- 1Ecology and Evolutionary Biology, University of Toronto, Toronto, ON, Canada
- 2Biological Sciences, University of Toronto Scarborough, Scarborough, ON, Canada
- 3Aquatic Research and Monitoring Section, Ontario Ministry of Natural Resources and Forestry, Trent University, Peterborough, ON, Canada
The integration of invasive species into native communities may result in the destabilization of food webs through predation of native prey and competition with native predators. These negative effects are likely to be exacerbated by climate change, increasing the frequency of heat waves, droughts, and high-flow events. Invasive Round Goby (Neogobius melanostomus) and native White Sucker (Catostomus commersonii) were collected from the wild and acclimated to a range of temperatures expected to capture increases in temperature under climate change. In the laboratory, we measured the effects of temperature (19, 22, 25, and 28°C) and velocity gradients (0, 0.15, 0.3, 0.6 m/s at 22 and 25°C) on the prey consumption and foraging behavior of each species. We found that the prey consumption and number of attacks (lunges at prey) of both species increased with temperature but were consistently higher in Round Goby. Velocity had an interactive effect with temperature for prey consumption and foraging behavior, where generally, velocity tolerance for both species was higher at 22°C than at 25°C. White Sucker tolerance to velocity was higher than Round Goby, suggesting that systems with higher velocities may provide a competitive advantage in high-flow environments. Our results stress the importance of interactive experiments when examining the impact of invasive species on native competitors under shifting climatic conditions.
1 Introduction
Invasive species are a significant driver of the decline of native biodiversity (Sala et al., 2000). As predation and competition are driving forces that structure natural communities (Rehage et al., 2005; Mofu et al., 2019), competition between native and invasive species can significantly reduce populations of native species and alter community structure and function (Ricciardi, 2004; Pintor and Sih, 2009). While invasion biology lacks formalized methods for testing invasive species impacts, prey consumption rates and patterns among invaders and trophically similar native species allow for predictions of invader impact (Alexander et al., 2014; Dick et al., 2014). Successful invaders are often characterized by their ability to utilize resources efficiency compared to native species, resulting in prey resource populations vulnerable to severe declines and possible extinctions (Alexander et al., 2014). Methods such as functional response, which measures the prey consumption of a species along a prey abundance gradient, and maximum feeding rate have been proposed to help elucidate, and make predictions about impacts (Dick et al., 2013; Alexander et al., 2014; Dick et al., 2014; Mofu et al., 2019). These methods are based on the assumption that invaders are efficient resource users, thus putting them in direct competition with native fishes (Alexander et al., 2014). Although the impacts of invaders through predation and competition with native species are well documented, these processes are largely context dependent, confounding the ability to make generalized predictions in invasion biology (Ricciardi et al., 2013; Mofu et al., 2019).
Understanding the context-dependencies of invasion are particularly relevant as changing environmental conditions driven by climate change will affect all biological aspects of organisms including physiology, morphology, and behavior that will result in cascading effects that alter the strength of interactions between species and prey resources (Rehage and Blanchard, 2016). Climate change is expected to increase fluctuations in temperature and precipitations increasing the frequency of heat waves, severe storms, droughts, and flooding events that will impact native ecosystems (Rahel and Olden, 2008; Rehage and Blanchard, 2016). As global species distributions are influenced by climatic suitability, climate change will have a major impact on the invasibility of native ecosystems (Diez et al., 2012) and the relative impacts of those successful invaders (Walther et al., 2009). Invasive species typically have the ability to cope with environmental variability and have wide environmental tolerances (Rehage and Blanchard, 2016), enabling them to outperform native species in changing conditions, like those caused by climate change. Therefore, robust experimental methods that include environmental context are needed in invasion studies to improve forecasting ability (Mofu et al., 2019).
Fishes are ectotherms and fundamentally linked to environmental temperatures that governs physiological processes such as metabolism (Morgan et al., 2001; Pörtner and Farrell, 2008; Kuehne et al., 2012; Figueiredo et al., 2019). Higher temperatures increase metabolism, which can lead to higher growth rates if prey consumption also increases. However, once species’ thermal optima are exceeded, thermal stress reduces the ability to feed and reduces growth (Nowicki et al., 2012), diverting energy to maintenance (Viant et al., 2003). In the Laurentian Great Lakes, climate change predictions indicate a temperature increases of 3-8 °C (Zhang et al., 2020), and species feeding rates will likely increase with increased metabolic demands. The relationship between temperature and metabolism differs among species, including native and non-native species, due to difference in evolutionary history (geographical origins) and species-specific physiological tolerances (Sorte, 2013). Climatic conditions shifting to warmer temperatures, often towards the physiological optima of non-native species, will promote increased feeding rates, growth, and reproduction than amplify negative competitive effects (Iacarella et al., 2015; Mofu et al., 2019). While a substantial body of knowledge exists regarding the relationship between temperature and feeding, there is limited understanding of the role that water flow velocity plays in changing feeding rates. For example, in a study by Hazelton and Grossman (2009) Rosyside Dace (Clinostomus funduloides) changed their feeding strategy to cope with the increased energy demands of increased velocity. While this does not link directly to metabolic demands, it demonstrates that velocity gradients may influence feeding ability in fishes through the increased energy expenditure to capture prey. Lack of empirical studies examining the influence of velocity on feeding rates represents a gap in our understanding of climate-change effects on predator-prey interactions. Understanding the relationship between feeding and water velocity may aid in predicting which streams which streams will be colonized by invasive species.
Environmental conditions influence individual behavior, including feeding behavior, and the outcomes of the predator-prey resource interactions (Lienart et al., 2014; Nagelkerken and Munday, 2016; Ren et al., 2020). As temperature increases metabolic rate, fishes increases their time spent foraging (Nowicki et al., 2012; Como et al., 2014; Nagelkerken and Munday, 2016; Scott et al., 2017). While the context dependency of foraging rate and physiology are well known, few studies have explicitly examined the effect of temperature on fish behavior (Theodorou et al., 2012) and feeding behavior of fishes (e.g., Biro et al., 2010; Nowicki et al., 2012; Theodorou et al., 2012). However, it is unclear how such behavioral responses to environmental gradients may influence invasion impacts. As invasive species are often characterized with bold and aggressive traits that allow for establishment and dispersal into novel environments (Pintor and Sih, 2009), shifts in behaviour relating to environmental conditions could not only affect individual fitness, but alter species interactions, ecosystem structure, and function (Scott et al., 2017).
Our study focuses on a native and a non-native fish species that co-occur in Laurentian Great Lakes tributaries of North America. Round Goby (Neogobius melanostomus, Pallas 1814) is an invasive fish species, native to the Ponto-Caspian region of Europe, with life-history characteristics similar to native benthic species, resulting in direct competition for resources such as food and habitat (Janssen and Jude, 2001), with many studies documenting its implication in the decline of native benthic species (Dubs and Corkum, 1996; French and Jude, 2001; Lauer et al., 2004; Balshine et al., 2005). Recent studies have shown a secondary invasion of Round Goby through continued range expansion through tributaries of the Laurentian Great Lakes (Lederer et al., 2006; Poos et al., 2010; Gutowsky and Fox, 2011; Myles-Gonzalez et al., 2015). The expansion of Round Goby into tributaries creates concerns for other species already experiencing declines that may be exacerbated by competition for resources with the Round Goby (Poos et al., 2010). One such species is White Sucker (Catostomus commersonii, Lacepède, 1803), a benthic species native to North America, hypothesized to be an important link in the transfer of offshore benthic energy to nearshore habitats and tributaries of the Laurentian Great Lakes (Flecker et al., 2010; Jones and Mackereth, 2016). White Sucker abundance in the Lake Ontario basin has been in decline, as indicated by the declining size of their spawning runs (Toronto Region Conservation Authority, unpublished data). It is hypothesized that a contributor to this decline is the forage competition between Round Goby juvenile White Sucker in tributaries (Kindree et al., 2023). These two species were chosen to examine the role of environmental context on maximum feeding rate and behaviour due to their hypothesized competitive interactions and difference in environmental tolerances. Round Goby is a generalist species with a wide thermal tolerance, ranging -1-30 °C (Kornis et al., 2012) with an energetic optima of 26°C (Lee and Johnson, 2005), while the optimal temperature range of White Sucker is 17-23 °C (Coker et al., 2001). White Sucker has a greater tolerance for high stream flows and has been found in streams with flows up to 0.9 m/s (Minckley, 1963), whereas Round Goby has a much lower tolerance, up to 0.34 m/s (Marsden et al., 1996). These differences in temperature and velocity tolerances can create competitive advantages that result in species being a superior competitor under optimal conditions, but inferior beyond that range (Taniguchi et al., 1998; Iacarella et al., 2015). Understanding the physiological and behavioural responses along environmental gradients is useful for disentangling the context-dependency of invasive species impacts on native communities (Iacarella et al., 2015).
Here, we experimentally test the interactive effects of elevated temperature and flow velocity on the prey consumption and foraging behaviour of two benthic fishes, invasive Round Goby and native White Sucker. A maximum feeding-rate framework was used to examine the effects of environmental gradients to determine the conditions where invasive species may have the greatest per capita effect on native food webs (Dick et al., 2013; Dick et al., 2014; Iacarella et al., 2015). Specifically, we aim to determine (i) whether temperature outside the thermal optima of these species will result in declines of feeding and foraging behaviour associated with thermal stress, and (ii) the interactive effects of temperature and stream velocity on these metrics. We expect that both species will experience a reduction in feeding and foraging behaviour at temperatures outside of their optimal range. Additionally, as coping with velocity has energetic consequences for species, we expect that as velocity exceeds species optima, feeding and foraging behaviour will decrease.
