- 1Zoological Survey of India, Kolkata, West Bengal, India
- 2School of Biological and Environmental Sciences, Faculty of Basic Sciences, Shoolini University, Solan, Himachal Pradesh, India
Introduction
In terrestrial ecosystems, soil organisms contribute to a variety of biological and biochemical processes, which play an important role in maintaining healthy and functional ecosystems (Lavelle et al., 2006). Among soil macro-invertebrates, earthworms make up a substantial part of the soil invertebrate biomass across various terrestrial habitats and are often used as bioindicators in soil quality assessments (Barros et al., 2002; Fründ et al., 2011; Pérès et al., 2011; Pauli et al., 2011). Earthworms play a crucial role by contributing to litter decomposition, nutrient cycling, soil aeration, and the maintenance of soil structure (Edwards and Bohlen, 1996).
In natural conditions, earthworms move at an extremely slow pace of approximately 1.4–9 m/year; however, their long-distance migration is facilitated through various means, such as by the feet of birds and other animals, through the roots of displaced plants, and even through transportation of wooden logs as well as long-distance transportation of the organism for commercial purposes (Julka, 1988; Díaz Cosín et al., 2011; Tóth et al., 2020; Chen et al., 2021). While colonizing a new environment beyond their native range, earthworm species face various ecological challenges. These include changes in behavioral traits, such as dietary adaptability and physiological tolerance, as well as changes in life history characteristics like parthenogenesis, short generation times, and dispersal modes (Nouri-Aiin et al., 2022). Some studies have shown that parthenogenesis and polyploidy benefit migrating earthworm species (Edwards and Bohlen, 1996; Díaz Cosín et al., 2011).
Earthworms are generally considered hermaphrodites; cross-fertilization is the most common reproductive strategy among most earthworm species. Self-fertilization has only been observed in Eisenia andrei Bouché, 1972, wherein the worm bends itself, allowing its spermathecal pores to contact the ventral zone of its clitellum and to pass the sperm from the male pores to the spermathecae (Domínguez et al., 2003). In addition to hermaphroditism, parthenogenetic reproduction is observed in some species, most of which are polyploid (Díaz Cosín et al., 2011). Parthenogenesis is significant due to its ability to preserve polyploidy and promote the expansion of polyploid variations in new regions; a single worm can initiate a new colony (Gates, 1972). Parthenogenetic reproduction is common in the family Lumbricidae (Terhivuo and Saura, 2003; Lowe and Butt, 2008; Sosa et al., 2017), with more than 30 species being found in North America (Reynolds, 1974). Parthenogenetic morphs typically have a high reproductive capacity, produce resistant cocoons, and exhibit wide environmental or feeding tolerances, a high dispersal rate, and the ability to withstand higher parasitic burdens (Gates, 1972; Jaenike and Selander, 1979; Blakemore, 2012).
In their study, Martay and Pearce-Higgins (2020) have reported a significant decline in the bird species that rely on earthworms, due to a decline in the earthworm population. Thus, it can be concluded that the role of soil biodiversity in maintaining ecosystem health and conserving habitats for higher vertebrates is invaluable, but often gets ignored in conservation policies (Martay and Pearce-Higgins, 2020; Cui et al., 2022; Duarte et al., 2024). This oversight leads to insufficient data on the conservation status of soil organisms, raising concerns about the effectiveness of conservation areas in preserving overall ecosystem functionality.
Analyzing earthworm populations and behavior will yield valuable insights into soil conditions and the broader environment. Moreover, an understanding of the spatial distribution of earthworm species along the protected areas is crucially important as baseline information against which future changes in the protected areas can be monitored and assessed. Therefore, the primary objective of this study was to analyze the spatial distribution of earthworm species across several wildlife sanctuaries, namely, Bethuadahari Wildlife Sanctuary, Bibhutibhushan Wildlife Sanctuary, Raiganj Wildlife Sanctuary, Ballavpur Wildlife Sanctuary, and Ramnabagan Wildlife Sanctuary. Our investigation aimed to address the following questions: Does species richness vary along these wildlife sanctuaries? What is the status of native and exotic peregrine species within these protected areas? Does the natural forest support native and epigeic species? To achieve these goals, earthworm samples were collected from different wildlife sanctuaries using the TSBF (Tropical Soil Biology and Fertility) method.
Material and methods
The present study was part of the Zoological Survey of India’s in-house program entitled “Faunal Diversity of Wildlife Sanctuaries of West Bengal”, covering all faunal groups. Two surveys were conducted for earthworms, one in December 2021 [Ramnabagan Wildlife Sanctuary (RAWLS) and Ballavpur Wildlife Sanctuary (BAWLS)] and another in October 2023 [Bibhutibhushan Wildlife Sanctuary (BIWLS), Bethuadahari Wildlife Sanctuary (BEWLS), and Raiganj Wildlife Sanctuary, also known as Kulik Bird Sanctuary (KUWLS)]. In the West Bengal state, these sanctuaries are situated in the districts of North 24 Parganas (BIWLS), Nadia (BEWLS), North Dinajpur (KUWLS), Birbhum (BAWLS), and Purba Bardhaman (RAWLS). The Nadia district has an average yearly precipitation of 1,245 mm, while the North Dinajpur district has an average of 1,592 mm. The Birbhum district with an average yearly precipitation of 1,321 mm, the Purba Bardhaman district with an average yearly precipitation of 1,400 mm, and the North 24 Parganas district with an average yearly precipitation of 1,579 mm are the intermediate districts concerning the rainfall level. The majority of the rainy season falls between June and September, during the Southwest monsoon. According to data from the WB State Government, January is the coldest month with the lowest points of up to 10°C, while May has the greatest peak of up to 41°C. Alluvial soil is the typical soil found in these regions. These sanctuaries are situated between 45 and 66 m above mean sea level and are a part of the Gangetic biogeographical zone. The selected Wildlife Sanctuaries characterized by the tropical deciduous forest comprise the dominant tree species, Acacia auriculiformis A. Cunn. ex Benth., Anacardium occidentale Linn., Shorea robusta Gaertn., Phyllanthus emblica Linn., Terminalia bellirica (Gaertn.) Roxb., Terminalia chebula Retz., Tectona grandis L.F., Terminalia arjuna (Roxb.) Wight and Arn., Dalbergia sissoo Roxb., Bambusa tulda Roxb., Neolamarckia cadamba (Roxb.) Bosser, Limonia acidissima Linn., and Ficus racemosa Linn.
