- 1Department of Geography and Spatial Information Techniques, Ningbo University, Ningbo, China
- 2Zhejiang Collaborative Innovation Center & Ningbo Universities Collaborative Innovation Center for Land and Marine Spatial Utilization and Governance Research, Ningbo University, Ningbo, China
- 3Laboratory of Island Biogeography and Conservation Biology, College of Life Sciences, Nanjing Normal University, Nanjing, China
Insight into the non-random distribution patterns of species in different regions is a foundational aim of research in community ecology and biogeography. The nestedness pattern, which investigates changes in species composition and abundance, has been widely used in numerous studies. However, studies on the nestedness of benthic diatoms are extremely rare, and consequently little has been mentioned of their assemblage mechanisms. To fill this gap, based on 168 benthic diatom species from 147 sampling sites in the Thousand Island Lake (TIL) catchment, we calculated their nestedness and niche width with the aim of i) analyzing the nestedness of benthic diatoms communities with different attachment abilities in TIL; ii) calculating niche width differences between nested and idiosyncratic species with different attachment abilities; iii) investigating the differences in alpha and beta diversity between nested and idiosyncratic sites; iv) examining whether environmental variables influencing the nestedness of benthic diatom communities are dependent on attachment ability. The results demonstrated a significant nestedness pattern in the benthic diatom metacommunity, and the sampling sites of low attachment species not only exhibited a nestedness pattern, but also with a lower nestedness value compared to the sampling sites of all species. Nested and idiosyncratic species differed in niche width, whereas differences between nested and idiosyncratic species of low attachment species were smaller. Additionally, significant differences in alpha and beta diversity were observed between nested and idiosyncratic sites. Furthermore, it was revealed that the nestedness of benthic diatom metacommunity in our study area were mostly influenced by local environmental variables. Our study contributes to the understanding of the significant nestedness observed in benthic diatom metacommunity in TIL, highlighting its relevance to biodiversity conservation efforts.
1 Introduction
It is universally acknowledged that beta diversity patterns consist of two separate components: turnover and nestedness (Harrison et al., 1992; Baselga, 2010). Turnover is mainly caused by the replacement of species between different communities, while nestedness indicates the difference in species richness between communities (Baselga, 2010; Legendre, 2014; Podani, 2016). Advancements in beta diversity measures have further expanded their applications in ecology and conservation biology, such as for biogeographic region delineation (Williams, 1996; Baselga, 2012; Mouillot et al., 2013), protected area siting and protected area network setup (Wiersma and Urban, 2005; Mcknight et al., 2007; Socolar et al., 2016). Hence, understanding the distribution pattern of beta diversity components and its influencing mechanism is of great importance in the fields of ecology, biogeography and conservation biology (Potapova and Charles, 2002; Passy, 2008). Nested structures occur when species-poor assemblages are subsets of species-rich assemblages (Mitsuo et al., 2010). However, an extreme case exists, when communities contain the same number of species but no overlapping species between them, their differences are purely driven by species replacement (Almeida-Neto et al., 2012). At this point, the nestedness pattern calculated by using the multipoint anisotropy index still yields a non-zero result, which is not a nestedness pattern in the strict sense (Almeida-Neto et al., 2012). This should be quantified in terms of nested measures, i.e., evaluated using consistent measures that depend on paired overlap and matrix filling, such as NODF (the Nestedness metric based on Overlap and Decreasing Fill) (Almeida-Neto et al., 2008). Hence, the matrix’s temperature (T) was used to determine the degree of nestedness by measuring how the presence/absence pattern deviates from perfect nestedness (Guimaraesjr and Guimaraes, 2006).
Nestedness patterns have been frequently observed in both metacommunities and networks of interacting species (Bascompte and Jordano, 2007; Soininen, 2008; Tornés and Ruhí, 2013). Nestedness quantifies the overlap in species composition between high and low diversity sites (Atmar and Patterson, 1993) and measures the degree of order in species gain and loss (Almeida-Neto et al., 2008). As a nestedness pattern is an orderly arrangement of species distribution patterns, any factor that facilitates the orderly arrangement of the community will increase the nestedness of the community and vice versa (Baselga, 2012). Nestedness can also be defined as a situation where a relatively large number of idiosyncratic species occupy a subset of locations where a wider range of species are discovered (Almeida-Neto et al., 2008). An important aspect of the nestedness pattern is the extent to which distinct species contribute to achieving perfect nestedness (Heino et al., 2009). Among them idiosyncratic species refer to species that deviate from nestedness patterns and unexpectedly appear in areas where species are scarce and/or abundant (Atmar and Patterson, 1993). Nested and idiosyncratic species often differ in ecology: idiosyncratic groups tend to possess extensive ecological tolerance and efficient transmissibility, whereas nested species are more prone to limited tolerance and restricted transmissibility (Mcabendroth et al., 2005). Combined with previous research, the extent to which species are nested or idiosyncratic is still inadequately comprehended. An intriguing area of research would be to explore the ecological traits that distinguish nested from idiosyncratic species in assemblages (Soininen, 2008).
Diatoms are distributed in almost all types of aquatic ecosystems worldwide, and their species richness is usually the highest among the main producers of aquatic systems (Pan et al., 1999). The distribution pattern of diatoms is often related to various factors, including biogeography, biogeochemistry, human influence and higher trophic levels in stream food webs (Verreydt et al., 2012). Astorga et al. (2012) found that diatoms (efficient passive dispersers) exhibited greater regulation by their environment and less spatial structuring compared to bryophytes (passive dispersers with intermediate dispersal ability) and invertebrates (inefficient active dispersers). Therefore, diatoms are an ideal model taxon for exploring the drives of passively dispersed microbial spatial patterns and determining the ultimate connection between the contributions of individual species to this pattern. The autoecology of particular species may be linked to its spatial structure, because of individual species’ traits that affect their ability to colonize new habitats or their likelihood of extinction (Mcabendroth et al., 2005). In general, diatoms are usually categorized as low attachment, medium attachment and high attachment, and those with higher attachment ability are better adapted to highly disturbed environments compared to others (Passy, 2007; Rimet and Bouchez, 2012; Lange et al., 2015; Wu et al., 2017). Rich and widespread species with large niches and the ability to attach the substrates can support sustained populations in diverse stream habitats (Virtanen et al., 2011). Attached diatom species were, on average, more widely distributed and had wider niche width than non-attached species (Heino and Soininen, 2006). Therefore, robust identification of nested structures in natural communities requires collecting environmental data and functional traits related to their constituent species (Ulrich et al., 2017).
The existence of nestedness patterns is often considered to facilitate the maintenance of species diversity and ensure the stability of network structure (Tornés and Ruhí, 2013). This nestedness pattern has been observed in various taxonomic groups, such as plants, in both terrestrial and aquatic environments (Honnay et al., 1999; Sasaki et al., 2012), birds (Cox and Blake, 1991; Simberloff and Martin, 1991), invertebrates (Mcabendroth et al., 2005), insects (Schouten et al., 2007; Valdovinos et al., 2009), mammals (Fischer et al., 2010) and macroinvertebrate (Ruhi et al., 2013), including diatoms (Soininen, 2008; Tornés and Ruhí, 2013). Currently, research on diatom nestedness patterns has been conducted only in intermittent rivers in the Mediterranean (Tornés and Ruhí, 2013) and in multiple rivers in Finland (Soininen, 2008). Given that previous studies have found freshwater biota to be highly nested in general (Heino, 2011), we conducted this study on the nestedness pattern of benthic diatom metacommunity in the catchment of TIL.
The proper understanding of the relationship between environment and metacommunity is a central theme in ecology (Liu et al., 2023; Wu et al., 2023). Therefore, the general objective of this study was to investigate the nestedness of benthic diatom metacommunity in a large natural catchment. Specifically, we aimed to obtain the environmental variables affecting nestedness when attachment ability varies, and also to obtain the differences between nested and idiosyncratic sampling sites (species). Because low attachment species are more susceptible to passive dispersal and are more abundant in all types of sampling sites, we hypothesized that (i) benthic diatom metacommunity in the TIL shows a clear nestedness pattern, and the degree of nestedness is higher in sampling sites with low attachment species. Previous studies also found that idiosyncratic species have a wider niche width with higher numbers than nested species even in highly nested regions, and the niche width of low attachment species is narrower (Heino and Soininen, 2006), so our hypothesis (ii) was that the difference in niche width between nested and idiosyncratic species is smaller in low attachment species than in high attachment species. We wanted to characterize nested and idiosyncratic sites with regards to their community attributes, so we hypothesized (iii) that idiosyncratic sites tend to have a higher level of species dissimilarity (beta diversity) than nested sites, irrespective of differences in species/functional richness (alpha diversity). If the diatoms have high attachment, they will not susceptible to environmental variables (Moore, 1977; Krejci and Lowe, 1987; Round and Bukhtiyarova, 1996; Passy, 2007). Hence, we hypothesized that (iv) the nestedness of benthic diatom metacommunity in the TIL catchment would be affected by environmental variables, and sampling sites where low attachment species were located would be more affected by environmental variables.
