- 1Laboratory of Biocorrosion and Biotechnology, Corporación para la Investigación de la Corrosión (CIC), Piedecuesta, Colombia
- 2Food Microbiome and Health, Quadram Institute, Norwich Research Park, Norwich, United Kingdom
- 3Research Group in Industrial Biotechnology and Molecular Biology - CINBIN, Escuela de Biología, Facultad de Ciencias, Universidad Industrial de Santander (UIS), Bucaramanga, Colombia
Citrus cultivation is vital to global agriculture, necessitating a comprehensive understanding of the soil microbiome’s diversity for sustainable practices. This study provides initial insights into the bacteriome in citrus crops in Santander, Colombia, employing a holistic approach combining culture-based techniques, sequencing methods, and bioinformatics analyses. The study explores organic and non-organic cultivation systems, revealing statistically significant differences in bacterial community composition between both practices. In general, the communities are dominated by members of the Actinobacteria and Proteobacteria, along with bacterial orders Gaiellales and Burkholderiales, all contributing to intricate ecological processes. Culture-based methods aided in the isolation of potential biotechnologically relevant strains. Among them, strain CP102 showed a pronounced carboxymethylcellulose (CMC) degradation capacity. Genetic analysis of the isolate resulted in the generation of the first closed genome of a member of the species Enterobacter soli and identified an unreported 109 kb plasmid. Further genomic examination revealed genes potentially associated with cellulose degradation in this species, which provides the isolate with biotechnological potential. This research significantly advances the global understanding of citrus-associated bacteriomes, shaping future agricultural practices and promoting the development of sustainable bioproducts.
1 Introduction
Citrus cultivation stands as a cornerstone of global agriculture, renowned for its substantial economic and nutritional impact. At the heart of this importance lies the role of the bacteriome in soil and plant health. These complex microbial communities are instrumental in facilitating nutrient uptake, promoting growth, and providing robust protection against a range of pathogens, thus ensuring the vitality and productivity of citrus crops (Berendsen et al., 2012; Liu et al., 2012; Donkersley et al., 2018). The symbiotic relationship between citrus plants and their bacteriome is gaining scientific interest, particularly in the face of challenges such as rising global temperatures, nutrient scarcity, and prevalent plant diseases that compromise yield and quality (Zhang et al., 2021; Srivastava et al., 2022). Furthermore, soil microbiomes represent a rich reservoir of resources for biotechnologically relevant processes such as nitrogen fixation, phosphate solubilization, biocatalysts, secondary metabolites, and other biomolecules, etc (Cherni et al., 2019; Timofeeva et al., 2023). Understanding soil microbiomes is a critical step towards improving aspects of citriculture and agriculture as a whole (Berg, 2009; Chaparro et al., 2012). As agriculture grapples with various challenges, the study of soil bacteriomes in citrus cultivation becomes increasingly vital. Their comprehension and the harnessing of beneficial microbes in contemporary agricultural practices are indispensable for sustaining production levels and matching the demands of a growing population (Nihorimbere et al., 2011; Xu et al., 2018; Su et al., 2023).
The aim of this study is to comprehensively characterize the microbial communities associated with citrus crops in the localities of Girón and Páramo in Santander, Colombia. By using an integrative approach that combines 16S rRNA gene sequencing and conventional culture-based methodologies, this research endeavors to provide a glimpse into the soil microbiome profiles from these understudied citrus-producing areas in the eastern Andes region of Colombia. Such a dual approach leverages the strengths of both high-throughput sequencing for broad microbial identification and culture-based techniques for isolating and characterizing specific microorganisms with biotechnological potential. The elucidation of unique microbial signatures in these regions provides critical knowledge that is essential for shaping future agricultural practices and driving biotechnological innovations, particularly within the varied ecological landscapes of Colombia. These pioneer insights are anticipated to help in the development of sustainable farming strategies, improve soil management techniques, and catalyze the discovery and application of microbial-derived bioproducts.
2 Materials and methods
2.1 Sample collection
Soil samples were collected from the municipalities of Girón (Sotavento farm - 6° 58´ 20.4024” N 73° 8´ 13.47” W) and Páramo (Vista Hermosa farm – 6° 27´ 42.0552” N 73° 9´ 4.3308” W) in the department of Santander, Colombia. Permission for the sampling and processing of Colombian biodiversity was granted by the National Ministry of Environment through contract ARG-167-2017, addenda N°1 and N°2. Seventeen soil samples were collected in Girón from three different locations (plots 1-3), dedicated to the organic production of Citrus latifolia and C. sinensis. As reference, one additional sample from a non-cultivated plot in Girón (plot 0) was collected. Also, samples from the two organic fertilizers used in the farm, prepared from chicken manure (B20) and worm castings (B21), were retrieved for microbiological analysis. In Páramo, the non-organic production crops of C. latifolia and C. limon within the farm are organized as a single plot (plot 4). From there, six soil samples were retrieved. To collect the top layer of the mineral soil (A horizon), 3 cm of topsoil was removed, and samples were taken at 30 cm from the plant stem and from a depth of 20-30 cm. Each sample was processed by resuspending 2 g of soil or fertilizing material in 18 mL PBS for microbiological analysis, and 5 g were collected in sterile centrifuge tubes for molecular analyses. Subsequently, 500g of soil were placed in sterile vacuum-sealed bags for physicochemical analysis (for methods see Supplementary Material).
2.2 Microbial enrichment and isolation
Enrichment cultures in liquid modified M9 (Reasoner and Geldreich, 1985; Puentes-Cala et al., 2023), and R2A (Chaudhary et al., 2019) were prepared from each sampled plot. According to the type of microorganism to be enriched the medium and carbon source varied. For cellulose-degrading bacteria the modified M9 medium was supplemented with 2 g/L carboxymethylcellulose (CMC), while for plastic degrading microorganisms 2 g/L 500 µm low-density polyethylene granules (LDPE) were added. When preparing solid M9 medium, agar-agar (20 g/L) was washed three times with distilled water to eliminate traces of contaminants. The M9 enrichments were incubated for 10 days at room temperature to allow for microbial growth. This was followed by a transfer to fresh media and allowed to grow for 10 additional days. Subsequently, 100µL of 10-6 and 10-8 dilutions were transferred to solid M9. Cellulolytic isolates were identified by the formation of a clear halo stained with a 0.1% w/v Congo red solution (Teather and Wood, 1982; Dantur et al., 2015). Potential low-density polyethylene (LDPE) degraders were identified by their ability to grow on M9 agar plates with LDPE as the only carbon source. Concurrently, R2A enrichments supplemented with heavy metals were employed to select for metal-tolerant bacteria. The modified R2A medium contained (g/L): yeast extract 0.5, peptone 0.5, sodium casein 0.5, glucose 0.5, soluble starch 0.5, sodium pyruvate 0.3, K2HPO4 0.3, and MgSO4 0.024. pH was adjusted to 7 before autoclaving. To select for tolerant microbes, metal concentrations were gradually increased. First, samples were used to inoculate R2A broth supplemented with 1 mM Pb(NO3)2 or 5mM ZnSO4. After 14 days at room temperature, enriched organisms were subjected to two cycles of inoculation in fresh R2A. In the first round, twice the initial concentrations of Pb2+ or Zn2+ (i.e., 2mM or 10mM, respectively) were used, followed by a second transfer into R2A with three times the initial metal concentration (3 mM Pb(NO3)2 or 15mM ZnSO4.7H2O). Finally, tolerant bacteria were isolated on solid R2A medium and preserved at -80°C in 20% glycerol.