Earthworms were collected from the aforementioned five wildlife sanctuaries. Within each sanctuary, four sampling sites (each with an area of 10 × 10 m) were selected (Supplementary Table 1; Supplementary Figure 1). At each sampling site, earthworms were collected by digging and hand sorting nine quadrants, each measuring 25 × 25 cm and up to 30 cm depth, following the TSBF method (Anderson and Ingram, 1993). In this way, a total of 20 sampling sites (5 × 4) and 180 subunits (20 × 9) were explored for quantitative and qualitative earthworm studies. The collected specimens were washed with water and preserved in 5% formalin for subsequent taxonomic identification. All pertinent morphological and anatomical characterizations of the earthworms were carried out using a Leica stereomicroscope (Model: Leica EZ4). The family level classification followed was based on the criteria set by Misirlioğlu et al. (2023). Additionally, considering the vertical distribution, size, and color of the specimens, the species were categorized into ecological groups (Bouché, 1977; Bottinelli et al., 2020). Finally, the specimens were deposited in the National Zoological Collection in the ZSI, GNC section, Kolkata. Earthworm community structure and species richness were assessed with the help of species diversity indices and a Mondrian plot using PRIMER v.7 (Clarke and Gorley, 2015), respectively. Additionally, Indicator Species Analysis (ISA) was also performed using Past version 4.13 (Hammer et al., 2001) to identify the strength of the species (p < 0.05) with the probability of association to the wildlife sanctuaries.
Results
A total of 22 species belonging to 12 genera and 6 families, viz., Benhamiidae, Megascolecidae, Acanthodrilidae, Rhinodrilidae, Almidae, and Moniligastridae, were collected. Table 1 shows the highest number of species recorded at KUWLS (n = 15) followed by BEWLS (n = 14), BAWLS (n = 11), BIWLS (n = 10), and RAWLS (n = 8). Although KUWLS had the highest number of 15 species, the Shannon diversity index (H’ = 2.34) and Simpson’s index (D = 0.89) were the highest at BEWLS (Table 1). The variance could be attributed to an uneven distribution—where a few species [Drawida nepalensis Michaelsen, 1907, Glyphidrilus gangeticus Gates, 1955 and Pontoscolex corethrurus (Müller, 1857)] dominate the ecosystem while others have significantly fewer individuals (Table 1 and Figure 1A). This disparity within the 15 species might contribute to the lower Shannon diversity index in KUWLS than that in BEWLS. Furthermore, the species were classified into ecological categories, viz., (1) Epigeic: Dichogaster affinis (Michaelsen, 1890), Dichogaster bolaui (Michaelsen, 1891), and Perionyx sp.; (2) Epi-endogeic: Amynthas alexandri (Beddard, 1900), Metaphire houlleti (Perrier, 1872), and Metaphire peguana (Rosa, 1890); (3) Endogeic: Lennogaster chittagongensis (Stephenson, 1917), Lennogaster yeicus (Stephenson, 1931), Octochaetona beatrix (Beddard, 1902), Octochaetona surensis (Michaelsen, 1910), Pontoscolex corethrurus (Müller, 1857), Glyphidrilus gangeticus Gates, 1955, Drawida barwelli (Beddard, 1886), Drawida calebi Gates, 1945, Drawida nepalensis Michaelsen, 1907, Dichogaster modiglianii (Rosa, 1896), Lampito mauritii Kinberg, 1867, Metaphire planata (Gates, 1926), Metaphire posthuma (Vaillant, 1868), and Polypheretima elongata (Perrier, 1872); (4) Anecic: Eutyphoeus orientalis (Beddard, 1883) and Eutyphoeus nicholsoni (Beddard, 1901). Among the identified earthworm communities, the endogeic was the most dominant (13 species), followed by the epigeic (3 species), the epi-endogeic (3 species), and the anecic (2 species). Moreover, the earthworm communities were composed of native and exotic peregrine species with the exotic species Pontoscolex corethrurus being found at most of the sites and dominating the earthworm communities at RAWLS (Table 1).
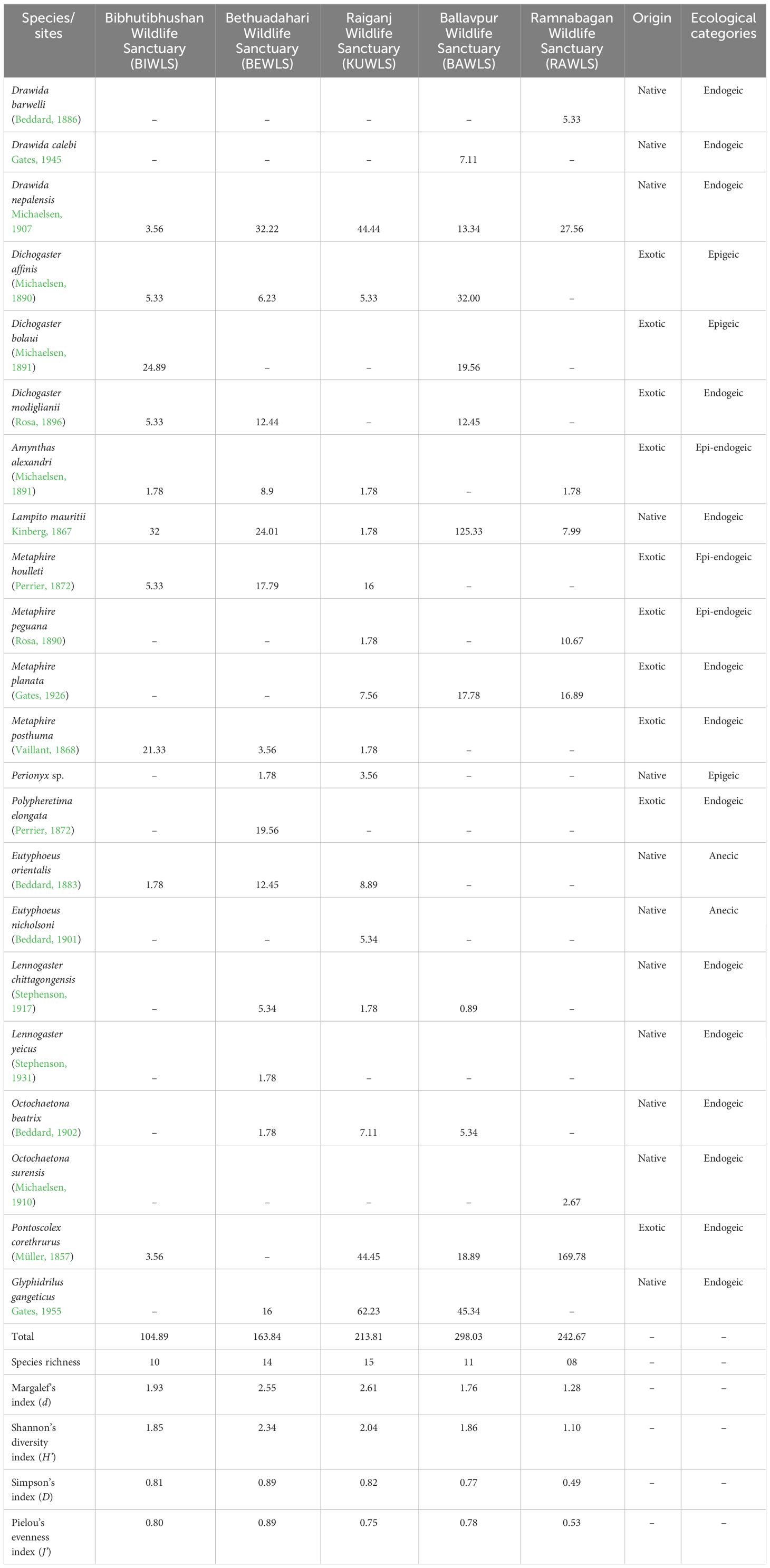
Table 1 Earthworm species abundance (ind. m−2) and diversity indices across different wildlife sanctuaries of West Bengal.
