- 1Department of Environmental Science and Policy, Università degli Studi di Milano, Milan, Italy
- 2Department of Biosciences, Università degli Studi di Milano, Milan, Italy
Serotonin (5 hydroxytryptamine, 5-HT) is a biogenic amine of ancient origin that is widespread among animals. It plays multiple roles during development and in adults as neurotransmitter at synaptic level and neuro hormone controlling complex behaviors in both vertebrates and invertebrates. Tunicates occupy a key phylogenetic position to understand the evolution of serotonin functions since they are the sister group of vertebrates. The presence of serotonin in tunicates was first reported in adults of the ascidian Ciona robusta (formerly Ciona intestinalis) in the 1946. Since then, serotonin systems have been in many tunicate species and its functions during embryogenesis and metamorphosis explored. We reviewed the current knowledge about serotonin in these animals first by comparing its presence and localization in larvae and adults of different species. Then, we focused on the model organism Ciona for which data regarding sequences and expression patterns of genes involved in serotonin synthesis and function have been reported. Overall, we provided a comprehensive overview of serotonergic machinery in tunicates and gave hints for future studies in this field.
Introduction
Serotonin [5-hydroxytryptamine (5-HT)] is a biogenic amine of ancient origin that dates back at least 600 million years. It can be found in various unicellular eukaryotes and nearly all metazoans. Its origin clearly predates the emergence of a centralized nervous system, playing a key role in chemotaxis and chemo-signaling in unicellular organisms and functioning as intracellular regulator in multicellular animals (Greczek-Stachura, 2002; Azmitia, 2007; Nichols and Nichols, 2008; Berger et al., 2009; Lv and Liu, 2017). Very early in evolution, 5-HT acquired the additional function as a morphogenetic factor, controlling the proliferation and differentiation of various cell types, including those of the enteric nervous system (Fiorica-Howells et al., 2000; Azmitia, 2001; Najjar et al., 2023). Its morphogenetic role is also prominent during animal development. In sea urchin and Drosophila, 5-HT is involved in early embryogenesis, acting as modulator of gastrulation (Colas et al., 1995; Buznikov et al., 2005). In mammalian and avian development, 5-HT regulates cardiac morphogenesis and neural crest cell migration (Yavarone et al., 1993a, 1993b; Moiseiwitsch and Lauder, 1995; Choi et al., 1997; Neumann et al., 2023).
Later, while maintaining this variety of developmental roles, 5-HT also began to function as neuromodulator. In many invertebrates, 5-HT neurons are primary sensory neurons modulating food intake, defense and locomotor behavior. 5-HT-synthetizing cells are involved in defense mechanisms in both cnidarians and insects (Horen, 1972; Weiger, 1997); in leech and in sea urchin they regulate swimming activity (Kristan and Nusbaum, 1982; Yaguchi and Katow, 2003) while in lobsters they control complex social behavior (Kravitz, 2000). In vertebrates, 5-HT role as modulator of sleep, mood, appetite, and temperature is also well-known (Azmitia, 2007).
5-HT neurons and their organization are completely different among animals reaching the higher complexity in vertebrates. Heterogeneity of 5-HT neurons concerns both their number, varying from hundreds in mollusks to several thousands in mammals, and their localization. In invertebrates, serotonergic system is often associated with sensory organs, and different type of neurotransmission have been described (Bacqué-Cazenave et al., 2020). In humans, 5-HT producing cells are found in brainstem, in the enteric nervous system and in mast cells and modulate the activity of a variety of other neurons or even of the entire neural circuits (Azmitia, 2007).
Tunicates have been recognized as the sister group of vertebrates (Delsuc et al., 2006). Along with cephalochordates and vertebrates, they form the group of chordates, whose common body plan is clearly demonstrated by their larvae. Tunicate larva consists of a trunk that houses the rudiments of the digestive tract and the anterior part of the central nervous system (CNS), and a locomotory tail flanked by muscle cells. The tail contains the neural tube running dorsally to the chord, which is an apomorphy of the chordate clade. The swimming larva undergoes a deep metamorphosis that transforms it, in most cases, into a sessile adult. Additionally, several tunicates exhibit a colonial lifestyle, making them the only chordates to have evolved this particular way of life. As a result, these animals possess a combination of both conserved and derived traits, with the formers mainly displayed by the larva, the latter mainly found in the adult form (Lemaire, 2011).
Traditionally tunicates include three classes, the pelagic appendicularians and thaliaceans and the sessile ascidians, with the last encompassing three orders: Aplousobranchia, Phlebobranchia and Stolidobranchia, identified mainly by gill features. Recent phylogenetic analysis has revisited the phylogeny of tunicates, revealing that the traditionally recognized ascidian group is indeed paraphyletic, with the thaliaceans being the sister group of Aplousobranchia plus Phlebobranchia (Delsuc et al., 2018; Kocot et al., 2018). For the aims of this review, we used the term “ascidian” to indicate a sessile species and maintained the traditional subdivisions for comparative purposes, being well aware that thaliaceans are derived and nested in the ascidian group.
Considering tunicate peculiar features and their key evolutionary position, 5-HT role and localization have been explored in different species, applying both morphological and molecular approaches. The evolution of this ancient and widespread monoamine represents indeed an intriguing topic with still many unsolved questions. Defining its role in tunicates may thus contribute to unveil which aspects are conserved and which are unique to this group.
In this review, we thoroughly examined the existing literature of 5-HT in tunicates to gather information and provide a comprehensive overview of its localization in both larvae and adults. We focused on Ciona robusta and Ciona intestinalis (from here on Ciona) (Brunetti et al., 2015; Pennati et al., 2015), well-established ascidian model systems in many research fields (Dehal et al., 2002; Satoh, 2013; Mercurio et al., 2019a, 2021). Our aim was to identify both novel and conserved features in comparison to phylogenetically related organisms. Furthermore, we documented the current knowledge regarding the genes involved in the synthesis and functioning of 5-HT, as well as the functional characterization of the serotonergic system.
Localization in ascidian larvae
5-HT localization by immunohistochemistry proved to be quite challenging in ascidian larvae, likely due to the low levels of this molecule. In fact, in Ciona, even if localization in larvae by means of anti 5-HT antibodies failed, 5-HT was detected by fluorometry after HPLC from early embryonic stages. In the egg, the reported concentration was 3.5 ± 2.9 fmoles/individual, and fell to 1.8 ± 1.5 fmoles/individual in the larva and the authors suggested a maternal origin of 5-HT throughout embryogenesis even if they did not investigate any enzyme expressions or activities (Razy-Krajka et al., 2012). 5-HT concentration increased again in the post-metamorphic stages and these findings align with De Bernardi’s study, which reported 5-HT immunolocalization in the Ciona visceral ganglion only in individuals at the onset of metamorphosis (De Bernardi et al., 2006). To partially overcome this obstacle and clearly visualize at least the 5-HT-accumulating cells, larvae were treated with exogenous 5-HT before immunostaining experiments. In this condition, 5-HT positive cells were easily observed in the dopaminergic neurons of the ventral sensory vesicle, further suggesting that results were affected by the low sensitivity of the technique (Razy-Krajka et al., 2012).
