- 1Department of Ethology and Companion Animal Science, Faculty of Agrobiology, Food and Natural Resources, Czech University of Life Sciences Prague, Prague, Czechia
- 2Department of Ethology, Institute of Animal Science, Prague, Czechia
- 3Department of Game Management and Wildlife Biology, Faculty of Forestry and Wood Sciences, Czech University of Life Sciences Prague, Prague, Czechia
- 4Department of Ecology and Environmental Sciences, Faculty of Natural Sciences and Informatics, Constantine the Philosopher University in Nitra, Nitra, Slovakia
- 5Section of Ecology, Department of Biology, University of Turku, Turku, Finland
Offspring survival rates in altricial birds during the post-fledging period are an essential factor in determining the fitness of parents and have a significant impact on general population dynamics. However, our current knowledge of post-fledging mortality and its causes remains fragmentary in most bird species, and even less information is available on the mortality of individuals of the same species in different environments. In order to address this gap in our knowledge, we studied fledgling mortality and its causes in Tengmalm’s owls (Aegolius funereus) during six breeding seasons in Central and North Europe using radio-telemetry. A total of 80 nestlings from 18 nests in Czechia (2010–2012, 2015) and 60 nestlings from 24 nests in Finland (2019, 2021) were radio-tracked during the post-fledging dependence period. The overall survival rate was much higher in Czechia (83%) than in Finland (53%), with predation identified as the primary cause of mortality in both areas. Avian predation was far higher in Finland, but mammalian predation was equivalent at both study sites. Pine martens (Martes martes) and goshawks (Accipiter gentilis) were the most common predators in Czechia and Finland, respectively. Starvation and disease, or mostly a combination of both, formed the second most common cause of death in both areas but were much more frequent in Finland than in Czechia. Offspring survival in both study sites was considerably higher in years of food abundance than in those of food scarcity. We suggest that the interactive effects of infections and poor body condition due to scarcity of main prey species induced higher mortality rates in offspring, particularly in the more challenging environment of North Europe. In contrast, fledgling owls were found to be able to fight off infections more successfully during rich food seasons. Finally, we encourage researchers to pay greater attention to the mutual influences of parasites and their definitive hosts and stress the importance of using radio or satellite tracking for mortality studies to identify causes of death more accurately.
1 Introduction
The significance of the post-fledging period for parents’ reproductive output and in the population dynamics and life history evolution of altricial birds has long been recognized (Lack, 1948). However, despite the numerous studies addressing the post-fledging dependence period (PFDP hereafter), our current knowledge regarding the causes of death and post-fledging survival rates remains fragmentary (Naef-Daenzer and Grüebler, 2016). A greater knowledge and understanding of survival rates and the causes of fledglings’ death are essential for formulating accurate estimates of population dynamics and recruitment probability in birds (Lack, 1966; Clutton-Brock, 1988; Newton, 1989) and are crucial in conserving species with declining populations (Vormwald et al., 2011). The lack of information regarding PFDP juvenile survival rates stems from the fact that earlier studies have typically focused on the incubation and/or nestling period/s, primarily due to the difficulties associated with tracking flying individuals after fledging, especially in nocturnal species. However, recent decades have seen considerable progress in overcoming these difficulties through the use of radio or satellite telemetry (White and Garrott, 1990; Kenward, 2001; Millspaugh and Marzluff, 2001).
Recent reviews have confirmed a widespread pattern of low juvenile survival across bird species, particularly during the first two weeks after leaving the nest (Cox et al., 2014; Naef-Daenzer and Grüebler, 2016). Interestingly, the pattern was the same for all ground-, open- (above ground in shrubs and trees) and cavity-nesting species; offspring survival rates steadily increased after the first two weeks of fledging, but survival was much lower after fledging than during the nestling period in open- and cavity-nesting species (reviewed by Naef-Daenzer and Grüebler, 2016). The vast majority of studies examining the causes of mortality noted that predation was the most frequent cause across different bird species/families (e.g., Naef-Daenzer et al., 2001; Todd et al., 2003; Robles et al., 2007; Salinas-Melgoza and Renton, 2007), a finding which, interestingly, has also been reported for many meso-predators and even for apex predators in birds of prey (e.g., Rohner, 1996; Coles et al., 1997; Sunde, 2005; Wiens et al., 2006).
After predation, the next most commonly reported proximate causes of death among fledglings are starvation, disease, human causes and structures (for example, collisions, artificial traps, electrocution, killing and hunting) and unknown reasons (e.g., Overskaug et al., 1999; Šálek et al., 2019; Kouba et al., 2021, 2023), but different variables can affect all of these causes of death. Among the factors most frequently mentioned in studies dealing with offspring survival during the PFDP are prey abundance, offspring sex, the timing of hatching and fledging within the breeding season, clutch and brood size, offspring age at dispersal, body condition at fledging (i.e., body mass and/or wing length), habitat composition and weather conditions (e.g., Tarwater et al., 2011; Maness and Anderson, 2013; Cox et al., 2014; Naef-Daenzer and Grüebler, 2016; Kouba et al., 2023).
Birds of prey fledglings are entirely dependent upon their parents for food during the PFDP and remain within the natal area until the initiation of natal dispersal (Kenward et al., 1993). The PFDP ranges from a few weeks to several months depending on species (Newton, 1979; Mikkola, 1983) and is the most critical period in terms of offspring survival due to the incomplete feather growth and inexpert flying skills of fledglings (McFadzen and Marzluff, 1996; Todd et al., 2003; Sunde, 2005). In owls and diurnal raptors, predation and starvation are also considered to be the most frequent causes of death (e.g., Petty and Thirgood, 1989; Varland et al., 1993; Overskaug et al., 1999), while disease, drowning, collisions and other or unknown cases were relatively rare (e.g., Bendel and Therres, 1993; Hunter et al., 1997; Todd, 2001; Larrat et al., 2012). Importantly, higher mortality rates have been observed in years with poor prey abundance in comparison with rates in rich years (Rohner and Hunter, 1996; Todd et al., 2003; Sunde, 2005). The risk of mortality is highest immediately after leaving the nest primarily due to predation by mammalian predators (Sunde, 2005), and the fact that mortality rates peak just after fledging has been confirmed by many other studies (e.g., Varland et al., 1993; McFadzen and Marzluff, 1996; Tome, 2011). However, even in a majority of birds of prey, the mortality of fledglings during the PFDP has never been studied, despite the fact that the PFDP is one of the most sensitive life history stages across all species of birds (Newton, 1979; Weathers and Sullivan, 1989).
The earliest estimate of the survival rate of Tengmalm’s owl (Aegolius funereus) fledglings was based on research using ring recoveries. Of a total of 4311 Tengmalm’s owl fledglings ringed between 1964 and 1985, only 53 (1.2%) were recovered and had survived at least their first winter. Abundance of main prey (voles) during the PFDP and independence phase was a crucial factor in determining the first-year survival of offspring (Korpimäki and Lagerström, 1988). Based on 281 ring recoveries of Tengmalm’s owls found dead in Finland, the estimates of mean annual survival were 50% during the first year of life and 67% thereafter (Korpimäki, 1992).
