- 1Department of Agronomy, Institute of Agronomy, Hungarian University of Agriculture and Life Sciences, Kaposvár, Hungary
- 2Department of Horticulture, Faculty of Technical and Human Sciences, Sapientia Hungarian University of Transylvania, Calea Sighişoarei, Romania
A well-known insect phenomenon is colour polymorphism, from which the species hopes to gain some ecological advantage. Herbivores adapt to the colours of their environment to reduce their chances of becoming prey, while predators use camouflage colours to succeed in hiding from prey. The larvae of the cosmopolitan, highly invasive cotton bollworm (Helicoverpa armigera Hbn., Lep.: Noctuidae) also benefit from this morphological polymorphism. Their fully-developed larvae can take on various colours when feeding on foliage. Our study aimed to determine the host plant-related colour adaptation of H. armigera larvae collected from different plant organs using different spectral analysis methods. Our studies, based on colour analysis of photographic images, showed that the colour of fully-developed larvae of the species is highly correlated with the colour of the damaged nutrient plant. The dominant colours of the larvae show a high similarity with the colours of the consumed host plant parts. The RGB-based analysis confirmed that larval colours are clustered according to the host plant’s organs and are mainly located in the yellow and green regions of the visible light spectrum. All these results confirm the extraordinary adaptive capabilities of this invasive species, which, among other things, verify its worldwide distribution.
1 Introduction
The cotton bollworm, Helicoverpa armigera Hbn. 1805 (Lepidoptera: Noctuidae) is an original Mediterranean fauna element (Anderson et al., 2016). It is a typically facultative migrant species, which wandered every 16-17 years only to northern areas in former years (Feng et al., 2004). Surprisingly, a significant escalation of its range was observed in the last decade of the 20th century. After this outbreak, It established stable colonies in many countries worldwide (Pedgley, 1985; Keszthelyi et al., 2013; Kriticos et al., 2015). It has appeared in the Australian (Fitt et al., 1995) and the American (Czepak et al., 2013) continents, too, where it is gradually gaining ground in many agrohabitats, displacing the native corn earworm (Helicoverpa zea Boddie, Lepidoptera: Noctuidae) (Bentivenha et al., 2016).
The species develops in various generations as a function of its distribution range. Hungary has two to three generations and overwinters as a pupa (Keszthelyi et al., 2013). The pest is highly polyphagous. It has more than 300 host plants. It can damage maize, sunflower, pepper, tomato, bean and many other arable, horticultural and ornamental crops (Firempong and Zalucki, 1989; Liu et al., 2004). A common characteristic of damage in different host crops is that the larvae specifically damage the generative organs. Thus, first of all, it masticates the ears and tassels of maize; on sunflowers, it bores into the blooms; on pepper and tomato, it damages the berries (Virić Gašparić et al., 2022). Due to its damage, the wounds may be secondarily colonised by various pathogens, which can further reduce the quantity and quality of the crop (Darvas et al., 2011; Dimitrov et al., 2013).
Therefore, the insect can be found on a wide range of host plants with different organic matter compositions and a wide variety of colours, where it is intensively feeding. As a canopy-dwelling herbivorous organism, it has a fundamental interest in matching the colour of its direct environment, its host plant, to reduce the likelihood of becoming prey. Colour changes often occur in lepidopterous larvae, which are based on a physiological colour change due to the migration of pigments (Raabe, 1982). Many factors can influence the development of these colour variants (Lehtonen et al., 2009), representing environmental effects between different populations and various selection forces (Raabe, 1982; Mallet and Joron, 1999). In most cases, environmental factors such as light, temperature and diet often have a decisive effect on this manifested colour composition (Raabe, 1982). It is a well-known biological fact that the larval colour of H. armigera is polymorphic (Deepa and Srivastava, 2010). Its larvae can be green, brown, beige, yellow, or any shade. In H. armigera, different body colours appear during the middle larvae stadiums (L3, L4) and become pronounced until the fully-developed larval stage. Ramos and Morallo-Rejesus (1976) showed that the source mainly determines the colour of H. armigera larvae.
The formation of different coloured larvae is explained by the experimental results of Yamasaki et al. (2009). Their results-based laboratory experiments pointed out that H. armigera larvae fed on different plant organs differ in colour. Thus, the larvae feeding on leaves are green, in contrast to those developing on a diet of flowers and fruits. Their results suggested that the colouration of the larvae was mainly determined by the part of the plant on which the larvae were reared. Chakravarty et al. (2020) analysed the larval colours of H. armigera populations originating from different locations and showed that the dominant colours differed significantly in their place of origin. It is hypothesised that diet-induced body colour polymorphism in H. armigera may play a role in larvae avoiding visual predation (Yamasaki et al., 2009).
Objective informatics values based on colour image analysis (such as RGB, HUE) have been known since 1989 (Chang and Reid, 1996). They are becoming increasingly popular as methodological methods based on colour comparison. RGB is represented digitally by 256 values each, representing more than 16 million possible colour combinations. Their objective identification allows for comparing certain physiological phenomena through the parameterisation of individual colours (McColl and Martin, 1989)). RGB and HSV models were used in the current study for several reasons. RGB directly corresponds to modern display hardware, and it is easily interpretable and implementable in analysis pipelines. Moreover, it is widely used in colour composition analyses. HSV can be easily derived from an RGB colour cube by projecting it onto a plane with R+G+B=1. It can also be statistically advantageous, as the position of the examined colour on the colour spectrum is expressed by a single variable (Hue) (Schwarz et al., 1987). Their application in entomological research has also provided solutions to several problems that were previously unresolved or difficult to understand. Colours and colour patterns have been used to study a wide range of ecological and practical plant conservation topics, including colour changes caused by pests on food plants (Clement et al., 2015), to assess phenotypic peculiarities accompanying insect development (Lacotte et al., 2023), to provide data for prognostic tools (Hassan et al., 2014), or to understand different mechanisms of crypsis (Sherratt et al., 2007).
