- 1Laboratório de Ecologia Marinha, Departamento de Oceanografia e Limnologia, Universidade Federal do Rio Grande do Norte, Natal, RN, Brazil
- 2Programa de Pós-Graduação em Ecologia, Universidade Federal do Rio Grande do Norte, Natal, Rio Grande do Norte, Brazil
- 3Marine Macroecology and Conservation Laboratory, Departamento de Ecologia Evolução, Universidade Federal de Santa Maria, Santa Maria, Brazil
- 4Programa de Pós-Graduação em Biodiversidade Animal, Departamento de Ecologia e Evolução, Universidade Federal de Santa Maria, Santa Maria, Brazil
The Southwestern Atlantic (SWA) harbors a relatively species poor but highly endemic coral assemblage due to historical processes, environmental and ecological drivers. Despite its low to moderate cover, corals still have a disproportionate contribution to ecosystem function and stability in this region. In the context of global change, it is imperative to know corals’ diversity and biogeographic patterns, yet a comprehensive approach is still missing for SWA corals. We integrated occurrence data from 21 sites and nine functional traits across 20 coral (scleractinian and hydrozoan) species to explore the taxonomic and functional diversity of coral assemblages in the SWA (1°N-27°S). We identified eight regions based on coral species composition, and then described their functional diversity using four metrics: functional richness (FRic), functional dispersion (FDis), functional evenness (FEve), and functional originality (FOri). Taxonomic and functional diversity peak between latitudes 13°S-20°S, decreasing with increasing distance from this diversity center, known as the Abrolhos Bank that harbors a wide continental platform. Our findings reveal a prevalent pattern of high functional redundancy across these eight regions (indicated by low functional originality), with species occupying the edges of the trait space (high functional evenness) and converging around few trait values (low functional dispersion). Such patterns resulted in low taxonomic and functional beta diversity and increased nestedness among regions caused by dispersal barriers and environmental filtering. The Southernmost region (24°-27°S) has the lowest taxonomic and functional diversity and comprises only two species that share similar traits, with these corals being: hermaphrodites, brooders and depth-tolerant, and having a wide corallite. As this region might become critical for corals in a future tropicalization scenario, tropical corals that share similar traits to those of the southernmost region can be more likely to thrive. Knowledge on taxonomic and functional diversity patterns can offer critical information to conservation by helping prioritizing areas with higher diversity and species with traits that enhance survival under climate change.
1 Introduction
Biodiversity patterns and its evolutionary and environmental drivers across different geographic scales have been a core subject in ecology and biogeography (Connell, 1978; Gaston, 2000). Biogeographic patterns have been investigated across scales, for several marine groups, including coralline algae (Sissini et al., 2022), sponges (Pansini and Longo, 2003; van Soest et al., 2012), crustaceans (Teschima et al., 2016; Freire et al., 2021), reef fishes (Floeter et al., 2008; Bender et al., 2013; Cowman and Bellwood, 2013; Siqueira et al., 2019), sea anemones (Targino and Gomes, 2020), and corals (Veron, 1995; Veron et al., 2009; Veron et al., 2011; Obura, 2012; McClanahan et al., 2014), as well as with a multi-taxa approach (Cord et al., 2022). Specifically, biogeographical patterns of the coral fauna were vastly explored under different approaches including reproductive ecology (Baird et al., 2009), recruitment (Price et al., 2019), genetic diversity and connectivity (Peluso et al., 2018; Teschima et al., 2022; van der Ven et al., 2022), symbiont (Picciani et al., 2016) and functional diversity (McWilliam et al., 2018). These efforts attempted to unravel the complexities that shape the distribution of species within marine ecosystems.
Coral assemblages are influenced by multiple environmental and ecological drivers. Geographic isolation, oceanic currents and river plumes directly affect coral dispersion and are key drivers of benthic community structure through dispersion limitation (e.g., Veron et al., 2009). The distribution of corals is largely influenced by species’ traits, biotic interactions and abiotic conditions (Gleason and Hofmann, 2011). Coral larvae disperse through ocean currents occasionally confronting environmental filters such as distance (van der Ven et al., 2022), semi-permeable river plumes (Tosetto et al., 2022) or ocean currents themselves (Treml et al., 2008; Thompson et al., 2018; van der Ven et al., 2022). Reproductive traits, such as the reproductive mode (broadcaster or brooder) and pelagic larval duration (PLD), can determine how far a coral larvae can disperse, with broadcasting corals usually having a greater PLD and, therefore, higher dispersal potential than brooder corals (Ritson-Williams et al., 2009; Nunes et al., 2011). When coral larvae reach ocean currents, they are subject to environmental filters, such as distance (van der Ven et al., 2022) and semi-permeable river plumes (Tosetto et al., 2022). Finally, there are small-scale environmental filters, including the availability of suitable habitat for coral recruitment. Despite having a limited swimming speed (Hata et al., 2017), coral larvae have advanced sensory abilities to choose where to settle by following cues of healthy reef locations (Vermeij et al., 2010; Tran and Hadfield, 2013). Both settlement and establishment processes can be influenced by environmental variables and biological resistance depending on which benthic organisms are priorly established.
Coral distribution is often a combination of establishment potential, driven by biological resistance or facilitation from other benthic organisms, and environmental filtering. For example, corals may struggle to settle and survive in reefs dominated by macroalgae, due to competition for resources (Kuffner et al., 2006; Birrell et al., 2008; Diaz-Pulido et al., 2010; Webster et al., 2015). Crustose coralline algae, on the other hand, can enhance coral recruitment, development, growth, and survival (Tebben et al., 2015). Among the environmental factors, light availability plays a critical role in shaping the establishment and consequently the distribution of coral species along depth gradients, ultimately determining differences in the assemblage composition between shallow and mesophotic reefs (Kahng et al., 2019; Tamir et al., 2019). Temperature and light are often correlated and tend to decrease with depth and higher latitudes, leading to distinct coral assemblages between shallow and mesophotic reefs (Kahng et al., 2019) and tropical versus subtropical reefs (Santana et al., 2023).
While nutrient-rich waters from upwelling events and river inputs, can increase productivity in coastal reefs (Braga and Müller, 1998; Braga et al., 2008; Han et al., 2012), mud and other terrestrial debris carried by riverine input into these reefs might reduce favorable conditions, increasing turbidity and affecting coral diversity (Golbuu et al., 2008, Golbuu et al., 2011). Along the SWA, massive river inputs result in nutrient-rich and highly turbid waters (Mies et al., 2020), a set of conditions that structure marine benthic communities in this region, including corals (Cord et al., 2022; Santana et al., 2023). Southwestern Atlantic (SWA) reefs have a higher nutrient concentration when compared to the Indo-Pacific and Caribbean reefs (Mies et al., 2020), and harbor low coral species richness summing 20 species when scleractinian coral and hydrocoral species are combined. Yet, these species compose a highly endemic assemblage (7 endemic species: 4 corals and 3 hydrocorals; Leão et al., 2003; Amaral et al., 2008). The SWA coral assemblages have thrived in highly turbid and nutrient-rich conditions over time and are well adapted to turbidity (Loiola et al., 2013; Loiola et al., 2019; Mies et al., 2020). On the other hand, SWA corals occupy a reduced portion of the functional space when compared to corals from the Indo-Pacific and Caribbean regions (McWilliam et al., 2018). Despite the reduced functional diversity, corals are still keystone species in favoring the occurrence of fish species and supporting reef fish assemblages (Luza et al., 2022). Such limited coral diversity and functional redundancy might imply greater vulnerability and lower resistance and/or resilience of these assemblages to disturbances. However, an approach combining spatial patterns of taxonomic and functional diversity of coral and hydrocoral assemblages in the SWA that could contribute to understanding this potential vulnerability is still lacking in the literature (but see Luza et al., 2022 for a functional perspective).
Essential in functional approaches, species traits can help us capture diversity patterns, and understand the responses of biological communities to changes in environmental conditions, and its potential effects on ecosystem processes (Lavorel and Garnier, 2002; Violle et al., 2007). A multitude of functional diversity metrics are available (e.g. Mason et al., 2005; Villéger et al., 2008; Mouillot et al., 2013), frequently relying on organisms’ or species traits, their occurrences and/or abundance values. Functional richness and functional evenness are considered as the primary components of functional diversity (Mason et al., 2005; Villéger et al., 2008) that along with other metrics, such as functional dispersion and functional originality, can track how communities were structured (Mouillot et al., 2013). When applied across biogeographical scales, these trait-based approaches and functional metrics can offer insights into the drivers of community assembly, from regional-scale environmental filters to historical-evolutionary large-scale processes (Mouillot et al., 2014; McWilliam et al., 2018). A more detailed description of functional diversity patterns and its drivers remain to be investigated in Southwestern Atlantic coral assemblages.
In this study, we provide a comprehensive approach on the taxonomic and functional diversity patterns of shallow water zooxanthellate corals and hydrocorals of Southwestern Atlantic reefs, by presenting a regionalization based on coral species, quantifying the taxonomic and functional diversity of each region and between regions, and investigating the influence of species richness and composition on functional richness, evenness, dispersion, and redundancy. We expected that the Abrolhos bank region (~12°S - ~20°S) would have the highest taxonomic and functional diversity, due to its larger continental shelf and its potential role as a past refugium to sea level changes in this region (Leão et al., 2003; Menezes et al., 2020). Therefore, taxonomic and functional diversity would exhibit a gradual decline both southwards and northwards with increasing distance from this center of diversity, transitioning from taxonomic and functional nestedness to turnover patterns (Figure 1). In the taxonomic approach we used occurrence data on 20 coral species to identify: (i) regions based on coral assemblages (using a cluster analysis); and (ii) the taxonomic beta diversity patterns. In the functional approach, we used nine coral traits related to dispersion, persistence, and ecological role, to assess: (iii) the functional richness (FRic); (iv) the functional dispersion (FDis), functional evenness (FEve) and functional originality (FOri) in each region; (v) the functional beta diversity patterns; and (vi) the potential environmental, ecological, and evolutionary drivers among regions. These functional metrics can offer valuable insights into the roles of species in local assemblages, their degree of uniqueness and redundancy. Since biological diversity supports ecosystem functioning and stability, it is important to assess the taxonomic and functional diversity patterns of coral assemblages, as well as the role of its multiple environmental and ecological drivers, particularly in a biodiversity loss due to a climate change scenario.
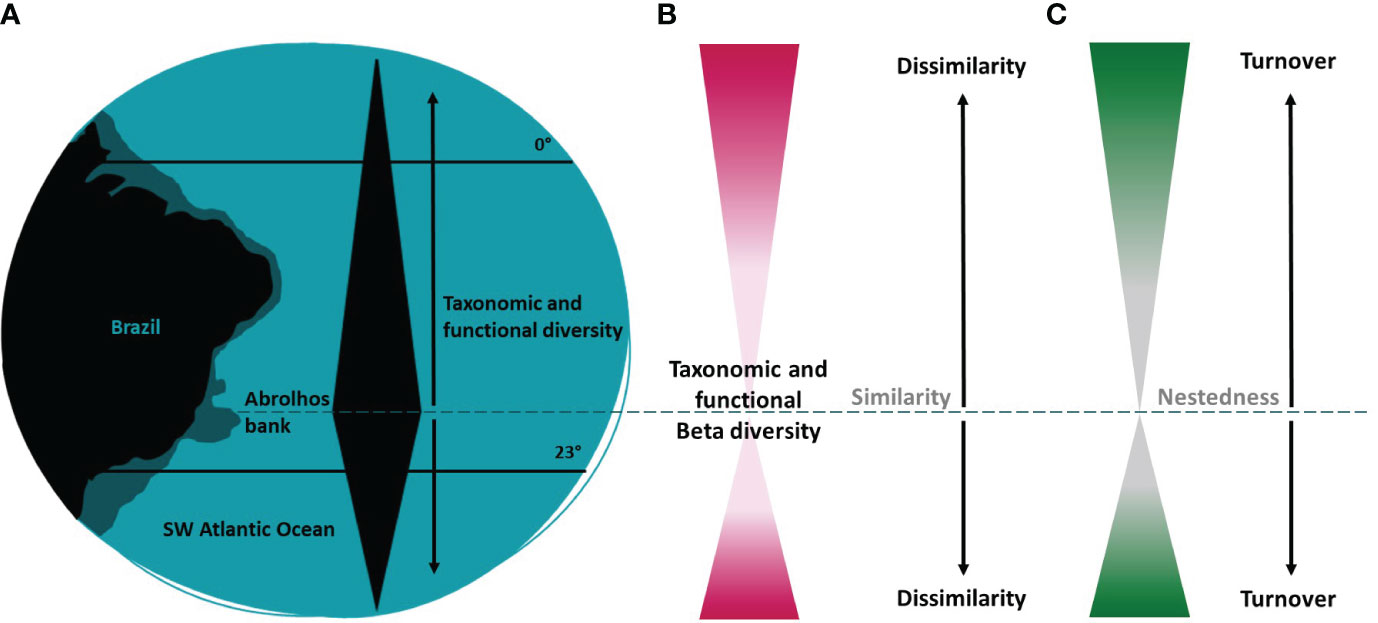
Figure 1 Visual representation of this study hypothesis. (A) The Abrolhos bank region (~13°S - ~19°S) would have the highest taxonomic and functional diversity, due to its larger continental shelf and potential role as a past refugium to sea level changes. Consequently, (B) taxonomic and functional diversity would reduce both southward and northward with increasing distance from this center of diversity, (C) changing from species loss to species replacement (nestedness to turnover drivers).
