- 1Laboratory of Sensory Ecology, Department of Physiology and Behavior, Biosciences Center, Federal University of Rio Grande do Norte, Natal, RN, Brazil
- 2Evolutionary Ecology Laboratory, Department of Zoology, Institute of Biological Sciences, University of Brasilia, Brasilia, DF, Brazil
- 3Insect Ecology Laboratory, Department of Ecology, Institute of Biology, Federal University of Rio de Janeiro, Rio de Janeiro, RJ, Brazil
The study of butterfly coloration has helped to identify the ecological pressures involved in the evolution of animal coloration. However, almost all studies that addressed this issue have focused on species that inhabit more temperate environments, leaving the species and ecological factors of tropical regions mostly understudied. Here, our purpose was to evaluate whether butterfly assemblages from two distinct Neotropical biomes (i.e., tropical rainforest and xeric white forest) differ regarding their melanism and/or color saturation. Our hypotheses were that (1) tropical rainforest butterflies should be more melanic and color saturated, and that (2) butterflies from more open/arid tropical environments should be more melanic on their dorsal wing surfaces than on their ventral wings. Therefore, we quantified melanism and color saturation from dorsal and ventral surfaces of 121 different butterfly species. Comparisons show that rainforest butterflies, when contrasted to white forest butterflies, have more melanic dorsal wing surfaces, which might be seen as a form of protection against parasites. Our data also show that rainforest butterflies, but not white forest species, have darker dorsal wing surfaces, when compared to their own ventral surfaces, a trend that was also found for species inhabiting both biomes, which might be associated to thermoregulatory advantages. At last, our results also point that butterflies' dorsal wing sides present a higher variance between species (regardless of Biome), when compared to their own ventral wing side, an indication that some ecological factor (e.g., predation avoidance) might be exerting a strong homogenizing force on ventral wing coloration.
1. Introduction
Butterflies are excellent study models for examining melanism and animal coloration because such features can be used as markers to understand which selective forces have been in action (Endler, 1982; Andersson, 1994). For instance, comparable color patterns that are seen in distinct species might result from convergent evolution driven by similar environmental pressures (Hoekstra, 2006). The color patterns of butterfly wings are formed through a set of physical mechanisms and pigments, such as melanin (Watt, 1964), and might play a role in mate recognition (Wiernasz, 1995), thermoregulation (Clusella-Trullas et al., 2007), protection against predators (Kettlewell, 1955) and parasites (True, 2003), as well as in desiccation and UV damage prevention (Majerus, 1998).
Organisms are expected to have darker colors in environments with lower temperatures because the concentration of melanin in the cuticle increases the absorption of thermal energy (Clusella-Trullas et al., 2007). As ectothermic and diurnal individuals, butterflies need heat to carry out their activities, such as foraging and looking for mates (Brakefield and Willmer, 1985; Wiernasz, 1995). So, the presence of darker patches might favor butterflies by quickly increasing their body temperature to carry out their activities (Kingsolver, 1983). Dark melanic butterflies also seem to be associated with humid environments (Stelbrink et al., 2019) as a form of protection against parasites. Alternatively, in warmer, highly sun-exposed, environments, insects are expected to be brighter to avoid overheating (Schmitz, 1994; Heidrich et al., 2018). However, melanism may not always be expressed homogeneously throughout the animal's body, as is the case for melanic differences found between dorsal and ventral surfaces of butterfly wings, a thermoregulatory strategy (Zeuss et al., 2014; Stelbrink et al., 2019) that captures more thermal energy when exposing dark melanic dorsal surfaces and dissipates more heat when exposing less pigmented ventral surfaces (Tsai et al., 2020). In addition to affecting lightness, melanin might also influence other spectral parameters of animal coloration, such as saturation (i.e., the purity of the color of an area judged in proportion to its brightness). For instance, melanic colors mostly result in patterns with lower saturation (i.e., less vivid colors), such as black, gray, and brown (McGraw, 2006), although the opposite relation has also been shown to be true for monarch butterflies (Hanley et al., 2013).
Whereas, most studies of lepidopteran coloration assemblages have addressed species and environmental conditions from the Palearctic (Zeuss et al., 2014; Heidrich et al., 2018; Stelbrink et al., 2019), Nearctic (Zeuss et al., 2014; Stelbrink et al., 2019), or Australian (Xing et al., 2016, 2018; Dalrymple et al., 2018) regions, the Neotropical species have been considerably understudied. In a country of continental dimensions, which holds an astonishing biodiversity and has seven different morphoclimatic and phytogeographic domains (Ab'Saber, 1967), it is surprising that only one study, conducted with Amazonian species, aimed to investigate lepidopteran color assemblages in Brazil (Spaniol et al., 2019).
