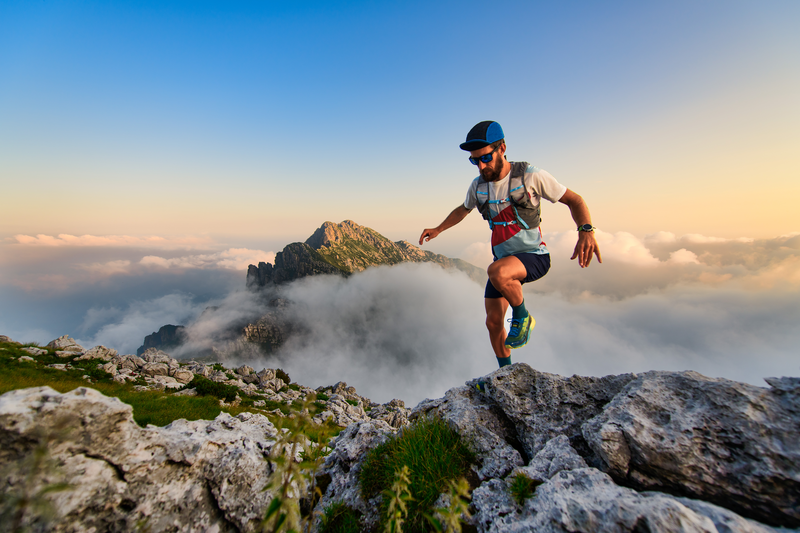
94% of researchers rate our articles as excellent or good
Learn more about the work of our research integrity team to safeguard the quality of each article we publish.
Find out more
ORIGINAL RESEARCH article
Front. Ecol. Evol. , 10 July 2024
Sec. Paleoecology
Volume 12 - 2024 | https://doi.org/10.3389/fevo.2024.1381539
This article is part of the Research Topic Changes in Plant-Herbivore Interactions Across Time Scales: Bridging Paleoecology and Contemporary Ecology View all 6 articles
Plants and insects are two of the more diverse and abundant organisms in terrestrial ecosystems. The fossil record of plant-insect interactions offers crucial insights into the coevolutionary dynamics between these groups, shedding light on the intricate relationships that have shaped terrestrial ecosystems. The study of fossil interactions is especially relevant in mid-Cretaceous ecosystems, a time of dramatic changes in the composition of floras and, consequently, in plant-insect relationships. Here, we describe the first suite of plant-insect interactions from the mid-Cretaceous of Mexico. We studied 554 plant fossils from the El Chango Lagerstätte (Cintalapa Formation, Chiapas, Mexico), including vegetative (leaves) and reproductive structures (fruits and seeds). The flora was dominated by gymnosperms (89.3%) followed by angiosperms (10.7%); other groups, such as pteridophytes and bryophytes, were absent. In total, 5.4% of the plant specimens hosted some damage. Angiosperms (all broad-leafed forms), despite being much less common than gymnosperms, expressed more evidence of damage by herbivores (35.6% of specimens damaged). In contrast, the narrow-leafed gymnosperms, the dominant group in the flora, hosted a much lower proportion of herbivory damage (1.8% of specimens damaged). The diversity of damage types (DTs) was relatively low: 14 DTs were identified, corresponding to seven FFGs, including margin feeding, hole feeding, surface feeding, piercing and sucking, oviposition, galling, and mining. Comparison with the other mid-Cretaceous plant-insect assemblages reveals a similar richness of DTs for angiosperms but a lower richness and diversity of DTs on gymnosperms from El Chango. These results indicate preferential herbivory on angiosperms (rather than on the available gymnosperms in the assemblage) by terrestrial arthropods during a period of major changes in the structure of terrestrial ecosystems. However, it is challenging to resolve whether this apparent preference is because insects particularly targeted angiosperms or if the herbivores simply targeted broad leaves in general, since most of the available gymnosperms from El Chango are scale-leafed forms.
Plants and arthropods represent the two dominant groups with respect to biodiversity and biomass in terrestrial ecosystems (Bar-On et al., 2018). Their importance extends back to the earliest terrestrial biotas, where fossil interactions provide evidence of the structure of the first land-based ecosystems (Labandeira and Wappler, 2023). Fossil evidence of plant-insect interactions provides one of the few windows into the past relationships between these two groups, offering tangible records of coevolutionary processes and provides valuable data on the evolutionary history of both plants and insects. These data enhance our understanding of the structure and development of ancient ecosystems, biodiversity patterns, and the intricate web of biotic relationships that have shaped the natural world over geological time scales (e.g., Mcloughlin et al., 2015; Santos et al., 2021, Santos et al., 2022a, Santos et al., 2022b, Santos et al., 2022c, Santos et al., 2023; Labandeira and Wappler, 2023).
Plant-arthropod interactions have been recorded in the fossil record since the Silurian-Devonian (Scott et al., 1992; Hagström and Mehlqvist, 2012), during the early colonization of terrestrial environments by plants. Since then, the interactions between these two groups have become more complex and abundant in the fossil record and have experienced successive phases of development. During the mid-Cretaceous, the Cretaceous Terrestrial Revolution (KTR), and the closely related Angiosperm Terrestrial Revolution (ATR), occurred. This interval encompassed significant changes in the composition, structure and complexity of terrestrial ecosystems, especially marked by the diversification and expansion of angiosperms, which came to dominate plant communities worldwide (McLoughlin et al., 1995, 2010; Villanueva-Amadoz et al., 2010; Coiffard et al., 2012; Condamine et al., 2020; Santos et al., 2022a; Xiao et al., 2022a; 2023). These changes at the base of the food chain led to significant shifts in the representation, morphology, and behaviour of numerous groups of terrestrial parasites, saprotrophs, and consumers, among which insects were key (Peris and Condamine, 2024). Some previous works (see Table 1 for details) suggested that, in Europe, insect herbivores adapted rapidly to angiosperms, targeting them as their preferred food source in some areas (Santos et al., 2022a). However, little is known about how these floristic changes affected the dynamics of herbivory in other regions of the world.
Table 1 Selection of Cretaceous plant-insect interactions. Modified from Santos et al. (2022a).
Evidence of fossil plant-insect interactions from Mexico is scarce, thus far being confined to studies of just a few specimens. The oldest fossil evidence of interactions from that country is from the Permian Matzitzi Formation (Flores-Barragán et al., 2023). There has been a single study of Jurassic interactions from the Otlaltepec Formation from which 22 bennettitalean and fern remains were reported with some evidence of herbivory damage (Velasco de León et al., 2015). There are a few reports of fungal-insect interactions in Miocene amber samples from Chiapas (Arroyo-Sánchez et al., 2023). Thus far, no formal studies have been published on plant-insect interactions from the Cretaceous of Mexico. Our study provides the first evidence of plant-insect interactions from the mid-Cretaceous of Mexico and southern portion of North America. This offers the opportunity to explore how insects reacted to the profound changes in terrestrial ecosystems in the region that would become the precursor to the modern Neotropical biogeographic realm (Carvalho et al., 2021). The main objectives of this work are to: 1, document the first occurrences of plant-insect interactions from the El Chango Lagerstätte; 2, identify and discuss the diversity and intensity of interactions expressed by Damage Types (DTs) and Functional Feeding Groups (FFGs) in this paleoforest; and 3, statistically appraise the palaeoecology of this fossil site.
The El Chango outcrop hosts a fossil Lagerstätte and is located 30 km southeast of the Guadalupe Victoria locality, Chiapas, southeastern Mexico (coordinates: N 16°34’14”, W 93°16’11”: Figures 1A, B). Strata exposed at this site belong to the Cintalapa Formation within the Sierra Madre Group. This group consists of a 700–1600-m-thick succession of marine laminated dolomites with sporadic flint levels (Moreno-Bedmar et al., 2014).
Figure 1 (A, B) Geographical and geological map showing (white dot in A and star in B) the position of El Chango Lagerstätte locality (Chiapas, Mexico). (C) Stratigraphic section of the studied interval of the Cintalapa Formation indicating the plant-bearing levels (star) and the lithological characters. M: Mudstone; W: Wackestone; P: Packstone. (Modified from Moreno-Bedmar et al., 2014).