2 Methods
To simulate climate-change impacts, feeding trials were conducted across thermal and velocity gradients to examine how they influence prey consumption and foraging behaviour of White Sucker and Round Goby. Feeding trials were conducted on each species acclimated to temperatures between 19 and 28°C to examine the influence of temperature. The influence of water velocity on feeding was examined across a gradient of 0 to 0.6 m/s at two testing temperatures of 22 and 25°C. This research was conducted with the approval of the University of Toronto’s Animal Care Committee (Animal Use Protocol# 20012053).
2.1 Fish sampling
Juvenile White Sucker (mean mass = 5.54 ± 2.67 g, mean total length = 85.63 ± 12.81 mm) and adult Round Goby of similar size (mean mass = 16.79 ± 6.50 g, mean total length = 105.55 ± 18.48 mm) were captured from a tributary to Lake Ontario, Highland Creek, in summer 2019 and 2020 using a combination of standard non-lethal sampling gear: minnow traps and backpack electrofishing. Owing to sampling constraints across the different species, total lengths of White Suker were on average smaller than Round Goby. Captured White Sucker and Round Goby were transported in aerated containers to the nearby (~1 km) University of Toronto Scarborough Aquatics Facility.
Fish were separated by species and held in 20-gallon tanks (76.2 cm x 30.5cm x 30.5cm) at a density of 7 individuals per tank. Fishes were held at ambient temperature (22 ± 1.0°C) and the photoperiod was maintained for 12-hour dark: 12-hour light conditions for a minimum acclimation time of two weeks before acclimation to experimental temperatures (Bergstrom and Mensinger, 2009). As these species were collected from the wild and prefer benthic habitats, aquaria were equipped with gravel substrate and tube shelters (PVC pipe) to reduce stress while housed. During the laboratory and temperature acclimation, fish were fed frozen blood worms, frozen Daphnia, and dry pellets once per day ad libitum.
Following the initial acclimation period, tanks housing each species were randomly assigned a treatment temperature and, through the use of heaters and chillers, each tank was brought to its experimental temperature at a rate of change of 1°C per day to reduce the physiological stress of temperature changes on the fishes (Taniguchi et al., 1998). Fishes were held at the testing temperature for a minimum of 14 days prior to feeding trials to allow for thermal acclimation (Taniguchi et al., 1998; Chrétien and Chapman, 2016). To achieve the reduced temperature from ambient (22 ± 1.0°C) to trial temperatures below 22, chillers were used to decrease the temperature and hold. For trials at higher temperatures than ambient (22 ± 1.0°C), heaters were used to increase and maintain the tank temperatures. Fishes were fasted for 48 hours prior to the trials to allow for standardization of hunger levels (Alexander et al., 2014).
2.2 Temperature feeding experiments
Trials to examine the influence of temperature on feeding behaviour and prey consumption were completed at four testing temperatures (19, 22, 25, and 28°C), chosen to encompass temperatures below and exceeding the thermal optima with a minimum of 10 replicates per species. Feeding trials were conducted in 10-gallon aquariums (50.8 cm x 25.4 cm x 30.5 cm), each trial tank was held at the appropriate trial temperature ( ± 0.5°C) using either heaters or chillers. Individual fish were moved from the acclimation tank to the trail tank 24 hours before trails began to reduce the effect of handling stress. Each individual trial tank was surrounded by black-out curtains to reduce the influence of the investigator and fish in adjacent tanks. Following the acclimation period, a fixed number of prey (225 thawed chironomid larvae, Chironomus sp., previously kept frozen, mean total thawed mass 1.60 g ± 0.04SE). This prey abundance was chosen to ensure oversaturation of prey items in the tank by Round Goby and White Sucker [preliminary experiments unpublished, (Reid and Ricciardi, 2021)]. Once chironomids were introduced, feeding behaviour was recorded for 2 hours. Prior to trial initiation, the filters in each treatment aquarium were stopped to prevent the removal of prey items via water filtration, and one aeration stone was provided per tank to ensure adequate dissolved oxygen (DO) across all temperature treatments (mean DO ± SE = 8.47 ± 0.13 mg/L). A camera (Swann™ security camera 1080 p) was mounted above each tank to capture the movements of fish throughout the duration of trial to be scored for feeding behaviour (section 2.4). After each trial was completed, each fish was measured (total length) and weighed. The remaining prey items were collected and counted to determine the number consumed by the fish during the trial period.
2.3 Velocity feeding experiments
Trials to examine the influence of temperature and velocity on feeding behaviour and prey consumption were completed at four testing velocities (0, 0.15, 0.3, 0.6 m/s) at two temperatures (22 and 25°C) with minimum of 6 replicates per species. Feeding trials were conducted in a flow chamber (71.1 cm x 23.4 cm x 21.6 cm) (Figure 1), the flow chamber was held at the appropriate trial temperature ( ± 0.5°C) using a heater. The trial area of the flow chamber was outfitted with 30 cork discs (1.9 cm x 1.9 cm x 1.0 cm) randomly glued across flat rocks to allow for pinning of prey items, pinning the prey items prevented prey displacement during trials. Thirty thawed chironomid larvae, (Chironomus sp., previously kept frozen, mean total thawed mass 0.44 g ± 0.17SE) were counted and weighed (with excess moisture removed) before one was pinned to each cork. Individual fish were placed in the flow chamber for two hours and feeding behaviour was video recorded. The flow chamber was surrounded by black-outs curtain to shield fish from observers and to reduce the influence of the investigator. A camera was mounted above the testing chamber to capture fish activity and feeding behaviour during the trial. Aeration was provided through an air stone in the flow chamber to ensure adequate DO across all temperature treatments (mean DO ± SE = 8.33 ± 0.10 mg/L). Upon completion of the trial time, the fish was removed from the flow chamber and total length and biomass were measured. The remaining prey items were removed from the corks and counted. As much water as possible was removed and the remaining unconsumed prey were weighed.
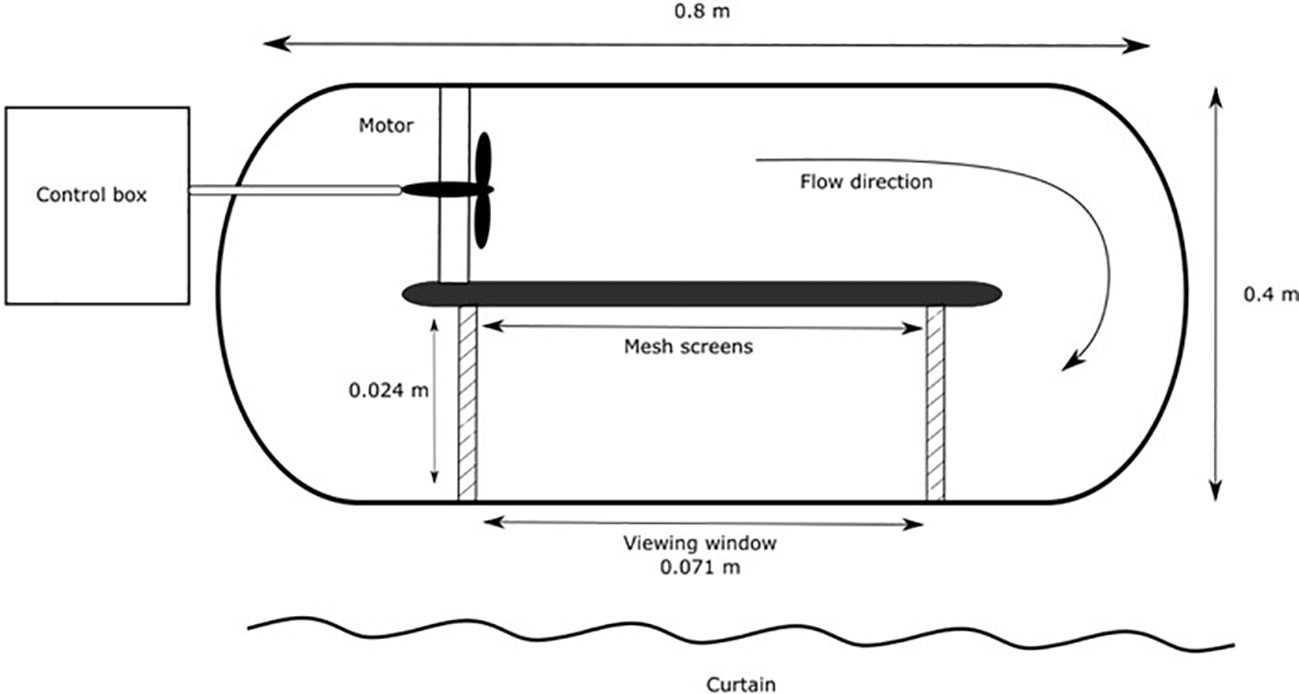
Figure 1 Top-view schematic of the flow chamber, not drawn to scale. Overhead camera was mounted to capture the viewing window where prey items were pinned to cork.
2.4 Foraging behaviour
Each trial video was examined using the event-logging software BORIS (Friard and Gamba, 2016). All behavioural scoring was conducted by one individual to reduce observer-level bias. Each video was scored, and the following variables were extracted from the video: number of attacks, total duration of foraging time, and time until fish began foraging. An attack was defined as a lunge towards or active consumption of one or more prey items. Foraging duration was the sum of foraging events defined as the movement or orientation towards prey items and ended when no movement was recorded for a duration of 5 seconds. The time until foraging event is the duration of time from the start of the trial until the first foraging event (observation of prey consumption).
2.5 Statistical analysis
All data were analyzed using R v. 4.0.3 (R Core Team, 2021). Although the fish were of similar size, we first conducted a series of linear regressions of the dependent variables with body size (mass and total length) to determine if any size variation influenced prey consumption of feeding behaviour metrics. As there was a relationship between size (length or biomass) and each individual response variable (prey consumption and the four behaviors) (p<0.05, in all cases), biomass was included as a covariate in all subsequent analyses.
