- 1U.S. Geological Survey, Forest and Rangeland Ecosystem Science Center, Olympic Field Station, Port Angeles, WA, United States
- 2Lower Elwha Klallam Tribe, Natural Resources, Port Angeles, WA, United States
- 3Olympic National Park, National Park Service, Port Angeles, WA, United States
Terrestrial wildlife species are important yet often overlooked taxa in the recovery of ecosystems following dam removal. Their presence can shape ecosystem recovery, signal restoration of ecosystem function, and influence food web dynamics and nutrient transfer. We used camera traps to examine seasonal use of two former reservoir beds and an upstream reference reach by the mammalian community following the removal of two large dams on the Elwha River, Washington, USA. For certain taxa, we compared current species use to data collected prior to dam removal. Camera traps revealed use by at least fifteen mammal species, including but not limited to American black bear (Ursus americanus), Columbian black-tailed deer (Odocoileus hemionus columbianus), Roosevelt elk (Cervus elaphus roosevelti), puma (Puma concolor), coyotes (Canis latrans), bobcats (Lynx rufus), and snowshoe hares (Lepus americanus). Coyotes were found mostly lower in the watershed outside the Olympic National Park boundary, while other species were distributed throughout the restoration area. We did not see major differences in species composition between the restoration areas and the upstream reference reach, though number of detections across study reaches differed for most species. Unlike previous findings, black bears were observed across all seasons in this study, suggesting a shift in seasonal use since dam removal. Full restoration of the terrestrial wildlife community could take decades to unfold, but early patterns demonstrate rapid establishment and use by wildlife on new riparian surfaces that are expected to continue to evolve with restoration of fish and vegetation communities.
1 Introduction
Opportunities to study large ecosystem restoration are rare and have been limited primarily to studies conducted after large-scale environmental perturbations like volcanic eruptions (Major et al., 2009), forest fires (Vallejo and Alloza, 2015; Alayan et al., 2022; Souza-Alonso et al., 2022), or long-term ecological degradation like occurred in the Florida Everglades (Doren et al., 2008). Dam removals are a relatively new but critical tool in ecological restoration efforts (Hart and Poff, 2002; O’Connor et al., 2015), and provide invaluable opportunities for monitoring ecosystem recovery over large spatial extents and long temporal scales. The increasing frequency of dam removals, particularly large dams, has amplified interest in comprehensive ecological monitoring and research programs to gauge the success of ecosystem restoration after these large-scale projects (Babbitt, 2002; Bellmore et al., 2016). While research and monitoring programs following dam removals are beginning to increase, research to date has been more focused on the physical or abiotic processes of dam removal than biotic restoration (Bellmore et al., 2016). Furthermore, ecological studies have focused primarily on aquatic biodiversity, with very little work on terrestrial vegetation and wildlife (Bellmore et al., 2016). To achieve restoration of ecosystem function following dam removal, restoration ecologists would benefit from understanding terrestrial and aquatic ecological processes as they relate to changes in the physical environment (Bellmore et al., 2016). Here, we focus on terrestrial wildlife as a facet of ecosystem restoration following large dam removal.
The removal of two large dams on the Elwha River, Washington, USA between 2011 and 2014 provided an unprecedented opportunity to understand how terrestrial wildlife both respond to and interact with the restoration process following dam removal (McCaffery et al., 2018). Removal of the Elwha dams reconnected anadromous fish to the upper reaches of this 72-km river after nearly 100 years of impoundment that restricted their movements to the lower 7.9 km of the river (Pess et al., 2008). Prior to deconstruction, the two dams inundated nearly 324 hectares of land (Department of the Interior, 1994) and trapped more than 19 million cubic meters of sediment (Bountry et al., 2010; Warrick et al., 2012). In the years since dam removal, eight species of anadromous fish have passed above the former dam sites (Duda et al., 2021) and nearly 324 hectares of land in two former reservoir beds have been restored through revegetation efforts (Chenoweth et al., 2022). Restoration has added habitat for terrestrial wildlife and reconnected nutrient linkages from the sea to the upper reaches of the watershed. For terrestrial wildlife, changes in fish availability and the creation and restoration of floodplain habitats may lead to changes in species distribution and seasonal use of the watershed, as well as increased wildlife use in the restored former reservoirs.
In addition to responding to new habitat, wildlife can play a large role in restoring ecosystem functions following dam removal (McCaffery et al., 2018, McCaffery et al., 2020). Wildlife species modify habitats, alter community structure, and shift ecosystem dynamics over short and long time scales (Naiman, 1988). For example, several species in terrestrial mammalian and avian communities may provide beneficial services in the form of seed dispersal (Willson, 1993; Wunderle, 1997; McLaughlin, 2013; Albert et al., 2015) and nutrient transport (Hobbs, 1996; Seagle, 2003; Helfield and Naiman, 2006; Quinn et al., 2009), while others could influence plant reestablishment, mostly through browsing planted seedlings (Ostfield et al., 1997; Gill and Beardall, 2001), seed predation (e.g., Bricker et al., 2010), or stripping woody stems of their bark (Ostfield et al., 1997). Herbivory by Columbian black-tailed deer (Odocoileus hemionus columbianus) and Roosevelt elk (Cervus elaphus roosevelti) has the potential to be an important driver of plant successional development (Schreiner et al., 1996; Horsley et al., 2003; Woodward et al., 2021). In the Elwha restoration area, Roosevelt elk demonstrated a strong initial influence on woody plants in the restoration zone through browsing activity (McCaffery et al., 2020). Further, species as varied as American black bears (Ursus americanus), weasels (Mustela spp.), and raccoons (Procyon lotor) may distribute marine-derived nutrients in the form of salmon (Oncorhynchus spp.) carcasses throughout early successional forests, providing nutrients important for plant establishment (e.g., Cederholm et al., 1989; Helfield and Naiman, 2006; Quinn et al., 2009). Finally, beavers (Castor canadensis) may provide beneficial services by constructing small dams in tributary or side-channel habitats, impounding water and increasing habitat complexity for fish, mammals, and birds (Leidholt-Bruner et al., 1992; Pollock et al., 2004, Pollock et al., 2015).
A key step to understanding how wildlife could interact with the restoration process is to describe patterns and drivers of distribution and activity of these species over time. We used remote cameras to document mammalian wildlife use of the two former reservoir beds and a reference upstream river valley 10 years after dam removal on the Elwha River in Washington, USA. Here, we examine seasonal use of the restoration sites by a suite of mammalian species in comparison to the reference reach over a two-year period. We expected to see use by a diversity of mammalian species, with variation in activity among the seasons of the year as well as across the three different study reaches (i.e., the restoring floodplains above each former dam location and the reference upstream valley). Specifically, we predicted that Roosevelt elk and American black bear use would be higher in the reference valley than in the restoration areas and would show strong seasonal patterns. We expected bear use to be highest in the spring but consistent throughout the summer, with potential for seeing use in the fall and winter coincident with returning salmon. We expected that Columbian black-tailed deer would be commonly detected, but again with seasonal and study reach differences. For these and other taxa, our results provide a baseline with which to compare ongoing restoration of these dynamic landscapes while providing initial insights into how patterns of wildlife distribution and use have changed since dam removal.
2 Materials and methods
2.1 Study area
The Elwha River (Washington, USA) flows from its headwaters in Olympic National Park to where it empties into the Strait of Juan de Fuca (Figure 1). The region has a maritime climate characterized by cool, wet winters and dry summers. We examined wildlife presence in three distinct areas (hereafter ‘study reaches’) along the Elwha River (Figure 1): 1) within the floodplain of the former reservoir Lake Aldwell (60 m elevation; hereafter “Aldwell”); 2) within the floodplain of the former reservoir Lake Mills (181 m elevation; hereafter “Mills”); and 3) within Geyser Valley, a reference reach located approximately three km upstream of Mills (235 m elevation; hereafter “Geyser”). We selected Geyser as a reference reach to represent a mature riparian system that was not affected by dam removal activities yet is still relatively low in elevation in the watershed. The reference reach was defined by elevation contours that approximated the water level of the former Mills reservoir.
