- 1Department of Entomology and Nematology, University of Florida, Gainesville, FL, United States
- 2Department of Plant Pathology, University of Nebraska – Lincoln, Lincoln, NE, United States
- 3Department of Communication and Department of Biology, University of Nebraska – Kearney, Kearney, NE, United States
Introduction: Global change events (e.g., worsening drought) are increasing environmental stress in a variety of terrestrial and aquatic habitats. The degree to which communities in soils and sediments are driven by temporal environmental changes across multiple habitat types from the same region is not clear.
Methods: We used nematodes, a common bioindicator of soil and sediment health, to determine how community diversity and composition are altered by rising alkalinity across lakes, shorelines, and prairies in the western Nebraska Sandhills. We sampled these three habitats from five lake basins spanning an alkalinity gradient (pH 7–11) across three years (2019, 2020, 2021). During our sampling, the Sandhills experienced a range of drought intensities, with 2019 being a wet year, followed by severe drought in 2020, and abnormally dry/moderate drought in 2021. To determine if diversity and composition of nematodes responded to increased alkalinity and drought-induced stress we used different modelling approaches, including Random Forest and pairwise comparisons.
Results and discussion: Overall, nematode diversity in lakes was most affected by increasing alkalinity over time, whereas in shorelines and prairies diversity was most reliant on bacterial diversity and potential nematode-nematode interactions. In comparison to shorelines and prairies, community composition in lakes was the least variable and consistently driven by pH and lake water levels. In contrast, compositions in the shorelines and prairies were more variable and explained at varying degrees by pH, year, lake basin, and climate-associated variables. In addition, relative abundance and compositional nature of select copious taxa were highly unpredictable, indicating potential instability in these habitats. Future research is necessary to address the ecologic stability of the Sandhills and determine where conservation efforts are most needed.
Introduction
Soil and sediment communities change over time in response to dynamic deterministic and stochastic processes. Deterministic selection modifies community diversity and composition via shifts in abiotic and biotic conditions (Fargione et al., 2003; Vellend, 2010; Dini-Andreote et al., 2015; Zhou and Ning, 2017), whereas stochastic processes provide opportunities for new species introductions (i.e., drift) (Chave, 2004; Chase and Myers, 2011; Nemergut et al., 2013). Temporal change in soil and sediment communities are often linked to changes in assembly processes. Specifically, as global change events (e.g., fire and drought) intensify over time, simultaneous shifts in assembly processes related to shifts in abiotic conditions have been observed. For example, as a result of drought and decreased precipitation, pH in bodies of water, sediments, and soils moved from neutral to more acidic/alkaline (Godbold and Calosi, 2013; Strang and Aherne, 2015; Jiao et al., 2016; Houle et al., 2020), and in response microbial diversity and functionality decreased (Wang et al., 2014; Tan et al., 2020; Jia et al., 2022). In addition to shifts in diversity, temporal variation in pH can also modify bacterial inter- and intraspecific interactions (Ratzke and Gore, 2018). These temporal shifts in diversity, interactions, and assembly could threaten ecosystem stability and ultimately functionality (i.e., ability to resist environmental disturbances) (Grimm and Wissel, 1997; Liu et al., 2018).
Nematodes are considered excellent bioindicators of soil and sediment conditions and health (Neher, 2001; Sánchez-Moreno and Ferris, 2018; Schenk et al., 2020; Ridall and Ingels, 2021) and ideal model organisms to study temporal stability in an ecosystem. They are the most diverse and abundant animals on the planet (Van Den Hoogen et al., 2019; Hodda, 2022). With a diversity of feeding habits (i.e., bacterial-feeding, fungal-feeding, omnivores, predators, and plant and animal parasites) and positioning at all trophic levels in food webs, nematodes perform key ecosystem functions (De Mesel et al., 2004; Pascal et al., 2008; Heidemann et al., 2014; Majdi and Traunspurger, 2015). For example, bacterial- and fungal-feeding nematodes exert control over decomposition and nutrient mineralization by feeding on microbes as well as keeping them in their active growth phase (Gerlach, 1978; Bonaglia et al., 2014; Creamer et al., 2016). Although they are integral components of soil and sediment communities, knowledge of how nematode community diversity and/or composition respond to change over time and increased environmental stress is limited. In high elevation successional gradient studies where the effects of temporal change were studied indirectly by substituting space for time, soil nematode diversity responded positively to some climate driven shifts in abiotic (e.g. increased soil moisture and pH) and biotic (e.g. higher diversity of plant communities and microbes) factors (e.g. Li et al., 2020; Hu et al., 2021; Porazinska et al., 2021). In a more direct temporal study of beach sediments in Qingdao, China, as pH decreased over time, aquatic nematode diversity decreased as well (Hua et al., 2019), however the effect was context dependent, with nematode diversity in finer sediments more resistant to changing pH. These examples suggest that nematode community stability across habitats may vary in response to drought-driven shifts in abiotic conditions. However, the consistency of response to drought across a habitat gradient remains unclear. Thus, the goal of this study was to examine how drought and subsequently more stressful environmental conditions affected the diversity, composition, and stability of nematode communities from three distinct habitats.
The Nebraska Sandhills is a region threatened by drought events that result in subsequent shifts in soil and sediment biogeochemical characteristics (Adane et al., 2019; Gattoni et al., 2022; National Drought Mitigation Center, 2023). While the landscape of the Sandhills is dominated by grass-stabilized sand dunes, there are also hundreds of embedded, natural shallow lakes. The western region is known for the presence of an alkalinity gradient (pH 7–11) driven by potassium, sodium, and chloride within the lakes but also in the surrounding dunes. During our study period (2019-2021), the Sandhills experienced intermittent drought (National Drought Mitigation Center, 2023). Decreased precipitation and drought can be linked to fluctuations in pH and biogeochemical concentrations in both soils and lake sediments (Jiao et al., 2016; Li et al., 2017). However, the effect of drought-induced change in pH can be habitat specific. For example, pH shifts in prairie soils have been shown to be influenced by plant productivity more than precipitation, suggesting a potential for the plant community to buffer the effect of drought on soil communities (Waldrop et al., 2017). Additionally, high levels of plant and microbial diversity act as buffers for grassland ecosystems by decreasing the negative effect of drought on overall biodiversity (Castillioni et al., 2020; Jin et al., 2022). In contrast, lakes may be affected by drought more directly with decreased water levels causing acidification or alkalization (Li et al., 2017), modifying sediment carbon content (Weise et al., 2016), and destabilizing microbial communities (Pu et al., 2023).
In our previous study of nematodes from three habitat types (i.e., lake, shoreline, and prairies) in the western Sandhills, we showed that within a single year, communities had distinct compositions and structures and experienced different assembly processes (Gattoni et al., 2022). Specifically, communities were differentially explained by deterministic factors (abiotic and biotic) with most variation explained in the lakes (59%) and least in the prairies (18%). While abiotic factors (i.e., pH and ion concentrations) were equally important to communities in all habitats (~16%), the role of biotic factors (i.e., diversity of microbial communities) varied and dominated (48%) in lake communities, contributed equally to abiotic factors in shoreline communities, and played a seemingly minor part in the prairie communities, albeit dependent on the identity of the nematode trophic group.
Given recent variability of drought conditions in the Nebraska Sandhills, we set out to examine potential shifts in nematode communities in the three habitats. We hypothesized that drought-induced increase in stress over time (e.g., more extreme alkalinity and reduced plant productivity) would alter nematode community diversity and composition, hence stability, but the response would be habitat specific with the lake communities affected by temporal change the most, followed by shorelines, and prairies, respectively.
Methods
The research was conducted at Crescent Lake National Wildlife Refuge (CLNWR) (41° 45’ 41.004” N, 102° 26’ 12.012”W) in the western Nebraska Sandhills in 2019, 2020, and 2021 (Figure 1). For this study, we selected five lake basins representing an alkalinity gradient ranging from ~7 to 11 pH with the two most neutral basins (Gimlet and Island) situated ~14 km from the most alkaline basins (Bean, Border, and Kokjohn). Island Lake was the largest, followed by Bean, Gimlet, Border, and Kokjohn. Importantly, Kokjohn was wet in 2019 but periodically dry in 2020 and 2021. More information regarding the study site can be found in Gattoni et al., 2022.
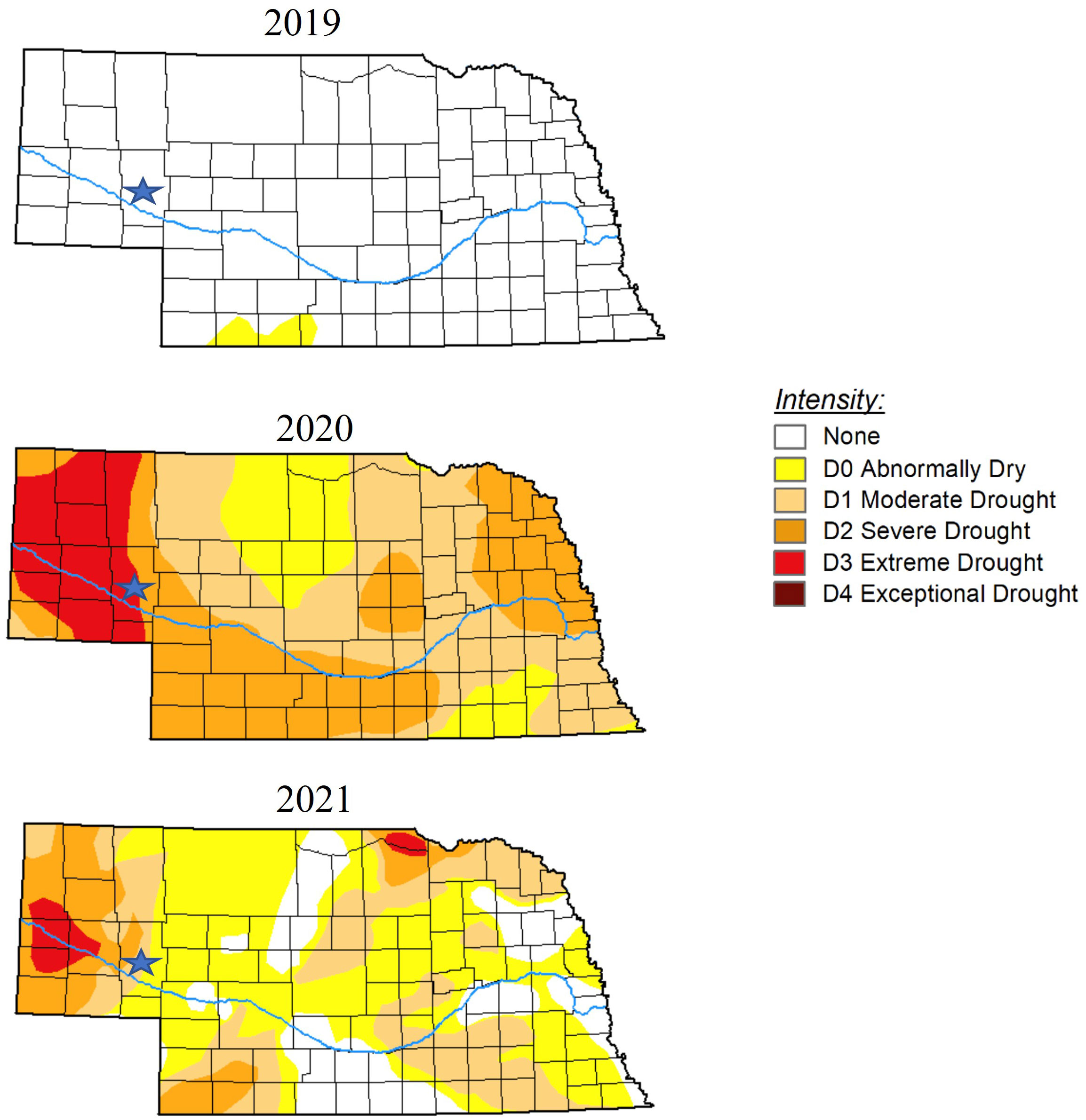
Figure 1 U.S. Drought Monitor maps indicating dry or drought conditions throughout the state of Nebraska in October 2019, 2020, and 2021. Stars indicate location of Crescent Lake National Wildlife Refuge where samples were obtained. The U.S. Drought Monitor is jointly produced by the National Drought Mitigation Center at the University of Nebraska-Lincoln, the United States Department of Agriculture, and the National Oceanic and Atmospheric Administration. Maps courtesy of National Drought Mitigation Center (2023).
