- 1Department of Integrative Marine Ecology, Stazione Zoologica Anton Dohrn, Villa Comunale, Napoli, Italy
- 2NBFC, National Biodiversity Future Center, Palermo, Italy
- 3Department of Veterinary Medicine and Animal Production, University of Naples Federico II, Naples, Italy
- 4Department of Ecosustainable Marine Biotechnology, Stazione Zoologica Anton Dohrn, Calabria Marine Centre, Amendolara, Italy
- 5Department of Integrative Marine Ecology, Stazione Zoologica Anton Dohrn, Calabria Marine Centre, Amendolara, Italy
- 6Department of Biology and Evolution of Marine Organisms, Sicily Marine Centre, Stazione Zoologica Anton Dohrn, Milazzo, Italy
- 7National Institute for Environmental Protection and Research, Milazzo, Italy
- 8Department of Life Sciences, University of Trieste, Trieste, Italy
This manuscript explores the role of European anchovies (Engraulis encrasicolus) in the central Mediterranean Region, shedding light on their ecological significance, conservation challenges, and sustainable utilization. The European anchovy is one of Europe’s most important fish resources in the Mediterranean basin, and it is considered a keystone species, playing a pivotal role in both ecological and socio-economic dimensions. However, in recent decades, European anchovy, together with Sardina pilchardus (commonly known as European sardine), has suffered a population decline for several reasons. Consequently, it is necessary to improve the management of anchovy fisheries by understanding the reproductive modes and characteristics, the influence of currents on the passive transport of eggs and larvae, the feeding habits, the environmental adaptability (e.g., salinity), and the distribution of ecotypes along the Italian coasts. Such information is fundamental for the management of fisheries, especially artisanal ones, and to control frauds, especially in protected, geographically referred, and traditional high-quality commercial products. Various aspects, ranging from their population dynamics to their industrial processing and the ecological implications of these activities were delved, highlighting the knowledge about anchovy populations and ecotypes and its importance in maintaining ecosystem balance and sustaining human communities. The ecological interaction of anchovies within the food web, as essential data in the conservation actions and management of these resources was emphasized. In addition, the metabolic and stomach contents diversity among anchovy populations and ecotypes was discussed, enhancing our understanding of their adaptability to varying environmental conditions. The manuscript then explores the traditional and industrial processing of anchovies, encompassing aspects ranging from fishing techniques (i.e., methods of capture) to their industrial significance, sustainability concerns, issues of fraud, and the establishment of geographical traceability. Finally, the opportunities for sustainable and biotechnological utilization of anchovy discards were also further explored, demonstrating the potential for waste reduction and resource optimization.
1 Anchovies: ecological significance and challenges in marine ecosystems
Anchovy species are abundant in most of the world’s marine ecosystems and are an important food source for other marine organisms (Tudela and Palomera, 1997; Gibson and Atkinson, 2016). Most species are marine, although many tolerate low salinity during one or more stages of their life cycle (Castillo et al., 2019; Liu et al., 2023). In addition, some species are adapted to freshwater environments, as in the case of several American and Asian species (Bloom and Lovejoy, 2017; Cheng et al., 2019). Marine species usually inhabit the continental shelf and adjacent deep-water areas and several of them show a seasonal vertical migration (Giannoulaki et al., 2013). Anchovies have both top-down and bottom-up effects on the ecosystem (Saraux et al., 2019), and mainly feed on zooplankton and, partially, on phytoplankton (Costalago and Palomera, 2014; Awad et al., 2022) although are considered indiscriminate feeders (Koslow, 1981; James, 1987; Borme et al., 2009). Stomach samples from adults show zooplankton, eggs, and small fish as primary prey items, and even some cannibalism activity, whereas larvae primarily consume copepods (James, 1987; Borme et al., 2009; Awad et al., 2022). They contribute to regulate plankton populations, thus influencing the entire trophic pyramid. A clear relationship has been demonstrated among anchovy populations, zooplankton, phytoplankton, and remineralization in the water column (James et al., 1989; Attayde and Hansson, 2001; Oguz et al., 2008; Politikos et al., 2015). Anchovy predation pressure on zooplankton populations helps control their numbers, preventing overgrazing on phytoplankton (Wollrab et al., 2012). Additionally, their role as prey for higher trophic levels, such as seabirds (Velarde et al., 2015), marine mammals (Ouled-Cheikh et al., 2022), and larger fish species (Glaser, 2011; Cardona et al., 2015) contributes to the overall biodiversity and stability of the ecosystem (Lavoué et al., 2022). Anchovies belong to the family of Engraulidae, in the order of Clupeiformes, and are divided into two subfamilies: Coilinae and Engraulinae (Lavoué et al., 2013). The subfamily Coilinae inhabits the Indo-Pacific Ocean, while the family Engraulinae is found in both the Indo-Pacific and Atlantic Oceans (Lavoué et al., 2013).
Into the family Engraulidae, which consists of about 17 genera and 141 species, genus Engraulis includes the European anchovy Engraulis encrasicolus (Bloom and Lovejoy, 2012). European anchovy is a small pelagic fish that inhabits the Mediterranean and the Black Seas, as well as several African areas (alongside the Moroccan coasts) and the Northeast Atlantic (FAO, 2020). In the Mediterranean Sea, changes in European anchovy’ population structure have been registered (Saraux et al., 2019; Fernández-Corredor et al., 2021) coupled with a gradual decline in their populations (Van Beveren et al., 2014). Several hypotheses explaining population decline have been formulated, taking into consideration one or more stressors such as overfishing (Klanjšček and Legović, 2007), habitat degradation (Fujita et al., 2021), food availability (Thoral et al., 2021), alteration in plankton community (Bat et al., 2007), pollution (Savoca et al., 2017; Basilone et al., 2018), microplastics (Collard et al., 2017), and climate-change (Checkley et al., 2017), with consequences on anchovy’s abundance and distribution as well as on their behavior, biochemistry, and physiology. Changes in the plankton community affect the diet of this species with consequences on the size of zooplankton species predated (Van Beveren et al., 2014; Queirós et al., 2019; Thoral et al., 2021).
As a case of study, the Black Sea has undergone significant transformations in recent years, leading to notable alterations in both the qualitative and quantitative composition of phytoplankton and zooplankton. These changes have been primarily driven by eutrophication, resulting from an increased influx of nutrients through major rivers over the past few decades (Oguz et al., 2008; Gücü et al., 2017). These changes in the plankton composition are related to interspecific dynamics among pelagic fishes, non-gelatinous and gelatinous plankton (Deibel and Lowen, 2011; Lucas and Dawson, 2013). In fact, in the Black Sea, the presence of non-native species (e.g., the ctenophore Mnemiopsis leidyi) has contributed to the decline of anchovy and other pelagic fish populations in the region (Kideys, 1994). The situation has become more intricate with the introduction of other alien species, such as the ctenophore Beroe ovata able to feed on M. leidyi, creating an ecological feedback system that also impacts other components of the planktonic community (Gücü et al., 2017). In the framework of fisheries management, a multidisciplinary approach is needed for the identification of fish stock units, the presence of subpopulations or sibling species, and the prediction of potential threats (Jemaa et al., 2015; Pita et al., 2016). Such considerations should be integrated from the social and economic point of view, due to the importance of anchovies for regional pelagic fisheries (Ruiz et al., 2017; Falautano et al., 2018; Sartor et al., 2019). In fact, anchovy fishing represents a fundamental resource for many human communities living in coastal areas with economical consequences on industrial and local fisheries in national and international markets. Its nutrient value, and the status of several semi-industrial production as a “protected-food” with characteristics of unicity (FAO, 2018; European Union, 2020) makes its management extremely important in the Mediterranean Basin (Consonni and Cagliani, 2022). This is particularly relevant considering “The Global Deal for Nature” proposal (Dinerstein et al., 2019) that led to the European Union long-term plan (EU’s biodiversity strategy for 2030) called “30x30 action”, with the aim to protect nature, reverse the degradation of ecosystems, address biodiversity loss and promote sustainable development (Baldock and Charveriat, 2018; Hermoso et al., 2022).
In this review, we focused our attention on European anchovies inhabiting the Mediterranean Sea, highlighting the essential role of anchovies in both ecological and human contexts (Figure 1). This manuscript covers a wide range of topics related to European anchovy including its population dynamics, ecological implications, adaptability to varying environmental conditions, metabolic and trophic diversity. The information presented here are fundamental in the framework of anchovy fishery management, taking into consideration stocks and ecotypes, sustainable and responsible fishing practices, artisanal fisheries, fraud issues, and traditional high-quality commercial productions. Regarding such high-quality productions, this manuscript explores the traditional and industrial processing of anchovies, encompassing aspects such as fishing techniques and their industrial significance. This study also explores the potential of anchovy discards for sustainable and biotechnological utilization, which aligns with the principles of sustainability for green and blue economy, by aiming to reduce waste and optimize the use of resources.
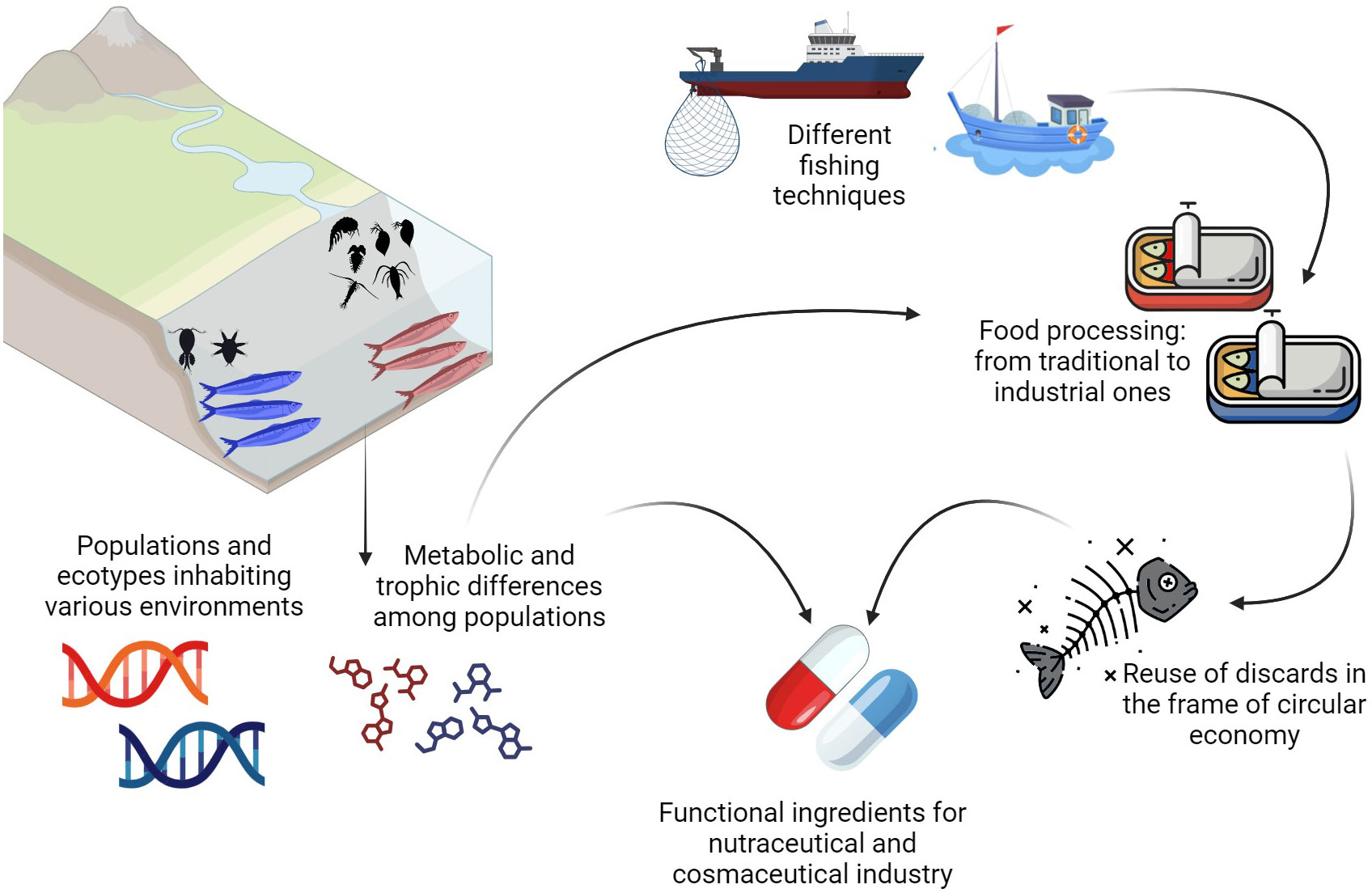
Figure 1 The Mediterranean Sea is characterized by different populations of anchovies inhabiting different environments. Through genetic and metabolomic approaches it is possible to identify such different classes of individuals inside the species. At the same time, anchovies are fished using different techniques and, in addition, are processed using industrial and traditional methods. In this review, we propose the reuse of anchovy discards for both nutraceutical and cosmeceutical purposes.
2 Anchovy populations and ecotypes: ecology and research approaches
2.1 The importance of ecotypic diversity in fishery and conservation science
Fishing and connectivity patterns, as well as morphological, behavioral, trophic, and demographic traits of marine species, are commonly used to designate fish stocks (Cadrin, 2020; Huret et al., 2020). The use of population genetic markers is fundamental to improve our understanding of connectivity patterns among marine populations. It could also help to define populations’ dynamics across spatial and temporal scales, possibly leading to the identification of ecotypes inside species (Casey et al., 2016; Nordahl et al., 2019).
Although there is widespread acknowledgment about the potential populations’ complexity of several marine species, fisheries are frequently managed considering subpopulations into singular management units. The discrepancy between species ecology/genetics and fishing management could result in diminished biodiversity (Benson et al., 2015). When fisheries management fails to account for genetic diversity in natural populations and instead treats them as a single homogeneous unit, it can lead to issues such as overfishing of loss of fixed rare alleles (McKinney et al., 2017), leading to the loss of these unique genetic traits from the overall gene pool (Svedäng et al., 2010). Rare alleles are genetic variants that occur at low frequencies within a population. These alleles may confer specific advantages or adaptations to environmental conditions (Bernatchez, 2016). However, if a subpopulation with such rare alleles is overfished, it can lead to the loss of these unique genetic traits from the overall gene pool (Svedäng et al., 2010). Indeed, safeguarding genetic resources stands as a pivotal component within conservation endeavors, and neglecting spatial dynamics in fish management could result in unforeseen hazards of overexploitation (Ying et al., 2011). The possibility to consider sub-species level taxonomic units (e.g., ecotypes) for conservation efforts has long been under discussion (Phillimore and Owens, 2006; Burbrink et al., 2022). Ecotypes are defined as populations of the same species that have inherited different traits that are closely associated with environmental parameters, integrating ecological inference with genetic variation (Stronen et al., 2022). Ecotypes correct or refine the delineation of management units and its application to fishery and conservation science, linking together the genetic structure of the population, the trophic preferences, and the behavior, to the exploitation of the fish resource (Stronen et al., 2022). This implies to disentangle predictions on the fate of single populations from that one referred to the species in toto, possibly allocating resources to more targeted restoration efforts (Engelhard et al., 2010). Overfishing and habitat degradation can disproportionately affect specific ecotypes, leading to imbalances in the ecosystem (Şahin et al., 2008; Engelhard et al., 2010; Chung et al., 2015).
In the European Union, the exploitation of fish stocks is managed under the Common Fisheries Policy, which ensures that fishing activities are environmentally, economically, and socially sustainable (Casey et al., 2016). European anchovies (Engraulis encrasicolus) stand out as an example of a species displaying remarkable ecotypic diversity (Silva et al., 2014; Rumolo et al., 2016; Catanese et al., 2017). Genetic studies of anchovies in the Mediterranean Sea have revealed distinct populations, suggesting limited gene flow between different regions (Magoulas et al., 2006; Huret et al., 2020). This genetic differentiation has led to variations in size, shape, and coloration which likely reflect physiological adaptations to specific environmental conditions, including temperature, salinity, and food availability (Gordina et al., 1997; Tudela, 1999; Turan et al., 2004) with consequences on growth rates, lifespan, and reproductive strategies (Politikos et al., 2015; Raybaud et al., 2017; Bang et al., 2022). The significance of understanding these biological variations, and eventually the existence of ecotypes, is essential for the conservation and management of these key species.
2.2 Populations and ecotypes and putative species
Phylogeographic investigations indicate that marine fish species tend to exhibit less population structuring compared to their freshwater counterparts. However, numerous marine species have revealed an intraspecific diversity among populations, as demonstrated in holothurians (Holoturia tubulosa) and clams (Venus verrucosa) (Feidantsis et al., 2022) but not in other species, such as European sardine (Sardina pilchardus) (Imsiridou et al., 2021) and cuttlefish (Sepia officinalis) (Feidantsis et al., 2022). It has been demonstrated that a clear population structuring exists in marine species with limited dispersal capabilities (Doherty et al., 1995; Baus et al., 2005; Cowen and Sponaugle, 2009; Truelove et al., 2017; Gaines and Lafferty, 2020) or living under selective conditions (Zardoya et al., 2004; Bargelloni et al., 2005; Swart et al., 2016; Cheng et al., 2018). This is not always true for species with a strong dispersal potential. The variability in allelic frequencies within natural populations over time and space can be shaped by diverse evolutionary processes, including migration, mutation, selection, and genetic drift (Foll and Gaggiotti, 2006; Jacquard, 2012). Ecological conditions lead to regional scales of local adaptation (Gkafas et al., 2019), giving rise to phylogeographic structures in marine species.
More than two decades ago, mitochondrial clade analysis unveiled a general heterogeneity among European anchovy populations (Magoulas et al., 1996, 2006). Admixture of individuals from different spawning sites (Tudela, 1999), and enhanced population genetic structure has been detected with species-specific polymorphic microsatellite loci (SSRs) (Landi et al., 2005; Zarraonaindia et al., 2009; Turan et al., 2017). Such investigations demonstrated the non-panmictic characteristics of anchovy population in bay of Biscay (Zarraonaindia et al., 2009) but not in the Adriatic sea (Turan et al., 2017), suggesting that European anchovy populations at least in some areas are genetically subdivided. An analysis of allozyme data suggested the presence of two distinct taxonomic entities: a coastal group called by Gulf of Lion’ fishermen “white anchovies” and a more pelagic cohort known as “blue anchovy” (Oueslati et al., 2014). Morphological observations also hinted at the existence of a distinct species, identified as Engraulis rissoi, inhabiting several lagoons in Sicily Island (Dulzetto, 1947), and Tunisian areas (Quignard et al., 1973; Messaoud et al., 2011). In the Black Sea, researchers identified two distinct geographical “populations” of anchovy (called Engraulis encrasicolus ponticus and Engraulis encrasicolus meaticus) that have adapted to reproducing in waters with different salinity (Chashchin et al., 2015; Chesalin and Nikolsky, 2023). However, the taxonomic categorization of anchovies living in the Black Sea has been a topic of extended discussion, and the debate continues regarding whether they represent two distinct subspecies, constituting separate stocks, or if they are populations of the same species (Ivanova et al., 2013; Chashchin et al., 2015; Gücü et al., 2017). In the Gulf of Lion, observations raised the potential presence of another species, Engraulis albidus, thriving in coastal lagoons (Borsa et al., 2004). In Moroccan coastal sites, researchers described a clear set of morphological and biological traits distinct from the ones of the European anchovy (Kada et al., 2009).
Beyond the potential identification of new anchovy species in the Mediterranean Sea, investigations based on electrophoretic and morphological variances (Bembo et al., 1996a; Borsa, 2002), nuclear ePIC marker CK6-2 (Borsa et al., 2004; Bouchenak-Khelladi et al., 2008) as well as mtDNA (Bembo et al., 1996b), confirmed the presence of distinct anchovy stocks. This idea was supported by other approaches, such as using nuclear and mitochondrial single nucleotide polymorphisms (SNPs), where two distinct groups were identified. The first one was associated with areas of deep-water upwelling on narrow continental shelves, and the second one with wide continental shelves (Zarraonaindia et al., 2012). SNPs markers technique have been utilized to identify population characteristics of adults, juveniles, and larvae of several species and to evaluate the population origin of several species, as in the case of Atlantic cod (Gadus morhua) (Jorde et al., 2018) and Atlantic bluefin tuna (Thunnus thynnus) (Rodríguez-Ezpeleta et al., 2019). In the frame of the Molecular Ecology Resources Primer Development Consortium, it was developed a SNPs panel composed of 47 nuclear DNA SNPs and 15 mtDNA SNPs of E. encrasicolus samples collected in several areas of the species range (Mediterranean, eastern Atlantic, North Sea, and English Channel, Bay of Biscay, Galicia to southern Africa). This tool allowed the researchers to identify two distinct E. encrasicolus groups, the first mainly present in the Bay of Biscay, the North Sea, and the English Channel, and the second one present in Mediterranean, with the exception of the Alboran Sea, and the Gulf of Cadiz (Zarraonaindia et al., 2012). Shortly afterward, Montes and colleagues identified in 2013 a much larger number of SNPs (around 19,000) by using a Next Generation Sequencing approach combining transcriptome and genome information of E. encrasicolus (Montes et al., 2013). These authors selected a panel of 530 SNPs, which were tested in five populations, four Atlantic and one Mediterranean, using the TaqMan OpenArray technique. This allowed the validation of around 480 markers that could be useful for understanding the genetic structure of the anchovy populations (Montes et al., 2013). Three years later, the same authors proposed the use of a panel of 482 SNPs, 481 from the Montes’ previous study and one from the Molecular Ecology Resources Primer Development Consortium (Zarraonaindia et al., 2012), to study both the genetic structure and adaptation of the Bay of Biscay anchovy population (Montes et al., 2016). SNPs panel was also used in the area from the southern Bay of Biscay to the Irish and North Seas across the English Channel (Huret et al., 2020) confirming the results obtained by the previous study (Montes et al., 2016), but with more robust data as the sampling was broader in space and time. Starting from the panel of SNPs used in a previous study (Montes et al., 2013), Catanese and colleagues selected a subset of 96 highly discriminating SNPs that can be used for genetic studies of European anchovy stocks, demonstrating that the SNPs selected based on Fst (Bhatia et al., 2013) are efficient in capturing much of the genetic variation identified by a larger SNPs panel (Catanese et al., 2016). Through the SNPs analysis of anchovy populations from the Italian seas (Tyrrhenian, Ionian, and Adriatic Seas), two genetic/ecological ecotypes of E. encrasicolus have been identified, namely coastal and offshore (Catanese et al., 2017, 2020). Such ecotypes coexist in several areas occupying partially overlapping niches (Figure 2). Variations in the timing of reproduction and larval growth among distinct ecotypes are notably influenced by environmental factors such as temperature, salinity, and dissolved oxygen (Ruggeri et al., 2016). Transcriptome analysis has further elucidated the presence of adaptive evolutionary mechanisms that drive differential selection between these ecotypes, correlated with fluctuations in salinity, with the coastal ecotype abundant in presence of rivers and higher chlorophyll concentrations (Montes et al., 2016; Catanese et al., 2017). The offshore ecotype, instead, shows enhanced environmental resilience and opportunistic feeding behavior (Catanese et al., 2017). Eleven molecular markers indicated divergent selection between the two ecotypes, with most of these markers being linked to salinity adaptation. Five differently expressed genes were associated with metabolic pathways (TIMM10, eif-2b, annexin A4, ADH, and CHAF1A) which may be important by enhancing the efficiency of food conversion in low-saline waters (Montes et al., 2013). In addition, two differently expressed genes (TSSK6 and basigin) are involved in a critical stage of fertilization process and could be associated with reproductive isolation of the two ecotypes (Montes et al., 2013).
