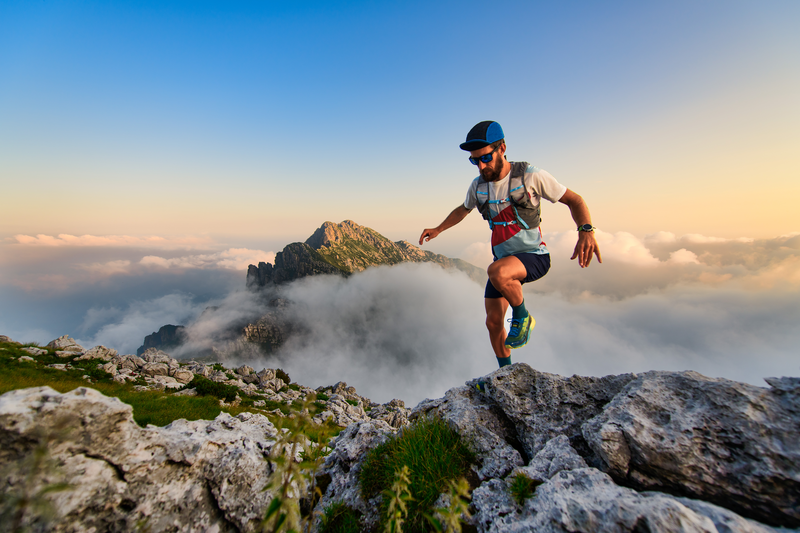
94% of researchers rate our articles as excellent or good
Learn more about the work of our research integrity team to safeguard the quality of each article we publish.
Find out more
ORIGINAL RESEARCH article
Front. Ecol. Evol. , 09 January 2024
Sec. Paleoecology
Volume 11 - 2023 | https://doi.org/10.3389/fevo.2023.1338865
This article is part of the Research Topic Changes in Plant-Herbivore Interactions Across Time Scales: Bridging Paleoecology and Contemporary Ecology View all 6 articles
Plants and insects are the most diverse and ecologically important organisms in the terrestrial biosphere. Their interactions are also among the richest biotic relationships, and offer significant insights into the evolution of terrestrial ecosystem complexity through the geological record. This investigation of the late Rhaetian Xujiahe and the earliest Jurassic Zhenzhuchong floral assemblages provides the first data on foliar herbivory generated by terrestrial arthropods across the Triassic–Jurassic transition in the eastern Tethys (East Asia) region. The damage types from two fossil assemblages are collectively attributed to seven functional feeding and egg-laying categories (i.e., hole feeding, margin feeding, surface feeding, skeletonization, piercing and sucking, oviposition, and galling). Most feeding strategies are spread across the major plant groups and persist through the Triassic–Jurassic boundary, with the exception of skeletonization (a category of external foliage feeding), which was restricted to the latest Triassic within dipteridacean ferns. The survey reveals that the respective frequency and diversity of interactions between plants and insects prior to and following the end-Triassic mass extinction event are almost the same, despite a substantial turnover of floral components. This suggest that insect herbivores were largely able to transfer to alternative (but commonly related) plant groups during the dramatic floristic turnover and environmental changes at the end of the Triassic. Sporadic occurrences of foliar modifications, such as marginal cusps on pinnules of Pterophyllum and prominent ridges on the rachises of some ferns and bennettites are interpreted as adaptations for defense against insect herbivores. A few differences in taxonomic composition and herbivory representation between the latest Triassic Xujiahe flora and the earliest Jurassic Zhenzhuchong flora are more likely to be related to collection and preservational biases rather than reflecting palaeoecological changes. We encourage further investigations exploring the distribution of insect damage in fossil floras from other palaeolatitudinal zones and spanning other major extinction events to develop a better understanding of terrestrial ecosystem responses to major crises in Earth’s history.
