- 1Department of Geography and Planning, School of Environmental Sciences, University of Liverpool, Liverpool, United Kingdom
- 2GEOTOP-UQAM, Montréal, QC, Canada
Over the last four decades, organic-walled dinoflagellate cysts have shown high potential as tracers of past sea-surface conditions during the Quaternary. These microfossils relate to the pelagic productivity of both phototrophic and heterotrophic protist organisms and are recovered in high numbers in almost all marine environment settings from the nearshore and estuarine systems to the distal continental margin. In polar environments, where other conventional proxies are rare or absent, dinoflagellate cysts showed a relatively high diversity of species and a close relationship with sea-ice cover duration, winter and summer temperature, and salinity, enabling quantitative reconstructions of several oceanic variables over time. From the temperate to the tropical latitudes of the Atlantic Ocean, their modern distribution highlights a response to primary productivity and seasonal contrasts in surface temperature. They also have proven that they could be used as tracers of eutrophication in stratified systems and can also highlight human impact on their distribution. In this paper, we present an overview of dinoflagellate cysts as ecological tracers in recent and past sediments of the Atlantic Ocean, from the Arctic to the Antarctic. We provide examples of their use as proxies in paleoclimatic-palaeoceanographic studies at glacial to interglacial time scales, with emphasis on the last ice age to recent (last 25 kyr), the northern North Atlantic and western-eastern tropic North Atlantic. We also discuss their potential as tracers of anthropogenic stress in coastal environments.
1 Introduction
The phylum Dinoflagellata encompasses a large diversity of unicellular eukaryotic organisms, including phototrophic taxa, hence playing a major role in the biological pump (Falkowski, 2012). Dinoflagellates are currently found in all aquatic systems, mostly in marine waters, where at least 2377 species are known (Gómez, 2012a). They occur from high latitudes to the tropics and can be found even in sea ice (e.g., Mudie, 1992; Montresor et al., 2003). Some of them are known to create Harmful Algal Blooms (HABs) (i.e., Alexandrium, Dinophysis, Karenia species, etc.).
Dinoflagellates have a complex feeding strategy. Among them, several species are phototrophic and account for an important part of the primary productivity of the ocean together with haptophytes and diatoms (e.g., Sigman and Hain, 2012). However, about 58% of extant taxa are heterotrophic, and several are described as mixotrophs, symbionts or parasites (Gómez, 2012b). One of the striking features of this group is that about 20% of the species form cysts that protect hypnozygote during their life cycle and the cyst is made of highly resistant organic matter enabling preservation in the sediments (e.g., Head, 1996) (Figure 1). These cysts are recovered in palynological slides after chemical treatments involving hydrochloric and hydrofluoric acids, and they are commonly analysed by palynologists as other organic-resistant microfossils such as pollen and spores (Traverse, 1974).
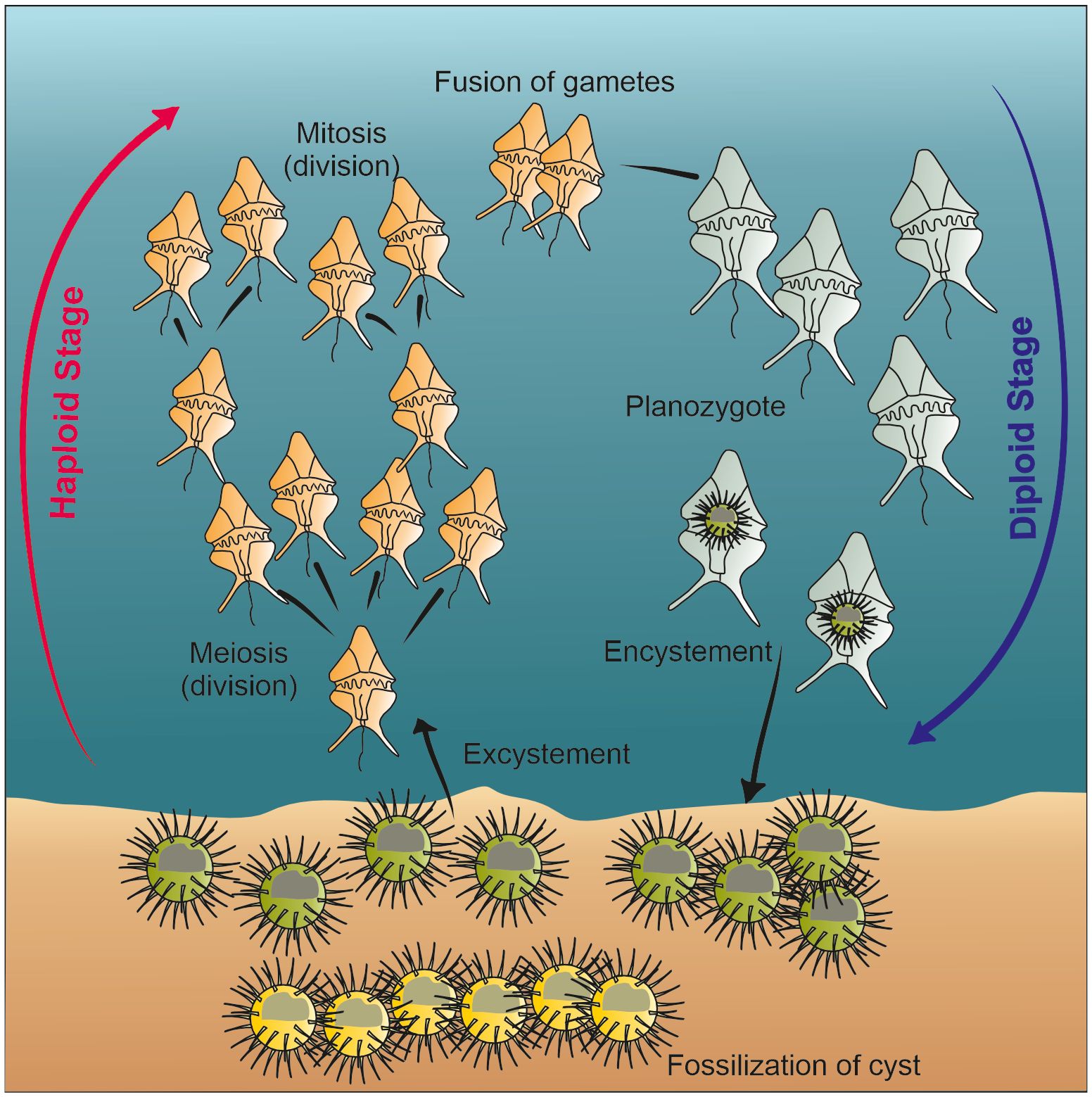
Figure 1 Simplified schematic of dinoflagellate life cycle, modified after de Vernal et al. (2013c) and Bravo and Figueroa (2014).
Close to a hundred organic-walled dinoflagellate cyst (also called dinocyst) taxa have been described from recent sediments (Van Nieuwenhove et al., 2020a; Limoges et al., 2020; Mertens et al., 2020). In addition, 35 known extant marine taxa make a calcareous cyst that can also be found in marine sediments (Zonneveld et al., 2005).
Dinoflagellate cysts have been observed in sedimentary rocks dating since the Triassic and used with success as biostratigraphic markers in Mesozoic and Cenozoic sequences (e.g., Fensome et al., 1993). In the 1970s, their significance as a palaeoceanographical indicator emerged with the pioneering studies of Williams (1971), Davey and Rogers (1975), Wall et al. (1977) and Williams and Bujak (1977) dealing with deep-sea sediment of the Atlantic Ocean. Earlier on, the ground-breaking studies of Braarud (1945) and later on of Nordii (1951), Wall (1965) and Wall and Dale (1966) elucidated the relationship between the motile form found in surface waters and the cyst, settled in the sediments, helping to understand the ecological affinities of these organisms. Since then, numerous studies focused both on culture and rDNA sequencing have further increased our knowledge of this relationship (e.g., Wang et al., 2019; Gu et al., 2021, 2022), clarifying taxonomical conundrums.
In the last thirty years, the use of dinoflagellate cyst assemblages in palaeoecology and palaeoclimatology increased considerably, notably with the development of approaches allowing quantitative estimates of past climate and ocean parameters. Here, we present the state of the knowledge concerning dinoflagellate cysts and their ecology, we provide some insight into the use of assemblages to document palaeoceanographical changes in the Atlantic Ocean during cold and warm episodes of the late Quaternary, and we briefly outline some environmental issues involving dinoflagellates and their cysts. Furthermore, this review integrates up-to-date considerations on the distribution of dinocysts both in time (biostratigraphy and ecostratigraphy) and space, from the high latitudes of the Northern Hemisphere to the Southern Ocean, following a major update of the taxonomy (Van Nieuwenhove et al., 2020b).
2 General characteristics of dinoflagellate cysts as a palaeoecological indicator from sediment studies
The life cycle of dinoflagellate is complex, with a hiatus between the thecal stage of living populations and the cyst stage, related to sexual reproduction, that fossilises in the sediment. Whereas thousands of living species are known, less than a hundred recent dinoflagellate cyst taxa were described. Hence, the cyst record only provides a fragmentary picture of the original populations. Furthermore, the morphology of the cyst is distinct from that of the theca, which makes cyst identification one of the most challenging aspects of the analysis. This is particularly critical for some taxa that exhibit a large range of morphological variability. The Spiniferites species are a typical example with shapes showing large variations (e.g., de Vernal et al., 2018; Mudie et al., 2018; Gu et al., 2021). The cyst morphology is the basis for the identification. The shape and the tabulation of the cyst body, the shape and position of the archeopyle, the ornamentation, processes density, shape and position, the wall structure, and sometimes the colour of the wall are all typical characteristics used to identify species. Several comprehensive atlases have been produced over the last decades, illustrating most of the modern dinoflagellate cyst species (e.g., Rochon et al., 1999; Zonneveld et al., 2013; Zonneveld and Pospelova, 2015; Mudie et al., 2017; Limoges et al., 2018, 2020; Mertens et al., 2020; Van Nieuwenhove et al., 2020a).
Another layer of complexity in the study of fossil dinoflagellate cysts is related to the dual terminology used to identify dinoflagellates and their cysts that are observed in distinct types of samples (it follows the International Code for the Nomenclature of Algae, Fungi and Plants (the Shenzhen Code, Turland et al., 2018). On the one hand, we have biological names for the thecate stage, and on the other hand, we have micropalaeontological names for the cyst stage yielding fossils (see Head, 1996; Ellegaard et al., 2018; Head et al., 2024). Although it has not been officially agreed, in general, studies dealing with cysts use the micropalaeontological name, unless the cyst was originally described from specimens of known biological affinity (see Table 1).
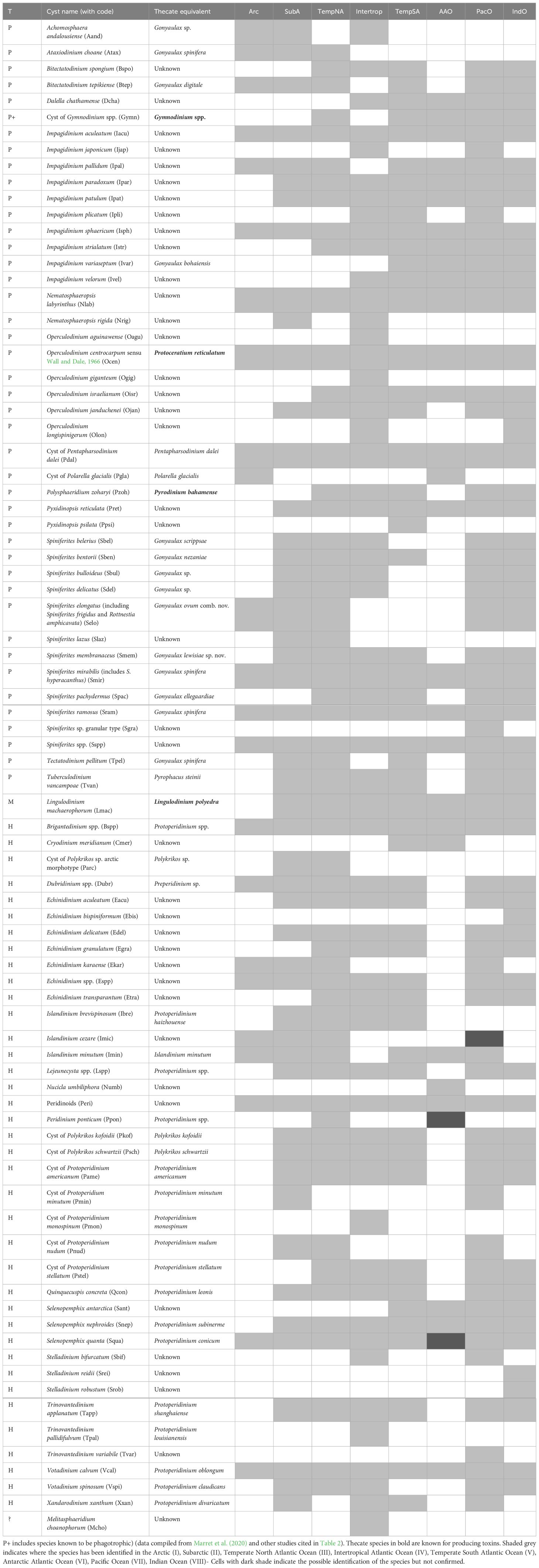
Table 1 List of organic-walled dinoflagellate cyst species found worldwide, with motile stage and trophic status (T is for Trophic habit, with P including phototrophs and autotrophs, H for heterotrophs and M for mixotrophs.
The wall of organic-walled dinoflagellate cysts is made of a strong molecules, often called dinosporin although recent studies have shown complex compositions depending upon the taxa. Studies based on micro-Fourier-transformed infrared spectroscopy have highlighted distinct compositions whether the taxon is phototrophic (cellulose-like glucan) or heterotrophic (nitrogen-rich glucan) (e.g., Bogus et al., 2014; Gurdebeke et al., 2018, 2020; Meyvisch et al., 2022, 2023). Some studies on the preservation aspect of organic matter in oxic and anoxic environments have tentatively established a classification of taxa according to a sensitivity gradient, with most phototrophic species being relatively resistant whereas heterotrophic species would be more prone to early diagenetic processes (e.g., Zonneveld et al., 2008, 2019; Versteegh and Zonneveld, 2022).
The complexity of the life cycle of dinoflagellates and the fact that the production of cysts is still not fully understood, as most of the observations are based on controlled conditions in laboratories (e.g., Bravo and Figueroa, 2014), has long been a limitation in palaeoecology. Consequently, the establishment of dinoflagellate cysts as a palaeoecological indicator slowly evolved, and from studies on the distribution of these microfossils in recent sediments (e.g., Wall et al., 1977; Williams and Bujak, 1977; Harland, 1983; Turon, 1984; Rochon et al., 1999). Mapping their occurrence in the oceans and relating their relative abundance with surface conditions was the stepping-stone to enable this microfossil group to become a valuable tracer of sea-surface conditions (e.g., Rochon et al., 1999; de Vernal et al., 2001; Marret and Zonneveld, 2003; Zonneveld et al., 2013; de Vernal et al., 2020).
Overall, the increasing number of studies investigating the distribution of dinoflagellate cyst species in recent sediments (Figure 2) has greatly contributed to refining our knowledge of their ecological affinity, sometimes shifting paradigms for some species. In parallel, progress in culture techniques and genetic studies has enabled to clarification of the idea that some thecate species may “create” different cyst species likely related to environmental conditions (the Spinifera complex, e.g., Ellegaard et al., 2018). Although there are taxonomical uncertainties and despite the hiatus between living and fossil populations, dinoflagellate cysts are now considered very useful palaeoceanographical tracers in marine environments of continental margins.