2.3 DNA extraction and sequencing
Metagenomic DNA was extracted from the soil samples with the DNeasy PowerSoil kit (Qiagen, Hilden, Germany) according to the manufacturer’s protocol. DNA concentration and quality were determined using a NanoPhotometer® NP80 (OD260/OD280). Subsequently, DNA samples were sent to Novogene Corporation Inc. (California, USA) for partially sequencing the 16S rRNA gene using primers 341F CCTAYGGGRBGCASCAG and 806R GGACTACNNGGGTATCTAAT on Illumina Novaseq PE250 platform (Illumina, USA). Genomic DNA of the isolates was extracted using the protocol described by (Martín-Platero et al., 2007). DNA quality was assessed by agarose gel electrophoresis and measuring the concentration using the Qubit™ Fluorometer (ThermoFisher-Scientific). Sequencing of the 16S rRNA gene was carried out with a MinION Mk1C (Oxford Nanopore Tech., UK), using the 16S Barcoding Kit 1-24 (SQK-16S024) and FLO-MIN106 (R9.4.1) flow cells. Whole genome of the cellulolytic strain CP102 was sequenced using Illumina NovaSeq 6000 (PE150) and Nanopore MinION platforms (Ligation Sequencing Kit (SQK-LSK109)).
2.4 Bioinformatics analyses
2.4.1 Metataxonomic composition with the amplicon 16S rRNA
Demultiplexed FASTQ files from the V3-V4 region were analyzed using Qiime2 v.2022.2. Sequences were joined, filtered, denoised, and trimmed to 405 bp with Deblur (Amir et al., 2017). The amplicon sequence variants (ASVs) were classified taxonomically using the Naive Bayes classifier trained on SILVA v.138.1 database (Quast et al., 2013). For further processing, the generated datasets were imported into the R environment v.4.3.1 (R Core Team, 2023). Relative abundances were assessed using phyloseq v.1.46.0 (McMurdie and Holmes, 2013). Alpha diversity was expressed as Shannon and Chao1 indexes. Statistical differences between locations were assessed with the Mann-Whitney U test. Beta diversity was evaluated by calculating dissimilarity using the Bray-Curtis distance metrics. For ordination, the ASV counts were log-transformed and standardized using the Hellinger method with the vegan (v.2.6) package in R. Multiple regression of the physicochemical soil properties and location with the ordination axis was estimated using the envfit function with 999 permutations.
2.4.2 Whole genome analysis and in silico species
A de novo hybrid assembly was conducted using Unicycler v.0.4.8 (Wick et al., 2017) on the European Galaxy server (The Galaxy Community, 2022). The assembly’s quality was evaluated by QUAST v.5.2.0 (Gurevich et al., 2013). Functional annotation of the genome and plasmid was carried out with prokka v.1.14.6 (Seemann, 2014). A whole genome-based taxonomic analysis was performed with the Genome-to-Genome Distance Calculator (GGDC) and the Type (Strain) Genome Server (TYGS) (Meier-Kolthoff and Göker, 2019). Determination of the closest type strains was made by comparing the CP102 genome against all type strains genomes in the TYGS database via the MASH algorithm (Ondov et al., 2016). In addition, other related strains were identified by 16S rDNA sequence comparison against the 19,412 type stains in the TYGS database (Nov.13.23). From the two approaches, the 10 closest type strains were determined using the Genome BLAST Distance Phylogeny approach (GBDP) (Meier-Kolthoff et al., 2013). Digital DDH values and confidence intervals were calculated using the default settings of the GGDC 4.0 (Meier-Kolthoff et al., 2013, Meier-Kolthoff et al., 2022). Average Nucleotide Identity (ANI) was calculated using OAT v.0.93.1 (Lee et al., 2016). Circular comparisons of the genome and plasmid with their closest relatives were performed using the BLAST Ring Image Generator (BRIG) (Alikhan et al., 2011).
3 Results
3.1 Chemical properties of collected samples
We determined the physicochemical properties of citrus production soils from four plots in Girón and one plot in Páramo, located in the eastern Andes region of Colombia (Supplementary Table 1). Although geographically close, Girón and Páramo exhibit slightly different climatic conditions. On average, Girón is situated 777 meters above sea level, has a mean annual temperature of 24.5°C, and receives annual precipitation of 933 mm. In contrast, Páramo is on average at 1,564 meters above sea level, with mean annual temperatures of 21°C and annual precipitation of 1,600 mm. Since Colombia lies close to the equator, no strong seasonal variations take place in any of the municipalities.
Differences in soil textures were observed between the two sampling locations. While soil samples from Giron exhibited a predominantly sandy texture, those from Páramo were classified as sandy loam. This distinction underscores the higher moisture retention capacity of soils in Páramo. Humidity, organic carbon, potassium, sodium, and iron content were higher in Páramo than in Girón (p ≤ 0.005) (Supplementary Table 1). Interestingly, the uncultivated and unfertilized plot in Girón (plot 0) exhibited the lowest levels of electrical conductivity, humidity, and nitrogen content. In all cases, the pH values were in the slightly acidic range, with plots 1-3 the closest to neutrality. The humidity content in Girón ranged from 4.96-9.8%, while in Páramo had a remarkable 25.03% despite being collected 2 weeks apart, suggesting substantial differences in soil water content, which is crucial for microbial activity and nutrient uptake. Organic carbon, a key component for soil fertility and structure, also varied, with Plot 4 showing the highest percentage (2.36%) and plot 1 the lowest (0.437%). This variation in organic carbon may impact the microbial diversity and activity in these soils.
Phosphorus (P) and nitrogen (N) levels, essential for plant growth varied within plots with the highest nitrogen content observed in Plot 4 (0.182%) and the highest phosphorus content in Plot 3 (0.3%). These physicochemical characteristics provide a foundation for understanding the diverse environmental conditions faced by citrus crops in this region and their potential impact on the soil-associated bacteriome.
3.2 Effect of farming system on bacteriome composition diversity
To characterize the microbiome in soil plots (A horizon) from two citrus plantations, 18 soil samples were collected from an organic citrus plantation and five 5 from a farm utilizing mineral fertilizers. These samples underwent metataxonomics analysis and bacterial isolation in minimal media. For the former, the amplicon sequencing and subsequent data processing generated 3,588,159 16S rRNA reads, which were classified into 8,650 amplicon sequence variants (ASV). The observed ASV counts displayed considerable variability, ranging from 1,103 to 1,925 across samples. The alpha diversity, which describes the richness (number of taxa) within a community, was contextualized using the Chao1 estimator, which suggested potential species richness values spanning from 1,220 to 2,480, indicating that the actual biodiversity might exceed the observed counts. Moreover, we used Shannon diversity index, which considers both species abundance and evenness, ranged from 4.64 to 6.73 across the samples. These values reflect the amount of species and their relative abundances within the soil communities among the different plots sampled in this study. Next, we assessed the richness differences between the Páramo and Girón localities, pairwise Wilcoxon rank-sum exact tests were applied to the observed ASVs, Chao1 richness estimates, and Shannon diversity indices. We did not detect significant differences in species richness between the two locations (p-values: 0.19 for ASVs, 0.22 for Chao1, and 0.49 for the Shannon index). To compare the variability in community composition (beta diversity) between Páramo and Girón, as well as the physicochemical soil properties, we used a constrained ordination analysis (Supplementary Figure S1). This analysis showed a clear separation between the plots from Páramo and Girón, driven by humidity, organic carbon, organic matter, potassium and iron, although none were significant. The location factor accounted for only a small proportion of the variance (r2 = 0.015).
At phylum level, the data revealed a significant presence of Actinobacteria and Proteobacteria (Figure 1), two key bacterial phyla in soil ecological processes (Huang et al., 2014; Deng et al., 2018). Actinobacteria are essential decomposers of organic matter, releasing nutrients crucial for plant growth and producing antibiotics and hormones (Kopecky et al., 2011; Lewin et al., 2016; Chen et al., 2023). Meanwhile, Proteobacteria contribute to nutrient cycling, soil fertility, and plant health (Wei et al., 2018; Mhete et al., 2020; Zhou et al., 2023). Other well-represented phyla included Acidobacteria and Bacteroidota, also relevant for their roles in decomposing complex organic substrates and contributing to carbon and nitrogen cycling, sulfur biogeochemistry and enzyme secretion (Banerjee et al., 2018; Kalam et al., 2020; Larsbrink and McKee, 2020; Kruczyńska et al., 2023). The soil microbiomes of Girón and Páramo portrayed a diverse array of bacterial orders, featuring the presence of Gaiellales, which among others like Burkholderiales, contribute to intricate processes such as promoting soil fertility, plant health, and ecosystem resilience through their involvement in organic matter decomposition and the nitrogen cycle (Tong et al., 2015; Zhang et al., 2019). The variation in Gaiellales abundance across samples suggests potential associations with specific soil characteristics or environmental conditions that favor their proliferation. These findings underscore not only the complexity of the microbial ecosystem, but also the specialized roles that distinct bacterial orders may play in soil health and functionality. At lower phylogenetic levels, it is noteworthy that the populations within the samples are predominantly dominated by taxa represented by less than 1% of the sequences. This observation emphasizes the prevalence of highly diverse and less abundant microbial taxa, further highlighting the nuanced composition and dynamics within the soil microbiomes under study.