2 Materials and methods
2.1 Coral data and localities
We used a presence absence database with information on 20 coral and hydrocoral species spanning shallow to mesophotic reefs (up to 180 meters) sourced from Bleuel et al. (2021), supplemented with updated records from coral specialists and authors’ personal observations (dataset will be available as a Supplementary File). This dataset comprises 1005 coral records from 33 publications, covering 163 coordinates (lat/lon) across 118 sites and 45 localities in the Southwestern Atlantic, spanning from 1993 to 2017 (for detailed information, refer to Bleuel et al., 2021 and Supplementary Material). The coral data was further organized into 21 localities based on geopolitical States, as appropriate, to facilitate analysis (Supplementary Material; Figure 1). The Amazon reefs (AM) locality comprises deeper reefs (~70 to 220m) that are influenced by the Amazon River plume and are characterized by low complexity and largely composed of crustose calcareous algae platforms and rhodoliths beds (Moura et al., 2016; Francini-Filho et al., 2018). The Parcel do Manuel Luís (PML) locality comprises shallower and more complex reefs (~30m) formed of pillar and wall pinnacles, patch reefs, rhodolith beds and shipwrecks (Leão et al., 2003; Moura et al., 2016; Francini-Filho et al., 2018; Cordeiro et al., 2021). The locality of Archipelago of São Pedro and São Paulo (SPSP), the most remote oceanic island, is formed of rocky shallow reefs up to 30 m depth and deeper reefs beyond 100 m depth (Magalhães et al., 2015). Reefs located at the coast of Piauí (PI) are composed of few patch and bank reefs close to the coast, with fewer coral species and are less explored and studied (Leão et al., 2003). The locality of Ceará (CE) comprises shallow and mesophotic reefs, including sandstone reefs and rhodolith beds, ranging from 16m to 40m depth (Soares et al., 2017; Barroso et al., 2022). The locality of Rocas atoll (AR), built on the flat top of a seamount chain that is part of the Fracture Zone of Fernando de Noronha, has an elliptical format composed of opened and closed pools that are formed of reef proper and sandy deposits (up to 20m) (Kikuchi and Leão, 1997; Leão et al., 2003; Longo et al., 2015). The locality of Fernando de Noronha (FN) is a volcanic archipelago of rocky reefs, that comprises shallow to mesophotic reefs, including some rhodolith beds (Amado-Filho et al., 2012; Matheus et al., 2019). The localities of Rio Grande do Norte (RN), Paraíba (PB), Pernambuco (PE), and Alagoas (AL) comprises reefs that are formed of coral knolls, patch reefs, elongated banks and attached bank reefs parallel to the coast (Maida and Ferreira, 1997; Leão et al., 2003). The locality of Sergipe (SE) has a great influence of the São Francisco River plume, the reefs (~30m-44m) are formed of siliciclastic sand and mud with rhodolith beds with bryozoan and foraminifera, and corals (Vale et al., 2022). The locality of Northern Bahia (BAN), from Praia do Forte (12.5°S) to Ilheus (14.8°S), comprises bank reefs and discontinuous attached bank reefs, fringing reefs and patch reefs of approximately 10 m depth (Leão et al., 2003). The locality of Southern Bahia and Abrolhos reef complex (BAS-ABR) from Aracaípe (16.1°S) to Viçosas Reefs (18°S). The locality of Espírito Santo (ES), south of the Abrolhos bank, comprises biogenic reefs at depths ranging from 12m to 25m that are influenced by the Doce River plume (Mazzei et al., 2017; Aued et al., 2018). The locality of Vitória-Trindade Seamount Chain (CVT) comprises seamounts mainly covered by rhodolith beds with low complexity ranging at depths between 20 m to 50 m with some reefs up to 90 m depth (Meirelles et al., 2015). The locality of Trindade island (TR), which is one of the easternmost and isolated islands of the CVT, comprises rocky reefs covered with rhodolith beds (Pereira-Filho et al., 2011; Meirelles et al., 2015). The locality of Rio de Janeiro (RJ), the southern limit of most coral species, comprises rocky reefs near the beach to rocky islands (Oigman-Pszczol and Creed, 2004; Lima and Coutinho, 2016). The locality of São Paulo (SP) comprises rocky reefs from Ubatuba (23°30’S; up to 5m depth), to Queimada Grande Island (24°20’S, up to 20 m depth) where only two scleractinian corals occur, Madracis decactis and Mussismilia hispida (Pereira-Filho et al., 2019; Banha et al., 2020; Grillo et al., 2021; Pereira-Filho et al., 2021). The locality of Paraná (PR) comprises a few rocky reef islands where only Madracis decactis occurs. The locality of Santa Catarina (SC) comprises the rocky reefs of Galé Island and Arvoredo Island (27°S), of 6 to 15m depth, where only the scleractinian coral Madracis decactis occurs (Capel et al., 2012).
2.2 Coral traits
We built a coral trait database by searching on coraltraits.org and google scholar using keywords related to traits, species names and both combined (e.g.: “functional traits”, “coral traits”, “sexual system”, “growth rate”, “corallite width”, “Scolymia”, “Mussismilia”, “Favia larval development”, “Millepora reproductive mode”). We used nine traits that are representative of key ecological functions of corals in reef systems, such as calcium carbonate production, habitat provision and nutrient cycling (Table 1). We used three reproductive traits (sexual system, mode of development and gamete release, and reproductive period) that are associated with repopulation and structure/dynamic of coral populations and dispersal capacity (Baird et al., 2009). The sexual system can influence fertilization success, in which hermaphroditic corals have an advantage compared to gonochoric species in processes of repopulation and population structure. Mode of development and gamete release influences the coral dispersal potential, in which spawning corals have a broader dispersal range than brooding corals. And the reproductive period can both be influenced by temperature change (i.e. climate change) and shape population dynamics through mass spawning events adding genetic diversity. Three growth traits (colony height, growth rate, and maximum diameter) that are indicative of calcium carbonate accretion (Madin et al., 2016), two of which also indicate reef complexity and the space provided to other reef organisms as shelter and microhabitat, potentially influencing the distribution of associated species (colony height and maximum diameter; Graham and Nash, 2013; Nogueira et al., 2015). Maximum diameter also indicates colony fecundity, in which larger colonies have higher fecundity and contribute with more gametes/larvae to the reef system (Álvarez-Noriega et al., 2016). We used maximum corallite width as proxy for coral’s mouth size (Lewis, 2006) for scleractinian corals that can indicate higher heterotrophic feeding capacity and thus higher heterotrophic contribution to the coral’s nutrition (Porter, 1976; Muscatine et al., 1989, but see Houlbrèque and Ferrier-Pagès, 2009). However, as hydrocorals do not have corallites, we used maximum gastropore width (Amaral et al., 2008) as their functional analog (Weiss and Ray, 2019). Depth limit is indicative of the vertical distribution of coral species, the vertical niche space occupied (habitat zonation) and habitat provision, which can change with light availability, all of which influence the structural composition and biodiversity of reef systems (Bongaerts et al., 2010; Kahng et al., 2016). We also included endosymbiont diversity of clades (Symbiodiniaceae) that indicates the number of different associations recorded between coral host and symbiont, in which higher associations with different Symbiodiniaceae lineages can indicate higher potential of resistance and/or resilience of the holobiont to coral bleaching through mechanisms such as symbiont shuffling (Thomas et al., 2019), a proxy for corals’ persistence in reef systems (see details in Table 1). When traits were not available for the Brazilian coral species, we used trait information of a coral species from the same genera from the Caribbean (details in the Supplementary File). For maximum growth rate, which was unavailable for seven coral species, we used the ‘missForest Nonparametric Missing Value Imputation using Random Forest’ to impute missing values (Stekhoven and Bühlmann, 2012).
2.3 Taxonomic diversity patterns
We used a cluster analysis to evaluate groupings among the Southwestern Atlantic reefs based on coral composition (presence/absence coral data). First, we built Jaccard dissimilarity matrix and then performed a cluster analysis with the Unweighted Pair Group Method with Arithmetic Average (UPGMA) as grouping algorithm, based on cophenetic analysis (Sneath and Sokal, 1973; cophenetic function from the “stats” package; R Core Team, 2022). Finally, we computed a similarity profile analysis using the simprof function from the “clustsig” package (Whitaker and Christman, 2010) to identify significant clusters and obtain significance values for each cluster branch from approximately Unbiased and Bootstrap probability calculated using pvclust function from the “pvclust” package (Suzuki and Shimodaira, 2006).
2.4 Functional diversity patterns
We built a functional trait-based distance matrix with nine mixed trait variables (categorical, ordinal, and quantitative, see Table 1) of 20 coral and hydrocoral species using the Gower distance (dist.ktab function; Gower and Legendre, 1986) as it allows the use of different types of variables when calculating distances while assigning traits with equal weight. With this distance matrix, we performed a Principal Coordinate Analysis (PCoA) to organize species into a functional space (Villéger et al., 2008) and maintained the first four PCoA axes which had the highest explanation of trait space variation and maximized trait space quality (Maire et al., 2015; Supplementary Figure 2). For graphical representation, we used the combinations among the first 3 axes that provided a more informative representation. To obtain the trait space volume for each region, we divided the convex hull of each region by the functional space occupied by the entire SWA coral fauna using the convhulln function from the “geometry” Package in R (R Core Team, 2022; Habel et al., 2023). We used four functional indices to measure the contribution of species composition and distribution to the functional trait space: Functional richness (FRic) indicates the proportion of space occupied by species; Functional Evenness (FEve) indicates how regularly distributed the species are within the trait space; and Functional dispersion (FDis) indicates how they are distributed considering trait range values, and the functional originality (FOri) is measured as the isolation of a species in the functional space (Mouillot et al., 2013). All indices were calculated using the multidimFD function from the “multidimFD” package (Mouillot et al., 2013). Here FEve and FDis were calculated on binary data (Villéger et al., 2008; Mouillot et al., 2013), and functional originality (FOri) indicates functional redundancy - lower originality values depict redundancy in trait space, while higher originality represents low redundancy among species in trait space (Mouillot et al., 2013). We are aware that FEve and FDis are abundance-weighted indices, yet they are also informative when measured with binary data. FEve, for instance, when calculated from binary data informs on the regularity of species distributions, while FOri informs on species traits originalities in each locality, here represented by the binary matrix (Villéger et al., 2008; Mouillot et al., 2013). In addition, we measured the community weighted means (CWM, for binomial data) using the Functcomp function from the “FD” package. Lastly, we evaluated the relationship between species richness and functional diversity using a Pearson correlation analysis and a linear regression model using the ggscatter function from the “ggpubr” package (Kassambara, 2019) in R (R Core Team, 2022).
2.5 Beta diversity patterns
We analyzed beta diversity patterns across eight Southwestern Atlantic reef regions indicated by the similarity profile analysis. To assess taxonomic beta diversity based on the occurrence of coral species across regions, we used the Jaccard dissimilarity matrix and partitioned beta diversity using the beta.div.comp function from the “adespatial” package (Legendre, 2014). For functional beta diversity, we calculated pairwise beta diversity using the functional space dimensions of the PCoA output and the Jaccard dissimilarity index using the functional.beta.pair function from “betapart” (Baselga, 2017). All analyses were conducted in R software (R Core Team, 2022).
2.6 Trait-environment patterns
To investigate how environmental variables may have shaped coral regions and the taxonomic and functional diversity patterns along the SWA we used a distance-based redundancy analysis (dbRDA). We used the presence and absence of corals organized by regions (R1 to R8) to build a resemblance matrix based on Jaccard Dissimilarity to use as biological data in the dbRDA. We obtained the annual mean of eight environmental variables between 2000 and 2014 from BioOracle (http://www.bio-oracle.org; Tyberghein et al., 2012; Assis et al., 2018): sea surface salinity (SSS), sea surface temperature (SST), chlorophyll, current velocity, phytoplankton, calcite, Photosynthetically Available Radiation (PAR), and Diffuse Attenuation Coefficient (KD). These variables were chosen because they are related to corals optimum environmental conditions, niche, and dispersion dynamics (Coles and Jokiel, 1992), considered primary determinants of reef distribution (Kleypas et al., 1999) and, to some extent, relate to the selected coral traits (Table 1). Environmental variables such as SST and SSS are related to several dimensions of coral’s success, development, and dispersion, while other variables may be associated with more specific coral traits. Current velocity can drive dispersion dynamics of coral larvae and repopulation in reef systems (White et al., 2010; Watson et al., 2011), while calcite and PAR are indicative of calcium carbonate accretion for calcifying organisms (Fabricius and De’ath, 2001). SSS, KD and PAR are variables associated to river input into the ocean, which may alter the preferable salinity and light incidence conditions of corals (Bleuel et al., 2021; Santana et al., 2023) and reflect species’ occurrence and carbonate accretion. These variables together with Chlorophyll and phytoplankton, indicate water nutrient availability (Kumar et al., 2010; Yang et al., 2020) and are associated with coral heterotrophic feeding traits. The environmental variables were also grouped by region (R1 to R8) and standardized with zero means to build a Euclidean distance matrix for the dbRDA. To select the environmental variables to perform the dbRDA, we considered the correlation, multicollinearity, and importance of the variables to the reef systems (Zuur et al., 2010). We checked the correlation between variables using Pearson’s correlation index, in which we excluded variables with correlation higher than 0.8 using the “corrplot” function (Wei and Simko, 2017). In addition, we checked the multicollinearity between variables using the generalized variance inflation factor (GVIF), removing variables with GVIF higher than 3 using the “covif” function (Fox and Weisberg, 2011). Only chlorophyll was forced to be maintained in the model due to its relevance as an environmental variable to our latitudinal gradient and reef ecosystems. Using the Vegan package in R (Oksanen et al., 2013; R Core Team, 2022), we ran the dbRDA using the function “capscale”, performed an analysis of variance (ANOVA; function “anova”) on the fitted model to assess the significance of terms, and used partial tests to assess the significance of individual predictor variables in the dbRDA while accounting for the effects of other variables (function “partial tests”).To include a functional interpretation of species traits among the eight regions and the environmental variables we displayed the dbRDA results together with the CWM results.
3 Results
3.1 Taxonomic diversity patterns
The Southwestern Atlantic reefs (SWA) harbors 20 zooxanthellate corals and hydrocorals species within 11 genera and 9 families. There are 16 coral species, from which 4 are endemic to the genus Mussismilia, and 4 hydrocoral species of the Millepora genus, from which 3 are endemic (Figure 2; Supplementary Figure 1). Despite the absence of a clear latitudinal gradient of coral species richness in the SWA, we found higher species richness and endemism in Eastern Brazil (12°S – 20°S), followed by Northeastern Brazil (5°S- 10°S) (Figure 2; Supplementary Figure 1). Based on cluster analysis, we identified eight regions across the SWA (Figure 2): R1= Amazon Reefs (AM; ~1°N) and Trindade-Martin Vaz Island (TR; ~20°S, 29°W); R2= Parcel do Manuel Luís (PML; ~0°), Rocas Atoll (RA; ~3°S) and Fernando de Noronha Archipelago (FN; ~3°S); R3 (2°S – 4°S) = Piauí (PI) and Ceará (CE); R4 (4°S – 10°S) = Rio Grande do Norte (RN), Paraíba (PB), Pernambuco (PE), Alagoas (AL); R5= Sergipe (11°S; SE) and Victoria-Trindade Seamount Chain (20°S, 31°W – 38°W; VTC); R6 (12°S – 20°S) = Northern Bahia (BAN), southern Bahia plus the Abrolhos region (ABR), and Espírito Santo (ES); R7 (~21°S – 23°S) = Rio de Janeiro (RJ); R8 (~24°S – 27°S) = São Paulo (SP), Paraná (PR), Santa Catarina (SC) and the Archipelago of São Pedro and São Paulo (ASPSP; ~0°, 29°W).