Generalized additive models (GAMs) were used to determine if the prey consumption and foraging behaviour between species across thermal and velocity gradients as trends were not linear. GAMs allow for non-linear relationships between the response variable and explanatory variables, allowing for both linear and complex additive responses through the use of smoothing curves (Scott et al., 2017). The GAMs were built with poisson distribution and log link function using the mgcv package in R. All models were fit with the REML (restricted maximum likelihood) method. The poisson distribution was chosen because when testing different distributions, we found that the poisson distribution was the best fit for the data.
To examine the effect of temperature, prey consumed (number of prey items consumed) or feeding behaviour were the response variable with smooth functions of acclimation temperature included in all GAMs, with factor-smooth interactions so that smooths could differ by species. This approach allows the model to apply unique smoothing curves for each species. To examine the effect of velocity, prey consumed (number of prey items consumed) or feeding behaviour were the response variable with smooth functions of water velocity included in all GAMs, with factor-smooth interactions so that smooths could differ by the interaction of temperature and species. This approach allows for the model to apply unique smoothing curves for each species and temperature. To allow some complexity in the functions while avoiding data overfitting, the basic dimension was defined as k=4 for each smooth. Species was included as a categorical explanatory variable. Fish mass was included as a smooth term, with factor-smooth interactions that could differ by species. Fish mass (g) and total length (mm) were strongly collinear (concurvity >0.8), leading to the exclusion of total length (mm) from the GAMs to meet the GAM assumption that there is an absence of multicollinearity (see Supplementary Material 1 for model forms for all GAMS).
The best-fitting models were selected using Akaike’s information criterion (AIC) scores, where lower scores were selected to balance model complexity with best fit (see Supplementary Materials 2 for full AIC tables used in model selection), where two models were within 2 AIC values of each other the more complex model was used to capture a larger deviance explained. All models were evaluated using normal Q–Q plots, histograms of the residuals, plots of residuals and responses versus fitted values to evaluate the residuals and test for departure from the model assumptions or other anomalies in the data or in the model fit.
3 Results
A total of 102 temperature-treatment trials were completed across White Sucker (n=51) and Round Goby(n=51) and 113 velocity-gradient trials at two temperatures were completed for White Sucker (n=64) and Round Goby (n=49) (Table 1). Although size matching was attempted, White Sucker on average were significantly smaller than Round Goby included in each trial (Temperature trials: t=10.949, df=101, p<0.0001; Velocity trials: t=14.218, df= 111, p<0.0001; Table 1). Fish mass was included in each model to account for species differences in body size.
3.1 Temperature feeding experiments: prey consumption
Prey consumption across the four testing temperatures varied significantly between species (Figure 2). Round Goby had significant higher prey consumption than White Sucker across all temperatures (p=0.004, Table 2) however, the biomass was significantly larger in the Round Goby (p<0.001), accounting for the difference in prey consumption between species. There was a significant interaction between temperature and species (Round Goby p<0.0001, White Sucker p<0.0001, Table 2). Round Goby and White Sucker had higher prey consumption at 22°C and 25°C, respectively.
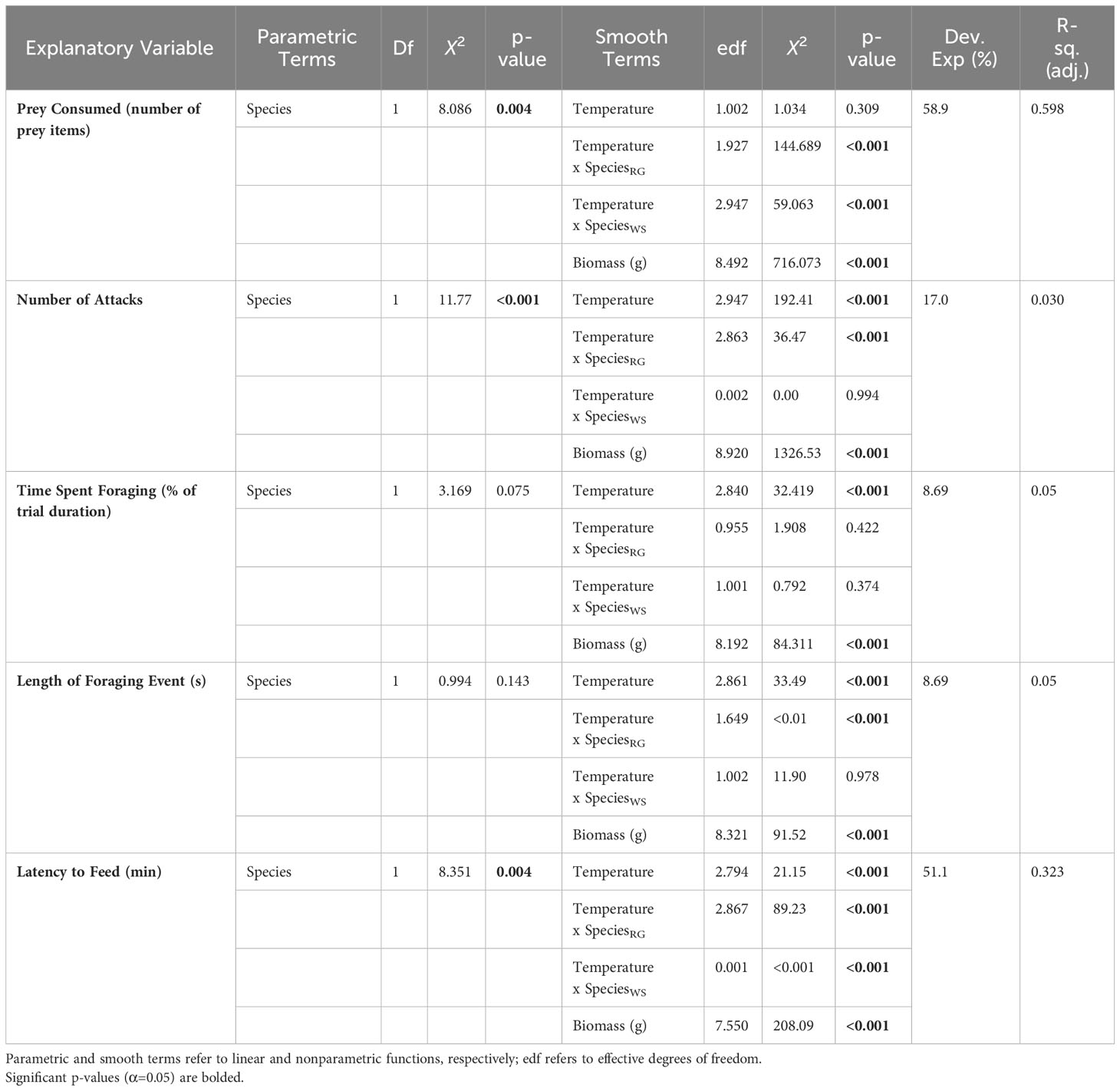
Table 2 Results of generalized additive models of prey consumption and foraging behaviours in White Sucker and Round Goby across the temperature gradient.
3.2 Temperature feeding experiments: foraging behaviour
The number of attacks was significant different between species, with Round Goby attempting more attacks at each temperature than White Sucker (p<0.001, Table 2). Generally, the number of attacks where different across all temperatures examined; however, there was also a significant interaction between Round Goby and temperature. Round Goby acclimated to 19 °C averaged 59% fewer attacks than individuals at acclimated to 22°C, indicating a temperature-driven effect on the attack behaviour of this species (Figure 3A). The number of attacks by White Sucker did not significantly change across temperatures. There was also a significant influence of biomass (p <0.001) on the number of attacks across both species, which was observed across all behavioural metrics measured.
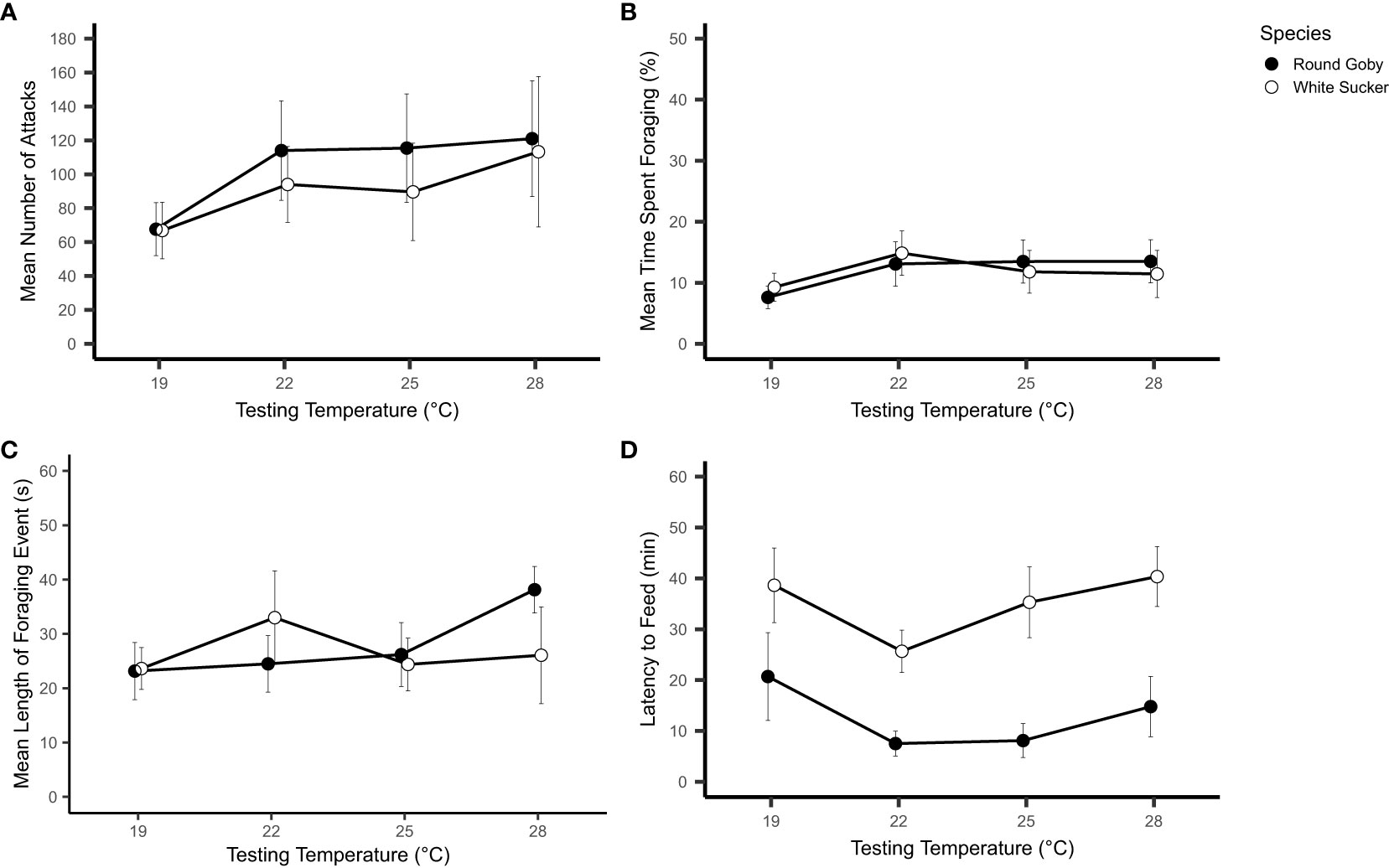
Figure 3 Feeding behaviours (mean ± SE) of White Sucker and Round Goby across a temperature gradient. (A) Number of attacks; (B) percent of trial time spent foraging; (C) average length of foraging event; (D) latency to begin feeding. N.B. Points offset to improve clarity.