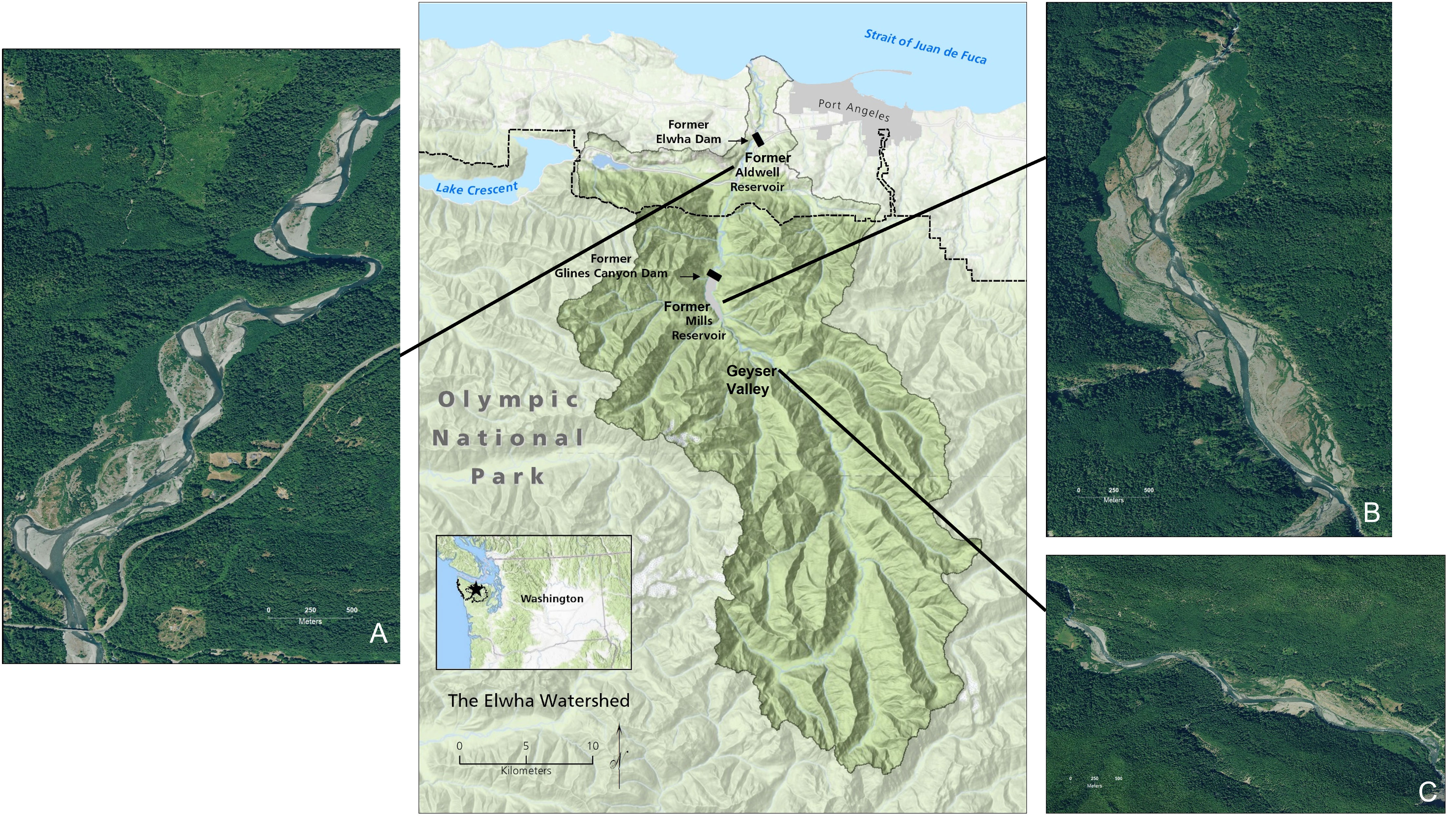
Figure 1 Map of the Elwha River watershed, Washington State, USA. The small inset map shows the location of the Elwha River on the Olympic Peninsula (dashed and dotted lines indicate the boundary of Olympic National Park). The central watershed map shows the locations of the three study reaches along the Elwha River, with zoomed-in imagery of the former (A) Aldwell and (B) Mills reservoirs, as well as (C) Geyser Valley, an upstream reference reach. Base map credit: R. Hoffman, Olympic National Park. Aerial photo credit: A. Ritchie, U.S. Geological Survey.
Vegetation and landform characteristics of the revegetating reservoir beds have been described previously (Chenoweth et al., 2022) and in this special issue (Shafroth et al., 2024). Vegetation in the reference reach was dominated by stands of pioneering willow, red alder (Alnus rubra), and black cottonwood (Populus balsamifera var. trichocarpa) communities on the active floodplain and mature mixed conifer/hardwood or conifer stands comprising Douglas fir (Pseudotsuga menziesii), western hemlock (Tsuga heterophylla), and bigleaf maple (Acer macrophyllum) on the older alluvial terraces and sidehills (McCaffery et al., 2020).
2.2 Sampling design
From July 2021 to July 2023, we installed and monitored 10 remote game cameras (Browning ProDCL) in each study reach for a total of 30 cameras over a combined 17,037 trap-nights. To determine camera placement, we used ArcGIS to create a systematic grid of points spaced at 200-meter intervals across each of the three study reaches (Aldwell, Mills, and Geyser). Every second grid point was removed sequentially until ten points remained in each study reach. In the field, we traveled to each GPS coordinate and selected a suitable site within 25–50 meters of the randomly generated grid point, aiming for a site where the camera’s view was unobstructed by vegetation. We limited site clearing where possible to leave native vegetation intact but removed light vegetation to reduce false triggers during wind events. We used webbing and cable locks to secure a metal lock box to a sturdy tree at an average height of 35 cm and no more than 0.6 m from the ground. When a sturdy tree was not available, such as on open gravel bars, we positioned rebar stakes into the substrate and attached cameras using heavy-gauge wire. We secured cameras within lock boxes to reduce thefts and limit damage caused by black bears. Each camera had a 42° wide angle of detection; within that range, we used a range finder to record six distance measurements (every 7°) to calculate the camera’s viewshed, which varied based on the size of the clearing and proximity of surrounding vegetation. Because our aim was to document presence of all species passively rather than target or attract certain species, and because bait influences detection of some species positively and some negatively (Holinda et al., 2020; Dart et al., 2022; Barcelos et al., 2023), we used unbaited cameras at all sites.
We equipped each camera with six lithium batteries and either a 32, 64, or 128 GB SD card depending on how frequently we checked cameras, with larger SD cards being checked less frequently. At the Aldwell and Mills stations, we visited cameras every two to three months to switch out SD cards and check battery life. Because of the logistical constraints of accessing the more remote Geyser Valley study reach, we checked those cameras every two to nine months. We set cameras on a hybrid mode of motion trigger and time-lapse. Motion trigger images had a one second delay. Time-lapse images were taken to ensure camera functionality throughout each survey period and consisted of two images captured each day—one hour after local sunrise and one hour before sunset. Time-lapse images were not included in the analyses.
We recorded general habitat characteristics associated with each camera station in the field while setting up our camera stations. We characterized the dominant overstory tree species and the physiographic description of each sampling site; for example, gravel bar, remnant alluvial terrace, or sloped valley wall. We later used GIS to measure the distance of each sampling station from the shoreline of the former reservoir.
2.3 Image identification and data analysis
We used both manual and machine learning models for image classification. We performed manual classifications using Camera Trap File Manager (CTFM), a Panthera-derived software application (http://www.pantheracameratrap.org, Version 2.1.18, accessed 02/2023). Machine learning programs included both PantheraIDS (http://pantheraids.org, accessed 02/2023) and Wildlife Insights (https://wildlifeinsights.org, accessed 06/2023). We verified all images classified by machine learning programs for accuracy. We extracted metadata, including date and time stamps associated with each image, which we then used to build a database.
We calculated the difference in time stamps between each motion-triggered image and the image immediately preceding it of the same species at the same camera. To reduce temporal correlation between sequential images, we retained the first image in each series and removed all subsequent images that were captured within 30 minutes of the immediately preceding image. Thus, for an image to be retained it had to be at least 30 minutes apart from other images of the same species at the same camera. We refer to images retained by this process as independent detection events. We chose a cutoff of 30 minutes based on an examination of our data showing that the number of images excluded would change minimally (by 1% or less) if we increased the cutoff to values greater than 30 minutes. Further, 30 minutes is commonly used in camera studies for rating photos as independent events (O'Brien et al., 2003; Burton et al., 2015, Iannarilli et al., 2021, Ayars et al., 2023).