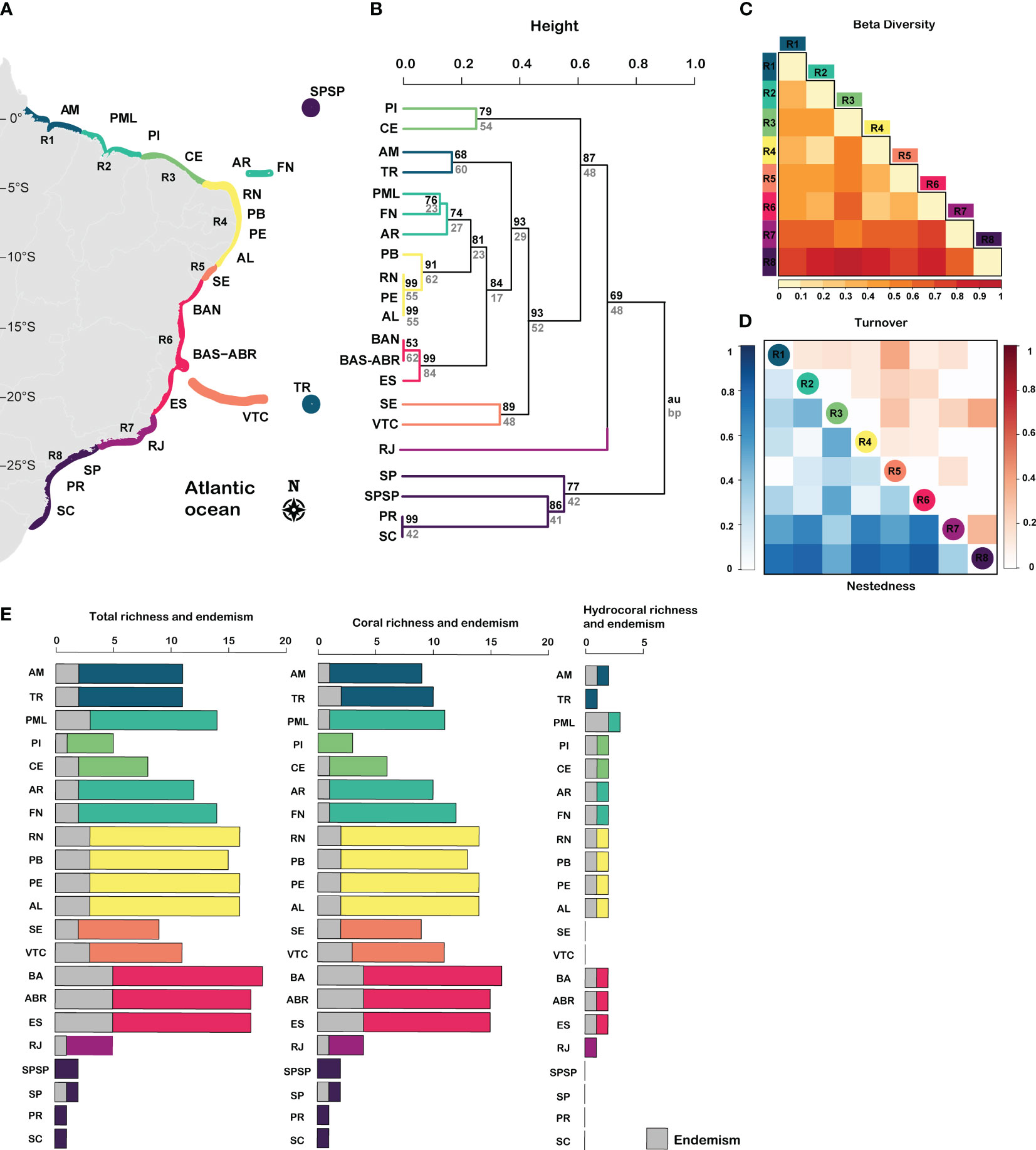
Figure 2 Taxonomic diversity of zooxanthellate corals and hydrocorals in Southwestern Atlantic reefs. (A) Regionalization, (B) Cluster of taxonomic diversity (C) total beta diversity, (D) turnover and nestedness, and (E) richness and endemism of corals and hydrocorals. R1= Amazon Reefs (AM; ~1°N) and Trindade-Martin Vaz Island (TR; ~20°S, 29°W); R2= Parcel do Manuel Luís (PML; ~0°), Rocas Atoll (RA; ~3°S) and Fernando de Noronha Archipelago (FN; ~3°S); R3 (2°S - 4°S) = Piauí (PI) and Ceará (CE); R4 (4°S - 10°S) = Rio Grande do Norte (RN), Paraíba (PB), Pernambuco (PE), Alagoas (AL); R5= Sergipe (11°S; SE) and Victoria-Trindade Seamount Chain (20°S, 31°W - 38°W; VTC); R6 (12°S - 20°S) = Northern Bahia (BAN), southern Bahia plus the Abrolhos region (ABR), and Espírito Santo (ES); R7 (~21°S - 23°S) = Rio de Janeiro (RJ); R8 (~24°S - 27°S) = São Paulo (SP), Paraná (PR), Santa Catarina (SC) and the Archipelago of São Pedro and São Paulo (ASPSP; ~0°, 29°W).
A large portion of the SWA, between latitudes 1°N and 20°S (R1-R6), had low variation in species composition, indicated by low taxonomic beta diversity values (Figure 2C; β ≤ 0.4), except for region R3 between latitudes 2°S – 4°S (Figure 2C; β = 0.5). This large portion has similar contributions of both turnover and nestedness. On the other hand, the Southeastern regions R7 and R8 (21°S to 27°S) had greater differences in species composition among sites, leading to higher beta-diversity values (β ≥ 0.6). Nestedness was the predominant taxonomic beta diversity component, except between R8 and R3 marked by a high turnover value (latitudes 2°S – 4°S) (Figure 2E).
3.2 Functional diversity patterns
Our principal coordinate analysis (PcoA) using nine functional traits of 20 coral species explained 83.16% of variation in four dimensions (axis) and had high quality (Qual.Fric = 0.65; Figure 3 and Supplementary Figures 2–4). In the first axis, species are arranged from small-sized brooder corals on the left to large-sized spawning corals on the right. In the second axis, species are distributed from gonochoric, fast-growing with small corallite width corals on the bottom to hermaphrodite, slow growing with large corallite width corals on the top. In axis 3, species are arranged from corals inhabiting shallow waters with high Symbiodiniaceae diversity to corals inhabiting deeper waters with low Symbiodiniaceae diversity (Figures 3B, C).
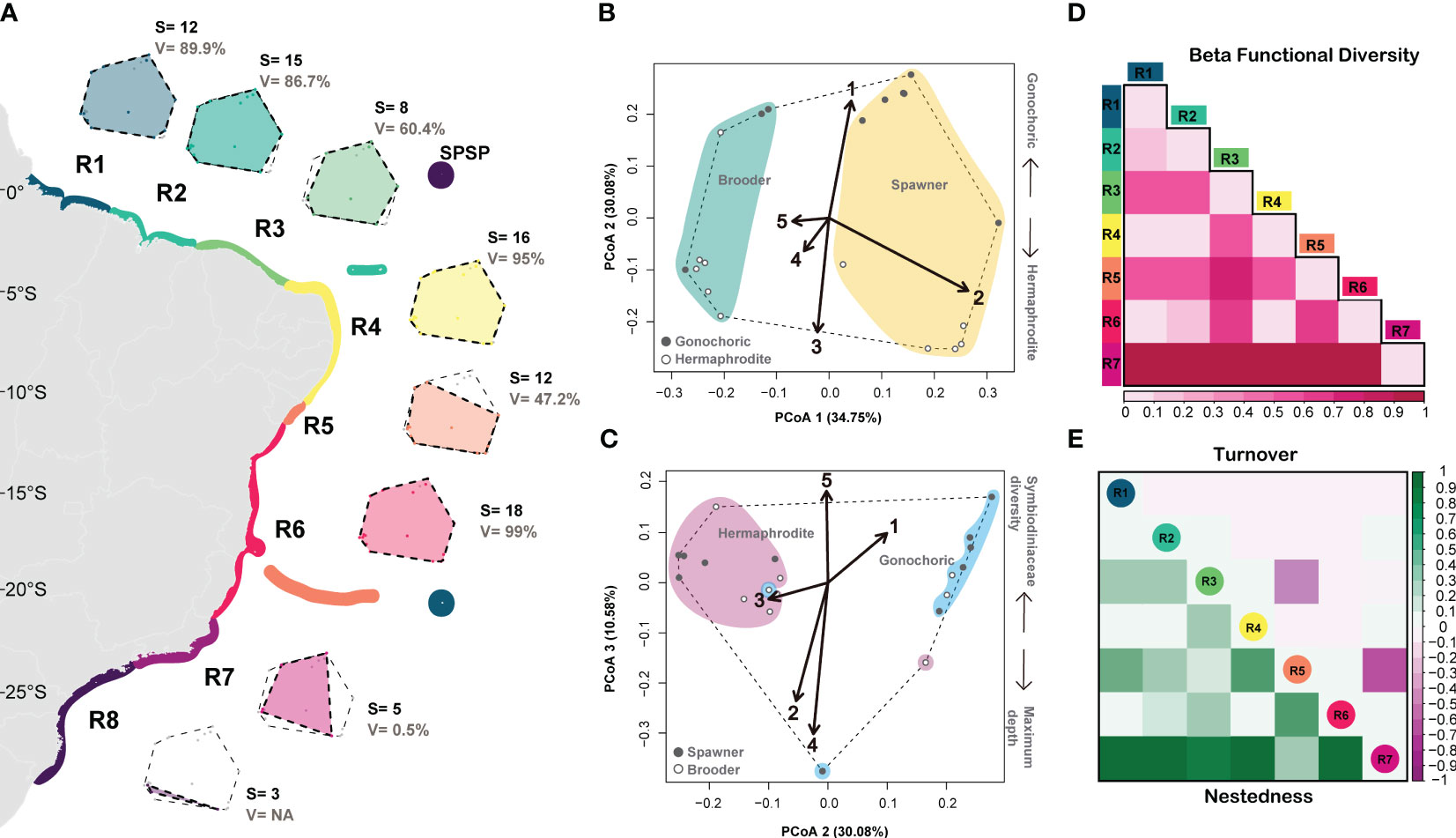
Figure 3 Functional diversity of zooxanthellate corals and hydrocorals in the SWA. (A) regionalization and functional trait spaces, (B, C) functional trait space (PCoA) and trait vectors (1) maximum growth rate (2) maximum diameter, (3) corallite width, (4) maximum depth limit, (5) Symbiodiniaceae diversity). (D) functional beta diversity and (E) functional turnover (in purple) and nestedness (in green). R1= Amazon Reefs (AM; ~1°N) and Trindade-Martin Vaz Island (TR; ~20°S, 29°W); R2= Parcel do Manuel Luís (PML; ~0°), Rocas Atoll (RA; ~3°S) and Fernando de Noronha Archipelago (FN; ~3°S); R3 (2°S - 4°S) = Piauí (PI) and Ceará (CE); R4 (4°S - 10°S) = Rio Grande do Norte (RN), Paraíba (PB), Pernambuco (PE), Alagoas (AL); R5= Sergipe (11°S; SE) and Victoria-Trindade Seamount Chain (20°S, 31°W - 38°W; VTC); R6 (12°S - 20°S) = Northern Bahia (BAN), southern Bahia plus the Abrolhos region (ABR), and Espírito Santo (ES); R7 (~21°S - 23°S) = Rio de Janeiro (RJ); R8 (~24°S - 27°S) = São Paulo (SP), Paraná (PR), Santa Catarina (SC) and the Archipelago of São Pedro and São Paulo (ASPSP; ~0°, 29°W).
In general, the SWA coral assemblage has high functional evenness (FEve) and dispersion (FDis) and low functional originality (FOri), which translates into high functional redundancy (Table 2). Species richness is highly correlated with functional richness (R=0.85; p-=0.015; Supplementary Figure 5). In addition to harboring the highest species richness (18), region R6 (12°S – 18°S) comprises the highest functional richness (Fric = 0.99), filling 99.9% of the SWA coral trait space. The region R4 (5°S – 10°S) had the second-highest species richness (16), functional diversity (Fric = 0.95) and volume, filling 95% of the SWA coral trait space. Between the most speciose regions (R6 and R4), R6 has the lowest functional originality, therefore the highest functional redundancy. The Southeastern and Southern regions R7 and R8, respectively (21°S to 27°S) have the lowest species richness (5 and 3, respectively), and R7 has the lowest functional richness (0.005), filling only 0.5% of the SWA trait space. We could not measure functional richness for R8 (24-27°S and the SPSP Archipelago ~0°, 29°W) given its low species richness (<4). The Regions R3 and R5 had comparable functional volumes (60.4% and 47.2%, respectively), despite harboring different species richness (8 in comparison to 12, respectively). These comparable functional volumes reflect differences in functional redundancy, region R3 (that also has high functional originality and functional evenness) presents lower redundancy when compared to region R5 (11°S-20°S). It may also reveal that Millepora and Mussismilia genera have disproportionately higher contributions to the functional diversity in region R3 relative to region R5. In contrast, regions R1(1°N) along with Trindade Island (20°S, 31°W) and R5 (11°S-20°S) had large differences in their functional volume (89.9% and 47.2%, respectively) despite harboring equivalent species richness (12). Coral species in the northern region and Trindade Island (R1) are functionally more original and less redundant in comparison to those of region R5 (11°S-20°S) that presents lower functional originality and, therefore, higher functional redundancy (Table 1). Regions R3 (2°S-4°S), R5 (11°S–20°S), and R7 21°-23°S) had the highest functional beta diversity and high turnover values compared to the other regions that had higher nestedness values (Figures 3D, E). These three regions (R3, R5 and R7) also had the greatest differences in their occupancy/filling of trait space (Supplementary Figure S4) which is supported by their high functional turnover values (Figure 3E).