Staining experiments with antibodies against 5-HT in the larvae of Eudistoma olivaceum (Clavelinidae), Aplidium stellatum (Polyclinidae), and Didemnum candidum (Didemnidae) yielded similar negative results. Conversely, immunohistochemistry analysis reported the presence of a 5-HT-like signal in 20 neural cells of the Herdmania momus (Stolidobranchia) and several of these neurons showed fibers projecting posteriorly forming a distinct network. 5-HT immunostaining signal close to the sensory vesicle was reported also in other Stolidobranchia larvae, such as Microcosmus exasperatus (Pyuridae), Styela plicata (Styelidae), and Molgula occidentalis (Molgulidae), and in the larva of the Phlebobranchia Ascidia interrupta (Stach, 2005). In Styela plicata larva, De Bernardi and colleagues detected 5-HT in some cells of the sensory vesicle surrounding the single sensory organ, the photolith, and in the adhesive papillae. Similarly, in the larva of Microcosmus vulgaris (Pyuridae) 5-HT was found in a few cells close to the gravity sensing otolith (De Bernardi et al., 2006). In Phallusia mammillata swimming larvae, 5-HT was detected in the sensory vesicle, adhesive papillae, epidermal trunk neurons, and epidermal tail neurons (Pennati et al., 2001) while in the larvae of Botrylloides leachi (Aplousobranchia), 5-HT signal was observed only in peripheral neurons of the papillae (Pennati et al., 2007b).
The larvae of Aplousobranchia species often exhibit partially differentiated adult tracts and organs, a phenomenon known as adultation (Jeffery and Swalla, 1992). In the larvae of these species, 5-HT could be detected both in larval typical organs and in differentiating adult ones. In the Clavelina oblunga (Aplouosobranchia) 5-HT immunoreactivity was found in cells close to the statocyte complex, a larval organ, and in the branchial basket, an adult organ (Stach, 2005). Similar findings were observed in C. lepadiformis and C. phlegraea where 5-HT was immunolocalized in same adult territories as in C. oblunga and in few marginal neurons of the adhesive papillae (Pennati et al., 2009).
The larva of Diplosoma listenarium revealed serotonin in several neurons of the complex papillae and in the posterior region of the cerebral vesicle, while differentiating zooids display adult-like localization (De Bernardi et al., 2006). Although 5-HT levels can vary among species, its presence remains widespread in tunicate nervous system and often associated with larval sensory activity. Moreover, the presence of serotonergic neurons in the adhesive papillae is a recurrent feature in most of the analyzed ascidian species. It has been suggested that 5-HT release by these neurons may play a role in the signaling cascade that triggers metamorphosis (Pennati and Rothbächer, 2015).
Localization in ascidian adults
Erspamer (1946) was the first to report the presence of 5-HT in adult tunicates. Since then, 5-HT has been found in adults of a variety of solitary ascidian including Ciona intestinalis, Ascidiella aspersa, Ascidia mentula, Styela plicata, and Phallusia mammillata (Erspamer, 1946; Welsh and Loveland, 1968; Pestarino, 1982; Sakharov and Salimova, 1982; Georges, 1985). More recently, immunolocalization experiments confirmed these results. In adults of Ciona, 5-HT containing cells were reported in the pharyngeal bands, in the esophagus and in the stomach; some of them were also aligned in a band extending from the peripharyngeal band of the branchial basket to the endostyle (Braun and Stach, 2016). In juvenile of P. mammillata, 5-HT-positive cells were found in the peripharyngeal band, in the gut and two bilaterally symmetric rows of cells between bands 7 and 8 of the endostyle (Pennati et al., 2001). This area also contains cells capable of fixing iodine (Nilsson et al., 1988) and calcitonin-like cells (Thorndyke and Probert, 1979). A similar localization pattern was also observed in adults of other ascidian species: Clavelina lepadiformis Diplosoma listerianum, Ascidiella scabra, Perophora japonica (Braun and Stach, 2016), Corella parallelogramma (Nilsson et al., 1988; Braun and Stach, 2016), Botryllus schlosseri (Tiozzo et al., 2009; Braun and Stach, 2016). Moreover, in the model organism B. schlosseri, 5-HT–positive cells were found in the buds during blastogenic development starting from early stages of bud differentiation, suggesting that 5-HT may play a role in controlling the morphogenetic processes (Tiozzo et al., 2009) (Figure 1).
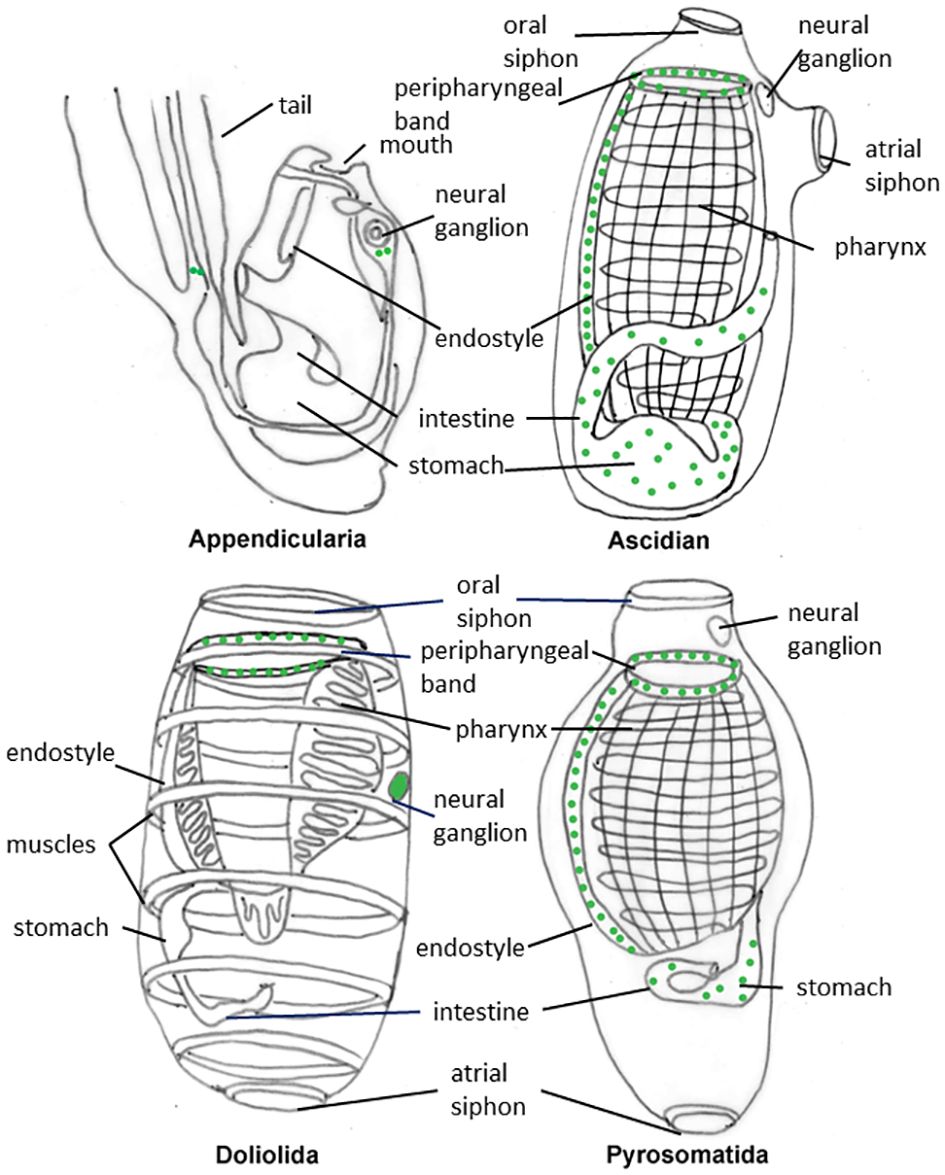
Figure 1 Schematic representation of the distribution pattern of serotonin positive cells (green dots) in adults of representative tunicates.
