- 1Laboratoire de Biologie du Développement de Villefranche-sur-Mer (LBDV, UMR 7009), Institut de la Mer de Villefranche (IMEV), Sorbonne Université, Centre National de la Recherche Scientifique (CNRS), Villefranche-sur-Mer, France
- 2Université Côte d’Azur, Centre National de la Recherche Scientifique (CNRS), Institut National de la Santé et de la Recherche Médicale (INSERM), Institute for Research on Cancer and Aging (IRCAN), Nice, France
Ascidians are among the most common invasive marine invertebrates worldwide. Many species of non-indigenous ascidians (NIAs) have successfully colonized the Mediterranean Sea, notably within anthropized coastal lagoons and harbors. Although invasive species are generally characterized by their broad ecological tolerance, different ascidian species exhibit varied responses to biotic and abiotic environmental stressors, including temperature and salinity. Acquiring a better understanding about of the impact of such parameters on ascidian life history is crucial for predicting the invasive potential of NIAs. In this study, we investigated the impact of various salinities on the reproduction of the colonial ascidian Polyandorcarpa zorritensis, a species indigenous to Peru and a thriving invader. P. zorritensis undergoes asexual reproduction via a peculiar form of budding named vasal budding and produces resistant spherules, which likely facilitated its dissemination over long distances. Despite its widespread distribution along the Pacific and Atlantic coasts, it is only found in a few Mediterranean coastal areas with a low salinity. We tested the impact of different salinity conditions on the sexual and asexual reproduction rates of P. zorritensis in a controlled laboratory setting. Our experiments showed that the rate of asexual reproduction in colonies bred at 29 or 36 ppt salinity levels, corresponding to the natural range inhabited by P. zorritensis, was higher than those grown in 40 ppt salinity, commonly found in Mediterranean marinas and harbors. The results suggest that, although P. zorritensis has been present in the Mediterranean for several decades, its potential for invasion could be constrained by an intolerance to high salinity.
Introduction
Ascidians are one of the most common invasive marine invertebrates worldwide (Lambert and Lambert, 1998; Izquierdo-Muñoz, 2009; Clarke Murray et al., 2014; Ulman et al., 2017; Cardeccia et al., 2018; Ulman et al., 2019a). When introduced to a new environment, non-indigenous ascidians (NIAs) can propagate on hard substrates, outcompete local species, and alter the native fouling communities (Lambert, 2002; Castilla et al., 2004; Bullard et al., 2007; Dias et al., 2008). Coastal anthropized areas like harbors and lagoons are particularly susceptible to being colonized by NIAs, which are mainly introduced by human-mediated vectors such as hull fouling and aquaculture activities (Lambert and Lambert, 2003; Clarke Murray et al., 2011; López-Legentil et al., 2015; Ulman et al., 2019b; Nichols et al., 2023). In these semi-enclosed habitats, environmental conditions differ from the open sea from which they are separated by natural or artificial barriers, leading to short-scale instability in terms of eutrophication, pollution, temperature, and salinity (Cognetti and Maltagliati, 2000; Gewing et al., 2017). In particular, temperature and salinity have a strong influence on the distribution of marine species, especially ascidians (Dybern, 1967; Dybern, 1969; Sims, 1984; Vázquez and Young, 1996; Vázquez and Young, 2000; Bullard et al., 2007; Epelbaum et al., 2009; Chebbi et al., 2010; Pineda et al., 2012; Gewing et al., 2018). With limited dispersal capacity due to a biphasic life cycle dominated by the sessile adult stage, ascidians‘ survival is compromised by local unfavorable conditions (Carballo, 2000). Therefore, although successful ascidian invaders are generally recognized for their broad ecological tolerance (Lambert and Lambert, 2003; Gröner et al., 2011; Granot et al., 2017; Rocha et al., 2017; Platin and Shenkar, 2023), NIAs can also display poor survival or reproductive outcomes when exposed to temperature and salinity that differ from their region of origin (Shenkar and Loya, 2008; Nagar and Shenkar, 2016; Gewing et al., 2018). Enhancing our understanding of how environmental factors influence the reproductive strategies of NIAs is essential for more accurately predicting their invasive potential (Rocha et al., 2017; Platin and Shenkar, 2023).
Polyandrocarpa zorritensis (Stolidobranchia: Styelidae) is a colonial ascidian that was first described from the coast of Peru (Van Name, 1931). In recent decades P. zorritensis has become a prevalent invasive species in temperate coastal areas (Lambert and Lambert, 1998; Brunetti and Mastrototaro, 2004). One of the oldest reports of P. zorritensis outside its native range was on the Italian Mediterranean coast (Brunetti, 1978) and the species has recently been reported in several Mediterranean harbors and gulfs (Brunetti and Mastrototaro, 2004; Mastrototaro et al., 2008; Stabili et al., 2015; Tempesti et al., 2022). It was also found in several Pacific and Atlantic regions including Japan (Nishikawa et al., 1993; Otani, 2002; Iwasaki et al., 2004), Southern California (Lambert and Lambert, 1998; Nichols et al., 2023), Hawaii (Abbott et al., 1997), the Galapagos Islands (Lambert, 2019), the Panama Canal (Carman et al., 2011), the Caribbean (Monniot, 2018; Streit et al., 2021), Brazil (Millar, 1958), the Gulf of Mexico (Lambert et al., 2005), Florida (Vázquez and Young, 1996) and North Carolina (Villalobos et al., 2017). From 1994 to 2020, there was a noticeable rise in P. zorritensis abundance in Californian marinas, accompanied by a concomitant northward expansion of the species (Nichols et al., 2023). Similarly, subsequent to its initial introduction to Japan in 1991, P. zorritensis has emerged as a common NIA detected along the country’s coasts (Iwasaki et al., 2004). The successful invasiveness of P. zorritensis has been attributed to its apparent tolerance to fluctuations in temperature and salinity (Lambert and Lambert, 1998) and its singular mode of asexual reproduction by vasal budding (Brunetti and Mastrototaro, 2004; Alié et al., 2018; Scelzo et al., 2019). During this process, the species produces resistant dormant forms (spherules) that plausibly allow long-distance human-mediated transport and colony restoration even in the absence of adult individuals and after extensive dormancy (Scelzo et al., 2019; Hiebert et al., 2022). Previous experimental studies demonstrated that larval behavior (Vázquez and Young, 1996) as well as zooid and spherule survival (Hiebert et al., 2022) are impacted by extreme temperature or salinity. However, we still have a poor understanding of the relationship between environmental parameters and asexual and sexual reproduction in P. zorritensis.
Coastal lagoons, harbors and marinas of the Mediterranean are known as hotspots for the entry and secondary spread of non-indigenous invasive species, whose diversity and distribution are influenced by environmental factors such as temperature, primary productivity or climate type (Ulman et al., 2017; Ulman et al., 2019a; Ulman et al., 2019b). While some non-indigenous ascidians such as Ascidiella aspersa, Styela plicata, Ciona robusta, and Pyura dura, are widely distributed throughout the Mediterranean, NIAs from the Red Sea are mostly found in the eastern Mediterranean (e.g. Microcosmus exasperatus, Herdmania momus), suggesting that west-east temperature and salinity gradients in the Mediterranean (Coll et al., 2010) may influence the distribution of non-indigenous ascidians, in relation to the species-specific ecological tolerances (Shenkar and Loya, 2008; Platin and Shenkar, 2023). Despite its local abundance, P. zorritensis is not among the most widely spread NIAs in the Mediterranean (Izquierdo-Muñoz, 2009; Cardeccia et al., 2018; Ulman et al., 2019a). P. zorritensis was not found on the 583 vessel hulls examined by Ulman et al. (2019b), although 71% of these vessels hosted at least one NIA. P. zorritensis was only recorded in one out of the 50 marinas analyzed by Ferrario et al. (2017), as well as Ulman et al. (Ulman et al., 2017; Ulman et al., 2019a). Furthermore, it was present in only one of the 32 marinas sampled in northern Spain (López-Legentil et al., 2015) and is absent from NIA-rich areas along the Tunisian and Israeli coasts (Chebbi et al., 2010; Gewing and Shenkar, 2017). Therefore, despite the long-standing observation of P. zorritensis in the Mediterranean (Brunetti, 1978), its distribution in the region has remained relatively restricted to the northwestern basins (Figure 1A). While salinity levels in marinas rich in NIAs are frequently higher than those found in surrounding open water (Ulman et al., 2019a), several Mediterranean sites where P. zorritensis is found have a salinity below the average 38 ppt of the western Mediterranean. In Taranto harbor’s Mare Piccolo in Italy and the Thau lagoon in France, salinity levels have an annual average of 36-37 ppt (Audouin, 1962; Brunetti and Mastrototaro, 2004; André et al., 2021). Additionally, a salinity of 34 ppt was measured at the Santa Carla de la Rapita harbor in the Ebro delta (López-Legentil et al., 2015). In La Spezia harbor, the spring salinity fluctuates between 32 and 37 ppt (Brunetti, 1978), while in Livorno harbor, the range spans from 35 to 39 ppt (Tempesti et al., 2022). In this study we investigated the influence of salinity on the reproduction of P. zorritensis, with emphasis on asexual reproduction by budding, analyzing in laboratory-controlled conditions the effects of different salinity values reported in regions where the species is found.