3.3 Trait-environment patterns
The dbRDA model revealed a statistically significant relationship between the environmental variables and the variation in biological data, accounting for a substantial proportion of the variation in the data (F = 2.39, p = 0.011; R2 = 0.86). Notably, the first two constrained axes, dbRDA1 and dbRDA2 (Figure 4), captured a considerable amount of variation, collectively explaining 78.86% of the total variation observed in the biological data. These findings underscore the importance of the environmental variables in shaping the composition and structure of these coral assemblages. Additionally, partial tests of individual predictor variables revealed a significant contribution of Chlorophyll (F=4.03; p= 0.018) which was mostly related to the dbRDA1 (eigenvalue= -0.74), and a marginal significance of PAR (F=2.05; p= 0.084) that had the major contribution to dbRDA1 (eigenvalue = 0.92), and KD (F=2.28; p= 0.083) that had a higher contribution to dbRDA2 (eigenvalue= -0.84). The predictor SSS was not significant (F=1.20; p= 0.352) and had a greater contribution to dbRDA 3 (eigenvalue = 0.83) that added about 10% of the total explanation of the model. The regions from latitude 1°N-0° (R1 and R2) were positively related to salinity and PAR, and negatively related to chlorophyll. The regions between latitudes 5°S-18°S (R4-R6) were positively related to salinity and KD. The regions from latitude 21°S-27°S (R7 and R8) were positively related to chlorophyll, with R8 also being positively affected by increased KD. The region from latitude 2°S-4°S (R3) was negatively related to KD and was positively affected by PAR (Figure 4A). In general, SWA reefs have proportionately more species with gonochoric sexual systems and brooding mode of development, with no remarkable pattern in the timing of reproduction, with generally low symbiont diversity, and with the potential of reaching large diameters. The regions between latitudes 11°S-20°S (R6) and 21°S-27°S (R7 and R8) have proportionately more species with hermaphrodite sexual system, and the southern region (R8, 24°S-27°S) has proportionately more brooder species with hermaphrodite sexual system, annual reproduction, smaller colony-heights (incrusting, massive-low-height to intermediate-height hemispheric, branching-pilar with thick branches), with a widest depth range distribution and larger corallite size (Figure 4B).
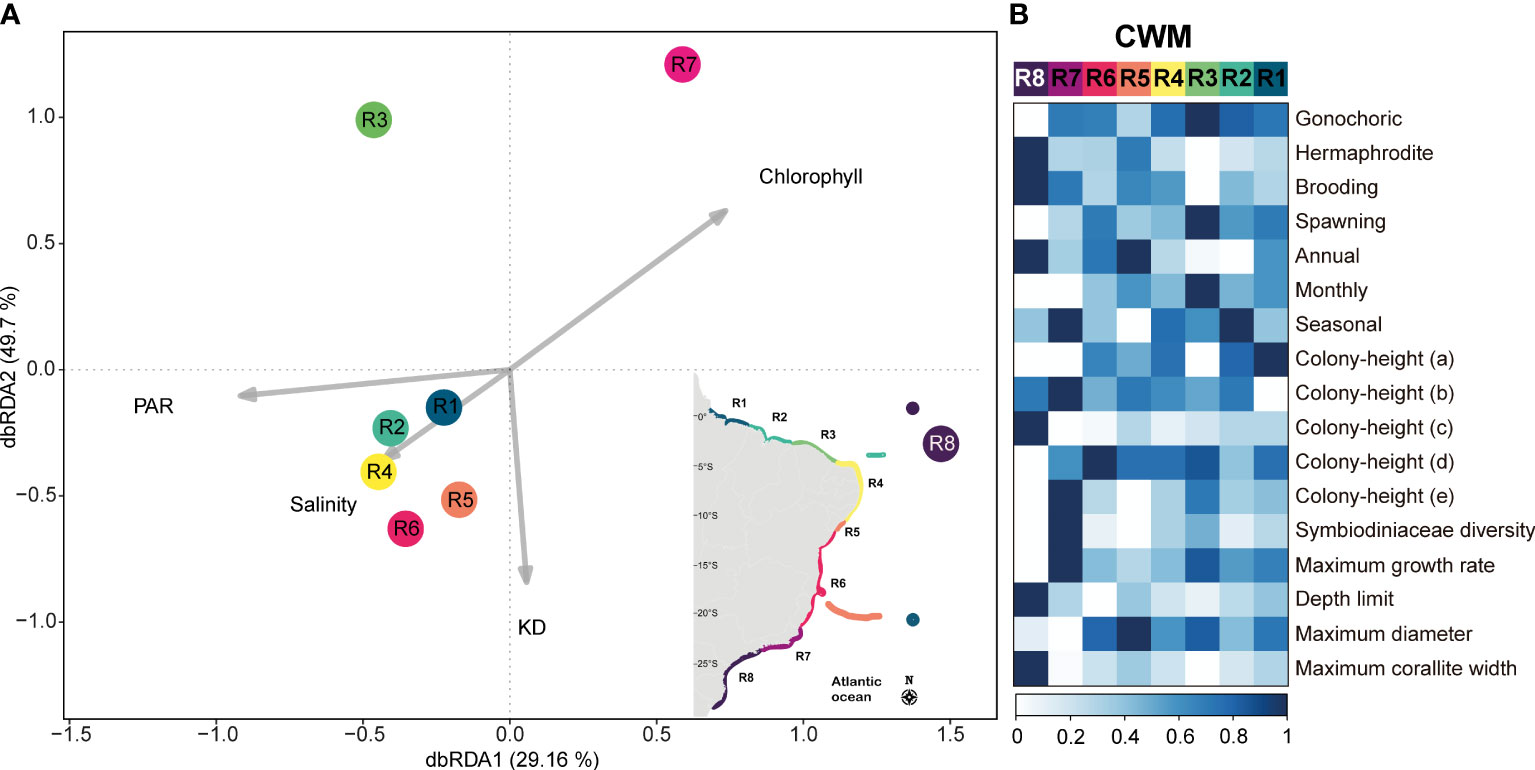
Figure 4 Trait-Environment patterns. (A) Distance based redundancy analysis (dbRDA) and (B) community weighted means (CWM). Darker tones of blue indicate higher contribution of the trait to the regions that are represented by the different colors (following the clustering colors for each region). R1= Amazon Reefs (AM; ~1°N) and Trindade-Martin Vaz Island (TR; ~20°S, 29°W); R2= Parcel do Manuel Luís (PML; ~0°), Rocas Atoll (RA; ~3°S) and Fernando de Noronha Archipelago (FN; ~3°S); R3 (2°S - 4°S) = Piauí (PI) and Ceará (CE); R4 (4°S - 10°S) = Rio Grande do Norte (RN), Paraíba (PB), Pernambuco (PE), Alagoas (AL); R5= Sergipe (11°S; SE) and Victoria-Trindade Seamount Chain (20°S, 31°W - 38°W; VTC); R6 (12°S - 20°S) = Northern Bahia (BAN), southern Bahia plus the Abrolhos region (ABR), and Espírito Santo (ES); R7 (~21°S - 23°S) = Rio de Janeiro (RJ); R8 (~24°S - 27°S) = São Paulo (SP), Paraná (PR), Santa Catarina (SC) and the Archipelago of São Pedro and São Paulo (ASPSP; ~0°, 29°W). Acronyms: KD, Diffuse Attenuation Coefficient; PAR, Photosynthetically Available Radiation; SST, Sea Surface Temperature.
4 Discussion
By examining the taxonomic diversity of shallow water zooxanthellate corals and hydrocorals, we identified eight distinct regions along the SWA. These regions align with previous studies on marine biogeography, further supporting their validity (Leão et al., 2003; Spalding et al., 2007; Cord et al., 2022). We found two centers of coral diversity: the Abrolhos Bank region (R6, ~12°S - ~20°S) with the highest taxonomic and functional diversity followed by region R4 (5°S - 10°S). Both taxonomic and functional diversity of coral assemblages decrease with increasing distance from these two diversity centers. Contrary to our hypothesis turnover components led to differences in taxonomic composition between the center of diversity (R6, 12°S - 20°S) and northward regions (R1 to R5), while nestedness was predominant in driving differences between R6 and the regions further south (R7 and R8; ~21°S-27°S). Nestedness (species loss) caused changes in functional composition/structure between regions (except between R3 and R5 and R5 and R7). Functional diversity increased with species richness, but not necessarily leading to increased functional redundancy, as there were regions with equivalent species richness but with different redundancy. The main drivers of coral assemblages’ composition in the SWA were river plume, ocean temperature (including an upwelling zone), geographic isolation, distance from diversity centers and currents that act as barriers for species dispersion and modify habitat conditions such as nutrient, light availability and temperature through latitudinal and depth gradients (Santana et al., 2023). Despite the low species richness and high nestedness, the combined effects of environmental factors have resulted in taxonomically and functionally variable coral assemblages in the SWA.
The global diversity center of zooxanthellate corals at the Coral Triangle (CT) results from the combination of geological (repeated environmental perturbations and sea-level fluctuations), biogeographic (endemism, overlap of species with wider geographic ranges and dispersion patterns through ocean currents and distance from the CT) and evolutionary factors (all combined plus Darwin’s Centers of Origin, Vicariance, and Reticulate Evolution) operating over time and space (Veron et al., 2011). Currently, the Indo Pacific and the Atlantic regions do not share any coral species, with the Caribbean being the richest Atlantic region and sharing few species with the Brazilian province given the biogeographic barriers like the Amazon River plume (Nunes et al., 2009; Veron et al., 2011). Within the SWA, the Abrolhos Bank region (R6) is a wide continental shelf that has accumulated richness, endemism, and diversification of coral species thought sea-level fluctuations during the Pleistocene (Leão et al., 2003), while ocean currents, ocean temperature, river discharge and isolation are among the biogeographic factors that influenced coral dispersion and establishment along the SWA. In addition, seamounts near the Abrolhos bank have also been hypothesized as refugia to past sea level fluctuations and may have favored recolonization along coastal reefs (Leão et al., 2003; Menezes et al., 2020). Collectively, these factors and events have structured coral assemblages in the Abrolhos Bank region (R6) as the current center of coral diversity in the SWA, while environmental filtering, which can favor the establishment of species with similar traits (McLean et al., 2021), was the main driver of the SWA low taxonomic and functional beta diversity, and the highly nested regions.
River discharge into the ocean decreases salinity and increases water turbidity and sedimentation, forming a plume that may act as a barrier to dispersion and settlement of marine invertebrates, including corals (Conaco and Cabaitan, 2020; Tosetto et al., 2022). Despite having relatively high turbidity levels (KD), the Northeastern and Eastern regions R4 and R6 (5°S-10°S and 12°S-20°S) presented the greater species richness and functional diversity, suggesting these are sediment-tolerant coral assemblages (Loiola et al., 2019). This pattern raises two hypotheses that are not mutually exclusive: (1) turbidity has provided an advantage to coral species that can reach larger diameters, allowing for better light acquisition, and possessing wider corallites – a trait that could potentially be linked to heterotrophic strategies (Porter, 1976), enabling corals to benefit from the increased nutrient input from rivers; and/or (2) turbidity is an environmental filter, that in conjunction with river plumes affecting salinity can select species with these traits, enabling them to thrive in a turbid and nutrient-rich environment. The major rivers that have influenced such regionalization at the SWA are the Amazon River (5°N - 0°), the São Francisco River (10°S) and the Doce River (19°S).
Our results also suggest that the dispersion of the Amazon River plume interacting with local currents may have contributed to the biogeographic separation of the Amazon reefs (R1; ~1°N) from Parcel do Manuel Luís reefs (R2; ~0°) despite their geographic proximity. The Amazon River plume is a soft barrier (semi-permeable sensu Floeter et al., 2008) that limits the faunal exchange of several marine taxa, including reef fishes and coral, between the Caribbean and the Southwestern Atlantic (Rocha, 2003; Floeter et al., 2008; Nunes et al., 2009, Nunes et al., 2011; Pinheiro et al., 2018). Reefs in these two regions differ in structure and complexity. The Great Amazon Reef System (GARS) is more influenced by the Amazon River plume and comprise deeper reefs (between 70 - 220 m) mostly formed of crustose calcareous algae (CCA) ledges and rhodolith beds with lower complexity (Moura et al., 2016; Francini-Filho et al., 2018). Whereas the Parcel do Manuel Luís reefs are shallower (<30m) and more complex, positioned at the southern limit of the GARS and thus less influenced by the Amazon River Plume. Reefs in this region are composed of pillar and wall pinnacles, patchy reefs and rhodolith beds, but there are also shipwrecks that sustain coral assemblages (Leão et al., 2003; Moura et al., 2016; Francini-Filho et al., 2018; Cordeiro et al., 2021). Parcel do Manuel Luís is also the only location where the Brazilian endemic hydrocoral species Millepora laboreli occurs (Amaral et al., 2008). The role of these soft barriers in separating biogeographic regions are consistent with previous studies within several taxa (Cord et al., 2022), including reef fishes (Floeter et al., 2008) and coral symbionts (Picciani et al., 2016), that also highlight differences in reef formation between these regions (Leão et al., 2003).
The Northeastern and Eastern regions (5°S to 20°S, R4 to R6) are associated with high salinity and water turbidity (KD), reinforcing the subdivision of this region by the plumes of two major rivers (São Francisco and Doce rivers). The São Francisco River, for example, has strongly influenced the distribution of two endemic Millepora species, with Millepora braziliensis being restricted to the north of the river and Millepora nitida, to its south (de Souza et al., 2017). In contrast, these rivers have not been effective barriers for the widely distributed and endemic brain coral Mussismilia hispida (Peluso et al., 2018). Still, such rivers strongly influence the coral-host patterns with corals hosting different Symbiodiniaceae clades North and South of the São Francisco (10°S) and Doce (19°S) rivers (Picciani et al., 2016). The riverine input is an important source of nutrient for phytoplankton, zooplankton and contributes to the carbonate cycle by influencing the availability of carbon forms (particulate organic carbon POC and dissolved organic carbon DOC) (Moura et al., 2016). These factors can influence coral growth and calcium carbonate production (Bouillon and Connolly, 2009; Bruce et al., 2012; Meirelles et al., 2015). However, river input may jeopardize coral larvae dispersion, settlement, and development, as they have limited tolerance to salinity fluctuations, high turbidity and sedimentation, and low light environments that usually result from the influence of river plumes (Liaño-Carrera et al., 2019). The numerous rivers that discharge in the Southwestern Atlantic coast might have a great influence on nutrient availability, the dispersion and connectivity of coral species, which may have been pivotal to shape the current distribution and composition of coral assemblages.
