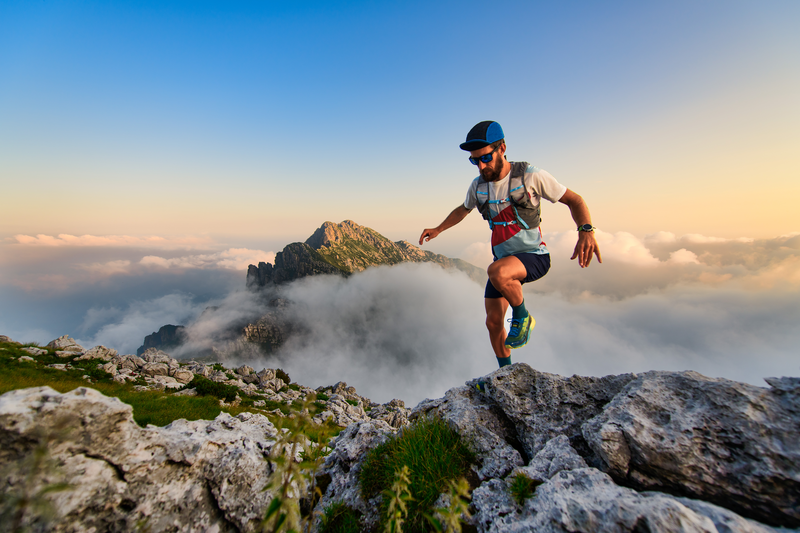
94% of researchers rate our articles as excellent or good
Learn more about the work of our research integrity team to safeguard the quality of each article we publish.
Find out more
ORIGINAL RESEARCH article
Front. Ecol. Evol. , 23 May 2024
Sec. Behavioral and Evolutionary Ecology
Volume 12 - 2024 | https://doi.org/10.3389/fevo.2024.1360395
Intraspecific variation in sex pheromones is a driver of reproductive isolation and speciation in insects. The False Codling Moth (FCM) Thaumatotibia leucotreta (Lepidoptera: Tortricidae) is a quarantine pest endemic to sub-Saharan Africa (SSA). The currently available precision control measures for FCM use female sex pheromone components to lure males into traps. However, the existing data on the composition of the female sex pheromone, especially the isomer ratios of the main pheromone component (E/Z)-8-dodecenyl acetate, are inconsistent for populations in SSA. This inconsistency led to speculation about possible reproductive isolation between geographically separated FCM populations and the potential need for local adjustment of pheromone-based FCM control tools. This, however, requires a comparative evaluation of geographic variation in FCM sexual communication and inter-population mating compatibility. We therefore investigated genetic isolation and mating compatibility between five geographically isolated FCM populations in South Africa and analysed the ratio of (E)- and (Z)-8-dodecenyl acetate in females from these populations. The five studied populations were found to form three genetically distinct groups with high genetic distances between each other. Mating compatibility tests showed that mating is possible across these groups, however, males preferred females of their own population when given choices; without a choice, males successfully mated with and transferred spermatophores to females from all other populations. The ratio of (E)- and (Z)-8-dodecenyl acetate was similar (c. 4:1) across the populations, indicating that this main female pheromone component does not cause the observed intra-population mating preferences. It remains to be investigated if qualitative/quantitative variation in other sex pheromone components influences intra-population recognition in South African FCM, providing a base for the development of regionally-specific lures for area-wide control programmes.
Environmentally friendly methods for managing insect pests are crucial for ecosystem functioning, biodiversity, and conservation (Araújo et al., 2023; Arp et al., 2023). Behavioural manipulation, using specific chemical signals (semiochemicals), is a strategy that has received much attention in the past 80 years (Steurer et al., 2024, but see Foster and Harris, 1997 for a review). One approach to manipulating the behaviour of insect pests is through pheromones, including sex pheromones (Bengtsson et al., 1994; Witzgall et al., 2010; Miller and Gut, 2015). Sex pheromones are crucial for the recognition, attraction, and selection of potential conspecific mates, and for preventing the attraction of heterospecific individuals to maintain species integrity (Wyatt, 2003; De Pasqual et al., 2021). Since the identification of the pheromone bombykol in the silkworm moth Bombyx mori L. (Lepidoptera: Bombycidae) in the 1950s, the first description of an insect sex pheromone (Butenandt et al., 1959), the identification, isolation, and use of sex pheromones has advanced and is now an essential tool in area-wide integrated pest management (AW-IPM) strategies (Stern et al., 1959; Wright, 1964; Witzgall et al., 2010; Gao et al., 2020). These strategies exploit the response of male insects to female sex pheromones, in particular male attraction, using inanimate objects baited with the specific female sex pheromone blend (Regnier and Law, 1968). However, sex pheromones (see De Pasqual et al., 2021 for a detailed review), the key signals for sexual communication, can overlap interspecifically (De Pasqual et al., 2021), and vary intraspecifically in many insects, including pests with wide geographic distributions (Rodriguez-Saona and Stelinski, 2009; Gao et al., 2020; De Pasqual et al., 2021).
In moth species, geographic variation may arise as a result of avoiding inbreeding with closely related sympatric species that share key pheromone components (McElfresh and Millar, 1999; Groot et al., 2008; Sadek et al., 2012). Variation can also be manifested in the timing of sexual behaviours or be a consequence of physiological, biotic, and abiotic factors such as age (Swier et al., 1977; Noldus and Potting, 1990; Xavier et al., 2018), nutrition (Casimero et al., 2001), temperature (Conner et al., 1985; Delisle and McNeil, 1987), relative humidity (Webster and Cardé, 1982; Royer and McNeil, 1991), photoperiod (Delisle and McNeil, 1986; Noldus and Potting, 1990; Kamimura and Tatsuki, 1994), host plants (Landolt and Phillips, 1997), wind speed (Conner et al., 1985), and insecticides (Shen et al., 2013; Navarro-Roldán and Gemeno, 2017). Therefore, it is essential to understand the local sex pheromone blends and local timings of sexual communication for effective field implementation of sex pheromone-dependent control tools (Gao et al., 2020).
The False Codling Moth (hereafter “FCM”), Thaumatotibia leucotreta Meyrick (Lepidoptera: Tortricidae), endemic to sub-Saharan Africa (hereafter “SSA”), is a pest of several economically important crops, including citrus, corn, cotton, pepper, pomegranate, peach, and plum (Venette et al., 2003; Hofmeyr et al., 2005; Adom et al., 2020). FCM is one of the biggest biotic threats to the citrus industry of southern Africa because of its classification as a phytosanitary or quarantine pest in international markets, such as the United States of America (USA), European Union (EU), and the Far East (Venette et al., 2003; Moore, 2021). Rejection of export consignments due to FCM detection is devastating to the economies of affected countries in SSA, such as the southern African citrus industry, comprising three citrus-exporting countries (South Africa, Zimbabwe, and Eswatini), with an estimated annual export of 2.2 million tons, valued at USD 1.5 billion (CGA, 2021). Consequently, FCM is diligently controlled in citrus orchards in South Africa (Hattingh et al., 2020). Due to the stringent restrictions on synthetic chemical pesticide residues on exported fruit by certain markets, FCM control is focused on IPM; the most important options of IPM against FCM are based on the use of female sex pheromones (Hofmeyr and Pretorius, 2010; Hattingh et al., 2020; Moore, 2021). This includes monitoring, attract-and-kill and mating disruption (Moore and Hattingh, 2012).