The amount of total trial time spent foraging did not significantly differ between species (p=0.075, Table 2) but was significantly different between temperatures for both species (Figure 3B). For example, the time spent foraging by Round Goby was 7.6% (± 1.84 SE) and White Sucker 9.3% (± 2.31 SE) when acclimated to 19 °C. (Figure 3B).
Round Goby and White Sucker did not significantly differ in the length of individual foraging events (Figure 3C). Mean length of each individual foraging period within a trial was 35.69 second (± 13.60 SE) for Round Goby and 30.94 seconds (± 24.01 SE) for White Sucker. Length of foraging events increased significantly for Round Goby with increasing temperatures (p<0.001, Table 2).
Latency to begin feeding showed a significant difference between the species (p=0.004, Table 2). White Sucker took much longer to begin feeding across all temperatures compared to Round Goby (Figure 3D). There was a significant difference across temperatures for both Round Goby and White Sucker, fish acclimated to the 19 and 28°C have a significantly longer latency than fish acclimated to 22 and 25°C (Figure 3D).
3.3 Velocity feeding experiments: prey consumption
There was no significant difference between species (p=0.959) in prey consumption but was significantly higher at 22°C for both species (p<0.001) (Figure 4 and Table 3). Across velocities, there was no significant difference in prey consumption in Round Goby and White Sucker, likely due to the high variability in prey consumption in each treatment and significant influence of biomass (p<0.001). There was a significant interaction between velocity, temperature and species(p<0.05) with higher prey consumption was higher at 22°C than 25°C and decreased as velocity increased. Also, both species consumed fewer prey items at 25°C.
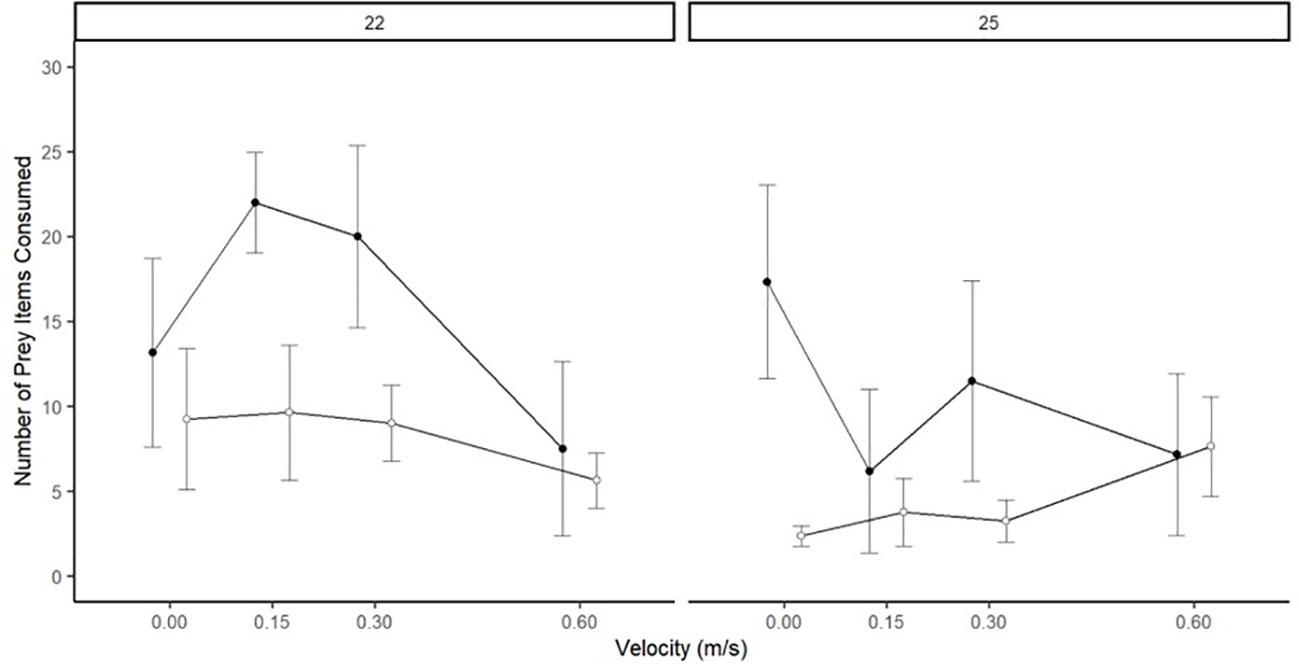
Figure 4 Prey consumption (mean ± SE) of White Sucker and Round Goby across a flow velocity gradient at two testing temperatures. N.B. Points offset to improve clarity.
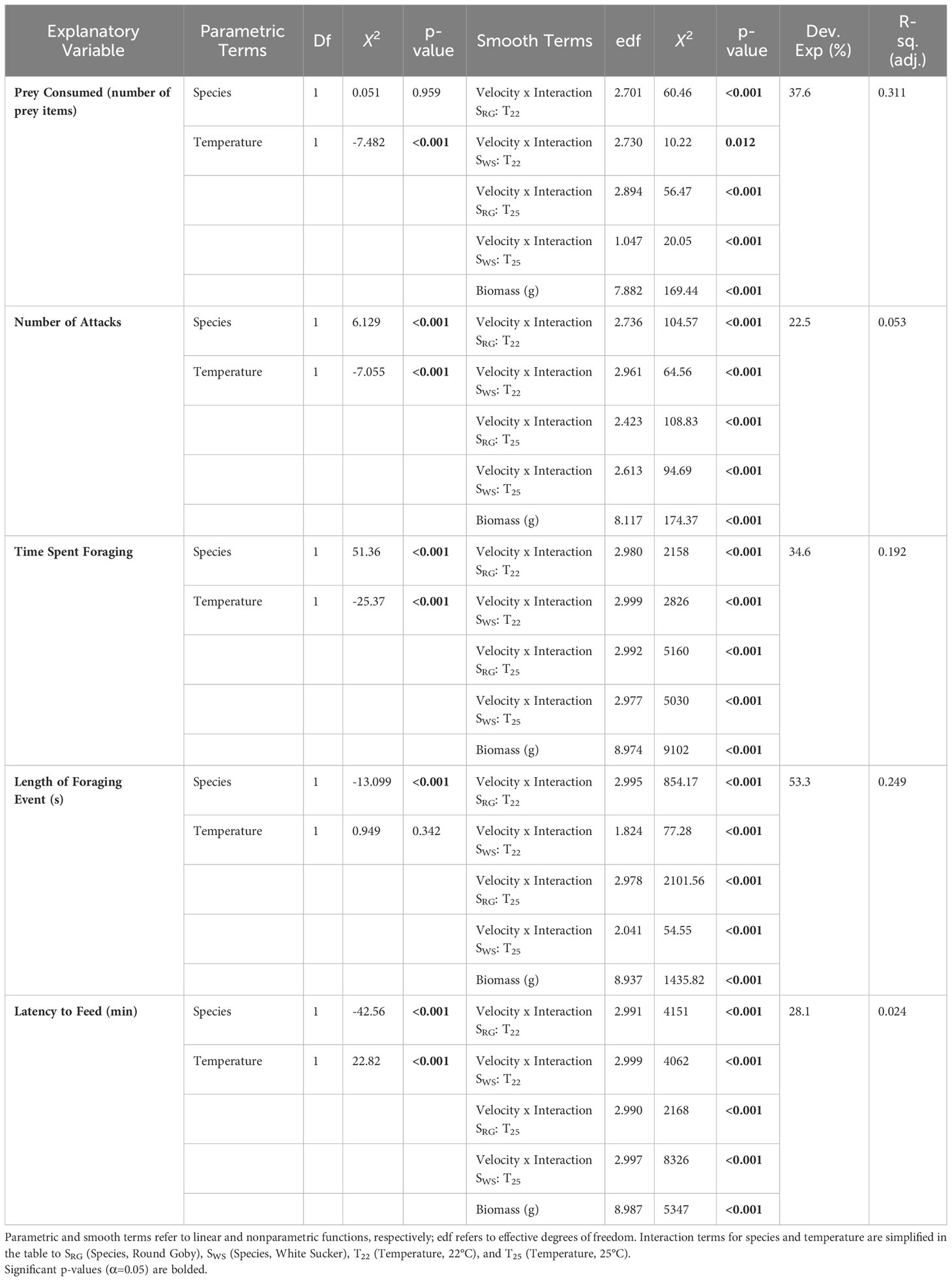
Table 3 Results of generalized additive models of prey consumption and foraging behaviours in White Sucker and Round Goby across the flow velocity gradient at two testing temperatures.
