- 1Coastal Bend Bays & Estuaries Program, Corpus Christi, TX, United States
- 2Department of Natural Resource Ecology and Management, Iowa State University, Ames, IA, United States
- 3Caesar Kleberg Wildlife Research Institute, Texas A&M University-Kingsville, Kingsville, TX, United States
- 4Wildlife Restoration Partnerships, Greenwich, NJ, United States
- 5Department of Ecology, Evolution, and Natural Resources, Rutgers University, New Brunswick, NJ, United States
Highly migratory shorebirds are among the fastest declining avian guilds, so determining causes of mortality is critically important for their conservation. Most of these species depend on a specific geographic arrangement of suitable sites that reliably provide resources needed to fuel physiologically demanding life histories. Long-term mark-resight projects allow researchers to investigate specific potential sources of variation in demographic rates between populations. Red Knots (Calidris canutus) occur in three relatively distinct regions across the northern Gulf of Mexico, and two of these areas have been experiencing episodic harmful algal blooms (red tide) with increased frequency in recent decades. Since knots are mostly molluscivorous during the nonbreeding season in the Gulf, they are potentially exposed to red tide toxins at high concentrations via their filter-feeding prey. We used long-term mark-resight data from Texas, Louisiana, and Florida (USA) to estimate apparent survival, and to assess the effects of red tides on survival of Red Knots. We also assessed effects of tracking devices deployed in conjunction with the projects over the years. While overall apparent annual survival rates were similar across the three locations (0.768 – 0.819), several red tide events were associated with catastrophically low seasonal (fall) survival in Florida (as low as 0.492) and Texas (as low as 0.510). Leg-mounted geolocators, but not temporary glued-on VHF tags, were associated with a reduction in apparent survival (~8%/year). Movement of knots between the three areas was rare and site fidelity is known to be high. Harmful algal blooms are predicted to increase in frequency and severity with climate change and increased anthropogenic degradation of coastal habitats, which may further endanger these as well as other shorebird populations around the world.
1 Introduction
Understanding demographic parameters is fundamental to monitoring and managing wildlife populations, but the highly migratory nature of many shorebird species makes estimation of these parameters distinctly challenging (Faaborg et al., 2010). Species may have broad or disjunct breeding ranges, geographically distinct nonbreeding populations, and rely differentially on migratory stopovers between the two. Being able to isolate parameters (and factors that may affect them) to specific populations requires knowledge of connectivity (Webster et al., 2002; Rushing et al., 2017), since consequences of factors affecting one part of the annual cycle can have carry-over effects on subsequent ones (Goss-Custard et al., 1995; Norris, 2005; Duijns et al., 2017). Survival rates of adults and post-fledged juveniles have been demonstrated to be the most consequential to population growth rates of several migratory shorebirds (Hitchcock and Gratto-Trevor, 1997; Calvert et al., 2006). For migratory shorebirds that use different geographic areas for discrete parts of their annual cycle, changes in habitat quality in any part of the cycle can have a strong effect on survival (Johnson et al., 2006; Duriez et al., 2012).
Coastal habitats worldwide have been degraded by human activities such as shoreline development, pollution, and freshwater diversions (Kennish, 2002), decreasing their capacity to support populations of migratory shorebirds (Fernández and Lank, 2006). Beyond direct losses, anthropogenic disturbance can be functionally equivalent to habitat loss or degradation by rendering sites unusable (Gill and Sutherland, 2000). Norris and Marra (2007) demonstrated that differences in habitat quality in one part of the annual cycle can have interseasonal effects on population dynamics depending on the strength of migratory connectivity. When connectivity is strong, further habitat loss from projected sea level rise is likely to result in bottlenecks with potential consequences to populations proportionately larger than the habitat loss itself (Iwamura et al., 2013).
Harmful algal blooms (HABs) occur in aquatic environments and can be considered extreme biological events resulting in major disruption to coastal ecosystems through complex food web dynamics (Landsberg et al., 2009). HABs have occurred in the Gulf of Mexico far back into recorded history (Magaña et al., 2003). They have increased in frequency and now occur commonly on the coasts of Texas/Mexico and western Florida (Hallegraeff, 1993, van Dolah 2000, Walsh et al., 2006; Brand and Compton, 2007; Tominack et al., 2020). Blooms in the Gulf of Mexico resulting in fish kills associated with the dinoflagellate Karenia brevis are typically known as “red tides.” The organism produces brevetoxin, a very potent neurotoxin that kills fish through absorption across gill membranes (Abbott et al., 1975) or consumption of toxic biota (Tester et al., 2000). These toxins can accumulate and result in mortalities of higher vertebrates directly and indirectly through food web dynamics (Landsberg et al., 2009). Filter-feeding molluscs – especially bivalves – readily accumulate brevetoxins in high concentrations (Bricelj et al., 2012; Van Hemert et al., 2022) and occasionally experience direct lethal effects, as well as sublethal effects that result in subsequent recruitment failure (Summerson and Peterson, 1990). However, most mollusk species survive exposure to brevetoxins, accumulating high concentrations of toxins that can then be ingested by consumers (Landsberg, 2002). In addition to effects from direct consumption, brevetoxin from lysed cells can reach extremely high concentrations that can persist in waters and sediments for several weeks after the bloom organism has dissipated (Pierce and Henry, 2008; Castle et al., 2013), exposing probe-feeding shorebirds to additional dosages through passive uptake. Despite strong evidence correlating bird mortalities with HABs (Van Hemert et al., 2021, 2022), data from experimental studies or laboratory examination of tissue samples are relatively scarce (Shumway et al., 2003). Impacts are likely underestimated due to depredation and decomposition of carcasses, and removal of carcasses through tidal action (Sutherland et al., 2012). Further, a lack of long-term demographic monitoring of affected avian species has confounded determination of population level effects, though a recent study found a relationship between HAB occurrence and survival in Gulf-wintering Piping Plovers (Ellis et al., 2021).
The Red Knot (Calidris canutus) is a Holarctic breeding shorebird comprising six currently recognized subspecies. In the Western Hemisphere, the C. c. rufa subspecies spends nonbreeding seasons in the southern US and neighboring Mexico, especially the states bordering the Gulf of Mexico (henceforth, the “Gulf”), the Caribbean, and several regions in South America from northern Brazil to Tierra del Fuego (Niles et al., 2008). Additionally, some knots wintering on the Pacific coast of southern Mexico (Oaxaca) south to Chiloé Island, Chile occur in Texas and Louisiana during migration – primarily during spring – and consist of both C. c. rufa and C. c. roselaari (Newstead, unpubl. data). Though the total population of knots that do this is not known, it is suspected to be considerably less than those wintering in the Gulf. Knots in the Gulf are concentrated primarily in three general areas: southwestern Florida, the barrier islands of Louisiana, and the coast of south Texas and Tamaulipas. These Gulf states are among the highest latitude wintering sites (~24° – 29° N) of the C.c. rufa subspecies, used not only during the extensive nonbreeding season but also for pre-migratory and post-breeding stages. Observations of marked individuals (Tuma and Powell, 2021, Newstead, unpubl. data) confirm high site fidelity to each of these locations, consistent with studies on other subspecies (Harrington et al., 1998; Leyrer et al., 2006; Buchanan et al., 2012; Musmeci et al., 2022).
Geolocator studies (Newstead et al., 2013, Newstead, unpubl. data) show that the Texas and Louisiana populations migrate almost exclusively through the interior of the North American continent rather than using sites along the Atlantic coast. The decline of more than 75% of the Atlantic Flyway rufa population over the course of two decades (Niles et al., 2008) prompted its listing as Endangered in Canada in 2007 (COSEWIC, 2007) and as Threatened under the US Endangered Species Act in 2014 (USFWS, 2014a). Recognition and understanding of the Gulf populations – particularly the Texas and Louisiana populations – have been relatively recent discoveries, and there has been no previous estimation of survival parameters that can be compared across the three locations. The Red Knot is considered primarily a molluscivore during the non-breeding season (van Gils et al., 2006; Baker et al., 2013). The species’ reliance on coquina clams (Donax spp.) when using Gulf beaches makes it particularly vulnerable to HABs and they have been observed exhibiting symptoms of neurotoxic shellfish poisoning during red tide events (DN, personal observation). Carcasses of knots encountered freshly dead or dying were found to have exceptionally high levels of brevetoxin in all tissues tested, with the highest levels in the liver and gastrointestinal tract (Rafalski, 2012).
New tracking technologies continue to contribute major breakthroughs in our understanding of avian life histories (Bridge et al., 2010; Robinson et al., 2010; Wilmers et al., 2015). The use of archival light-level data loggers (geolocators), radiotransmitters, GPS and cellular technologies has drastically expanded our understanding of migratory strategies and revealed previously-unknown sites of essential importance (Stutchbury et al., 2009; Newstead et al., 2013; McKellar et al., 2015; Chan et al., 2019). While these discoveries have been critical in directing further research and conservation actions to places that can best benefit the species, the effects of tracking devices on the movements, activities, and, ultimately, survival of tracked animals remains a source of concern (Barron et al., 2010; Elliott et al., 2012; Scarpignato et al., 2016). Meta-analyses on device effects on birds (survival, behavior, reproductive success and others) have revealed some significant negative consequences varying by species, device type, attachment method, migration distance, and many other factors (Barron et al., 2010; Costantini and Møller, 2013). Specific to shorebirds, most studies have reported no significant impact of leg-mounted geolocators based on metrics from the year following deployment (Conklin and Battley, 2010; Pakanen et al., 2015; Mondain-Monval et al., 2020). Reductions in one-year return rates were detected for only two of 23 Arctic-breeding shorebird populations carrying geolocators relative to individuals carrying only a unique leg marker, with no detectable effect on the Great and Red knots included in the analysis (Weiser et al., 2016). However, Pakanen et al. (2020) found that when they extended their analysis of Dunlin (C. alpina) tracked over multiple years, apparent survival was lower for birds carrying geolocators compared to those without. These findings suggest that negative effects may accumulate over time or result in incremental increases in mortality risk. When possible, longer-term datasets should be analyzed to determine consequences that may not be evident based on one-year return rates alone. Small VHF transmitters attached to birds tracked using direct or automated radiotelemetry have also yielded important findings for many shorebirds, especially for local movements (Green et al., 2002; Warnock and Takekawa, 2003; Rogers et al., 2006; Duijns et al., 2019). Most VHF tag deployments on shorebirds have utilized an adhesive to affix the transmitter to the back, which subsequently falls off the bird with the next molt cycle or sooner, and these studies have generally reported no short-term survival consequences (Drake et al., 2001; Barron et al., 2010; Buchanan et al., 2019; Stantial et al., 2019).
Annual survival is a key underlying demographic parameter that can vary with environmental conditions, and strongly influences population trends. When data are sufficient, annual survival can be apportioned into partial (e.g. seasonal or semi-annual) components, providing greater insight into what particular locations or processes are contributing to demographic change (Gauthier et al., 2001; Leyrer et al., 2013; Piersma et al., 2016; van Irsel et al., 2022). We used mark-resight data from three projects involving captures of Red Knots in the three main Gulf of Mexico wintering areas to compare annual (and seasonal when possible) survival rates between populations, and to assess effects of an increasingly prevalent coastal ecosystem stressor (HABs) and the use of tracking devices on survival.