It has been proposed that 5-HT occurrence in the pharyngeal bands of the branchial basket of these filter feeder animals may serve to control mucus secretion and ciliary beating (Braun and Stach, 2016). In fact, the branchial basket is bordered by a ciliated epithelium and covered by mucus secreted by the endostyle. A role of 5-HT in ciliary beating control was also reported in several invertebrates as well as in numerous larval forms (Hay-Schmidt, 2000). As an alternative hypothesis, serotonin in peripharingeal band may serves in mechanosensory neurons (Valero-Gracia et al., 2016).
The tunicate endostyle is a ventral organ made by folds of the branchial basket epithelium that displays five/nine zones of specialized cells, comprising supporting and glandular cells and cells with iodinating capacity. Serotonin positive cells of the endostyle are innervated by the subendostylar nerve (Braun and Stach, 2016). The endostyle is considered homologous to the vertebrate thyroids due to its capability to produce iodinated molecules (Godeaux, 1989). Interestingly, 5-HT is also found in the parafollicular cells of the thyroid, further supporting the hypothesis of homology between the two organs (Barasch et al., 1987).
In vertebrates, it is known that 95% of the 5-HT is produced in the intestine (Banskota et al., 2019). Actually, this was first noted by Erspamer who discovered “enteramine” in gastrointestinal in a rabbit (Erspamer and Asero, 1952). Intestinal 5-HT has been found to modulate various aspects of intestinal function, including enteric nervous system development, motility, secretion, inflammation, sensation, and epithelial development (Banskota et al., 2019; Najjar et al., 2023).
Based on the detailed morphological description provided by Burighel and Milanesi, the serotonergic cells described in the digestive system of ascidians may be identified as endocrine cells (Burighel and Milanesi, 1975), and considered homolog to the vertebrate enterochromaffin cells (Gershon, 2004). 5-HT-positive cells are also present in the enteric system of cephalochordates (Candiani et al., 2001) but not in hemichordates or echinoderms (Strano et al., 2019; Mercurio et al., 2019b), suggesting that enteric 5-HT may be a novel feature of chordate evolution.
Noteworthy, in all the analyzed species, 5-HT was not detected in the neural ganglion of the adults. It has been proposed that, after metamorphosis, which leads to a reduction of the sense organs and a sessile life style, the function of 5-HT as a neurotransmitter in the CNS were reduced and could even disappear altogether (Braun and Stach, 2018).
Localization in other tunicates
Tunicates also include free-living thaliaceans encompassing three clades, Doliolida, Salpida and Pyrosomatida, of holoplanktonic organisms with diverse and complex life cycles. Doliolida and Salpida present two different forms, the blastozooid generation, which is produced through asexual reproduction, and the oozooid generation, which is derived from zygote development. Pysosomatida are colonial animals with direct development.
5-HT-positive cells have been reported in many thaliacean species: Thalia democratica (Pennati et al., 2012; Braun and Stach, 2016, 2018; Valero-Gracia et al., 2016); Doliolum nationalis (Stach, 2005; Braun and Stach, 2018); Pyrosomella verticillata (Valero-Gracia et al., 2016; Braun and Stach, 2018), Salpa fusiformis, Iasis cylindrical, Pyrosoma atlanticum, Pyrostremma agassizi (Braun and Stach, 2018); Doliolina muelleri and Ihlea punctate (Valero-Gracia et al., 2016) (Figure 1).
In Salpida and Doliolida species, similar to adult ascidians, serotonergic cells were found in the peripharyngeal band of the digestive tract. However, unlike ascidians, 5-HT immunolabelling was not detected in the endostyle but was instead present in the cerebral ganglion. It has been proposed that the absence of 5-HT in the endostyle of salpids and doliolids could be a character associated with changes in the control of thyroid hormone production (Valero-Gracia et al., 2016). In Pyrosomatida, 5-HT localization appears more comparable to that of adult ascidians: in Pyrosoma agassizi, for example, 5-HT-positive cells were found in the peripharyngeal band, endostyle, and intestine, but not in the central nervous system (Braun and Stach, 2018). As Pyrosomatida are basal thaliaceans (Delsuc et al., 2018; Kocot et al., 2018), they may have retained plesiomorphyc characters from their sessile ancestor. It has been proposed that serotonergic neurons might have a locomotory function by controlling body muscles that have been lost in sessile ascidians and retained or re-acquired in free living thaliaceans. 5-HT absence in the CNS of planktonic pyrosomes could be attributed to their reliance on water flow generated by ciliary beating, rather than muscle movement, for locomotion (Braun and Stach, 2018).
Since the complex life cycles of salps and doliolids, the pattern of distribution of 5-HT-containing cells can differ between oozooid and blastozooid stages (Braun and Stach, 2018). In salps, fertilization occurs internally and the zygote develops inside the blastozooid, in close contact with maternal tissues where a placenta forms. 5-HT immunolocalization has been observed in placenta of T. democratica (Pennati et al., 2012), but, as viviparity is a derived tract in salps, serotonin presence in the placenta should be considered a derived tract not inherited by the common ancestor of tunicates. Interestingly, 5-HT and its receptors has also been reported in the mammalian placenta, where they are believed to play a role not only in placental development and pregnancy maintenance, but also in fetal development (Yavarone et al., 1993a; Huang et al., 1998). This should be considered a homoplasy, most probably due to a conserved and ancient role of serotonin in embryos development.
