When and Why Did Human Brains Decrease in Size? A New Change-Point Analysis and Insights From Brain Evolution in Ants
A Commentary on:
Human brains have shrunk: the questions are when and why
DeSilva J, Fannin L, Cheney I, Claxton A, Ilieş I, Kittelberger J, Stibel J and Traniello J (2023). Front. Ecol. Evol. 11:1191274. doi: 10.3389/fevo.2023.1191274
1 Introduction
Brain size is a very important topic for paleoneurology and paleoanthropology, even if this topic has generated more controversies than answers, and there is little consensus on the actual dynamics of brain size evolution. A recent example of these controversial issues is the human brain reduction from the Late Pleistocene/Holocene to the modern days, supported by the analysis of a large compilation of fossil and recent human crania in DeSilva et al. (2021, 2023), but not confirmed by the analysis on a subset of the same data performed by Villmoare and Grabowski (2022), which have found that there is no reduction in brain size in modern humans since the origins of our species. Indeed, DeSilva et al. (2021) determined changepoints in the time evolution of human cranial capacity using the package changepoint (Killick and Eckley, 2014) and segmented regression models (Muggeo, 2008). They found three changepoints, two positive rate changes in hominin brain evolution and one very recent negative rate change. The first, 2.1 million years ago, coincided with the early evolution of the genus Homo. The second, 1.5 million years ago, related to technological innovations seen in the archaeological records. The last changepoint, a negative rate change, is regarding the recent Homo sapiens evolution at an age of 3±1 ka. Villmoare and Grabowski (2022) argued that the dataset of DeSilva et al. (2021) was inadequate, and the mean endocranial volume of H. sapiens for age ∼0.1 ka was not correct. Due to the scarcity of fossils that, for H. sapiens, spans a huge interval (300 ka), Villmoare and Grabowski (2022) argued that a dataset with too many data in the range age ∼0.1 ka (N=578) over a total of N=836 is time unbalanced towards recent ages, biasing the research of changepoints, leading to a false negative rate change in H. sapiens’ endocranial-volume evolution for the 3–5 ka age. DeSilva et al. (2023) replied to the criticisms of Villmoare and Grabowski (2022) changing partially the endocranial volume dataset and confirming the reduction in the last 3–5 ka age. This surprising recent brain reduction was linked by the authors to the ability to store information externally in social groups, which relaxed the strong forces of selection for maintaining large brains. In particular, according to DeSilva et al. (2021), also studies of ants’ social systems could aid in interpreting patterns of brain evolution identified in humans.
These controversial conclusions should be related to the fact that H. sapiens’ cranial capacity can differ by over 1,000 cc (Holloway et al., 2004). Indeed, these big differences of endocranial volume within our species complicate the study of cranial capacity’s evolution, starting from few available fossil samples. To handle data consistent with the analysis reported in DeSilva et al. (2023), we summarize the statistical results of some key studies about the endocranial volume of contemporary H. sapiens (age ∼1 ka). Beals et al. (1984) analyzed data on 5,288 crania from 122 different ethnic groups and reported an endocranial volume of 1,349 ± 78cc. Henneberg (1988) indicated 1,387cc for a sample N=245. The Dekaban and Sadowsky (1978) adult dataset (N=3,399) indicates an average value of 1,334.5 ± 205.9cc. A fundamental point to be considered is that the distribution of endocranial volumes of adult humans has a bimodal shape, with two most frequent values, one for male and the other for female. Rushton (1994) reported in Table 1 of his paper average cranial capacities for individuals of almost all geographical regions of the earth. He found a value of 1,401 ± 42cc for men and 1,186 ± 53cc for women. The difference of the two means is greater than two standard deviations, implying a distribution of endocranial volumes characterized by two separate maxima. By considering studies with an equal sex representation, average endocranial volumes vary between 1,335 ± 206cc (Dekaban and Sadowsky, 1978; N=3,399) and 1,344 ± 137cc (Ho et al., 1980; N=1,261). The average of these two datasets is 1,337 ± 187cc, very close to the value indicated by Beals et al. (1984). Thus, we can consider also this last dataset balanced in terms of sex representation, obtaining a final average, for N=3,399 + 1,261 + 5,288 = 9,948 adult individuals, equal to 1,343 ± 129cc. DeSilva et al. (2023) reported, for the more recent age of 0.1 ka, N=415 entries with an average value of 1,297 ± 152cc. Adding this last dataset to the above ones, a final dataset of N=10,363 is obtained, with an average endocranial volume of 1,341 ± 130cc, statistically well representative of the endocranial volume of contemporary humans (age ∼0.1 ka). Supplementary Figure S1 shows the data reported in DeSilva et al. (2023) excepted the values corresponding to 0.1 ka, substituted with the N=10,363 average value. Supplementary Figure S1 clearly shows that the endocranial volumes of H. sapiens, corresponding to other ages than 0.1 ka, fall mainly over the 0.1 ka value. A fossil of the past could have an endocranial volume value that differs from the 0.1 ka average value both due to the natural variability of the cranial capacity of humans and due to a time evolution of the cranial capacity. Supplementary Figure S2 shows the differences, with respect to the mean value obtained at age ∼0.1 ka (N=10,363), of all the fossils corresponding to the H. sapiens. Numerical values have been reported in Supplementary Table S1. The unequal sex representation of endocranial volumes for the few fossils available, belonging to a specific age, could become a source of bias when studying time evolution of the H. sapiens’ cranial capacity.