3.4 Velocity feeding experiments: foraging behaviour
The number of attacks performed, regardless of success, was significantly different between the two species (p<0.001), with Round Goby performing more attacks at the lower velocities than White Sucker when acclimated to the higher temperature (25°C) (Table 3 and Figure 5A). However, White Sucker preformed more attacks at flow rates of 0.6m/s. There was an interaction between species, temperature and flow velocity (p<0.001) (Table 3 and Figure 5A). Number of attacks for both species at 22 °C were higher at intermediate velocities of 0.15 m/s and 0.6 m/s, while at 25 °C, more attacks were performed at 0m/s and 0.6m/s. There was also a significant influence of biomass (p <0.001) on the number of attacks across both species, which was observed across all behavioural metrics measured.
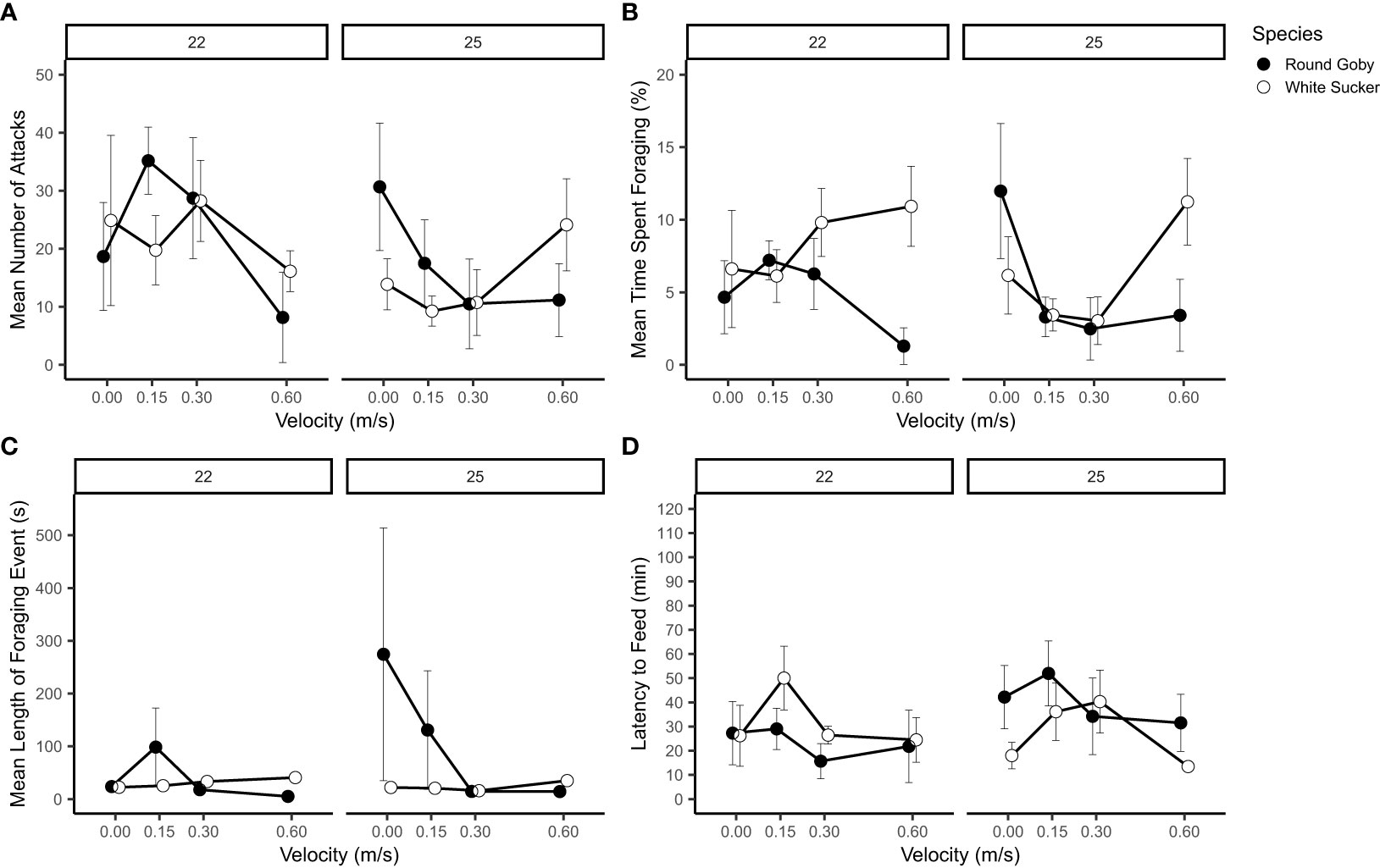
Figure 5 Feeding behaviours (mean ± SE) of White Sucker and Round Goby across a velocity gradient at two temperatures. (A) Number of attacks; (B) percent of trial time spent foraging; (C) average length of foraging event; (D) latency to begin feeding. N.B. Points offset to improve clarity.
The amount of total time spent foraging was significantly different between species (p<0.001) and temperature (p<0.001), with Round Goby spending more time foraging at low flow rates (0 in 25°C and 0.15 m/s in 22°C) and White Sucker spending more time foraging at the higher velocities (0.3 and 0.6 m/s at 22 °C and 0.6 m/s at 25 °C) (Table 3 and Figure 5B). The total time spent foraging varied significantly across flow velocities and had an interaction with species and temperature. The foraging time for Round Goby decreased significantly across both temperatures with velocity.
The duration of individual foraging events differed significantly between species (p<0.001), with Round Goby spending more time foraging at lower velocities than White Sucker (Figure 5C). The time spent foraging also differed between temperatures and velocities. The trends in foraging duration were consistent with other behavioural traits, as there was a significant interaction between temperature and velocity. White Sucker foraging duration varied across temperature, particularly in the highest velocity (0.6m/s), they spent more time foraging. Round Goby spent less time foraging at the higher velocities (0.3 and 0.6 m/s) in both temperatures.
Latency to begin feeding differed significantly between the two species and between the two testing temperatures (p<0.001) (Figure 5D). There were significant interactions between velocity, species and temperature. Flow velocity had a significant interaction with temperature and species; the amount of time until feeding began varied between the temperatures and across the flow velocity gradient. White Sucker taking longer to begin feeding at intermediate velocities (0.15 and 0.3 m/s), while Round Goby took longer to begun feeding at lower velocities (0 and 0.15 m/s).
4 Discussion
Our study found that prey consumption and foraging behaviour changed along environmental gradients of temperature and velocity. Generally, both species had a higher prey consumption within their thermal optimal temperatures with prey consumption decreasing at the thermal extremes of 19 and 28 °C, likely due to metabolic demands and stress shifting in response to temperature. Invasive Round Goby consumed consistently more prey across temperatures than native White Sucker. Within the optimal temperatures of both species across a velocity gradient, we found that prey consumption responded to velocity, with significant differences in the trends between temperatures demonstrating an interactive effect of these environmental variables on prey consumption. Temperature also influenced foraging behaviour in both species, with the number of attacks increasing with increasing temperature. Behavioural responses in both species varied along velocities, exhibiting different patterns of response between temperatures, further providing evidence for context dependency in feeding in both native and invasive species. The results from this comparative study provide evidence for Round Goby superiority in foraging across all temperature treatments, however, this competitive advantage appears to decline in high velocity conditions. The differences observed between native and non-native species prey consumption could suggest that with the continued invasion of Round Goby there would be likely be a shift species that comprise the benthic invertebrate community. Round Goby exhibits aggressive behavioral traits (Dubs and Corkum, 1996; Balshine et al., 2005) and high prey capture efficiencies, we would expect to see more Round Goby given their competitive advantage, and fewer benthic competitors, like the White Sucker.
Total prey consumption increased for both species in intermediate temperatures of 22 and 25 °C before declining, consistent with our expectation prey consumption in these species would decline outside of their thermal optima. The increase in prey consumption in response to increasing temperature is well documented as individuals need to consume additional energy to compensate for metabolic costs associated with higher temperatures (Morgan et al., 2001; Biro et al., 2010; Nowicki et al., 2012). Similar to Englund et al. (2011), our prey consumption curves followed a modal relationship, with optimal temperatures where performance is expected to be highest within the range of species’ thermal optima. We expect that, if fish were acclimated to higher temperatures beyond 28 °C, consumption would continue to decline as thermal stress required fishes to shift energy from foraging to maintenance of critical physiological functions (Viant et al., 2003). The decline in prey consumption with temperature found in this study are consistent with Zanuzzo et al. (2019) as temperature exceeded the thermal optima of Atlantic Cod (Gadus morhua), individual food intake is reduced due to the physiological stress of elevated temperatures. As climate warming increases stream temperatures, species that live near the edge of their thermal limits may be disproportionally affected. White Sucker is a coolwater species with a lower thermal tolerance than Round Goby (Kindree et al. submitted). Unexpectedly, the highest prey consumption rate did not reflect the species thermal optima based on previous studies, which suggested that optima would be reached in White Sucker at 22 °C (n=3, Reutter and Herdendorf, 1976; Spotila et al., 1979; Coker et al., 2001) and in Round Goby near 26 °C (n=78, Lee and Johnson, 2005). In our study, the optima temperatures, those with highest prey consumption reversed, 25 °C for White Sucker and 22 °C for Round Goby. This suggests that White Sucker may have a wider tolerance than previously reported in the literature, which is likely given the restricted sample size of previous thermal optimum work. Consistent with other studies comparing invasive species to native congeners (Rehage et al., 2005), we found that invasive Round Goby prey consumption was 2-4 times higher, depending on temperature, compared to the native White Sucker. While the prey consumption was higher in Round Goby, the impact of higher biomass in the Round Goby tested in our study is likely influencing those patterns. Similar results have been found in native and invasive species comparisons in South Africa, where Smallmouth Bass (Micropterus dolomieu) introduced for angling had a higher prey consumption compared to a functionally similar native species (Alexander et al., 2012). The lack significant differences between species may be related to the similar physiological optimal temperatures and generalist behavioural traits of Round Goby and White Sucker.