2 Methods
2.1 Study area
The northern Gulf of Mexico is bordered by a fairly contiguous extent of sandy beaches punctuated by passes connecting to bays and other receiving waters. Sediment grain size composition and origin (biogenic and terrigenous) vary widely across the Gulf, which affects the character of benthic infaunal communities and consequently the distribution of shorebirds that use them. Red Knots occur regularly in three primary areas across the Gulf – Florida, where they are most concentrated in the southwestern region between Clearwater and Marco Island; Louisiana, where they occur on the beaches of Grand Isle and the adjacent Caminada Headlands as well as the offshore barrier islands of the Breton Island National Wildlife Refuge; and Texas, where they are most common on the southern half of the coast from the Corpus Christi area to the border with Mexico, and likely well into contiguous parts of Tamaulipas where habitat is very similar. These three main areas are at least 600 km from one another and are considered as separate population units for the purpose of recovery planning (USFWS, 2021). These three geopolitical states are henceforth referred to as “locations” to avoid potential confusion with conditional states related to the analysis.
2.2 Field methods
For this project, captures of Red Knots occurred in Texas on Mustang and North Padre Islands between October 2009 – October 2019, in Louisiana on Grand Isle and the Caminada Headlands from the eastern end of Elmer’s Island west to Port Fourchon between April 2014 – April 2019; and in Florida from Longboat Key to Sanibel Island between October 2005 – March 2010.
All Red Knots were captured using a cannon-net (~ 9 m X 9 m, or ~10 m X 25 m) on beaches where birds were foraging or resting. Standard processing included a federal metal band on tarsus or tibia, a uniquely inscribed alphanumeric green flag on the opposite tibia, measures of bill and total head length (nearest 0.1mm), flattened wing chord length (mm), and mass (grams). A clip of the distal portion of the 6th primary covert was retained from most captured birds for isotopic analysis (carbon, nitrogen, hydrogen isotopes; for a project to assign migrants to wintering sites), and a blood sample was taken by brachial venipuncture on a smaller sample of birds for future genetic analysis.
In Florida, capture effort was concentrated between November – March (>95% of all captures) between years 2005-2010. Capture effort in Texas was mostly focused on fall and spring periods (>90% of all captures between September-November, or April-May) with smaller catches in other months, between 2009-2019. Louisiana captures were all in April, from 2014-2019. The distribution of resights by month was similar to that of the captures, except for Florida when many resights were recorded in months before and after the main winter months which constituted the bulk of the capture efforts.
Multiple tracking projects were conducted during the course of the projects. Archival light-level dataloggers (henceforth, “geolocators”; British Antarctic Survey [BAS] Model MK10 and MK12 or Migrate Technologies Intigeo W65) were mounted on leg flags and attached to the tibiotarsus as described in Niles et al. (2010). All assemblies weighed < 1.4 g. Radiotelemetry studies in Louisiana and Texas included deployment of small VHF transmitters (Lotek NTQB-4-2, 0.9 g) glued to the intrascapular region, as described in Newstead (2014).
2.3 Encounter histories and covariates
Encounter data were compiled from multiple resight projects and public domain records in bandedbirds.org; additional records were made available directly to the author. Encounter occasions began with the first capture effort in Florida in winter 2005/6 and ended in winter 2019/20 season.
Only records from Florida, Louisiana and Texas were used to build encounter histories. Birds were assigned to one of the three locations based on their original capture location. If an individual was encountered outside the location of initial capture (i.e., in one of the other two locations) and there were no subsequent records within the capture location it was removed from the dataset. This eliminated only a small number of birds from the dataset that may have switched wintering location or underwent atypical migrations.
Resightings were divided into three encounter occasions per year: the fall encounter (July 20 – October 31; 104 d; midpoint September 9), winter encounter (December 15 – January 31; 48 d; midpoint January 7), and spring encounter (April 1 – May 30; 60 d; midpoint May 1; Figure 1). Based on the midpoints of the encounter occasions, the year was thus divided into three intervals: (fall to winter – 120 d; winter to spring – 114 d; spring to fall – 131 d). These are referred to as the fall, winter, and summer intervals, respectively. The time range from the earliest captures to the most recent encounters spans 43 occasions (42 intervals).
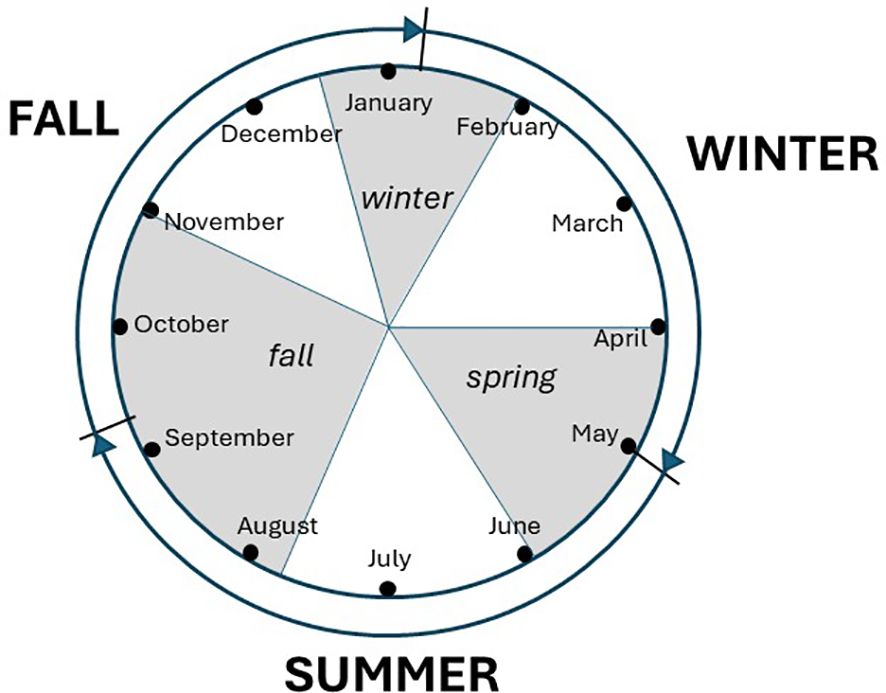
Figure 1 Encounter periods (grey), occasion midpoints (lines extending from outer circle), and season interval names for apparent survival analysis on Red Knots in the northern Gulf of Mexico.
As defined, the intervals generally reflect distinct and important phases in the annual cycle: during “fall” birds are returning from the Arctic and undergoing a body molt including flight feathers; during “winter” birds are managing a balance of predation risk, prey resource availability and maintaining sufficient fat reserves; during “summer” adult birds undertake a major migratory journey to Arctic breeding grounds, spend two to three months attempting to breed, and then return to nonbreeding areas. While juvenile birds nearly all remain on nonbreeding areas in their first full summer, they are exposed to factors such as extreme heat and increased human disturbance that adults mostly escape.
Individuals were grouped into one of three age classes based on age at capture. Birds that were not aged upon capture were classified as “unknown” age. Birds classified as hatch-year prior to, or second-year during, a spring occasion were classified as juvenile. Birds aged as second-year or after-hatch-year following a spring occasion (i.e., they had survived the first full oversummer interval so were > 1 year old), and all birds aged as after-second-year were classified as adult. Juveniles and birds of unknown/unspecified age were assumed to recruit into the adult age class following the first summer interval.
Since occasions are assumed to be instantaneous, the initial occasion for birds captured during intervals was assigned to be the subsequent occasion, so that estimates would not be biased by partial interval effects.
We included covariates in the dataset to test whether negative effects of tracking devices resulted in lower apparent survival. Effects of leg-mounted geolocators and glue-on VHF transmitters were assessed using a set of time-varying binary covariates for each. Once deployed, an individual with a geolocator was assumed to retain the geolocator permanently unless it was removed. VHF transmitters glued to the intrascapular region typically fall off within a few months of deployment, so the covariate was applied for only the subsequent interval.
Since HABs (especially “red tides”) have been observed to result in direct mortality to Red Knots, we hypothesized that exposure to toxins could result in lower apparent survival either through additional (undetected) direct mortality or sublethal effects. Effects of red tide were assessed using several approaches. Red tide sampling occurs in Florida (inshore and offshore) with good spatial and temporal coverage through the HABSOS system (NOAA National Centers for Environmental Information, 2014). The monthly bloom severity index (BSI) developed by Stumpf et al. (2022) was used to identify intervals when red tide blooms were affecting the southwest Florida coast. Red tide effects can occur at relatively low concentrations, but generally begin having pronounced effects resulting in fish kills at concentrations >1,000,000 cells/L. The summed BSIs for months corresponding to intervals in this study were used to classify red tide as absent/minimal (summed BSI = 0, covariate = -1), moderate (summed BSI > 0 but < 5, covariate = 0), or severe (summed BSI > 5, covariate = 1). In Texas, red tide monitoring is conducted mostly in response to known or suspected occurrences. Since events vary greatly in their range and extent of impact to marine life, fish kill reports were used as a secondary source to confirm an event to a degree that would have resulted in high likelihood of the shoreline being exposed to the effects of the bloom. For Texas, red tide events were ascribed to seasons based on Tominack et al. (2020), and severity was assigned as appropriate to the geography utilized by knots. A covariate set was thus created for each location based on red tide being absent/minimal, moderate, or severe (-1, 0, and 1, respectively) during each interval. A covariate set including all red tide events was made for each location separately, and another that included all locations together (but the red tide covariates applicable to each location separately).
Because knots are highly mobile and likely vary in their degree of exposure to harmful algal blooms depending on various environmental factors, we also tested the effect of each individual red tide season against all others. Separate covariate sets were created for each red tide season occurrence in Florida and Texas to assess the effect of red tide events independently. The covariate value of 1 was assigned to intervals when red tide was present (either moderate or severe), and 0 for all others. Based on our criteria, there were a total of 17 and 6 red tide season events for Florida and Texas, respectively, applicable to the 42 intervals of the study, so a covariate set was created for each of these.
2.4 Statistical analyses
Models were evaluated using a Cormack-Jolly-Seber (CJS) framework in Program MARK (v. 9.0, White and Burnham, 1999) to estimate apparent survival (φ) and encounter (p) probabilities. Apparent survival is the probability that a knot alive at occasion i was alive and in the study area at occasion i + 1. Its inverse includes mortality and permanent emigration from the study area. Goodness-of-fit testing was run on the fully time-varying model and contingency tables were examined individually to assess whether patterns indicated lack of independence in the data. The median ĉ approach was applied to account for overdispersion in all subsequent models. Model evaluation was based on quasi-Akaike’s Information Criterion adjusted for sample size (QAICc) and model weights (ωi). We built models in an ordered 3-step process described below.
2.4.1 Step 1: determining best underlying model structures
Preliminary evaluation of the dataset indicated major differences in the distribution of encounters between locations and seasons, so model fitting began with a series of models holding φ constant by location and allowing for variation in p by location, season, and age. Using the best parameter structure for p, models incorporating variability in φ by location, season and age (and combinations thereof) were then tested to determine the best fit for a base model. Models in which covariate parameters were poorly estimated (standard errors of effect coefficient very close to zero or greater than 2.0) were removed from the resulting model set. Models within 2 ΔQAICc of the top model were considered well-supported, and the top model was carried forward for testing of the time-varying covariate datasets.
2.4.2 Step 2: building a candidate model set with red tide index and tracking device effects
We then built a candidate set of models that included covariates added to the most competitive base model. We considered the effect of tracking devices (geolocators, VHF transmitters) independently as well as combined. Given differences in habitat distribution and the character and duration of red tide events between Texas and Florida, we considered the effect of red tide on each location modeled independently, as well as together. We then considered models that included both tracking device and red tide effects. Covariates were considered predictive if the 95% confidence intervals (C.I.) of effect coefficients did not include zero. Apparent survival and encounter probabilities were reported based on the top model that did not include a red tide effect. If all parameters were well estimated in a model including seasonal variation within a location, the model including those terms and the tracking device effects was used to estimate those season-specific parameters (i.e. to provide estimates unaffected by tracking devices). To facilitate comparison with other studies, apparent seasonal survival (φ a) estimates and 95% C.I.s were converted to apparent annual estimates using the delta method (Powell, 2007), either as a product of the three separate seasonal estimates or exponentiation of the non-season specific estimates.