The main objectives of the current study were as follows. Firstly, we aimed to determine the survival rates and causes of death of Tengmalm’s owl fledglings during the PFDP. Secondly, we intended to compare survival rates and causes of death between two different environments, more specifically in Central and North Europe. Thirdly, we wanted to identify potential predictors which could be responsible for any differences identified between the two environments. Finally, we compared the results of the current study with earlier research conducted in Finland, which showed that the fledglings’ probability of dying by starvation was 3.7 times higher in the decreasing than during the increasing abundance of main foods of owls (Kouba et al., 2023).
Because nights are at least two times shorter in Finland than in Czechia, nocturnal parent owls have less time in which to feed their offspring (Korpimäki, 1981; Zárybnická et al., 2012). Therefore, we predicted that (i) the total mortality rate will be lower in Czechia than in Finland. Because the populations of small rodents, the main prey species of Tengmalm’s owls, usually increase from early spring to autumn (i.e., over the course of the breeding season of owls) in Czechia (Zárybnická et al., 2015a) but decrease from early spring to autumn in the decline phase of the three-year high-amplitude cycle prevailing in Finland (Korpimäki et al., 2005a), we further expected that (ii) the starvation rate of offspring during the PFDP will be higher in Finland than in Czechia. However, the abundance of the main prey of owls in particular years was expected to be a decisive factor in terms of starvation rates in both study sites. The air pollution calamity which severely damaged the study area in the Ore Mts. of Czechia in the 1970s led to the widespread loss of nest sites for birds of prey, and therefore there were almost no observations of species which predate Tengmalm’s owls (Kouba et al., 2023). As a result, we predicted that (iii) avian predation will be lower in Czechia than in Finland. Finally, because nest predation rates of Tengmalm’s owls were substantially higher in Central Europe (ca. 20% in the Ore Mts.; Zárybnická et al., 2015b) than in North Europe (ca. 5%; Korpimäki, 1987; Korpimäki and Hakkarainen, 2012), we predicted that (iv) Tengmalm’s owl fledglings will be more subject to predation by pine martens (Martes martes) in Czechia than in Finland.
2 Materials and methods
2.1 Study areas
The study was conducted over the course of six breeding seasons in Czechia in 2010–2012, 2015 and in Finland in 2019 and 2021. The Czech site (50° N, 13° E; 730–960 m a. s. l.; ca. 120 square km) is located in the Ore Mts. and included approximately 120–170 nest boxes (Kouba et al., 2014; 2017). The study area was severely damaged by historical air pollution events (for more information, see, e.g., Kouba et al., 2013). Currently, the area is mainly forested, predominantly by blue spruce (Picea pungens, covering approximately 28% of the area), Norway spruce (Picea abies, 26%), birch (Betula spp., 11%), European mountain ash (Sorbus aucuparia, 5%), European beech (Fagus sylvatica, 4%), and European larch (Larix decidua, 4%).
The Finnish site is situated in the Kauhava region of west-central Finland (63° N, 23° E; 50–110 m a. s. l.; ca. 1000 square km) and included approximately 450 nest boxes (Korpimäki and Hakkarainen, 1991; 2012). Approximately 61% of this study area is forested, with the predominant species being Scots pine (Pinus sylvestris, forming ca. 65% of the local forests), Norway spruce (>30%) and a minority of deciduous trees (birches and Eurasian aspen, Populus tremula) (for more information, see, e.g., Korpimäki and Hakkarainen, 1991; 2012).
The nest boxes in both areas were similar in design; they were made of wood, square in section, with a base of 18–25 x 18–25 cm, 40–60 cm height, and an 8–10 cm diameter entrance hole.
2.2 Environmental variables
In order to ensure uniformity for comparisons, the habitat in both study areas was divided into sections with a 500 m radius around each of the studied nest boxes which were categorized into three classes (with the first class being subdivided into three forest subclasses, see below) according to the methodology used in our previously published study (Kouba et al., 2023). The classes were (1) forest stands and individual areas of either young, middle-aged or old-growth forests, (2) clear-cuts, and (3) agricultural land. For further details of the categorization of the habitat into the three variables (1–3) and the three forest subclasses, see Kouba et al. (2023).
Weather data for the Ore Mts. was obtained from weather stations near to the study site. The mean daily temperature (°C; an average of hourly measurements) was taken from the station in Nová Ves v Horách and daily precipitation (mm) from the station in Český Jiřetín. Mean daily temperatures (°C; an average of hourly measurements) and daily precipitation (mm) for the Kauhava study area were obtained from the Finnish Meteorological Institute, more specifically from the weather station at Kauhava airport which is located in approximately the middle of the study area.
In both study areas, the abundance of main prey species (small rodents) was assessed using snap traps set in late spring (early June in the Czech and early May in the Finnish site). Snap-traps were set up in squares (with 10 m spacing) and were checked each morning for three consecutive days. For each year of the study, the total trapping effort in the Czech study site was 1089 trap nights (n = 3 locations), with ca. 600 trap nights (n = 8 locations) carried out in the Finnish study site. The number of captured mammals per 100 trap nights was calculated for each trapping site and breeding season (Table 1).
2.3 Field procedures
All nest boxes in both study areas and across all study years were visited regularly from early March to late June to find nests. If nesting was identified, nests were checked sufficiently often to determine the number of eggs and hatchlings and the exact hatching date (± 1 day). The age of the nestlings was based on the recorded dates of hatching.
From 25 days after the hatching of the first chick, the nest boxes were checked at one or two-day intervals; a total of 80 nestlings from 18 nests in Czechia and 60 nestlings from 24 nests in Finland. All individuals were weighed, and their wing length was measured to estimate the appropriate time for tagging (Kouba et al., 2013; 2014). Nestlings were equipped with type PIP4 leg-mount transmitters (Biotrack Ltd., UK) about five days before fledging. For more details about the transmitters used in the study, see Kouba et al. (2023) and Stehlíková Sovadinová et al. (2024). The nest boxes were visited at 12-hour intervals during the night and daylight until all siblings had fledged and the exact date of nest box departure had been determined.
After fledging, the young were radio-tracked using the “homing-in” method (Kenward, 2001), in which the signal is followed to a particular tree until the individual is observed, until they become independent, or until they are found dead, disappeared or dispersed. Fledglings were located at intervals of either 12 hours or 24 hours during the day and night (e.g., Kouba et al., 2023; Stehlíková Sovadinová et al., 2024) which should be sufficient to allow the fates of individual fledglings to be recorded. Radio signals were received using Yupiteru MVT-9000 receivers (Yupiteru Industries Co. Ltd., Japan) and 3-element Yagi antennas. The PFDP starts with the departure of the owlets from the nest, but the end is obviously less clearly demarcated. We defined the end of the PFDP with the first rapid and abrupt movement away from fledglings’ habitual locations, an event which previous studies have suggested may correspond with the cessation of begging for food (Kouba et al., 2013; 2014).
Across both study areas, the fates of 130 of the 140 monitored fledglings were identified (Table 2); the fate of 9 of the remaining 10 individuals could not be determined because they fledged before tagging or their transmitters failed during the study, but these fledglings were regularly spotted and/or heard during the PFDP; they were last recorded 34 ± 12 (mean ± SD; range 13–53) days after fledging and were therefore included in the five below survival function analyses. The last individual disappeared on day 30 after fledging (Table 2).