As climate change is expected to increase temperatures and alter local hydrology of streams (Rahel and Olden, 2008), our understanding of the context dependency of flow-velocity impacts on feeding are lacking. In addition to climate change, anthropogenic changes to the landscape through urbanization can have significant impacts on the hydrology and temperature of streams. The context dependency of velocity on feeding is particularly relevant to Round Goby invasion into lotic environments from lentic areas where velocity is less important, may result in changes to their feeding ability and behaviour thus affecting the strength of their ecological effects (i.e., competition) on native species. There is a clear interaction between temperature and velocity with prey consumption of both species with the higher prey consumption, on average, occurring across velocities at 22 °C. As expected, Round Goby prey consumption decreased with increasing velocity, although the response of that decline was stronger at 25 °C than 22 °C, suggesting that the compounding effects of temperature on physiology and velocity affecting energy expenditure decreased overall performance. White Sucker did not have a strong response to flow velocity, as expected given their reported higher flow velocity tolerance (Minckley, 1963), and prey consumption surpassed Round Goby at 0.6 m/s (25°C). Similar studies, only examining the role of velocity on foraging, found that juvenile Chinook Salmon (Oncorhynchus tshawytscha) decreased prey capture at velocities exceeding 0.3 m/s (Donofrio et al., 2018). Our results suggest that White Sucker may have competitive advantage in higher flow velocities over Round Goby. Habitats with higher velocities, e.g., riffles, may provide refugia zones for White Sucker that are more resistant to Round Goby invasion due to environmental conditions not suitable for feeding, thus lowering individual fitness. These results are supported by the findings of Kuehne et al. (2012), which suggested that fish living near the physiological limit for one environmental parameters will exhibit a magnified response to additional stressors, such as velocity in our study. A limitation of our study is that we could not acclimate individuals to flow velocities prior to experimental treatment; housing fishes at treatment velocities could allow for the evaluation of prey consumption in response to higher energy demands required in high velocity zones. When fishes exist in areas of high velocity, they will need to expend more energy to feed and maintain position than in areas of low velocity.
Metabolism not only drives prey consumption, but is also linked to increases in feeding activity and shifts in behaviour (Theodorou et al., 2012). While all behavioural metrics measures were affected significantly by temperature, there was not a consistent response (Figure 3). The number of attacks differed significantly between our species, with native White Sucker consistently exhibiting fewer prey capture attempts. In both species, the number of attacks increased with temperature, with the highest number of attacks being performed at 28 °C. This is consistent with existing studies, in which rising temperatures increased metabolism, resulting in higher foraging activity (Theodorou et al., 2012; Como et al., 2014; Pink and Abrahams, 2016; Figueiredo et al., 2019) and aggressive behaviours. For example, Theodorou et al. (2012) found that fishes held that 13 °C were more aggressive than individuals held at 3 °C. Interestingly, while the number of attacks increased with temperature, the time spent foraging did not increase in temperatures greater than 19 °C. Shifting more attacks into the same foraging duration could be a feeding strategy to conserve energy costs in feeding activity as metabolism increases (Como et al., 2014). While this provides evidence for a shift in feeding strategy, i.e., the fishes have a higher rate of attacks, the total prey consumption of both species declined at 28 °C suggesting the prey capture efficiency or the ability to capture prey decreased with thermal stress (Scott et al., 2017). While both species spent equal time foraging, Round Goby were able to consume significantly more prey over time compared to White Sucker. Time spent foraging is often associated with increased risk of predation (Theodorou et al., 2012), as White Sucker consistently consume less prey at all temperatures, they benefit less in terms of energy acquired during risk-taking behaviour. Finally, we found evidence that bold behavioural traits were more readily expressed within optimal thermal temperatures, as expressed by the latency to begin feeding. Both species begin foraging sooner at temperatures within their thermal optima, but Round Goby began foraging 2-4 times faster than White Sucker, exhibiting a generally bolder behaviour across individuals. Studies on personality have shown that fishes in temperatures closer to thermal optima were bolder and commenced feeding three times sooner than fishes held at lower temperatures (Theodorou et al., 2012).
There is a clear interactive effect between temperature and velocity on the response of fish foraging behaviour observed in our study. At 25 °C, there is a clear decline in Round Goby behavioural foraging activity traits of attacks, time spent foraging, and length of foraging intervals with increasing velocity (Figure 4). However, at 22 °C, the highest levels of activity are found at flow velocities of 0.3 m/s, providing further evidence of a thermal optimum existing at 22 °C for Round Goby. At the highest velocity treatment of 0.6 m/s, Round Goby had difficulty maintaining position in the stream and spent more time among refuge substrates and less time foraging. These results could suggest that velocity tolerance of Round Goby may limit its ability to have substantial impacts on native prey abundance in high flow velocity areas (e.g. riffles). The effect of temperature and flow velocity interactions are less clear for White Sucker, although we did find a higher velocity tolerance, as expected. Most studies examining the influence of velocity on the foraging activity of fishes is restricted to drift-feeding salmonid species (e.g. Piccolo et al., 2008; Bozeman and Grossman, 2019a; Bozeman and Grossman, 2019b) rather than active foraging species like Round Goby and White Sucker, limiting comparison of these results due to differences in energy allocation between the foraging strategies. As the first study to investigate the interactive effects of temperature and flow velocity on the behaviour of active foraging species, it is clear that species’ flow velocity tolerance is a good predictor of species performance. Interactive studies regarding elevated temperature and CO2 on fish behaviour suggested that long-term acclimation and adaptation potential may play a role in future responses (Nowicki et al., 2012), although it unlikely these mechanisms will function similarity with velocity as flow tolerance is linked to morphology and physiology (e.g. endurance and swimming ability).
Although we size-matched the individuals in each treatment to the best of our ability, the Round Goby were consistently larger than White Sucker and biomass was a significant covariate in each model. These results are consistent with other studies that found body size affected prey consumption and foraging in native and invasive species (Lee and Johnson, 2005; Rehage et al., 2005; Como et al., 2014). The positive relationship between biomass and foraging (prey consumption and behaviour) suggests an influence of higher metabolic demands. While the species sizes were different, with Round Goby being larger, individuals from both groups were collected from the same stream and time period. Thus, the size disparity would reflect the individuals interacting in natural stream conditions biologically relevant to species-interactions outside laboratory conditions. The higher prey consumption of the Round Goby and more aggressive behaviours, given their larger size, in stream environments could suggest an advantage over the native White Sucker.
The utilization of GAMs in this analysis allowed for the assessment of the non-linear feeding and foraging behaviour trends observed. As with all statistical models, GAMs have limitations, principally the flexibility of GAMs can lead to overfitting of data. Therefore, proper parameterization and model evaluation are necessary to ensure the interpretation is statistically robust. Additionally, low sample sizes or replicates may not capture the full suite of phenotypes to the treatments. However, given replicates in the literature on feeding studies are often not large (often ranging from 3 to 20, Taniguchi et al., 1998; Alexander et al., 2014; Bozeman and Grossman, 2019a; Reid and Ricciardi, 2021) to balance the number of animals needed to draw conclusions and experimental effort. We feel confident that the number of replicates produced in this work are sufficient to demonstrate the difference in prey consumption and feeding behaviour within these two species across environmental gradients.
This study provides an understanding of the possible predator-prey interactions of native and invasive stream fishes under environmental gradients as a proxy for climate change. Further understanding of the impact of Round Goby and interactions with trophically similar species like White Sucker should be explored using a variety of methods including functional response experiments. While the present study presents novel results regarding the interaction of temperature and water velocity using a single prey density, studies have shown that the shape of the feeding response to environmental contexts vary with prey densities (Dick et al., 2013; Alexander et al., 2014; Dick et al., 2014). Additionally, further understanding of the context dependencies related to other environmental factors, such as turbidity, and population characteristics, including density, sex, and sex rations should be explored. It has been shown that turbidity affects the foraging strategies of Brook Trout as they switch to energetically costly methods when turbidity increases (Sweka and Hartman, 2001). The additional foraging requirements to capture prey will have consequences on species energy budgets and, ultimately, fitness.
Our study demonstrates the importance of assessing the interactive effects of ubiquitous stressors (e.g., climate change). Few experimental studies have been carried out to understand behavioural change in co-occurring species under climate change. Our results demonstrate the interactions between environmental factors, species behaviour, and food consumption. We demonstrated that competitive dominance of resource use is skewed in favor of an invasive species as temperature increases. However, the native species appears to be better suited for these conditions at higher velocities. These higher-velocity streams within stream networks might prove to be a refuge for White Sucker and mitigate competition with the less velocity-tolerant Round Goby. Our study enhances the current understanding of the interactive effects of temperature and velocity on the foraging behaviour of native and invasive stream fishes.
Data availability statement
The raw data supporting the conclusions of this article will be made available by the authors, without undue reservation.