In mid-October 2019, 2020, and 2021, we collected three (2019) and four (2020, 2021) replicate lake sediment (denoted as lake), shoreline sediment/soil (denoted as shoreline), and prairie soil (denoted as prairie) samples. To ensure sample independence among replicates within each habitat, sampling locations were separated by 30 – 100 m. In 2019, lake samples were obtained by wading into the water (~10 m from the shore) and using a soil corer (see below). In 2020 and 2021, they were obtained from the middle of the lake accessed by a kayak using a 15 cm x 15 cm x 20 cm dredge and consisted of three composite dredges. Each shoreline and prairie sample (as well as lake samples in 2019) consisted of ~15 – 20 soil cores (~2 cm x 10 cm) from an area equivalent to 20 m2. Prairie samples were taken in proximity to plant stems. Generally, plant diversity was limited (~10 plant species) and similar among lake basins. Sediment and soil samples were collected in Whirlpacks or gallon Ziplocks, gently homogenized, and placed in a cooler with ice until processed upon return to the University of Nebraska-Lincoln (2019) or University of Florida (2020, 2021).
Nematode sample processing
In 2019, nematodes were extracted from 50 g of soil/sediment subsamples at the University of Nebraska-Lincoln (Gattoni et al., 2022), but in 2020 and 2021 from 100 g at the University of Florida. Briefly, two concurrent methods (sugar centrifugation and 48-hour modified Baermann funnels) were used to extract nematodes. All nematode extractions were enumerated and morphologically identified using inverted microscopy and keys (Goodey, 1963) to at least family level and further categorized into trophic groups (i.e., bacterial feeder, fungal feeder, plant parasite, predator, omnivore, or animal parasite) (Yeates et al., 1993). Because food sources of Tylenchidae are not well known (Qing and Bert, 2019) and could involve root hairs or root-associated fungi, nematodes in this family were classified as a separate group of root associates. This morphology-based data provided baseline information to ensure the accuracy of DNA-based analyses at a finer level of taxonomic resolution.
Nematode DNA from morphologically identified communities was extracted with the Qiagen® DNeasyPowerSoil Kit according to the manufacturer’s instructions (Qiagen Inc., Toronto, Canada). Extracted DNA samples were amplified using NF1/18Sr2b (Porazinska et al., 2009) following EMP protocols (Thompson et al., 2017). DNA was also extracted directly from 0.35 g of soil as described above and was amplified using the 16S (515f/926r) (Apprill et al., 2015; Parada et al., 2016) and 18S (1391f/EukBr) (Amaral-Zettler et al., 2009; Caporaso et al., 2012) rRNA metabarcoding gene markers for bacteria and eukaryotes.
All amplifications were done in triplicates for each sample, and all amplicons were verified by gel electrophoresis. Triplicates were pooled and all samples sent to the Hubbard Center for Genome Studies, University of New Hampshire, for the attachment of primer indexes (using Golay barcodes) (Caporaso et al., 2012), library preparation, and sequencing using Illumina sequencing (HiSeq 2×250 bp, Illumina Inc., CA, USA). Negative controls were implemented during DNA extraction and PCR and sequenced together with other samples.
Amplicon data processing
Qiime2 v2021.4 was used to remove primer regions of the demultiplexed sequences with cutadapt software (Martin, 2011). Forward and reverse reads were joined using vsearch in Qiime2 (Rognes et al., 2016), and joined sequences were filtered for quality using q-score-joined in Qiime2 with default settings (Bokulich et al., 2013). Sequences were clustered into operational taxonomic units (OTUs) using vsearch at 99% similarity for nematodes and 97% for bacteria and eukaryotes to produce OTU tables. Taxonomic assignment of OTUs was performed using BLAST with 18S-NemaBase (Gattoni et al., 2023) for nematodes and SILVA v138 for all other microbial OTU tables.
OTUs representing less than 0.001% of total reads and OTUs with low percent ID (<85%) and low query coverage (<95%) were removed from all OTU tables. The nematode OTU table was filtered to only include nematodes, bacterial table to only include bacteria, and the eukaryotic table to include only fungi and simple eukaryotes (i.e., protists and algae) split into two independent tables. Using taxonomic trees, the nematode OTU table was collapsed as directed by “Head-Tail” patterns (Porazinska et al., 2010) (see Gattoni et al., 2022 for more details), and the number of reads was standardized to per 100 g of dry soil/sediment for nematodes and 1 g for all soil microbes.
Biogeochemical and climatic data analysis
Biogeochemistry and climate-associated data were used as explanatory variables. Gravimetric soil moisture (%) was measured by drying 20 g of soil/sediment subsamples for 24 hrs at 60°C. Subsamples of ~100 g were sent to Ward Laboratories, Inc., Nebraska, USA for biogeochemical analyses including pH, % organic matter (OM), potassium (K), calcium (Ca), sodium (Na), zinc (Zn), iron (Fe), and chloride (Cl).
The extent of surface water in lakes and the productivity of vegetation were measured using data obtained from Copernicus Sentinel-2 satellite imagery (Copernicus Sentinel data [2019, 2020, 2021]) from the available date closest to each sampling period. From satellite imagery, the Green Normalized Difference Vegetation Index (GNDVI) isolated water from vegetation. A polygon was fit to each of the lakes based on the GNDVI, and from each polygon the extent of surface water in lakes (hereafter referred to as “lake level”) was calculated. Normalized Difference Vegetation Index (NDVI) was used to measure vegetative production at the prairie sampling sites. NDVI values were taken for all prairie samples.
Statistical analyses
Statistical analyses were performed in R V.3.6.1 (R Core Team, 2019). Treatments were defined by habitat (lake, shore, prairie), lake basin (in order from lowest to highest pH: Gimlet, Island, Bean, Border, Kokjohn), and year (2019, 2020, 2021). First, Shannon’s alpha diversity was calculated for nematode and all microbial communities using the ‘estimate richness’ function in ‘phyloseq’ package V.1.34.0 (McMurdie and Holmes, 2013). To select variables for downstream analyses and address the relationships among abiotic (biochemical variables), biotic (i.e., Shannon’s diversity), climate-associated variables (i.e., lake water level, soil moisture and NDVI), and time, correlation matrices were created with Pearson’s correlation using the ‘cor’ function in ‘stats’ V3.6.2 and visualized using ‘corrplot’ V0.84 (Wei and Simko, 2017).
The effect of abiotic, biotic, and climatic variables on the diversity of nematode communities was tested using Random Forest (RF) models via the ‘randomforest’ function from the ‘RandomForest’ package (Breiman, 2001). In RF models, multiple decision trees are used to assess the importance of independent variables on the outcome of the dependent variable. For our models, we used 1,500 trees and 4 variables per split (i.e., each branch of the tree considers 4 variables at a time). All models were tested for significance using the ‘rf.significance’ function from the ‘rfUtilities’ package, and the importance of each independent variable was calculated with the ‘rfPermute’ function in the ‘rfPermute’ package. For each model, the importance of the independent variables was reported as the percentage of mean standard error increase (%IncMSE) if the variable was removed from the model. To ensure Random Forest models captured how the change in abiotic, biotic, and climate-associated variables as well as lake basin structure nematode diversity (i.e., the dependent variable), each independent variable in the model was paired with year as an interaction term. Lake basin was included as a variable to encompass characteristics of each basin that were not addressed with the other variables, such as geography or plant community composition. Year was also a lone independent variable in the model to account for the effect of any additional temporal shift on diversity. Based on previous knowledge from the region, pH, chloride, sodium, and potassium were decided as the abiotic variables for lake and shoreline communities with the addition of organic matter for prairie communities. For all models, bacterial, microbial eukaryotic, and fungal diversity were included as biotic factors. As climate-associated variables, lake water level was used for the lake and shoreline communities, and soil moisture and NDVI for the prairie community. All variables that had a negative %IncMSE value were removed. Finally, due to consistent differences in community assembly processes among the terrestrial trophic groups (Gattoni et al., 2022), models were run independently for bacterial-feeding, fungal-feeding, plant-parasitic, omnivore, and predatory taxa in the prairies. The diversity of selected nematode feeding types was added as independent variables with year as an interaction term for each.