2.4.3 Step 3: evaluating survival in specific red tide seasons
To evaluate the effect of specific red tide events, we used the most competitive base model and independently added each red tide season to the model as applicable to each location. We considered a red tide event to be poorly estimated if its inclusion resulted in other parameters being poorly estimated. Red tide events (seasons) were considered significant if the 95% C.I.s of the effect coefficient did not overlap zero. For significant seasons, the magnitude of the effect on φ was calculated as the percentage difference between the mean estimate of the survival probability in that season relative to the survival probability of all other seasons for that location.
To estimate survival for each significant red tide season, we ran a post-hoc model treating each of those seasons individually and accounted for any significant tracking device effects. If any coefficient became non-significant in this model, that covariate was removed and the reduced model run until all terms were significant.
The strength of differences between locations was assessed by whether 95% C.I.s overlapped, and covariate effects were assessed by whether the 95% C.I. included zero. C.I.s are presented in brackets following the mean, unless otherwise noted.
3 Results
Encounter histories were constructed from 2,412 knots (Florida: 1,373 captured between 2005-2010; Louisiana: 255 captured between 2014-2019; Texas: 784 captured between 2009-2019), and 4,078 resights (Florida: 3,013; Louisiana: 188, Texas, 877; Supplementary Table 1). Geolocators were deployed on 68, 49, and 114 knots in Florida, Louisiana and Texas, respectively. VHF transmitters were deployed on 18 and 115 knots in Louisiana and Texas, respectively.
There were 17 red tide seasons in Florida (8 severe, 9 moderate) during the 42 intervals since marking began. Two were in summer (one moderate, one severe). Both summer events preceded severe fall events. Of nine fall events (three moderate, six severe), six persisted into the subsequent winter interval. There were no winter events that were not preceded by a fall red tide event. In Texas, there were 6 red tide seasons (3 severe, 3 moderate) during the 31 intervals since marking began. All Texas red tide seasons were in fall.
3.1 Best underlying model structures
The goodness-of-fit test indicated some overdispersion in the data but examination of contingency tables did not suggest any systematic source of bias. Differences in resighting effort (p) between years and locations were likely responsible for high model deviance. Subsequently, all models were adjusted using median ĉ = 1.155. The best models for the encounter parameters included location and season. All models including age resulted in multiple parameters being poorly estimated, so these were removed from further consideration. All subsequent model runs utilized the p(location, season) parameterization.
The top base model for explaining variation in Red Knot apparent survival included a constant seasonal survival term (φ c) for each location. A competing model allowed for season-specific (φ f, φ w, φ s) parameters for Florida, but not for Texas and Louisiana. A model with constant seasonal survival across locations received the lowest model weight of the three. The two most competitive models were carried forward for model development incorporating HAB and tracking device covariates.
3.2 Assessment of candidate models including red tide index and tracking device effects
All models testing tracking device and red tide effects on the base model that included seasonal variation in survival in Florida had uniformly higher QAICc than the corresponding models based on the constant seasonal survival base model. Since the inclusion of variation in seasonal survival in Florida did not improve model fit in any case, these models were removed from the candidate model set.
The best fitting model included effects of geolocators and red tide in Florida (Table 1). The four top models each had a likelihood >0.125 (indicating support; Burnham and Anderson, 2002), and all included the geolocator covariate. The geolocator effect was negative and significant in all models that included it. VHF transmitter and red tide covariates were also all negative but non-significant when included in the models. Multiple parameters were poorly estimated in all models that included red tide in Texas only. The effect of geolocator in the top-ranked model without a red tide effect ( = -0.445 [-0.655, -0.236]) equates to an estimated reduction in seasonal apparent survival of 4.1%, 3.2%, and 3.8% for Texas, Louisiana, and Florida, respectively.
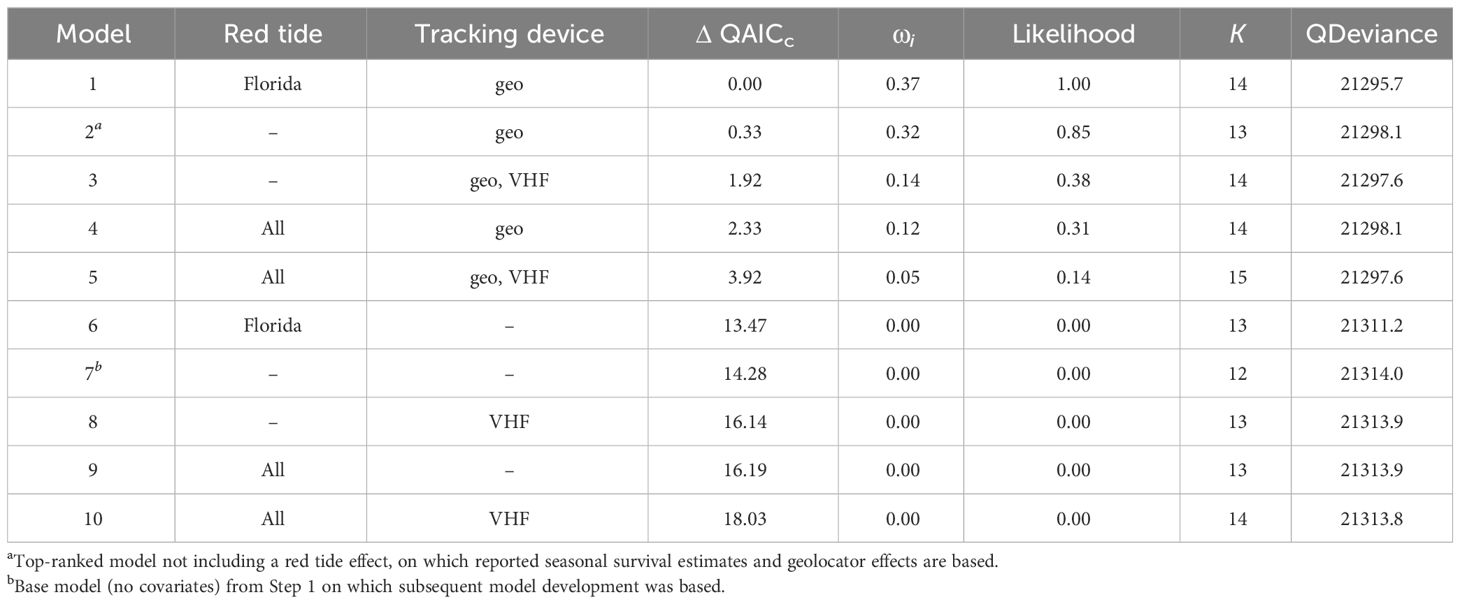
Table 1 Model ranking including combinations of red tide and tracking device covariates applied to the best-fitting base model (Φ location, p location, season) for Red Knots from Texas, Louisiana, and Florida populations from 2005-2019.
The top-ranked model that did not include a red tide effect was used to estimate apparent survival for each location. With tracking devices accounted for separately in the model, mean apparent seasonal survival was highest for Louisiana, intermediate in Florida, and lowest in Texas, though C.I.s overlapped (Table 2). Resighting probabilities varied between seasons within each location.
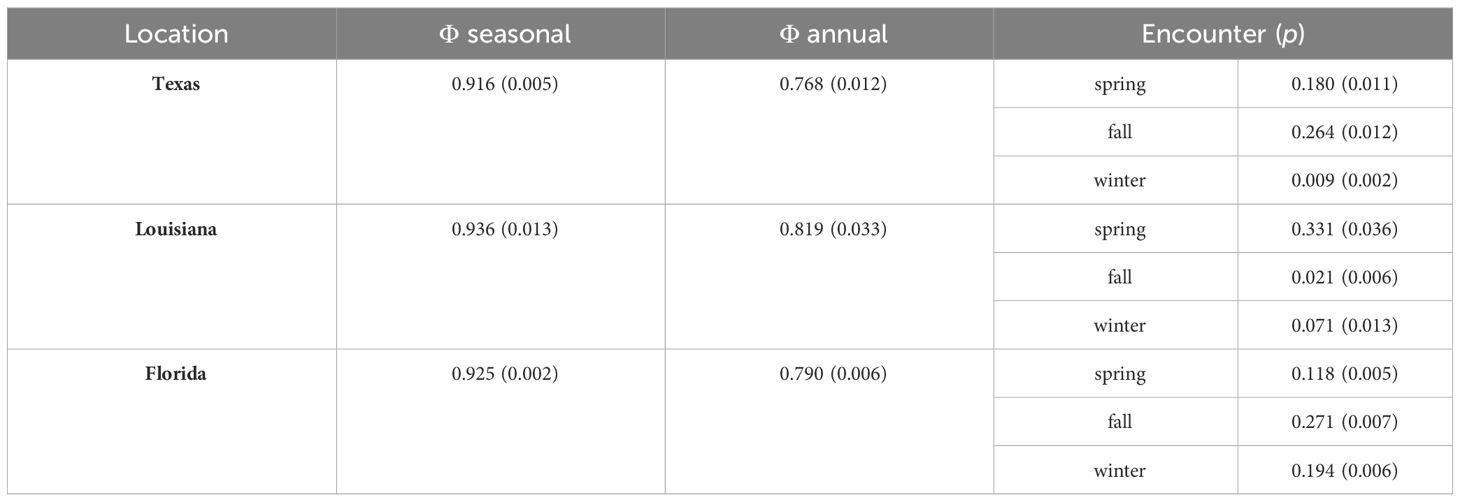
Table 2 Mean estimates and standard errors (SE) for apparent seasonal and annual survival and encounter probabilities of Red Knots for each location from the φ(location, geolocator) p (location, season) base model.
Estimation of distinct seasonal apparent survival probabilities was only possible for Florida. When seasonal variation for Florida was added to the top-ranked model, mean apparent survival was highest during winter (0.944 [0.915, 0.963], intermediate in fall (0.914 [0.834, 0.957] and lowest in summer (0.907 [0.821, 0.954]), though C.I.s were wide and overlapping.
3.3 Individual red tide season effects
Parameters were estimable for models including individual red tide seasons on the base model for one (of six) Texas seasons, and nine (of seventeen) Florida seasons (Table 3). The 2009 fall red tide season in Texas was significant ( = -2.515 [-3.291, -1.739]), as were four total seasons in Florida comprising two extended events in 2012 (fall: ( = -1.553 [-1.742, -0.764]; winter: ( = -1.470 [-1.930, -1.010]) and 2018 (fall: ( = -2.504 [-3.169, -1.840]; winter: ( = -1.831 [-2.817, -0.845]). Red tide seasons with non-significant terms had higher standard errors, indicating data was insufficient to estimate an effect.
