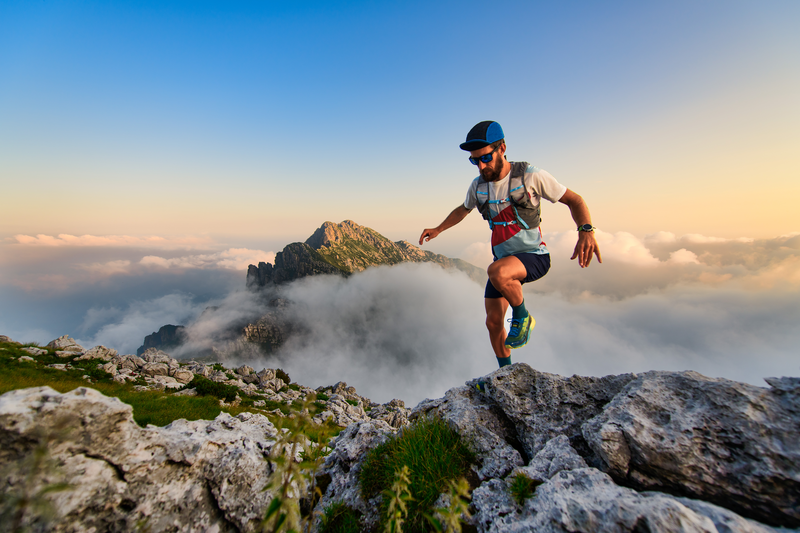
95% of researchers rate our articles as excellent or good
Learn more about the work of our research integrity team to safeguard the quality of each article we publish.
Find out more
ORIGINAL RESEARCH article
Front. Ecol. Evol. , 26 January 2024
Sec. Population, Community, and Ecosystem Dynamics
Volume 12 - 2024 | https://doi.org/10.3389/fevo.2024.1325275
This article is part of the Research Topic The Interactions Between Invasive Species and Resident Communities Under Environmental Change View all articles
Forests support a variety of functions essential for the provisioning of ecosystem services. However, invasive plants can disrupt forest functioning, particularly in temperate forest understories that commonly experience invasive plant encroachment, by altering community-level trait distributions. Invasive plants, frequently characterized by acquisitive traits such as higher specific leaf area and nitrogen content, may either outcompete native species with different trait values (displacement), or add trait values to the existing community (complementarity). Therefore, complementarity may still allow restoration of the original community function by removing invasive species, and consequently the added provision of function when compared to the natives, but displacement may not. While understanding which process occurs is critical in managing ecosystem functionality, little is known about the impact of invasion on the co-occurring native community trait distributions. To address this knowledge gap, we leveraged survey data on temperate forest understories that varied in their degree of invasion (i.e., gradient of invasion). We calculated the community-weighted mean of three leaf traits: leaf nitrogen, specific leaf area, and leaf dry matter content – traits strongly linked to invasion, sensitive to environmental changes, and that affect ecosystem functions. Our findings show that increasing invasion had a significant positive effect on total (native and invasive) community leaf nitrogen, but did not alter native community trait distributions. This suggests that potential impacts on ecosystem functions, such as increased primary productivity and nitrogen cycling, would be primarily driven by trait complementarity and not displacement. We did not find any significant differences in the total or native communities with respect to specific leaf area or leaf dry matter content. Differentiating trait displacement from trait complementarity contributes to our understanding of how invasion affects functionality of understory plant communities in forests. Additionally, it provides a valuable framework to facilitate evidence-based decisions for the management of ecosystem functionality.
Forests play a crucial role in providing a multitude of ecosystem services, including carbon storage, water supply, pollination, food production and climate regulation (Thompson et al., 2011; Decocq et al., 2016; Liang et al., 2016; Brockerhoff et al., 2017; Mori et al., 2017; Gauthier et al., 2023). These services are supported by ecosystem functions, e.g., net primary productivity, decomposition, and nutrient cycling, which arise from the interaction and arrangement of biotic and abiotic components and ecosystem processes (Brockerhoff et al., 2017). The primary biotic component of forest ecosystems is the plant community (Wallace, 2007; Fu et al., 2013). Alterations in plant community composition, species relative abundance, and trait distributions (i.e., within community pattern of species traits) as a result of plant invasions are expected to modify the functionality of these ecosystems (Chacón-Labella et al., 2023; Girona et al., 2023). Understanding the nature of these alterations to native ecosystems can inform management approaches geared at maintaining function. On one hand, if invasive plants possessing distinct traits compared to traits of native species are displacing the latter, the removal of invasive species might not lead to a full recovery of the system’s function. On the other hand, if invasive plants are complementing, i.e., adding to the native community, their removal may return functionality to its original level. While research has extensively documented linkages between traits and ecosystem functions [EFs; e.g., (Lavorel and Garnier, 2002; Lavorel and Grigulis, 2012; van der Plas et al., 2020; Hagan et al., 2023)], there is little knowledge on how invasive species impact EFs through changes in the trait distribution of natives or of total communities, natives plus invasives (Jo et al., 2015; Lee et al., 2017; Livingstone et al., 2020).
The long-term persistence of forests relies on the maintenance of their structure, composition and diversity. Although most of the focus is on the canopy due to its role in carbon storage and acting as a carbon sink during growth (Pan et al., 2011; Castro et al., 2021), understories play a crucial role for tree recruitment and regeneration, as well as harboring most of the forest biodiversity (Landuyt et al., 2019; Spicer et al., 2020). However, introduced plants often dominate forest understories when they become invasive, leading to biodiversity loss, suppression of tree recruitment, and impacts on ecosystem functions and services (Mack et al., 2000; Simberloff et al., 2013; Link et al., 2018; Díaz et al., 2019). Invasive plants can disrupt some of these ecosystem functions (Ehrenfeld, 2003; Castro-Díez et al., 2014; Seabloom et al., 2015; Lee et al., 2017; Parra-Tabla and Arceo-Gómez, 2021) mostly via alterations of competitive hierarchies within the total community, and within the native community (Pysek et al., 2012). These invasions could then alter functional trait distributions and, therefore, lead to changes in the functionality of ecosystems which should be reflected in the makeup of both the native community and the total community (Díaz and Cabido, 2001; Lavorel and Garnier, 2002).
Plant traits can be aggregated into ecological strategies (Weigelt et al., 2021). One of the most well-established strategies is related to the conservation gradient in leaf traits, the leaf economic spectrum, LES (Wright et al., 2004). The LES describes a resource use trade-off from the acquisitive, ‘fast’ end, to conservative strategies, or ‘slow’ end in terms of carbon processing (Wright et al., 2004; Weigelt et al., 2021). Within the LES commonly studied traits are high leaf nitrogen (leaf N), and high specific leaf area (SLA) representing the ‘fast’ end and high leaf dry matter content (LDMC) representing the ‘slow’ end. These three traits are considered both response and effect traits as they respond to resource availability and have effects on ecosystem properties (Díaz and Cabido, 2001; Lavorel and Garnier, 2002). The correlation of traits at the ends of the LES leads to the expectation that multiple traits are associated with a given ecosystem function (Chacón-Labella et al., 2023). For example, increases in both leaf N and SLA have positive links with key ecosystem functions, including aboveground productivity (Lavorel and Garnier, 2002; Garnier et al., 2004; Violle et al., 2007; Reich, 2012), leaf decomposition rates (Cornwell et al., 2008; Pietsch et al., 2014; Jo et al., 2016), N cycling (mainly, nitrification), soil N pools, and soil C pools (Ehrenfeld, 2003; Orwin et al., 2010; Laughlin, 2011; Lienin and Kleyer, 2012; Castro-Díez et al., 2014; Lee et al., 2017). Conversely, increases in LDMC are negatively associated with aboveground productivity (Lavorel and Garnier, 2002; Garnier et al., 2004), and nitrification rates (Laughlin, 2011). Therefore, trait covariation should be accounted for as a pathway to improve the accuracy of predicted impacts of invasion on forest functionality.