The Triassic–Jurassic transition is characterized by one of the five major extinctions of the Phanerozoic—the so-called end-Triassic extinction (ETE: Sepkoski, 1996; McGhee et al., 2013). The event is characterized by the disappearance of almost 300 families of marine invertebrates and vertebrates, along with major extinctions or dramatic representational changes in terrestrial realms (Benton, 1995; McElwain et al., 1999; Olsen et al., 2002; McElwain et al., 2007; van de Schootbrugge et al., 2009; Lindström et al., 2012; Peng et al., 2018; Li et al., 2020; Zhou et al., 2021). Additionally, some groups of plants show abnormal abundances or morphological changes preceding the extinction event that reflect strong perturbations in plant physiology or ecology associated with the deepening environmental crisis (Vajda et al., 2023). The emplacement of the Central Atlantic Magmatic Province (CAMP) volcanism, releasing greenhouse gases (e.g., CO2, CH4) in the order of c. 3000 Gt of carbon, and raising atmospheric pCO2 by up to c. 2400 ppm (Rampino et al., 2023), has been generally considered as the dominant trigger for the extreme environmental conditions experienced during the end-Triassic biotic crisis (Marzoli et al., 2004; van de Schootbrugge et al., 2009; Ruhl et al., 2010; Ruhl et al., 2011; Greene et al., 2012; Percival et al., 2017; Lindström et al., 2019; Panfili et al., 2019; He et al., 2020; Ruhl et al., 2020; Schoepfer et al., 2022).
The responses of the terrestrial biosphere and particular biological groups to this event are still debated. Questions remain as to the timing of events, the degree and abruptness of the extinctions, and how different trophic levels responded in both marine and terrestrial settings (McElwain et al., 2007; Lucas and Tanner, 2015; Barbacka et al., 2017). Although some biotic groups have been widely investigated, e.g., terrestrial vertebrates (Olsen et al., 1987; Olsen et al., 2002; Lucas and Tanner, 2015); corals (Lathuilière and Marchal, 2009); macrofloras (Rees et al., 2000; McElwain et al., 2007; Steinthorsdottir et al., 2015; Barbacka et al., 2017; Zhou et al., 2021) and microfloras (van de Schootbrugge et al., 2009; Mander et al., 2010; Mander et al., 2013; Vajda and Bercovici, 2014; Barbacka et al., 2017; Li et al., 2020), sparse stratigraphic sampling has enabled resolution of only generalized patterns of biotic turnover for other groups, e.g., insects (Labandeira and Sepkoski, 1993; Clapham et al., 2016; Condamine et al., 2016).
Plants and insects are the two most diverse and important terrestrial autotrophic and heterotrophic groups in the modern biosphere. Together, they represent around 70% of Earth’s biodiversity (Schoonhoven et al., 2005; Calatayud et al., 2018), and their intricate trophic network and close interactions play a vital role in the maintenance and evolution of terrestrial ecosystems (Labandeira and Wappler, 2023). Their body fossil record and their trace interactions on fossil plants provide us with important information on taxon and ecosystem evolution through time and space (Liu et al., 2015).
In the absence of a rich and continuous record of insect body fossils through most regions on Earth, the trace fossil record of plant-arthropod interactions potentially provides our richest source of information on the turnover of insect herbivore guilds through many of Earth’s major biotic crises. The earliest evidence of plant-insect interactions can be traced back to the latest Silurian to Early Devonian (Labandeira, 2007; Hagström and Mehlqvist, 2012). An obvious diversification of interactions occurred during the Late Carboniferous as new forest communities and novel seed-plant architectures and reproductive strategies were established (Labandeira, 2002). To date, the vast majority of studies on fossil plant-insect interactions represent incidental reports of specific examples of herbivory, oviposition, or other processes (Scott et al., 2004; Currano et al., 2008; Feng et al., 2014; McLoughlin et al., 2015; Gandolfo and Zamaloa, 2021). Fewer studies have attempted to resolve the patterns of change in insect herbivory through particular major Earth crises, e.g., the Permian–Triassic extinction (Krassilov and Karasev, 2008; Labandeira et al., 2016; Cariglino et al., 2021) and the end-Cretaceous impact event (Labandeira et al., 2002a; Labandeira et al., 2002b; Donovan et al., 2016; Donovan et al., 2018; Zambon et al., 2023). Few studies have specifically investigated changes in plant-insect associations across the end-Triassic event. Of these studies, Steinthorsdottir et al. (2015) reported the frequency change in endophytic oviposition (egg-laying traces) in ginkgoalean leaves across the Triassic–Jurassic transition at Astartekløft, East Greenland and noted a profound change in the ecosystem and food webs caused by environmental degradation at this time. Other studies have noted long-term shifts in the hosts of particular herbivore guilds (e.g., gallers) across this event (Labandeira, 2021), but data for the critical Rhaetian–Hettangian interval remains sparse (Labandeira, 2006; Labandeira, 2013). Clearly, if the patterns of biotic turnover and shifts in plant host-herbivore associations are to be resolved for this major perturbation in Earth’s history, higher-resolution sampling and analyses of plant-insect interactions are required for this key interval.