Low temperatures were also important drivers of coral assemblage composition, distinguishing tropical from colder, subtropical areas: Rio de Janeiro region (R7, ~22°S), recognized as an upwelling zone (Palma and Matano, 2009; Coelho-Souza et al., 2012) and Southern Brazil (R8, ~24°S - 27°S) as a colder subtropical area. Water temperature is a limiting factor for some coral species and along with seasonal upwelling could influence the dispersion and establishment of corals in these subtropical regions (Leão et al., 2003; Lima and Coutinho, 2016). The lower temperatures in the upwelling region (R7) have been identified as a soft barrier between provinces and marine ecoregions in the SWA (Spalding et al., 2007), a major environmental driver of taxonomic subdivisions across several taxa (Cord et al., 2022) and the southern limit for most coral species that occur along the SWA (Castro and Pires, 2001; Leão et al., 2003, Leão et al., 2016; Lima and Coutinho, 2016). Such patterns might be related to the thermal limits of most coral species with tropical affinity, which generally lies within an average annual minimum and maximum of 21°C and 29.5°C, respectively (Kleypas et al., 1999). The southern region (R7) exhibited a stronger positive association with Chlorophyll, which reinforces the influence of upwelling in the area, as higher chlorophyll concentrations typically correspond with the upwelling season (Walter et al., 2018). Additionally, this region displayed a negative correlation with PAR, which might be associated with reduced light penetration during nutrient-rich waters of the upwelling season. Most corals in this region are brooders that reproduce in the summer, following the peak of upwelling season that decreases water temperature but increases nutrient availability (Oliveira et al., 2013), stimulating phytoplankton blooms that might nourish coral species for pre-reproductive stages and the reproductive season to come. However, colder upwelling waters can slow-down the development of coral larvae (e.g., gastrulation and larvae formation; (Woolsey, 2014)) and reduce settlement success (Rodríguez et al., 2009), which potentially reduces dispersion and repopulation. It seems that among zooxanthellate shallow-water corals only those with greater temperature range plasticity, thermal tolerance and adaptability would thrive in such cold environments (e.g., Siderastrea stellata, Mussismilia hispida, Madracis decactis).
Geographic isolation and distance from diversity centers played an important role in grouping coral assemblages from Saint Peter and Saint Paul’s Archipelago (0°S) with those from the Southern Region (24°S-27°S; R8) but not with Victoria-Trindade Seamount Chain (20°S). Both groupings may be linked to reproductive or dispersal limitations that resulted in a predominance of hermaphrodite brooders in those assemblages. The geographic isolation of oceanic islands and offshore reefs may have driven the differences in species composition when compared to coastal areas, both because of the divergent environmental conditions and the dispersal limitations. The region R3 comprising the oceanic islands of Fernando de Noronha and Rocas Atoll (~3°S) and the region of Parcel do Manuel Luis (0°; ~80km from the coast) clustered apart from the coastal regions exhibiting a mild positive relation to Photosynthetically Available Radiation (PAR) and a stronger negative relation to turbidity (KD). These findings indicate that R3 represents an environment characterized by oligotrophic conditions, where sunlight can penetrate deeply into the ocean and provide an ample light spectral range for photosynthesis. This stands in contrast to coastal regions where the presence of river plumes leads to reduced values for both PAR and turbidity. Differences between oceanic and coastal assemblages were also observed for Brazilian reef fishes. In this case, endemic species within the Labridae, Epinephelidae and Gobiidae families are more diverse in coastal regions and some families are even absent in oceanic islands (e.g., Grammatidae, Microdesmidae, Ophidiidae; Floeter et al., 2008; Pinheiro et al., 2018). For corals, we see the opposite pattern, in which the coral populations of the endemic brain coral Mussismilia hispida have higher genetic diversity at oceanic sites compared tocoastal populations (Peluso et al., 2018). The influence of distance from the coast on larval flow and population connectivity can limit the survival and dispersal capacity of coral larvae (Nunes et al., 2011; Peluso et al., 2018). This mechanism could also explain the unexpected clustering of coral assemblages from Trindade Island (20°S) as distinct from the Victoria-Trindade Seamount Chain (20°S), in contrast with previous studies on reef fish assemblages that found no significant differences between seamounts and islands (Pinheiro et al., 2015). However, fishes have higher dispersal capacity and mobility when compared to corals, a factor that likely contributes to the higher connectivity of fishes between Trindade Island and the Victoria-Trindade Seamount Chain. A possible explanation for differences in coral assemblages between Trindade Island and Victoria-Trindade Seamount Chain is that sea level changes during the Pleistocene (~130 m bellow current sea level during Last Glacial Maximum) could have isolated the sea-mount chain and islands changing the dispersion dynamics long enough to result in different composition of coral assemblages (Pinheiro et al., 2017; Menezes et al., 2020).
Compared to the two centers of diversity (R4 and R6, ~4°S to ~20°S) in the SWA, the southern region (R8, ~24°S to ~27°S) has high taxonomic beta diversity and is highly nested, indicating species loss through dispersal limitation or environmental filtering. The long-distance separating the centers of diversity regions (R4 and R6) and the southern region (R8) hampers the survival and therefore arrival of coral larvae. Even though some coral species can survive and reach distant reefs using closer reefs as steppingstones (Peluso et al., 2018), it does not imply that they can survive in colder waters and settle in such habitat. Differences in coral assemblages might be more related to the limited ability of species in establishing a viable population after dispersal instead of dispersal limitation itself, as seen in the case of the coral assemblages of Lord Howe Island (subtropical Australia) compared to the Great Barrier Reef (Australia’s diversity center) (Keith et al., 2015). In addition, the southern region comprises rocky reefs dominated by turf algae and macroalgae (Aued et al., 2018), which could impose biological resistance to coral settlement (Kuffner et al., 2006; Birrell et al., 2008; Webster et al., 2015). The lower light incidence and photosynthetic available radiation (PAR) of these southernmost reefs have possibly favored larger corallite size and a more heterotrophic feeding mode in coral species (Lesser et al., 2010). Also, increased distance from the center of diversity could have caused the predominance of hermaphroditism among the local coral fauna (Ghiselin, 1969). Environmental harshness has certainly influenced the low taxonomic and functional diversity within this region, limiting habitat-forming and higher-growth-rate coral species (e.g., the Millepora genera), and other dominant reef builders of SWA reefs (e.g. Siderastrea stellata and Montastraea cavernosa, respectively). Nevertheless, the endemic brain coral Mussismilia hispida and the widespread Madracis decactis thrive, sometimes even in high abundances, in these cold and isolated reefs (Capel et al., 2012; Grillo et al., 2021). Southern coral assemblages share traits that were probably shaped by the interaction of colder waters, lower light incidence and biological resistance to larval settling.
Compared to the global coral fauna, the SWA has lower species richness and functional diversity, therefore being less redundant and potentially more vulnerable to disturbances (McWilliam et al., 2018). Nevertheless, SWA corals have thriven over time as sediment-tolerant species (Loiola et al., 2019) with historically fewer bleaching events and lower mortality (Mies et al., 2020), except for the sensitive ramified and phaceloid species Millepora alcicornis and Mussismilia harttii (Duarte et al., 2020). Overall, coral assemblages in the SWA exhibit both high redundancy (indicated by the low functional originality values)) and vulnerability due to a limited number of species concentrated in smaller groups at the extremes of trait space (indicated by the high functional dispersion). When evaluating functional originality among the regions, there is almost a latitudinal gradient with higher values (lower redundancy) concentrated in assemblages/regions northward (R1, R2, R3) and lower values in regions further south (R7, R8; greater redundancy). Low functional redundancy regions are also those of high species richness, despite the positive correlation between species richness and functional diversity, suggesting there are different levels of vulnerability in such coral assemblages. Such high richness and low redundancy values differ from the positive species richness-functional redundancy relationship usually expected for reef assemblages (Mouillot et al., 2014; Yeager et al., 2017). In the SWA, southern assemblages are structured by strong environmental filters, which selected coral species with more similar traits and therefore redundant in trait space (low originality values). In the north, more amenable environmental conditions have enabled the establishment of coral species with additional trait values, increasing local richness and originality (distances in trait space). Finally, the predominance of the nestedness component in functional beta diversity indicates that poorer coral assemblages of the SWA are subsets of richer ones, as that of the Abrolhos Bank or the Northeastern coast of Brazil.
Our original expectation was that the Abrolhos Bank would harbor the richest coral assemblage in SWA, both taxonomically and functionally, and that the richness of adjacent coral assemblages would decline with increasing distance from Abrolhos, both north and southwards. This expectation was based on the role of Abrolhos as a refugium during the last minimum glacial period (Menezes et al., 2020) and the wide continental platform that, combined, could be a diversity center to colonize adjacent areas. In this case, nestedness would be the major driver of coral beta diversity among regions. Our results confirmed that Abrolhos is indeed a diversity center but also indicates the northeastern portion of Brazil (R4) as a secondary diversity center. This secondary diversity center would explain higher turnover between the Abrolhos region (R6) and the northern regions (e.g. R2, R3), suggesting it also had a pivotal role in historically maintaining coral diversity. Differences in the assemblages between these regions also reflected on functional diversity, with northern assemblages being less redundant even comprising a lower richness. In contrast, differences between the Abrolhos and the southern assemblages (e.g R7, R8) were driven by nestedness, generating higher functional redundancy even with few species, corroborating our initial expectation of impoverishment with increasing distance. These patterns are also related to different environmental filters. While in R7 and R8, low temperatures, high turbidity, a predominantly rocky reef framework, and isolation may be driving the impoverishment of coral assemblages as distance increases from Abrolhos, diversity patterns between R1 and R4 seem more related to riverine inputs, salinity, and turbidity. The interplay between these two diversity centers (R4 and R6) and environmental filters acting on dispersal, recruitment and establishment of coral populations prompted this scenario of heterogenous coral assemblages in Brazil, despite its low species richness.
Understanding coral taxonomic and functional diversity patterns, and how it interacts with biogeographical barriers and environmental filters is fundamental to predict future outcomes of coral distribution and inform conservation actions. Under warming scenarios, the probability of coral occurrence tends to increase towards southern regions (R7-R8) (Bleuel et al., 2021). Coral species such as Mussismilia hispida and Madracis decactis that already predominate in this region (Capel et al., 2012; Grillo et al., 2021) may have traits that facilitate their survival under severe conditions, such as greater dependence on heterotrophy or symbiont photosynthetic efficiency. This may partially explain the high resistance and resilience of Mu. hispida to coral bleaching through heterotrophic compensation leading to low post-bleaching mortality (Mies et al., 2018; Banha et al., 2020). Trait composition can be strongly consistent across geographic scales and be highly influenced by environmental conditions (McLean et al., 2021). The currently dominant traits in the southern region (large corallite size, depth limit) can provide insights on species with potential to expand their distributions towards this region (e.g., Mussismilia harttii and Montastraea cavernosa). Further studies could investigate traits that might be more affected by ocean warming and acidification, including those related to reproduction and growth that are important for calcium carbonate accretion, reef structure and habitat provisioning. In addition, investigating traits that can help corals cope with climate change, such as heterotrophic feeding (nourishment and compensation during coral bleaching) and Symbiodiniaceae diversity of clades (symbiont shuffling and thermal tolerance) is also important to understand species resistance and resilience to such stressors. Here we documented a diverse, variable, highly endemic coral fauna with high functional redundancy. This information can bring critical insights on the future of SWA and more diverse reef ecosystems.
Data availability statement
The original contributions presented in the study are included in the article/Supplementary Material. Further inquiries can be directed to the corresponding authors.
Ethics statement
The manuscript presents research on animals that do not require ethical approval for their study.
Author contributions
JB: Conceptualization, Data curation, Formal analysis, Investigation, Methodology, Visualization, Writing – original draft, Writing – review & editing. LW: Data curation, Formal analysis, Methodology, Visualization, Writing – review & editing. MB: Data curation, Formal analysis, Funding acquisition, Methodology, Resources, Visualization, Writing – review & editing. GL: Conceptualization, Funding acquisition, Investigation, Project administration, Resources, Supervision, Writing – review & editing.
Funding
The author(s) declare financial support was received for the research, authorship, and/or publication of this article. This study was supported by Serrapilheira Institute (Grant No. Serra-1708-15364) awarded to GL. This study was financed in part by the Coordenação de Aperfeiçoamento de Pessoal de Nível Superior - Brasil (CAPES) - Finance Code 001 through PhD scholarships to JB and LW. This work is also part of the ReefSYN Working Group, within the Synthesis on Biodiversity and Ecosystem Services Program (SinBiose) supported by CNPq (442417/2019–5) awarded to MB. GL is also grateful to a research productivity scholarship provided by the Brazilian National Council for Scientific and Technological Development (CNPq; numbers 310517/2019-2; 308072/2022-7).
Acknowledgments
We are thankful to Juliana Dias and Amana Garrido for revising earlier versions of this manuscript.
Conflict of interest
The authors declare that the research was conducted in the absence of any commercial or financial relationships that could be construed as a potential conflict of interest.
The author(s) declared that they were an editorial board member of Frontiers, at the time of submission. This had no impact on the peer review process and the final decision.
Publisher’s note
All claims expressed in this article are solely those of the authors and do not necessarily represent those of their affiliated organizations, or those of the publisher, the editors and the reviewers. Any product that may be evaluated in this article, or claim that may be made by its manufacturer, is not guaranteed or endorsed by the publisher.