2 Materials and methods
2.1 Study area
Our study was carried out in the catchment of the TIL (Figure 1), located in eastern China. The region has a catchment area of approximately 10,080 km2, with an elevation gradient of over 500 m (Liu et al., 2023; Lin et al., 2024). This region has a humid subtropical monsoonal climate, with an average annual precipitation of 1430 mm. TIL was formed by damming the rivers in the lower reach to build hydroelectric power station in 1959 (Lu and Bao, 2010). In the TIL catchment, the proportion of forest is the highest among all land use types (Supplementary Figure S1). Hence, this catchment is a large near-natural watershed, implying critical relevance to the ecological conservation of the TIL.
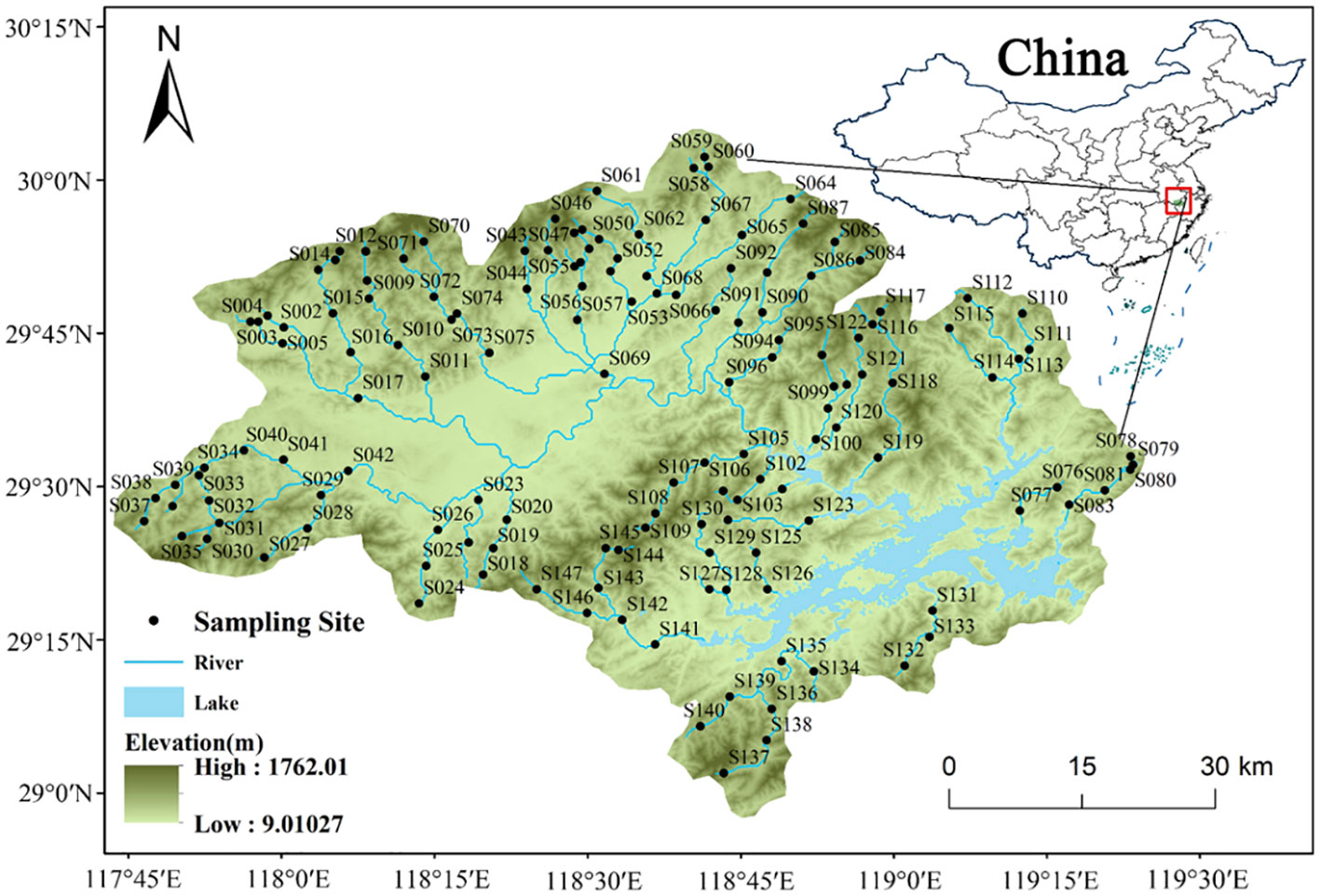
Figure 1 Distribution of the 147 sampling sites within the catchment area of the Thousand Islands Lake (TIL) in China.
2.2 Sampling and identification of benthic diatoms
The 147 sites within the TIL catchment area were surveyed in April and May of 2021. The selection of sampling sites aimed to cover wadable reaches from the headwaters to the larger river in a balanced manner (Liu et al., 2023). To minimize the impact of human activities, such as wastewater outlets, bridges, and villages, the sampling sites were carefully chosen. At each sampling site, we meticulously selected five representative stones from different microhabitats, with an approximate diameter of 6 cm. These stones were then systematically brushed to collect algae samples, specifically diatoms, within the defined sampling area. Subsequently, the stones were thoroughly washed with 380 ml of distilled water in order to obtain the diatom samples for further analysis, and samples were immediately preserved in 5% non-acetic Lugol’s iodine solution and sent to the laboratory for further analysis (Wang et al., 2023). Permanent diatom slides were then prepared in the laboratory using 30% H2O2 (Lane et al., 2003). At least 300 diatom valves were enumerated at 1000x magnification under oiled conditions and diatoms were identified to the lowest taxonomic level (Wang et al., 2022a, Wang et al., 2022b). The attachment ability of diatom species described in Supplementary Table S1, which was judged on the basis of previous studies (Passy, 2007; Rimet and Bouchez, 2012; Lange et al., 2015; Wu et al., 2017).
2.3 Explanatory variables
2.3.1 Local environment and Ions variables
Hydro-morphological parameters were measured in the field, encompassing river width (Width), depth (Depth) and flow velocity (measured with a Global Water Flow Probe FP201). Furthermore, pH, conductivity and water temperature were recorded using a water quality handheld meter (YSI Multiparameter instrument professional plus) (Liu et al., 2023). At each location, water samples were collected and stored at -4°C prior to further analysis in the laboratory. Concentrations of total phosphorus (TP), total nitrogen (TN), chemical oxygen demand (CODMn), nitrate nitrogen (NO3-N), ammonia nitrogen (NH3-N), soluble reactive phosphorus (PO4-P) and different metal ions (e.g., Cu and As) were measured in the laboratory according to the Analytical Methods for Water and Wastewater Monitoring (State Environmental Protection Administration, 2002). Habitat characteristics (including percentage of different hydrologic conditions and substrates) were evaluated according to the Qualitative Habitat Evaluation Index (QHEI), a multi-scale qualitative habitat evaluation index published by the U.S. Environmental Protection Agency in 1989 to measure the physical integrity of streams (Taft and Koncelik, 2006). It considers six factors including substrate, instream cover, channel morphology, riparian zone and bank erosion, pool/glide and riffle/run quality, and gradient, and is a fast, complete, and user-friendly approach (Gazendam et al., 2013). For this reason, we used the QHEI to assess habitat conditions in the study area.
2.3.2 Geo-climatic variables
We classified climate (e.g., annual mean temperature and annual precipitation), land use and topographic data as Geo-climatic variables (Liu et al., 2023). Data on land use were obtained from Yang and Huang (2021). After calculating the percentage of each land-use type in the upstream watershed of each sampling site, the land-use types were reclassified into six categories based on the characteristics of the study area, namely, grassland, forest, cropland, shrub, water surface, and imperviousness (Wu et al., 2021). The topographic variables, including elevation, slope and gradient (Amatulli et al., 2018). The gradient displays the stream’s elevation change over a longitudinal range, and the proportion of the length to the width illustrates the site’s orientation along the north-south and east-west axis (Amatulli et al., 2018). The WorldClim 2 (Fick and Hijmans, 2017) database was utilized to extract nineteen bioclimatic variables (Bio1 to Bio19) for each sampling site. These variables include annual mean temperature, maximum temperature of the warmest month, minimum temperature of the coldest month, and annual precipitation. The data, averaged from 1970 to 2000, were obtained at a spatial resolution of 1-km (Fick and Hijmans, 2017).
2.4 Data analysis
Firstly, we used the software ANINHADO to determine the overall nestedness of the biota matrix, which orders matrices based on the number of occurrences per row and draws a diagonal between species presence and absence (Guimaraesjr and Guimaraes, 2006). ANINHADO computes two nestedness metrics: the classical matrix temperature (T) (Atmar and Patterson, 1993) and the more recently proposed nestedness metric based on overlap and decreasing fill, i.e., NODF (Almeida-Neto et al., 2008). T can be interpreted as a thermodynamic disordered dynamic measurement, ranging from 0° (maximum nestedness) for ordered systems to 100° for disordered systems. We used T to determine whether each location and taxonomic group is nested or idiosyncratic (Tornés and Ruhí, 2013). Idiosyncratic species or sites were considered to be those with T values greater than the original matrix values, and nesting species were considered to be those with T values less than or equal to the original matrix values (Heino et al., 2009). Moreover, the T can be compared with previous studies.