Considering that environmental conditions can drive behaviors and select different coloration patterns (Endler, 1992) and that the spectral composition of sunlight can be modified by the physical characteristics of the environment (Endler, 1993), the present study aimed to compare the visual patterns displayed by assemblages of butterflies from two distinct Brazilian biomes, namely, the tropical rainforest and the xeric white forest. We expect butterfly assemblages from each biome to differ regarding their melanism and/or color saturation; our hypotheses are as follows: (i) butterflies that occur in the rainforest should be more melanic than those occurring exclusively in the white forest since in warmer, more sun-exposed environments, such as the xeric white forest, insects are expected to be brighter to avoid overheating (Schmitz, 1994; Heidrich et al., 2018), while in moist environments, such as the tropical rainforest, butterflies should tend to be darker (Stelbrink et al., 2019) as a form of protection against parasites; (ii) also due to thermoregulatory advantages (Zeuss et al., 2014; Stelbrink et al., 2019), differences in melanism between dorsal and ventral wing surfaces should be more pronounced in more heat-stressful environments (Tsai et al., 2020), such as the xeric white forest; finally, (iii) in highly shaded environments, such as rainforests, in opposition to considerably leafless ecosystems, such as the xeric white forest, individuals should have more saturated colors to enhance their contrast against the green dappled foliage background and compensate for the greenish illuminant created by sunlight filtering through the treetops (Endler, 1993).
2. Materials and methods
This study was approved by the UFRN's Animal Ethics Committee (CEUA, protocol number 013/2020) and followed all legal requirements of Brazilian law (number 11.794/2008).
2.1. Selection of specimens
We used well-preserved butterfly specimens from the scientific collection of the Laboratory of Ecology and Evolution of Butterflies, of the Federal University of Rio Grande do Norte (LEEB-UFRN), from which we also assembled information regarding the municipality, locality, date, and geographic coordinates regarding each specimen's capture.
We also checked the occurrence of species according to the available literature to identify those species that occurred in both biomes, in opposition to those occurring in only one biome: the Brazilian Atlantic Forest (referred to here as tropical rainforest) and the Brazilian Caatinga (referred to here as a xeric white forest). The taxonomic classification of the species followed Lamas (2004) and Mielke (2005).
2.2. Morphoclimatic and phytogeographic domains
The Brazilian Caatinga (hereafter referred to as xeric white forest), a kind of semi-arid forest/scrubland, is a poorly studied and highly threatened biome (Olson et al., 2001; Leal et al., 2003). This xeric and thorny scrub forest, which consists mostly of cacti and small thorny trees that lose their leaves seasonally, presents a high degree of floristic endemism with extreme meteorological patterns, which make it completely devoid of leaves in the dry season, when only the gray of the trunks and branches are evident, which made it known in the native-American language by the name of Caatinga (i.e., white forest; Prado, 2003). Rainfall in Caatinga (300–1,000 mm/per year) is usually concentrated for about 3–5 months (Sampaio, 1995), with an annual mean temperature of 28°C (Sampaio, 1995; Campos and Andrade, 2021).
The Brazilian Atlantic Forest (hereafter referred to as tropical rainforest), a tropical and subtropical moist broadleaf forest biome (Olson et al., 2001), has a hot and humid climate without a defined dry season, usually occupies regions of lower altitude (<1,000 m) and extends from northeast to southeast Brazil. Currently, the Atlantic forest is restricted to ~98,000 km2, or 7.6% of its original extension, and the last remnants of the forest still suffer intense anthropic pressure and imminent risk of extinction (Ministry of the Environment of Brazil, 1999), showing high levels of endemism (Morellato and Haddad, 2000) that exceed those found on most of the Amazon Forest, in such a way that the Atlantic forest, with its high biodiversity and elevated risk of extinction, is deemed as a hotspot (Sechrest et al., 2002). Precipitation in the tropical rainforest is 1.455 mm/per year, with an annual mean temperature of 26°C.
2.3. Color metrics quantification
We adopted a well-established protocol (Stevens et al., 2007) to obtain standardized photographs using a Canon EOS REBEL T2i camera (Canon 550D), coupled with an EF-S18-55mm lens and mounted on a tripod. Individually, and in a sequential manner, we chose one specimen from 121 different species of butterflies, and positioned each of them on a gray background, next to a color chart (X-Rite ColorChecker Passport), before capturing digital images of their dorsal and ventral surfaces (see Supplementary Figure S1 for a pictorial explanation on how the parameters were obtained). The use of a color chart ensured the maintenance of color information fidelity and allowed us to balance distortions caused by the camera's sensors and ambient lighting variation. After verifying the occurrence and geographic distribution of these 121 species of butterflies, data from those that did not occur in both environments (N = 46; Supplementary Table S1) were selected, rejecting those co-occurring in both biomes (N = 75; Supplementary Table S2). We evaluated each photo regarding its exposure through RawTherapee 5.4 software, converted the digital files to 8 bits, and obtained gray values for each pixel by using ImageJ software (Schneider et al., 2012). This procedure of 8-bit conversion and scaling of values followed what has been done in previous articles (Zeuss et al., 2014; Stelbrink et al., 2019). After photo calibration in ImageJ, we used a custom-made R package (Mello et al., 2022) to select regions of interest (i.e., wing area, excluding the thoracic and the abdominal region of the specimen) and extracted calibrated RGB values for each pixel. From each scaled image, we determined the mean gray value for each channel (R, G, and B), and then calculated the overall mean gray value by averaging the three channels. In the RGB system, lighter pixels are coded by higher values, while darker pixels lead to lower values, so the lower the mean gray value, the greater the melanism presented by the animal (each channel varies from 0 to 255).