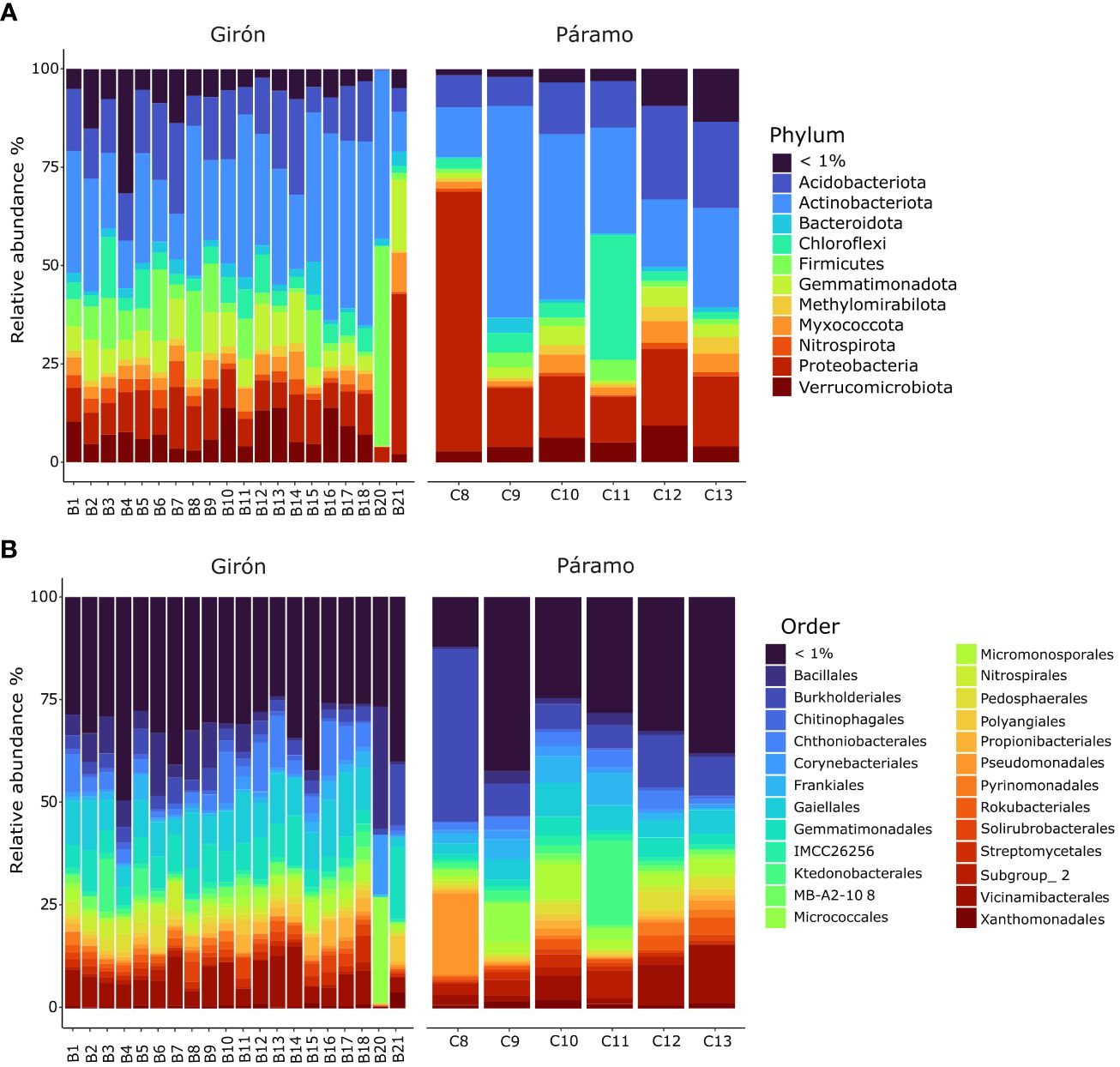
Figure 1 Barplots representing the bacterial taxonomy based on 16S rRNA gene. Data are shown at the (A) phylum and (B) order level for soils samples from organic (Girón municipality; B1-B21) and non-organic (Páramo municipality, C8-C13) citrus fruits plantations. B1 to B9 belong to Plot 1, B10 to B13 belong to Plot 2, B14 to B17 to Plot 3, Sample B18 belongs to Plot 0 (non-cultivated), B20 and B21 are chicken manure compost and worm castings, respectively. Conversely, within the non-organic citrus plantation, Samples C8 to C13 are designated to Plot 4.
The microbial communities in the fertilizer based on chicken manure were mostly dominated by Gram positive bacteria of the phyla Firmicutes (class Bacilli) and Actinobacteria (class Actinobacteria) (Figure 1). Even though fresh chicken manure is rich in diverse gut microbes, specially Proteobacteria, these usually do not reach the soil, since microbiomes of composted chicken manure are usually dominated by Gram-positive bacteria (Maeda et al., 2011; Zhang et al., 2018; Haq et al., 2021). Such dominance is explained by the ability of Gram-positives to withstand the relatively high temperatures reached during the composting process (Partanen et al., 2010; Aguilar-Paredes et al., 2023). In contrast, the worm castings were mostly populated by Gram negatives of the phyla Proteobacteria (Beta-, Delta- and Gammaproteobacteria) and Gemmatimonadetes (C_Gemm-1). Vermicomposting occurs at room temperature and under aerobic conditions, eliminating the temperature-based selection of microbes (Huang et al., 2014; Cai et al., 2018).
Culture-based methods were focused on isolating k-strategist bacteria capable of cellulase production, heavy metal resistance and LDPE degradation. Twenty-five isolates from 8 bacterial genera were identified through full 16S rRNA gene sequencing (Table 1). The strains showcased a range of morphological colony traits, and functional activities such as the degradation of natural (CMC) and artificial (LDPE) polymers, and tolerance to heavy metals. These isolates, with their promising traits, are prime candidates for further research and potential applications in bioremediation, bioprospecting, and sustainable agricultural practices (Kyaw et al., 2012; Ahmed et al., 2018; Alvarado-Campo et al., 2023; Nademo et al., 2023).
Isolate CP102 emerged as a particularly interesting candidate due to its pronounced capability for degrading carboxymethyl cellulose (CMC) on agar plates. Genomic sequencing techniques were employed for the production of a closed bacterial genome and the identification of an accompanying plasmid (Figure 2). Comparative genomic analysis showed that the closest relative of CP102 is Enterobacter soli ATCC BAA-2102 as determined by 16S rRNA gene comparison (identity: 99.54%), genome average nucleotide identity (95.73%, Figure 3) and dDDH (d6: 82.2%). These results suggest that isolate CP102 is most likely affiliated to the species Enterobacter soli. CP102’s genome, as detailed in Supplementary Table 2, consists of a single circular contig with a total length of 4,781,449 bp. The genome harbors 4,389 coding DNA sequences (CDS) and has a GC content of 53.9%. The chromosomal DNA includes 22 rRNA genes, 84 tRNA genes, and a single tmRNA, indicating a robust capacity for protein synthesis.