The presence-absence matrix we constructed includes 168 diatom species and 147 sites. We compared nested and idiosyncratic sites for differences in Local environmental variables, Geo-climatic variables, and Ions variables. After that, the data processing was realized in R (Version 4.2.2, R Core Team, 2022). Species richness was calculated in the R package vegan, and functional richness (FRic) was calculated by the “dbFD” function in the R package FD (Laliberté et al., 2014). Taxonomic beta diversity matrices, using the beta.pair function in the R package betapart (Baselga and Orme, 2012); functional beta diversity matrices, in which total beta diversity is divided into turnover and nestedness components using the function functional.beta.pair in the R package betapart. Analysis of variance (ANOVA) was used to test whether alpha and beta diversity differed between nested and idiosyncratic sites (hypothesis iii), with alpha or beta diversity as the dependent variable and nested structure as the fixed factor. Permutational Multivariate Analysis of Variance (PERMANOVA) was conducted using the betadisper function from the vegan package to assess the significance of differences between sites in terms of dispersion. The Tukey’s post hoc test, implemented with the TukeyHSD function from the stats package, was employed to determine specific pairwise differences between groups when significant differences were detected.
In order to measure whether the contribution of each species to nestedness is related to its own ecology (hypothesis ii), we use Levins niche width index to calculate the niche width (using niche.width function in R package spaa) (Zhang and Ma, 2014). This method has been widely used in similar studies in different taxa (Ahmad et al., 2021; De Lima et al., 2023). The niche widths were calculated for all species and their differences between nested and idiosyncratic species were tested using analysis of variance (ANOVA), where niche width was the dependent variable and nesting structure (nested and idiosyncratic species) was the fixed factor. We also analyzed the relationship between river physicochemical factors and nestedness (i.e., environmental variables that triggered low T-values). To examine the main drivers of nested sampling sites’ T-values (hypothesis iv), we applied multiple linear regression models between T-values and environmental variables. Before multivariate linear regression, we tested the linearity and then computed it in R using the construct_fourmula function of the remotes package (Guo et al., 2020). To simplify the model, a stepwise model selection was performed using AICc (function stepAIC in R package MASS) (Venables and Ripley, 2002) and the model with the lowest AICc value was considered the best fit (Zhou et al., 2020).
3 Results
From the 147 sampling sites, a total of 168 species were identified in the benthic diatom communities of the TIL (Wang et al., 2023). Among them, 95 species exhibited low attachment ability, 42 species had medium attachment ability, and 25 species displayed high attachment ability (Supplementary Table S1). The dominant species was Achnanthidium delmontii, while in the upstream the dominant idiosyncratic species was Fragilaria vaucheriae and the dominant idiosyncratic species was Melosira varians in the mid- and downstream sites. Throughout the study area, high attachment species consistently exhibited a higher abundance compared to low attachment species, which in turn surpassed the abundance of medium attachment species (Supplementary Table S2). The observed temperature (Tobs = 13.53) was considerably lower than the value predicted by the ‘fixed-fixed’ null model (Tpre = 51.45), providing evidence for a highly nested metacommunity structure (p < 0.001). This nested structure was further supported by the NODF metric, with the observed global value (NODFobs = 34.08) being much higher compared to the null model prediction (NODFpre = 12.53). Thus, there is a remarkably nestedness pattern of benthic diatom metacommunity in the TIL. Similarly, the sampling sites of low attachment species (ATT_Low) not only exhibited the high nestedness pattern, but also with a lower nested value (6.89) compared to the sampling sites of all species (Figure 2).
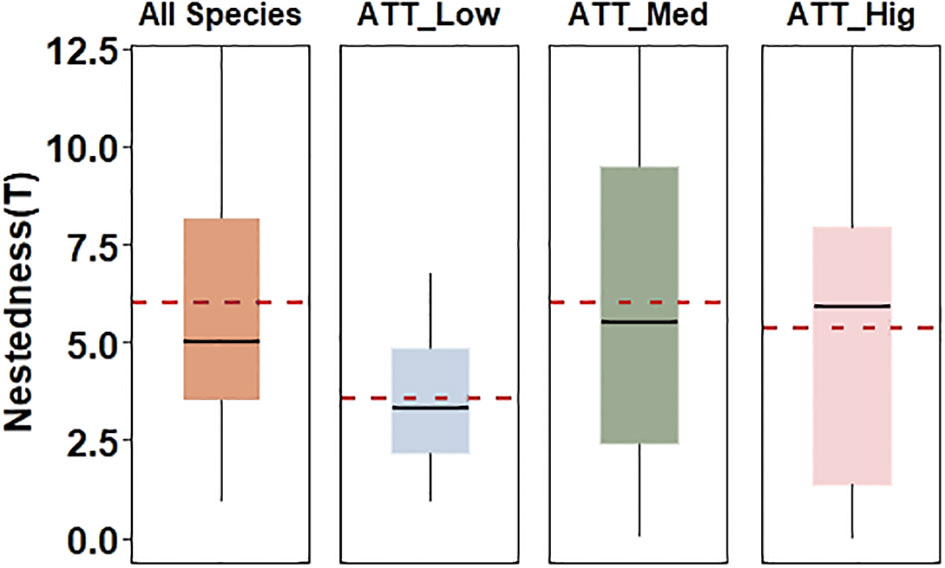
Figure 2 The temperature T of the sampling sites where all species are located is compared with those of sites where species with different attachments are located. All Species represented all sampling sites, ATT_Low, ATT_Med and ATT_Hig represented the sites where low, medium and high attachment species were located, respectively. The boxplots display the median (middle line), 25th and 75th percentiles of the data, with the lower and upper whiskers corresponding the 10th and 90th percentiles. The horizontal red dashed lines represent the mean values.
On the other hand, the sampling sites of medium attachment (ATT_Med) and high attachment (ATT_Hig) species also demonstrated a nestedness pattern but had high nestedness values (TATT_Med = 13.09, TATT_Hig = 13.02) closer to values of TIL. Despite the significant nestedness pattern observed in the benthic diatom communities, a substantial number of idiosyncratic species (54) were found, accounting for 32.00% of the total species (168). The percentage of idiosyncratic species in the upstream was 42.00%, exceeding the 38.00% in the mid- and downstream. The upstream idiosyncratic sampling sites accounted for 43.48%, much higher than the 32.50% in the midstream and 21.05% in the downstream. It is noteworthy that the idiosyncratic species included 37 species which accounted for 39.00% of the species with low attachment ability. While 36.00% of high attachment species were idiosyncratic, 31.00% of medium attachment species were idiosyncratic. In summary, this confirmed our hypothesis (i) that benthic diatom metacommunity in the TIL show a clear nestedness pattern, and the degree of nestedness is higher in sampling sites with low attachment species.
Taxa with different attachment ability showed different patterns of niche widths. Specifically, species with nested structures as well as idiosyncratic species displayed broader niche widths as their species’ attachment ability increased. Furthermore, as the species’ attachment ability increased, both nested and idiosyncratic species demonstrated wider niche widths. However, the niche width of idiosyncratic species was consistently broader than that of nested species (p<0.05), irrespective of species’ attachment ability (Figure 3). The differences in niche width between idiosyncratic and nested species in the sampling sites of low attachment species (ATT_Low) were smaller compared to other attachment species. When considering species occurrence, the species contributing most to the nested structure displayed lower occurrence, whereas idiosyncratic species were more frequently observed across all hydrotypologies (Figure 4). Overall, in combination with species occurrence, idiosyncratic species occur more frequently in all sampling sites and their niche widths are greater. It happens that idiosyncratic species make up a larger proportion of low attachment species, and that low attachment species are themselves vulnerable to dispersal, which effectively proved our hypothesis (ii).