Supplementary material
The Supplementary Material for this article can be found online at: https://www.frontiersin.org/articles/10.3389/fevo.2024.1322751/full#supplementary-material
References
Álvarez-Noriega M., Baird A. H., Dornelas M., Madin J. S., Cumbo V. R., Connolly S. R. (2016). Fecundity and the demographic strategies of coral morphologies. Ecology 97, 3485–3493. doi: 10.1002/ecy.1588
Amado-Filho G. M., Pereira-Filho G. H., Bahia R. G., Abrantes D. P., Veras P. C., Matheus Z. (2012). Occurrence and distribution of rhodolith beds on the Fernando de Noronha Archipelago of Brazil. Aquat. Bot. 101, 41–45. doi: 10.1016/j.aquabot.2012.03.016
Amaral F. M., Steiner A. Q., Broadhurst M. K., Cairns S. D. (2008). An overview of the shallow-water calcified hydroids from Brazil (Hydrozoa: Cnidaria), including the description of a new species. Zootaxa. 1930, 56–68. doi: 10.11646/ZOOTAXA.1930.1.4
Assis J., Tyberghein L., Bosch S., Verbruggen H., Serrão E. A., De Clerck O. (2018). Bio-ORACLE v2.0: Extending marine data layers for bioclimatic modelling. Global Ecol. Biogeography 27, 277–284. doi: 10.1111/geb.12693
Aued A. W., Smith F., Quimbayo J. P., Cândido D. V., Longo G. O., Ferreira C. E. L., et al. (2018). Large-scale patterns of benthic marine communities in the Brazilian province. PloS One 13 (6), e0198452. doi: 10.1371/journal.pone.0198452
Baird A. H., Guest J. R., Willis B. L. (2009). Systematic and biogeographical patterns in the reproductive biology of scleractinian corals. Annu. Rev. Ecol. Evol. Syst. 40, 551–571. doi: 10.1146/annurev.ecolsys.110308.120220
Banha T. N. S., Capel K. C. C., Kitahara M. V., Francini-Filho R. B., Francini C. L. B., Sumida P. Y. G., et al. (2020). Low coral mortality during the most intense bleaching event ever recorded in subtropical Southwestern Atlantic reefs. Coral Reefs 39, 515–521. doi: 10.1007/s00338-019-01856-y
Barroso H. D. S., Lima I. D. O., Bezerra A. D. A., Garcia T. M., Tavares T. C. L., Alves R. S., et al. (2022). Distribution of nutrients and chlorophyll across an equatorial reef region: Insights on coastal gradients. Ocean Coast. Res. 71, e23002. doi: 10.1590/2675-2824071.22016hdsb
Baselga A. (2017). Partitioning abundance-based multiple-site dissimilarity into components: balanced variation in abundance and abundance gradients. Methods Ecol. Evol. 8, 799–808. doi: 10.1111/2041-210X.12693
Bender M. G., Pie M. R., Rezende E. L., Mouillot D., Floeter S. R. (2013). Biogeographic, historical and environmental influences on the taxonomic and functional structure of Atlantic reef fish assemblages. Global Ecol. Biogeography 22, 1173–1182. doi: 10.1111/geb.12099
Birrell C. L., McCook L. J., Willis B. L., Harrington L. (2008). Chemical effects of macroalgae on larval settlement of the broadcast spawning coral Acropora millepora. Mar. Ecol. Prog. Ser. 362, 129–137. doi: 10.3354/meps07524
Bleuel J., Pennino M. G., Longo G. O. (2021). Coral distribution and bleaching vulnerability areas in Southwestern Atlantic under ocean warming. Sci. Rep. 11, 12833. doi: 10.1038/s41598-021-92202-2
Bongaerts P., Ridgway T., Sampayo E. M., Hoegh-Guldberg O. (2010). Assessing the “deep reef refugia” hypothesis: Focus on Caribbean reefs. Coral Reefs 29, 309–327. doi: 10.1007/s00338-009-0581-x
Bouillon S., Connolly R. M. (2009). Carbon Exchange Among Tropical Coastal Ecosystems. In: Nagelkerken I. (eds) Ecological Connectivity among Tropical Coastal Ecosystems. Springer, Dordrecht. doi: 10.1007/978-90-481-2406-0_3
Bourmaud C. A. F., Leung J. K. L., Bollard S., Gravier-Bonnet N. (2013). Mass spawning events, seasonality and reproductive features in Milleporids (Cnidaria, Hydrozoa) from Reunion Island. Mar. Ecol. 34, 14–24. doi: 10.1111/maec.12024
Braga E. S., Chiozzini V. C., Berbel G. B. B., Maluf J. C. C., Aguiar V. M. C., Charo M., et al. (2008). Nutrient distributions over the Southwestern South Atlantic continental shelf from Mar del Plata (Argentina) to Itajaí (Brazil): Winter-summer aspects. Cont Shelf Res. 28, 1649–1661. doi: 10.1016/j.csr.2007.06.018
Braga E. D. S., Müller T. J. (1998). Observation of regeneration of nitrate, phosphate and silicate during upwelling off Ubatuba, Brazil, 23 S. Continental Shelf Research 18 (8), 915–922. doi: 10.1016/S0278-4343(98)00002-8
Bruce T., Meirelles P. M., Garcia G., Paranhos R., Rezende C. E., de Moura R. L., et al. (2012). Abrolhos bank reef health evaluated by means of water quality, microbial diversity, benthic cover, and fish biomass data. PloS One 7. doi: 10.1371/journal.pone.0036687
Caparelli A. (2004). Esforço Reprodutivo de Mussismilia Braziliensis (Verrill 1868) (Cnidaria, Scleractinia, Mussidae) no Complexo Recifal dos Abrolhos, BA, Brasil. (Rio de Janeiro: UFRJ/ MN), 2004 xvi, 53pp.
Capel K. C. C., Segal B., Bertuol P., Lindner A. (2012). Corallith beds at the edge of the tropical South Atlantic. Coral Reefs 31, 75. doi: 10.1007/s00338-011-0818-3
Castro C. B., Pires D. O. (2001). Brazilian coral reefs: what we already know and what is still missing. Bull. Mar. Sci. 69, 357–371.
Coelho-Souza S. A., López M. S., Guimarães J. R. D., Coutinho R., Candella R. N. (2012). Biophysical interactions in the Cabo Frio upwelling system, Southeastern Brazil. Braz. J. Oceanography 60, 353–365. doi: 10.1590/S1679-87592012000300008
Coles S. L., Jokiel P. L. (1992). “Effects of salinity on coral reefs” in Connell DW, , Hawker DW (ed) Pollution in tropical aquatic systems. CRC Press, Boca Raton, FL, p 147–166.
Conaco C., Cabaitan P. C. (2020). Influence of salinity and temperature on the survival and settlement of Heliopora coerulea larvae. Mar. pollut. Bull. 150. doi: 10.1016/j.marpolbul.2019.110703
Coni E. O. C., Ferreira C. M., Meirelles P. M., Menezes R., Santana E. F. C., Moreira A. P. B., et al. (2017). Modeling abundance, growth, and health of the solitary coral Scolymia wellsi (Mussidae) in turbid SW Atlantic coral reefs. Mar. Biol. 164. doi: 10.1007/s00227-017-3090-4
Connell J. H. (1978). Diversity in tropical rain forests and coral reefs: high diversity of trees and corals is maintained only in a nonequilibrium state. Science 199 (4335), 1302–1310. doi: 10.1126/science.199.4335.13
Cord I., Nunes L. T., Barroso C. X., Freire A. S., Gadig O. B. F., Gomes P. B., et al. (2022). Brazilian marine biogeography: a multi-taxa approach for outlining sectorization. Mar. Biol. 169. doi: 10.1007/s00227-022-04045-8
Cordeiro C. A. M. M., Quimbayo J. P., Nunes J. A. C. C., Nunes L. T., Sissini M. N., Sampaio C. L. S., et al. (2021). Conservation status of the southernmost reef of the Amazon Reef System: The Parcel de Manuel Luís. Coral Reefs 40, 165–185. doi: 10.1007/s00338-020-02026-1
Cowman P. F., Bellwood D. R. (2013). The historical biogeography of coral reef fishes: Global patterns of origination and dispersal. J. Biogeogr 40, 209–224. doi: 10.1111/jbi.12003
de Barros M. M. L., de Oliveira Pires D. (2003). Sexual reproduction of the Brazilian reef coral Siderastrea stellata Verrill 1868 (Anthozoa, Scleractinia). Bull. Mar. Sci. 73, 713–724.
de Souza J. N., Nunes F. L. D., Zilberberg C., Sanchez J. A., Migotto A. E., Hoeksema B. W., et al. (2017). Contrasting patterns of connectivity among endemic and widespread fire coral species (Millepora spp.) in the tropical Southwestern Atlantic. Coral Reefs 36, 701–716. doi: 10.1007/s00338-017-1562-0
Diaz-Pulido G., Harii S., McCook L. J., Hoegh-Guldberg O. (2010). The impact of benthic algae on the settlement of a reef-building coral. Coral Reefs 29, 203–208. doi: 10.1007/s00338-009-0573-x
Duarte G. A. S., Villela H. D. M., Deocleciano M., Silva D., Barno A., Cardoso P. M., et al. (2020). Heat waves are a major threat to turbid coral reefs in Brazil. Front. Mar. Sci. 7. doi: 10.3389/fmars.2020.00179
Fabricius K., De’ath G. (2001). Environmental factors associated with the spatial distribution of crustose coralline algae on the Great Barrier Reef. Coral Reefs 19, 303–309. doi: 10.1007/s003380000120
Fadlallah Y. H. (1983). Sexual reproduction, development and larval biology in scleractinian corals: a review. Coral reefs 2, 129–150. doi: 10.1007/BF00336720
Floeter S. R., Rocha L. A., Robertson D. R., Joyeux J. C., Smith-Vaniz W. F., Wirtz P., et al. (2008). Atlantic reef fish biogeography and evolution. J. Biogeogr 35, 22–47. doi: 10.1111/j.1365-2699.2007.01790.x
Francini-Filho R. B., Asp N. E., Siegle E., Hocevar J., Lowyck K., D’Avila N., et al. (2018). Perspectives on the Great Amazon Reef: Extension, biodiversity, and threats. Front. Mar. Sci. 5. doi: 10.3389/fmars.2018.00142
Freire A. S., Teschima M. M., Brandão M. C., Iwasa-Arai T., Sobral F. C., Sasaki D. K., et al. (2021). Does the transport of larvae throughout the south Atlantic support the genetic and morphometric diversity of the Sally Lightfoot Crabs Grapsus grapsus (Linnaeus 1758) and grapsus adscensionis (Osbeck 1765) (Decapoda: Grapsidae) among the oceanic islands? J. Mar. Syst. 223. doi: 10.1016/j.jmarsys.2021.103614
Gaston K. J. (2000). Global patterns in biodiversity. Nature 405 (6783), 220–227. doi: 10.1038/35012228
Ghiselin M. T. (1969). The evolution of hermaphroditism among animals. Q. Rev. Biol. 44, 189–208. doi: 10.1086/406066
Gleason D. F., Hofmann D. K. (2011). Coral larvae: From gametes to recruits. J. Exp. Mar. Biol. Ecol. 408, 42–57. doi: 10.1016/j.jembe.2011.07.025
Golbuu Y., Fabricius K., Victor S., Richmond R. H. (2008). Gradients in coral reef communities exposed to muddy river discharge in Pohnpei, Micronesia. Estuar. Coast. Shelf Sci. 76, 14–20. doi: 10.1016/j.ecss.2007.06.005
Golbuu Y., van Woesik R., Richmond R. H., Harrison P., Fabricius K. E. (2011). River discharge reduces reef coral diversity in Palau. Mar. pollut. Bull. 62, 824–831. doi: 10.1016/j.marpolbul.2010.12.015
Gower J. C., Legendre P. (1986). Metric and Euclidean properties of dissimilarity coefficients. J. classification 3, 5–48. doi: 10.1007/BF01896809
Graham N. A. J., Nash K. L. (2013). The importance of structural complexity in coral reef ecosystems. Coral Reefs 32, 315–326. doi: 10.1007/s00338-012-0984-y
Grillo A. C., Candido C. F., Giglio V. J., Longo G. O. (2021). Unusual high coral cover in a Southwestern Atlantic subtropical reef. Mar. Biodiversity 51. doi: 10.1007/s12526-021-01221-9
Habel K., Grasman R., Gramacy R., Mozharovskyi P., Sterratt D. (2023). “_geometry: Mesh generation and surface tessellation_,” in R package version 0.4.7. Available at: https://CRAN.R-project.org/package=geometry.
Han A., Dai M., Kao S. J., Gan J., Li Q., Wang L., et al. (2012). Nutrient dynamics and biological consumption in a large continental shelf system under the influence of both a river plume and coastal upwelling. Limnol Oceanogr 57, 486–502. doi: 10.4319/lo.2012.57.2.0486
Hata T., Madin J. S., Cumbo V. R., Denny M., Figueiredo J., Harii S., et al. (2017). Coral larvae are poor swimmers and require fine-scale reef structure to settle. Sci. Rep. 7. doi: 10.1038/s41598-017-02402-y
Houlbrèque F., Ferrier-Pagès C. (2009). Heterotrophy in tropical scleractinian corals. Biol. Rev. 84, 1–17. doi: 10.1111/j.1469-185X.2008.00058.x
Hubbard D. K., Scaturo D. (1985). Growth rates of seven species of scleractinean corals from Cane Bay and Salt River, St. Croix, USVI. Bull. Mar. Sci. 36, 325–338.
Kahng S. E., Akkaynak D., Shlesinger T., Hochberg E. J., Wiedenmann J., Tamir R., et al. (2019). “Light, Temperature, Photosynthesis, Heterotrophy, and the Lower Depth Limits of Mesophotic Coral Ecosystems” in Mesophotic Coral Ecosystems, eds. Loya Y., Puglise K., Bridge T. Coral Reefs of the World. Springer, Cham. 12, 801–828. doi: 10.1007/978-3-319-92735-0_42
Kahng S., Copus J. M., Wagner D. (2016). “Mesophotic coral ecosystems,” in Marine Animal Forests (Springer International Publishing), 1–22. doi: 10.1007/978-3-319-17001-5_4-1
Keith S. A., Woolsey E. S., Madin J. S., Byrne M., Baird A. H. (2015). Differential establishment potential of species predicts a shift in coral assemblage structure across a biogeographic barrier. Ecography 38, 1225–1234. doi: 10.1111/ecog.01437
Kikuchi R. K. P., Leão Z. M. A. N. (1997). “Rocas (Southwestern equatorial atlantic, brazil): an atoll built primarily by coralline algae,” in Proc. 8th. int. coral reef symposium, vol. 1, 736 p.