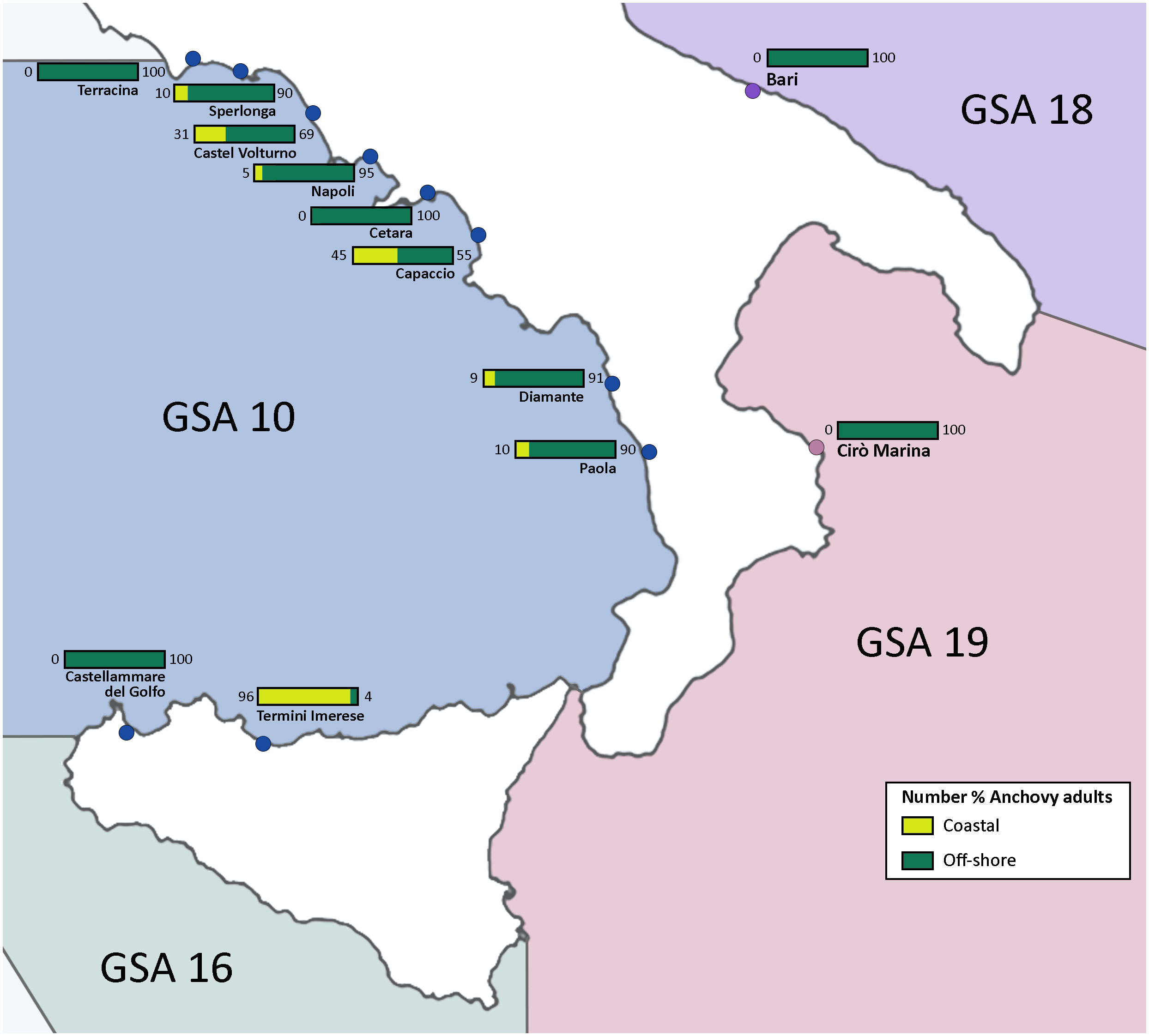
Figure 2 Presence in percentage of coastal (in yellow) and offshore (in green) ecotype in various sampling sites in the Central Mediterranean Area (Catanese et al., 2017).
European anchovy populations exhibit intricate dynamics of egg and larval dispersal, facilitating the transportation and mingling of ecotypes over short and moderate distances (Norcross and Shaw, 1984; Catanese et al., 2020). Such passive transport has consequences on the connectivity of anchovy populations that often consist of individuals from different areas. The presence of individuals belonging to different ecotypes, and presenting distinct ecological attributes and lifestyles poses challenges for effective management actions (Erisman et al., 2017) requiring in several cases a multi-basin integrated approach (Catanese et al., 2020). The differentiated ecotypes, in fact, should be considered as separate fishery stocks, though they coexist within the same population. Phylogenetic studies have concluded that these ecotypes represent a case of partial reproductive isolation, probably due to geographical isolation, which has led to parallel genetic differentiation as effects of quaternary climatic oscillations on evolutionary diversification (Le Moan et al., 2016). Indeed, following the Messinian Salinity Crisis, which devastated the marine fauna of the Mediterranean, the basin was inundated by Atlantic water and biota during the Pliocene around 5.33 million years ago (Roveri et al., 2014; Evangelisti et al., 2017). This fauna underwent significant environmental shifts due to oceanographic and climatic changes associated with the transition from the tropical-subtropical conditions of the Pliocene to the cooler climate of the Pleistocene (Sabelli and Taviani, 2014; Agiadi et al., 2018). The Pleistocene epoch was characterized by numerous glacial-interglacial cycles, leading to substantial alterations in organism distribution (Wilson and Eigenmann Veraguth, 2010), with the Last Glacial Maximum (LGM) occurring approximately between 24 and 16.5 thousand years ago in the Mediterranean Sea (Cacho et al., 2001). The genetic footprint of postglacial recolonization has been identified in numerous marine species with an Atlantic-Mediterranean distribution, such as seabass (Tine et al., 2014) and holothurians (Gkafas et al., 2023). However, the impact of coastal glaciation is not uniform, prompting speculation that species might inherently vary in their capacity to adapt to the environmental shifts linked with glacial cycles (Wilson and Eigenmann Veraguth, 2010). During LGM glacial periods, anchovies found refuge in multiple locations in the eastern Mediterranean seas and, in the Atlantic, in the Gulf of Senegal and Guinea (Le Moan et al., 2016; Evangelisti et al., 2017). In the current interglacial period, an independent secondary contact between anchovy ecotypes in the Atlantic and Mediterranean regions or a post-contact colonization of one or both ecotypes from one region to the other has taken place (Le Moan et al., 2016).
A similar pattern of genetic divergence has been observed in a small intertidal fish, Coryphoblennius galerita, that is characterized by the existence of two clades: one in the Mediterranean and another in the eastern Atlantic (Domingues et al., 2007a). Such differences can be explained by the existence of an effective barrier during LGM in Gibraltar Strait or/and the ‘Almerian-Oran jet’ that prevents gene flow between Mediterranean and Atlantic populations (Duran et al., 2004; Bargelloni et al., 2005; Lemaire et al., 2005). The degree of isolation observed in European anchovy and other marine species cannot be found in other small pelagic fish like European sardine (Imsiridou et al., 2021) and in sparidae such as white seabream (Diplodus sargus) (Domingues et al., 2007b). In fact, research has shown that Greek populations of European sardines share haplotypes with those found worldwide, suggesting a single evolutionary unit for this species and no sign of phylogenetic Atlantic-Mediterranean division (Imsiridou et al., 2021). The same situation has been found in white seabream, with Atlantic and Mediterranean populations showing no signs of genetic differentiation (Domingues et al., 2007b).
2.3 Reproduction and passive transport dynamics
Engraulis encrasicolus experiences demographic fluctuations throughout the year (Ma et al., 2019). Interannual variability of the stock biomass in several small pelagic fish species has been related to environmental forcing drivers (Cingolani et al., 1996; Leitão et al., 2014). Such variability is a characteristic of several R-strategist fish species with short life spans, early age at first reproduction, high number of offspring, and low offspring survival rates, which in turn can heavily affect recruitment success and stock biomass levels (Saraux et al., 2019; Patti et al., 2020). These natural fluctuations are of considerable ecological importance, as these fishes make up a significant proportion of the available biomass in the Mediterranean, supporting both fishing activities and the wider ecosystem (Patti et al., 2020). Preserving the reproductive cycle of anchovies and effectively managing it, while addressing potential disturbances, takes on paramount importance to ensure the viability of productive fishing zones. It is the responsibility of governments to manage these disturbances to ensure the survival of these vital species (Ramírez-Valiente et al., 2021). Anchovies are oviparous species with external fertilization and iteroparous strategy, capable of spawning several times within a reproductive season (Regner, 1996). The spawning season for anchovy populations living in the central Mediterranean Sea covers a period from April to October, with spawning peaks during the warmer months (Basilone et al., 2006). The processes of migration from the coast towards deeper waters and the beginning of the reproductive season are temperature-dependent mechanisms (Alheit et al., 2012). During the fertile period, the anchovies expel ellipsoidal-shaped eggs which float between 30 and 100 meters in depth and hatch within 24-36 hours at a temperature of 21°C (Palomera et al., 1988; Dulčić, 1997). Anchovies show a prolonged breeding season that is considered a key adaptation that contributes to its reproductive success. Anchovies necessitate specific spawning habitats characterized by factors called “fundamental triad” such as nutrient enrichment, concentration of larval food distributions, and local retention of eggs and larvae (Bakun, 1997). Consequently, a nexus between environmental conditions and the reproductive process is imperative (Lasker and Zweifel, 1978). In many cases, spawning areas correspond to coastal zones characterized by coastal upwelling events, which are able to enrich the oligotrophic waters (Patti et al., 2010; Torri et al., 2018). A study conducted in the Strait of Sicily, a study examined a 12-year time series spanning from 2005 to 2016, aiming to investigate the relationships between European anchovy density, growth, reproduction, and habitat dynamics within an upwelling system (Basilone et al., 2020). The mechanisms governing anchovy dispersal, encompassing both passive drifting and active swimming, wield substantial influence over their life history, growth, and survival dynamics (Cuttitta et al., 2016).
Evidence suggests a direct correlation between physical phenomena and the abundance of larval populations (Cuttitta et al., 2015). This implies that conducive conditions for the survival of anchovy larvae exist, with implications for adult stocks (Lafuente et al., 2002, 2005; Cuttitta et al., 2015). It can be expected that the early dispersal of the two European anchovy ecotypes is linked to both their physical characteristics and dietary preferences, which play a crucial role in their survival. On one hand, passive dispersal from various spawning grounds and a certain level of active movement capacity influences the spatial distribution of each ecotype. Additionally, their preference for specific prey items, which are influenced by environmental factors, could further influence survival and consequently population structure. Such additional evolutionary force can advantage genetic variants with greater fitness in specific environment maintaining also in early life stages a certain balance among alleles, increasing the level of genetic differentiation between populations (Malavolti, 2017). Nonetheless, fisheries studies focused on analyzing environmentally induced stock fluctuations rely on variables that are comparatively easier to analyze than the other aforementioned driving forces affecting the species’ habitat and recruitment variability, which are more challenging to monitor (Lafuente et al., 2002, 2005).
Passive dispersal is a prominent feature of anchovy early life stages. Anchovy eggs and larvae are subject to ocean currents, which can carry them over considerable distances, transporting them from spawning grounds to various nursery areas (Ospina-Alvarez et al., 2012). This passive dispersal can occur on varying spatial scales, depending on the strength and persistence of marine currents, within an estimated distance covered of about regional scales. In any case, these observations underscore the importance of understanding oceanographic processes in predicting anchovy distribution and the central role of ocean circulation in driving recruitment strength and, definitely, resource abundance (Ospina-Álvarez et al., 2013; Ospina-Alvarez et al., 2015). The high rate of egg dispersion caused by marine currents and the different degrees of local retention could explain the genetic heterogeneity observed in the adult populations along the Mediterranean Sea, with different sites harboring coastal ecotype, offshore ecotype, or both of them in different percentage degrees (Catanese et al., 2020). Such clustering could be based on the diet composition of coastal and offshore ecotypes but the presence of different prey in different coastal areas depends on their geomorphology and hydrodynamics, e.g., the presence of rivers (Catanese et al., 2017). The analysis of SNP markers in European anchovy egg samples helped to identify anchovy population parental origins on a small spatial scale (200 km) through the assignment of genotypes to adult anchovy stocks (Catanese et al., 2020). Results underscored the significance of habitat type (offshore versus coastal/estuarine) as a crucial factor in the genetic differentiation among anchovy populations. In the central Tyrrhenian Sea, only two specific locations, called “Capaccio” and “Cetara” by Catanese and colleagues (Figure 2), served as the donor populations for all the sampled adult populations belonging to the offshore ecotype (Catanese et al., 2020). While some degree of mixing among the sampled egg hauls may exist, the assignment of egg groups to adult populations allowed for the differentiation of contributions from distinct ecotypes to new wild generations. The study also described how the high rate of egg dispersion, driven by marine currents, and the varying degrees of local retention could account for the genetic heterogeneity observed within the adult populations, where eggs from neighboring spawning sites tended to intermingle.
As anchovies metamorphose from the larval to juvenile stages, they become more capable of horizontal and vertical active dispersal (Smith et al., 2001) often occurring within specific habitats (Parada et al., 2008). It has been hypothesized that diel vertical migration of both anchovy larvae/juveniles and their prey (copepods) plays an important role in their spatial distribution (Baldó et al., 2006; Ospina-Alvarez et al., 2012; Casaucao et al., 2021). The use of 3D advanced models, that consider vertical daily migrations, biological behavior, egg-buoyancy, and growth, provides a more precise insight into the degree of connectivity between spawning and nursery areas, as well as a better understanding of the species/ecotypes recruitment and management (Ospina-Alvarez et al., 2012; Casaucao et al., 2021). It has been demonstrated that such simulations taking into account juveniles behaviors better describe the formation of schools in anchovy larvae and juveniles, evidencing a transport associated with filaments and meanders depending on the mesoscale oceanographic structures (Ospina-Alvarez et al., 2012). This idea is in agreement with previous researches, who proposed that temperate fish larvae and juveniles, such as anchovies, can detect preferable nursery areas and swim toward them by using a set of sensory cues (infotaxis strategy) (Teodósio et al., 2016).
In the adult stage, anchovies actively move in response to changing environmental conditions, such as temperature and prey availability, influencing their distribution (Guraslan et al., 2017). For all these reasons, success in the reproductive endeavors of pelagic fish, especially those that congregate in sizable schools, hinges upon an intricate interplay of variables. These factors encompass the environment’s conditions, the influence of oceanic currents, the salinity of the water, the availability of nourishing sustenance and chlorophyll, etc. The Mediterranean Sea, rife with diverse habitats teeming with anchovies, hosts an assortment of phenotypic and behavioral adaptations among these populations. These distinct groups exploit different ecological niches for the development of their communities (Cardinale et al., 2004; Rumolo et al., 2016; Catanese et al., 2017).
3 Dietary habits and metabolomics
3.1 Trophic ecology and dietary preferences
Engraulis encrasicolus stomach contents offer intriguing insights into their dietary habits and their role within the marine ecosystem thanks to their position as a critical link in the marine food web (Chouvelon et al., 2015; Essington et al., 2015; Awad et al., 2022). Distinct foraging behaviors and dietary preferences are pivotal components of resource partitioning, allowing different species to coexist while reducing competition (Colloca et al., 2010; Liedke et al., 2018; Da Ros et al., 2023). Additionally, different European anchovy prey-preferences impacts the composition of lower trophic levels, influencing the entire food web structure (Colloca et al., 2010; Chouvelon et al., 2015; Rumolo et al., 2016; Awad et al., 2022). Several studies have examined the interactions between various pelagic species, such as Sardinella aurita, Sardina pilchardus, and Engraulis encrasicolus, revealing competition for food resources and overlapping trophic niches among these zooplanktivorous fish species (Bachiller et al., 2020, 2021). While the differences in diet and behavior among sympatric species have been established, it is worth noting that different populations and ecotypes of anchovies may exhibit variations in depth distribution and diet, indicating niche differentiation. This differentiation in resource utilization helps reduce competition, promote species diversity, and enhance ecosystem stability (Awad et al., 2022). Additionally, the presence of distinct ecotypes is influenced by various environmental factors, which in turn shape their physiology, behavior, and interactions with the zooplankton community, thus affecting the trophic web (Catanese et al., 2017). Anchovies exhibit dietary variations in response to environmental food availability revealing a complex mosaic of prey items across various populations (Table 1) (Tudela et al., 2002; Bacha and Amara, 2009; Borme et al., 2009; Raab et al., 2013; Chouvelon et al., 2015; Bachiller et al., 2020, 2021; Basilone et al., 2020; Awad et al., 2022). European anchovy larvae in the north-western Mediterranean primarily feed on copepod eggs, nauplii, and copepodites, with heightened activity during daylight hours. As the larvae grow, their diet broadens to include a variety of prey sizes, particularly an increased consumption of copepodites (Tudela et al., 2002). A study analyzing data from the years 2010-2011 on Northeast Atlantic anchovy’s stomach contents, revealed that they exhibited a feeding pattern characterized by copepod-dominated, particularly small and medium-sized organisms. The former study also highlighted trophic segregation as the cause of a negative fluctuation in anchovy abundance during the spring season (Chouvelon et al., 2015).
Raab et al. offered a different viewpoint regarding the European anchovy population in the North Sea. Their study aimed to investigate the influence of temperature, along with food availability, on this particular population (Raab et al., 2013). Through the utilization of spatiotemporal statistical models that correlate anchovy abundance with environmental factors, specifically temperature and food availability, the research revealed that temperature played a more significant role in explaining the distribution and abundance. These findings suggest that, in the case of North Sea anchovies where food resources are not the limiting factor, variations in temperature hold greater significance than alterations in food availability in facilitating the attainment of the necessary size for winter survival (Raab et al., 2013). Two recent studies (Bachiller et al., 2020, 2021) examined the stomach contents of E. encrasicolus during the spring of 2018 in the western Mediterranean Sea. The results reveal a significant presence of Calanoidae, order Euphausiacea, and order Decapoda. Diatoms also feature prominently, particularly within the taxonomic families of Chaetocerotaceae, Bacillariaceae, Rhizosoleniaceae, and Thalassiosiraceae (Bachiller et al., 2020, 2021). While the amount of diatoms in anchovy stomach contents is typically limited if compared with Sarda sarda (Campo et al., 2006; Fletcher et al., 2013; Albo-Puigserver et al., 2016), it has been demonstrated that these diatoms are abundant in secondary metabolites, which exert diverse effects on the cellular and tissue processes of several species (Mutalipassi et al., 2019; Levy et al., 2021; Bahi et al., 2023). Although the effects of diatoms bioactive compounds on anchovy population have been demonstrated only regarding toxic effects (Scholin et al., 2000; Lefebvre et al., 2001; Fire et al., 2010), it is known that secondary metabolites effects can be elusive to trace and have the potential to alter animal behavior and physiological functions, ultimately influencing population dynamics, as demonstrated in previous studies in other taxa (Gorbi et al., 2014; Lopes et al., 2019; Mutalipassi et al., 2019, 2022; Zupo et al., 2019). Moreover, variations in the frequency of prey ingestion within the same species appear to correspond to a latitudinal signal, suggesting potentially more efficient predation on larger prey like krill. This latitudinal pattern signifies alterations in the trophic ecology of anchovies, aligning with enhanced biological conditions in the southern region of the study area (Bachiller et al., 2020, 2021). An earlier examination of the stomach contents of European anchovies, collected in the Northern Adriatic in October 2002 (Borme et al., 2009), revealed a predominantly zooplanktivorous diet centered around a limited selection of small copepod species (measuring 0.2 to 0.3 or 0.5 to 0.6 mm). Throughout the daylight hours, the copepods Euterpina acutifrons and Oncaea spp. were the dominant components of the anchovy diet, both in terms of their frequency, abundance, and overall biomass, across all size categories of anchovies. During nighttime hours, bivalve larvae also played a significant role in the anchovy’s dietary composition, accounting for more than 69% of the total prey consumed across all anchovy size groups, along with the aforementioned copepods Oncaea spp. and E. acutifrons (Borme et al., 2009). Bacha and Amara conducted a comprehensive study on European anchovies, examining spatial, temporal, and ontogenetic variations in three regions: Bejaia, Benisaf, and Ghazaouet along the Algerian coast in the southwestern Mediterranean (Bacha and Amara, 2009). Irrespective of factors like season, region, or size, the anchovies’ stomach contents were found to consist exclusively of zooplankton, primarily comprising 87% copepods. Nevertheless, the presence and abundance of copepods varied over time. In the first year of life, anchovies consume primarily small to medium-sized copepods. As they matured, these were gradually supplanted by larger decapods and amphipods (e.g., crustaceans). Statistical analyses, including cluster analysis, ANOSIM, and SIMPER, revealed a distinct anchovy diet in Bejaia Bay compared to the other two regions, likely due to differences in hydrological conditions (Bacha and Amara, 2009). Furthermore, differences in diet composition were observed across seasons. Summer and spring exhibited unique prey groups and demonstrated lower dietary similarity compared to the other two seasons. In contrast, winter (with 36 prey species) and autumn (with 30 prey species) featured a more diverse diet. Interestingly no traces of phytoplankton were found in the stomachs of anchovies in this study. The trophic ecology of adult European anchovy in different regions of the central Mediterranean Sea, including the Strait of Sicily and the Tyrrhenian Sea (South Campania, Gulf of Gaeta, South Elba, Strait of Sicily) was examined and compared through stable carbon and nitrogen isotope analysis (Rumolo et al., 2016). Results revealed a distinct geographical pattern in anchovy feeding behavior during the summer, characterized by an increasing trend towards the northward where anchovies captured in the northern Tyrrhenian Sea exhibited higher isotope values if compared to individuals sampled in southern region. These elevated carbon and nitrogen values may suggest that anchovies tend to inhabit coastal waters, which contain a greater concentration of 13C derived from benthic primary producers in addition to phytoplankton. The findings of Rumolo and colleagues imply that the feeding behavior of E. encrasicolus is influenced, either directly or indirectly, by local factors and resource distribution, in relation to the size of zooplankton.