Invasive plants usually possess traits that confer advantages in resource acquisition and use, such as higher values of SLA, foliar nitrogen per mass and maximum photosynthetic rate per mass when compared to native species [Figure 1A, (Ordonez and Olff, 2013; van Kleunen et al., 2015; Montesinos, 2022)], facilitating them to dominate the community (Bernard-Verdier and Hulme, 2019). By dominating the forest understory, invasive species might either locally extirpate native species displaying certain traits (Figure 1C) or favor native species with similar traits (Figure 1B). Both situations would alter the levels of ecosystem functionality that the native community provides. When competitive advantages lead to native community displacement, the potential for amplified effects on ecosystem functions arises (Vilà et al., 2011; Vilà and Hulme, 2017). However, if invasive species do not alter the trait distribution of the native community, even if the native species composition differs across a gradient of invasion, but rather adds to or complements it, then the impact of invasion on functionality is limited to the dominance of invasive species, and the native community continues to provide similar functionality (Figure 1D). These pathways accentuate the need to understand the interplay between increasing levels of invasion, trait distributions, and ecosystem function.
Figure 1 Conceptual figure of hypothesized ways in which invasion can affect total and native community functionality. CWM = community weighted mean of a generic trait. Lines represent hypothesized potential responses of the native community CWM of a generic trait with increasing invasive species cover (gradient of invasion). Dash types are used for visual differentiation among potential responses of the native community.
The extent to which invasive species alter community trait distribution, particularly, of the native community and the potential implications of these changes in ecosystem functions have only recently been considered (Livingstone et al., 2020; Fernandez et al., 2021). Effects of invasion on native communities are usually quantified by comparing invaded and non-invaded communities [e.g., (Dostal et al., 2013; Gallien and Carboni, 2017)]. While this approach captures the changes in the community, it does not isolate the changes in the co-occurring native community (Thomsen et al., 2016). By isolating the impact on the native communities, some studies have found that invasion does not always impact native communities [e.g., (Livingstone et al., 2020; Fernandez et al., 2021; Parra-Tabla and Arceo-Gómez, 2021)]. As a result, recent literature recommends excluding invasive species from response metrics (e.g., diversity, similarity, abundance, trait values) to understand the net impact of invasive species on the trait distributions of the co-occurring native community (Thomsen et al., 2016) with increasing levels of invasion. In our study, we adopted this framework, i.e., analysis of community weighted mean trait values calculated with and without the invasive species, to assess whether the traits of the native community changed or not in response to invasion and what kind of change took place – where invasion could result in increases, decreases or no change in total and native community trait values (Figure 1).
In this study, we surveyed plant communities in temperate forest understories across a gradient of invasion, and linked these surveys to trait data of leaf N, SLA, and LDMC from the TRY database (Kattge et al., 2011). We then investigated the effect of invasive species on both modifying total community and native community trait distributions, by quantifying changes in functional trait at the community level. Such changes could then result in potential alterations in ecosystem function as a consequence of invasion. Specifically, we asked: (Q1) how does the total, native and invasive, community change in trait functionality with invasion? (Q2) How does invasion impact native community trait distribution? Due to resource limitations, particularly of water, light, and nutrients resulting from intense competition among trees and among trees and understory plants in forest ecosystems, we hypothesize that the predominant mechanism influencing changes in community trait values and, therefore, ecosystem functionality, following invasion is complementarity rather than displacement. This expectation is based on the premise that highly acquisitive invasive species may face challenges thriving in low-resource environments typical of forest understories (Martin et al., 2009). By understanding the effects of invasion-driven changes in community functionality through alterations in trait distributions, we can improve our ability to predict the consequences of invasions on ecosystems and tailor management practices.
We conducted this study at the Edwin S. George Reserve (ESGR), a 525-ha ecological reserve in southeast Michigan (-84.022079 W, 42.457242 N). ESGR features a deciduous temperate forest primarily dominated by oak (Quercus spp.) and hickory (Carya spp.), with common sub-canopy species being red maple (Acer rubrum L.), black cherry (Prunus serotina Ehrh.), and witch-hazel (Hamamelis virginiana L.) (Allen et al., 2020). The most prevalent invasive species in the study area are the shrubs autumn-olive (Elaeagnus umbellata Thunb.), and barberry (Berberis thunbergii DC.) and the vine multiflora rose (Rosa multiflora Murray; see Supplementary Table S1 for a full list of invasive species based on our survey). The soils at ESGR are Boyer–Oshtemo sandy loam or Miami loam in areas above 275 m, and Carlisle and Rifle muck otherwise (Allen et al., 2020). The climate generally is defined as warm-summer humid continental (Allen et al., 2020). In this area, from 1991 to 2020, the mean annual temperature is 8.7°C, with a minimum mean temperature in January of -4.7°C and a maximum in July of 21.3°C, the average annual rainfall is 822 mm (Arguez et al., 2010).
To understand how plant communities in temperate forests may change their functionality under invasion, we carried out an observational field study in summer 2021 and sampled plant communities in forest understories with different levels of invasion. We assessed species identity, native or invasive status, and their abundance. We then calculated the community weighted mean for three representative leaf functional traits: leaf nitrogen, specific leaf area, and leaf dry matter content.
In the summer of 2021, we selected 9 forest patches within ESGR with varying invasive species cover (i.e., from non-invaded to heavily invaded), canopy openness and soil water conditions (Supplementary Figure S1; Supplementary Table S2). Within each forest, we installed three transects 50 x 2 m positioned at least 50 m apart (see Supplementary Figure S2 for a diagram of the transect set up). We further divided each transect into five 20 m2 plots, yielding a total of 135 plots. We sampled two forest strata [classification modified from Spicer et al. (2020)], understory and forest floor, where plants were identified to species based on Taxonomic Name Resolution Service (Boyle et al., 2013). For the understory stratum, we collected data in five 5 m2 subplots per transect on shrubs and trees 0.5 m< height< 2m. In this stratum, we recorded the maximum diameter and the diameter at a 90-degree angle to maximum for each individual. We then calculated crown area as an ellipse based on the two diameters, summed areas by species and obtained abundance data as the percent overlap of each species’ crown area to the plot area. To sample the forest floor, we visually estimated percent cover (with a precision of 1%) of all growth forms with height > 0.5 in five 1 m2 subplots. No individual stratum exceed 100% cover, but the cumulative species-level cover summed across strata could surpass 100% cover. We assigned nativity (i.e., native vs. introduced/invasive) following Reznicek et al. (2014), and considered all introduced species as invasive species given our knowledge of the studied area.
We obtained species level trait data on leaf N (mg/g), specific leaf area (SLA; mm2/mg) and leaf dry matter content (LDMC; g/g, oven-dry mass of leaf/water-saturated fresh mass of leaf) from TRY database (Kattge et al., 2011). Species values were obtained in a two-step process. First, from trait values obtained from TRY, we calculated the mean value of each trait for each site. Second, we obtained an overall species trait value by taking the mean across site means. We then used these species-level values to compute the community weighted mean (CWM) – the average trait value of species within a community (Lavorel et al., 2008; Le Bagousse-Pinguet et al., 2017). The CWM is determined by weighting individual trait values by the relative abundance (e.g., percent cover) of each species. For each trait we calculated CWM of each plot for (a) total community, encompassing both invasive and native species, and (b) native community, consisting of native species only. To maintain a representative sample of each plot-level community, we analyzed only plots with a minimum of 80% of cover with associated trait values across all three traits as suggested by Pakeman and Quested (2007). The plot-level Pielou’s Evenness index, which quantifies the evenness of species abundance distribution in a community, was derived from the Shannon index (i.e., a measure of species diversity of the community, with higher values representing communities with high species richness and a relatively even distribution of individuals among species) divided by the logarithm of richness. A Pielou’s Evenness index value of 1 indicates maximum evenness in species abundance distribution (i.e., all species are equally abundant within a community). The Shannon index was obtained via the ‘vegan’ package (Oksanen et al., 2022). Given the aforementioned criteria, we analyzed data from 90 plots as some had no plants (n = 2 plots), or only one species (n = 3 plots) which makes the calculation of the Pielou’s Evenness Index undefined, and insufficient cover with associated trait values across all three traits (n = 40 plots).