Existing reports of the composition of the female FCM pheromone are remarkably varied, in terms of both the particular compounds and the ratios of different isomers (reviewed in Levi-Zada et al., 2020). The most recent study of female moths from Israel reported eleven compounds in the female FCM sex pheromone blend (Levi-Zada et al., 2020). Corroborating previous studies, only (E)-8-dodecenyl acetate (E8–12:Ac) and (Z)-8-dodecenyl acetate (Z8–12:Ac) were shown to play a key role in FCM sexual communication (Zagatti et al., 1983; Hall et al., 1984; Attygalle et al., 1986; Levi-Zada et al., 2020). However, studies from SSA have reported discrepant ratios of these two compounds as the optimal blend both in laboratory assays and field evaluations. To date, there is no clarity on the composition of the most effective blend of the pheromone for IPM or the natural ratio of isomers in SSA FCM populations. For example, following the extraction and evaluation of female sex pheromone glands, Persoons et al. (1977) reported the E/Z ratio as 1:1, Angélini et al. (1981) and Zagatti et al. (1983) found 3:1, Hall et al. (1984) reported 2:3, while Attygalle et al. (1986) found 9:1. Newton et al. (1993) highlighted 8:2 and 9:1 as effective blends. Recently, Levi-Zada et al. (2020) reported 9:1, but the population tested by the latter authors was from Israel, not SSA. The discrepancies in the published isomer ratios from different populations may reflect intraspecific variation in the sex pheromone across SSA. Attygalle et al. (1986) took this one step further and postulated the occurrence of subspecies of FCM in SSA, which differ in the ratio of E8–12:Ac and Z8–12:Ac. More recent laboratory and field studies performed in South Africa provide some support for the possibility of FCM subspecies (e.g., Timm et al., 2008; 2010; Joubert, 2018; Upfold, 2019).
Considering that the functionality of the established IPM programme for FCM in southern Africa relies on sex pheromone-based control tools (i.e., monitoring, attract-and-kill, mating disruption, and SIT), it is paramount to fully establish whether the FCM pheromone blend varies geographically and whether intraspecific variation in the pheromone blend may influence the effectiveness of existing control tools. Exploring the potential role of intraspecific variation in the pheromone blend in premating isolation would also inform discussions of incipient speciation within FCM. This study specifically addresses these questions by investigating the mating compatibility of FCM from different geographic populations in South Africa and assessing the ratios of E8–12:Ac and Z8–12:Ac in females from these populations. The implications of our findings are discussed in the context of AW-IPM of FCM.
FCM populations from four citrus-producing regions in South Africa were sampled. The populations originated from wild FCM individuals collected from Addo (33°34’S, 25°41’E, Eastern Cape Province), Marble Hall (24°58’S, 24°18’E, Limpopo Province), Citrusdal (32°36’S,19°01’E, Western Cape Province) and Nelspruit (25°28’S, 30°58’E, Mpumalanga Province) (Opoku-Debrah et al., 2014). A fifth population was reared from individuals originating from unspecified locations in the Western Cape and Eastern Cape provinces (Moore, 2002). The populations were named after their place of origin; the mixed population is referred to as Old culture (hereafter “Old Colony”). Each of these five FCM populations was maintained separately in a controlled environment (CE) room at the Centre for Biological Control in the Department of Zoology and Entomology at Rhodes University, Makhanda, South Africa. In order to prevent adaptations to laboratory rearing conditions or “evolution” of laboratory strains, the genetic pool of all five populations was maintained by intermittent re-supply from the wild populations. The CE rearing conditions included a constant temperature of 26°C ± 2°C, 50–60% relative humidity, and a 12-hour photoperiod (L12:D12). Each population was kept in a separate rearing cage (size: 120 x 100 x 75 cm; model: Perspex®) to prevent interbreeding, and maintained on an artificial diet developed by Moore et al. (2014).
In order to assess genetic differentiation as an indicator for genetic isolation between the five studied South African FCM populations, the genetic integrity of each population was determined using the Amplified Fragment Length Polymorphism (AFLP) microsatellite analysis protocol developed by Paun and Schönswetter (2012), albeit with minor modifications, using adapters and primers as determined by Timm et al. (2010). Genomic DNA was isolated from entire crushed larvae (1 per sample) and preserved in 95% ethanol, according to standard procedures involving proteinase K digestion, followed by a salt-extraction method (Hunt, 1997), which is optimal for whole-insect DNA extraction. Five replicates per population were prepared.
To investigate inter-population mating behaviour, mating compatibility tests were performed with laboratory reared individuals from the five geographically isolated FCM populations. The moths were sexed using key sexual diagnostic features of the pupal stage, i.e., the presence of two notches on the fifth pupal segment of male pupae, absent in females (Daiber, 1979). Sexed individuals of all populations were then placed into separate glass vials sealed with dampened cotton wool and labelled with the name of the population and sex. To distinguish between individuals of different populations in each test, moths of one population were dyed using Calco Oil Red N-1700® added to the larval diet with manufacturers’ instructions. The dye is taken up by fat bodies and does not reduce the fitness of the moth (Davis, 1973). As a double measure, moths from the dyed population were also patted down gently using cotton wool to remove some of the moths’ scales, giving them a lighter appearance. The loss of wing scales, common for moths used in the FCM SIT programmes, does not significantly influence flight ability or other behaviours (Craig Chambers, pers. comm.). Nevertheless, to avoid any possible bias from the dye or removal of scales, these identification methods were rotated among the populations for each replicate. Mating compatibility was then assessed via no-choice and choice tests.
In order to test if males can successfully mate with females from a different population, no-choice tests were performed. For these tests, five 2-day-old virgin females and one male were placed in a nylon mesh cage (30 cm × 30 cm) for two consecutive nights. After 48 h, the females were freeze-killed at -20°C overnight and each female was dissected under a dissecting microscope (Leica EZ4 Fluorescence Stereo Microscope) in order to assess the presence of spermatophores in their bursa copulatrix. Five assays were performed per mating combination. Assays with males and females from the same population were performed as the control treatment to compare the ability of males to transfer spermatophores to females from other populations.
Given that in this species the males are those that select the partner (Aigbedion-Atalor et al., 2024), in order to assess whether males discriminated females from their own or other population, choice tests in which males were offered females from their own population and from one of the other populations were conducted, yielding a total of 10 pairwise comparisons (Supplementary Figure S1). These mating compatibility tests were conducted in a large 3 m × 3 m square white nylon mesh cage with a white canvas base, in a large temperature-controlled greenhouse, with natural light (see Supplementary Figure S1). Trays of females holding glass vials (n = 30) containing 2-day-old virgin female moths (one per vial) from two different populations (n=60) were placed on a tray upwind from the males. Reciprocally, vials (n = 30) containing the 2-day old males (one per vial) from a single population were placed on a tray downwind from the females. A large ventilated fan provided airflow in the facility. The glass vials were opened at 17h00, allowing the moths to exit the vials voluntarily and independently. Every 30 minutes, an observer entered the cage to look for mating pairs, which were collected into glass vials, and the population of individuals in each mating pair was recorded. Males that were not responsive (morbid or dead) were replaced. The test ended either after 90 minutes of no mating activity or a maximum duration of five hours if mating activities were observed. The trial was repeated three times for each population combination.
In order to determine the sexual compatibility and mating performance of the geographically isolated FCM populations, different performance indices were calculated. The suitability of the adults and the environmental conditions (nylon mesh cages) for mating were determined by calculating the participation in mating (PM), representing overall mating activity. According to the Food and Agriculture Organisation (FAO), the International Atomic Energy Agency (IAEA) and the United States Department of Agriculture (USDA), the minimum valid PM value is 0.2, i.e., at least 20% of the total amount of possible couples need to have mated (FAO/IAEA/USDA, 2003).
In addition, sexual compatibility between the five populations was assessed using the index of sexual isolation (ISI) as well as the male and female relative performance index (MRPI, FRPI) (Cayol et al., 1999; Taret et al., 2010). ISI accounts for the number of pairs obtained for each possible mating combination, and ranges from -1, where most couples consisted of the second population listed, to 1 where most couples consist of the first population listed. A value of 0 represents random mating: equal proportion of the four possibilities of mating, i.e., homotypic (AA and BB) or heterotypic (AB and BA), where A and B represent two different populations.