Compositional matrices were created based on Aitchison distance (Aitchison et al., 2000) to test change in nematode community composition over time. Compositional differences among habitats and basins were tested with permutational analysis of variance (PERMANOVA) with 999 permutations and visualized with principal coordinate analysis (PCoA) ordinations. Pairwise comparisons were conducted for each habitat type using the ‘pairwiseAdonis’ function in the ‘pairwiseAdonis’ package (Martinez Arbizu, 2020). Pairwise comparisons were used to determine which variables were most important to the differences in composition seen in each year. The first independent term in each comparison was defined by year to dictate the term of main interest which dictates comparisons. Other independent variables included pH, climate-associated variables, and lake basin blocked by lake basin. As climate-associated variables were habitat specific, lake water level was used for lakes and shorelines and NDVI for prairies.
General linear models (GLM) were used to identify how highly abundant nematode genera responded to shifts in abiotic variations over time. From each habitat type, the most abundant genera were evaluated with the relative abundance of each as the dependent variable in the models. pH, climate (i.e., lake level for shoreline and sediment and soil moisture and NDVI for prairie), basin, and year as well as the interaction of year with both pH and climate were the independent variables. All models were created in ‘glmmTMB’ and visualized with ‘ggplot2’.
Results
Relationships among variables
As expected, potassium, sodium, and chloride were positively correlated with pH and each other in all habitat types (Figure 2). In lakes, abiotic variables showed strong negative relationships with all biotic variables, where both microbial and nematode diversities decreased as pH and associated ion concentrations increased (Figure 2A). These relationships in shorelines were also negative, albeit weaker than in lakes (Figure 2B). However, there was no relationship with nematode diversity. And finally, in prairies, positive relationships between abiotic and biotic variables were only observed for nematodes and not for bacteria, fungi, or microbial eukaryotes (Figure 2C). Biotic variables in the lakes positively correlated with each other, as did those in the shorelines except for fungal and nematode diversity. In prairies, bacterial diversity positively correlated with fungal and eukaryotic diversity. There were negative relationships between pH and ion concentrations with lake water levels in both the lakes and shorelines (Figures 2A, B). Bacterial and nematode diversity positively correlated with lake water level in lakes, and all biotic variables positively correlated with water level in shorelines. In prairies, soil moisture positively correlated with fungal diversity and NDVI with eukaryotic diversity.
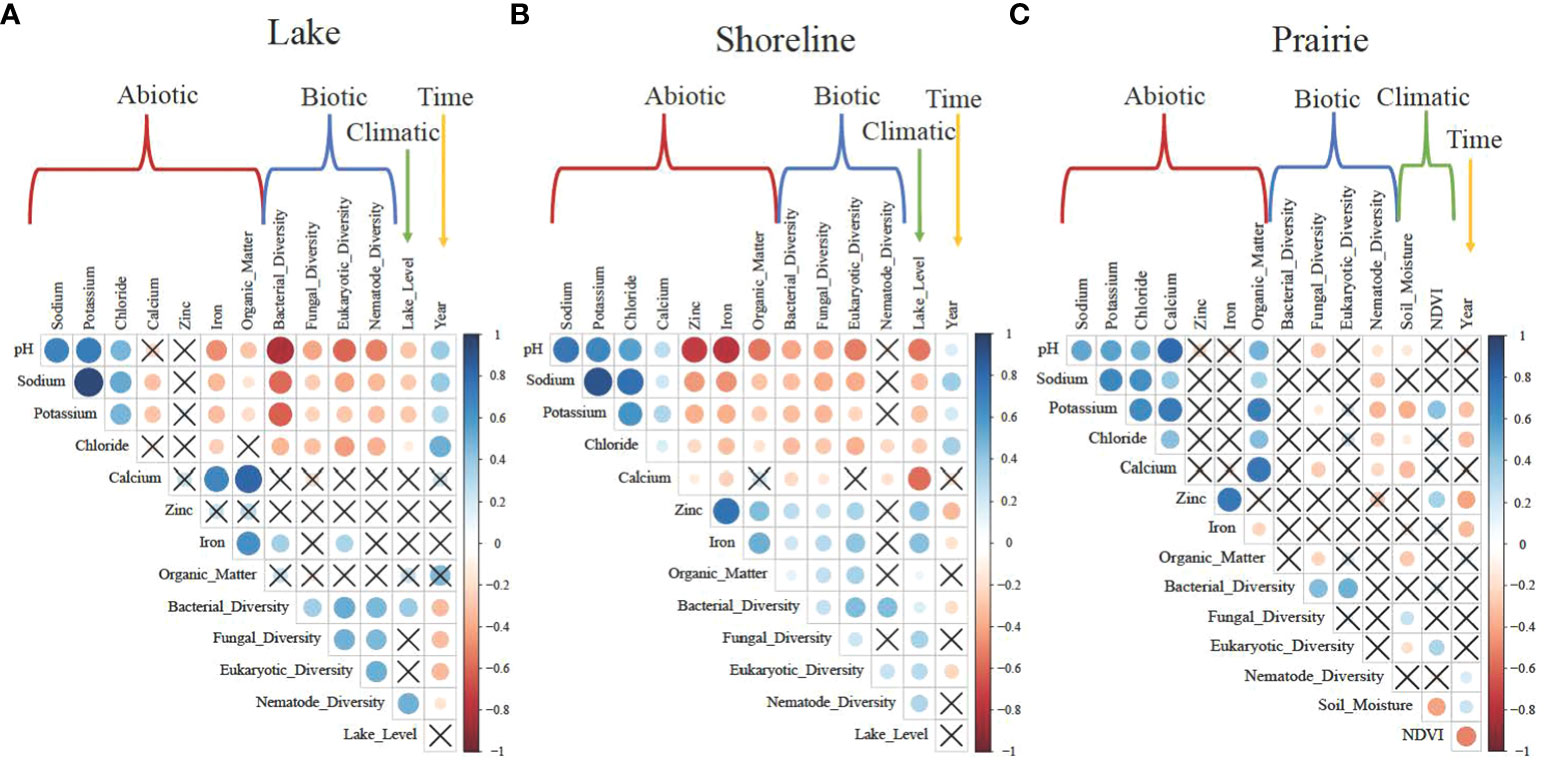
Figure 2 Correlation plots indicating all potential relationships between abiotic, biotic, climatic and time variables in (A) Lake, (B) Shoreline, and (C) Prairie habitats. Abiotic variables (red brackets) include habitat biogeochemistry (e.g., pH, concentrations of sodium and potassium). Biotic variables (blue brackets) are Shannon's diversity of bacterial, fungal, eukaryotic, and nematode communities. Climate-associated (climatic) variables (green arrows/brackets) are water lake level for Lake and Shoreline habitats and soil moisture and NDVI for the Prairie habitat. Time (yellow arrow) is a year of sampling (3 years). Color scale represents the direction and strength of relationships from +1 (blue for positive) to -1 (red for negative). Boxes without "X" indicate significantly correlated variables.
There were strong positive relationships between pH and ion concentrations with time (year) in lakes and shorelines, but there was no significant relationship between time and lake water levels (Figures 2A, B). Overall, in prairies, neither abiotic nor biotic variables correlated with time or climate-associated variables (Figure 2C).
Nematode diversity
In general, lake nematode communities had the lowest diversity followed by the shorelines and then prairies (Supplementary Figure S1). Except for year alone, all other factors including habitat, basin, and all factor combinations significantly explained variation in diversity (Supplementary Table S1).
To determine how the importance of change over time in abiotic, biotic, and climatic variables and time alone (year) to nematode diversity varied among habitats, we used RF models. Overall, the effect of change in all variables over time on nematode diversity was habitat specific. While the temporal change in eukaryotic diversity, pH, and lake level were the most important to lake communities (Variance Explained= 35.7%, Mean Sum of Square Residuals= 0.22, Figure 3A), bacterial diversity, year alone, and lake basin alone were the most important to shoreline communities (Variance Explained= 20.0%, Mean Sum of Square Residuals= 0.40, Figure 3B). RF models were not run for specific trophic groups due to the low trophic diversity in the lakes and shorelines.
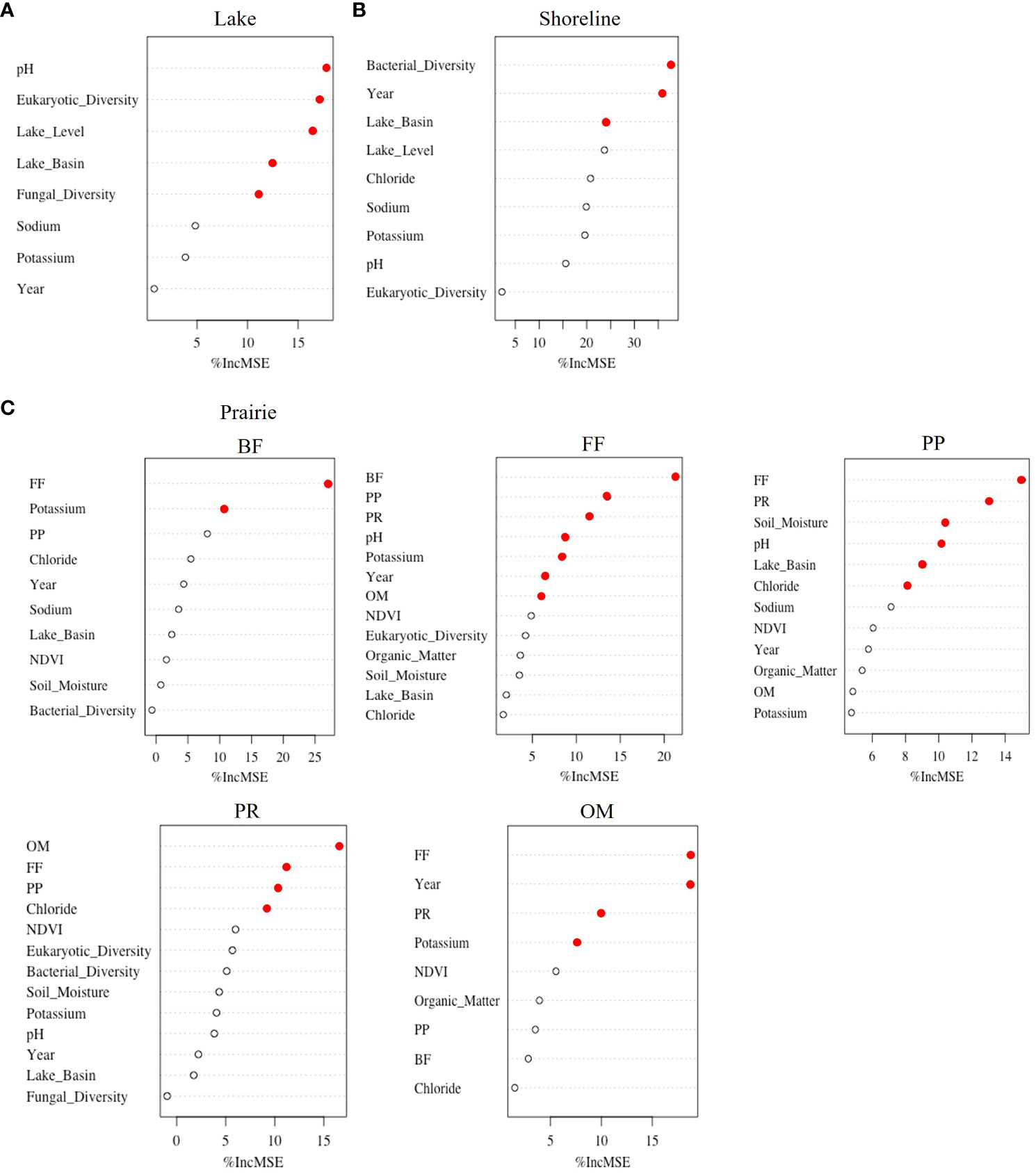
Figure 3 Random Forest models explaining variance, accuracy, and importance of abiotic, biotic, climatic, and time variables to nematode Shannon’s diversity in (A) Lake, (B) Shoreline, and (C) Prairie habitats. Importance is shown by percentage of mean standard error increase (%IncMSE) if the variable is removed from the model, with filled red circles indicating significant variables and empty black circles nonsignificant variables. Models for the Prairie habitat were run at the level of nematode trophic group (i.e., BF, bacterial feeders; FF, fungal feeders; PP, plant parasites; PR, predators; and OM, omnivores).