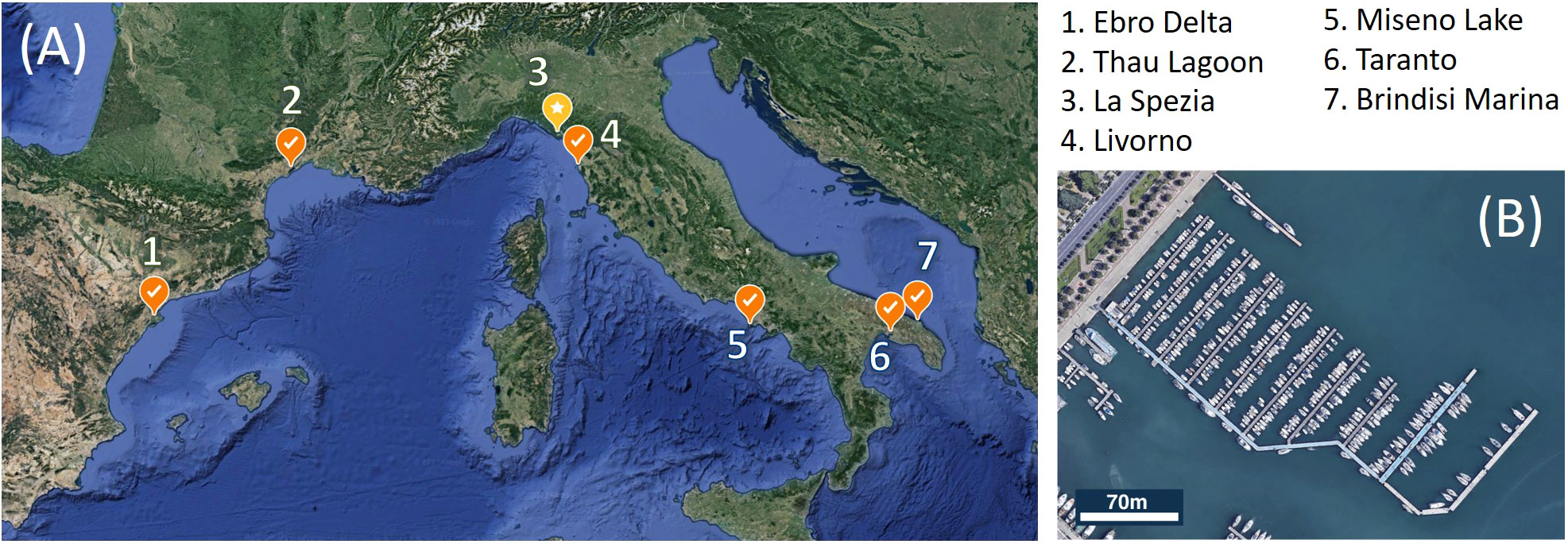
Figure 1 (A) Sites in the Mediterranean Sea where the presence of Polyandrocarpa zorritensis was reported. 1: Turon and Perera, 1988; 2: https://doris.ffessm.fr/Especes/Polyandrocarpa-zorritensis-Polyandrocarpe-de-Zorritos-5004; 3: Brunetti, 1978; 4: Tempesti et al., 2022; 5: Virgili et al., 2022; 6: Mastrototaro et al., 2008; 7: Stabili et al., 2015. The yellow spot marks La Spezia, where the samples for this study were collected. (B) Satellite view of the Assonautica Marina in La Spezia. Credit: Landsat/Copernicus Data SIO, NOAA, US Navy, NGA, GEBCO Inst. Geogr. Nacional GeoBasis-DE/BKG (©2009) 3D.
Materials and methods
Polyandrocarpa zorritensis collection, identification and husbandry
Colonies of Polyandrocarpa zorritensis were collected in the harbor of La Spezia, Italy (Assonautica Benedetti, 44°06′10.7″N, 9°49′34.5″E) (Figure 1B). The presence of P. zorritensis was recorded by visual inspection of the mooring lines along the jetties of the marina on six occasions from May 2016 to February 2023. Salinity and temperature (Supplementary Table) were measured close to the surface, at the depth where P. zorritensis colonies were present. Taxonomic identification follows Van Name (1931), and molecular barcoding of colonies from La Spezia was conducted previously (Alié et al., 2018). The spherules were gently separated from the colony and stored in tanks containing seawater at 11°C (at salinity of 38-39ppt) before being used for experiments. To obtain zooids used for the experiments, the spherules were transferred to water tanks containing 12 liters of artificial seawater (ASW) at 24°C, prepared with deionized water and marine salt (Red Sea Salt, Red Sea) at the desired concentration. The spherules were placed on microscope glass slides (5x7cm) on the bottom of the tanks, with 5 to 12 spherules per slide and 3 to 4 slides per tank (see Supplementary Table). Since the number of stolons depends on spherule size (Scelzo et al., 2019), we took care to use spherules of similar sizes. The day-night light cycle was 12:12.
Upon hatching, bubblers were added to oxygenate the water, and animals were fed twice a day with a mix of 15ml of live Tisochrysis lutea, 15ml of live Chaetoceros gracilis, 250µl ISO800, and 250µl of Shellfish Diet 1800 (Reed Mariculture) per tank. Water was changed every two days, temperature was maintained around 24-24.5°C and colorimetric tests (Ammonia Marine Test Kit and Nitrate\Nitrite Marine Test Kit, Red Sea) were performed on a regular basis to ensure that NH3/NH4, NO2 and NO3 wastes did not reach toxic values throughout the experiments.
Asexual reproduction monitoring and quantification
Experiments were conducted from spherules collected in the harbor of La Spezia from 2019 to 2022. A first set of experiments was conducted on two different batches of spherules that were collected in autumn (November 5, 2019 and November 10, 2022) and maintained at 11°C for 80 and 99 days, respectively. Results from these two experiments were combined and analyzed as a single experiment (hereafter called “autumn”). Another set of experiments was conducted on a single batch of spherules collected in winter (February 2, 2022, called winter batch) and maintained at 11°C for 73 days and 97 days, respectively. A last experiment was conducted on spherules collected in spring (May 12, 2022, called spring batch) and used immediately after collection. Zooids were cultivated at 22, 29, 36 and 40ppt. Photos of the zooids were taken at regular intervals (see Supplementary Figure 1 as an example), up to 24 days after spherule seeding, from which date image-based quantification of asexual reproduction tends to become complicated due to the high number of overlapping stolons, which makes the individual assignment difficult. Pictures were taken with a Canon EOS 6D camera equipped with a 100mm macro lens. Stolon bases, stolon tips, budding nests, and newly hatched zooids were quantified from pictures of the last day of each experiment, using the Cell Counter plugin on ImageJ.
Quantification of sexual reproduction
Sexual reproduction was evaluated by counting the number of embryos and larvae produced per zooid, which were obtained from one experiment using the autumn 2022 spherules (Supplementary Table). Upon having reached sexual maturity (approximately a month after spherule seeding), zooids were relaxed in seawater with MS-222 (E10521-10G, Sigma Aldrich), then fixed in a solution of 4% paraformaldehyde diluted in ASW according to the salinity of each sample, at room temperature and then kept at 4°C. After several days of fixation, zooids were dissected and the number of gonads and brooded embryos in the atrial chamber was counted for each individual (see Supplementary Figures 2B-B’ as an example). The swimming larvae and the embryos accidentally released by the zooids during the 9 days preceding their fixation were also counted, by visually inspecting the tanks every morning (from the time the light goes on, 8 a.m., to early afternoon, when they stopped releasing larvae) and before every water change. In this experiment, we raised 19 spherules at 40ppt, 22 spherules at 36 ppt and 20 spherules at 29 ppt (Supplementary Table).
Morphological measures, heartbeat rate and stolon pulsation period
Zooid size was measured using ImageJ, on the one-month-old animals hatched from spherules of the autumn 2022 batch, relaxed in MS-222 (a pinch of powder in 15 ml of sea water), and before fixing. Size was measured between the tip of the inhalant siphon and the posterior-most point of the body cavity (see Supplementary Figure 2A as an example). Heart and stolon beating rates were measured in an additional experiment using 14-days-old animals from the autumn batch (Supplementary Table). Heart beating rate was measured visually under a stereomicroscope, thanks to the transparency of the tunic, as the number of pulsations per minute. Stolon pulsation period was measured using timelapse imaging taken with a Leica M165-FC stereomicroscope equipped with an MC170-HD camera. One picture was taken every 5 seconds, a pulsation period being the lag time until the beating tip of a stolon comes back to its initial condition (see example movies at https://drive.google.com/drive/folders/1Z522byRFvnHer677YMKoQZMvjaDs6fKX?usp=sharing).