To clarify the ISI value, the two other indices MRPI and FRPI were calculated in order to account for variations in male and female mating propensity, regardless of their mating partners (Cayol et al., 1999). MRPI and FRPI values range from 1, where all mating events are performed by males (MRPI) or females (FRPI) of one type (the first population to be listed), through an equilibrium at 0 where there is an equal participation in mating by males (MRPI) or females (FRPI) of both types, to -1 where all mating events are performed by males (MRPI) or females (FRPI) of the other type (second population listed). The MRPI and FRPI explain the role of the males and females from two different populations compared in each experiment, and thus complement the ISI very well (Taret et al., 2010).
Pheromones were extracted from FCM females of all five studied populations. From each population, unmated, 2-day-old females were frozen at 3:00 am and stored at -20°C until extraction of the pheromone. FCM females are known to release sex pheromones from a membranous gland situated dorsally between the 8th and 9th segment of the abdomen (see Attygalle et al., 1986). In order to extract the pheromone, the last three segments (“abdominal tip”) were dissected and placed in a 1.5 ml conical glass vial containing 80 µl dichloromethane (DCM; 99.8%, Honeywell Riedel-de Haën). Abdominal tips from a total of 25 females per population were pooled in one vial. Each vial was vortexed for ca. 30 seconds and stored at room temperature for 30 minutes. The solvent was subsequently transferred to a clean glass vial and stored at -20°C. For each population, extracts were replicated five times. Highly viscous extracts were diluted with an additional 5–10 µl of DCM.
The pheromone extracts were analysed using gas chromatography (GC) with a flame ionisation detector (FID). These analyses were performed on a Varian CP3800 GC equipped with an FID and Varian 1079 PTV injector port. Volatiles in extracts were separated on a polar SGE SolGel wax capillary column (30 m x 0.32 mm inner diameter, 0.25 μm film thickness). For each run, 1.0 μl of extract was injected with a 10:1 split on the injector, and the injector was held at 200°C for 5 min, and then increased to 250°C at 200°C per min and held for the remainder of the run. The GC oven temperature was increased from 40°C to 260°C at 10°C per min, held at 260°C for 12 min and then increased to 280°C at 20°C per min and held at 280°C for five min. The GC was operated in constant flow mode using helium at a flow rate of 2.0 ml per min as the carrier gas. Pheromone peaks were identified by comparison with retention times of synthetic standards of both isomers E8–12:Ac (Purity: 99.4% Insect Science®) and Z8–12:Ac (Purity: 98.9% Insect Science®) injected under identical conditions. The ratios of the two isomers were calculated based on peak areas.
The absence of co-eluting compounds in the E8–12:Ac and Z8–12:Ac peaks in the pheromone extract chromatograms was confirmed by injecting a subset of extracts from each population on a Scion 8500 gas chromatograph coupled to a SCION SQ single quadrupole mass spectrometer (GC-MS) with a 1079 PTV injector port and a Scion-WaxMS polar column (30 m x 0.25 mm inner diameter x 0.25 μm film thickness). This stationary phase has similar polarity to the column used in the GC-FID analyses described above. For each run, 1 µl of extract was injected. The injector was held at 40°C for 2 min (split ratio 20:1) and then increased to 200°C at 200°C min−1 in splitless mode. The GC oven temperature was held at 40°C for 3 min then increased to 240°C at 10°C per min, held at 240°C for 12 min and then increased to 250°C at 20°C per min and held at 250°C for 24.5 min. The GC was operated in constant flow mode using helium at a flow rate of 1.0 ml per min as the carrier gas. Pheromone peaks were identified by comparison with retention times of synthetic standards of both isomers (E8–12:Ac and Z8–12:Ac) injected under identical conditions. Purity of peaks was confirmed by comparison with mass spectra from the standard and close scrutiny of the mass spectra throughout the width of the peak.
To investigate the genetic relationships among populations, the resulting binary matrix from the population-level genetic differentiation was imported and consolidated in Microsoft® Excel. This matrix was then converted to a pairwise similarity matrix using Bray-Curtis dissimilarity index. Differences between populations were tested with a one-way Analysis of Similarities (ANOSIM), where R = 1 was the most distant, and R = 0 was the most related. Relationships among individuals and populations were viewed as Principal Coordinates Analysis (PCoA) plots using Jaccard’s permutation in the statistical software PAST (Kosman and Leonard, 2007). At the population level, the percentage of polymorphic loci (PPL) was calculated using GenAlEx v6.5 Excel software package (Peakall and Smouse, 2012). Shannon Information Index (Ho), and Nei’s genetic diversity (Hj) were calculated using POPGENE v3.0 (Yeh et al., 1999; Fu et al., 2016). The relationships between populations were determined based on pairwise measures of genetic distance (D) and genetic identity (I), calculated using unbiased genetic distance algorithm (Nei, 1978) with the software POPGENE v3.0 (Yeh et al., 1999).
The indices of sexual compatibility and mating performance were assessed across five populations via a one-sample Student’s t-test to determine whether the Index (ISI, MRPI and FRPI) differed significantly from the theoretical value expected for equal competitiveness or random mating (Tejeda et al., 2017). This was performed using R (v4.2.3) foundation environment for statistical computing (R Core Team, 2022).
To evaluate the number of spermatophores per male transferred into females, Shapiro-Wilks test for normality was used, followed by a Generalised Linear Model (GLM) with a Poisson distribution, and log-link function was used to analyse the count data (McElduff et al., 2010) in R (v4.2.3). Female population was the explicated or predictor variable for the response variable (i.e., spermatophores)
To compare the mean ratios of E8–12:Ac to Z8–12:Ac between the different populations, the proportions of each isomer were logit transformed and the mean proportions compared using a one-way Analysis of Variance (ANOVA) using IBS SPSS Statistics (Version 28.0.0.0). Means and standard errors were calculated from the transformed values and back-transformed for graphs.
The principal coordinate analysis (PCoA) for individuals of five geographically isolated FCM populations (five larvae per population) revealed three genetically distinct groups. Individuals from Addo, Citrusdal, and the Old Colony populations grouped together, while individuals from Nelspruit and individuals from Marble Hall formed separated groups each (Figure 1).
Figure 1 Principal coordinate analysis representing 14.38% (PCoA 1) and 11.72% (PCoA 2) of the genetic variance displaying the relationships of individuals from five geographically isolated populations of Thaumatotibia leucotreta (False Codling Moth, FCM).
The total percentage polymorphic loci (PPL) for the studied populations ranged from 33.44% (Marble Hall) to 49.35% (Old Colony) (Table 1). The Old Colony population had the highest genetic diversity (Hj = 0.1849; Ho = 0.1575), while the lowest genetic diversity was found in the Marble Hall population (Hj = 0.135; Ho = 0.1282) (Table 1).
Table 1 Genetic diversity among populations of Thaumatotibia leucotreta (False Codling Moth, FCM) from different geographical regions.
The total genetic diversity was significantly different with high genetic distances between the studied populations (one-way ANOSIM: R = 0.77; P = 0.001) (Table 2).
Table 2 Genetic distances (shaded grey) and genetic identity (italicised) indices (Nei, 1978) estimated based on AFLP analysis for five populations of Thaumatotibia leucotreta (False Codling Moth, FCM).
In the no-choice tests mating was not selective, meaning that successful mating occurred between all pairwise comparisons and there were no significant differences in the number of spermatophores recovered from the female bursa copulatrix (χ2 = 103. 73; df = 24; P = 0.722; Figures 2, 3).
Figure 2 Mean (± SE) number of spermatophores transferred by Thaumatotibia leucotreta (False Codling Moth, FCM) males to females in no-choice mating compatibility tests. The number of transferred spermatophores is used as an indicator for the mating ability of males from Addo, Citrusdal, Marble Hall, Nelspruit and Old Colony with females from these populations. There were no significant differences in the number of spermatophores found in the bursa copulatix of females from Addo, Citrusdal, Marble Hall, Nelspruit and the Old Colony population after mating with males from these populations (Generalized Linear Modle: P>0.05).