3.2 Metabolomics for ecotypes identification
The existence of distinct populations was suggested despite the significant considerations given to the interaction between the food web and the distribution of European anchovies, but an exhaustive worldwide assessment is still absent. Nowadays, the distinction between the different ecotypes becomes a categorical imperative, essential to reach a deeper understanding of all economic, ecological, and biological processes related to the presence/absence of one ecotype rather than the other. The development of omics techniques has undoubtedly revolutionized the characterization of the functional responses of biological individuals, populations, or whole communities. Among these techniques, metabolomics represents the endpoint of the omics cascade as well as the level following high throughput and high-resolution analyses of RNA and proteins (transcriptomics and proteomics, respectively) (Macel et al., 2010). As a matter of fact, the set of metabolites synthesized by an organism reflects the surrounding environments representing a helpful tool in studying the mechanisms of interactions between organisms and their environment (Ryan and Robards, 2006; Bundy et al., 2009). Over time, metabolomics has found extensive application in the ecological field, so much that this combination for some authors has given rise to a new discipline, the “ecometabolomic” (Sardans et al., 2021; Uthe et al., 2021). Typically, metabolomics has been applied to explore the effects of pollutants and emerging contaminants and generally to physiological changes (Fasulo et al., 2012; Farré and Jha, 2020; Zhang et al., 2021; Liu et al., 2022), to determine the nutrient profile for aquaculture purposes/effects (Maruhenda Egea et al., 2015), for food security (Yueqi et al., 2023) or to determine habitat qualities (Goode et al., 2020). In addition, metabolomics is supposed to provide reliable information on the geographic origin of the species under examination (Zhao et al., 2020). This methodology has already found widespread application in the field of plant ecology for distinguishing, for example, between ecotypes of Chenopodium quinoa, Ipomoea batatas, Arabidopsis thaliana, and Cistanche deserticola (Shekhar et al., 2016; Lalaleo et al., 2020; Sun et al., 2020; Segarra-Medina et al., 2023). However, in the realm of differentiating between ecotypes of marine organisms, its use remains relatively limited. To date, this approach has allowed us to obtain data related to the traceability of geographic origin and detection of species-mislabeling in marine bivalves (Santos et al., 2023) or sponges and deep-sea corals (Vohsen et al., 2019; Bayona et al., 2020; Mohanty et al., 2021). Interestingly, Heal and co-authors demonstrated that in 21 cultured phytoplankton species, the bulk community metabolomes reflect the chemical composition of the phytoplankton community identifying a (chemical) fingerprint of the community across different environmental gradients (Heal et al., 2021). Thus, driven by the close dynamic relationship that exists between the metabolites measured and the physiological status of the whole organism, metabolomic fingerprinting approaches could provide useful information on interspecific relationships (Ivanišević et al., 2011). In this context, the application of fingerprint metabolomics would seem to be a very valid tool for distinguishing and characterizing the offshore and coastal ecotypes of E. encrasicolus. Disparities in prey composition closely align with the genetic separation observed between distinct anchovy ecotypes. Offshore ecotype has been demonstrated to predate preferentially larger zooplankton, showing higher environmental tolerance and opportunistic feeding behavior (Catanese et al., 2017). Conversely, their coastal counterparts thrive in the vicinity of river mouths, showcasing a positive correlation with lower salinity and elevated chlorophyll values (Catanese et al., 2017). The dietary preferences of coastal anchovy ecotypes center around smaller prey, including small copepods like Euterpina, Farranula, Oithona, Acartia, and various calanoids, along with their nauplii, together with other small-sized prey like bivalve larvae. The diverse prey preferences of anchovy populations have been demonstrated to be not correlated with the difference in prey abundances. These nuanced interactions resonate throughout marine ecosystems, shaping species coexistence, lower trophic levels, and the overall structure and function of marine food webs (Bachiller and Irigoien, 2015; Poiesz et al., 2020). These feeding behavior differences not only have cascade effects along the trophic chain but can confer a different characteristic composition in terms of levels of amino acids, lipids, and other elements such as to allow the possibility of complex biochemical fingerprints for definitively identifying the two different ecotypes. In the context of Protected Designation of Origin (PDO) regulations governing products derived from marine sources, these unique biochemical fingerprints hold immense significance. They emerge as interesting markers that can facilitate the precise classification and authentication of products deriving from specific ecotypes. This intricate differentiation mechanism acts as a safeguard, upholding the authenticity and integrity integral to PDO guidelines. As emphasized by the European Union in 2020, this ensures that consumers consistently receive products that mirror the anticipated characteristics and superior quality associated with their designated origin (European Union, 2020). For example, investigations has demonstrated the prevalence of offshore ecotypes in the vicinity of Cetara juxtaposed with the existence of coastal ecotypes in more distant southern and northern regions (Catanese et al., 2017). This variance underscores the necessity for distinguishing between these populations or ecotypes, a critical aspect in the realm of stock assessment science. Such identification forms a cornerstone in the development of effective fishery management plans, integrating crucial information into the framework of PDO, Protected Geographical Indication (PGI), or Traditional Speciality Guaranteed (TSG) food products (Di Pinto et al., 2019; Minos and Loukovitis, 2019; Girard, 2022). By integrating these findings into regulatory frameworks and management strategies, stakeholders can navigate the nuances of distinct ecotypes, thereby enhancing the precision of product categorization while bolstering sustainable fishing practices. This approach ensures that PDO and related designations uphold their reputation for authenticity, empowering consumers to trust in the specific origin and exceptional quality of the marine-derived products they choose.
In summary, metabolomics is a valuable and evolving approach for distinguishing between two different ecotypes within a species. By revealing the unique metabolic adaptations of these populations, it enhances our understanding of how organisms respond to their environments and provides insights into the ecological and evolutionary processes that shape biodiversity. No less important is the potential support that would arise in distinguishing ecotypes from the perspective of the fraudulent misdescription of seafood, including the mislabeling of raw material, production, and origin. While metabolomics is a promising tool for distinguishing ecotypes coupled also with other molecular techniques, challenges such as sample collection, data analysis, cost-effectiveness, time and standardization must be addressed (Santos et al., 2023). Additionally, to date, the metabolome of anchovies is still poorly explored. Therefore, there is a need to expand the metabolite databases to support a robust comparative analyses also considering the sensitivity of metabolomics to external factors such as age, diet and diurnal and reproductive cycles (Jones et al., 2013).
4 Traditional and industrial processing: from fishing to industries
4.1 Industrial and traditional fishing in the Mediterranean Sea
Engraulis encrasicolus stands as one of the most targeted species of the Mediterranean fishery (FAO, 2022a). This pursuit traditionally employs active techniques such as the lampara system, the pelagic trawl (volante), or the traditional menaica (Cingolani et al., 1996; Tsagarakis et al., 2014; Sartor et al., 2019).
In the lampara fishing techniques (Figure 3), anchovies are typically lured by boat equipped with powerful lights, known as lampara, and then encircled by a purse seiner (Di Cintio et al., 2022). In the Tyrrhenian Italian region of Campania, this technique generally is carried out using fishing vessels with a length overall of less than 24 meters (Lucchetti et al., 2017). This technique takes advantage from the attraction to light of several fish species (Fitzpatrick et al., 2013; Nguyen and Winger, 2019) assuming primary roles in triggering a diverse array of stimuli (Marchesan et al., 2005; Liao et al., 2007; Sabbah et al., 2010; Bryhn et al., 2014; Matsui et al., 2016). Light plays a pivotal role in triggering schooling behavior in various fish species, whether as a defense mechanism or a predatory strategy (Pavlov et al., 2000), enabling them to discern prey, seek shelter, and locate conspecifics, standing as a fundamental sensory input vital for their day-to-day survival (Cortesi et al., 2020; Oteiza and Baldwin, 2021). The utilization of light in fishing has emerged as one of the most advanced, efficient, and successful methods for capturing commercially valuable species, as in the case of the lampara technique. This fishing activity takes place during the night and fish are attracted to the surface using artificial light. The illumination is created by lamps mounted on auxiliary vessels called lampara, which are lowered into the fishing area from the main vessel (Pertierra and Lleonart, 1996; Cingolani and Santojanni, 2003; Edwin, 2019; Giráldez, 2021). In order to maximize fish capture, two auxiliary vessels often can operate both at the same time in a joint fishing operation force for the catch. The illumination system remains activated for a period of approximately 2 hours, during this time anchovies swim relatively close to the surface. When the aggregation is complete, another boat called stazza is released at sea from the main vessel quickly as possible moving in a circle to the shoal to close the net by winch. At the end of each fishing operation, fish are hoisted on board with large nets and put into tanks full of water and ice (Cingolani and Santojanni, 2003; Edwin, 2019).
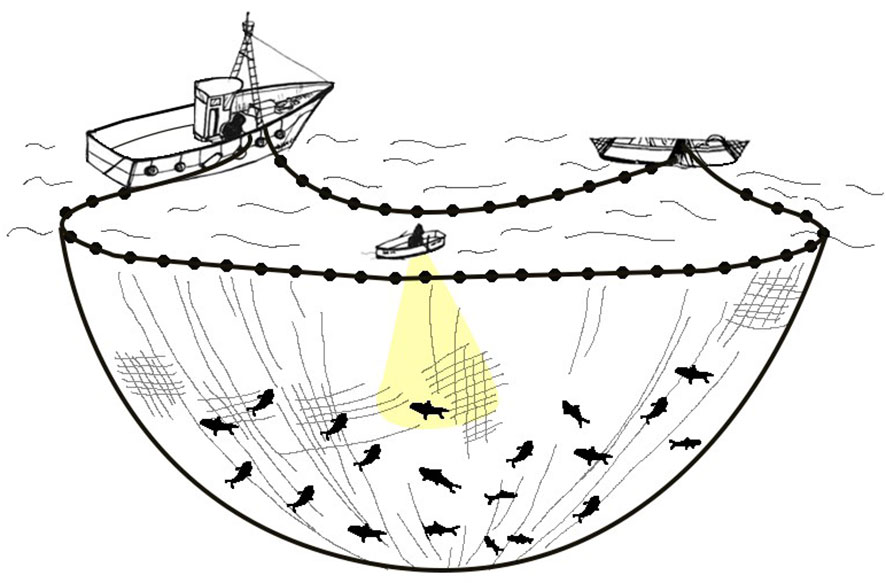
Figure 3 Lampara fishing techniques. To catch anchovy using a purse seiner with lampara, a large net is deployed around a school of anchovies, encircling them. The central lampara light attracts the anchovies towards the net, and then the net bottom is drawn closed to trap the fish, allowing for their easy retrieval.
The second method involves towing a net in mid-water without contacting the seabed, called the volante, which is used to catch European anchovies and other small pelagic species (Figure 4). At the national scale, this technique represents less than 1% in terms of vessels. This technique is present in the Adriatic Sea fleet, Geographical sub-areas (GSA) 17, which represents the larger volante fish fleet in terms of numbers, followed by the Southern and Central Tyrrhenian fleet in the GSA 10 (MIPAAF, 2021). In this case, the fish are captured through the movement of the net towed by the boat. Volante vessels fish only in the daytime and land their product every evening. Before beginning this phase, a particular echo sounder installed on board is used to locate and estimate the size of the shoals of anchovies in the water column. The fishing process involves a series of well-coordinated actions that unfold in a particular sequence, each step designed to optimize the capture of anchovies (Pertierra and Lleonart, 1996; Cingolani and Santojanni, 2003; Russo et al., 2015). Firstly, the vessel initiates a deliberate reduction in speed, a crucial maneuver that sets the stage for the subsequent activities. The net is then skillfully deployed at a carefully chosen distance from the seabed. This placement is a strategic decision aimed at increasing the chances of a productive catch. As the trawling operation commences, the vessel’s speed is methodically increased. This acceleration is a calculated effort to cover a larger area and ensnare as many anchovies as possible. Once the trawl has been executed, the net is expertly hoisted back onto the vessel. The anchovies, now captured, are gently transferred, as seen for the lampara fishing technique, in a container containing water and ice. This careful handling is essential to ensure the anchovies’ freshness and preserve their quality until they reach their final destination (Cingolani and Santojanni, 2003; Russo et al., 2015).
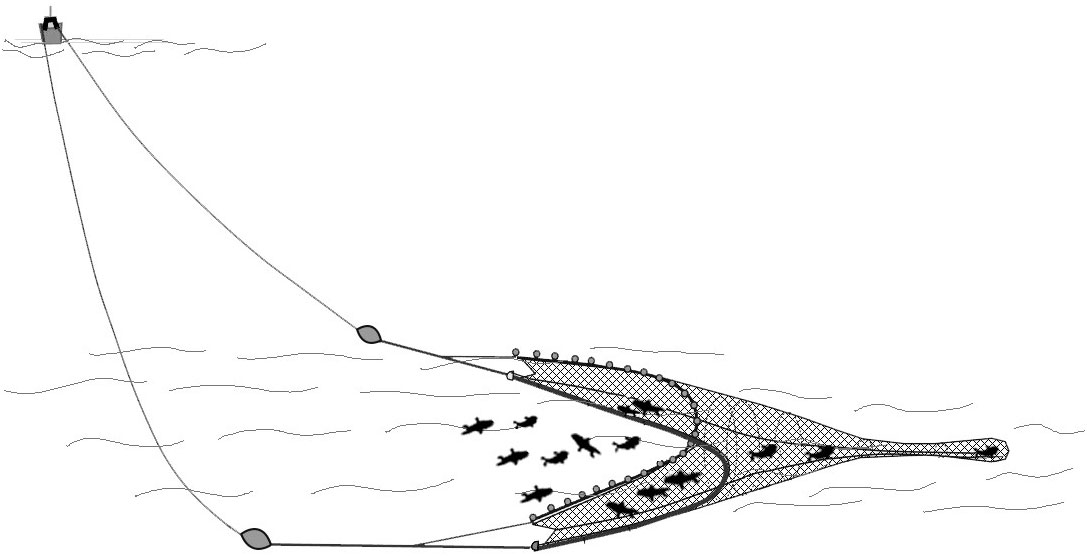
Figure 4 Volante fishing technique. To catch anchovy using the mid-water volante net technique, a vessel deploys a rectangular net designed to encircle schools of anchovies swimming at mid-depths. The net is suspended horizontally in the water column and equipped with floats and weights to keep it at the desired depth. As the vessel tows the net through the water, the anchovies were caught into the net, and once a sufficient amount is captured, the net is hauled in to harvest the fish, making it an effective method for targeting midwater schools of anchovy.
However, a third distinct fishing gear, typical of small-scale fisheries with a length overall of less than 12 meters, is notably present along the coasts of southern Italy, particularly in GSA 10 and 19 (Figure 5), and is known as menaica or menaide (Sartor et al., 2019). The menaica method is characterized by scarcely mechanized gear generating an activity strictly correlated with ancient traditions of small fishing fleets (Sartor et al., 2015, 2019). It is performed by a relatively modest menaica fleet, which comprises only around 40 boats in Sicily and 19 in Campania (Sartor et al., 2019). In compliance with Italian legislation, which has governed its technical specifications since 2012, menaica is a passive net categorized as a sub-group of the small-scale driftnet (SSD) passive net subcategory (Lucchetti et al., 2017). As a driftnet, menaica was banned in 1998 along with large-scale driftnets targeting swordfish (Ferretti et al., 1995, 2002), the latter producing numerous instances of the unwanted catch of protected, endangered, and threatened species, such as marine mammals and reptiles, generating great environmental concerns (Di Natale, 1995; Di Natale et al., 1995; Tudela, 2004). Menaica has been recognized as a highly selective and specialized fishing gear and it has been demonstrated, in fact, that anchovy is the only target species for the fleets of Catania (Sicily) and Cilento (Campania). The European sardine (S. pilchardus) is the second target species and it has been reported that it represents the main target species for the menaica vessels from Slovenia (Sartor et al., 2019). They have been monitored in certain areas under the context of the European Union Data Collection Regulation (DCR) and subsequent Data Collection Framework (DCF). However, monitoring is limited to specific locations due to the scattered distribution of SSD fisheries and the low level of landings or fishing efforts. For instance, the menaica fishery (Figure 6) is monitored primarily in the FAO‐GFCM Geographical Sub‐Area (GSA) 19 (western Ionian Sea), in GSA 10 (Central-Southern Tyrrhenian Sea) and occasionally in GSA 16 (Central Mediterranean sea, south of Sicily island) (Sartor et al., 2019). The menaica method employs a single netting panel made of nylon, deployed no more than three miles from the coast. The net has a maximum length of 500 meters, a height of 10 meters, and a range of mesh size (19 -29 mm) (Sala et al., 2018). Depending on the fishing area and target species, menaica operations typically occur from April to September, primarily during the nighttime (Sartor et al., 2019). One of the notable advantages of the menaica technique is its inherent selectivity, predominantly capturing adult anchovies while minimizing issues related to by-catch (Sartor et al., 2019). The design of the net prevents larger fish from breaking through it, while allowing smaller ones to pass, contributing to its efficiency in target species retention. Along the South Italian coastline, anchovies caught especially using the menaica method are traditionally preserved in wooden barrens in alternating layers separated by sea salt, after being gutted and beheaded by hand (Corona et al., 2022).
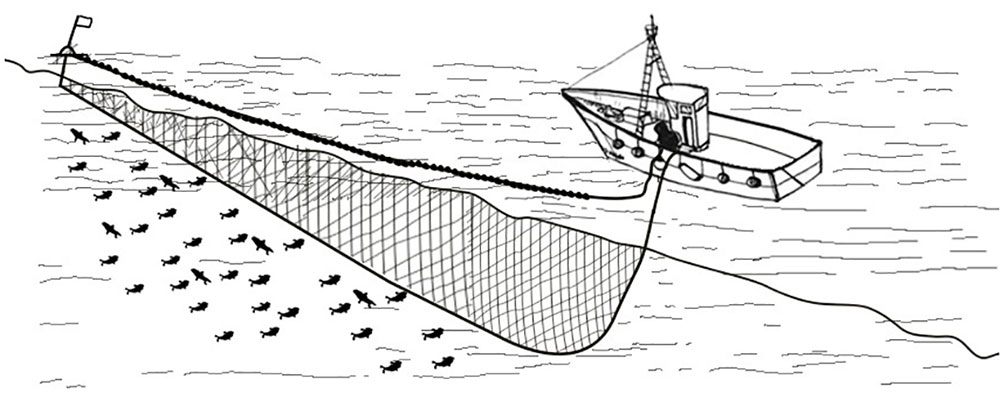
Figure 5 Menaica fishing technique. In this fishing technique, a specialized type of drift-net is used to catch anchovies. The menaica is deployed vertically in the water, suspended from surface floats and tensioned in the water thanks to the lead-file placed below. Schools of anchovies swim in the net as it drifts, and the mesh size of the gill net is designed to entangle fish with their gills. This fishing gear is highly selective for anchovies and, partially, sardines, and the selectivity is given by the type of mesh used, reducing by-catch to a minimum.
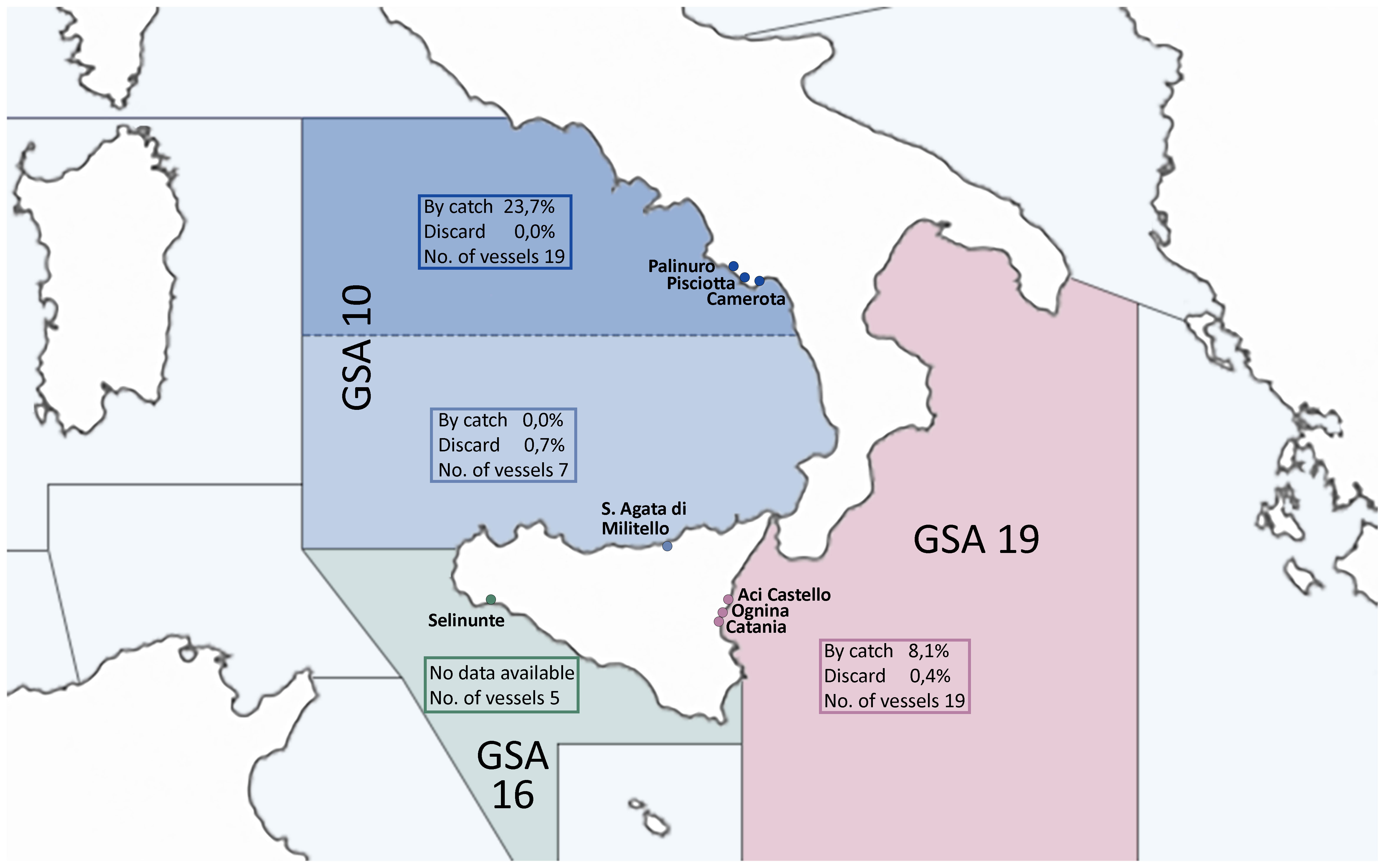
Figure 6 Menaica fishing activity in central Mediterranean Sea (South of Italy). The image includes GSA numbers for each specific geographical area, as well as percentage data about bycatch and discards, along with information about the count of vessels engaged in menaica fishing in the waters of Italy. Notably, the image also brings attention to the ports where menaica vessels have been recorded (Sartor et al., 2015, 2019).