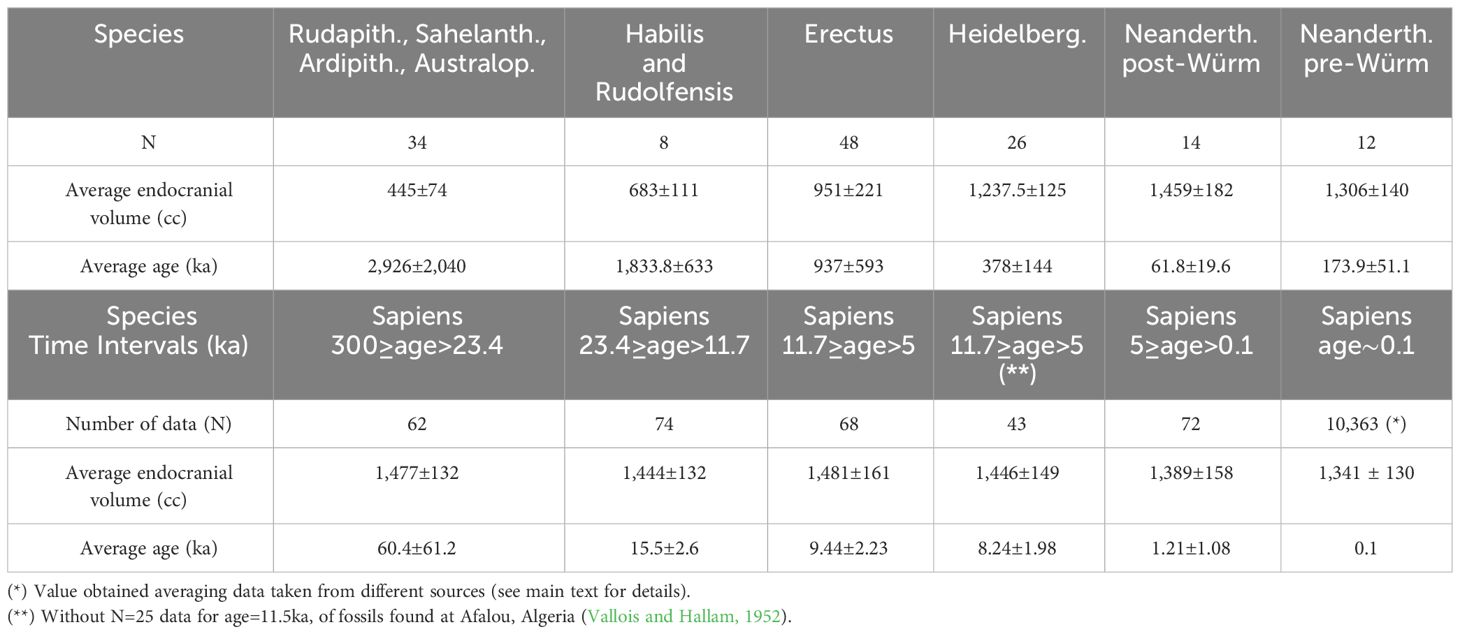
Table 1 Mean endocranial volumes and mean ages averaging data taken from (DeSilva et al., 2023).