We collected all environmental data during the summer of 2021 (see Supplementary Table S1 for summary data). To estimate light availability (% of full sun), we took canopy photos using a fish-eye lens attached to a tripod-mounted smartphone positioned at a height of 1.2 m at three points within each plot in August. Photos were analyzed following methods outlined by Bianchi et al. (2017). To estimate soil water availability, we measured soil volumetric water content (%) in May, June and August using the Fieldscout TDR300 Soil Moisture Meter at the same locations we took canopy photos within each plot (see Figure S2 for detailed diagram). We obtained one measurement per plot for both light and soil water measurements by calculating the mean and associated standard deviation across the three points. We obtained soil nutrient availability by deploying one ion-exchange resin capsule at a randomly selected transect within each forest. We purchased the capsules from UNIBEST which was also responsible for their analysis. We performed all data wrangling using the family of packages “tidyverse” (Wickham et al., 2019) in R (R Core Team, 2022).
To answer how invasion may affect plant community functionality via changes in trait distribution (Q1), for each plot, we analyzed community weighted mean (CWM) of each trait for the total community (native and invasive; Figure 1 green). We used a multinormal (MNR) likelihood to analyze the three traits simultaneously. We used this approach to account for the established relationships across the three selected traits in the LES (Wright et al., 2004). Plot-level (i) community weighted mean for each trait (k) was estimated as a function of invasive percent cover, to assess the effect of invasion (), of native richness () and Pielou’s Evenness index for the total community () to account for any effects of diversity on trait distributions, and of light () and soil water availability (; we only used August measurements, the driest month) as these resources may also drive the distribution of traits (Chacón-Labella et al., 2023). We also included each forest as different intercepts. The likelihood (or data model):
Being the process model:
Parameter was estimated from an overall intercept value, hyperparameter . Parameter represents a variance-covariance matrix of the three CWM. We estimated all parameters from non-information prior distributions, , and . We calculated correlation values between each pair of CMW () from the covariance and standard deviation in , .
To assess how invasion impacts native community trait distribution (Q2), we ran a similar analysis as above by changing the response variable to the plot-level community weighted mean of each trait for native species only (Figure 1 purple). Among the covariates, we only modified the Pielou’s Evenness index to reflect calculation of the native species only.
We generated model predictions of each CWM, total community and only natives, along a gradient of invasion (i.e., percent cover of invasive species, ranging from uninvaded to heavily invaded) by setting all other model covariates to their mean values. To quantify changes in community functionality due to invasion, total community and only natives, we calculated the difference between the predicted community weighted mean for each trait under its maximum invasion and at no invasion (Figure 1).
We ran the MNR likelihoods in JAGS (Plummer, 2003) through R using “rjags” (Plummer, 2022). We retrieved posterior distributions and parameters values after convergence, using the least 100,000 iterations with a thinning interval of 500. We checked model convergence by visually inspecting plots of posterior distributions of each model parameter retrieved. Lastly, we conducted a simple t-test to compare Pielou’s Evenness index values calculated for the total community (i.e., comprising native and invasive species) with those calculated exclusively for native species. Analysis code is available on GitHub at https://github.com/laispetri/FunctionalChanges.git, and data are available in Figshare at https://doi.org/10.6084/m9.figshare.24415648.v3.
The invasive percent cover, two strata summed: understory and forest floor, varied from 0 (13 plots) to 184% (1 plot), with a mean of 56.1%. The plot-level richness of native species ranged from four (3 plots) to 20 species (1 plot), with a mean of 10 species. The mean value of Pielou’s Evenness index for the total community was 0.69 and for the natives only, 0.73 (statistically significant difference, t(177.79) = 2.97, p< 0.05). At the plot level, light availability ranged from 6.85% to 54.84% of full sunlight across plots. And soil water, measured as volumetric water content, ranged from 2.7% to 20% (see Supplementary Table S2 for summaries of environmental data per forest). The general patterns of trait distributions (i.e., raw data) of leaf N CWM of all species, i.e., total community, indicates a shift toward higher values, whereas no clear distinctions are evident for the native community alone as invasion increases (Figure 2A). For SLA, the mean CWM remains consistent across the invasion gradient, but its variance decreases with higher invasion (Figure 2B). Lastly, both the total and native-only CWMs of LDMC show slightly higher variance with increased invasion, but maintaining a similar overall pattern across the invasion gradient (Figure 2C). All parameter values from the analyses are reported in Supplementary Table S3, and model fit (goodness of fit) in Supplementary Figure S3.
Figure 2 Trait distributions of plot-level community weighted mean (CWM) values [leaf N, leaf nitrogen concentration (A); SLA, specific leaf area (B); LDMC, leaf dry matter content (C)]. Levels of invasion (from lowest to highest, left to right) were defined to contain an equal number of areas (respectively, 29, 34 and 27 plots). Bandwidth is set to Silverman’s ‘rule of thumb’ (Silverman, 2018).
Increase in percent cover of invasive species was positively and significantly associated with total community weighted mean distributions of both leaf N and SLA (Figure 3). Conversely, across all traits, CWM of only natives remained unchanged during invasion (Figure 3). Increases in native richness were significantly associated with a decrease in leaf N community trait distributions irrespective of invasive species being in the CWM estimates (Figure 3). Native species richness was not significantly associated with the CWM of SLA or LDMC, regardless of CWM including or not invasives. Evenness was significant and positively associated with leaf N CWM calculated with natives only; for all other combinations of trait and type of community, associations were not significant. Higher soil water was significantly associated with higher CWM of SLA, regardless of community grouping, with no significant association for any other trait (Figure 3). Light availability had no significant associations with CWMs.
Figure 3 Posterior means and 95% CI of parameter values (standardized for comparisons) of covariates included in the multivariate regression models. Posterior means of covariates, therefore, signify the impact of each covariate on the response variable, i.e., the community weighted mean (CWM) for each trait computed both with (green) and without (purple) invasive species. Leaf N, leaf nitrogen concentration (A); SLA, specific leaf area (B); LDMC, leaf dry matter content (C); Cover Inv, invasive percent cover; Nat richness, native richness. Credible intervals (CI) that do not cross zero are statically significant (solid symbols).
Simulations showed an increase in the total community leaf N with increasing level of invasion (Figure 4A). Total community values of CWM leaf N at maximum invasion were significantly different from those at no invasion (Figure 5A). The total community values of CWM SLA tended to increase with higher levels of invasion (Figure 4B), but the association was not significant (Figure 5A). Total community CWM of LDMC values remained similar across the gradient of invasion (Figure 4C), with no significant difference between CWM of LDMC values at no invasion and maximum invasion (Figure 5A).
Figure 4 Model predictions (line = mean model predictions, shaded area = 95% credible intervals) of community weighted mean (CWM) for each trait and community type across the gradient of invasion. Symbols are calculated CWM at each plot. Leaf N, leaf nitrogen concentration (A); SLA, specific leaf area (B); LDMC, leaf dry matter content (C).
Figure 5 (A) Differences of predicted community weighted mean trait values between maximum invasion and no invasion. (B) Correlation values of each paired trait comparison derived from parameter . Leaf N, leaf nitrogen concentration; SLA, specific leaf area; LDMC, leaf dry matter content. Credible intervals [CI] that do not cross zero are statically significant (solid symbols).
There was a gradual positive slope in the native community CWM SLA values with higher levels of invasion (Figure 4B), whereas values were similar across invasive cover levels for the other two traits (Figures 4A, C). Corroborating these results, the difference between predicted trait values under high invasion and no invasion was not significant for any trait (Figure 5A).
Furthermore, the correlations derived from the variance-covariance matrix of the CWM for the three traits indicate a positive and significant association between CWM leaf N and SLA trait values in communities computed with and without invaders (Figure 5B). Additionally, both CWM of leaf N and of SLA trait values were negatively associated with LDMC, although relationships were not significant (Figure 5B).