For each species, we calculated the total number of independent detection events in each study reach, as well as the number of detection events per season in each study reach. We then standardized independent detection events by the number of functional trap nights. This standardization allowed us to correct for occasional camera battery failures that occurred between checks and more accurately compare results across seasons and study reaches. When examining seasonal patterns, we followed typical season lengths for spring (March 21st–June 20st), summer (June 21st–September 20st), fall (September 21st–December 20th), and winter (December 21st–March 20th). Results are summarized as number of independent detections per 100 functional trap nights for ease of interpretation.
We used generalized linear mixed-effects models to examine the relationship between wildlife detection events and various temporal and site-specific covariates for 6 species: bobcat, snowshoe hare, coyote, Columbian black-tailed deer, Roosevelt elk, and American black bear. We fit Poisson models with the response variable defined as the daily count of independent detection events for a particular species. The covariates we assessed were sampling year, season (as defined above), study reach, distance from forest edge, and camera viewshed. We tested 10 candidate models related to our hypotheses for patterns of detections: a null model; single-variable models for each of the five covariates; additive models for season and year, study reach and season, and study reach and year; and an interactive model for study reach and season. We included random effects for camera station and date in all candidate models. We fit the set of models for each species using the glmmTMB package (Brooks et al., 2017) in R (Version 4.3.1, R Core Team, 2023). We ranked models according to the Akaike information criterion (AIC) using the AICcmodavg package (Mazerolle, 2023) and considered well-supported models to be those with a ΔAIC of less than or equal to 2 (Burnham and Anderson, 2002). We assessed the fit of top-ranked models using the diagnostic functions and workflow of the DHARMa package (Hartig, 2022).
3 Results
3.1 Habitat characteristics
We placed cameras in Aldwell primarily in deciduous stands; of 10 camera locations, 8 were in stands dominated by a cottonwood overstory, and one each had overstories dominated by alder and willow. Two locations were on the sloped walls of the former reservoir (“valley wall”), 4 were on the gravel bar, and 4 on remnant terraces. Similarly, camera locations on Mills were all located in deciduous (60%) or gravel bar (40%) habitats. Dominant overstory was split between cottonwood (3 of 10), alder (3 of 10), and willow (3 of 10), with one open site. Two locations were on valley walls, 3 were on the gravel bar, and 5 were on remnant terraces. In contrast, camera locations in Geyser (our established reference reach) were more mixed, with 4 locations in conifer habitats, 3 in mixed conifer/hardwood habitats, and 3 in gravel or deciduous habitats. Six of 10 locations had a conifer overstory (Douglas fir or western hemlock), one was dominated by bigleaf maple, and 3 were alder dominated. Four were located in upland forest, one was on the valley wall, 3 were on remnant terraces, and 2 were on the gravel bar.
3.2 Species composition and seasonal use
After removing blank and unknown images as well those of humans, domestic dogs, birds, and small rodents, we documented a total of 107,658 images, consisting of 5,473 individual detection events (McCaffery et al., 2024). We identified 15 species of mammals over the two years of the study (Table 1) including American black bear, bobcat, chipmunk (Tamias spp.), Columbian black-tailed deer, coyote, Douglas squirrel (Tamiasciurus douglasii), fisher (Pekania pennanti), unspecified mustelid, northern flying squirrel (Glaucomys sabrinus), puma, raccoon, Roosevelt elk, snowshoe hare, spotted skunk (Spilogale gracilis), and opossum (Didelphis virginiana). Most species exhibited variations in detections across both study reach and season (Table 1, Supplementary Table S1).
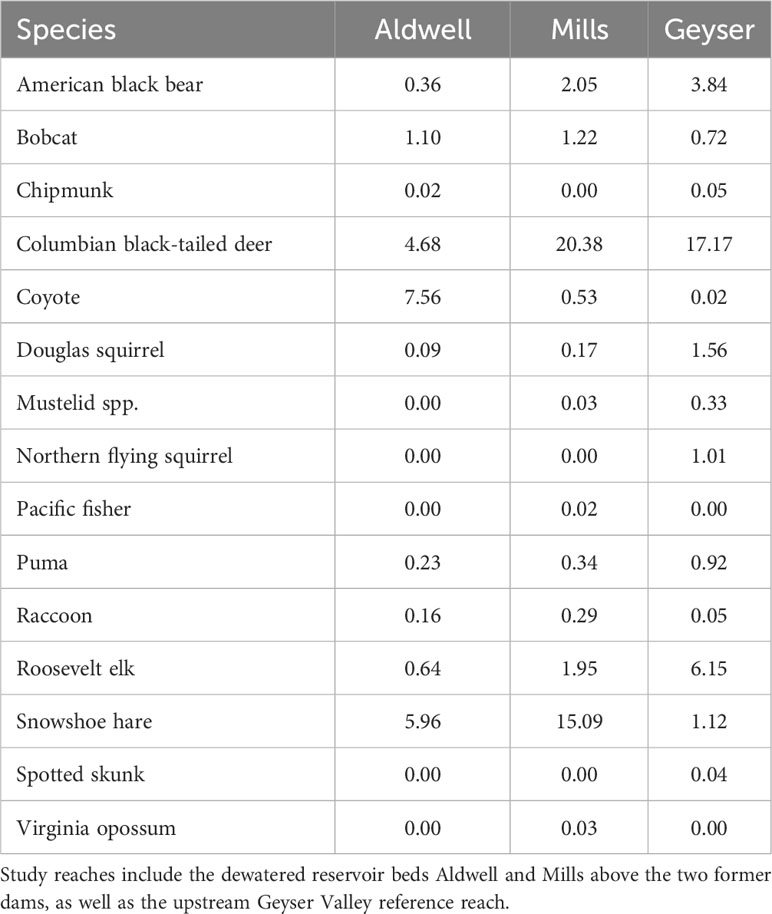
Table 1 Summary of mammalian detections per 100 trap nights by study reach in the Elwha River watershed, Washington, USA from July 2021-June 2023.
We fit all 10 candidate models for bobcat, snowshoe hare, coyote, and Columbian black-tailed deer. For all four species, the best supported model contained an interactive effect of study reach and season, with no support for any other models (Table 2, Supplementary Table S2). Model diagnostics did not indicate any problems with model misspecification. We detected snowshoe hares most frequently during the summer, and much more often in Mills (15 detections per 100 trap nights), than in Aldwell (6 detections per 100 trap nights), or Geyser (1 detection per 100 trap nights; Tables 1, 2, Supplementary Table S1). We observed coyotes, a species not native to the Olympic Peninsula, almost exclusively in Aldwell, which is located outside of Olympic National Park. We saw 8 coyote detections per 100 trap nights in Aldwell, compared to less than 1 in Mills and Geyser (Table 1). Coyotes were observed most frequently in the fall (Table 2, Supplementary Table S1). For deer, Mills and Geyser had much higher detection rates (20 and 17 detections per 100 trap nights, respectively) than Aldwell (5 detections per 100 trap nights; Table 1, Figure 2A). Columbian black-tailed deer were most common in the spring and fall seasons, and least common in winter (Table 2, Supplementary Table S1). In contrast to other species, bobcat detections occurred more evenly among seasons and among study reaches, at a rate of approximately 1 detection per 100 trap nights (Tables 1, 2, Supplementary Table S1).