In the prairies, separate RF models were run for each trophic group. All models indicated that the temporal change in diversity of another nematode trophic group was the most important variable (Figure 3C). For example, the diversity of bacterial-feeding nematodes was the most significantly influenced by the change in diversity of fungal-feeding nematodes (Variance Explained= 27.8%, Mean Sum of Square Residuals= 0.16). Similarly, the diversity of fungal-feeding nematodes was explained the most by the change in diversity of bacterial feeders, along with that of plant-parasitic and predatory nematodes (Variance Explained= 45.1%, Mean Sum of Square Residuals= 0.25). The change in each basin over time was significant only for plant parasites (Variance Explained= 30.3%, Mean Sum of Square Residuals= 0.16). From all tested abiotic factors, temporal change in pH, potassium, and chloride were indicated as significant in explaining diversity of some but not all nematode trophic groups. For example, predatory nematodes were most strongly correlated with changes in omnivore, fungal-feeding, and plant-parasitic diversity with chloride also important (Variance Explained= 30.9%, Mean Sum of Square Residuals= 0.13). Overall, there was little evidence that changes in diversity of microbial groups were significantly important to diversity of any prairie nematode trophic group. Similarly, time alone was only important to fungal feeders and omnivores (Variance Explained= 23.4%, Mean Sum of Square Residuals= 0.23).
Community composition
Compositionally, nematode communities separated by habitat type along the PCo1 axis with the lake and shorelines distinct from prairies (Figure 4). In addition, composition of communities from lakes and shorelines separated along the PCo2 axis, interpreted as alkalinity, with the most alkaline basins distinct from most neutral. Using PERMANOVA, habitat, basin, year, and all interactions of the three factors significantly affected composition. Of all factors, habitat was the most important (R2 = 0.23) (Supplementary Table S2) with others explaining a similar amount of compositional variance (R2 = 0.02-0.08).
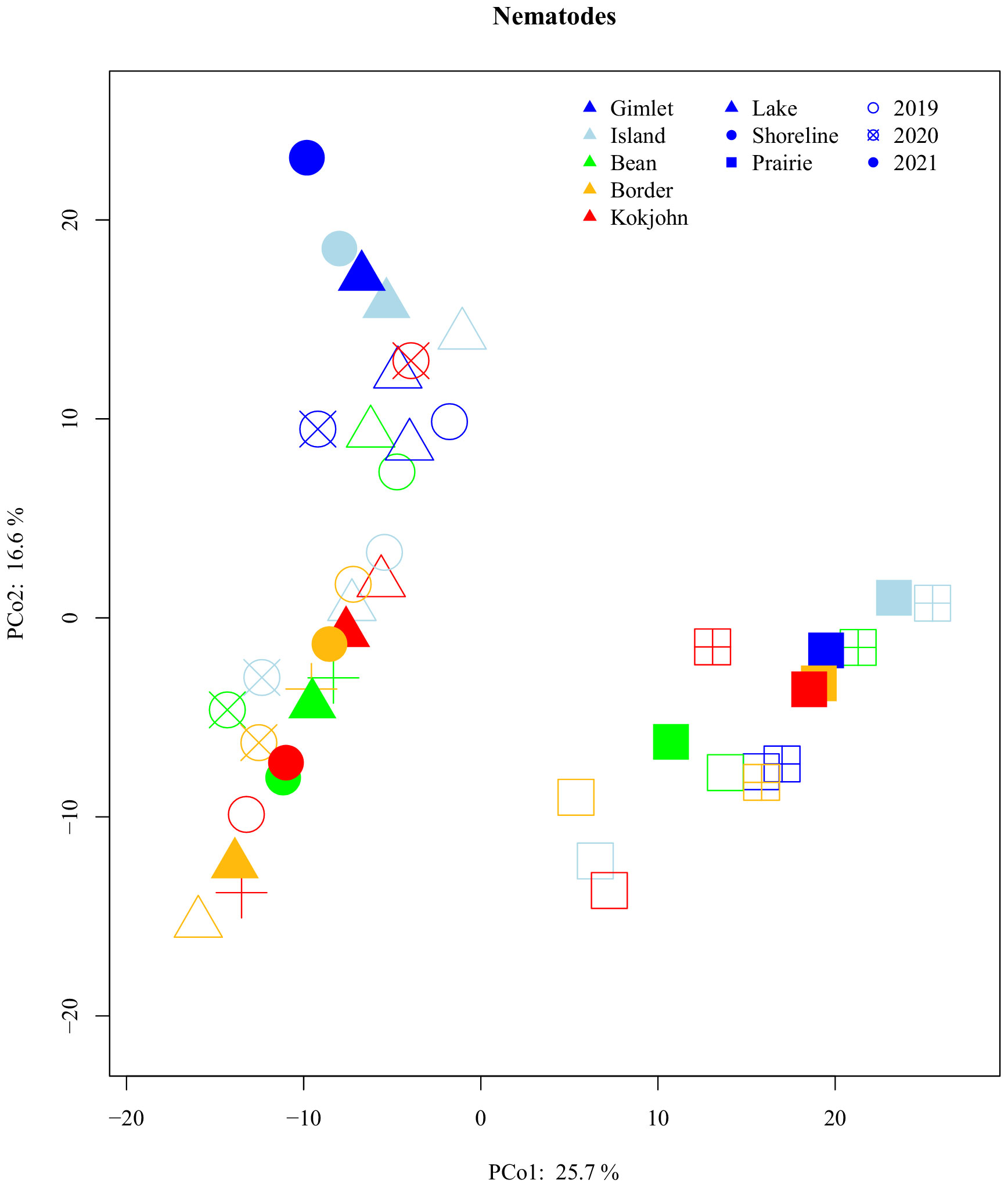
Figure 4 Principal coordinate analysis (using Aitchison's distances) of nematode compositional community dissimilarity. Points indicate centroids. Communities show a general clustering by both habitat (Lake, Shoreline, and surrounding Prairie) and lake basin (Island, Gimlet, Bean, Kokjohn, and Border), but not Year (2019, 2020, and 2021). Color of each point indicates the lake, shape indicates habitat, and fill (i.e., hollow shape, "x" through shape, filled shape) indicates year.
Pairwise PERMANOVAs were used to compare compositional differences between sampling years for each habitat type and determine how they were affected by pH, climate-associated variables (i.e., lake water level in lakes and shorelines and NDVI in prairies), lake basin, year, and the interactions of these terms. Lake communities were relatively similar among all years. Although pH was indicated as the most consistently significant factor (Table 1) shaping the communities, the degree to which it explained compositional variability varied with year. For example, pH played a more significant role in shifts of lake compositional differences between 2020 and 2021 (R2 = 0.22) than 2019 and 2020 (R2 = 0.12) or 2019 and 2021 (R2 = 0.15). In addition to pH, lake level was also important across all years but explained a considerably lower amount of variation (R2 = 0.06-0.10) than pH. Lake basin played a role in compositional differences only between 2019 and 2021 (R2 = 0.30).
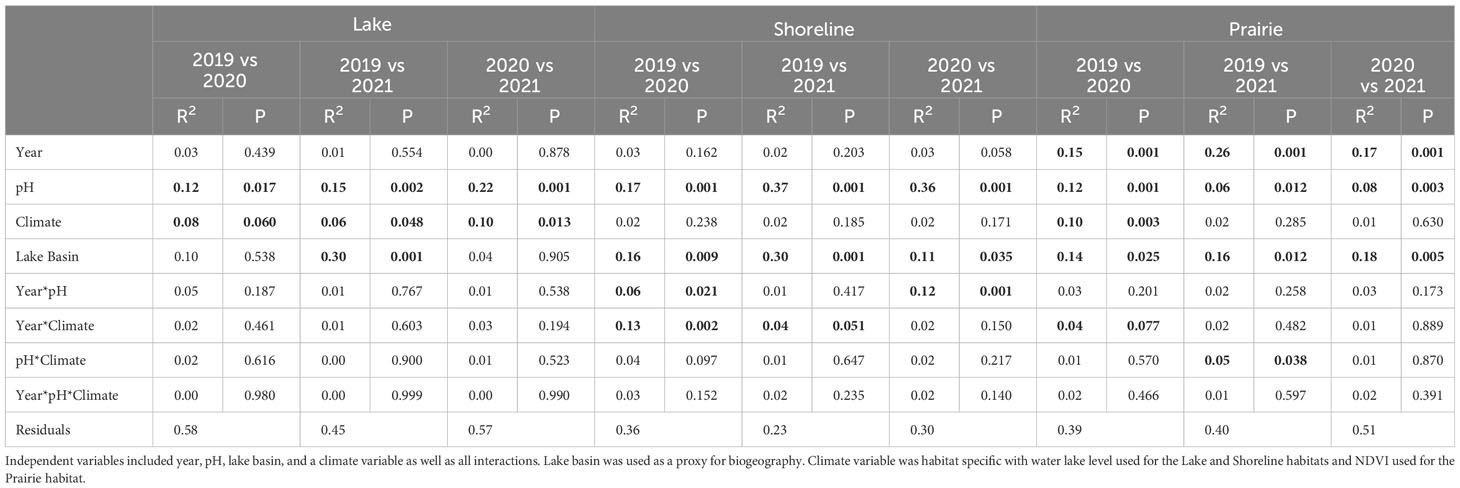
Table 1 Pairwise comparison of nematode community composition via Pairwise Adonis among years for each habitat type.