In light of this scientific background, our studies, based on field and retrospective image processing, aimed to confirm the colour differences in fully-developed larvae feeding on different host plants and plant organs. Besides, we aimed to elucidate the host-plant-related colour polymorphism of H. armigera larvae using digital colour analysis methods. We wanted to determine the dominant colours in their positions in the visible light range. Finally, we wanted to see if the colour of the larvae was related to the colour of the damaged organs of the host plant. The anticipated outcomes may provide crucial insights into the crypsis of this significant herbivore. They may also illuminate the physiological processes underlying the success of a pest with exceptional adaptability, and contribute to the discovery of the distinctive ecological characteristics underpinning invasive species’ spread.
2 Materials and methods
2.1 Production and classified of experimental photographic material
Photographs of fully-developed H. armigera larvae from different plants and plant organs were taken during field visits. The digital pictures were taken in September 2022 and 2023 at the end of the vegetation cycle of Central Europe, in arable fields, in the regions of Kaposvár (46°22’36.90” N 17°47’34.38” E) and Somogyszil (46°32’40.22” N 17°58’41.40” E) in Hungary. The photos were taken so that the whole larva and its host plant were visible. The equipment used for photography includes a Canon EOS 80D SLR camera, Canon EF 100mmf/2.8 Macro USM lens and Canon 580EX II flash. The photography method was standardised, and photos were taken from an equal distance (≈30 cm), standard flash fire, with an exposure time of 1/250 sec and an aperture of 7.1. The resolution of the photos was 3888×2592 pixels.
The records were divided into four groups: 1st group the larvae on the ear of maize (hereinafter: on maize ears),.2nd group: on the blooms of sunflower plants (hereinafter: on sunflower blooms), 3rd group on green leaves (regardless of plant species) (hereinafter: on leaves) as well as 4th on the green blooms of wind-pollinated plants (regardless of plant species) (hereinafter: on green, wind pollinated blooms) (Figure 1). From these photographs, 10-10 records were classified into each group for further analysis. The criterion for the selection of the images was that the plant organ should entirely occupy the larva analysed and that this plant organ should be visible in the background The dominant larval colours, the specific colours of larvae from different host plants and plant organs, and the degree of similarity to the typical colours of the host plant organ were determined.
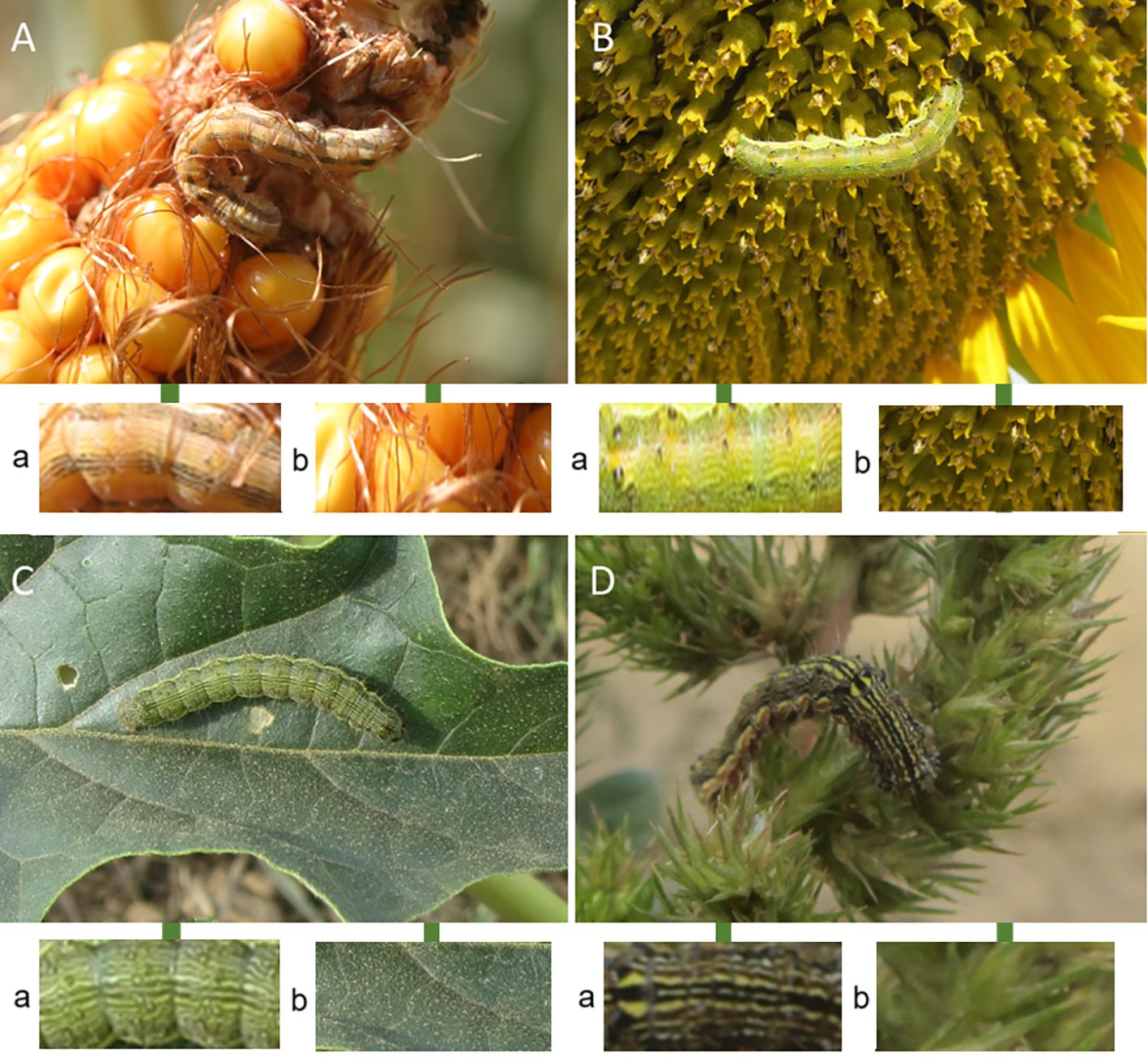
Figure 1 Records of H armigera larvae from the different host plants and plant parts. (A) on maize ears; (B) on sunflower blooms; (C) on leaves; (D) on green, wind-pollinated blooms; a: larva skin samples; b: host plant samples.