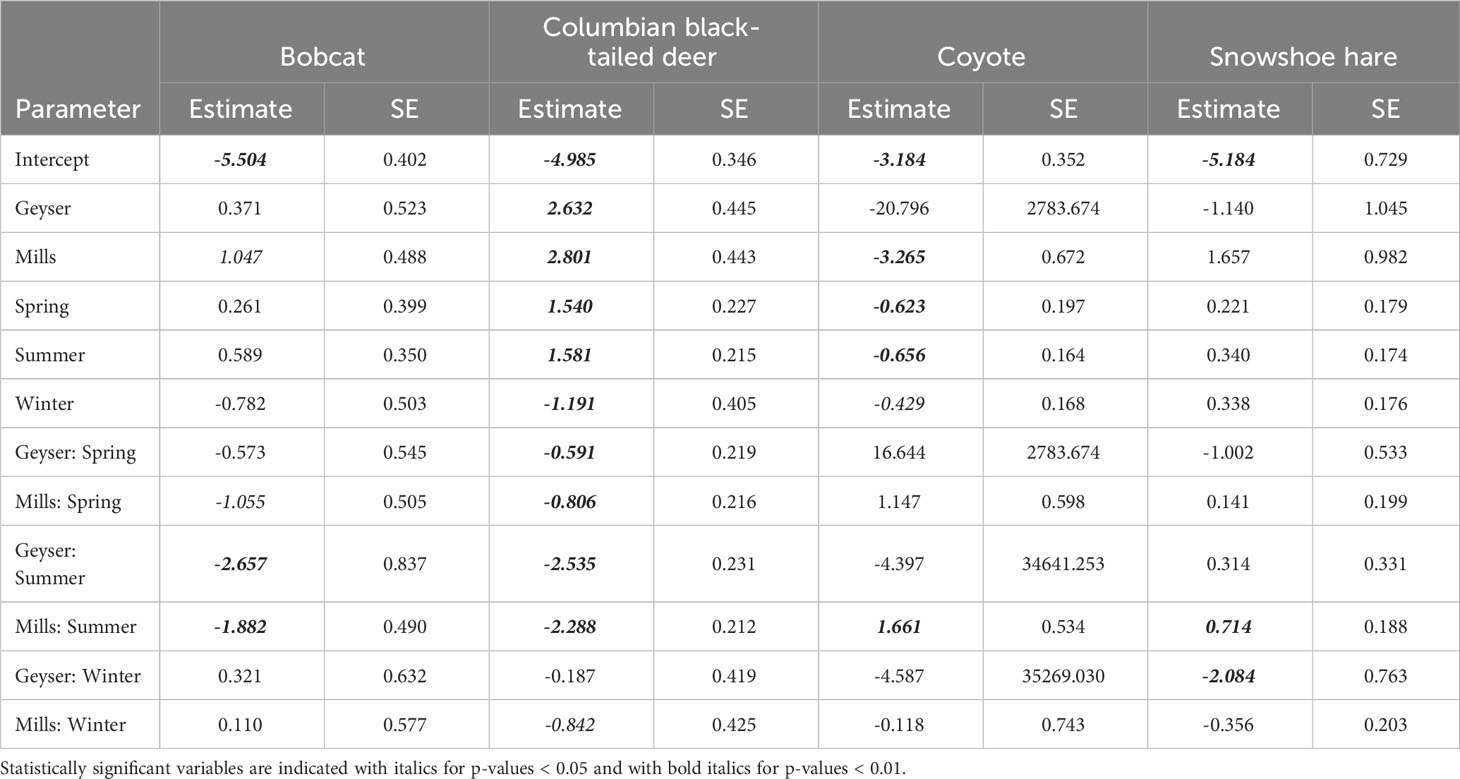
Table 2 Parameter estimates (log scale) and standard errors for the four species with a top-ranked model of Study Reach * Season.
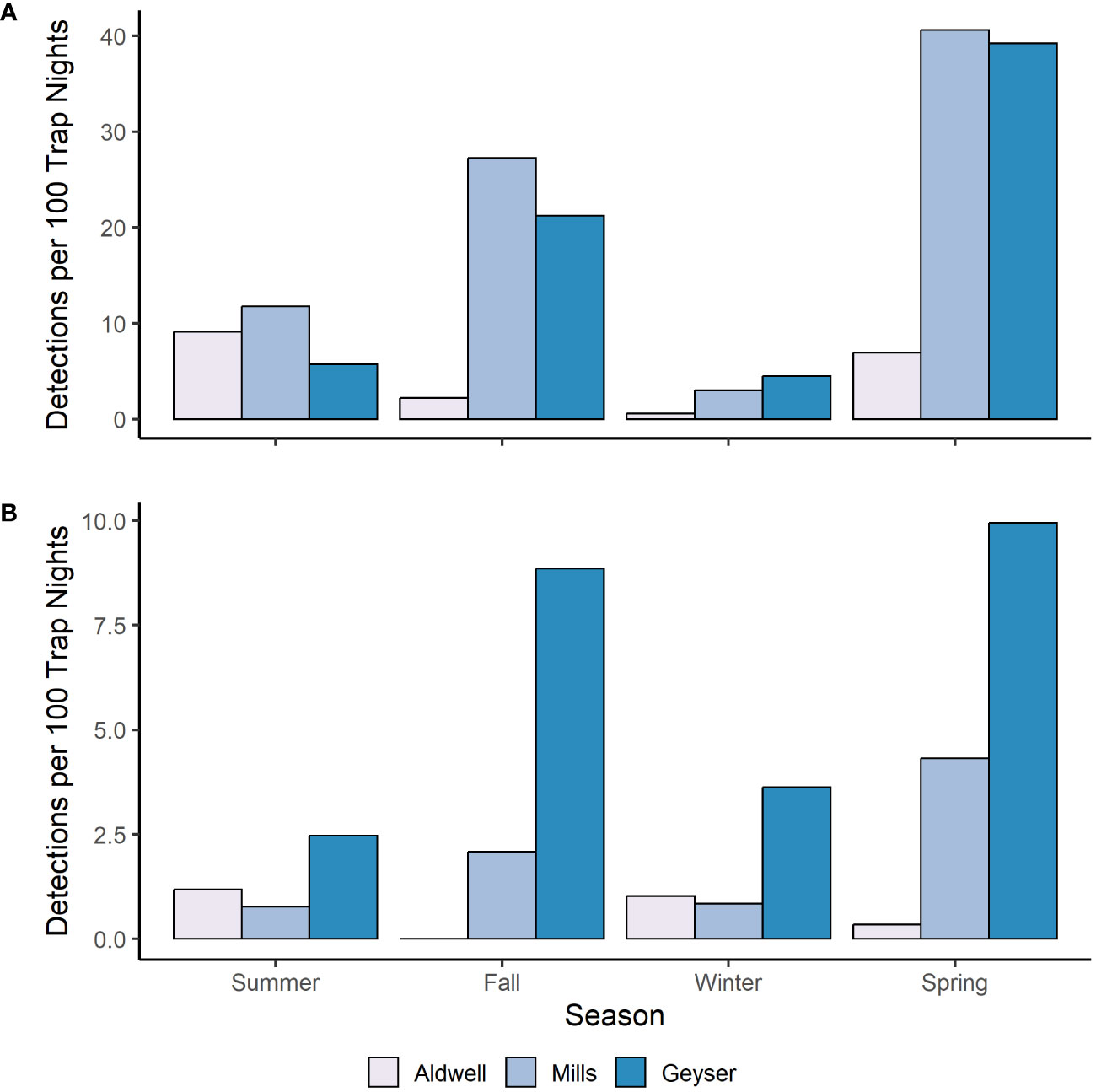
Figure 2 Number of independent photo events per 100 camera trap nights for (A) Columbian black-tail deer and (B) Roosevelt elk and in each study reach and season in the Elwha River from July 2021-June 2023.
For Roosevelt elk and black bear, the candidate model with an interactive effect of study reach and season produced a singular convergence warning due to the lack of detections at certain combinations of study reach and season. We therefore excluded it from further consideration. Among the other 9 models, the best supported model for both species contained an additive effect of study reach and season (Table 3, Supplementary Table S2), with no support for any other models. Model diagnostics did not indicate any problems with model misspecification. For Roosevelt elk, Geyser had the highest frequency of detections at 6 detections per 100 trap nights (Figure 2B; Table 1). Mills and Aldwell had less than 2 elk detections per 100 trap nights. We observed elk more often in spring and fall than in summer and winter (Figure 2B; Supplementary Table S1). Black bear detections were highest in Geyser (4 detections per 100 trap nights) and Mills (2 detections per 100 trap nights; Figure 3; Table 1). Bear detections were more frequent in the spring than in any other season (Figure 3; Supplementary Table S1).