Invasive plants can affect the functioning of ecosystems by impacting the growth, abundance, diversity, and richness of native plants (Vilà et al., 2011; Pysek et al., 2012), as well as by altering trait distributions of the co-occurring native community (Loiola et al., 2018; Fried et al., 2019; Sodhi et al., 2019; Livingstone et al., 2020; Fernandez et al., 2021). The consequences of plant invasion on ecosystem functions (EFs) through changes in native community trait distributions have only recently begun to be explored (Livingstone et al., 2020; Fernandez et al., 2021). We investigated these changes by analyzing trait distributions of both total, natives plus invasives, and native communities. Our results revealed a significant and positive change in total community leaf N and a marginal change for SLA due to invasion. In contrast, native community functionality remained largely unchanged (i.e., no loss of function) across the invasion gradient for the traits we analyzed, indicating invasive species are complementing and not displacing native function. Overall, our results provide insights into the extent of alterations in ecosystem functions via community-level responses to invasion.
Traits from plant invaders often show substantial differences compared to their native counterparts (van Kleunen et al., 2010; Ordonez and Olff, 2013). Invasive plants tend to have higher trait values for both leaf N and SLA while maintaining low investment in leaf tissue resulting in low LDMC trait values (Leishman et al., 2007; van Kleunen et al., 2010; Ordonez and Olff, 2013; Henn et al., 2019). These trait differences are particularly relevant in explaining the success and dominance of invasive species in high-resource environments, such as post-disturbance or canopy gaps (Funk, 2013; Jauni et al., 2015; Ibáñez et al., 2021). However, these differences are also present, albeit less frequently, in low-resource environments, such as forest understories (Funk and Vitousek, 2007; Funk, 2013). Our results show that in our study system, temperate forest understories, communities with high dominance of invasive plants were associated with higher levels of leaf N (Figures 4A and 5A), and had a tendency towards higher levels of SLA (Figure 4B). Shifts in total community trait values due to invasion have been documented in other study systems (Cadotte et al., 2017; Livingstone et al., 2020; Fernandez et al., 2021), also suggesting that as invasive species become abundant, community-level trait characteristics change and, therefore, community functionality likely also changes. Increases in leaf N and SLA at the community level have have been linked to positive effects on aboveground biomass, N cycling rates, and soil N pools (Lavorel and Garnier, 2002; Ehrenfeld, 2003; Garnier et al., 2004; Violle et al., 2007; Orwin et al., 2010; Laughlin, 2011; Lienin and Kleyer, 2012; Reich, 2012; Castro-Díez et al., 2014; Lee et al., 2017). And, in fact, we observed a positive association between elevated levels of soil ammonium, soil nitrate, and total soil N with increasing invasion abundance at the forest patch level (Supplementary Table S2).
Contrary to expectations, we found no significant effect of invasion on LDMC (Figures 4C, 5A), suggesting that invasive species exhibit similar strategies with respect to this trait when compared to natives. Invasive species tend to exploit abundant-resource events, such as disturbances or the existence of canopy gaps, as opportunities for colonizing forest understories (Funk, 2013; Gioria and Osborne, 2014). It is likely that regardless of their acquisitive ability, invaders of forest understories also need to have some level of shade tolerance to cope with low-light conditions and become abundant (Funk and Vitousek, 2007; Funk, 2013). A considerable body of evidence underscores that specific invasive plants, when dominant in forest understories possess traits that confer advantage in these low-resource environments [e.g., (Funk and Vitousek, 2007; Heberling and Fridley, 2013; Martinez and Fridley, 2018; Fridley et al., 2022)]. They, for example, invest in leaf construction, e.g., high LDMC, increases leaf lifespan and confers shade tolerance advantages in forested environments (Funk, 2013; Pérez-Harguindeguy et al., 2016). Also, LDMC tends to positively correlate with physical resistance and protection against herbivory (Pérez-Harguindeguy et al., 2016), characteristics that also slow tissue breakdown by associated biota (Pichon et al., 2020) and are common in forest understory plants. Lastly, decomposition rates likely interact with LDMC trait values which were unchanged across invasion levels.
We attempted to capture invasive impact on the native community and changes in ecosystem’s functionality by differentiating between displacement and complementarity of native traits with increasing levels of invasion (Figure 1). After excluding invasive species from our response metric, our results showed no changes in the CWM of the native community for all three traits across the invasion gradient (Figures 4 and 5A). This result indicates that the shifts observed in CWM trait distributions of the total community (discussed above) and consequent changes in ecosystem functionality are a sole consequence of invasive species complementing the native community. Our findings align with most other studies that explored a similar approach in other ecosystems (Fried et al., 2019; Livingstone et al., 2020; Fernandez et al., 2021), where native community trait distributions remained unchanged given invasion. Specifically, these studies also found no effects of invasion on the CWM of SLA (Fried et al., 2019; Livingstone et al., 2020; Fernandez et al., 2021) or LDMC (Fried et al., 2019) of the native community [but see Loiola et al. (2018)]. One potential explanation for this pattern relies on likely strong selective forces in forest understories as they are extremely competitive with respect to essential resources for plant establishment and growth, such as light, soil water, and soil nutrients (Dawson et al., 2015). Consequently, native species, even when co-occurring with invasives, need to have specific trait values or characteristics to be able to tolerate such conditions. Our results, in concordance with the literature (Fried et al., 2019; Livingstone et al., 2020; Fernandez et al., 2021), underscore the importance of distinguishing between invasion effects on the total community (including invasives) and the native community alone. Regarding management implications, our findings suggest that removing invasive species would restore functional trait levels, and likely ecosystem functionality in temperate forest understories, comparable to uninvaded conditions.
Leaf traits, arranged along the acquisitive-conservation gradient, exhibit a trade-off between life-span and resource acquisition and allocation (Wright et al., 2004). Consistent with expectations, our results align with this gradient, revealing significant positive correlations between leaf N and SLA (Figure 5B). In order words, traits associated with lower survival, shorter leaf lifespan, higher light demands, and higher maximum photosynthetic rates (Wright et al., 2004; Diaz et al., 2016) were positively correlated in our analysis. Additionally, both traits tended to show negative correlations with LDMC, although the latter was not statistically significant (Figure 5B). This result highlights that LDMC, characterized by high values in conservative leaves that exhibit tolerance to low resource environments (Wright et al., 2004; Diaz et al., 2016), was accurately positioned to be on the opposite end from the other two acquisitive traits. Our analytical approach, utilizing multinormal distributions, enabled simultaneous consideration of trait covariation while exploring the individual effects of environmental and biotic covariates on community-level trait variation.
Richness and evenness are important metrics for understanding both ecosystem functionality and the impact of invasion. Native richness is expected to have positive effects on functionality, particularly on productivity, such as in the context of biodiversity-ecosystem-function (BEF) framework where positive relationships have been extensively documented (Hector and Bagchi, 2007; Isbell et al., 2011; Maestre et al., 2012; Tilman et al., 2014; Jing et al., 2015; Liang et al., 2016). Invaded areas, however, are usually associated with low native diversity or low richness, either because invaders outcompete natives or because they colonize areas with low native richness, while being highly productive (Livingstone et al., 2020). Therefore, it seems counter-intuitive that invaded areas which are usually associated with low native diversity or low richness, either because invaders outcompete natives or because they colonize areas already with low native richness, still are highly productive (Livingstone et al., 2020). The loss of functionality by native communities under invasion can be then compensated by the high performance of invaders with high dispersal abilities (Rejmanek and Richardson, 1996; van Kleunen et al., 2015), high resource-use efficiency (Funk and Vitousek, 2007; Heberling and Fridley, 2013) and/or presenting novel strategies, e.g., N-fixing invasive species (Paschke et al., 1989; Perry et al., 2010). Contrary to this pattern, our findings reveal that richer native communities, irrespective of community grouping (i.e., total or natives only), showed lower leaf N (Figure 3A). For all other traits, we did not find any significant association with richness (Figures 3B, C). These results suggest that changes in functionality via increases in leaf N due to invasion are dissociated from native richness and related to the complementary effect of invasives only (Figure 4). One potential explanation for this pattern is that if resources are under-explored by natives, invasive species likely occupy empty niches, complementing the system’s functionality (Elton, 1958; Chase and Leibold, 2003; Hierro et al., 2005). Additionally, a widespread invader in our study area, Eleagnus umbellata is an N-fixing species (Paschke et al., 1989; Perry et al., 2010) possibly driving most of the complementary effect on CWM of leaf N.