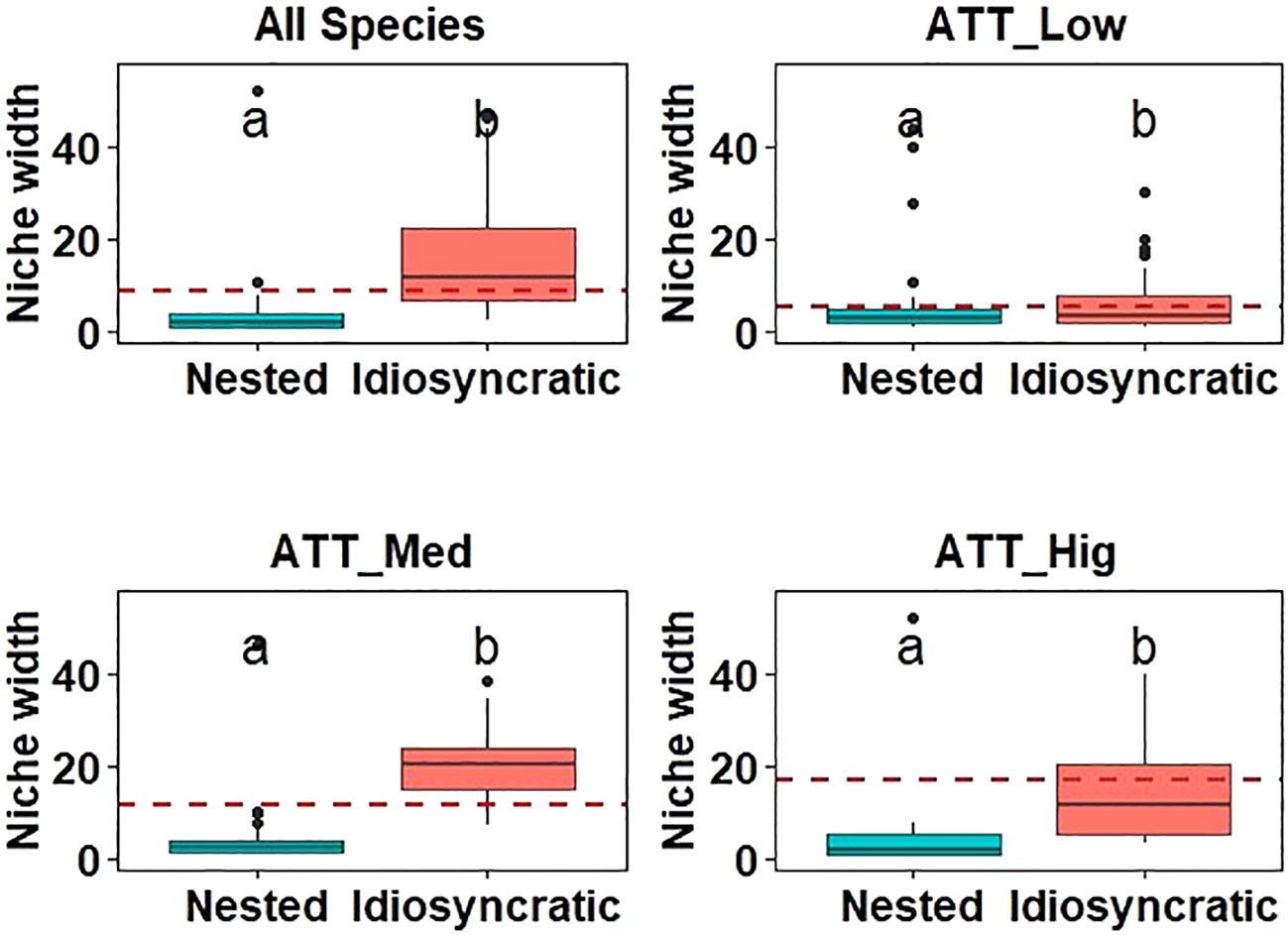
Figure 3 Comparison of niche widths between nested and idiosyncratic sites. The abbreviations are as in Figure 2. ANOVA analysis was performed to compare differences among groups, with different letters above the boxes indicating significant differences (p<0.05).
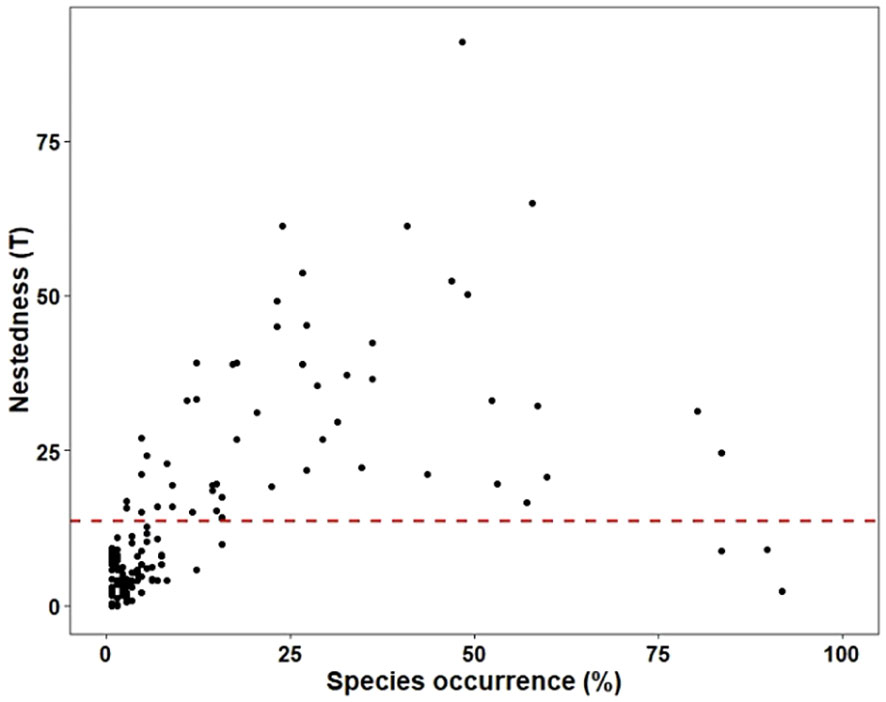
Figure 4 Relationship between nestedness (T) and species occurrence (%). The horizontal red dashed line indicates the observed nestedness (T = 13.53). Species falling below the red line are considered as nested, whereas those above the line are classified as idiosyncratic.
Furthermore, our study revealed notable differences between nested and idiosyncratic sites in terms of species/functional richness (alpha diversity) (Figure 5) and spatial species/functional variations (beta diversity) (Figure 6). The results verified our hypothesis (iii) that idiosyncratic sites, which are characterized by unique and distinct species composition exhibited significant lower species richness (SR) and functional richness (FRic) compared to nested sites. This indicated that idiosyncratic sites had fewer total species and a narrower range of functional traits. Regardless of differences in species/functional richness (alpha diversity), idiosyncratic sites consistently showed higher species dissimilarity (beta diversity) than nested sites. These observations indicated that the spatial heterogeneity of community composition was more prominent in idiosyncratic sites, whereas nested sites displayed a more consistent pattern of species richness across diverse habitats.
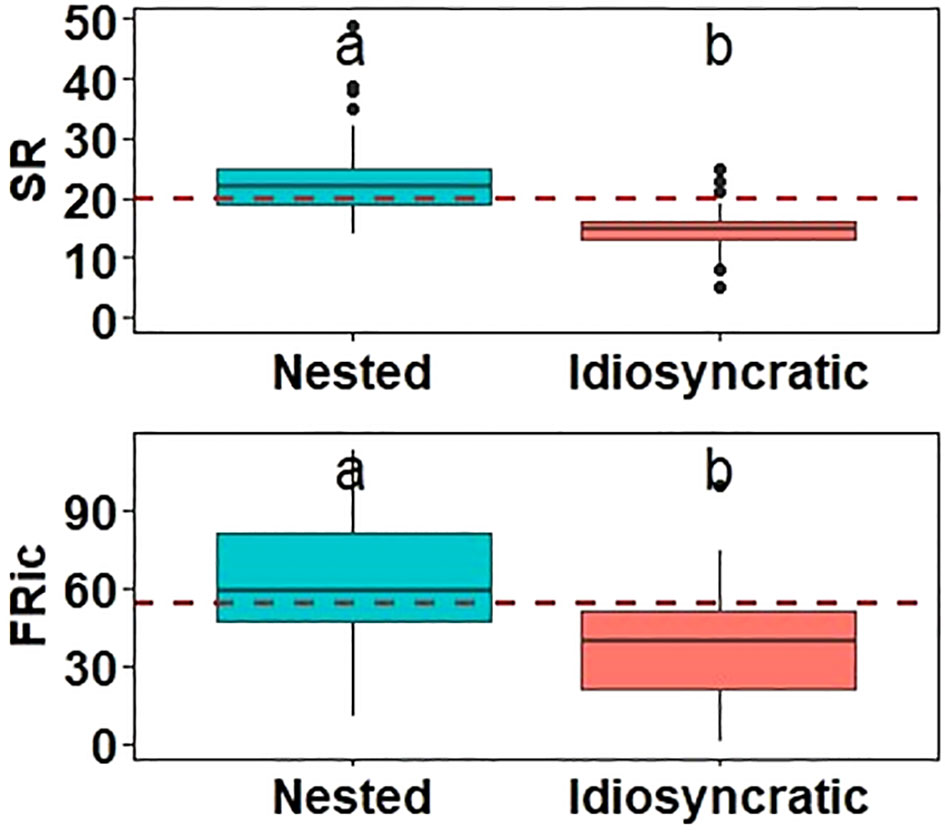
Figure 5 Comparison between taxonomic (species richness, SR) and functional (functional richness, FRic) alpha diversity at nested and idiosyncratic sites. Explanation of boxplot is as in Figure 2, while comparison between groups as in Figure 3, with different letters above the boxes indicating significant differences (p<0.05).