In the case of appendicularians, the third and most basal group of tunicates, only two cells in the posterior part of the neural ganglion of Oikopleura fusiformis show positive staining against 5-HT antibodies (Stach, 2005), while no 5-HT-like signal has been detected in Oikopleura dioica (Braun and Stach, 2016).
A complete overview of serotonin localization in tunicates is provided in Table 1.
The serotonin machinery
5-HT synthesis pathway involved two fundamental enzymes: tryptophan hydroxylase (TPH) and 5-hydroxytryptophan decarboxylase, an aromatic amino acid decarboxylase (AADC). Moreover, 5-HT concentration in extracellular fluid is regulated by selective membrane serotonin transporter (SERT) and 5-HT can be further transported into storage vesicles via a vesicular mono amine transporter (VMAT). The molecular machinery of serotonin system has been extensively studied in Ciona.
TPH is the rate limiting enzyme in the biosynthesis of serotonin and is considered a good marker of serotonergic neurons (Goridis and Rohrer, 2002). In the genome of Ciona a single gene coding for a TPH (Ci-TPH) is present and its sequence shares a high similarity level with those of vertebrates’ ones (Pennati et al., 2007a). In posterior larval trunk, Ci-TPH expression is restricted to a few cells grouped into two distinct clusters (Pennati et al., 2007a; Razy-Krajka et al., 2012) and in posterior muscle cells of the tail (Pennati et al., 2007a). One gene encoding a vesicular monoamine transporter (Ci-VMAT) is expressed specifically in the TPH domain of the trunk (Razy-Krajka et al., 2012).
Serotonin transporters (SERTs) are plasma membrane transporters involved in neurotransmitter re-uptake. They are Na+-dependent transporters belonging to the solute-linked carrier family 6 (SLC6) of membrane co-transporters. SERTs are present in the genomes of practically all Metazoa (Caveney et al., 2006). In Ciona, Ci-SERT transcripts were detected in the territory of Ci-Tyrosine hydroxylase, the gene coding for the rate-limiting enzyme of dopamine, but not in the Ci-TPH domains of the tail-bud embryos and larvae. It has been proposed that 5-HT could be stored together with dopamine in the Ci-TH-expressing cells of the sensory vesicle, a property shared by some vertebrate amacrine cells of the retina. Although there is no evidence that TPH-positive cells express any of the two genes encoding aromatic amino acid decarboxylase (Ci-AADC) described in Ciona, in both TPH and TH domains the transcripts of GTP cyclohydrolase I (Ci-GCH) are present. This latter is the enzyme responsible for the synthesis of tetrahydrobiopterin, an essential cofactor of serotonin and dopamine synthesis (Razy-Krajka et al., 2012). The absence of recognized AADC genes expression in TPH positive cells needs to be further investigated, also exploring the possibility of a maternal origin of the protein. Interestingly, TPH expression in Ciona adults indicates that serotonergic neurons are present also in the tentacle of coronal cells in the oral siphon, considered homologous to vertebrate hair cells (Rigon et al., 2018).
5-HT exerts its function by binding to different types of receptors that in human have been classified into seven families (5HT 1-7) (Pytliak et al., 2011). With the exception of 5HTR3s, that are ligand-gated ion channels, all the other serotonin receptors (5HTRs) are G-protein coupled receptors.
In the genome of Ciona, five genes corresponding to serotonin receptors have been identified (Mercurio et al., 2023). The phylogenetic analysis showed that two sequences, 5HT1.1 and 5HT1.2, are basal to all 5HT1 paralogs of vertebrates. One sequence is ortholog to 5HT2 and has five protein isoforms, one is an ortholog of 5HT7s, and the last one, 5HT-like, is highly divergent and basal to all G-coupled 5HT classes. In Ciona savigny, the coding region of 5-HT2 is included in the largest intron of the Psmd1-like gene that encodes the non-ATP regulatory subunit 1 (RPN2) of the 26S proteasome. The 26S proteasome is a highly conserved multicatalytic protease from yeast to mammals, which functions to degrade proteins following ubiquitination. None of the other 5-HT receptor genes show a similar genomic localization while this organization is shared with the 5-HT2B gene of all mammals, that are encoded in a large intron of the proteasome Psmd1 too (Moutkine et al., 2019). Interestingly, it was suggested that this genomic arrangement may outcome in a conserved co-regulatory relationship (Assis et al., 2008).
During development, by in situ hybridization, it was possible to identify the territories of expression of four out of the five identified 5HTRs, with the exception of Ci-5HT2. All analyzed genes showed an early expression starting from early gastrula. Ci-5HT-like transcripts were identified with strong signal mainly in territories of presumptive nervous system, in precursors of the anterior sensory vesicle and pigment cells, in cells that will contribute to form larval visceral ganglion and in posterior precursors of the bipolar tail neurons. The other identified Ci-5HTR genes showed a broader expression, mainly in mesenchyme cells with a sharp anterior limit of expression. Ci-5HT7 expression was observed in cells of the anterior most row of neural plate progenitors including palp neuron progenitors. The expression of all genes decreased or were no longer detectable at larva stage (Mercurio et al., 2023). Importantly, all the Ci-5HTRs were expressed in some territories of CNS lineage, suggesting their contribution in neural development, as reported in vertebrates (Romero-Reyes et al., 2021).
Functional evidence
5-HT is a multifaceted molecule that plays many diverse roles (Berger et al., 2009). Its functions in tunicates are still largely to be elucidated. Some hints come from pharmacological inhibition/stimulation of its receptors and transporter (Pennati et al., 2001, 2003; Mercurio et al., 2023). 5-HT involvement in ascidian embryogenesis has been suggested by the early expression of 5HTRs genes in Ciona, and it was confirmed by treatments with 5-HT active drugs. P. mammillata larvae developed by embryos exposed at gastrula stage to Ritanserin, a selective 5HT2 antagonist, showed a roundish head and flat papillae. Juveniles exposed to the same drug had an enlarged heart with blood cells accumulating in it (Pennati et al., 2001). Interestingly, in mouse embryos exposure to Ritanserin induced morphological defects in the head, neural tube and heart, probably by preventing the differentiation of cranial neural crest cells and myocardial precursor cells (Choi et al., 1997). Exposure WAY-100635, an antagonist of 5-HT1 receptors, caused an impairment on anterior trunk with malformed palps and a curved tail both in P. mammillata and Ciona larvae (Pennati et al., 2001; Mercurio et al., 2023). In treated Ciona embryos, the expression of Ci-Pou IV, a specific marker of sensory neurons, indicated that the deactivation of 5HTRs disrupted the development of neurons of palps and tail. Is has been suggested that the lack of most of the sensory neurons detected in WAY-100635-treated embryos could be related to drug interference with the complex interactions between diffusible molecules involved in sensory neuron specification such as retinoic acid, FGF/MAPK signal, and the Wnt pathway (Mercurio et al., 2023). Moreover, larvae exposed to WAY-100635 showed a reduction of pigment in otolith and ocellus, the sensory organs of the sensory vesicle. The pigment organs were recognizable by their shape but melanin content was drastically reduced (Pennati et al., 2003; Mercurio et al., 2023). It was demonstrated that WAY- 100635 treated embryos displayed a drastic decrease in expression of Ci-Tcf. This gene is specifically expressed in the precursor cells of the ocellus and otolith and its perturbation led to larval sensory organs being only partially melanized, suggesting a role in pigment cell terminal differentiation (Squarzoni et al., 2011). 5-HT involvement in melanin synthesis has been reported in different animals. Disrupting the serotonin synthesis impaired pigment synthesis in eyes of Platyhelminthes (Lambrus et al., 2015). Stress conditions and alterations of 5-HT levels can reduce the production of melanin in humans as in mouse. Agonists of 5-HT1A and 5-HT1B receptors proved to be efficient at restoring pigmentation (Wu et al., 2014). In zebrafish, fluoxetine, a selective inhibitor of serotonin reuptake, increases melanin synthesis via 5-HT1A receptor (Liu et al., 2019).