Figure 3 Details of Thaumatotibia leucotreta (False Codling Moth, FCM) female reproductive structures after copulation. (A) Abdominal tip with external reproductive organ, (B) dissected bursa copulatrix showing spermatophores and (C) spermatophore recovered from bursa copulatrix. Magnification: 10× (A, B), 40× (C).
The average participation of mating (PM) during the performed mating choice tests between the studied populations was 0.34 (34% of possible mating pairs in the cages). This PM value is higher than the valid minimum proportion of mating inclusion (PM = 0.2) (FAO/IAEA/USDA, 2003), and we can therefore assume that the conditions under which the tests were run were adequate. The ISI revealed that mating was generally random (ISI = 0) in the pairwise combinations tested (Table 3), however, in four population combinations the mating choice was significantly selective for intra-population mating partners, i.e., Addo × Nelspruit (ISI = 0.13; t2 = 6.23; P = 0.02), Addo × Marble Hall (ISI = 0.11; t2 = 4.72; P = 0.04), Citrusdal × Nelspruit (ISI = 0.11; t2 = 4.95; P = 0.04) and Citrusdal × Marble Hall (ISI = 0.12; t2 = 4.31; P = 0.04) (Table 3). In these combinations, there were more homogenous couples from Addo and Citrusdal populations than from Nelspruit and Marble Hall populations. The MRPI values were significant for Addo × Nelspruit (MRPI = 0.19; t2 = 6.23; P = 0.02), Addo × Marble Hall (MPRI = 0.17; t2 = 6.65; P = 0.02) and Citrusdal × Nelspruit (MRPI = 0.13; t2 = 4.39; P = 0.04) (Table 3), indicating that males originating from Addo and Citrusdal populations participated in more mating events than males from Nelspruit and Marble Hall; FRPI values for these population combinations were not significant indicating that the mating choices are actively made by males while the females are passive (“male mate choice”). The MRPI and FRPI values for the fourth significant combinations, Citrusdal × Marble Hall, were inconclusive, as no significance for either MRPI or FRPI was found (Table 3).
Table 3 The mean, 95% confidence interval (CI) and p-value for the index of sexual isolation (ISI), male relative performance index (MRPI), female relative performance index (FRPI), relative sterility index (RSI), and indices for intraspecific mating compatibility in mating choice tests using individuals of five populations of Thaumatotibia leucotreta (False Codling Moth, FCM).
The ratios of E8–12:Ac to Z8–12:Ac did not significantly differ between the five studied populations (F4,20 = 0.341, P = 0.847; Figure 4). The ratio of E8–12:Ac to Z8–12:Ac ranged from 3.8:1 to 4.2:1 across the five populations (Table 4).
Figure 4 Amount (%) of (E)-8-dodecenyl acetate (E8–12:Ac) relative to (Z)-8-dodecenyl acetate (Z8–12:Ac) in extracts from abdominal tips of Thaumatotibia leucotreta (False Codling Moth, FCM) females from five different populations in South Africa. The ratio of the two isomers was not significantly different between the populations.
Table 4 Ratio of (E)-8-dodecenyl acetate (E8-12:Ac) to (Z)-8-dodecenyl acetate (Z8-12:Ac) for each FCM population.
This study investigated genetic differentiation and mating compatibility in five geographically isolated populations of the False Codling Moth (FCM) Thaumatotibia leucotreta in South Africa. The obtained data showed genetic differentiation between these populations whereby three genetically distinct groups with high genetic distances between each other were identified, i.e., Nelspruit, Marble Hall, and a group formed by Old Colony, Citrusdal and Addo (Tables 1, 2). These genetic differences were reflected in the mating choices made during pairwise choice tests where individuals from genetically distant groups were selected against (Table 3). Nonetheless, in the absence of a choice, males did successfully mate with females from any other population. The mating success, measured as the number of transferred spermatophores, was similar in inter- and intra-population mating events (Figure 2); however, males from populations with high genetic diversity participated more actively in mating with females from both their own and different populations compared to males from populations with a low genetic diversity.
Assessing the levels of genetic variation between different populations is a powerful method to identify or confirm gene flow limitations that can lead to reproductive isolation and eventually speciation (Riesch et al., 2017; Taylor and Friesen, 2017; Doellman et al., 2019). With regard to pest insects, geographically isolated populations with restricted gene flow can rapidly diverge in traits targeted by region-wide applied pest management strategies and cause these to become less effective (Riesch et al., 2017; Taylor and Friesen, 2017; Gao et al., 2020). In the case of FCM, the currently available data from SSA indicate divergence, particularly with regard to the composition of the female sex pheromone which is widely used in FCM control tools (Attygalle et al., 1986; Upfold, 2019). Consequently, local changes in mating behaviour are worth testing as these may require adjusting the commonly used sex pheromone-based management strategies on a local basis.
Previous studies with geographically isolated FCM populations in SSA found both high genetic diversity (Timm et al., 2008; 2010) as well as low genetic diversity, regardless of host crops, geographical locations, and country (e.g., Mkiga et al., 2021). However, to date, no study has assessed genetic diversity of, and differentiation between, populations on a smaller geographic scale, i.e., within a single country. This study is the first to focus on a smaller geographic scale, combining data for FCM populations from four South African provinces, encompassing the southern and northern parts of South Africa, including the Eastern Cape, Limpopo, Mpumalanga, and Western Cape provinces, and a population of mixed origin (Old Colony). Remarkably, even at this geographic scale, there were significant differences in genetic diversity with the five studied populations falling into three distinct genetic groups, indicating divergence of FCM within South Africa. Low genetic diversity was found in samples from the Marble Hall and Nelspruit populations, which both formed their own genetic groups (Figure 1). These were separate from the third genetic group, comprising the other three studied populations (Figure 1). High genetic diversity was found in samples from the Addo and Citrusdal populations, while the Old Colony population was intermediate.
Males from populations with a higher recorded genetic diversity were observed to be more competitive (significant MRPI values) and participated in more mating events with females of both their own and other populations, than were males from populations with a low calculated genetic diversity (Tables 1, 3). Although not significant, this observed trend indicates that maintaining high genetic diversity amongst commercially produced cultures used in AW-IPM programmes, in particular the sterile insect technique (SIT), could be crucial to improve the competitiveness of SIT males in the field. Maintaining genetic diversity and avoiding phenotypic, as well as genetic, effects are key challenges faced in the mass rearing of insects (Leftwich et al., 2021). Inbreeding depression and random genetic drift may result in the loss of genetic diversity, which can lead to loss of fitness and reduced field performance (Mackauer, 1976; Willi et al., 2006; Hoffmann et al., 2017). A decrease in vigour (phenotypic effect) often results from overcrowding and poor diet (Reynolds, 2012). Genetic effects and changes in behaviour may arise as a result of a ‘domestication’ effect when long-term laboratory rearing results in selection for traits better adapted to the laboratory rearing conditions, which in turn affects the reproductive or behavioural traits of the insect (Bartlett, 1984; Hoffmann and Ross, 2018). In order to counteract the “evolution” of laboratory strains, the genetic pool of cultured populations should be re-supplied intermittently with field collected individuals from the corresponding wild populations.
The genetic divergence between the five populations identified in this study was partly reflected in the mating choices during mating compatibility assays with males and females of two different populations. While mating was random in assays with population pairs from the same genetic group, mating was selective towards the own population in choice assays with populations from two different genetic groups (Table 3). This indicates that individuals are capable of identifying conspecifics on a population level, which allows us to assume divergence in recognition signals, likely the sex pheromone, and pre-mating reproductive isolation between some of the South African populations. the FRPI values were never significantly different (Table 3) which indicates that males actively choose female mating partners (“male mate choice”, Bonduriansky, 2001) and thereby select on female phenotypic traits – most likely the female sex pheromone. However, in the absence of a choice, males from all the populations would mate with any female and were able to transfer spermatophores to females originating from a different population with similar success as to females from their own population (Figures 2, 3). It was not assessed in this study whether the resulting offspring of inter-population mating events would be viable. However, previous studies did not find significant differences in the number of viable offspring produced in no-choice cross-mating tests. Thus, while pre-mating reproductive isolation seems established at a certain level, post-mating reproductive isolation among South African FCM populations does not (yet) exist, as previously underscored by Mgocheki and Addison (2016).