Terrestrial sequences spanning the Triassic–Jurassic transition are well developed in the northeastern Sichuan Basin, South China. These units (the Upper Triassic Xujiahe Formation and the Lower Jurassic Zhenzhuchong Formation) constitute one of the most continuous Triassic–Jurassic continental successions in the eastern Tethyan realm and have yielded diverse macro- and micro-floras (Ye et al., 1986; Wang et al., 2010; Li et al., 2020; Zhou et al., 2021; Lu et al., 2023), together with key fossil insects (Lin, 1978; Li et al., 2007; Fang et al., 2013). A magnetostratigraphic study dated the Xujiahe Formation as ranging from 207.2 Ma to 201.3 Ma in the Qilixia section at Xuanhan, northeastern Sichuan Basin, South China. At this locality, the Triassic–Jurassic boundary was placed between the Xujiahe and Zhenzhuchong formations (Li et al., 2017). A recent palynological investigation obtained results consistent with the magnetostratigraphic age interpretations by Li et al. (2017) and revealed the co-dominance of Cyatheaceae/Dicksoniaceae (ferns) and Cheirolepidiaceae (conifers) in the lower part of the Zhenzhuchong Formation—features typifying it as Early Jurassic (Hettangian–Sinemurian) in age (Li et al., 2020). This refined chronostratigraphic framework enables more detailed studies of the temporal changes in terrestrial ecosystems through the Triassic–Jurassic transition in the eastern Tethys (East Asia) region.
In recent years, we collected abundant and diverse leaf fossils with multiple insect damage features from the Xujiahe and Zhenzhuchong formations in the Qilixia section of Xuanhan County, northeastern Sichuan Basin, South China. Here we present the first analysis of plant-arthropod interactions from uppermost Triassic and lowermost Jurassic sedimentary strata in South China. We also provide preliminary interpretations of ecosystem changes across the Triassic–Jurassic transition in this region based on the composition of the macroflora and evidence from the representation of insect damage types.
The Sichuan Basin developed on the western margin of the South China Block in the eastern Tethyan region (Figure 1A) during the Late Triassic to Early Jurassic (Li et al., 2017). The basin covers c. 2.6 × 105 km2 in southwestern China, and occupies the eastern Sichuan Province and the majority of Chongqing City (Figure 1B). The Sichuan foreland basin formed during the Late Triassic in response to the collision of the South China Block, North China Block and other Asian plates. Foreland basin filling resulted in the shift of depositional environments from marine to terrestrial, and the sedimentary succession records numerous important environmental oscillations prior to and after the end-Triassic mass extinction (Wang et al., 2010).
Figure 1 Location of the Qilixia section at Xuanhan, northern Sichuan Basin, South China, and a geological map of the studied area along with the lithological column of the Qilixia section. (A) The palaeogeographical location of the studied area during the Latest Triassic (after Li et al., 2017). (B) Geographical extent of the Sichuan Basin and the location of the Qilixia section. (C) Simplified geological map of the studied section and its adjacent areas (after Wang et al., 2010). (D) Upper Triassic (Xujiahe Formation)–Lower Jurassic (Zhenzhuchong (ZZH) Formation) lithological column of the Qilixia section (after Li et al., 2020).
Upper Triassic to Lower Jurassic terrestrial lacustrine-fluvial to coal-swamp deposits are well-developed along the eastern and northeastern margins of the basin (Wang et al., 2010). This study focuses on the uppermost Triassic Xujiahe Formation and the lowermost Jurassic Zhenzhuchong Formation in the Qilixia section, one of the best-exposures of Upper Triassic–Lower Jurassic successions in the northeastern Sichuan Basin (Figures 1C, D). The Qilixia section (31°11′49″N, 107°44′37″E) is located close to Qili Town in Xuanhan County, Dazhou City (Figures 1B, C). In the Qilixia section, the Xujiahe Formation unconformably overlies the dolomitic strata of the Middle Triassic Leikoupo Formation, and it is conformably overlain by the Lower Jurassic siliciclastic Zhenzhuchong Formation (Figure 1D).
The Xujiahe Formation consists mainly of sandstones, siltstones, mudstones and coals, and is divided into seven informal lithostratigraphic members (Member I–VII according to local usage; Wang et al., 2010). Members I, III, V and VII consist of fine-grained sandstones, mudstones and coals, yielding rich and diverse plant fossils, indicating floodplain–lacustrine depositional settings (Huang, 1992; Wang et al., 2010; Figure 1D). Members II, IV and VI represent fluvial–deltaic strata, which are characterized by medium- to coarse-grained sandstones (Huang, 1992; Wang et al., 2010; Figure 1D). The Zhenzhuchong Formation is composed mainly of a thin basal bed of conglomerate and quartzose sandstones succeeded by feldspathic sandstones, siltstones, and several thin coal beds in the lower part (Wang et al., 2010; Figure 1D).