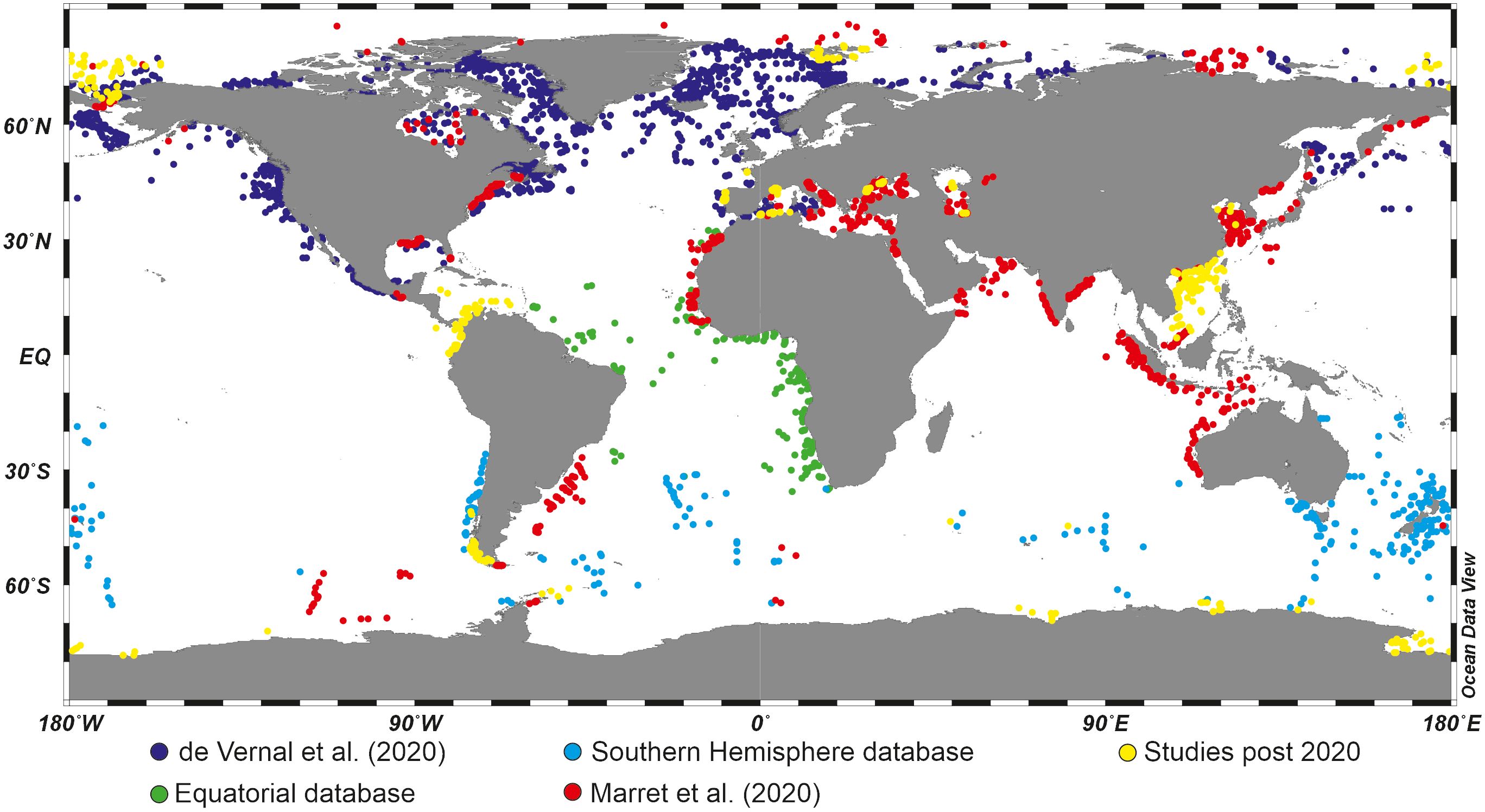
Figure 2 Global distribution of organic-walled dinoflagellate cyst assemblages in surface sediments, based on a compilation from Marret et al. (2020) with additional studies published post 2020 (see Table 2 for references).
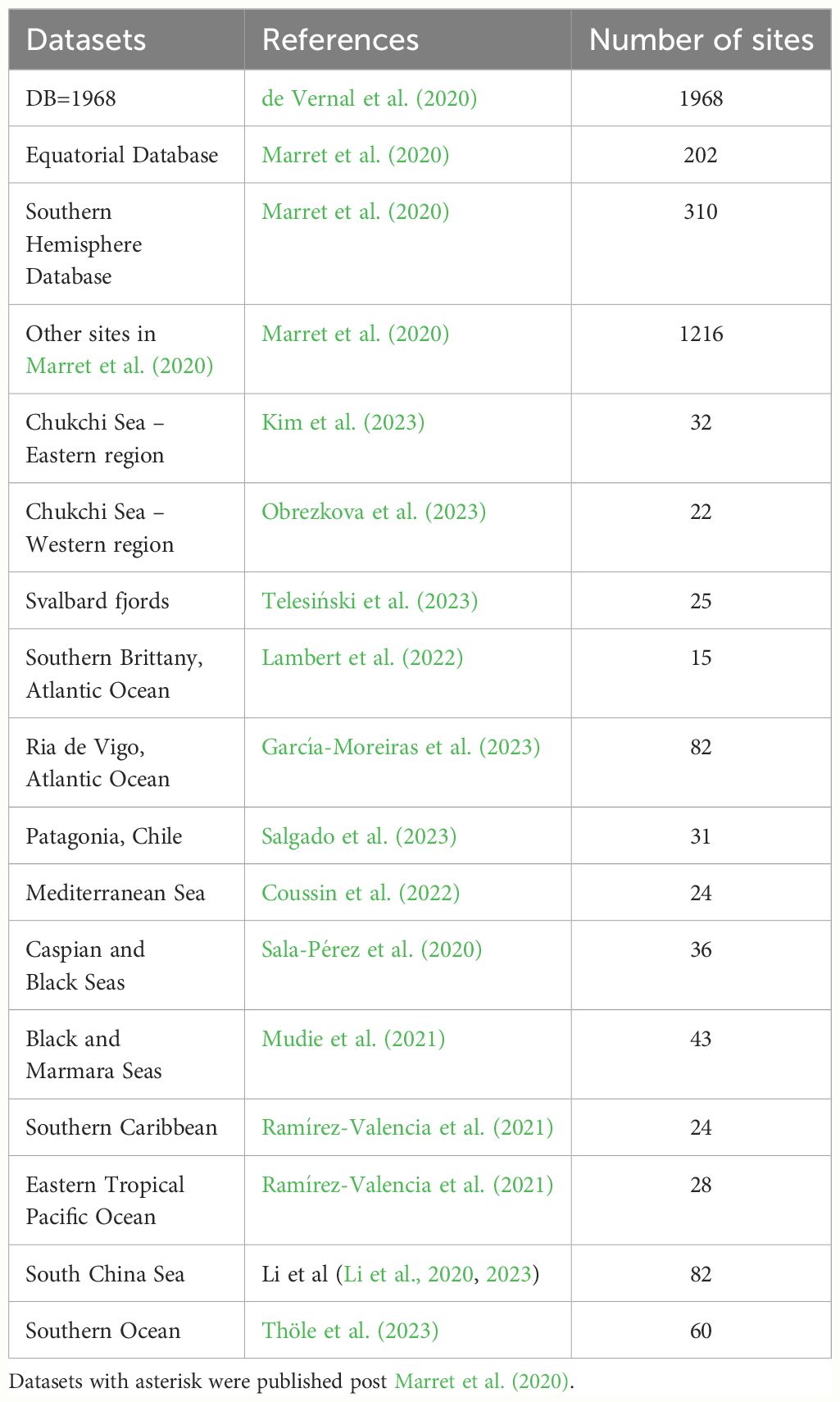
Table 2 Location of datasets used in Figure 2 here with references.
3 Modern distribution of dinoflagellate cysts
Until the 1970s, most studies dealing with fossil dinoflagellate cysts, then designated as hystrichospheres, were undertaken by sedimentary geologists to document the Mesozoic-Cenozoic biostratigraphy. The demonstration of the relationships between dinoflagellates and their cysts based on detailed morphological observations of fossils (Evitt, 1963), observation of hystrichospheres in the plankton (Wall, 1965) and culture experiments (Wall and Dale, 1966, 1968) were an important step toward the use of dinoflagellate cysts in palaeoecology. Based on dinoflagellate cyst taxa counts in 35 surface sediment samples from the North Atlantic Ocean, Williams (1971) demonstrated a distribution related to environmental gradients, from nearshore to offshore settings and with latitudes. Later, Wall et al. (1977) made a comprehensive analysis of the distribution of dinoflagellate cysts in sediments collected at 168 sites from tropical to temperate latitudes of the Atlantic Ocean showing further distinct associations related to the latitudinal gradients, in addition to assemblages having affinities for either estuarine, neritic or oceanic environments. Hence, they proposed an ecological classification of taxa in categories and associations, which could be used as a conceptual model for the understanding of taxa distribution (Figure 3).
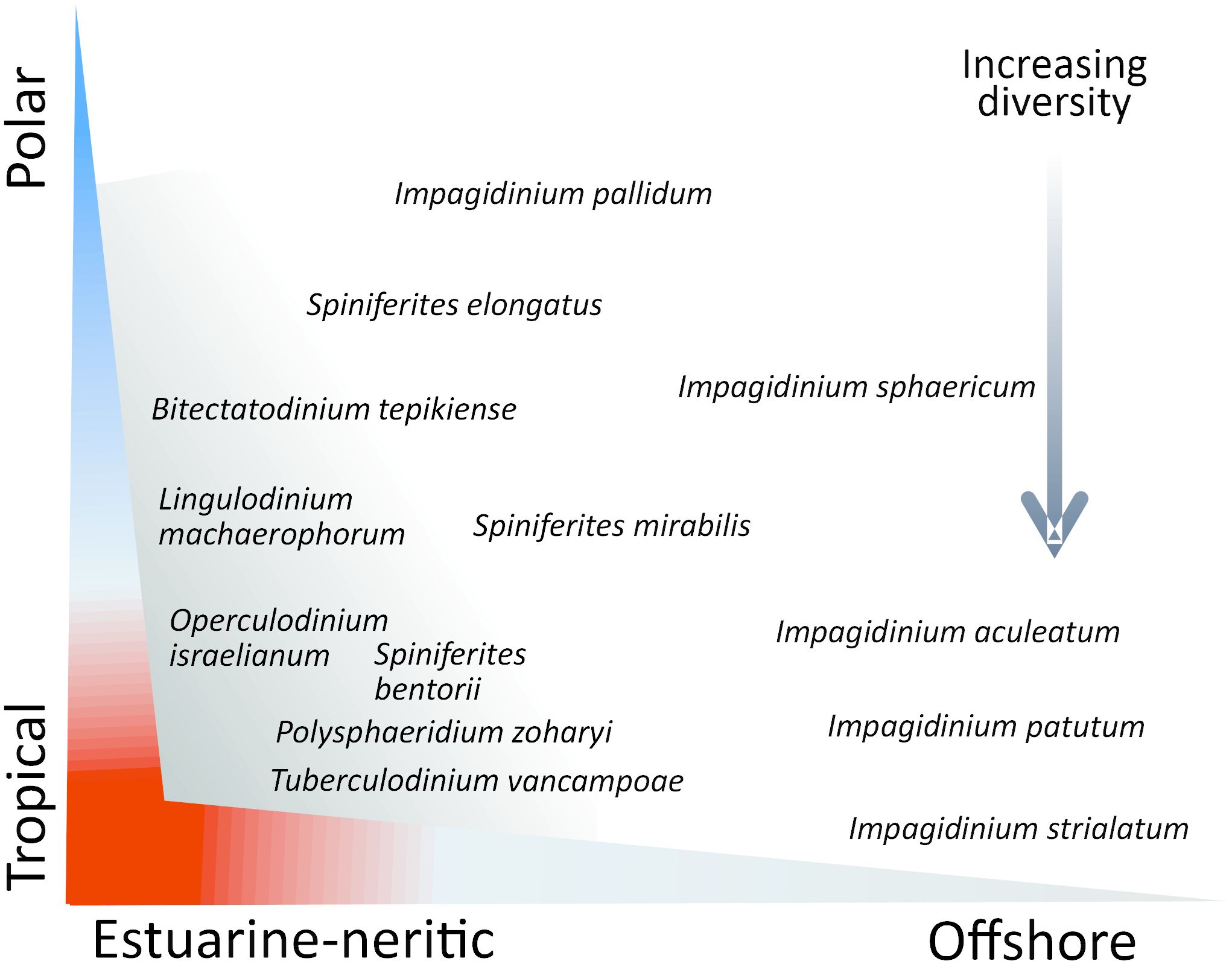
Figure 3 Schematic distribution of some taxa according to general ecosystems and stress vectors determined by estuarine-neritic to offshore gradients (salinity, turbulence, water depths, nutrients) and tropical to polar latitudes (temperature, seasonal contrasts). The sketch with the categories of dinocyst taxa having affinities for neritic, estuarine and offshore settings in tropical to cool temperate environment was inspired by Wall et al. (1977). Only a few taxa are indicated in the figure, most being categorized by Wall et al. (1977). The cosmopolitan taxa Brigantedinium spp., Operculodinium centrocarpum and Nematosphaeropsis labyrinthus are not shown.
Since the early works of Williams (1971) and Wall et al. (1977), regional studies permitted to develop distribution maps of dinoflagellate cysts in sediments of the North Atlantic and adjacent seas (e.g., Harland, 1983; Turon, 1984; de Vernal et al., 1994; Rochon et al., 1999), the Northern Hemisphere Oceans (Radi and de Vernal, 2008; de Vernal et al., 2013a, 2020) and at World Ocean scale (Marret and Zonneveld, 2003; Zonneveld et al., 2013; Marret et al., 2020; see Figure 2). Hence, much progress in the knowledge of the distribution of dinoflagellate cysts in surface sediments has been made in the last decades, strengthening the early observation and inferences by Wall et al. (1977).
Dinoflagellate cysts recovered in sediment are related to pelagic productivity but the taphonomic processes between the dinoflagellate population in upper water mass and their cysts in the thanatocoenoses have been a matter of debate. Comparison of dinoflagellate in plankton tows (theca) and sediment traps or sediments at the sea floor (cysts) have shown a general agreement in the taxonomic content allowing inferences about dinoflagellate populations in surface water and cyst assemblages in the sediment (e.g., Dodge and Harland, 1991; Dodge, 1994; Heikkilä et al., 2016) although only a small proportion of dinoflagellate taxa produces fossilisable cysts (e.g., Head, 1996). While a large part of the cyst fluxes is likely to derive from the upper water masses through settling with marine snow and faecal pellets (Turner, 2015), hydrodynamical factors can be involved in the sedimentation of the cysts (Wall et al., 1977; Nooteboom et al., 2020). Hence, lateral transport of dinoflagellate cysts before burial as any other small-size particles cannot be ruled out. Nevertheless, the sharp boundaries in the cyst distribution on the sea floor suggest a limited impact of distal transport on the assemblages (e.g., de Vernal et al., 2020), as also shown at the zonal scale through modelling experiment (Thöle et al., 2023). The analyses of other microfossils recovered in palynological slides such as pollen and spores that are necessarily allochthonous provide clues about potential nearshore-offshore transport, which appears very limited beyond the estuarine and inner neritic zone (e.g., de Vernal and Giroux, 1991; Rochon and de Vernal, 1994). Among taphonomical processes, selective preservation could be considered. It has been shown that the organic-walled cysts of some taxa, notably those belonging to protoperidinales, could be affected by oxidation (Zonneveld et al., 2008, 2019). Nevertheless, despite possible biases due to differential preservation, the analysis of cyst distribution in recent sediments highlights close relationships with sea-surface conditions (e.g., de Vernal et al., 2020). Abundant protoperidiniale cysts recovered in the sediment may also suggest a limited impact of oxidation, at least along continental margins where productivity is high and sedimentary inputs result in rapid burial.
In general, the concentrations of organic-walled dinoflagellate cysts are high in neritic marine environments and decrease offshore, showing very little fluxes in the distal deep ocean settings (Figure 4A). Such a feature may be due to generally low dinoflagellate productivity in central parts of the ocean, but also to poor preservation or both. Hence, dinoflagellate cysts appear particularly useful for the study of continental margin areas, where their species diversity and abundance are high (Figure 4B).
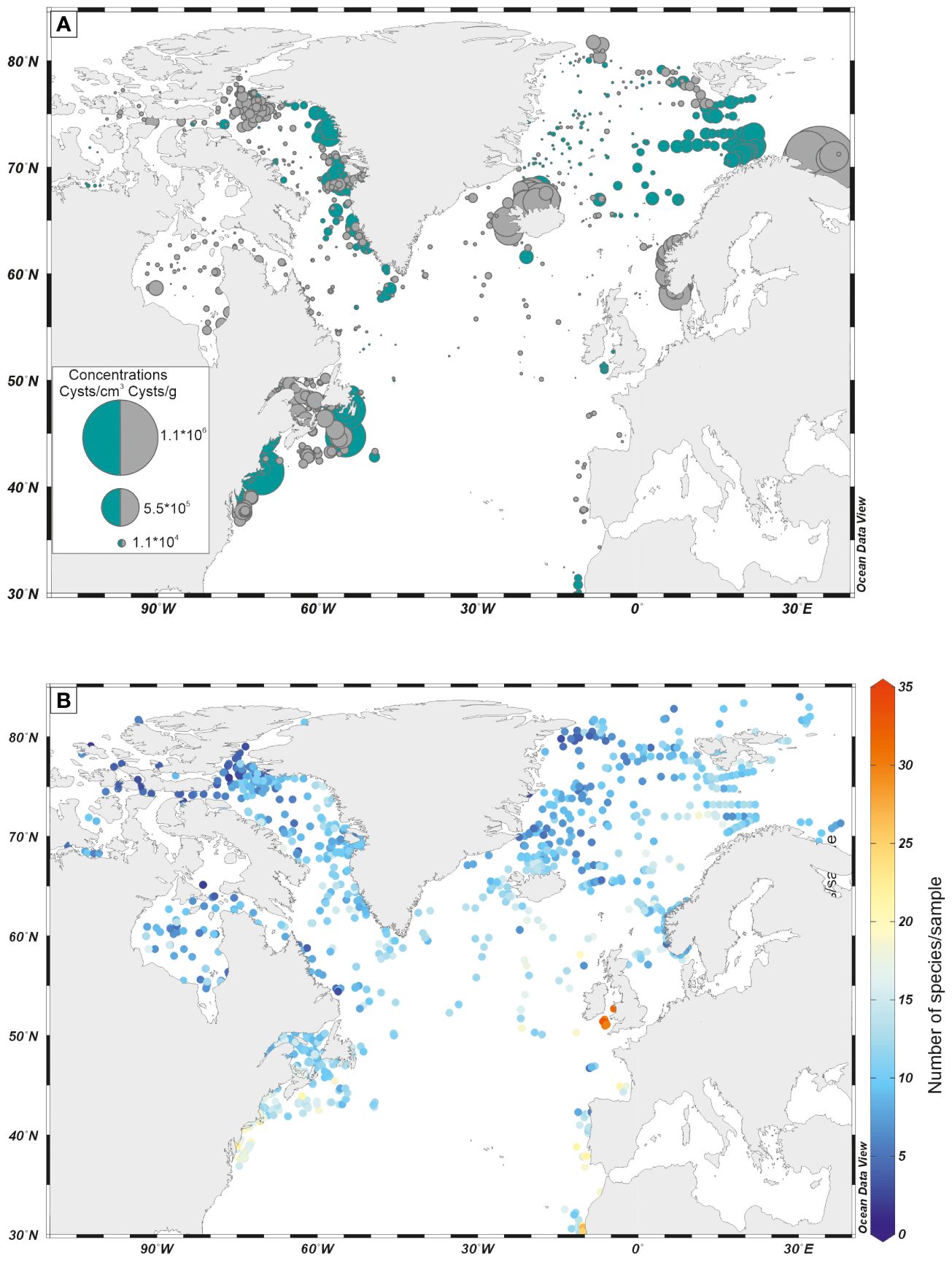
Figure 4 (A) Distribution map of cyst concentration (in specimen/g and specimen/cm3) in surface samples, in the North Atlantic Ocean; (B) Number of species per assemblage in surface samples, in the North Atlantic Ocean. Dinoflagellate cyst data are from the database DB=1968 (de Vernal et al., 2020).