2 The condition of equal sex representation of endocranial volumes
Distribution of endocranial volumes of adult humans has a bimodal shape, with two most frequent modes or values, one for male and the other for female (Rushton, 1994). An equal sex representation of cranial capacity of a dataset should lead to a histogram distribution characterized by two maxima (male and female modes) of nearly the same height. Supplementary Figure S3 shows a histogram of the N=26 cranial capacity data for Homo neanderthalensis reported by (DeSilva et al., 2023), divided in pre- and post-Würm ages. The histograms for both periods confirm that the endocranial volume distribution also for H. neanderthalensis is bimodal, as for H. sapiens. The subset of data of H. neanderthalensis, reported by DeSilva et al. (2023), is quite well-balanced in terms of sex representation. Conversely, analyzing the H. sapiens’ data, we should note that one of the subsets consisting of N=25 specimens found at Afalou, Algeria (Vallois and Hallam, 1952), dated at an age of 11.5 ka, just at the beginning of the Holocene, is not well-balanced in terms of sex representation. Supplementary Figure S4 shows the histogram of these N=25 endocranial volumes. It can be noted that this subset of data is strongly unbalanced in terms of sex representation, most probably 5 females and 20 males, as it can be estimated by the difference in the integrals of the two peaks. Indeed, the mean cranial capacity falls within the second peak of the distribution (male peak) and not in between the two peaks, as it should happen for a balanced sex representation of endocranial volumes (see Supplementary Materials for more details). Therefore, this subset of data should be eliminated by the whole (DeSilva et al, 2023) dataset because the overall available data of H. sapiens’ endocranial volumes for age >1 ka is of only N = 278 individuals, and a subset of N = 25 of sex-representation unbalanced data is approximately 9% of the total, a too high percentage of the whole dataset, causing strong bias in any further analysis of the time evolution of the endocranial volume.
3 Was there an endocranial volume reduction during the end of the Holocene?
By means of the histogram analysis of Supplementary Figure S4, it has been shown that at least one subset of data—N=25 data corresponding to the age=11.5ka of the whole dataset (DeSilva et al., 2023)—used for the changepoints search was strongly biased towards male endocranial volumes. Therefore, this biased subset of data must be discarded as a not-representative value of endocranial volumes. If this sex-representation unbalanced subset of data is eliminated, the mean for the 11.7 ka ≥ age > 5 ka interval changes remarkably, as it has been shown by the red square in Supplementary Figure S2. The elimination of this subset of data affects time-evolution analysis of the endocranial volume, questioning if the changepoint of DeSilva et al. (2021, 2023) for an age of 3–5 ka is reliable.
Supplementary Figure S5 shows the histogram for the N=72 endocranial volumes of H. sapiens reported by DeSilva et al. (2023), for the 5 ka ≥ age > 0.15 ka interval. The dashed line indicates the mean cranial capacity, which falls at the end of the first main peak of the histogram. This subset of data is slightly biased towards female endocranial volumes, as it can be estimated by the difference in the integrals of the two peaks of the distribution. Therefore, the actual average cranial capacity for the 5 ka ≥ age > 0.15 ka interval should be also slightly greater than the value of 1,389 cc obtained averaging these N=72 data. In any case, if we calculate the difference between the mean of these 72 individuals corresponding to the 5 ka ≥ age > 0.15 ka interval, with respect to the mean at an age of 0.1 ka, we obtain and . Thus, the reduction in the endocranial volume in the period 5 ka ≥ age > 0.15 ka should be considered as a reliable conclusion. A better sex-balanced 5 ka ≥ age > 0.15 ka dataset would have given a still lower value of p. Thus, the above analysis confirms the result of DeSilva et al. (2021, 2023) of an endocranial volume reduction during the last 3–5 ka and discussed by several other authors (von Bonin, 1934; Tobias, 1970; Schwidetzky, 1976; Wiercinski, 1979; Beals et al., 1984; Henneberg, 1988, 1998, 2004; Brown, 1992; Henneberg and Steyn, 1993, 1995; Ruff et al., 1997; Brown and Maeda, 2004; Wu et al., 2007; Bailey and Geary, 2009; Hawks, 2011; Balzeau et al., 2013; Bednarik, 2014; Liu et al., 2014; Stibel, 2021, 2023; Wu et al., 2022).