Furthermore, we used a kernel density estimation method to extract the most common (mode) RGB triplet pixel values (see Mello et al., 2022 for details). Therefore, in cases where wing coloration has many different color patches, this method allows for capturing the RGB values of the most common color patch. We then used these RGB values to estimate the color saturation by calculating the mean absolute difference between channels divided by the overall mean gray value (as calculated earlier). This metric varies from zero to two, with higher values indicating higher color saturation. Finally, we measured the relative contribution of each channel by dividing each value by the sum of all three channels.
2.4. Statistical analysis
We performed Bayesian phylogenetic multilevel statistical analyses. We extracted the phylogeny from Chazot et al. (2019) and obtained a new tree with an “ape” package (Paradis and Schliep, 2019) using species phylogenetically close to ours. We generated a branch size distance matrix which was used to model their phylogenetic dependence in our statistical analyses. We ran one model for each color metric (mean gray value, saturation, R proportion, G proportion, and B proportion). The color metric was entered as the response variable. Wing side (dorsal or ventral), biome (tropical rainforest or xeric white forest), and their interaction entered as population-level effects (fixed effects). The species identification and the variance-covariance matrix of phylogenetic distances were entered as group-level effects (random effects). To fulfill statistical assumptions, we log-transformed mean gray value and square-root transformed saturation before analyses. Furthermore, because there was evidence of a difference in variance between wing sides for saturation, we included sigma as a function of the wing side in the saturation analysis. We ran models in R (v4.2.2; R Core Team, 2022) using the package brms (v2.18.0; Bürkner, 2018), which implements Bayesian models in Stan (Carpenter et al., 2017). For each model, we ran four independent chains for 10,000 iterations, 5,000 as a warm-up, in a total of 20,000 sampled iterations. We set weakly informative priors scaled to our data. We evaluated model fits by checking chain convergence, the presence of transitions with diverging errors, visual posterior predictive checks, and leave-one-out cross-validation. Figures were produced with ggplot2 and introdataviz packages (Wickham, 2016; Nordmann et al., 2021). Statistical estimates are shown in the original, back-transformed, scale as the mode and the 95% high-density credible interval.
3. Results
3.1. Mean gray value
The statistical analysis indicated a lower mean gray value for the dorsal wing surfaces of butterflies from the tropical rainforest (n = 31 species) when compared to xeric white forest butterflies (n = 15 species) (Figure 1 and Supplementary Figure S2; mode and 95% high-density credible interval: −11 [−28, −1]). For the ventral side, although in the same direction as the dorsal side, the difference between biomes does not exclude the possibility of no difference at 95% CI (−6 [−21, 6]).
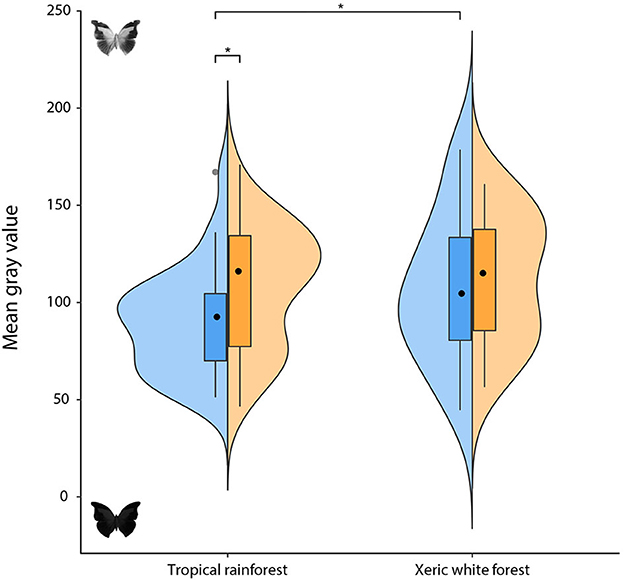
Figure 1. Mean gray value of butterflies from two biomes (tropical rainforest and xeric white forest). Blue and orange violin areas represent the frequency distribution of values for the dorsal and the ventral sides of butterflies' wings, respectively. Boxes indicate interquartile ranges, whiskers show 95% confidence intervals, and median values are represented by black dots. Gray dots represent outliers, while asterisks and brackets indicate statistically significant differences. The lower the mean gray value, the greater the melanism exhibited.
Furthermore, while butterflies from the xeric white forest did not show clear differences between their dorsal and ventral wing surfaces (−1 [−11, 7]), we found the wings of tropical rainforest butterflies to have darker dorsal than ventral surfaces (Figure 1 and Supplementary Figure S2; −7 [−15, −3]).