The modern distribution of dinoflagellate cysts around the world documents the geographical range and ecological affinities of the species. Many taxa can be considered cosmopolitan, meaning they occur in most of the oceans and seas, while others have a more restricted spatial distribution (e.g., Marret et al., 2020). Table 1 highlights that most taxa are found in the three oceans, but their relative abundance can vary in importance. Among the 83 taxa that we discuss here, very few are strictly endemic, and these are mostly found in the Southern Ocean, close to the Antarctic continent (Figure 5). Although McMinn (1995) initially reported the absence of Quaternary dinoflagellate cysts south of 60°S, subsequent studies highlighted a relatively the occurrence of up to 15 taxa, including the new species Cryodinium meridianum, Nucicla umbiliphora, and Selenopemphix antarctica (Marret and de Vernal, 1997; Esper and Zonneveld, 2002; Hartman et al., 2019). Another spot of endemism well documented is the Black Sea-Caspian Sea region, with some species being a legacy from the Paratethyan Sea (e.g., Mudie et al., 2018). It can be noted that recent human activities have enabled the spread of some endemic species. One example is that of Peridinium ponticum, an endemic species from the Ponto-Caspian region abundant in the northwestern part of the Black Sea (Mudie et al., 2017), but recently reported to occur in the Ria de Vigo (Atlantic margin of NW Iberia; García-Moreiras et al., 2023) and off Brittany (Mudie et al., 2017). In these cases, ballast waters, which are rich in micro-organisms that can relocate and thrive in different geographical regions (e.g., Cheniti et al., 2018), may have constituted an efficient mechanism for cyst dispersal.
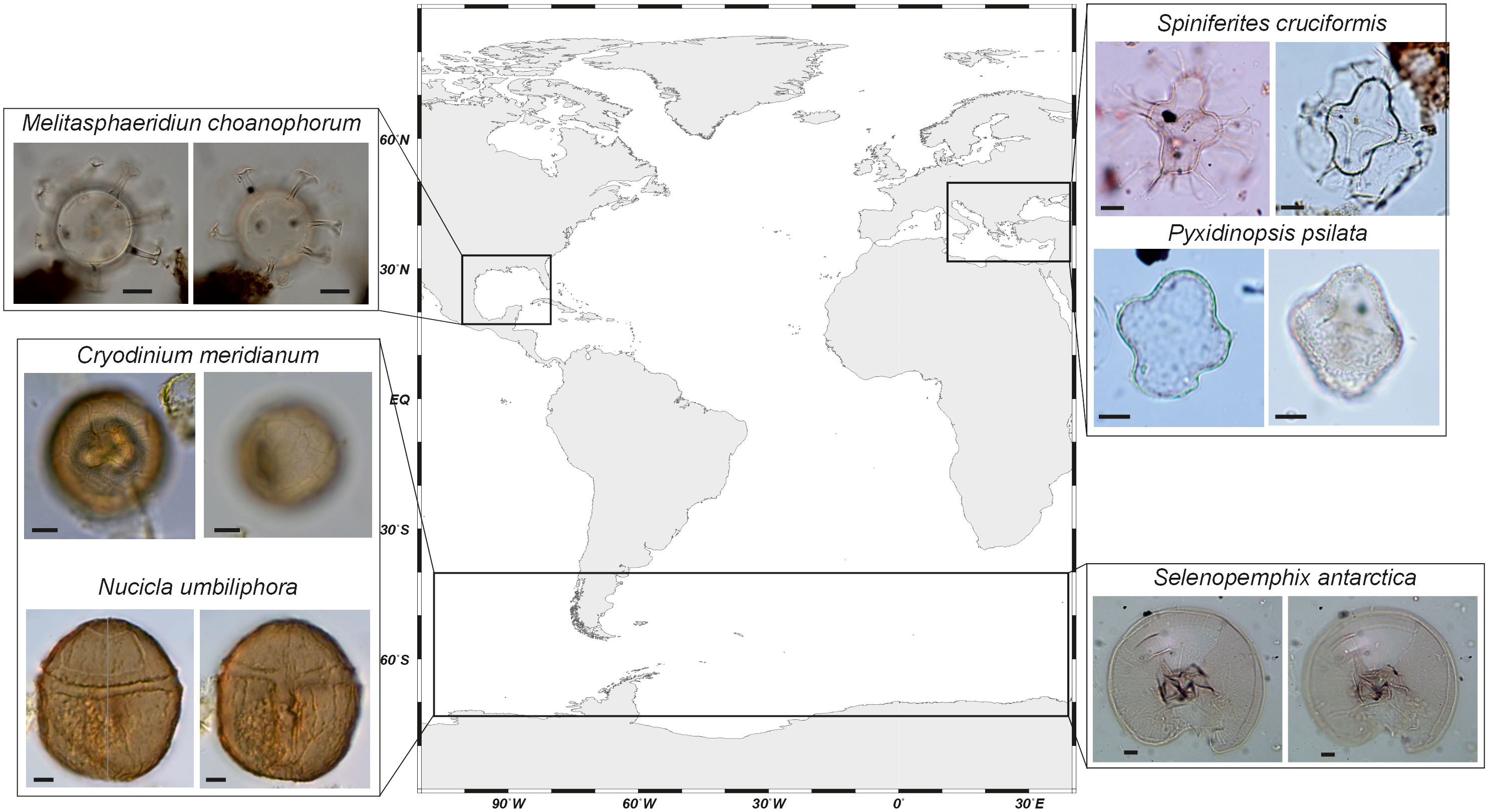
Figure 5 Endemism in dinoflagellate cyst population. Photos from Spiniferites cruciformis and Pyxidinopsis psilata are from the Holocene of the Black Sea (core MAR02–45P, Marret et al., 2009; photos are from courtesy of Fabienne Marret). Photos of Selenopemphix antarctica are from the Holocene off Adélie Land (core MD03–2601, photos are from courtesy of Fabienne Marret). Photos of Melitasphaeridium choanophorum are from the Cariaco Basin, ODP Site 1002, core section 1H 5W 11–13, courtesy of Kenneth Mertens). Photos of Cryodinium meridianum and Nucicla umbiliphora from recent sediments off Antarctica are courtesy of Julian Hartman and Francesca Sangiorgi (Hartman et al., 2018, 2019). Scale bar is 10µm.
4 From biostratigraphy to quantitative palaeoceanography in the Northern Hemisphere
4.1 Biostratigraphy, ecostratigraphy and climate changes
At the scale of the Mesozoic and Cenozoic, dinoflagellate cysts were used as biostratigraphy markers, and their distribution revealed maximum taxonomic diversity during the Cretaceous and Palaeogene (Fensome et al., 1993, 2019; Riding et al., 2022). Hence, dinoflagellates, or at least the taxa yielding organic-walled cysts, show affinities with warm oceans. During the Neogene, since about 23 Ma, a decreasing diversity of species appears to be a common feature in many time series (e.g., Wrenn and Kokinos, 1986; Head and Wrenn, 1992). It seems to have been accompanied by a decrease in the proportion of warm water relative to cold water taxa and was thus associated with a global cooling trend (Boyd et al., 2018). From the early Pliocene to the Quaternary (5.33–2.58 Ma), which is an interval marked by the development of ice sheets in the Northern Hemisphere, the decrease in species diversity of dinoflagellate cysts continued with many disappearances and rare new occurrences of new taxa. This has been documented from several drilling sites in the northern North Atlantic (e.g., De Schepper and Head, 2008, 2009; De Schepper et al., 2017; Aubry et al., 2020), where assemblage changes could result from ocean re-organisation linked to global cooling, sea-level variations and Arctic gateway configuration changes at the onset of glaciations (e.g., De Schepper et al., 2015). A relationship between dinocyst biodiversity and global climate thus emerges from biostratigraphical schemes of the Cenozoic (De Schepper et al., 2015; Boyd et al., 2018). However, the timing of the biostratigraphic events and their distribution in space is not documented well enough to elucidate the mechanisms. In addition to the large-scale climate changes involving temperature shifts, the sea-level variations and the rapid modification of the physiography and coastlines during the glaciations and the transitions from glacial to interglacial stages might have represented major stresses for cyst-forming dinoflagellates. In particular, the emergence of shelves and ice advances over the continental margins might have been critical for the taxa with a life cycle involving a benthic stage before germination, and cell migration to the upper water column.
The few biostratigraphic schemes available for the Quaternary period suggest little turnover of dinoflagellate cyst taxa. However, the last occurrences of a few taxa including Habibacysta tectata and Filisphaera filifera, which progressively disappeared from the Arctic Ocean and the northern North Atlantic over the last two million years (de Vernal et al., 1992; Matthiessen et al., 2018; Figure 6), seems to correspond to the intensification of glaciations (Lisiecki and Raymo, 2005) and possibly the development of perennial sea-ice cover in the Arctic Ocean (Polyak et al., 2013). Conversely, some taxa such as the cyst of Pentapharsodinium dalei recorded their first occurrence and increase in abundance at high latitudes during the Quaternary (Figures 6, 7), which could also relate to a global cooling trend.
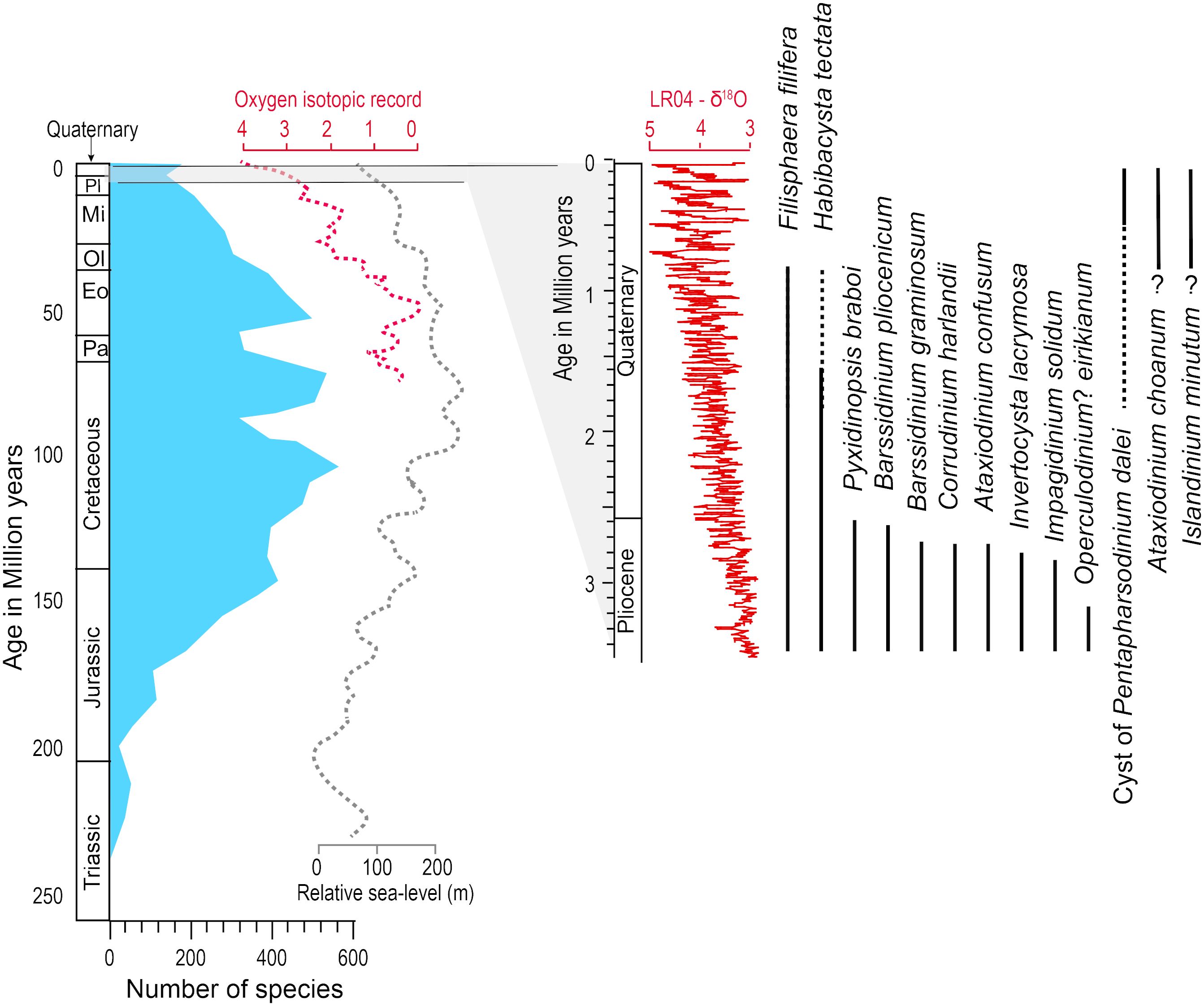
Figure 6 Figure illustrating the taxonomic diversity of dinoflagellate cyst though time. At the scale of the Mesozoic and Cenozoic, the figure is redrafted from Sluijs et al. (2005), with the number of species being from MacRae et al. (1996) and sea level curve from Haq et al. (1987). The Oxygen isotopic curve of the Cenozoic and the last 3.5 million years are from Cramer et al. (2009) and Lisiecki and Raymo (2005), respectively. At the scale of the latest Pliocene and Quaternary, the biostratigraphic ranges of a few species typical of the northern North Atlantic is illustrated from de Vernal et al. (1992), De Schepper et al. (2017) and Aubry et al. (2020).
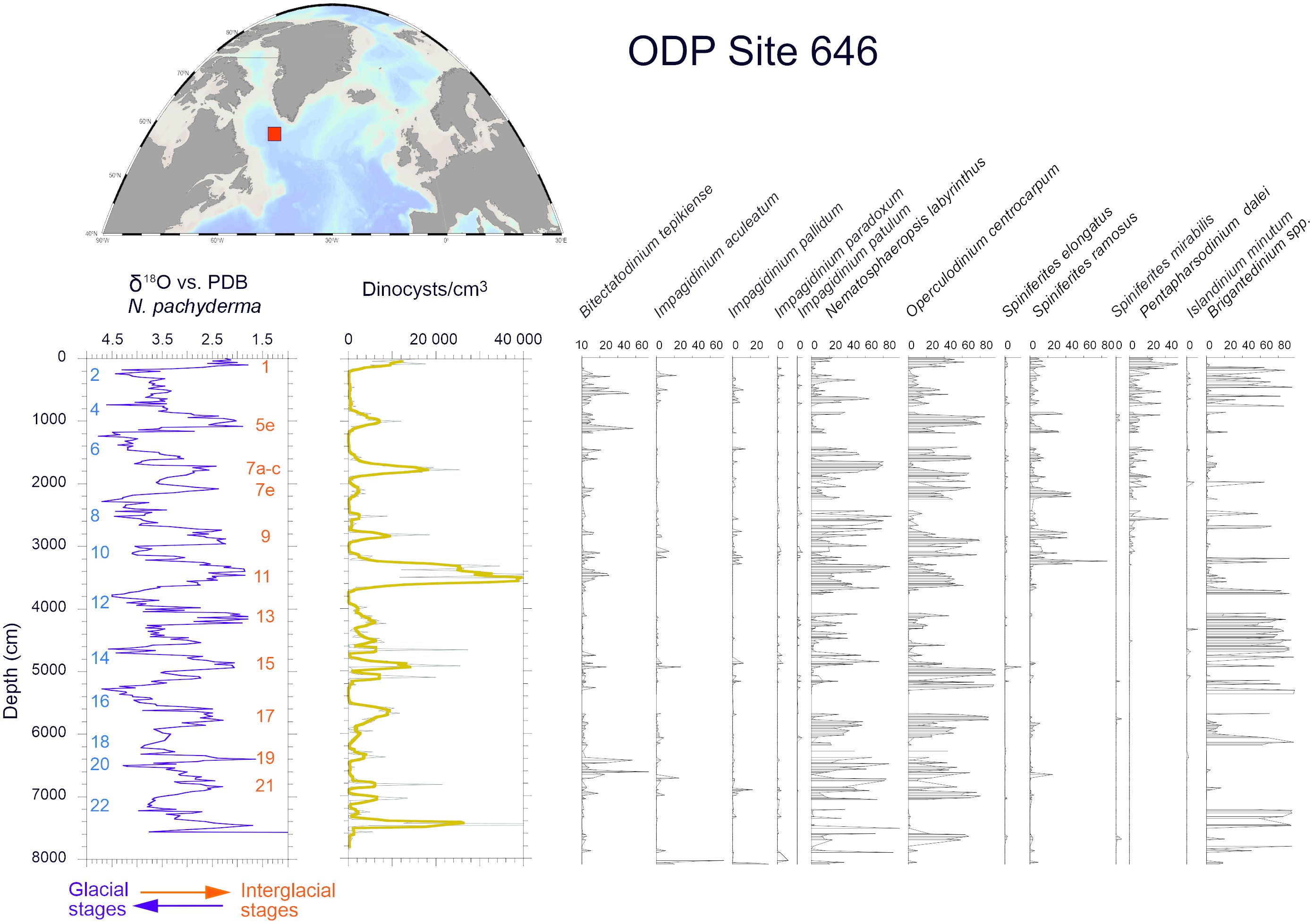
Figure 7 Percentages of the main dinocyst taxa in the sedimentary sequence spanning the last 800,000 years at the Ocean Drilling Programme (ODP) 646. Dinoflagellate cysts data are from de Vernal and Mudie (1992) and the isotope stratigraphy from Aksu et al. (1989).