4.2 Economic significance and challenges of anchovy fisheries: sustainability, fraud, and geographical traceability
The economic importance of anchovies in the Mediterranean cannot be understated. European anchovies are a vital component of regional fisheries, supporting livelihoods and providing a source of income for coastal communities (FAO, 2018). They are processed into various products, including canned goods, fishmeal, and fish oil, contributing to local and international markets (Dinçer, 2018). The long-term survival of anchovy populations depends on implementing sustainable fisheries management, which includes enforcing catch limits and creating protected zones (Di Franco et al., 2016). Due to its importance in regional pelagic fisheries, this species is highly appreciated in Mediterranean markets. The especially rich nutritional profile contributes to its appeal as a commercial fish species. It is characterized by a high concentration of important polyunsaturated fatty acids (PUFAs), including omega-3 fatty acids like docosahexaenoic acid (DHA) and ecosapentaenoic acid (EPA), as well as omega-6 fatty acids such as arachidonic acid (ARA) (Zlatanos and Laskaridis, 2007). Anchovies, including European ones, are commonly found in the European market as processed food, as in the case of brine-fermented or fileted and canned (preserved in oil) anchovies, in addition to the common use as fresh fish (FAO, 2020). The fisheries industry is vulnerable to dishonest practices, and it is not sufficiently safeguarded. The main factors impeding the fight against fraud include the high level of complexity of the fish supply chain, the large number of parties involved, and the quick perishability of the product. These illegal procedures have adverse economic and sanitary consequences on different levels of the production and supply chain, from producers to transformers and final consumers (FAO, 2018). Consumers’ perceptions of fresh or processed fish and seafood items’ quality are the result of a variety of objective and subjective elements, and this perception has a direct impact on the product’s market and worldwide economic values. The so-called country-of-origin effect, which states that consumers increasingly tend to associate high-quality fish products with specific production areas due to specific sensorial characteristics, and ethical or ecological motivations, is currently encouraging mislabeling or misrepresenting the origin of fish products (Varrà et al., 2021b). According to several authors, fraud is a common occurrence concerning processed fish products made from the industrial transformation of the extremely valuable European anchovy (Velasco et al., 2016). Using misleading labels or other dishonest marketing techniques in the seafood supply chain about the quality, origin, quantity, or species it is a widespread problem in European and American fisheries (Petrossian and Pezzella, 2018). Up to one-third of all seafood consumed in the United States has been misidentified and sold as something different from what these customers thought they were buying, according to recent estimates of species substitution (Silva et al., 2021). Additionally, substitute rates for seafood vary by species, with some substitutions being as high as 70%. Anywhere in the seafood supply chain can experience seafood fraud (Silva et al., 2021; Lawrence et al., 2022). European legislation (General Food Law, regulation (EC) No 178/2002) mandates the identification of species through distinct commercial names solely in fresh and prepared fishery products. However, in processed products, generic designations can be used instead of specific species names leading to misleading information given to consumers and several techniques has been develop to identify species in processed products (Muñoz-Colmenero et al., 2016; Cutarelli et al., 2018). This pertains to European anchovy paste, where the PCR-RFLP technique has revealed the presence in different percentage of undisclosed species, spanning from Sardinella aurita to Sprattus sprattus, and S. pilchardus (Rea et al., 2009). In other investigations, COI-DNA barcoding was used in combination with PCR-RFLP method to investigate labeling species accuracy in different processed food (i.e., anchovy fillets in vegetal oil, canned anchovies, and anchovy paste) demonstrating that up to the 14% of samples contains unlabeled species, e.g., Engraulis japonicus, S. aurita and S. pilchardus (Pappalardo and Ferrito, 2015). Similar results has been achieved using BLAST techniques where other species belonging to Engraulis genus has been found in mislabeled anchovy products, such as anchoveta (E. ringens), Argentine anchovy (E. anchoita) and Japanese anchovy (E. japonicus) (Giusti et al., 2019).
Despite the successful application of various techniques for fish species identification (Black et al., 2017), detecting fraud associated with fishing areas remains challenging for the majority of commercial species found in the fish market. Scientific research dealing with the identification of fish and seafood origin used several different approaches. Among these approaches, methods involving inorganic components like stable isotopes (Carrera and Gallardo, 2017), trace elements (Smith and Watts, 2009), a combination of the two previous techniques (Li et al., 2019; Varrà et al., 2021b), or the use of multi-elemental analysis (Rodushkin et al., 2007) have proven successful in several cases. In particular, the multiple identification of elements using techniques such as inductively coupled plasma-optical emission spectroscopy (ICP-OES) and inductively coupled plasma-mass spectrometry (ICP-MS) was used successfully to track back to the origin of processed or highly processed products (Varrà et al., 2021b). Furthermore, near-infrared (NIR) spectroscopy has been employed to evaluate the geographical traceability of salted ripened anchovies originating from diverse Mediterranean regions. Disparities in protein, peptide, amino acid, and proteolytic compound signatures, and particularly in fatty acid profiles, signify distinctive features of aquatic environments, influencing the overall chemical composition of the final product (Varrà et al., 2021a).
Another different approach is linked to the possibility of imposing the use of monitoring software for fishermen to track their fishing activity. These reports are expected to encourage fishing vessel owners to practice self-control and accountability and to increase their perception of being caught and sanctioned if they contravene the law (Petrossian and Pezzella, 2018). In addition, it should be important to check licenses, gear, fish caught, and catch registered through the support of local port authorities to ensure that the fishers take the necessary steps and follow the regulations more carefully, as this will “alert conscience” about possible penalties if they fail to do so (Petrossian and Pezzella, 2018). Several food items have been objects of interest for the European Union, which decided to protect foods with characteristics of unicity. For this reason, PDO, PGI, and TSG labels have been adopted to avoid fraudulent practices (Consonni and Cagliani, 2022). European anchovies are not immune to this activity, especially considering that such high-quality commercial products require a clear statement of Geographical traceability and consequently of the fishing areas, as in the case of traditional Italian anchovy sauce with PDO called “colatura di alici di Cetara”. On the Amalfi Coast (Italy), since 1807, anchovies caught are gutted, beheaded by hand, and placed in wooden vats in alternating layers separated by sea salt. Once the layers are ready, a wooden disk with weights is placed on top of the vats, and the aging process starts. A clear liquid called “colatura”, obtained from pressed anchovies matured in wooden vats under salt for at least 9 months, is finally collected (Corona et al., 2022). During the maturation process, lipolytic and proteolytic activities exert a significant influence on the commercial attributes of the product, leading to the formation of distinctive volatile compounds and the production of biogenic amines, such as histamine. This phenomenon represents one of the foremost hygienic and health concerns in fish products belonging to the Engraulidae family and their enzymatic maturation-derived products. Preliminary investigations suggest that elevated histamine levels, surpassing safe level thresholds, have exclusively present in processed anchovies that have not undergone evisceration (D’Anza et al., 2023). These findings pinpoint a specific condition associated with histamine accumulation, highlighting the significance of the processing method in mitigating histamine content.
4.3 Valorization of anchovy discards in a circular economy: challenges and opportunities for sustainable and biotechnological utilization
Recently, the Food and Agriculture Organization estimated that, in 2020, fisheries and aquaculture production reached 214 million tones and it is forecasted to grow for another 14% by 2030. Asian countries are at the top of the world ranking with 75% of production in 2020, followed by countries in the Americas (10%), Europe (8%), Africa (6%) and Oceania (1%) (FAO, 2022b). Such increasing global fishery production has resulted in several consequences related to the massive production of waste deriving from industrial processing and by-catch of inedible species. Discards may represent up to 70% of processed fish, depending on the size, species, and type of processing, including heads (9–12% of total fish weight), viscera (12–18%), skin (1–3%), bones (9–15%) and scales (5%) (FAO, 2022b). Due to the increasing attention towards such concerning issues, many EU policies and strategies, in terms of fishing management, have been addressed to a strong reduction of waste and re-utilization of them for industrial and biotechnological purposes (Damalas, 2015; Soto-Oñate and Lemos-Nobre, 2021). Within this context, a circular bio-economy approach has been introduced to promote the production of high-value-added compounds from renewable biological resources (Cooney et al., 2023). Several parts of fish and seafood are generally discarded although they store a lot of important compounds including proteins and peptides, enzymes, oils, and biopolymers of biotechnological interest, e.g., collagen, chitin, chitosan (Coppola et al., 2021; Racioppo et al., 2021; Nag et al., 2022; Lal et al., 2023). At present, a concrete alternative to incineration is the re-use of fish wastes as fishmeal for aquaculture purposes and as a fertilizing agent since the rich content in oils, minerals, and essential amino acids reported good results in gonad/tissue fattening and plant growth (Beheshti Foroutani et al., 2018; Ashraf et al., 2020; Ciriminna et al., 2021).
Chemical methods are conventional approaches that have been used for the isolation of high-value compounds from fish discards, although a high quantity of solvents is needed (Ideia et al., 2020). For this reason, green and more sustainable approaches are starting to be applied such as supercritical carbon dioxide (SC–CO2) extraction, microwave-assisted extraction (MAE), and enzymatic hydrolysis (Bruno et al., 2019; Ozogul et al., 2021; Thirukumaran et al., 2022). So far, fish protein hydrolysates have been widely obtained through the addition of endogenous or commercial enzymes that cleave the aminoacidic bonds with highly selective reactions (Ozogul et al., 2021). Interestingly, to reinforce the circular economy approach such enzymes have also been extracted from fish viscera and other parts to obtain protein hydrolysates with antioxidant properties (Khiari, 2022; Borges et al., 2023). Recent findings also allowed the development of novel eco-friendly bioconversion processes taking advantage of microbial fermentation or algal metabolism to recover valuable ingredients and produce biofuels from seafood discards (Marti-Quijal et al., 2020; Vázquez et al., 2020; Venugopal, 2021).
So far, several seafood by-products have reported valuable biological activities and potential applications in marine biotechnology by offering new solutions to improve human health and well-being (Atef and Mahdi Ojagh, 2017; Ashraf et al., 2020; Mutalipassi et al., 2021; Cardeira et al., 2022; Siahaan et al., 2022). For instance, depending on fish species, hydrolysis condition, isolation, and so on, protein hydrolysates rich in amino acids, proteins, peptides, and antioxidants, have shown great potential in aquaculture since a significant improvement of growth, immune function, and disease resistance was observed (Siddik et al., 2021; Moya Moreira et al., 2023). Protein hydrolysates, from sources that are normally discarded during industrial processing such as shrimp shells, reported antioxidant properties and potential applications in nutraceutical and cosmeceutical fields (Henriques et al., 2021; Messina et al., 2021).
Concerning anchovies, high-value compounds have been isolated from discards with different applications in biotechnological fields (Table 2). Chemical approaches have led to the isolation of bioactive compounds from wastes of Cantabrian individuals. In particular, hexane, aqueous, butanol, and ethyl-acetate fractions, from the methanol extract of gut and head, showed anti-mutagenic, antifungal, anti-bacterial, and antioxidant activities (Burgos-Hernández et al., 2016). Extensive chemical analyses from head, frame, and viscera of the European anchovy from the Black Sea also reported good potential in the fabrication of protein powder and hydrolysates, fish oils, and mineral supplements (Gencbay and Turhan, 2016). The majority of products isolated from anchovy wastes are protein hydrolysates with interesting bioactivities that make them suitable functional ingredients for biotechnological purposes (He et al., 2014; Rodrigues Freitas et al., 2016; Mangano et al., 2021).
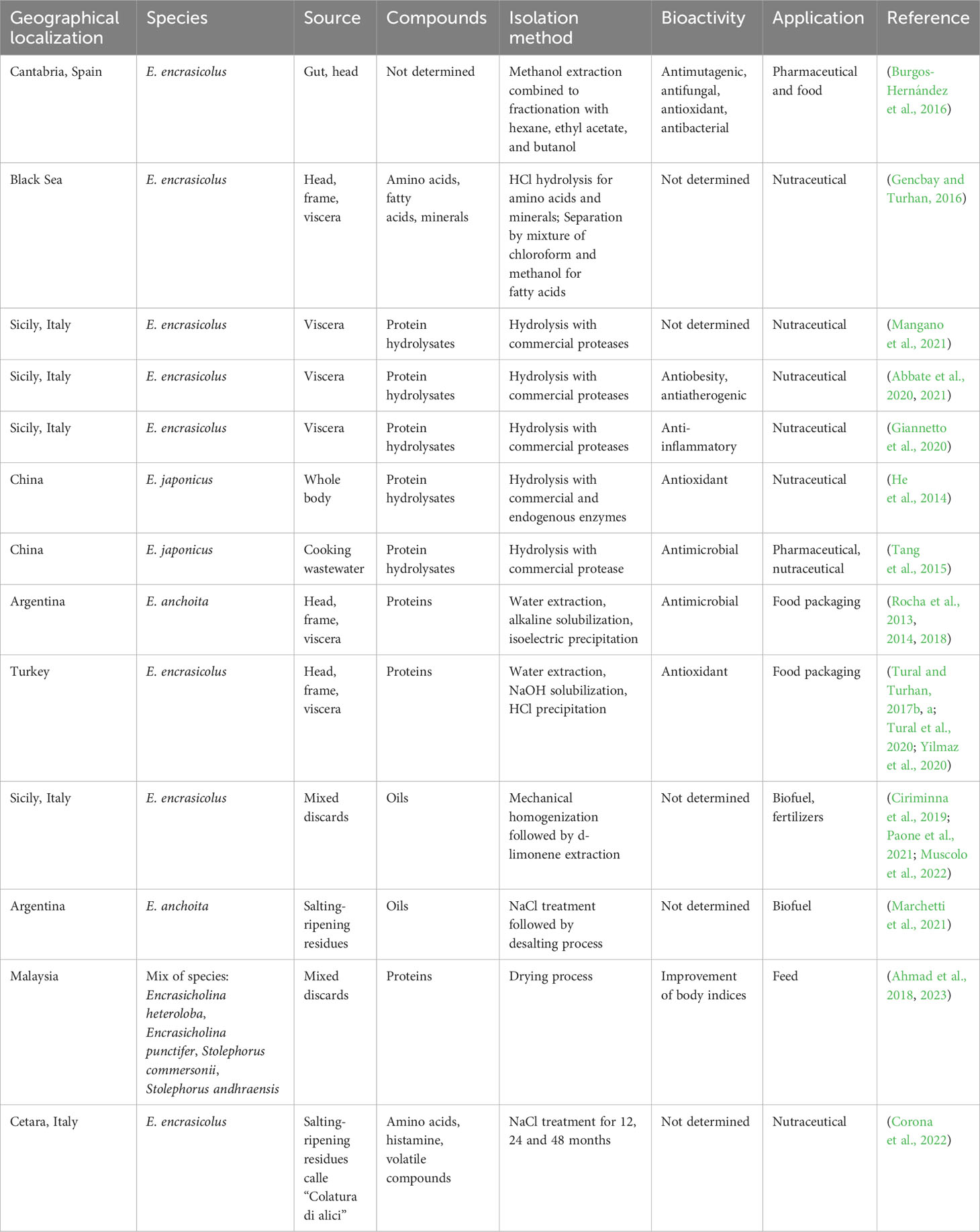
Table 2 Valorization and potential use of anchovy discards: different geographical areas, different species materials, bioactivity and field of application.
In the European anchovies, several studies have been already done on protein hydrolysates characterization and bioactivity screening in both in vitro and in vivo models. High-performance liquid chromatography with diode array detection (HPLC-DAD), nuclear magnetic resonance (NMR), and inductively coupled plasma mass spectrometry (ICP-MS), led to the characterization of protein hydrolysates with high nutritional value obtained through enzymatic digestion of viscera (Mangano et al., 2021). Such protein hydrolysates showed anti-obesity properties and a significant reduction of atherosclerotic plaques in dysfunction-associated fatty liver disease (MAFLD) ApoE–/– mice models with a considerable enhancement of lipid metabolism after 12 weeks of administration (Abbate et al., 2020, 2021). In addition, a clear reduction of protein levels and gene expression of pro-inflammatory mediators such as COX-2 and cytokines TNF-α, IL-1 α, IL-1 β, IL-6, in LPS-induced RAW264.7 treated cells and ApoE knockout administered mice, was also observed (Giannetto et al., 2020). The aminoacidic composition and antioxidant capacity were also evaluated in protein hydrolysates obtained from the Chinese anchovy E. japonicus. DPPH assay revealed a radical scavenging activity with higher efficiency in hydrolysates obtained with commercial enzymes in comparison to endogenous ones (He et al., 2014) and, interestingly, similar results have been reported from the anchovy sprat Clupeonella engrauliformis (Ovissipour et al., 2013). Antimicrobial peptides were instead identified in the hydrolysates obtained from the cooking wastewater of E. japonicus (Tang et al., 2015) and tissues of the half-fin Setipinna taty (Song et al., 2019).
Discarded parts of European and Argentine anchovies were used for the extraction of proteins and the development of edible films to be used in food packaging (Rocha et al., 2013; Tural and Turhan, 2017b). To improve the physiochemical, mechanical, thermal, antioxidant, and antimicrobial properties, some modifications have been applied such as the addition of cross-linking agents (Yilmaz et al., 2020), essential oils (Tural and Turhan, 2017a; Tural et al., 2020), and organic acids (Rocha et al., 2014, 2018).
Fish oils also represent an important source of functional ingredients due to the high content in omega-3 polyunsaturated fatty acids which exert several benefits for humans (Pike and Jackson, 2010). The accomplishment of green extractions through renewable solvents, plus the re-use of both fish oils and solid biomass, allows the highest standards of a circular economy approach. High-quality oils have been successfully extracted from anchovy discards in the fileting process by using limonene obtained from orange peel (Ciriminna et al., 2019). The remaining solid waste was employed for biogas production through microbial anaerobic digestion (Paone et al., 2021), and for the manufacturing of nutrients-rich fertilizers promoting the growth of Tropea’s red onion with higher efficiency in comparison to common organic and chemical products (Muscolo et al., 2022). Oils from anchovy discarded tissues have been also used for biodiesel production with good combustion properties and reduced pollutant emission (Behçet, 2011). As already mentioned, industrial processing produces plenty of residues that are commonly wasted although such material could represent a resource of compounds for animal feed or nutraceuticals. For instance, by-products generated by salting-ripening procedures of fresh anchovies (E. anchoita) harvested from the Southwestern Atlantic Ocean were found rich in proteins, EPA, and DHA that can be recovered through desalting processes (Marchetti et al., 2021). Recently, feeding experiments on the Red-Hybrid Tilapia (Oreochromis spp.) with dried materials obtained from Malaysian anchovies were found to give a significant protein supply and growth performance improvement (Ahmad et al., 2018, 2023). In the Gulf of Salerno, Cetara anchovies have been highly consumed food since 1800 due to their undeniable taste. The salt ripening process that takes, at least, 9 months, allows the production of the so-called “colatura di alici”, a savory liquid that received the status of PDO by the European Union in 2020 and is currently used as dressing sauce for several dishes. A very recent study analyzed the chemical content of Cetara anchovies’ sauce to investigate its nutritional value and safety. Interestingly, when the ripening process was applied for up to 48 months, the “colatura di alici” was found richer in essential amino acids and volatile organic compounds, enhancing nutritional properties and flavor, while histamine significantly reduced by improving sauce safety (Corona et al., 2022). Since the Cetara anchovy is highly appreciated and widely consumed food by both locals and foreigners, every year a lot of discarded material is produced. However, there are no studies on the possible re-use of such discards for biotechnological applications, although recent chemical analyses have suggested a good nutritional value of its salt-ripening sauce (Corona et al., 2022). Furthermore, it is important to note the absence of available data pertaining to variations in metabolite compositions among distinct populations and ecotypes situated along the expanse of the Mediterranean Sea. Therefore, there exists a compelling imperative for future investigations aimed at comprehending any disparities, should they exist, in the potential utility of anchovy catches in diverse geographical regions.
5 Conclusions
In conclusion, this comprehensive review provides valuable insights into the biology of the European anchovies (Engraulis encrasicolus), and the essential role they play in the central Mediterranean Region. The research sheds light on the ecological significance, conservation challenges, and sustainable utilization of Engraulis encrasicolus, highlighting its status as a keystone species critical for both ecological and socio-economic dimensions in the Mediterranean basin. The declining numbers of European anchovy, along with Sardina pilchardus, have raised concerns, emphasizing the urgent need for improved management of anchovy fisheries. This improvement calls for a deep understanding of their reproductive modes, passive transport dynamics influenced by ocean currents, feeding habits, adaptability to environmental conditions, and the distribution of ecotypes along the Italian coasts. Anchovies are pivotal for maintaining ecosystem balance and supporting human communities reliant on marine resources. Information reported in this review serves as a basis for improving effective fisheries management, based as first on the correct identification of management units, i.e. populations and ecotypes. Management also involves the identification and valorization of artisanal practices, and the prevention of fraud, particularly in the context of protected, geographically specific, and traditional high-quality commercial productions. Reported information about anchovy population dynamics, their industrial processing, and the ecological implications of these activities, ultimately contributing to our knowledge of anchovy populations and ecotypes. It is imperative to proceed with research to develop a more straightforward way to identify anchovy ecotypes. This would greatly enhance our ability to understand and manage these distinct populations and ecotypes, helping in the identification of fishery frauds, for example through the development of ready-to-use population/ecotype identification kits. Special attention should be given to their ecological interactions within the food web, with a focus on metabolic and stomach contents diversity among anchovy populations and ecotypes, thus enhancing our understanding of their adaptability to varying environmental conditions. It’s essential to pay attention to potential metabolic differences among populations and ecotypes. This could open doors to the production of nutraceutical and cosmeceutical products, further enhancing the value and sustainability of anchovy resources. Furthermore, there is a clearly reported importance for traditional and industrial processing of anchovies, spanning from fishing techniques to the economic significance and sustainability challenges of anchovy fisheries. It also addresses issues of fraud and the establishment of geographical traceability. It is important to work toward creating a circular economy around anchovy discards, forging agreements with food processing industries. This would not only reduce waste but also contribute to sustainable resource utilization, creating opportunities for the sustainable and biotechnological utilization of anchovy discards, highlighting the potential for waste reduction and resource optimization.