Results
Presence of Polyandrocarpa zorritensis coincides with lower salinity in La Spezia marina
In 2016, we investigated several spots in the La Spezia Bay, including marinas (Circolo Velico Palmito 44.086377, 9.878521; Darsena Pagliari 44.104680, 9.859106; Porto La Grazie 44.068307, 9.835713; Assonautica 44.103388, 9.82633) and open water oyster racks (44.071649, 9.857886; 44.057952, 9.842249). We only found P. zorritensis in the Assonautica marina (Figure 1B), the innermost investigated spot of the bay. From 2016 to 2023, we regularly found Polyandrocarpa zorritensis in this marina, usually as large aggregate colonies and/or as mats of dormant spherules on the immersed boat lines, in the proximity of a freshwater outlet (Figure 2). In May 2016 and 2022, the species was only visible on the proximal-most pontoons of the marina, where the salinity was the lowest, i.e., 29 and 34 ppt respectively (Figures 2A, D). While we cannot totally rule out that small residual colonies were present on the other pontoons, they were likely inconspicuous enough not to be detected. In the autumn and winter seasons, the colonies had a wider distribution, with measured salinities ranging between 36 and 38 ppt (Figures 2B, C, E, F).
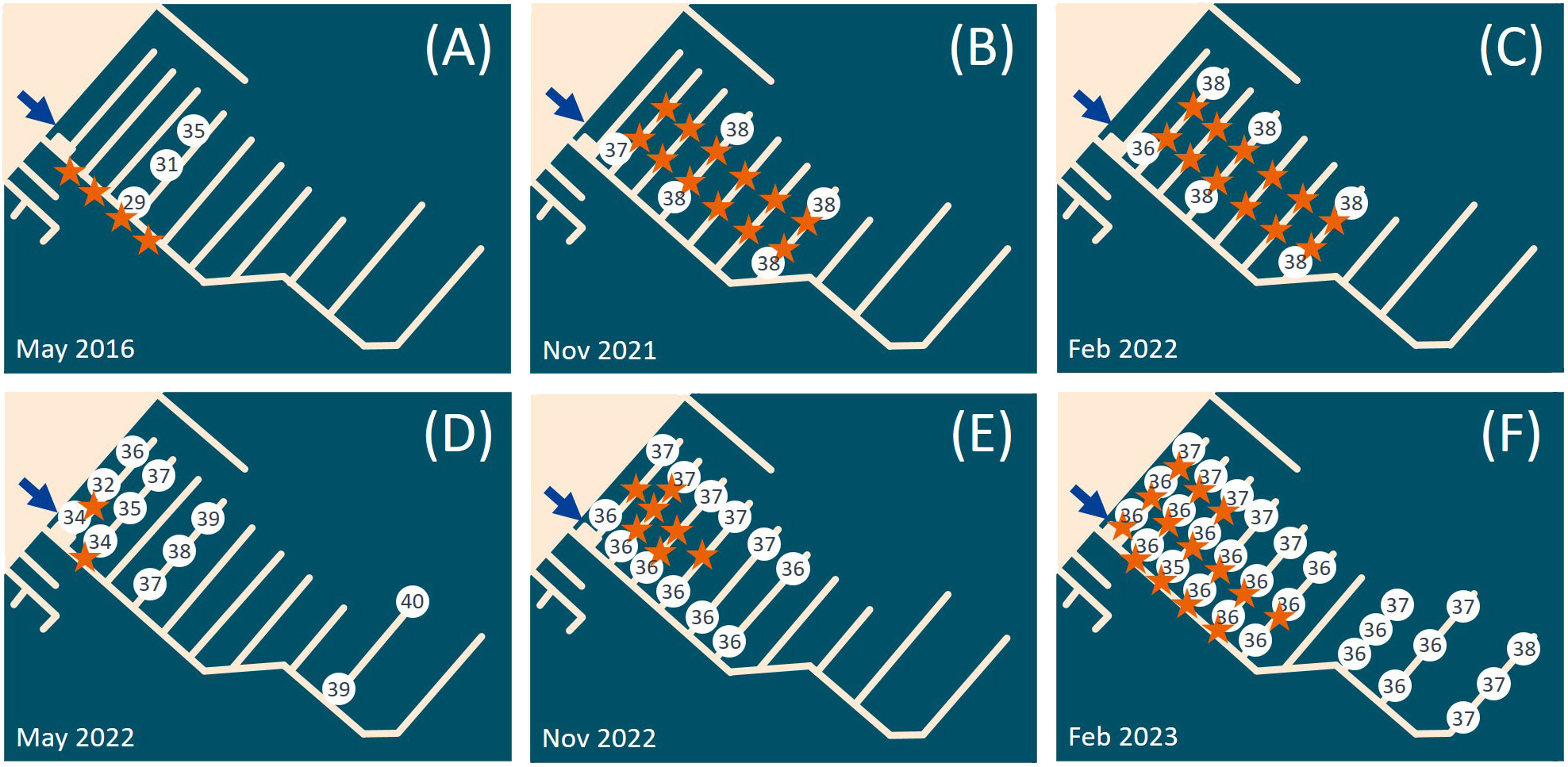
Figure 2 Polyandrocarpa zorritensis presence and salinity values observed in (A) May 2016, (B) May 2021, (C) February 2022, (D) May 2022, (E) November 2022 and (F) February 2023. The blue arrow indicates the inflow of fresh water in the marina. The white circles contain the salinity values (in ppt) observed in the different sites of the marina. The orange stars indicate the spots where P. zorritensis was found.
High salinity negatively affected the rate of asexual reproduction
To study the effect of the observed salinity on the asexual budding in P. zorritensis, we quantified the production of stolons, budding nests (also called buds) and new zooids produced by individual adult zooids (illustrated in Figure 3) cultivated at 22, 29, 36 and 40 ppt (Supplementary Table). These adults were obtained from dormant spherules collected in the field and later placed in experimental conditions in the laboratory. While none of the spherules placed at 22 ppt hatched, nearly every spherule in the other salinity conditions hatched after 5 to 7 days and gave rise to a functional zooid (Supplementary Table). When using spherules collected in autumn (Figures 4A-A”) and maintained in dormancy for three months before the experiment, the salinity significantly affected the number of stolonial tips, which was significantly lower at 40 than at 36 ppt (Mann-Whitney p-value = 0.0071) (Figure 4A’), as well as the number of buds and of newly hatched zooids, which was significantly lower at 40 than at 29 (p-value = 0.0099) and 36 ppt (p-value = 6.05e-5) (Figure 4A”). The number of stolon bases was also significantly lower at 40 ppt than at 36 ppt (p-value = 0.014) and did not differ between 29 and the two other conditions (Figure 4A).
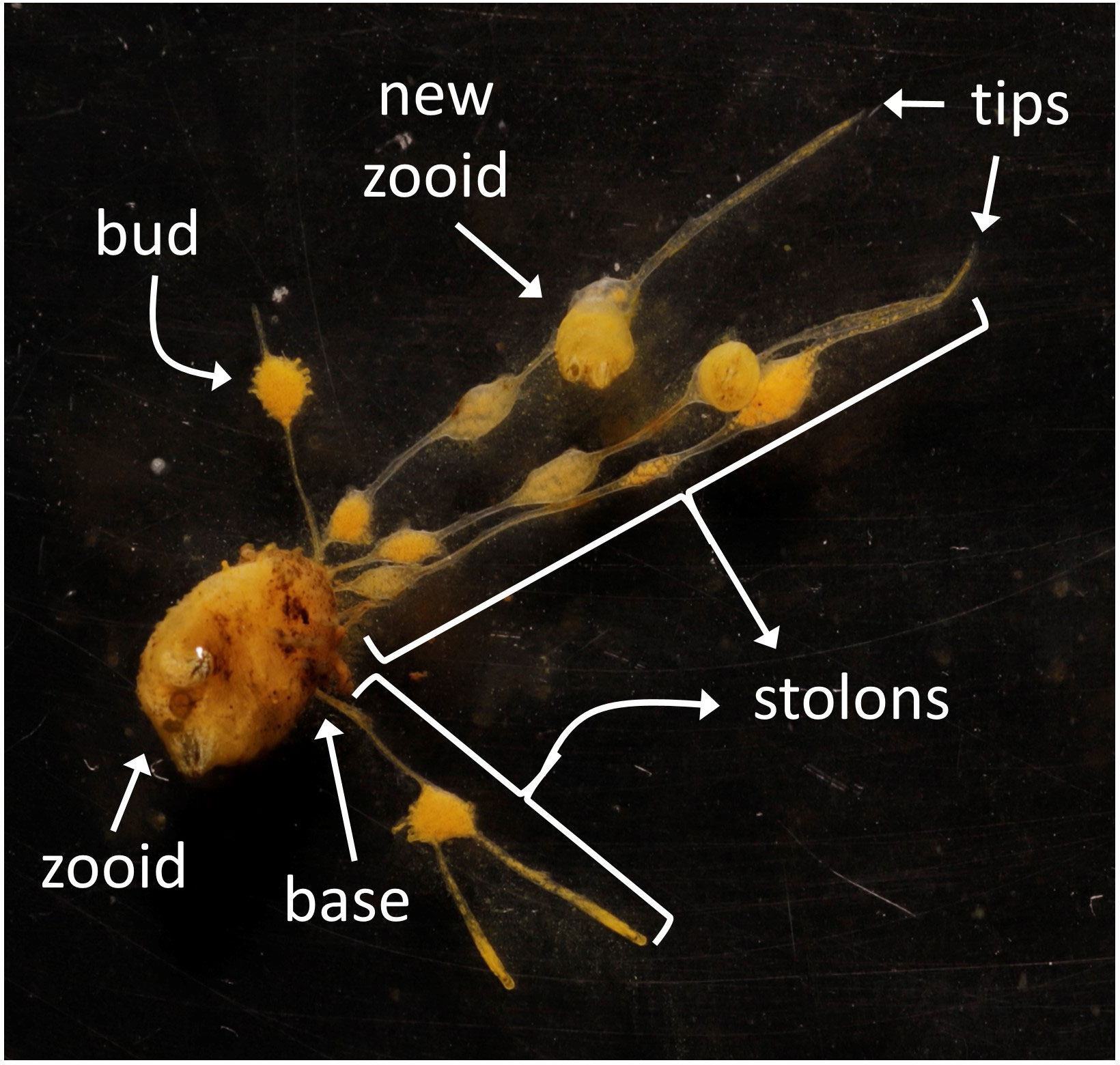
Figure 3 Parameters measured for the rate of asexual reproduction. Image showing a zooid originated from a dormant spherule, indicating the base of a stolon, tips of stolons, a bud (or budding nest), and a new zooid formed from a bud.