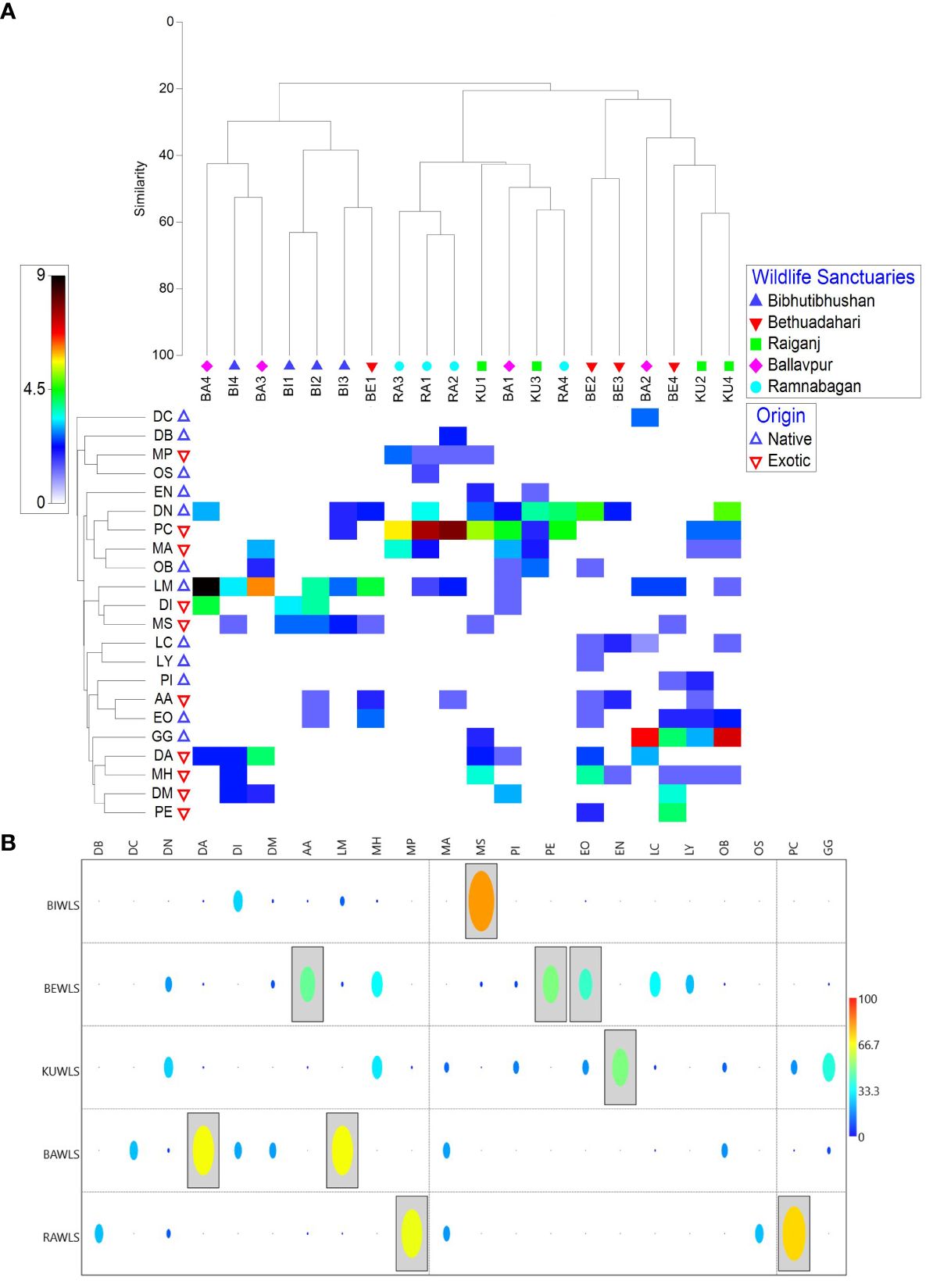
Figure 1 (A) Mondrian plot and (B) Indicator Species Analysis illustrating the strength of the species (p<0.05) with respect to the wildlife sanctuaries, West Bengal, DC, Drawida calebi Gates, 1945; PI, Perionyx sp.; PE, Polypheretima elongata (Perrier, 1872); LM, Lampito mauritii Kinberg, 1867; DI, Dichogaster bolaui (Michaelsen, 1891); MS, Metaphire posthuma (Vaillant, 1868); DM, Dichogaster modiglianii (Rosa, 1896); MA, Metaphire planata (Gates, 1926); OB, Octochaetona beatrix (Beddard, 1902); LY, Lennogaster yeicus (Stephenson, 1931); LC, Lennogaster chittagongensis (Stephenson, 1917); AA, Amynthas alexandri (Beddard, 1900); EO, Eutyphoeus orientalis (Beddard, 1883); DN, Drawida nepalensis Michaelsen, 1907; PC, Pontoscolex corethrurus (Müller, 1857); GG, Glyphidrilus gangeticus Gates, 1955; DA, Dichogaster affinis (Michaelsen, 1890); MH, Metaphire houlleti (Perrier, 1872); EN, Eutyphoeus nicholsoni (Beddard, 1901); DB, Drawida barwelli (Beddard, 1886); MP, Metaphire peguana (Rosa, 1890); OS, Octochaetona surensis (Michaelsen, 1910); BI1–BI4, Bibhutibhushan Wildlife Sanctuary (BIWLS); BE1–BE4, Bethuadahari Wildlife Sanctuary (BEWLS); KU1–KU4, Raiganj Wildlife Sanctuary (KUWLS); BA1–BA4, Ballavpur Wildlife Sanctuary (BAWLS); RA1–RA4, Ramnabagan Wildlife Sanctuary (RAWLS).