The relationship between evenness and EFs are believed to be closely linked to environmental variability, particularly with respect to productivity (Hillebrand et al., 2008). This is because more even communities (i.e., less dominance by one or a few species) are expected to have higher productivity as those communities would hold a larger diversity of species with different strategies to adapt to environmental variability (Hillebrand et al., 2008). Our results, however, revealed no significant association of evenness with any of the traits and community groupings (Figure 3), suggesting that changes in species dominance with increasing invasion was not a significant factor driving alterations in ecosystem functionality. We speculate that the range of variability in environmental conditions (namely, light and soil water availability) in our understory plots were insufficient to capture significant relationships between evenness and CWM traits.
Environmental factors shape both plant community composition and structure, as well as the overall functioning of the ecosystems (Jing et al., 2015; Chacón-Labella et al., 2023). In forested ecosystems below tree canopies, in particular, light availability and soil water content are among the main limiting resources for species survival and growth (Landuyt et al., 2019; De Frenne et al., 2021), likely influencing community trait distributions (Chacón-Labella et al., 2023). Our results revealed that soil water was positively associated with community-level SLA for both community types (Figure 3B), while no other significant associations with soil water or light availability were observed (Figures 3A, C). These significant results align with the literature as SLA is associated with higher growth and photosynthesis rates, which heavily rely on water and nutrient supply (Xu and Zhou, 2011; Flexas et al., 2012; McAdam and Brodribb, 2015; Novick et al., 2016; Lambers and Oliveira, 2019; Westerband et al., 2023). Surprisingly, soil water was not associated with leaf N, while this trait is correlated with SLA (Figure 5B) and their shared position on the “fast” end of the LES (Wright et al., 2004; Diaz et al., 2016). Also surprising was the lack of significant association observed between light availability and the CWM values of the three traits across community types, which is contrary to the expected strong effect of light on shaping understory communities (Depauw et al., 2019; Blondeel et al., 2020). This absence of association may be attributed to the potential lack of sensitivity of the CWM we calculated to the local environmental variability of our study system. Incorporating local trait values and intraspecific variation into our analysis, as demonstrated to be crucial in capturing variation in the trajectories of understory plant communities in other temperate forests (Blondeel et al., 2020), could provide a more nuanced understanding of CWM trait values and available light.
Invasion can impact community-level trait distributions, and consequently, ecosystem functionality through either displacement or complementarity of native species. To distinguish between these two processes, the effects of invasives on total (i.e., invasives and natives) versus native community trait distributions need to be differentiated (Thomsen et al., 2016). Our results indicate that for our study system, invasive plants indeed lead to changes in ecosystem functionality by increasing the mean trait values of leaf N, while having no significant impact on the trait distributions of the native community. Therefore, alterations in ecosystem functionality within our study system, based on representative traits of the LES, are primarily driven by the increased abundance of invasive species complementing the functionality exhibited by the native community, as we hypothesized. These findings have important management implications: if invasive species were to be removed, the co-occurring native community could recover its original trait distribution with little impact on ecosystem functionality. In contrast, if invasion had displaced native species function, the removal of invasive species would likely fail to achieve a full recovery of functionality. By quantifying invasion-driven changes in ecosystem function and differentiating between complementarity versus displacement, our study provides essential information and framework to allow land managers to make informed decisions regarding such consequences on ecosystem functionality of temperate forest understories.
The original contributions presented in the study are publicly available. This data can be found here: https://doi.org/10.6084/m9.figshare.24415648.v3.
LP: Conceptualization, Data curation, Formal analysis, Methodology, Visualization, Writing – original draft, Writing – review & editing. II: Conceptualization, Formal analysis, Funding acquisition, Methodology, Writing – original draft, Writing – review & editing.
The author(s) declare financial support was received for the research, authorship, and/or publication of this article. LP thanks the Matthaei Botanical Gardens and Nichols Arboretum at the University of Michigan for financial support via the Chase Fellowship Award (2021). LP was also supported by funding from NSF DEB-1252664 granted to II.
We thank Dr. Robyn Burnham, Dr. Natalia Umaña, Dr. Karen Alofs and two reviewers for their valuable manuscript comments. We also thank all that assisted with field work.
The authors declare that the research was conducted in the absence of any commercial or financial relationships that could be construed as a potential conflict of interest.
All claims expressed in this article are solely those of the authors and do not necessarily represent those of their affiliated organizations, or those of the publisher, the editors and the reviewers. Any product that may be evaluated in this article, or claim that may be made by its manufacturer, is not guaranteed or endorsed by the publisher.
The Supplementary Material for this article can be found online at: https://www.frontiersin.org/articles/10.3389/fevo.2024.1325275/full#supplementary-material
Allen D., Dick C., Burnham R. J., Perfecto I., Vandermeer J. (2020). The michigan big woods research plot at the Edwin S (Pinckney, MI, USA: George Reserve).
Arguez A., Durre I., Applequist S., Squires M., Vose R., Yin X., et al. (2010). NOAA’s U.S. Climate Normals (1981-2010). NOAA National Centers for Environmental Information. doi: 10.7289/V5PN93JP
Bernard-Verdier M., Hulme P. E. (2019). Alien plants can be associated with a decrease in local and regional native richness even when at low abundance. J. Ecol. 107, 1343–1354. doi: 10.1111/1365-2745.13124
Bianchi S., Cahalan C., Hale S., Gibbons J. M. (2017). Rapid assessment of forest canopy and light regime using smartphone hemispherical photography. Ecol. Evol. 7, 10556–10566. doi: 10.1002/ece3.3567
Blondeel H., Perring M. P., Depauw L., De Lombaerde E., Landuyt D., De Frenne P., et al. (2020). Light and warming drive forest understorey community development in different environments. Global Change Biol. 26, 1681–1696. doi: 10.1111/gcb.14955
Boyle B., Hopkins N., Lu Z., Garay J. A. R., Mozzherin D., Rees T., et al. (2013). The taxonomic name resolution service: an online tool for automated standardization of plant names. BMC Bioinf. 14, 16. doi: 10.1186/1471-2105-14-16
Brockerhoff E. G., Barbaro L., Castagneyrol B., Forrester D. I., Gardiner B., González-Olabarria J. R., et al. (2017). Forest biodiversity, ecosystem functioning and the provision of ecosystem services. Biodiversity Conserv. 26, 3005–3035. doi: 10.1007/s10531-017-1453-2
Cadotte M. W., Yasui S. L. E., Livingstone S., Macivor J. S. (2017). Are urban systems beneficial, detrimental, or indifferent for biological invasion? Biol. Invasions 19, 3489–3503. doi: 10.1007/s10530-017-1586-y
Castro J., Morales-Rueda F., Navarro F. B., Löf M., Vacchiano G., Alcaraz-Segura D. (2021). Precision restoration: a necessary approach to foster forest recovery in the 21st century. Restor. Ecol. 29, e13421. doi: 10.1111/rec.13421
Castro-Díez P., Godoy O., Alonso A., Gallardo A., Saldaña A. (2014). What explains variation in the impacts of exotic plant invasions on the nitrogen cycle? A meta-analysis. Ecol. Lett. 17, 1–12. doi: 10.1111/ele.12197
Chacón-Labella J., Hinojo-Hinojo C., Bohner T., Castorena M., Violle C., Vandvik V., et al. (2023). How to improve scaling from traits to ecosystem processes. Trends Ecol. Evol. 38, 228–237. doi: 10.1016/j.tree.2022.10.007
Chase J. M., Leibold M. A. (2003). Ecological niches: linking classical and contemporary approaches (Chicago: University of Chicago Press).