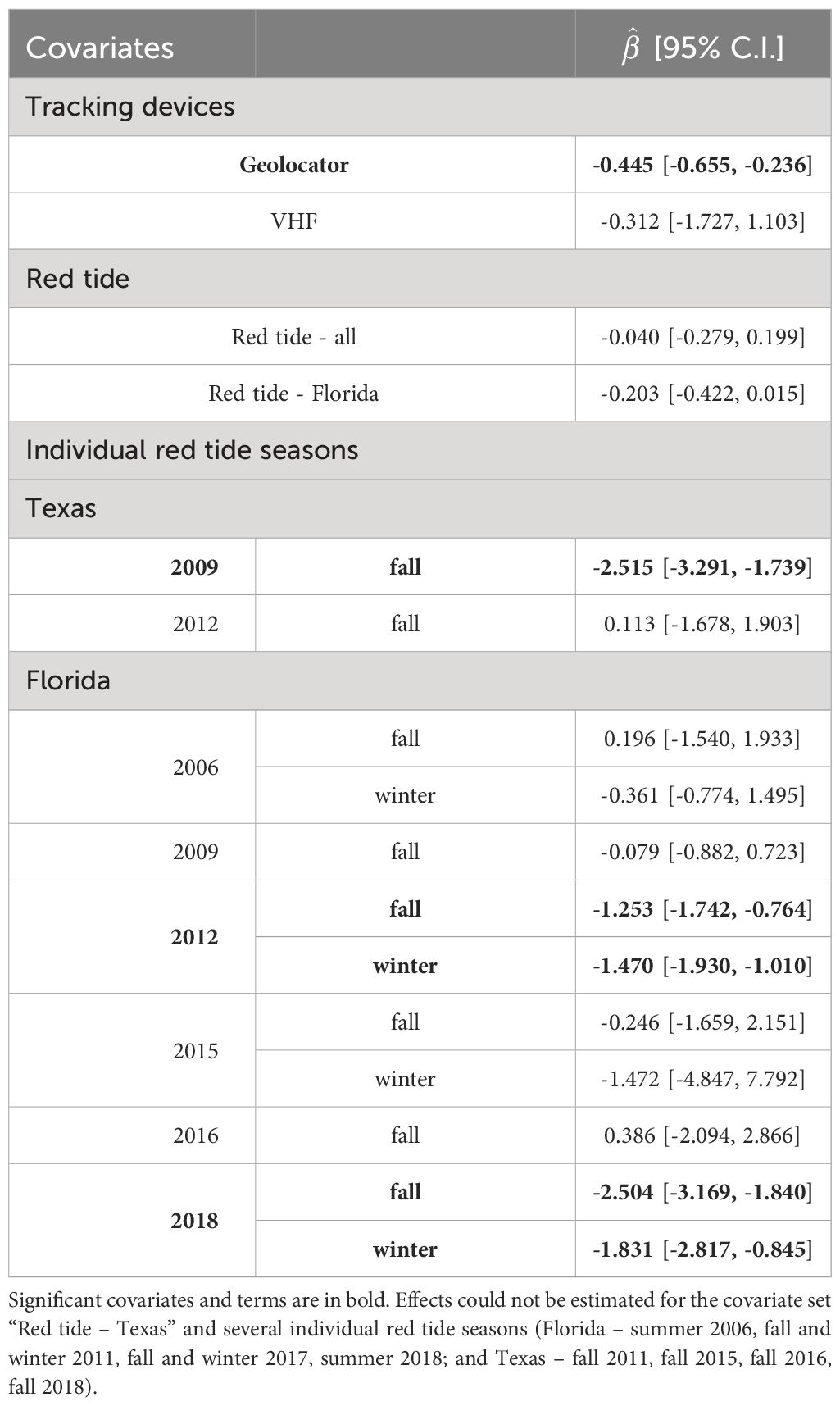
Table 3 Effect coefficients () and 95% confidence intervals for covariates tested individually on the Φ (location) p (location, season) base model for Red Knot apparent survival in the northern Gulf of Mexico.
The post-hoc model retaining all significant covariates included the geolocator effect and four of the five significant red tide seasons (Table 4). Point estimates of seasonal survival during red tide events in Florida ranged from 0.492 (fall 2018) to 0.884 (fall 2012). Seasonal survival during the Texas fall 2009 red tide was 0.510.
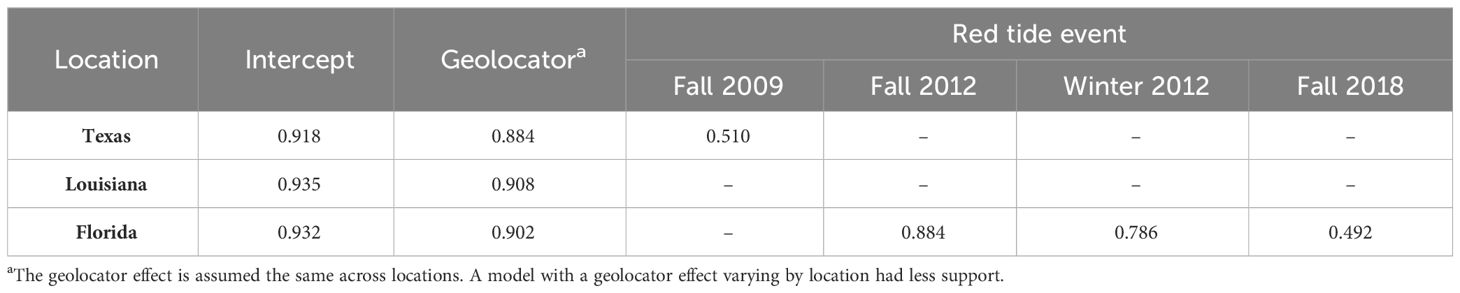
Table 4 Seasonal apparent survival estimates of Red Knots in each location based on the highest-supported post-hoc model incorporating five significant covariates – geolocators, and the four red tide events as applicable to the affected location.
4 Discussion
Our results confirm episodes of sharply reduced survival of Red Knots during red tide events, and suggest this could be a significant driver of survival in Texas and Florida. While only a red tide effect in Florida was included in the top model of the candidate set, tests on individual seasons – when all parameters were estimable – were all either strong and significant, or were weak with relatively high standard errors. This is indicative of sparseness of data in some seasons (especially low winter resight probability in Texas) which likely resulted in a failure to find an effect when one may have occurred. Instead of chronically lower annual survival, knots in these locations may be experiencing relatively high survival punctuated by acute episodes of high mortality from red tide.
Several studies on knots have demonstrated often sharply contrasting survival estimates comparing different time series (Baker et al., 2004; González et al., 2006; Leyrer et al., 2013), population segments (Harrington et al., 1998) and body condition (McGowan et al., 2011), and age (Schwarzer et al., 2012). A robust model accounting for transience, temporary emigration, persistence and food availability at a stopover site illustrated that many different processes can affect estimates of apparent survival over short timeframes (Tucker et al., 2021). Further, the focal populations of these studies often preclude simple comparison of survival estimates across studies. For example, knots captured in Delaware Bay during spring migration are primarily breeding age individuals who have already survived nearly two full years during which mortality is expected to be highest (and thus unaccounted for in estimates), whereas estimates based on populations that included those younger cohorts (including ours) would be expected to be lower. Nevertheless, our estimates of apparent annual survival rates of Red Knots from the three Gulf of Mexico locations were within the ranges of those reported by most other studies on rufa Red Knots. Of the three Gulf locations, mean apparent annual survival was lowest in Texas and highest in Louisiana, though differences were not significant.
An effect of age on survival was not detectable in our models, but we note that the first occasion a knot becomes “available” to our study sites follows a critical and typically very high-mortality time interval following hatching in the Arctic, including surviving to fledging and the first southbound migration (~first 3 months of life). However, we are aware of no published survival estimates for this species which include that highly sensitive period. Accurate estimation of age-specific survival in the first- and second-year periods (prior to the first return to the Arctic as a breeder for most knots) was likely related to limitations in data for these age groups.
Our study estimated apparent survival, which is the complement of both mortality and permanent emigration. These are the first published survival estimates for knots in Texas and Louisiana, but a relatively recent study examined true survival in Florida. Between 2005-2010, true annual survival of Florida-wintering knots was estimated at 0.89 for adults and 0.95 for juveniles, using a Barker model (Schwarzer et al., 2012). The Barker model accounts for emigration and re-immigration based on encounters in a secondary encounter area (in this case, James Bay, Ontario, and the US Atlantic coast), resulting in annual survival estimates that separate the two processes by which an individual can leave the population (mortality or permanent emigration). Our dataset encompasses the same individuals and years of the Schwarzer et al. (2012) study, but because of the use of different modeling approaches and longer timespan of our study, we would not expect our estimates to be consistent. However, comparison may provide some insight into the potential population dynamics of the Florida winterers. We explore two potential explanations, which are not mutually exclusive: 1) during the course of the past decade the survival rate has in fact declined since the Schwarzer et al. (2012) study; and, 2) more knots formerly associated with Florida wintering areas are spending extended periods of time or the full nonbreeding period at sites along the southeast US coast, or into the Caribbean.
The significant reduction in survival associated with several red tide events in Florida provides some support for the hypothesis that mean survival rates truly have declined particularly in the past decade. It must be noted that because there were no new birds marked in Florida beyond 2010 in this analysis, it is possible that an age-related effect (i.e. senescence) could have depressed our apparent survival rates. However, the five-year timespan of the Schwarzer et al. (2012) study encompassed only four seasons (two events) that met our criteria as moderate or severe in terms of BSI. Three of these were the contiguous summer-fall-winter seasons during the bloom of 2006-7 (two of those were moderate severity), and the other was the brief and moderate bloom of fall 2009. By contrast, red tide occurred in thirteen seasons over the subsequent decade. Each bloom affected multiple consecutive seasons (including the one beginning in fall 2017 that lasted well over a year and a half), potentially compounding the effects. The years assessed in the Schwarzer et al. (2012) study (the same as the first five years of ours) represent a relative lull in red tide frequency and severity in Florida compared to the latter decade included in our study.
There is also evidence that our apparent survival estimates for Florida could be lower because of permanent shifts in wintering range outside of Florida. Lyons et al. (2018) estimated the wintering population of the southeast US (including Florida) at 10,400 individuals using data from the fall migration in 2011, while surveyors conducting the International Piping Plover Census (Elliott-Smith et al., 2015) counted 5,069 Red Knots during the 2006 count and approximately 3,900 in 2011. These numbers are not directly comparable, as they are based on different methodologies, but they reflect uncertainties as to where specifically Red Knots are wintering in the southeastern U.S. While there are not consistent repeated estimates from each location within this region over that time, resight data indicates that some birds have indeed shifted from the Florida wintering group to the Atlantic coasts of Georgia and South Carolina (USFWS 2014b, Pelton et al., 2022). The parameter estimates for fidelity and re-immigration based on the Barker model used by Schwarzer et al. (2012) indicate some support for this hypothesis. The apparent survival estimates for Florida in this study confound permanent emigration (such as a shift in wintering area from Florida to Georgia/South Carolina) with mortality, so it is possible that some portion of the decrease in apparent survival was attributable to emigration.
Apparent survival estimates for the Texas and Louisiana populations from this study could also be biased low (relative to true survival), if some proportion of those birds had also shifted to other wintering sites. However, there is currently no solid evidence to support this, and relatively minimal exchange of individuals even between the locations suggests it is unlikely.
The four significant red tide seasons in Florida were actually two prolonged events that lasted through the fall and winter intervals of the 2012 and 2018 nonbreeding season, compounding the effect on annual survival. In those years, estimated annual survival (assuming mean of non-red-tide survival for the unaffected season) would have been ~0.56 (in 2012) and ~0.33 (in 2018). While the 2009 red tide in Texas primarily affected one season (fall), it was severe enough that annual survival would have been ~0.43. These estimates indicate the loss of large proportions (~44 – 67%) of the entire population in a single year. Though there is no fixed quantitative threshold of a “catastrophe” in population dynamics, certainly the scale of these losses for a K-selected species are alarming. Simulation studies have demonstrated that population trends tend to be depressed when variability in survival is high, relative to a population where it is low, given the same arithmetic mean of survival (Boyce, 1977; Hitchcock and Gratto-Trevor, 1997). Indeed, catastrophic events, especially when combined with other environmental stressors, can drastically accelerate negative population growth rates towards extinction in closed populations (Simberloff, 1988). In this case, the effect of catastrophes on one wintering population may be tempered somewhat depending on the degree of migratory connectivity between breeding and wintering areas. As the processes by which young Red Knots recruit into a particular wintering population remain poorly understood, it is not clear that high recruitment could offset low survival years to stabilize a wintering population over the long term. Population declines documented in other wintering areas for C. c. rufa suggest a negative long-run population growth rate, and our results indicate red tides could be contributing to very high variability in Red Knot survival, at least in the Texas and Florida populations. Under these conditions, populations become more vulnerable to extinction especially when the frequency and magnitude of random catastrophes are increasing (Lande, 1993).