Author contributions
MM: Conceptualization, Data curation, Supervision, Writing – original draft, Writing – review & editing. ED: Conceptualization, Data curation, Supervision, Writing – original draft, Writing – review & editing. MP: Writing – original draft, Writing – review & editing. RF: Data curation, Writing – original draft, Writing – review & editing. CM: Data curation, Writing – original draft, Writing – review & editing. NR: Data curation, Writing – original draft, Writing – review & editing. CP: Data curation, Writing – original draft, Writing – review & editing. GP: Investigation, Supervision, Writing – review & editing. TR: Investigation, Supervision, Writing – review & editing. AT: Funding acquisition, Project administration, Writing – review & editing. VP: Conceptualization, Investigation, Project administration, Writing – review & editing.
Funding
The author(s) declare that financial support was received for the research, authorship, and/or publication of this article. This work has been funded under the National Recovery and Resilience Plan (NRRP), Mission 4 Component 2 Investment 1.4 - Call for tender No. 3138 of 16 December 2021, rectified by Decree n.3175 of 18 December 2021 of Italian Ministry of University and Research funded by the European Union – NextGenerationEU. Project code CN_00000033, Concession Decree No. 1034 of 17 June 2022 adopted by the Italian Ministry of University and Research, CUP C63C22000520001, Project title “National Biodiversity Future Center - NBFC”.
Acknowledgments
We acknowledge architects Gabriele Marino and Ernesto Bianchino for drawing the images used in this manuscript.
Conflict of interest
The authors declare that the research was conducted in the absence of any commercial or financial relationships that could be construed as a potential conflict of interest.
Publisher’s note
All claims expressed in this article are solely those of the authors and do not necessarily represent those of their affiliated organizations, or those of the publisher, the editors and the reviewers. Any product that may be evaluated in this article, or claim that may be made by its manufacturer, is not guaranteed or endorsed by the publisher.
References
Abbate J. M., Macrì F., Arfuso F., Iaria C., Capparucci F., Anfuso C., et al. (2021). Anti-atherogenic effect of 10% supplementation of anchovy (Engraulis encrasicolus) waste protein hydrolysates in ApoE-deficient mice. Nutrients 13, 2137. doi: 10.3390/nu13072137
Abbate J. M., Macrì F., Capparucci F., Iaria C., Briguglio G., Cicero L., et al. (2020). Administration of protein hydrolysates from anchovy (Engraulis encrasicolus) waste for twelve weeks decreases metabolic dysfunction-associated Fatty liver disease severity in ApoE-/-mice. Anim. (Basel) 10, 2303. doi: 10.3390/ani10122303
Agiadi K., Girone A., Koskeridou E., Moissette P., Cornée J.-J., Quillévéré F. (2018). Pleistocene marine fish invasions and paleoenvironmental reconstructions in the eastern Mediterranean. Quat. Sci. Rev. 196, 80–99. doi: 10.1016/j.quascirev.2018.07.037
Ahmad F., Ayub M. N. A., Mohamad S. N., Ibrahim S. (2018). Proximate and amino acid compositions of selected dried anchovies (Whole and processed) from Pangkor Island, Malaysia. Malaysian Fisheries J. 17, 39–50.
Ahmad R., Shapawi R., Seng L. L., Kian A. Y. S., Tuzan A. D. (2023). Evaluation of Anchovy by-products as an ingredient in the diets developed for Red Hybrid Tilapia (Oreochromis spp.) juveniles. MAbs 52, 37–48. doi: 10.55230/mabjournal.v52i3.2711
Albo-Puigserver M., Navarro J., Coll M., Layman C. A., Palomera I. (2016). Trophic structure of pelagic species in the northwestern Mediterranean Sea. J. Sea Res. 117, 27–35. doi: 10.1016/j.seares.2016.09.003
Alheit J., Pohlmann T., Casini M., Greve W., Hinrichs R., Mathis M., et al. (2012). Climate variability drives anchovies and sardines into the North and Baltic Seas. Prog. Oceanogr. 96, 128–139. doi: 10.1016/j.pocean.2011.11.015
Ashraf S. A., Adnan M., Patel M., Siddiqui A. J., Sachidanandan M., Snoussi M., et al. (2020). Fish-based bioactives as potent nutraceuticals: Exploring the therapeutic perspective of sustainable food from the sea. Mar. Drugs 18, 265. doi: 10.3390/md18050265
Atef M., Mahdi Ojagh S. (2017). Health benefits and food applications of bioactive compounds from fish byproducts: A review. J. Funct. Foods 35, 673–681. doi: 10.1016/j.jff.2017.06.034
Attayde J. L., Hansson L.-A. (2001). The relative importance of fish predation and excretion effects on planktonic communities. Limnol. Oceanogr. 46, 1001–1012. doi: 10.4319/lo.2001.46.5.1001
Awad J. E., Okba Z., Talborjt E. H. A., Ouizgani H. E. (2022). Feeding habit and prey selection of anchovy, Engraulis encrasicolus (Engraulidae), from the Moroccan Atlantic coast. Aquaculture Aquarium Conserv. Legislation 15, 927–940.
Bacha M., Amara R. (2009). Spatial, temporal and ontogenetic variation in diet of anchovy (Engraulis encrasicolus) on the Algerian coast (SW Mediterranean). Estuar. Coast. Shelf Sci. 85, 257–264. doi: 10.1016/j.ecss.2009.08.009
Bachiller E., Albo-Puigserver M., Giménez J., Pennino M. G., Marí-Mena N., Esteban A., et al. (2020). A trophic latitudinal gradient revealed in anchovy and sardine from the Western Mediterranean Sea using a multi-proxy approach. Sci. Rep. 10, 17598. doi: 10.1038/s41598-020-74602-y
Bachiller E., Giménez J., Albo-Puigserver M., Pennino M. G., Marí-Mena N., Esteban A., et al. (2021). Trophic niche overlap between round sardinella (Sardinella aurita) and sympatric pelagic fish species in the Western Mediterranean. Ecol. Evol. 11, 16126–16142. doi: 10.1002/ece3.8293
Bachiller E., Irigoien X. (2015). Trophodynamics and diet overlap of small pelagic fish species in the Bay of Biscay. Mar. Ecol. Prog. Ser. 534, 179–198. doi: 10.3354/meps11375
Bahi A., Ramos-Vega A., Angulo C., Monreal-Escalante E., Guardiola F. A. (2023). Microalgae with immunomodulatory effects on fish. Rev Aquac. 15, 1522–1539. doi: 10.1111/raq.12792
Bakun A. (1997). Patterns in the ocean: ocean processes and marine population dynamics. Oceanographic Literature Rev. 5, 530.
Baldó F., García-Isarch E., Jiménez M. P., Romero Z., Sánchez-Lamadrid A., Catalán I. A. (2006). Spatial and temporal distribution of the early life stages of three commercial fish species in the northeastern shelf of the Gulf of Cádiz. Deep Sea Res. Part 2 Top. Stud. Oceanogr. 53, 1391–1401. doi: 10.1016/j.dsr2.2006.04.004
Baldock D., Charveriat C. (2018). 30x30 Actions for a Sustainable Europe #Think2030 Action Plan (Brussels, Belgium: Institute for European Environmental Policy (IEEP)).
Bang M., Sohn D., Kim J. J., Choi W., Jang C. J., Kim C. (2022). Future changes in the seasonal habitat suitability for anchovy (Engraulis japonicus) in Korean waters projected by a maximum entropy model. Front. Mar. Sci. 9. doi: 10.3389/fmars.2022.922020
Bargelloni L., Alarcon J. A., Alvarez M. C., Penzo E., Magoulas A., Palma J., et al. (2005). The Atlantic–Mediterranean transition: Discordant genetic patterns in two seabream species, Diplodus puntazzo (Cetti) and Diplodus sargus (L.). Mol. Phylogenet. Evol. 36, 523–535. doi: 10.1016/j.ympev.2005.04.017
Basilone G., Ferreri R., Barra M., Bonanno A., Pulizzi M., Gargano A., et al. (2020). Spawning ecology of the European anchovy (Engraulis encrasicolus) in the Strait of Sicily: Linking variations of zooplankton prey, fish density, growth, and reproduction in an upwelling system. Prog. Oceanogr. 184, 102330. doi: 10.1016/j.pocean.2020.102330
Basilone G., Gargano A., Corriero A., Zupa R., Santamaria N., Mangano S., et al. (2018). Liver melanomacrophage centres and CYP1A expression as response biomarkers to environmental pollution in European anchovy (Engraulis encrasicolus) from the western Mediterranean Sea. Mar. pollut. Bull. 131, 197–204. doi: 10.1016/j.marpolbul.2018.04.028
Basilone G., Guisande C., Patti B., Mazzola S., Cuttitta A., Bonanno A., et al. (2006). Effect of habitat conditions on reproduction of the European anchovy (Engraulis encrasicolus) in the Strait of Sicily. Fish. Oceanogr. 15, 271–280. doi: 10.1111/j.1365-2419.2005.00391.x
Bat L., Șahİn F., Satılmıș H. H., Üstün F., Özdemir Z. B., Kıdeys A. E., et al. (2007). The changed ecosystem of the black sea and its impact on anchovy fisheries. J. FisheriesSciences.com 1, 191–227. doi: 10.3153/jfscom.2007024
Baus E., Darrock D. J., Bruford M. W. (2005). Gene-flow patterns in Atlantic and Mediterranean populations of the Lusitanian sea star Asterina gibbosa. Mol. Ecol. 14, 3373–3382. doi: 10.1111/j.1365-294X.2005.02681.x
Bayona L. M., van Leeuwen G., Erol Ö., Swierts T., van der Ent E., de Voogd N. J., et al. (2020). Influence of geographical location on the metabolic production of giant barrel sponges (Xestospongia spp.) revealed by metabolomics tools. ACS Omega 5, 12398–12408. doi: 10.1021/acsomega.0c01151
Behçet R. (2011). Performance and emission study of waste anchovy fish biodiesel in a diesel engine. Fuel Process. Technol. 92, 1187–1194. doi: 10.1016/j.fuproc.2011.01.012
Beheshti Foroutani M., Parrish C. C., Wells J., Taylor R. G., Rise M. L., Shahidi F. (2018). Minimizing marine ingredients in diets of farmed Atlantic salmon (Salmo salar): Effects on growth performance and muscle lipid and fatty acid composition. PloS One 13, e0198538. doi: 10.1371/journal.pone.0198538
Bembo D. G., Carvalho G. R., Cingolani N., Arneri E., Giannetti G., Pitcher T. J. (1996a). Allozymic and morphometric evidence for two stocks of the European anchovy Engraulis encrasicolus in Adriatic waters. Mar. Biol. 126, 529–538. doi: 10.1007/BF00354635
Bembo D. G., Carvalho G. R., Snow M., Cingolani N., Pitcher T. J. (1996b). Stock discrimination among European anchovies, Engraulis encrasicolus, by means of PCR-amplified mitochondrial DNA analysis. Oceanographic Literature Rev. 12, 1274.
Benson A. J., Cox S. P., Cleary J. S. (2015). Evaluating the conservation risks of aggregate harvest management in a spatially-structured herring fishery. Fish. Res. 167, 101–113. doi: 10.1016/j.fishres.2015.02.003
Bernatchez L. (2016). On the maintenance of genetic variation and adaptation to environmental change: considerations from population genomics in fishes. J. Fish Biol. 89, 2519–2556. doi: 10.1111/jfb.13145
Bhatia G., Patterson N., Sankararaman S., Price A. L. (2013). Estimating and interpreting FST: the impact of rare variants. Genome Res. 23, 1514–1521. doi: 10.1101/gr.154831.113
Black C., Chevallier O. P., Haughey S. A., Balog J., Stead S., Pringle S. D., et al. (2017). A real time metabolomic profiling approach to detecting fish fraud using rapid evaporative ionisation mass spectrometry. Metabolomics 13, 153. doi: 10.1007/s11306-017-1291-y
Bloom D. D., Lovejoy N. R. (2012). Molecular phylogenetics reveals a pattern of biome conservatism in New World anchovies (family Engraulidae). J. Evol. Biol. 25, 701–715. doi: 10.1111/j.1420-9101.2012.02464.x
Bloom D. D., Lovejoy N. R. (2017). On the origins of marine-derived freshwater fishes in South America. J. Biogeogr. 44, 1927–1938. doi: 10.1111/jbi.12954
Borges S., Odila J., Voss G., Martins R., Rosa A., Couto J. A., et al. (2023). Fish by-products: A source of enzymes to generate circular bioactive hydrolysates. Molecules 28, 1155. doi: 10.3390/molecules28031155
Borme D., Tirelli V., Brandt S. B., Fonda Umani S., Arneri E. (2009). Diet of Engraulis encrasicolus in the northern Adriatic Sea (Mediterranean): ontogenetic changes and feeding selectivity. Mar. Ecol. Prog. Ser. 392, 193–209. doi: 10.3354/meps08214
Borsa P. (2002). Allozyme, mitochondrial-DNA, and morphometric variability indicate cryptic species of anchovy (Engraulis encrasicolus). Biol. J. Linn. Soc Lond. 75, 261–269. doi: 10.1046/j.1095-8312.2002.00018.x
Borsa P., Collet A., Durand J.-D. (2004). Nuclear-DNA markers confirm the presence of two anchovy species in the Mediterranean. C. R. Biol. 327, 1113–1123. doi: 10.1016/j.crvi.2004.09.003
Bouchenak-Khelladi Y., Durand J.-D., Magoulas A., Borsa P. (2008). Geographic structure of European anchovy: A nuclear-DNA study. J. Sea Res. 59, 269–278. doi: 10.1016/j.seares.2008.03.001
Bruno S. F., Ekorong F. J. A. A., Karkal S. S., Cathrine M. S. B., Kudre T. G. (2019). Green and innovative techniques for recovery of valuable compounds from seafood by-products and discards: A review. Trends Food Sci. Technol. 85, 10–22. doi: 10.1016/j.tifs.2018.12.004
Bryhn A. C., Königson S. J., Lunneryd S.-G., Bergenius M. A. J. (2014). Green lamps as visual stimuli affect the catch efficiency of floating cod (Gadus morhua) pots in the Baltic Sea. Fish. Res. 157, 187–192. doi: 10.1016/j.fishres.2014.04.012
Bundy J. G., Davey M. P., Viant M. R. (2009). Environmental metabolomics: a critical review and future perspectives. Metabolomics 5, 3–21. doi: 10.1007/s11306-008-0152-0
Burbrink F. T., Crother B. I., Murray C. M., Smith B. T., Ruane S., Myers E. A., et al. (2022). Empirical and philosophical problems with the subspecies rank. Ecol. Evol. 12, e9069. doi: 10.1002/ece3.9069
Burgos-Hernández A., Rosas-Burgos E.-C., Martínez M., Nuncio-Jauregui P.-N., Marhuenda F., Kačániová M., et al. (2016). Bioactive fractions from cantabrian anchovy (Engraulis encrarischolus) viscera. Food Sci. Technol. 36, 426–431. doi: 10.1590/1678-457X.10115
Cacho I., Grimalt J. O., Canals M., Sbaffi L., Shackleton N. J., Schönfeld J., et al. (2001). Variability of the western Mediterranean Sea surface temperature during the last 25,000 years and its connection with the Northern Hemisphere climatic changes. Paleoceanography 16 (1), 40–52. doi: 10.1029/2000PA000502
Cadrin S. X. (2020). Defining spatial structure for fishery stock assessment. Fish. Res. 221, 105397. doi: 10.1016/j.fishres.2019.105397
Campo D., Mostarda E., Castriota L., Scarabello M. P., Andaloro F. (2006). Feeding habits of the Atlantic bonito, Sarda sarda (Bloch 1793) in the southern Tyrrhenian sea. Fish. Res. 81, 169–175. doi: 10.1016/j.fishres.2006.07.006
Cardeira M., Bernardo A., Leonardo I. C., Gaspar F. B., Marques M., Melgosa R., et al. (2022). Cosmeceutical potential of extracts derived from fishery industry residues: Sardine wastes and codfish frames. Antioxidants (Basel) 11, 1925. doi: 10.3390/antiox11101925
Cardinale M., Doering-Arjes P., Kastowsky M., Mosegaard H. (2004). Effects of sex, stock, and environment on the shape of known-age Atlantic cod (Gadus morhua) otoliths. Can. J. Fish. Aquat. Sci. 61, 158–167. doi: 10.1139/f03-151
Cardona L., Martínez-Iñigo L., Mateo R., González-Solís J. (2015). The role of sardine as prey for pelagic predators in the western Mediterranean Sea assessed using stable isotopes and fatty acids. Mar. Ecol. Prog. Ser. 531, 1–14. doi: 10.3354/meps11353
Carrera M., Gallardo J. M. (2017). Determination of the geographical origin of all commercial hake species by stable isotope ratio (SIR) analysis. J. Agric. Food Chem. 65, 1070–1077. doi: 10.1021/acs.jafc.6b04972
Casaucao A., González-Ortegón E., Jiménez M. P., Teles-MaChado A., Plecha S., Peliz A. J., et al. (2021). Assessment of the spawning habitat, spatial distribution, and Lagrangian dispersion of the European anchovy (Engraulis encrasicolus) early stages in the Gulf of Cadiz during an apparent anomalous episode in 2016. Sci. Total Environ. 781, 146530. doi: 10.1016/j.scitotenv.2021.146530
Casey J., Jardim E., Martinsohn J. T. (2016). The role of genetics in fisheries management under the E.U. common fisheries policy. J. Fish Biol. 89, 2755–2767. doi: 10.1111/jfb.13151
Castillo R., Dalla Rosa L., García Diaz W., Madureira L., Gutierrez M., Vásquez L., et al. (2019). Anchovy distribution off Peru in relation to abiotic parameters: A 32-year time series from 1985 to 2017. Fisheries Ocenography 28, 389–401. doi: 10.1111/fog.12419
Catanese G., Di Capua I., Iriondo M., Bonanno A., Estonba A., Procaccini G. (2020). Application of high-throughput single nucleotide polymorphism genotyping for assessing the origin of Engraulis encrasicolus eggs. Aquat. Conserv. 30, 1313–1324. doi: 10.1002/aqc.3321
Catanese G., Montes I., Iriondo M., Estonba A., Iudicone D., Procaccini G. (2016). High resolution SNPs selection in Engraulis encrasicolus through Taqman OpenArray. Fish. Res. 177, 31–38. doi: 10.1016/j.fishres.2016.01.014
Catanese G., Watteaux R., Montes I., Barra M., Rumolo P., Borme D., et al. (2017). Insights on the drivers of genetic divergence in the European anchovy. Sci. Rep. 7, 4180. doi: 10.1038/s41598-017-03926-z
Chashchin A., Shlyakhov V. A., Dubovik V. E., Negoda S. (2015). “Stock assessment of Anchovy (Engraulis encrasicolus) in northern Black Sea and Sea of Azov,” in Progressive Engineering Practices in Marine Resource Management (Hershey, Pennsylvania, USA: IGI Global), 209–243. doi: 10.4018/978-1-4666-8333-4.ch006
Checkley D. M. J. R., Asch R. G., Rykaczewski R. R. (2017). Climate, anchovy, and sardine. Ann. Rev. Mar. Sci. 9, 469–493. doi: 10.1146/annurev-marine-122414-033819
Cheng J., Han Z., Song N., Gao T., Yanagimoto T., Strüssmann C. A. (2018). Effects of Pleistocene glaciation on the phylogeographic and demographic histories of chub mackerel Scomber japonicus in the north-western Pacific. Mar. Freshw. Res. 69, 514–524. doi: 10.1071/MF17099
Cheng F., Wang Q., Maisano Delser P., Li C. (2019). Multiple freshwater invasions of the tapertail anchovy (Clupeiformes: Engraulidae) of the Yangtze River. Ecol. Evol. 9, 12202–12215. doi: 10.1002/ece3.5708
Chesalin M. V., Nikolsky V. N. (2023). A Comparative Morphometric Analysis of the Azov Anchovy (Engraulis encrasicolus maeoticus Pusanov 1926) and the Black Sea Anchovy (E. encrasicolus ponticus Alexandrov 1927) Based on Body Shape. Russ. J. Mar. Biol. 49, 87–96. doi: 10.1134/S1063074023020049
Chouvelon T., Violamer L., Dessier A., Bustamante P., Mornet F., Pignon-Mussaud C., et al. (2015). Small pelagic fish feeding patterns in relation to food resource variability: an isotopic investigation for Sardina pilchardus and Engraulis encrasicolus from the Bay of Biscay (north-east Atlantic). Mar. Biol. 162, 15–37. doi: 10.1007/s00227-014-2577-5
Chung S., Suzaki H., Kasai A., Nakata H. (2015). The response of fish communities to climate and human-induced changes inferred from fishery landings in an enclosed bay. Estuaries Coasts 38, 1365–1375. doi: 10.1007/s12237-014-9884-4
Cingolani N., Giannetti G., Arneri E. (1996). Anchovy fisheries in the Adriatic sea. Scientia Marina 60, 269–277.
Cingolani N., Santojanni A. (2003). AdriaMed training course on data collection and biological sampling system on small pelagics (Ancona, Italy: FAO).