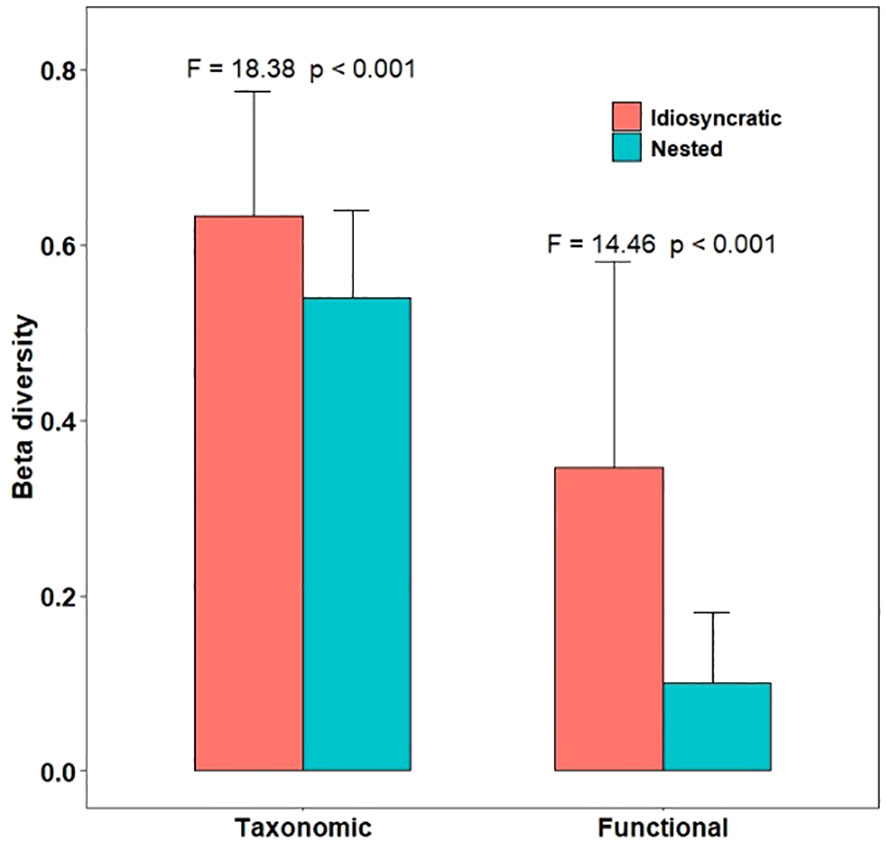
Figure 6 Comparison of taxonomic and functional beta diversity between nested and idiosyncratic sites. F and p values were generated by permutational analysis of multivariate dispersions (PERMDISP).
We found that there were some differences between the idiosyncratic sites, nested sites and the average of all sites (Supplementary Table S3). We analyzed the effects of various environmental factors on nested sites (Figure 7). Among them, PO4-P, As and Elevation had a positive, while Cu and NO3-N had a negative, correlation with the nestedness for nested sites. In parallel, the nestedness of sampling sites with different attachment ability species were also affected by environmental variables to varying degrees. Among them, the nestedness of sampling sites with low attachment ability species (ATT_Low) were affected by more environmental variables, which confirmed our hypothesis (iv). The nestedness of sampling sites with high attachment species (ATT_Hig) were mainly influenced by Depth and NH3-N. However, the nestedness of nested sampling sites with medium attachment species (ATT_Med) were only affected by the Slope.
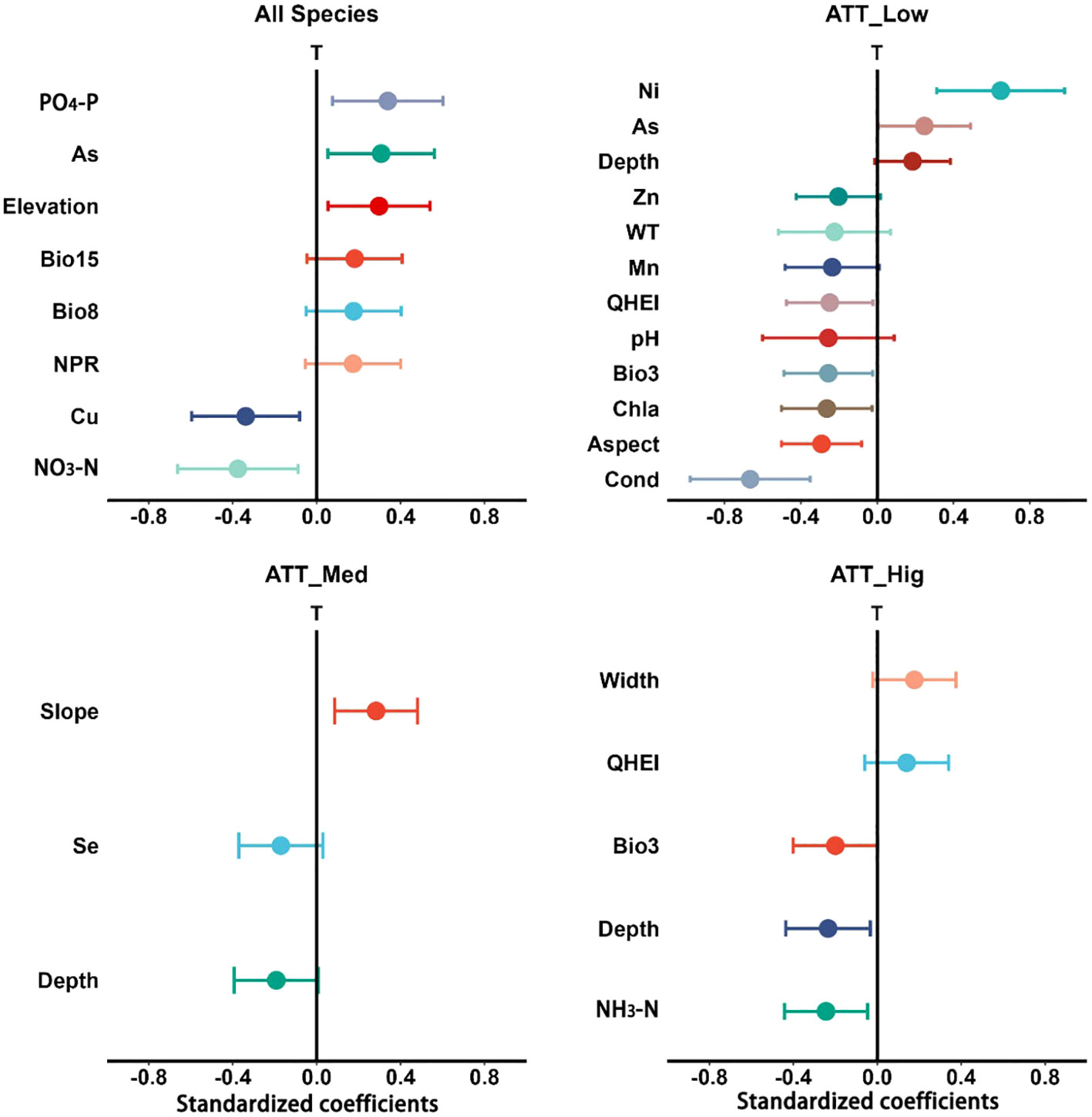
Figure 7 Standardized regression coefficients of model predictors and associated 95% confidence intervals for nestedness (T) at nested sites (n=96). Standardized regression coefficients are generated from model-averaging procedures and are averaged across all of the threshold values. Predictors that have confidence intervals not crossing the zero line are significant (p < 0.05).
4 Discussion
4.1 Relationships between benthic diatom metacommunity nestedness, attachment ability, niche width, and environmental variables
Our study aligned with prior research (Soininen, 2012), suggested that the TIL serves as a natural catchment area where closely attached small pioneer species, such as members of the genus Achnanthidium, often dominate these communities. There was a clear nestedness pattern of benthic diatoms in the TIL, but there was no linear relationship between species attachment ability and nestedness values. Previous studies have found that if the diatom was able to attach to the substrate, it should occur more frequently because the cells were not easily separated from the bottom by the water current (Stevenson et al., 1996). This coincided with the nestedness of the sampling sites with high attachment species, which is higher than that with all species. However, this did not apply to the sampling sites of low attachment species, which can be explained by our results: there was small niche width difference between nested and idiosyncratic species in low attachment species, so there may less competition between species with a higher degree of nestedness. From our study, benthic diatoms in the TIL had the largest proportion of low attachment species and idiosyncratic species, and the proportion of idiosyncratic species among low attachment species was the largest. High occurrence of idiosyncratic species, which represents the high occurrence of low attachment species in all types of sampling sites. Combined with the fact that low attachment species are susceptible to environmental variables, therefore, the susceptibility of low attachment species to passive dispersal is thus the reason for the high nestedness of the sampling sites where they are located. The regional ubiquity and lack of diversity of single-celled organisms (Heino and Soininen, 2005; Soininen and Heino, 2007) may result in less ordered gains and losses of species, with taxa occurring everywhere. Therefore, although significant nestedness may occur in aquatic biota, species with high inter population diffusion rates, effective passive diffusion in the region seems to be logical (Rundle et al., 2002).
Compared with previous studies of benthic diatom nestedness patterns in other regions, we found an interesting phenomenon. In the study carried out in Finland (Soininen, 2008), T was 28.41 and the percentage of idiosyncratic species was 44%; and in the study conducted in the north-east Iberian Peninsula (Tornés and Ruhí, 2013), T was 14.5 and the percentage of idiosyncratic species was 34%; and we found that T was 13.53 and the percentage of idiosyncratic species was 32% in the catchment of TIL, It seems that there is a positive correlation between the frequency of idiosyncratic species occurrence and nestedness. The wider niche width represents the species’ ability to adapt to the rich and diverse resources and complex environmental conditions, with a high degree of adaptability and ecological flexibility (Astorga et al., 2012). The results of hypothesis (ii) showed that niche width of idiosyncratic species was the largest among medium attachment species, but the degree of nestedness was the lowest in the sampling sites where medium attachment species were located. Therefore, when studying nestedness in terms of species’ functional traits, the nestedness value of sampling sites with higher attachment ability species is not necessarily the highest.