Fluoxetine is a selective blocker of SERT that generate an increase in extracellular 5-HT. In Ciona larvae, exposure to Fluoxetine reduced spontaneous swimming and the shadow response that is a light-triggered bout of high-speed swimming. It was suggested that the DA-synthesizing/5-HT accumulating cells of the ascidian sensory vesicle play a role in controlling the swimming behavior in response to light, strengthening the hypothesis of their homology with the amacrine cells of vertebrates (Razy-Krajka et al., 2012). The function of 5-HT in modulating swimming behavior is further supported by Ci-TPH expression in some Ciona muscle cells of the larval tail, probably at neuro-muscular junctions, suggesting that 5-HT may regulates the left-right alternate tail contractions during larval swimming (Pennati et al., 2007a).
The blocking of SERT have effects also on ascidian metamorphosis. Larvae of P. mammillata exposed to Fluoxetine exhibited an early onset of metamorphosis. A similar stimulating effect was obtained exposing competent larvae to an agonist of 5-HT1 receptor, 8-OH-DPAT. Conversely, 5-HT depletion, by means of antagonists and by antibody incubation, delayed tail resorption and the onset of metamorphosis. Therefore, it is likely that 5-HT plays a key role in the mechanism triggering metamorphosis in P. mammillata larvae (Pennati et al., 2001). 5-HT role in modulating the metamorphosis has been assessed in many different pelagic larvae of marine animals such as barnacles, cnidarians and mollusks (Barlow and Truman, 1992; Couper and Leise, 1996; McCauley, 1997; Zega et al., 2007a, 2007b), suggesting that this could be a conserved and probably ancient role of 5-HT (Zega et al., 2007b).
Discussion
Serotonin is an ancient molecule that plays crucial roles in tunicates. Due to their phylogenetic position, tunicates are pivotal in understanding at least part of the evolutionary history of this eclectic molecule. They preserved some ancient 5-HT roles, such as control of ciliary beating and locomotion coordination, which are also evident in cnidarians (Mayorova and Kosevich, 2013) and the larvae of various invertebrates (Hay-Schmidt, 2000). 5-HT roles as neurotransmitter, in morphogenesis and melanogenesis, are likely to be ancient ones since they have been described also in platyhelminthes (Lambrus et al., 2015). Conversely, the presence of 5-HT positive cells in the intestine in tunicates (Pennati et al., 2001; Tiozzo et al., 2009; Braun and Stach, 2016, 2018), cephalochordates (Candiani et al., 2001) and vertebrates (Gershon, 2004) could be a chordate specific novelty, since it has never been described in other groups (Strano et al., 2019; Mercurio et al., 2019b). Within the tunicate clade, several events of loss and gain of function might have occurred in relation to the evolution of different life cycles. Some of these functions have been probably lost in appendicularians, the clade for which many details are still in need of exploration. Swimming larvae of sessile forms use 5-HT to control locomotion in response to perception of light stimuli and keep 5-HT role in controlling the timing of metamorphosis. Adults of these sessile forms might have lost 5-HT in CNS consequently to loss of locomotion control. Presence of serotonergic neurons in CNS were regained in doliolids and salps that derive from sessile forms but that have reconquered a pelagic life style, with locomotion driven by muscle contractions. An alternative less parsimonious scenario presumes that Stolidobranchia and the clade of Phlebobranchia plus Aplousobranchia have lost 5-HT in adult CNS independently and the sessile ancestor of Doliolida and Salpida retained it as a plesiomorphic character. The absence of 5-HT containing cells in the endostyle of salps and doliolids compared to pyrosomes may result from a secondary loss of serotonin control over ciliary beating and mucus secretion, as suggested by Valero-Gracia et al. (2016).
Overall, the multifaceted roles of 5-HT in tunicates underscore its importance and versatility, prompting further questions about its evolutionary journey. Despite significant advancements, many aspects remain unresolved with functional studies often fragmentary and focused on a few ascidian species. Moreover, molecular characterization of the serotonergic system has only been accomplished in Ciona. Future research should aim to fill these knowledge gaps and better delineate the specific roles of 5-HT in these animals, thus providing valuable insight into its conserved functions and lineage-specific traits.
Author contributions
RP: Conceptualization, Writing – original draft. GB: Data curation, Writing – review & editing. SM: Conceptualization, Supervision, Writing – review & editing. GS: Resources, Supervision, Writing – review & editing.
Funding
The author(s) declare that no financial support was received for the research, authorship, and/or publication of this article.
Acknowledgments
The authors acknowledge the support of the APC central fund of the University of Milan.
Conflict of interest
The authors declare that the research was conducted in the absence of any commercial or financial relationships that could be construed as a potential conflict of interest.
Publisher’s note
All claims expressed in this article are solely those of the authors and do not necessarily represent those of their affiliated organizations, or those of the publisher, the editors and the reviewers. Any product that may be evaluated in this article, or claim that may be made by its manufacturer, is not guaranteed or endorsed by the publisher.