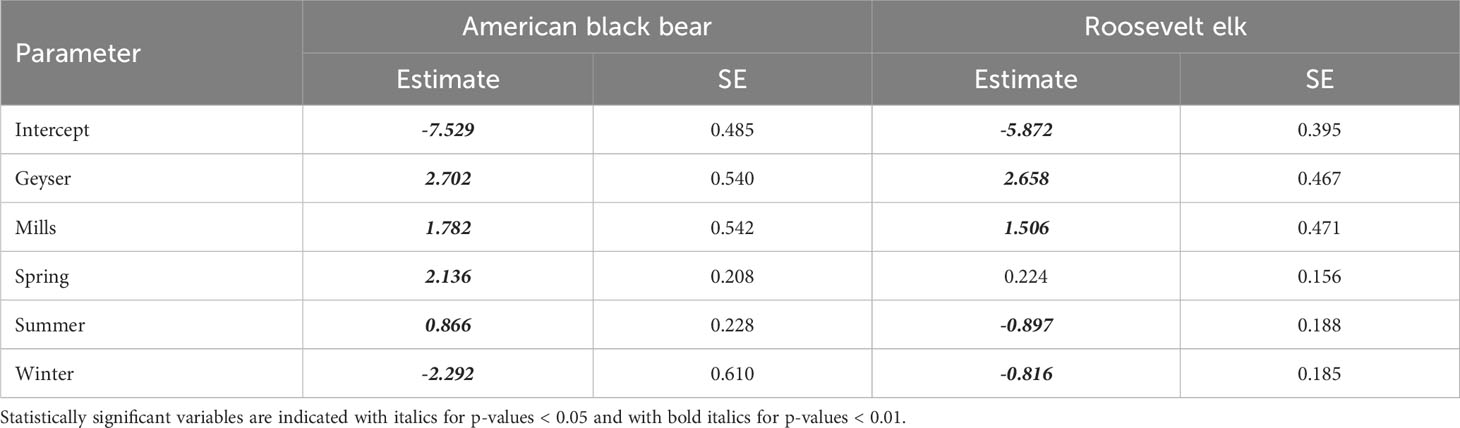
Table 3 Parameter estimates (log scale) and standard errors for the two species with a top-ranked model of Study Reach + Season.
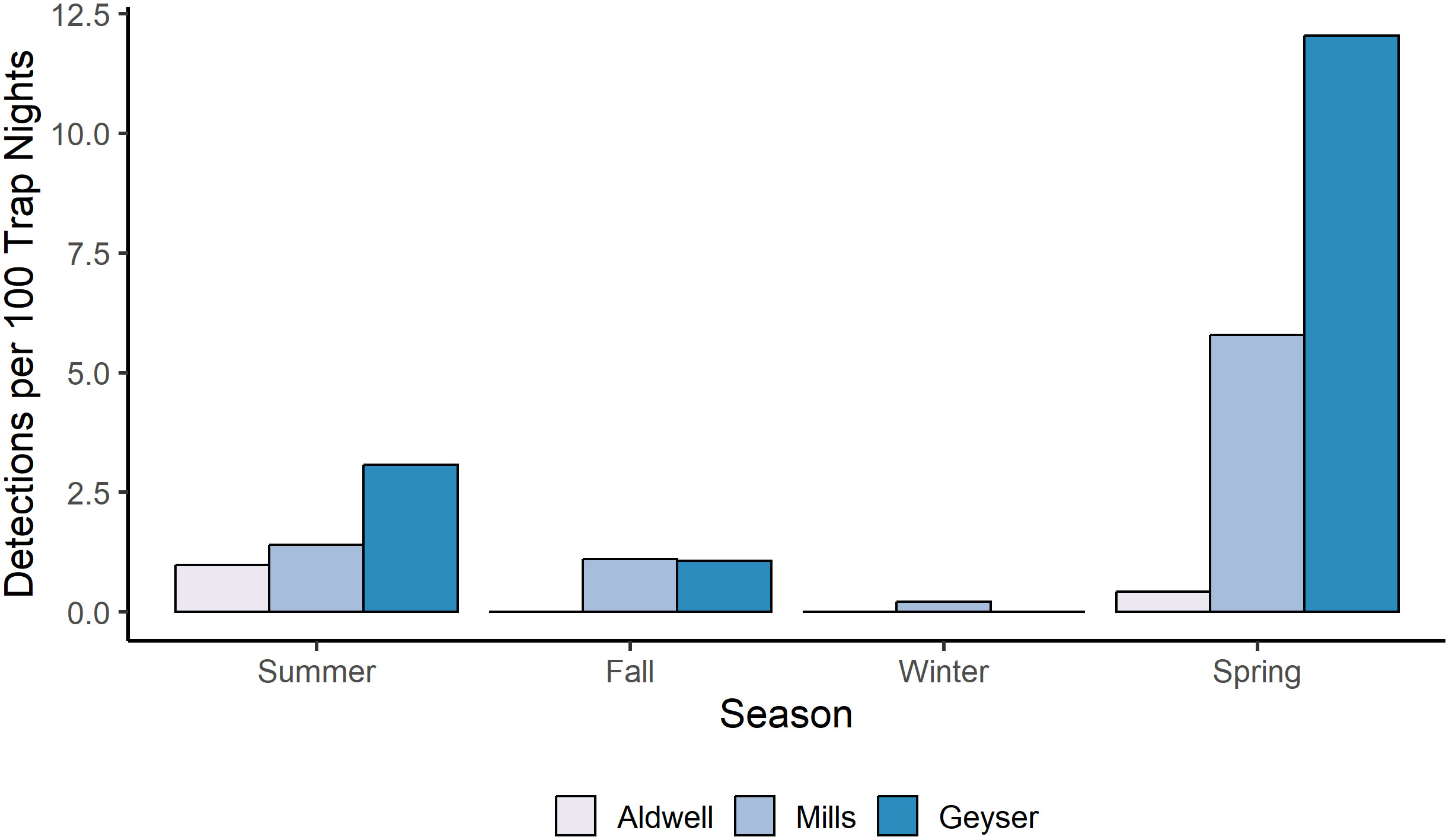
Figure 3 Number of independent photo events per 100 camera trap nights for American black bears in each study reach and season in the Elwha River from July 2021-June 2023.
We were unable to model factors associated with puma detections due to the small number of photos of this species. We detected pumas more frequently in Geyser (about 1 detection per 100 trap nights) than in Aldwell and Mills (less than 0.4 detections per 100 trap nights; Table 1). Puma detections were lowest in the spring (Supplementary Table S1). Detections of other species were variable and mostly infrequent (Table 1, Supplementary Table S1). We detected one Pacific fisher (Pekania penannti) on Mills in February 2023, a rare species that was reintroduced to the Olympic Peninsula between 2008 and 2010 after decades of absence. We detected raccoons on only three occasions in the Geyser study reach, but more frequently in Mills and Aldwell. We had two detections of the non-native Virginia opossum (Didelphis virginiana) at Mills in summer 2022 and two detections of the spotted skunk (Spilogale gracilis) at Geyser in fall 2021. Weasel (Mustela spp) detections were infrequent and occurred almost entirely in Geyser.
4 Discussion
Over two years of camera trapping, we revealed early patterns of use by a diverse suite of mammalian species on revegetating former reservoirs behind the two former dams, as well as in an upstream reference reach on the Elwha River. These data provide an initial snapshot of mammalian use of the two novel, revegetating habitats in comparison to an upstream river valley 10 years following dam removal, showing different patterns of use in newly forming riparian zones (Aldwell and Mills) relative to an established, mature riparian zone (Geyser). We were able to broadly compare distributions of American black bear (Sager-Fradkin et al., 2008) and small carnivores (Jenkins et al., 2013) in this study to studies conducted prior to dam removal. Further, we compared current patterns of use by Columbian black-tailed deer and Roosevelt elk to a study conducted in the three years immediately following dam removal (McCaffery et al., 2020). For other species, the data presented here provide a baseline to which we can compare future work on species distribution and use of the Elwha watershed as restoration and successional processes continue to unfold. Finally, our data can be used as a basis to understand how mammalian wildlife contribute to restored ecosystem function over time.
Our data showed preliminary patterns that suggest American black bear use of the watershed may be expanding seasonally to encompass a larger portion of the year than documented prior to dam removal. A study of black bears prior to dam removal in the Elwha watershed found that they exhibited predictable and synchronous patterns of elevation change throughout the year (Sager-Fradkin et al., 2008). Bears used low-elevation conifer and hardwood forests during spring, mid-elevation forests and meadows during summer, and mid-to-high-elevation forests and meadows during late summer and fall, with limited movement during the winter denning period (Sager-Fradkin et al., 2008). Researchers concluded that bears may change annual distribution patterns after dam removal and salmon restoration by increasing use along the floodplain during late summer and fall when coho and Chinook runs return upriver (Sager-Fradkin et al., 2008). Our detections indicated some evidence that bear presence is higher in the fall to winter than previously documented in Sager-Fradkin et al. (2008 [Figure 2]); moreover, we have recently documented bear use of the Mills reservoir during December, including fishing for coho salmon in a tributary stream (K. Sager-Fradkin, personal observation). However, we expect that fuller shifts in seasonal use could take longer to establish and be a function of both time and recovery of salmon populations. While anadromous fish have now moved upstream past both dams and into the upper watershed (Duda et al., 2021), the restoration process is early and ongoing and fish numbers are influenced by many factors beyond the restoration of the river. Future work should aim to quantify bear use more comprehensively and to understand the role of bears in moving fish and marine-derived nutrients into the surrounding ecosystem once salmon are more established.