The carcasses of all deceased fledglings were collected, preserved and transported to the State Veterinary Institute Prague in Czechia where autopsies were performed to determine the cause of death and to carry out parasitological and bacteriological investigations. It is important to note here that the pathologists always only stated what was present and observed; they never determined a direct cause of death. However, we further speculate that this might be the reason why the fledglings died. Avian predators were identified as causes of death by direct observation or where typical plucking sites or transmitters in the nests were found. Predation by mammals was determined if the fledglings were found buried in the ground or some remains of body parts, bones and/or transmitters with obvious teeth bite marks. In a few cases, when the fledglings disappeared without a trace close to birds of prey nests or where other raptor predation was confirmed, we considered bird predation as the reason for their disappearance (Table 2).
Owls in Czechia were trapped, handled and tagged under Permits Nos. 530/758 R/08-Abt/UL, 35016/02-OOP/8751/02 and 173/049/ZPZ/2015/ZD-838 issued by the Ministry of the Environment of the Czech Republic. They were ringed under the supervision of the Ringing Centre of the National Museum in Prague, Permit No. 329. Fledglings in Finland were tagged and radio-tracked, and the cadavers were transported to Czechia under the approval of the Centre for Economic Development, Transport and the Environment (Permit Nos. VARELY/1389/2018, and VARELY/5933/2019). They were ringed under the ringing license of the Finish Museum of Natural History (Permit No. 524). Every effort was made to minimize suffering during the study.
2.4 Statistical analyses
All data was analyzed using SAS System version 9.4 (SAS Institute Inc.). The countable variables were first checked for intercorrelation and multicollinearity. A significant correlation was found between the dates of hatching and fledging (0.95, P<0.001), spring prey abundance and number of fledglings (0.54, P<0.01), and areas of all forests and agricultural lands within a 500 m radius of the studied nest boxes (-0.88, P<0.001). The extent of collinearity was then investigated using the Tolerance value or Variance Inflation factor, Eigenvalue, and Condition Number (in PROC REG) following the approach described by Schreiber-Gregory and Jackson (2017). No collinearity was identified during the analysis, but any variables which were found to be intercorrelated were not used together in the same set of predictors in any subsequent analysis.
The data was then analyzed in more detail in three steps. Step 1. Kaplan-Meier curves were created for two countries (STRATA) of Finland and Czechia using PROC LIFETEST. In order to compare the survival functions (covering all causes of death) for the two countries, PROC LIFETEST offers six non-parametric tests of equality over strata: Log-rank, Wilcoxon, Tarone-Ware, Peto-Peto, Modified Peto-Peto and Harrington-Fleming tests. In this study, only the information obtained through the Log-rank test was used to compare the two countries. The time statement was the number of days between fledging and death for individuals who had been found dead. There were five censored values for Czechia but none for Finland. The numeric predictor variables which were tested for their association with survival time are given in Table 3. The Chi-Squares of the Wilcoxon test estimated the effect of numeric predictors. A %newsurv macro (Meyers, 2017) was used to determine the survivor function plots with confidence intervals (CI) of 95%.
Step 2. The cause of death for the fledglings in each study site was compared. Predation (e.g., Sunde, 2005; Kouba et al., 2023) and starvation (e.g., Overskaug et al., 1999; Kouba et al., 2023) account for the vast majority of fledgling deaths, and therefore, we first separated all cases of fledgling death caused by predation and applied the same procedure described in Step 1. We then separated the cases caused by starvation and other cases not caused by predation and applied the same method as that used for the cases of predation. Step 3. We analyzed the predation data depending on whether the fledgling had been predated by bird or mammal predators.
Since the Kaplan-Meier analysis does not allow direct testing of interaction terms, we constructed a composite variable of “survival/precipitation”. We used it as one of the predictors listed in Table 3. The “survival” aspect reflected the days between fledging and death for individuals who died before independence. The prematurely dying fledglings died up to 18 days of PFDP in most cases (only 4 out of 40 died later). We regarded these 18 days as a period critical for survival. Our previous study (Kouba et al., 2023) showed that precipitation was the main factor of that period. The part of the composite variable for “precipitation” was the average precipitation (mm/day) for the number of days between an individual owl’s fledging and death within the PFDP or the average precipitation up to 18 days for survivors.
3 Results
A total of 140 Tengmalm’s owl fledglings were monitored during the course of this study: 80 individuals from 18 nests during four breeding seasons in Czechia and 60 individuals from 24 nests during two breeding seasons in Finland (all siblings from the studied nests were monitored). Within the monitored nests, the clutch size was 5.8 ± 1.5 (mean ± SD), the number of hatchlings was 5.2 ± 1.8, and the number of fledglings was 4.3 ± 2.1 in Czechia and 6.0 ± 1.0, 5.7 ± 1.2, and 2.5 ± 1.4 in Finland, respectively (for details regarding basic breeding data, including the study seasons and the numbers of studied young, see Table 1). Combining the data from both study sites, the survival rate for fledglings during the PFDP was 69.2%. The pooled mortality rate due to starvation, disease or mostly their combination was 12.3%, the predation mortality rate was 17.7%, while other causes accounted for 0.8% (Table 2). The PFDP duration roughly ranged between one to two months in both study areas.
3.1 Survival rates and factors affecting survival
Step 1. The proportion of fledglings estimated to have survived was larger in Czechia than in Finland (Log-rank test: χ2(1)=11.20, P=0.0008, Figure 1A; overall mortality rate 17.1% vs 46.7%, respectively). The univariate chi-squares for the Wilcoxon test showed that composite survival/precipitation was a highly significant predictor variable (χ2=12.91, P=0.0003), and individual fledging date (χ2=4.82, P=0.03) and the spring abundance of main prey species (χ2=4.44, P=0.04) were only marginally significant. No other numeric predictor, for example, that of habitat variables, reached a significant level (Table 3). For all three mentioned significant predictors, fledglings with larger values had more prolonged survival periods.
Step 2. The level of mortality caused by the merged factors of starvation, disease or a combination of both (“starvation”) was lower in Czechia than in Finland (Log-rank test: χ2(1)=3.88, P=0.05, Figure 1B; starvation mortality rate 5.7% vs 20.0%, respectively). None of the numeric predictors in Table 3 had a significant influence on mortality caused by starvation. The incidence of predation caused by both mammals and birds was also lower in Czechia than in Finland (Log-rank test: χ2(1)=5.02, P=0.03, Figure 1C; predation mortality rate 10.0% vs 26.7%, respectively). This was influenced by the composite survival/precipitation variable (Wilcoxon test: χ2=7.86, P=0.005) and individual fledging date (Wilcoxon test: χ2=4.78, P=0.03); the survival periods of individual fledglings were found to be longer at higher levels of precipitation and later fledging dates.
Step 3. Predation by avian predators was much more frequent in Finland than in Czechia (Log-rank test χ2(1)=9.93, P=0.002, Figure 1D; avian predation rate 23.3% vs 1.4%, respectively), and the date of fledging had only a marginal effect on the survival rate (Wilcoxon test: χ2=3.84, P=0.05), with individual fledglings which left the nest box later tending to survive better. In contrast, there was no difference between Czechia and Finland in terms of predation by mammals (Log-rank test: χ2(1)=1.80, NS, Figure 1E; mammal predation rate 8.6% vs 3.3%, respectively).