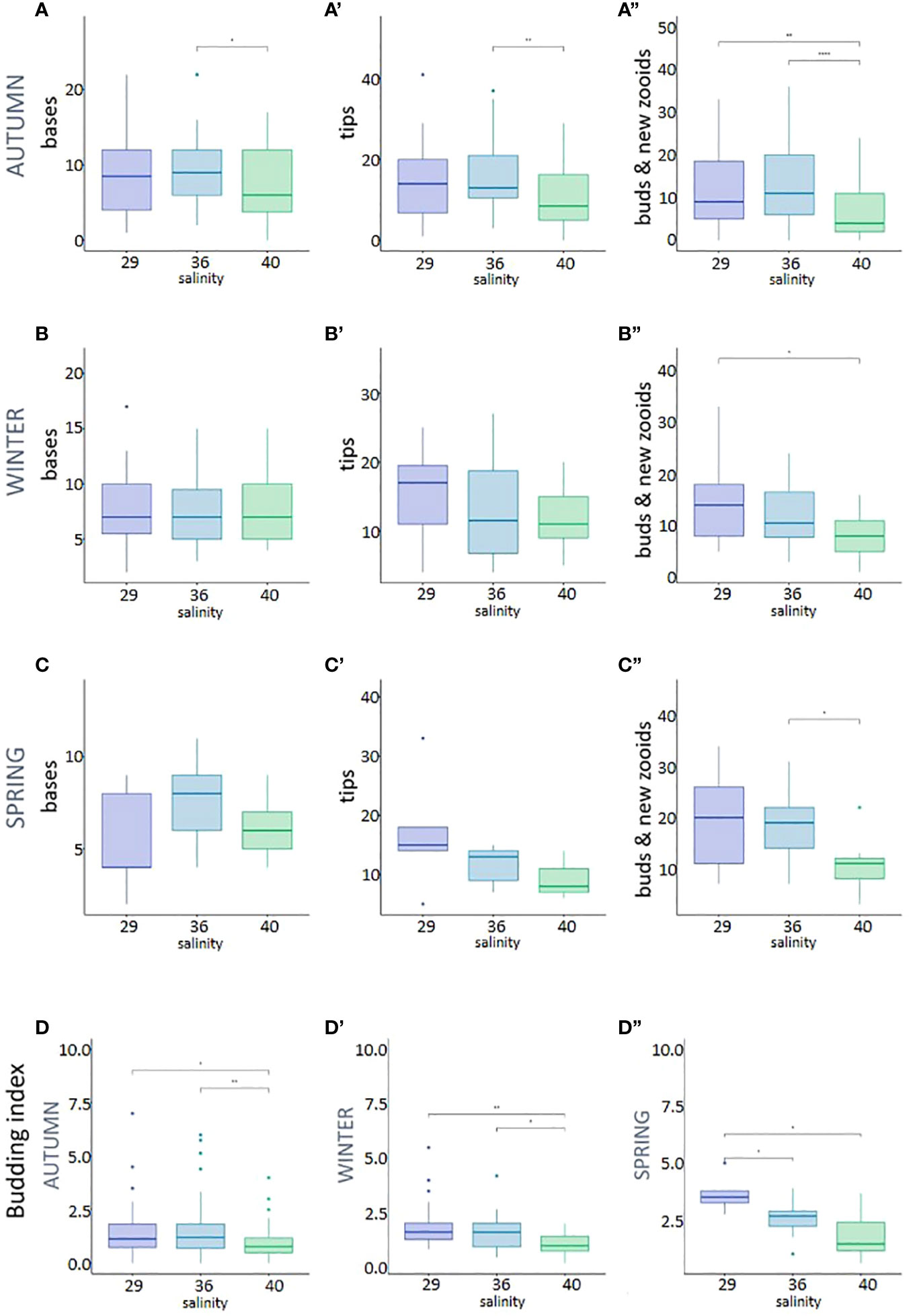
Figure 4 Asexual reproduction in the three different salinities: 29, 36 and 40 ppt, measured by the production of stolons bases, stolons tips, buds and new zooids, per adult zooid; (A-A”) Autumn batch; (B-B”) Winter batch; (C-C”) Spring batch; (D-D”) Budding index, i.e. ratio of buds and new zooids to stolon bases, per adult zooid. Stars correspond to the statistical analysis [Mann-Withney test with *p-value < 0.05, **p-value <0.01, and ****p-value < 0.001; absence of asterisk means no statistical significance (p-value > 0.05)].
Experiments conducted with spherule batches collected in different seasons gave a similar, albeit less pronounced trend. When using spherules collected in winter (Figures 4B-B”), the number of stolon bases and stolon tips did not differ between the three treatments (Figures 4B-B’), but the salinity affected the number of nests and new zooids, which was lower at 40 than at 29 ppt (p-value = 0.011) (Figure 4B”). Finally, for spherules collected in spring (Figures 4C-C”), the number of stolon bases and stolon tips did not differ between the three treatments (Figures 4C-C’) but the number of nest and new zooids was lower at 40 than 36 ppt (p-value = 0.037) (Figure 4C”).
In order to estimate the yield between the growth of the colony and the actual reproduction via budding, we calculated a budding index, defined as the ratio between the number of nests and new zooids to the number of stolon bases. Regardless of the spherule batch used, as salinity increased, the budding index decreased (Figures 4D-D”). Using autumn and winter spherules, the budding index was significantly lower at 40 ppt when compared to 29 and 36 ppt (Figures 4D, D’). Using spring spherules the index was significantly higher at 29 ppt when compared to 40 and 36 ppt (Figure 4D”). All p-values associated with these experiments are given in the Supplementary Table.
Salinity did not affect zooid physiology
Physiological variables reflecting the general zooid health (Dijkstra et al., 2008) were measured on the animals exposed to the tested salinities. The average size of the zooids, measured from the tip of the inhalant siphon to the posterior-most point of the body cavity, were 1.14, 1.24 and 1.31 cm for 50 days-old zooids grown at 29, 36 and 40 ppt respectively, with a significant difference between 29 and 40 ppt (p-value = 0.043) (Figure 5A; Supplementary Table). The average heartbeats rate (Figure 5B) did not significantly differ between the three conditions (56, 60 and 57.2 pulsations per minute at 29, 36 and 40 ppt respectively), neither did the period of stolon beating (110, 112 and 107 seconds at 29, 36 and 40 ppt respectively) (Figure 5C). These results suggest that zooids grow slightly slower at 29 ppt but do not experience chronic stress significantly affecting blood circulation in the colony.