All yearly compositional differences in shoreline communities were influenced by pH and lake basin. Similar to lake communities, the magnitude of these relationships was variable. For example, the degree to which pH affected composition was lower between 2019 and 2020 (R2 = 0.17) than between 2019 and 2021 (R2 = 0.37) and 2020 and 2021 (R2 = 0.36). In addition, interaction of pH and year from 2019 to 2020 (R2 = 0.06) and 2020 to 2021 (R2 = 0.12) and the interaction of climate and year from 2019 to 2020 (R2 = 0.13) and 2019 to 2021 (R2 = 0.04) were significant to compositional differences.
Finally, differences in prairie community composition between years were influenced by pH, lake basin, and year. In contrast to diversity, year alone was the most influential of composition (R2 = 0.16-0.26) followed by NDVI (R2 = 0.10) and the interaction of NDVI and year (R2 = 0.04) contributed to difference in composition between 2019 and 2020. Lastly, the interaction of pH and NDVI influenced composition between 2019 and 2021 (R2 = 0.05) but no other years.
Nematode relative abundance
Across the lake and shoreline, Tobrilidae nematodes were the most abundant regardless of year (Supplementary Figure S2). In the neutral lakes and shorelines, there was more trophic and family diversity than in alkaline basins and differences in the relative abundance of certain families. For example, Dorylaimidae increased in abundance from 2019 to 2020 but decreased again in 2021. The prairies had the highest taxonomic and trophic diversity. While not dependent on alkalinity, relative abundance of families was most variable across basins in 2019. In contrasts, basins in both 2020 and 2021 were similar with 2020 dominated by bacterial-feeding nematodes, specifically Cephalobidae. Similarly, prairies in 2021 supported high numbers of bacterial feeders as well as fungal feeders and plant parasites.
The relative abundance of most common predatory nematodes in the lake communities were not impacted by year, pH, lake level, lake basin, and the combination of these factors in the same manner (Supplementary Figures S2, S3A, Supplementary Table S3). For example, Tobrilus and Brevitobrilus, common in the most neutral lakes, were not affected by any variables, whereas Eutobrilus, common in the most alkaline lakes, was affected by year, pH, and basin. While the bacterial-feeding nematodes in the lakes were largely present in the neutral lakes, these were also affected by the variables differently. For example, Eumonhystera was affected by basin, the interaction of pH and lake level, and the interaction of all variables, whereas Fictor and Monhystera were independent of these variables.
With a higher level of trophic diversity (Supplementary Figure S2), all highly abundant shoreline nematode genera were influenced by at least one variable considered (Supplementary Table S3). For example, unlike in the lakes, abundance of Tobrilus and Brevitobrilus were driven by year, climate, and pH in some combination (Supplementary Figure S3B; Supplementary Table S2). While lake level was not important to Epitobrilus in the lakes, it was in the shorelines in combination with both pH and year. In contrast, the last highly abundant Tobrilidae, Brevitobrilus, decreased over time and was influenced by lake level, basin, year, the interaction of lake level and year, and the interaction of pH, lake level, and year. The two highly abundant bacterial-feeding nematodes, Fictor and Plectus, both had the highest relative abundance in 2020 and were not influenced by pH or year but rather by basin and the interaction of pH and lake level, respectively. Lastly, the only omnivore considered, Mesodorylaimus, also had the highest relative abundance in 2020 and was solely influenced by year alone.
Prairies contained the highest level of trophic diversity (Supplementary Figure S2). Unlike the aquatic and semi-aquatic habitats, all genera in the prairies were affected by year alone or year in combination with pH and NDVI (Supplementary Figure S3C, Supplementary Table S2). Bacterial-feeding nematodes were generally highest in abundance and differentially driven by all factors. For example, Acrobeles increased from 2019 to 2020, remained consistent from 2020 to 2021, and was most influenced by basin, year, the interaction of pH and NDVI, and the interaction of pH, climate, and year. But two other nematodes in the same family of Cephalobidae, Acrobeloides and Cephalobus, were only influenced by pH and the interaction of pH, climate, and year. Moreover, their relative abundance patterns were opposite, especially in 2020. Pseudacrobeles, yet another representative of Cephalobidae, was the most variable over time and driven by all factors and combination of factors except the interaction of climate and year. Similarly, while both plant parasitic taxa were driven by year, Mesocriconema increased in abundance over time and was influenced by the interaction of pH and climate, and Xiphinema decreased in abundance and was more sensitive to climate, basin, the interaction of pH and year, and the interaction of climate and year. Lastly, the two taxa, Aporcelaimellus (predator) and Prodorylaimus (omnivore), were both driven by basin, year and the interaction of pH, climate, and year.
Discussion
Community diversity and composition are subject to climate-driven temporal shifts in abiotic conditions. The extent to which these alterations in assembly influence nematode communities over time is not clear. With variable drought-like conditions over our sampling period, the Nebraska Sandhills were an ideal system to provide insight into the impact of temporal shifts in abiotic conditions and subsequently on nematode community diversity and composition across a distinct habitat gradient allowing us to hypothesize on the potential stability of these communities/habitats.
Nematode community diversity in response to drought-induced abiotic stress
Correlations indicated significant relationships between the abiotic/biotic characteristics of the Sandhills environment and sampling time (i.e., year). In the lakes and shorelines, strong positive relationships among pH, abiotic variables tied to pH (i.e., sodium, potassium, and chloride), lake level, and time suggested that abiotic shifts in these habitats occurred over our sampling period. The increased pH/alkalinity in the lakes, specifically, correlated to harsher, more extreme environments and resulted in decreased microbial and nematode diversity. In the lakes of the Tibetan Plateau, the most similar lakes in the world to those of the alkaline lakes of the western Sandhills, increased pH also reduced microbial diversity (Ji et al., 2019). Our RF models also indicated that change in pH over time as well as change in lake level were the most determining factors of nematode community diversity in lakes. We predict that with continued droughts reducing lake water levels and higher alkalinity, nematode and microbial diversity in aquatic habitats will likely continue to decrease.
Importantly, change in eukaryotic diversity over time was also among the most significant factors shaping nematode community diversity in lakes, aligning well with Tobrilidae, the most common nematodes in the lakes. Although Tobrilidae are generally classified as predators (Yeates et al., 1993), many specimens in our samples often displayed green guts indicating they may be feeding on food sources other than classical prey (i.e., other nematodes) including unicellular algae that are common in these lakes. This is in contrast to our previous report based on 2019 sampling (Gattoni et al., 2022) when bacterial diversity, rather than eukaryotic, was the major factor contributing to lake nematode community assembly. With overall trophic diversity decreasing over time, due in large part to using a more appropriate sampling in the following two years (i.e., kayaking to the center of the lakes instead of wading near the shorelines), there were fewer bacterial-feeding nematodes in our 2020 and 2021 lake samples.
In the shorelines, where pH was also variable and conditions became more extreme in a similar manner to lakes, there was no significant relationship between pH and nematode diversity. Additionally, in the RF models, no abiotic variable was important to shoreline community diversity. With few studies of nematode or microbial communities in shorelines, this result is in contrast to our results in the lake sediment as well as a singular study of the hypersaline Ebinur Lake where microbial diversity was directly affected by lake pH (Zhao et al., 2018). Instead of abiotic variables, only year, change in bacterial diversity, and lake basin were important to nematode diversity. With abiotic variables unimportant, we expected lake water level to be influential, specifically because lake water levels were highly variable during our three sampling years. It is possible that since shoreline communities experience this change continuously (Loope and Swinehart, 2000; Guretzky et al., 2016), they may be more tolerant to water level shifts than their lake counterparts, and other factors are likely to be more important to their assembly. For example, our variable “lake basin” may encompass fluctuations in decomposition. Previous work on Lake Sinclair in Michigan, USA, found that shorelines were prone to build-up of large quantities of plant materials and thus very high decomposition rates (Wensink and Tiegs, 2016). While there was no clear evidence of decomposition changing over time along shorelines in our system, this would agree with RF models indicating the importance of changes in bacterial diversity in structuring nematode community diversity, hinting that there may be a connection to decomposition. More work will need to be done to clarify this relationship. Lastly, time alone was important to nematode community diversity. With the most dramatic changes in community composition between years, the effect of time alone on diversity may reflect the transitory nature of the shorelines. Temporal change also relies on the stochastic processes of dispersal and drift (Chave, 2004; Chase and Myers, 2011; Nemergut et al., 2013). As transition zones experience input from two distinct ecosystems (Steibl et al., 2021) (i.e., lake sediments and prairie soils), this not only includes direct dispersal of nematodes to shorelines, but also movement of bacteria, fungi, and microbial eukaryotes, key food sources for various nematodes. In this way, the importance of time to shoreline nematode diversity in the RF models may be reflective of their transient and potentially stochastic nature.
Finally, nematode diversity in the prairie was unique from both the lakes and shorelines being largely driven by potential nematode-nematode interactions. For example, diversity of the bacterial-feeding nematodes was most reliant on diversity of fungal-feeding nematodes rather than other factors. There are two probable reasons for the importance of nematode-nematode interactions, specifically that of fungal-feeding nematodes. First, it is well known that bacterial- and fungal-feeding nematodes alter bacterial and fungal diversity and thus decomposition and nutrient cycling in soils via trophic interactions (Bonaglia et al., 2014). Recently, it has been shown that nematodes may not be generalists as commonly expected but rather have more specialist diets (Estifanos et al., 2013; Xiao et al., 2014), so it is not unlikely that nematodes shape their own abiotic and biotic habitats through feeding. Other organisms have been observed shaping their ecosystems to their benefit and the benefit of similar organisms (Banerjee et al., 2018). For example, plant identity determines the diversity and composition of the rhizobial microbiome (Dagher et al., 2019), which in turn benefits the plant community by buffering them against abiotic stress (Porter et al., 2020; Markalanda et al., 2022). Secondly, fungal-feeding nematodes have been observed feeding on nematode-parasitic fungi (Bae and Knudsen, 2001), potentially keeping their populations at low levels and preventing high levels of parasitism thus benefitting the larger nematode community.