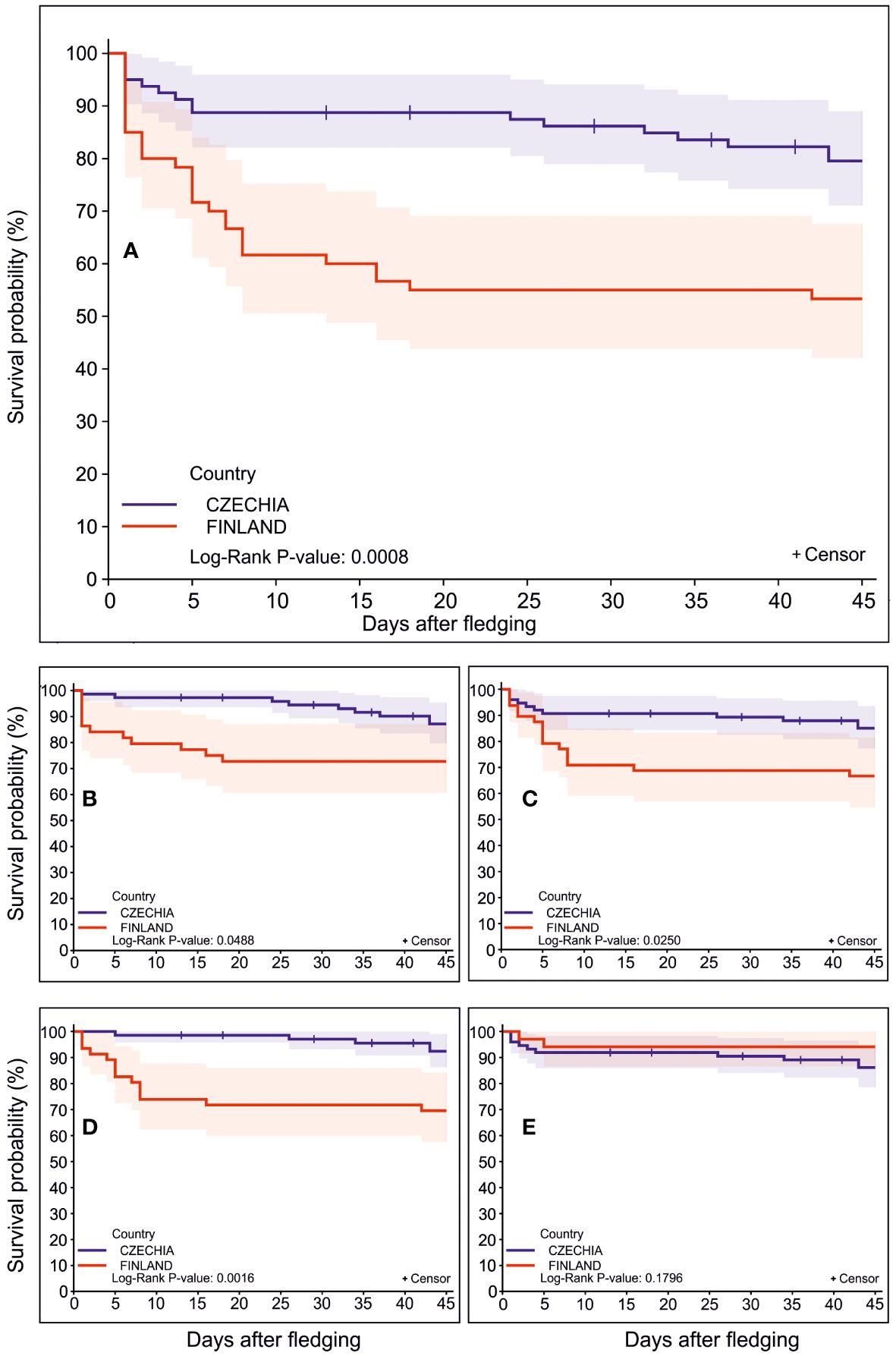
Figure 1 Fledglings’ mortality risk curves after leaving the nest. Tengmalm’s owl fledglings’ survival function curves with ± 95% confidence intervals calculated for the post-fledging phase within five different combinations of mortality sources, with data for the Czech (blue) and Finnish (red) study sites shown separately. The total mortality risk comprising all death events (A), mortality risk caused by starvation, disease and a combination of both (B), predation mortality risk merging death events caused by birds and mammals (C), avian-only predation mortality risk (D), and mammal-only predation mortality risk (E) are shown. The censored individuals are marked by a plus (+) symbol.
3.2 Causes of death during the post-fledgling phase
In both study sites, predation was the most frequent cause of death, with mammalian predation being more common in Czechia and avian predation in Finland. All eight cases of mammal predation occurred within the first five days after fledging. Avian predation mostly took place soon after leaving the nest; in 13 out of 15 cases, young birds were taken within eight days of fledging. Starvation, either by itself or in combination with disease, was the second most frequent cause of death; in contrast to death by predation, individual cases occurred more evenly throughout the study but were also most common soon after fledging.
The pine marten and the goshawk (Accipiter gentilis) were the most common predators in the Czech and Finnish study areas, respectively (Table 4). During the autopsy examinations, the most frequently recorded parasite was Sarcocystis funereus, which was identified in the carcasses of 4 out of 10 Czech fledglings and in all 16 Finnish fledglings.
4 Discussion
Our results show a higher total mortality rate for Tengmalm’s owl fledglings during the PFDP in the North European study area of Finland than in the Central European area of Czechia, a finding which is consistent with our first prediction (i). There were two main reasons for this difference. Firstly, the number of fledglings predated by avian predators was higher in Finland than in Czechia (14 vs 1 individual), and secondly, the mortality of fledglings caused by combined starvation and disease was 3.5 times higher in Finland than in Czechia, a finding which is in line with our second prediction (ii). It should be noted here that the autopsies conducted on the recovered carcasses did not intend to distinguish between deaths caused by starvation and those caused by disease; we only speculate about these possibilities in cases where the young birds were unnaturally underweight without any infections or, vice versa, in birds which had an appropriate body mass but were heavily parasitized. In all other cases, the cause of death most probably was starvation, possibly in combination with infection. However, though this remains speculative, we suggest that starvation was a more significant factor in most cases. Our overall results regarding the rate and frequency of predation and starvation did not differ markedly from other studies on birds of prey such as, for example, tawny owls (Strix aluco) (Overskaug et al., 1999; Sunde, 2005), long-eared owls (Asio otus) (Tome, 2011) or prairie falcons (Falco mexicanus) (McFadzen and Marzluff, 1996).
Mortality due to starvation varied widely depending on the abundance of small rodents, the main prey species of Tengmalm’s owls. Mortality induced by starvation during the PFDP was widespread in the Finnish site in 2019, a decrease year in the three-year high-amplitude population cycle of voles: the vole population declined from spring to autumn, and vole numbers were very low during the PFDP and the fledglings’ independence period. This steep decline in the abundance of main prey species induced a very high mortality rate in owl offspring (Kouba et al., 2023). Mortality due to starvation was much lower in 2021 which was an increasing year of main prey populations, although brood reduction during the nestling period was as frequent as in 2019. These results are consistent with the first-year survival and recruitment rates of Tengmalm’s owl offspring, which are three times higher for individuals which fledged in the increase than in the decrease phase of the vole cycle (Korpimäki and Lagerström, 1988; Korpimäki and Hakkarainen, 2012). During the increase phase in 2021, some fledglings also starved to death, but this was only recorded within broods of yearling male parents or in those of polygynous males [only three males (all in Finland) in 2021 were recorded as polygynous during the whole study]. The fledgling production of yearling males is generally lower than that of older males (Laaksonen et al., 2002), and nestling mortality is markedly more frequent in the secondary and tertiary nests of polygynous male owls than in their primary nests (Korpimäki, 1991). Polygynous male owls prefer to provision their earliest (primary) nest after hatching; as a consequence, many nestlings in later secondary and tertiary nests starve to death (Korpimäki, 1991).