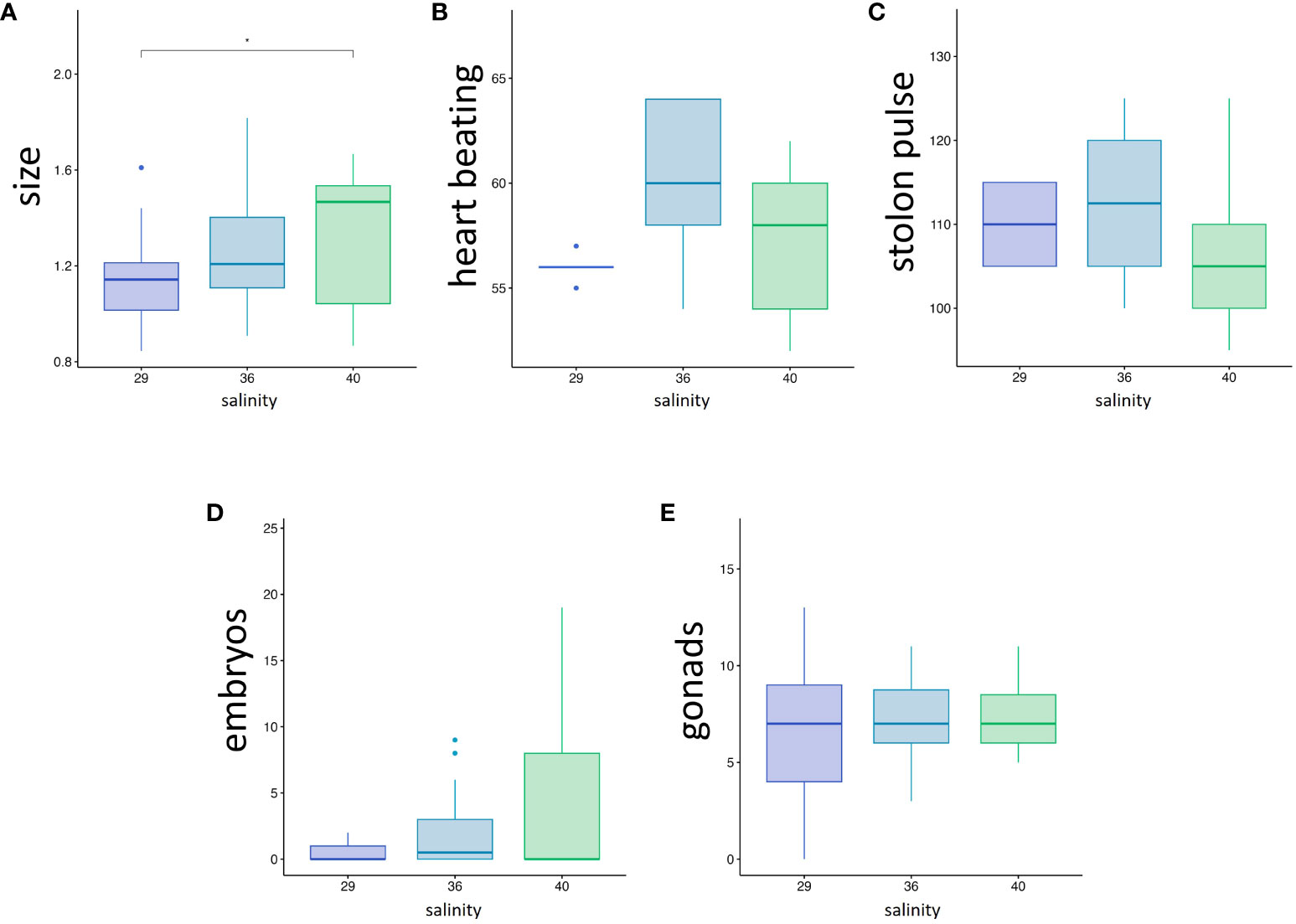
Figure 5 (A-C) Colony health parameters, (A) zooid size in cm, (B) heart beating rate (pulsations.min-1), (C) Stolon pulsation period (time between two consecutive pulsation, in seconds). (D, E) Influence of salinity on sexual reproduction: (D) Number of brooded embryos per 1 month-old zooids. (E) Number of gonads per 1 month-old zooid. Stars correspond to the statistical analysis [Mann-Withney test with *p-value < 0.05; absence of asterisk means no statistical significance (p-value > 0.05)].
Production of embryos and larvae
To assess whether sexual reproduction was equally affected by the salinity, we counted the number of gonads and offspring (larvae and embryos) present in the body cavity of one-month-old zooids obtained from autumn spherules (Figures 5D, E). The number of gonads did not significantly differ between the three conditions (Figure 5E). An average of 0.50 (± 0.82 SD), 2.04 (± 2,76) and 4.42 (± 6,6) offspring per zooid at 29, 36 and 40 ppt, respectively (Figure 5D) was counted, with no statistical difference between the three conditions probably due to high inter-individual variance. Indeed, while some zooids did not contain any offspring, others were bearing up to 19 embryos (Supplementary Table). Thus, we also counted the larvae and embryos present in the tanks during the nine days before the end of the experiment (Supplementary Figure 3). Adding these numbers to the offspring found inside the body cavity, we obtain a total of 11 larva and 23 embryos released by 20 zooids at 29 ppt (0.24 offspring per zooid/day), 112 larvae and 37 embryos released by the zooids at 36 ppt (0.73 offspring per zooid/day), and 104 larvae and 17 embryos released by 19 zooids at 40 ppt (0.70 offspring per zooid/day). Experimental replicates would be necessary to test for statistical significance, yet these results suggest that the number of offspring is approximately ten times lower at 29 ppt than at higher salinities.
Discussion
Salinity effect on asexual budding in P. zorritensis
Our results suggest that the rate of asexual reproduction in P. zorritensis, i.e. the number of asexually produced zooids 14 days after hatching of the spherules, is lower at 40 ppt salinity compared to 29 and 36 ppt. The salinity values of this study were selected on the basis of experimental evidence: 29 ppt was selected as it is the minimum level at which P. zorritensis was found in the sampling site (La Spezia, Assonautica Marina), while 36 ppt was chosen as it corresponds to the average level in semi-enclosed basins colonized by P. zorritensis in the Mediterranean Sea (Audouin, 1962; Brunetti and Mastrototaro, 2004). This salinity level also closely matches the surface salinity in the northern Peruvian coast (Chaigneau et al., 2013), where P. zorritensis was originally described. On the other hand, a salinity of 40 ppt is frequently observed in Mediterranean marinas (Ulman et al., 2019a; present study), which are the primary entry points and habitats for most non-indigenous ascidians (NIAs). Our experiments indicate that the quantity of zooids produced by spherules is smaller at 40 ppt than at lower salinities, indicating a negative impact of relatively high salinity on the asexual propagation of P. zorritensis.
Salinity has a notable impact on the budding index, which represents the average number of buds per stolon and which was used as a proxy for the relative energy allocation to colony expansion versus asexual replication (budding). This index was generally lower at 40 ppt, except for the comparisons between 36 and 40 ppt with spring spherules, where the difference is not statistically significant. Yet, while salinity affected the number of buds and zooids in most experiments, a significant effect on stolon bases and tips was only observed between 36 and 40 ppt with the autumn batch. This observation could reflect a different dynamic of colony expansion related to the larger size of autumn spherules (Hiebert et al., 2022). Additionally, it is also important to note that zooids raised at 40 ppt showed a slightly larger size than those raised at lower salinities. Taken as a whole, these results suggest that individuals raised at 40 ppt may prioritize somatic growth and colony expansion over asexual reproduction compared to those at lower salinities.
Overwintering spherules are probably a seasonal population bottleneck for P. zorritensis. Notably, in March 2018 and May 2019, P. zorritensis appeared to disappear almost completely from the La Spezia site, where only a few scattered resting spherules were found attached to boat mooring lines (Hiebert et al., 2022). Similarly, in May 2022, the animals were confined to the immediate proximity zone. This scenario contrasts sharply with the autumn situation, where P. zorritensis extensively dominated the benthic community by forming dense colonies consisting of numerous stolons and buds (see Figure 5 in Hiebert et al., 2022 or Figure 2 in Scelzo et al., 2019). This suggests that only a limited number of spherules are overwintering and that successful hatching in spring may be crucial for replenishing the population of P. zorritensis, which mainly relies on budding (Brunetti and Mastrototaro, 2004; Hiebert et al., 2022). Consequently, adverse conditions such as high salinity may significantly decrease the rate of asexual reproduction and greatly impact the fitness of P. zorritensis.
The above observations further support that salinity has a pronounced effect on the budding index in colonies originating from spring spherules. Indeed, these spherules were collected when the strongest salinity gradients were observed in the La Spezia marina, together with the lowest salinities recorded (34 to 40 ppt in May 2022 and 29 to 35 ppt in May 2016). In our experiments the spring batch was the only group to show a statistical difference between 29 and 36 ppt, with a budding index that was 2.5 times higher at 29 ppt compared to 40 ppt. This finding may have significant implications for adaptability to salinity gradients, because spring spherules could be particularly vulnerable to water salinity. Therefore, strong salinity gradients during this critical season may have a significant impact on the reproductive outcomes of P. zorritensis, as the overwintering spherules are the ones that replenish the population.
The dispersal of P. zorritensis may be limited by extremely low salinities. Previous research has shown that the hatching potential of dormant spherules is halved after exposure to 15 ppt salinity for 48 hours and subsequent return to higher salinity (Hiebert et al., 2022). Thus, spherules are capable to withstand acute hypo-osmotic stress. However, our present findings suggest that spherules are unable to produce zooids when maintained at 22 ppt. In addition, it appears that a low salinity prevents larval recruitment. Indeed, Vázquez and Young (1996) reported that P. zorritensis larvae displayed abnormal swimming behavior at 26 ppt or lower, and actively escaped from water at 22 ppt when exposed to vertical haloclines. Taking these findings together with our present results, it is clear that water with a salinity as low as 22 ppt does not facilitate asexual reproduction and hinders larval recruitment, suggesting that P. zorritensis may not be able to thrive in brackish environments.