Ciriminna R., Scurria A., Fabiano-Tixier A.-S., Lino C., Avellone G., Chemat F., et al. (2019). Omega-3 extraction from Anchovy fillet leftovers with Limonene: Chemical, economic, and technical aspects. ACS Omega 4, 15359–15363. doi: 10.1021/acsomega.9b01168
Ciriminna L., Signa G., Vaccaro A. M., Visconti G., Mazzola A., Vizzini S. (2021). Turning waste into gold: Sustainable feed made of discards from the food industries promotes gonad development and colouration in the commercial sea urchin Paracentrotus lividus (Lamarck 1816). Aquaculture Rep. 21, 100881. doi: 10.1016/j.aqrep.2021.100881
Collard F., Gilbert B., Compère P., Eppe G., Das K., Jauniaux T., et al. (2017). Microplastics in livers of European anchovies (Engraulis encrasicolus). Environ. pollut. 229, 1000–1005. doi: 10.1016/j.envpol.2017.07.089
Colloca F., Carpentieri P., Balestri E., Ardizzone G. (2010). Food resource partitioning in a Mediterranean demersal fish assemblage: the effect of body size and niche width. Mar. Biol. 157, 565–574. doi: 10.1007/s00227-009-1342-7
Consonni R., Cagliani L. R. (2022). Quality assessment of traditional food by NMR analysis. Food Control 142, 109226. doi: 10.1016/j.foodcont.2022.109226
Cooney R., de Sousa D. B., Fernández-Ríos A., Mellett S., Rowan N., Morse A. P., et al. (2023). A circular economy framework for seafood waste valorisation to meet challenges and opportunities for intensive production and sustainability. J. Clean. Prod. 392, 136283. doi: 10.1016/j.jclepro.2023.136283
Coppola D., Lauritano C., Palma Esposito F., Riccio G., Rizzo C., de Pascale D. (2021). Fish waste: From problem to valuable resource. Mar. Drugs 19, 116. doi: 10.3390/md19020116
Corona O., Cinquanta L., Li Citra C., Mazza F., Ferrantelli V., Cammilleri G., et al. (2022). Evolution of free aminoacids, histamine and volatile compounds in the italian Anchovies (Engraulis encrasicolus L.) sauce at different ripening times. Foods 12, 126. doi: 10.3390/foods12010126
Cortesi F., Mitchell L. J., Tettamanti V., Fogg L. G., de Busserolles F., Cheney K. L., et al. (2020). Visual system diversity in coral reef fishes. Semin. Cell Dev. Biol. 106, 31–42. doi: 10.1016/j.semcdb.2020.06.007
Costalago D., Palomera I. (2014). Feeding of European pilchard (Sardina pilchardus) in the northwestern Mediterranean: From late larvae to adults. Sci. Mar. 78, 41–54. doi: 10.3989/scimar.03898.06D
Cowen R. K., Sponaugle S. (2009). Larval dispersal and marine population connectivity. Ann. Rev. Mar. Sci. 1, 443–466. doi: 10.1146/annurev.marine.010908.163757
Cutarelli A., Galiero G., Capuano F., Corrado F. (2018). Species identification by means of mitochondrial cytochrome B DNA sequencing in processed anchovy, sardine and tuna products. Food Nutr. Sci. 09, 369–375. doi: 10.4236/fns.2018.94029
Cuttitta A., Patti B., Maggio T., Quinci E. M., Pappalardo A. M., Ferrito V., et al. (2015). Larval population structure of Engraulis encrasicolus in the Strait of Sicily as revealed by morphometric and genetic analysis. Fish. Oceanogr. 24, 135–149. doi: 10.1111/fog.12098
Cuttitta A., Quinci E. M., Patti B., Bonomo S., Bonanno A., Musco M., et al. (2016). Different key roles of mesoscale oceanographic structures and ocean bathymetry in shaping larval fish distribution pattern: A case study in Sicilian waters in summer 2009. J. Sea Res. 115, 6–17. doi: 10.1016/j.seares.2016.04.005
Damalas D. (2015). Mission impossible: Discard management plans for the EU Mediterranean fisheries under the reformed Common Fisheries Policy. Fish. Res. 165, 96–99. doi: 10.1016/j.fishres.2015.01.006
D’Anza E., Di Paolo M., Marano M., Alvino S., Danese A., Bruno T., et al. (2023). “Valutazione della contaminazione chimica ambientale e del contenuto di istamina nella produzione di colatura di Alici di Cetara DOP,” in Nuovi approcci della Medicina Veterinaria per uno sviluppo sostenibile di produzioni e consumi alimentari, vol. 39. (Vibo Valentia, Italy: XXXII Convegno Nazionale A.I.V.I).
Da Ros Z., Fanelli E., Cassatella S., Biagiotti I., Canduci G., Menicucci S., et al. (2023). Resource Partitioning among “Ancillary” Pelagic Fishes (Scomber spp., Trachurus spp.) in the Adriatic Sea. Biology 12, 272. doi: 10.3390/biology12020272
Deibel D., Lowen B. (2011). A review of the life cycles and life-history adaptations of pelagic tunicates to environmental conditions. ICES J. Mar. Sci. 69, 358–369. doi: 10.1093/icesjms/fsr159
Dinçer M. T. (2018). An overview of the seafood processing sector in some Mediterranean countries. MedFAR 1, 23–30.
Di Cintio A., Labanchi L., Spagnolo M., Musella G., Romeo T., Garozzo V., et al. (2022). Fishing capacity in Southern Italy: An insight into the status and trends of the Campanian fishing fleet. Regional Stud. Mar. Sci. 49, 102102. doi: 10.1016/j.rsma.2021.102102
Di Franco A., Thiriet P., Di Carlo G., Dimitriadis C., Francour P., Gutiérrez N. L., et al. (2016). Five key attributes can increase marine protected areas performance for small-scale fisheries management. Sci. Rep. 6, 38135. doi: 10.1038/srep38135
Di Natale A. (1995). Driftnets impact on protected species: Observers data from the Italian fleet and proposal for a model to assess the number of cetaceans in the by-catch. ICCAT Collective Volume of Scientific Papers 44, 255–263.
Di Natale A., Mangano A., Maurizi A., Montaldo L. (1995). A review of driftnet catches by the Italian fleet: species composition, observers data and distribution along the net. Collect. Vol. Sci. Pap. ICCAT/Recl. Doc. Sci. CICTA/Colecc. Doc. Cient. CICAA, 226–235.
Dinerstein E., Vynne C., Sala E., Joshi A. R., Fernando S., Lovejoy T. E., et al. (2019). A Global Deal For Nature: Guiding principles, milestones, and targets. Sci. Adv. 5, eaaw2869. doi: 10.1126/sciadv.aaw2869
Di Pinto A., Mottola A., Marchetti P., Savarino A., Tantillo G. (2019). Fraudulent species substitution in e-commerce of protected denomination origin (pdo) products. J. Food Compost. Anal. 79, 143–147. doi: 10.1016/j.jfca.2019.03.018
Doherty P. J., Planes S., Mather P. (1995). Gene flow and larval duration in seven species of fish from the great barrier reef. Ecology 76, 2373–2391. doi: 10.2307/2265814
Domingues V. S., Faria C., Stefanni S., Santos R. S., Brito A., Almada V. C. (2007a). Genetic divergence in the Atlantic-Mediterranean Montagu’s blenny, Coryphoblennius galerita (Linnaeus 1758) revealed by molecular and morphological characters. Mol. Ecol. 16, 3592–3605. doi: 10.1111/j.1365-294X.2007.03405.x
Domingues V. S., Santos R. S., Brito A., Alexandrou M., Almada V. C. (2007b). Mitochondrial and nuclear markers reveal isolation by distance and effects of Pleistocene glaciations in the northeastern Atlantic and Mediterranean populations of the white seabream (Diplodus sargus, L.). J. Exp. Mar. Bio. Ecol. 346, 102–113. doi: 10.1016/j.jembe.2007.03.002
Dulčić J. (1997). Growth of anchovy, Engraulis encrasicolus (L.), larvae in the Northern Adriatic Sea. Fish. Res. 31, 189–195. doi: 10.1016/S0165-7836(97)00030-1
Duran S., Giribet G., Turon X. (2004). Phylogeographical history of the sponge Crambe crambe (Porifera, Poecilosclerida): range expansion and recent invasion of the Macaronesian islands from the Mediterranean Sea. Mol. Ecol. 13, 109–122. doi: 10.1046/j.1365-294X.2003.02022.x
Edwin L. (2019). Surrounding nets and seines: structure, operation and conservation aspects (Kochi, India: ICAR:: Central Institute of Fisheries Technology).
Engelhard G. H., Ellis J. R., Payne M. R., ter Hofstede R., Pinnegar J. K. (2010). Ecotypes as a concept for exploring responses to climate change in fish assemblages. ICES J. Mar. Sci. 68, 580–591. doi: 10.1093/icesjms/fsq183
Erisman B., Heyman W., Kobara S., Ezer T., Pittman S., Aburto-Oropeza O., et al. (2017). Fish spawning aggregations: where well-placed management actions can yield big benefits for fisheries and conservation. Fish Fish 18, 128–144. doi: 10.1111/faf.12132
Essington T. E., Moriarty P. E., Froehlich H. E., Hodgson E. E., Koehn L. E., Oken K. L., et al. (2015). Fishing amplifies forage fish population collapses. Proc. Natl. Acad. Sci. U. S. A. 112, 6648–6652. doi: 10.1073/pnas.1422020112
European Union (2020) Colatura Alici di Cetara EU No: PDO-IT-02440. Available online at: https://eur-lex.europa.eu/legal-content/EN/TXT/PDF/?uri=CELEX:52020XC0622(02)&from=IT.
Evangelisti F., Bellucci A., Sabelli B., Albano P. G. (2017). The periwinkle Echinolittorina punctata (Mollusca: Gastropoda) tracked the warming of the Mediterranean Sea following the Last Glacial Maximum. Mar. Biol. 164, 34. doi: 10.1007/s00227-017-3071-7
Falautano M., Castriota L., Cillari T., Vivona P., Finoia M. G., Andaloro F. (2018). Characterization of artisanal fishery in a coastal area of the Strait of Sicily (Mediterranean Sea): Evaluation of legal and IUU fishing. Ocean Coast. Manag 151, 77–91. doi: 10.1016/j.ocecoaman.2017.10.022
FAO (2018). Overview of food fraud in the fisheries sector (Rome, Italy: Food and Agriculture Organization of the United Nations).
FAO (2020). The State of World Fisheries and Aquaculture 2020: Sustainability in action. (Rome, Italy: Food and Agriculture Organization of the United Nations). doi: 10.4060/ca9229en
FAO (2022a). The State of Mediterranean and Black Sea Fisheries. (Rome, Italy: Food and Agriculture Organization of the United Nations). doi: 10.4060/cc3370en
FAO (2022b). The State of World Fisheries and Aquaculture 2022. Towards Blue Transformation (Rome, Italy: FAO). doi: 10.4060/cc0461en
Farré M., Jha A. N. (2020). “Chapter 9 - Metabolomics effects of nanomaterials: an ecotoxicological perspective,” in Environmental Metabolomics. Eds. Álvarez-Muñoz D., Farré M. (Barcelona, Spain: Elsevier), 259–281. doi: 10.1016/B978-0-12-818196-6.00009-1
Fasulo S., Iacono F., Cappello T., Corsaro C., Maisano M., D’Agata A., et al. (2012). Metabolomic investigation of Mytilus galloprovincialis (Lamarck 1819) caged in aquatic environments. Ecotoxicol. Environ. Saf. 84, 139–146. doi: 10.1016/j.ecoenv.2012.07.001
Feidantsis K., Gkafas G. A., Exadactylos A., Michaelidis B., Staikou A., Hatziioannou M., et al. (2022). Different interspecies demographic histories within the same locality: A case study of sea cucumbers, cuttlefish and clams in greek waters. Sustainability 14, 14380. doi: 10.3390/su142114380
Fernández-Corredor E., Albo-Puigserver M., Pennino M. G., Bellido J. M., Coll M. (2021). Influence of environmental factors on different life stages of European anchovy (Engraulis encrasicolus) and European sardine (Sardina pilchardus) from the Mediterranean Sea: A literature review. Regional Stud. Mar. Sci. 41, 101606. doi: 10.1016/j.rsma.2020.101606
Ferretti M., Mangano A., Manzueto L., Palladino S., Tarulli E. (1995). Le reti derivanti (Roma Italy: ICRAM).
Ferretti M., Tarulli E., Palladino A. (2002) Classificazione e descrizione degli attrezzi da pesca in uso nelle marinerie italiane con particolare riferimento al loro impatto ambientale. Available online at: https://www.isprambiente.gov.it/contentfiles/00010100/10119-icram-vol3.pdf.
Fire S. E., Wang Z., Berman M., Langlois G. W., Morton S. L., Sekula-Wood E., et al. (2010). Trophic transfer of the harmful algal toxin domoic acid as a cause of death in a minke whale (Balaenoptera acutorostrata) stranding in Southern California. Aquat. Mamm. 36, 342–350. doi: 10.1578/AM.36.4.2010.342
Fitzpatrick C., McLean D., Harvey E. S. (2013). Using artificial illumination to survey nocturnal reef fish. Fish. Res. 146, 41–50. doi: 10.1016/j.fishres.2013.03.016
Fletcher N., Batjakas I. E., Pierce G. J. (2013). Diet of the Atlantic bonito Sarda sarda (Bloch 1793) in the Northeast Aegean Sea. J. Appl. Ichthyol. 29, 1030–1035. doi: 10.1111/jai.12164
Foll M., Gaggiotti O. (2006). Identifying the environmental factors that determine the genetic structure of populations. Genetics 174, 875–891. doi: 10.1534/genetics.106.059451
Fujita T., Yamamoto M., Kono N., Tomiyama T., Sugimatsu K., Yoneda M. (2021). Temporal variations in hatch date and early survival of Japanese anchovy (Engraulis japonicus) in response to environmental factors in the central Seto Inland Sea, Japan. Fish. Oceanogr. 30, 527–541. doi: 10.1111/fog.12535
Gaines S. D., Lafferty K. D. (2020). “Modeling the dynamics of marine species: the importance of incorporating larval dispersal,” in Ecology of Marine Invertebrate Larvae. Ed. McEdward L. (London, UK: Taylor and Francis Group), 24. doi: 10.1201/9780138758950
Gencbay G., Turhan S. (2016). Proximate composition and nutritional profile of the Black Sea Anchovy (Engraulis encrasicholus) whole fish, fillets, and by-products. J. Aquat. Food Prod. Technol. 25, 864–874. doi: 10.1080/10498850.2014.945199
Giannetto A., Esposito E., Lanza M., Oliva S., Riolo K., Di Pietro S., et al. (2020). Protein hydrolysates from Anchovy (Engraulis encrasicolus) waste: In vitro and in vivo biological activities. Mar. Drugs 18, 86. doi: 10.3390/md18020086
Giannoulaki M., Iglesias M., Tugores M. P., Bonanno A., Patti B., de Felice A., et al. (2013). Characterizing the potential habitat of European anchovy Engraulis encrasicolus in the Mediterranean Sea, at different life stages. Fish. Oceanogr. 22, 69–89. doi: 10.1111/fog.12005
Gibson R. N., Atkinson R. J. A. (2016). “Anchovy and sardine in the Adriatic sea - an ecological review,” in Oceanography and Marine Biology (London UK: CRC Press), 221–268. doi: 10.1201/9781420094220-8
Giráldez A. (2021). “Small pelagic resources: A historic perspective and current state of the resources,” in Alboran Sea - Ecosystems and Marine Resources. Eds. Báez J. C., Vázquez J.-T., Camiñas J. A., Idrissi M.M. (Springer International Publishing, Cham), 559–576. doi: 10.1007/978-3-030-65516-7_16
Girard S. (2022). Can Geographical Indications promote sustainable shellfish farming? The example of Bay of Mont-Saint-Michel mussels. Mar. Policy 135, 104845. doi: 10.1016/j.marpol.2021.104845
Giusti A., Tinacci L., Sotelo C. G., Acutis P. L., Ielasi N., Armani A. (2019). Authentication of ready-to-eat anchovy products sold on the Italian market by BLAST analysis of a highly informative cytochrome b gene fragment. Food Control 97, 50–57. doi: 10.1016/j.foodcont.2018.10.018
Gkafas G. A., Hatziioannou M., Malandrakis E. E., Tsigenopoulos C. S., Karapanagiotidis I. T., Mente E., et al. (2019). Heterozygosity fitness correlations and generation interval of the Norway lobster in the Aegean Sea, eastern Mediterranean. J. Biol. Res. 26, 14. doi: 10.1186/s40709-019-0103-0
Gkafas G. A., Sarantopoulou J., Apostologamvrou C., Antoniadou C., Exadactylos A., Fleris G., et al. (2023). Admixture of holothurian species in the hellenic seas (Eastern mediterranean) as revealed by RADseq. sustainability 15, 11493. doi: 10.3390/su151511493
Glaser S. M. (2011). Do albacore exert top-down pressure on northern anchovy? Estimating anchovy mortality as a result of predation by juvenile north pacific albacore in the California current system. Fish. Oceanogr. 20, 242–257. doi: 10.1111/fog.2011.20.issue-3
Goode K. L., Dunphy B. J., Parsons D. M. (2020). Environmental metabolomics as an ecological indicator: Metabolite profiles in juvenile fish discriminate sites with different nursery habitat qualities. Ecol. Indic. 115, 106361. doi: 10.1016/j.ecolind.2020.106361
Gorbi S., Giuliani M. E., Pittura L., d’Errico G., Terlizzi A., Felline S., et al. (2014). Could molecular effects of Caulerpa racemosa metabolites modulate the impact on fish populations of Diplodus sargus? Mar. Environ. Res. 96, 2–11. doi: 10.1016/j.marenvres.2014.01.010
Gordina A. D., Nikolskiy V. N., Niermann U., Bingel F., Subbotin A. A. (1997). New data on the morphological differences of anchovy eggs (Engraulis encrasicolus) in the Black Sea. Fish. Res. 31, 139–145. doi: 10.1016/S0165-7836(96)00563-2
Gücü A. C., Genç Y., Dağtekin M., Sakınan S., Ak O., Ok M., et al. (2017). On black sea anchovy and its fishery. Rev. Fisheries Sci. Aquaculture 25, 230–244. doi: 10.1080/23308249.2016.1276152
Guraslan C., Fach B. A., Oguz T. (2017). Understanding the impact of environmental variability on anchovy overwintering migration in the Black Sea and its implications for the fishing industry. Front. Mar. Sci. 4. doi: 10.3389/fmars.2017.00275
He S., Wang F., Ning Z., Yang B., Wang Y. (2014). Preparation of anchovy (Engraulis japonicus) protein hydrolysates with high free radical-scavenging activity using endogenous and commercial enzymes. Food Sci. Technol. Int. 20, 567–578. doi: 10.1177/1082013213496418
Heal K. R., Durham B. P., Boysen A. K., Carlson L. T., Qin W., Ribalet F., et al. (2021). Marine community metabolomes carry fingerprints of phytoplankton community composition. mSystems 6, e01334–20. doi: 10.1128/mSystems.01334-20
Henriques A., Vázquez J. A., Valcarcel J., Mendes R., Bandarra N. M., Pires C. (2021). Characterization of protein hydrolysates from fish discards and by-products from the North-West Spain fishing fleet as potential sources of bioactive peptides. Mar. Drugs 19, 338. doi: 10.3390/md19060338
Hermoso V., Carvalho S. B., Giakoumi S., Goldsborough D., Katsanevakis S., Leontiou S., et al. (2022). The EU Biodiversity Strategy for 2030: Opportunities and challenges on the path towards biodiversity recovery. Environ. Sci. Policy 127, 263–271. doi: 10.1016/j.envsci.2021.10.028
Huret M., Lebigre C., Iriondo M., Montes I., Estonba A. (2020). Genetic population structure of anchovy (Engraulis encrasicolus) in North-western Europe and variability in the seasonal distribution of the stocks. Fish. Res. 229, 105619. doi: 10.1016/j.fishres.2020.105619
Ideia P., Pinto J., Ferreira R., Figueiredo L., Spínola V., Castilho P. C. (2020). Fish processing industry residues: A review of valuable products extraction and characterization methods. Waste Biomass Valorization 11, 3223–3246. doi: 10.1007/s12649-019-00739-1
Imsiridou A., Karnezi S., Minos G., Exadactylos A. (2021). Comparison of European sardine (Sardina pilchardus, Walbaum 1792) Greek haplotypes with those found in the global distribution of the species. Appl. Ecol. Environ. Res. 19, 4025–4035. doi: 10.15666/aeer/1905_40254035
Ivanišević J., Thomas O. P., Lejeusne C., Chevaldonné P., Pérez T. (2011). Metabolic fingerprinting as an indicator of biodiversity: towards understanding inter-specific relationships among Homoscleromorpha sponges. Metabolomics 7, 289–304. doi: 10.1007/s11306-010-0239-2
Ivanova P. P., Dobrovolov I. S., Bat L., Kideys A. E., Nikolsky V. (2013). Application of esterase polymorphism to specify population genetic structure of Engraulis encrasicolus. Mors’kyi Ekolohichnyi Zhurnal. Sevastopol volume 12, 45–52.
Jacquard A. (2012). The Genetic Structure of Populations (Berlin, Germany: Springer Science & Business Media).
James A. G. (1987). Feeding ecology, diet and field-based studies on feeding selectivity of the Cape anchovy Engraulis capensis. S. Afr. J. Mar. Sci./S.-Afr. Tydskr. Seewet. 5, 673–692. doi: 10.2989/025776187784522784
James A. G., Probyn T., Seiderer L. J. (1989). Nitrogen excretion and absorption efficiencies of the Cape anchovy Engraulis capensis Gilchrist fed upon a variety of plankton diets. J. Exp. Mar. Bio. Ecol. 131, 101–124. doi: 10.1016/0022-0981(89)90002-6
Jemaa S., Bacha M., Khalaf G., Amara R. (2015). Evidence for population complexity of the European anchovy (Engraulis encrasicolus) along its distributional range. Fish. Res. 168, 109–116. doi: 10.1016/j.fishres.2015.04.004
Jones O. A. H., Maguire M. L., Griffin J. L., Dias D. A., Spurgeon D. J., Svendsen C. (2013). Metabolomics and its use in ecology. Austral Ecol. 38, 713–720. doi: 10.1111/aec.2013.38.issue-6
Jorde P. E., Elin S. A., Espeland S. H., Sodeland M., Knutsen H. (2018). Can we rely on selected genetic markers for population identification? Evidence from coastal Atlantic cod. Ecol. Evol. 8, 12547–12558. doi: 10.1002/ece3.4648
Kada O., Abdellaoui S., Driss R. M. N. (2009). Contribution à l’identification et à la caractérisation biologique et dynamique de l’anchois de la lagune de Nador (Maroc). Bull. l’Institut Scientifique (Rabat) Sect Scince la Vie 31, 91–98.
Khiari Z. (2022). Sustainable upcycling of fisheries and aquaculture wastes using fish-derived cold-adapted proteases. Front. Nutr. 9. doi: 10.3389/fnut.2022.875697
Kideys A. E. (1994). Recent dramatic changes in the Black Sea ecosystem: The reason for the sharp decline in Turkish anchovy fisheries. J. Mar. Syst. 5, 171–181. doi: 10.1016/0924-7963(94)90030-2
Klanjšček J., Legović T. (2007). Is anchovy (Engraulis encrasicolus, L.) overfished in the Adriatic Sea? Ecol. Modell. 201, 312–316. doi: 10.1016/j.ecolmodel.2006.09.020
Koslow J. A. (1981). Feeding selectivity of schools of northern Anchovy, Engraulis mordax, in the southern california bight. Fish. Bull. 79, 131–142.