Many other lepidopteran pests also in South Africa have formed locally adapted populations, due to fragmented host ranges and in part due to limited hosts, such as Grapholita molesta (Busck) (Lepidoptera: Tortricidae), Cydia pomonella (L.) (Lepidoptera: Tortricidae), Thaumatotibia batrachopa (Meyrick) (Lepidoptera: Tortricidae) and Cryptophlebia peltastica (Meyrick) (Lepidoptera: Tortricidae) (Meyrick, 1930; Quilici et al., 1988; Newton, 1998). The genetic differences amongst locally and regionally adapted FCM populations raises apprehensions and questions of whether the efficacy of semiochemical-based technologies can be enhanced through the manufacturing of regionally specific pheromone lures and dispensers.
Studies investigating sexual behaviour in other lepidopteran species found that in cases where males exhibited a significant selection for females of their own population compared to females of a different population, the composition of the sex pheromone was different between both populations (e.g., Tòth et al., 1992; Groot et al., 2008; Duménil et al., 2014). Over time, these differences in the sex pheromone or intraspecific variation may act as a driver for sexual selection and mating incompatibility via reproductive isolation (see De Pasqual et al., 2021 for a detailed review). It has been abundantly clarified that even subtle differences in pheromone composition may influence the degree of attraction within species populations (Campion and Nesbitt, 1981; Newton et al., 1993; Ando et al., 2004; Ando and Yamakawa, 2011). Hence, chemical analyses of sex pheromones in pest insects with a wide distribution range is important to understand locally manifested pre-mating reproductive isolation (Gao et al., 2020).
In fact, there are contradictory data regarding the composition of the female sex pheromone (Read et al., 1968; Persoons et al., 1977; Angélini et al., 1981; Zagatti et al., 1983; Hall et al., 1984; Attygalle et al., 1986) and it is unknown whether these differences are simply a result of different methodologies used or indeed reflect geographic isolation. Attygalle et al. (1986) concluded that the differences were most likely a result of populations originating from different geographic ranges and that sympatric speciation may have occurred due to ecological boundaries, encompassing spatial structure and functions, and temporal dynamics.
Earlier attempts to investigate intraspecific sex pheromone variation between geographically isolated FCM populations in South Africa using sample enrichment probes (Burger et al., 2017) were unsuccessful (Upfold, 2019). Here a solvent extraction method established by Attygalle et al. (1986), and recently validated by researchers in Israel (Levi-Zada et al., 2020), was used to assess differences in the ratios of the main pheromone components E8–12:Ac and Z8–12:Ac. These isomers are the main attractant for males and have been shown to play a key role in FCM mating behaviour. However, there is controversy in the precise ratio of the two isomers published in earlier studies (Zagatti et al., 1983; Attygalle et al., 1986; Levi-Zada et al., 2020). We have determined a E:Z-ratio of c. 4:1 and found this to be consistent across all five studied populations (Table 4). The absence of variation in the key attractive components E8–12:Ac and Z8–12:Ac could explain the general mating compatibility between all five studied populations when mating partners from the own population are not available. This finding provides important empirical evidence that South African FCM populations have not diverged in the key sexual signal. However, the observed trends for intra-population mating preferences and “male mate choice” (MRPI values) in choice-tests between the five studied FCM populations suggests some divergence in the female sex pheromone composition. It is thus very likely that other minor components of the sex pheromone (not explored in this study) vary between the populations and that such variation accounts for intra-population recognition and mating preference, which over time could lead to pre-mating reproductive isolation between geographically isolated FCM populations.
The raw data supporting the conclusions of this article will be made available by the authors, without undue reservation.
The animal study was approved by Animal Research Ethics Committee at Rhodes University. The study was conducted in accordance with the local legislation and institutional requirements.
PA: Conceptualization, Data curation, Formal analysis, Investigation, Methodology, Project administration, Resources, Software, Validation, Visualization, Writing – original draft, Writing – review & editing. AH: Data curation, Formal analysis, Investigation, Methodology, Project administration, Software, Validation, Visualization, Writing – review & editing. JU: Investigation, Writing – review & editing. AS: Formal analysis, Funding acquisition, Methodology, Project administration, Resources, Software, Supervision, Validation, Visualization, Writing – review & editing. SM: Conceptualization, Funding acquisition, Project administration, Resources, Supervision, Validation, Visualization, Writing – review & editing. MH: Conceptualization, Funding acquisition, Project administration, Resources, Supervision, Validation, Visualization, Writing – review & editing. CC: Conceptualization, Funding acquisition, Methodology, Project administration, Resources, Supervision, Validation, Visualization, Writing – review & editing.
The author(s) declare financial support was received for the research, authorship, and/or publication of this article. We gratefully acknowledge Citrus Research International (CRI) and the Citrus Growers’ Association (CGA) of Southern Africa for funding the postdoctoral fellowship of PA-A through the Centre for Biological Control, Rhodes University, South Africa; and Citrus Research International (CRI) for funding the postdoctoral fellowship of AH. PA-A was also supported by a Rhodes University Research Committee (RC) grant, awarded in 2021.The South African Research Chairs Initiative, of the Department of Science and Innovation and the National Research Foundation of South Africa provided further funding for the work.
We would also like to thank Professor Steve Johnson (University of KwaZulu-Natal) for access to the analytical equipment used in the pheromone analyses.
The authors declare that the research was conducted in the absence of any commercial or financial relationships that could be construed as a potential conflict of interest.
All claims expressed in this article are solely those of the authors and do not necessarily represent those of their affiliated organizations, or those of the publisher, the editors and the reviewers. Any product that may be evaluated in this article, or claim that may be made by its manufacturer, is not guaranteed or endorsed by the publisher.
Any opinion, finding, conclusion, or recommendation expressed in this material is that of the authors, and the National Research Foundation does not accept any liability in this regard.
The Supplementary Material for this article can be found online at: https://www.frontiersin.org/articles/10.3389/fevo.2024.1360395/full#supplementary-material
Adom M., Fening K. O., Billah M. K., Wilson D. D., Hevi W., Clottey V. A., et al. (2021). Pest status, bio-ecology and management of the false codling moth, Thaumatotibia leucotreta (Meyrick) (Lepidoptera: Tortricidae) and its implication for international trade. Bull. Entomol. Res. 111, 17–30. doi: 10.1017/S0007485320000358
Aigbedion-Atalor P., Upfold J., Coombes C., Moore S., Hill M. (2024). Sexual selection in Thaumatotibia leucotreta (Meyrick) is shaped by contrasting geographic adaptations, but does it matter for area-wide sex pheromone control tools? Ann. Appl. Biol. doi: 10.1111/aab.12898
Ando T., Inomata S. I., Yamamoto M. (2004). “Lepidopteran sex pheromones. The chemistry of pheromones and other semiochemicals I,” in Topics in Current Chemistry (Springer, Heidelberg, Berlin), 51–96.
Ando T., Yamakawa R. (2011). Analyses of lepidopteran sex pheromones by mass spectrometry. TrAC Trends Anal. Chem. 30, 990–1002. doi: 10.1016/j.trac.2011.03.010
Angélini A., Descoins L., Lhoste J., Trijau J. P., Zogatti P. (1981). Essai de nouvelles formulations d’attractifs de synthèse pour le piégeage sexuel de Cryptophlebia leucotreta Meyr.(Lepidoptera). Coton Fibres Tropicales 36, 259–264.