Collectively, 840 plant-bearing rock samples were recovered from the uppermost unit (Member VII) of the Xujiahe Formation (708 slabs) and the lowermost (Bed 2) Zhenzhuchong Formation (132 slabs) at the Qilixia section in the northeastern Sichuan Basin, South China (Figure 1D). The fossil plants from the Member VII of the Xujiahe Formation are assigned a late Rhaetian age based on magnetostratigraphic data (Li et al., 2017), whereas the fossil material from the Bed 2 of the Zhenzhuchong Formation is interpreted to be Hettangian–Sinemurian in age according to palynological results (Li et al., 2020). All specimens, registered as QLX2014001–QLX2014099, QLX2014287–QLX2014459 and QLX-2105-001–QLX-2105-033, QLX2105-211–QLX2105-745, are stored in the Palaeobotanical Collection in the Nanjing Institute of Geology and Paleontology, Chinese Academy of Sciences (NIGPAS). Only compression-impression floras are considered for this study. Permineralized/carbonized woods/stems/roots and samples with extremely fragmentary plant detritus were excluded from the quantitative analysis. Totally, 596 slabs containing 740 identifiable leaf fragments were analyzed (564 plant fossils from Member VII of the Xujiahe Formation and 176 specimens from Bed 2 of the Zhenzhuchong Formation; Appendix 1).
Each fragment was carefully examined and assigned to genus level for evaluation of the diversity and abundance of plant-arthropod interactions. Owing to the incomplete nature of many fossils making species assignments equivocal, identification to genus was deemed the most effective level for comparison of floras across the Triassic–Jurassic transition. The identification of damage features documented in this study follow the protocols and standard descriptive scheme of damage types (DTs) developed by Labandeira et al. (2007) – and updated versions of this atlas). Each damage type was classified into its corresponding functional feeding group (FFG). The catalogue of identified plant fossils and the presence of DTs on each plant fragment is presented in Appendix 1.
Photographs of plant fossils were taken with a Nikon Z7 digital camera with an Z 24–70 mm f/4S lens. Strong lateral lights at various angles were applied to highlight subtle morphological or herbivory features when needed. Standard graphics software (Adobe Photoshop® CC) was used only to correct brightness and enhance the contrast of images.
We identified 13 plant genera with insect damage features from the uppermost Triassic (upper Rhaetian) Xujiahe Formation in the Qilixia section: Equisetites, Dictyophyllum, Clathropteris, Cladophlebis, Pterophyllum, Anomozamites, Ptilophyllum, Zamites, Taeniopteris, Baiera, Ginkgoites, Sphenobaiera, Podozamites (Appendix 1). The flora is dominated by Bennettitales (i.e., Pterophyllum, Anomozamites, Ptilophyllum) and ferns (i.e., Dictyophyllum, Clathropteris, Cladophlebis), but it also contains abundant cycads (including Zamites and Taeniopteris), Ginkgoales (including Ginkgoites, Baiera, and Sphenobaiera) and conifers (Podozamites). Sphenopsids (Equisetites) are less common (Figures 2A, C).
Figure 2 Floral composition and proportion of fragments with insect damage on different plant groups across the Triassic–Jurassic boundary in the Qilixia section. (A) Late Rhaetian flora from the uppermost Xujiahe Formation. (B) Earliest Jurassic flora from the lowermost Zhenzhuchong Formation. (C) Proportion of fragments with DTs on different plant groups in the latest Triassic flora. (D) Proportion of fragments with DTs on different plant groups in the earliest Jurassic flora.
Twenty-eight DTs attributed to seven FFGs were identifiable on these plants (Figure 3A), i.e., hole feeding (DT01, DT02, DT03, DT05 and DT07), margin feeding (DT12, DT13, DT14, DT15, DT26 and DT81), surface feeding (DT28, DT29 and DT103), skeletonization (DT16 and DT21), piercing and sucking (DT46, DT48, DT77 and DT133), oviposition (DT100, DT100 and DT134) and galling (DT33, DT84, DT87, DT117 and DT122) (Appendix 1). External foliar feedings, such as hole feeding, margin feeding and surface feeding are most common herbivory categories (Figure 3C).