4 Is the endocranial volume reduction characterizing all Homo sapiens evolution?
Figure 1 shows the mean endocranial volumes versus mean ages for the following: H. sapiens (black); H. neanderthalensis (red); H. heidelbergensis (blue); H. erectus (dark yellow); H. habilis and H. rudolfensis (magenta); Rudapithecus, Sahelanthropus, Ardipithecus, and Australopithecines (green). Data have been taken by DeSilva et al. (2023). The mean values shown in Figure 1 have been reported in Table 1.
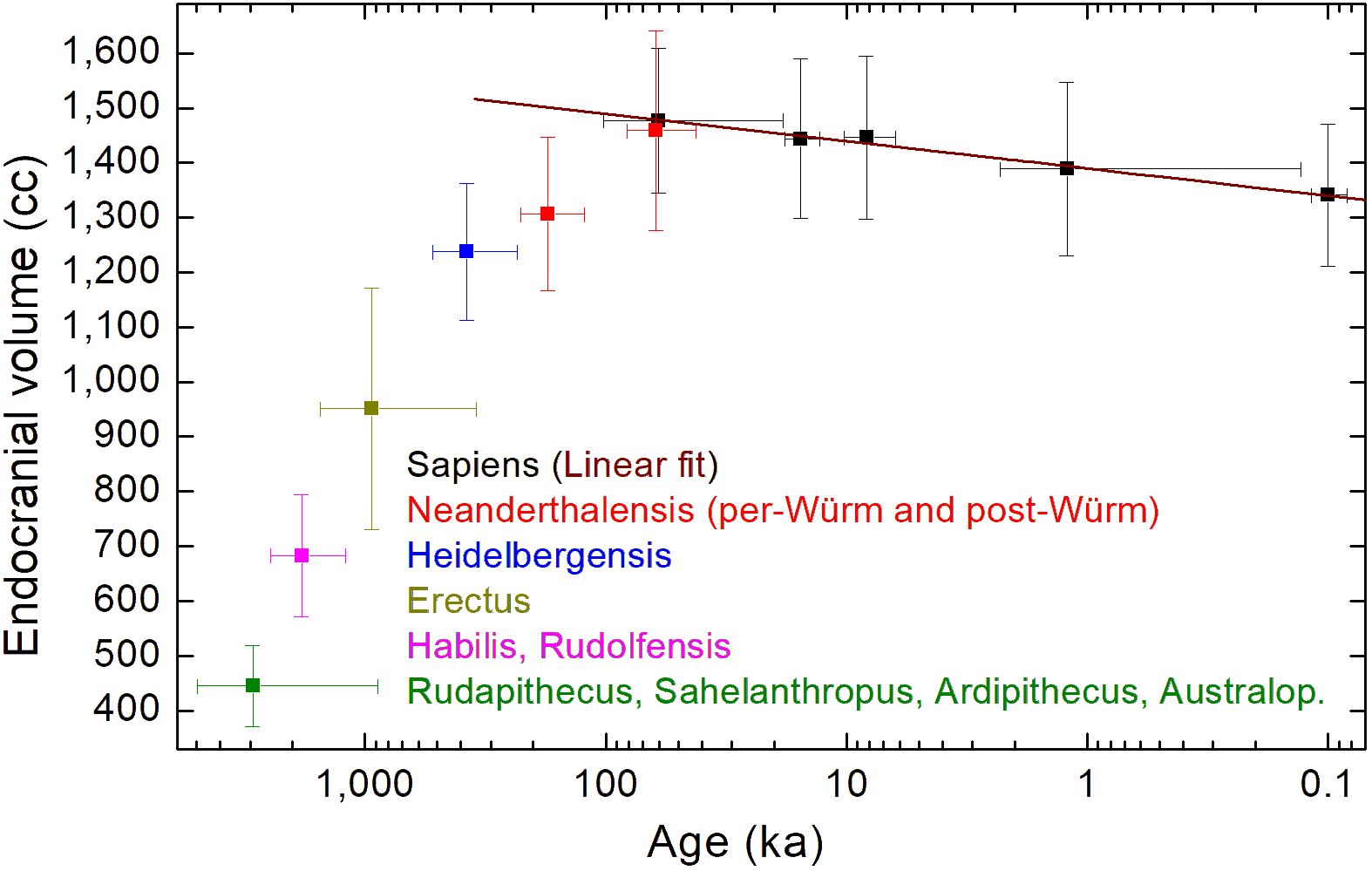
Figure 1 Mean endocranial volumes versus mean ages for H. sapiens (black); H. neanderthalensis (red); H. heidelbergensis (blue); H. erectus (dark yellow); H. habilis and H. rudolfensis (magenta); Rudapithecus, Sahelanthropus, Ardipithecus, and Australopithecines (green). Data taken by (DeSilva et al., 2023). The brown line is a linear fit of the H. sapiens’ mean data reported in Table 1.