Lafuente J. G., García A., Mazzola S., Quintanilla L., Delgado J., Cuttita A., et al. (2002). Hydrographic phenomena influencing early life stages of the Sicilian Channel anchovy. Fish. Oceanogr. 11, 31–44. doi: 10.1046/j.1365-2419.2002.00186.x
Lafuente J. G., Vargas J. M., Criado F., Garcia A., Delgado J., Mazzola S. (2005). Assessing the variability of hydrographic processes influencing the life cycle of the Sicilian Channel anchovy, Engraulis encrasicolus, by satellite imagery. Fish. Oceanogr. 14, 32–46. doi: 10.1111/j.1365-2419.2004.00304.x
Lal J., Deb S., Singh S. K., Biswas P., Debbarma R., Yadav N. K., et al. (2023). Diverse uses of valuable seafood processing industry waste for sustainability: a review. Environ. Sci. pollut. Res. Int. doi: 10.1007/s11356-023-28890-2
Lalaleo L., Hidalgo D., Valle M., Calero-Cáceres W., Lamuela-Raventós R. M., Becerra-Martínez E. (2020). Differentiating, evaluating, and classifying three quinoa ecotypes by washing, cooking and germination treatments, using HNMR-based metabolomic approach. Food Chem. 331, 127351. doi: 10.1016/j.foodchem.2020.127351
Landi M., Garoia F., Piccinetti C., Tinti F. (2005). Isolation of polymorphic microsatellite loci from the European anchovy, Engraulis encrasicolus. Mol. Ecol. Notes 5, 266–268. doi: 10.1111/j.1471-8286.2005.00892.x
Lasker R., Zweifel J. R. (1978). “Growth and survival of first-feeding northern anchovy larvae (Engraulis mordax) in patches containing different proportions of large and small prey,” in Spatial pattern in plankton communities. Ed. Steele J. H. (Springer US, Boston, MA), 329–354. doi: 10.1007/978-1-4899-2195-6_13
Lavoué S., Hata H., Halim S. A. A. A., Jamaluddin J. A. F., Ridzwan M. F. B. M., Ratmuangkhwang S., et al. (2022). Species diversity of anchovies (Teleostei: Engraulidae) along the northwest coast of Peninsular Malaysia. Regional Stud. Mar. Sci. 55, 102599. doi: 10.1016/j.rsma.2022.102599
Lavoué S., Miya M., Musikasinthorn P., Chen W., Nishida M. (2013). Mitogenomic evidence for an Indo-West Pacific origin of the Clupeoidei (Teleostei: Clupeiformes). PloS One 8, e56485. doi: 10.1371/journal.pone.0056485
Lawrence S., Elliott C., Huisman W., Dean M., van Ruth S. (2022). The 11 sins of seafood: Assessing a decade of food fraud reports in the global supply chain. Compr. Rev. Food Sci. Food Saf. 21, 3746–3769. doi: 10.1111/1541-4337.12998
Lefebvre K. A., Dovel S. L., Silver M. W. (2001). Tissue distribution and neurotoxic effects of domoic acid in a prominent vector species, the northern anchovy Engraulis mordax. Mar. Biol. 138, 693–700. doi: 10.1007/s002270000509
Leitão F., Alms V., Erzini K. (2014). A multi-model approach to evaluate the role of environmental variability and fishing pressure in sardine fisheries. J. Mar. Syst. 139, 128–138. doi: 10.1016/j.jmarsys.2014.05.013
Lemaire C., Versini J.-J., Bonhomme F. (2005). Maintenance of genetic differentiation across a transition zone in the sea: discordance between nuclear and cytoplasmic markers. J. Evol. Biol. 18, 70–80. doi: 10.1111/j.1420-9101.2004.00828.x
Le Moan A., Gagnaire P.-A., Bonhomme F. (2016). Parallel genetic divergence among coastal-marine ecotype pairs of European anchovy explained by differential introgression after secondary contact. Mol. Ecol. 25, 3187–3202. doi: 10.1111/mec.13627
Levy T., Zupo V., Mutalipassi M., Somma E., Ruocco N., Costantini M., et al. (2021). Protandric transcriptomes to uncover parts of the crustacean sex-differentiation puzzle. Front. Mar. Sci. 8. doi: 10.3389/fmars.2021.745540
Li L., Han C., Dong S., Boyd C. E. (2019). Use of elemental profiling and isotopic signatures to differentiate Pacific white shrimp (Litopenaeus vannamei) from freshwater and seawater culture areas. Food Control 95, 249–256. doi: 10.1016/j.foodcont.2018.08.015
Liao C.-H., Chyn S.-S., Tsai T.-Y., Lu H.-J. (2007). Fishing capacity and conditions of light fishery under different fishing light intensity. J. Fisheries Soc. Taiwan 34, 207–223. doi: 10.29822/JFST.200706.0007
Liedke A. M. R., Bonaldo R. M., Segal B., Ferreira C. E. L., Nunes L. T., Burigo A. P., et al. (2018). Resource partitioning by two syntopic sister species of butterflyfish (Chaetodontidae). J. Mar. Biol. Assoc. U. K. 98, 1767–1773. doi: 10.1017/S0025315417001321
Liu S., Tian Y., Liu Y., Alabia I. D., Cheng J., Ito S. (2023). Development of a prey-predator species distribution model for a large piscivorous fish: A case study for Japanese Spanish mackerel Scomberomorus niphonius and Japanese anchovy. Engraulis japonicus. Deep Sea Res. Part 2 Top. Stud. Oceanogr. 207, 105227. doi: 10.1016/j.dsr2.2022.105227
Liu L., Wu Q., Miao X., Fan T., Meng Z., Chen X., et al. (2022). Study on toxicity effects of environmental pollutants based on metabolomics: A review. Chemosphere 286, 131815. doi: 10.1016/j.chemosphere.2021.131815
Lopes V. M., Costa P. R., Rosa R. (2019). “Effects of harmful algal bloom toxins on marine organisms,” in Ecotoxicology of marine organisms, vol. 47 . Eds. Duarte B., Caçador M. I. V. (Boca Ranton USA: CRC Press). doi: 10.1201/b22000
Lucas C. H., Dawson M. N. (2014). “What are jellyfishes and thaliaceans and why do they bloom? In: Pitt K., Lucas C. (eds) Jellyfish Blooms. Springer, Dordrecht. doi: 10.1007/978-94-007-7015-7_2
Lucchetti A., Carbonara P., Colloca F., Lanteri L., Spedicato M. T., Sartor P. (2017). Small-scale driftnets in the Mediterranean: Technical features, legal constraints and management options for the reduction of protected species bycatch. Ocean Coast. Manage. 135, 43–55. doi: 10.1016/j.ocecoaman.2016.11.002
Ma S., Cheng J., Li J., Liu Y., Wan R., Tian Y. (2019). Interannual to decadal variability in the catches of small pelagic fishes from China Seas and its responses to climatic regime shifts. Deep Sea Res. Part 2 Top. Stud. Oceanogr. 159, 112–129. doi: 10.1016/j.dsr2.2018.10.005
Macel M., Van Dam N. M., Keurentjes J. J. B. (2010). Metabolomics: the chemistry between ecology and genetics. Mol. Ecol. Resour. 10, 583–593. doi: 10.1111/j.1755-0998.2010.02854.x
Magoulas A., Castilho R., Caetano S., Marcato S., Patarnello T. (2006). Mitochondrial DNA reveals a mosaic pattern of phylogeographical structure in Atlantic and Mediterranean populations of anchovy (Engraulis encrasicolus). Mol. Phylogenet. Evol. 39, 734–746. doi: 10.1016/j.ympev.2006.01.016
Magoulas A., Tsimenides N., Zouros E. (1996). Mitochondrial DNA phylogeny and the reconstruction of the population history of a species: the case of the European anchovy (Engraulis encrasicolus). Mol. Biol. Evol. 13, 178–190. doi: 10.1093/oxfordjournals.molbev.a025554
Malavolti S. (2017) Stock structure, estimation of the biomass, spawning and nursery areas of the anchovy in the Adriatic Sea. Available online at: https://iris.univpm.it/retrieve/e18b8790-9e9e-d302-e053-1705fe0a27c8/Tesi_Malavolti.pdf.
Mangano V., Gervasi T., Rotondo A., De Pasquale P., Dugo G., Macrì F., et al. (2021). Protein hydrolysates from anchovy waste: purification and chemical characterization. Nat. Prod. Res. 35, 399–406. doi: 10.1080/14786419.2019.1634711
Marchesan M., Spoto M., Verginella L., Ferrero E. A. (2005). Behavioural effects of artificial light on fish species of commercial interest. Fish. Res. 73, 171–185. doi: 10.1016/j.fishres.2004.12.009
Marchetti M. D., Jimenez Lugo R. J., Massa Á.E., Czerner M. (2021). Valorization of residues from anchovy (Engraulis anchoita) salting-ripening process: Impact of desalting procedures in retention of biologically-active compounds. LWT 150, 111896. doi: 10.1016/j.lwt.2021.111896
Marti-Quijal F. J., Remize F., Meca G., Ferrer E., Ruiz M.-J., Barba F. J. (2020). Fermentation in fish and by-products processing: an overview of current research and future prospects. Curr. Opin. Food Sci. 31, 9–16. doi: 10.1016/j.cofs.2019.08.001
Maruhenda Egea F. C., Toledo-Guedes K., Sanchez-Jerez P., Ibanco-Cañete R., Uglem I., Saether B.-S. (2015). A metabolomic approach to detect effects of salmon farming on wild saithe (Pollachius virens) populations. J. Agric. Food Chem. 63, 10717–10726. doi: 10.1021/acs.jafc.5b04765
Matsui H., Takayama G., Sakurai Y. (2016). Physiological response of the eye to different colored light-emitting diodes in Japanese flying squid Todarodes pacificus. Fish. Sci. 82, 303–309. doi: 10.1007/s12562-015-0965-5
McKinney G. J., Seeb J. E., Seeb L. W. (2017). Managing mixed-stock fisheries: genotyping multi-SNP haplotypes increases power for genetic stock identification. Can. J. Fish. Aquat. Sci. 74, 429–434. doi: 10.1139/cjfas-2016-0443
Messaoud H., Bouriga N., Yahia M. N. N., Boumaiza M., Faure E., Quignard J. P., et al. (2011). Discrimination de trois populations d’anchois du genre Engraulis (Clupeiforme, Engraulidae) des cotes tunisiennes par analyse de forme des otolithes. Bull. Inst. Natl. Sci. Technol. Mer 38, 21–27.
Messina C. M., Manuguerra S., Arena R., Renda G., Ficano G., Randazzo M., et al (2021). In vitro bioactivity of astaxanthin and peptides from hydrolisates of shrimp (Parapenaeus longirostris) by-products: From the extraction process to biological effect evaluation, as pilot actions for the strategy “from waste to profit”. Mar Drugs. 19, 216. doi: 10.3390/md19040216
Minos G., Loukovitis D. (2019). Can the population structure of three Greek marine species (Sardina pilchardus, Penaeus kerathurus, Mullus barbatus) become a tool for their future characterization as PGI products? J. Nutrition Food Lipid Sci. 2019, 54–83. doi: 10.33513/nfls/1901-08
MIPAAF (2021). Programma nazionale triennale della pesca e dell’acquacoltura 2022-2024. Ital. Ministry Agricultural Alimentary Forestry Policies.
Mohanty I., Tapadar S., Moore S. G., Biggs J. S., Freeman C. J., Gaul D. A., et al. (2021). Presence of Bromotyrosine alkaloids in marine sponges is independent of metabolomic and microbiome architectures. mSystems 6, e01387–20. doi: 10.1128/mSystems.01387-20
Montes I., Conklin D., Albaina A., Creer S., Carvalho G. R., Santos M., et al. (2013). SNP discovery in European anchovy (Engraulis encrasicolus) by high-throughput transcriptome and genome sequencing. PloS One 8, e70051. doi: 10.1371/journal.pone.0070051
Montes I., ZarraonaIndia I., Iriondo M., Grant W. S., Manzano C., Cotano U., et al. (2016). Transcriptome analysis deciphers evolutionary mechanisms underlying genetic differentiation between coastal and offshore anchovy populations in the Bay of Biscay. Mar. Biol. 163, 205. doi: 10.1007/s00227-016-2979-7
Moya Moreira T. F., Gonçalves O. H., Leimann F. V., Ribeiro R. P. (2023). Fish protein hydrolysates: Bioactive properties, encapsulation and new technologies for enhancing peptides bioavailability. Curr. Pharm. Des. 29, 824–836. doi: 10.2174/1381612829666230110141811
Muñoz-Colmenero M., Blanco O., Arias V., Martinez J. L., Garcia-Vazquez E. (2016). DNA authentication of fish products reveals mislabeling associated with seafood processing. Fisheries 41, 128–138. doi: 10.1080/03632415.2015.1132706
Muscolo A., Mauriello F., Marra F., Calabrò P. S., Russo M., Ciriminna R., et al. (2022). AnchoisFert: A new organic fertilizer from fish processing waste for sustainable agriculture. Glob Chall 6, 2100141. doi: 10.1002/gch2.202100141
Mutalipassi M., Esposito R., Ruocco N., Viel T., Costantini M., Zupo V. (2021). Bioactive compounds of nutraceutical value from fishery and aquaculture discards. Foods 10, 1495. doi: 10.3390/foods10071495
Mutalipassi M., Mazzella V., Zupo V. (2019). Ocean acidification influences plant-animal interactions: The effect of Cocconeis scutellum parva on the sex reversal of Hippolyte inermis. PloS One 14, e0218238. doi: 10.1371/journal.pone.0218238
Mutalipassi M., Riccio G., Ruocco N., Galasso C., Zupo V., Greco S. (2022). Editorial: Cyanobacterial and microalgal compounds: Chemical ecology and biotechnological potentials. Front. Mar. Sci. 9. doi: 10.3389/fmars.2022.984160
Nag M., Lahiri D., Dey A., Sarkar T., Pati S., Joshi S., et al. (2022). Seafood discards: A potent source of enzymes and biomacromolecules with nutritional and nutraceutical significance. Front. Nutr. 9. doi: 10.3389/fnut.2022.879929
Nguyen K. Q., Winger P. D. (2019). Artificial light in commercial industrialized fishing applications: A review. Rev. Fisheries Sci. Aquaculture 27, 106–126. doi: 10.1080/23308249.2018.1496065
Norcross B. L., Shaw R. F. (1984). Oceanic and estuarine transport of fish eggs and larvae: A Review. Trans. Am. Fish. Soc 113, 153–165. doi: 10.1577/1548-8659(1984)113<153:OAETOF>2.0.CO;2
Nordahl O., Koch-Schmidt P., Sunde J., Yıldırım Y., Tibblin P., Forsman A., et al. (2019). Genetic differentiation between and within ecotypes of pike (Esox lucius) in the Baltic Sea. Aquat. Conserv. 29, 1923–1935. doi: 10.1002/aqc.3196
Oguz T., Salihoglu B., Fach B. (2008). A coupled plankton–anchovy population dynamics model assessing nonlinear controls of anchovy and gelatinous biomass in the Black Sea. Mar. Ecol. Prog. Ser. 369, 229–256. doi: 10.3354/meps07540
Ospina-Álvarez A., Bernal M., Catalán I. A., Roos D., Bigot J.-L., Palomera I. (2013). Modeling fish egg production and spatial distribution from acoustic data: a step forward into the analysis of recruitment. PloS One 8, e73687. doi: 10.1371/journal.pone.0073687
Ospina-Alvarez A., Catalán I. A., Bernal M., Roos D., Palomera I. (2015). From egg production to recruits: Connectivity and inter-annual variability in the recruitment patterns of European anchovy in the northwestern Mediterranean. Prog. Oceanogr. 138, 431–447. doi: 10.1016/j.pocean.2015.01.011
Ospina-Alvarez A., Parada C., Palomera I. (2012). Vertical migration effects on the dispersion and recruitment of European anchovy larvae: From spawning to nursery areas. Ecol. Modell. 231, 65–79. doi: 10.1016/j.ecolmodel.2012.02.001
Oteiza P., Baldwin M. W. (2021). Evolution of sensory systems. Curr. Opin. Neurobiol. 71, 52–59. doi: 10.1016/j.conb.2021.08.005
Oueslati S., Fadhlaoui-Zid K., Kada O., Augé M. T., Quignard J. P., Bonhomme F. (2014). Existence of two widespread semi-isolated genetic entities within Mediterranean anchovies. Mar. Biol. 161, 1063–1071. doi: 10.1007/s00227-014-2399-5
Ouled-Cheikh J., Giménez J., Albo-Puigserver M., Navarro J., Fernández-Corredor E., Bellido J. M., et al. (2022). Trophic importance of small pelagic fish to marine predators of the Mediterranean Sea. Mar. Ecol. Prog. Ser. 696, 169–184. doi: 10.3354/meps14125
Ovissipour M., Rasco B., Shiroodi S. G., Modanlow M., Gholami S., Nemati M. (2013). Antioxidant activity of protein hydrolysates from whole anchovy sprat (Clupeonella engrauliformis) prepared using endogenous enzymes and commercial proteases. J. Sci. Food Agric. 93, 1718–1726. doi: 10.1002/jsfa.5957
Ozogul F., Cagalj M., Šimat V., Ozogul Y., Tkaczewska J., Hassoun A., et al. (2021). Recent developments in valorisation of bioactive ingredients in discard/seafood processing by-products. Trends Food Sci. Technol. 116, 559–582. doi: 10.1016/j.tifs.2021.08.007
Palomera I., Morales-Nin B., Lleonart J. (1988). Larval growth of anchovy, Engraulis encrasicolus, in the Western Mediterranean Sea. Mar. Biol. 99, 283–291. doi: 10.1007/BF00391991
Paone E., Fazzino F., Pizzone D. M., Scurria A., Pagliaro M., Ciriminna R., et al. (2021). Towards the Anchovy Biorefinery: towards the anchovy biorefinery: Biogas production from anchovy processing waste after fish oil extraction with biobased Limonene. Sustainability 13, 2428. doi: 10.3390/su13052428
Pappalardo A. M., Ferrito V. (2015). A COIBar-RFLP strategy for the rapid detection of Engraulis encrasicolus in processed anchovy products. Food Control 57, 385–392. doi: 10.1016/j.foodcont.2015.03.038
Parada C., Mullon C., Roy C., Fréon P., Hutchings L., van der Lingen C. D. (2008). Does vertical migratory behaviour retain fish larvae onshore in upwelling ecosystems? A modelling study of anchovy in the southern Benguela. Afr. J. Mar. Sci. 30, 437–452. doi: 10.2989/AJMS.2008.30.3.1.635
Patti B., Guisande C., Bonanno A., Basilone G., Cuttitta A., Mazzola S. (2010). Role of physical forcings and nutrient availability on the control of satellite-based chlorophyll a concentration in the coastal upwelling area of the Sicilian Channel. scimar 74, 577–588. doi: 10.3989/scimar.2010.74n3577
Patti B., Torri M., Cuttitta A. (2020). General surface circulation controls the interannual fluctuations of anchovy stock biomass in the Central Mediterranean Sea. Sci. Rep. 10, 1554. doi: 10.1038/s41598-020-58028-0
Pavlov D. S., Kasumyan A. O. (2000). Patterns and mechanisms of schooling behavior in fish: a review. J. Ichthyol. 40, S163.
Petrossian G. A., Pezzella F. S. (2018). IUU Fishing and seafood fraud: Using crime script analysis to inform intervention. Ann. Am. Acad. Pol. Soc Sci. 679, 121–139. doi: 10.1177/0002716218784533
Phillimore A. B., Owens I. P. F. (2006). Are subspecies useful in evolutionary and conservation biology? Proc. Biol. Sci. 273, 1049–1053. doi: 10.1098/rspb.2005.3425
Pike I. H., Jackson A. (2010). Fish oil: production and use now and in the future. Lipid Technol. 22, 59–61. doi: 10.1002/lite.201000003
Pita A., Casey J., Hawkins S. J., Villarreal M. R., Gutiérrez M.-J., Cabral H., et al. (2016). Conceptual and practical advances in fish stock delineation. Fish. Res. 173, 185–193. doi: 10.1016/j.fishres.2015.10.029
Poiesz S. S. H., Witte J. I. J., van der Veer H. W. (2020). Only a few key prey species fuel a temperate coastal fish food web. Mar. Ecol. Prog. Ser. 653, 153–166. doi: 10.3354/meps13472
Politikos D., Somarakis S., Tsiaras K. P., Giannoulaki M., Petihakis G., Machias A., et al. (2015). Simulating anchovy’s full life cycle in the northern Aegean Sea (eastern Mediterranean): A coupled hydro-biogeochemical–IBM model. Prog. Oceanogr. 138, 399–416. doi: 10.1016/j.pocean.2014.09.002
Queirós A. M., Stephens N., Widdicombe S., Tait K., McCoy S. J., Ingels J., et al. (2019). Connected macroalgal-sediment systems: blue carbon and food webs in the deep coastal ocean. Ecol. Monogr. 89, e01366. doi: 10.1002/ecm.1366
Quignard J. P., Hamdouni T., Zaouali J. (1973). Donnees preliminaires sur les caracteres biometriques des anchois Engraulis encrasicolus (Linne 1758) des cotes de Tunisie et du Lac Ichkeul. Rev. Des. Travaux l’Institut Des. Pêches Maritimes 37, 191–196.
Raab K., Llope M., Nagelkerke L. A. J., Rijnsdorp A. D., Teal L. R., Licandro P., et al. (2013). Influence of temperature and food availability on juvenile European anchovy Engraulis encrasicolus at its northern boundary. Mar. Ecol. Prog. Ser. 488, 233–245. doi: 10.3354/meps10408
Racioppo A., Speranza B., Campaniello D., Sinigaglia M., Corbo M. R., Bevilacqua A. (2021). Fish loss/waste and low-value fish challenges: State of art, advances, and perspectives. Foods 10, 2725. doi: 10.3390/foods10112725
Ramírez-Valiente J. A., del Blanco L. S., Alía R., Robledo-Arnuncio J. J., Climent J. (2021). Adaptation of Mediterranean forest species to climate: Lessons from common garden experiments. J. Ecol. doi: 10.1111/1365-2745.13730
Raybaud V., Bacha M., Amara R., Beaugrand G. (2017). Forecasting climate-driven changes in the geographical range of the European anchovy (Engraulis encrasicolus). ICES J. Mar. Sci. 74, 1288–1299. doi: 10.1093/icesjms/fsx003
Rea S., Storani G., Mascaro N., Stocchi R., Loschi A. R. (2009). Species identification in anchovy pastes from the market by PCR-RFLP technique. Food Control 20, 515–520. doi: 10.1016/j.foodcont.2008.08.001
Regner S. (1996). Effects of environmental changes on early stages and reproduction of anchovy in the Adriatic Sea. Sci. Mar. 60, 167–177.