The sedimentary succession at El Chango (Figure 1C) was deposited in a brackish estuary or salty lagoon with episodic influx of freshwater (Vega et al., 2006; Moreno-Bedmar et al., 2014). The remains of several marine/coastal organisms have been found in the Cintalapa Formation. The fossil assemblages are recognized globally for their exceptional marine fish diversity and preservation, as documented by, e.g., Alvarado-Ortega et al. (2009), Alvarado-Ortega and Than-Marchese (2012, 2013), Díaz-Cruz et al. (2019, 2020), Than-Marchese et al. (2020) and Cantalice et al. (2021). Additionally, deposits from this formation contain significant fossils of decapods (e.g., Vega et al., 2006; 2022; Bruce et al., 2021), plants (González-Ramírez et al., 2013; Guerrero-Márquez et al., 2013; this study), and even insects, including remains of Odonata and Hemiptera (Vega et al., 2006).
According to Vega et al. (2006), the El Chango deposits are late Albian in age. However, Moreno-Bedmar et al. (2014) suggested an earliest Cenomanian age for the upper part of the stratigraphic section at El Chango based on the presence of diagnostic ammonoids (Graysonites: Acanthoceratidae). The lower part of the section has not been calibrated with ammonoids, and consequently, a late Albian age for this interval cannot be excluded. Mexico was located in low northern latitudes (<15°N) during the mid-Cretaceous (McLoughlin, 2001).
We noted 858 plant remains from the El Chango site. Only well-preserved terrestrial plant remains with dimensions of at least 2 × 2 cm were selected for this study. This left an inventory of 554 plant fossils (See details in the Supplementary Data), including vegetative remains (536 leaves, 97%; Figures 2–6) and reproductive structures (18 fruits and seeds, 3%; Figures 3, 5). The plant fossils are preserved as carbonaceous films or impressions that were analyzed for evidence of interactions. The relatively good preservation of the leaves suggests that transport distances were short, indicating a parautochthonous origin of the plant fossils and, consequently, of the interactions.
Figure 2 Various DTs of margin feeding on basal angiosperms at El Chango. (A) Two occurrences of DT012 on Eucalyptolaurus sp. Scale Bar = 1 cm (10 mm); (B) Margin feeding with developed reaction rim (DT200) and hole feeding on the left side of the leaf (DT113) in Angiosperm MF16. Scale Bar = 10 mm; (C) Evidence of DT012 on aff. Sapindopsis sp. Scale Bar = 10 mm; (D) Detail of A showing the dark reaction rim of one DT012 (see white arrow) on Eucalyptolaurus sp. Scale Bar = 5 mm; (E) Detail of B, white arrow shows the slightly cuspate margin and thickened reaction rim of a DT200. Scale Bar = 5 mm; (F) Detail of C, showing the DT012 on aff. Sapindopsis sp.; Scale Bar = 5 mm.
Figure 3 Various DTs of hole feeding on early angiosperms from the studied site. (A) Leaf of Angiosperm MF7 with some hole-feeding interactions (DT001). Scale Bar = 10 mm; (B) Polylobate hole feeding (DT005) on Angiosperm MF3. Scale Bar = 10 mm; (C) Angiosperm fruit (Papaveraceae-like fruit) bearing some herbivory-defense structures (spines) and with a possible hole-feeding scar (DT001). Scale Bar = 10 mm; (D) Detail of A, white arrow shows the reaction rim around the small hole (DT001) in the angiosperm leaf. Scale Bar = 1 mm; (E) Detail of A, white arrow shows the plant reaction to the hole-feeding damage (DT001). Scale Bar = 1 mm; (F) Detail of the irregular (polylobate) hole feeding (DT005) illustrated in (B). Scale Bar = 5 mm; (G) Detailed photo of C, showing more detail on the spines of the Papaveraceous-like fruit and the putative DT001 (see white arrow).
Figure 4 Various DTs of surface feeding, oviposition, piercing and sucking, and mining on basal angiosperms of El Chango site. (A) Angiosperm MF13 with linear mining in the right side of the leaf (DT129). Scale Bar = 10 mm; (B) aff. Sapindopsis sp. with an oviposition scar in the midvein (DT076; see detail of red square in (D). Scale Bar = 10 mm; (C) Surface feeding on Angiosperm MF11 (DT030; Detail in G). Scale Bar = 10 mm; (D) Detail of the oviposition mark (see white arrow) in aff. Sapindopsis sp. Scale Bar = 5 mm; (E) Possible piercing and sucking interaction (aff. DT053) in Angiosperm MF11. Scale Bar = 5 mm; (F) Enlargement of A, showing details of the linear mining (DT129), white arrows indicate the reaction rim surrounding this interaction. Scale Bar = 5 mm; (G) Detail of the damage patch corresponding to Surface Feeding (DT030). Scale Bar = 5 mm.
Figure 5 Various DTs of piercing and sucking, and galling on some gymnosperms and fruits from the El Chango site. (A) Gymnosperm branch of cf. Geinitzia sp. showing a putative gall (similar structure to DT336). Scale Bar = 10 mm; (B) Indeterminate angiosperm fruit showing an attached fish coprolite (upper white arrow) and a gall-like structure (lower white arrow). Scale Bar = 10 mm; (C) Piercing and sucking marks on a leaf of Angiosperm MF8 consisting of several slightly convex styletal punctures (DT047) Scale Bar = 5 mm; (D) Detail of the pedunculate gall emerging from a cf. Geinitzia sp. branch: Scale Bar = 5 mm; (E) Zoom on C, showing details on the styletal punctures (DT047). Scale Bar = 1 mm; (F) Specimen of cf. Geinitzia sp. bearing falcate leaves spreading from the axis, showing piercing and sucking marks on the upper leaf (see details on G). Scale Bar = 5 mm; (G) Detail of (F), showing one falcate leaf of cf. Geinitzia sp. with a concave styletal puncture (DT046). Scale Bar = 1 mm; (H) Enlargement of the piercing and sucking mark (see white arrow).
Figure 6 Putative plant-algae interaction (epibiont of marine/brackish algae on terrestrial gymnosperms from El Chango site. (A) Specimen of cf. Geinitzia sp. is affected by attached filaments of putative Chlorophyta sensu lato (see discussion). Scale Bar = 10 mm; (B) Unaffected specimen of cf. Geinitzia sp. from the same site). Scale Bar = 10 mm; (C) Detail of the putative algae filaments coating the leaves and the axis. Scale Bar = 5 mm; (D) Detail on the thin filaments that divide in a dichotomous pattern (see arrows). Scale Bar = 1 mm.
The fossils were prepared following standard techniques in the Department of Palaeontology in the IGL-UNAM, using a micropneumatic hammer and needles. Selected fossils were photographed using a Canon EOSM50, and detailed photos were taken with a micro-camera Axiocam 503 Color attached to a Zeiss Axio Zoom V.16 microscope. Some samples were photographed submerged in distilled H2O to improve image contrast and quality. The fossils will be stored in the Laboratory of Palaeobotany of Dr. Sergio RS Cevallos-Ferriz in the IGL-UNAM, Ciudad de México.
We have followed the classification system proposed by Labandeira et al. (2007) to identify plant-arthropod interactions. In this system, the interactions observed on the leaves are classified into different morphotypes, named Damage Types (hereafter DTs), followed by an identifying number, where most of the DTs belong to specific Functional Feeding Groups (hereafter FFGs). Additionally, each DT is connected to a certain degree of specialization, based on the occurrence on different host plants (Labandeira et al., 2007).
The statistical analyses were undertaken using R i386 3.6.0 (R Development Core Team) and R Commander. We have used codes based on Gunkel and Wappler (2015) to calculate the richness and rarefaction of DTs and FFGs (Figures 7, 8); to obtain standard deviations, we employed the procedures of Heck Jr et al. (1975). Rarefaction was calculated using both damaged and undamaged plant remains. Statistically analyzed plant-insect associations from Cretaceous deposits are scarce, which limits the options for comparisons with other floras. To make comparisons between the interactions of El Chango and other assemblages of mid-Cretaceous (especially Cenomanian) plant-insect interactions, we re-processed the data of Santos et al. (2022a) from the early Cenomanian plant assemblage from Puy-Puy (France), which was the only available relevant dataset from this time interval (Figure 8). We analyzed the rarefaction of DTs and FFGs of the Puy-Puy dataset following the same methodology applied to our Mexican data.