The mean values plotted in Figure 1 shows that the mean endocranial volumes of hominids non-Homo, and of H. habilis, H. rudolfensis, and H. erectus are, in a Log(age)-scale, aligned along a nearly linear growth. The same happens for H. heidelbergensis, pre-Würm H. neanderthalensis, and post-Würm H. neanderthalensis, although the slope of the nearly linear growth evidently changes. The brown line plotted in Figure 1 is a linear regression of the endocranial volumes versus log values of the age, reported in Table 1 for H. sapiens, after having discarded the male-biased 11.5 ka subset of data, as already discussed. The linear fit for the Cranial Capacity (CC) gives the following time dependence:
with the age expressed in years and the cranial capacity expressed in cc.
In the above linear fit, we have considered mean values for avoiding the time unbalance of data towards more recent ages because reducing data to means is a standard practice in timeseries analysis calculated to represent specific temporal slices (Auger and Lawrence, 1989; Wagner et al., 2002; Villmoare and Grabowski, 2022). Supplementary Figure S6 shows details of the fit. All experimental points fall within the 95% interval of confidence if we neglect the data at 11.5 ka. The adjusted R-square is 0.98, showing that these mean values follow just a linear regression as a function of the Log(age).
If we calculate a linear regression of all data for H. sapiens reported by DeSilva et al. (2023) in the range 300 ka ≥ age > 0.1 ka, substituting only their data for the age ∼1 ka with the mean value of 1,431cc (N=10,363), without performing any averaging of data, the following linear regression is obtained:
In this last linear regression, the adjusted R-square is only 0.05, indicating that the origin of the high variance of data, with respect to the model, cannot be explained by the endocranial volume reduction as a function of Log(age). Indeed, the origin of the high variance of data is related to the high variability of cranial capacity of H. sapiens, which spans a 1,000 cc range (Holloway et al., 2004), together with the very low number of fossils available for different ages. From the linear regression, we have a reduction of approximately 50 cc for each log-decade (Equations 1, 2); 50 divided 1,000 is just 0.05, the adjusted R-square value. Therefore, due to the paucity of fossils, 95% of the variance of data of (DeSilva et al., 2023) is related to the high variability of H. sapiens’ endocranial volumes, only 5% to time evolution.
There is also another reason that affects this high variance of data. Indeed, the natural logarithm of endocranial volumes versus the natural logarithm of age has been recently shown also in Wu et al. (2022; see Figure 7 therein) for datasets published in Holloway et al. (2004), Li et al. (2017), and Ni et al. (2021). An overall increasing cranial capacity is evident, excepted in the late Pleistocene period, so confirming a period of negative rate change in H. sapiens’ endocranial-volume evolution. However, there are also important exceptions to the general trend: we have found species with very low cranial capacity (Homo floresiensis, Homo luzonensis, and Homo naledi) compared to average H. sapiens’ endocranial volumes; moreover, there is the earliest evidence of a 200–160 ka hominin (XJY6 fossil) with brain size in the upper range of H. neanderthalensis’ and modern H. sapiens’ values (Wu et al., 2022). All these findings imply a dependence of the cranial capacity not only on time but also on the geographical region, contributing further to the big variance of data.