Temporal changes in composition are habitat and taxon-driven
Composition of nematode communities was distinct by habitat type. In the lakes, composition was largely driven by pH and lake level, particularly between 2020 and 2021 when lake level changed the most (Gattoni et al., in press). Typically, a decrease in lake level, as has occurred in the Sandhills, can cause an increase in salt concentration (Senner et al., 2018). Together with decreased diversity in lakes, this may provide additional evidence for a harshening environment affecting nematode community composition. While no studies have reported on the effects of lake boundary shifts on nematode community composition, sea water penetrating coastal lagoon sediments can cause instability in community composition (Netto and Fonseca, 2017). With similar results in this work and historic use of nematode communities to answer questions of sediment disturbance (Schenk et al., 2020; Ridall and Ingels, 2021), differences in composition between years may point to instability in the western Nebraska Sandhill lakes. With large scale droughts and flooding events threatening lakes across the globe (Yao et al., 2023), more research is needed to understand potential shifts in community composition in this climate-sensitive region.
Of the taxa commonly found in the lakes, only two were affected by abiotic variables, climate, basin, or year. The abundance of Epitobrilus, the most common genus of Tobrilidae found predominantly in highly alkaline lakes, was influenced by pH, basin, and year. Abundance of the other genera of Tobrilidae, limited to neutral lakes, was not impacted by the variables in the model. With very little known about the family other than being an ancient aquatic clade commonly found in lake and ocean sediments (Traunspurger, 2000; Tahseen, 2012), our results provide evidence of different niche preferences among members of this nematode family. In this, abiotic environments are directly more important in defining the niche of Epitobrilus, while that of Tobrilus must involve other factors. Potential interactions with other eukaryotes and nematodes may be important, but these relationships need further study. Overall, these results indicate that compositionally, the nematode communities in the lake sediments are relatively stable across time with few individual taxa being significantly affected by changing abiotic or climate-associated conditions.
In the shorelines, composition was similarly distinct among years with pH, basin, and the interaction of year and pH or year and climate structuring all compositional differences. As one of the most disregarded habitat types in community ecology, there are no other studies of nematodes in terrestrial-aquatic transitional zones to compare these results. However, there is evidence of functional changes in shoreline microbial communities as water levels change over time (Shahraki et al., 2021). As discussed previously, with strong ties between nematode and microbial communities, it is imperative to define how the dual impact of change in nematode community composition and microbial community functioning will impact this habitat in the future, specifically regarding decomposition.
As for the abundance of individual shoreline taxa, Epitobrilus increased with pH while Tobrilus decreased with the former most affected by basin and the interaction between pH and climate and climate and year and the later by basin and the interaction between climate and year. With the fluctuation of both climate (i.e., lake water level) and pH over time (Gattoni et al., in press), this pattern supports the idea that different genera and perhaps species within Tobrilidae exhibit unique niche preferences. With more individual taxa varying in abundance over time in the shorelines than lakes in addition to temporal differences in community composition, the shorelines may be less stable than the lakes.
In the prairies, community compositions were most explained by pH, basin, and year. This pattern was consistent across individual genera as well. There was no impact of climate on composition. Whereas the sediment and shorelines are constantly changing, as demonstrated by correlations between pH and time and changes in lake level, the prairies nematode communities may be buffered from climate changes by their associated plant communities. Not only do plants alter soil biota composition (Dagher et al., 2019), but they also play a pivotal role is soil physical stability, biogeochemistry, and moisture retention (Johnston et al., 2020; Morris et al., 2022). The last of these could provide some protection to soil communities, including nematodes, in drought or drought-like conditions if it is not so severe to destabilize the plant communities themselves. However, while this buffering capacity might have protected general patterns of community composition, individual nematodes were still responsive to changes in climate.
In the prairies, nematode genera had unique responses to the variables considered, like previously reported studies. For example, changes in precipitation in grasslands resulted in a reduction of plant ectoparasites whereas endoparasites remained unaffected (Ankrom et al., 2022). Similarly, the two plant ectoparasites in the Sandhills (Mesocriconema and Xiphinema) were distinct with the only significant variable shared being year. Additionally, as Mesocriconema abundance increased over time, that of Xiphinema decreased. Interestingly, these two nematodes are morphologically and behaviorally very different. While Mesocriconema is small, slow, and exhibits very little movement, Xiphinema is large, relatively fast, and more consistently in motion (Taylor, 1936; Wram and Zasada, 2020). In addition, cuticles of the two nematodes also vary (heavy annulated in Mesocriconema and a smooth in Xiphinema). Perhaps, some of these characteristics may define Mesocriconema as a hardier and more resistant to and Xiphinema more susceptible to drought-induced temporal changes in the ecosystem.
Other nematodes increased over time (Acrobeles, Acrobeloides, Ditylenchus, Plectus) and some experienced instability (Pseudacrobeles, Cephalobus). The other major Dorylaimida (Aporcellaimellus) decreased over time similar to Xiphinema, indicting this order may be more susceptible to temporal change. These patterns from individual genera indicate the soil in the prairies may be more disturbed and less stable than the compositional analyses of the whole community indicated. Further research is needed to better identify patterns of disturbance in the grasslands as a potential result of climate change.
Conclusion
Here we hypothesized that drought-driven increase in stress would impact nematode community diversity and composition indicating a potential threat to ecosystem stability in each habitat distinctly. With community diversity and composition from all three habitat types clearly shaped by abiotic, biotic, time, and climate-associated variables uniquely, our major hypothesis was supported. However, our expectation that lakes would be affected by temporal changes in stress the most, followed by shorelines, and prairies, respectively, was incorrect. While nematode diversity of inland lakes was largely driven by increasing pH and lake water level, this was not consistent with community composition and individual taxa, which instead indicated a relatively stable lake sediment community. In contrast to the lakes, nematode diversity in shorelines and prairies were most reliant on biotic interactions, with shoreline also heavily influenced by lake basin and year but independent of abiotic variables or lake water levels. In combination with compositional patterns, the shoreline communities may be transient and less stable than the lakes. Nematode diversity of all individual trophic groups in the prairies pointed to the potential role of nematode-nematode interactions in shaping their communities. Also, prairie community composition was largely dependent on the lone effect of pH, basin, and time, with individual taxa largely influenced by the change in these three variables over time. With the most taxa that varied in relative abundance over time, the prairies may also be unstable. Future studies should focus on specifically quantifying the potential for stability in these ecoregions to determine how they could be a target for future conservation efforts.
Data availability statement
The datasets presented in this study can be found in online repositories. The names of the repository/repositories and accession number(s) can be found below: https://www.ncbi.nlm.nih.gov/genbank/, PRJNA740183.
Ethics statement
The manuscript presents research on animals that do not require ethical approval for their study.
Author contributions
KG: Conceptualization, Formal analysis, Investigation, Visualization, Writing – original draft, Writing – review & editing. EG: Methodology, Supervision, Writing – review & editing. KP: Methodology, Project administration, Resources, Writing – review & editing. TP: Funding acquisition, Methodology, Project administration, Resources, Writing – review & editing. MH: Funding acquisition, Resources, Writing – review & editing. DP: Conceptualization, Formal analysis, Funding acquisition, Investigation, Methodology, Project administration, Resources, Supervision, Writing – original draft, Writing – review & editing.
Funding
The author(s) declare financial support was received for the research, authorship, and/or publication of this article. This project was supported in part by the Nebraska Research Initiative through the University of Nebraska Collaboration Initiative (Sandhills Alkaline Lakes Collaborative Initiative) and the University of Florida.
Acknowledgments
We thank the SALCI team (David Dunigan, Ethan Freese, Jayadra Ghosh, Roger Carlson, Jessica Corman, Mirae Guenther, Daniel Gschwentner, Innocent Byiringiro, Peter Mullin, Abigail Borgmeier, Emma Brinley Buckley, and others) and the Worms et al. Lab (J. Parr McQueen, Lauren Uy, and Ariella Jacobson) for their contributions, as well as Diane Whited from the Flathead Lake Biological Station, University of Montana for assistance with analysis of satellite images.
Conflict of interest
The authors declare that the research was conducted in the absence of any commercial or financial relationships that could be construed as a potential conflict of interest.
Publisher’s note
All claims expressed in this article are solely those of the authors and do not necessarily represent those of their affiliated organizations, or those of the publisher, the editors and the reviewers. Any product that may be evaluated in this article, or claim that may be made by its manufacturer, is not guaranteed or endorsed by the publisher.