Figure 7 (A) Number of specimens used in this study indicating the total quantity of gymnosperms and angiosperms; (B) Incidence of affected specimens for angiosperms, gymnosperms, and the whole flora. (C) Rarefaction curves showing the diversity of all DTs, specialized feeding, and external feeding at El Chango. (D) Differences in the rarefaction of diversity damage in angiosperms and gymnosperms from the El Chango site.
Figure 8 Comparison between DT rarefaction from the Lagerstätte floras of El Chango (Mexico, North America) and Puy-Puy (France, Europe; Santos et al., 2022a). Including the whole floras (A), the angiosperms (B), and the gymnosperms (C). Puy-Puy is represented by blue, and El Chango by orange.
The fossil assemblage at El Chango is dominated by gymnosperms (495 specimens; 89.3%; Figure 7A). Angiosperm remains are remarkably scarce in the assemblage (59 specimens; 10.7%; Figure 7A). The most abundant remains were attributed to the conifer Geinitzia sp. (62.3% relative abundance), followed by Podocarpaceae indet. (13.9%) and by the cupressacean Brachyphyllum sp. (10.1%). Other taxa or morphotypes represent less than 10% of the assemblage. Angiosperms, such as Sapindopsis sp. (Platanaceae; Figures 1C, 2) and Eucalyptolaurus sp. (Lauraceae; Figure 1C), were also present in the assemblage. Poor preservation of some angiosperm leaves did not permit identification to genus or species level. Consequently, some were classified into various “angiosperm morphotypes”.
The taxon that dominates the plant fossil assemblage, Geinitzia sp., has xerophytic adaptations (Moreau et al., 2021). The third-most abundant genus (Brachyphyllum: 9.1%) also has xerophytic characters, e.g., small appressed leaves, thick cuticle, and sunken stomata to limit water loss (Moreau et al., 2022). There is also a remarkable dearth of hygrophilous plants, such as ferns and bryophytes, in this mid-Cretaceous assemblage. These groups, especially ferns, are common in other Cretaceous floras (being represented in both macrofloras and palynofloras) from the Northern Hemisphere (e.g., Vajda et al., 2013; Villanueva-Amadoz et al., 2014; Santos et al., 2018, 2022a; Estrada-Ruiz et al., 2018; Rodríguez-Barreiro et al., 2022; 2024; Cevallos-Ferriz et al., 2022; Herman and Domogatskaya, 2023; Martínez de Espronceda et al., 2024). Cycadales and Bennettitales were not recorded in the fossil flora. The absence of these taxa in the assemblage does not necessarily mean their absence in the biocenosis since taphonomic processes may have excluded them. On the other hand, the flora also contained seeds and fruits, some of which bore potential anti-herbivore defences, e.g., the spinescence expressed in the Papaveraceae-like fruit (Figures 3C, G).
Fourteen DTs were identified in the plant assemblage (Figures 7C, D) corresponding to seven FFGs, including two DTs of margin feeding (DT012, DT200), four DTs of hole feeding (DT001, DT005, DT008, DT113), one DT of surface feeding (DT030), three DTs of piercing and sucking (DT046, DT047, DT053), one example of oviposition (DT076), two putative DTs of galling (DT080, and a galling structure that shares some affinities with DT336), and one DT corresponding to mining (DT129).
Of the 554 plant remains included in the study, only 5.4% show evidence of herbivory or oviposition scars (Figure 7B). Nevertheless, when this richness of damage is analyzed by botanical groups, the results show high disparity. Only 1.8% of gymnosperm specimens were damaged, whereas 35.6% of angiosperms bore damage features (Figure 7B). The frequency of arthropod damage varied among each FFG. Hole feeding was the most common functional feeding group (2.5%), followed by margin feeding (present in 1.1% of specimens), piercing and sucking (1.1% of affected plants), galling (0.9%), mining (0.2%), and surface feeding (0.2%). No clear evidence of skeletonization, seed predation, or pathogen attack was found in the El Chango flora.
Two specimens of cf. Geinitzia sp. host intriguing plant-algae interactions (Figure 6). Delicate filamentous structures coat the branch axis and its helically arranged leaves, forming dense threads around these gymnosperms. The distinct arrangement of these filaments, exclusive to the gymnosperms and absent in the adjacent rock matrix, strongly suggests a preferential association with the plant. Detailed discussion of this specimen is included in the end of the following section.
We identified seven FFGs and 14 DTs in the El Chango Lagerstätte, representing a generally low diversity of interactions. Herbivory is represented predominantly by relatively simple hole feeding (2.5% incidence in the flora), piercing and sucking (1.1% of affected plants), and margin feeding (1.1% of the flora). Most identified DTs were found on broad-leafed angiosperm remains (12 DTs), whereas only two DTs were identified in gymnosperms (Figure 7D). The absence of other FFGs, such as skeletonization or pathogen damage, does not necessarily imply the absence of insects, fungi, or other micro-organisms with these feeding styles in the parent biota but may indicate taphonomic biases. We also note that the rarefaction curve for all DTs is not stabilized at the 536 specimen level (Figure 7C), suggesting that if more samples are recovered from El Chango more diversity of damage would likely be identified.
According to the host specificity index, the interactions from the El Chango site have similar diversity of specific damage (six DTs) to that of generalist damage (external damage; seven DTs). Specialized interactions in the fossil record are commonly attributed to monophagous and particularly oligophagous insects (Currano et al., 2008, 2010; Adroit et al., 2016). In contrast, polyphagous insects are generally associated with more general interactions (Currano et al., 2008). The similar levels of diversity and abundance between specific and generalist damage in the El Chango flora suggest that oligophagous and polyphagous insects were equally important in this gymnosperm-dominated mid-Cretaceous paleoforest.
The plant assemblage at the El Chango site shows a significant disparity in the richness of damage for each plant group and genus. Gymnosperms represent only 1.8% of the specimens with one or more DTs. This contrasts with the dominance of this group in the total flora (89.3%). In comparison, 35.6% of angiosperms were damaged by insects or other arthropods or mollusks, yet flowering plants constitute only 10.7% of the plant specimens in this Cretaceous flora. This indicates that the regional entomofauna targeted broad angiosperm leaves in preference to the available scale-leafed gymnosperms, but it is not clear whether this differential distribution was due to preferences for broad leaf form or for flowering plants per se. No broad-leafed gymnosperms or scale-leafed angiosperms are available in the El Chango assemblage to test these alternative possibilities. In any case, the relatively abundance of damage on angiosperms is striking given that, during the Albian–Cenomanian, angiosperms had only recently emerged as a significant component of the vegetation and were still in their early phase of diversification (Friis et al., 2011). Insects, having adapted to interactions with pteridophytes and gymnosperms over hundreds of millions of years, might be expected to require significant time to adjust to herbivory on this new plant group. However, our findings indicate that the entomofauna were already well adapted to feed on early angiosperms during the mid-Cretaceous in the tropics. This implies a relatively rapid adjustment of diverse insect clades to the emerging plant group that would soon dominate terrestrial ecosystems.
To resolve whether the insects from El Chango had a preference for feeding on angiosperms is challenging in the absence of broad-leafed gymnosperms, but we hypothesize that the small, thick and appressed leaves of the preserved conifers may have been inhibitory to some insect herbivores. Among the most dominant taxa in the El Chango assemblage were the conifers Geinitzia and Brachyphyllum (collectively accounting for 72.4% of the flora), which have narrow and coriaceous leaves and thick cuticles. Brachyphyllum has been interpreted as coniferous (with various species affiliated to Araucariaceae, Cheirolepidiaceae, or Cupressaceae) and having xerophytic adaptations to semi-arid conditions (Moreau et al., 2022). Geinitzia sp. also bears reduced coriaceous leaves, and some low-palaeolatitude species of this genus have been linked by morphological adaptations and histology to high temperatures and aridity (Moreau et al., 2021; see also discussion by Santos et al., 2022a). These adaptations may have served as deterrents to herbivorous arthropods.