Ethics statement
The animal study was approved by University of Toronto Animal Care Committee. The study was conducted in accordance with the local legislation and institutional requirements.
Author contributions
MK: Conceptualization, Data curation, Formal analysis, Investigation, Methodology, Project administration, Validation, Visualization, Writing – original draft, Writing – review & editing. NJ: Conceptualization, Supervision, Writing – review & editing. NM: Conceptualization, Funding acquisition, Resources, Supervision, Writing – review & editing.
Funding
The author(s) declare financial support was received for the research, authorship, and/or publication of this article. This research was supported by Natural Sciences and Engineering Research Council of Canada (NSERC) Strategic Project grant awarded to NM [Grant number (506528)] and a NSERC CREATE Great Lakes Program to MK [Grant number (432269)].
Acknowledgments
The authors are grateful to K. Featherston, K. Brougham, K. Gohil, and E. Quider for assistance in the field and I. Ashtarieh for his help scoring feeding behaviors. We thank the University of Toronto Scarborough Aquatics Facility staff and veterinarians for providing helpful insights into fish husbandry. We also thank the two reviewers whose comments and suggestions greatly improved the manuscript.
Conflict of interest
The authors declare that the research was conducted in the absence of any commercial or financial relationships that could be construed as a potential conflict of interest.
Publisher’s note
All claims expressed in this article are solely those of the authors and do not necessarily represent those of their affiliated organizations, or those of the publisher, the editors and the reviewers. Any product that may be evaluated in this article, or claim that may be made by its manufacturer, is not guaranteed or endorsed by the publisher.
Supplementary material
The Supplementary Material for this article can be found online at: https://www.frontiersin.org/articles/10.3389/fevo.2024.1290083/full#supplementary-material
References
Alexander M. E., Dick J. T. A., O’Connor N. E., Haddaway N. R., Farnsworth K. D. (2012). Functional responses of the intertidal amphipod Echinogammarus marinus: Effects of prey supply, model selection and habitat complexity. Mar. Ecol. Prog. Ser. 468, 191–202. doi: 10.3354/meps09978
Alexander M. E., Dick J. T. A., Weyl O. L. F., Robinson T. B., Richardson D. M. (2014). Existing and emerging high impact invasive species are characterized by higher functional responses than natives. Biol. Lett. 10 (2), 20130946–20130946. doi: 10.1098/rsbl.2013.0946
Balshine S., Verma A., Chant V., Theysmeyer T. (2005). Competitive interactions between round gobies and logperch. J. Great Lakes Res. 31 (1), 68–77. doi: 10.1016/S0380-1330(05)70238-0
Bergstrom M. A., Mensinger A. F. (2009). Interspecific resource competition between the invasive round goby and three native species: logperch, slimy sculpin, and spoonhead sculpin. Trans. Am. Fish. Soc. 138 (5), 1009–1017. doi: 10.1577/T08-095.1
Biro P. A., Beckmann C., Stamps J. A. (2010). Small within-day increases in temperature affects boldness and alters personality in coral reef fish. Proc. R. Soc. B. Biol. Sci. 277 (1678), 71–77. doi: 10.1098/rspb.2009.1346
Bozeman B., Grossman G. (2019a). Foraging behaviour and optimal microhabitat selection in Yukon River Basin nonanadromous dolly varden charr (Salvelinus malma). Ecol. Freshw. Fish. 28 (4), 586–601. doi: 10.1111/eff.12477
Bozeman B., Grossman G. D. (2019b). Mechanics of foraging success and optimal microhabitat selection in alaskan arctic grayling (Thymallus arcticus). Can. J. Fish. Aquat. Sci. 76 (5), 815–830. doi: 10.1139/cjfas-2018-0115
Chrétien E., Chapman L. J. (2016). Tropical fish in a warming world: thermal tolerance of Nile perch Lates niloticus (L.) in Lake Nabugabo, Uganda. Conserv. Physiol. 4 (1), cow062. doi: 10.1093/conphys/cow062
Coker G. A., Portt C. B., Minns C. K. (2001). Morphological and ecological characteristics of Canadian freshwater fishes. Manuscr. Rep. Fish. Aquat. Sci. 2554, iv–89.
Como S., Lefrancois C., Maggi E., Antognarelli F., Dupuy C. (2014). Behavioral responses of juvenile golden gray mullet Liza aurata to changes in coastal temperatures and consequences for benthic food resources. J. Sea Res. 92, 66–73. doi: 10.1016/j.seares.2013.10.004
Dick J. T. A., Alexander M. E., Jeschke J. M., Ricciadi A., MacIsaac H. J., Robinson T. B., et al. (2014). Advancing impact prediction and hypothesis testing in invasion ecology using a comparative functional response approach. Biol. Invasions 16, 735–753. doi: 10.1007/s10530-013-0550-8
Dick J. T. A., Gallagher K., Avlijas S., Clarke H. C., Lewis S. E., Leung S., et al. (2013). Ecological impacts of an invasive predator explained and predicted by comparative functional responses. Biol. Invasions 15, 837–846. doi: 10.1007/s10530-012-0332-8
Diez J. M., D’Antonio C. M., Dukes J. S., Grosholz E. D., Olden J. D., Sorte C. J. B., et al. (2012). Will extreme climatic events facilitate biological invasions? Front. Ecol. Environ. 10 (5), 249–257. doi: 10.1890/110137
Donofrio E., Simon T., Neuswanger J. R., Grossman G. D. (2018). Velocity and dominance affect prey capture and microhabitat selection in juvenile chinook (Oncorhynchus tshawytscha). Environ. Biol. Fishes 101, 609–622. doi: 10.1007/s10641-018-0723-5
Dubs D. O. L., Corkum L. D. (1996). Behavioral interactions between round gobies (Neogobius melanostomus) and mottled sculpins (Cottus bairdi). J. Great Lakes Res. 22 (4), 838–844. doi: 10.1016/S0380-1330(96)71005-5
Englund G., Öhlund G., Hein C. L., Diehl S. (2011). Temperature dependence of the functional response. Ecol. Lett. 14 (9), 914–921. doi: 10.1111/j.1461-0248.2011.01661.x
Figueiredo B. R. S., Calvo C., López-Rodríguez A., Mormul R. P., Teixeira-de Mello F., Benedito E., et al. (2019). Short-term interactive effects of experimental heat waves and turbidity pulses on the foraging success of a subtropical invertivorous fish. Water 11 (10), 1–13. doi: 10.3390/w11102109
Flecker A. S., McIntyre P. B., Moore J. W., Anderson J. T. (2010). Migratory fishes as material and process subsidies in riverine ecosystems. Am. Fish. Soc. Symp 73, 559–592. doi: 10.1899/04-028.1
French J. R. P. III, Jude D. J. (2001). Diets and diet overlap of nonindigenous gobies and small benthic native fishes co-inhabiting the St. Clair River Michigan. J. Great Lakes Res. 27 (3), 300–311. doi: 10.1016/S0380-1330(01)70645-4
Friard O., Gamba M. (2016). BORIS: a free, versatile open-source event-logging software for video/audio coding and live observations. Methods Ecol. Evol. 7 (11), 1325–1330. doi: 10.1111/2041-210X.12584
Gutowsky L. F. G., Fox M. G. (2011). Occupation, body size and sex ratio of round goby (Neogobius melanostomus) in established and newly invaded areas of an Ontario river. Hydrobiologia 671, 27–37. doi: 10.1007/s10750-011-0701-9
Hazelton P. D., Grossman G. D. (2009). The effects of turbidity and an invasive species on foraging success of rosyside dace (Clinostomus funduloides). Freshw. Biol. 54 (9), 1977–1989. doi: 10.1111/j.1365-2427.2009.02248.x
Iacarella J. C., Dick J. T. A., Alexander M. E., Ricciardi A. (2015). Ecological impacts of invasive alien species along temperature gradients: testing the role of environmental matching. Ecol. Appl. 25 (3), 706–716. doi: 10.1890/14-0545.1
Janssen J., Jude D. J. (2001). Recruitment failure of mottled sculpin Cottus bairdi in Calumet Harbor, southern Lake Michigan, induced by the newly introduced round goby Neogobius melanostomus. J. Great Lakes Res. 27 (3), 319–328. doi: 10.1016/S0380-1330(01)70647-8
Jones N. E., Mackereth R. W. (2016). Resource subsidies from adfluvial fishes increase stream productivity. Freshw. Biol. 61 (6), 991–1005. doi: 10.1111/fwb.12762
Kindree M. M., Kindree M. M., Jones N. E., Nicholas. N. E. (2023). Competitive interactions between invasive round goby and native white sucker in streams. Can. J. Fish. Aquat. Sci. 80 (6), 978–991. doi: 10.1139/cjfas-2022-0022
Kornis M. S., Mercado-Silva N., Vander Zanden M. J. (2012). Twenty years of invasion: a review of round goby Neogobius melanostomus biology, spread and ecological implications. J. Fish. Biol. 80 (2), 235–285. doi: 10.1111/j.1095-8649.2011.03157.x
Kuehne L. M., Olden J. D., Duda J. J. (2012). Costs of living for juvenile chinook salmon (Oncorhynchus tshawytscha) in an increasingly warming and invaded world. Can. J. Fish. Aquat. Sci. 69 (10), 1621–1630. doi: 10.1139/f2012-094
Lauer T. E., Allen P. J., McComish T. S. (2004). Changes in mottled sculpin and johnny darter trawl catches after the appearance of round gobies in the Indiana waters of Lake Michigan. Trans. Am. Fish. Soc. 133 (1), 185–189. doi: 10.1577/T02-123
Lederer A., Massart J., Janssen J. (2006). Impact of round gobies (Neogobius melanostomus) on Dreissenids (Dreissena polymorpha and Dreissena bugensis) and the associated macroinvertebrate community across an invasion front. J. Great Lakes Res. 32 (1), 1–10. doi: 10.3394/0380-1330(2006)32[1:IORGNM]2.0.CO;2
Lee V. A., Johnson T. B. (2005). Development of a bioenergetics model for the round goby (Neogobius melanostomus). J. Great Lakes Res. 31 (2), 125–134. doi: 10.1016/S0380-1330(05)70244-6
Lienart G. D. H., Mitchell M. D., Ferrari M. C. O., McCormick M. I. (2014). Temperature and food availability affect risk assessment in an ectotherm. Anim. Behav. 89, 199–204. doi: 10.1016/j.anbehav.2013.12.031
Marsden E. J., Charlebois P., Wolfe K., Jude D. J., Rudnicka S. (1996). The Round Goby (Neogobius melanostomus): a review of European and North American literature. Illinois Nat. Hist. Surv. Aquat. Ecol. Tech. Rep. 96 (10).