Araújo M. F., Castanheira E. M., Sousa S. F. (2023). The buzz on insecticides: a review of uses, molecular structures, targets, adverse effects, and alternatives. Molecules 28, 3641. doi: 10.3390/molecules28083641
Arp H. P. H., Aurich D., Schymanski E. L., Sims K., Hale S. E. (2023). Avoiding the next silent spring: our chemical past, present, and future. Environ. Sci. Technol. 57, 6355–6359. doi: 10.1021/acs.est.3c01735
Attygalle A. B., Schwarz J., Vostrowsky O. (1986). Individual variation in the sex pheromone components of the false codling moth, Cryptophlebia leucotreta (Lepidoptera: Tortriciade). Z. für Naturforschung C 41, 1077–1081. doi: 10.1515/znc-1986-11-1222
Bartlett A. C. (1984). “Genetic changes during insect domestication,” in Advances and Challenges in Insect Rearing. Eds. King E. G., Leppla N. C. (USDA/ARS, New Orleans, USA), 2–8.
Bengtsson J., Fagerström T., Rydin H. (1994). Competition and coexistence in plant communities. Trends Ecol. Evol. 9, 246–250. doi: 10.1016/0169-5347(94)90289-5
Bonduriansky R. (2001). The evolution of male mate choice in insects: a synthesis of ideas and evidence. Biol. Rev. 76, 305–339. doi: 10.1017/S1464793101005693
Burger B. V., de Klerk C. A., Morr M., Burger W. J. (2017). Identification, synthesis, and field tests of the sex pheromone of Margarodes prieskaensis (Jakubski). J. Chem. Ecol. 43, 94–105. doi: 10.1007/s10886-016-0801-0
Butenandt V. A. (1959). Über den sexsual-lockstoff des seidenspinners Bombyx mori. Reindarstellung und Konstitutionsermittlung. Z. Naturforschg 14b, 283–884.
Campion D. G., Nesbitt B. F. (1981). Lepidopteran sex pheromones and pest management in developing countries. Int. J. Pest Manage. 27, 53–61. doi: 10.1080/09670878109414172
Casimero V., Nakasuji F., Fujisaki K. (2001). The influences of larval and adult food quality on the calling rate and pre-calling period of females of the cotton bollworm, Helicoverpa armigera Hübner (Lepidoptera: Noctuidae). Appl. Entomol. Zool. 36, 33–40. doi: 10.1303/aez.2001.33
Cayol J. P., Vilardi J., Rial E., Vera M. T. (1999). New indices and method to measure the sexual compatibility and mating performance of Ceratitis capitata (Diptera: Tephritidae) laboratory-reared strains under field cage conditions. J. Econ. Entomol. 92, 140–145. doi: 10.1093/jee/92.1.140
CGA (2021) Key Industry Statistics (Hillcrest, South Africa: CGA). Available online at: https://citrusresourcewarehouse.co.za/home/document-home/internal-citrus-industry-organisationpublications/annual-reports/cga-annual-reports/7440-cga-annual-report-2020/file (Accessed 21st October 2021).
Conner W. E., Webster R. P., Itagaki H. (1985). Calling behaviour in arctiid moths: the effects of temperature and wind speed on the rhythmic exposure of the sex attractant gland. J. Insect Physiol. 31, 815–820. doi: 10.1016/0022-1910(85)90074-5
Daiber C. C. (1979). A study of the biology of the false codling moth [Cryptophlebia leucotreta (Meyr.)]: The cocoon. Phytophylactica 11, 151–157.
Davis F. M. (1973). Calco oil red N-1700® for marking adults and eggs of the southwestern corn borer. Ann. Entomol. Soc. America 66, 1167–1168. doi: 10.1093/aesa/66.5.1167
Delisle J., McNeil J. N. (1986). The effect of photoperiod on the calling behaviour of virgin females of the true armyworm, Pseudaletia unipuncta (Haw.) (Lepidoptera: Noctuidae). J. Insect Physiol. 32, 199–206. doi: 10.1016/0022-1910(86)90059-4
Delisle J., McNeil J. N. (1987). Calling behaviour and pheromone titre of the true armyworm Pseudaletia unipuncta (Haw.) (Lepidoptera: Noctuidae) under different temperature and photoperiodic conditions. J. Insect Physiol. 33, 315–324. doi: 10.1016/0022-1910(87)90119-3
De Pasqual C., Groot A. T., Mappes J., Burdfield-Steel E. (2021). Evolutionary importance of intraspecific variation in sex pheromones. Trends Ecol. Evol. 36, 848–859. doi: 10.1016/j.tree.2021.05.005
Doellman M. M., Schuler H., Jean Saint G., Hood G. R., Egan S. P., Powell T. H., et al. (2019). Geographic and ecological dimensions of host plant-associated genetic differentiation and speciation in the Rhagoletis cingulata (Diptera: Tephritidae) sibling species group. Insects 10, 275. doi: 10.3390/insects10090275
Duménil C., Judd G. J., Bosch D., Baldessari M., Gemeno C., Groot A. T. (2014). Intraspecific variation in female sex pheromone of the codling moth Cydia pomonella. Insects 5, 705–721. doi: 10.3390/insects5040705
FAO/IAEA/USDA (Food and Agriculture Organization of the United Nations/International Atomic Energy Agency/United States Department of Agriculture (2003). Manual for product quality control and shipping procedures for sterile mass-reared Tephritid fruit flies (Vienna, Austria: IAEA).
Foster S. P., Harris M. O. (1997). Behavioral manipulation methods for insect pest-management. Annu. Rev. Entomol. 42, 123–146. doi: 10.1146/annurev.ento.42.1.123
Fu K., Guo Z., Zhang X., Fan Y., Wu W., Li D., et al. (2016). Insight into the genetic variability analysis and cultivar identification of tall fescue by using SSR markers. Hereditas 153, 1–9. doi: 10.1186/s41065-016-0013-1
Gao K., Torres-Vila L. M., Zalucki M. P., Li Y., Griepink F., Heckel D. G., et al. (2020). Geographic variation in sexual communication in the cotton bollworm, Helicoverpa armigera. Pest Manage. Sci. 76, 3596–3605. doi: 10.1002/ps.5893
Groot A. T., Marr M., Schöfl G., Lorenz S., Svatos A., Heckel D. G. (2008). Host strain specific sex pheromone variation in Spodoptera frugiperda. Front. Zool. 5, 1–13. doi: 10.1186/1742-9994-5-20
Hall D. R., Beevor P. S., Cork A., Nesbitt B. F., La Croix E. A. (1984). (Z)-8-dodecenyl acetate: the major component of the female sex pheromone of Cryptophlebia batrachopa, a Tortricid pest of macadamia in Malawi. Entomol. Experimentalis Applicata 35, 33–36. doi: 10.1111/j.1570-7458.1984.tb03355.x
Hattingh V., Moore S., Kirkman W., Goddard M., Thackeray S., Peyper M., et al. (2020). An improved systems approach as a phytosanitary measure for Thaumatotibia leucotreta (Lepidoptera: Tortricidae) in export citrus fruit from South Africa. J. Econ. Entomol. 113, 700–711. doi: 10.1093/jee/toz336
Hoffmann A. A., Ross P. A. (2018). Rates and patterns of laboratory adaptation in (mostly) insects. J. Econ. Entomol. 111, 501–509. doi: 10.1093/jee/toy024
Hoffmann A. A., Sgrò C. M., Kristensen T. N. (2017). Revisiting adaptive potential, population size, and conservation. Trends Ecol. Evol. 32, 506–517. doi: 10.1016/j.tree.2017.03.012
Hofmeyr J. H., Carpenter J. E., Bloem S. (2005). Developing the sterile insect technique for Cryptophlebia leucotreta (Lepidoptera: Tortricidae): influence of radiation dose and release ratio on fruit damage and population growth in field cages. J. Econ. Entomol. 98, 1924–1929. doi: 10.1093/jee/98.6.1924
Hofmeyr J. H., Pretorius J. (2010). Development of a device to collect mass-reared false codling moth, Thaumatotibia leucotreta (Meyrick) (Lepidoptera: Tortricidae), in a commercial insectary. Afr. Entomol. 18, 374–378. doi: 10.4001/003.018.0204
Hunt G. J. (1997). “Insect DNA extraction protocol,” in Fingerprinting methods based on arbitrarily primed PCR. (Berlin, Heidelberg: Springer Berlin Heidelberg), 21–24. doi: 10.1007/978-3-642-60441-6
Joubert F. D. (2018). Assessment of pheromone specificity in Thaumatotibia leucotreta (Meyrick) populations with focus on pest monitoring and the regional rollout of the sterile insect technique in citrus. [MSc. Thesis]. (Makhanda, South Africa: Rhodes University), 103 pp.