At the scale of the Quaternary, marked by the alternation of glacial and interglacial stages, with global sea-level changes ranging up to 140 meters (Lisiecki and Raymo, 2005; Lambeck et al., 2014; Dutton et al., 2015), dinoflagellate cysts were used as ecostratigraphic markers. A few studies from the Northern North Atlantic document changes over several glacial to interglacial cycles (de Vernal and Mudie, 1992; Eynaud, 1999). Among those, the analysis of an 800,000-year long sequence at the Ocean Drilling Site (ODP) 646 in the northwest North Atlantic (Figure 7), shows large variations following the pacing of glacial-interglacial cycles. High concentrations, thus cyst fluxes, characterized the interglacial stages while changes in assemblages point to variations of temperatures from cool to temperate.
Among phototrophic taxa, the cosmopolitan species Nematosphaeropsis labyrinthus and Operculodinium centrocarpum are abundant and the accompanying taxa having affinities for more distinct environments illustrate important changes. For example, Spiniferites mirabilis, a taxon presently characteristic of warm temperate conditions (Figure 8A, de Vernal et al., 2018), recorded a small peak at ODP Site 646 during the last interglacial (marine isotope stage -MIS- 5e). It recorded maximum occurrences in MIS 5e sediments of several sites of the northern North Atlantic and Nordic Seas (Penaud et al., 2008), pointing to the spreading of warm temperate waters in the northern North Atlantic (Figure 8B). Another example is provided by the distribution of Bitectatodinium tepikiense, which presently characterizes the margin of southeastern Canada where low sea-surface salinity results in strong stratification, low thermal inertia and large contrasts of temperatures from freezing winters to warm summers (de Vernal et al., 2005, 2020; Figure 9A). This taxon invaded the North Atlantic episodically, indicating episodes of large meltwater-freshwater discharges, notably during the last ice age (cf. de Vernal et al., 2005; Figure 9B).
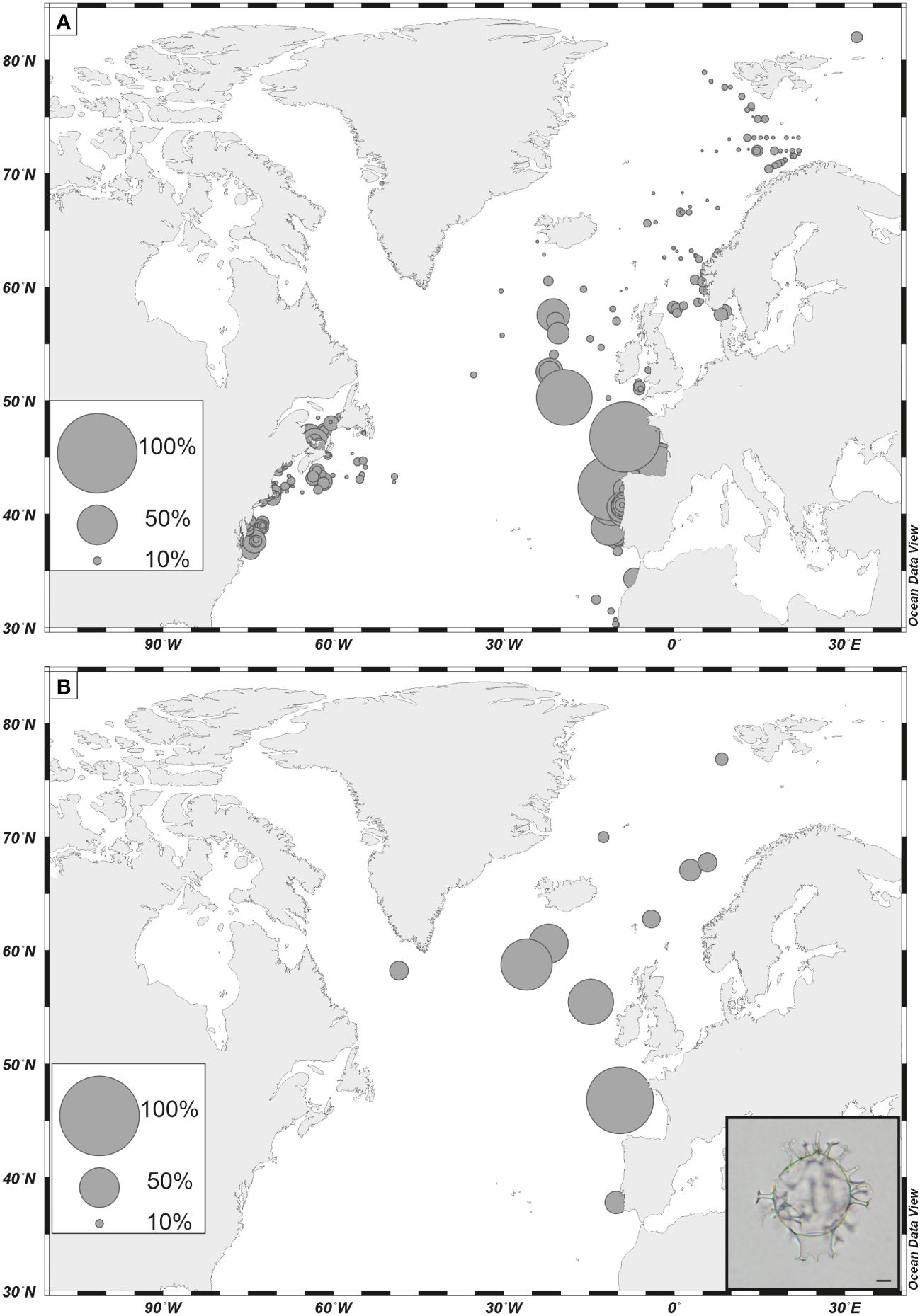
Figure 8 (A) Percentages of Spiniferites mirabilis in dinoflagellate cyst assemblages of surface sediment as reported in the updated n=1968 database (de Vernal et al., 2020); (B) percentages of Spiniferites mirabilis in assemblages of the last interglacial (marine isotope 5e). Data are from de Vernal and Hillaire-Marcel (2008), (Van Nieuwenhove et al., 2008, 2011, 2013), Van Nieuwenhove and Bauch (2008), Eynaud (1999), Eynaud et al (Eynaud et al., 2000, 2004), Matthiessen et al. (2001); Penaud et al. (2008). Photos is courtesy of André Rochon, scale bar is 10 µm.
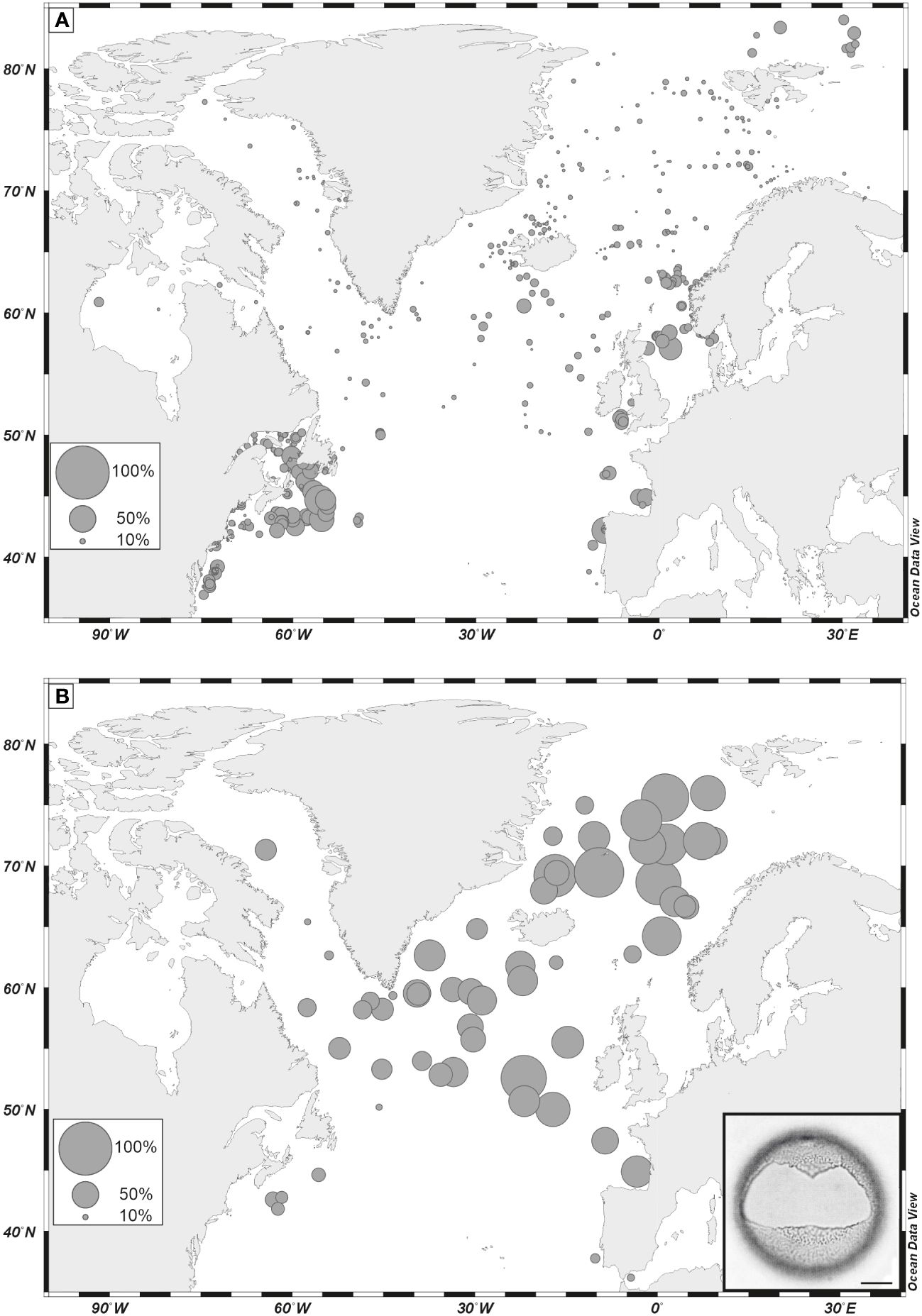
Figure 9 (A) Percentages of Bitectatodinium tepikiense in dinoflagellate cyst assemblages of surface sediment as reported in the updated n=1968 database (de Vernal et al., 2020); (B) percentages of Bitectatodinium tepikiense in assemblages of the Last Glacial Maximum. Data from de Vernal et al. (2005). Scale bar is 10 µm.
In general, phototrophic taxa appear dominant during interglacial stages while heterotrophic taxa often characterize glacial intervals. In the northern North Atlantic, the dominance of heterotrophic taxa is common along continental margins marked by sea ice spreading during glacial times (Figure 10A). A high proportion of heterotrophic taxa, low species diversity, and common occurrence of Islandinium minutum (Figure 10B) are typical features of seasonal sea ice environments (de Vernal et al., 2001, 2013b, 2020). This could be related to primary productivity dominated by diatoms, which have the particularity to make blooms at the sea-ice edges, thus being more competitive than phototrophic dinoflagellates but providing food for heterotrophic taxa (e.g. Hamel et al., 2002; Radi and de Vernal, 2008).
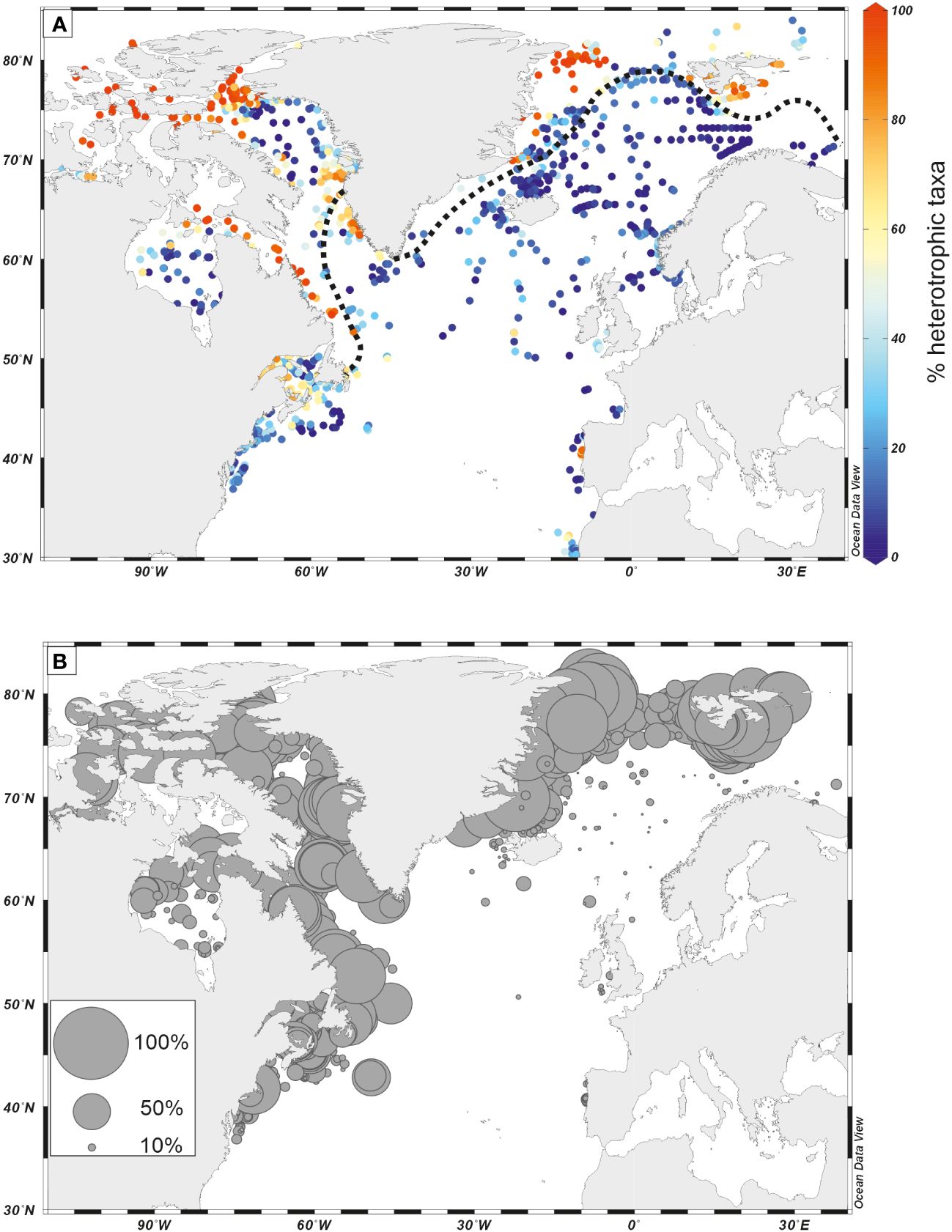
Figure 10 (A) Distribution of percentages of heterotrophic taxa in surface sediment assemblages; (B) Distribution of percentages of Islandinium minutum in surface sediment assemblages. Dinoflagellate cyst data from the updated n=1968 database (de Vernal et al., 2020). Maximum sea-ice (black dotted line) was drawn based on the compiled average position in March (1981–2010) after the National Snow and Ice Data Center (NSDC) online data.
4.2 Quantifying ocean changes from dinoflagellate cyst assemblages
Quantitative interpretations using transfer functions or analogue methods require standardised databases. The uniformization of the laboratory procedures and dinoflagellate cyst taxonomy undertaken permitted the development of a modern database of the Northern Hemisphere that was regularly updated since the 1990s (de Vernal et al., 1994; Rochon et al., 1999; de Vernal et al., 2001, 2013a, 2020). The last update includes 1968 sites and 71 taxa (de Vernal et al., 2020; Van Nieuvenhowe et al., 2020b). Before making reconstructions, it was necessary to demonstrate the linkages between the assemblages and the parameters to estimate. The standardized database was thus analysed through multivariate techniques such as canonical correspondence analyses (cf. Ter Braak and Smilauer, 1998) to evaluate the strength of the relationships between dinoflagellate cyst assemblages and sea-surface parameters including seasonal temperature, salinity, sea-ice cover and primary productivity. All trials demonstrated that temperature is a determinant parameter but that other parameters are important, including salinity, seasonality and productivity (e.g. Radi and de Vernal, 2008; Bonnet et al., 2010; de Vernal et al., 2013a, 2020; Hohmann et al., 2023). They further show that the strength of the relationship between the assemblage and given parameters may vary depending on the ocean basin, where the range of different ocean parameters may differ. In the Pacific Ocean, for example, temperature and productivity were revealed to be the most determinant parameters, while in the North Atlantic, temperature and salinity in addition to sea-ice cover explain most of the variance in assemblages (cf. Radi and de Vernal, 2008). On these grounds, different methods of reconstruction were attempted, including the analogue techniques, and calibration techniques such as the artificial neural network and the weighted average partial-least square (Rochon et al., 1999; Peyron and de Vernal, 2001; Bonnet et al., 2010; Hohmann et al., 2023). Validation tests on modern samples have shown good results with all approaches. Low prediction errors could be obtained from the application of calibration techniques on regional data sets. However, when dealing with fossil assemblages, one cannot assume that the modern conditions at the regional scale comprise all past situations. For this reason, the use of modern analogue techniques with databases as large as possible was generally prioritized for palaeo-reconstruction (see Bonnet et al., 2010). Reconstructions from dinoflagellate cyst assemblages in the subpolar North Atlantic have indeed shown extra-regional analogues for late Quaternary samples (e.g. Allan et al., 2018). Moreover, the analogue techniques also identify non-analogue or poor-analogue situations leading to the assessment of the reliability of reconstruction and discarding results when representative analogues are missing.