Small and mesocarnivore detections were low and variable in this study, in contrast to a pre-dam removal study conducted along the entire river between 2006 and 2008 (Jenkins et al., 2013). The pre-dam removal research documented that populations of some small carnivore species were most common on the lower river reaches (i.e., below and between the dams), although the potential effects of salmon availability (i.e., no salmon present above the lower dam) were confounded with other human uses of the lower river (Jenkins et al., 2013). In this study, small carnivores were rarely detected and in small numbers. Notably, the previous study used bait to attract carnivores (Jenkins et al., 2013), while we used unbaited cameras. Use of baited stations would likely be necessary to better characterize use by this group of species and compare them to pre-dam removal distributions. We had one detection of the Pacific fisher in the second year of our study, a rare mesocarnivore that was reintroduced to the Olympic Peninsula between 2008-2010 after decades of absence presumably due to overtrapping and habitat loss (Hayes and Lewis, 2006), and which was not present when the pre-dam removal study was conducted. This detection adds to other camera detections of this rarely seen species in the area, contributing to our understanding of post-reintroduction use of Olympic National Park and surrounding areas (e.g., Happe et al., 2020). Primary prey for fisher in this area includes snowshoe hares, which we commonly detected in Aldwell and Mills (where the fisher was observed), and which thrive in disturbed or early-stage vegetation (Parsons et al., 2020; Table 1). The regenerating riparian area may provide beneficial hunting areas for fishers adjacent to mature forests typically used for denning (Lewis et al., 2016). In fact, the fisher we photographed on Mills had a snowshoe hare in its mouth.
We found widespread use of the revegetating reservoir beds and Geyser Valley by Roosevelt elk and Columbian black-tailed deer but continue to see differences across the three study reaches and across seasons. Floodplain bottomlands and riparian zones are key habitats for Columbian black-tailed deer and Roosevelt elk on the Olympic Peninsula (Jenkins and Starkey, 1984; Schroer et al., 1993), and both species used the former Elwha reservoirs relatively quickly after dam removal (McCaffery et al., 2020). In previous work, we examined presence of deer and elk in the three study reaches over the winter and into spring by using pellet counts as indices of use (McCaffery et al., 2020). In that study, we found elk use to be highest in Geyser, low but increasing over time in Mills, and virtually absent from Aldwell (McCaffery et al., 2020). Overall, these general patterns have been maintained in this camera-based study: Geyser still has the highest elk use, but there is some suggestion that winter-to-spring elk use has increased in Aldwell and Mills compared to five years ago (Figure 2B). We found very little overwinter use by deer in study plots in Aldwell and Mills in our previous study (McCaffery et al., 2020). Patterns of use appeared similar in our current study, with deer detections being lowest in the winter season, and lowest in Aldwell compared to the other two study areas (Figure 2A). The area surrounding the Aldwell reservoir bed is more developed and more human-influenced than Mills and Geyser. The availability of safe deer habitat in residential yards and fields as well as the prevalence of hunting in Aldwell and on adjacent public lands may lead to diminished deer use of the Aldwell lakebed. Again, since methodologies differ and rely on indices of use in these two studies, these data provide a qualitative comparison that can serve as a basis for ongoing monitoring of use.
Our results showed differences in detections by species in the regenerating lakebeds compared to the upstream Geyser reference reach, which is dominated by conifer overstory and represents an older, mature riparian system that was relatively unaffected by dam removal activities. Moreover, Geyser is the furthest upriver reach that we studied, is located in wilderness within Olympic National Park, and is much harder to access and thus has a much smaller human footprint than both Aldwell and Mills. We documented bear, elk, and puma more frequently in this upper part of the river (Figures 2B, 3; Table 1), and documented more forest-associated species like squirrels and chipmunks in Geyser compared to Mills and Aldwell (Table 1). Differences between Geyser and the two regenerating lakebeds were less striking for deer (Figure 2A), which, despite their lower detection numbers in Aldwell, were ubiquitous across study areas. Further, we detected coyotes – a nonnative species that has become relatively widespread on the Olympic Peninsula – predominantly in Aldwell, with some detections in Mills but only one coyote detection in Geyser in the second year (Table 1). Coyotes are found at higher elevations in other parts of the park (Witczuk et al., 2013), and it is unknown what led to the strong differences between detections in Aldwell versus the two other areas. Over time, we will be able to monitor if use by this species shifts further upstream in this system. Finally, snowshoe hare detections were much higher in Mills and Aldwell where forests are still in early stages of regenerating compared to Geyser. These differences in use and composition likely reflect both habitat differences (early seral stage riparian-dominated habitats in the regenerating lakebeds above the former dams v. mature riparian zone in Geyser) as well as elevational position in the watershed.
While cameras provide a useful tool for remotely monitoring a suite of rare or elusive species alongside more common ones, they have some limitations. We aimed to control for obvious repeat detections of the same individuals by removing adjacent pictures of the same species, but we had no way to identify individuals, so our data only represented an index of current use. Camera location likely affected the diversity and numbers of species captured in our images. For example, more targeted studies for individual species may have led to different approaches in the study design such as camera baiting or targeting specific habitat characteristics (i.e. closer to river channels or beaver dams). However, as this is the first attempt to capture the suite of mammalian species using the dewatered lakebeds following dam removal, this work should have increasing value going forward as successional processes in these habitats continue and a longitudinal record of species composition in relation to habitat changes develops.
As changes in the Elwha ecosystem continues to unfold, terrestrial mammals are expected to both respond to and interact with the changing landscape. In addition to becoming established as permanent or seasonal residents in novel and changing habitats, species may interact with new vegetation in the former reservoirs (e.g., McCaffery et al., 2020), disperse seeds across the restoration area and beyond (e.g., McLaughlin, 2013), or consume and transport marine-derived nutrients brought to the system by anadromous fish (e.g., Tonra et al., 2015). Comprehensive restoration of the Elwha ecosystem could take decades but should ultimately include reestablishment of important terrestrial-aquatic linkages. Ultimately, wildlife should continue to play a pivotal role in ongoing revegetation and succession following dam removal and should not be overlooked as players in and beneficiaries of restoration of large watersheds following dam removal. This study provides a baseline of data from which to develop hypotheses for studies of wildlife roles in restored ecosystem function; establishes patterns of use that can be monitored over time as the system changes; and provides a template for understanding the role of wildlife within recovery processes in other large dam removal systems around the world.
Data availability statement
The original contributions presented in the study are publicly available. This data can be found here: https://doi.org/10.5066/P1BJD7OH.
Ethics statement
Ethical approval was not required for the study involving animals in accordance with the local legislation and institutional requirements because the research was non-invasive and did not involve any handling of any animal. Remote cameras were used to monitor animal presence without any manipulation or contact.
Author contributions
RM: Conceptualization, Formal analysis, Funding acquisition, Investigation, Methodology, Writing – original draft, Writing – review & editing. SC: Investigation, Writing – original draft, Writing – review & editing, Data curation, Formal analysis. KG: Formal analysis, Writing – review & editing, Methodology, Writing – original draft. KJ: Conceptualization, Methodology, Writing – review & editing. PH: Writing – review & editing, Conceptualization, Methodology. KS: Conceptualization, Funding acquisition, Investigation, Methodology, Project administration, Supervision, Writing – original draft, Writing – review & editing.
Funding
The author(s) declare financial support was received for the research, authorship, and/or publication of this article. This work was supported by the United States Fish and Wildlife Service (USFWS) Tribal Wildlife Grant [grant number F21AP00714], the Resources Legacy Fund (RLF) - grant number 15594, and the United States Geological Survey (USGS). USFWS funding covered equipment costs and staff time for field work, data processing, and data analysis. RLF paid the publication fee. USGS funding covered equipment costs as well as staff time for field work, data analysis, and writing.