5 Discussion
If data of different species are merged, as done in the last linear regression reported in the Supplementary Materials, the main characteristic of the H. sapiens’ evolution is lost: a gradual and continuous decrease in the endocranial volume from the origin of the species, set 300 ka ago according to the oldest fossils, until now. Some studies have placed the beginning of the cranial capacity reduction in the late Pleistocene (approximately 35 ka) (Ruff et al., 1997) and others in the more recent Holocene (about 10 ka) (Henneberg, 1988; Hawks, 2011). Instead, the linear fit of Figure 1 indicates that endocranial volume reduction of H. sapiens is not a characteristic only of the last 3–5 ka, but it is going on at least from the late Pleistocene, as suggested in (Ruff et al., 1997), and probably from the beginning, from the oldest fossils’ age (300 ka). Figure 1 shows that different species have different processes of endocranial volume increase/decrease (Bruner, 2017). In particular, the evolution of H. sapiens’ endocranial volume seems to be in countertrend to the overall increase common to other hominid/hominin species, if we discard few exceptions, related to the small-brained Middle and Late Pleistocene hominins Homo naledi (Berger et al., 2015; Montgomery, 2018) and Homo floresiensis (Brown et al., 2004b). Homo neanderthalensis is characterized by a time increment of the average endocranial volume, which reaches the H. sapiens’ value when interbreeding of the two species has, probably, become more pronounced (Gokcumen, 2020), as shown by the pre-Würm and post-Würm mean values plotted in Figure 1, with the post-Würm value for Homo neanderthalensis very close to the H. sapiens’ value of the same age.
Figure 1 shows that H. sapiens’ evolution seems to be an exception, because its mean cranial capacity has been decreasing as a function of time, from the oldest age of the first fossils found. This finding could imply that H. sapiens’ origin might be related to more ancient hominid species than those until now discovered. Future work could aim to extend the analysis of DeSilva et al. (2023) also to other dataset not considered in their work, such as the dataset reported in (Stanyon et al., 1993). Also the analysis of brain weights normalized to the body weight (Nooranipour and Farahani, 2008), for fossils for which this is possible, could allow to obtain new insights on endocranial volume time evolution. In any case, future discoveries of new fossils, particularly of very ancient Homo-sapiens’ fossils (perhaps also for age >300 ka), and new genetic studies of the archaic interbreeding of hominins (Rogers et al., 2020; Bergström et al., 2021), should allow to better understand the origin of our species and its endocranial volume evolution.
Author contributions
LD: Conceptualization, Data curation, Formal analysis, Investigation, Methodology, Project administration, Resources, Software, Supervision, Validation, Visualization, Writing – original draft, Writing – review & editing.
Funding
The author(s) declare that no financial support was received for the research, authorship, and/or publication of this article.
Conflict of interest
The author declares that the research was conducted in the absence of any commercial or financial relationships that could be construed as a potential conflict of interest.
Publisher’s note
All claims expressed in this article are solely those of the authors and do not necessarily represent those of their affiliated organizations, or those of the publisher, the editors and the reviewers. Any product that may be evaluated in this article, or claim that may be made by its manufacturer, is not guaranteed or endorsed by the publisher.
Supplementary material
The Supplementary Material for this article can be found online at: https://www.frontiersin.org/articles/10.3389/fevo.2024.1368347/full#supplementary-material
References
Auger I., Lawrence C. (1989). Algorithms for the optimal identification of segmented neighborhoods. Bull. Math. Biol. 51, 39–54. doi: 10.1016/S0092-8240(89)80047-3
Bailey D. H., Geary D. C. (2009). Hominid brain evolution. Hum. Nat. 20, 67–79. doi: 10.1007/s12110-008-9054-0
Balzeau A., Grimaud-Hervé D., Détroit F., Holloway R. L., Combès B., Prima S. (2013). First description of the Cro-Magnon 1 endocast and study of brain variation and evolution in anatomically modern Homo sapiens. Bull. Mém. Soc Anthropol. Paris 25, 1–18. doi: 10.1007/s13219-012-0069-z
Beals K. L., Smith C. L., Dodd S. M. (1984). Brain size, cranial morphology, climate and time machines. Curr. Anthropol. 25, 301–330. doi: 10.1086/203138
Bednarik R. G. (2014). Doing with less: hominin brain atrophy. Homo 65, 433–449. doi: 10.1016/j.jchb.2014.06.001
Berger L. R., Hawks J., de Ruiter D. J., Churchill S. E., Schmid P., Delezene L., et al. (2015). Homo naledi, a new species of the genus Homo from the Dinaledi chamber. South Africa. eLife 4, e09560. doi: doi.org/10.7554/eLife.09560
Bergström A., Stringer C., Hajdinjak M., Scerri E. M. L., Skoglund P. (2021). Origins of modern human ancestry. Nature 590, 229–237. doi: 10.1038/s41586-021-03244-5
Brown P. (1992). Recent human evolution in East Asia and Australasia. Philos. Trans. R. Soc London B Biol. Sci. 337, 235–242. doi: 10.1098/rstb.1992.0101
Brown P., Maeda T. (2004). Post-Pleistocene diachronic change in east Asian facial skeletons: the size, shape and volume of the orbits. Anthropol. Sci. 112, 29–40. doi: 10.1537/ase.00072
Brown P., Sutikna T., Morwood M. J., Soejono R. P., Saptomo E. W., Due R. A. (2004b). A new small-bodied hominin from the Late Pleistocene of Flores, Indonesia. Nature 431, 1055–1061. doi: 10.1038/nature02999
Bruner E. (2017). “The Fossil Evidence of Human Brain Evolution,” in Evolution of Nervous Systems, 2nd ed., Elsevier, Oxford vol. 4. , 63–69.