Three examples of time series illustrating the reconstructions of sea-surface conditions across the North Atlantic cover from the last glacial maximum to the late Holocene are presented here (Figure 11). The example from the Labrador Sea (core HU91–045-094), off southeastern Canada, shows large amplitude changes from glacial to interglacial, with generally dominant heterotrophic taxa and common occurrence of Islandinium minutum during glacial time, pointing to seasonal sea-ice cover. The assemblages of the postglacial sediments are dominated by the cosmopolitan phototrophic taxa Operculodinium centrocarpum and Nematosphaeropsis labyrinthus, and the interval from 11,700 to ~7000 years BP marks the transition with decreasing percentages of heterotrophic taxa. It is also characterized by maximum occurrences of Bitectatodinium tepikiense and Impagidinium sphaericum, thus showing both warm and cold conditions. The reconstructions indicate indeed a large seasonal contrast of temperature with optimum conditions in summer and cold winter in a context of strongly stratified surface waters due to low salinity because of meltwater discharge during ice retreat. The record demonstrates that dinoflagellate cyst assemblages permit to disentangling of winter and summer temperatures, and temperature and salinity. In the example of the core from the Labrador Sea, the simultaneous reconstruction of several ocean parameters is possible because the modern analogues comprise the combination of high-low temperatures under variable salinities in addition to a wide range of seasonal contrasts of temperature (de Vernal et al., 2005, 2020).
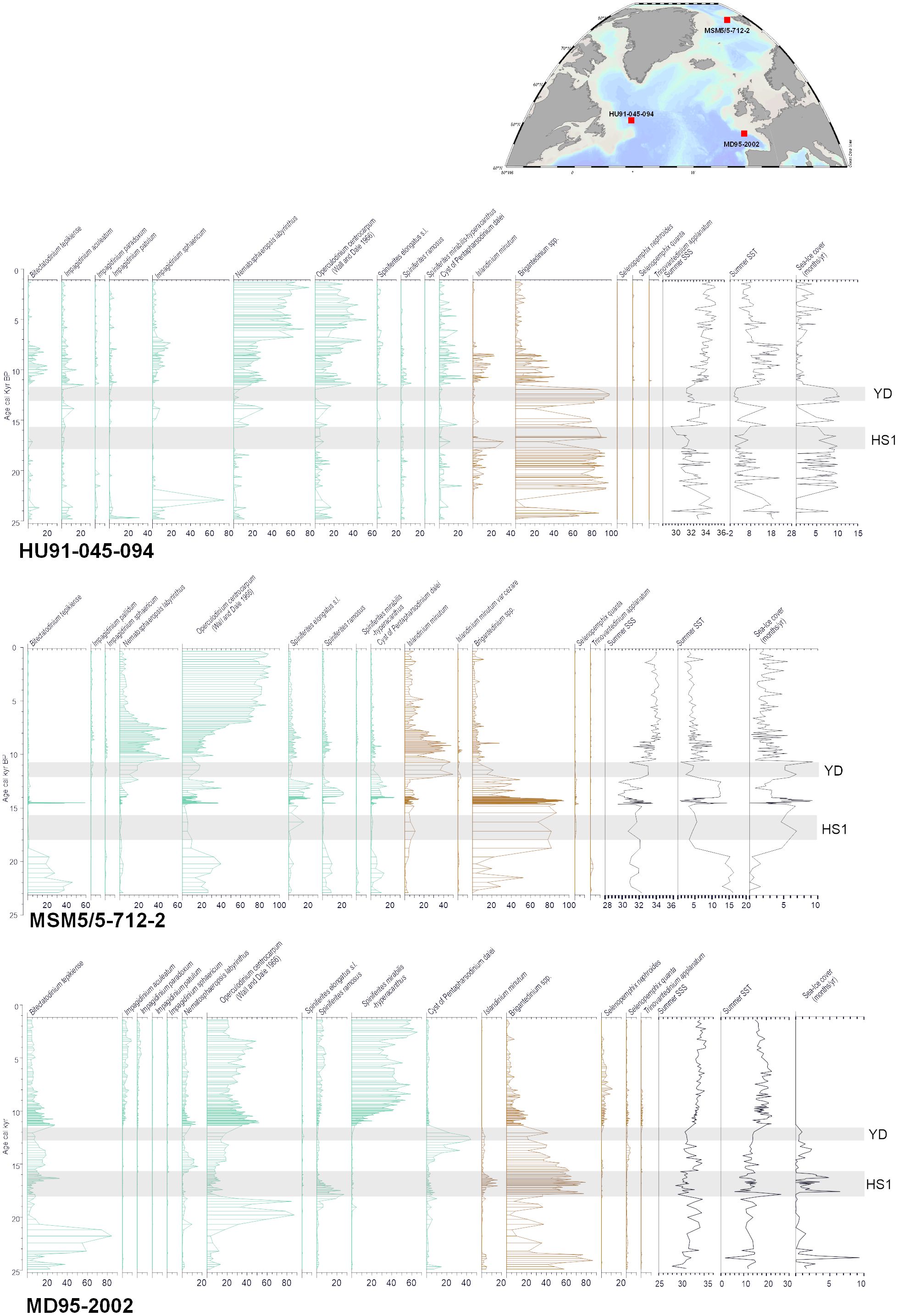
Figure 11 Percentage diagram of selected indicator taxa in sediment encompassing the last 25,000 years in cores MD95–2002 (Eynaud, 1999; Zumaque et al., 2017), MSM5–712 (Falardeau et al., 2018) and HU91–045-094 (de Vernal et al., 2000) from the northern North Atlantic. The histograms are in green and brown for phototrophic and heterotrophic taxa respectively. The reconstructions were made from the modern analogue technique using the n=1968 database (de Vernal et al., 2020). The cold pulses of the Younger Dryas (YD) and Heinrich Stadial 1 (HS1) are shown by gray bands.
In a polar context, the record from the Svalbard margins (core MSM5/5–712-2; Falardeau et al., 2018; Figure 11) in the axis of the North Atlantic Current flowing northward into the Arctic Ocean illustrates also the transition from glacial to interglacial conditions. The Last Glacial Maximum was characterised by Bitectatodinium tepikiense (see Figure 9B) pointing to a warm summer but cold winter, and the Heinrich events were characterised by dominant heterotrophic taxa, leading to reconstructed cold conditions. The transition of the early postglacial, from ~12,000 to 7500 years BP was marked by common Operculodinium centrocarpum and Nematosphaeropsis labyrinthus and occurrences of both cold water (Islandinium minutum) and warm water taxa (Spiniferites mirabilis, Impagidinium sphaericum), which permitted to reconstructed strong seasonal contrasts of temperatures in a context of low salinity (Falardeau et al., 2018). This example also illustrates large amplitude changes from glacial to interglacial and shows the decoupling of temperatures and salinity.
In a middle latitude context, the data from the western European margins (Core MD95–2002; Eynaud, 1999; Zumaque et al., 2017; Figure 11) illustrate high diversity in dinoflagellate cyst assemblages and large changes from glacial to interglacial, but also within the last ice age, which was marked by the succession of dominant heterotrophic taxa and Bitectatodinium tepikiense or Operculodinium centrocarpum, indicating large variations of salinity and cold pulses with some winter sea-ice cover spreading (Eynaud et al., 2012). At this site, the postglacial interval is marked by abundant Spiniferites mirabilis and Impagidinium sphaericum that illustrate warm temperate conditions (Zumaque et al., 2017). The data led to the reconstruction of a mid-Holocene thermal optimum ending around 5000 years BP as at many other North Atlantic sites (Kaufman et al., 2020), but the particularity of the dinoflagellate cyst record is to evidence seasonal contrast, with summer warmth without winter optimum.
5 Palaeo intertropical environments and Southern Hemisphere
Among sea-surface conditions that we reconstruct from biogeochemical proxies and microfossil assemblages, palaeoproductivity may be the most challenging one, besides sea-ice cover. Dinocysts have proved to be good indicators of palaeoproductivity, in particular in regions where net primary productivity is possibly the most dominant control on dinoflagellates (see Radi and de Vernal, 2008; Penaud et al., 2018). Coastal regions, where important river discharges (or plumes) occur, are usually characterized by rich dinocyst assemblages, with a zonation linked to the presence of nutrients and hydrographic conditions. The Atlantic Ocean contains zones where Chlorophyll-a (reflecting the abundance of phytoplankton) is highly abundant, mostly along coastal regions where eastern boundary currents occur (Figure 12A). The West African coastline has several sectors with permanent upwelling systems (Canary Current, Benguela Current). The equatorial region has also upwellings linked to the latitudinal movement of the Intertropical Convergence Zone (ITCZ). In the eastern equatorial Atlantic Ocean, major rivers (Congo, Niger) bring in the marine environment freshwater and nutrients, affecting coastal waters up to a certain distance from the shore. The Congo River discharge is known to create the phenomenon of river-induced upwelling, bringing up to the surface nutrients (e.g., Cadée, 1978; Eisma and Van Bennekom, 1978).
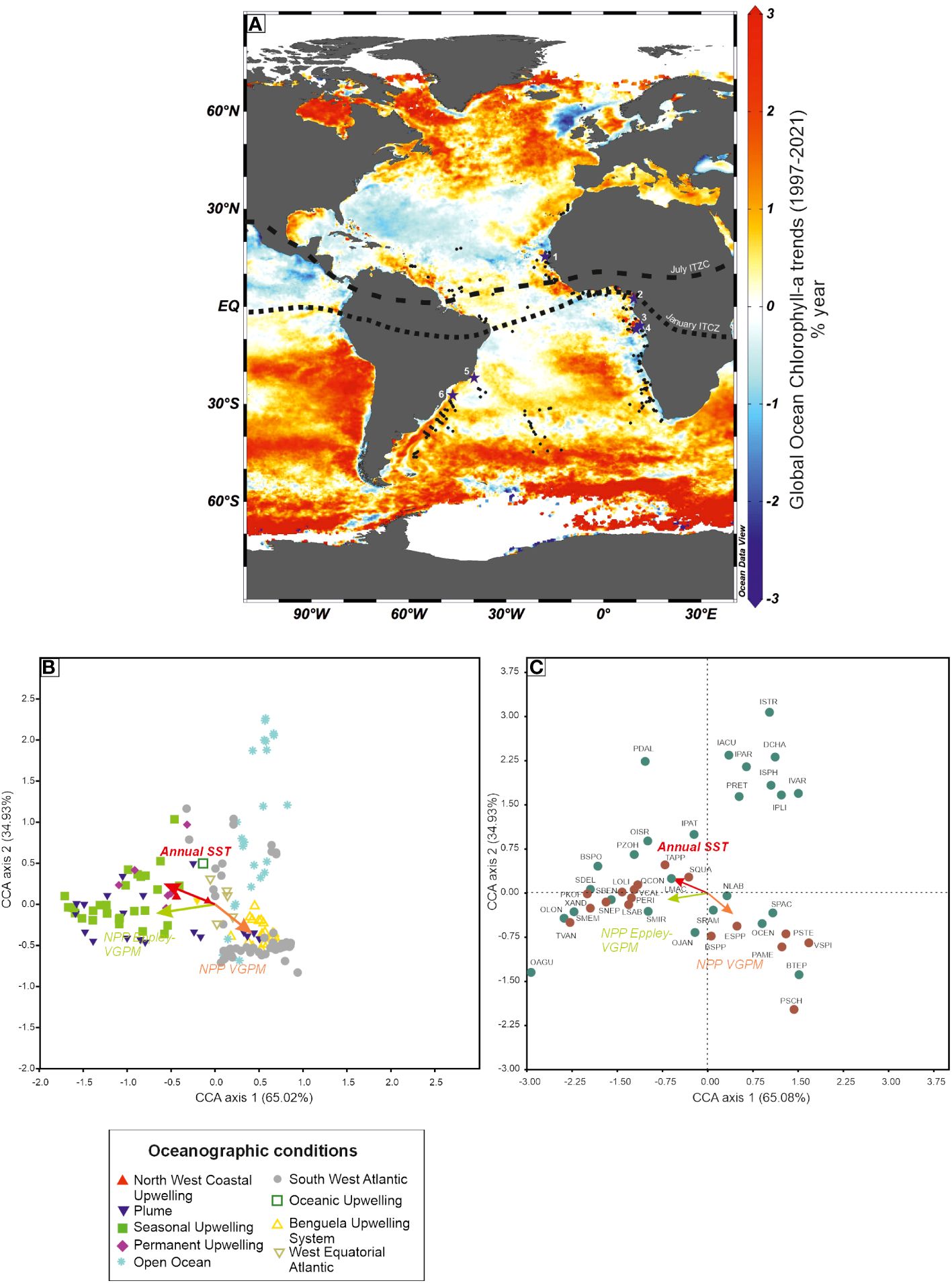
Figure 12 (A) Global distribution of ocean Chlorophyll-a (change in mass concentration over time) (DOI (product): https://doi.org/10.48670/moi-00230); location of dinoflagellate cyst assemblages in surface sediments (from Hardy et al., 2018); position of the Intertropical Convergence Zone; location of cores cited in the text and Figure 13 [1: core GeoB9508–5 (Bouimetarhan et al., 2013); 2: core GeoB4905–4 (Marret et al., 2013); 3: core KZAI-01 (Hardy et al., 2016); 4: Core GeoB1008–3 (Dupont et al., 1999); 5: Core GeoB3202–1 (Gu et al., 2020); 6: Core GeoB2107–3 (Gu et al., 2017)]. (B) Canonical correspondence analysis on the 164 assemblages from Hardy et al. (2018) with position of the controlling factors (Annual Sea-Surface Salinity, Annual Sea-Surface Temperature and Net Primary Productivity (NPP) (see Hardy et al., 2018). Symbols are allocated to assemblages in relation to the oceanographic conditions at their location. (C) Canonical correspondence analysis on the taxa occurring in the 164 assemblages, with phototrophic taxa in green and heterotrophic taxa in brown (adapted from Hardy et al., 2018), and delimited by four quarters by dotted lines. Variances in % are indicated between brackets. CCA was run on percentage data of the 164 assemblages.
A regional database of dinoflagellate cyst assemblages was reviewed in Hardy et al. (2018), evaluating their potential to reconstruct sea-surface conditions, in particular past productivity. Of the 237 sites that were selected, 164 were retained as they could be related to pre-industrial conditions for productivity (using Vertically Generalised Production Model, VGPM) and SST. In this study, two NPP models were used, the VGPM and the Eppley-VGPM ones, where the Eppley-VGPM tends to give higher NPP values when SSTs are high. The statistical analysis (CCA) performed on these 164 assemblages (Figure 12B) shows a clustering of assemblages that more or less identifies the different regimes of oceanographical conditions. A canonical correspondence analysis carried out on the taxa occurring in the 164 assemblages shows that taxa are ordinated along three dominant directions (Figure 12C), which strongly suggests that the species are predominantly controlled by SST and productivity, even though the two NPP vectors have a different direction. Taxa in the upper right quarter are usually found in open ocean and western boundary current systems. Taxa in the bottom left quarter usually respond to warmer temperatures compared to the taxa in the bottom right quarter. Most of the heterotrophic taxa are ordinated closely to both NPP vectors.
This situation is similar back in time. Despite rare organic-walled dinoflagellate cyst records in this region, contrasting data highlight the complex response of dinoflagellate cysts to environmental changes. Selected records covering the last 25,000 years, with robust age models, show that the eastern side of the tropical Atlantic Ocean was mostly dominated by heterotrophic taxa, notably Brigantedinium spp., during the last glaciation, and that the Holocene is characterised by a dominance of phototrophic taxa, mainly O. centrocarpum and some Spiniferites species (Figure 13). Heterotrophic taxa also captured the latitudinal migration of the ITCZ, in particular near the deltas of large rivers (e.g., Marret et al., 2013; Hardy et al., 2018). In the western side of the tropical Atlantic Ocean, the records show the dominance of phototrophic taxa, essentially O. centrocarpum, with very little change between glacial and interglacial conditions.