In Czechia, the starvation of fledglings was only observed during the 2011 breeding season, the year with the lowest prey abundance. This year, eight nestlings from five broods that starved to death in the box were even at the fledging age (three were already radio-tagged; no one was included in any analysis of mortality during the PFDP). As a result, brood reduction was very high in 2011 in comparison with the other three breeding seasons during which the research was conducted in this site. We suggest that the explanation for higher mortality due to starvation during PFDP in Finland is a consequence of the more challenging environment of North Europe; during the mid-summer breeding season, nightly darkness lasts only two to three hours in the north (Korpimäki, 1981), whereas it is twice as long in the south (Zárybnická et al., 2012), meaning that only a short period of time is available for prey deliveries among the Finnish population of the strictly nocturnal Tengmalm’s owls. In addition, steep summer declines in the populations of small rodents do not occur in the Czech study site (Zárybnická et al., 2015a), whereas they happen every three years in the north through high-amplitude 3-year vole cycles (Korpimäki et al., 2005a; 2005b). We suggest that short prey delivery times and steep summer declines in the north can account for the higher fledgling mortality rates caused by a combination of starvation and disease in the Finnish study site, a rate which is 3.5 times greater than that recorded in Czechia. Moreover, these results are also in agreement with the findings of an earlier study comparing our two study areas (Zárybnická et al., 2012) which reported higher brood reduction during the nestling period in Finland; the study also found that local males are required to hunt throughout the whole night to satiate their offspring.
The overall higher predation of Tengmalm’s owl fledglings in Finland is primarily a reflection of the above-mentioned difference regarding the presence of avian predators in the Kauhava area and their absence in the Ore Mts. (see Introduction), which aligns with prediction iii. The occurrence of avian predation was much harder to confirm than predation by mammals (see below); seven fledglings disappeared, and their tag signals were never detected again (Table 4). However, two cases occurred in the same stand of forest in which a tag of a Tengmalm’s owl fledgling was found in the nest cavity of Ural owls (Strix uralensis). The other five cases were attributed to goshawks because they occurred at sites where this raptor species had taken their siblings. Predation by goshawks was confirmed in cases where feathers of Tengmalm’s owls were found at typical plucking sites. The recorded avian predators are well-known enemies of Tengmalm’s owl fledglings and adults (Mikkola, 1983; Hakkarainen and Korpimäki, 1996; Tornberg et al., 2006), but one interesting find was the predation of a fledgling by carrion crows (Corvus corone), a species which has not previously been described as a predator of Tengmalm’s owl in existing literature.
Predation by mammals appeared to be more frequent at the Czech site than in the Finnish study area, but the difference was insignificant. Insufficient data could have caused the lack of significance. Still, we could not confirm the prediction iv anyway. In four out of seven cases of pine marten predation, the carcass was found buried in the ground for use as a future food source. The fledglings were eaten on the spot in the three other cases, and only some feather, bone and leg remains were found. In all of these cases, we concede that the fledglings might also have been predated by red foxes (Vulpes vulpes), although this is somewhat unlikely because most of the young were observed higher up in trees during their previous tracking.
We found that predator-induced mortality mainly occurred immediately after fledging, a finding which is in agreement with earlier studies and which appears to be a widespread phenomenon in birds across different species and families (e.g., Adams et al., 2001; Naef-Daenzer et al., 2001; Keedwell, 2003; Todd et al., 2003; Sunde, 2005; Robles et al., 2007; Salinas-Melgoza and Renton, 2007). The improvement of flying and climbing skills in fledglings most probably involves elements of motor learning that can only be learned outside the nest (as suggested by Sunde, 2005). Moreover, flight feathers are not fully grown at the time of leaving the nest, making the naive newly-fledged young birds an easy target even in the case of Tengmalm’s owls, which can usually fly relatively well upon fledgling in comparison to tawny owl fledglings which can only climb in branches at that time.
Survival periods of fledglings were longer for those who fledged later during the breeding seasons. Thus, higher mortality occurred within parents’ broods with the earliest nesting timing. This finding contrasts many studies on bird of prey species that described the earliest breeders as the most successful, including the Tengmalm’s owl (e.g., Korpimäki and Hakkarainen, 1991; Korpimäki and Wiehn, 1998; Ferrero et al., 2003; Sergio, 2003). However, this comparison of the nestling and post-fledging phases may be inappropriate because the mortality sources might notably differ between the two periods. More importantly, our result also contradicts the finding that mortality risk in tawny owl fledglings increased with the fledging date due to an increasing raptor predation risk later in the breeding season (Sunde, 2005). As we found the opposite, it is evident that the selection pressures regarding fledgling dates (the selection for early breeding) differ in these two species.
The parasitological and bacteriological investigations of recovered carcasses of succumbed or predated young revealed a broad scale of present parasites and/or bacteria. Only a few studies to date have addressed the potential impact of particular parasites on adults or fledglings in different birds of prey worldwide (but see, e.g., Hunter et al., 1997; Larrat et al., 2012). However, we found no other cause of death besides parasites in one fledgling in Czechia and one in Finland, infected by Sarcocystis funereus and species of the order Ascaridida, respectively. Both were in good physical condition because the deaths occurred during seasons with a high abundance of main prey. Several studies have noted that the synergy between parasites and other stressors, such as inclement weather, environmental disturbance, food scarcity, crowding or a combination of these factors can result in a substantial increase in bird mortality (Howe, 1992; Hunter et al., 1997; Work et al., 2004; Larrat et al., 2012). However, it is not clear whether poor body condition as a consequence of low food abundance induces individuals to be an easy target for different parasites due to decreased host resistance or vice versa; the infections which can weaken individuals as a parasite burden might have metabolic costs for their hosts (Behnke et al., 1992; Wobeser, 2009) and thereby contribute to deteriorations in their physical condition. Our results seem consistent with the theory that individuals are more capable of successfully fighting and recovering from infections under conditions of high food supply, with the survival of fledglings remaining high in such conditions (as suggested by Hunter et al., 1997); infections usually do not kill their hosts directly but may weaken individuals to such an extent that other factors can reach life-threatening levels or combine to cause death (as suggested by Howe, 1992).
A prevalent parasite found in the studied carcasses was Sarcocystis funereus (Máca et al., 2021; 2024). It is known that Sarcocystis cernae influences the behaviour of its intermediate host (the common vole Microtus arvalis) by enhancing the probability of parasite transmission to the final host (the Eurasian kestrel Falco tinnunculus) and increasing the risk of its predation (Hoogenboom and Dijkstra, 1987), and this is likely also the case with the intermediate host of S. funereus, which is still unknown but which was suggested may be the harvest mouse (Micromys minutus) (Máca et al., 2024). However, very little is known about the effects of parasitation by Sarcocystis sp. on their definitive hosts, various species of birds of prey. We surmise that the presence of large numbers of parasites in the intestines must burden any organism and adversely affect the health of the host. In this context, we would recommend that future research should focus on parasite-host relationships and interactions because the issue of unstudied parasites within animal populations and their status and long-term development may be as crucial as more comprehensively studied topics such as animal interactions, habitat loss and global climate change.