Sparse data (low encounter probability) for certain seasons in some locations likely resulted in the inability to fully estimate parameters for multiple red tide events, but is it possible that birds are able to avoid red tide effects in some years, but not in others? Knots could potentially reduce their exposure to toxins either through a shift in prey selection, or a shift in range.
There is evidence that some shorebirds avoid prey with high concentrations of algal toxins. Black oystercatchers (Haematopus bachmani) shifted diet to prey items that did not harbor algal toxins when those toxins were present in sea mussels – their preferred prey – and discarded mussel tissue with high toxin concentrations when they did capture it (Kvitek and Bretz, 2005), while other shorebird species tended to avoid areas where toxins were present. Red knots, however, consume bivalve prey whole and crush it in their gizzard rather than removing the flesh first (which would provide an opportunity to taste and reject), potentially making them more susceptible to accumulate high amounts of toxin. A prey selection mechanism to reduce exposure would only be viable if a suitable non-toxic alternate prey source were available. On the Gulf-facing beaches, Donax spp. is by far the dominant bivalve mollusk that is most likely to occur in ample densities to support knots, and it is known to concentrate HAB toxins at extremely high levels (Cummins et al., 1971). It is also possible that red tides could affect birds by negatively affecting recruitment of their bivalve prey (Summerson and Peterson, 1990; Rolton et al., 2016), which might have both immediate and long-term effects. A study comparing two red tide outbreaks (2006 and 2011) on beaches of south Texas found that one event resulted in a near complete die-off of the benthic macrofauna while that same faunal community was virtually unaffected in the other event, despite extensive fish-kills occurring in both (Lerma, 2013).
As discussed previously, permanent emigration of birds from the Florida wintering population to another site in the southeast US would be one way to avoid red tide effects. However, avoidance may not require permanent emigration. Since red tides most commonly occur during fall months, simply prolonging a southeast US stopover before moving on to Florida could reduce the degree of exposure. The abundance and duration of knots stopping at the Altamaha River delta (Georgia) varies between years and is likely influenced by availability of the dwarf surf clam (Mulinia lateralis; Lyons et al., 2018), so “good years” at this site might reduce the proportion of birds arriving in southwest Florida to toxic conditions, at a time when they are already under high physiological stress due to the demands of molt which is coupled with decreased immunological function (Buehler et al., 2008). If knots stay in the southeast US long enough to complete their molt, they would also likely arrive in better condition. There is isotopic evidence that some knots in the Florida wintering population do in fact complete their molt prior to arrival in Florida (Newstead, unpubl. data). Staying longer further north would also reduce the risk of exposure to tropical storms during the peak of hurricane season (Niles et al., 2012).
In Texas, knots are known to utilize the extensive tidal flats of the Laguna Madre when water levels allow (Newstead, 2014), and when red tides do occur, they tend to be most severe and extensive on the Gulf beach, only occasionally affecting the Laguna Madre. Also, the Laguna Madre complex and the interspersed flats of the Rio Grande Delta extend over 400 km from Corpus Christi, Texas southward to La Pesca, Tamaulipas, Mexico. Aerial radiotelemetry documented that knots move extensively throughout this system during the nonbreeding season (Newstead, 2014), so they could potentially avoid red tide effects by moving to unaffected parts of the same extensive system.
Red tides typically occur beginning in late summer and often persist until early to mid-winter, though in the past decade some events have been initiated or prolonged into the spring and summer seasons (Brand and Compton, 2007; Stumpf et al., 2022). Comparing models allowing seasonal variation in survival for Florida, estimates were lower in all seasons when red tide was not included as a covariate, but within all models season-specific estimates were lower in summer relative to fall and winter. This suggests that, absent red tide, survival in Florida during the extensive nonbreeding period is higher relative to the breeding period, which includes lengthy round-trip migrations for breeding adults. This finding is in contrast to Leyrer et al. (2013) for C.c. canutus wintering at Banc d’Arguin in Mauritania, where survival during the migratory and breeding seasons was close to 1.0, with most mortality occurring on the wintering area. Banc d’Arguin, at roughly 20.5 N latitude, is extremely arid and hot even during the boreal winter. Leyrer et al. (2013) suggested that during the period following arrival from breeding grounds, environmental and interspecific competitive constraints may depress survival at a time when birds are already under high physiological stress due to flight feather molt (Leyrer et al., 2013). Additionally, during this phase knots tend to suppress costly immune functions which may make them more vulnerable to novel stressors (Buehler et al., 2008). Climate conditions on wintering sites are more moderate in the subtropical latitudes of this study, though birds may occasionally experience stress from short bouts of cold winter temperatures in addition to a wider array of other stressors such as disturbance from heavy recreational use of beaches. Such conditions could simultaneously increase maintenance metabolism costs and place constraints on foraging opportunity. Prey depletion, or prey toxicity, from red tide events during this time period would introduce another lethal or sublethal stressor on top of those already normally experienced by knots during the nonbreeding period.
Boyd and Piersma (2001) found that relative population stability of Red Knots (C.c. islandica) wintering in Great Britain was maintained by alternating trends of survival and recruitment, implicating a potential role of density-dependent processes in population regulation. Knots using Delaware Bay during spring migration experienced consistently high apparent survival which was offset by consistently low recruitment between 2005-2018, resulting in a slightly positive population growth rate (Tucker et al., 2023). Using data from two large shorebird monitoring datasets, Bart et al. (2007) suggested the most likely mechanisms of North American shorebird population declines are reduction in breeding population size and poor reproduction, rather than an artifact potentially explicable by shifting distributions. This is almost certainly the case with Red Knots, as nearly all regular monitoring at key sites across the range indicate a declining trend, while no “new” sites of importance have been discovered in the meantime that balance for losses seen elsewhere. The relatively acute mortality episodes associated with red tides in this study would clearly result in reduced breeding population, but it is not known whether reproductive capacity can offset such population reductions when they occur relatively frequently.
While red tide toxins have been directly tied to the mortality of Red Knots in Texas (Rafalski, 2012) and closely related shorebirds in Florida (van Deventer et al., 2012) through necropsy and tissue sampling, only one other study has quantitatively estimated the effect of HABs on shorebird survival at the population level. Ellis et al. (2021) detected a negative effect of HABs on Piping Plover (Charadrius melodus) survival during the nonbreeding season along the Gulf of Mexico coast. This species is not only faithful to wintering areas generally (similar to knots) but even more highly faithful to specific individual territories with small home ranges (Drake et al., 2001; Cohen et al., 2008; Newstead, 2014) and may have a greater disinclination to move away from an area affected by red tide or other factors that may negatively affect survival. Our study provides additional evidence that HABs can negatively impact shorebird populations even when sudden mass mortality events are not observed or perhaps do not occur.
Another HAB dinoflagellate, Aureoumbra lagunensis, creates “brown tides” in the Laguna Madre of Texas which could be affecting knots in other ways. Though this organism does not produce potent toxins, it is considered disruptive to ecosystems because of its ability to bloom at low light and nutrient levels, and create a positive feedback mechanism that results in losses to seagrasses and benthic organisms (Gobler and Sunda, 2012). One brown tide event in the 1990s persisted in the Laguna Madre for nearly eight years, the longest HAB ever recorded (Buskey et al., 2001), and blooms have recurred intermittently and at varying spatial extents since then (DeYoe et al., 2007). Major die-offs of Mulinia lateralis, formerly the dominant bivalve mollusk in the Laguna Madre, have been coincident with these blooms (Montagna et al., 1993). The diet of Red Knots during the winter months in the Laguna Madre has not been described, but given that M. lateralis is a dominant prey item in other parts of the species’ range, it is likely that these crashes in local populations would also impact prey availability, and potentially survival, for knots.
While this study focused on populations affected by HABs in the Gulf of Mexico, blooms have been suggested as a potential cause of several significant mortality events on the Atlantic coast of South America, affecting the long-distance migrant rufa population wintering in Tierra del Fuego. In Uruguay in April 2007, approximately 1300 knots were found dead in a single event that may have been associated with a HAB, though samples were not collected to confirm the cause of mortality (Aldabe et al., 2015). The loss of ~6% of the total rufa population in a single documented event, and the possibility that this may not have been a one-off event but could even occur with some regularity in remote parts of its range provides a potential partial explanation for the dramatic collapse of the Red Knot population that winters on the Atlantic coast of South America. During mortality events in 1997 and 2000 in southern Brazil, Buehler et al. (2010) described similar condition of Red Knots immediately prior to mortality – disorientation, lethargy, unresponsiveness – as witnessed in red tide events in Texas (Newstead, pers. obs.) and Florida, but pathology reports were inconclusive as to the primary cause of death.
Further, Red Knots that winter along the Pacific coasts of Central and South America (the majority of which are suspected to use the focal locations of this study as stopovers; Newstead, unpublished data) may also be encountering increased frequency and intensity of HABs (Band-Schmidt et al., 2019), including several recent events in Ecuador (Torres, 2015; Borbor-Cordova et al., 2019) and Chile (Mardones et al., 2010; Paredes et al., 2019). Several dinoflagellate species that produce paralytic or diarrhetic shellfish poisons can reach bloom concentrations resulting in fish kills and other toxic effects in areas of Central and South America known to be important stopovers. Among these, Gymnodinium catenatum, the Alexandrium tamarense complex, and Dinophysis spp. produce toxins that become highly concentrated in bivalve species such as wedge clams, Donax hanleyanus, and blue mussels, Mytilus edulis (Carreto et al., 1986; Mee et al., 1986; Méndez and Carreto, 2018), both known to be favored prey items of red knots. The distribution and frequency of HABs appear to be increasing in Central and South America (Band-Schmidt et al., 2019), as well as in the Gulf of Mexico (Tominack et al., 2020).
The magnitude of the geolocator effect was a ~3% reduction in seasonal survival (or ~8% over a year). While many studies reporting tracking device effects on survival have focused on the short-term (often one-year return rates) with projects having highly variable numbers of birds with and without devices, the results of this study are consistent with others (Rodriguez-Ruiz et al., 2019; Pakanen et al., 2020) finding that negative effects of some tracking devices may be statistically undetectable in the short term but accrue to the level of significance over the course of longer-term studies. The use of tracking devices on wildlife has yielded transformative new insights into our understanding of life histories and factors affecting distribution and movements of animals, especially Red Knots (Niles et al., 2010; Burger et al., 2012; Niles et al., 2012; Newstead et al., 2013; Tomkovich et al., 2013; Piersma et al., 2021). However, consideration must be given to the potential costs of such deployments on survival, reproduction, movement, and other concerns. As new findings are added to the literature and technological advances lead to ever smaller and more efficient tracking devices, researchers should continue to assess the potential benefits to be gained for species conservation relative to the potential impacts to birds when planning new studies.
This study provides the first long-term apparent survival estimates for Red Knot populations in the Gulf, and strong evidence that HABs are negatively affecting populations in Texas and Florida. Preventing such large-scale events presents many challenges, although where their apparent causes are linked to excessive nutrients these factors can be mitigated by better managing anthropogenic landscape changes along the coast and through the watershed. Since HABs are considered a “co-stressor” associated with climate change (Griffith and Gobler, 2020), these findings indicate the impacts to knots could become even more severe in the future.
Accurate estimation of population size of these three Gulf wintering groups has not been possible, and is hindered by several factors including the potential shift of some portion of the Florida wintering population to the southeast US (Pelton et al., 2022), logistical difficulties in accessing habitats used by the Louisiana and Texas populations during winter, and the fact that some knots that pass through the northern Gulf in spring likely wintered somewhere further south. These are all surmountable obstacles provided adequate support for dedicated and coordinated monitoring programs. While we have presented estimates of one key demographic parameter (survival) for these populations, a better understanding of processes and rates of recruitment is needed to evaluate population trajectories.