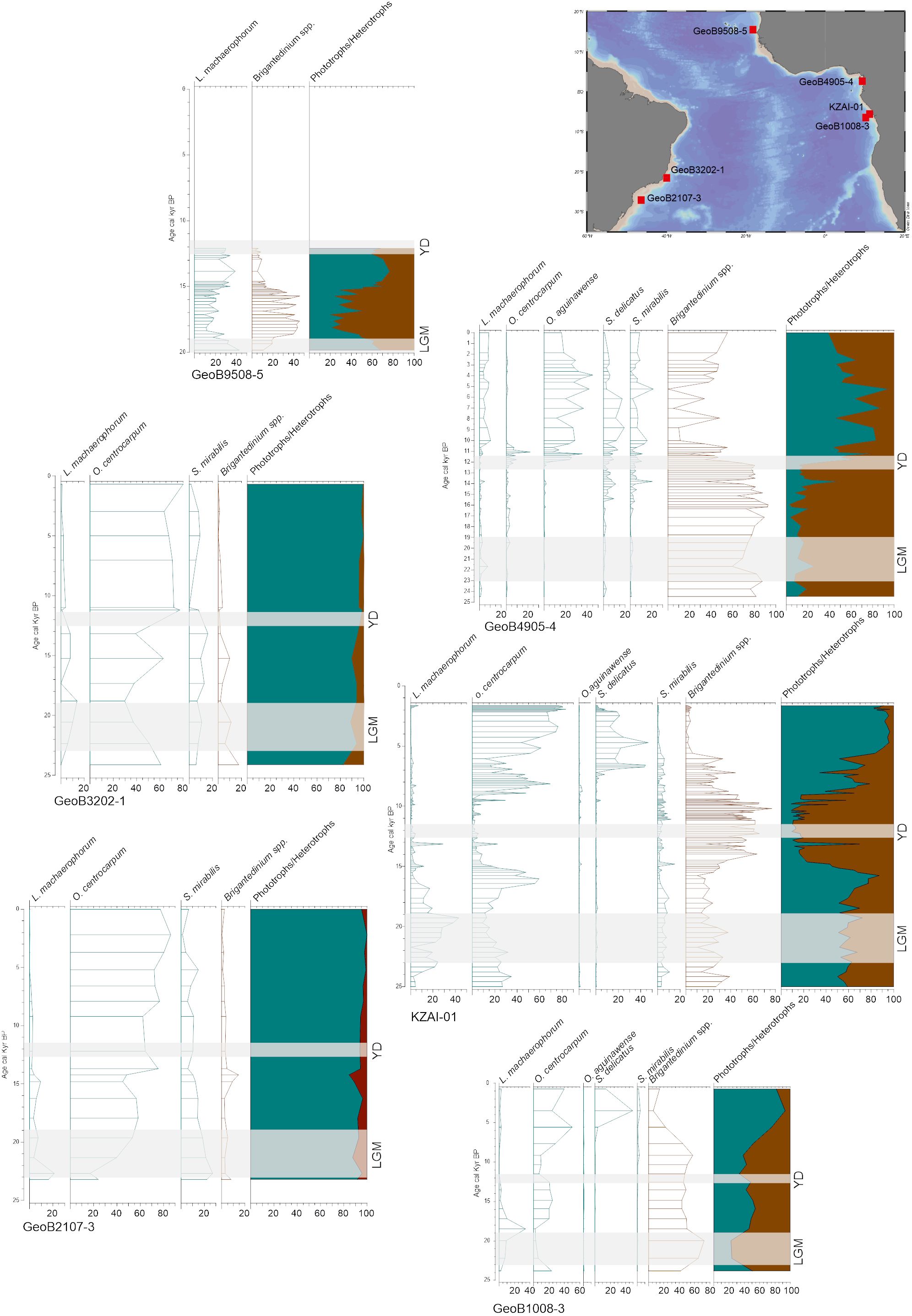
Figure 13 Selected cores in the tropical regions of the Atlantic Ocean, covering the last 25,000 years, with percentages of dominant dinoflagellate cysts (see caption in Figure 12 for details about cores). LGM, Last Glacial Maximum; YD, Younger Dryas.
Further south, very few Quaternary records are available, but they all show a dominance of heterotrophic taxa during glacial marine isotopic stages, notably the MIS2; they also have demonstrated strong potential to trace latitudinal movements of the different fronts (e.g., Esper and Zonneveld, 2007). Off the southwest African coast, ODP Site 1087 integrates signal of Agulhas leakages over the last 500,000 years based on biogeochemical and microfossil proxies; dinoflagellate cysts were used to reconstruct sea-surface temperature, showing a good agreement with alkenone-based SSTs (Petrick et al., 2015). These few studies provide evidence that dinoflagellate cysts can be used to reconstruct complex oceanographic changes over the Quaternary.
6 Environmental issues and dinoflagellates
Some dinoflagellates are notoriously known for lethal toxin production (e.g., Alexandrium sp.); others are red-tide producers, and while not being lethal, can severely affect aquatic ecosystems through the process of eutrophication (e.g., Anderson, 2019). Global monitoring shows that most coastal regions are now affected by HABs (either red tide or toxicity, or both) (https://data.hais.ioc-unesco.org/); that was not the case half a century ago where fewer events were reported or recorded. Causes of such widespread phenomenon are, among others, related to increased agricultural activities, with the release of nutrients (N and P), favouring algal blooms. Transport of organisms in ship ballast waters has also been a contributor. Recent studies have been linking changes in climatic conditions to phytoplankton communities (e.g., Hinners et al., 2019). Therefore, it was very tempting to trace back in time the occurrence of species related to eutrophication to better understand the mechanisms behind their distribution. The Gulf of Mexico is an exemplary case study where the clear relationship between the abundance of specific cyst taxa (mostly heterotrophic species) and nutrient enrichments due to the use of agricultural fertiliser has been established (Price et al., 2018). Of the known species producing toxins or red-tides, very few produce a cyst that can preserve well in sediments (see Table 1, thecate species in bold). Cysts of Alexandrium sp. are often observed in recent sediments and up to a few hundred years but do not seem to be preserved beyond that (e.g., Head et al., 2006). It was debated that these cysts may have been confused with the ones produced by the calcareous dinoflagellate species, Scrippsiella trifida. Mudie et al. (2002) reported sporadic presence of Alexandrium cysts off Newfoundland during the Holocene period, their abundance linked to warming SST. However, Head et al. (2006) demonstrated that these cysts were produced by S. trifida. Nevertheless, other species, which produced resistant cysts, have shown high abundance during warm episodes (L. machaerophorum during the Holocene in the NW Atlantic Ocean, Mudie et al. (2002); Polysphaeridium zoharyi during the Middle Eocene Climatic Optimum warming in east equatorial Atlantic, Cramwinckel et al., 2019).
Fjords have been particularly scrutinised, due to their propensity of becoming eutrophic in recent times. Dale et al. (1999) introduced the concept of using abundance of dinoflagellate cysts as “indicators of cultural eutrophication”. L. machaerophorum, a species occurring worldwide, with a large tolerance to salinity and temperature, has seen their abundance largely increase when additional nutrients entered the waters of the inner basin of the studied fjord (Oslofjord, Norway). Not far from this site, another record from Gullmar Fjord (Sweden) highlights a more complex story, with no strong evidence of the abundance of L. machaerophorum being related to nutrient enrichments (Harland et al., 2006).
Our increasing knowledge of the ecology of dinoflagellate cyst species and their biogeographical distribution highlights that their past occurrence reflects a complex history of oceanographic changes, in particular for more recent times where human activities had a profound impact on coastal ecosystems. However, it is possible to use past assemblages during interglacial periods to obtain an insight into environmental conditions in a warmer world.
7 Closing remarks and perspectives
Compared to other microfossils, dinoflagellate cysts are very particular palaeoecological-palaeoceanographical indicators as summarized in a few points below.
(1) They are organic-walled biogenic indicators of productivity in the photic zone that are usually well preserved in marine sediments unlike those formed of opal silica and calcium carbonate, such as diatoms and coccoliths respectively, that may dissolve when there is undersaturation of silica and carbonate in the water column. From this viewpoint, dinoflagellate cysts are useful and complementary proxies of primary productivity, providing there is no oxidation of organic matter.
(2) The fossilisable cysts of dinoflagellates are mostly abundant in neritic areas and along continental margins and rare in distal offshore settings, possibly because they constitute the benthic stage of hypnozygotes with only a few taxa having the ability for dispersal over long distances in surface waters of the deep ocean. Among those, Impagidinium species stand out in addition to the cosmopolitan taxa Operculodinium centrocarpum and Nematosphaeropsis labyrinthus.
(3) Dinoflagellates include taxa with a wide range of trophic behaviors, phototrophic, heterotrophic and mixotrophic, notably. While phototrophic taxa usually dominate in oceanic settings, heterotrophic ones are often abundant in nearshore areas, and also along the sea-ice edges and the upwelling zones, where diatom blooms dominate the primary production and sustain the development of heterotrophic dinoflagellates. Hence, dinoflagellate cysts offer a holistic view of productivity, beyond what is strictly related to primary production.
(4) Cyst-forming dinoflagellate occur from the equator to the Arctic and Antarctic, where they form assemblages typical of sea-ice environments, often dominated by heterotrophic taxa. Hence, they are useful for making inferences about sea-surface temperature, from tropical to freezing.
(5) Dinoflagellate cyst assemblages with different taxonomical compositions encompass low to high-salinity environments, from estuaries to open oceans, which make them one of the rare proxies of salinity. Similarly, because salinity is closely tied to stratification and thermal inertia making large seasonal contrasts at high latitudes, dinoflagellate cysts also appear to be one of the rare indicators of seasonality.
For all the reasons mentioned above, dinoflagellate cyst assemblages are unique proxies allowing the documenting of past climate and ocean conditions with regard not only to temperature but also considering seasonality, salinity, sea-ice cover, and productivity. However, the taxonomy of dinoflagellate cysts is not necessarily easy, especially when considering the polymorphism of some taxa and the existence of cryptic species. Moreover, the processing of the sediment before microscope observation and the analyses of the dinoflagellate cyst population are time-consuming. As a consequence, the scientific community is currently exploring techniques such as those based on molecular biomarkers and ancient DNA that are less tedious than micropalaeontology to document past dinoflagellate populations and/or species as proxies for key environmental parameters.
Author contributions
FM: Conceptualization, Writing – original draft, Writing – review & editing. AV: Conceptualization, Writing – original draft, Writing – review & editing.
Funding
The author(s) declare that no financial support was received for the research, authorship, and/or publication of this article.
Acknowledgments
The authors deeply thank their colleagues Francesca Sangiorgi, Julian Hartman, Kenneth Mertens and André Rochon for providing dinoflagellate cyst photos to illustrate some of the figures. The authors are very grateful for the constructive comments of two reviewers, helping us to improve our manuscript.
Conflict of interest
The authors declare that the research was conducted in the absence of any commercial or financial relationships that could be construed as a potential conflict of interest.
Publisher’s note
All claims expressed in this article are solely those of the authors and do not necessarily represent those of their affiliated organizations, or those of the publisher, the editors and the reviewers. Any product that may be evaluated in this article, or claim that may be made by its manufacturer, is not guaranteed or endorsed by the publisher.
References
Aksu A. E., de Vernal A., Mudie P. J. (1989). High-resolution foraminiferal, palynological and stable isotopic records of upper Pleistocene sediments from Labrador Sea: paleoclimatic and paleoceanographic trends. Proc. Ocean Drill. Program 105B, 617–652.
Allan E., de Vernal A., Knudsen M. F., Hillaire-Marcel C., Moros M., Ribeiro S., et al. (2018). Late holocene sea surface instabilities in the disko Bugt area, west Greenland, in phase with δ18O oscillations at camp century. Paleoceanogr. Paleoclimatol. 33, 227–243. doi: 10.1002/2017PA003289
Anderson D. M. (2019). “Harmful Algal Blooms,” in Encyclopedia of Ocean Sciences, 3rd ed. Eds. Cochran J. K., Bokuniewicz H. J., Yager P. L. (Academic Press, Oxford), 309–321.
Aubry A. M. R., De Schepper S., de Vernal A. (2020). Dinocyst and acritarch biostratigraphy of the Late Pliocene to Early Pleistocene of IODP Site U1307, Labrador Sea. J. Micropaleontol. 39, 41–60. doi: 10.5194/jm-39-41-2020
Bogus K., Mertens K. N., Lauwaert J., Harding I. C., Vrielinck H., Zonneveld K. A. F., et al. (2014). Differences in the chemical composition of organic-walled dinoflagellate resting cysts from phototrophic and heterotrophic dinoflagellates. J. Phycol. 50, 254–266. doi: 10.1111/jpy.12170
Bonnet S., de Vernal A., Hillaire-Marcel C., Radi T., Husum K. (2010). Variability of sea-surface temperature and sea-ice cover in the Fram Strait over the last two millennia. Mar. Micropaleontol. 74, 59–74. doi: 10.1016/j.marmicro.2009.12.001
Bouimetarhan I., Groeneveld J., Dupont L., Zonneveld K. (2013). Low- to high-productivity pattern within Heinrich Stadial 1: Inferences from dinoflagellate cyst records off Senegal. Global Planet. Change 106, 64–76. doi: 10.1016/j.gloplacha.2013.03.007
Boyd J. L., Riding J. B., Pound M. J., De Schepper S., Ivanovic R. F., Haywoo A. M., et al. (2018). The relationship between Neogene dinoflagellate cysts and global climate dynamics. Earth-Sci. Rev. 177, 366–385. doi: 10.1016/j.earscirev.2017.11.018
Braarud T. (1945). Morphological observations on marine dinoflagellate cultures. Norsk Videnskaps-Acad Oslo Avh Mat.-Naturvidensk 11, 1–18.
Bravo I., Figueroa R. I. (2014). Towards an ecological understanding of dinoflagellate cyst functions. Microorganisms 2, 11–32. doi: 10.3390/microorganisms2010011
Cadée G. C. (1978). Primary production and chlorophyll in the Zaire River, estuary and plume. Netherlands J. Sea Res. 12, 368–381. doi: 10.1016/0077-7579(78)90040-6
Cheniti R., Rochon A., Frihi H. (2018). Ship traffic and the introduction of diatoms and dinoflagellates via ballast water in the port of Annaba, Algeria. J. Sea Res. 133, 154–165. doi: 10.1016/j.seares.2017.07.008
Coussin V., Penaud A., Combourieu-Nebout N., Peyron O., Schmidt S., Zaragosi S., et al. (2022). Distribution of modern dinocysts and pollen in the western Mediterranean Sea (Algerian margin and Gulf of Lion). Mar. Micropaleontol. 175. doi: 10.1016/j.marmicro.2022.102157
Cramer B. S., Toggweiler J. R., Wright J. D., Katz M. E., Miller K. G. (2009). Ocean overturning since the Late Cretaceous: Inferences from a new benthic foraminiferal isotope compilation. Paleoceanography 24, PA4216. doi: 10.1029/2008PA001683
Cramwinckel M. J., van der Ploeg R., Bijl P. K., Peterse F., Bohaty S. M., Röhl U., et al. (2019). Harmful algae and export production collapse in the equatorial Atlantic during the zenith of Middle Eocene Climatic Optimum warmth. Geology 47, 247–250. doi: 10.1130/G45614.1
Dale B., Thorsen T. A., Fjellså A. (1999). Dinoflagellate cysts as indicators of cultural eutrophication in the Oslofjord, Norway. Estuarine Coast. Shelf Sci. 48, 371–382. doi: 10.1006/ecss.1999.0427
Davey R. J., Rogers J. (1975). Palynomorph distribution in Recent offshore sediments along two traverses off South West Africa. Mar. Geol. 18, 213–225. doi: 10.1016/0025-3227(75)90097-3
De Schepper S., Beck K. M., Mangerud G. (2017). Late Neogene dinoflagellate cyst and acritarch biostratigraphy for Ocean Drilling Program Hole 642B, Norwegian Sea. Rev. Palaeobot. Palynol. 236, 12–32. doi: 10.1016/j.revpalbo.2016.08.005
De Schepper S., Head M. J. (2008). New dinoflagellate cyst and acritarch taxa from the Pliocene and Pleistocene of the eastern North Atlantic (DSDP Site 610). J. System. Palaeontol. 6, 101–117. doi: 10.1017/S1477201907002167
De Schepper S., Head M. J. (2009). Pliocene and pleistocene dinoflagellate cyst and acritarch zonation of dsdp hole 610a, eastern north Atlantic. Palynology 33, 179–218. doi: 10.2113/gspalynol.33.1.179
De Schepper S., Schreck M., Beck K. M., Matthiessen J., Fahl K., Mangerud G. (2015). Early Pliocene onset of modern Nordic Seas circulation related to ocean gateway changes. Nat. Commun. 6. doi: 10.1038/ncomms9659
de Vernal A., Giroux L. (1991). Distribution of organic-walled microfossils in recent sediments from the estuary and Gulf of St. Lawrence. Can. Special Publ. Fish. Aquat. Sci. 113, 189–199.
de Vernal A., Eynaud F., Henry M., Hillaire-Marcel C., Londeix L., Mangin S., et al. (2005). Reconstruction of sea-surface conditions at middle to high latitudes of the Northern Hemisphere during the Last Glacial Maximum (LGM) based on dinoflagellate cyst assemblages. Quater. Sci. Rev. 24, 897–924. doi: 10.1016/j.quascirev.2004.06.014
de Vernal A., Eynaud F., Henry M., Limoges A., Londeix L., Matthiessen J., et al. (2018). Distribution and (palaeo)ecological affinities of the main Spiniferites taxa in the mid-high latitudes of the Northern Hemisphere. Palynology 42, 182–202. doi: 10.1080/01916122.2018.1465730
de Vernal A., Henry M., Matthiessen J., Mudie P. J., Rochon A., Boessenkool K. P., et al. (2001). Dinoflagellate cyst assemblages as tracers of sea-surface conditions in the northern North Atlantic, Arctic and sub-Arctic seas: the new ‘n=677’ data base and its application for quantitative palaeoceanographic reconstruction. J. Quater. Sci. 16, 681–698. doi: 10.1002/jqs.659
de Vernal A., Hillaire-Marcel C. (2008). Natural variability of Greenland climate, vegetation and ice volume during the last million years. Science 320, 1622–1625. doi: 10.1126/science.1153929
de Vernal A., Hillaire-Marcel C., Rochon A., Fréchette B., Henry M., Solignac S., et al. (2013a). Dinocyst-based reconstructions of sea ice cover concentration during the Holocene in the Arctic Ocean, the northern North Atlantic Ocean and its adjacent seas. Quater. Sci. Rev. 79, 111–121. doi: 10.1016/j.quascirev.2013.07.006
de Vernal A., Hillaire-Marcel C., Turon J.-L., Matthiessen J. (2000). Reconstruction of sea-surface conditions in the northern North Atlantic during the last glacial maximum based on dinocyst assemblages. Can. J. Earth Sci. 37, 725–750. doi: 10.1139/e99-091
de Vernal A., Londeix L., Harland R., Morzadec-Kerfourn M.-T., Mudie P. J., Turon J.-L., et al. (1992). “The Quaternary organic walled dinoflagellate cyst of the North Atlantic Ocean and adjacent seas: ecostratigraphic and biostratigraphic records,” in Neogene and Quaternary dinoflagellate cysts and acritarchs. Eds. Head M. J., Wrenn J. H. (American Association of Stratigraphic Palynologists Foundation), 289–328.