In the Finnish study area, we recorded that 57% of dead fledglings had been predated and 43% had died due to a combination of starvation and disease. The main causes of death of Tengmalm’s owls ringed in Finland during 1913–2012 (636 inds.) were traffic collision (26%), window collision (15%), entering buildings (10%), predation (25%), sickness (8%) and other (16%) (Valkama et al., 2014). Although we compared young and adult individuals over different periods, the difference between the two study results is in agreement with similar comparisons made in other studies, as the conclusions about survival rates and causes of mortality based exclusively on ring recoveries can be misleading (Newton et al., 2016; Naef-Daenzer et al., 2017). For example, a study on the causes of death in little owls (Athene noctua) based on ring recoveries substantially overestimated traffic and human structures and underestimated predation (Naef-Daenzer et al., 2017). All these researchers stressed the importance of using radio or satellite tracking for mortality studies to determine the actual causes of death, an approach which is crucial to establishing proper conservation management for endangered species.
We conclude that the abundance of main prey species was the most crucial factor regarding the survival of Tengmalm’s owl fledglings during the PFDP, a finding which has also been observed in other species of birds of prey (e.g., Rohner and Hunter, 1996; Todd et al., 2003; Sunde, 2005). We further suggest that the interactive effects of infection and malnourishment due to low prey abundance were also responsible for a high degree of mortality, particularly in the Finnish study area in which the more challenging environment possibly acts as another stressor factor. In contrast, we might speculate that Tengmalm’s owl fledglings were more successful in fighting off infections in seasons with high prey abundance, as has also been hypothesized for great horned owls (Bubo virginianus) (Hunter et al., 1997). Finally, we recommend that researchers pay greater attention to the interacting influences and long-term effects of parasites on their hosts, particularly their definitive hosts, as this appears to be an understudied topic of crucial importance.
Data availability statement
The raw data supporting the conclusions of this article will be made available by the authors, without undue reservation.
Ethics statement
All applicable international, national and institutional guidelines for the care and use of animals were followed in this study. Owls in Czechia were trapped, handled and tagged under Permits Nos. 530/758 R/08-Abt/UL, 35016/02-OOP/8751/02 and 173/049/ZPZ/2015/ZD-838 issued by the Ministry of the Environment of the Czech Republic. They were ringed under the supervision of the Ringing Centre of the National Museum in Prague, Permit No. 329. Fledglings in Finland were tagged and radio-tracked, and the cadavers were transported to Czechia under the approval of the Centre for Economic Development, Transport and the Environment (Permit Nos. VARELY/1389/2018, and VARELY/5933/2019). They were ringed under the ringing license of the Finish Museum of Natural History (Permit No. 524). Every effort was made to minimize suffering during the study. The study was conducted in accordance with the local legislation and institutional requirements.
Author contributions
MK: Conceptualization, Data curation, Formal analysis, Funding acquisition, Investigation, Methodology, Project administration, Resources, Writing – original draft, Writing – review & editing. LB: Formal analysis, Visualization, Writing – original draft, Writing – review & editing. FT: Investigation, Writing – review & editing. SS: Investigation, Writing – review & editing. EK: Writing – original draft, Writing – review & editing, Conceptualization, Supervision.
Funding
The author(s) declare financial support was received for the research, authorship, and/or publication of this article. The research project was financially supported by two grants provided to MK by the Regional Fund of the South Ostrobothnia of the Finnish Cultural Foundation (Business ID 0116947-3 and 10201775). LB was supported by the Ministry of Agriculture of the Czech Republic (No. MZE-RO0723).
Acknowledgments
We thank Alena Popelková for her help with nest box controls and tagging the nestlings during 2010–2012, Alessandro Fantoni for his help with tagging the nestlings and radio-tracking in 2015, and Jiří Šindelář for his help with nest box controls in 2015 in Czechia. We also thank Tomáš Bušina, Jorma Nurmi, Kari Hongisto and Martin Janouš for their help in the field during the study in Finland. We thank Mikko Hänninen for finding the transmitter in the Ural owl nest. We greatly appreciate Lubomír Peške for technical assistance with radio-telemetry and manufacturing Yagi antennas for the whole study. Next, we would like to thank HW, SH and PK for their comments and suggestions to the earlier draft of the manuscript.
Conflict of interest
The authors declare that the research was conducted in the absence of any commercial or financial relationships that could be construed as a potential conflict of interest.
Publisher’s note
All claims expressed in this article are solely those of the authors and do not necessarily represent those of their affiliated organizations, or those of the publisher, the editors and the reviewers. Any product that may be evaluated in this article, or claim that may be made by its manufacturer, is not guaranteed or endorsed by the publisher.
References
Adams A. A. Y., Skagen S. K., Adams R. D. (2001). Movements and survival of lark bunting fledglings. Condor 103, 643–647. doi: 10.1093/condor/103.3.643
Behnke J. M., Barnard C. J., Wakelin D. (1992). Understanding chronic nematode infections: Evolutionary considerations, current hypotheses and the way forward. Int. J. Parasitol. 22, 861–907. doi: 10.1016/0020-7519(92)90046-N
Bendel P. R., Therres G. D. (1993). Differential mortality of barn owls during fledging from marsh and off-shore nest sites. J. Field Ornithol. 64, 326–330.
Coles C. F., Petty S. J., Johnson D. H. (1997). “Dispersal behavior and survival of juvenile tawny owls (Strix aluco) during the low point in a vole cycle,” in Biology and conservation of owls of the northern hemisphere. Eds. Duncan J. R., Nicholls T. H. (USDA Forest Service, General Technique Report NC-190, St. Paul, Minnesota), 111–118.
Cox W. A., Thompson F. R., Cox A. S., Faaborg J. (2014). Post-fledging survival in passerine birds and the value of post-fledging studies to conservation. J. Wildl. Manage. 78, 183–193. doi: 10.1002/jwmg.670
Ferrero J. J., Grande J. M., Negro J. J. (2003). Copulation behavior of a potentially double-brooded bird of prey, the black-winged kite (Elanus caeruleus). J. Raptor Res. 37, 1–7.
Hakkarainen H., Korpimäki E. (1996). Competitive and predatory interactions among raptors: An observational and experimental study. Ecology 77, 1134–1142. doi: 10.2307/2265582
Hoogenboom I., Dijkstra C. (1987). Sarcocystis cernae: A parasite increasing the risk of predation of its intermediate host, Microtus arvalis. Oecologia 74, 86–92. doi: 10.1007/BF00377350
Howe F. P. (1992). Effects of Protocalliphora braueri (Diptera: Calliphoridae) parasitism and inclement weather on nestling sage thrashers. J. Wildl. Dis. 28, 141–143. doi: 10.7589/0090-3558-28.1.141
Hunter D. B., Rohner C., Currie D. C. (1997). Mortality in fledgling great horned owls from black fly hematophaga and leucocytozoonosis. J. Wildl. Dis. 33, 486–491. doi: 10.7589/0090-3558-33.3.486
Keedwell R. J. (2003). Does fledging equal success? Post-fledging mortality in the black-fronted tern. J. Field Ornithol. 74, 217–221. doi: 10.1648/0273-8570-74.3.217
Kenward R. E., Marcström V., Karlbom M. (1993). Post-nestling behavior in goshawks, Accipiter gentilis: 1. The causes of dispersal. Anim. Behav. 46, 365–370. doi: 10.1006/anbe.1993.1198
Korpimäki E. (1981). On the ecology and biology of Tengmalm’s owl (Aegolius funereus) in southern Ostrobothnia and Soumenselkä, western Finland. Acta Univ Oul A 118 Biol. 13, 1–84.