3.2. Color saturation
In general, we found that, on average, butterfly wings have similar saturation values. The statistical analysis did not show any clear difference between biomes (i.e., rainforest vs. white forest), either for the dorsal (mode and 95% CI: 0.02 [−0.12, 0.14]) or ventral wing surfaces (0.07 [−0.02, 0.15]). We also did not find support for differences between wing surfaces (i.e., dorsal vs. ventral) from the rainforest (−0.03 [−0.11, 0.06]) and the white forest (0.02 [−0.09, 0.14]). We found, however, the dorsal wing side to present a higher variance between species than the ventral side (Supplementary Figure S2; dorsal side estimated sigma of 0.15 [0.12, 0.19]; ventral side estimated sigma of 0.09 [0.06, 0.12]).
In addition, we did not find evidence for differences in the proportion of R, G, and B between locations (i.e., rainforest vs. white forest; dorsal R: 0.010 [−0.027, 0.039]; dorsal G: −0.009 [−0.026, 0.011]; dorsal B: 0.002 [−0.022, 0.026]; ventral R: 0.019 [−0.014, 0.052]; ventral G: 0.000 [−0.020, 0.017]; ventral B: −0.015 [−0.039, 0.008]) nor wing side (i.e., dorsal vs. ventral; rainforest R: 0.001 [−0.022, 0.025]; rainforest G: −0.001 [−0.020, 0.017]; rainforest B: 0.009 [−0.006, 0.024]; white forest R: 0.013 [−0.020, 0.047]; white forest G: −0.004 [−0.022, 0.017]; white forest B: −0.009 [−0.030, 0.012]).
4. Discussion
The comparison of butterfly assemblages from each biome did show differences regarding the melanism of their wings, confirming our first hypothesis and previous evidence showing that the lightness of butterflies' wings contrasts between different ecosystems, a trait that has been linked to thermoregulation (Tsai et al., 2020). Our expectation was that tropical rainforest butterflies should be more melanic (i.e., to present lower mean gray values) to counteract a higher parasitic rate found in humid environments (Stelbrink et al., 2019). Studies have shown that there is a pleiotropic effect between melanocortins and the immune function of animals (Ducrest et al., 2008) and that more melanic animals could defend themselves against parasites by strengthening their immunocompetence or directly preventing the proliferation of parasites due to the toxicity of melanin molecules (Côte et al., 2018). In addition, we also expected that white forest butterflies should be less melanic to avoid overheating (Schmitz, 1994; Forsman et al., 2002; Heidrich et al., 2018) since the xeric white forest is notoriously known for its high solar radiation, high average annual temperature, low cloud cover, and low relative humidity rates (Prado, 2003).
Our data also revealed that butterflies from the rainforest have wings that are significantly more melanic on their dorsal faces when compared to their own ventral wing surfaces, but the same was not true for white forest assemblies, which is the opposite of what we would expect according to our second hypothesis. Differences in melanism between dorsal and ventral wing surfaces have been associated with thermoregulatory advantages (Tsai et al., 2020), in such a way that heat-stressful environments, such as the xeric white forest, should have selected for more prominent differences, although it has also been observed that butterflies from regions with less sun exposure tend to be darker (Dalrymple et al., 2018). Here it is worth noting that the studies that proposed the relationship between melanism and thermoregulatory strategies were based on assemblages of butterflies from colder regions (Zeuss et al., 2014; Stelbrink et al., 2019; Tsai et al., 2020), a rule that might not fully apply to tropical environments. Having a darker dorsal surface and a lighter ventral area might also be a thermoregulatory adaptation related to more heterogenous biomes, which consist of different phytophysiognomies, as in the case of the tropical rainforest. The tropical moist broadleaf forests biome (Olson et al., 2001) presents two distinct phytophysiognomies that were considered in this study, the Coastal Atlantic Forest, a true tropical rainforest, with tall trees that form high and closed canopies, and the Atlantic Coast Restinga, a type of coastal tropical forest, characterized by medium-sized trees and shrubs adapted to drier conditions. Those tropical rainforest butterflies that frequently transition from a dryer and open phytophysiognomy (e.g., the Atlantic Coast Restinga) to another that is much more shaded and humid (e.g., Coastal Atlantic Forest) should benefit from the thermoregulatory advantages (Tsai et al., 2020) of having a darker dorsum and a lighter ventral surface. If these interpretations are correct, it would be expected that butterflies inhabiting both biomes (i.e., rainforest and white forest) would also show differences in melanism between their ventral and dorsal wing surfaces. Indeed, a posteriori analysis, following the same method described in this study, but using 75 butterfly species that occur in both biomes (Supplementary Table S2; the statistical analysis used the taxonomy as a proxy for the phylogenetic dependence), showed a significant difference in melanism between their dorsal and ventral wing surfaces (9.89 [4.37, 19.4]), with a darker dorsum and a lighter ventral surface (Figure 2 and Supplementary Figure S2). In contrast, for a considerably dryer, open, and leafless biome, like what is found in the Xeric Shrublands (Olson et al., 2001), known in Brazil as the Caatinga or white forest, a tendency to show a similar degree of melanism on both sides of their wings (Figure 1) could be understood as a strategy to avoid desiccation and UV damage (Majerus, 1998).