Kamimura M., Tatsuki S. (1994). Effects of photoperiodic changes on calling behavior and pheromone production in the oriental tobacco budworm moth, Helicoverpa assulta (Lepidoptera: Noctuidae). J. Insect Physiol. 40, 731–734. doi: 10.1016/0022-1910(94)90101-5
Kosman E., Leonard K. J. (2007). Conceptual analysis of methods applied to assessment of diversity within and distance between populations with asexual or mixed mode of reproduction. New Phytol. 174, 683–696. doi: 10.1111/j.1469-8137.2007.02031.x
Landolt P. J., Phillips T. W. (1997). Host plant influences on sex pheromone behavior of phytophagous insects. Annu. Rev. Entomol. 42, 371–391. doi: 10.1146/annurev.ento.42.1.371
Leftwich P. T., Spurgin L. G., Harvey-Samuel T., Thomas C. J., Paladino L. C., Edgington M. P., et al. (2021). Genetic pest management and the background genetics of release strains. Philos. Trans. R. Soc. B. 376(1818), 20190805. doi: 10.1098/rstb.2019.0805
Levi-Zada A., Fefer D., Madar R., Steiner S., Kaspi R. (2020). Evaluation of pheromone of false codling moth Thaumatotibia leucotreta in Israel by sequential SPME/GCMS analysis and field trials. J. Pest Sci. 93, 519–529. doi: 10.1007/s10340-019-01138-0
Mackauer M. (1976). Genetic problems in the production of biological control agents. Annu. Rev. Entomol. 21, 369–385. doi: 10.1146/annurev.en.21.010176.002101
McElduff F., Cortina-Borja M., Chan S. K., Wade A. (2010). When t-tests or Wilcoxon-Mann-Whitney tests won’t do. Adv. Physiol. Educ. 34, 128–133. doi: 10.1152/advan.00017.2010
McElfresh J. S., Millar J. G. (1999). Geographic variation in sex pheromone blend of Hemileuca electra from Southern California. J. Chem. Ecol. 25, 2505–2525. doi: 10.1023/A:1020826208715
Meyrick E. (1930). Microlepidoptera of Mauritius. Trans. Entomol. Soc. London 78, 309–323. doi: 10.1111/j.1365-2311.1930.tb00389.x
Mgocheki N., Addison P. (2016). Investigating the validity of the species status of the false codling moth in South African deciduous fruit orchards using mating studies and mtDNA. Bull. Entomol. Res. 106, 598–605. doi: 10.1017/S0007485316000304
Miller J. R., Gut L. J. (2015). Mating disruption for the 21st century: matching technology with mechanism. Environ. Entomol. 44(3), 427–453. doi: 10.1093/ee/nvv052
Mkiga A. M., Mohamed S. A., du Plessis H., Khamis F. M., Ekesi S. (2021). Bio-ecology of false codling moth, Thaumatotibia leucotreta (Meyrick) (Lepidoptera: Tortricidae) within citrus orchards in Kenya and Tanzania. Agric. For. Entomol. 23, 13–22. doi: 10.1111/afe.12398
Moore S. D. (2002). The development and evaluation of Cryptophlebia leucotreta granulovirus (CrleGV) as a biological control agent for the management of false codling moth, Cryptophlebia leucotreta, on citrus. (Doctoral dissertation, Rhodes University).
Moore S. D. (2021). Biological control of a phytosanitary pest (Thaumatotibia leucotreta): A case study. Int. J. Environ. Res. Public Health 18, 1198. doi: 10.3390/ijerph18031198
Moore S., Hattingh V. (2012). A review of current pre-harvest control options for false codling moth in citrus in southern Africa. SA Fruit J. 11, 82–85.
Moore S. D., Richards G. I., Chambers C., Hendry D. (2014). An improved larval diet for commercial mass rearing of the false codling moth, Thaumatotibia leucotreta (Meyrick) (Lepidoptera: Tortricidae). Afr. Entomol. 22, 216–219. doi: 10.4001/003.022.0125
Navarro-Roldán M. A., Gemeno C. (2017). Sublethal effects of neonicotinoid insecticide on calling behavior and pheromone production of tortricid moths. J. Chem. Ecol. 43, 881–890. doi: 10.1007/s10886-017-0883-3
Nei M. (1978). Estimation of average heterozygosity and genetic distance from a small number of individuals. Genetics 89, 583–590. doi: 10.1093/genetics/89.3.583
Newton P. J. (1998). “False codling moth Cryptophlebia leucotreta (Meyrick),” in Citrus Pests in the Republic of SOUTH AFRICA. Eds. Bedford E. C. G., van den Berg M. A., de Villiers E. A. (Dynamic Ad, Nelspruit, South Africa), 192–200.
Newton P. J., Thomas C. D., Mastro V. C., Schwalbe C. P. (1993). Improved two-component blend of the synthetic female sex pheromone of Cryptophlebia leucotreta, and identification of an attractant for C. peltastica. Entomol. Experimentalis Applicata 66, 75–82. doi: 10.1111/j.1570-7458.1993.tb00694.x
Noldus L. P. J. J., Potting R. P. J. (1990). Calling behaviour of Mamestra brassicae: effect of age and photoperiod. Entomol. Experimentalis Applicata 56, 23–30. doi: 10.1111/j.1570-7458.1990.tb01378.x
Opoku-Debrah J. K., Hill M. P., Knox C., Moore S. D. (2014). Comparison of the biology of geographically distinct populations of the citrus pest, Thaumatotibia leucotreta (Meyrick) (Lepidoptera: Tortricidae), in South Africa. Afr. Entomol. 22, 530–537. doi: 10.4001/003.022.0317
Paun O., Schönswetter P. (2012). Amplified fragment length polymorphism: an invaluable fingerprinting technique for genomic, transcriptomic, and epigenetic studies. Plant DNA Fingerprinting Barcoding: Methods Protoc., 75–87. doi: 10.1007/978-1-61779-609-8_7
Persoons C. J., Ritter F. J., Nooyen W. J. (1977). Sex pheromone of the false codling moth Cryptophlebia leucotreta (Lepidoptera: Tortricidae). J. Chem. Ecol. 3, 717–722. doi: 10.1007/BF00988070
Quilici S., Verbizier B., Trahais B., Manikom R. (1988). Note sur les ravageurs du litchi à la Réunion. Fruits 43, 459–464.
R Core Team. (2022). R: A language and environment for statistical computing. R Foundation for Statistical Computing.
Read J. S., Warren F. L., Hewitt P. H. (1968). Identification of the sex pheromone of the false codling moth (Argyroploce leucotreta). Chem. Commun. (London) 14), 792–793. doi: 10.1039/c19680000792
Regnier F. E., Law J. H. (1968). Insect pheromones. J. Lipid Res. 9, 541–551. doi: 10.1016/S0022-2275(20)42699-9
Reynolds M. P. (2012). “Introduction,” in Physiological Breeding I: Interdisciplinary Approaches to Improve Crop Adaptation. Eds. Reynolds M. P., Pask A. J. D., Mullan D. (CIMMYT, Mexico: D.F), pp.692 2–4.