Rocha M., Loiko M. R., Gautério G. V., Tondo E. C., Prentice C. (2013). Influence of heating, protein and glycerol concentrations of film-forming solution on the film properties of Argentine anchovy (Engraulis anchoita) protein isolate. J. Food Eng. 116, 666–673. doi: 10.1016/j.jfoodeng.2013.01.004
Rocha M., Loiko M. R., Tondo E. C., Prentice C. (2014). Physical, mechanical and antimicrobial properties of Argentine anchovy (Engraulis anchoita) protein films incorporated with organic acids. Food Hydrocoll. 37, 213–220. doi: 10.1016/j.foodhyd.2013.10.017
Rocha M., Prietto L., de Souza M. M., Furlong E. B., Prentice C. (2018). Effect of organic acids on physical-mechanical and antifungicidal properties of Anchovy protein films. J. Aquat. Food Prod. Technol. 27, 316–326. doi: 10.1080/10498850.2018.1433736
Rodrigues Freitas I., Cortez-Vega W. R., Prentice C. (2016). Physicochemical and functional properties of protein recovered from fish waste. J. Aquat. Food Prod. Technol. 25, 1034–1044. doi: 10.1080/10498850.2015.1008714
Rodríguez-Ezpeleta N., Díaz-Arce N., Walter J. F. III, Richardson D. E., Rooker J. R., Nøttestad L., et al. (2019). Determining natal origin for improved management of Atlantic bluefin tuna. Front. Ecol. Environ. 17, 439–444. doi: 10.1002/fee.2090
Rodushkin I., Bergman T., Douglas G., Engström E., Sörlin D., Baxter D. C. (2007). Authentication of Kalix (N.E. Sweden) vendace caviar using inductively coupled plasma-based analytical techniques: evaluation of different approaches. Anal. Chim. Acta 583, 310–318. doi: 10.1016/j.aca.2006.10.038
Roveri M., Flecker R., Krijgsman W., Lofi J., Lugli S., Manzi V., et al. (2014). The Messinian Salinity Crisis: Past and future of a great challenge for marine sciences. Mar. Geol. 352, 25–58. doi: 10.1016/j.margeo.2014.02.002
Ruggeri P., Splendiani A., Occhipinti G., Fioravanti T., Santojanni A., Leonori I., et al. (2016). Biocomplexity in populations of European anchovy in the adriatic sea. PloS One 11, e0153061. doi: 10.1371/journal.pone.0153061
Ruiz J., Rincón M. M., Castilla D., Ramos F., del Hoyo J. J. G. (2017). Biological and economic vulnerabilities of fixed TACs in small pelagics: An analysis of the European anchovy (Engraulis encrasicolus) in the Gulf of Cádiz. Mar. Policy 78, 171–180. doi: 10.1016/j.marpol.2017.01.022
Rumolo P., Bonanno A., Barra M., Fanelli E., Calabrò M., Genovese S., et al. (2016). Spatial variations in feeding habits and trophic levels of two small pelagic fish species in the central Mediterranean Sea. Mar. Environ. Res. 115, 65–77. doi: 10.1016/j.marenvres.2016.02.004
Russo T., Pulcinella J., Parisi A., Martinelli M., Belardinelli A., Santojanni A., et al. (2015). Modelling the strategy of mid-water trawlers targeting small pelagic fish in the Adriatic Sea and its drivers. Ecol. Modell. 300, 102–113. doi: 10.1016/j.ecolmodel.2014.12.001
Ryan D., Robards K. (2006). Metabolomics: The greatest omics of them all? Anal. Chem. 78, 7954–7958. doi: 10.1021/ac0614341
Şahin C., Akin S., Hacimurtazaoglu N., Mutlu C., Verep B. (2008). The stock parameter of anchovy (Engraulis encrasicolus) population on the coasts of the eastern Black Sea: reason and implications in declining of anchovy population during the 2004–2005 and 2005–2006 fishing seasons. Fresenius Environ. Bull. 17, 2159–2169.
Sabbah S., Laria R. L., Gray S. M., Hawryshyn C. W. (2010). Functional diversity in the color vision of cichlid fishes. BMC Biol. 8, 133. doi: 10.1186/1741-7007-8-133
Sabelli B., Taviani M. (2014). “The making of the mediterranean molluscan biodiversity,” in The Mediterranean Sea: Its history and present challenges. Eds. Goffredo S., Dubinsky Z. (Springer Netherlands, Dordrecht), 285–306. doi: 10.1007/978-94-007-6704-1_16
Sala A., Lucchetti A., Sartor P. (2018). Technical solutions for European small-scale driftnets. Mar. Policy 94, 247–255. doi: 10.1016/j.marpol.2018.05.019
Santos A., Ricardo F., Domingues M. R. M., Patinha C., Calado R. (2023). Current trends in the traceability of geographic origin and detection of species-mislabeling in marine bivalves. Food Control 152, 109840. doi: 10.1016/j.foodcont.2023.109840
Saraux C., Van Beveren E., Brosset P., Queiros Q., Bourdeix J.-H., Dutto G., et al. (2019). Small pelagic fish dynamics: A review of mechanisms in the Gulf of Lions. Deep Sea Res. Part 2 Top. Stud. Oceanogr. 159, 52–61. doi: 10.1016/j.dsr2.2018.02.010
Sardans J., Gargallo-Garriga A., Urban O., Klem K., Holub P., Janssens I. A., et al. (2021). Ecometabolomics of plant–herbivore and plant–fungi interactions: a synthesis study. Ecosphere 12, e03736. doi: 10.1002/ecs2.3736
Sartor P., Carbonara P., Cerasi S., Garibaldi F., Lanteri L., Lembmbo G., et al. (2015). Identification and characterisation of the small-scale driftnet fisheries in the Italian seas. Biol. Mar. Mediterr. 22, 180.
Sartor P., Carbonara P., Cerasi S., Lembo G., Facchini M. T., Lucchetti A., et al. (2019). A selective and low impacting traditional fishery, sustaining the economy of small coastal villages in central Mediterranean: Keep or replace the small-scale driftnets? Fish. Manage. Ecol. 26, 661–673. doi: 10.1111/fme.12397
Savoca M. S., Tyson C. W., McGill M., Slager C. J. (2017). Odours from marine plastic debris induce food search behaviours in a forage fish. Proc. Biol. Sci. 284, 20171000. doi: 10.1098/rspb.2017.1000
Scholin C. A., Gulland F., Doucette G. J., Benson S., Busman M., Chavez F. P., et al. (2000). Mortality of sea lions along the central California coast linked to a toxic diatom bloom. Nature 403, 80–84. doi: 10.1038/47481
Segarra-Medina C., Pascual L. S., Alseekh S., Fernie A. R., Rambla J. L., Gómez-Cadenas A., et al. (2023). Comparison of metabolomic reconfiguration between Columbia and Landsberg ecotypes subjected to the combination of high salinity and increased irradiance. BMC Plant Biol. 23, 406. doi: 10.1186/s12870-023-04404-7
Shekhar S., Mishra D., Gayali S., Buragohain A. K., Chakraborty S., Chakraborty N. (2016). Comparison of proteomic and metabolomic profiles of two contrasting ecotypes of sweetpotato (Ipomoea batata L.). J. Proteomics 143, 306–317. doi: 10.1016/j.jprot.2016.03.028
Siahaan E. A., Agusman, Pangestuti R., Shin K.-H., Kim S.-K. (2022). Potential cosmetic active ingredients derived from marine by-products. Mar. Drugs 20, 734. doi: 10.3390/md20120734
Siddik M. A. B., Howieson J., Fotedar R., Partridge G. J. (2021). Enzymatic fish protein hydrolysates in finfish aquaculture: a review. Rev. Aquac. 13, 406–430. doi: 10.1111/raq.12481
Silva A. J., Hellberg R. S., Hanner R. H. (2021). “Chapter 7 - seafood fraud,” in Food Fraud. Eds. Hellberg R. S., Everstine K., Sklare S. A. (Academic Press), 109–137. doi: 10.1016/B978-0-12-817242-1.00008-7
Silva G., Lima F. P., Martel P., Castilho R. (2014). Thermal adaptation and clinal mitochondrial DNA variation of European anchovy. Proc. Biol. Sci. 281, 20141093. doi: 10.1098/rspb.2014.1093
Smith P. E., Horne J. K., Schneider D. C. (2001). Spatial dynamics of anchovy, sardine, and hake pre-recruit stages in the California Current. ICES J. Mar. Sci. 58, 1063–1071. doi: 10.1006/jmsc.2001.1092
Smith R. G., Watts C. A. (2009). Determination of the country of origin of farm-raised shrimp (family Penaeide) using trace metal profiling and multivariate statistics. J. Agric. Food Chem. 57, 8244–8249. doi: 10.1021/jf901658f
Song R., Jia Z., Shi Q., Wei R., Dong S. (2019). Identification of bioactive peptides from half-fin anchovy (Setipinna taty) hydrolysates and further modification using Maillard reaction to improve antibacterial activities. J. Funct. Foods 58, 161–170. doi: 10.1016/j.jff.2019.05.001
Soto-Oñate D., Lemos-Nobre A. C. (2021). The European Union landing obligation: The compliance problems derived from its multilevel approach. Mar. Policy 132, 104666. doi: 10.1016/j.marpol.2021.104666
Stronen A. V., Norman A. J., Vander Wal E., Paquet P. C. (2022). The relevance of genetic structure in ecotype designation and conservation management. Evol. Appl. 15, 185–202. doi: 10.1111/eva.13339
Sun X., Li L., Pei J., Liu C., Huang L.-F. (2020). Metabolome and transcriptome profiling reveals quality variation and underlying regulation of three ecotypes for Cistanche deserticola. Plant Mol. Biol. 102, 253–269. doi: 10.1007/s11103-019-00944-5
Svedäng H., Stål J., Sterner T., Cardinale M. (2010). Consequences of subpopulation structure on fisheries management: cod (Gadus morhua) in the kattegat and öresund (North sea). Rev. Fish. Sci. 18, 139–150. doi: 10.1080/10641260903511420
Swart B. L., Bester-van der Merwe A. E., Kerwath S. E., Roodt-Wilding R. (2016). Phylogeography of the pelagic fish Seriola lalandi at different scales: confirmation of inter-ocean population structure and evaluation of southern African genetic diversity. Afr. J. Mar. Sci. 38, 513–524. doi: 10.2989/1814232X.2016.1238410
Tang W., Zhang H., Wang L., Qian H., Qi X. (2015). Targeted separation of antibacterial peptide from protein hydrolysate of anchovy cooking wastewater by equilibrium dialysis. Food Chem. 168, 115–123. doi: 10.1016/j.foodchem.2014.07.027
Teodósio M. A., Paris C. B., Wolanski E., Morais P. (2016). Biophysical processes leading to the ingress of temperate fish larvae into estuarine nursery areas: A review. Estuar. Coast. Shelf Sci. 183, 187–202. doi: 10.1016/j.ecss.2016.10.022
Thirukumaran R., Anu Priya V. K., Krishnamoorthy S., Ramakrishnan P., Moses J. A., Anandharamakrishnan C. (2022). Resource recovery from fish waste: Prospects and the usage of intensified extraction technologies. Chemosphere 299, 134361. doi: 10.1016/j.chemosphere.2022.134361
Thoral E., Queiros Q., Roussel D., Dutto G., Gasset E., McKenzie D. J., et al. (2021). Changes in foraging mode caused by a decline in prey size have major bioenergetic consequences for a small pelagic fish. J. Anim. Ecol. 90, 2289–2301. doi: 10.1111/1365-2656.13535
Tine M., Kuhl H., Gagnaire P.-A., Louro B., Desmarais E., Martins R. S. T., et al. (2014). European sea bass genome and its variation provide insights into adaptation to euryhalinity and speciation. Nat. Commun. 5, 5770. doi: 10.1038/ncomms6770
Torri M., Corrado R., Falcini F., Cuttitta A., Palatella L., Lacorata G., et al. (2018). Planktonic stages of small pelagic fishes (Sardinella aurita and Engraulis encrasicolus) in the central Mediterranean Sea: The key role of physical forcings and implications for fisheries management. Prog. Oceanogr. 162, 25–39. doi: 10.1016/j.pocean.2018.02.009
Truelove N. K., Kough A. S., Behringer D. C., Paris C. B., Box S. J., Preziosi R. F., et al. (2017). Biophysical connectivity explains population genetic structure in a highly dispersive marine species. Coral Reefs 36, 233–244. doi: 10.1007/s00338-016-1516-y
Tsagarakis K., Palialexis A., Vassilopoulou V. (2014). Mediterranean fishery discards: review of the existing knowledge. ICES J. Mar. Sci. 71, 1219–1234. doi: 10.1093/icesjms/fst074
Tudela S. (1999). Morphological variability in a Mediterranean, genetically homogeneous population of the European anchovy, Engraulis encrasicolus. Fish. Res. 42, 229–243. doi: 10.1016/S0165-7836(99)00052-1
Tudela S. (2004). Ecosystem effects of fishing in the Mediterranean: An analysis of the major threats of fishing gear and practices to biodiversity and marine habitats (Roma Italy: Food & Agriculture Org).
Tudela S., Palomera I. (1997). A Trophic ecology of the European anchovy Engraulis encrasicolus in the Catalan Sea (northwest Mediterranean). Mar. Ecol. Prog. Ser. 160, 121–134. doi: 10.3354/meps160121
Tudela S., Palomera I., Quílez G. (2002). Feeding of anchovy Engraulis encrasicolus larvae in the north-west Mediterranean. J. Mar. Biol. Assoc. U. K. 82, 349–350. doi: 10.1017/S0025315402005568
Tural S., Turhan S. (2017a). Properties and antioxidant capacity of anchovy (Engraulis encrasicholus) by-product protein films containing thyme essential oil. Food Technol. Biotechnol. 55, 77–85. doi: 10.17113/ftb.55.01.17.4824
Tural S., Turhan S. (2017b). Properties of edible films made from anchovy by-product proteins and determination of optimum protein and glycerol concentration by the TOPSIS method. J. Aquat. Food Prod. Technol. 26, 640–654. doi: 10.1080/10498850.2016.1251998
Tural S., Turhan S., Öz F. (2020). Characterization and antioxidant capacity of anchovy by-product protein films enriched with rosemary and laurel essential oils. Su Urun. Derg./J. Fish. Aquat. Sci. 37, 379–387. doi: 10.12714/egejfas.37.4.09
Turan C., Ergüden D., Gürlek M., Başusta N., Turan F. (2004). Morphometric structuring of the anchovy (Engraulis encrasicolus) in the Black, Aegean and Northeastern Mediterranean seas. Turkish J. Veterinary Anim. Sci. 28, 865–871.
Turan C., Tuncay S. S., Soldo A., Bosnic N., Bardakçi F. (2017). Genetic structure of anchovy Engraulis encrasicolus in the Adriatic Sea using microsatellite DNA analysis. Nat. Eng. Sci. 2, 85–99. doi: 10.28978/nesciences.337793
Uthe H., van Dam N. M., Hervé M. R., Sorokina M., Peters K., Weinhold A. (2021). “Chapter Six - A practical guide to implementing metabolomics in plant ecology and biodiversity research,” in Advances in Botanical Research. Eds. Pétriacq P., Bouchereau A. (Academic Press), 163–203. doi: 10.1016/bs.abr.2020.09.019
Van Beveren E., Bonhommeau S., Fromentin J.-M., Bigot J.-L., Bourdeix J.-H., Brosset P., et al. (2014). Rapid changes in growth, condition, size and age of small pelagic fish in the Mediterranean. Mar. Biol. 161, 1809–1822. doi: 10.1007/s00227-014-2463-1
Varrà M. O., Ghidini S., Ianieri A., Zanardi E. (2021a). Near infrared spectral fingerprinting: A tool against origin-related fraud in the sector of processed anchovies. Food Control 123, 107778. doi: 10.1016/j.foodcont.2020.107778
Varrà M. O., Husáková L., Patočka J., Ghidini S., Zanardi E. (2021b). Classification of transformed anchovy products based on the use of element patterns and decision trees to assess traceability and country of origin labelling. Food Chem. 360, 129790. doi: 10.1016/j.foodchem.2021.129790
Vázquez J. A., Fraguas J., Mirón J., Valcárcel J., Pérez-Martín R. I., Antelo L. T. (2020). Valorisation of fish discards assisted by enzymatic hydrolysis and microbial bioconversion: Lab and pilot plant studies and preliminary sustainability evaluation. J. Clean. Prod. 246, 119027. doi: 10.1016/j.jclepro.2019.119027
Velarde E., Ezcurra E., Anderson D. W. (2015). Seabird diet predicts following-season commercial catch of Gulf of California Pacific Sardine and Northern Anchovy. J. Mar. Syst. 146, 82–88. doi: 10.1016/j.jmarsys.2014.08.014
Velasco A., Aldrey A., Pérez-Martín R. I., Sotelo C. G. (2016). Assessment of the labelling accuracy of spanish semipreserved anchovies products by FINS (forensically informative nucleotide sequencing). Heliyon 2, e00124. doi: 10.1016/j.heliyon.2016.e00124
Venugopal V. (2021). Valorization of seafood processing discards: Bioconversion and bio-refinery approaches. Front. Sustain. Food Syst. 5. doi: 10.3389/fsufs.2021.611835
Vohsen S. A., Fisher C. R., Baums I. B. (2019). Metabolomic richness and fingerprints of deep-sea coral species and populations. Metabolomics 15, 34. doi: 10.1007/s11306-019-1500-y
Wilson A. B., Eigenmann Veraguth I. (2010). The impact of Pleistocene glaciation across the range of a widespread European coastal species. Mol. Ecol. 19, 4535–4553. doi: 10.1111/mec.2010.19.issue-20
Wollrab S., Diehl S., De Roos A. M. (2012). Simple rules describe bottom-up and top-down control in food webs with alternative energy pathways. Ecol. Lett. 15, 935–946. doi: 10.1111/j.1461-0248.2012.01823.x
Yilmaz K., Turhan S., Saricaoglu F. T., Tural S. (2020). Improvement of physicochemical, mechanical, thermal and surface properties of anchovy by-product protein films by addition of transglutaminase, and the correlation between secondary structure and mechanical properties. Food Packag. Shelf Life 24, 100483. doi: 10.1016/j.fpsl.2020.100483
Ying Y., Chen Y., Lin L., Gao T. (2011). Risks of ignoring fish population spatial structure in fisheries management. Can. J. Fish. Aquat. Sci. 68, 2101–2120. doi: 10.1139/f2011-116
Yueqi A., Qiufeng R., Li W., Xuezhen Z., Shanbai X. (2023). Comparison of volatile aroma compounds in commercial surimi and their products from freshwater fish and marine fish and aroma fingerprints establishment based on metabolomics analysis methods. Food Chem. 433, 137308. doi: 10.1016/j.foodchem.2023.137308
Zardoya R., Castilho R., Grande C., Favre-Krey L., Caetano S., Marcato S., et al. (2004). Differential population structuring of two closely related fish species, the mackerel (Scomber scombrus) and the chub mackerel (Scomber japonicus), in the Mediterranean Sea. Mol. Ecol. 13, 1785–1798. doi: 10.1111/j.1365-294X.2004.02198.x
Zarraonaindia I., Iriondo M., Albaina A., Pardo M. A., Manzano C., Grant W. S., et al. (2012). Multiple SNP markers reveal fine-scale population and deep phylogeographic structure in European anchovy (Engraulis encrasicolus L.). PloS One 7, e42201. doi: 10.1371/journal.pone.0042201
Zarraonaindia I., Pardo M. A., Iriondo M., Manzano C., Estonba A. (2009). Microsatellite variability in European anchovy (Engraulis encrasicolus) calls for further investigation of its genetic structure and biogeography. ICES J. Mar. Sci. 66, 2176–2182. doi: 10.1093/icesjms/fsp187
Zhang L.-J., Qian L., Ding L.-Y., Wang L., Wong M. H., Tao H.-C. (2021). Ecological and toxicological assessments of anthropogenic contaminants based on environmental metabolomics. Environ. Sci. Ecotechnol 5, 100081. doi: 10.1016/j.ese.2021.100081
Zhao G., Zhao W., Han L., Ding J., Chang Y. (2020). Metabolomics analysis of sea cucumber (Apostichopus japonicus) in different geographical origins using UPLC-Q-TOF/MS. Food Chem. 333, 127453. doi: 10.1016/j.foodchem.2020.127453
Zlatanos S., Laskaridis K. (2007). Seasonal variation in the fatty acid composition of three Mediterranean fish: sardine (Sardina pilchardus), anchovy (Engraulis encrasicholus) and picarel (Spicara smaris). Food Chem. 103, 725–728. doi: 10.1016/j.foodchem.2006.09.013
Keywords: Engraulis encrasicolus, ecotypes, Mediterranean, menaica, discards, high-value compounds, traceability, trophic ecology
Citation: Mutalipassi M, D’Anza E, Pugliano M, Firmamento R, Murano C, Ruocco N, Pennesi C, Procaccini G, Romeo T, Terlizzi A and Peretti V (2024) Casting light on the European anchovy: from biology to conservation and industry. Front. Ecol. Evol. 12:1352055. doi: 10.3389/fevo.2024.1352055
Received: 07 December 2023; Accepted: 16 February 2024;
Published: 04 March 2024.
Edited by:
Genuario Belmonte, University of Salento, ItalyReviewed by:
Arnold Rakaj, University of Rome Tor Vergata, ItalyAthanasios Exadactylos, University of Thessaly, Greece
Patrícia Jesus Gonçalves, Portuguese Institute for Sea and Atmosphere (IPMA), Portugal
Copyright © 2024 Mutalipassi, D’Anza, Pugliano, Firmamento, Murano, Ruocco, Pennesi, Procaccini, Romeo, Terlizzi and Peretti. This is an open-access article distributed under the terms of the Creative Commons Attribution License (CC BY). The use, distribution or reproduction in other forums is permitted, provided the original author(s) and the copyright owner(s) are credited and that the original publication in this journal is cited, in accordance with accepted academic practice. No use, distribution or reproduction is permitted which does not comply with these terms.
*Correspondence: Antonio Terlizzi, YW50b25pby50ZXJsaXp6aUBzem4uaXQ=; Mirko Mutalipassi, bWlya28ubXV0YWxpcGFzc2lAc3puLml0
†These authors have contributed equally to this work and share first authorship