In contemporary plant studies, it has been established that environmental conditions play a pivotal role in determining plant palatability across various species (Münzbergová and Skuhrovec, 2013; Kuglerová et al., 2019). Specifically, arid and semi-arid conditions have been identified as factors that decrease plant palatability to insects across multiple plant species (e.g., Blumenthal et al., 2020). Consequently, the presence of plants adapted to semi-arid conditions may have contributed to a reduction in foliar palatability at El Chango. This reduction may have resulted in low insect populations and diversity, consequently contributing to the relatively low richness and diversity of DTs in this flora. This observation aligns with contemporary research on plant-insect interactions (e.g., Münzbergová and Skuhrovec, 2013; Kuglerová et al., 2019), indicating that aridity is associated with decreased network metrics related to plant-insect interactions.
Moreover, aridity negatively correlates with network specialization and the modularity of local webs (e.g., Santos-Neto et al., 2022). Furthermore, recent studies on herbivory have revealed that certain insects alleviate pressure on their host plants in response to rising temperatures (Laws and Belovsky, 2010). In this context, some of the discussed gymnosperms are indicative arid or semi-arid conditions, at least during certain parts of the year. This climatic influence might also contribute to the lower palatability observed in most specimens within this flora.
In the El Chango flora, various anti-herbivore adaptations have been identified, exemplified by the Papaveraceae-like fruit (Figures 3C, D). Features such as spines and thorns, found in multiple parts of the plants, are commonly interpreted as defences against herbivores (Hanley et al., 2007). Notably, spinescence is generally deemed more effective against vertebrates than invertebrates (Cooper and Owen-Smith, 1986; Hanley et al., 2007). Most plants in this assemblage bore leaves with at least some morphological feature(s) contributing to unpalatability and anti-herbivore defences. This aligns with the observed greater herbivory and trace diversity in angiosperms, which appeared more vulnerable to attacks by generalist insects.
The herbivory rates found in the El Chango fossil flora are relatively low (5.4% of affected specimens), especially when compared to quantitative data on plant-arthropod interactions from other Cretaceous sites, such as the Albian flora of Estercuel, Spain (Santos et al., 2023), the Cenomanian flora of Puy-Puy, France (Santos et al., 2022a), which reach 20.4% and 22.2%, respectively, or the Albian flora from Rose Creek in USA (Xiao et al., 2022a), which has a higher proportion (45.6%) of damaged specimens. Only those three mid-Cretaceous floras from the North Hemisphere have been subjected to quantitative or semi-quantitative analyses of plant-insect interactions (Table 1). They are relatively close in age to the El Chango flora. Additionally, the fact that some authors of the current study participated in identifying interactions in two of the other floras reduces the potential human bias for detecting and interpreting different damage types. However, other influences, such as taphonomic bias, should be considered and may ultimately limit the scope of comparisons between these various mid-Cretaceous floras. Sampling limitations (only 72 specimens available) on the mid-Cretaceous flora from Spain (Santos et al., 2023) potentially bias the results from that assemblage. However, the interactions recorded in the mid-Cretaceous flora of France were based on an assemblage of more than 1500 plant specimens (Santos et al., 2022a), enabling more confident comparisons.
The richness of interactions at El Chango is relatively low if the flora is considered as a whole (5.4%), notably lower than the 20–22% observed in other paralic floras from the mid-Cretaceous of Spain (Santos et al., 2023) or France (Santos et al., 2022a). However, this percentage at El Chango must be considered in context. Although, superficially, it may seem like a low ratio, when the interactions are assessed by botanical groups, we note that these values reach percentages of around 36% in angiosperms, representing similar or even higher values than those found in other mid-Cretaceous floras. For example, the herbivory rate on the angiosperms of the Estercuel flora (East Spain) was 20.4%, and on the angiosperms of the Cenomanian flora at Puy-Puy was 34%. Nevertheless, the small number of angiosperms at El Chango might have influenced the results, and the patterns of herbivory should be interpreted cautiously. Ideally, more fossils of angiosperms from El Chango should be recovered from the site to support this interpretation.
The slightly older Albian flora from Rose Creek (USA) includes 114 DTs on about 2000 plant specimens (Xiao et al., 2022a), suggesting much greater diversity of herbivory styles than in the El Chango flora (14 DTs). This difference might be due to greater sampling effort (554 specimens vs 2084 at Rose Creek), but also to the higher plant diversity of the Rose Creek flora (about 24 species/morphotypes in El Chango vs 50 species/morphotypes in the Rose Creek flora). In addition, the Rose Creek flora includes a greater abundance of angiosperms (which are usually more herbivorized than gymnosperms; e.g., Santos et al., 2022a), whereas at El Chango, angiosperms are scarce. On the other hand, DT diversity in the early Cenomanian of France (coeval with the assemblage at El Chango) is significantly greater than that in the Mexican flora, with 71 DTs compared to 14 DTs. This evident disparity is linked to the difference in sample sizes, i.e., 1605 specimens analyzed from Puy-Puy versus 554 (536 leaves) specimens from El Chango. When considering rarefaction curves of DT diversity at an equivalent sampling level (554 specimens; 536 leaves), they confirm the heightened diversity of damage types in the Cenomanian of France (43 DTs) compared to Mexico (14 DTs; Figure 8A). Examining rarefaction curves specifically for DT diversity in angiosperms (Figure 8B) reveals a notable similarity in damage diversity at the same sampling level (approximately 13 DTs in France versus 11 DTs in Mexico at 50 angiosperms of rarefaction). Crucially, when considering the standard deviation of damage diversity in angiosperms, it is evident that the mid-Cretaceous assemblages demonstrate comparable variability at the same sampling level. The disparity in general DT diversity is attributed to differences in damage on gymnosperms. At an equivalent sampling level (about 500 specimens), gymnosperms from Puy-Puy host a higher diversity of traces than those from El Chango (26 DTs vs 4 DTs). The most parsimonious explanation lies in the anatomical differences in the gymnosperms between the two localities.
In Puy-Puy, gymnosperms are represented predominantly by the coniferous Dammarophyllum (constituting 46.3% of the assemblage), a genus characterized by broad leaves that are more susceptible to insect damage (Santos et al., 2022a). Most of gymnosperms at El Chango have small, appressed awl-shaped leaves with thick cuticles that are presumably less palatable. On this basis, angiosperms were a proportionately more significant food source for insects and other herbivorous arthropods and mollusks in the coastal paleoforests of North America than in equivalent settings in Europe during the mid-Cretaceous.
Despite the Puy-Puy flora hosting markedly more diverse and common interactions, potential taxonomic biases (owing to fewer plant species in El Chango) and taphonomic biases (greater preservational potential of robust conifers at El Chango) offer plausible explanations for these differences. Although marked differences exist between the assemblages, a common pattern is evident in the plant-insect interactions. In both gymnosperm-dominated floras from Europe and North America, insects clearly preferred angiosperms. Although there are variations in the specific compositions of the assemblages, the higher amounts of damage on angiosperms leaves in these mid-Cretaceous paleoforests prompts the need for further research. More comprehensive investigations into mid-Cretaceous plant assemblages and plant-insect interactions are essential to unravel the role that this apparent herbivory pressure on early angiosperms played in the radiation and dominance of terrestrial ecosystems by flowering plants during the KTR.
Other types of biotic associations have been found in El Chango assemblage, for example, the plant-algae interaction found on cf. Geinitzia sp. (Figure 6). Given their morphology and dichotomous habit, we confidently exclude these filaments from constituting another part of the host plant, such as branches or roots of Geinitzia sp. (white arrows in Figure 6D). Additionally, the minute size of these filaments (around 0.1 mm in diameter) rules out the possibility that they are filaments from another parasitic angiosperm (such as Cuscuta sp.); and they are too large and robust to be fungal hyphae. Although the simple morphology of these tangled filaments makes their assignment to a specific taxonomic group impossible, we argue that the most parsimonious explanation is that they are filaments of some epibiont green algae growing on the robust branch in a marine setting before burial. Plants washed into fluvial, estuarine and marine systems can have long and complex taphonomic histories, developing interactions with a broad range of epibionts and saprotrophs (Philippe et al., 2022). The depositional environment at the El Chango site was a lagoon or estuary (e.g., Moreno-Bedmar et al., 2014). Hence, terrestrial plants may have been washed into this brackish water environment and submerged for extended periods before burial, allowing colonization by filamentous green algae epibionts.