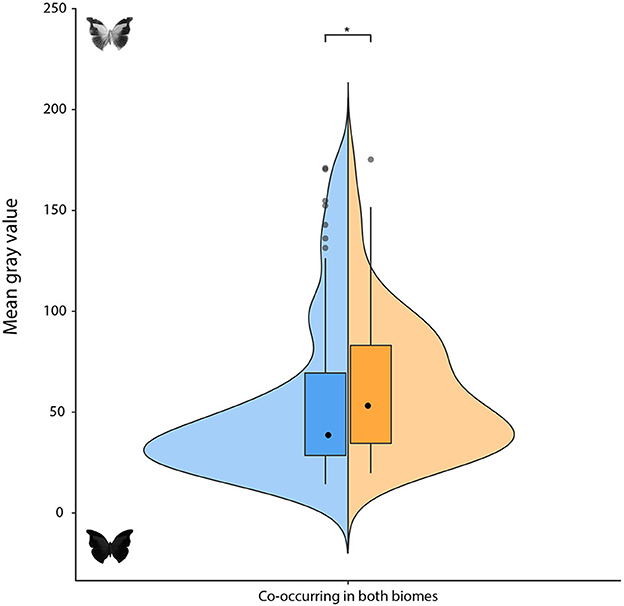
Figure 2. Mean gray value of butterflies co-occurring in both biomes (tropical rainforest and xeric white forest). Other conventions as in Figure 1.
An alternative explanation for the difference in melanism between the dorsal and the ventral faces of rainforest butterflies' wings would be that it works as an anti-predatory strategy (Murali, 2018), making it difficult for predators to capture them (Pinheiro et al., 2016). When butterflies present a different pattern of melanism between ventral and dorsal areas they can produce an effect called dynamic flash when flapping their wings during flight, which would help to confuse predators about their exact location (Murali and Kodandaramaiah, 2020). Although literature still lacks studies comparing the predation pressure of butterflies in different tropical environments, we believe is reasonable to guess that the abundance of butterfly predators is greater in the rainforest than in the white forest, due to the greater general diversity of species found in the rainforest, which would be in line with our proposition.
Even though the latitude in which our specimens were captured did not vary much (Supplementary Tables S1, S2), and despite the fact that the solar incidence of different regions of the Brazilian northeast is comparably high (Lopo et al., 2014), differences in solar incidence between localities with diverse kinds of phytophysiognomies were expected (although the solar incidence was not directly accessed in the present study), since vegetation cover reduces the intensity of sunlight and considerably decreases the proportion of short wavelength light from the illuminant (Endler, 1993). However, our third hypothesis, stating that shaded environments (i.e., rainforest) should have butterflies with more saturated colors, was not corroborated.
Since the illuminant spectrum also influences the production of animal coloration (Endler, 1993), changes in the geometry of canopies, resulting from deforestation, could also influence the dynamics and spatial distribution of organisms of different colors (Spaniol et al., 2019). Spaniol et al. (2020) showed that degraded environments might be experiencing a loss in the diversity of butterflies' color patterns since in degraded and more exposed environments butterflies with more conspicuous colors may have been reduced by predator activity. In line with this, we have found that butterflies' dorsal wing sides present a higher variance between species (regardless of the environment) when compared to their ventral wing side. On the one hand, a lower color variance on ventral wing surfaces might be seen as an indication that some ecological factor (e.g., predation avoidance) is exerting a strong homogenizing force on ventral wing coloration. On the other hand, for dorsal wing surfaces, a greater variety of active selective forces (e.g., thermoregulation and socio-sexual signaling) could explain the existence of a higher color variance.
To our knowledge, this is the first study to compare the coloration of butterfly assemblages in humid and semi-arid tropical environments. Due to its occurrence in a variety of ecosystems, future research with Neotropical butterflies must be encouraged and should incorporate data on communities from savannahs, prairies, and floodlands, in addition to comparing butterfly populations from a specific biome (e.g., rainforests from the Northeast and the Southeast regions of Brazil), but that occur in very different latitudes (Zeuss et al., 2014). It would also be interesting to investigate the relationship that other body regions, such as butterflies' thorax and the basal region of their wings (Munro et al., 2019), have with melanism and color saturation, as well as how Neotropical butterflies reflect infrared radiation (see Kang et al., 2021). Furthermore, since most studies that assess macroecological patterns focus on the consequences of population dynamics and changes in coloration patterns (Zeuss et al., 2014; Pinkert et al., 2017; Xing et al., 2018), experiments that relate environmental degradation to the degree of melanism in butterfly populations and communities should also be pursued. Future studies could also include visual modeling techniques to assess how changes in the sensory environment of organisms (e.g., changes in vegetation structure and environmental light) may affect the trade-off between animal communication, predation, and thermoregulation.
Data availability statement
The raw data supporting the conclusions of this article will be made available by the authors, without undue reservation.
Author contributions
SS: conceptualization, methodology, software, formal analysis, investigation, data curation, writing—original draft, and visualization. FG: formal analysis and writing—review and editing. MC: conceptualization, resources, and writing—review and editing. DP: conceptualization, methodology, resources, writing—review and editing, visualization, supervision, project administration, and funding acquisition. All authors contributed to the article and approved the submitted version.