de Vernal A., Mudie P. J. (1992). “Pliocene and Quaternary dinoflagellate cyst stratigraphy in Labrador Sea: paleoecological implications,” in Neogene and Quaternary dinoflagellate cysts and acritarchs. Eds. Head M. J., Wrenn J. H. (American Association of Stratigraphic Palynologists Foundation), 329–346.
de Vernal A., Radi T., Zaragosi S., Van Nieuwenhove N., Rochon A., Allan E., et al. (2020). Distribution of common modern dinoflagellate cyst taxa in surface sediments of the Northern Hemisphere in relation to environmental parameters: The new =1968 database. Mar. Micropaleontol. 159. doi: 10.1016/j.marmicro.2019.101796
de Vernal A., Rochon A., Fréchette B., Henry M., Radi T., Solignac S. (2013b). Reconstructing past sea-ice cover of the Northern hemisphere from dinocyst assemblages: status of the approach. Quater. Sci. Rev. 79, 122–134. doi: 10.1016/j.quascirev.2013.06.022
de Vernal A., Rochon A., Radi T. (2013c). “Dinoflagellates,” in The Encyclopedia of Quaternary Science, vol. 2 . Ed. Elias S. A. (Elsevier, Amsterdam), 800–815. doi: 10.1016/B978-0-444-53643-3.00283-1
de Vernal A., Turon J. L., Guiot J. (1994). Dinoflagellate cyst distribution in high-latitude marine environments and quantitative reconstruction of sea-surface salinity, temperature, and seasonality. Can. J. Earth Sci. 31, 48–62. doi: 10.1139/e94-006
Dodge J. D. (1994). Biogeography of marine armoured dinoflagellates and dinocysts in the NE Atlantic and North Sea. Rev. Palaeobot. Palynol. 84, 169–180. doi: 10.1016/0034-6667(94)90049-3
Dodge J. D., Harland R. (1991). The distribution of planktonic dinoflagellates and their cysts in the eastern and northeastern Atlantic Ocean. New Phytol. 118, 593–603. doi: 10.1111/j.1469-8137.1991.tb01000.x
Dupont L., Schneider R., Schmüser A., Jahns S. (1999). Marine-terrestrial interaction of climate changes in West Equatorial Africa of the last 190,000 years. Palaeoecol. Afr. 26, 61–84.
Dutton A., Webster J. M., Zwartz D., Lambeck K., Wohlfarth B. (2015). Tropical tales of polar ice: Evidence of Last Interglacial polar ice sheet retreat recorded by fossil reefs of the granitic Seychelles islands. Quater. Sci. Rev. 107, 182e196–196. doi: 10.1016/j.quascirev.2014.10.025
Eisma D., Van Bennekom J. (1978). The Zaire river and estuary and the Zaire outflow in the Atlantic Ocean. Netherlands J. Sea Res. 12, 255–272. doi: 10.1016/0077-7579(78)90030-3
Ellegaard M., Head M. J., Versteegh G. J. M. (2018). Linking biological and geological data on dinoflagellates using the genus Spiniferites as an example: the implications of species concepts, taxonomy and dual nomenclature. Palynology 42, 221–230. doi: 10.1080/01916122.2018.1465732
Esper O., Zonneveld K. A. F. (2002). Distribution of organic-walled dinoflagellate cysts in surface sediments of the Southern Ocean (eastern Atlantic sector) between the Subtropical Front and the Weddell Gyre. Mar. Micropaleontol. 46, 177–208. doi: 10.1016/S0377-8398(02)00041-5
Esper O., Zonneveld K. A. F. (2007). The potential of organic-walled dinoflagellate cysts for the reconstruction of past sea-surface conditions in the Southern Ocean. Mar. Micropaleontol. 65, 185–212. doi: 10.1016/j.marmicro.2007.07.002
Evitt W. R. (1963). A discussion and proposals concerning fossil dinoflagellates, Hystrichospheres, and Acritarchs, 1. Natl. Acad. Sci. Proc. 49, 158–164. doi: 10.1073/pnas.49.2.158
Eynaud F. (1999). Kystes de dinoflagellés et évolution paléoclimatique et paléohydrologique de l’Atlantique Nord au cours du dernier cycle climatique du Quaternaire. , Bordeaux. I. PhD: 291.
Eynaud F., Malaizé B., Zaragosi S., de Vernal A., Scourse J., Pujol C., et al. (2012). New constraints on European glacial freshwater releases to the North Atlantic Ocean. Geophys. Res. Lett. 39, L15601. doi: 10.1029/2012GL052100
Eynaud F., Turon J. L., Duprat J. (2004). Comparison of the Holocene and Eemian palaeoenvironments in the South Icelandic Basin: dinoflagellate cysts as proxies for the North Atlantic surface circulation. Rev. Palaeobot. Palynol. 128, 55–79. doi: 10.1016/S0034-6667(03)00112-X
Eynaud F., Turon J. L., Sanchez-Goni M. F., Gendreau S. (2000). Dinoflagellate cyst evidence of “Heinrich-like events” off Portugal during the marine isotopic stage 5. Mar. Micropaleontol. 40, 9–21. doi: 10.1016/S0377-8398(99)00045-6
Falardeau J., de Vernal A., Spielhagen R. (2018). Paleoceanography of northeastern Fram Strait since the last glacial maximum: palynological evidence of large amplitude change. Quater. Sci. Rev. 195, 133–152. doi: 10.1016/j.quascirev.2018.06.030
Falkowski P. (2012). Ocean Science: The power of plankton. Nature 483, S17–S20. doi: 10.1038/483S17a
Fensome R. A., Taylor F. J. R., Norris G., Sarjeant W. A. S., Wharton D. I., Williams G. L. (1993). “A Classification of Living and Fossil Dinoflagellates,” in American Museum of Natural History, Micropaleontology, vol. 7. , 1–351. Special publication.
Fensome R. A., Williams G. L., MacRae R. A. (2019). The Lentin and Williams index of fossil dinoflagellates. 2019 edition Vol. 50 (American Association of Stratigraphic Palynologists), 1173. Continuous Series.
García-Moreiras I., Vila Costas S., García-Gil S., Muñoz Sobrino C. (2023). Organic-walled dinoflagellate cyst assemblages in surface sediments of the Ría de Vigo (Atlantic margin of NW Iberia) in relation to environmental gradients. Mar. Micropaleontol. 180. doi: 10.1016/j.marmicro.2023.102217
Gómez F. (2012a). A checklist and classification of living dinoflagellates (Dinoflagellata, Alveoloata). CICIMAR Oceánides 27, 65–140. doi: 10.37543/oceanides.v27i1.111
Gómez F. (2012b). A quantitative review of the lifestyle, habitat and trophic diversity of dinoflagellates (Dinoflagellata, Alveolata). System. Biodivers. 10, 267–275. doi: 10.1080/14772000.2012.721021
Gu H. F., Huo K., Krock B., Bilien G., Pospelova V., Li Z., et al. (2021). Cyst-theca relationships of Spiniferites bentorii, S. hyperacanthus, S. ramosus, S. scabratus and molecular phylogenetics of Spiniferites and Tectatodinium (Gonyaulacales, Dinophyceae). Phycologia 60, 332–353. doi: 10.1080/00318884.2021.1930796
Gu H. F., Mertens K. N., Derrien A., Bilien G., Li Z., Hess P., et al. (2022). Unraveling the Gonyaulax baltica Species Complex: Cyst-theca Relationship of Impagidinium variaseptum, Spiniferites pseudodelicatus sp. nov. and S. ristingensis (Gonyaulacaceae, Dinophyceae), With Descriptions of Gonyaulax bohaiensis sp. nov, G. amoyensis sp. nov. and G. portimonensis sp. nov. J. Phycol. 58, 465–486. doi: 10.1111/jpy.13245
Gu F., Pätzold J., Behling H. (2020). Evidence of cooling in the tropical South Atlantic off southeastern Brazil during the last 50 kyr. Rev. Palaeobot. Palynol. 272, 104128. doi: 10.1016/j.revpalbo.2019.104128
Gu F., Zonneveld K. A. F., Chiessi C. M., Arz H. W., Pätzold J., Behling H. (2017). Long-term vegetation, climate and ocean dynamics inferred from a 73,500 years old marine sediment core (GeoB2107–3) off southern Brazil. Quater. Sci. Rev. 172, 55–71. doi: 10.1016/j.quascirev.2017.06.028
Gurdebeke P. R., Mertens K. N., Bogus K., Marret F., Chomerat N., Vrielinck H., et al. (2018). Taxonomic re-investigation and geochemical characterization of Reid’s, (1974) species of spiniferites from holotype and topotype material. Palynology 42, 93–110. doi: 10.1080/01916122.2018.1465735
Gurdebeke P. R., Mertens K. N., Pospelova V., Matsuoka K., Li Z., Gribble K. E., et al. (2020). Taxonomic revision, phylogeny, and cyst wall composition of the dinoflagellate cyst genus Votadinium Reid (Dinophyceae, Peridiniales, Protoperidiniaceae). Palynology 44, 310–335. doi: 10.1080/01916122.2019.1580627
Hamel D., de Vernal A., Gosselin M., Hillaire-Marcel C. (2002). Organic-walled microfossils and geochemical tracers: sedimentary indicators of productivity changes in the North Water and northern Baffin Bay during the last centuries. Deep-Sea Res. Part Ii-Topical Stud. Oceanogr. 49, 5277–5295. doi: 10.1016/S0967-0645(02)00190-X
Haq B. U., Hardenbol J., Vail P. R. (1987). Chronology of fluctuating sea levels since the Triassic. Science 235, 1156–1167. doi: 10.1126/science.235.4793.1156
Hardy W., Marret F., Penaud A., Le Mezo P., Droz L., Marsset T., et al. (2018). Quantification of last glacial-Holocene net primary productivity and upwelling activity in the equatorial eastern Atlantic with a revised modern dinocyst database. Palaeogeogr. Palaeoclimatol. Palaeoecol. 505, 410–427. doi: 10.1016/j.palaeo.2018.06.025
Hardy W., Penaud A., Marret F., Bayon G., Marsset T., Droz L. (2016). Dinocyst assemblage constraints on oceanographic and atmospheric processes in the eastern equatorial Atlantic over the last 44 kyr. Biogeosciences 13, 4823–4841. doi: 10.5194/bg-13-4823-2016
Harland R. (1983). Distribution maps of recent dinoflagellate cysts in bottom sediments from the North-Atlantic Ocean and adjacent seas. Palaeontology 26, 321–387.
Harland R., Nordberg K., Filipsson H. L. (2006). Dinoflagellate cysts and hydrographical change in Gullmar Fjord, west coast of Sweden. Sci. Total Environ. 355, 204. doi: 10.1016/j.scitotenv.2005.02.030
Hartman J. D., Bijl P. K., Sangiorgi F. (2018). A review of the ecological affinities of marine organic microfossils from a Holocene record offshore of Adelie Land (East Antarctica). J. Micropalaeontol. 37, 445–497. doi: 10.5194/jm-37-445-2018
Hartman J. D., Sangiorgi F., Bijl P. K., Versteegh G. J. M. (2019). Nucicla umbiliphora gen. et sp. nov.: a Quaternary peridinioid dinoflagellate cyst from the Antarctic margin. Palynology. 43 (1), 94–103
Head M. J. (1996). “Modern dinoflagellate cysts and their biological affinities,” in Palynology: principles and applications, vol. 3 . Eds. Jansonius J., McGregor D. C. (American Association of Stratigraphic Palynologists Foundation, Salt Lake City), 1197–1248.
Head M. J., Lewis J., de Vernal A. (2006). The cyst of the calcareous dinoflagellate Scrippsiella trifida: Resolving the fossil record of its organic wall with that of Alexandrium tamarense. J. Paleontol. 80, 1–18. doi: 10.1666/0022-3360(2006)080[0001:TCOTCD]2.0.CO;2
Head M. J., Mertens K. N., Fensome R. A. (2024). Dual nomenclature in organic-walled dinoflagellate cysts I: concepts, methods and applications. Palynology, 1–99. doi: 10.1080/01916122.2023.2290200
Head M. J., Wrenn J. H. (1992). Neogene and Quaternary dinoflagellate cysts and acritarchs (Dallas: American Association of Stratigraphic Palynologists Foundation). 438pp.
Heikkilä M., Pospelova V., Forest A., Stern G. A., Fortier L., MacDonald R. W. (2016). Dinoflagellate cyst production over an annual cycle in seasonally ice-covered Hudson Bay. Mar. Micropaleontol. 125, 1–24. doi: 10.1016/j.marmicro.2016.02.005
Hinners J., Hense I., Kremp A. (2019). Modelling phytoplankton adaptation to global warming based on resurrection experiments. Ecol. Model. 400, 27–33. doi: 10.1016/j.ecolmodel.2019.03.006
Hohmann S., Kucera M., De Vernal A. (2023). Disentangling environmental drivers of subarctic dinocyst assemblage compositional change during the Holocene. Climate Past 19, 2027–2051. doi: 10.5194/cp-19-2027-2023
Kaufman D., McKay N., Routson C., Erb M., Davis B., Heiri O., et al. (2020). A global database of Holocene paleotemperature records. Sci. Data 7. doi: 10.1038/s41597-020-0445-3
Kim S. Y., Park T., Marret F., Potvin É., Cho K. H., Jung J., et al. (2023). Strong regionalism in dinoflagellate cysts in recent sediments from the Chukchi-East Siberian Seas, Arctic Ocean. Prog. Oceanogr. 211. doi: 10.1016/j.pocean.2023.102970
Lambeck K., Rouby H., Purcell A., Sun Y., Sambridge M. (2014). Sea level and global ice volumes from the Last Glacial Maximum to the Holocene. Proc. Natl. Acad. Sci. United States America 111, 15296–15303. doi: 10.1073/pnas.1411762111
Lambert C., Penaud A., Poirier C., Goubert E. (2022). Distribution of modern dinocysts in surface sediments of southern Brittany (NW France) in relation to environmental parameters: Implications for paleoreconstructions. Rev. Palaeobot. Palynol. 297. doi: 10.1016/j.revpalbo.2021.104578
Li Z., Pospelova V., Kawamura H., Luo C. X., Mertens K. N., Hernandez-Almeida I., et al. (2020). Dinoflagellate cyst distribution in surface sediments from the South China Sea in relation to hydrographic conditions and primary productivity. Mar. Micropaleontol. 159. doi: 10.1016/j.marmicro.2019.101815
Li Z., Pospelova V., Mertens K. N., Liu L., Wu Y., Li C., et al. (2023). Evaluation of organic-walled dinoflagellate cyst distributions in coastal surface sediments of the China Seas in relation with hydrographic conditions for paleoceanographic reconstruction. Quater. Int. 661, 60–75. doi: 10.1016/j.quaint.2023.03.007
Limoges A., Londeix L., Mertens K. N., Rochon A., Pospelova V., Cuellar T., et al. (2018). Identification key for Pliocene and Quaternary Spiniferites taxa bearing intergonal processes based on observations from estuarine and coastal environments. Palynology 42, 72–88. doi: 10.1080/01916122.2018.1465733
Limoges A., Van Nieuwenhove N., Head M. J., Mertens K. N., Pospelova V., Rochon A. (2020). A review of rare and less well known extant marine organic-walled dinoflagellate cyst taxa of the orders Gonyaulacales and Suessiales from the Northern Hemisphere. Mar. Micropaleontol. 159. doi: 10.1016/j.marmicro.2019.101801
Lisiecki L. E., Raymo M. E. (2005). A Pliocene-Pleistocene stack of 57 globally distributed benthic δ18O records (vol 20, art no PA1003, 2005). Paleoceanography 20. doi: 10.1029/2004PA001071
MacRae R. A., Fensome R. A., Williams G. L. (1996). Fossil dinoflagellate diversity, originations, and extinctions and their significance. Can. J. Bot. 74, 1687–1694. doi: 10.1139/b96-205
Marret F., Bradley L., de Vernal A., Hardy W., Kim S. Y., Mudie P., et al. (2020). From bi-polar to regional distribution of modern dinoflagellate cysts, an overview of their biogeography. Mar. Micropaleontol. 159. doi: 10.1016/j.marmicro.2019.101753
Marret F., de Vernal A. (1997). Dinoflagellate cyst distribution in surface sediments of the southern Indian Ocean. Mar. Micropaleontol. 29, 367–392. doi: 10.1016/S0377-8398(96)00049-7
Marret F., Kim S. Y., Scourse J. (2013). A 30,000 yr record of land-ocean interaction in the eastern Gulf of Guinea. Quater. Res. 80, 1–8. doi: 10.1016/j.yqres.2013.04.003
Marret F., Mudie P., Aksu A., Hiscott R. N. (2009). A Holocene dinocyst record of a two-step transformation of the Neoeuxinian brackish water lake into the Black Sea. Quater. Int. 197, 72–86. doi: 10.1016/j.quaint.2007.01.010
Marret F., Zonneveld K. A. F. (2003). Atlas of modern organic-walled dinoflagellate cyst distribution. Rev. Palaeobot. Palynol. 125, 1–200. doi: 10.1016/S0034-6667(02)00229-4
Matthiessen J., Knies J., Nowaczyk N. R., Stein R. (2001). Late Quaternary dinoflagellate cyst stratigraphy at the Eurasian continental margin, Arctic Ocean: indications for Atlantic water inflow in the past 150,000 years. Global Planet. Change 31, 65–86. doi: 10.1016/S0921-8181(01)00113-8
Matthiessen J., Schreck M., De Schepper S., Zorzi C., de Vernal A. (2018). Quaternary dinoflagellate cysts in the Arctic Ocean: Potential and limitations for stratigraphy and paleoenvironmental reconstructions. Quater. Sci. Rev. 192, 1–26. doi: 10.1016/j.quascirev.2017.12.020
McMinn A. (1995). Why are there no post-Paleogene dinoflagellate cysts in the Southern Ocean? Micropaleontology 41, 383–386. doi: 10.2307/1485813
Mertens K. N., Gu H., Gurdebeke P. R., Takano Y., Clarke D., Aydin H., et al. (2020). A review of rare, poorly known, and morphologically problematic extant marine organic-walled dinoflagellate cyst taxa of the orders Gymnodiniales and Peridiniales from the Northern Hemisphere. Mar. Micropaleontol. 159. doi: 10.1016/j.marmicro.2019.101773
Meyvisch P., Gurdebeke P. R., Vrielinck H., Neil Mertens K., Versteegh G., Louwye S. (2022). Attenuated total reflection (ATR) micro-fourier transform infrared (Micro-FT-IR) spectroscopy to enhance repeatability and reproducibility of spectra derived from single specimen organic-walled dinoflagellate cysts. Appl. Spectrosc. 76, 235–254. doi: 10.1177/00037028211041172
Meyvisch P., Mertens K. N., Gurdebeke P. R., Sandt C., Pospelova V., Vrielinck H., et al. (2023). Does dinocyst wall composition really reflect trophic affinity? New evidence from ATR micro-FTIR spectroscopy measurements. J. Phycol. doi: 10.1111/jpy.13382
Montresor M., Lovejoy C., Orsini L., Procaccini G., Roy S. (2003). Bipolar distribution of the cyst-forming dinoflagellate Polarella glacialis. Polar Bio. 26, 186–194. doi: 10.1007/s00300-002-0473-9
Mudie P. J. (1992). “Circum-arctic Quaternary and Neogene marine palynofloras: paleoecology and statistical analysis,” in Neogene and Quaternary Dinoflagellate Cysts and Acritarchs. Eds. Head M. J., Wrenn J. H. (American Association of Stratigraphic Palynologists Foundation, Dallas).