The mid-Cretaceous El Chango Lagerstätte in Mexico provides unique insights into plant-insect interactions during a pivotal period of changes in the evolution of terrestrial ecosystems in the tropics of North America. A total of 554 fossil plant specimens were analyzed for plant-insect interactions. Our analysis revealed a relatively low overall herbivory rate of 5.4%. However, examination by botanical groups demonstrated a stark contrast between the insect damage on the available gymnosperms and the angiosperms from El Chango. Although gymnosperms dominated the palaeoflora (89.4% abundance), they hosted a low herbivory incidence of 1.8%. In contrast, despite constituting a smaller proportion of the El Chango flora (10.7%), early angiosperms were significantly more affected by herbivores, with 35.6% of specimens showing some form of damage.
The diversity of DTs was also relatively low in the flora, with 14 identified DTs and seven FFGs. Angiosperms hosted a higher diversity of damage (12 DTs), attributed to various FFGs, such as margin feeding, hole feeding, piercing and sucking, galling, oviposition, and mining. The available gymnosperms in the assemblage, in contrast, hosted a small range of interactions (five DTs belonging to galling and piercing and sucking, and hole feeding), potentially due to their anatomical structure and xeromorphic adaptations, making them less palatable to herbivores.
Comparison with coeval European plant assemblages, such as Puy-Puy (France), reveals similarities in gymnosperm dominance but notable differences in the diversity and frequency of plant-insect interactions. The Puy-Puy flora hosts more DTs and rates of herbivory, but potential biases in plant species diversity and taphonomy offer plausible explanations for the observed disparities. Nevertheless, a pattern emerges across these mid-Cretaceous ecosystems, with insects inflicting greater feeding damage on broad-leafed angiosperms than co-preserved scale/needle-leafed gymnosperms. These findings underscore the need for further research on mid-Cretaceous plant assemblages and plant-insect interactions to unravel the relative roles of the targeting of angiosperms as a clade versus a potential preference of herbivores for feeding on broad-leafed plants in general during the mid-Cretaceous. Our study contributes to the broader understanding of coevolutionary dynamics between plants and insects during a critical phase in Earth’s vegetation history.
The original contributions presented in the study are included in the article/Supplementary Material. Further inquiries can be directed to the corresponding authors.
AS: Conceptualization, Data curation, Formal analysis, Funding acquisition, Investigation, Methodology, Project administration, Resources, Software, Supervision, Validation, Visualization, Writing – original draft, Writing – review & editing. SM: Conceptualization, Data curation, Formal analysis, Funding acquisition, Investigation, Methodology, Project administration, Resources, Software, Supervision, Validation, Visualization, Writing – original draft, Writing – review & editing. MR-K: Conceptualization, Data curation, Formal analysis, Investigation, Methodology, Resources, Writing – review & editing. AH-D: Conceptualization, Data curation, Formal analysis, Investigation, Methodology, Resources, Writing – review & editing. UV-A: Conceptualization, Data curation, Formal analysis, Funding acquisition, Investigation, Methodology, Project administration, Resources, Supervision, Validation, Visualization, Writing – review & editing. SC-F: Conceptualization, Data curation, Formal analysis, Funding acquisition, Investigation, Methodology, Project administration, Resources, Supervision, Validation, Visualization, Writing – review & editing.
The author(s) declare financial support was received for the research, authorship, and/or publication of this article. AS and SM are supported by a grant from the Swedish Research Council (VR grant number 2022-03920). AS was also supported by a postdoctoral fellowship from the UNAM (Mexico, ref.: UNAM-DGAPA 2023-2024). SRSCF recognizes financial support from DGAPA-UNAM project IN105522, Conacyt (Conahcyt) project CF61501, and MR-K thanks Conacyt (Conahcyt) a Graduate school scholarship, 762397.
Authors thank Enoch Ortiz Montejo, Alma Rosa Huerta Huerta Vergara, and all those in the Palaeobotany Group from IGL-UNAM who have collaborated in the excavations from the El Chango site since 2005. AS also thanks Dr. Torsten Wappler (University of Bonn), for his kind help. Also, thanks to Drs. Jesús Alvarado Ortega and Violeta Amparo Romero Mayén from the IGL-Colección Nacional de Paleontología for their support. We are grateful to the editor and anonymous reviewers for their useful suggestions that improved this manuscript. This work had the fieldwork authorization permit number 401.1S.3-2024/137 of the Council of Paleontology of the National Archeology Coordination of the National Institute of Anthropology and History (INAH).
The authors declare that the research was conducted in the absence of any commercial or financial relationships that could be construed as a potential conflict of interest.
All claims expressed in this article are solely those of the authors and do not necessarily represent those of their affiliated organizations, or those of the publisher, the editors and the reviewers. Any product that may be evaluated in this article, or claim that may be made by its manufacturer, is not guaranteed or endorsed by the publisher.
The Supplementary Material for this article can be found online at: https://www.frontiersin.org/articles/10.3389/fevo.2024.1381539/full#supplementary-material
Adroit B., Wappler T., Terral J. F., Ali A. A., Girard V. (2016). Bernasso, a paleoforest from the early Pleistocene: New input from plant–insect interactions (Hérault, France). Palaeogeogr Palaeoclimatol Palaeoecol. 446, 78–84. doi: 10.1016/j.palaeo.2016.01.015
Alvarado-Ortega J., Ovalles-Damián E., Blanco-Piñón A. (2009). The fossil fishes from the Sierra Madre Formation, Ocozocoautla, Chiapas, Southern Mexico. Palaeontol. Electron. 12, 1–22.
Alvarado-Ortega J., Than-Marchese B. A. (2012). A Cenomanian aipichthyoid fish (Teleostei, Acanthomorpha) from America, Zoqueichthys carolinae gen. and sp. nov. from El Chango quarry (Cintalapa Member, Sierra Madre Formation), Chiapas, Mexico. Rev. Mex. Cienc. Geol. 29, 735–748.