Medium attachment species were affected by the lowest number of environmental factors, and the sampling sites with medium attachment species had the lowest level of nestedness compared to the other attachment sampling sites. The gap in niche width between nested and idiosyncratic species was greatest among medium attachment species. In the Niche Variation Hypothesis (Valen, 1965), populations with wider niche widths consist of more ‘specialized’ individuals, indicating increased heterogeneity among individuals. This suggests that individual niche width and specialization can operate concurrently, expanding the population’s niche. Thus, the broader niche width of idiosyncratic species among medium attachment species resulted in a more stable community less affected by environmental variables, although the degree of nestedness in the sampling sites where they were located was low in the TIL. In summary, the relationship between species attachment ability and nestedness is diverse and needs to be considered in the context of niche width and environmental variables.
4.2 Potential influence of spatial process on nestedness patterns of benthic diatoms
The idiosyncrasy is greater in aquatic organisms compared to terrestrial organisms, which may be due to high dispersal ability of aquatic organisms (Tornés and Ruhí, 2013). The numbers of idiosyncratic species and idiosyncratic sites were higher in the upstream than in the midstream and downstream, which coincides with the content of nestedness: the strongest dispersers only occur in a subset of sites where their strict habitat requirements are met (Honnay et al., 1999). It has been demonstrated that the idiosyncratic species have a broader ecological tolerance (Mcabendroth et al., 2005), wider occupancy of sites (Soininen, 2008) and differing relationships to environmental conditions (Malmqvist and Hoffsten, 2000). Combined with our results, it can be surmised that the upstream has more typical environmental conditions suitable for diatom survival. Therefore, the abundance of idiosyncratic species in the upstream may reduce nestedness. However, the complex diversity exhibited in the upstream of TIL highlights the importance of its conservation in future conservation-oriented stream studies (Heino et al., 2009).
Small-bodied organisms in the aquatic organisms are more susceptible to passive dispersion and may exhibit reduced likelihood of displaying spatial patterns in comparison to large-bodied organisms (Hajek et al., 2011; De Bie et al., 2012). Nevertheless, incorporating spatial processes enhances the reliability of bioassessments (Liu et al., 2024), while taking dispersal processes into account improves the accuracy of bioassessments (Leboucher et al., 2021). At smaller spatial scales, our study also observed weak dispersal limitations (Soininen, 2012), with Achnanthidium delmontii being the dominant species throughout the watershed and belonging to nested species. Normally, the downstream dispersal limitations will be less due to better connectivity (Demars and Harper, 2005; Gothe et al., 2017), and even among the idiosyncratic species, the dominant species in both the mid- and downstream was Melosira varians in the TIL. Weak dispersal limitation represents greater ability to exchange individuals between sampling sites, and the more obvious the homogeneity of the communities (Lopes et al., 2014). Therefore, our study suggested that when the spatial scale is smaller, the nestedness of communities may be more pronounced. A higher variety of idiosyncratic species and number of idiosyncratic sampling sites were found in the upstream than in the mid- and downstream. This also verifies that the increased heterogeneity of the upstream environment may promote changes in diatom community composition, which is important for the conservation of regional diversity (Jyrkankallio-Mikkola et al., 2018).
In terms of spatial structure, the absence of a direct source of colonist in the upstream and the limited ability to receive migrants may lead to a high degree of spatial turnover in the species composition of upstream sites (Clarke et al., 2008; Miyasono and Taylor, 2013; Heino et al., 2015). In the context of benthic diatoms, seasonality also plays a significant role in shaping spatial patterns of turnover and nestedness. Therefore, it is crucial for future research to investigate their nestedness patterns across multiple rivers and seasons, aiming to uncover the underlying factors that influence these patterns. Furthermore, studying the functional characteristics of both nested and idiosyncratic species will provide valuable insights into the distribution patterns of diverse biological taxa, as well as the mechanisms driving these patterns in different spatial and temporal dimensions. Overall, the relative influence of environmental and spatial filtering on community structure varies across seasons, and future research on assemblage communities should aim to reveal the mechanisms that influence community assemblages in rivers.
5 Conclusion
Nestedness is the characteristic of metacommunities and is relatively common in aquatic communities. In our study, we found that the benthic diatom assemblage community in the near-natural catchment of the TIL exhibited a distinct nestedness pattern. Idiosyncratic sites exhibited greater spatial heterogeneity in community composition, while nested sites demonstrated a more uniform distribution of species richness across a variety of habitats. Whereas spatially structured upstream areas may possess typical habitats more suitable for different types of diatoms, not only because of the higher environmental heterogeneity in the upstream compared to other river reaches, but also because a higher number of idiosyncratic species was found in the upstream areas. These idiosyncratic species typically had wider niche widths, and the abundance of idiosyncratic species was especially most pronounced in medium attachment species. Overall, our study showed that there are diverse relationships between the attachment ability of species and the nestedness of communities, and that the effects of ecological traits and environmental variables need to be considered together. Our study provides important insights into the intricate patterns of nestedness within benthic diatom communities and underscores the necessity for additional studies to gain a comprehensive understanding of the factors influencing nestedness and community structure in aquatic ecosystems.
Data availability statement
The original contributions presented in the study are included in the article/Supplementary Material, further inquiries can be directed to the corresponding author.
Author contributions
XQ: Writing – original draft. GL: Data curation, Visualization, Writing – review & editing. CC: Software, Visualization, Writing – review & editing. YiW: Formal analysis, Writing – review & editing. YaW: Formal analysis, Writing – review & editing. ZL: Formal analysis, Writing – review & editing. NW: Conceptualization, Methodology, Visualization, Writing – review & editing.
Funding
The author(s) declare financial support was received for the research, authorship, and/or publication of this article. This study was supported by grants from the National Natural Science Foundation of China (No. 52279068, 42350410434).
Acknowledgments
We thank Wanxiang Jiang and colleagues of Anhui Normal University for their field supports. Dr. Fengzhi He helped with land use extraction and Chengxiu Zhan supported with data analysis. Two reviewers gave constructive comments on this manuscript.
Conflict of interest
The authors declare that the research was conducted in the absence of any commercial or financial relationships that could be construed as a potential conflict of interest.
Publisher’s note
All claims expressed in this article are solely those of the authors and do not necessarily represent those of their affiliated organizations, or those of the publisher, the editors and the reviewers. Any product that may be evaluated in this article, or claim that may be made by its manufacturer, is not guaranteed or endorsed by the publisher.
Supplementary material
The Supplementary Material for this article can be found online at: https://www.frontiersin.org/articles/10.3389/fevo.2024.1339946/full#supplementary-material
References
Ahmad M., Sharma P., Rathee S., Singh H. P., Batish D. R., Lone G. R., et al. (2021). Niche width analyses facilitate identification of high-risk endemic species at high altitudes in western Himalayas. Ecol. Indic. 126, 107653. doi: 10.1016/j.ecolind.2021.107653
Almeida-Neto M., Frensel D. M. B., Ulrich W. (2012). Rethinking the relationship between nestedness and beta diversity: a comment on Baselga, (2010). Glob Ecol. Biogeogr. 21, 772–777. doi: 10.1111/j.1466-8238.2011.00709.x
Almeida-Neto M., Guimarães P., Guimarães P. R., Loyola R. D., Ulrich W. (2008). A consistent metric for nestedness analysis in ecological systems: reconciling concept and measurement. Oikos. 117, 1227–1239. doi: 10.1111/j.0030-1299.2008.16644.x
Amatulli G., Domisch S., Tuanmu M. N., Parmentier B., Ranipeta A., Malczyk J., et al. (2018). A suite of global, cross-scale topographic variables for environmental and biodiversity modeling. Sci. Data. 5, 180040. doi: 10.1038/sdata.2018.40
Astorga A., Oksanen J., Luoto M., Soininen J., Virtanen R., Muotka T. (2012). Distance decay of similarity in freshwater communities: do macro- and microorganisms follow the same rules? Glob Ecol. Biogeogr. 21, 365–375. doi: 10.1111/j.1466-8238.2011.00681.x
Atmar W., Patterson B. (1993). The measure of order and disorder in the distribution of species in fragmented habitat. Oecologia. 96, 373–382. doi: 10.1007/BF00317508
Bascompte J., Jordano P. (2007). Plant-animal mutualistic networks: The architecture of biodiversity. Annu. Rev. Ecol. Evol. Syst. 38, 567–593. doi: 10.1146/annurev.ecolsys.38.091206.095818
Baselga A. (2010). Partitioning the turnover and nestedness components of beta diversity. Glob Ecol. Biogeogr. 19, 134–143. doi: 10.1111/j.1466-8238.2009.00490.x
Baselga A. (2012). The relationship between species replacement, dissimilarity derived from nestedness, and nestedness. Glob Ecol. Biogeogr. 21, 1223–1232. doi: 10.1111/j.1466-8238.2011.00756.x
Baselga A., Orme C. D. L. (2012). betapart: an R package for the study of beta diversity. Methods Ecol. Evol. 3, 808–812. doi: 10.1111/j.2041-210X.2012.00224.x
Clarke A., Mac Nally R., Bond N., Lake P. S. (2008). Macroinvertebrate diversity in headwater streams: a review. Freshw. Biol. 53, 1707–1721. doi: 10.1111/j.1365-2427.2008.02041.x
Cox T., Blake S. (1991). Managing cultural diversity: implications for organizational competitiveness. Acad. Manag Perspect. 5, 45–56. doi: 10.5465/ame.1991.4274465
De Bie T., De Meester L., Brendonck L., Martens K., Goddeeris B., Ercken D., et al. (2012). Body size and dispersal mode as key traits determining metacommunity structure of aquatic organisms. Ecol. Lett. 15, 740–747. doi: 10.1111/j.1461-0248.2012.01794.x
De Lima R. C., De Albernaz T. L., Secchi E. R., Cebuhar J. D., Botta S. (2023). Feeding habits of South American and sub-Antarctic fur seals during their nonbreeding season in the southwestern Atlantic Ocean. Mar. Mamm Sci. 40, 26–53. doi: 10.1111/mms.13049
Demars B., Harper D. (2005). Distribution of aquatic vascular plants in lowland rivers: separating the effects of local environmental conditions, longitudinal connectivity and river basin isolation. Freshw. Biol. 50, 418–437. doi: 10.1111/j.1365-2427.2004.01329.x
Fick S. E., Hijmans R. J. (2017). WorldClim 2: new 1-km spatial resolution climate surfaces for global land areas. Int. J. Climatol. 37, 4302–4315. doi: 10.1002/joc.5086
Fischer J., Lindenmayer D. B., Hobbs R. J. (2010). "IV.2 Landscape pattern and biodiversity," in Princeton Guide to Ecology, ed. S. A. Levin. (Princeton University Press). 431–437 p.