Funding
This study was financed in part by the Coordenacao de Aperfeicoamento de Pessoal de Nivel Superior – Brasil CAPES (Finance Codes 001 and 043/2012), by Conselho Nacional de Desenvolvimento Cientifico e Tecnologico – Brasil (CNPq) (Finance Codes 478222/2006-8 and 474392/2013-9), and by Programa de Apoio aos Nucleos de Excelencia – FAPERN/CNPq (Finance Code 25674/2009). An M.Sc. Scholarship was granted to SS and a Researcher Scholarship was granted to DP, both by Conselho Nacional de Desenvolvimento Cientifico e Tecnologico – Brasil CNPq.
Acknowledgments
We are deeply indebted to L.N. dos Santos and all staff of the Laboratory of Ecology and Evolution of Butterflies, UFRN, for granting us access to their scientific collection during the stressful period of the COVID-19 pandemic. We also thank C.A. Iserhard for helping with the phylogenies.
Conflict of interest
The authors declare that the research was conducted in the absence of any commercial or financial relationships that could be construed as a potential conflict of interest.
Publisher's note
All claims expressed in this article are solely those of the authors and do not necessarily represent those of their affiliated organizations, or those of the publisher, the editors and the reviewers. Any product that may be evaluated in this article, or claim that may be made by its manufacturer, is not guaranteed or endorsed by the publisher.
Supplementary material
The Supplementary Material for this article can be found online at: https://www.frontiersin.org/articles/10.3389/fevo.2023.932755/full#supplementary-material
References
Ab'Saber, A. N. (1967). Domínios morfoclimáticos e províncias fitogeográficas no Brasil. Orientação 3, 45–48.
Brakefield, P. M., and Willmer, P. G. (1985). The basis of thermal melanism in the ladybird Adalia bipunctata: differences in reflectance and thermal properties between the morphs. Heredity 54, 9–14. doi: 10.1038/hdy.1985.3
Bürkner, P. C. (2018). Advanced bayesian multilevel modeling with the R package brms. R J. 10, 395–411. doi: 10.32614/RJ-2018-017
Campos, D. A., and Andrade, E. M. (2021). Seasonal trend of climate variables in an area of the Caatinga phytogeographic domain. Revista Agro@mbiente On-line 15, 1–18. doi: 10.18227/1982-8470ragro.v15i0.6833
Carpenter, B., Gelman, A., Hoffman, M. D., Lee, D., Goodrich, B., Betancourt, M., et al. (2017). Stan: a probabilistic programming language. J. Stat. Softw. 76, 7223. doi: 10.18637/jss.v076.i01
Chazot, N., Wahlberg, N., Freitas, A. V. L., Mitter, C., Labandeira, C., Sohn, J.-C., et al. (2019). Priors and posteriors in bayesian timing of divergence analyses: the age of butterflies revisited. Syst. Biol. 68, 797–813. doi: 10.1093/sysbio/syz002
Clusella-Trullas, S., van Wyk, J. H., and Spotila, J. R. (2007). Thermal melanism in ectotherms. J. Therm. Biol. 32, 235–245. doi: 10.1016/j.jtherbio.2007.01.013
Côte, J., Boniface, A., Blanchet, S., Hendry, A. P., Gasparini, J., and Jacquin, L. (2018). Melanin-based coloration and host–parasite interactions under global change. Proc. R. Soc. B. 285, 20180285. doi: 10.1098/rspb.2018.0285
Dalrymple, R. L., Flores-Moreno, H., Kemp, D. J., White, T. E., Laffan, S. W., Hemmings, F. A., et al. (2018). Abiotic and biotic predictors of macroecological patterns in bird and butterfly coloration. Ecol. Monogr. 88, 204–224. doi: 10.1002/ecm.1287
Ducrest, A. L., Keller, L., and Roulin, A. (2008). Pleiotropy in the melanocortin system, coloration and behavioural syndromes. Trends Ecol. Evol. 23, 502–510. doi: 10.1016/j.tree.2008.06.001
Endler, J. (1992). Signals, signal conditions, and the direction of evolution. Am. Nat. 139, S125–S153.
Endler, J. A. (1982). Convergent and divergent effects of natural selection on color patterns in two fish faunas. Evolution 36, 178–188. doi: 10.2307/2407979
Endler, J. A. (1993). The color of light in forests and its implications. Ecol. Monogr. 63, 1–27. doi: 10.2307/2937121
Forsman, A., Ringblom, K., Civantos, E., and Ahnesjo, J. (2002). Coevolution of color pattern and thermoregulatory behavior in polymorphic pygmy grasshoppers Tetrix undulata. Evolution 56, 349–360. doi: 10.1111/j.0014-3820.2002.tb01345.x
Hanley, D., Miller, N. G., Flockhart, D. T. T., and Norris, D. R. (2013). Forewing pigmentation predicts migration distance in wild-caught migratory monarch butterflies. Behav. Ecol. 24, 1108–1113. doi: 10.1093/beheco/art037
Heidrich, L., Friess, N., Fiedler, K., Brändle, M., Hausmann, A., Brandl, R., et al. (2018). The dark side of lepidoptera: colour lightness of geometrid moths decreases with increasing latitude. Glob. Ecol. Biogeogr. 27, 407–416. doi: 10.1111/geb.12703
Hoekstra, H. E. (2006). Genetics, development and evolution of adaptive pigmentation in vertebrates. Heredity 97, 222–234. doi: 10.1038/sj.hdy.6800861
Kang, C., Im, S., Lee, W. Y., Choi, Y., Stuart-Fox, D., and Huertas, B. (2021). Climate predicts both visible and near-infrared reflectance in butterflies. Ecol. Lett. 24, 1869–1879. doi: 10.1111/ele.13821
Kettlewell, H. (1955). Selection experiments on industrial melanism in the Lepidoptera. Heredity 9, 323–342. doi: 10.1038/hdy.1955.36
Kingsolver, J. G. (1983). Thermoregulation and flight in Colias butterflies: elevational patterns and mechanistic limitations. Ecology 64, 534–545. doi: 10.2307/1939973
Lamas, G. (2004). “Checklist: Part 4A. Hesperioidea-papilionoidea,” in Atlas of Neotropical Lepidoptera, ed J. B. Heppner (Gainesville: Association for Tropical Lepidoptera/Scientific Publishers).