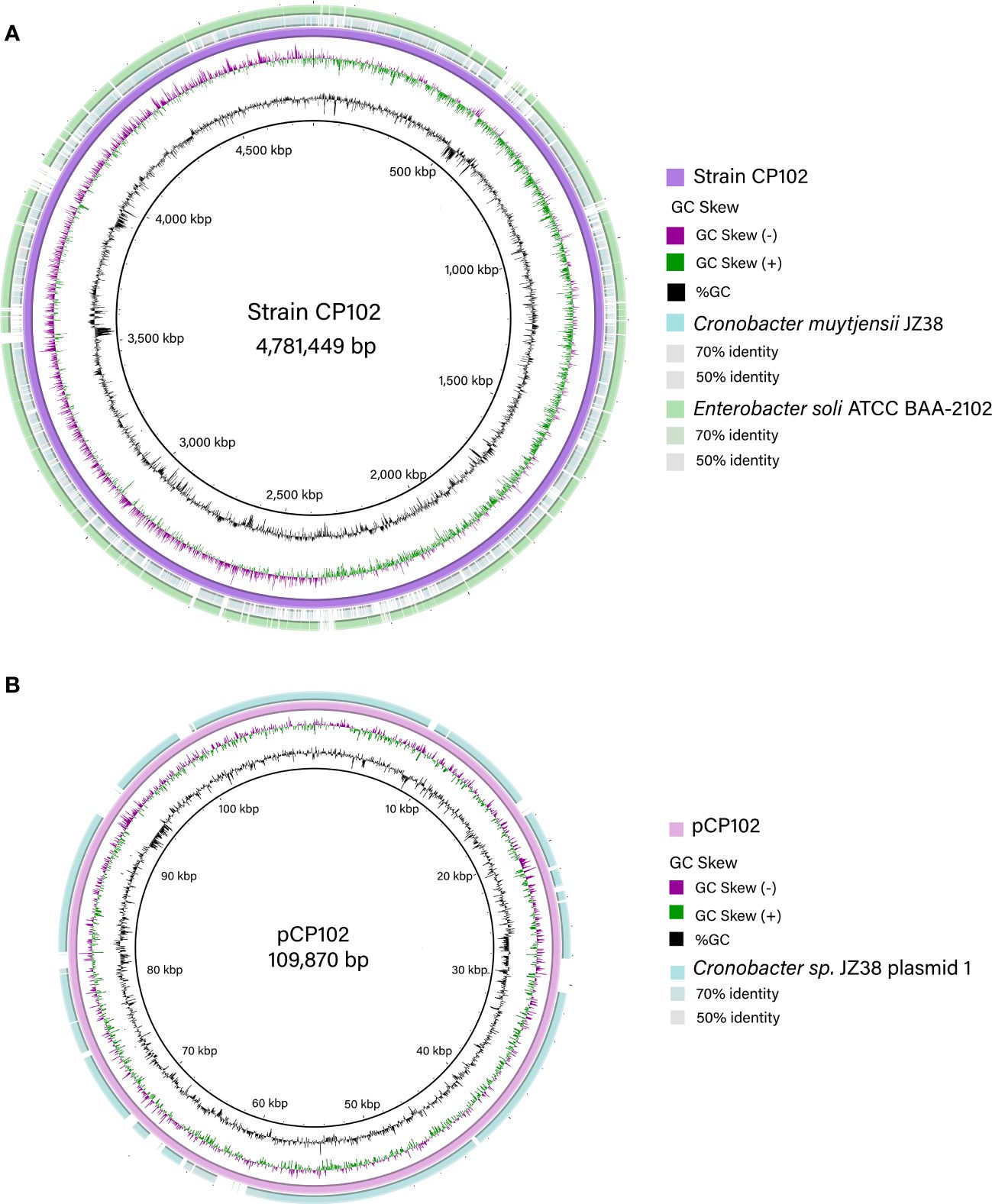
Figure 2 Maps of the genetic elements found isolate CP102. (A) The bacterial chromosome (purple ring) is 4.78 Mb in size. In the two outer concentric rings, the chromosomes of two closely related species are aligned. Their nucleotide identities to CP102 are depicted in color gradients. (B) A 109 kb plasmid was assembled and aligned in the outer most ring to plasmid p1 of Cronobacter muytjensii JZ38. For both the chromosome and plasmid, the middle circle indicates de GC skew [(G-C)/(G+C)] positive (green) and negative (purple). The inner circle (black) indicates the % GC content.
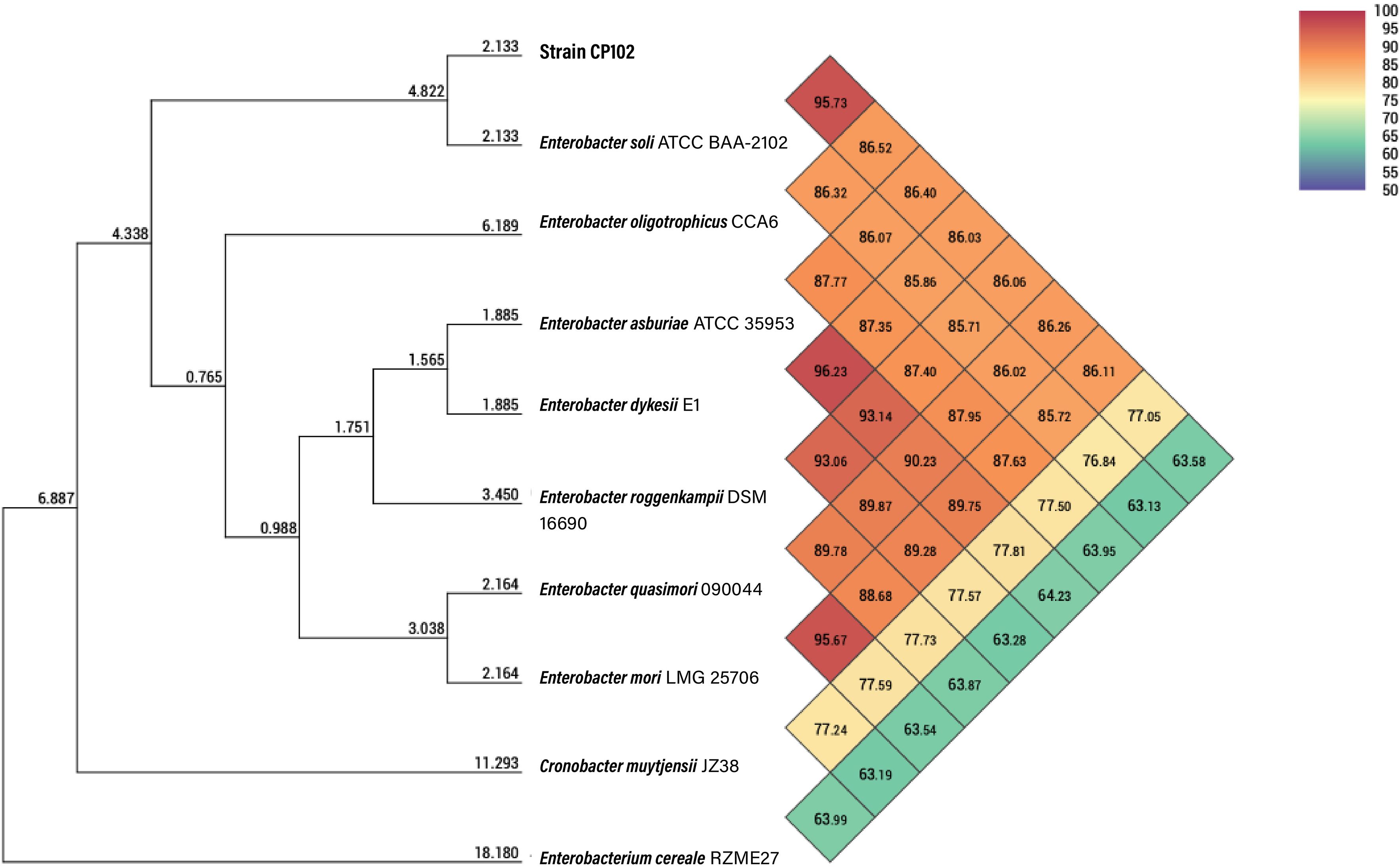
Figure 3 Heatmap of the Average Nucleotide Identity between strain CP102 and closely-related type strains. Similarity percentages in a color scale among the genomes are shown. The values on the branches indicate the intergenomic genetic distance calculated in the Orthologous Average Nucleotide Identity Tool (OAT) software.