The Mondrian plot (Figure 1A) revealed that mixed clustering might be attributed to the presence of peregrine species at each wildlife sanctuary. The exotic peregrine species Pontoscolex corethrurus and the native peregrine species Lampito mauritii and Drawida nepalensis were found in most of the study sites within the wildlife sanctuaries. Although the population of these species varied significantly among the sites, Pontoscolex corethrurus, for example, was predominantly abundant at site RA2 in RAWLS; similarly, Drawida nepalensis was predominantly abundant at KU4 in KUWLS and Lampito mauritii was predominantly abundant at BA4 in BAWLS (Figure 1A). It is interesting to note that the populations of other species were found to be minimal in areas where these species predominated. Furthermore, the study sites within each wildlife sanctuary showed some clustering based on the occurrence and abundance of the observed species (Figure 1A). Four distinct clusters were formed among the sampling sites in the wildlife sanctuaries, namely, BIWLS, KUWLS, RAWLS, and BEWLS, each with similarity scores of 63.08%, 57.32%, 56.78%, and 46.95%, respectively. Additionally, the assessment of ISA identified 9 out of 22 species as significant indicators (p < 0.05) in the selected Wildlife sanctuaries. It included three species in BEWLS, two species each in BAWLS and RAWLS, and one species each in KUWLS and BIWLS (Figure 1B). Except for KUWLS, all other sanctuaries harbor at least one exotic species as an indicator, namely, BIWLS, Metaphire posthuma; BEWLS, Amynthas alexandri; BAWLS, Dichogaster affinis; and RAWLS, Pontoscolex corethrurus. Interestingly, Eutyphoeus nicholsoni was found to be the only endemic species serving as an indicator at KUWLS, which also supports a greater number of species (Figure 1B).
In earthworm reproduction, the clitellum secretes a girdle-like protective and nutritious layer that moves toward the anterior side, collecting the ova from the ovary and sperms from spermathecae (sperm storage) and finally shedding through the anterior-most segment and forming a cocoon (Julka, 1988). A colony of the earthworm species Metaphire houlleti was observed with the parthenogenetic mode of reproduction. This species was found at 7 sites out of 20 in five different wildlife sanctuaries with a population range of 1.78–14.22 ind. m−2. The parthenogenetic morphs were found in a single colony with a population of 14.22 ind. m−2 at only one site near Gharial pound in BEWLS (BE2), where all were athecal morphs. In natural conditions, the formation of a cocoon mostly remains unabsorbed. Interestingly, we managed to collect specimens showing the movement of the girdle toward the anterior, indicating the formation of cocoons in parthenogenetic species. We highlighted this phenomenon in two specimens by varying the degree of movement, one at segment 8 (Figure 2A) and one at segment 11 (Figure 2B). All the parthenogenetic morphs lack spermathecae, in addition to one lacking male pores, but all have well-developed testes, seminal vesicles, and prostate glands. Moreover, the population per meter square was slightly higher (14.22 ind. m−2) in the parthenogenetic colony, as compared to the maximum (12.44 ind. m−2) at another site [Badhur tower (KU1), KUWLS] in a sexually reproducing colony.
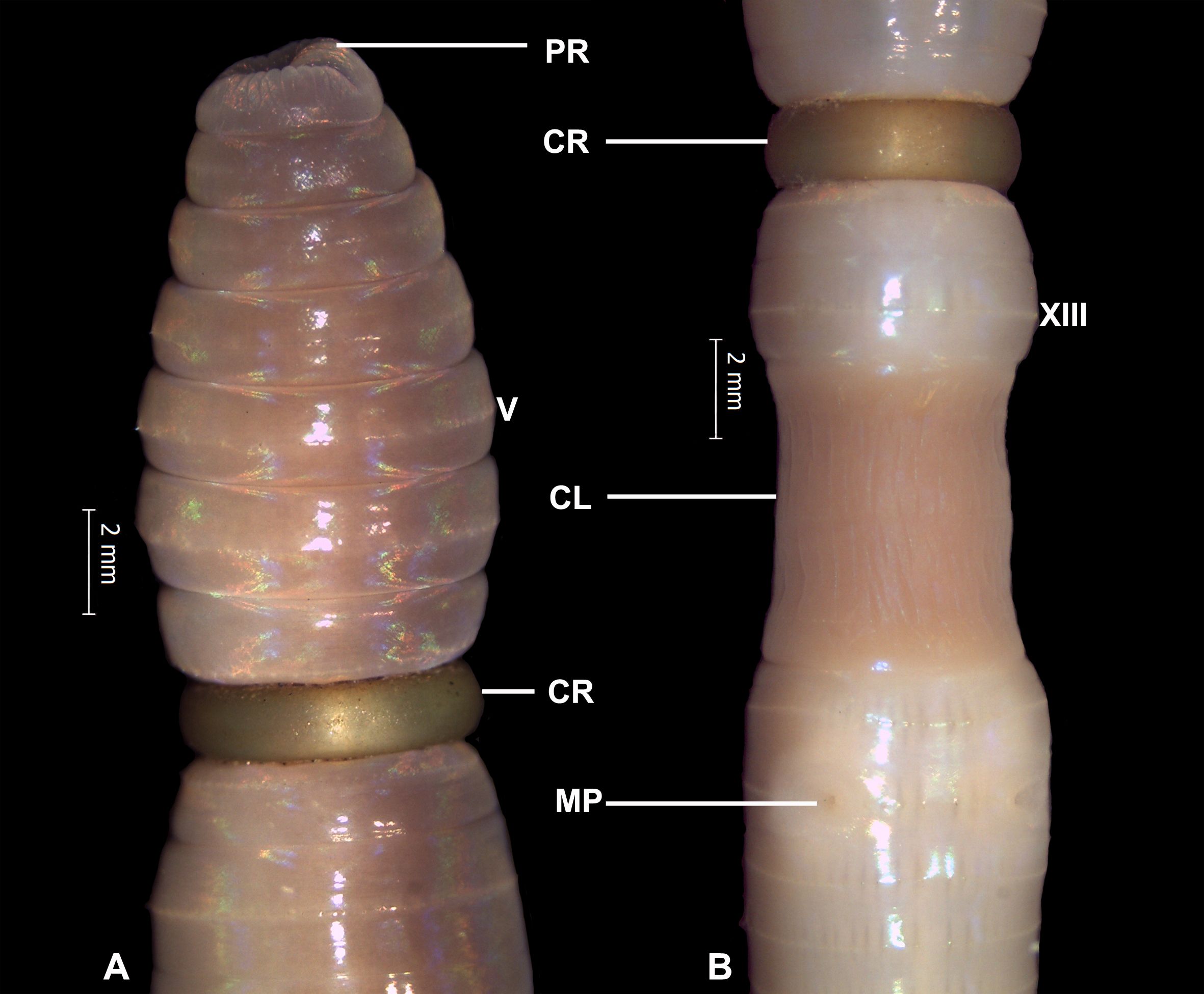
Figure 2 Metaphire houlleti (Perrier, 1872) showing the formation of cocoon in parthenogenetic morphs. (A) Anterior; (B) genital region. PR, Prostomium; CR, Cocoon ring (advance stage); CL, Clitellum; MP, Male pores.