Effect of different salinities on asexual versus sexual reproduction
Although previous work on a colonial stolidobranch showed that temperature and salinity have different effects on sexual and asexual reproduction (Brunetti, 1984), the present study showed no statistically significant difference in the number of gonads and brooded embryos between the different experimental conditions. However, the number of larvae observed swimming in the tanks was approximately ten times lower at 29 ppt when compared to 36 and 40 ppt, suggesting that 36 ppt may be an ideal salinity at which both asexual and sexual reproduction rates are high. Further research into the effects of salinity on sexual reproduction could be carried out using histological analyses to examine gonadal maturity. In addition, other aspects related to sexual reproduction could be studied, such as the age at which zooids reach sexual maturity, or larval swimming behavior and larval recruitment at different salinities.
Coloniality in ascidians is often associated with reduced zooid size, ovoviviparity, and fewer embryos compared to solitary species (Berrill, 1935; Svane and Young, 1989; Zega et al., 2006; Alié et al., 2018). Accordingly, the gonads of P. zorritensis contain relatively few eggs, each zooid bearing approximately 20 embryos at different developmental stages (Van Name, 1945; Monniot, 2016; present study), and the embryonic development takes several days (personal observations). Each day a zooid releases only a few larvae (this study), which are lecithotrophic and low-dispersive with swimming times ranging from only a few minutes to a few hours (Vázquez and Young, 1996, personal observations). Therefore, a decrease in asexual reproduction in P. zorritensis, as observed at 40 ppt, may severely limit its fitness because sexual reproduction is unlikely to compensate for reproductive success in this species. In contrast, solitary Polycarpa species, which are closely related to P. zorritensis (Alié et al., 2018), are usually larger and have more and larger gonads (Van Name, 1945; Vazquez et al., 1995). They can spawn several dozens of planktonic eggs per week (Gordon et al., 2020), thus promoting long-distance dispersal by currents (Berrill, 1935; Svane and Young, 1989; Havenhand, 1991). Styela plicata, another Stolidobanch that is also very abundant in La Spezia marina, is a broadcast spawner of floating eggs that develop for several hours before metamorphosis (Yamaguchi, 1975; Villa and Patricolo, 2000; Crean and Marshall, 2015). Notably, there are reports of solitary oviparous phlebobranchs whose larvae can survive for ten days or disperse up to 1.5 km away (Svane and Young, 1989; Havenhand, 1991). In conclusion, while the colonial lifestyle is thought to provide competitive advantages over solitary organisms by allowing for indeterminate growth, colonization of complex microhabitats, or high regenerative capacity (Jackson, 1977; Kott, 1989; Dias et al., 2008; Hiebert et al., 2019), when a colonial ascidian such as P. zorritensis is in an environment where asexual propagation is hindered, the relatively few poorly dispersing larvae may limit its ability to compete for space with other fouling benthic organisms.
Conclusions
Understanding the ecological tolerance of P. zorritensis is crucial for global invasive potential forecasting, aiding targeted conservation efforts in high-priority ecosystems (Locke, 2009; Nichols et al., 2023). Salinity affects sexual and asexual reproduction, while previous research shows its impact on spherule and zooid survival under temperature stress (Hiebert et al., 2022). These findings, coupled with the predicted rise in Mediterranean Sea salinity (Parras-Berrocal et al., 2020), suggest a limited expansion potential of P. zorritensis. However, further research is required to characterize its ecological tolerance range, especially regarding the unknown impact of temperature on colony growth.
Data availability statement
The original contributions presented in the study are included in the article/Supplementary Material. Further inquiries can be directed to the corresponding author.
Ethics statement
Ethical approval was not required for the study involving animals in accordance with the local legislation and institutional requirements because the used species is not under restriction for ethical purpose.
Author contributions
VT-S: Conceptualization, Data curation, Formal analysis, Investigation, Writing – original draft, Writing – review & editing. RA-P: Conceptualization, Data curation, Formal analysis, Investigation, Writing – review & editing. DEG: Data curation, Formal analysis, Investigation, Writing – review & editing. ML: Formal analysis, Investigation, Writing – review & editing. ST: Conceptualization, Supervision, Writing – original draft, Writing – review & editing. AA: Conceptualization, Data curation, Formal analysis, Investigation, Supervision, Writing – original draft, Writing – review & editing.
Funding
The author(s) declare financial support was received for the research, authorship, and/or publication of this article. This study was supported by Sorbonne University EMERGENCE 2021/22 and INSB-DBM2021-2024.
Acknowledgments
We would like to thank the “Service Aquariologie” of CRB (IMEV-FR3761) supported by EMBRC-France for the help with the marine-culture facility. We warmly thank Laurent Gilleta for assistance with animal collection and husbandry. We are also grateful to the team of Assonautica marina (La Spezia) and to lab members who helped collecting P. zorritensis: Irene Kopelman, Manon Boosten, Marta Scelzo, Laurel Hiebert and Sonia Lotito. We thank Laurel Hiebert and Roland Zimm for advice on the manuscript. Finally, we thank Alexandre Jan and Christian Sardet for their help and advice on the photographic setup.
Conflict of interest
The authors declare that the research was conducted in the absence of any commercial or financial relationships that could be construed as a potential conflict of interest.
Publisher’s note
All claims expressed in this article are solely those of the authors and do not necessarily represent those of their affiliated organizations, or those of the publisher, the editors and the reviewers. Any product that may be evaluated in this article, or claim that may be made by its manufacturer, is not guaranteed or endorsed by the publisher.
Supplementary material
The Supplementary Material for this article can be found online at: https://www.frontiersin.org/articles/10.3389/fevo.2024.1332780/full#supplementary-material
Supplementary Figure 1 | Example of asexual reproduction photo documentation; (A) First day of image acquisition, when the zooid starts shooting stolons (seven days after the experiment’s start); (B) Intermediate stage of the experiment; (C) Last day of image acquisition, at which the experiment was stopped.
Supplementary Figure 2 | (A) The zooid size is the result from the sum of two measures: 1. oral siphon length, 2. zooid body (from the base to the region between the two siphons; (B) Zooid dissection for embryos and gonads counting. (B’) Dissected zooid showing the location of gonads.
Supplementary Figure 3 | Larvae released by the zooids during the nine days prior sample fixation.
References
Abbott D. P., Newberry A. T., Morris K. M. (1997). Section 6B: Ascidians (Urochordata). Reef and Shore Fauna of Hawaii Vol. 64 (Honolulu, Hawai’i: Bishop Museum Special Publ).
Alié A., Hiebert L. S., Simion P., Scelzo M., Prünster M. M., Lotito S., et al. (2018). Convergent acquisition of nonembryonic development in styelid ascidians. Mol. Biol. Evol. 35 (7), 1728–1743. doi: 10.1093/molbev/msy068
André F., Girard P., Alié A. (2021) DORIS, 08/07/2021: Polyandrocarpa zorritensis (Van Name 1931). Available at: https://doris.ffessm.fr/ref/specie/5004.
Audouin J. (1962). Hydrologie de l’étang de Thau. Revue des Travaux de l’Institut des Pêches Maritimes 26, 5–104.
Berrill N. J. (1935). Studies in tunicate development. Part IV. Asexual reproduction. Philos. Trans. R. Soc. London Ser. B Biol. Sci. 225 (526), 327–379. doi: 10.1098/rstb.1935.0014
Brunetti R. (1978). Polyandrocarpa zorritensis (Van name 1931) A colonial ascidian new to the mediterranean record. Vie Milieu XXVIII–XXIX (AB), 647–652.
Brunetti R. (1984). Combined Effects of Temperature and Salinity on Colonies of Botryllus schlosseri and Botfylloides leachi (Ascidiacea) from the Venetian Lagoon. Mar. Ecol. Prog. Ser. 2 (4), 303–314. doi: 10.3354/meps002303
Brunetti R., Mastrototaro F. (2004). The non-indigenous stolidobranch ascidian Polyandrocarpa zorritensis in the mediterranean: description, larval morphology and pattern of vascular budding. Zootaxa 528 (1), 1. doi: 10.11646/zootaxa.528.1.1
Bullard S. G., Lambert G., Carman M. R., Byrnes J., Whitlatch R. B., Ruiz G., et al. (2007). The colonial ascidian didemnum sp. A: current distribution, basic biology and potential threat to marine communities of the Northeast and West coasts of North America. J. Exp. Mar. Biol. Ecol. 342 (1), 99–108. doi: 10.1016/j.jembe.2006.10.020
Carballo J. L. (2000). Larval ecology of an ascidian tropical population in a mediterranean enclosed ecosystem. Mar. Ecol. Prog. Ser. 195, 159–167. doi: 10.3354/meps195159
Cardeccia A., Marchini A., Occhipinti-Ambrogi A., Galil B., Gollasch S., Minchin D., et al. (2018). Assessing biological invasions in European seas: biological traits of the most widespread non-indigenous species. Estuarine Coast. Shelf Sci. 201, 17–28. doi: 10.1016/j.ecss.2016.02.014
Carman M., Bullard S., Rocha R., Lambert G., Dijkstra J., Roper J., et al. (2011). Ascidians at the Pacific and Atlantic entrances to the Panama canal. Aquat. Invasions 6 (4), 371–380. doi: 10.3391/ai.2011.6.4.02
Castilla J. C., Guiñez R., Caro A. U., Ortiz V. (2004). Invasion of a rocky intertidal shore by the tunicate pyura praeputialis in the bay of antofagasta, Chile. Proc. Natl. Acad. Sci. 101 (23), 8517–8524. doi: 10.1073/pnas.0401921101
Chaigneau A., Dominguez N., Eldin G., Vasquez L., Flores R., Grados C., et al. (2013). Near-coastal circulation in the Northern humboldt current system from shipboard ADCP data: CIRCULATION OF THE NHCS FROM SADCP DATA. J. Geophys. Res.: Oceans 118 (10), 5251–5266. doi: 10.1002/jgrc.20328
Chebbi N., Mastrototaro F., Missaoui H. (2010). Spatial distribution of ascidians in two Tunisian lagoons of the Mediterranean sea. Cahiers Biol. Mar. 51 (2), 117–127.