Cornwell W. K., Cornelissen J. H. C., Amatangelo K., Dorrepaal E., Eviner V. T., Godoy O., et al. (2008). Plant species traits are the predominant control on litter decomposition rates within biomes worldwide. Ecol. Lett. 11, 1065–1071. doi: 10.1111/j.1461-0248.2008.01219.x
Dawson W., Burslem D. F. R. P., Hulme P. E. (2015). Consistent effects of disturbance and forest edges on the invasion of a continental rain forest by alien plants. Biotropica 47, 27–37. doi: 10.1111/btp.12183
Decocq G., Andrieu E., Brunet J., Chabrerie O., De Frenne P., De Smedt P., et al. (2016). Ecosystem services from small forest patches in agricultural landscapes. Curr. Forestry Rep. 2, 30–44. doi: 10.1007/s40725-016-0028-x
De Frenne P., Lenoir J., Luoto M., Scheffers B. R., Zellweger F., Aalto J., et al. (2021). Forest microclimates and climate change: Importance, drivers and future research agenda. Global Change Biol. 27, 2279–2297. doi: 10.1111/gcb.15569
Depauw L., Perring M. P., Landuyt D., Maes S. L., Blondeel H., De Lombaerde E., et al. (2019). Light availability and land-use history drive biodiversity and functional changes in forest herb layer communities. J. Ecol. 108, 1411–1425. doi: 10.1111/1365-2745.13339
Díaz S., Cabido M. (2001). Vive la différence: plant functional diversity matters to ecosystem processes. Trends Ecol. Evol. 16, 646–655. doi: 10.1016/S0169-5347(01)02283-2
Díaz S., Kattge J., Cornelissen J. H. C., Wright I. J., Lavorel S., Dray S., et al. (2016). The global spectrum of plant form and function. Nature 529, 167–171. doi: 10.1038/nature16489
Díaz S. M., Settele J., Brondízio E., Ngo H., Guèze M., Agard J., et al. (2019). The global assessment report on biodiversity and ecosystem services: Summary for policy makers.
Dostal P., Mullerova J., Pysek P., Pergl J., Klinerova T. (2013). The impact of an invasive plant changes over time. Ecol. Lett. 16, 1277–1284. doi: 10.1111/ele.12166
Ehrenfeld J. G. (2003). Effects of exotic plant invasions on soil nutrient cycling processes. Ecosystems 6, 503–523. doi: 10.1007/s10021-002-0151-3
Elton C. S. (1958). The ecology of invasions by animals and plants/Charles S. Elton (U.S.A: Chapman & Hall).
Fernandez R. D., Castro-Díez P., Aragón R., Pérez-Harguindeguy N. (2021). Changes in community functional structure and ecosystem properties along an invasion gradient of Ligustrum lucidum. J. Vegetation Sci. 32, e13098. doi: 10.1111/jvs.13098
Flexas J., Gallé A., Galmés J., Ribas-Carbo M., Medrano H. (2012). “The response of photosynthesis to soil water stress,” in Plant responses to drought stress: from morphological to molecular features. Ed. Aroca R. (Berlin, Heidelberg: Springer Berlin Heidelberg).
Fridley J. D., Bauerle T. L., Craddock A., Ebert A. R., Frank D. A., Heberling J. M., et al. (2022). Fast but steady: An integrated leaf-stem-root trait syndrome for woody forest invaders. Ecol. Lett. 25, 900–912. doi: 10.1111/ele.13967
Fried G., Carboni M., Mahaut L., Violle C. (2019). Functional traits modulate plant community responses to alien plant invasion. Perspect. Plant Ecology Evol. Systematics 37, 53–63. doi: 10.1016/j.ppees.2019.02.003
Fu B., Wang S., Su C., Forsius M. (2013). Linking ecosystem processes and ecosystem services. Curr. Opin. Environ. Sustainability 5, 4–10. doi: 10.1016/j.cosust.2012.12.002
Funk J. L. (2013). The physiology of invasive plants in low-resource environments. Conserv. Physiol. 1, cot026. doi: 10.1093/conphys/cot026
Funk J. L., Vitousek P. M. (2007). Resource-use efficiency and plant invasion in low-resource systems. Nature 446, 1079–1081. doi: 10.1038/nature05719
Gallien L., Carboni M. (2017). The community ecology of invasive species: where are we and what’s next? Ecography 40, 335–352. doi: 10.1111/ecog.02446
Garnier E., Cortez J., Billès G., Navas M.-L., Roumet C., Debussche M., et al. (2004). Plant functional markers capture ecosystem properties during secondary succession. Ecology 85, 2630–2637. doi: 10.1890/03-0799
Gauthier S., Kuuluvainen T., Macdonald S. E., Shorohova E., Shvidenko A., Bélisle A.-C., et al. (2023). “Ecosystem management of the boreal forest in the era of global change,” in Boreal forests in the face of climate change: sustainable management. Eds. Girona M. M., Morin H., Gauthier S., Bergeron Y. (Cham: Springer International Publishing).
Gioria M., Osborne B. A. (2014). Resource competition in plant invasions: emerging patterns and research needs. Front. Plant Sci. 5. doi: 10.3389/fpls.2014.00501
Girona M. M., Aakala T., Aquilué N., Bélisle A.-C., Chaste E., Danneyrolles V., et al. (2023). “Challenges for the sustainable management of the boreal forest under climate change,” in Boreal forests in the face of climate change: sustainable management. Eds. Girona M. M., Morin H., Gauthier S., Bergeron Y. (Cham: Springer International Publishing).
Hagan J. G., Henn J. J., Osterman W. H. A. (2023). Plant traits alone are good predictors of ecosystem properties when used carefully. Nat. Ecol. Evol. 7, 332–334. doi: 10.1038/s41559-022-01920-x
Heberling J. M., Fridley J. D. (2013). Resource-use strategies of native and invasive plants in Eastern North American forests. New Phytol. 200, 523–533. doi: 10.1111/nph.12388
Hector A., Bagchi R. (2007). Biodiversity and ecosystem multifunctionality. Nature 448, 188–190. doi: 10.1038/nature05947
Henn J. J., Yelenik S., Damschen E. I. (2019). Environmental gradients influence differences in leaf functional traits between native and non-native plants. Oecologia 191, 397–409. doi: 10.1007/s00442-019-04498-7
Hierro J. L., Maron J. L., Callaway R. M. (2005). A biogeographical approach to plant invasions: the importance of studying exotics in their introduced and native range. J. Ecol. 93, 5–15. doi: 10.1111/j.0022-0477.2004.00953.x
Hillebrand H., Bennett D. M., Cadotte M. W. (2008). Consequences of dominance: a review of evenness effects on local and regional ecosystem processes. Ecology 89, 1510–1520. doi: 10.1890/07-1053.1
Ibáñez I., Liu G., Petri L., Schaffer-Morrison S., Schueller S. (2021). Assessing vulnerability and resistance to plant invasions: a native community perspective. Invasive Plant Sci. Manage. 14, 64–74. doi: 10.1017/inp.2021.15
Isbell F., Calcagno V., Hector A., Connolly J., Harpole W. S., Reich P. B., et al. (2011). High plant diversity is needed to maintain ecosystem services. Nature 477, 199–202. doi: 10.1038/nature10282
Jauni M., Gripenberg S., Ramula S. (2015). Non-native plant species benefit from disturbance: a meta-analysis. Oikos 124, 122–129. doi: 10.1111/oik.01416
Jing X., Sanders N. J., Shi Y., Chu H., Classen A. T., Zhao K., et al. (2015). The links between ecosystem multifunctionality and above- and belowground biodiversity are mediated by climate. Nat. Commun. 6, 8159. doi: 10.1038/ncomms9159
Jo I., Fridley J. D., Frank D. A. (2015). Linking above- and belowground resource use strategies for native and invasive species of temperate deciduous forests. Biol. Invasions 17, 1545–1554. doi: 10.1007/s10530-014-0814-y
Jo I., Fridley J. D., Frank D. A. (2016). More of the same? In situ leaf and root decomposition rates do not vary between 80 native and nonnative deciduous forest species. New Phytol. 209, 115–122. doi: 10.1111/nph.13619
Kattge J., Díaz S., Lavorel S., Prentice I. C., Leadley P., BÖnisch G., et al. (2011). TRY-a global database of plant traits. Glob Chang Biol. 17, 2905–2935. doi: 10.1111/j.1365-2486.2011.02451.x
Lambers H., Oliveira R. S. (2019). “Plant water relations,” in Plant physiological ecology. Ed. Lambers H., Oliveira R. S. (Cham: Springer International Publishing).