Figure 3 Diversity of damage types among the various plant groups and the proportion of functional feeding groups represented within the uppermost Triassic and Lower Jurassic assemblages. (A) Diversity of damage types in the late Rhaetian flora. (B) Diversity of damage types in the earliest Jurassic flora. (C) Proportion of functional feeding groups in the late Rhaetian flora. (D) Proportion of functional feeding groups in the earliest Jurassic flora.
Traces of arthropod damage were present on 30.67% of the 564 examined leaf fragments (Figure 2C). External feeding, such as hole feeding (DT01; Figure 4A), margin feeding (DT12, DT13, DT14, DT15; Figures 4A–F, H), surface feeding (DT29, DT103; Figures 4D, F) and skeletonization (DT16, DT21; Figures 4C, G) are the most common herbivory categories on ferns and sphenopsids. Skeletonization is unique to Dipteridaceae (Clathropteris and Dictyophyllum) in this assemblage and is expressed by a continuous reticulum of remnant secondary and tertiary veins enclosing polygonal areas of tissue removal with indistinct reaction rims (DT16; Figure 4C), or removal of the highest orders of venation with an absence of broad swaths of interveinal tissue (DT21; Figure 4G). Piercing and sucking, oviposition and galling are rare on ferns and sphenopsids in the assemblage, with only six occurrences recorded (Appendix 1), e.g., galling types DT84 and DT87 (Figures 4A, H, I), characterized by elongate or lenticular galls with various surface textures occurring on a major vein or twig.
Figure 4 Examples of various DTs on sphenopsids and ferns from Member VII of the Xujiahe Formation. (A) Hole feeding (DT01, arrow and area A1), margin feeding (DT12, arrow A2) and galling (DT87, arrow A3) on pinnules and rachis of Cladophlebis integra (Ôishi et Takahashi) Frenguelli, QLX2014316. (B) Margin feeding (DT12, arrows B1; DT14, arrow B2; DT15, arrow B3) on pinnules of Cladophlebis denticulata (Brongniart) Nathorst, QLX2014322. (C) Margin feeding (DT12, arrow C1) and skeletonization (DT16, area C2) on pinna of Clathropteris platyphylla (Göeppert) Brongniart, QLX2014330. (D) Margin feeding (DT13, arrows D1) and surface feeding (DT103, arrows D2) on pinnules of Cladophlebis scariosa Harris, QLX2014455. (E) Margin feeding (DT12, arrow E1; DT14, arrow E2) on pinna of Dictyophyllum nathorstii Zeiller, QLX-2105-15-567. (F) Margin feeding (DT13, arrows F1) and surface feeding (DT29, arrow F2) on pinnules of Cladophlebis kaoiana Sze, QLX-2105-15-619. (G) Skeletonization (DT21) on pinna of Clathrophteris platyphylla, QLX2014438. (H) Margin feedings (DT12, arrows H1) and galling (DT87, arrow H2) on pinnules and rachis of Cladophlebis sp., QLX-2105-15-709. (I) Galling (DT84, arrow) on Equisetites sp., QLX2014394. All scale bars = 5 mm, except for A1 (= 1 mm).
Bennettites host the most diverse range of DTs (Figure 3A). Hole feeding (DT03; Figure 5A) and margin feeding (DT12, DT81; Figure 5B) are common on this plant group; piercing and sucking (Figures 5C, D) and oviposition (Figures 5E, F) are notably more frequent than on ferns and sphenopsids (Figure 3A). Piercing and sucking is generally represented by concave elliptical punctures into the leaf tissue with a vacant central region sporadically aligned in a series (DT48; Figure 5C), or as sparse occurrences of a single concave puncture with a central depression penetrating the mesophyll (DT46; Figure 5D). Oviposition on bennettites is characterized mostly by linear series of lenticular or ovoid oviposition scars parallel to venation on leaves (DT100, DT101; Figures 5E, F). For cycads, only the external foliar feeding categories of hole feeding (DT02; Figure 5G) and margin feeding (DT12, DT15, DT26; Figures 5H–J) were observed.