Dekaban A. S., Sadowsky D. (1978). Changes in brain weights during the span of human life: relation of brain weights to body heights and body weights. Ann. Neurol. 4, 345–356. doi: 10.1002/ana.410040410
DeSilva J. M., Fannin L. D., Chaney I., Claxton A. G., Ilies I., Kittelberger J., et al. (2023). Human brains have shrunk: the questions are when and why. Front. Ecol. Evol. 11. doi: 10.3389/fevo.2023.1191274
DeSilva J. M., Traniello J. F. A., Claxton A. G., Fannin L. D. (2021). When and why did human brains decrease in size? A new change-point analysis and insights from brain evolution in ants. Front. Ecol. Evol. 9. doi: 10.3389/fevo.2021.742639
Gokcumen O. (2020). Archaic hominin introgression into modern human genomes. Yearbook Phys. Anthropol. 171, 60–73. doi: 10.1002/ajpa.2395160
Hawks J. (2011). Selection for smaller brains in Holocene human evolution. arXIV. doi: 10.48550/arXiv.1102.5604
Henneberg M. (1998). Evolution of the human brain: is bigger better? Clin. Exp. Pharmacol. Physiol. 25, 745–749. doi: 10.1111/j.1440-1681.1998.tb02289.x
Henneberg M. (2004). The rate of human morphological microevolution and taxonomic diversity of hominids. Stud. Hist. Anthropol. 2006, 49–59.
Henneberg M., Steyn M. (1993). Trends in cranial capacity and cranial index in Subsaharan Africa during the Holocene. Am. J. Hum. Biol. 5, 473–479. doi: 10.1002/ajhb.1310050411
Henneberg M., Steyn M. (1995). Diachronic variation of cranial size and shape in the Holocene: a manifestation of hormonal evolution? Riv. Antropol. 73, 159–164.
Ho K. C., Roessmann U., Straumfjord J. V., Monroe G. (1980). Analysis of brain weight. I. Adult brain weight in relation to sex, race, and age. Arch. Pathol. Lab. Med. 104, 635–639. doi: 10.1038/nature10629
Holloway R. L., Broadfield D. C., Yuan M. S. (2004). “Brain Endocasts: The Paleoneurological Evidence,” in The Human Fossil Record, vol. 3 . Eds. Schwartz J. H., Tattersall I. (Wiley-Liss, New York). doi: 10.1002/0471663573
Killick R., Eckley I. A. (2014). changepoint: an R package for changepoint analysis. J. Stat. Software 58, 1–19. doi: 10.18637/jss.v058.i03
Li Z. Y., Wu X. J., Zhou L. P., Liu W., Gao X., Trinkaus E. (2017). Late Pleistocene archaic human crania from Xuchang, China. Science 355, 969e972. doi: 10.1126/science.aal2482
Liu C., Tang Y., Ge H., Wang F., Sun H., Meng H., et al. (2014). Increasing breadth of the frontal lobe but decreasing height of the human brain between two Chinese samples from a Neolithic site and from living humans. Am. J. Phys. Anthropol. 154, 94–103. doi: 10.1002/ajpa.22476
Montgomery S. H. (2018). Hominin brain evolution: the only way is up? Curr. Biol. 28, R788–R790. doi: 10.1016/j.cub.2018.06.021
Muggeo V. M. R. (2008). segmented: an R package to fit regression models with broken-line relationships. R News 8, 20–25.