We conducted a search for genes associated with cellulolytic activity within the genome of CP102 and its close relatives, using BLASTp. En. Soli CP102 encoded four proteins that are potentially involved in the cellulolytic activity: Candidate protein KOMHPLAP_04250, is similar to a terephthalic acid (TPA) cellulase from Enterobacter soli (Acc. Number HDX4050896.1). The candidate TPA cellulase KOMHPLAP_042 may catalyze the hydrolysis of cellulose into its constituent glucose units, positing a significant role in the primary degradation of cellulose, which could be a cornerstone in the CP102 strain’s cellulolytic arsenal. Similarly, KOMHPLAP_04258, is a ‘TPA: endoglucanase’, linked to Enterobacter soli (HDX4050904.1). This enzyme appears to be akin to endoglucanases that act on the internal β-1,4-glucosidic bonds within cellulose. This catalytic activity is critical, as it disrupts the orderly structure of cellulose, rendering it more accessible for subsequent enzymatic action, and suggests a synergistic participation of KOMHPLAP_04258 in cellulose breakdown. The third protein, KOMHPLAP_01362, bears the annotation ‘TPA: glycoside hydrolase family 1 protein’ and is aligned with a protein from Enterobacter asburiae (HDR2889362.1). As a member of the glycoside hydrolase family, this protein is anticipated to possess a broad range of activity on glycosidic bonds, which could include cellulolytic functions, depending on the specific substrates the enzyme interacts with. This implies a potential versatility in the carbohydrate-active enzymes of CP102, warranting further biochemical validation. Lastly, KOMHPLAP_01787 is close homologous of a ‘6-phospho-beta-glucosidase’, derived from Enterobacter species (WP_014069886.1.). This enzyme has a higher specificity compared to the wider-range cellulolytic enzymes, which could provide a specialized niche, potentially linked to a unique phosphorolytic cleavage mechanism.
Strain CP102 harbors a plasmid, pCP102, with a length of 109,870 bp and 141 CDS (Figure 2B). This is the first report of a plasmid in En. soli isolates. Interestingly, in a BLAST search, 81% of the genes encoded by pCP102 aligned to plasmid p1 (109 kbp) from Cronobacter muytjensii JZ38 showing 89.84% nucleotide identity over the aligned sequence. Cr. muytjensii JZ38 is described as an endophytic bacteria that promotes plant growth in stressing environments (Eida et al., 2020). En. soli CP102 and Cr. muytjensii JZ38 showed 96.49% 16S rRNA sequence identity and a mere 77% ANI value. Although originally all members of the genus Cronobacter were collapsed into a single species within Enterobacter sakazakii, a reclassification generated the formulation of a new genus with seven described species thus far (Eida et al., 2020). The roles of p1 and pCP102 in the metabolism or survival of their hosts are still to be determined.
4 Discussion
The pH levels in our study plots, ranging from 6.2 to 6.92, predominantly fall within the slightly acidic spectrum, a range known to be generally favorable for citrus cultivation (Long et al., 2017). Conversely, lower pH levels could trigger H+toxicity, impairing nutrient uptake and water absorption, thereby stunting plant growth. We noted a considerable heterogeneity in soil electrical conductivity (EC) across the plots, which indicates a non-homogeneous distribution of ions and nutrients in the soil influencing soil bacterial communities (Kim et al., 2016). Soil electrical conductivity (EC) is an indicator of nutrient availability, reflecting the concentration of dissolved ions in the soil water. Higher EC values typically suggest greater soil fertility, affecting microbial metabolism and growth (Darmawan et al., 2023; Kim and Park, 2024). Thus, EC is a useful proxy for assessing soil nutrient levels, which are critical for understanding soil fertility, ecosystem functioning, and microbial-mediated nutrient cycling. These communities are integral to nutrient cycling processes, thus directly impacting plant nutrient availability. Expanding on the intricacies of soil health, the observed variation in soil humidity within our plots corresponds with the research of García-Orenes et al (García-Orenes et al., 2010). Their work emphasized the significant influence of soil moisture levels on the composition of soil microbial communities and the abundance of nitrogen-cycling microbes. These factors are crucial for maintaining soil health and facilitating nutrient cycling. Finally, we observed fluctuations in the soil organic carbon content, a key factor in soil fertility and microbial diversity, known to influence the diversity and dynamics of both bacterial and fungal communities (Liu et al., 2017; Morugán-Coronado et al., 2019; Wu et al., 2021). Collectively, these findings underscore the intricate interplay of soil physicochemical properties in shaping the soil microbiome and, consequently, the health and productivity of citrus crops in this region.
In assessing the microbial diversity associated with citrus crops in the samples from the eastern Andes region of Santander, Colombia, and comparing them with global studies, a deeper analysis into the types of orders and phyla present becomes essential (Trivedi et al., 2012). Globally, the predominant taxa in citrus rhizospheres globally include Proteobacteria, Actinobacteria, Acidobacteria, and Bacteroidetes, as identified in the comprehensive analysis by (Xu et al., 2018). These groups are crucial for plant-microbe interactions, nutrient acquisition, and plant growth promotion, with core taxa like Pseudomonas, Agrobacterium, and Burkholderia playing significant roles in these processes (Bahram et al., 2018; Xu et al., 2018). In Santander, Colombia, we found similar microbial taxa to those reported in other citrus-related geographic locations due to the shared ecological characteristics. Nevertheless, local environmental conditions, such as soil composition, climate, and agricultural practices result in variations in the relative abundance and specific community structure. Our results enhance our understanding of the microbial diversity in the A horizon of citrus soils, which plays a crucial role in nutrient cycling and root health. This provides a solid foundation for further studies into plant-soil-microbe interactions and their impact on citrus crop productivity. By comparing these findings with global datasets, we can begin to understand the unique aspects of the Colombian citrus microbiome. This could lead to region-specific strategies for enhancing citrus production and health, leveraging the unique microbial properties of the local soils.
We employed sequencing and culturing techniques to explore the microbial diversity associated with citrus crops. These enabled the isolation and identification of bacteria from various culture media and substrates, leading to the identification of genera such as Bacillus and Pseudomonas. The occurrence of Bacillus species suggests its potential role in supporting the growth and health of citrus crops, echoing the global understanding of its beneficial properties in agriculture (Xu et al., 2018). Moreover, the detection of Pseudomonas species is noteworthy, considering their well-documented role in plant-associated activities, such as plant growth promotion abilities, degrading phenol, stimulating germination and seedling growth, biocontrol of pathogens by producing secondary metabolites, and for their potential to relieve plants from environmental stresses (Mercado-Blanco, 2015; Rajkumar et al., 2017; Biessy and Filion, 2021; Gao et al., 2023; Zboralski and Filion, 2023).
The genus Enterobacter has been increasingly recognized for its impact on host physiology and health. In a study highlighted by (Penyalver et al., 2022), the potential of surveying the bacterial microbiome to select host-beneficial microbes was emphasized. This approach is crucial in the modern citrus industry to overcome phytopathological threats and improve yield and quality. The presence of Enterobacter soli aligns with these findings, indicating its potential as a beneficial resource for citrus crops. This genus, known for its mutualistic interactions, could be a critical player in enhancing citrus health and productivity (Yaish, 2016; Lladó et al., 2017; Ludueña et al., 2019; Penyalver et al., 2022). This comparative analysis with global datasets on citrus rhizosphere microbiomes underscores the importance of understanding local microbial communities. In this sense, the genomic analysis of Enterobacter soli CP102 harboring an unreported plasmid for the genus, represents a significant insight in understanding the microbial dynamics of citrus soils in this region, highlighting the untapped diversity and potential within these ecosystems. Key to its role in the citrus soil microbiome is its robust cellulolytic capacity, as evidenced by the presence of enzymes like cellulases and endoglucanases, showing the biotechnological potential of the associated microorganisms.