Alvarado-Ortega J., Than-Marchese B. A. (2013). The first record of a North American Cenomanian Trachichthyidae fish (Acanthomorpha, Acanthopterygii), Pepemkay maya, gen. et sp. nov., from El Chango quarry (Sierra Madre Formation), Chiapas, Mexico. J. Vertebr. Paleontol. 33, 48–57. doi: 10.1080/02724634.2012.712585
Arroyo-Sánchez D. K., Víctor J., Córdova-Tabares V. M., Hernández-Damián A. L., Estrada-Ruiz E. (2023). Entomopathogenic fungi (Ascomycota: Hypocreales) on a termite (Blattodea: Mastotermitidae) trapped in Miocene amber from Chiapas, Mexico. Hist. Biol., 1–7. doi: 10.1080/08912963.2023.2291769
Banerji J. (2004). Evidence of insect-plant interactions from the Upper Gondwana sequence (Lower Cretaceous) in the Rajmahal Basin, India. Gondwana. Res. 7, 205–210. doi: 10.1016/S1342-937X(05)70320-8
Bar-On Y. M., Phillips R., Milo R. (2018). The biomass distribution on Earth. Proc. Natl. Acad. Sci. U.S.A. 115, 6506–6511.doi.10.1073/pnas.1711842115. doi: 10.1073/pnas.1711842115
Blumenthal D. M., Mueller K. E., Kray J. A., Ocheltree T. W., Augustine D. J., Wilcox K. R. (2020). Traits link drought resistance with herbivore defence and plant economics in semi-arid grasslands: The central roles of phenology and leaf dry matter content. J. Ecol. 108, 2336–2351. doi: 10.1111/1365-2745.13454
Bruce N. L., de Lourdes Serrano-Sánchez M., Carbot-Chanona G., Vega F. J. (2021). New species of fossil Cirolanidae (Isopoda, Cymothoida) from the Lower Cretaceous (Aptian) Sierra Madre Formation plattenkalk dolomites of El Espinal quarries, Chiapas, SE Mexico. J. South. Am. Earth. Sci. 109, 103285. doi: 10.1016/j.jsames.2021.103285
Cantalice K. M., Than-Marchese B. A., Villalobos-Segura E. (2021). A new Cenomanian acanthomorph fish from the El Chango quarry (Chiapas, south-eastern Mexico) and its implications for the early diversification and evolutionary trends of acanthopterygians. Pap. Palaeontol. 7, 1699–1726. doi: 10.1002/spp2.1359
Carvalho M. R., Jaramillo C., de la Parra F., Caballero-Rodríguez D., Herrera F., Wing S., et al. (2021). Extinction at the end-Cretaceous and the origin of modern Neotropical rainforests. Science 372, 63–68. doi: 10.1126/science.abf1969
Cevallos-Ferriz S. R., Hernández-Damián A. L., Beraldi-Campesi H., Ruvalcaba-Knoth M. A., Huerta-Vergara A. R. (2022). Paleobotany to understand evolution and biodiversity in Mexico. Bot. Sci. 100, 34–65. doi: 10.17129/botsci.3122
Coiffard C., Gomez B., Daviero-Gomez V., Dilcher D. L. (2012). Rise to dominance of angiosperm pioneers in European Cretaceous environments. Proc. Natl. Acad. Sci. 109, 20955–20959. doi: 10.1073/pnas.1218633110
Condamine F. L., Silvestro D., Koppelhus E. B., Antonelli A. (2020). The rise of angiosperms pushed conifers to decline during global cooling. Proc. Natl. Acad. Sci. 117, 28867–28875. doi: 10.1073/pnas.2005571117
Cooper S. M., Owen-Smith N. (1986). Effects of plant spinescence on large mammalian herbivores. Oecologia 68, 446–455. doi: 10.1007/BF01036753
Currano E. D., Labandeira C. C., Wilf P. (2010). Fossil insect folivory tracks paleotemperature for six million years. Ecol. Monogr. 80, 547–567. doi: 10.1890/09-2138.1
Currano E. D., Wilf P., Wing S. L., Labandeira C. C., Lovelock E. C., Royer D. L. (2008). Sharply increased insect herbivory during the Paleocene–Eocene Thermal Maximum. Proc. Natl. Acad. Sci. U.S.A. 105, 1960–1964. doi: 10.1073/pnas.0708646105
Díaz-Cruz J. A., Alvarado-Ortega J., Carbot-Chanona G. (2019). Dagon avendanoi gen. and sp. nov., an Early Cenomanian Enchodontidae (Aulopiformes) fish from the El Chango quarry, Chiapas, southeastern Mexico. J. South. Am. Earth. Sci. 91, 272–284. doi: 10.1016/j.jsames.2019.01.014
Díaz-Cruz J. A., Alvarado-Ortega J., Giles S. (2020). A long snout enchodontid fish (Aulopiformes: Enchodontidae) from the Early Cretaceous deposits at the El Chango quarry, Chiapas, southeastern Mexico: A multi-approach study. Palaeont. Electr. 23, 1–27. doi: 10.26879/1065
Ding Q., Labandeira C. C., Meng Q., Ren D. (2015). Insect herbivory, plant-host specialization and tissue partitioning on mid-Mesozoic broadleaved conifers of Northeastern China. Palaeogeogr. Palaeoclimatol. Palaeoecol. 440, 259–273. doi: 10.1016/j
Donovan M. P., Iglesias A., Wilf P., Labandeira C. C., Cuneo N. R. (2016). Rapid recovery of Patagonian plant–insect associations after the end-Cretaceous extinction. Nat. Ecol. Evol. 1, 1–12. doi: 10.1038/s41559–016-0012
Donovan M. P., Iglesias A., Wilf P., Labandeira C. C., Cuneo N. R. (2018). Diverse plant-insect associations from the latest Cretaceous and early Paleocene of Patagonia, Argentina. Ameghiniana. 55, 303–338. doi: 10.5710/AMGH.15.02.2018.3181
Donovan M. P., Wilf P., Iglesias A., Cúneo N. R., Labandeira C. C. (2020). Persistent biotic interactions of a Gondwanan conifer from Cretaceous Patagonia to modern Malesia. Commun. Biol. 3, 708. doi: 10.1038/s42003-020-01428-9
Donovan M. P., Wilf P., Labandeira C. C., Johnson K. R., Peppe D. J. (2014). Novel insect leaf-mining after the end-Cretaceous extinction and the demise of Cretaceous leaf miners, Great Plains, USA. PloS One 9, e103542. doi: 10.1371/journal.pone.0103542
Estévez-Gallardo P., Sender L. M., Mayoral E., Diez J. B. (2018). First evidence of insect herbivory on Albian aquatic angiosperms of the NE Iberian Peninsula. Earth. Environ. Sci. Trans. R. Soc Edinb. 108, 429–435. doi: 10.1017/S1755691018000555
Estrada-Ruiz E., Centeno-González N. K., Aguilar-Arellano F., Martínez-Cabrera H. I. (2018). New record of the aquatic fern Marsilea, from the Olmos Formation (upper Campanian), Coahuila, Mexico. Int. J. Plant Sci. 179, 487–496. doi: 10.1086/697729
Flores-Barragán M. A., Velasco de León M. P., Labandeira C. (2023). Plant-Insect Interactions in Central-eastern Mexico during the upper Paleozoic. (Amsterdam: Elsevier). doi: 10.2139/ssrn.4677024
Friis E. M., Crane P. R., Pedersen K. R. (2011). The Early Flowers and Angiosperm Evolution (Cambridge: Cambridge University Press), 600. doi: 10.1017/CBO9780511980206
Genise J. F. (1995). Upper Cretaceous trace fossils in permineralized plant remains from Patagonia, Argentina. Ichnos 3, 287–299. doi: 10.1080/10420949509386399
Genise J. F., Sánchez M. V., Poiré D., González M. G. (2020). A fossorial petalurid trace fossil from the Albian of Patagonia. Cretac. Res. 116, 104591. doi: 10.1016/j.cretres.2020.104591
González-Ramírez I., Calvillo-Canadell L., Cevalloz-Ferríz S. R. S. (2013). Coníferas cupresáceas fósiles de “El Chango”, Chiapas (Aptiano). Paleontol. Mex. 63, 24–31.
Guerrero-Márquez G., Calvillo-Canadell L., Cevallos-Ferriz S. R., Avendaño-Gil J. (2013). Angiospermas cretácicas de la localidad “El Chango”, Chiapas, México. Paleontol. Mex. 63, 32–39.
Gunkel S., Wappler T. (2015). Plant-insect interactions in the upper Oligocene of Enspel (Westerwald, Germany), including an extended mathematical framework for rarefaction. Paleobiodivers. Paleoenviron. 95, 55–75. doi: 10.1007/s12549-014-0176-6
Hagström J., Mehlqvist K. (2012). The dawn of terrestrial ecosystems on Baltica: First report on land plant remains and arthropod coprolites from the Upper Silurian of Gotland, Sweden. Palaeogeogr. Palaeoclimatol. Palaeoecol. 317, 162–170. doi: 10.1016/j.palaeo.2012.01.001
Hanley M. E., Lamont B. B., Fairbanks M. M., Rafferty C. M. (2007). Plant structural traits and their role in anti-herbivore defence. Perspect. Plant Ecol. Evol. Syst. 8, 157–178. doi: 10.1016/j.ppees.2007.01.001
Heck K. L. Jr., van Belle G., Simberloff D. (1975). Explicit calculation of the rarefaction diversity measurement and the determination of sufficient sample size. Ecology 56, 1459–1461. doi: 10.1016/j.ppees.2007.01.001
Herman A. B., Domogatskaya K. V. (2023). Ochotopteris—An endemic fern of the mid-cretaceous arctic. Geosciences 13, 279. doi: 10.3390/geosciences13090279
Klymiuk A. A., Stockey R. A., Rothwell G. W. (2015). Plant-arthropod interactions in Acanthostrobus edenensis (Cupressaceae), a new conifer from the Upper Cretaceous of Vancouver Island, British Columbia. Int. J. Plant Sci. 176, 378–392. doi: 10.1086/680684
Krassilov V. A. (2007). Mines and galls on fossil leaves from the Late Cretaceous of southern Negev, Israel. Afr. Invertebr. 48, 13–22.