Mudie P. J., Marret F., Mertens K. N., Shumilovskikh L., Leroy S. A. G. (2017). Atlas of modern dinoflagellate cyst distributions in the Black Sea Corridor: from Aegean to Aral Seas, including Marmara, Black, Azov and Caspian Seas. Mar. Micropaleontol. 134, 1–152. doi: 10.1016/j.marmicro.2017.05.004
Mudie P. J., Rochon A., Levac E. (2002). Palynological records of red tide-producing species in Canada: Past trends and implications for the future. Palaeogeogr. Palaeoclimatol. Palaeoecol. 180, 159. doi: 10.1016/S0031-0182(01)00427-8
Mudie P., Rochon A., Richards K., Ferguson S., Warny S. (2018). Spiniferites cruciformis, Pterocysta cruciformis and Galeacysta etrusca: morphology and palaeoecology. Palynology 42, 135–161. doi: 10.1080/01916122.2018.1465737
Mudie P. J., Yanko-Hombach V. V., Mudryk I. (2021). Palynomorphs in surface sediments of the North-Western Black Sea as indicators of environmental conditions. Quater. Int. 590, 122–145. doi: 10.1016/j.quaint.2020.05.014
Nooteboom P. D., Delandmeter P., van Sebille E., Bijl P. K., Dijkstra H. A., von der Heydt A. S. (2020). Resolution dependency of sinking Lagrangian particles in ocean general circulation models. PloS One 15. doi: 10.1371/journal.pone.0238650
Nordii E. (1951). Resting spores in Gonyaulax polyedra Stein. Nytt Magasinf Naturvitenskapene 88, 207–212.
Obrezkova M. S., Pospelova V., Kolesnik A. N. (2023). Diatom and dinoflagellate cyst distribution in surface sediments of the Chukchi Sea in relation to the upper water masses. Mar. Micropaleontol. 178. doi: 10.1016/j.marmicro.2022.102184
Penaud A., Eynaud F., Turon J. L., Zaragosi S., Marret F., Bourillet J. F. (2008). Interglacial variability (MIS 5 and MIS 7) and dinoflagellate cyst assemblages in the Bay of Biscay (North Atlantic). Mar. Micropaleontol. 68, 136–155. doi: 10.1016/j.marmicro.2008.01.007
Penaud A., Hardy W., Lambert C., Marret F., Masure E., Servais T., et al. (2018). Dinoflagellate fossils: Geological and biological applications. Rev. Micropaléontol. 61, 235–254. doi: 10.1016/j.revmic.2018.09.003
Petrick B. F., McClymont E. L., Marret F., van der Meer M. T. J. (2015). Changing surface water conditions for the last 500 ka in the Southeast Atlantic: Implications for variable influences of Agulhas leakage and Benguela upwelling. Paleoceanography 30, 1153–1167. doi: 10.1002/2015PA002787
Peyron O., de Vernal A. (2001). Application of Artificial Neural Network (ANN) to high latitude dinocyst assemblages for the reconstruction of past sea-surface conditions in Arctic and sub-arctic seas. J. Quater. Sci. 16, 699–711. doi: 10.1002/jqs.651
Polyak L., Best K. M., Crawford K. A., Council E. A., St-Onge G. (2013). Quaternary history of sea ice in the western Arctic Ocean based on foraminifera. Quater. Sci. Rev. 79, 145–156. doi: 10.1016/j.quascirev.2012.12.018
Price A. M., Baustian M. M., Turner R. E., Rabalais N. N., Chmura G. L. (2018). Dinoflagellate cysts track eutrophication in the northern gulf of Mexico. Estuaries Coasts 41, 1322–1336. doi: 10.1007/s12237-017-0351-x
Radi T., de Vernal A. (2008). Dinocysts as proxy of primary productivity in mid-high latitudes of the Northern Hemisphere. Mar. Micropaleontol. 68, 84–114. doi: 10.1016/j.marmicro.2008.01.012
Ramírez-Valencia V., Paez-Reyes M., Salgado J., Sangiorgi F., Zúñiga-González A. C., Amézquita A., et al. (2021). Distribution of organic-walled dinoflagellate cysts in surface sediments of the southern Caribbean and the eastern tropical Pacific and its environmental implications. Mar. Micropaleontol. 167, 102000. doi: 10.1016/j.marmicro.2021.102000
Riding J. B., Soyer-Gobillard M.-O., Medlin L. K. (2022). A review of the dinoflagellates and their evolution from fossils to modern. J. Mar. Sci. Eng. 11, 1. doi: 10.3390/jmse11010001
Rochon A., de Vernal A. (1994). Palynomorph distribution in Recent sediments from the Labrador Sea. Can. J. Earth Sci. 31, 115–127. doi: 10.1139/e94-010
Rochon A., de Vernal A., Turon J.-L., Matthiessen J., Head M. J. (1999). Distribution of dinoflagellate cyst assemblages in surface sediments from the North Atlantic Ocean and adjacent basins and quantitative reconstructions of sea-surface parameters (Dallas: American Association of Stratigraphic Palynologists).
Sala-Pérez M., Lattuada M., Flecker R., Anesio A., Leroy S. A. G. (2020). Dinoflagellate cyst assemblages as indicators of environmental conditions and shipping activities in coastal areas of the Black and Caspian Seas. Region. Stud. Mar. Sci. 39. doi: 10.1016/j.rsma.2020.101472
Salgado P., Pizarro G., Frangopulos M., Pinto-Torres M., Toro C., Torres R., et al. (2023). Distribution of dinoflagellate cyst assemblages in surface sediments of Magellan fjords and channels (Patagonia, Chile) with a focus on harmful species: An overview on environmental scenario. Prog. Oceanogr. 213. doi: 10.1016/j.pocean.2023.103000
Sigman D. M., Hain M. P. (2012). The biological productivity of the ocean. Nat. Educ. Knowledge 3, 1–16.
Sluijs A., Pross J., Brinkhuis H. (2005). From greenhouse to icehouse; organic-walled dinoflagellate cysts as paleoenvironmental indicators in the Paleogene. Earth-Sci. Rev. 68, 281–315. doi: 10.1016/j.earscirev.2004.06.001
Telesiński M. M., Pospelova V., Mertens K. N., Kucharska M., Zajączkowski M. (2023). Dinoflagellate cysts and benthic foraminifera from surface sediments of Svalbard fjords and shelves as paleoenvironmental indicators. Oceanologia 65, 571–594. doi: 10.1016/j.oceano.2023.06.007
Ter Braak C. J. F., Smilauer P. (1998). Canoco Regerence Manuel and User’s Guide to Canoco for Windows, Software for Canonical Community Ordinatio, (version 5.1) (Wageningen: Center of Biometry). 351 pp.
Thöle L. M., Nooteboom P. D., Hou S. N., Wang R. J., Nie S. Y., Michel E., et al. (2023). An expanded database of Southern Hemisphere surface sediment dinoflagellatecyst assemblages and their oceanographic affinities. J. Micropalaeontol. 42, 35–56. doi: 10.5194/jm-42-35-2023
Traverse A. (1974). Paleopalynology - 1947–1972. Ann. Missouri Bot. Garden 61, 203–236. doi: 10.2307/2395191
Turland N. J., Wiersema J. H., Barrie F. R., Greuter W., Hawksworth D. L., Herendeen P. S., et al. (2018). International Code of Nomenclature for algae, fungi, and plants (Shenzhen Code) adopted by the Nineteenth International Botanical Congress Shenzhen, China, July 2017. Int. Code Nomenclature Algae Fungi Plants (Shenzhen Code) 159, 3–3. doi: 10.12705/Code.2018
Turner J. T. (2015). Zooplankton fecal pellets, marine snow, phytodetritus and the ocean’s biological pump. Prog. Oceanogr. 130, 205–248. doi: 10.1016/j.pocean.2014.08.005
Turon J. L. (1984). Le palynoplancton dans l’environnement actuel de l’Atlantique Nord-Oriental. Evol. clim. hydrol. depuis le dernier maxrimum glaciaire.
Van Nieuwenhove N., Bauch H. A. (2008). Last interglacial (MIS 5e) surface water conditions at the Vøring Plateau (Norwegian Sea), based on dinoflagellate cysts. Polar Res. 27, 175–186. doi: 10.3402/polar.v27i2.6175
Van Nieuwenhove N., Bauch H. A., Andruleit H. (2013). Multiproxy fossil comparison reveals contrasting surface ocean conditions in the western Iceland Sea for the last two interglacials. Palaeogeogr. Palaeoclimatol. Palaeoecol. 370, 247–259. doi: 10.1016/j.palaeo.2012.12.018
Van Nieuwenhove N., Bauch H. A., Eynaud F., Kandiano E., Cortijo E., Turon J.-L. (2011). Evidence for delayed poleward expansion of North Atlantic surface waters during the last interglacial (MIS 5e). Quater. Sci. Rev. 30, 934–946. doi: 10.1016/j.quascirev.2011.01.013
Van Nieuwenhove N., Bauch H. A., Matthiessen J. (2008). Last interglacial surface water conditions in the eastern Nordic Seas inferred from dinocyst and foraminiferal assemblages. Mar. Micropaleontol. 66, 247–263. doi: 10.1016/j.marmicro.2007.10.004
Van Nieuwenhove N., Head M. J., Limoges A., Pospelova V., Mertens K. N., Matthiessen J., et al. (2020a). An overview and brief description of common marine organic-walled dinoflagellate cyst taxa occurring in surface sediments of the Northern Hemisphere. Mar. Micropaleontol. 159. doi: 10.1016/j.marmicro.2019.101814
Van Nieuwenhove N., Pospelova V., de Vernal A., Rochon A. (2020b). Preface: A historical perspective on the development of the Northern Hemisphere modern dinoflagellate cyst database. Mar. Micropaleontol. 159. doi: 10.1016/j.marmicro.2020.101824
Versteegh G. J. M., Zonneveld K. A. F. (2022). Micro-Fourier transform infrared spectroscopy of degradation-resistant organic microfossils; Influence of preservation environment and phylogeny. Front. Mar. Sci. 9. doi: 10.3389/fmars.2022.1040543
Wall D. (1965). Modern hystrichospheres and dinoflagellate cysts from the Woods Hole region. Grana Palynol. 6, 297–314. doi: 10.1080/00173136509429150
Wall D., Dale B. (1966). “Living fossils” in western Atlantic plankton. Nature 211, 1025–1026. doi: 10.1038/2111025a0
Wall D., Dale B. (1968). Modern dinoflagellate cysts and the evolution of the Peridiniales. Micropaleontology 14, 265–304. doi: 10.2307/1484690
Wall D., Dale B., Lohmann G. P., Smith W. K. (1977). The environmental and climatic distribution of dinoflagellate cysts in the North and South Atlantic Oceans and adjacent seas. Mar. Micropaleontol. 2, 121–200. doi: 10.1016/0377-8398(77)90008-1
Wang N., Mertens K. N., Krock B., Luo Z. H., Derrien A., Pospelova V., et al. (2019). Cryptic speciation in Protoceratium reticulatum (Dinophyceae): Evidence from morphological, molecular and ecophysiological data. Harmful Algae 88. doi: 10.1016/j.hal.2019.05.003
Williams D. B. (1971). “14. The occurence of dinoflagellates in marine sediments. The Micropalaeontology of Oceans,” in Proceedings of the Symposium Held in Cambridge from 10 to 17 September 1967 Under the Title’Micropalaeontology of Marine Bottom Sediments (Cambridge University Press).
Williams G. L., Bujak J. P. (1977). Distribution patterns of some North-Atlantic cenozoic dinoflagellate cysts. Mar. Micropaleontol. 2, 223–233. doi: 10.1016/0377-8398(77)90012-3
Wrenn J. H., Kokinos J. P. (1986). “Preliminary comments on Miocene through Pleistocene dinoflagellate cysts from De Soto Canyon, Gulf of Mexico,” in Papers from the First Symposium on Neogene Dinoflagellate Cyst Biostratigraphy, vol. 17 . Eds. Wrenn J. H., Duffield S. L., Stein J. A. (American Association of Stratigraphic Palynologists Contributions Series), 169–225.
Zonneveld K. A. F., Gray D. D., Kuhn G., Versteegh G. J. M. (2019). Postdepositional aerobic and anaerobic particulate organic matter degradation succession reflected by dinoflagellate cysts: The Madeira Abyssal Plain revisited. Mar. Geol. 408, 87–109. doi: 10.1016/j.margeo.2018.11.010
Zonneveld K. A. F., Marret F., Versteegh G. J. M., Bogus K., Bonnet S., Bouimetarhan I., et al. (2013). Atlas of modern dinoflagellate cyst distribution based on 2405 data points. Rev. Palaeobot. Palynol. 191, 1–197. doi: 10.1016/j.revpalbo.2012.08.003
Zonneveld K. A. F., Meier K. J. S., Esper O., D. S., Wendler I., Willems H. (2005). The (palaeo-) environmental significance of modern calcareous dinoflagellate cysts: a review. Paläontol. Z. 79, 61–77. doi: 10.1007/BF03021754
Zonneveld K. A. F., Pospelova V. (2015). A determination key for modern dinoflagellate cysts. Palynology 39, 387–409. doi: 10.1080/01916122.2014.990115
Zonneveld K. A. F., Versteegh G., Kodrans-Nsiah M. (2008). Preservation and organic chemistry of Late Cenozoic organic-walled dinoflagellate cysts: A review. Mar. Micropaleontol. 68, 179–197. doi: 10.1016/j.marmicro.2008.01.015
Keywords: sea-surface conditions, palaeoproductivity, biostratigraphy, palaeoecology, palaeoceanography
Citation: Marret F and de Vernal A (2024) Dinoflagellate cysts as proxies of environmental, ocean and climate changes in the Atlantic realm during the quaternary. Front. Ecol. Evol. 12:1378931. doi: 10.3389/fevo.2024.1378931
Received: 30 January 2024; Accepted: 14 May 2024;
Published: 04 June 2024.
Edited by:
Thiago Pereira Santos, Fluminense Federal University, BrazilReviewed by:
Debra Willard, United States Department of the Interior, United StatesAnil Gupta, Indian Institute of Technology Kharagpur, India
Copyright © 2024 Marret and de Vernal. This is an open-access article distributed under the terms of the Creative Commons Attribution License (CC BY). The use, distribution or reproduction in other forums is permitted, provided the original author(s) and the copyright owner(s) are credited and that the original publication in this journal is cited, in accordance with accepted academic practice. No use, distribution or reproduction is permitted which does not comply with these terms.
*Correspondence: Fabienne Marret, f.marret@liverpool.ac.uk