Figure 5 Examples of various DTs on bennettite and cycad foliage from Member VII of the Xujiahe Formation. (A) Hole feeding (DT03, arrow) on pinnule of Pterophyllum ptilum Harris, QLX2014298. (B) Margin feeding (DT12, arrow B1; DT81, arrow B2) on pinnules of Pterophyllum sp., QLX2014308. (C) Piercing and sucking damage (DT48, arrow) on pinnules of Pterophyllum sp., QLX2014405. (D) Piercing and sucking damage (DT46, arrow) on pinnules of Pterophyllum sp., QLX-2105-15D-333. (E) Oviposition scars (DT100, arrow) on pinnules of Pterophyllum sp., QLX-2105-15U-652. (F) Oviposition scars (DT101, arrows) on pinnules of Pterophyllum angustum (Braun) Gothan, QLX- 2015-15-278. (G) Hole feeding (DT02, arrow) on pinna of Nilssonia sp., QLX-2105-15D-460. (H) Margin feeding (DT15, arrow H1; DT26, arrow H2) on pinna of Taeniopteris sp., QLX-2015-15-364. (I) Margin feeding (DT12, arrows) on pinna of Taeniopteris sp., QLX-2105-15D-251. (J) Margin feeding (DT15, arrow) on pinna of Taeniopteris sp., QLX-2105-15D-465. All scale bars = 5 mm, except for A1 (= 2 mm).
External foliage feeding is moderately common on the leaves of ginkgophytes, and is generally represented by small circular perforations (DT01; Figure 6A), shallow excisions of the leaf margin (DT12; Figure 6B), and excisions of the leaf apex (DT13; Figure 6C). Piercing and sucking damage is sporadic, usually characterized by small, convex and circular structures surrounded by one concentric ridge on the leaf surface, and no more than 1 mm in diameter (DT77; Figure 6D). Leaves of Podozamites (conifers) are abundant in this flora, and possess the second-most diverse DTs (Figures 2C, 3A). External foliage feeding is common (DT01, DT03, DT28; Figures 6E, F), with an especially high frequency of surface feeding represented by elongate abraded lamina surfaces between parallel veins bounded by a discernable reaction rim (DT28; Figure 6F). Three types of piercing and sucking damage are evident, mostly represented by a concave styletal puncture into the mesophyll with a central depression, and circular structures with a concentric ridge on leaf surfaces (DT46, DT77; Figure 6G). Oviposition damage is mostly characterized by distinctive thin, linear scars oriented longitudinally along the parallel veins (DT134; Figure 6H). Two galling DTs (e.g., circular to ellipsoid galls occurring on the leaf surface, DT33, Figure 6I; rounded ellipsoid galls with smooth surfaces located between longitudinally arranged parallel veins and causing distortion of adjacent veins, DT122, Figure 6J), occur sporadically on the leaves of Podozamites.
Figure 6 Examples of various DTs on ginkgoales and conifers from Member VII of the Xujiahe Formation. (A) Hole feeding (DT01, arrow) on Baiera sp., QLX-2105-15D-216. (B) Margin feeding (DT12, arrow) on Baiera elegans Ôishi, QLX-2105-15D-513. (C) Margin feeding (DT13, arrow) on Baiera elegans, QLX-2105-15U-267. (D) Piercing and sucking damage (DT77, area D1 and arrow) on Ginkgoites sp., QLX-2105-15D-497. (E) Hole feeding (DT01, arrow E1; DT03, arrow E2) on Podozamites sp., QLX-2105-15D-490. (F) Surface feeding (DT28, arrow) on Podozamites sp., QLX-2105-15U-534. (G) piercing and sucking damage (DT46, arrow G1; DT77, arrow G2) on Podozamites sp., QLX2014430. (H) Oviposition (DT134, arrow) on Podozamites sp., QLX-2105-15U-628. (I) Galling (DT33, arrow) on Podozamites sp., QLX-2105-15U-547. (J) Galling (DT122, area J1 and arrow) on Podozamites sp., QLX-2105-15U-642. All scale bars = 5 mm, except for D1 and J1 (= 2 mm).
Within the lowermost Zhenzhuchong Formation (Hettangian–Sinemurian age) in the Qilixia section, the plant diversity decreases by nearly half at genus level, with only seven genera recorded, i.e., Neocalamites, Todites, Thaumatopteris, Coniopteris, Cladophlebis, Storgaardia and Podozamites. This Early Jurassic fossil assemblage is notably characterized by a high frequency of ferns (i.e., Todites, Thaumatopteris, Coniopteris, Cladophlebis) and conifers (including Storgaardia and Podozamites), whereas sphenopsids (Neocalamites) are sparse (Figures 2B, D).