Kikuchi R. K. P., Oliveira M. D. M., Leão Z. M. A. N. (2013). Density banding pattern of the south western Atlantic coral Mussismilia Braziliensis. J. Exp. Mar. Biol. Ecol. 449, 207–214. doi: 10.1016/j.jembe.2013.09.019
Kleypas J. A., Mcmanusi J. W., B Meñdez L. A. (1999). Environmental Limits to Coral Reef Development: Where Do We Draw the Line? American Zoologist. 39(1), 146–159. doi: 10.1093/icb/39.1.146
Kuffner I. B., Walters L. J., Becerro M. A., Paul V. J., Ritson-Williams R., Beach K. S. (2006). Inhibition of coral recruitment by macroalgae and cyanobacteria. Mar. Ecol. Prog. Ser. 323, 107–117. doi: 10.3354/meps323107
Kumar S. P., Narvekar J., Nuncio M., Kumar A., Ramaiah N., Sardesai S., et al. (2010). Is the biological productivity in the bay of bengal light limited? Curr. Sci. 98, 1331–1339.
Lavorel S., Garnier E. (2002). Predicting changes in community composition and ecosystem functioning from plant traits: revisiting the holy grail. Funct. Ecol. 16 (5), 545–556. doi: 10.1046/j.1365-2435.2002.00664.x
Leão Z. M. A. N., Kikuchi R. K. P., Ferreira B. P., Neves E. G., Sovierzoski H. H., Oliveira M. D. M., et al. (2016). Brazilian coral reefs in a period of global change: A synthesis. Braz. J. Oceanogr 64, 97–116. doi: 10.1590/S1679-875920160916064sp2
Leão Z. M., Kikuchi R. K., Testa V. (2003). “Corals and coral reefs of Brazil,” in Latin American coral reefs (Elsevier Science B.V.), 9–52. doi: 10.1016/B978-044451388-5/50003-5
Legendre P. (2014). Interpreting the replacement and richness difference components of beta diversity. Global Ecol. Biogeography 23, 1324–1334. doi: 10.1111/geb.12207
Lesser M. P., Marc S., Michael S., Michiko O., Gates R. D., Andrea G. (2010). Photoacclimatization by the coral Montastraea cavernosa in the mesophotic zone: Light, food, and genetics. Ecology 91, 990–1003. doi: 10.1890/09-0313.1
Lewis J. B. (2006). Biology and ecology of the hydrocoral millepora on coral reefs. Adv. Mar. Biol. 50, 1–55. doi: 10.1016/S0065-2881(05)50001-4
Liaño-Carrera F., Camarena-Luhrs T., Gómez-Barrero A., Martos-Fernández F. J., Ramírez-Macias J. I., Salas-Monreal D. (2019). New coral reef structures in a tropical coral reef system. Lat Am. J. Aquat Res. 47, 270–281. doi: 10.3856/vol47-issue2-fulltext-7
Lima L. F. O., Coutinho R. (2016). The reef coral Siderastrea stellata thriving at its range limit: population structure in Arraial do Cabo, southeastern Brazil. Bull. Mar. Sci. 92, 107–121. doi: 10.5343/bms.2015.1029
Loiola M., Oliveira M. D. M., Kikuchi R. K. P. (2013). Tolerance of Brazilian brain coral Mussismilia Braziliensis to sediment and organic matter inputs. Mar. pollut. Bull. 77, 55–62. doi: 10.1016/j.marpolbul.2013.10.033
Loiola M., Cruz I. C., Lisboa D. S., Mariano-Neto E., Leão Z. M., et al (2019). Structure of marginal coral reef assemblages under different turbidity regime. Marine environmental research. 147, 138–148. doi: 10.1016/j.marenvres.2019.03.013
Longo G. O., Morais R. A., Martins C. D. L., Mendes T. C., Aued A. W., Cândido D. V., et al. (2015). Between-habitat variation of benthic cover, reef fish assemblage and feeding pressure on the benthos at the only atoll in south atlantic: Rocas atoll, NE brazil. PloS One 10 (6), e0127176. doi: 10.1371/journal.pone.0127176
Lueg J. R., Moulding A. L., Kosmynin V. N., Gilliam D. S. (2012). Gametogenesis and Spawning of Solenastrea bournoni and Stephanocoenia intersepta in Southeast Florida, USA. J. Mar. Biol. 2012, 1–13. doi: 10.1155/2012/370247
Luza A. L., Quimbayo J. P., Ferreira C. E. L., Floeter S. R., Francini-Filho R. B., Bender M. G., et al. (2022). Low functional vulnerability of fish assemblages to coral loss in Southwestern Atlantic marginal reefs. Sci. Rep. 12. doi: 10.1038/s41598-022-20919-9
Madin J. S., Hoogenboom M. O., Connolly S. R., Darling E. S., Falster D. S., Huang D., et al. (2016). A trait-based approach to advance coral reef science. Trends Ecol. Evol. 31, 419–428. doi: 10.1016/j.tree.2016.02.012
Maire E., Grenouillet G., Brosse S., Villéger S. (2015). How many dimensions are needed to accurately assess functional diversity? A pragmatic approach for assessing the quality of functional spaces. Global Ecol. Biogeography 24, 728–740. doi: 10.1111/geb.12299
Magalhães G. M., Amado-Filho G. M., Rosa M. R., de Moura R. L., Brasileiro P. S., De Moraes F. C., et al. (2015). Changes in benthic communities along a 0–60 m depth gradient in the remote st. peter and st. paul archipelago (Mid-atlantic ridge, brazil). Bull. Mar. Sci. 91 (3), 377–396. doi: 10.5343/bms.2014.1044
Maida M., Ferreira B. P. (1997). “Coral reefs of brazil: an overview,” in Proc 8th int coral reef sym, vol. 1, 263–274.
Mason N. W., Mouillot D., Lee W. G., Wilson J. B. (2005). Functional richness, functional evenness and functional divergence: the primary components of functional diversity. Oikos 111 (1), 112–118. doi: 10.1111/j.0030-1299.2005.13886.x
Matheus Z., Francini-Filho R. B., Pereira-Filho G. H., Moraes F. C., Moura R. L. D., Brasileiro P. S., et al. (2019). Benthic reef assemblages of the Fernando de Noronha Archipelago, tropical South-west Atlantic: Effects of depth, wave exposure and cross-shelf positioning. PloS One 14, e0210664. doi: 10.1371/journal.pone.0210664
Mazzei E. F., Bertoncini A. A., Pinheiro H. T., MaChado L. F., Vilar C. C., Guabiroba H. C., et al. (2017). Newly discovered reefs in the southern Abrolhos Bank, Brazil: Anthropogenic impacts and urgent conservation needs. Mar. pollut. Bull. 114, 123–133. doi: 10.1016/j.marpolbul.2016.08.059
McClanahan T. R., Ateweberhan M., Darling E. S., Graham N. A. J., Muthiga N. A. (2014). Biogeography and change among regional coral communities across the western Indian Ocean. PloS One 9. doi: 10.1371/journal.pone.0093385
McLean M., Stuart-Smith R., Villéger S., Auber A., Edgar G., MacNeil M. A., et al. (2021). Trait similarity in reef fish faunas across the world’s oceans. Proceedings of the National Academy of Sciences. 118 (12), 2012318118. doi: 10.1073/pnas.2012318118/-/DCSupplemental
McWilliam M., Hoogenboom M. O., Baird A. H., Kuo C. Y., Madin J. S., Hughes T. P. (2018). Biogeographical disparity in the functional diversity and redundancy of corals. Proc. Natl. Acad. Sci. U.S.A. 115, 3084–3089. doi: 10.1073/pnas.1716643115
Meirelles P. M., Amado-Filho G. M., Pereira-Filho G. H., Pinheiro H. T., De Moura R. L., Joyeux J. C., et al. (2015). Baseline assessment of mesophotic reefs of the Vitória-Trindade Seamount Chain based on water quality, microbial diversity, benthic cover and fish biomass data. PloS One 10. doi: 10.1371/journal.pone.0130084
Menezes N., Sobral-Souza T., Silva M., Solferini V. N. (2020). Paleoclimatic distribution and phylogeography of Mussismilia Braziliensis (Anthozoa, Scleractinia), an endemic Brazilian reef coral. Mar. Biodiversity 50. doi: 10.1007/s12526-020-01063-x
Mies M., Francini-Filho R. B., Zilberberg C., Garrido A. G., Longo G. O., Laurentino E., et al. (2020). South atlantic coral reefs are major global warming refugia and less susceptible to bleaching. Front. Mar. Sci. 7. doi: 10.3389/fmars.2020.00514
Mies M., Güth A. Z., Castro C. B., Pires D. O., Calderon E. N., Pompeu M., et al. (2018). Bleaching in reef invertebrate larvae associated with Symbiodinium strains within clades A–F. Mar. Biol. 165. doi: 10.1007/s00227-017-3263-1
Mouillot D., Graham N. A. J., Villéger S., Mason N. W. H., Bellwood D. R. (2013). A functional approach reveals community responses to disturbances. Trends Ecol. Evol. 28, 167–177. doi: 10.1016/j.tree.2012.10.004
Mouillot D., Villéger S., Parravicini V., Kulbicki M., Arias-González J. E., Bender M., et al. (2014). Functional over-redundancy and high functional vulnerability in global fish faunas on tropical reefs. Proceedings of the National Academy of Sciences. 111(38), 13757–13762. doi: 10.1073/pnas.1317625111
Moura R. L., Amado-Filho G. M., Moraes F. C., Brasileiro P. S., Salomon P. S., Mahiques M. M., et al. (2016). An extensive reef system at the Amazon River mouth. Sci. Adv. 2. doi: 10.1126/sciadv.1501252
Muscatine L., Porter J. W., Kaplan I. R. (1989). Resource partitioning by reef corals as determined from stable isotope composition: I. δ 13 C of zooxanthellae and animal tissue vs depth. Mar. Biol. 100, 185–193. doi: 10.1007/BF00391957
Neal B. P., Khen A., Treibitz T., Beijbom O., O’Connor G., Coffroth M. A., et al. (2017). Caribbean massive corals not recovering from repeated thermal stress events during 2005–2013. Ecol. Evol. 7, 1339–1353. doi: 10.1002/ece3.2706
Nogueira M. M., Neves E., Johnsson R. (2015). Effects of habitat structure on the epifaunal community in Mussismilia corals: does coral morphology influence the richness and abundance of associated crustacean fauna? Helgol Mar. Res. 69, 221–229. doi: 10.1007/s10152-015-0431-x
Nunes F., Norris R. D., Knowlton N. (2009). Implications of isolation and low genetic diversity in peripheral populations of an amphi-Atlantic coral. Mol. Ecol. 18, 4283–4297. doi: 10.1111/j.1365-294X.2009.04347.x
Nunes F. L. D., Norris R. D., Knowlton N. (2011). Long distance dispersal and connectivity in Amphi-Atlantic corals at regional and basin scales. PloS One 6. doi: 10.1371/journal.pone.0022298
Obura D. (2012). The diversity and biogeography of western Indian ocean reef-building corals. PloS One 7. doi: 10.1371/journal.pone.0045013
Oigman-Pszczol S. S., Creed J. C. (2004). Size structure and spatial distribution of the corals mussismilia hispida and siderastrea stellata (Scleractinia) at armação dos búzios, brazil. Bull. Mar. Sci. 74 (2), 433–448.
Oksanen J., Blanchet F. G., Kindt R., Legendre P., Minchin P. R., O hara R. B., et al (2013). Community ecology package. R package version. 2(0), 321–326. Available online at: http://cc.oulu.fi/˜jarioksa/.
Oliveira D. R. P., Cordeiro L. G. M. S., Carreira R. S. (2013). Characterization of organic matter in cross-margin sediment transects of an upwelling region in the Campos Basin (SW Atlantic, Brazil) using lipid biomarkers. Biogeochemistry 112, 311–327. doi: 10.1007/s10533-012-9726-z
Palma E. D., Matano R. P. (2009). Disentangling the upwelling mechanisms of the South Brazil Bight. Cont Shelf Res. 29, 1525–1534. doi: 10.1016/j.csr.2009.04.002
Pansini M., Longo C. (2003). A review of the Mediterranean Sea sponge biogeography with, in appendix, a list of the demosponges hitherto recorded from this sea. Biogeographia 24. doi: 10.21426/B6110107
Peluso L., Tascheri V., Nunes F. L. D., Castro C. B., Pires D. O., Zilberberg C. (2018). Contemporary and historical oceanographic processes explain genetic connectivity in a Southwestern Atlantic coral. Sci. Rep. 8. doi: 10.1038/s41598-018-21010-y
Pereira-Filho G. H., Amado-Filho G. M., Guimarães S. M., Moura R. L., Sumida P. Y., Abrantes D. P., et al. (2011). Reef fish and benthic assemblages of the Trindade and Martin Vaz island group, southwestern Atlantic. Brazilian Journal of Oceanography. 59, 201–212.
Pereira-Filho G. H., Shintate G. S., Kitahara M. V., Moura R. L., Amado-Filho G. M., Bahia R. G., et al. (2019). The southernmost Atlantic coral reef is off the subtropical island of Queimada Grande (24 S), Brazil. Bull. Mar. Sci. 95, 277–287. doi: 10.5343/bms.2018.0056
Pereira-Filho G. H., Mendes V. R., Perry C. T., Shintate G. I., Niz W. C., Sawakuchi A. O., et al. (2021). Growing at the limit: Reef growth sensitivity to climate and oceanographic changes in the South Western Atlantic. Global and Planetary Change. 201, 103479. doi: 10.1016/j.gloplacha.2021.103479
Picciani N., de Lossio e Seiblitz I. G., de Paiva P. C., e Castro C. B., Zilberberg C. (2016). Geographic patterns of Symbiodinium diversity associated with the coral Mussismilia hispida (Cnidaria, Scleractinia) correlate with major reef regions in the Southwestern Atlantic Ocean. Mar. Biol. 163. doi: 10.1007/s00227-016-3010-z
Pinheiro H. T., Bernardi G., Simon T., Joyeux J. C., Macieira R. M., Gasparini J. L., et al. (2017). Island biogeography of marine organisms. Nature 549, 82–85. doi: 10.1038/nature23680
Pinheiro H. T., Mazzei E., Moura R. L., Amado-Filho G. M., Carvalho-Filho A., Braga A. C., et al. (2015). Fish biodiversity of the Vitória-Trindade seamount chain, southwestern Atlantic: An updated database. PloS One 10. doi: 10.1371/journal.pone.0118180
Pinheiro H. T., Rocha L. A., Macieira R. M., Carvalho-Filho A., Anderson A. B., Bender M. G., et al. (2018). South-western Atlantic reef fishes: Zoogeographical patterns and ecological drivers reveal a secondary biodiversity centre in the Atlantic Ocean. Divers. Distrib 24, 951–965. doi: 10.1111/ddi.12729
Pinzón C. J. H., Weil E. (2011). Cryptic species within the atlanticcaribbean genus meandrina (scleractinia): A multidisciplinary approach and description of the new species meandrina jacksoni. Bull. Mar. Sci. 87, 823–853. doi: 10.5343/bms.2010.1085
Pires D. O., Castro C. B., Ratto C. C. (1999). Reef coral reproduction in the Abrolhos Reef Complex, Brazil: the endemic genus Mussismilia. Mar. Biol. 135, 463–471. doi: 10.1007/s002270050646
Pires D. O., Castro C. B., Ratto C. C. (2000). Reproduction of the solitary coral Scolymia wellsi Laborel (Cnidaria, Scleractinia) from the Abrolhos reef complex, Brazil. In Proceedings of the 9th International Coral Reef Symposium, Bali. 1, 382–384.