In conclusion, this study offers a first step in a comprehensive view of the soil health and microbial diversity essential for citrus cultivation in this region. The physicochemical properties of the soil provide a conducive environment for citrus growth, while the diverse microbial community, exemplified by beneficial bacteria like Bacillus, Pseudomonas, and Enterobacter, plays a vital role in maintaining soil health and supporting crop productivity. The novel insights gained from Enterobacter soli CP102 underscore the significant biotechnological potential of these microorganisms, paving the way for future agricultural innovations. Collectively, these findings contribute to the understanding of the intricate relationships between soil properties, microbial communities, and citrus crop health, emphasizing the importance of tailored agricultural practices to leverage these interactions for enhanced productivity in the Santander region.
Data availability statement
The datasets presented in this study can be found in online repositories. The names of the repository/repositories and accession number(s) can be found below: https://www.ncbi.nlm.nih.gov/, PRJNA870475.
Author contributions
GAC-V: Conceptualization, Formal analysis, Funding acquisition, Methodology, Project administration, Resources, Supervision, Writing – original draft, Writing – review & editing. VT-P: Conceptualization, Data curation, Formal analysis, Investigation, Methodology, Visualization, Writing – original draft. JM-P: Conceptualization, Data curation, Formal analysis, Investigation, Methodology, Visualization, Writing – original draft. PS-G: Investigation, Methodology, Writing – original draft. LS: Data curation, Formal analysis, Writing – review & editing. JH-T: Formal analysis, Writing – review & editing. EP-C: Conceptualization, Formal analysis, Funding acquisition, Methodology, Project administration, Resources, Supervision, Writing – original draft, Writing – review & editing.
Funding
The authors declare financial support was received for the research, authorship, and/or publication of this article. This research was funded by the Sistema General de Regalı́as from the Colombian Ministry of Science (Grant no. BPIN2020000100373). LS was funded by the UK’s Biotechnology and Biological Sciences Research Council (BBSRC) under the Institute Strategic Grant: Food Microbiome and Health BB/X011054/1.
Conflict of interest
The authors declare that the research was conducted in the absence of any commercial or financial relationships that could be construed as a potential conflict of interest.
Publisher’s note
All claims expressed in this article are solely those of the authors and do not necessarily represent those of their affiliated organizations, or those of the publisher, the editors and the reviewers. Any product that may be evaluated in this article, or claim that may be made by its manufacturer, is not guaranteed or endorsed by the publisher.
Supplementary material
The Supplementary Material for this article can be found online at: https://www.frontiersin.org/articles/10.3389/fevo.2024.1372284/full#supplementary-material
References
Aguilar-Paredes A., Valdés G., Araneda N., Valdebenito E., Hansen F., Nuti M. (2023). Microbial community in the composting process and its positive impact on the soil biota in sustainable agriculture. Agronomy 13. doi: 10.3390/agronomy13020542
Ahmed S., Rahman M. S., Hasan M. M., Paul N., Sajib A. A. (2018). Microbial degradation of lignocellulosic biomass: Discovery of novel natural lignocellulolytic bacteria. Biotechnologia 99, 137–146. doi: 10.5114/bta.2018.75657
Alikhan N.-F., Petty N. K., Ben Zakour N. L., Beatson S. A. (2011). BLAST Ring Image Generator (BRIG): simple prokaryote genome comparisons. BMC Genomics 12, 402. doi: 10.1186/1471-2164-12-402
Alvarado-Campo K. L., Quintero M., Cuadrado-Cano B., Montoya-Giraldo M., Otero-Tejada E. L., Blandón L., et al. (2023). Heavy metal tolerance of microorganisms isolated from coastal marine sediments and their lead removal potential. Microorganisms 11. doi: 10.3390/microorganisms11112708
Amir A., Daniel M., Navas-Molina J., Kopylova E., Morton J., Xu Z. Z., et al. (2017). Deblur rapidly resolves single-. Am. Soc. Microbiol. 2, 1–7. doi: 10.1128/mSystems.00191-16
Bahram M., Hildebrand F., Forslund S. K., Anderson J. L., Soudzilovskaia N. A., Bodegom P. M., et al. (2018). Structure and function of the global topsoil microbiome. Nature 560, 233–237. doi: 10.1038/s41586-018-0386-6
Banerjee S., Schlaeppi K., van der Heijden M. G. A. (2018). Keystone taxa as drivers of microbiome structure and functioning. Nat. Rev. Microbiol. 16, 567–576. doi: 10.1038/s41579-018-0024-1
Berendsen R. L., Pieterse C. M. J., Bakker P. A. H. M. (2012). The rhizosphere microbiome and plant health. Trends Plant Sci. 17, 478–486. doi: 10.1016/j.tplants.2012.04.001
Berg G. (2009). Plant–microbe interactions promoting plant growth and health: perspectives for controlled use of microorganisms in agriculture. Appl. Microbiol. Biotechnol. 84, 11–18. doi: 10.1007/s00253-009-2092-7
Biessy A., Filion M. (2021). Phloroglucinol derivatives in plant-beneficial Pseudomonas spp.: Biosynthesis, regulation, and functions. Metabolites 11. doi: 10.3390/metabo11030182
Cai L., Gong X., Sun X., Li S., Yu X. (2018). Comparison of chemical and microbiological changes during the aerobic composting and vermicomposting of green waste. PloS One 13, e0207494. doi: 10.1371/journal.pone.0207494
Chaparro J. M., Sheflin A. M., Manter D. K., Vivanco J. M. (2012). Manipulating the soil microbiome to increase soil health and plant fertility. Biol. Fertil Soils 48, 489–499. doi: 10.1007/s00374-012-0691-4
Chaudhary D. K., Khulan A., Kim J. (2019). Development of a novel cultivation technique for uncultured soil bacteria. Sci. Rep. 9, 1–11. doi: 10.1038/s41598-019-43182-x
Chen W., Yu T., Zhao C., Li B., Qin Y., Li H., et al. (2023). Development and determinants of topsoil bacterial and fungal communities of afforestation by aerial sowing in Tengger Desert, China. J. Fungi 9. doi: 10.3390/jof9040399
Cherni M., Ferjani R., Mapelli F., Boudabous A., Borin S., Ouzari H. I. (2019). Soil parameters drive the diversity of Citrus sinensis rhizosphere microbiota which exhibits a potential in plant drought stress alleviation. Appl. Soil Ecol. 135, 182–193. doi: 10.1016/j.apsoil.2018.12.006
Dantur K. I., Enrique R., Welin B., Castagnaro A. P. (2015). Isolation of cellulolytic bacteria from the intestine of Diatraea saccharalis larvae and evaluation of their capacity to degrade sugarcane biomass. AMB Express 5. doi: 10.1186/s13568-015-0101-z
Darmawan D., Perdana D., Ismardi A., Fathona I. W. (2023). Investigating the electrical properties of soil as an indicator of the content of the NPK element in the soil. Measurement Control 56, 351–357. doi: 10.1177/00202940221122177
Deng J., Yin Y., Zhu W., Zhou Y. (2018). Variations in soil bacterial community diversity and structures among different revegetation types in the Baishilazi nature reserve. Front. Microbiol. 9. doi: 10.3389/fmicb.2018.02874
Donkersley P., Silva F. W. S., Carvalho C. M., Al-Sadi A. M., Elliot S. L. (2018). Biological, environmental and socioeconomic threats to citrus lime production. J. Plant Dis. Prot. 125, 339–356. doi: 10.1007/s41348-018-0160-x