Krassilov V. A. (2008a). Mine and gall predation as top down regulation in the plant–insect systems from the Cretaceous of Negev, Israel. Palaeogeogr. Palaeoclimatol. Palaeoecol. 261, 261–269. doi: 10.1016/j.palaeo.2008.01.017
Krassilov V. A. (2008b). Evidence of temporary mining in the Cretaceous fossil mine assemblage of Negev, Israel. Insect Science. 15, 285–290. doi: 10.1111/j.1744-7917.2008.00212.x
Krassilov V. A., Rasnitsyn A. P. (2008). “Plant-arthropod interactions in the early angiosperm history,” in Evidence from the Cretaceous of Israel (Pensoft: Sofia and Moscow, BRILL Leiden and Boston). doi: 10.1163/ej.9789004170711.1-229
Krassilov V. A., Shuklina S. (2008). Arthropod trace diversity on fossil leaves from the mid-Cretaceous of Negev, Israel. Alavesia 2, 239–245.
Krassilov V. A., Silantieva N., Hellmund M., Hellmund W. (2007). Insect egg sets on angiosperm leaves from the Lower Cretaceous of Negev, Israel. Cretac. Res. 28, 803–811. doi: 10.1016/j.cretres.2006.11.004
Krassilov V., Silantieva N., Lewy Z. (2008). “Traumas on fossil leaves from the Cretaceous of Israel,” in Plant–Arthropod Interactions in the Early Angiosperm History: Evidence from the Cretaceous of Israel. Eds. Krassilov V., Rasnitsyn A. (Sofia–Moscow, Pensoft (and Leiden–Boston), 7–187.
Kuglerová M., Skuhrovec J., Münzbergová Z. (2019). Relative importance of drought, soil quality, and plant species in determining the strength of plant–herbivore interactions. Ecol. Entomol. 44, 665–677. doi: 10.1111/een.12745
Labandeira C. C., Wappler T. (2023). Arthropod and pathogen damage on fossil and modern plants: exploring the origins and evolution of herbivory on land. Annu. Rev. Entomol. 68, 341–361. doi: 10.1146/annurev-ento-120120-102849
Labandeira C. C., Wilf P., Johnson K. R., Marsh F. (2007). Guide to Insect (and other) Damage Types on Compressed Plant Fossils (Washington, D.C: Smithsonian Institution). Version 3.0.
Laws A. N., Belovsky G. E. (2010). How will species respond to climate change? Examining the effects of temperature and population density on an herbivorous insect. Environ. Entomol. 39, 312–319. doi: 10.1603/EN09294
Maccracken S. A., Miller I. M., Labandeira C. C. (2019). Late Cretaceous domatia reveal the antiquity of plant-mite mutualisms in flowering plants. Biol. Lett. 15, 20190657. doi: 10.1098/rsbl.2019.0657
Maccracken S. A., Sohn J.-C. C., Miller I. M., Labandeira C. C. (2021). A new Late Cretaceous leaf mine Leucopteropsa spiralae gen. et sp. nov. (Lepidoptera: lyonetiidae) represents the first confirmed fossil evidence of the Cemiostominae. J. Syst. Palaeontol. 19, 131–144. doi: 10.1080/14772019.2021.1881177
Martínez de Espronceda P., Rodríguez-Barreiro I., Pérez-Pueyo M., Bádenas B., Canudo I., Puértolas-Pascual E., et al. (2023). Palynostratigraphical review of the K–Pg boundary from the Ibero-Armorican Island: New data from the Maastrichtian dinosaur outcrop Veracruz 1 (Pyrenees, NE Iberian Peninsula). Cretac. Res. 154, 105757. doi: 10.1016/j.cretres.2023.105757
McLoughlin S. (2001). The breakup history of Gondwana and its impact on pre-Cenozoic floristic provincialism. Aust. J. Bot. 49, 271–300. doi: 10.1071/BT00023
McLoughlin S., Drinnan A. N., Rozefelds A. C. (1995). The Cenomanian flora of the winton formation, eromanga basin, queensland, Australia. Mem. Qd Mus. 38, 273–313.
McLoughlin S., Martin S. K., Beattie R. (2015). The record of Australian Jurassic plant-arthropod interactions. Gondwana Res. 27, 940–959. doi: 10.1016/j.gr.2013.11.009
McLoughlin S., Pott C., Elliott D. (2010). The Winton Formation flora (Albian–Cenomanian, Eromanga Basin): implications for vascular plant diversification and decline in the Australian Cretaceous. Alcheringa 34, 303–323. doi: 10.1080/03115511003669944
Mikuláš R., Milàn J., Genise J. F., Bertling M., Bromley R. G. (2020). An insect boring in an Early Cretaceous wood from Bornholm, Denmark. Ichnos 27, 284–289. doi: 10.1080/10420940.2020.1744587
Moreau J. D., Trincal V., Deconinck J. F., Philippe M., Bourel B. (2021). Lowermost Jurassic dinosaur ecosystem from the Bleymard Strait (southern France): sedimentology, mineralogy, palaeobotany and palaeoichnology of the Dolomitic Formation. Geol. Magaz. 158, 1830–1846. doi: 10.1017/S001675682100039X
Moreau J. D., Vullo R., Charbonnier S., Jattiot R., Trincal V., Néraudeau D., et al. (2022). Konservat-Lagerstätten from the Upper Jurassic lithographic limestone of the Causse Méjean (Lozère, southern France): palaeontological and palaeoenvironmental synthesis. Geol. Mag. 159, 761–781. doi: 10.1017/S0016756821001382
Moreno-Bedmar J. A., Latil J. L., Villanueva-Amadoz U., Calvillo-Canadell L., Calvillo-Canadell S. (2014). Ammonite age-calibration of the EL Chango Fossil-Lagerstätte, Chiapas state (SE Mexico). R. S. J. South. Am. Earth. Sci. 56, 447–453. doi: 10.1016/j.jsames.2014.09.022
Münzbergová Z., Skuhrovec J. (2013). Effect of habitat conditions and plant traits on leaf damage in the Carduoideae subfamily. PloS One 8, e64639. doi: 10.1371/journal.pone.0064639
Peris D., Condamine F. L. (2024). The angiosperm radiation played a dual role in the diversification of insects and insect pollinators. Nat. Commun. 15, 552. doi: 10.1038/s41467-024-44784-4
Petrov A. V. (2013). New ichnotaxon Megascolytinus zherikhini (Coleoptera: Curculionidae: Scolytinae) from Upper Cretaceous deposits of Mongolia. Paleont. J. 47, 597–600. doi: 10.1134/S0031030113060051
Philippe M., McLoughlin S., Strullu-Derrien C., Bamford M., Kiel S., Nel A., et al. (2022). Life in the woods: taphonomic evolution of a diverse saproxylic community within fossil woods from Upper Cretaceous submarine mass flow deposits (Mzamba Formation, southeast Africa). Gondw. Res. 109, 113–133. doi: 10.1016/j.gr.2022.04.008
Pires E. F., Sommer M. G. (2009). Plant–arthropod interaction in the Early Cretaceous (Berriasian) of the Araripe Basin, Brazil. J. South Am. Earth Sci. 27, 50–59. doi: 10.1016/j.jsames.2008.09.004
Rodríguez-Barreiro I., Santos A. A., Arribas M. E., Mas R., Arribas J., Villanueva-Amadoz U., et al. (2022). Jurassic–Cretaceous transition in the West Cameros Basin (Tera Group, Burgos, Spain): Sedimentological and palynostratigraphical insights. Cretac. Res. 139, 105300. doi: 10.1016/j.cretres.2022.105300
Rodríguez-Barreiro I., Santos A. A., Villanueva-Amadoz U., Gasulla J. M., Escaso F., Ortega F., et al. (2024). Palynological reconstruction of the habitat and diet of Iguanodon bernissartensis in the Lower Cretaceous Morella Formation, NE Iberian Peninsula. Cretac. Res. 156, 105804. doi: 10.1016/j.cretres.2023.105804
Santos A. A., Hernández-Orúe A., Wappler T., Diez J. B. (2022b). Plant–insect interactions from the Late Pennsylvanian of the Iberian Peninsula (León, northern Spain). Rev. Palaeobot. Palynol 301, 104658. doi: 10.1016/j.revpalbo.2022.104658