Twenty-one DTs belonging to six FFGs were recognized (Figures 3B, D), i.e., hole feeding (DT01, DT02, DT03, and DT05), margin feeding (DT12, DT13, and DT14), surface feeding (DT28, DT29 and DT103), piercing and sucking (DT46, DT48, DT77 and DT133), oviposition (DT72 and DT101), and galling (DT33, DT84, DT87, DT117, and DT122) (Appendix 1). Skeletonization was not detected in the earliest Jurassic Zhezhuchong flora, but the other six major FFGs occur in almost equivalent proportion to that recorded prior to the Triassic–Jurassic transition (Figure 3D).
Traces of plant-insect interactions appeared on 32.95% of the analyzed 176 leaf fragments (Figure 2D). Arthropod damage on Neocalamites was typically represented by oval oviposition scars oriented parallel to the axis of the stem with a distinct inner region surrounded by a rim of callus tissue (DT77; Figure 7F). Ferns represented by Todites, Coniopteris and Cladophlebis, mostly host hole feeding (e.g., DT01; Figure 7A) and margin feeding damage (e.g., DT12, DT13; Figures 7A, C, D) on pinnules. Piercing and sucking is locally represented by concave punctures into the leaf tissue with a central depression (DT46; Figure 7E). Galls occur sporadically on the rachis of the fern pinna (DT33; Figure 7B).
Figure 7 Examples of various DTs on sphenopsids and ferns from the Bed 2 of the Zhenzhuchong Formation. (A) Hole feeing (DT01, area A1 and arrow: insect) and margin feeding (DT12, arrows A2; DT13, arrow A3) on pinnules of Todites princeps (Presl) Gothan, QLX2014039. (B) Galling (DT33, arrow) on rachis of Todites pinceps, QLX2014044. (C) Margin feeding (DT12, arrows C1; DT13, arrow C2) on pinnules of Todites princeps, QLX2014048. (D) Margin feeding (DT12, arrow) on pinnules of Coniopteris tiehshanensis Ye et Lih, QLX2014005. (E) Piercing and sucking damage (DT46, area E1 and arrow) on pinnules of Cladophlebis sp., QLX2014001. (F) Oviposition (DT72, arrow) on stem of Neocalamites sp., QLX2014008. All scale bars = 5 mm, except for A1 (= 2 mm) and E1 (= 1mm).
Podozamites markedly dominates this earliest Jurassic flora (Figures 2B, D). Leaves of Podozamites host the most diverse array of DTs (Figure 3B). External foliar feeding damage, such as surface feeding (DT28, DT103; Figures 8A, B, E, H–I), hole feeding (DT01, DT02; Figure 8C) and margin feeding (DT12; Figures 8C, G, I) are represented. The surface feeding category DT28 is especially common and represented by strip-like abrasions between the parallel veins of Podozamites leaves (Figures 8A, E, H, I). Piercing and sucking (DT28, DT48; Figures 8E, H) and galling (DT84, DT122; Figures 8D, F, J) are also moderately important herbivory styles on Podozamites leaves.
Figure 8 Examples of various DTs of conifers from the Bed 2 of the Zhenzhuchong Formation. (A) Surface feeding (DT28, arrow) on leaflet of Podozamites sp., QLX2014069. (B) Surface feeding (DT103, area B1 and arrow) on leaflet of Podozamites sp. cf. P. issykkulensis Genkina, QLX-2105-15-012. (C) Hole feeding (DT01, arrow C1; DT02, arrow C2) and margin feeding (DT12, arrow C3) on leaflets of Podozamites distans (Presl) Braun, QLX-2105-Jzh2-019. (D) Galling (DT122, area D1 and arrow) on leaflet of Podozamites sp, QLX-2105-Jzh2-027. (E) Surface feeding (DT28, area E1), piercing and sucking (DT48, area E2 and arrows) and galling (DT122, arrow E3) on leaflets of Podozamites sp., QLX-2105-Jzh2-015. (F) Galling (DT84, arrow) on leaflet of Podozamites sp., QLX-2105-Jzh2-026. (G) Margin feeding (DT12, arrow) on leaflet of Podozamites sp., QLX-2105-Jzh2-031. (H) Surface feeding (DT28, arrows H1) and piercing and sucking (DT77, area H2 and arrow) on leaflets of Podozamites sp., QLX-2105-Jzh2-014. (I) Margin feeding (DT12, arrows I1) and surface feeding (DT28, arrow I2) on leaflets of Podozamites sp., QLX2014068. (J) Galling (DT122, arrow) on leaflet of Podozamites sp., QLX-2105-Jzh2-028. All scale bars = 5 mm, except for B1, D1, E1, E2 and H2 (= 2 mm).