2.2 Composition of the larval colours, clustering according to origin
Ten pictures were analysed for each group (maize ears, sunflower bloom, leaves, and wind-pollinated blooms). From each analysed larval and host plant section, an area of 200×200 pixels was cut out using GIMP 2.10.32 software so that the selected area was representative of the colour composition of the analysed section. All individual colours of larval skins were determined using IMGONLINE software (https://www.imgonline.com.ua/). Dominant larval colours from different locations, their dominance and frequency percentages were identified using the Palette Generator (https://palettegenerator.com/) and ICS (http://mkweb.bcgsc.ca/) software, which provide natural image palettes extracted using the k-means algorithm. The extracted colours were positioned in the visible light spectrum using RGB (Red, Green, Blue) and HSV (Hue, Saturation, Value) parameters. The information originated from RGB analysis and was used to determine the position of the dominant larval colours in the visible light range and the clustering of larvae from different host plants and plant parts in the light spectrum and their possible overlapping. By analysing the HSV (Hue, Saturation, Value) data from the same recordings, we determined the Hue values of larvae from different plant organs, their percentage distribution on the colour palette, and the average (mean ± SE) saturation and value parameters of these groups Main Hue and RGB values were assessed on 100 equal-sized subparts of each picture, resulting in 100 mean Hue and mean R, G, and B values for each image.
To better assess the visual distinctness of the grouped larval colours, 3-D concentration ellipsoidal plots were generated using RGB data of these colours using Rcmdr 2.8.0 under R 4.2.2. Statistically verifiable differences between larval colours from different plants or plant sites were analysed using one-way multivariate analysis of variance (one-way MANOVA).In this analysis, there were three dependent variables (raw R, G, and B values) and a single independent variable,” host”, with 4 different values (maize, sunflower, green leaves, and wind-pollinated green flowers). A Kruskal-Wallis test was conducted to determine if there were differences in Hue scores of the images depicting larval skin according to their location on the host plants. Subsequently, pairwise comparisons were performed using Dunn’s test with Bonferroni correction for multiple comparisons. The statistical analysis was conducted by the SPSS 29.0 software package (p ≤ 0.05).
Spearman’s rank-order correlations were carried out to assess the relationship between raw R, G, and B values of different host and larval skin pairs (corn ear, green wind pollinated, leaf, sunflower). Preliminary analyses showed the relationships to be monotonic, as assessed by visual inspection of scatterplots.
3 Results
3.1 Colour composition of H. armigera larvae depending on host plant
Figure 2 shows the colour composition and most dominant colours of H. armigera larvae developing on different plant organs are shown in Figure 3. Although similar colours in the different groups overlap (RGB codes: #C9C581, #A3A376, #8C7556, #1A1209), the separation of the colour composition of these different groups is visible.
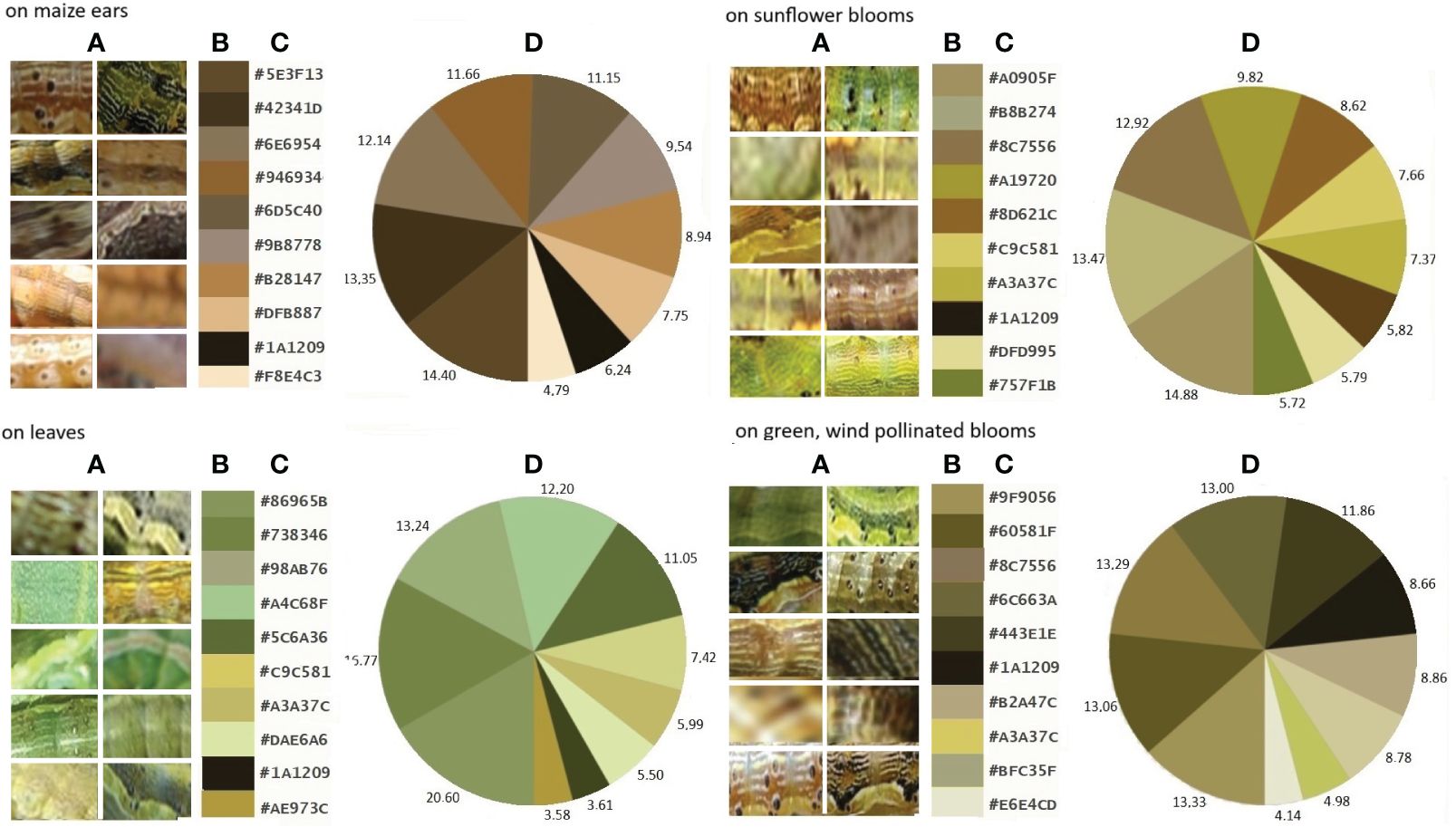
Figure 2 Larval skins of H armigera (A), dominant colours classified by RGB of these larvae from different host plants (B), plant organs, their colour codes (C) and percentages (D).