Data availability statement
The raw data supporting the conclusions of this article will be made available by the authors, without undue reservation.
Ethics statement
Ethical approval was not required for the study involving animals in accordance with the local legislation and institutional requirements because Researchers conducting field work were not affiliated with institutions with their own ethics review process. USFWS Recovery Permits (which were obtained for this work) require extensive explanation of capture/handling procedures and contingencies.
Author contributions
DN: Conceptualization, Data curation, Formal analysis, Investigation, Methodology, Project administration, Writing – original draft, Writing – review & editing. SD: Formal analysis, Methodology, Writing – review & editing. BB: Methodology, Supervision, Writing – review & editing. LN: Conceptualization, Writing – review & editing. JB: Writing – review & editing.
Funding
The author(s) declare financial support was received for the research, authorship, and/or publication of this article. Partial funding for captures and resight efforts were provided by grants from US Fish & Wildlife Service (201818G909, F16AP00835, F19AC00712, F19AP00937) and Florida Fish and Wildlife Conservation Commission (9713-720-6328).
Acknowledgments
Thanks to Humphrey Sitters, Amanda Dey, and Bill Howe for initiating the study of knots in Texas, and to Delaina LeBlanc of Barataria-Terrebonne National Estuary Program for assistance with logistics, capture, and resighting since the beginning of the study in Louisiana. Thanks to Jeannine Parvin and Lena Usyk for managing and corralling encounter data from www.bandedbirds.org, and our sincerest appreciation to all the dedicated biologists and volunteers for their time assisting in catches and reporting resights over many years and many sites. This is manuscript 24-103 of the Caesar Kleberg Wildlife Research Institute.
Conflict of interest
The authors declare that the research was conducted in the absence of any commercial or financial relationships that could be construed as a potential conflict of interest.
Publisher’s note
All claims expressed in this article are solely those of the authors and do not necessarily represent those of their affiliated organizations, or those of the publisher, the editors and the reviewers. Any product that may be evaluated in this article, or claim that may be made by its manufacturer, is not guaranteed or endorsed by the publisher.
Supplementary material
The Supplementary Material for this article can be found online at: https://www.frontiersin.org/articles/10.3389/fevo.2024.1375412/full#supplementary-material
References
Abbott B. C., Siger A., Spigelstein M. (1975). “Toxins from the blooms of Gymnodinium breve,” in Proceedings of the First International Conference on Toxic Dinoflagellate Blooms. Ed. LoCicero V. R. (Massachusetts Science and Technology Foundation, Wakefield, Massachusetts), 355–366.
Aldabe J., Rocca P. I., González P. M., Caballero-Sadi D., Baker A. J. (2015). Migration of endangered Red Knots Calidris canutus rufa in Uruguay: important sites, phenology, migratory connectivity and a mass mortality event. Wader Study 122, 221–235. doi: 10.18194/ws.00024
Baker A. J., González P. M., Morrison R. I. G., Harrington B. (2013). “Red Knot (Calidris canutus), v.2.0,” in The Birds of North America. Ed. Poole A. F. (Cornell Lab of Ornithology, Ithaca, NY, USA). doi: 10.2173/bna
Baker A. J., González P. M., Piersma T., Niles L. J., de Lima Serrano do Nascimento I., Atkinson P. W., et al. (2004). Rapid population decline in red knots: fitness consequences of decreased refuelling rates and late arrival in Delaware Bay. Proc. R. Soc London 271, 875–882. doi: 10.1098/rspb.2003.2663
Band-Schmidt C. J., Durán-Riveroll L. M., Bustillos-Guzmán J. J., Leyva-Valencia I., López-Cortés D. J., Núñez-Vázquez, et al. (2019). Paralytic toxin producing dinoflagellates in Latin America: ecology and physiology. Front. Mar. Sci. 6. doi: 10.3389/fmars.2019.00042
Barron D. G., Brawn J. D., Weatherhead P. J. (2010). Meta-analysis of transmitter effects on avian behavior and ecology. Methods Ecol. Evol. 1, 180–187. doi: 10.1111/j.2041-210X.2010.00013.x
Bart J., Brown S., Harrington B., Morrison. R. I. G. (2007). Survey trends of North American shorebirds: population declines or shifting distirbutions? J. Avian Biol. 38, 73–82. doi: 10.1111/j.2007.0908-8857.03698.x
Borbor-Cordova M. J., Torres G., Mantilla-Saltos G., Casierra-Tomala A., Bermúdez J. R., Renteria W., et al. (2019). Oceanography of harmful algal blooms on the Ecuadorian Coast, (1997–2017): Integrating remote sensing and biological data. Front. Mar. Sci. 6. doi: 10.3389/fmars.2019.00013
Boyce M. S. (1977). Population growth with stochastic fluctuations in the life table. Theor. Popul. Biol. 12, 366–373. doi: 10.1016/0040-5809(77)90050-8
Boyd H., Piersma T. (2001). Changing balance between survival and recruitment explains population trends in Red Knots Calidris canutus islandica wintering in Britain 1969-1995. Ardea 89, 301–317.
Brand L. E., Compton A. (2007). Long-term increase in Karenia brevis abundance along the Southwest Florida Coast. Harmf. Algae 6, 232–252. doi: 10.1016/j.hal.2006.08.005
Bricelj V. M., Haubois A.-G., Sengco M. R., Pierce R. H., Culter J. K., Anderson D. M. (2012). Trophic transfer of brevetoxins to the benthic macrofaunal community during a bloom of the harmful dinoflagellate Karenia brevis in Sarasota Bay, Florida. Harmf. Algae 16, 27–34. doi: 10.1016/j.hal.2012.01.001
Bridge E. S., Thorup K., Bowlin M. S., Chilson P. B., Diehl R. H., Fléron R. W. (2010). Technology on the move: recent and forthcoming innovations for tracking migratory birds. BioScience 61, 689–698. doi: 10.1525/bio.2011.61.9.7
Buchanan J. B., Johnson J. A., Bishop M. A., DeCicco L. H., Hajdukovich N., Salzer L. J., et al. (2019). Does radio transmitter attachment influence resighting rates of Red Knots in the Pacific Flyway, USA? Wader Study 126, 151–154. doi: 10.18194/ws
Buchanan J. B., Lyons J. E., Salzer L. J., Carmona R., Arce N., Wiles G. J., et al. (2012). Among-year site fidelity of Red Knots during migration in Washington. J. Field Ornithol. 83, 282–289. doi: 10.1111/jofo.2012.83.issue-3
Buehler D. M., Bugoni L., Dorrestein G. M., González P. M., Pereira-Jr J., Proença L., et al. (2010). Local mortality events in migrating sandpipers (Calidris) at a staging site in southern Brazil. Wader Study Group Bull. 117, 150–156.
Buehler D. M., Piersma T., Matson K., Tieleman I. (2008). Seasonal redistribution of immune function in a migrant shorebird: annual-cycle effects override adjustments to thermal regime. Am. Nat. 172, 783–796. doi: 10.1086/592865
Burger J., Niles L. J., Porter R. R., Dey A. D., Koch S., Gordon C. (2012). Migration and over-wintering of red knots (Calidris canutus rufa) along the atlantic coast of the united states. Condor 114 (2), 302–313. doi: 10.1525/cond.2012.110077
Burnham K. P., Anderson D. R. (2002). Model Selection and Multimodel Inference: a Practical Information-theoretic Approach. 2nd ed (New York: Springer-Verlag).
Buskey E. J., Liu H., Collumb C., Bersano J. G. F. (2001). The decline and recovery of a persistent Texas brown tide algal bloom in the Laguna Madre (Texas, USA). Estuaries 24, 337–346. doi: 10.2307/1353236
Calvert A. M., Amirault D. L., Shaffer F., Elliot R., Hanson A., McKnight J., et al. (2006). Population assessment of an endangered shorebird: the Piping Plover (Charadrius melodus melodus) in eastern Canada. Avian Conserv. Ecol. - Écol. Conserv. Des. oiseaux 1, 4. doi: 10.5751/ACE-00091-010304
Carreto J. I., Benavides H. R., Negri R. M., Glorioso P. D. (1986). Toxic red-tide in the Argentine Sea. Phyoplankton distribution and survival of the toxic dinoflagellate Gonyaulax excavata in a frontal area. J. Plankton Res. 8, 15–28. doi: 10.1093/plankt/8.1.15
Castle K. T., Flewelling L. J., Bryan J. II, Kramer A., Lindsay J., Nevada C., et al. (2013). Coyote (Canis latrans) and domestic dog (Canis familiaris) mortality and morbidity due to a Karenia brevis red tide in the Gulf of Mexico. J. Wildl. Dis. 49, 955–964. doi: 10.7589/2012-11-299
Chan Y.-C., Tibbitts T. L., Lok T., Hassell C. J., Peng H.-B., Ma Z., et al. (2019). Filling knowledge gaps in a threatened shorebird flyway through satellite tracking. J. Appl. Ecol. 56, 2305–2315. doi: 10.1111/1365-2664.13474
Cohen J. B., Karpanty S. M., Catlin D. H., Fraser J. D., Fischer R. A. (2008). Winter ecology of Piping Plovers at Oregon Inlet, North Carolina. Waterbirds 31, 472–479. doi: 10.1675/1524-4695-31.3.472
Conklin J. R., Battley P. F. (2010). Attachment of geolocators to Bar-tailed Godwits: a tibia-mounted method with no survival effects or loss of units. Wader Study Group Bull. 117, 56–58.
COSEWIC. (2007). COSEWIC assessment and status report on the Red Knot Calidris canutus in Canada (Ottawa: Committee on the Status of Endangered Wildlife in Canada). Available at: www.sararegistry.gc.ca/status/status_e.cfm. Vii + 58 pp.
Costantini D., Møller A. P. (2013). A meta-analysis of the effects of geolocator application on birds. Curr. Zool. 59, 697–706. doi: 10.1093/czoolo/59.6.697
Cummins J. M., Jones A. C., Stevens A. A. (1971). Occurrence of toxic bivalve molluscs during a Gymnodinium breve ‘‘red tide’’. Trans. Am. Fish. Soc 100, 112–116. doi: 10.1577/1548-8659(1971)100
DeYoe H. R., Buskey E. J., Jochem F. J. (2007). Physiological responses of Aureoumbra lagunensis and Synechococcus sp. to nitrogen addition in a mesocosm experiment. Harmf. Algae 6, 48–55. doi: 10.1016/j.hal.2006.06.001
Drake K. R., Thompson J. E., Drake K. L., Zonick C. (2001). Movements, habitat use, and survival of nonbreeding Piping Plovers. Condor 103, 259–267. doi: 10.1093/condor/103.2.259
Duijns S., Anderson A. M., Aubry Y., Dey A., Flemming S. A., Francis C. M., et al. (2019). Long-distance migratory shorebirds travel faster towards their breeding grounds, but fly faster post-breeding. Sci. Rep. 9, 9420. doi: 10.1038/s41598-019-45862-0
Duijns S., Niles L. J., Dey A., Aubry Y., Friis C., Koch S., et al. (2017). Body condition explains migratory performance of a long-distance migrant. Proc. R. Soc B 284, 20171374. doi: 10.1098/rspb.2017.1374
Duriez O., Ens B. J., Choquet R., Pradel R., Klaassen M. (2012). Comparing the seasonal survival of resident and migratory oystercatchers: carry-over effects of habitat quality and weather conditions. Oikos 121, 862–873. doi: 10.1111/j.1600-0706.2012.20326.x
Elliott K. H., McFarlane-Tranquilla L., Burke C. M., Hedd A., Montevecchi W. A., Anderson W. G. (2012). Year-long deployments of small geolocators increase corticosterone levels in murres. Mar. Ecol. Prog. Ser. 466, 1–7. doi: 10.3354/meps09975
Elliott-Smith E., Bidwell M., Holland A. E., Haig S. M. (2015). Data from the 2011 International Piping Plover Census (Reston, Virginia, USA: U.S. Geological Survey).