Discussion
Except for a few studies (Hale et al., 2006; Ransom, 2011; Loss et al., 2012; Craven et al., 2017), earthworms are considered an important community for the conservation of a healthy ecosystem and habitat for other vertebrates (Blouin et al., 2013; Martay and Pearce-Higgins, 2020). In this study, 22 species were documented across various wildlife sanctuaries, with species counts ranging from two to eight at each specific site, falling well within the reported range of 1–15 species (Edwards and Bohlen, 1996). The observed variability in species occurrence among these sites may be due to the factors elucidated in various studies such as environmental conditions, food availability, and specific habitat characteristics (Ekschmitt et al., 2003; Phillips et al., 2019; Tóth et al., 2020). Considering the wildlife sanctuaries, a maximum of 15 species were recorded from KUWLS; this site is also known for hosting a substantial annual bird population and has witnessed notable counts of approximately 99,383 birds, including 64,055 open bill storks, 8,969 night herons, 19,841 egrets, and 6,528 cormorants (Wildlife Sanctuary Information). Sometimes, birds and animals play an important role in the migration of earthworm species through soil adhering to their feet (Gates, 1972; Blakemore, 2009). Despite having the highest number of species at KUWLS, the values of the Shannon diversity index (H’ = 2.34) and Simpson’s index (D = 0.89) were the highest at BEWLS, which may be due to the equal distribution of earthworm species. Our findings support those of Lenka et al. (2023), who noted that an even distribution of species in an ecosystem tends to give higher Shannon diversity index values. Furthermore, according to Callaghan et al. (2019), a higher value of H’ indicates greater ecological stability and a more resilient ecosystem capable of supporting various ecological niches. Therefore, it can be concluded that the ecosystem at BEWLS is likely more suitable for the colonization of earthworm species when compared to other wildlife sanctuaries. In the present study, all the sanctuaries were characterized by the distinct indicator species. The changes in the indicator species may be reflected by the changes in the ecosystem (Siddig et al., 2016). To ensure better management strategies and conservation efforts, knowledge of both species diversity and edaphic parameters is necessary (Magray et al., 2022). Hence, it is essential to interpret the edaphic factors with respect to earthworm species diversity in wildlife sanctuaries.
The clustering of nearby study sites associated with each wildlife sanctuary might be due to the local environmental conditions, which play a significant role in the colonization and abundance of earthworm species. Previous studies contributed to the conclusion that local edaphic factors were responsible for the formation of earthworm species population patches (González et al., 2007; Demetrio et al., 2019; Singh et al., 2020; Ahmed et al., 2022). The species Lennogaster yeicus was initially described in Chaungson, Myanmar (Stephenson, 1931), and in India, it has been reported from Himachal Pradesh (Julka, 1988), Uttarakhand (Bhadauria et al., 1997), and Tripura (Chaudhuri et al., 2012; Jamatia and Chaudhuri, 2017). The report of this species in the wildlife sanctuaries is an addition to the earthworm fauna of the state of West Bengal. Overall, the presence of 54.5% native species in the protected forest supports Demetrio et al. (2023) findings, which showed that forest ecosystems support more native species than exotic ones.
Earthworms play an important role in nutrient recycling and plant growth (van Groenigen et al., 2014; Hussain et al., 2018), which is strongly influenced by their ecological categories (Huang et al., 2020). It was observed that litter decomposition substantially increased when worms from all categories were present in an ecosystem (Huang et al., 2020). Moreover, the species recorded in the present study were categorized into ecological groups, aligning with the finding that an earthworm community in an ecosystem is composed of one to two epigeic species, two to four endogeic species, and zero to two species of large anecics (Pop, 1997). In protected areas, the presence of all the ecological categories indicates greater ecological stability and a more resilient ecosystem that can support various ecological niches. Alteration of natural vegetation to pasture, cropland, and tree plantation often alters functional diversity (Decaëns and Jiménez, 2002; Smith et al., 2008). The widespread occurrence of endogeic species such as Pontoscolex corethrurus, Lampito mauritii, and Drawida nepalensis indicates their tolerance to a wide range of edaphic factors.
Parthenogenesis is considered significant due to its ability to preserve polyploidy and promote the expansion of polyploid variations in new regions, as a single worm can initiate a new colony (Gates, 1972). In the present study, a colony of parthenogenetic morphs of the species Metaphire houlleti was recorded from a site in BEWLS. Similar to the parthenogenetic morphs observed in the present study, Blakemore (2012) also reported the presence of iridescent testes and funnels in parthenogenetic morphs, whereas other studies suspected this phenomenon in parthenogenesis (Gates, 1972; Jaenike and Selander, 1979). The findings of Jaenike and Selander (1979) are supported by the current observation of Metaphire houlleti, a parthenogenetic earthworm, in the upper soil organic layer. Interestingly, we observed that this species exhibited both parthenogenetic and sexual reproduction, with the population recording the highest in the former. Our study also supports the findings that parthenogenetic morphs typically have a high reproductive capacity, contribute to rapid population growth, produce resistant cocoons, and exhibit wide environmental or feeding tolerances, a high dispersal rate, and the ability to withstand higher parasitic burdens (Gates, 1972; Jaenike and Selander, 1979; Blakemore, 2012).
Conclusion
Among 22 species identified from various wildlife sanctuaries of West Bengal, the number of species has varied across the sanctuaries. In comparison with the other sanctuaries, BEWLS is determined to be more appropriate for the perpetuation of earthworm communities based on the Shannon diversity index. With the exception of KUWLS, ISA has revealed exotic species as indicators across the majority of the wildlife sanctuaries. At KUWLS, it is observed that the endemic species Eutyphoeus nicholsoni might function as a possible indicator species, which also supports a greater number of species. It is also observed that the range extension of Lennogaster yeicus to the West Bengal state and the widespread occurrence of various species such as Pontoscolex corethrurus, Lampito mauritii, and Drawida nepalensis indicate their high tolerance of a wide range of edaphic factors. It is the need of the hour to assess and interpret the physical process data with respect to the diversity of earthworm species in wildlife sanctuaries to validate such indicator species. Moreover, further molecular studies are required to find out the genetic variations between parthenogenetic and sexually reproducing colonies of the earthworm species Metaphire houlleti.
Data availability statement
The original contributions presented in the study are included in the article/Supplementary Material. Further inquiries can be directed to the corresponding authors.
Ethics statement
The manuscript presents research on animals that do not require ethical approval for their study.
Author contributions
SA: Data curation, Investigation, Methodology, Resources, Writing – original draft, Writing – review & editing. JJ: Data curation, Formal analysis, Writing – review & editing. DB: Conceptualization, Supervision, Writing – review & editing. NM: Data curation, Software, Validation, Writing – original draft, Writing – review & editing.