References
Assis R., Kondrashov A. S., Koonin E. V., Kondrashov F. A. (2008). Nested genes and increasing organizational complexity of metazoan genomes. Trends Genet. 24, 475–478. doi: 10.1016/j.tig.2008.08.003
Azmitia E. C. (2001). Modern views on an ancient chemical: serotonin effects on cell proliferation, maturation, and apoptosis. Brain Res. Bull. 56, 413–424. doi: 10.1016/S0361-9230(01)00614-1
Azmitia E. C. (2007). Serotonin and brain: evolution, neuroplasticity, and homeostasis. Int. Rev. Neurobiol. 77, 31–56. doi: 10.1016/S0074-7742(06)77002-7
Bacqué-Cazenave J., Bharatiya R., Barrière G., Delbecque J.-P., Bouguiyoud N., Di Giovanni G., et al. (2020). Serotonin in animal cognition and behavior. Int. J. Mol. Sci. 21, 1649. doi: 10.3390/ijms21051649
Banskota S., Ghia J.-E., Khan W. I. (2019). Serotonin in the gut: blessing or a curse. Biochimie 161, 56–64. doi: 10.1016/j.biochi.2018.06.008
Barasch J. M., Tamir H., Nunez E. A., Gershon M. D. (1987). Serotonin-storing secretory granules from thyroid parafollicular cells. J. Neurosci. 7, 4017–4033. doi: 10.1523/JNEUROSCI.07-12-04017.1987
Barlow L. A., Truman J. W. (1992). Patterns of serotonin and SCP immunoreactivity during metamorphosis of the nervous system of the red abalone, Haliotis rufescens. J. Neurobiol. 23, 829–844. doi: 10.1002/neu.480230705
Berger M., Gray J. A., Roth B. L. (2009). The expanded biology of serotonin. Annu. Rev. Med. 60, 355–366. doi: 10.1146/annurev.med.60.042307.110802
Braun K., Stach T. (2016). Comparative study of serotonin-like immunoreactivity in the branchial basket, digestive tract, and nervous system in tunicates. Zoomorphology 135, 351–366. doi: 10.1007/s00435-016-0317-8
Braun K., Stach T. (2018). Distribution and evolution of serotonin-like immunoreactive cells in Thaliacea (Tunicata). Zoomorphology 137, 565–578. doi: 10.1007/s00435-018-0416-9
Brunetti R., Gissi C., Pennati R., Caicci F., Gasparini F., Manni L. (2015). Morphological evidence that the molecularly determined Ciona intestinalis type A and type B are different species: Ciona robusta and Ciona intestinalis. J. Zoolog Syst. Evol. Res. 53, 186–193. doi: 10.1111/jzs.12101
Burighel P., Milanesi C. (1975). Fine structure of the gastric epithelium of the ascidian Botryllus schlosseri. Mucous, endocrine and plicated cells. Cell Tissue Res. 158, 481–496. doi: 10.1007/BF00220214
Buznikov G. A., Peterson R. E., Nikitina L. A., Bezuglov V. V., Lauder J. M. (2005). The pre-nervous serotonergic system of developing sea urchin embryos and larvae: pharmacologic and immunocytochemical evidence. Neurochem. Res. 30, 825–837. doi: 10.1007/s11064-005-6876-6
Candiani S., Augello A., Oliveri D., Passalacqua M., Pennati R., De Bernardi F., et al. (2001). Immunocytochemical localization of serotonin in embryos, larvae and adults of the lancelet, branchiostoma floridae. Histochem. J. 33, 413–420. doi: 10.1023/A:1013775927978
Caveney S., Cladman W., Verellen L., Donly C. (2006). Ancestry of neuronal monoamine transporters in the Metazoa. J. Exp. Biol. 209, 4858–4868. doi: 10.1242/jeb.02607
Choi D.-S., Ward S. J., Messaddeq N., Launay J.-M., Maroteaux L. (1997). 5-HT2B receptor-mediated serotonin morphogenetic functions in mouse cranial neural crest and myocardiac cells. Dev. Camb. Engl. 124, 1745–1755. doi: 10.1242/dev.124.9.1745
Colas J. F., Launay J. M., Kellermann O., Rosay P., Maroteaux L. (1995). Drosophila 5-HT2 serotonin receptor: coexpression with fushi-tarazu during segmentation. Proc. Natl. Acad. Sci. 92, 5441–5445. doi: 10.1073/pnas.92.12.5441
Couper J. M., Leise E. M. (1996). Serotonin injections induce metamorphosis in larvae of the gastropod mollusc ilyanassa obsoleta. Biol. Bull. 191, 178–186. doi: 10.2307/1542921
De Bernardi F., Pennati R., Candiani S., Biggiogero M., Zega G., Groppelli S., et al. (2006). Serotonin in the morphogenesis of ascidian nervous system. Caryologia 59, 379–383.
Dehal P., Satou Y., Campbell R. K., Chapman J., Degnan B., De Tomaso A., et al. (2002). The draft genome of Ciona intestinalis: insights into chordate and vertebrate origins. Science 298, 2157–2167. doi: 10.1126/science.1080049
Delsuc F., Brinkmann H., Chourrout D., Philippe H. (2006). Tunicates and not cephalochordates are the closest living relatives of vertebrates. Nature 439, 965–968. doi: 10.1038/nature04336
Delsuc F., Philippe H., Tsagkogeorga G., Simion P., Tilak M.-K., Turon X., et al. (2018). A phylogenomic framework and timescale for comparative studies of tunicates. BMC Biol. 16, 39. doi: 10.1186/s12915-018-0499-2
Erspamer V. (1946). Presence of enteramine or an enteraminosimilar substance in the gastrointestinal and splenic extracts of fish and in the gastroenteric extracts of ascidians. Experientia 2, 369–371. doi: 10.1007/BF02163944
Erspamer V., Asero B. (1952). Identification of Enteramine, the specific hormone of the enterochromaffin cell system, as 5-Hydroxytryptamine. Nature 169, 800–801. doi: 10.1038/169800b0
Fiorica-Howells E., Maroteaux L., Gershon M. D. (2000). Serotonin and the 5-HT2B receptor in the development of enteric neurons. J. Neurosci. 20, 294–305. doi: 10.1523/JNEUROSCI.20-01-00294.2000
Georges D. (1985). Presence of cells resembling serotonergic elements in four species of tunicates. Cell Tissue Res. 242, 341–348. doi: 10.1007/BF00214546
Gershon M. D. (2004). Review article: serotonin receptors and transporters — roles in normal and abnormal gastrointestinal motility. Aliment. Pharmacol. Ther. 20, 3–14. doi: 10.1111/j.1365-2036.2004.02180.x
Goridis C., Rohrer H. (2002). Specification of catecholaminergic and serotonergic neurons. Nat. Rev. Neurosci. 3, 531–541. doi: 10.1038/nrn871
Greczek-Stachura M. (2002). The regulation of swimming speed and the rate of cell division by serotonin in Paramecium aurelia. Cell. Mol. Biol. Lett. 07.