Acknowledgments
We are grateful to M. Sheldon, D. Manson, V. Castle, J. Bauman and her students from Western Washington University, and others for assistance in the field. We thank J. Hagar and two reviewers for reviewing the manuscript. Any use of trade, firm, or product names is for descriptive purposes only and does not imply endorsement by the U.S. Government.
Conflict of interest
The authors declare that the research was conducted in the absence of any commercial or financial relationships that could be construed as a potential conflict of interest.
Publisher’s note
All claims expressed in this article are solely those of the authors and do not necessarily represent those of their affiliated organizations, or those of the publisher, the editors and the reviewers. Any product that may be evaluated in this article, or claim that may be made by its manufacturer, is not guaranteed or endorsed by the publisher.
Supplementary material
The Supplementary Material for this article can be found online at: https://www.frontiersin.org/articles/10.3389/fevo.2024.1266474/full#supplementary-material
References
Alayan R., Rotich B., Lakner Z. (2022). A comprehensive framework for forest restoration after forest fires in theory and practice: a systematic review. Forests 13, 1354. doi: 10.3390/f13091354
Albert A., Auffret A. G., Cosyns E., Cousins S. A. O., D’hondt B., Eichberg C., et al. (2015). Seed dispersal by ungulates as an ecological filter: a trait-based meta-analysis. Oikos 124, 1109–1120. doi: 10.1111/oik.02512
Ayars J., Emmet R. L., Bassing S. B., Sanderfoot O. V., Raby S., Karambelas A., et al. (2023). Camera traps link population-level activity patterns with wildfire smoke events for mammals in Eastern Washington State. Fire Ecol. 19, 49. doi: 10.1186/s42408-023-00207-1
Babbitt B. (2002). What goes up, may come down: Learning from our experiences with dam construction in the past can guide and improve dam removal in the future. BioScience 52, 656–658. doi: 10.1641/0006-3568(2002)052[0656:WGUMCD]2.0.CO;2
Barcelos D. C., Alvarenga G. C., Grabin D. M., Baccaro F., Ramalho E. E. (2023). Divergent effects of lure on multi-species camera-trap detections and quality of photos. J. Nat. Conserv. 71, 1–9. doi: 10.1016/j.jnc.2022.126317
Bellmore J. R., Duda J. J., Craig L. S., Greene S. L., Torgerson C. E., Collins M. J., et al. (2016). Status and trends of dam removal research in the United States. WIREs Water 4, e1164. doi: 10.1002/wat2.1164
Bountry J. A., Ferrari R., Wille K., Randle T. J. (2010). Survey Report and Area-Capacity Tables for Lake Mills and Lake Aldwell on the Elwha River, Washington (Denver, CO: US Department of the Interior, Bureau of Reclamation). Technical Service Center Report No. SRH-2010-23.
Bricker M., Pearson D., Maron J. (2010). Small-mammal seed predation limits the recruitment and abundance of two perennial grassland forbs. Ecology 91, 85–92. doi: 10.1890/08-1773.1
Brooks M. E., Kristensen K., van Benthem K. J., Magnusson A., Berg C. W., Nielsen A., et al. (2017). glmmTMB balances speed and flexibility among packages for zero-inflated generalized linear mixed modeling. R J. 9, 378–400. doi: 10.32614/RJ-2017-066
Burnham K. P., Anderson D. R. (2002). Model selection and multimodel inference: A practical information-theoretic approach. 2nd ed. (New York: Springer).
Burton A. C., Neilson E., Moreira D., Ladle A., Steenweg R., Fisher J. T., et al. (2015). REVIEW: Wildlife camera trapping: a review and recommendations for linking surveys to ecological processes. J. Appl. Ecol. 52, 675–685. doi: 10.1111/1365-2664.12432
Cederholm C. J., Houston D. B., Cole D. L., Scarlett W. J. (1989). Fate of coho salmon carcasses in spawning streams. Can. J. Fisheries Aquat. Sci. 46, 1347–1355. doi: 10.1139/f89-173
Chenoweth J., Bakker J. D., Acker S. A. (2022). Planting, seeding, and sediment impact restoration success following dam removal. Restor. Ecol. 30, e13506. doi: 10.1111/rec.13506
Dart M. M., Perkins L. B., Jenks J. A., Hatfield G., Lonsinger R. C. (2022). The effect of scent lures on detection is not equitable among sympatric species. Wildlife Res. 50, 199–200. doi: 10.1071/WR22094
Department of the Interior (DOI), Department of Commerce, and the Lower Elwha S’Klallam Tribe (1994). The Elwha report: Restoration of the Elwha River ecosystem and native anadromous fisheries. (Washington D.C.: Department of Interior, United States Government Publishing Office)
Doren R. F., Trexler J. C., Gottlieb A. D., Harwell M. C. (2008). Ecological indicators for system-wide assessment of the greater everglades ecosystem restoration program. Ecol. Indic. 9S, S2–S16. doi: 10.1016/j.ecolind.2008.08.009
Duda J. J., Torgersen C. E., Brenkman S. J., Peters R. J., Sutton K. T., Connor H. A., et al. (2021). Reconnecting the Elwha River: spatial patterns of fish response to dam removal. Front. Ecol. Evol. 9, 765488. doi: 10.3389/fevo.2021.765488
Gill R. M. A., Beardall V. (2001). The impact of deer on woodlands: the effects of browsing and seed dispersal on vegetation structure and composition. Forestry 74, 209–218. doi: 10.1093/forestry/74.3.209
Happe P., Jenkins K. J., McCaffery R., Lewis J., Pilgrim K., Schwartz M. K. (2020). Occupancy patterns in a reestablishing fisher population following reintroduction. J. Wildlife Manage. 84, 344–358. doi: 10.1002/jwmg.21788
Hart D. D., Poff N. L. (2002). A special section on dam removal and river restoration. BioScience 52, 653–655. doi: 10.1641/0006-3568(2002)052[0653:ASSODR]2.0.CO;2
Hartig F. (2022) DHARMa: Residual diagnostics for hierarchical (multi-level/mixed) regression models. R package version 0.4.6. Available online at: https://CRAN.R-project.org/package=DHARMa.
Hayes G. E., Lewis J. C. (2006). Washington State Recovery Plan for the Fisher (Olympia, WA: Washington Department of Fish and Wildlife, 62+ viii pp).
Helfield J. M., Naiman R. J. (2006). Keystone interactions: salmon and bear in riparian forests of Alaska. Ecosystems 9, 167–180. doi: 10.1007/s10021-004-0063-5
Hobbs N. T. (1996). Modification of ecosystems by ungulates. J. Wildlife Manage. 60, 695–713. doi: 10.2307/3802368
Holinda D., Burgar J. M., Burton A. C. (2020). Effects of scent lure on camera trap detections vary across mammalian predator and prey species. PloS One 15, e0229055. doi: 10.1371/journal.pone.0229055
Horsley S. B., Stout S. L., DeCalesta D. S. (2003). White-tailed deer impact on the vegetation dynamics of norther hardwood forest. Ecol. Appl. 13, 98–118. doi: 10.1890/1051-0761(2003)013[0098:WTDIOT]2.0.CO;2
Iannarilli F., Erb J., Arnold T. W., Fieberg J. R. (2021). Evaluating species-specific responses to camera-trap survey designs. Wildlife Biol. 2021, 1–12wlb.00726. doi: 10.2981/wlb.00726
Jenkins K. J., Chelgren N. D., Sager-Fradkin K. A., Happe P. J., Adams M. J. (2013). Occupancy patterns of mammals and lentic amphibians in the Elwha River riparian zone before dam removal. River Res. Appl. 31, 193–206. doi: 10.1002/rra.2723
Jenkins K. J., Starkey E. E. (1984). Habitat use by Roosevelt elk in unmanaged forests of the Hoh Valley, Washington. J. Wildlife Manage. 48, 642–646. doi: 10.2307/3801209
Leidholt-Bruner K., Hibbs D. E., McComb W. C. (1992). Beaver dam locations and their effects on distribution and abundance of coho salmon fry in two coastal Oregon streams. Northwest Sci. 66, 218–223.