Porter J. W. (1976). Autotrophy, heterotrophy, and resource partitioning in Caribbean reef-building corals. Am. Nat. 110, 731–742. doi: 10.1086/283100
Price N. N., Muko S., Legendre L., Steneck R., Van Oppen M. J. H., Albright R., et al. (2019). Global biogeography of coral recruitment: Tropical decline and subtropical increase. Mar. Ecol. Prog. Ser. 621, 1–17. doi: 10.3354/meps12980
R Core Team. (2022). R: A language and environment for statistical computing. (Vienna, Austria: R Foundation for Statistical Computing). Available at: https://www.R-project.org/.
Ritson-Williams R., Arnold S. N., Fogarty N. D., Steneck R. S., Vermeij M. J., Paul V. J. (2009). New perspectives on ecological mechanisms affecting coral recruitment on reefs. Smithsonian Contributions to Mar. Sci. 38, 437. doi: 10.5479/si.01960768.38.437
Rocha L. A. (2003). Patterns of distribution and processes of speciation in Brazilian reef fishes. J. Biogeography 30, 1161–1171. doi: 10.1046/j.1365-2699.2003.00900.x
Rodríguez S., Alvizu A., Tagliafico A., Bastidas C. (2009). Low natural repopulation of marginal coral communities under the influence of upwelling. Hydrobiologia 624, 1–11. doi: 10.1007/s10750-008-9662-z
Santana E. F. C., Mies M., Longo G. O., Menezes R., Aued A. W., Luza A. L., et al. (2023). Turbidity shapes shallow Southwestern Atlantic benthic reef communities. Mar. Environ. Res. 183, 105807. doi: 10.1016/j.marenvres.2022.105807
Silva I. C. B. S., Liparini A., Pereira N. S., Braga B. L. S. S., Sial A. N., Liu S. C., et al. (2019). Assessing the growth rate of the South Atlantic coral species Mussismilia hispida (Verrill 1902) using carbon and oxygen stable isotopes. J. South Am. Earth Sci. 96. doi: 10.1016/j.jsames.2019.102346
Siqueira A. C., Bellwood D. R., Cowman P. F. (2019). Historical biogeography of herbivorous coral reef fishes: The formation of an Atlantic fauna. J. Biogeogr 46, 1611–1624. doi: 10.1111/jbi.13631
Sissini M. N., Koerich G., de Barros-Barreto M. B., Coutinho L. M., Gomes F. P., Oliveira W., et al. (2022). Diversity, distribution, and environmental drivers of coralline red algae: the major reef builders in the Southwestern Atlantic. Coral Reefs 41, 711–725. doi: 10.1007/s00338-021-02171-1
Sneath P. H., Sokal R. R. (1973). Numerical taxonomy (The principles and practice of numerical classification).
Soares M. D. O., Rossi S., Martins F. A. S., Carneiro P. B. D. M. (2017). The forgotten reefs: benthic assemblage coverage on a sandstone reef (Tropical South-western Atlantic). J. Mar. Biol. Assoc. United Kingdom 97, 1585–1592. doi: 10.1017/S0025315416000965
Spalding M., Fox H., Allen G., Davidsin N., Ferdana Z., Finlayson M., et al. (2007). Marine ecoregions of the world: A bioregionalization of coastal and shelf areas. Bioscience 57, 573–583. doi: 10.1641/B570707
Stekhoven D. J., Bühlmann P. (2012). Missforest-Non-parametric missing value imputation for mixed-type data. Bioinformatics 28, 112–118. doi: 10.1093/bioinformatics/btr597
Suzuki R., Shimodaira H. (2006). Pvclust: An R package for assessing the uncertainty in hierarchical clustering. Bioinformatics 22, 1540–1542. doi: 10.1093/bioinformatics/btl117
Szmant A. M. (1986). Reproductive ecology of Caribbean reef corals. Coral reefs 5, 43–53. doi: 10.1007/BF00302170
Szmant A. (1991). Sexual reproduction by the Caribbean reef corals Montastrea annularis and M. cavernosa. Mar. Ecol. Prog. Ser. 75, 13–25. doi: 10.3354/meps075013
Tamir R., Eyal G., Kramer N., Laverick J. H., Loya Y. (2019). Light environment drives the shallow-to-mesophotic coral community transition. Ecosphere 10. doi: 10.1002/ecs2.2839
Targino A. K. G., Gomes P. B. (2020). Distribution of sea anemones in the Southwest Atlantic: biogeographical patterns and environmental drivers. Mar. Biodiversity 50. doi: 10.1007/s12526-020-01099-z
Tebben J., Motti C. A., Siboni N., Tapiolas D. M., Negri A. P., Schupp P. J., et al. (2015). Chemical mediation of coral larval settlement by crustose coralline algae. Sci. Rep. 5. doi: 10.1038/srep10803
Teschima M. M., Ströher P. R., Firkowski C. R., Pie M. R., Freire A. S. (2016). Large-scale connectivity of Grapsus grapsus (Decapoda) in the Southwestern Atlantic oceanic islands: integrating genetic and morphometric data. Mar. Ecol. 37, 1360–1372. doi: 10.1111/maec.12347
Teschima M. M., Zilberberg C., Nunes F. L. D. (2022). Strong genetic differentiation demarks populations of Favia across biogeographic regions of the Atlantic Ocean. Coral Reefs 41, 523–534. doi: 10.1007/s00338-021-02203-w
Thomas L., López E. H., Morikawa M. K., Palumbi S. R. (2019). Transcriptomic resilience, symbiont shuffling, and vulnerability to recurrent bleaching in reef-building corals. Mol. Ecol. 28, 3371–3382. doi: 10.1111/mec.15143
Thompson D. M., Kleypas J., Castruccio F., Curchitser E. N., Pinsky M. L., Jönsson B., et al. (2018). Variability in oceanographic barriers to coral larval dispersal: Do currents shape biodiversity? Prog. Oceanogr 165, 110–122. doi: 10.1016/j.pocean.2018.05.007
Tosetto E. G., Bertrand A., Neumann-Leitão S., Nogueira Júnior M. (2022). The Amazon River plume, a barrier to animal dispersal in the Western Tropical Atlantic. Sci. Rep. 12. doi: 10.1038/s41598-021-04165-z
Tran C., Hadfield M. G. (2013). Localization of sensory mechanisms utilized by coral planulae to detect settlement cues. Biol. (Basel) 132(3), 195–206. doi: 10.1111/ivb.12025
Treml E. A., Halpin P. N., Urban D. L., Pratson L. F. (2008). Modeling population connectivity by ocean currents, a graph-theoretic approach for marine conservation. Landsc Ecol. 23, 19–36. doi: 10.1007/s10980-007-9138-y
Tyberghein L., Verbruggen H., Pauly K., Troupin C., Mineur F., De Clerck O. (2012). Bio-ORACLE: A global environmental dataset for marine species distribution modelling. Global Ecol. Biogeography 21, 272–281. doi: 10.1111/j.1466-8238.2011.00656.x
Vale N. F., Braga J. C., Bastos A. C., Moraes F. C., Karez C. S., Bahia R. G., et al. (2022). Structure and composition of rhodolith beds from the Sergipe-Alagoas basin (NE Brazil, southwestern Atlantic). Diversity 14, 282. doi: 10.3390/d14040282
van der Ven R. M., Ratsimbazafy H. A., Kochzius M. (2022). Large-scale biogeographic patterns are reflected in the genetic structure of a broadcast spawning stony coral. Coral Reefs 41, 611–624. doi: 10.1007/s00338-022-02220-3
van Soest R. W. M., Boury-Esnault N., Vacelet J., Dohrmann M., Erpenbeck D., de Voogd N. J., et al. (2012). Global diversity of sponges (Porifera). PloS One 7. doi: 10.1371/journal.pone.0035105
Vermeij M. J. A., Marhaver K. L., Huijbers C. M., Nagelkerken I., Simpson S. D. (2010). Coral larvae move toward reef sounds. PloS One 5. doi: 10.1371/journal.pone.0010660
Vermeij M. J. A., Sampayo E., Bröker K., Bak R. P. M. (2004). The reproductive biology of closely related coral species: Gametogenesis in Madracis from the southern Caribbean. Coral Reefs 23, 206–214. doi: 10.1007/s00338-004-0368-z
Veron J. E. N. (1995) Corals in Space and Time: The Biogeography and Evolution of the Scleractinia. Available online at: https://books.google.com.br/books?hl=pt-BR&lr=&id=piQvtbFUicAC&oi=fnd&pg=PR9&dq=veron+1995&ots=ZVdqel-33p&sig=2Hzui8JpReTG0gZl.
Veron J. E. N., Devantier L. M., Turak E., Green A. L., Kininmonth S., Stafford-Smith M., et al. (2009). Delineating the coral triangle. Galaxea J. Coral Reef Stud. 11 (2), 91–100. doi: 10.3755/galaxea.11.91
Veron J. E. N., Devantier L. M., Turak E., Green A. L., Kininmonth S., Stafford-Smith M., et al. (2011). “The coral triangle,” in Coral Reefs: An Ecosystem in Transition (Springer Netherlands), 47–55. doi: 10.1007/978-94-007-0114-4_5
Veron J. E. N., Stafford-Smith M. G., Turak E., DeVantier L. M. (2016) Corals of the world. Available online at: http://www.coralsoftheworld.org/species_factsheets/ (Accessed 02 Oct 2023).
Villéger S., Mason N. W., Mouillot D. (2008). New multidimensional functional diversity indices for a multifaceted framework in functional ecology. Ecology 89, 2290–2301. doi: 10.1890/07-1206.1
Violle C., Navas M. L., Vile D., Kazakou E., Fortunel C., Hummel I., et al. (2007). Let the concept of trait be functional. Oikos 116 (5), 882–892. doi: 10.1111/j.0030-1299.2007.15559.x
Walter R. K., Armenta K. J., Shearer B., Robbins I., Steinbeck J. (2018). Coastal upwelling seasonality and variability of temperature and chlorophyll in a small coastal embayment. Cont Shelf Res. 154, 9–18. doi: 10.1016/j.csr.2018.01.002
Watson J. R., Hays C. G., Raimondi P. T., Mitarai S., Dong C., Mcwilliams J. C., et al. (2011). Currents connecting communities: Nearshore community similarity and ocean circulation. Ecology 92, 1193–1200. doi: 10.1890/10-1436.1
Webster F. J., Babcock R. C., Van Keulen M., Loneragan N. R. (2015). Macroalgae inhibits larval settlement and increases recruit mortality at Ningaloo Reef, Western Australia. PloS One 10. doi: 10.1371/journal.pone.0124162
Wei T., Simko. V. (2017). R package “corrplot”: Visualization of a Correlation Matrix (Version 0.84). Available at: https://github.com/taiyun/corrplot.
Weiss K. C. B., Ray C. A. (2019). Unifying functional trait approaches to understand the assemblage of ecological communities: synthesizing taxonomic divides. Ecography 42, 2012–2020. doi: 10.1111/ecog.04387
White C., Selkoe K. A., Watson J., Siegel D. A., Zacherl D. C., Toonen R. J. (2010). “Ocean currents help explain population genetic structure,” in Proceedings of the Royal Society B: Biological Sciences (Royal Society). 1685–1694. doi: 10.1098/rspb.2009.2214
Woolsey E. S. (2014). Coral larval ecology and biogeography in a warming ocean. Doctoral dissertation, James Cook University, Townswille, Australia. Available at: http://researchonline.jcu.edu.au/40732/.
Yang C., Ye H., Tang S. (2020). Seasonal variability of diffuse attenuation coefficient in the Pearl River estuary from long-term remote sensing imagery. Remote Sens (Basel) 12. doi: 10.3390/rs12142269
Yeager L. A., Deith M. C., McPherson J. M., Williams I. D., Baum J. K. (2017). Scale dependence of environmental controls on the functional diversity of coral reef fish communities. Global Ecol. Biogeogr. 26 (10), 1177–1189. doi: 10.1111/geb.12628
Zilberberg C., Abrantes D. P., Marques J. A., MaChado L. F., Fernandes de Barros Marangoni L. (2016). Conhecendo os recifes brasileiros: rede de pesquisas Coral Vivo. Rio de Janeiro: Museu Nacional, UFRJ, 2016, 360 pp.
Keywords: biogeography, diversity patterns, beta diversity, trait space, functional redundancy
Citation: Bleuel J, Waechter L, Bender M and Longo GO (2024) Taxonomic and functional diversity of zooxanthellate corals and hydrocorals in Southwestern Atlantic reefs. Front. Ecol. Evol. 12:1322751. doi: 10.3389/fevo.2024.1322751
Received: 16 October 2023; Accepted: 04 April 2024;
Published: 01 May 2024.
Edited by:
Lorenzo Alvarez-Filip, National Autonomous University of Mexico, MexicoReviewed by:
Manuel Olán-González, National Autonomous University of Mexico, MexicoFabian Alejandro Rodriguez-Zaragoza, University of Guadalajara, Mexico
Copyright © 2024 Bleuel, Waechter, Bender and Longo. This is an open-access article distributed under the terms of the Creative Commons Attribution License (CC BY). The use, distribution or reproduction in other forums is permitted, provided the original author(s) and the copyright owner(s) are credited and that the original publication in this journal is cited, in accordance with accepted academic practice. No use, distribution or reproduction is permitted which does not comply with these terms.
*Correspondence: Guilherme O. Longo, Z3VpbGhlcm1lLm8ubG9uZ29AZ21haWwuY29t