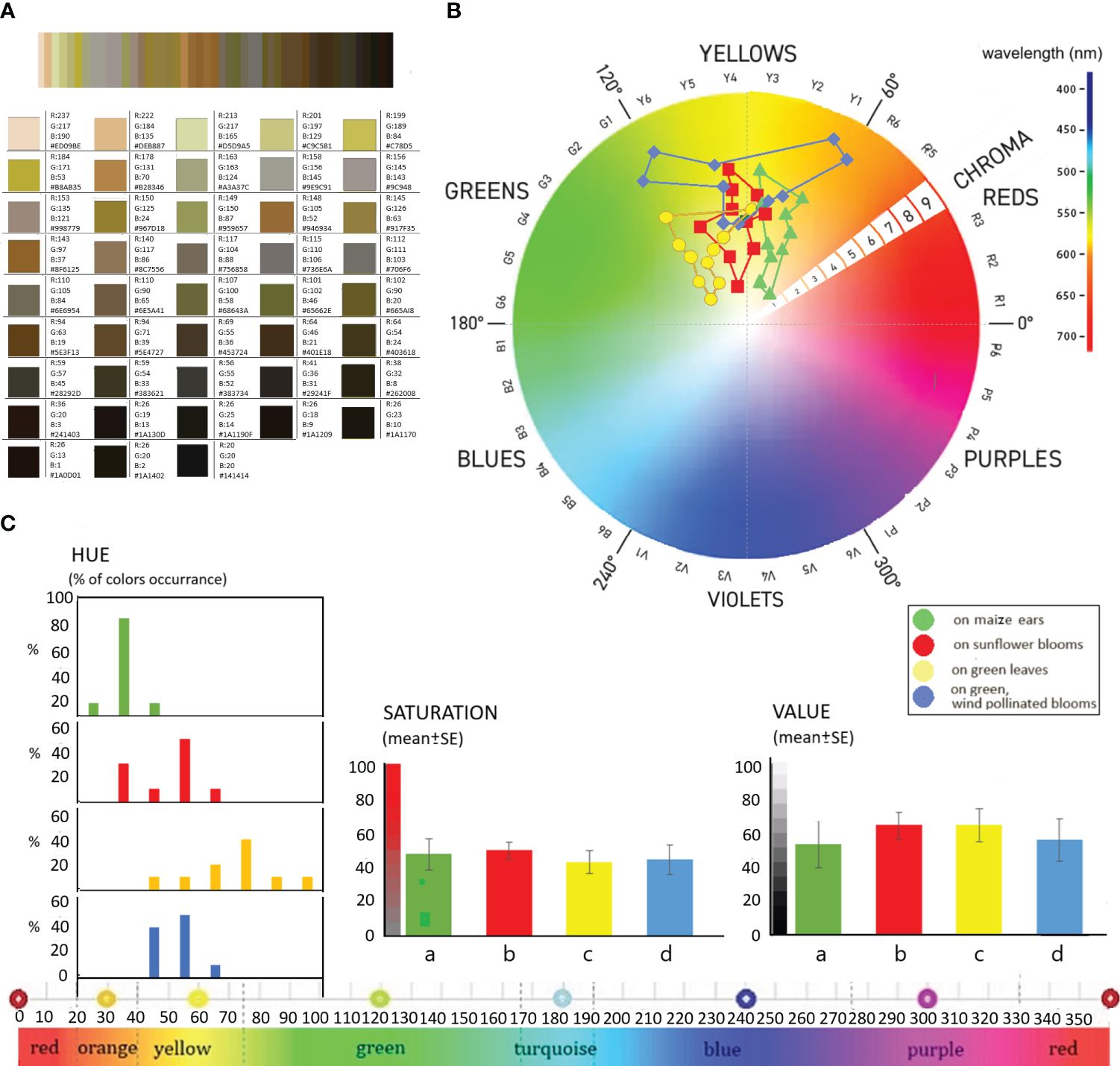
Figure 3 The RGB parameters of dominant colours (A), their locations in the visible light range (B) and distribution of HSV-based colour determination (C) of examined H. armigera larvae; (a) on maize ears, (b) on sunflower blooms, (c) on green leaves, (d) on green, wind-pollinated blooms.
The 43 most predominant colours and their RGB colour codes and HSV values of all H. armigera larvae analysed are shown in Figure 3A. The combined analysis of the larval colours, containing about 214.620 pixels, comprises 126.027 individual colours. It can be seen that the larval colours of insects from different parts of the plant are mainly in the “yellow” range of the visible light spectrum. Only the colours of the larvae developing on vegetative leaves cluster in the border areas of the “green” range close to yellow. The colours of the larvae observed on maize ears, however, are close to the “red” wavelength range. Without exception, the colour of H. armigera larvae was in the warm wavelength range of 565-590 nm.