Lewis J. C., Jenkins K. J., Happe P. J., Manson D. J., McCalmon M. (2016). Landscape-Scale habitat selection by fishers translocated to the Olympic Peninsula of Washington. For. Ecol. Manage. 369, 170–183. doi: 10.1016/j.foreco.2016.02.032
Major J. J., Crisafulli C. M., Frenzen P., Bishop J. (2009). “After the disaster: The hydrogeomorphic, ecological, and biological responses to the 1980 eruption of Mount St. Helens, Washington,” in Volcanoes to Vineyards: Geologic Field Trips through the Dynamic Landscape of the Pacific Northwest: Geological Society of America Field Guide, vol. 15 . Eds. O’Connor J. E., Dorsey R. J., Madin I. P. (Boulder, CO: The Geological Society of America, Inc.), 111–134.
Mazerolle M. J. (2023) AICcmodavg: Model selection and multimodel inference based on (Q)AIC(c). R package version 2.3.3. Available online at: https://cran.r-project.org/package=AICcmodavg.
McCaffery R. M., Cendejas-Zarelli S. J., Goodwin K. R., Happe P. J., Jenkins K. J., Sager-Fradkin K. A. (2024). Camera trap photo detections of mammals along three reaches of the Elwha River, Washington (U.S. Geological Survey Data Release). doi: 10.5066/P1BJD7OH
McCaffery R., Jenkins K. J., Cendejas-Zarelli S., Happe P. J., Sager-Fradkin K. A. (2020). Small mammals and ungulates respond to and interact with revegetation processes following dam removal. Food Webs 25, e00159. doi: 10.1016/j.fooweb.2020.e00159
McCaffery R., McLaughlin J., Sager-Fradkin K., Jenkins K. J. (2018). Terrestrial fauna are agents and endpoints in ecosystem restoration following dam removal. Ecol. Restor. 36, 97–107. doi: 10.3368/er.36.2.97
McLaughlin J. F. (2013). Engaging birds in vegetation restoration after Elwha dam removal. Ecol. Restor. 31, 46–56. doi: 10.3368/er.31.1.46
Naiman R. J. (1988). Animal influences on ecosystem dynamics. Bioscience 38, 750. doi: 10.2307/1310783
O'Brien T. G., Kinnaird M. F., Wibisono H. T. (2003). Crouching tigers, hidden prey: Sumatran tiger and prey populations in a tropical forest landscape. Anim. Conserv. 6, 131–139. doi: 10.1017/S1367943003003172
O’Connor J. E., Duda J. J., Grant G. E. (2015). 1000 dams and counting. Science 348, 496–497. doi: 10.1126/science.aaa9204
Ostfield R. S., Manson R. H., Canham C. D. (1997). Effects of rodents on survival or tree seeds and seedlings invading old fields. Ecology 78, 1531–1542. doi: 10.1890/0012-9658(1997)078[1531:EOROSO]2.0.CO;2
Parsons M. A., Lewis J. C., Pauli J. N., Chestnut T., Ranson J. I., Werntz D. O., et al. (2020). Prey of reintroduced fishers and their habitat relationships in the Cascades Range, Washington. For. Ecol. Manage. 460, 117888. doi: 10.1016/j.foreco.2020.117888
Pess G. R., McHenry M. L., Beechies T. J., Davies J. (2008). Biological impacts of the Elwha River dams and potential salmonid responses to dam removal. Northwest Sci. 82, 72–90. doi: 10.3955/0029-344X-82.S.I.72
Pollock M. M., Lewallen G., Woodruff K., Jordan C. E., Castro J. M. (2015). The Beaver Restoration Guidebook: Working with Beaver to Restore Streams, Wetlands, and Floodplains. Version 1.02 (Portland: U.S. Fish and Wildlife Service).
Pollock M. M., Pess G. R., Beechie T. J. (2004). The importance of beaver ponds to coho salmon productions in the StillaGuamish River Basin, Washington, USA. North Am. J. Fisheries Manage. 24, 749–760. doi: 10.1577/M03-156.1
Quinn T. P., Carlson S. M., Gende S. M., Rich H. B. Jr (2009). Transportation of Pacific salmon carcasses from streams to riparian forests by bears. Can. J. Zoology 87, 195–203. doi: 10.1139/Z09-004
R Core Team (2023). R: A language and environment for statistical computing (Vienna, Austria: R Foundation for Statistical Computing). Available at: https://www.R-project.org/.
Sager-Fradkin K. A., Jenkins K. J., Happe P. J., Beecham J. J., Wright R. G., Hoffman R. A. (2008). Space and habitat use by black bears in the Elwha Valley prior to dam removal. Northwest Sci. 82, 164–178. doi: 10.3955/0029-344X-82.S.I.164
Schreiner E. G., Krueger K. A., Happe P. J., Houston D. B. (1996). Understory patch dynamics and ungulate herbivory in old-growth forests of Olympic National Park, Washington. Can. J. For. Res. 26, 255–265. doi: 10.1139/x26-029
Schroer G. L., Jenkins K. J., Moorhead B. B. (1993). Roosevelt elk selection of temperate rain forest seral stages in western Washington. Northwest Sci. 67, 23–29.
Seagle S. W. (2003). Can ungulates foraging in a multiple-use landscape alter forest nitrogen budgets? Oikos 103, 230–234. doi: 10.1034/j.1600-0706.2003.12287.x
Shafroth B., Perry L. G., Helfield J. M., Chenowith J., Brown R. L. (2024). Vegetation responses to large dam removal on the Elwha River, Washington, USA. Front. Ecol. Evol. doi: 10.3389/fevo.2024.1272921
Souza-Alonso P., Saiz G., García R. A., Pauchard A., Ferreira A., Merino A. (2022). Post-fire ecological restoration in Latin American forest ecosystems: Insights and lessons from the last two decades. For. Ecol. Manage. 509, 1–20. doi: 10.1016/j.foreco.2022.120083
Tonra C. M., Sager-Fradkin K., Morley S. A., Duda J. J., Marra P. P. (2015). The rapid return of marine-derived nutrients to a freshwater food web following dam removal. Biol. Conserv. 192, 130–134. doi: 10.1016/j.biocon.2015.09.009
Vallejo V. R., Alloza J. A. (2015). “Chapter 12 - postfire ecosystem restoration,” in Wildfire hazards, risks and disasters. Eds. Shroder J. F., Paton D. (Cambridge, MA: Elsevier), 229–246.
Warrick J. A., Duda J. J., Magril C. S., Curran C. A. (2012). River turbidity and sediment loads during dam removal. Eos 93, 43, 425–426. doi: 10.1029/2012EO430002
Willson M. F. (1993). Mammals as seed-dispersal mutualists in North America. Oikos 67, 159–176. doi: 10.2307/3545106
Witczuk J., Pagacz S., Mills L. S. (2013). Disproportionate predation on endemic marmots by invasive coyotes. J. Mammalogy 94, 702–713. doi: 10.1644/12-MAMM-A-199.1
Woodward A., Jenkins K. J., Harmon M. E. (2021). Plant community succession following ungulate exclusion in a temperate rainforest. Ecosphere 12, e03889. doi: 10.1002/ecs2.3889
Keywords: camera-trapping, dam removal, Elwha, restoration, wildlife
Citation: McCaffery RM, Cendejas-Zarelli SJ, Goodwin KR, Happe PJ, Jenkins KJ and Sager-Fradkin KA (2024) Establishment of terrestrial mammals on former reservoir beds following large dam removal on the Elwha River, Washington, USA. Front. Ecol. Evol. 12:1266474. doi: 10.3389/fevo.2024.1266474
Received: 25 July 2023; Accepted: 11 March 2024;
Published: 26 March 2024.
Edited by:
Arnaldo Marín, University of Murcia, SpainReviewed by:
Laura E. D'Acunto, Wetland and Aquatic Research Center, United StatesThomas Neeson, University of Oklahoma, United States
Copyright © 2024 McCaffery, Cendejas-Zarelli, Goodwin, Happe, Jenkins and Sager-Fradkin. This is an open-access article distributed under the terms of the Creative Commons Attribution License (CC BY). The use, distribution or reproduction in other forums is permitted, provided the original author(s) and the copyright owner(s) are credited and that the original publication in this journal is cited, in accordance with accepted academic practice. No use, distribution or reproduction is permitted which does not comply with these terms.
*Correspondence: Rebecca M. McCaffery, rmccaffery@usgs.gov; Kimberly A. Sager-Fradkin, kim.sager@elwha.org