Gazendam E., Gharabaghi B., Jones F., Whiteley H. (2013). Evaluation of the qualitative habitat evaluation index as a planning and design tool for restoration of rural ontario waterways. CWRJ/RCRH. 36, 149–158. doi: 10.4296/cwrj3602827
Gothe E., Baattrup-Pedersen A., Wiberg-Larsen P., Graeber D., Kristensen E. A., Friberg N. (2017). Environmental and spatial controls of taxonomic versus trait composition of stream biota. Freshw. Biol. 62, 397–413. doi: 10.1111/fwb.12875
Guimaraesjr P. R., Guimaraes P. (2006). Improving the analyses of nestedness for large sets of matrices. Environ. Model. Software 21, 1512–1513. doi: 10.1016/j.envsoft.2006.04.002
Guo K., Wu N., Manolaki P., Baattrup-Pedersen A., Riis T. (2020). Short-period hydrological regimes override physico-chemical variables in shaping stream diatom traits, biomass and biofilm community functions. Sci. Total Environ. 743, 140720. doi: 10.1016/j.scitotenv.2020.140720
Hajek M., Rolecek J., Cottenie K., Kintrova K., Horsak M., Poulickova A., et al. (2011). Environmental and spatial controls of biotic assemblages in a discrete semi-terrestrial habitat: comparison of organisms with different dispersal abilities sampled in the same plots. J. Biogeogr. 38, 1683–1693. doi: 10.1111/jbi.2011.38.issue-9
Harrison S., Ross S. J., Lawton J. H. (1992). Beta diversity on geographic gradients in britain. J. Anim. Ecol. 61, 151–158. doi: 10.2307/5518
Heino J. (2011). A macroecological perspective of diversity patterns in the freshwater realm. Freshw. Biol. 56, 1703–1722. doi: 10.1111/fwb.2011.56.issue-9
Heino J., Melo A. S., Siqueira T., Soininen J., Valanko S., Bini L. M. (2015). Metacommunity organization, spatial extent and dispersal in aquatic systems: patterns, processes and prospects. Freshw. Biol. 60, 845–869. doi: 10.1111/fwb.12533
Heino J., Mykrä H., Muotka T. (2009). Temporal variability of nestedness and idiosyncratic species in stream insect assemblages. Divers. Distrib. 15, 198–206. doi: 10.1111/j.1472-4642.2008.00513.x
Heino J., Soininen J. (2005). Assembly rules and community models for unicellular organisms: patterns in diatoms of boreal streams. Freshw. Biol. 50, 567–577. doi: 10.1111/j.1365-2427.2005.01346.x
Heino J., Soininen J. (2006). Regional occupancy in unicellular eukaryotes: a reflection of niche breadth, habitat availability or size-related dispersal capacity? Freshw. Biol. 51, 672–685. doi: 10.1111/j.1365-2427.2006.01520.x
Honnay O., Hermy M., Coppin P. (1999). Nested plant communities in deciduous forest fragments: species relaxation or nested habitats? Oikos. 84, 119–129. doi: 10.2307/3546872
Jyrkankallio-Mikkola J., Siljander M., Heikinheimo V., Pellikka P., Soininen J. (2018). Tropical stream diatom communities – The importance of headwater streams for regional diversity. Ecol. Indic. 95, 183–193. doi: 10.1016/j.ecolind.2018.07.030
Krejci M. E., Lowe R. L. (1987). Spatial and temporal variation of epipsammic diatoms in a spring-fed brook. J. Phycol. 23, 585–590. doi: 10.1111/j.1529-8817.1987.tb04210.x
Laliberté E., Legendre P., Shipley B. (2014). FD: Measuring functional diversity (FD) from multiple traits, and other tools for functional ecology (R Package).
Lane C. M., Taffs K. H., Corfield J. L. (2003). A comparison of diatom community structure on natural and artificial substrata. Hydrobiologia. 493, 65–79. doi: 10.1023/A:1025498732371
Lange K., Townsend C. R., Matthaei C. D. (2015). A trait-based framework for stream algal communities. Ecol. Evol. 6, 23–36. doi: 10.1002/ece3.1822
Leboucher T., Mignien L., Wach M., Boutry S., Jamoneau A., Passy S. I., et al. (2021). Consideration of mass effect processes in bioindication allows more accurate bioassessment of water quality. Ecol. Indic. 127, 107791. doi: 10.1016/j.ecolind.2021.107791
Legendre P. (2014). Interpreting the replacement and richness difference components of beta diversity. Glob Ecol. Biogeogr. 23, 1324–1334. doi: 10.1111/geb.12207
Lin Z., Liu G., Guo K., Wang K., Wijewardene L., Wu N. (2024). Scales matter: regional environment factors affect α diversity but local factors affect β diversity of macroinvertebrates in Thousand Islands Lake catchment area. Ecol. Indic. 158, 111561. doi: 10.1016/j.ecolind.2024.111561
Liu G., Lin Z., Qi X., Wang Y., Wang Y., Jiang W., et al. (2023). Environmental filtering, spatial processes and biotic interactions jointly shape different traits communities of stream macroinvertebrates. Front. Ecol. Evol. 11. doi: 10.3389/fevo.2023.1196296
Liu G., Qi X., Lin Z., Lv Y., Khan S., Qu X., et al. (2024). Comparison of different macroinvertebrates bioassessment indices in a large near-natural watershed under the context of metacommunity theory. Ecol. Evol. 14, e10896. doi: 10.1002/ece3.10896
Lopes P. M., Bini L. M., Declerck S. A. J., Farjalla V. F., Vieira L. C. G., Bonecker C. C., et al. (2014). Correlates of zooplankton beta diversity in tropical lake systems. PLoS One 9, e109581. doi: 10.1371/journal.pone.0109581
Lu L., Bao J. (2010). The course and mechanism of evolution about qiandao lake based on the theory of dissipative structure. Acta Geographica Sinica. 65, 755–768. doi: 10.11821/xb201006013
Malmqvist B., Hoffsten P. (2000). Macroinvertebrate taxonomic richness, community structure and nestedness in Swedish streams. Arch. Hydrobiol. 150, 29–54. doi: 10.1127/archiv-hydrobiol/150/2000/29
Mcabendroth L., Foggo A., Rundle S., Bilton D. (2005). Unravelling nestedness and spatial pattern in pond assemblages. J. Anim. Ecol. 74, 41–49. doi: 10.1111/j.1365-2656.2004.00895.x
Mcknight M. W., White P. S., Mcdonald R. I., Lamoreux J. F., Sechrest W., Ridgely R. S., et al. (2007). Putting beta-diversity on the map: broad-scale congruence and coincidence in the extremes. PLoS Biol. 5, e272. doi: 10.1371/journal.pbio.0050272
Mitsuo Y., Tsunoda H., Ohira M., Doi M., Senga Y. (2010). Nested subset patterns of species composition in a pond-dwelling fish fauna. Ecol. Res. 26, 311–316. doi: 10.1007/s11284-010-0785-0
Miyasono S., Taylor C. M. (2013). Effects of habitat size and isolation on species immigration-extinction dynamics and community nestedness in a desert river system. Freshw. Biol. 58, 1303–1312. doi: 10.1111/fwb.12127
Moore J. W. (1977). Seasonal succession of algae in a eutrophic stream in southern England. Hydrobiologia. 53, 181–192. doi: 10.1007/BF00029297
Mouillot D., De Bortoli J., Leprieur F., Parravicini V., Kulbicki M., Bellwood D. R. (2013). The challenge of delineating biogeographical regions: nestedness matters for Indo-Pacific coral reef fishes. J. Biogeogr. 40, 2228–2237. doi: 10.1111/jbi.12194
Pan Y., Stevenson. R. J., Hill. B. H., Herlihy. A. T., Kaufmann. P. R. (1999). Spatial patterns and ecological determinants of benthic algal assemblages in mid-atlantic streams, USA. J. Phycol. 35, 460–468. doi: 10.1046/j.1529-8817.1999.3530460.x
Passy S. I. (2007). Diatom ecological guilds display distinct and predictable behavior along nutrient and disturbance gradients in running waters. Aquat Bot. 86, 171–178. doi: 10.1016/j.aquabot.2006.09.018
Passy S. I. (2008). Continental diatom biodiversity in stream benthos declines as more nutrients become limiting. PNAS. 105, 9663–9667. doi: 10.1073/pnas.0802542105
Podani J. (2016). Once again on the components of pairwise beta diversity. Ecol. Inform. 32, 63–68. doi: 10.1016/j.ecoinf.2016.01.002
Potapova M. G., Charles D. F. (2002). Benthic diatoms in USA rivers: distributions along spatial and environmental gradients. J. Biogeogr. 29, 167–187. doi: 10.1046/j.1365-2699.2002.00668.x
R Core Team (2022). R: A language and environment for statistical computing (Austria, Vienna: R Foundation for Statistical Computing).