The colours classified by RGB of larvae originating from different host plants were well separated (Figure 3B). The colour composition of the larvae feeding on sunflower inflorescences was mainly located in the centre of the “yellow” colour range between 575-580nm wavelength. Larvae collected on wind-pollinated green plant inflorescences had the most diverse colour composition, which spread from green to red. In terms of colour tone, the larvae of this group had the darkest colours, with chroma values ranging from 6 to 9, compared to chroma values of 1 to 7 for the other sample groups.
Based on HSV-based colour determination, the larval colours have HUE values between 31 and 97°, i.e. they lie within a well-defined narrow spectrum of the whole colour spectrum, representing about 18.33% of the total 360° spectrum. 27.5% of the colours are in the 31°-40° range and 27.5% in the 51°-60° range Figure 3C. 17.5% of colours are found in the 41-50° range, 10-10% in the 61-70° and 71-80° ranges, and 2.5% each in the 21-30°, 81-90° and 91-100° ranges. The narrowest colour range of about 30° is found in the colours of larvae developing on maize (31-46°) and on the inflorescences of wind-pollinated plants (44-62°). The colour composition of the larvae developing on sunflower inflorescences is slightly more diverse, covering a wider spectrum, with Hue values ranging from 38° to 66°. The broadest spectrum is occupied by the colours of larvae developing on leaves, with hue values ranging from 48° to 97°. The colours of larvae developing on these vegetative organs are essentially distinct from those of larvae developing on other plant organs. The colours of these larvae tend to be in the border regions of the green-yellow spectrum or the green spectrum.
Figure 4A shows the RGB-based scatterplot with 3D concentration of larvae from different parts of the host plants. The colours of the caterpillars developing in other parts of the larvae are clustered in largely different groups. Naturally, similar to visible light analysis, there are also colour overlaps.
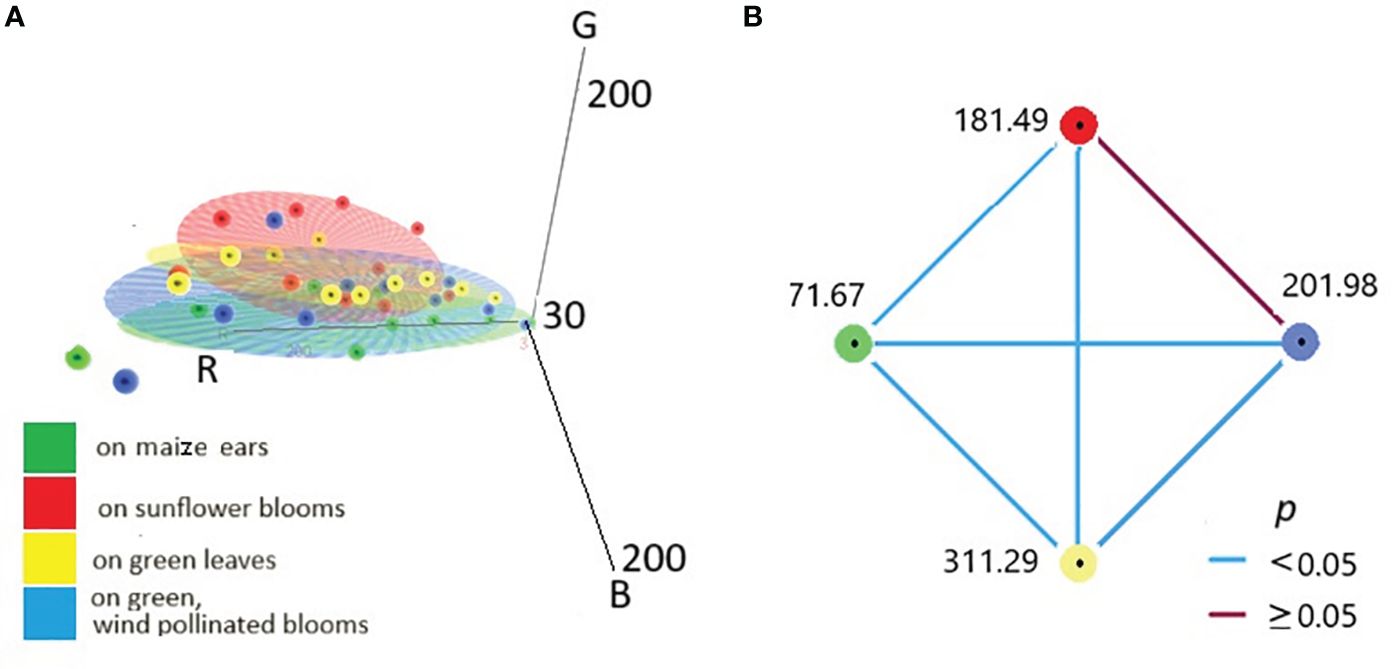
Figure 4 RGB-based scatterplot with 3D concentration ellipsoid of colours of larvae from different food plants and plant organs (A). Relation of mean Hue values of larvae from various plant parts visualised by pairwise comparison. Each node and belonging numbers show the mean rank of other groups (B).
The assumptions of one-way MANOVA (p ≤0.05) were satisfied, as judged by testing for homogeneity of variances, univariate and multivariate outliers, normality, a linear relation between dependent variables, homogeneity of variance-covariance matrices, and multi-collinearity. There was a statistically significant difference between the caterpillars on different host plants in the combined dependent variables (R, G, B values), F(9, 108)= 4,825, p = 0.00002; Pillai’s V = 0.860; partial η2 = 0.287.
Median Hue scores significantly differed between the different locations, χ2(3) = 215.866, p < 0.001. Adjusted p-values for all pairwise comparisons are presented in Figure 4B. It can be seen that each cluster significantly differed from the others (p < 0.001), except for one relation (in the case of larvae originating from sunflower and wind-pollinated blooms). Blue lines represent statistically significant pairwise comparisons (the red line is nonsignificant).