Eida A. A., Bougouffa S., L’Haridon F., Alam I., Weisskopf L., Bajic V. B., et al. (2020). Genome Insights of the Plant-Growth Promoting Bacterium Cronobacter muytjensii JZ38 With Volatile-Mediated Antagonistic Activity Against Phytophthora infestans. Front. Microbiol. 11. doi: 10.3389/fmicb.2020.00369
Gao H., Feng G.-D., Feng Z., Yao Q., Li J., Deng X., et al. (2023). Pseudomonas citri sp. nov., a potential novel plant growth promoting bacterium isolated from rhizosphere soil of citrus. Antonie Van Leeuwenhoek 116, 281–289. doi: 10.1007/s10482-022-01803-y
García-Orenes F., Guerrero C., Roldán A., Mataix-Solera J., Cerdà A., Campoy M., et al. (2010). Soil microbial biomass and activity under diferent agricultural management systems in a semiarid Mediterranean agroecosystem. Soil Tillage Res. 109, 110–115. doi: 10.1016/j.still.2010.05.005
Gurevich A., Saveliev V., Vyahhi N., Tesler G. (2013). QUAST: quality assessment tool for genome assemblies. Bioinformatics 29, 1072–1075. doi: 10.1093/bioinformatics/btt086
Haq H. U., Li Y., Jin L., Zhang T., Cheng L., Li Z., et al. (2021). Effect of chicken manure-based fertiliser on bacterial communities and diversity of tomato endosphere microbiota. Agric. (Pol’nohospodárstvo) 67, 144–154. doi: 10.2478/agri-2021-0013
Huang K., Li F., Wei Y., Fu X., Chen X. (2014). Effects of earthworms on physicochemical properties and microbial profiles during vermicomposting of fresh fruit and vegetable wastes. Bioresour Technol. 170, 45–52. doi: 10.1016/j.biortech.2014.07.058
Kalam S., Basu A., Ahmad I., Sayyed R. Z., El-Enshasy H. A., Dailin D. J., et al. (2020). Recent understanding of soil acidobacteria and their ecological significance: A critical review. Front. Microbiol. 11. doi: 10.3389/fmicb.2020.580024
Kim H. N., Park J. H. (2024). Monitoring of soil EC for the prediction of soil nutrient regime under different soil water and organic matter contents. Appl. Biol. Chem. 67. doi: 10.1186/s13765-023-00849-4
Kim J. M., Roh A.-S., Choi S.-C., Kim E.-J., Choi M.-T., Ahn B.-K., et al. (2016). Soil pH and electrical conductivity are key edaphic factors shaping bacterial communities of greenhouse soils in Korea. J. Microbiol. 54, 838–845. doi: 10.1007/s12275-016-6526-5
Kopecky J., Kyselkova M., Omelka M., Cermak L., Novotna J., Grundmann G. L., et al. (2011). Actinobacterial community dominated by a distinct clade in acidic soil of a waterlogged deciduous forest. FEMS Microbiol. Ecol. 78, 386–394. doi: 10.1111/j.1574-6941.2011.01173.x
Kruczyńska A., Kuźniar A., Podlewski J., Słomczewski A., Grządziel J., Marzec-Grządziel A., et al. (2023). Bacteroidota structure in the face of varying agricultural practices as an important indicator of soil quality – a culture independent approach. Agric. Ecosyst. Environ. 342. doi: 10.1016/j.agee.2022.108252
Kyaw B. M., Champakalakshmi R., Sakharkar M. K., Lim C. S., Sakharkar K. R. (2012). Biodegradation of low density polythene (LDPE) by pseudomonas species. Indian J. Microbiol. 52, 411–419. doi: 10.1007/s12088-012-0250-6
Larsbrink J., McKee L. S. (2020). “Bacteroidetes bacteria in the soil: Glycan acquisition, enzyme secretion, and gliding motility,” in Advances in Applied Microbiology (Wilmington, United States: Academic Press Inc), 63–98. doi: 10.1016/bs.aambs.2019.11.001
Lee I., Ouk Kim Y., Park S.-C., Chun J. (2016). OrthoANI: An improved algorithm and software for calculating average nucleotide identity. Int. J. Syst. Evol. Microbiol. 66, 1100–1103. doi: 10.1099/ijsem.0.000760
Lewin G. R., Carlos C., Chevrette M. G., Horn H. A., McDonald B. R., Stankey R. J., et al. (2016). Evolution and ecology of actinobacteria and their bioenergy applications. Annu. Rev. Microbiol. 70, 235–254. doi: 10.1146/annurev-micro-102215-095748
Liu Y., Heying E., Tanumihardjo S. A. (2012). History, global distribution, and nutritional importance of citrus fruits. Compr. Rev. Food Sci. Food Saf. 11, 530–545. doi: 10.1111/j.1541-4337.2012.00201.x
Liu Z., Rong Q., Zhou W., Liang G. (2017). Effects of inorganic and organic amendment on soil chemical properties, enzyme activities, microbial community and soil quality in yellow clayey soil. PloS One 12, e0172767. doi: 10.1371/journal.pone.0172767
Lladó S., López-Mondéjar R., Baldrian P. (2017). Forest soil bacteria: diversity, involvement in ecosystem processes, and response to global change. Microbiol. Mol. Biol. Rev. 81. doi: 10.1128/MMBR.00063-16
Long A., Zhang J., Yang L. T., Ye X., Lai N. W., Tan L. L., et al. (2017). Effects of low pH on photosynthesis, related physiological parameters, and nutrient profiles of citrus. Front. Plant Sci. 8. doi: 10.3389/fpls.2017.00185
Ludueña L. M., Anzuay M. S., Angelini J. G., McIntosh M., Becker A., Rupp O., et al. (2019). Genome sequence of the endophytic strain Enterobacter sp. J49, a potential biofertilizer for peanut and maize. Genomics 111, 913–920. doi: 10.1016/j.ygeno.2018.05.021
Maeda K., Hanajima D., Toyoda S., Yoshida N., Morioka R., Osada T. (2011). Microbiology of nitrogen cycle in animal manure compost. Microb. Biotechnol. 4, 700–709. doi: 10.1111/j.1751-7915.2010.00236.x
Martín-Platero A. M., Valdivia E., Maqueda M., Martínez-Bueno M. (2007). Fast, convenient, and economical method for isolating genomic DNA from lactic acid bacteria using a modification of the protein “salting-out” procedure. Anal. Biochem. 366, 102–104. doi: 10.1016/j.ab.2007.03.010
McMurdie P. J., Holmes S. (2013). phyloseq: an R package for reproducible interactive analysis and graphics of microbiome census data. PloS One 8, e61217. doi: 10.1371/journal.pone.0061217
Meier-Kolthoff J. P., Auch A. F., Klenk H.-P., Göker M. (2013). Genome sequence-based species delimitation with confidence intervals and improved distance functions. BMC Bioinf. 14, 60. doi: 10.1186/1471-2105-14-60
Meier-Kolthoff J. P., Carbasse J. S., Peinado-Olarte R. L., Göker M. (2022). TYGS and LPSN: a database tandem for fast and reliable genome-based classification and nomenclature of prokaryotes. Nucleic Acids Res. 50, D801–D807. doi: 10.1093/nar/gkab902
Meier-Kolthoff J. P., Göker M. (2019). TYGS is an automated high-throughput platform for state-of-the-art genome-based taxonomy. Nat. Commun. 10, 2182. doi: 10.1038/s41467-019-10210-3
Mercado-Blanco J. (2015). “Pseudomonas strains that exert biocontrol of plant pathogens,” in Pseudomonas: Volume 7: New Aspects of Pseudomonas Biology (New York, NY: Springer Netherlands), 121–172. doi: 10.1007/978-94-017-9555-5_6
Mhete M., Eze P. N., Rahube T. O., Akinyemi F. O. (2020). Soil properties influence bacterial abundance and diversity under different land-use regimes in semi-arid environments. Sci. Afr 7. doi: 10.1016/j.sciaf.2019.e00246
Morugán-Coronado A., García-Orenes F., McMillan M., Pereg L. (2019). The effect of moisture on soil microbial properties and nitrogen cyclers in Mediterranean sweet orange orchards under organic and inorganic fertilization. Sci. Total Environ. 655, 158–167. doi: 10.1016/j.scitotenv.2018.11.174
Nademo Z. M., Shibeshi N. T., Gemeda M. T. (2023). Isolation and screening of low-density polyethylene (LDPE) bags degrading bacteria from Addis Ababa municipal solid waste disposal site “Koshe”. Ann. Microbiol. 73. doi: 10.1186/s13213-023-01711-0
Nihorimbere V., Ongena M., Smargiassi M., Thonart P. (2011). Beneficial effect of the rhizosphere microbial community for plant growth and health. Biotechnologie Agronomie Société Environnement 15, 327.