Leal, I. R., Tabarelli, M., and Silva, J. M. C. (2003). Ecologia e conservação da Caatinga. Recife: Editora Universitária, Universidade Federal de Pernambuco.
Lopo, A. B., Spyrides, M. H. C., Lucio, P. S., and Silva, S. M. P. (2014). Uv extreme events in northeast of Brazil. Ciência E Natura 36, 482–490. doi: 10.5902/2179460X12816
McGraw, K. J. (2006). “Mechanics of melanin-based coloration,” in Bird Coloration. Volume 1 Mechanisms and measurements, eds G. E. Hill, and K. J. McGraw (Cambridge, MA: Harvard University Press). doi: 10.2307/j.ctv22jnscm.9
Mello, N. D., Sanchez, L. G. F., and Gawryszewski, F. M. (2022). Spatio-temporal colour variation of arthropods and their environment. Evol. Ecol. 36, 117–133. doi: 10.1007/s10682-021-10144-7
Mielke, O. H. H. (2005). Catalogue of the American Hesperioidea: Hesperiidae (Lepidoptera). Curitiba: Sociedade Brasileira de Zoologia.
Ministry of the Environment of Brazil (1999). Diretrizes Para a Política de Conservação e Desenvolvimento Sustentável da Mata Atlântica. Brasília: MMA, Caderno No. 13.
Morellato, L. P. C., and Haddad, C. F. B. (2000). Introduction: the Brazilian Atlantic forest. Biotropica 32, 786–792. doi: 10.1111/j.1744-7429.2000.tb00618.x
Munro, J. T., Medina, I., Walker, K., Moussalli, A., Kearney, M. R., Dyer, A. G., et al. (2019). Climate is a strong predictor of near-infrared reflectance but a poor predictor of colour in butterflies. Proc. R. Soc. B 286, 20190234. doi: 10.1098/rspb.2019.0234
Murali, G. (2018). Now you see me, now you don't: dynamic flash coloration as an antipredator strategy in motion. Anim. Behav. 142, 207–220. doi: 10.1016/j.anbehav.2018.06.017
Murali, G., and Kodandaramaiah, U. (2020). Size and unpredictable movement together affect the effectiveness of dynamic flash coloration. Anim. Behav. 162, 87–93. doi: 10.1016/j.anbehav.2020.02.002
Nordmann, E., McAleer, P., Toivo, W., Paterson, H., and DeBruine, L. (2021). Data visualization using R, for researchers who don't use R. Adv. Methods Pract. Psychol. Sci. 5, 25152459221074654. doi: 10.31234/osf.io/4huvw
Olson, D. M., Dinerstein, E., Wikramanayake, E. D., Burgess, N. D., Powell, G. V. N., Underwood, E. C., et al. (2001). Terrestrial ecoregions of the world: a new map of life on earth. BioScience 51, 933–938. doi: 10.1641/0006-3568(2001)051[0933:TEOTWA]2.0.CO;2
Paradis, E., and Schliep, K. (2019). Ape 5.0: an environment for modern phylogenetics and evolutionary analyses in R. Bioinformatics 35, 526–528. doi: 10.1093/bioinformatics/bty633
Pinheiro, C. E. G., Freitas, A. V. L., Campos, V. C., DeVries, P. J., and Penz, C. M. (2016). Both palatable and unpalatable butterflies use bright colors to signal difficulty of capture to predators. Neotrop. Entomol. 45, 107–113. doi: 10.1007/s13744-015-0359-5
Pinkert, S., Brandl, R., and Zeuss, D. (2017). Colour lightness of dragonfly assemblages across North America and Europe. Ecography 40, 1110–1117. doi: 10.1111/ecog.02578
Prado, D. E. (2003). “As caatingas da América do Sul,” in Ecologia e Conservação da Caatinga, eds I. R. Leal, M. Tabarelli, and J. M. C. Silva (Recife: Universitária da UFPE), 3–73.