Funding
The author(s) declare financial support was received for the research, authorship, and/or publication of this article. Through ZSI In-house program, "Faunal diversity of Wildlife Sanctuaries of West Bengal (F206-13/2021-22/15866 & F206-13/2023-24/25413).
Acknowledgments
Thanks are due to the facility for conducting the study to the Ministry of Environment, Forest and Climate Change, Government of India. SA acknowledges the Director, Zoological Survey of India, Kolkata for providing fellowship in the form of PDF to complete the task.
Conflict of interest
The authors declare that the research was conducted in the absence of any commercial or financial relationships that could be construed as a potential conflict of interest.
Publisher’s note
All claims expressed in this article are solely those of the authors and do not necessarily represent those of their affiliated organizations, or those of the publisher, the editors and the reviewers. Any product that may be evaluated in this article, or claim that may be made by its manufacturer, is not guaranteed or endorsed by the publisher.
Supplementary material
The Supplementary Material for this article can be found online at: https://www.frontiersin.org/articles/10.3389/fevo.2024.1372706/full#supplementary-material
References
Ahmed S., Marimuthu N., Tripathy B., Julka J. M., Chandra K. (2022). Earthworm community structure and diversity in different land-use systems along an elevation gradient in the Western Himalaya, India. App. Soil Ecol. 176, 104468. doi: 10.1016/j.apsoil.2022.104468
Anderson J. M., Ingram J. S. (1993). Tropical soil biology and fertility: a handbook of methods. Wallingford: CAB International.
Barros E., Pashanasi B., Constantino R., Lavelle P. (2002). Effects of land-use system on the soil macrofauna in western Brazilian Amazonia. Biol. Fertil. Soils 35, 338–347. doi: 10.1007/s00374-002-0479-z
Beddard F. E. (1883). Note on some earthworms from india. Ann. Mag. Nat. Hist. 12, 213–224. doi: 10.1080/00222938309459619
Beddard F. E. (1886). Notes on earthworms from ceylon and the philippine islands, including a description of two new species. Ann. Mag. Nat. Hist. 17, 89–98. doi: 10.1080/00222938609460120
Beddard F. E. (1900). On a new species of earthworm from india, belonging to the genus amyntas. Proc. Zool. Soc Lond. 1900, 998–1002.
Beddard F. E. (1901). Contribution to the knowledge of the structure and systematic arrangement of earthworms. Proc. Zool. Soc Lond. 1901 (1), 187–206. doi: 10.1111/j.1469-7998.1901.tb08539.x
Beddard F. E. (1902). On two new earthworms of the family megascolecidae. Ann. Mag. Nat. Hist. 9 (7), 456–463. doi: 10.1080/00222930208678621
Bhadauria T., Ramakrishnan P. S., Srivastava K. N. (1997). Population dynamics of earthworms during crop rotation under rainfed agriculture in central Himalayas, India. Appl. Soil Ecol. 6, 205–215. doi: 10.1016/S0929-1393(97)00008-5
Blakemore R. J. (2009). “Cosmopolitan earthworms: A global and historical perspective,” in Annelids in Modern Biology. Ed. Shain D. H. (Wiley-Blackwell, Hoboken, NJ), 257–283. doi: 10.1002/9780470455203.ch14
Blakemore R. J. (2012). Cosmopolitan Earthworms an Eco-taxonomic Guide to the Peregrine Species of the World. 5th ed (Yokohama, Japan: Verm Ecology Solutions), 850.
Blouin M., Hodson M. E., Delgado E. A., Baker G., Brussaard L., Butt K. R., et al. (2013). 19A review of earthworm impact on soil function and ecosystem services. Eur. J. Soil Sci. 64, 161–182. doi: 10.1111/ejss.12025
Bottinelli N., Hedde M., Jouquet P., Capowiez Y. (2020). An explicit definition of earthworm ecological categories–Marcel Bouché’s triangle revisited. Geoderma 372, 114361. doi: 10.1016/j.geoderma.2020.114361
Bouché M. B. (1972). Lombriciens de France. Écologie et Systématique. INRA Publication. (Paris: INRA Publication; Institut National des Recherches Agriculturelles), 1–671.
Bouché M. B. (1977). Strategies lombriciennes. Ecol. Bull. 25, 122–132. Available at: https://www.jstor.org/stable/20112572.
Callaghan C. T., Bino G., Major R. E., Martin J. M., Lyons M. B., Kingsford R. T. (2019). Heterogeneous urban green areas are bird diversity hotspots: insights using continental-scale citizen science data. Landsc. Ecol. 34, 1231–1246. doi: 10.1007/s10980-019-00851-6
Chaudhuri P. S., Dey A., Bhattacharjee G., Nath S. (2012). Earthworm diversity in Tripura - present status. Sci. Cult. 78, 343–346. Available at: https://www.researchgate.net/publication/277312724.
Chen S. Y., Hsu C. H., Soong K. (2021). How to cross the sea: Testing the dispersal mechanisms of the cosmopolitan earthworm Pontodrilus litoralis. R. Soc Open Sci. 8, 202297. doi: 10.1098/rsos.202297
Clarke K. R., Gorley R. N. (2015) Getting started with PRIMER v7. PRIMER-E: Plymouth. Available online at: https://it.scribd.com/document/268811163/Getting-Started-With-PRIMER-7.
Craven D., Thakur M. P., Cameron E. K., Frelich L. E., Beauséjour R., Blair R. B., et al. (2017). The unseen invaders: introduced earthworms as drivers of change in plant communities in North American forests (a meta-analysis). Glob. Change Biol. 23, 1065–11074. doi: 10.1111/gcb.13446
Cui H., Wagg C., Wang X., Liu Z., Liu K., Chen S., et al. (2022). The loss of above-and belowground biodiversity in degraded grasslands drives the decline of ecosystem multifunctionality. Appl. Soil Ecol. 172, 104370. doi: 10.1016/j.apsoil.2021.104370
Decaëns T., Jiménez J. J. (2002). Earthworm communities under an agricultural intensification gradient in Colombia. Plant Soil 240, 133–143. doi: 10.1023/A:1015844610604
Demetrio W. C., PM D. F., Dudas R., Zagatto M. G., Feijoo A., Brown G. G. (2023). Earthworm species in native and planted forests in Brazil. Zootaxa 5255, 304–323. doi: 10.11646/zootaxa.5255.1
Demetrio W. C., Ribeiro R. H., Nadolny H., Bartz M. L., Brown G. G. (2019). Earthworms in Brazilian no-tillage agriculture: Current status and future challenges. Eur. J. Soil Sci. 71, 988–1005. doi: 10.1111/ejss.12918
Díaz Cosín D. J., Novo M., Fernández R. (2011). “Reproduction of earthworms: sexual selection and parthenogenesis,” in Biology of Earthworms, Soil Biology, vol. 24 . Ed. Karaca A. (Springer-Verlag, Berlin, Heidelberg), 69–86. doi: 10.1007/978–3-642–14636-7_5
Domínguez J., Velando A., Aira M., Monroy F. (2003). Uniparental reproduction of Eisenia fetida and E. andrei (Oligochaeta: Lumbricidae): evidence of self-insemination. The 7th international symposium on earthworm ecology · Cardiff · Wales · 2002. Pedobiologia 47, 530–534. doi: 10.1078/0031–4056-00224
Duarte A. C., Guerra C. A., Cano-Díaz C., Zeiss R., Carvalho-Santos C., Carvalho R. P., et al. (2024). Effects of protected areas on soil nematode communities in forests of the North of Portugal. Biodivers. Conserv. 33, 73–89. doi: 10.1007/s10531-023-02732-6
Edwards C. A., Bohlen P. J. (1996). “The influence of environmental factors on earthworms,” in Biology and ecology of earthworms. Eds. Edwards C. A., Bohlen P. J. (Chapman & Hall, London), 134–162.