Hay-Schmidt A. (2000). The evolution of the serotonergic nervous system. Proc. R. Soc B Biol. Sci. 267, 1071. doi: 10.1098/rspb.2000.1111
Horen W. P. (1972). Insect and scorpion sting. JAMA 221, 894–898. doi: 10.1001/jama.1972.03200210038009
Huang W. Q., Zhang C. L., Di X. Y., Zhang R. Q. (1998). Studies on the localization of 5-hydroxytryptamine and its receptors in human placenta. Placenta 19, 655–661. doi: 10.1016/S0143-4004(98)90027-3
Jeffery W. R., Swalla B. J. (1992). Evolution of alternate modes of development in ascidians. BioEssays 14, 219–226. doi: 10.1002/bies.950140404
Kocot K. M., Tassia M. G., Halanych K. M., Swalla B. J. (2018). Phylogenomics offers resolution of major tunicate relationships. Mol. Phylogenet. Evol. 121, 166–173. doi: 10.1016/j.ympev.2018.01.005
Kravitz E. A. (2000). Serotonin and aggression: insights gained from a lobster model system and speculations on the role of amine neurons in a complex behavior. J. Comp. Physiol. A 186, 221–238. doi: 10.1007/s003590050423
Kristan W. B., Nusbaum M. P. (1982). The dual role of serotonin in leech swimming. J. Physiol. 78, 743–747.
Lambrus B. G., Cochet-Escartin O., Gao J., Newmark P. A., Collins E.-M. S., Iii J. J. C. (2015). Tryptophan hydroxylase is required for eye melanogenesis in the planarian Schmidtea mediterranea. PloS One 10, e0127074. doi: 10.1371/journal.pone.0127074
Lemaire P. (2011). Evolutionary crossroads in developmental biology: the tunicates. Development 138, 2143–2152. doi: 10.1242/dev.048975
Liu L., Fu M., Pei S., Zhou L., Shang J. (2019). R-fluoxetine increases melanin synthesis through a 5-HT1A/2A receptor and p38 MAPK signaling pathways. Int. J. Mol. Sci. 20, 80. doi: 10.3390/ijms20010080
Lv J., Liu F. (2017). The Role of Serotonin beyond the Central Nervous System during Embryogenesis. Front. Cell. Neurosci. 11. doi: 10.3389/fncel.2017.00074
Mayorova T. D., Kosevich I. A. (2013). Serotonin-immunoreactive neural system and contractile system in the hydroid Cladonema (Cnidaria, Hydrozoa). Invert. Neurosci. 13, 99–106. doi: 10.1007/s10158-013-0152-2
McCauley D. W. (1997). Serotonin plays an early role in the metamorphosis of the hydrozoan phialidium gregarium. Dev. Biol. 190, 229–240. doi: 10.1006/dbio.1997.8698
Mercurio S., Bozzo M., Pennati A., Candiani S., Pennati R. (2023). Serotonin receptors and their involvement in melanization of sensory cells in ciona intestinalis. Cells 12, 1150. doi: 10.3390/cells12081150
Mercurio S., Cauteruccio S., Manenti R., Candiani S., Scarì G., Licandro E., et al. (2019a). miR-7 knockdown by peptide nucleic acids in the ascidian ciona intestinalis. Int. J. Mol. Sci. 20, 5127. doi: 10.3390/ijms20205127
Mercurio S., Gattoni G., Messinetti S., Sugni M., Pennati R. (2019b). Nervous system characterization during the development of a basal echinoderm, the feather star Antedon mediterranea. J. Comp. Neurol. 527, 1127–1139. doi: 10.1002/cne.24596
Mercurio S., Messinetti S., Manenti R., Ficetola G. F., Pennati R. (2021). Embryotoxicity characterization of the flame retardant tris(1-chloro-2-propyl)phosphate (TCPP) in the invertebrate chordate Ciona intestinalis. J. Exp. Zool. Part Ecol. Integr. Physiol. 335, 339–347. doi: 10.1002/jez.2446
Moiseiwitsch J. R., Lauder J. M. (1995). Serotonin regulates mouse cranial neural crest migration. Proc. Natl. Acad. Sci. U.S.A. 92, 7182–7186. doi: 10.1073/pnas.92.16.7182
Moutkine I., Collins E. L., BéChade C., Maroteaux L. (2019). Evolutionary considerations on 5-HT2 receptors. Pharmacol. Res. 140, 14–20. doi: 10.1016/j.phrs.2018.09.014
Najjar S. A., Hung L. Y., Margolis K. G. (2023). Serotonergic control of gastrointestinal development, motility, and inflammation. Compr. Physiol. 13, 4851–4868. doi: 10.1002/cphy.c220024
Neumann J., Hofmann B., Dhein S., Gergs U. (2023). Cardiac roles of serotonin (5-HT) and 5-HT-receptors in health and disease. Int. J. Mol. Sci. 24, 4765. doi: 10.3390/ijms24054765
Nichols D. E., Nichols C. D. (2008). Serotonin receptors. Chem. Rev. 108, 1614–1641. doi: 10.1021/cr078224o
Nilsson O., Fredriksson G., Öfverholm T., Ericson L. E. (1988). Electron-microscopic immunocytochemistry of 5-hydroxytryptamine in the ascidian endostyle. Cell Tissue Res. 253, 137–143. doi: 10.1007/BF00221748
Pennati R., Candiani S., Biggiogero M., Zega G., Groppelli S., Oliveri D., et al. (2007a). Developmental expression of tryptophan hydroxylase gene in Ciona intestinalis. Dev. Genes Evol. 217, 307–313. doi: 10.1007/s00427-007-0138-3
Pennati R., Dell’Anna A., Zega G., De Bernardi F. (2012). Immunohistochemical study of the nervous system of the tunicate Thalia democratica (Forsskal 1775). Eur. J. Histochem. EJH 56, e16. doi: 10.4081/ejh.2012.16
Pennati R., Ficetola G. F., Brunetti R., Caicci F., Gasparini F., Griggio F., et al. (2015). Morphological differences between larvae of the Ciona intestinalis species complex: hints for a valid taxonomic definition of distinct species. PloS One 10, e0122879. doi: 10.1371/journal.pone.0122879
Pennati R., Groppelli S., De Bernardi F., Mastrototaro F., Zega G. (2009). Immunohistochemical analysis of adhesive papillae of Clavelina lepadiformis (Müller 1776) and Clavelina phlegraea (Salfi 1929) (Tunicata, Ascidiacea). Eur. J. Histochem. EJH 53, e4. doi: 10.4081/ejh.2009.e4
Pennati R., Groppelli S., Sotgia C., Candiani S., Pestarino M., De Bernardi F. (2001). Serotonin localization in Phallusia mammillata larvae and effects of 5-HT antagonists during larval development. Dev. Growth Differ. 43, 647–656. doi: 10.1046/j.1440-169X.2001.00608.x
Pennati R., Groppelli S., Sotgia C., Zega G., Pestarino M., De Bernardi F. (2003). WAY-100635, an antagonist of 5-HT1A receptor, causes malformations of the CNS in ascidian embryos. Dev. Genes Evol. 213, 187–192. doi: 10.1007/s00427-003-0311-2