Supplementary material
The Supplementary Material for this article can be found online at: https://www.frontiersin.org/articles/10.3389/fevo.2024.1305930/full#supplementary-material
References
Adane Z., Zlotnik V. A., Rossman N. R., Wang T., Nasta P. (2019). Sensitivity of potential groundwater recharge to projected climate change scenarios: A site-specific study in the Nebraska Sand Hills, USA. Water 11, 950. doi: 10.3390/w11050950
Aitchison J., Barceló-Vidal C., Martín-Fernández J. A., Pawlowsky-Glahn V. (2000). Logratio analysis and compositional distance. Math. Geol. 32, 271–275. doi: 10.1023/A:1007529726302
Amaral-Zettler L. A., McCliment E. A., Ducklow H. W., Huse S. M. (2009). A method for studying protistan diversity using massively parallel sequencing of V9 hypervariable regions of small-subunit ribosomal RNA genes. PLoS One 4, e6372. doi: 10.1371/journal.pone.0006372
Ankrom K. E., Franco A. L. C., Fonte S. J., Gherardi L. A., de Tomasel C. M., Wepking C., et al. (2022). Ecological maturity and stability of nematode communities in response to precipitation manipulations in grasslands. Appl. Soil Ecol. 170, 104263. doi: 10.1016/j.apsoil.2021.104263
Apprill A., McNally S., Parsons R., Weber L. (2015). Minor revision to V4 region SSU rRNA 806R gene primer greatly increases detection of SAR11 bacterioplankton. Aquat Microb. Ecol. 75, 129–137. doi: 10.3354/ame01753
Bae Y.-S., Knudsen G. R. (2001). Influence of a fungus-feeding nematode on growth and biocontrol efficacy of Trichoderma harzianum. Phytopathology 91, 301–306. doi: 10.1094/PHYTO.2001.91.3.301
Banerjee S., Schlaeppi K., van der Heijden M. G. A. (2018). Keystone taxa as drivers of microbiome structure and functioning. Nat. Rev. Microbiol. 16, 567–576. doi: 10.1038/s41579-018-0024-1
Bokulich N. A., Subramanian S., Faith J. J., Gevers D., Gordon J. I., Knight R., et al. (2013). Quality-filtering vastly improves diversity estimates from Illumina amplicon sequencing. Nat. Methods 10, 57–59. doi: 10.1038/nmeth.2276
Bonaglia S., Nascimento F. J. A., Bartoli M., Klawonn I., Brüchert V. (2014). Meiofauna increases bacterial denitrification in marine sediments. Nat. Commun. 5, 5133. doi: 10.1038/ncomms6133
Caporaso J. G., Lauber C. L., Walters W. A., Berg-Lyons D., Huntley J., Fierer N., et al. (2012). Ultra-high-throughput microbial community analysis on the Illumina HiSeq and MiSeq platforms. ISME J. 6, 1621–1624. doi: 10.1038/ismej.2012.8
Castillioni K., Wilcox K., Jiang L., Luo Y., Jung C. G., Souza L. (2020). Drought mildly reduces plant dominance in a temperate prairie ecosystem across years. Ecol. Evol. 10, 6702–6713. doi: 10.1002/ece3.6400
Chase J. M., Myers J. A. (2011). Disentangling the importance of ecological niches from stochastic processes across scales. Philos. Trans. R. Soc B: Biol. Sci. 366, 2351–2363. doi: 10.1098/rstb.2011.0063
Chave J. (2004). Neutral theory and community ecology: Neutral theory and community ecology. Ecol. Lett. 7, 241–253. doi: 10.1111/j.1461-0248.2003.00566.x
Creamer R. E., Hannula S. E., Leeuwen J. P. V., Stone D., Rutgers M., Schmelz R. M., et al. (2016). Ecological network analysis reveals the inter-connection between soil biodiversity and ecosystem function as affected by land use across Europe. Appl. Soil Ecol. 97, 112–124. doi: 10.1016/j.apsoil.2015.08.006
Dagher D. J., de la Providencia I. E., Pitre F. E., St-Arnaud M., Hijri M. (2019). Plant identity shaped rhizospheric microbial communities more strongly than bacterial bioaugmentation in petroleum hydrocarbon-polluted sediments. Front. Microbiol. 10. doi: 10.3389/fmicb.2019.02144
De Mesel I., Derycke S., Moens T., van der Gucht K., Vincx M., Swings J. (2004). Top-down impact of bacterivorous nematodes on the bacterial community structure: a microcosm study. Environ. Microbiol. 6, 733–744. doi: 10.1111/j.1462-2920.2004.00610.x
Dini-Andreote F., Stegen J. C., van Elsas J. D., Salles J. F. (2015). Disentangling mechanisms that mediate the balance between stochastic and deterministic processes in microbial succession. PNAS 112 (11). doi: 10.1073/pnas.1414261112
Estifanos T. K., Traunspurger W., Peters L. (2013). Selective feeding in nematodes: a stable isotope analysis of bacteria and algae as food sources for free-living nematodes. Nematology 15, 1–13. doi: 10.1163/156854112X639900
Fargione J., Brown C. S., Tilman D. (2003). Community assembly and invasion: An experimental test of neutral versus niche processes. PNAS 100, 8916–8920. doi: 10.1073/pnas.1033107100
Gattoni K., Gendron E. M. S., McQueen J. P., Powers K., Powers T. O., Harner M. J., et al. (2024). Microbial community diversity is governed by stochasticity and determinism across a habitat gradient regardless of temporal change in biogeochemical properties. (In Press) Community Ecol.
Gattoni K., Gendron E. M. S., Borgmeier A., McQueen J. P., Mullin P. G., Powers K., et al. (2022). Context-dependent role of abiotic and biotic factors structuring nematode communities along two environmental gradients. Mol. Ecol. 31, 3903–3916. doi: 10.1111/mec.16541
Gattoni K., Gendron E. M. S., Sandoval-Ruiz R., Borgemeier A., McQueen J. P., Shepherd R. M., et al. (2023). 18S-nemaBase: curated 18S rRNA database of nematode sequences. J. Nematol. 55, 20230006. doi: 10.2478/jofnem-2023-0006
Gerlach S. A. (1978). Food-chain relationships in subtidal silty sand marine sediments and the role of meiofauna in stimulating bacterial productivity. Oecologia 33, 55–69. doi: 10.1007/BF00376996
Godbold J. A., Calosi P. (2013). Ocean acidification and climate change: advances in ecology and evolution. Philos. Trans. R. Soc B: Biol. Sci. 368, 20120448. doi: 10.1098/rstb.2012.0448
Goodey T. (1963). Soil and freshwater nematodes. 2nd ed. Ed. Goodey J. B. (London: Methuen & Co.), xvi + 544. p.
Grimm V., Wissel C. (1997). Babel, or the ecological stability discussions: an inventory and analysis of terminology and a guide for avoiding confusion. Oecologia 109, 323–334. doi: 10.1007/s004420050090
Guretzky J. A., Dunn C., Hillhouse H. (2016). Long-term Sandhills prairie responses to precipitation, temperature, and cattle stocking rate. Plant Ecol. 217, 969–983. doi: 10.1007/s11258-016-0622-9
Heidemann K., Hennies A., Schakowske J., Blumenberg L., Ruess L., Scheu S., et al. (2014). Free-living nematodes as prey for higher trophic levels of forest soil food webs. Oikos 123, 1199–1211. doi: 10.1111/j.1600-0706.2013.00872.x
Hodda M. (2022). Phylum Nematoda: Trends in species descriptions, the documentation of diversity, systematics, and the species concept. Zootaxa 5114, 290–317. doi: 10.11646/zootaxa.5114.1.2
Houle D., Marty C., Augustin F., Dermont G., Gagnon C. (2020). Impact of climate change on soil hydro-climatic conditions and base cations weathering rates in forested watersheds in eastern Canada. Front. For. Glob. Change. 3. doi: 10.3389/ffgc.2020.535397
Hu W., Schmidt S. K., Sommers P., Darcy J. L., Porazinska D. L. (2021). Multiple-trophic patterns of primary succession following retreat of a high-elevation glacier. Ecosphere 12 (3), e03400. doi: 10.1002/ecs2.3400
Hua E., Sun Y., Zhang Z., He L., Cui C., Mu F. (2019). Effects of reduced seawater pH on nematode community composition and diversity in sandy sediments. Mar. Environ. Res. 150, 104773. doi: 10.1016/j.marenvres.2019.104773
Ji M., Kong W., Yue L., Wang J., Deng Y., Zhu L. (2019). Salinity reduces bacterial diversity, but increases network complexity in Tibetan Plateau lakes. FEMS Microbiol. Ecol. 95 (12), fiz190. doi: 10.1093/femsec/fiz190
Jia R., Fan F., Li L., Qu D. (2022). Temporal response of bacterial community associated Fe(III) reduction to initial pH shift of paddy soils. Agronomy 12, 1304. doi: 10.3390/agronomy12061304
Jiao F., Shi X.-R., Han F.-P., Yuan Z.-Y. (2016). Increasing aridity, temperature and soil pH induce soil C-N-P imbalance in grasslands. Sci. Rep. 6, 19601. doi: 10.1038/srep19601
Jin H., Chang L., Van Kleunen M., Liu Y. (2022). Soil mesofauna may buffer the negative effects of drought on alien plant invasion. J. Ecol. 110, 2332–2342. doi: 10.1111/1365-2745.13950
Johnston M. R., Balster N. J., Thompson A. M. (2020). Vegetation alters soil water drainage and retention of replicate rain gardens. Water 12, 3151. doi: 10.3390/w12113151
Li S., Bush R. T., Mao R., Xiong L., Ye C. (2017). Extreme drought causes distinct water acidification and eutrophication in the Lower Lakes (Lakes Alexandrina and Albert), Australia. J. Hydrol. 544, 133–146. doi: 10.1016/j.jhydrol.2016.11.015
Li X., Chen X., Zhu H., Ren Z., Jiao J., Hu F., et al. (2020). Effects of historical legacies on soil nematode communities are mediated by contemporary environmental conditions. Ecol. Evol. 10, 6732–6740. doi: 10.1002/ece3.6406
Liu Z., Cichocki N., Bonk F., Günther S., Schattenberg F., Harms H., et al. (2018). Ecological stability properties of microbial communities assessed by flow cytometry. MSphere 3, e00564–e00517. doi: 10.1128/mSphere.00564-17
Loope D. B., Swinehart J. (2000). Thinking like a dune field: geologic history in the Nebraska Sand Hills. Gt. Plains Res. 10, 32.
Majdi N., Traunspurger W. (2015). Free-living nematodes in the freshwater food web: a review. J. Nematol. 47, 28–44.
Markalanda S. H., McFadden C. J., Cassidy S. T., Wood C. W. (2022). The soil microbiome increases plant survival and modifies interactions with root endosymbionts in the field. Ecol. Evol. 12 (1), e8283. doi: 10.1002/ece3.8283
Martin M. (2011). Cutadapt removes adapter sequences from high-throughput sequencing reads. EMBnet J. 17, 10–12. doi: 10.14806/ej.17.1.200
Martinez Arbizu P. (2020). pairwiseAdonis: Pairwise multilevel comparison using adonis. R package version 0.4. (Vienna, Austria: The Comprehensive R Archive Network). Available at: https://github.com/pmartinezarbizu/pairwiseAdonis.
McMurdie P. J., Holmes S. (2013). phyloseq: An R package for reproducible interactive analysis and graphics of microbiome census data. PLoS One 8 (4), e61217. doi: 10.1371/journal.pone.0061217
Morris K. A., Saetre P., Norton U., Stark J. M. (2022). Plant community effects on soil moisture and nitrogen cycling in a semi-arid ecosystem. Biogeochemistry 159, 215–232. doi: 10.1007/s10533-022-00922-y
National Drought Mitigation Center. (2023). (University of Nebraska-Lincoln). Available at: https://drought.unl.edu/Home.aspx.
Neher D. A. (2001). Role of nematodes in soil health and their use as indicators. J. Nematol. 33, 161–168.