Ekschmitt K., Stierhof T., Dauber J., Kreimes K., Wolters V. (2003). On the quality of soil biodiversity indicators: abiotic and biotic parameters as predictors of soil faunal richness at different spatial scales. Agr. Ecosyst. Environ. 98, 273–283. doi: 10.1016/S0167-8809(03)00087-2
Fründ H. C., Graefe U., Tischer S. (2011). “Earthworms as bio-indicators of soil quality,” in Biology of Earthworms, Soil Biology, vol. 24 . Ed. Karaca A. (Springer-Verlag, Berlin, Heidelberg), 261–278. doi: 10.1007/978–3-642–14636-7_16
Gates G. E. (1926). Note on a new species of Pheretima from rangoon. Ann. Mag. Nat. Hist. 17 (100), 411–415. doi: 10.1080/00222932608633432
Gates G. E. (1945). On some indian earthworms. Proc. Natl. Acad. Sci. India B 21 (4), 208–283. doi: 10.1007/BF03049816
Gates G. E. (1955). On indian and burmese earthworms of the genus Glyphidrilus. Rec. Zool. Surv. India 53 (1-2), 53–65. doi: 10.26515/rzsi/v53/i1-2/1955/162021
Gates G. E. (1972). Burmese earthworms: an introduction to the systematics and biology of megadrile oligochaetes with special reference to Southeast Asia. Trans. Amer. Philos. Soc 62, 1–326. doi: 10.2307/1006214
González G., García E., Cruz V., Borges S., Zalamea M., Rivera M. M. (2007). Earthworm communities along an elevation gradient in Northeastern Puerto Rico. Eur. J. Soil Biol. 43, S24–S32. doi: 10.1016/j.ejsobi.2007.08.044
Hale C. M., Frelich L. E., Reich P. B. (2006). Changes in hardwood forest understory plant communities in response to European earthworm invasions. Ecology 87, 1637–1649. doi: 10.1890/0012-9658(2006)87[1637:CIHFUP]2.0.CO;2
Hammer O., Harper D. A. T., Ryan P. D. (2001). PAST: paleontological statistics software package for education and data analysis. Palaeont. Electr. 4, 9. Available at: http://palaeo-electronica.org/2001_1/past/issue1_01.htm.
Huang W., González G., Zou X. (2020). Earthworm abundance and functional group diversity regulate plant litter decay and soil organic carbon level: A global meta-analysis. Appl. Soil Ecol. 150, 103473. doi: 10.1016/j.apsoil.2019.103473
Hussain N., Abbasi T., Abbasi S. A. (2018). Generation of highly potent organic fertilizer from pernicious aquatic weed Salvinia molesta. Environ. Sci. pollut. Res. 25, 4989–5002. doi: 10.1007/s11356-017-0826-0
Jaenike J., Selander R. K. (1979). Evolution and ecology of parthenogenesis in earthworms. Am. Zool. 19, 729–737. doi: 10.1093/icb/19.3.729
Jamatia S. K. S., Chaudhuri P. S. (2017). Earthworm community structure under tea plantation (Camellia sinensis) of Tripura (India). Trop. Ecol. 58, 105–113. Available at: https://www.researchgate.net/publication/316543348.
Julka J. M. (1988). The fauna of India and the adjacent countries. Megadrile: Oligochaeta (Earthworms). Haplotaxida: Lumbricina: Megascolecoidea: Octochaetidae (Calcutta: Zoological Survey of India), 400.
Kinberg J. G. H. (1867). “Annulata nova,” in Öfversigt af kongl, vol. 23. (Stockholm: Vetenskaps–akademiens forhandlingar), 97–103.
Lavelle P., Decaens T., Aubert M., Barot S., Blouin M., Bureau F., et al. (2006). Soil invertebrates and ecosystem services. Eur. J. Soil Biol. 42, S3–S15. doi: 10.1016/j.ejsobi.2006.10.002
Lenka R., Tripathy B., Sharma L. K., Chandra K. (2023). Understanding the bird species assemblages found in and around Thane creek, Maharashtra, India. Ornithol. Res. 31, 282–293. doi: 10.1007/s43388-023-00153-1
Loss S. R., Niemi G. J., Blair R. B. (2012). Invasions of non-native earthworms related to population declines of ground-nesting songbirds across a regional extent in northern hardwood forests of North America. Landsc. Ecol. 27, 683–696. doi: 10.1007/s10980-012-9717-4
Lowe C. N., Butt K. R. (2008). Life cycle traits of the parthenogenetic earthworm Octolasion cyaneum (Savigny 1826). Eur. J. Soil Biol. 44, 541–544. doi: 10.1016/j.ejsobi.2008.08.002
Magray J. A., Wani B. A., Islam T., Ganie A. H., Nawchoo I. A. (2022). Phyto-ecological analysis of Phytolacca acinosa Roxb. assemblages in Kashmir Himalaya, India. Front. For. Glob. Change 5. doi: 10.3389/ffgc.2022.976902
Martay B., Pearce-Higgins J. W. (2020). Opening a can of worms: can the availability of soil invertebrates be indicated by birds? Ecol. Indic. 113, 106222. doi: 10.1016/j.ecolind.2020.106222
Michaelsen W. (1890). Beschreibung der von herrn dr. stuhlmann im mudungsgebiet des sambesi gesammelten terricolen. Jahrbuch der hamburgischen wissenschaftlichen Anstalten 7, 1–30.
Michaelsen W. (1891). Oligochaeten des naturhistorischen museums in hamburg. IV Vol. 8 (Jahrbuch der hamburgischen wissenschaftlichen Anstalten), 3–42.
Michaelsen W. (1907). Neue oligochaten von vorder-indien, ceylon, birma und den andaman-inseln. Mitt. naturhist. Mus. Hamburg 24 (2), 143–188.