Clarke Murray C., Gartner H., Gregr E. J., Chan K., Pakhomov E., Therriault T. W. (2014). Spatial distribution of marine invasive species: environmental, demographic and vector drivers. Diversity Distrib. 20 (7), 824–836. doi: 10.1111/ddi.12215
Clarke Murray C., Pakhomov E. A., Therriault T. W. (2011). Recreational boating: A large unregulated vector transporting marine invasive species: transport of NIS by recreational boats. Diversity Distrib. 17 (6), 1161–1172. doi: 10.1111/j.1472-4642.2011.00798.x
Cognetti G., Maltagliati F. (2000). Biodiversity and adaptive mechanisms in brackish water fauna. Mar. pollut. Bull. 40 (1), 7–14. doi: 10.1016/S0025-326X(99)00173-3
Coll M., Piroddi C., Steenbeek J., Kaschner K., Lasram F. B. R., Aguzzi J., et al. (2010). The biodiversity of the Mediterranean Sea: estimates, patterns, and threats. PloS One 5 (8), e11842. doi: 10.1371/journal.pone.0011842
Crean A. J., Marshall D. J. (2015). Eggs with larger accessory structures are more likely to be fertilized in both low and high sperm concentrations in styela plicata (Ascidiaceae). Mar. Biol. 162 (11), 2251–2256. doi: 10.1007/s00227-015-2755-0
Dias G. M., Delboni C. G. M., Duarte L. F. L. (2008). Effects of competition on sexual and clonal reproduction of a tunicate: the importance of competitor identity. Mar. Ecol. Prog. Ser. 362, 149–156. doi: 10.3354/meps07447
Dijkstra J., Dutton A., Westerman E., Harris L. (2008). Heart Rate Reflects Osmotic Stress Levels in Two Introduced Colonial Ascidians Botryllus schlosseri and Botrylloides violaceus. Mar. Biol. 154 (5), 805–811. doi: 10.1007/s00227-008-0973-4
Dybern B. I. (1967). The distribution and salinity tolerance of ciona intestinalis (L.) F. Typica with special reference to the waters around Southern Scandinavia. Ophelia 4 (2), 207–226. doi: 10.1080/00785326.1967.10409621
Dybern B. I. (1969). Distribution and ecology of ascidians in kviturdvikpollen and Vågsböpollen on the West coast of Norway. Sarsia 37 (1), 21–40. doi: 10.1080/00364827.1969.10411143
Epelbaum A., Herborg L. M., Therriault T. W., Pearce C. M. (2009). Temperature and salinity effects on growth, survival, reproduction, and potential distribution of two non-indigenous botryllid ascidians in British Columbia. J. Exp. Mar. Biol. Ecol. 369 (1), 43–52. doi: 10.1016/j.jembe.2008.10.028
Ferrario J., Caronni S., Occhipinti-Ambrogi A., Marchini A. (2017). Role of commercial harbours and recreational marinas in the spread of non-indigenous fouling species. Biofouling 33 (8), 651–660. doi: 10.1080/08927014.2017.1351958
Gewing M.-T., Goldstein E., Buba Y., Shenkar N. (2018). Temperature resilience facilitates invasion success of the solitary ascidian herdmania momus. Biol. Invasions 21 (2), 349–361. doi: 10.1007/s10530-018-1827-8
Gewing M.-T., López-Legentil S., Shenkar N. (2017). Anthropogenic factors influencing invasive ascidian establishment in natural environments. Mar. Environ. Res. 131, 236–242. doi: 10.1016/j.marenvres.2017.10.001
Gewing M.-T., Shenkar N. (2017). Monitoring the magnitude of marine vessel infestation by non-indigenous ascidians in the Mediterranean. Mar. pollut. Bull. 121 (1–2), 52–59. doi: 10.1016/j.marpolbul.2017.05.041
Gordon T., Roth L., Caicci F., Manni L., Shenkar N. (2020). Spawning induction, development and culturing of the solitary ascidian polycarpa mytiligera, an emerging model for regeneration studies. Front. Zool. 17 (1), 19. doi: 10.1186/s12983-020-00365-x
Granot I., Shenkar N., Belmaker J. (2017). Habitat niche breadth predicts invasiveness in solitary ascidians. Ecol. Evol. 7 (19), 7838–7847. doi: 10.1002/ece3.3351
Gröner F., Lenz M., Wahl M., Jenkins S. R. (2011). Stress resistance in two colonial ascidians from the Irish sea: the recent invader didemnum vexillum is more tolerant to low salinity than the cosmopolitan diplosoma listerianum. J. Exp. Mar. Biol. Ecol. 409 (1–2), 48–52. doi: 10.1016/j.jembe.2011.08.002
Havenhand J. N. (1991). Fertilisation and the potential for dispersal of gametes and larvae in the solitary ascidian ascidia Mentula Müller. Ophelia 33 (1), 01–15. doi: 10.1080/00785326.1991.10429738
Hiebert L. S., Scelzo M., Alié A., De Tomaso A. W., Brown F. D., Tiozzo S. (2022). Comparing dormancy in two distantly related tunicates reveals morphological, molecular, and ecological convergences and repeated co-option. Sci. Rep. 12 (1), 12620. doi: 10.1038/s41598-022-16656-8
Hiebert L. S., Vieira E. A., Dias G. M., Tiozzo S., Brown F. D. (2019). Colonial ascidians strongly preyed upon, yet dominate the substrate in a subtropical fouling community. Proc. R. Soc. B 286 (1903), 20190396. doi: 10.1098/rspb.2019.0396
Iwasaki K., Kimura T., Kinoshita K., Yamaguchi T., Nishikawa T., Nishi E., et al. (2004). Human-mediated introduction and dispersal of marine organisms in Japan: Results of a questionnaire survey by the Committee for the Preservation of the Natural Environment, the Japanese Association of Benthology. Japan. J. Benthol. 59 (1), 22–44. doi: 10.5179/benthos.59.22
Izquierdo-Muñoz A. (2009). Recent non-indigenous ascidians in the Mediterranean Sea. Aquat. Invasions 4 (1), 59–64. doi: 10.3391/ai.2009.4.1.5
Jackson J. B. C. (1977). Competition on marine hard substrata: The adaptive significance of solitary and colonial strategies. Am. Nat. 111, 743–767. doi: 10.1086/283203
Lambert C. C., Lambert G. (1998). Non-indigenous ascidians in Southern California harbors and marinas. Mar. Biol. 130 (4), 675–688. doi: 10.1007/s002270050289
Lambert C. C., Lambert G. (2003). Persistence and differential distribution of nonindigenous ascidians in harbors of the Southern California bight. Mar. Ecol. Prog. Ser. 259, 145–161. doi: 10.3354/meps259145
Lambert G. (2002). Nonindigenous ascidians in tropical waters. Pacific Sci. 56 (3), 291–298. doi: 10.1353/psc.2002.0026
Lambert G. (2019). Fouling ascidians (Chordata: ascidiacea) of the galápagos: Santa Cruz and Baltra Islands. Aquat. Invasions 14 (1), 132–149. doi: 10.3391/ai.2019.14.1.05
Lambert G., Faulkes Z., Lambert C. C., Scofield V. L. (2005). Ascidians of South Padre Island, Texas, with a key to species. Texas J. Sci. 57, 251–262.