R Core Team (2022). R: A Language and Environment for Statistical Computing. Vienna: R Foundation for Statistical Computing. Available online at: https://www.R-project.org/
Sampaio, E. V. S. B. (1995). “Overview of the Brazilian caatinga,” in Seasonally Dry Tropical Forests, eds S. H. Bullock, H. A. Mooney, and E. Medina (Londres: Cambridge University Press), 35–58.
Schmitz, H. (1994). Thermal characterization of butterfly wings: absorption in relation to different color, surface structure and basking type. J. Therm. Biol. 19, 403–412. doi: 10.1016/0306-4565(94)90039-6
Schneider, C. A., Rasband, W. S., and Eliceiri, K. W. (2012). NIH image to ImageJ: 25 years of image analysis. Nat. Methods 9, 671–675. doi: 10.1038/nmeth.2089
Sechrest, W., Brooks, T. M., da Fonseca, G. A. B., Konstant, W. R., Mittermeier, R. A., Purvis, A., et al. (2002). Hotspots and the conservation of evolutionary history. Proc. Nat. Acad. Sci. USA 99, 2067–2071. doi: 10.1073/pnas.251680798
Spaniol, R. L., Duarte, L. S., Mendonça, M. S., and Iserhard, C. A. (2019). Combining functional traits and phylogeny to disentangling Amazonian butterfly assemblages on anthropogenic gradients. Ecosphere 10, e02837. doi: 10.1002/ecs2.2837
Spaniol, R. L., Mendonça, M. S., Hartz, S. M., Iserhard, C. A., and Stevens, M. (2020). Discolouring the Amazon Rainforest: how deforestation is affecting butterfly coloration. Biodiv. Conserv. 29, 2821–2838. doi: 10.1007/s10531-020-01999-3
Stelbrink, P., Pinkert, S., Brunzel, S., Kerr, J., Wheat, C. W., Brandl, R., et al. (2019). Colour lightness of butterfly assemblages across North America and Europe. Sci. Rep. 9, 1760. doi: 10.1038/s41598-018-36761-x
Stevens, M., Párraga, C. A., Cuthill, I. C., Partridge, J. C., and Troscianko, T. S. (2007). Using digital photography to study animal coloration. Biol. J. Linn. Soc. 90, 211–237. doi: 10.1111/j.1095-8312.2007.00725.x
True, J. R. (2003). Insect melanism: the molecules matter. Trends Ecol. Evol. 18, 640–647. doi: 10.1016/j.tree.2003.09.006
Tsai, C. C., Childers, R. A., Nan Shi, N., Ren, C., Pelaez, J. N., Bernard, G. D., et al. (2020). Physical and behavioral adaptations to prevent overheating of the living wings of butterflies. Nat. Commun. 11, 551. doi: 10.1038/s41467-020-14408-8
Watt, W. (1964). Pteridine components of wing pigmentation in the butterfly Colias eurytheme. Nature 201, 1326–1327. doi: 10.1038/2011326b0
Wickham, H. (2016). ggplot2: Elegant Graphics for Data Analysis. New York, NY: Springer. doi: 10.1007/978-3-319-24277-4
Wiernasz, D. C. (1995). Male choice on the basis of female melanin pattern in Pieris butterflies. Anim. Behav. 49, 45–51. doi: 10.1016/0003-3472(95)80152-9
Xing, S., Bonebrake, T. C., Ashton, L. A., Kitching, R. L., Cao, M., Sun, Z., et al. (2018). Colors of night: climate–morphology relationships of geometrid moths along spatial gradients in southwestern China. Oecologia 188, 537–546. doi: 10.1007/s00442-018-4219-y
Xing, S., Bonebrake, T. C., Tang, C. C., Pickett, E. J., Cheng, W., Greenspan, S. E., et al. (2016). Cools habitats support darker and bigger butterflies in Australian tropical forests. Ecol. Evol. 6, 8062–8074. doi: 10.1002/ece3.2464
Keywords: photography, ImageJ, sexual selection, sensory ecology, lepidoptera, Caatinga, Atlantic forest, camouflage
Citation: Schirmer SC, Gawryszewski FM, Cardoso MZ and Pessoa DMA (2023) Melanism and color saturation of butterfly assemblages: A comparison between a tropical rainforest and a xeric white forest. Front. Ecol. Evol. 11:932755. doi: 10.3389/fevo.2023.932755
Received: 30 April 2022; Accepted: 16 January 2023;
Published: 16 February 2023.
Edited by:
David Andrew Gray, California State University, Northridge, United StatesReviewed by:
Doekele G. Stavenga, University of Groningen, NetherlandsEduardo Carneiro, Federal University of Paraná, Brazil
Copyright © 2023 Schirmer, Gawryszewski, Cardoso and Pessoa. This is an open-access article distributed under the terms of the Creative Commons Attribution License (CC BY). The use, distribution or reproduction in other forums is permitted, provided the original author(s) and the copyright owner(s) are credited and that the original publication in this journal is cited, in accordance with accepted academic practice. No use, distribution or reproduction is permitted which does not comply with these terms.
*Correspondence: Daniel Marques Almeida Pessoa, ZGFuaWVsLnBlc3NvYUB1ZnJuLmJy