Minckley W. L. (1963). The ecology of a spring stream: Doe Run, Meade County, Kentucky. Wildl. Monogr. 11, 3–124.
Mofu L., Cuthbert R. N., Dalu T., Woodford D. J., Wasserman R. J., Dick J. T. A., et al. (2019). Impacts of non-native fishes under a seasonal temperature gradient are forecasted using functional responses and abundances. NeoBiota 49, 57–75. doi: 10.3897/neobiota.49.34986
Morgan I. J., Macdonald D. G., Wood C. M. (2001). The cost of living for freshwater fish in a warmer, more polluted world. Glob. Chang Biol. 7 (4), 345–355. doi: 10.1046/j.1365-2486.2001.00424.x
Myles-Gonzalez E., Burness G., Yavno S., Rooke A., Fox M. G. (2015). To boldly go where no goby has gone before: boldness, dispersal tendency, and metabolism at the invasion front. Behav. Ecol. 26 (4), 1083–1090. doi: 10.1093/beheco/arv050
Nagelkerken I., Munday P. L. (2016). Animal behaviour shapes the ecological effects of ocean acidification and warming: moving from individual to community-level responses. Glob. Chang Biol. 22 (3), 974–989. doi: 10.1111/gcb.13167
Nowicki J. P., Miller G. M., Munday P. L. (2012). Interactive effects of elevated temperature and CO2 on foraging behavior of juvenile coral reef fish. J. Exp. Mar. Bio Ecol. 412, 46–51. doi: 10.1016/j.jembe.2011.10.020
Piccolo J. J., Hughes N. F., Bryant M. D. (2008). Water velocity influences prey detection and capture by drift-feeding juvenile coho salmon (Oncorhynchus kisutch) and steelhead (Oncorhynchus mykiss irideus). Can. J. Fish. Aquat. Sci. 65 (2), 266–275. doi: 10.1139/F07-172
Pink M., Abrahams M. V. (2016). Temperature and its impact on predation risk within aquatic ecosystems. Can. J. Fish. Aquat. Sci. 73 (6), 869–876. doi: 10.1139/cjfas-2015-0302
Pintor L. M., Sih A. (2009). Differences in growth and foraging behavior of native and introduced populations of an invasive crayfish. Biol. Invasions 11, 1895–1902. doi: 10.1007/s10530-008-9367-2
Poos M., Dextrase A. J., Schwalb A. N., Ackerman J. D. (2010). Secondary invasion of the round goby into high diversity Great Lakes tributaries and species at risk hotspots: potential new concerns for endangered freshwater species. Biol. Invasions 12, 1269–1284. doi: 10.1007/s10530-009-9545-x
Pörtner H. O., Farrell A. P. (2008). Physiology and climate change. Science 322 (5902), 690–692. doi: 10.1126/science.1163156
R Core Team (2021). R: A language and environment for statistical computing. R Foundation for Statistical Computing, Vienna, Austria. Available at: https://www.R-project.org/.
Rahel F. J., Olden J. D. (2008). Assessing the effects of climate change on aquatic invasive species. Conserv. Biol. 22 (3), 521–533. doi: 10.1111/j.1523-1739.2008.00950.x
Rehage J. S., Barnett B. K., Sih A. (2005). Foraging behaviour and invasiveness: do invasive Gambusia exhibit higher feeding rates and broader diets than their noninvasive relatives? Ecol. Freshw. Fish. 14 (4), 352–360. doi: 10.1111/j.1600-0633.2005.00109.x
Rehage J. S., Blanchard J. R. (2016). What can we expect from climate change for species invasions? Fisheries 41 (7), 405–407. doi: 10.1080/03632415.2016.1180287
Reid H. B., Ricciardi A. (2021). Ecological responses to elevated water temperatures across invasive populations of the round goby (Neogobius melanostomus) in the Great Lakes basin. Can. J. Fish. Aquat. Sci. 79 (2), 277–288. doi: 10.1139/cjfas-2021-0141
Ren Y., Xie D., Li B., Lui Y., Hu S., Liu H., et al. (2020). Influence of water temperature, habitat complexity and light on the predatory performance of the dark sleeper Odontobutis potamophila (Günther 1861). J. Freshw. Ecol. 35 (1), 367–378. doi: 10.1080/02705060.2020.1816227
Reutter J. M., Herdendorf C. E. (1976). Thermal discharge from a nuclear power plant: predicted effects on Lake Erie fish. Ohio J. Sci. 76, 39–45.
Ricciardi A. (2004). Assessing species invasions as a cause of extinction. Trends Ecol. Evol. 19 (12), 619–620. doi: 10.1016/j.tree.2004.09.021
Ricciardi A., Hoopes M. F., Marchetti M. P., Lockwood J. L. (2013). Progress toward understanding the ecological impacts of nonnative species. Ecol. Monogr. 83 (3), 263–282. doi: 10.1890/13-0183.1
Sala O. E., Stuart Chapin F. I. I. I., Armesto J. J., Berlow E., Bloomfield J., Dirzo R., et al. (2000). Global biodiversity scenarios for the year 2100. Science 287 (5459), 1770–1775. doi: 10.1126/science.287.5459.1770
Scott M., Heupel M., Tobin A., Pratchett M. (2017). A large predatory reef fish species moderates feeding and activity patterns in response to seasonal and latitudinal temperature variation. Sci. Rep. 7, 1–9. doi: 10.1038/s41598-017-13277-4
Sorte C. J. B. (2013). Predicting persistence in a changing climate: Flow direction and limitations to redistribution. Oikos 122 (2), 161–170. doi: 10.1111/j.1600-0706.2012.00066.x
Spotila J. R., Terpin K. M., Koons R. R., Bonati R. L. (1979). Temperature requirements of fishes from eastern Lake Erie and the upper Niagara River: a review of the literature. Envir. Biol. Fish. 4, 281–307. doi: 10.1007/BF00005485
Sweka J. A., Hartman K. J. (2001). Effects of turbidity on prey consumption and growth in brook trout and implications for bioenergetics modeling. Can. J. Fish. Aquat. Sci. 58 (2), 386–393. doi: 10.1139/cjfas-58-2-386
Taniguchi Y., Rahel F. J., Novinger D. C., Gerow K. G. (1998). Temperature mediation of competitive interactions among three fish species that replace each other along longitudinal stream gradients. Can. J. Fish. Aquat. Sci. 55 (8), 1894–1901. doi: 10.1139/f98-072
Theodorou P., Olafsdóttir G.Á., Snorrason S. S. (2012). Reaching the limit: constrained behavioural flexibility of juvenile Atlantic cod (Gadus morhua) at current coastal temperatures. J. Exp. Mar. Bio Ecol. 413, 192–197. doi: 10.1016/j.jembe.2011.12.009
Viant M. R., Werner I., Rosenblum E. S., Gantner A. S., Tjeerdema R. S., Johnson M. K. (2003). Correlation between heat-shock protein induction and reduced metabolic condition in juvenile steelhead trout (Oncorhynchus mykiss) chronically exposed to elevated temperature. Fish. Physiol. Biochem. 29, 159–171. doi: 10.1023/B:FISH.0000035938.92027.81
Walther G. R., Roques A., Hulme P. E., Sykes M. T., Pyšek P., Kühn I., et al. (2009). Alien species in a warmer world: risks and opportunities. Trends Ecol. Evol. 24 (12), 686–693. doi: 10.1016/j.tree.2009.06.008
Zanuzzo F. S., Bailey J. A., Garber A. F., Gamperl A. K. (2019). The acute and incremental thermal tolerance of Atlantic cod (Gadus morhua) families under normoxia and mild hypoxia. Comp. Biochem. Physiol. Part A. 233, 30–38. doi: 10.1016/j.cbpa.2019.03.020
Keywords: foraging behavior, climate change, resource use, invasive species, Round Goby (Neogobius melanostomus), White Sucker (Catostomus commersonii)
Citation: Kindree MM, Jones NE and Mandrak NE (2024) Interactive effects of temperature and velocity on the feeding behavior of competing native and invasive stream fishes. Front. Ecol. Evol. 12:1290083. doi: 10.3389/fevo.2024.1290083
Received: 06 September 2023; Accepted: 04 January 2024;
Published: 23 January 2024.
Edited by:
Congyan Wang, Jiangsu University, ChinaReviewed by:
Sukran Yalcin Ozdilek, Çanakkale Onsekiz Mart University, TürkiyeFraser John Combe, Ceva Animal Health, LLC, United States
Copyright © 2024 Kindree, Jones and Mandrak. This is an open-access article distributed under the terms of the Creative Commons Attribution License (CC BY). The use, distribution or reproduction in other forums is permitted, provided the original author(s) and the copyright owner(s) are credited and that the original publication in this journal is cited, in accordance with accepted academic practice. No use, distribution or reproduction is permitted which does not comply with these terms.
*Correspondence: Meagan M. Kindree, meagan.kindree@gmail.com