3.2 Correlating host plant and larval skin colour pairs
Paired maize ear host-larval skin R, G, and B values showed weak and very weak significant correlations rs(998) = 0.386, p < 0.0001; rs(998) = 0.135, p < 0.0001; rs(998) = 0.165, p < 0.0001, for R, G, and B values respectively. Similarly, paired R, G, and B values from green wind-pollinated host-larvae images also showed weak and very weak significant correlations rs(998) = 0.167, p < 0.0001; rs(998) = 0.153, p < 0.0001; rs(998) = 0.272, p < 0.0001, for R, G, and B values respectively. Only the R and G values showed moderate significant correlations on paired green leaf host-larval skin image pairs rs(998) = 0.432, p < 0.0001; rs(998) = 0.417, p < 0.0001 for R and G values, respectively. In the case of the paired sunflower host-larvae images, weak significant correlations were observed between the paired R, G, and B raw values rs(998) = 0.310, p < 0.0001; rs(998) = 0.368, p < 0.0001; rs(998) = 0.360, p < 0.0001, for R, G, and B values respectively.
4 Discussion
Our study has confirmed that H. armigera can successfully adapt to the colour of its host plant. The colour polymorphism of the larvae of this species is a known fact, the triggers and causes of which are reported in detail in Yamasaki et al. (2009) and Chakravarty et al. (2020). Based on the results of their laboratory studies, they point out that the colour of the larvae is related to the colour composition of the host plant currently consumed, which is mainly a function of the incorporation of carotenoid content of the plant (Ramos and Morallo-Rejesus, 1976),
As a diurnal herbivore, its adaptation of H. armigera to its environment’s colour is crucial for its survival. This phenomenon is known as crypsis (Endler, 1981) and has been identified as a survival mechanism in several prey-predator relationships. H. armigera has successfully used crypsis to ensure successful larval survival. In their study, Rodríguez-Morales et al. (2021) showed that the visual perception of a predator depends on the spectral sensitivity of the prey’s eye, spatial understanding and temporal resolution. In addition, abiotic factors, such as wind or obstacles, can increase the sensitivity of visual perception (Thery and Gomez, 2010).
The colour variation of larvae developing on various plants is different. Development on the maize ear effectively forces the larva into a narrow spectrum of colours to achieve perfect crypsis. In contrast, a broader range of colours appears on larvae on leaves with varying shades of green or wind-pollinated blooms. The significant difference in colour composition of larvae developing on the inflorescence of wind pollinated blooms should be noted compared to larvae developing on other hosts. This segregation may initially lead to the emergence of new ecotypes as an initial step of adaptation to ecological segregation (Lowry, 2012). Later, as certain host plants become dominant, it may lead to genetic fixation of individuals, i.e. the emergence of new biotypes.
A study by De Moraes and Mescher (2004) showed that the colour variability in larvae of some butterfly species depends on the colour of the food plant consumed and the colour of the leaves and plant organs consumed by the female laying her eggs. Thus, genetic and epigenetic origin is also a determining factor. Several genes responsible for larval colour have been identified in moths. For example, the juvenile hormone is responsible for the manifest larval colouration of Bombyx mori L (Futahashi et al., 2008; Zhao et al., 2012) and some butterflies (Shirataki et al., 2010), as well as for the abrupt changes in colour patterns of these larvae (Futahashi and Fujiwara, 2008). In addition, melanin synthesis is under ecdysteroid regulation in larvae of the butterfly species Papilio xuthus L. (Futahashi and Fujiwara, 2007) and Manduca sexta L (Hiruma and Riddiford, 2009). Overall, in these cases, despite hormonal regulation, larval body colour is genetically determined without being influenced by environmental factors.
In addition to genetic factors, environmental factors are crucial in determining larval colouration in some species (Hazel, 2002). In the case of Battus philenor L., larval body colour is determined by prevailing temperature, probably as a consequence of adaptation to high temperature environments (Nice and Fordyce, 2006).
Our studies raise the question of the analysis from the perspective of different predator species, which has been analysed in other prey-predator relationships by Bennett and Cuthill (1994) as well as Rodríguez-Morales et al. (2021) among others. The question arises whether H. armigera can successfully use crypsis against predators that sense different light ranges (such as various spider, bird, and wasp species). The invasive characteristic of this species follows that when it conquers new areas, it can encounter not only food plants but also predators that were previously unknown to it. The successful application of crypsis prior to these species may even be a critical factor in colonising a new area. The demonstrated colour polymorphism of H. armigera larvae could be a key moment in the invasive insect’s conquest of new agricultural areas. This sophisticated ecological adaptive tool, crypsis, supports the chances of avoiding becoming a potential prey in new habitats and thus establishing a stable population as soon as possible. This phenomenon, in turn, points towards an increase in agricultural damage through the success of this important herbivore species.
Colour analysis and image processing may become an essential tool in future entomological studies, as Byers (2006) has successfully demonstrated that modern high-resolution cameras can detect a sufficient number of colours and isolate them with high reliability even under natural conditions. However, scientific results on the comparative colour analysis of herbivores and their host plants are still relatively scarce in the literature. By applying these approaches, several unresolved insect phenomena could be identified and analysed.
Data availability statement
The original contributions presented in the study are included in the article/supplementary materials. Further inquiries can be directed to the corresponding author.
Ethics statement
The manuscript presents research on animals that do not require ethical approval for their study.
Author contributions
SK: Conceptualization, Formal Analysis, Investigation, Visualization, Writing – original draft. SO-G: Data curation, Investigation, Writing – review & editing. FP-F: Investigation, Writing – review & editing. KS-T: Data curation, Formal Analysis, Investigation, Writing – review & editing. AB: Conceptualization, Supervision, Writing – review & editing.
Funding
The author(s) declare financial support was received for the research, authorship, and/or publication of this article. This work was supported by the Flagship Research Groups Programme of the Hungarian University of Agriculture and Life Sciences.
Conflict of interest
The authors declare that the research was conducted in the absence of any commercial or financial relationships that could be construed as a potential conflict of interest.