Ni X. J., Ji Q., Wu W. S., Shao Q. F., Ji Y., Zhang C., et al. (2021). Massive cranium from Harbin establishes a new Middle Pleistocene human lineage in China. Innovation 2, 100130. doi: 10.1016/j.xinn.2021.100130
Nooranipour M., Farahani R. M. (2008). Estimation of cranial capacity and brain weight in 18–22-year-old Iranian adults. Clin. Neurol. Neurosurg. 110, 997–1002. doi: 10.1016/j.clineuro.2008.06.006
Rogers A. R., Harris N. S., Achenback A. A. (2020). Neanderthal-Denisovan ancestors interbred with a distantly related hominin. Sci. Adv. 6, 5483. doi: 10.1126/sciadv.aay5483
Ruff C. B., Trinkaus E., Holliday T. W. (1997). Body mass and encephalization in Pleistocene Homo. Nature 387, 173–176. doi: 10.1038/387173a0
Rushton J. P. (1994). Sex and race differences in cranial capacity from international labour office data. Intelligence 19, 281–294. doi: 10.1016/0160-2896(94)90002-7
Schwidetzky I. (1976). Postpleistocene evolution of the brain? Am. J. Phys. Anthropol. 45, 605–611. doi: 10.1002/ajpa.1330450327
Stanyon R., Consigliere S., Morescalchi M. A. (1993). Cranial capacity in hominid evolution. Hum. Evol. 8, 205–216. doi: 10.1007/BF02436715
Stibel J. M. (2021). Decreases in brain size and encephalization in anatomically modern humans. Brain Behav. Evol. 96, 64–77. doi: 10.1159/000519504
Stibel J. M. (2023). Climate change influences brain size in humans. Brain Behav. Evol. 98, 93–106. doi: 10.1159/000528710
Tobias P. V. (1970). Brain-size, grey matter and race—fact or fiction? Am. J. Phys. Anthropol. 32, 3–25. doi: 10.1002/ajpa.1330320103
Vallois H. V., Hallam L. M. (1952). “Catalogue des hommes fossiles,” in Proceedings of the 19th Session of the International Congress of Geologists, Algiers, Vol. 5.
Villmoare B., Grabowski M. (2022). Did the transition to complex societies in the Holocene drive a reduction in brain size? A reassessment of the DeSilva et al., (2021) hypothesis. Front. Ecol. Evol. 10. doi: 10.3389/fevo.2022.963568
von Bonin G. (1934). On the size of man's brain as indicated by skull capacity. J. Comp. Neurol. 59, 1–28. doi: 10.1002/cne.900590102
Wagner A. K., Soumerai S. B., Zhang F., Ross-Degnan D. (2002). Segmented regression analysis of interrupted time series studies in medication use research. J. Clin. Pharm. Ther. 27, 299–309. doi: 10.1046/j.1365-2710.2002.00430.x
Wiercinski A. (1979). Has the brain size decreased since the upper paleolithic period? / La taille du cerveau a-t-elle diminué depuis le Paléolithique supérieur? Bull. Mém. Soc Anthropol. Paris 6, 419–427. doi: 10.3406/bmsap.1979.1979
Wu X. J., Bae C. J., Friess M., Xing S., Athreya S., Liu W. (2022). Evolution of cranial capacity revisited: A view from the late Middle Pleistocene cranium from Xujiayao, China. J. Hum. Evol. 163, 103119. doi: 10.1016/j.jhevol.2021.103119
Keywords: encephalization, bimodal distribution, Homo sapiens, Holocene, Pleistocene
Citation: De Caro L (2024) Commentary: Human brains have shrunk: the questions are when and why. Front. Ecol. Evol. 12:1368347. doi: 10.3389/fevo.2024.1368347
Received: 10 January 2024; Accepted: 25 March 2024;
Published: 11 April 2024.
Edited by:
Mathieu Lihoreau, Centre National de la Recherche Scientifique (CNRS), FranceReviewed by:
Emmanuel Paul Gilissen, Royal Museum for Central Africa, BelgiumCopyright © 2024 De Caro. This is an open-access article distributed under the terms of the Creative Commons Attribution License (CC BY). The use, distribution or reproduction in other forums is permitted, provided the original author(s) and the copyright owner(s) are credited and that the original publication in this journal is cited, in accordance with accepted academic practice. No use, distribution or reproduction is permitted which does not comply with these terms.
*Correspondence: Liberato De Caro, bGliZXJhdG8uZGVjYXJvQGNuci5pdA==