Michaelsen W. (1910). Die oligochäten fauna der vorderindisch-ceylonischen region. Abh. Geb. Naturw. Hamburg 19 (5), 1–108.
Müller F. (1857). Lumbricus corethrurus, busten-schwanz. Archiv für Naturgeschichte 23 (1), 113–116.
Misirlioğlu M., Reynolds J. W., Stojanović M., Trakić T., Sekulić J., James S. W., et al. (2023). Earthworms (Clitellata, Megadrili) of the world: an updated checklist of valid species and families, with notes on their distribution. Zootaxa 5255, 417–438. doi: 10.11646/zootaxa.5255.1.33
Nouri-Aiin M., Connolly S., Keough C., Smigelsky A. J., Wen Y., Howland J., et al. (2022). Genetic population structure and reproductive system of two invasive Asian earthworms, Amynthas tokioensis and Amynthas agrestis. PeerJ 10, e13622. doi: 10.7717/peerj.13622
Pauli N., Barrios E., Conacher A. J., Oberthür T. (2011). Soil macrofauna in agricultural landscapes dominated by the Quesungual Slash-and-Mulch Agroforestry System, western Honduras. Appl. Soil Ecol. 47, 119–132. doi: 10.1016/j.apsoil.2010.11.005
Pérès G., Vandenbulcke F., Guernion M., Hedde M., Beguiristain T., Douay F., et al. (2011). Earthworm indicators as tools for soil monitoring, characterization and risk assessment. An example from the national Bioindicator programme (France). Pedobiologia 54, S77–S87. doi: 10.1016/j.pedobi.2011.09.015
Perrier E. (1872). Recherches pour servir à l’historie des lombriciens terrestres. Nouvelles Arch. du Museum d’Histoire Naturelle Paris 8, 5–198. doi: 10.5962/bhl.title.12201
Phillips H. R. P., Guerra C. A., Bartz M. L. C., Briones M. J. I., Brown G., Crowther T. W., et al. (2019). Global distribution of earthworm diversity. Science 366, 480–485. doi: 10.1126/science.aax4851
Pop V. V. (1997). Earthworm-vegetation-soil relationships in the Romanian carpathians. Soil Biol. Biochem. 29, 223–229. doi: 10.1016/S0038-0717(96)00168-X
Ransom T. S. (2011). Earthworms, as ecosystem engineers, influence multiple aspects of a salamander's ecology. Oecologia 165, 745–754. doi: 10.1007/s00442-010-1775-1
Reynolds J. W. (1974). Are oligochaetes really hermaphroditic anphimictic organisms? Biologist 56, 90–99. Available at: https://www.researchgate.net/publication/288940049.
Rosa D. (1890). Viaggio di leonardo fea in birmania e regioni vicine. XXVI. perichetidi. Annali Mus. civ. Stor. Nat. Giacomo Doria Genova 2, 107–122.
Rosa D. (1896). I lombrichi raccolti a sumatra dal dott. Elio Modigliani. Annali del Museo Civico di Storia Naturale di Genova Serie 2 (16), 502–532.
Siddig A. A., Ellison A. M., Ochs A., Villar-Leeman C., Lau M. K. (2016). How do ecologists select and use indicator species to monitor ecological change? Insights from 14 years of publication in Ecological Indicators. Ecol. Ind. 60, 223–230. doi: 10.1016/j.ecolind.2015.06.036
Singh S., Sharma A., Khajuria K., Singh J., Vig A. P. (2020). Soil properties changes earthworm diversity indices in different agro-ecosystem. BMC Ecol. 20, 1–14. doi: 10.1186/s12898-020-00296-5
Smith R. G., McSwiney C. P., Grandy A. S., Suwanwaree P., Snider R. M., Robertson G. P. (2008). Diversity and abundance of earthworms across an agricultural land-use intensity gradient. Soil Tillage Res. 100, 83–88. doi: 10.1016/j.still.2008.04.009
Sosa I. D., Marchán D. F., Novo M., Cosín D. J. D., Giribet G., Fernández R. (2017). Insights into the origin of parthenogenesis in oligochaetes: strong genetic structure in a cosmopolitan earthworm is not related to reproductive mode. Eur. J. Soil Biol. 81, 31–38. doi: 10.1016/j.ejsobi.2017.06.003
Stephenson J. (1917). On a collection of oligochaeta from various parts of india and further india. Rec. Zool. Surv. India 13, 353–416. doi: 10.26515/rzsi/v13/i6/1917/163594
Stephenson J. (1931). Descriptions of Indian Oligochaeta II. Rec. Zool. Surv. India 33, 173–202. doi: 10.26515/rzsi/v33/i2/1931/162500
Terhivuo J., Saura A. (2003). Low clonal diversity and morphometrics in the parthenogenetic earthworm Octolasion cyaneum (Sav.): The 7th international symposium on earthworm ecology· Cardiff· Wales· 2002. Pedobiologia 47, 434–439. doi: 10.1016/S0031-4056(04)70218-8
Tóth Z., Szlavecz K., Epp Schmidt D. J., Hornung E., Setälä H., Yesilonis I. D., et al. (2020). Earthworm assemblages in urban habitats across biogeographical regions. Appl. Soil Ecol. 151, 103530. doi: 10.1016/j.apsoil.2020.103530
Vaillant L. (1868). Note sur l’anatomie de deux espèces du genre perichaeta etessai de classification des annelides lombricines. Annales Des. Sci. Naturelles 10, 225–256.
Keywords: ecological categories, parthenogenesis, range extension, spatial variability, wildlife sanctuaries
Citation: Ahmed S, Julka JM, Banerjee D and Marimuthu N (2024) Spatial distribution of earthworm community structure along the wildlife sanctuaries of West Bengal, with a glimpse of parthenogenesis in Metaphire houlleti (Perrier, 1872). Front. Ecol. Evol. 12:1372706. doi: 10.3389/fevo.2024.1372706
Received: 18 January 2024; Accepted: 25 April 2024;
Published: 14 May 2024.
Edited by:
Lise Dupont, Université Paris-Est Créteil Val de Marne, FranceReviewed by:
Lucio Lucadamo, University of Calabria, ItalyShweta Yadav, Dr. Hari Singh Gour University, India
Copyright © 2024 Ahmed, Julka, Banerjee and Marimuthu. This is an open-access article distributed under the terms of the Creative Commons Attribution License (CC BY). The use, distribution or reproduction in other forums is permitted, provided the original author(s) and the copyright owner(s) are credited and that the original publication in this journal is cited, in accordance with accepted academic practice. No use, distribution or reproduction is permitted which does not comply with these terms.
*Correspondence: Shakoor Ahmed, c2hha29vcmFobWVkMjA0QGdtYWlsLmNvbQ==; Nithyanandam Marimuthu, bWFyaW5lbWFyaUBob3RtYWlsLmNvbQ==