Landuyt D., De Lombaerde E., Perring M. P., Hertzog L. R., Ampoorter E., Maes S. L., et al. (2019). The functional role of temperate forest understorey vegetation in a changing world. Global Change Biol. 25, 3625–3641. doi: 10.1111/gcb.14756
Laughlin D. C. (2011). Nitrification is linked to dominant leaf traits rather than functional diversity. J. Ecol. 99, 1091–1099. doi: 10.1111/j.1365-2745.2011.01856.x
Lavorel S., Garnier E. (2002). Predicting changes in community composition and ecosystem functioning from plant traits: revisiting the Holy Grail. Funct. Ecol. 16, 545–556. doi: 10.1046/j.1365-2435.2002.00664.x
Lavorel S., Grigulis K. (2012). How fundamental plant functional trait relationships scale-up to trade-offs and synergies in ecosystem services. J. Ecol. 100, 128–140. doi: 10.1111/j.1365-2745.2011.01914.x
Lavorel S., Grigulis K., Mcintyre S., Williams N. S. G., Garden D., Dorrough J., et al. (2008). Assessing functional diversity in the field – methodology matters! Funct. Ecol. 22, 134–147. doi: 10.1111/j.1365-2435.2007.01339.x
Le Bagousse-Pinguet Y., Gross N., Maestre F. T., Maire V., De Bello F., Fonseca C. R., et al. (2017). Testing the environmental filtering concept in global drylands. J. Ecol. 105, 1058–1069. doi: 10.1111/1365-2745.12735
Lee M. R., Bernhardt E. S., Van Bodegom P. M., Cornelissen J. H. C., Kattge J., Laughlin D. C., et al. (2017). Invasive species’ leaf traits and dissimilarity from natives shape their impact on nitrogen cycling: a meta-analysis. New Phytol. 213, 128–139. doi: 10.1111/nph.14115
Leishman M. R., Haslehurst T., Ares A., Baruch Z. (2007). Leaf trait relationships of native and invasive plants: community- and global-scale comparisons. New Phytol. 176, 635–643. doi: 10.1111/j.1469-8137.2007.02189.x
Liang J., Crowther T. W., Picard N., Wiser S., Zhou M., Alberti G., et al. (2016). Positive biodiversity-productivity relationship predominant in global forests. Science 354, aaf8957. doi: 10.1126/science.aaf8957
Lienin P., Kleyer M. (2012). Plant trait responses to the environment and effects on ecosystem properties. Basic Appl. Ecol. 13, 301–311. doi: 10.1016/j.baae.2012.05.002
Link A. F., Turnblacer T., Snyder C. K., Daugherty S. E., Utz R. M. (2018). Low recruitment of native trees in a deciduous forest associated with Japanese barberry (Berberis thunbergii) invasion. Invasive Plant Sci. Manage. 11, 20–26. doi: 10.1017/inp.2018.1
Livingstone S. W., Isaac M. E., Cadotte M. W. (2020). Invasive dominance and resident diversity: unpacking the impact of plant invasion on biodiversity and ecosystem function. Ecol. Monogr. 90, e01425. doi: 10.1002/ecm.1425
Loiola P. P., De Bello F., Chytrý M., Götzenberger L., Carmona C. P., Pyšek P., et al. (2018). Invaders among locals: Alien species decrease phylogenetic and functional diversity while increasing dissimilarity among native community members. J. Ecol. 106, 2230–2241. doi: 10.1111/1365-2745.12986
Mack R. N., Simberloff D., Lonsdale W. M., Evans H., Clout M., Bazzaz F. A. (2000). Biotic invasions: Causes, epidemiology, global consequences, and control. Ecol. Appl. 10, 689–710. doi: 10.1890/1051-0761(2000)010[0689:BICEGC]2.0.CO;2
Maestre F. T., Quero J. L., Gotelli N. J., Escudero A., Ochoa V., Delgado-Baquerizo M., et al. (2012). Plant species richness and ecosystem multifunctionality in global drylands. Science 335, 214–218. doi: 10.1126/science.1215442
Martin P. H., Canham C. D., Marks P. L. (2009). Why forests appear resistant to exotic plant invasions: intentional introductions, stand dynamics, and the role of shade tolerance. Front. Ecol. Environ. 7, 142–149. doi: 10.1890/070096
Martinez K. A., Fridley J. D. (2018). Acclimation of leaf traits in seasonal light environments: Are non-native species more plastic? J. Ecol. 106, 2019–2030. doi: 10.1111/1365-2745.12952
McAdam S. A. M., Brodribb T. J. (2015). The evolution of mechanisms driving the stomatal response to vapor pressure deficit. Plant Physiol. 167, 833–843. doi: 10.1104/pp.114.252940
Montesinos D. (2022). Fast invasives fastly become faster: invasive plants align largely with the fast side of the plant economics spectrum. J. Ecol. 110, 1010–1014. doi: 10.1111/1365-2745.13616/v2/response1
Mori A. S., Lertzman K. P., Gustafsson L. (2017). Biodiversity and ecosystem services in forest ecosystems: a research agenda for applied forest ecology. J. Appl. Ecol. 54, 12–27. doi: 10.1111/1365-2664.12669
Novick K. A., Ficklin D. L., Stoy P. C., Williams C. A., Bohrer G., Oishi A. C., et al. (2016). The increasing importance of atmospheric demand for ecosystem water and carbon fluxes. Nat. Climate Change 6, 1023–1027. doi: 10.1038/nclimate3114
Oksanen J., Simpson G., Blanchet F., Kindt R., Legendre P., Minchin P., et al. (2022). Vegan: Community Ecology Package. R package version 2.6-4.
Ordonez A., Olff H. (2013). Do alien plant species profit more from high resource supply than natives? A trait-based analysis. Global Ecol. Biogeography 22, 648–658. doi: 10.1111/geb.12019
Orwin K. H., Buckland S. M., Johnson D., Turner B. L., Smart S., Oakley S., et al. (2010). Linkages of plant traits to soil properties and the functioning of temperate grassland. J. Ecol. 98, 1074–1083. doi: 10.1111/j.1365-2745.2010.01679.x
Pakeman R. J., Quested H. M. (2007). Sampling plant functional traits: What proportion of the species need to be measured? Appl. Vegetation Sci. 10, 91–96. doi: 10.1111/j.1654-109X.2007.tb00507.x
Pan Y., Birdsey R. A., Fang J., Houghton R., Kauppi P. E., Kurz W. A., et al. (2011). A large and persistent carbon sink in the world’s forests. Science 333, 988–993. doi: 10.1126/science.1201609
Parra-Tabla V., Arceo-Gómez G. (2021). Impacts of plant invasions in native plant–pollinator networks. New Phytol. 230, 2117–2128. doi: 10.1111/nph.17339
Paschke M. W., Dawson J. O., David M. B. (1989). Soil nitrogen mineralization in plantations of Juglans nigra interplanted with actinorhizal Elaeagnus umbellata or Alnus glutinosa. Plant Soil 118, 33–42. doi: 10.1007/BF02232788
Pérez-Harguindeguy N., Díaz S., Garnier E., Lavorel S., Poorter H., Jaureguiberry P., et al. (2016). Corrigendum to: New handbook for standardised measurement of plant functional traits worldwide. Aust. J. Bot. 64, 715–716. doi: 10.1071/BT12225_CO
Perry L. G., Blumenthal D. M., Monaco T. A., Paschke M. W., Redente E. F. (2010). Immobilizing nitrogen to control plant invasion. Oecologia 163, 13–24. doi: 10.1007/s00442-010-1580-x
Pichon N. A., Cappelli S. L., Soliveres S., Hölzel N., Klaus V. H., Kleinebecker T., et al. (2020). Decomposition disentangled: A test of the multiple mechanisms by which nitrogen enrichment alters litter decomposition. Funct. Ecol. 34, 1485–1496. doi: 10.1111/1365-2435.13560
Pietsch K. A., Ogle K., Cornelissen J. H. C., Cornwell W. K., Bönisch G., Craine J. M., et al. (2014). Global relationship of wood and leaf litter decomposability: the role of functional traits within and across plant organs. Global Ecol. Biogeography 23, 1046–1057. doi: 10.1111/geb.12172
Plummer M. (2003). “JAGS: A program for analysis of Bayesian graphical models using Gibbs sampling,” in Proceedings of the 3rd international workshop on distributed statistical computing. 124 (125.10), 1–10.