Nemergut D. R., Schmidt S. K., Fukami T., O’Neill S. P., Bilinski T. M., Stanish L. F., et al. (2013). Patterns and processes of microbial community assembly. Microbiol. Mol. Biol. 77, 342–356. doi: 10.1128/MMBR.00051-12
Netto S. A., Fonseca G. (2017). Regime shifts in coastal lagoons: Evidence from free-living marine nematodes. PLoS One 12, e0172366. doi: 10.1371/journal.pone.0172366
Parada A. E., Needham D. M., Fuhrman J. A. (2016). Every base matters: assessing small subunit rRNA primers for marine microbiomes with mock communities, time series and global field samples: Primers for marine microbiome studies. Environ. Microbiol. 18, 1403–1414. doi: 10.1111/1462-2920.13023
Pascal P.-Y., Dupuy C., Richard P., Rzeznik-Orignac J., Niquil N. (2008). Bacterivory of a mudflat nematode community under different environmental conditions. Mar. Bio. 154, 671–682. doi: 10.1007/s00227-008-0960-9
Porazinska D. L., Bueno De Mesquita C. P., Farrer E. C., Spasojevic M. J., Suding K. N., Schmidt S. K. (2021). Nematode community diversity and function across an alpine landscape undergoing plant colonization of previously unvegetated soils. Soil Bio. Biochem. 161, 108380. doi: 10.1016/j.soilbio.2021.108380
Porazinska D. L., Giblin-Davis R. M., Faller L., Farmerie W., Kanzaki N., Morris K., et al. (2009). Evaluating high-throughput sequencing as a method for metagenomic analysis of nematode diversity. Mol. Ecol. Resour. 9, 1439–1450. doi: 10.1111/j.1755-0998.2009.02611.x
Porazinska D. L., Giblin-Davis R. M., Sung W., Thomas W. K. (2010). Linking operational clustered taxonomic units (OCTUs) from parallel ultra sequencing (PUS) to nematode species. Zootaxa 2427, 55. doi: 10.11646/zootaxa.2427.1.6
Porter S. S., Bantay R., Friel C. A., Garoutte A., Gdanetz K., Ibarreta K., et al. (2020). Beneficial microbes ameliorate abiotic and biotic sources of stress on plants. Funct. Ecol. 34, 2075–2086. doi: 10.1111/1365-2435.13499
Pu H., Yuan Y., Qin L., Liu X. (2023). pH drives differences in bacterial community β-diversity in hydrologically connected lake sediments. Microorganisms 11, 676. doi: 10.3390/microorganisms11030676
Qing X., Bert W. (2019). Family Tylenchidae (Nematoda): An overview and perspectives. Org. Divers. Evol. 19, 391–408. doi: 10.1007/s13127-019-00404-4
Ratzke C., Gore J. (2018). Modifying and reacting to the environmental pH can drive bacterial interactions. PLoS Biol. 16, e2004248. doi: 10.1371/journal.pbio.2004248
Ridall A., Ingels J. (2021). Suitability of free-living marine nematodes as bioindicators: status and future considerations. Front. Mar. Sci. 8. doi: 10.3389/fmars.2021.685327
Rognes T., Flouri T., Nichols B., Quince C., Mahé F. (2016). VSEARCH: a versatile open source tool for metagenomics. PeerJ 4, e2584. doi: 10.7717/peerj.2584
R Core Team. (2019). R: a language and environment for statistical computing. Vienna, Austria: R Foundation for Statistical Computing. Available at: https://www.R-project.org/.
Sánchez-Moreno S., Ferris H. (2018). “Nematode ecology and soil health,” in Plant Parasitic Nematodes in Subtropical and Tropical Agriculture. Eds. Sikora R. A., Coyne D., Hallmann J., Timper P. (Wallingford UK: CAB International), 62–86. doi: 10.1079/9781786391247.0062
Schenk J., Höss S., Brinke M., Kleinbölting N., Brüchner-Hüttemann H., Traunspurger W. (2020). Nematodes as bioindicators of polluted sediments using metabarcoding and microscopic taxonomy. Environ. Int. 143, 105922. doi: 10.1016/j.envint.2020.105922
Senner N. R., Moore J. N., Seager S. T., Dougill S., Kreuz K., Senner S. E. (2018). A salt lake under stress: Relationships among birds, water levels, and invertebrates at a Great Basin saline lake. Biolog. Conservat. 220, 320–329. doi: 10.1016/j.biocon.2018.02.003
Shahraki A. H., Chaganti S. R., Heath D. (2021). Spatio-temporal dynamics of bacterial communities in the shoreline of Laurentian great Lake Erie and Lake St. Clair’s large freshwater ecosystems. BMC Microbiol. 21, 253. doi: 10.1186/s12866-021-02306-y
Steibl S., Sigl R., Bräumer P. E., Clauß V., Goddemeier S., Hamisch S., et al. (2021). Diversity patterns and community structure of the ground-associated macrofauna along the beach-inland transition zone of small tropical islands. Diversity 13, 377. doi: 10.3390/d13080377
Strang D., Aherne J. (2015). Potential influence of climate change on the acid-sensitivity of high-elevation lakes in the Georgia basin, British Columbia. Adv. Meteorol. 2015, 1–11. doi: 10.1155/2015/536892
Tahseen Q. (2012). Nematodes in aquatic environments adaptations and survival strategies. Biodiver. J. 3, 13–40.
Tan W., Wang J., Bai W., Qi J., Chen W. (2020). Soil bacterial diversity correlates with precipitation and soil pH in long-term maize cropping systems. Sci. Rep. 10, 6012. doi: 10.1038/s41598-020-62919-7
Taylor A. L. (1936). The genera and species of the criconematinae, a sub-family of the Anguillulinidae (Nematoda). Trans. Amer. Micr. Soc 55, 391. doi: 10.2307/3222522
Thompson L. R., Sanders J. G., McDonald D., Amir A., Ladau J., Locey K. J., et al. (2017). A communal catalogue reveals Earth’s multiscale microbial diversity. Nature 551, 457–463. doi: 10.1038/nature24621
Traunspurger W. (2000). The biology and ecology of lotic nematodes. Freshw. Biol. 44, 29–45. doi: 10.1046/j.1365-2427.2000.00585.x
Van Den Hoogen J., Geisen S., Routh D., Ferris H., Traunspurger W., Wardle D. A., et al. (2019). Soil nematode abundance and functional group composition at a global scale. Nature 572, 194–198. doi: 10.1038/s41586-019-1418-6
Vellend M. (2010). Conceptual synthesis in community ecology. Q. Rev. Biol. 85, 183–206. doi: 10.1086/652373
Waldrop M. P., Holloway J. M., Smith D. B., Goldhaber M. B., Drenovsky R. E., Scow K. M., et al. (2017). The interacting roles of climate, soils, and plant production on soil microbial communities at a continental scale. Ecology 98, 1957–1967. doi: 10.1002/ecy.1883
Wang S., Dong H., Hou W., Jiang H., Huang Q., Briggs B. R., et al. (2014). Greater temporal changes of sediment microbial community than its waterborne counterpart in Tengchong hot springs, Yunnan Province, China. Sci. Rep. 4, 7479. doi: 10.1038/srep07479
Wei T., Simko V. (2017) R Package “Corrplot”: Visualization of a Correlation Matrix (Version 0.84). Available at: https://github.com/taiyun/corrplot.
Weise L., Ulrich A., Moreano M., Gessler A., E. Kayler Z., Steger K., et al. (2016). Water level changes affect carbon turnover and microbial community composition in lake sediments. FEMS Microbiol. Ecol. 92, fiw035. doi: 10.1093/femsec/fiw035
Wensink S. M., Tiegs S. D. (2016). Shoreline hardening alters freshwater shoreline ecosystems. Freshw. Sci. 35, 764–777. doi: 10.1086/687279
Wram C. L., Zasada I. (2020). Differential response of Meloidogyne, Pratylenchus , Globodera , and Xiphinema species to the nematicide fluazaindolizine. Phytopathology® 110, 2003–2009. doi: 10.1094/PHYTO-05-20-0189-R
Xiao H.-F., Li G., Li D.-M., Hu F., Li H.-X. (2014). Effect of different bacterial-feeding nematode species on soil bacterial numbers, activity, and community composition. Pedosphere 24, 116–124. doi: 10.1016/S1002-0160(13)60086-7
Yao F., Livneh B., Rajagopalan B., Wang J., Crétaux J.-F., Wada Y., et al. (2023). Satellites reveal widespread decline in global lake water storage. Science 380, 743–749. doi: 10.1126/science.abo2812
Yeates G. W., Bongers T., De Goede R. G., Freckman D. W., Georgieva S. S. (1993). Feeding habits in soil nematode families and genera-an outline for soil ecologists. J. Nematol. 25, 315–331.
Zhao S., Liu J.-J., Banerjee S., Zhou N., Zhao Z.-Y., Zhang K., et al. (2018). Soil pH is equally important as salinity in shaping bacterial communities in saline soils under halophytic vegetation. Sci.c Rep. 8, 4550. doi: 10.1038/s41598-018-22788-7
Keywords: biodiversity, community assembly, drought, ecosystem stability, modelling, nematode, Sandhills alkaline lakes
Citation: Gattoni K, Gendron EMS, Powers K, Powers TO, Harner MJ and Porazinska DL (2024) Effects of drought-induced stress on nematode communities in aquatic and terrestrial habitats of the Nebraska Sandhills. Front. Ecol. Evol. 12:1305930. doi: 10.3389/fevo.2024.1305930
Received: 02 October 2023; Accepted: 23 January 2024;
Published: 21 February 2024.
Edited by:
Susana Gomes, University of Aveiro, PortugalReviewed by:
Eustachio Tarasco, University of Bari Aldo Moro, ItalyYudai Kitagami, Mie University, Japan
Ashleigh Smythe, Virginia Military Institute, United States
Copyright © 2024 Gattoni, Gendron, Powers, Powers, Harner and Porazinska. This is an open-access article distributed under the terms of the Creative Commons Attribution License (CC BY). The use, distribution or reproduction in other forums is permitted, provided the original author(s) and the copyright owner(s) are credited and that the original publication in this journal is cited, in accordance with accepted academic practice. No use, distribution or reproduction is permitted which does not comply with these terms.
*Correspondence: Dorota L. Porazinska, ZG9yb3RhbHBAdWZsLmVkdQ==; Kaitlin Gattoni, a2dhdHRvbml1ZmxAZ21haWwuY29t