Locke A. (2009). A screening procedure for potential tunicate invaders of Atlantic Canada. Aquat. Invasions 4 (1), 71–79. doi: 10.3391/ai.2009.4.1.7
López-Legentil S., Legentil M. L., Erwin P. M., Turon X. (2015). Harbor networks as introduction gateways: contrasting distribution patterns of native and introduced ascidians. Biol. Invasions 17 (6), 1623–1638. doi: 10.1007/s10530-014-0821-z
Mastrototaro F., D’Onghia G., Tursi A. (2008). Spatial and seasonal distribution of ascidians in a semi-enclosed basin of the Mediterranean Sea. J. Mar. Biol. Assoc. United Kingdom 88 (5), 1053–1061. doi: 10.1017/S0025315408001392
Millar R. H. (1958). Some ascidians from Brazil. Ann. Mag. Natural History 1 (8), 497–514. doi: 10.1080/00222935808650975
Monniot F. (2016). A new species of Polyandrocarpa (Ascidiacea, styelidae) in the Mediterranean sea. Zootaxa 4132 (1), 87. doi: 10.11646/zootaxa.4132.1.7
Monniot F. (2018). Ascidians collected during the madibenthos expedition in Martinique: 2. Stolidobranchia, styelidae. Zootaxa 4410 (2), 291. doi: 10.11646/zootaxa.4410.2.3
Nagar L., Shenkar N. (2016). Temperature and salinity sensitivity of the invasive ascidian microcosmus exasperatus Helle. Aquat. Invasions 11 (1), 33–43. doi: 10.3391/ai.2016.11.1.04
Nichols C. L., Lambert G., Nydam M. L. (2023). Continued persistence of non-native ascidians in Southern California harbors and marinas. Aquat. Invasions 18 (1), 1–22. doi: 10.3391/ai.2023.18.1.101962
Nishikawa T., Kajiwara Y., Kawamura K. (1993). Probable introduction of Polyandrocarpa zorritensis (Van Name) to Kitakyushu and Kochi. Japan. Zool. Sci. Suppl. Tokyo 10, 176.
Otani M. (2002). Appearance and latest trends of introduced marine sessile animals in Japanese waters. Sessile Org 19, 69–92. doi: 10.4282/sosj.19.69
Parras-Berrocal I. M., Vazquez R., Cabos W., Sein D., Mañanes R., Perez-Sanz J., et al. (2020). The climate change signal in the Mediterranean Sea in a regionally coupled atmosphere–ocean model. Ocean Sci. 16, 743–765. doi: 10.5194/os-16-743-2020
Pineda M. C., Turon X., López-Legentil S. (2012). Stress levels over time in the introduced ascidian styela plicata: the effects of temperature and salinity variations on hsp70 gene expression. Cell Stress Chaperones 17 (4), 435–444. doi: 10.1007/s12192-012-0321-y
Platin R., Shenkar N. (2023). Can Stand the Heat – Ecology of the Potentially Invasive Ascidian Styela plicata along the Mediterranean Coast of Israel. Front. Mar. Sci. 10. doi: 10.3389/fmars.2023.1159231
Rocha R. M., Castellano G. C., Freire C. A. (2017). Physiological tolerance as a tool to support invasion risk assessment of tropical ascidians. Mar. Ecol. Prog. Ser. 577, 105–119. doi: 10.3354/meps12225
Scelzo M., Alexandre A., Pagnotta S., Lejeune C., Henry P., Gilletta L., et al. (2019). Novel budding mode in Polyandrocarpa zorritensis: A model for comparative studies on asexual development and whole body regeneration. EvoDevo 10 (1), 7. doi: 10.1186/s13227-019-0121-x
Shenkar N., Loya Y. (2008). The solitary ascidian herdmania momus: native (Red sea) versus non-indigenous (Mediterranean) populations. Biol. Invasions 10 (8), 1431–1439. doi: 10.1007/s10530-008-9217-2
Sims L. L. (1984). Osmoregulatory capabilities of three macrosympatric stolidobranch ascidians, Styela clava Herdman, S. plicata (Lesueur), and S. montereyensis (Dall). J. Exp. Mar. Biol. Ecol. 82 (2–3), 117–129. doi: 10.1016/0022-0981(84)90098-4
Stabili L., Licciano M., Longo C., Lezzi M., Giangrande A. (2015). The Mediterranean non-indigenous ascidian Polyandrocarpa zorritensis: microbiological accumulation capability and environmental implications. Mar. pollut. Bull. 101 (1), 146–152. doi: 10.1016/j.marpolbul.2015.11.005
Streit O. T., Lambert G., Erwin P. M., López-Legentil S. (2021). Diversity and abundance of native and non-native ascidians in Puerto Rican harbors and marinas. Mar. pollut. Bull. 167, 112262. doi: 10.1016/j.marpolbul.2021.112262
Svane I., Young C. M. (1989). The ecology and behaviour of ascidian larvae. Oceanogr. Mar. Biol.: an Annu. Rev. 27, 45–90.
Tempesti J., Langeneck J., Romani L., Garrido M., Lardicci C., Maltagliati F., et al. (2022). Harbour type and use destination shape fouling community and non-indigenous species assemblage: A study of three Northern Tyrrhenian port systems (Mediterranean sea). Mar. pollut. Bull. 174, 113191. doi: 10.1016/j.marpolbul.2021.113191
Turon X., Perera M. (1988). Las ascidias del Delta del Ebro. Aspectos faunísticos y cuantitativos. P. Dept. Zool. Barcelona 14, 81–90.
Ulman A., Ferrario J., Forcada A., Arvanitidis C., Occhipinti-Ambrogi A., Marchini A. (2019a). A Hitchhiker’s guide to Mediterranean marina travel for alien species. J. Environ. Manage. 241, 328–339. doi: 10.1016/j.jenvman.2019.04.011
Ulman A., Ferrario J., Forcada A., Seebens H., Arvanitidis C., Occhipinti-Ambrogi A., et al. (2019b). Alien species spreading via biofouling on recreational vessels in the Mediterranean sea. J. Appl. Ecol. 56 (12), 2620–2629. doi: 10.1111/1365-2664.13502
Ulman A., Ferrario J., Occhipinti-Ambrogi A., Arvanitidis C., Bandi A., Bertolino M., et al. (2017). A massive update of non-indigenous species records in Mediterranean marinas. PeerJ 5, e3954. doi: 10.7717/peerj.3954
Vazquez E., Ramos-Espla A. A., Turon X. (1995). The genus polycarpa (Ascidiacea, styelidae) on the Atlantic and Mediterranean coasts of the Iberian Peninsula. J. Zool. 237 (4), 593–614. doi: 10.1111/j.1469-7998.1995.tb05017.x
Vázquez E., Young C. M. (1996). Responses of compound ascidian larvae to haloclines. Mar. Ecol. Prog. Ser. 133, 179–190. doi: 10.3354/meps133179
Vázquez E., Young C. M. (2000). Effects of low salinity on metamorphosis in estuarine colonial ascidians. Inverteb. Biol. 119 (4), 433–444. doi: 10.1111/j.1744-7410.2000.tb00113.x
Villa L. A., Patricolo E. (2000). The follicle cells of styela plicata (Ascidiacea, tunicata): A sem study. Zool. Sci. 17 (8), 1115–1121. doi: 10.2108/zsj.17.1115
Villalobos S., Lambert G., Shenkar N., López-Legentil S. (2017). Distribution and population dynamics of key ascidians in North Carolina harbors and marinas. Aquat. Invasions 12 (4), 447–458. doi: 10.3391/ai.2017.12.4.03
Virgili R., Tanduo V., Katsanevakis S., Terlizzi F., Villani G., Fontana A., et al. (2022). The miseno lake (Central-Western Mediterranean sea): an overlooked reservoir of non-indigenous and cryptogenic ascidians in a marine reserve. Front. Mar. Sci. 9. doi: 10.3389/fmars.2022.866906
Yamaguchi M. (1975). Growth and reproductive cycles of the marine fouling ascidians ciona intestinalis, styela plicata, botrylloides violaceus, and leptoclinum mitsukurii at aburatsubo-moroiso inlet (Central Japan). Mar. Biol. 29 (3), 253–259. doi: 10.1007/BF00391851
Keywords: tunicate, development, budding, salinity, invasive, non-indigenous species
Citation: Tobias-Santos V, Andreoni-Pham R, El Gharbi D, Lebel M, Tiozzo S and Alié A (2024) Salinity-mediated limitation of asexual reproduction in the colonial ascidian Polyandrocarpa zorritensis. Front. Ecol. Evol. 12:1332780. doi: 10.3389/fevo.2024.1332780
Received: 03 November 2023; Accepted: 24 January 2024;
Published: 15 February 2024.
Edited by:
Paolo Sordino, Anton Dohrn Zoological Station Naples, ItalyReviewed by:
Maria Flavia Gravina, University of Rome Tor Vergata, ItalyFederica Montesanto, University of Florida, United States
Copyright © 2024 Tobias-Santos, Andreoni-Pham, El Gharbi, Lebel, Tiozzo and Alié. This is an open-access article distributed under the terms of the Creative Commons Attribution License (CC BY). The use, distribution or reproduction in other forums is permitted, provided the original author(s) and the copyright owner(s) are credited and that the original publication in this journal is cited, in accordance with accepted academic practice. No use, distribution or reproduction is permitted which does not comply with these terms.
*Correspondence: Alexandre Alié, YWxleGFuZHJlLmFsaWVAaW1ldi1tZXIuZnI=
†These authors share first authorship