Publisher’s note
All claims expressed in this article are solely those of the authors and do not necessarily represent those of their affiliated organizations, or those of the publisher, the editors and the reviewers. Any product that may be evaluated in this article, or claim that may be made by its manufacturer, is not guaranteed or endorsed by the publisher.
References
Anderson C. J., Tay W. T., McGaughran A., Gordon K., Walsh T. K. (2016). Population structure and gene flow in the global pest, Helicoverpa armigera. Mol. Ecol. 25, 5296–5311. doi: 10.1111/mec.13841
Bennett A. T., Cuthill I. C. (1994). Ultraviolet vision in birds: what is its function? Vision Res. 34, 1471–1478. doi: 10.1016/0042-6989(94)90149-X
Bentivenha J. P., Paula-Moraes S. V., Baldin E. L., Specht A., da Silva I. F., Hunt T. E. (2016). Battle in the new world: Helicoverpa armigera versus Helicoverpa zea (Lepidoptera: Noctuidae). PloS One 11, e0167182. doi: 10.1371/journal.pone.0167182
Byers J. A. (2006). Analysis of insect and plant colors in digital images using java software on the internet. Ann. Entomol. Soc Am. 99, 865–874. doi: 10.1603/0013-8746(2006)99[865:AOIAPC]2.0.CO;2
Chakravarty S., Padwal K. G., Srivastava C. P. (2020). Larval body colour polymorphism in Helicoverpa armigera (hubner) populations across India. Ind. J. Entomol. 82, 415–422. doi: 10.5958/0974-8172.2020.00093.0
Chang Y. C., Reid J. F. (1996). RGB calibration for color image analysis in machine vision. IEEE Trans. Image Process. 5, 1414–1422. doi: 10.1109/TIP.83
Clement A., Verfaille T., Lormel C., Jaloux B. (2015). A new colour vision system to quantify automatically foliar discolouration caused by insect pests feeding on leaf cells. Biosyst. Eng. 133, 128–140. doi: 10.1016/j.biosystemseng.2015.03.007
Czepak C., Albernaz K. C., Vivan L. M., Guimarães H. O., Carvalhais T. (2013). First reported occurrence of Helicoverpa armigera (Hübner) (Lepidoptera: Noctuidae) in Brazil. Pesq. Agr. Trop. 43, 110–113. doi: 10.1590/S1983-40632013000100015
Darvas B., Bánáti H., Takács E., Lauber É., Szécsi Á., Székács A. (2011). Relationships of Helicoverpa armigera, Ostrinia nubilalis and Fusarium verticillioides on MON 810 maize. Insects 2, 1–11. doi: 10.3390/insects2010001
Deepa M., Srivastava C. P. (2010). Biological characteristics of Helicoverpa armigera. Ann. Plant Prot. Sci. 18, 370–372.
De Moraes C. M., Mescher M. C. (2004). Biochemical crypsis in the avoidance of natural enemies by an insect herbivore. Proc. Nat. Acad. Sci. 101, 8993–8997. doi: 10.1073/pnas.0403248101
Dimitrov Y., Palagacheva N., Dimova M., Vitanova M., Rashev S. (2013). Multiplication of cotton bollworm (Helicoverpa armigera Hubner) favors the development of fungal pathogen’s corn. Ecol. Future. J. Agric. Sci. For. Sci. 12, 24–27.
Endler J. A. (1981). An overview of the relationships between mimicry and crypsis. Biol. J. Linn. Soc 16, 25–31. doi: 10.1111/j.1095-8312.1981.tb01840.x
Feng H. Q., Wu K. M., Cheng D. F., Guo Y. Y. (2004). Northward migration of Helicoverpa armigera (Lepidoptera: Noctuidae) and other moths in early summer observed with radar in northern China. J. Econ. Entomol. 97, 1874–1883. doi: 10.1093/jee/97.6.1874
Firempong S., Zalucki M. P. (1989). Host plant-selection by Helicoverpa armigera (Hubner) (Lepidoptera, Noctuidae) role of certain plant attributes. Aust. J. Zool. 37, 675–683. doi: 10.1071/ZO9890675
Fitt G. P., Dillon M. L., Hamilton J. G. (1995). Spatial dynamics of Helicoverpa populations in Australia: simulation modelling and empirical studies of adult movement. Comp. Electr. Agric. 13, 177–192. doi: 10.1016/0168-1699(95)00024-X
Futahashi R., Fujiwara H. (2007). Regulation of 20-hydroxyecdysone on the larval pigmentation and the expression of melanin synthesis enzymes and yellow gene of the swallowtail butterfly, Papilio Xuthus. Insect Biochem. Mol. Biol. 37, 855–864. doi: 10.1016/j.ibmb.2007.02.014
Futahashi R., Fujiwara H. (2008). Juvenile hormone regulates butterfly larval pattern switches. Science 319, 1061. doi: 10.1126/science.1149786
Futahashi R., Sato J., Meng Y., Okamoto S., Daimon T., Yamamoto K., et al. (2008). Yellow and ebony are the responsible genes for the larval colour mutants of the silkworm Bombyx mori. Genetics 180, 1995–2005. doi: 10.1534/genetics.108.096388
Hassan S. N., Rahman N. S., Win Z. (2014). Automatic classification of insects using color-based and shape-based descriptors. Int. J. Appl. Contr. Electric. Electron. Eng. 2, 23–35.