Korpimäki E. (1987). Selection for nest-hole shift and tactics of breeding dispersal in Tengmalm’s owl Aegolius funereus. J. Anim. Ecol. 56, 185–196. doi: 10.2307/4808
Korpimäki E. (1991). Poor reproductive success of polygynously mated female Tengmalm’s owls: Are better options available. Anim. Behav. 41, 37–47. doi: 10.1016/s0003-3472(05)80501-9
Korpimäki E. (1992). Fluctuating food abundance determines the lifetime reproductive success of male Tengmalm’s owls. J. Anim. Ecol. 61, 103–111. doi: 10.2307/5513
Korpimäki E., Hakkarainen H. (1991). Fluctuating food supply affects the cluch size of Tengmalm’s owl independent of laying date. Oecologia 85, 543–552. doi: 10.1007/BF00323767
Korpimäki E., Hakkarainen H. (2012). The boreal owl: ecology, behaviour and conservation of a forest-dwelling predator (Cambridge: Cambridge University Press). doi: 10.1017/CBO9780511844164
Korpimäki E., Lagerström M. (1988). Survival and natal dispersal of fledglings of Tengmalm’s owl in relation to fluctuating food conditions and hatching date. J. Anim. Ecol. 57, 433–441. doi: 10.2307/4915
Korpimäki E., Norrdahl K., Huitu O., Klemola T. (2005a). Predator-induced synchrony in population oscillations of coexisting small mammal species. Proc. R. Soc B-Biol. Sci. 272, 193–202. doi: 10.1098/rspb.2004.2860
Korpimäki E., Oksanen L., Oksanen T., Klemola T., Norrdahl K., Banks P. B. (2005b). Vole cycles and predation in temperate and boreal zones of Europe. J. Anim. Ecol. 74, 1150–1159. doi: 10.1111/j.1365-2656.2005.01015.x
Korpimäki E., Wiehn J. (1998). Clutch size of kestrels: Seasonal decline and experimental evidence for food limitation under fluctuating food conditions. Oikos 83, 259–272. doi: 10.2307/3546837
Kouba M., Bartoš L., Šťastný K. (2013). Differential movement patterns of juvenile Tengmalm’s owls (Aegolius funereus) during the post-fledging dependence period in two years with contrasting prey abundance. PloS One 8, e67034. doi: 10.1371/journal.pone.0067034
Kouba M., Bartoš L., Šťastný K. (2014). Factors affecting vocalization in Tengmalm’s owl (Aegolius funereus) fledglings during post-fledging dependence period: Scramble competition or honest signalling of need? PloS One 9, e95594. doi: 10.1371/journal.pone.0095594
Kouba M., Bartoš L., Tomášek V., Popelková A., Šťastný K., Zárybnická M. (2017). Home range size of Tengmalm’s owl during breeding in central Europe is determined by prey abundance. PloS One 12, e0177314. doi: 10.1371/journal.pone.0177314
Kouba M., Bartoš L., Tulis F., Ševčík M., Sovadinová S., Bušina T., et al. (2023). Post-fledging survival of Tengmalm’s owl offspring in boreal forests: Interactive effects of varying dynamics of main prey and habitat composition. Front. Ecol. Evol. 11. doi: 10.3389/fevo.2023.1151622
Kouba M., Slobodník R., Chavko J. (2021). Post-fledging dependence period, dispersal movements and temporary settlement areas in saker falcons (Falco cherrug). Raptor J. 15, 75–87. doi: 10.2478/srj-2021-0005
Laaksonen T., Korpimäki E., Hakkarainen H. (2002). Interactive effects of parental age and environmental variation on the breeding performance of Tengmalm’s owls. J. Anim. Ecol. 71, 23–31. doi: 10.1046/j.0021-8790.2001.00570.x
Lack D. (1948). Natural selection and family size in the starling. Evolution 2, 95–110. doi: 10.2307/2405371
Larrat S., Dallaire A. D., Lair S. (2012). Emaciation and larval filarioid nematode infection in boreal owls (Aegolius funereus). Avian Pathol. 41, 345–349. doi: 10.1080/03079457.2012.688940
Máca O., Kouba M., Korpimäki E., González-Solís D. (2021). Molecular identification of Sarcocystis sp. (Apicomplexa, Sarcocystidae) in offspring of Tengmalm’s owls, Aegolius funereus (Aves, Strigidae). Front. Vet. Sci. 8. doi: 10.3389/fvets.2021.804096
Máca O., Kouba M., Langrová I., Panská L., Korpimäki E., González-Solís D. (2024). The Tengmalm’s owl Aegolius funereus (Aves, Strigidae) as the definitive host of Sarcocystis funereus sp. nov. (Apicomplexa). Front. Vet. Sci. 11. doi: 10.3389/fvets.2024.1356549
Maness T. J., Anderson D. J. (2013). Predictors of juvenile survival in birds. Ornithol. Monogr. 78, 1–55. doi: 10.1525/om.2013.78.1.1
McFadzen M. E., Marzluff J. M. (1996). Mortality of prairie falcons during the fledging-dependence period. Condor 98, 791–800. doi: 10.2307/1369859
Meyers J. (2017)The %NEWSURV family of macros: an update on the survival plotting macro %NEWSURV and an introduction to expansion macros (Accessed Proceeding of Pharmaceutical SAS Users Group Conference (PharmaSUG 2017)).