Rimet F., Bouchez A. (2012). Life-forms, cell-sizes and ecological guilds of diatoms in European rivers. Knowl. Manag Aquat Ecosyst. 406, 1–12. doi: 10.1051/kmae/2012018
Round F. E., Bukhtiyarova L. (1996). Epipsammic diatoms-communities of British rivers. Diatom Res. 11, 363–372. doi: 10.1080/0269249X.1996.9705390
Ruhi A., Boix D., Gascon S., Sala J., Quintana X. D. (2013). Nestedness and successional trajectories of macroinvertebrate assemblages in man-made wetlands. Oecologia. 171, 545–556. doi: 10.1007/s00442-012-2440-7
Rundle S. D., Foggo A., Choiseul V., Bilton D. T. (2002). Are distribution patterns linked to dispersal mechanism? An investigation using pond invertebrate assemblages. Freshw. Biol. 47, 1571–1581. doi: 10.1046/j.1365-2427.2002.00886.x
Sasaki T., Katabuchi M., Kamiyama C., Shimazaki M., Nakashizuka T., Hikosaka K. (2012). Nestedness and niche-based species loss in moorland plant communities. Oikos. 121, 1783–1790. doi: 10.1111/j.1600-0706.2012.20152.x
Schouten N., van der Ham L. G. J., Euverink G.-J. W., De Haan A. B. (2007). Selection and evaluation of adsorbents for the removal of anionic surfactants from laundry rinsing water. Water Res. 41, 4233–4211. doi: 10.1016/j.watres.2007.05.044
Simberloff D. S., Martin J. L. (1991). Nestedness of insular avifaunas: simple summary statistics masking complex species patterns. Ornis Fenn. 68, 178–192. doi: 10.1007/BF01640530
Socolar J. B., Gilroy J. J., Kunin W. E., Edwards D. P. (2016). How should beta-diversity inform biodiversity conservation? Trends Ecol. Evol. 31, 67–80. doi: 10.1556/ComEc.12.2011.2.2
Soininen J. (2008). The ecological characteristics of idiosyncratic and nested diatoms. Protist. 159, 65–72. doi: 10.1016/j.protis.2007.07.001
Soininen J. (2012). Macroecology of unicellular organisms - patterns and processes. Environ. Microbiol. Rep. 4, 10–22. doi: 10.1111/j.1758-2229.2011.00308.x
Soininen J., Heino J. (2007). Variation in niche parameters along the diversity gradient of unicellular eukaryote assemblages. Protist. 158, 181–191. doi: 10.1016/j.protis.2006.11.002
State Environmental Protection Administration (2002). Water and wastewater monitoring analysis methods (China Environmental Science Press). 38–47 p.
Stevenson R. J., Bothwell M. L., Lowe R. L. (1996). Algal ecology: freshwater benthic ecosystem (Cambridge, MA, USA: Academic Press), 3–30 p.
Taft B., Koncelik J. P. (2006). Methods for assessing habitat in flowing waters: Using the qualitative habitat evaluation index (QHEI). Ohio Environmental Protection Agency.
Tornés E., Ruhí A. (2013). Flow intermittency decreases nestedness and specialization of diatom communities in Mediterranean rivers. Freshw. Biol. 58, 2555–2566. doi: 10.1111/fwb.12232
Ulrich W., Kryszewski W., Sewerniak P., Puchałka R., Strona G., Gotelli N. J. (2017). A comprehensive framework for the study of species co-occurrences, nestedness and turnover. Oikos. 126, 1607–1616. doi: 10.1111/oik.04166
Valdovinos F., Ramos-Jiliberto R., Flores J., Espinoza C. (2009). Structure and dynamics of pollination networks: the role of alien plants. Oikos. 118, 1190–1200. doi: 10.1111/j.1600-0706.2009.17364.x
Valen L. M. V. (1965). Morphological variation and width of ecological niche. Am. Nat. 99, 377–390. doi: 10.1086/282379
Venables W. N., Ripley B. D. (2002). Modern Applied Statistics with S Vol. XII (New York, NY: Springer). 498 p.
Verreydt D., De Meester L., Decaestecker E., Villena M. J., van der Gucht K., Vannormelingen P., et al. (2012). Dispersal-mediated trophic interactions can generate apparent patterns of dispersal limitation in aquatic metacommunities. Ecol. Lett. 15, 218–226. doi: 10.1111/j.1461-0248.2011.01728.x
Virtanen L. K., Köngäs P., Aitto-Oja S., Soininen J. (2011). Is temporal occurrence of diatoms related to species traits, local abundance, and regional distribution? J. Phycol. 47, 1445–1453. doi: 10.1111/j.1529-8817.2011.01068.x
Wang Y., Wu N., Liu G., Mu H., Gao C., Wang Y., et al. (2023). Incorporating functional metrics into the development of a diatom-based index of biotic integrity (D-IBI) in Thousand Islands Lake (TIL) catchment, China. Ecol. Indic. 153, 110405. doi: 10.1016/j.ecolind.2023.110405
Wang Y., Wu N., Tang T., Wang Y., Cai Q. (2022a). Small run-of-river hydropower dams and associated water regulation filter benthic diatom traits and affect functional diversity. Sci. Total Environ. 813, 152566. doi: 10.1016/j.scitotenv.2021.152566
Wang Y., Wu N., Tang T., Zhou S., Cai Q. (2022b). Small run-of-river dams affect taxonomic and functional β-diversity, community assembly process of benthic diatoms. Front. Ecol. Evol. 10. doi: 10.3389/fevo.2022.895328
Wiersma Y. F., Urban D. L. (2005). Beta diversity and nature reserve system design in the Yukon, Canada. Conserv. Biol. 19, 1262–1272. doi: 10.1111/j.1523-1739.2005.00099.x
Williams P. H. (1996). Mapping variations in the strength and breadth of biogeographic transition zones using species turnover. Proc. R Soc. London B. 263, 579–588. doi: 10.1098/rspb.1996.0087
Wu N., Dong X., Liu Y., Wang C., Baattrup-Pedersen A., Riis T. (2017). Using river microalgae as indicators for freshwater biomonitoring: Review of published research and future directions. Ecol. Indic. 81, 124–131. doi: 10.1016/j.ecolind.2017.05.066
Wu N., Guo K., Zou Y., He F., Riis T. (2023). SER: An R package to characterize environmental regimes. Ecol. Evol. 13, e9882. doi: 10.1002/ece3.9882
Wu N., Zhou S., Zhang M., Peng W., Guo K., Qu X., et al. (2021). Spatial and local environmental factors outweigh geo-climatic gradients in structuring taxonomically and trait-based β-diversity of benthic algae. J. Biogeogr. 48, 1842–1857. doi: 10.1111/jbi.14108
Yang J., Huang X. (2021). The 30 m annual land cover dataset and its dynamics in China from 1990 to 2019. Earth Syst. Sci. Data. 13, 3907–3925. doi: 10.5194/essd-13-3907-2021
Zhang J., Ma K. (2014). “spaa: An R package for computing species association and niche overlap,” in Advance in biodiversity Conservation and research in China, vol. X. , 165–174.
Zhou S., Wu N., Zhang M., Peng W., He F., Guo K., et al. (2020). Local environmental, geo-climatic and spatial factors interact to drive community distributions and diversity patterns of stream benthic algae, macroinvertebrates and fishes in a large basin, Northeast China. Ecol. Indic. 117, 106673. doi: 10.1016/j.ecolind.2020.106673
Keywords: periphyton, nestedness, niche width, attachment ability, environment variables
Citation: Qi X, Liu G, Chen C, Wang Y, Wang Y, Lin Z and Wu N (2024) Nestedness of benthic diatom metacommunity in relation to species niche width and environmental variables in a large near-natural catchment. Front. Ecol. Evol. 12:1339946. doi: 10.3389/fevo.2024.1339946
Received: 17 November 2023; Accepted: 16 April 2024;
Published: 01 May 2024.
Edited by:
Muhammad Aasim, Sivas University of Science and Technology, TürkiyeReviewed by:
Xuhui Dong, Guangzhou University, ChinaNuman Emre Gümüş, Karamanoğlu Mehmetbey University, Türkiye
Copyright © 2024 Qi, Liu, Chen, Wang, Wang, Lin and Wu. This is an open-access article distributed under the terms of the Creative Commons Attribution License (CC BY). The use, distribution or reproduction in other forums is permitted, provided the original author(s) and the copyright owner(s) are credited and that the original publication in this journal is cited, in accordance with accepted academic practice. No use, distribution or reproduction is permitted which does not comply with these terms.
*Correspondence: Naicheng Wu, bmFpY2hlbmd3dTg4QGdtYWlsLmNvbQ==