Santos A. A., Nel A., Rodríguez-Barreiro I., Sender L. M., Wappler T., Diez J. B. (2022c). Insect and plant diversity in hot-Spring ecosystems during the Jurassic-Cretaceous boundary from Spain (Aguilar fm., palencia). Biology 11, 273. doi: 10.3390/biology11020273
Santos A. A., Sender L. M., Wappler T., Diez J. B. (2023). Plant–insect interactions on aquatic and terrestrial angiosperms from the latest Albian (Early Cretaceous) of Estercuel (northeastern Spain) and their paleoenvironmental implications. Plants 12, 508. doi: 10.3390/plants12030508
Santos A. A., Sender L. M., Wappler T., Engel M. S., Diez J. B. (2021). A Robinson Crusoe story in the fossil record: Plant-insect interactions from a Middle Jurassic ephemeral volcanic island (Eastern Spain). Palaeogeogr. Palaeoclimatol. Palaeoecol. 583, 110655. doi: 10.1016/j.palaeo.2021.110655
Santos A. A., Villanueva-Amadoz U., Royo-Torres R., Sender L. M., Cobos A., Alcalá L., et al. (2018). Palaeobotanical records associated with the first dinosaur defined in Spain: Palynostratigraphy, taxonomy and palaeoenvironmental remarks. Cretac. Res. 90, 318–334. doi: 10.1016/j.cretres.2018.04.023
Santos A. A., Xiao L., Labandeira C., Néraudeau D., Dépré P., Moreau D., et al. (2022a). Plant–insect interactions from the mid-Cretaceous at Puy-Puy (Aquitaine Basin, western France) indicate preferential herbivory for angiosperms amid a forest of ferns, gymnosperms, and angiosperms. Bot. Lett. 169, 568–587. doi: 10.1080/23818107.2022.2092772
Santos-Filho E. B. D., Adami-Rodrigues K., Lima F. J. D., Bantim R. A. M., Wappler T., Saraiva A.ÁF. (2019). Evidence of plant–insect interaction in the Early Cretaceous flora from the Crato Formation, Araripe Basin, northeast Brazil. Hist. Biol. 31, 926–937. doi: 10.1080/08912963.2017.1408611
Santos-Filho E. B., Brum A. S., Souza G. A., Figueiredo R. G., Usma C. D., Ricetti J. H. Z., et al (2023). First record of insect-plant interaction in Late Cretaceous fossils from Nelson Island (South Shetland Islands Archipelago), Antarctica. Anais Acad. Brasil. Ci. 95, e20231268.
Santos-Neto P. E., Arnan X., Ribeiro-Neto J. D., Wirth R., Leal I. R. (2022). Aridity, but not disturbance, reduces the specialization and modularity of plant–insect herbivore interaction networks in Caatinga dry forest. J. Insect Conserv. 26, 175–189. doi: 10.1007/s10841-022-00376-5
Scott A. C., Stephenson J., Chaloner W. G. (1992). Interaction and coevolution of plants and arthropods during the Palaeozoic and Mesozoic. Philos. Trans. R. Soc B: Biol. 335, 129–165. doi: 10.1098/rstb.1992.0016
Sender L. M., Wappler T., García-Ávila M., Santos A. A., Diez J. B. (2022). First plant-insect interactions on Cretaceous Caytoniales (genus Sagenopteris) from the Lower Cretaceous (Albian) of Spain. Cretac. Res. 138, 105295. doi: 10.1016/j.cretres.2022.105295
Than-Marchese B. A., Alvarado-Ortega J., Matamoros W. A., Velázquez-Velázquez E. (2020). Scombroclupea javieri sp. nov., an enigmatic Cenomanian clupeomorph fish (Teleostei, Clupeomorpha) from the marine deposits of the Cintalapa Formation, Ocozocoautla, Chiapas, southeastern Mexico. Cretac. Res. 112, 104448. doi: 10.1016/j.cretres.2020.104448
Vajda V., Lyson T. R., Bercovici A., Doman J. H., Pearson D. A. (2013). A snapshot into the terrestrial ecosystem of an exceptionally well-preserved dinosaur (Hadrosauridae) from the Upper Cretaceous of North Dakota, USA. Cretac. Res. 46, 114–122. doi: 10.1016/j.cretres.2013.08.010
Vega F. J., Alvarado-Ortega J., Luque J., Porras-Múzquiz H., Carbot-Chanona G., González-Rodríguez K. A., et al. (2022). Cretaceous Crustacea from plattenkalk deposits of Mexico. J. South. Am. Earth. Sci. 116, 103839. doi: 10.1016/j.jsames.2022.103839
Vega F. J., García-Barrera P., Perrilliat M. D. C., Coutiño M. A., Mariño-Pérez R. (2006). El Espinal, a new plattenkalk facies locality from the Lower Cretaceous Sierra Madre Formation, Chiapas, southeastern Mexico. Rev. Mex. Cienc. Geol. 23, 323–333.
Velasco de León M. P., Flores-Barragán M. A., Cadena-Gonzáles D. (2015). Interacción planta-insecto sobre hojas de Bennettitales en la localidad Cerro el Matador del Jurásico Medio, Formación Otlaltepec (Puebla). Paleontol. Mex. 4, 1–5.
Villanueva-Amadoz U., Calvillo-Canadell L., Cevallos-Ferriz S. R. S. (2014). Síntesis de los trabajos paleobotánicos del Cretácico en México. Boletin la Soc Geol. Mex. 66, 97–121.
Villanueva-Amadoz U., Pons D., Diez J. B., Ferrer J., Sender L. M. (2010). Angiosperm pollen grains of San Just site (Escucha Formation) from the Albian of the Iberian Range (north-eastern Spain). Rev. Palaeobot. Palynol. 162, 362–381. doi: 10.1016/j.revpalbo.2010.02.014
Xiao L., Labandeira C. C., Dilcher D. L., Ren D. (2022a). Arthropod and fungal herbivory at the dawn of angiosperm diversification: The Rose Creek plant assemblage of Nebraska, USA. Cretac. Res. 131, 105088. doi: 10.1016/j.cretres.2021.105088
Xiao L. F., Labandeira C. C., Ren D. (2022b). Insect herbivory immediately before the eclipse of the gymnosperms. Ins. Sci. 29, 1483–1520. doi: 10.1017/pab.2021.20
Keywords: angiosperms, gymnosperms, mid-Cretaceous, plant-arthropod interactions, palaeoecology, fruits, herbivory
Citation: Santos AA, McLoughlin S, Rubalcava-Knoth MA, Hernández-Damián AL, Villanueva-Amadoz U and Cevallos-Ferriz SR S (2024) Plant-insect interactions in the mid-Cretaceous paleotropical El Chango Lagerstätte (Cintalapa Fm., Mexico)—patterns of herbivory during the Angiosperm Terrestrial Revolution. Front. Ecol. Evol. 12:1381539. doi: 10.3389/fevo.2024.1381539
Received: 03 February 2024; Accepted: 17 June 2024;
Published: 10 July 2024.
Edited by:
Sandra Schachat, Stanford University, United StatesReviewed by:
Romulo Cenci, University of the Rio dos Sinos Valley, BrazilCopyright © 2024 Santos, McLoughlin, Rubalcava-Knoth, Hernández-Damián, Villanueva-Amadoz and Cevallos-Ferriz. This is an open-access article distributed under the terms of the Creative Commons Attribution License (CC BY). The use, distribution or reproduction in other forums is permitted, provided the original author(s) and the copyright owner(s) are credited and that the original publication in this journal is cited, in accordance with accepted academic practice. No use, distribution or reproduction is permitted which does not comply with these terms.
*Correspondence: Artai A. Santos, YXJ0YWlzYW50b3NAZ21haWwuY29t; Uxue Villanueva-Amadoz, dXh1ZUBnZW9sb2dpYS51bmFtLm14
Disclaimer: All claims expressed in this article are solely those of the authors and do not necessarily represent those of their affiliated organizations, or those of the publisher, the editors and the reviewers. Any product that may be evaluated in this article or claim that may be made by its manufacturer is not guaranteed or endorsed by the publisher.
Research integrity at Frontiers
Learn more about the work of our research integrity team to safeguard the quality of each article we publish.