Pennati R., Rothbächer U. (2015). Bioadhesion in ascidians: a developmental and functional genomics perspective. Interface Focus 5, 20140061. doi: 10.1098/rsfs.2014.0061
Pennati R., Zega G., Groppelli S., De Bernardi F. (2007b). Immunohistochemical analysis of the adhesive papillae of Botrylloides leachi (Chordata, Tunicata, Ascidiacea): Implications for their sensory function. Ital. J. Zool 74, 325–329. doi: 10.1080/11250000701562229
Pestarino M. (1982). Occurrence of different secretin-like cells in the digestive tract of the ascidian Styela plicata (Urochordata, Ascidiacea). Cell Tissue Res. 226, 231–235. doi: 10.1007/BF00217097
Pytliak M., Vargová V., Mechírová V., Felšöci M. (2011). Serotonin receptors - from molecular biology to clinical applications. Physiol. Res. 60, 15–25. doi: 10.33549/physiolres.931903
Razy-Krajka F., Brown E. R., Horie T., Callebert J., Sasakura Y., Joly J.-S., et al. (2012). Monoaminergic modulation of photoreception in ascidian: evidence for a proto-hypothalamo-retinal territory. BMC Biol. 10, 45. doi: 10.1186/1741-7007-10-45
Rigon F., Gasparini F., Shimeld S. M., Candiani S., Manni L. (2018). Developmental signature, synaptic connectivity and neurotransmission are conserved between vertebrate hair cells and tunicate coronal cells. J. Comp. Neurol. 526, 957–971. doi: 10.1002/cne.24382
Romero-Reyes J., Molina-Hernández A., Díaz N. F., Camacho-Arroyo I. (2021). Role of serotonin in vertebrate embryo development. Reprod. Biol. 21, 100475. doi: 10.1016/j.repbio.2020.100475
Sakharov D. A., Salimova N. (1982). Serotonin-containing cells in the ascidian endostyle. Experientia 38, 802–803. doi: 10.1007/BF01972280
Satoh N. (2013). “Ciona: A Model for Developmental Genomics,” in Encyclopedia of Life Sciences (Chichester: John Wiley & Sons, Ltd). doi: 10.1002/9780470015902.a0021411
Squarzoni P., Parveen F., Zanetti L., Ristoratore F., Spagnuolo A. (2011). FGF/MAPK/Ets signaling renders pigment cell precursors competent to respond to Wnt signal by directly controlling Ci-Tcf transcription. Development 138, 1421–1432. doi: 10.1242/dev.057323
Stach T. (2005). Comparison of the serotonergic nervous system among Tunicata: implications for its evolution within Chordata. Org. Divers. Evol. 5, 15–24. doi: 10.1016/j.ode.2004.05.004
Strano F., Micaroni V., Beli E., Mercurio S., Scarì G., Pennati R., et al. (2019). On the larva and the zooid of the pterobranch Rhabdopleura recondita Beli, Cameron and Piraino 2018 (Hemichordata, graptolithina). Mar. Biodivers 49, 1657–1666. doi: 10.1007/s12526-018-0933-2
Thorndyke M. C., Probert L. (1979). Calcitonin-like cells in the pharynx of the ascidian Styela clava. Cell Tissue Res. 203, 301–309. doi: 10.1007/BF00237244
Tiozzo S., Murray M., Degnan B. M., De Tomaso A. W., Croll R. P. (2009). Development of the neuromuscular system during asexual propagation in an invertebrate chordate. Dev. Dyn 238, 2081–2094. doi: 10.1002/dvdy.22023
Valero-Gracia A., Marino R., Crocetta F., Nittoli V., Tiozzo S., Sordino P. (2016). Comparative localization of serotonin-like immunoreactive cells in Thaliacea informs tunicate phylogeny. Front. Zool 13, 45. doi: 10.1186/s12983-016-0177-6
Weiger W. A. (1997). Serotonergic modulation of behaviour: A phylogenetic overview. Biol. Rev. 72, 61–95. doi: 10.1111/j.1469-185X.1997.tb00010.x
Welsh J. H., Loveland R. E. (1968). 5-Hydroxytryptamine in the ascidian, Ciona intestinalis L. Comp. Biochem. Physiol. 27, 719–722. doi: 10.1016/0010-406X(68)90613-0
Wu H.-L., Pang S.-L., Liu Q.-Z., Wang Q., Cai M.-X., Shang J. (2014). 5-HT1A/1B receptors as targets for optimizing pigmentary responses in C57BL/6 mouse skin to stress. PloS One 9, e89663. doi: 10.1371/journal.pone.0089663
Yaguchi S., Katow H. (2003). Expression of tryptophan 5-hydroxylase gene during sea urchin neurogenesis and role of serotonergic nervous system in larval behavior. J. Comp. Neurol. 466, 219–229. doi: 10.1002/cne.10865
Yavarone M. S., Shuey D. L., Sadler T. W., Lauder J. M. (1993a). Serotonin uptake in the ectoplacental cone and placenta of the mouse. Placenta 14, 149–161. doi: 10.1016/S0143-4004(05)80257-7
Yavarone M. S., Shuey D. L., Tamir H., Sadler T. W., Lauder J. M. (1993b). Serotonin and cardiac morphogenesis in the mouse embryo. Teratology 47, 573–584. doi: 10.1002/tera.1420470609
Zega G., Pennati R., Dahlström M., Berntsson K., Sotgia C., De Bernardi F. (2007a). Settlement of the barnacle Balanus improvisus: The roles of dopamine and serotonin. Ital. J. Zool 74, 351–361. doi: 10.1080/11250000701631594
Keywords: neurotransmitter, ascidian, serotonin receptor, serotonin transporter, 5-HT, development
Citation: Pennati R, Blumer G, Mercurio S and Scarì G (2024) Serotonin system in tunicates: insight from morphological and molecular approaches. Front. Ecol. Evol. 12:1378927. doi: 10.3389/fevo.2024.1378927
Received: 30 January 2024; Accepted: 05 April 2024;
Published: 18 April 2024.
Edited by:
Paolo Sordino, Anton Dohrn Zoological Station Naples, ItalyReviewed by:
Graziano Fiorito, Stazione Zoologica Anton Dohrn, ItalyShunsuke Yaguchi, University of Tsukuba, Japan
Copyright © 2024 Pennati, Blumer, Mercurio and Scarì. This is an open-access article distributed under the terms of the Creative Commons Attribution License (CC BY). The use, distribution or reproduction in other forums is permitted, provided the original author(s) and the copyright owner(s) are credited and that the original publication in this journal is cited, in accordance with accepted academic practice. No use, distribution or reproduction is permitted which does not comply with these terms.
*Correspondence: Silvia Mercurio, silvia.mercurio@unimi.it; sil.mercurio@gmail.com