Ellis K. S., Anteau M. J., Cuthbert F. J., Gratto-Trevor C. L., Jorgensen J. G., Newstead D. J., et al. (2021). Impacts of extreme environmental disturbances on piping plover survival are partially moderated by migratory connectivity. Biol. Conserv. 264, 109371. doi: 10.1016/j.biocon.2021.109371
Faaborg J., Holmes R. T., Anders A. D., Bildstein K. L., Dugger K. M., Gauthreaux J. S.A., et al. (2010). Recent advances in understanding migration systems of New World land birds. Ecol. Monogr. 80, 3–48. doi: 10.1890/09-0395.1
Fernández G., Lank D. B. (2006). Effects of habitat loss on shorebirds during the non-breeding season: current knowledge and suggestions for action. Ornitol. Neotro. 19, 633–640.
Gauthier G., Pradel R., Menu S., Lebreton J.-D. (2001). Seasonal survival of Greater Snow Geese and effect of hunting under dependence in sighting probability. Ecology 82, 3105–3119. doi: 10.1890/0012-9658(2001)082[3105:SSOGSG]2.0.CO;2
Gill J. A., Sutherland W. J. (2000). “Predicting the consequences of human disturbance from behavioural decisions,” in Behaviour and Conservation. Eds. Morris L. M., Sutherland W. J. (Cambridge Univ. Press, Cambridge, UK), 51–64.
Gobler C. J., Sunda W. G. (2012). Ecosystem disruptive algal blooms of the brown tide species, Aureococcus anophagefferens and Aureoumbra lagunensis. Harmf. Algae 14, 360–345. doi: 10.1016/j.hal.2011.10.013
González P. M., Baker A. J., Echave M. E. (2006). Annual survival of red knots (Calidris canutus rufa) using the san antonio oeste stopover site is reduced by domino effects involving late arrival and food depletion in delaware bay. El hornero 21 (2), 109–117.
Goss-Custard J. D., Clarke R. T., Durell S. E. A., dit. L., Caldow R. W. G., Ens B. J. (1995). Population consequences of winter habitat loss in a migratory shorebird. II. model predictions. J. Appl. Ecol. 32, 337–351.
Green M., Piersma T., Jukema J., De Goeij P., Spaans B., van Gils J. A. (2002). Radio-telemetry observations of the first 650 km of the migration of Bar-tailed Godwits Limosa lapponica from the Wadden Sea to the Russian Arctic. Ardea 90, 71–80.
Griffith A. W., Gobler C. J. (2020). Harmful algal blooms: A climate change co-stressor in marine and freshwater ecosystems. Harmf. Algae 91, 1–12. doi: 10.1016/j.hal.2019.03.008
Hallegraeff G. M. (1993). A review of harmful algal blooms and their apparent global increase. Phycology 322, 79–99. doi: 10.2216/i0031-8884-32-2-79.1
Harrington B. A., Hagan J. M., Leddy L. E. (1998). Site fidelity and survival differences between two groups of new world Red Knots (Calidris canutus). Auk 105, 439–445. doi: 10.1093/auk/105.3.439
Hitchcock C. L., Gratto-Trevor C. (1997). Diagnosing a shorebird local population decline with a stage-structured population model. Ecology 78, 522.534. doi: 10.1890/0012-9658(1997)078[0522:DASLPD]2.0.CO;2
Iwamura T., Possingham H. P., Chadès I., Minton C., Murray N. J., Rogers D. I., et al. (2013). Migratory connectivity magnifies the consequences of habitat loss from sea-level rise for shorebird populations. Proc. R Soc. B 280, 20130325. doi: 10.1098/rspb.2013.0325
Johnson M. D., Sherry T. W., Holmes R. T., Marra P. P. (2006). Assessing habitat quality for a migratory songbird wintering in natural and agricultural habitats. Conserv. Biol. 20, 1433–1444. doi: 10.1111/j.1523-1739.2006.00490.x
Kennish M. J. (2002). Environmental threats and environmental future of estuaries. Environ. Conserv. 29, 78–107. doi: 10.1017/S0376892902000061
Kvitek R., Bretz C. (2005). Shorebird foraging behavior, diet, and abundance vary with harmful algal bloom toxin concentrations in invertebrate prey. Mar. Ecol. Prog. Ser. 293, 303–309. doi: 10.3354/meps293303
Lande R. (1993). Risks of population extinction from demographic and environmental stochasticity and random catastrophes. Am. Nat. 142, 911–927. doi: 10.1086/285580
Landsberg J. H. (2002). The effects of harmful algal blooms on aquatic organisms. Rev. Fish. Sci. 10, 113–390. doi: 10.1080/20026491051695
Landsberg J. H., Flewelling L. J., Naar J. (2009). Karenia brevis red tides, brevetoxins in the food web, and impacts on natural resources: decadal advancements. Harmf. Algae 8, 598–607. doi: 10.1016/j.hal.2008.11.010
Lerma L. (2013). The effects of a red tide, Karenia brevis episode on the benthic macroinvertebrate communities of South Padre Island, TX. University of Texas at Brownsville, Brownsville, Texas.
Leyrer J., Lok T., Brugge M., Spaans B., Sandercock B. K., Piersma T. (2013). Mortality within the annual cycle: seasonal survival patterns in Afro-Siberian Red Knots Calidris canutus canutus. J. Ornithol. 154, 933–943. doi: 10.1007/s10336-013-0959-y
Leyrer J., Spaans B., Camara M., Piersma T. (2006). Small home ranges and high site fidelity in red knots (Calidris c. canutus) wintering on the Banc d’Arguin, Mauritania. J. Ornithol. 147, 376–384. doi: 10.1007/s10336-005-0030-8
Lyons J. E., Winn B., Keyes T., Kalasz K. S. (2018). Post-breeding migration and connectivity of Red Knots in the western Atlantic. J. Wildl. Manage. 82, 383–396. doi: 10.1002/jwmg.21389
Magaña H. A., Contreras C., Villareal T. A. (2003). A historical assessment of Karenia brevis in the western Gulf of Mexico. Harmf. Algae 2, 163–171. doi: 10.1016/S1568-9883(03)00026-X
Mardones J., Clement A., Rojas X., Aparicio C. (2010). Alexandrium catenella during 2009 in Chilean waters, and recent expansion to coastal ocean. Harmf. Algae News 41, 8-9.
McGowan C. P., Hines J. E., Nichols J. D., Lyons J. E., Smith D. R., Kalasz K. S., et al. (2011). Demographic consequences of migratory stopover: linking red knot survival to horseshoe crab spawning abundance. Ecosphere 2, 1–22. doi: 10.1890/ES11-00106.1
McKellar A. E., Ross R. K., Morrison R. I. G., Niles L. J., Porter R. R., Burger J., et al. (2015). Shorebird use of western Hudson Bay near the Nelson River during migration, with a focus on the Red Knot. Wader Study 122, 1–11. doi: 10.18194/ws.00020
Mee L. D., Espinosa M., Diaz G. (1986). Paralytic shellfish poisoning with a Gymnodinium catenatum red tide on the Pacific coast of Mexico. Mar. Environ. Res. 19, 77–92. doi: 10.1016/0141-1136(86)90040-1
Méndez S. M., Carreto J. I. (2018). “Harmful Algal Blooms in the Río de la Plata Region,” in Plankton Ecology of the Southwestern Atlantic: From the Subtropical to the Subantarctic Realm. Eds. Hoffmeyer M., Sabatini M. E., Brandini F., Calliari D. L., Santinelli N. H. (Springer International Publishing, Switzerland). doi: 10.1007/978-3-319-77869-3
Mondain-Monval T. O., du Feu R., Sharp S. P. (2020). The effects of geolocators on return rates, condition, and breeding success in Common Sandpipers Actitis hypoleucos. Bird Study 67, 217–223. doi: 10.1080/00063657.2020.1808592
Montagna P. A., Stockwell D. A., Kalke R. D. (1993). Dwarf surfclam Mulinia lateralis (Say 1822) populations and feeding during the Texas brown tide event. J. Shellfish Res. 12, 433–442.
Musmeci L. R., Bala L. O., Tschopp A., Hernandez M. A., Coscarella M. A. (2022). Red knot (Calidris canutus rufa) site fidelity at Península Valdés, Argentina. Wilson J. Ornithol. 134, 302-309. doi: 10.1676/19-00108
Newstead D. J. (2014). Habitat use of North Padre Island and Laguna Madre habitats by Piping Plovers (Charadrius melodus) and Red Knots (Calidris canutus) in the vicinity of current and proposed wind energy development (Austin, Texas: Report to Texas Parks & Wildlife Department, project E-137-R). 47 pp.
Newstead D. J., Niles L. J., Porter R. R., Dey A., Burger J., Fitzsimmons O. N. (2013). Geolocation reveals mid-continent migratory routes and Texas wintering areas of Red Knots Calidris canutus rufa. Wader Study Group Bull. 120, 53–59.
Niles L. J., Burger J., Porter R. R., Dey A. D., Koch S., Harrington B., et al. (2012). Migration pathways, migration speeds and non-breeding areas used by northern hemisphere wintering Red Knots Calidris canutus of the subspecies rufa. Wader Study Group Bull. 119, 195–203.
Niles L. J., Burger J., Porter R. R., Dey A. D., Minton C. D. T., Gonzalez P. M., et al. (2010). First results using light level geolocators to track Red Knots in the Western Hemisphere show rapid and long intercontinental flights and new details of migration pathways. Wader Study Group Bull. 117, 123–130.
Niles L. J., Sitters H. P., Dey A. D., Atkinson P. W., Baker A. J., Bennett K. A., et al. (2008). Status of the Red Knot, Calidris canutus rufa, in the Western Hemisphere. Stud. Avian Biol. 36, 1–185.
NOAA National Centers for Environmental Information. (2014). Physical and biological data collected along the texas, mississippi, alabama, and florida gulf coasts in the gulf of mexico as part of the harmful algal BloomS observing system from 1953-08-19 to 2023-07-06 (NCEI accession 0120767) (NOAA National Centers for Environmental Information). Dataset. Available at: https://www.ncei.noaa.gov/archive/accession/0120767.