Millspaugh J. J., Marzluff J. M. (2001). Radio tracking and animal populations (London: Academic Press). doi: 10.1016/B978-012497781-5/50016-5
Naef-Daenzer B., Grüebler M. U. (2016). Post-fledging survival of altricial birds: Ecological determinants and adaptation. J. Field Ornithol. 87, 227–250. doi: 10.1111/jofo.12157
Naef-Daenzer B., Korner-Nievergelt F., Fiedler W., Grüebler M. U. (2017). Bias in ring-recovery studies: causes of mortality of little owls Athene noctua and implications for population assessment. J. Avian Biol. 48, 266–274. doi: 10.1111/jav.00947
Naef-Daenzer B., Widmer F., Nuber M. (2001). Differential post-fledging survival of great and coal tits in relation to their condition and fledging date. J. Anim. Ecol. 70, 730–738. doi: 10.1046/j.0021-8790.2001.00533.x
Newton I., McGrady M. J., Oli M. K. (2016). A review of survival estimates for raptors and owls. Ibis 158, 227–248. doi: 10.1111/ibi.12355
Overskaug K., Bolstad J. P., Sunde P., Øien I. J. (1999). Fledgling behavior and survival in northern tawny owls. Condor 101, 169–174. doi: 10.2307/1370460
Petty S. J., Thirgood S. J. (1989). A radio tracking study of post-fledging mortality and movements of tawny owls in Argyll. Ringing Migr. 10, 75–82. doi: 10.1080/03078698.1989.9673943
Robles H., Ciudad C., Vera R., Baglione V. (2007). No effect of habitat fragmentation on post-fledging, first-year and adult survival in the middle spotted woodpecker. Ecography 30, 685–694. doi: 10.1111/j.2007.0906-7590.05179.x
Rohner C. (1996). The numerical response of great horned owls to the snowshoe hare cycle: Consequences of non-territorial ‘floaters’ on demography. J. Anim. Ecol. 65, 359–370. doi: 10.2307/5882
Rohner C., Hunter D. B. (1996). First-year survival of great horned owls during a peak and decline of the snowshoe hare cycle. Can. J. Zool.-Rev. Can. Zool. 74, 1092–1097. doi: 10.1139/z96-121
Šálek M., Poprach K., Opluštil L., Melichar D., Mráz J., Václav R. (2019). Assessment of relative mortality rates for two rapidly declining farmland owls in the Czech Republic (Central Europe). Eur. J. Wildl. Res. 65. doi: 10.1007/s10344-019-1253-y
Salinas-Melgoza A., Renton K. (2007). Postfledging survival and development of juvenile lilac-crowned parrots. J. Wildl. Manage. 71, 43–50. doi: 10.2193/2005-646
Schreiber-Gregory D. N., Jackson H. M. (2017). “Multicollinearity: What is it, why should we care, and how can it be controlled,” in Proceedings of the SAS R Global Forum 2017, Conference Paper. 1404.
Sergio F. (2003). From individual behaviour to population pattern: Weather-dependent foraging and breeding performance in black kites. Anim. Behav. 66, 1109–1117. doi: 10.1006/anbe.2003.2303
Stehlíková Sovadinová S., Kouba M., Ševčík M., Tulis F., Bušina T., Korpimäki E. (2024). Home range size of Tengmalm’s owl offspring during the post-fledging dependence period in Central and North Europe. Front. Ecol. Evol. 12. doi: 10.3389/fevo.2024.1347916
Sunde P. (2005). Predators control post-fledging mortality in tawny owls, Strix aluco. Oikos 110, 461–472. doi: 10.1111/j.0030-1299.2005.14069.x
Tarwater C. E., Ricklefs R. E., Maddox J. D., Brawn J. D. (2011). Pre-reproductive survival in a tropical bird and its implications for avian life histories. Ecology 92, 1271–1281. doi: 10.1890/10-1386.1
Todd L. D. (2001). Dispersal patterns and post-fledging mortality of juvenile burrowing owls in Saskatchewan. J. Raptor Res. 35, 282–287.
Todd L. D., Poulin R. G., Wellicome T. I., Brigham R. M. (2003). Post-fledging survival of burrowing owls in Saskatchewan. J. Wildl. Manage. 67, 512–519. doi: 10.2307/3802709
Tome D. (2011). Post-fledging survival and dynamics of dispersal in long-eared owls Asio otus. Bird Study 58, 193–199. doi: 10.1080/00063657.2011.559531
Tornberg R., Korpimäki E., Byholm P. (2006). Ecology of the northern goshawk in Fennoscandia. Stud. Avian. Biol. 31, 141–157.
Valkama J., Saurola P., Lehikoinen A., Lehikoinen E., Piha M., Sola P., et al. (2014). The finnish bird ringing atlas. Vol. II (Helsinki, Finland: Finnish Museum of Natural History and Ministry of Environment).
Varland D. E., Klaas E. E., Loughin T. M. (1993). Use of habitat and perches, causes of mortality and time until dispersal in postfledging American kestrels. J. Field Ornithol. 64, 169–178.
Vormwald L. M., Morrison M. L., Mathewson H. A., Cocimano M. C., Collier B. A. (2011). Survival and movements of fledgling willow and dusky flycatchers. Condor 113, 834–842. doi: 10.1525/cond.2011.110009
Weathers W. W., Sullivan K. A. (1989). Juvenile foraging proficiency, parental effort, and avian reproductive success. Ecol. Monogr. 59, 223–246. doi: 10.2307/1942600
White G. C., Garrott R. A. (1990). Analysis of wildlife radio-tracking data (San Diego: Academic Press).
Wiens J. D., Noon B. R., Reynolds R. T. (2006). Post-fledging survival of northern goshawks: The importance of prey abundance, weather, and dispersal. Ecol. Appl. 16, 406–418. doi: 10.1890/04-1915
Wobeser A. G. (2009). “Parasitism: costs and effects,” in Parasitic diseases of wild birds. Eds. Atkinson C. T., Thomas N. J., Hunter D. B. (Wiley-Blackwell, Ames: IA), 3–9.
Work T. M., Meteyer C. U., Cole R. A. (2004). Mortality in Laysan ducks (Anas laysanensis) by emaciation complicated by Echinuria uncinata on Laysan Island, Hawai. J. Wildl. Dis. 40, 110–114. doi: 10.7589/0090-3558-40.1.110
Zárybnická M., Korpimäki E., Griesser M. (2012). Dark or short nights: Differential latitudinal constraints in nestling provisioning patterns of a nocturnally hunting bird species. PloS One 7, e36932. doi: 10.1371/journal.pone.0036932
Zárybnická M., Riegert J., Kouba M. (2015b). Indirect food web interactions affect predation of Aegolius funereus nests by pine martens Martes martes according to the alternative prey hypothesis. Ibis 157, 459–467. doi: 10.1111/ibi.12265
Keywords: bacteria, birds of prey, mortality rate, parasites, predation, prey abundance, radio-telemetry, starvation
Citation: Kouba M, Bartoš L, Tulis F, Stehlíková Sovadinová S and Korpimäki E (2024) Survival rates and causes of death in Tengmalm’s owl offspring during the post-fledging dependence period in Central and North Europe. Front. Ecol. Evol. 12:1388643. doi: 10.3389/fevo.2024.1388643
Received: 20 February 2024; Accepted: 29 April 2024;
Published: 22 May 2024.
Edited by:
Pascual López-López, University of Valencia, SpainReviewed by:
Hermann Wagner, RWTH Aachen University, GermanySarah Hill, Appalachian State University, United States
Patrik Karell, Lund University, Sweden
Copyright © 2024 Kouba, Bartoš, Tulis, Stehlíková Sovadinová and Korpimäki. This is an open-access article distributed under the terms of the Creative Commons Attribution License (CC BY). The use, distribution or reproduction in other forums is permitted, provided the original author(s) and the copyright owner(s) are credited and that the original publication in this journal is cited, in accordance with accepted academic practice. No use, distribution or reproduction is permitted which does not comply with these terms.
*Correspondence: Marek Kouba, bWFyZWtrb3ViYThAZ21haWwuY29t
†ORCID: Marek Kouba, orcid.org/0000-0003-2262-5733
Luděk Bartoš, orcid.org/0000-0003-2667-5930
Filip Tulis, orcid.org/0000-0003-2673-5630
Simona Stehlíková Sovadinová, orcid.org/0009-0008-9431-5156
Erkki Korpimäki, orcid.org/0000-0001-7596-1955