Plummer M. (2022). rjags: Bayesian Graphical Models using MCMC. Available at: https://CRAN.R-project.org/package=rjags.
Pysek P., Jarosik V., Hulme P. E., Pergl J., Hejda M., Schaffner U., et al. (2012). A global assessment of invasive plant impacts on resident species, communities and ecosystems: the interaction of impact measures, invading species’ traits and environment. Global Change Biol. 18, 1725–1737. doi: 10.1111/j.1365-2486.2011.02636.x
R Core Team. (2022). R: A language and environment for statistical computing (Vienna, Austria: R Foundation for Statistical Computing). Available at: https://www.r-project.org/.
Reich P. B. (2012). Key canopy traits drive forest productivity. Proc. R. Soc. B: Biol. Sci. 279, 2128–2134. doi: 10.1098/rspb.2011.2270
Rejmanek M., Richardson D. M. (1996). What attributes make some plant species more invasive? Ecology 77, 1655–1661. doi: 10.2307/2265768
Reznicek A., Penskar M., Walters B., Slaughter B. (2014). Michigan floristic quality assessment database. Herbarium, University of Michigan (Lansing, MI: Ann Arbor, MI and Michigan Natural Features Inventory, Michigan State University).
Seabloom E. W., Borer E. T., Buckley Y. M., Cleland E. E., Davies K. F., Firn J., et al. (2015). Plant species’ origin predicts dominance and response to nutrient enrichment and herbivores in global grasslands. Nat. Commun. 6, 7710. doi: 10.1038/ncomms8710
Silverman B. W. A. (2018). Density estimation for statistics and data analysis (Boca Raton, FL: CRC Press).
Simberloff D., Martin J.-L., Genovesi P., Maris V., Wardle D. A., Aronson J., et al. (2013). Impacts of biological invasions: what’s what and the way forward. Trends Ecol. Evol. 28, 58–66. doi: 10.1016/j.tree.2012.07.013
Sodhi D. S., Livingstone S. W., Carboni M., Cadotte M. W. (2019). Plant invasion alters trait composition and diversity across habitats. Ecol. Evol. 9, 6199–6210. doi: 10.1002/ece3.5130
Spicer M. E., Mellor H., Carson W. P. (2020). Seeing beyond the trees: a comparison of tropical and temperate plant growth forms and their vertical distribution. Ecology 101, e02974. doi: 10.1002/ecy.2974
Thompson I. D., Okabe K., Tylianakis J. M., Kumar P., Brockerhoff E. G., Schellhorn N. A., et al. (2011). Forest biodiversity and the delivery of ecosystem goods and services: translating science into policy. BioScience 61, 972–981. doi: 10.1525/bio.2011.61.12.7
Thomsen M. S., Wernberg T., South P. M., Schiel D. R. (2016). To include or not to include (the invader in community analyses)? That is the question. Biol. Invasions 18, 1515–1521. doi: 10.1007/s10530-016-1102-9
Tilman D., Isbell F., Cowles J. M. (2014). Biodiversity and ecosystem functioning. Annu. Rev. Ecology Evolution Systematics 45, 471–493. doi: 10.1146/annurev-ecolsys-120213-091917
van der Plas F., Schröder-Georgi T., Weigelt A., Barry K., Meyer S., Alzate A., et al. (2020). Plant traits alone are poor predictors of ecosystem properties and long-term ecosystem functioning. Nat. Ecol. Evolution. 4, 1602–1611. doi: 10.1038/s41559-020-01316-9
van Kleunen M., Dawson W., Maurel N. (2015). Characteristics of successful alien plants. Mol. Ecol. 24, 1954–1968. doi: 10.1111/mec.13013
van Kleunen M., Weber E., Fischer M. (2010). A meta-analysis of trait differences between invasive and non-invasive plant species. Ecol. Lett. 13, 235–245. doi: 10.1111/j.1461-0248.2009.01418.x
Vilà M., Espinar J. L., Hejda M., Hulme P. E., Jarosik V., Maron J. L., et al. (2011). Ecological impacts of invasive alien plants: a meta-analysis of their effects on species, communities and ecosystems. Ecol. Lett. 14, 702–708. doi: 10.1111/j.1461-0248.2011.01628.x
Vilà M., Hulme P. E. (2017). Impact of biological invasions on ecosystem services. Invading nature - Springer Series in invasion (Springer).
Violle C., Navas M.-L., Vile D., Kazakou E., Fortunel C., Hummel I., et al. (2007). Let the concept of trait be functional! Oikos 116, 882–892. doi: 10.1111/j.0030-1299.2007.15559.x
Wallace K. J. (2007). Classification of ecosystem services: Problems and solutions. Biol. Conserv. 139, 235–246. doi: 10.1016/j.biocon.2007.07.015
Weigelt A., Mommer L., Andraczek K., Iversen C. M., Bergmann J., Bruelheide H., et al. (2021). An integrated framework of plant form and function: the belowground perspective. New Phytol. 232, 42–59. doi: 10.1111/nph.17590
Westerband A. C., Wright I. J., Maire V., Paillassa J., Prentice I. C., Atkin O. K., et al. (2023). Coordination of photosynthetic traits across soil and climate gradients. Global Change Biol. 29, 856–873. doi: 10.1111/gcb.16501
Wickham H., Averick M., Bryan J., Chang W., Mcgowan L. D. A., François R., et al. (2019). Welcome to the tidyverse. J. Open Source software 4, 1686. doi: 10.21105/joss.01686
Wright I. J., Reich P. B., Westoby M., Ackerly D. D., Baruch Z., Bongers F., et al. (2004). The worldwide leaf economics spectrum. Nature 428, 821–827. doi: 10.1038/nature02403
Keywords: alien species, ecosystem functioning, introduced species, invasion gradient, leaf economic spectrum, multinormal, non-native species, plant traits
Citation: Petri L and Ibáñez I (2024) Trait-related functional changes in understory forest community after invasion are driven by complementarity rather than displacement. Front. Ecol. Evol. 12:1325275. doi: 10.3389/fevo.2024.1325275
Received: 20 October 2023; Accepted: 08 January 2024;
Published: 26 January 2024.
Edited by:
Xiao Guo, Qingdao Agricultural University, ChinaReviewed by:
Martí March-Salas, Goethe University Frankfurt, GermanyCopyright © 2024 Petri and Ibáñez. This is an open-access article distributed under the terms of the Creative Commons Attribution License (CC BY). The use, distribution or reproduction in other forums is permitted, provided the original author(s) and the copyright owner(s) are credited and that the original publication in this journal is cited, in accordance with accepted academic practice. No use, distribution or reproduction is permitted which does not comply with these terms.
*Correspondence: Laís Petri, cGV0cmlsQHVtaWNoLmVkdQ==
Disclaimer: All claims expressed in this article are solely those of the authors and do not necessarily represent those of their affiliated organizations, or those of the publisher, the editors and the reviewers. Any product that may be evaluated in this article or claim that may be made by its manufacturer is not guaranteed or endorsed by the publisher.
Research integrity at Frontiers
Learn more about the work of our research integrity team to safeguard the quality of each article we publish.