Ondov B. D., Treangen T. J., Melsted P., Mallonee A. B., Bergman N. H., Koren S., et al. (2016). Mash: Fast genome and metagenome distance estimation using MinHash. Genome Biol. 17, 1–14. doi: 10.1186/s13059-016-0997-x
Partanen P., Hultman J., Paulin L., Auvinen P., Romantschuk M. (2010). Bacterial diversity at different stages of the composting process. BMC Microbiol. 10, 94. doi: 10.1186/1471-2180-10-94
Penyalver R., Roesch L. F. W., Piquer-Salcedo J. E., Forner-Giner M. A., Alguacil M., del M. (2022). From the bacterial citrus microbiome to the selection of potentially host-beneficial microbes. N Biotechnol. 70, 116–128. doi: 10.1016/j.nbt.2022.06.002
Puentes-Cala E., Atehortúa-Bueno M., Tapia-Perdomo V., Navarro-Escalante L., Hernández-Torres J., Castillo-Villamizar G. (2023). First insights into the gut microbiome of Diatraea saccharalis: From a sugarcane pest to a reservoir of new bacteria with biotechnological potential. Front. Ecol. Evol. 11. doi: 10.3389/fevo.2023.1027527
Quast C., Pruesse E., Yilmaz P., Gerken J., Schweer T., Yarza P., et al. (2013). The SILVA ribosomal RNA gene database project: improved data processing and web-based tools. Nucleic Acids Res. 41, D590–D596. doi: 10.1093/nar/gks1219
Rajkumar M., Bruno L. B., Banu J. R. (2017). Alleviation of environmental stress in plants: The role of beneficial Pseudomonas spp. Crit. Rev. Environ. Sci. Technol. 47, 372–407. doi: 10.1080/10643389.2017.1318619
Reasoner D. J., Geldreich E. E. (1985). A new medium for the enumeration and subculture of bacteria from potable water. Appl. Environ. Microbiol. 49, 1–7. doi: 10.1128/aem.49.1.1-7.1985
Seemann T. (2014). Prokka: rapid prokaryotic genome annotation. Bioinformatics 30, 2068–2069. doi: 10.1093/bioinformatics/btu153
Srivastava A. K., Das A. K., Jagannadham P. T. K., Bora P., Ansari F. A., Bhate R. (2022). Bioprospecting microbiome for soil and plant health management amidst huanglongbing threat in citrus: A review. Front. Plant Sci. 13. doi: 10.3389/fpls.2022.858842
Su J., Wang Y., Bai M., Peng T., Li H., Xu H. J., et al. (2023). Soil conditions and the plant microbiome boost the accumulation of monoterpenes in the fruit of Citrus reticulata ‘Chachi.’. Microbiome 11. doi: 10.1186/s40168-023-01504-2
R Core Team. (2023). A language and environment for statistical computing. R Found. Stat. Comput. Vienna, Austria. https://www.R-project.org.
Teather R. M., Wood P. J. (1982). Use of Congo red-polysaccharide interactions in enumeration and characterization of cellulolytic bacteria from the bovine rumen. Appl. Environ. Microbiol. 43, 777–780. doi: 10.1128/aem.43.4.777-780.1982
The Galaxy Community (2022). The Galaxy platform for accessible, reproducible and collaborative biomedical analyses: 2022 update. Nucleic Acids Res. 50, W345–W351. doi: 10.1093/nar/gkac247
Timofeeva A. M., Galyamova M. R., Sedykh S. E. (2023). Plant growth-promoting soil bacteria: nitrogen fixation, phosphate solubilization, siderophore production, and other biological activities. Plants 12. doi: 10.3390/plants12244074
Tong H., Hu M., Li F., Chen M., Lv Y. (2015). Burkholderiales participating in pentachlorophenol biodegradation in iron-reducing paddy soil as identified by stable isotope probing. Environ. Sci. Process Impacts 17, 1282–1289. doi: 10.1039/C4EM00530A
Trivedi P., He Z., Van Nostrand J. D., Albrigo G., Zhou J., Wang N. (2012). Huanglongbing alters the structure and functional diversity of microbial communities associated with citrus rhizosphere. ISME J. 6, 363–383. doi: 10.1038/ismej.2011.100
Wei H., Peng C., Yang B., Song H., Li Q., Jiang L., et al. (2018). Contrasting soil bacterial community, diversity, and function in two forests in China. Front. Microbiol. 9. doi: 10.3389/fmicb.2018.01693
Wick R. R., Judd L. M., Gorrie C. L., Holt K. E. (2017). Unicycler: Resolving bacterial genome assemblies from short and long sequencing reads. PloS Comput. Biol. 13, e1005595. doi: 10.1371/journal.pcbi.1005595
Wu Y., Wang X., Hu R., Zhao J., Jiang Y. (2021). Responses of soil microbial traits to ground cover in citrus orchards in central China. Microorganisms 9. doi: 10.3390/microorganisms9122507
Xu J., Zhang Y., Zhang P., Trivedi P., Riera N., Wang Y., et al. (2018). The structure and function of the global citrus rhizosphere microbiome. Nat. Commun. 9, 4894. doi: 10.1038/s41467-018-07343-2
Yaish M. W. (2016). Draft genome sequence of endophytic bacterium Enterobacter asburiae PDA134, isolated from date palm (Phoenix dactylifera L.) roots. Genome Announc 4. doi: 10.1128/genomeA.00848-16
Zboralski A., Filion M. (2023). Pseudomonas spp. can help plants face climate change. Front. Microbiol. 14. doi: 10.3389/fmicb.2023.1198131
Zhang L., Li L., Pan X., Shi Z., Feng X., Gong B., et al. (2018). Enhanced growth and activities of the dominant functional microbiota of chicken manure composts in the presence of maize straw. Front. Microbiol. 9. doi: 10.3389/fmicb.2018.01131
Zhang C., Tayyab M., Abubakar A. Y., Yang Z., Pang Z., Islam W., et al. (2019). Bacteria with different assemblages in the soil profile drive the diverse nutrient cycles in the sugarcane straw retention ecosystem. Diversity (Basel) 11. doi: 10.3390/d11100194
Zhang Y., Trivedi P., Xu J., Roper M. C., Wang N. (2021). The citrus microbiome: from structure and function to microbiome engineering and beyond. Phytobiomes J. 5, 249–262. doi: 10.1094/PBIOMES-11-20-0084-RVW
Keywords: citrus cultivation, agricultural practices, soil-associated bacteria, biodiversity, microbiome, bioprospection, biocatalysts
Citation: Castillo-Villamizar GA, Tapia-Perdomo V, Maldonado-Pava J, Santamaría-Gálvis P, Sayavedra L, Hernandez-Torres J and Puentes-Cala E (2024) Unveiling soil bacterial ecosystems in andean citrus orchards of Santander, Colombia. Front. Ecol. Evol. 12:1372284. doi: 10.3389/fevo.2024.1372284
Received: 17 January 2024; Accepted: 06 May 2024;
Published: 21 May 2024.
Edited by:
Antonio Castellano-Hinojosa, Universidad de Granada, SpainReviewed by:
Emma Dawson, University of Florida, United StatesYu-Pei Chen, Xiamen Medical College, China
Copyright © 2024 Castillo-Villamizar, Tapia-Perdomo, Maldonado-Pava, Santamaría-Gálvis, Sayavedra, Hernandez-Torres and Puentes-Cala. This is an open-access article distributed under the terms of the Creative Commons Attribution License (CC BY). The use, distribution or reproduction in other forums is permitted, provided the original author(s) and the copyright owner(s) are credited and that the original publication in this journal is cited, in accordance with accepted academic practice. No use, distribution or reproduction is permitted which does not comply with these terms.
*Correspondence: Edinson Puentes-Cala, ZXB1ZW50ZXNAY29ycm9zaW9uY2ljLmNvbQ==