Riesch R., Muschick M., Lindtke D., Villoutreix R., Comeault A. A., Farkas T. E., et al. (2017). Transitions between phases of genomic differentiation during stick-insect speciation. Nat. Ecol. Evol. 1, 0082. doi: 10.1038/s41559-017-0082
Rodriguez-Saona C. R., Stelinski L. L. (2009). “Behavior-Modifying Strategies in IPM: Theory and Practice,” in Integrated pest management: innovation-development process, eds. Peshin R., Dhawan A. K.. (Dordrecht: Springer). doi: 10.1007/978-1-4020-8992-3_11
Royer L., McNeil J. N. (1991). Changes in calling behaviour and mating success in the European corn borer (Ostrinia nubilalis), caused by relative humidity. Entomol. Experimentalis Applicata 61, 131–138. doi: 10.1111/j.1570-7458.1991.tb02405.x
Sadek M. M., von Wowern G., Löfstedt C., Rosén W. Q., Anderson P. (2012). Modulation of the temporal pattern of calling behavior of female Spodoptera littoralis by exposure to sex pheromone. J. Insect Physiol. 58, 61–66. doi: 10.1016/j.jinsphys.2011.09.016
Shen L. Z., Chen P. Z., Xu Z. H., Deng J. Y., Harris M. K., Wanna R., et al. (2013). Effect of larvae treated with mixed biopesticide Bacillus thuringiensis-abamectin on sex pheromone communication system in cotton bollworm, Helicoverpa armigera. PloS One 8, e68756. doi: 10.1371/journal.pone.0068756
Peakall R., Smouse P. E. (2012). GenAlEx 6.5: genetic analysis in Excel. Population genetic software for teaching and research—an update. Bioinformatics 28, 2537–2539. doi: 10.1093/bioinformatics/bts460
Stern V. M. R. F., Smith R., van den Bosch R., Hagen K. (1959). The integration of chemical and biological control of the spotted alfalfa aphid: the integrated control concept. Hilgardia 29, 81–101. doi: 10.3733/hilg.v29n02p081
Steurer M., Ruther J., Pokorny T. (2024). Behavioural consequences of intraspecific variability in a mate recognition signal. Proc. R. Soc. B 291, 20232518. doi: 10.1098/rspb.2023.2518
Swier S. R., Rings R. W., Musick G. J. (1977). Age-related calling behavior of the black cutworm, Agrotis ipsilon. Ann. Entomol. Soc. America 70, 919–924. doi: 10.1093/aesa/70.6.919
Taret G., Sevilla M., Wornoayporn V., Islam A., Ahmad S., Caceres C., et al. (2010). Mating compatibility among populations of codling moth Cydia pomonella Linnaeus (Lepidoptera: Tortricidae) from different geographic origins. J. Appl. Entomol. 134, 207–215. doi: 10.1111/j.1439-0418.2008.01375.x
Taylor R. S., Friesen V. L. (2017). The role of allochrony in speciation. Mol. Ecol. 26, 3330–3342. doi: 10.1111/mec.14126
Tejeda M. T., Arredondo-Gordillo J., Orozco-Dávila D., Quintero-Fong L., Díaz-Fleischer F. (2017). Directional selection to improve the sterile insect technique: survival and sexual performance of desiccation resistant Anastrepha ludens strains. Evol. Appl. 10, 1020–1030. doi: 10.1111/eva.12506
Timm A. E., Geertsema H., Warnich L. (2010). Population genetic structure of economically important Tortricidae (Lepidoptera) in South Africa: a comparative analysis. Bull. Entomol. Res. 100, 421–431. doi: 10.1017/S0007485309990435
Timm A. E., Warnich L., Geertsema H. (2008). Morphological and molecular identification of economically important Tortricidae (Lepidoptera) on deciduous fruit tree crops in South Africa. Afr. Entomol. 16, 209–219. doi: 10.4001/1021-3589-16.2.209
Tòth M., Löfstedt C., Blair B. W., Cabello T., Farag A. I., Hansson B. S., et al. (1992). Attraction of male turnip moths Agrotis segetum (Lepidoptera: Noctuidae) to sex pheromone components and their mixtures at 11 sites in Europe, Asia, and Africa. J. Chem. Ecol. 18, 1337–1347. doi: 10.1007/BF00994360
Upfold J. K. (2019). Sexual attraction and mating compatibility between Thaumatotibia leucotreta populations and implications for semiochemical dependent technologies [MSc. Thesis]. (Makhanda, South Africa: Rhodes University), 106 pp.
Venette R. C., Davis E. E., DaCosta M., Heisler H., Larson M. (2003). Mini risk assessment: false codling moth, Thaumatotibia (= Cryptophlebia) leucotreta (Meyrick) [Lepidoptera: Tortricidae] (St. Paul, MN: University of Minnesota, Department of Entomology, CAPS PRA), pp.1–pp30.
Webster R. P., Cardé R. T. (1982). Relationships among pheromone titre, calling and age in the omnivorous leafroller moth (Platynota stultana). J. Insect Physiol. 28, 925–933. doi: 10.1016/0022-1910(82)90108-1
Willi Y., Van Buskirk J., Hoffmann A. A. (2006). Limits to the adaptive potential of small populations. Annu. Rev. Ecol. Evol. Syst. 37, 433–458. doi: 10.1146/annurev.ecolsys.37.091305.110145
Witzgall P., Kirsch P., Cork A. (2010). Sex pheromones and their impact on pest management. J. Chem. Ecol. 36, 80–100. doi: 10.1007/s10886-009-9737-y
Wyatt T. D. (2003). Pheromones and animal behaviour Vol. Vol. 626 (Cambridge: Cambridge University Press). doi: 10.1017/CBO9780511615061
Xavier L. M. S., Magalhães D. M., Viana P. A., Blassioli-Moraes M. C., Borges M., Barrigossi J. A. F., et al. (2018). Age influence on sexual behavior of the lesser cornstalk borer, Elasmopalpus lignosellus (Zeller)(Lepidoptera: Pyralidae). Neotropical Entomol. 47, 205–210. doi: 10.1007/s13744-017-0527-x
Yeh F. C., Yang R. C., Boyle T. (1999). POPGENE. Microsoft Windows Based Freeware for Population Genetic Analysis. Release 1.31. (Edmonton: University of Alberta).
Keywords: (E/Z)-8-dodecyl acetate, geographic isolation, insect sex pheromone, intraspecific variation, mating choice, phytosanitary pest, reproductive behaviour
Citation: Aigbedion-Atalor P, Heiduk A, Upfold J, Shuttleworth A, Moore S, Hill M and Coombes C (2024) Geographic variation in genetic composition, sexual communication and mating compatibility of the False Codling Moth, Thaumatotibia leucotreta for optimisation of area-wide control. Front. Ecol. Evol. 12:1360395. doi: 10.3389/fevo.2024.1360395
Received: 22 December 2023; Accepted: 02 May 2024;
Published: 23 May 2024.
Edited by:
Ingo Schlupp, University of Oklahoma, United StatesReviewed by:
Björn Bohman, Swedish University of Agricultural Sciences, SwedenCopyright © 2024 Aigbedion-Atalor, Heiduk, Upfold, Shuttleworth, Moore, Hill and Coombes. This is an open-access article distributed under the terms of the Creative Commons Attribution License (CC BY). The use, distribution or reproduction in other forums is permitted, provided the original author(s) and the copyright owner(s) are credited and that the original publication in this journal is cited, in accordance with accepted academic practice. No use, distribution or reproduction is permitted which does not comply with these terms.
*Correspondence: Pascal Aigbedion-Atalor, cGFzY2FsYXRhbG9yMUB5YWhvby5jb20=
Disclaimer: All claims expressed in this article are solely those of the authors and do not necessarily represent those of their affiliated organizations, or those of the publisher, the editors and the reviewers. Any product that may be evaluated in this article or claim that may be made by its manufacturer is not guaranteed or endorsed by the publisher.
Research integrity at Frontiers
Learn more about the work of our research integrity team to safeguard the quality of each article we publish.