Hazel W. N. (2002). The environmental and genetic control of seasonal polyphenism in larval colour and its adaptive significance in a swallowtail butterfly. Evol 56, 248–342. doi: 10.1111/j.0014-3820.2002.tb01344.x
Hiruma K., Riddiford L. M. (2009). The molecular mechanisms of cuticular melanization: The ecdysone cascade leading to dopa decarboxylase expression in Manduca sexta. Insect Biochem. Mol. Biol. 39, 245–253. doi: 10.1016/j.ibmb.2009.01.008
Keszthelyi S., Nowinszky L., Puskás J. (2013). The growing abundance of Helicoverpa armigera in Hungary and its areal shift estimation. Centr. Eur. J. Biol. 8, 756–764. doi: 10.2478/s11535-013-0195-0
Kriticos D. J., Ota N., Hutchison W. D., Beddow J., Walsh T., Tay W. T., et al. (2015). The potential distribution of invading Helicoverpa armigera in North America: is it just a matter of time? PloS One 10, e0119618. doi: 10.1371/journal.pone.0119618
Lacotte V., Dell’Aglio E., Peignier S., Benzaoui F., Heddi A., Rebollo R., et al. (2023). A comparative study revealed hyperspectral imaging as a potential standardized tool for the analysis of cuticle tanning over insect development. Heliyon 9, e13962. doi: 10.1016/j.heliyon.2023.e13962
Lehtonen P. K., Laaksonen T., Artemyev A. V., Belskii E., Both C., Bures S., et al. (2009). Geographic patterns of genetic differentiation and plumage colo.ur variation are different in the pied flycatcher (Ficedula hypoleuca). Mol. Ecol. 18, 4463–4476. doi: 10.1111/j.1365-294X.2009.04364.x
Liu Z., Li D., Gong P., Wu K. (2004). Life table studies of the cotton bollworm, Helicoverpa armigera (Hübner) (Lepidoptera: Noctuidae), on different host plants. Environ. Entomol. 33, 1570–1576. doi: 10.1603/0046-225X-33.6.1570
Lowry D. B. (2012). Ecotypes and the controversy over stages in the formation of new species. Biol. J. Linn. Soc 106, 241–257. doi: 10.1111/bij.2012.106.issue-2
Mallet J., Joron M. (1999). Evolution of diversity in warning colour and mimicry: polymorphisms, shifting balance, and speciation. Ann. Rev. Ecol. Syst. 30, 201–233. doi: 10.1146/annurev.ecolsys.30.1.201
McColl R. W., Martin G. R. (1989). Compression of colour image data using histogram analysis and clustering techniques. Electron. Commun. Eng. J. 2, 93–100. doi: 10.1049/ecej:19890021
Nice C. C., Fordyce J. A. (2006). How caterpillars avoid overheating: Behavioral and phenotypic plasticity of pipevine swallowtail larvae. Oecologia 146, 541–548. doi: 10.1007/s00442-005-0229-7
Pedgley D. E. (1985). Windborne migration of Heliothis armigera (Hübner) (Lepidoptera: Noctuidae) to the British Isles. Entomol. Gaz. 36, 15–20.
Raabe M. (1982). “Morphological and Physiological Colour Change,” in Insect Neurohormones (Springer, Boston, MA). doi: 10.1007/978-1-4684-4013-3
Ramos V. E., Morallo-Rejesus B. (1976). Effects of nutrition on larval colouration of Helicoverpa armigera (Hübner). Philipp. J. Entomol. 3, 201–224.
Rodríguez-Morales D., Tapia-McClung H., Robledo-Ospina L. E., Rao D. (2021). Colour and motion affect a dune wasp’s ability to detect its cryptic spider predators. Sci. Rep. 11, 15442. doi: 10.1038/s41598-021-94926-7
Schwarz M. W., Cowan W. B., Beatty J. C. (1987). An experimental comparison of RGB, YIQ, LAB, HSV, and opponent color models. ACM Trans. Graph. 6, 123. doi: 10.1145/31336.31338
Sherratt T. N., Pollitt D., Wilkinson D. M. (2007). The evolution of crypsis in replicating populations of web-based prey. Oikos 116, 449–460. doi: 10.1111/j.0030-1299.2007.15521.x
Shirataki H., Futahashi R., Fujiwara H. (2010). Species-specific coordinated gene expression and trans-regulation of larval colour pattern in three swallowtail butterflies. Evol. Dev. 12, 305–314. doi: 10.1111/j.1525-142X.2010.00416.x
Thery M., Gomez D. (2010). Insect colours and visual appearance in the eyes of their predators. Adv. Ins. Physiol. 38, 267–353. doi: 10.1016/S0065-2806(10)38001-5
Virić Gašparić H., Vučemilović Jurić D., Bažok R. (2022). Assessment of a possible increase in the harmfulness of the cotton bollworm (Helicoverpa armigera Hubner) in Croatia. Entomol. Cro. 21, 1–9. doi: 10.17971/ec.21.1.1
Yamasaki A., Shimizu K., Eujisaki K. (2009). Effect of host plant part on larval body-colour polymorphism in Helicoverpa armigera (Lepidoptera: Noctuidae). Ann. Entomol. Soc Am. 102, 76–84. doi: 10.1603/008.102.0108
Keywords: colour analysis, crypsis, fully-developed larvae, Helicoverpa armigera, herbivore adaptive ability, image analysis, polymorphism
Citation: Keszthelyi S, Orsi-Gibicsár S, Pál-Fám F, Somfalvi-Tóth K and Balog A (2024) Colour polymorphism of cotton bollworm larvae as a function of the type of host plant providing its development. Front. Ecol. Evol. 12:1376435. doi: 10.3389/fevo.2024.1376435
Received: 25 January 2024; Accepted: 14 May 2024;
Published: 31 May 2024.
Edited by:
Lian-Sheng Zang, Guizhou University, ChinaReviewed by:
Xiaoling Tan, Chinese Academy of Agricultural Sciences (CAAS), ChinaHaneef Tariq, Niğde Ömer Halisdemir University, Türkiye
Copyright © 2024 Keszthelyi, Orsi-Gibicsár, Pál-Fám, Somfalvi-Tóth and Balog. This is an open-access article distributed under the terms of the Creative Commons Attribution License (CC BY). The use, distribution or reproduction in other forums is permitted, provided the original author(s) and the copyright owner(s) are credited and that the original publication in this journal is cited, in accordance with accepted academic practice. No use, distribution or reproduction is permitted which does not comply with these terms.
*Correspondence: Sándor Keszthelyi, keszthelyi.sandor@uni-mate.hu