Norris D. R. (2005). Carry-over effects and habitat quality in migratory populations. Oikos 109, 178–186. doi: 10.1111/j.0030-1299.2005.13671.x
Norris D. R., Marra P. P. (2007). Seasonal interactions, habitat quality, and population dynamics in migratory birds. Condor 109 (3), 535–547. doi: 10.1093/condor/109.3.535
Pakanen V.-M., Rönkä N., Thomson R. L., Blomqvist D., Koivula K. (2020). Survival probability in a small shorebird decreases with the time an individual carries a tracking device. J. Avian Biol. 51, e02555. doi: 10.1111/jav.02555
Pakanen V.-M., Rönkä N., Thomson R. L., Koivula K. (2015). No strong effects of leg-flagged geolocators on return rates or reproduction of a small long-distance migratory shorebird. Ornis Fennica 92, 101–111. doi: 10.51812/of.133872
Paredes J., Varela D., Martínez C., Zúñiga A., Correa K., Villarroel A., et al. (2019). Population Genetic Structure at the Northern Edge of the Distribution of Alexandrium catenella in the Patagonian Fjords and Its Expansion Along the Open Pacific Ocean Coast. Front. Mar. Sci. 5. doi: 10.3389/fmars.2018.00532
Pelton M. M., Padula S. R., Garcia-Walther J., Andrews M., Mercer R., Porter R., et al. (2022). Kiawah and Seabrook islands are a critical site for the rufa Red Knot. Wader Study 129, 105–118. doi: 10.18194/ws
Pierce R. H., Henry M. S. (2008). Harmful algal toxins of the Florida red tide (Karenia brevis): natural chemical stressors in South Florida coastal ecosystems. Ecotoxicology 17, 623–631. doi: 10.1007/s10646-008-0241-x
Piersma T., Kok E. M. A., Hassell C. J., Peng H.-B., Verkuil Y. I., Lei G., et al. (2021). When a typical jumper skips: itineraries and staging habitats used by Red Knots (Calidris canutus piersmai) migrating between northwest Australia and the New Siberian Islands. Ibis 163, 1235–1251. doi: 10.1111/ibi.12964
Piersma T., Lok T., Chen Y., Hassell C. J., Yang H.-Y., Boyle A., et al. (2016). Simultaneous declines in summer survival of three shorebird species signals a flyway at risk. J. Appl. Ecol. 53, 479–490. doi: 10.1111/1365-2664.12582
Powell L. A. (2007). Approximating variance of demographic parameters using the delta method: a reference for avian biologists. Condor 109, 949–954. doi: 10.1093/condor/109.4.949
Rafalski A. V. (2012). Evaluation of brevetoxin accumulation and degradation in coastal mammals, birds, and fish found moribund on Texas beaches during red tide blooms. Texas A&M University – Corpus Christi, Corpus Christi, Texas.
Robinson W. D., Bowlin M. S., Bisson I., Shamoun-Baranes J., Thorup K., Diehl R. H., et al. (2010). Integrating concepts and technologies to advance the study of bird migration. Front. Ecol. Environ. 8, 354–361. doi: 10.1890/080179
Rodriguez-Ruiz J., Mougeot F., Parejo D., de la Puente J., Bermejo A., Aviles J. M. (2019). Important areas for the conservation of the european roller coracias garrulus during the non-breeding season in southern africa. Bird Conserv. Int. 29 (1), 159–175. doi: 10.1111/ibi.12317
Rogers D. I., Piersma T., Hassell C. J. (2006). Roost availability may constrain shorebird distribution: Exploring the energetic costs of roosting and disturbance around a tropical bay. Biol. Conserv. 133, 225–235. doi: 10.1016/j.biocon.2006.06.007
Rolton A., Vignier J., Volety A. K., Pierce R. H., Henry M., Shumway S. E., et al. (2016). Effects of field and laboratory exposure to the toxic dinoflagellate Karenia brevis on the reproduction of the eastern oyster, Crassostrea virginica, and subsequent development of offspring. Harmf. Algae 57, 13–26. doi: 10.1016/j.hal.2016.04.011
Rushing C. S., Hostetler J. A., Sillett T. S., Marra P. P., Rotenberg J. A., Ryder T. B. (2017). Spatial and temporal drivers of avian population dynamics across the annual cycle. Ecology 98, 2837–2850. doi: 10.1002/ecy.1967
Scarpignato A. L., Harrison A.-L., Newstead D. J., Niles L. J., Porter R. R., van den Tillaart M., et al. (2016). Field-testing a new miniaturized GPS-Argos satellite transmitter (3.5 g) on migratory shorebirds. Wader Study 123, 240–246. doi: 10.18194/ws.00046
Schwarzer A. C., Collazo J. A., Niles L. J., Brush J. M., Douglass N. J., Percival H. F. (2012). Annual survival of Red Knots (Calidris canutus rufa) wintering in Florida. Auk 129, 725–733. doi: 10.1525/auk.2012.11269
Shumway S. E., Allen S. M., Boersma P. D. (2003). Marine birds and harmful algal blooms: sporadic victims or under-reported events? Harmf. Algae 2, 1–17.
Simberloff D. (1988). The contribution of population and community biology to conservation science. Annu. Rev. Ecol. Systematics 19 (1), 473–511.
Stantial M. L., Cohen J. B., Loring P. H., Paton P. W. C. (2019). Radio transmitters did not affect apparent survival rates of adult Piping Plovers (Charadrius melodus). Waterbirds 42, 205–209. doi: 10.1675/063.042.0207
Stumpf R. P., Li Y., Kirkpatrick B., Litaker R. W., Hubbard K. A., Currier R. D., et al. (2022). Quantifying Karenia brevis bloom severity and respiratory irritation impact along the shoreline of Southwest Florida. PloS One 17, e0260755. doi: 10.1371/journal.pone.0260755
Stutchbury B. J. M., Tarof S. A., Done T., Gow E. A., Kramer P. M., Tautin J., et al. (2009). Tracking long-distance songbird migration by using geolocators. Science 323, 896. doi: 10.1126/science.1166664
Summerson H. C., Peterson C. H. (1990). Recruitment failure of the bay scallop, Argopecten irradians concentricus, during the first red tide, Ptychodiscus brevis, outbreak recorded in North Carolina. Estuaries 13, 3, 322–331. doi: 10.2307/1351923
Sutherland W. J., Alves J. A., Amano T., Chang C. H., Davidson N. C., Finlayson C. M., et al. (2012). A horizon scanning assessment of current and potential future threats facing migratory shorebirds. Ibis 154, 663–679. doi: 10.1111/j.1474-919X.2012.01261.x
Tester P. A., Turner J. T., Shea D. (2000). Vectorial transport of toxins from the dinoflagellate Gymnodinium breve through copepods to fish. J. Plankton Res. 22, 47–62. doi: 10.1093/plankt/22.1.47
Tominack S. A., Coffey K. Z., Yoskowitz D., Sutton G., Wetz M. S. (2020). An assessment of trends in the frequency and duration of Karenia brevis red tide blooms on the South Texas coast (western Gulf of Mexico). PloS One 15, e0239309. doi: 10.1371/journal.pone.0239309
Tomkovich P. S., Porter R. R., Loktionov E. Y., Niles L. J. (2013). Pathways and staging areas of Red Knots Calidris canutus rogersi breeding in southern Chukotka, Far Eastern Russia. Wader Study Group Bull. 120, 181–193.
Torres G. (2015). Evaluación de mareas rojas durante 1968-2009 en Ecuador. Acta Oceanográfica del Pacífico 20, 89–98.
Tucker A. M., McGowan C. P., Lyons J. E., DeRose-Wilson A., Clark N. A. (2021). Species-specific demographic and behavioral responses to food availability during migratory stopover. Popul. Ecol. 2021, 1–16.
Tucker A. M., McGowan C. P., Nuse B. L., Lyons J. E., Moore C. T., Smith D. R., et al. (2023). Estimating recruitment rate and population dynamics at a migratory stopover site using an integrated population model. Ecosphere 14, 1–16. doi: 10.1002/ecs2.4439
Tuma M. E., Powell A. N. (2021). The southeastern U.S. as a complex of use sites for nonbreeding rufa Red Knots: fifteen years of band encounter data. Wader Study 128, 265–273. doi: 10.18194/ws
U.S. Fish and Wildlife Service [USFWS]. (2014a). Threatened species status for the Rufa red knot. 73706–73748, 79 Federal Register 238 (2014 December 11).
U.S. Fish and Wildlife Service [USFWS]. (2014b). Rufa red knot background information and threats assessment. Supplement to Endangered and Threatened Wildlife and Plants; Final Threatened Status for the Rufa red knot (Calidris canutus rufa) [Document No. FWS-R5-ES-2013-0097; RIN AY17] (Pleasantville, New Jersey, USA: U.S. Fish and Wildlife Service).
U.S. Fish and Wildlife Service [USFWS]. (2021). Rufa Red Knot Critical Habitat Methods [Document No. FWS-R5-ES-2021-0032-0009] (Pleasantville, New Jersey, USA: U.S. Fish and Wildlife Service). 12 pp.
van Deventer M., Atwood K., Vargo G. A., Flewelling L. J., Landsberg J. H., Naar J. P., et al. (2012). Karenia brevis red tides and brevetoxin-contaminated fish: a high risk factor for Florida’s scavenging shorebirds? Botanica Marina 55, 31–37. doi: 10.1515/bot.2011.122
van Dolah F. M. (2000). Marine algal toxins: origins, health effects, and their increased occurrence. Environ. Health Perspect. 108, 133–141. doi: 10.1289/ehp.00108s1133
van Gils J. A., Piersma T., Dekinga A., Battley P. F. (2006). Modelling phenotypic flexibility: an optimality analysis of gizzard size in Red Knots (Calidris canutus). Ardea 9), 409–420.
Van Hemert C., Dusek R. J., Smith M. M., Kaler R., Sheffield G., Divine L. M., et al. (2021). Investigation of algal toxins in a multispecies seabird die-off in the Bering and Chukchi Seas. J. Wildl. Dis. 57, 399–407. doi: 10.7589/JWD-D-20-00057
Van Hemert C., Harley J. R., Baluss G., Smith M. M., Dusek R. J., Lankton J. S., et al. (2022). Paralytic shellfish toxins associated with Arctic Tern mortalities in Alaska. Harmf. Algae 117, 102270. doi: 10.1016/j.hal.2022.102270
van Irsel J., Frauendorf M., Ens B. J., van de Pol M., Troost K., Oosterbeek K., et al. (2022). State-dependent environmental sensitivity of reproductive success and survival in a shorebird. Ibis 164, 692–710. doi: 10.1111/ibi.13038
Walsh J. J., Jolliff J. K., Darrow B. P., Lenes J. M., Milroy S. P., Remsen A., et al. (2006). Red tides in the Gulf of Mexico: Where, when, and why? J. Geophys. Res. 111, 1–46. doi: 10.1029/2004JC002813
Warnock N., Takekawa J. Y. (2003). Use of radio telemetry in studies of shorebirds: past contributions and future directions. Wader Study Group Bull. 100, 1–14.
Webster M. S., Marra P. P., Haig S. M., Bensch S., Holmes R. T. (2002). Links between worlds: unraveling migratory connectivity. Trends Ecol. Evol. 17, 76–83. doi: 10.1016/S0169-5347(01)02380-1
Weiser E. L., Lanctot R. B., Brown S. C., Alves J. A., Battley P. F., Bentzen R., et al. (2016). Effects of geolocators on hatching success, return rates, breeding movements, and change in body mass in 16 species of Arctic-breeding shorebirds. Move. Ecol. 4, 1–19. doi: 10.1186/s40462-016-0077-6
White G. C., Burnham K. P. (1999). Program MARK: Survival estimation for populations of marked animals. Bird Study 46, 120–139.
Keywords: survival, shorebird, harmful algal bloom, red tide, molluscivore, red knot, Gulf of Mexico, mark-resight
Citation: Newstead DJ, Dinsmore SJ, Ballard BM, Niles LJ and Burger J (2024) Survival of red knots in the northern Gulf of Mexico. Front. Ecol. Evol. 12:1375412. doi: 10.3389/fevo.2024.1375412
Received: 23 January 2024; Accepted: 18 March 2024;
Published: 09 April 2024.
Edited by:
Piotr Nowicki, Jagiellonian University, PolandReviewed by:
Joe Eisaguirre, United States Geological Survey, United StatesDaniel Robert Ruthrauff, U.S. Geological Survey, United States
Copyright © 2024 Newstead, Dinsmore, Ballard, Niles and Burger. This is an open-access article distributed under the terms of the Creative Commons Attribution License (CC BY). The use, distribution or reproduction in other forums is permitted, provided the original author(s) and the copyright owner(s) are credited and that the original publication in this journal is cited, in accordance with accepted academic practice. No use, distribution or reproduction is permitted which does not comply with these terms.
*Correspondence: David J. Newstead, ZG5ld3N0ZWFkQGNiYmVwLm9yZw==