The results indicate significant vegetation changes from the latest Triassic to the earliest Jurassic in the Qilixia section. The late Rhaetian flora from the Member VII of the Upper Triassic Xujiahe Formation was dominated by Bennettitales (mainly Pterophyllum) and ferns (mainly Dipteridaceae and Cladophlebis) (Figures 2A, C). The floral diversity and plant abundance of the earliest Jurassic fossil assemblages from the Bed 2 of the Zhenzhuchong Formation decrease markedly. This flora is dominated by conifers (Podozamites) and ferns (Todites and Coniopteris) (Figures 2B, D). The macrofloral changes confirm a distinct vegetation turnover across the Triassic–Jurassic boundary in the studied area, with the disappearance of bennettites, cycads and ginkgoaleans, and a marked rise in the abundance of the foliage of the ferns Todites and Coniopteris, and the conifer Podozamites (Figure 2).
Seven feeding strategies are recognized from the latest Triassic flora, of which six persist through the Triassic–Jurassic transition in the Qilixia section; only skeletonization disappears (Figure 3). Several examples of skeletonization are represented on pinna of Clathropteris platyphylla (Figures 4C, G) in the latest Triassic Xujiahe flora, and these are among the oldest know examples of insect-mediated skeletonization on the fern family Dipteridaceae (Feng et al., 2014). We contend that the disappearance of skeletonization in the earliest Jurassic Zhenzhuchong flora is possibly due to sampling biases or regional disparity in the floras, as the comparatively limited number of fossil specimens from the lowermost Zhenzhuchong Formation includes only one fragment of a reticulate-veined taxon (i.e., Thaumatopteris; Figure 2D). Other external foliage feeding categories, such as hole feeding, margin feeding and surface feeding are the most common damage types on essentially all plant groups (Figures 3A, B). In the latest Triassic Xujiahe flora, piercing and sucking damage is most common on Bennettitales (Figures 5C, D), Ginkgoales (Figure 6D) and Podozamites (Figure 6G), generally represented by concave/convex styletal punctures into leaf tissue.
During the earliest Jurassic, piercing and sucking damage types and frequency remained nearly constant from pre-ETE assemblages. It is conceivable that the terrestrial arthropods with this feeding strategy were able to transfer to alternative host plant groups, such as ferns (Figure 7E) and the conifer Podozamites (Figures 8E, H) that were obviously favored by mucivorous herbivores in the earliest Jurassic Zhenzhuchong flora.
Oviposition represents the response of egg insertion into or onto plant tissues. Three types of oviposition with a total of 10 occurrences (Appendix 1) were recorded in the latest Triassic Xujiahe flora (i.e., DT100, Figure 5E; DT101, Figure 5F; DT134, Figure 6H), whereas only two types with three occurrences (Appendix 1) were recognized from the earliest Jurassic Zhenzhuchong flora (i.e., DT72, Figure 7F; DT101).
Galling is also a common functional feeding group, including five DTs (i.e., DT33, Figures 6I, 7B; DT84, Figures 4I, 8F; DT87, Figures 4A, H; DT117; DT122, Figures 6J, 8D, E, J), which apparently all passed through the Triassic–Jurassic transition, and occurred on almost every plant group, mostly as rounded ellipsoid galls oriented parallel to venation on leaf surfaces or on twigs.
Although there was an obvious turnover in floral composition across the Triassic–Jurassic transition in the Qilixia section, it is notable that terrestrial arthropod herbivore guilds experienced little change based on the available evidence (Figures 2, 3; Appendix 1). Although fewer damage types were recorded from the earliest Jurassic Zhenzhuchong flora, the frequency and diversity of insect damage features remains proportionally equivalent, indicating that the slight decrease in the number of DTs after the end-Triassic event is more likely related to the biases of fossil collections or preservation conditions.
Previous studies of macro- and palyno-floral turnover during the end-Triassic mass extinction in Sichuan Basin, South China, suggested a significant increase in typical drought-resistant taxa, which agrees with inferences of global warming across the Triassic–Jurassic boundary and a dryer environment in the earliest Jurassic (Li et al., 2020; Zhou et al., 2021). It is likely that the study area experienced dramatic environmental changes and ecological perturbations, leading to the obvious regional vegetational turnover during this event. Since there is relatively little change in the proportional representation of major feeding categories, we infer that many arthropod herbivores were able to transfer to alternative host plants during this biotic crisis, which was possible less abrupt than the end-Cretaceous and less intense than the end-Permian events.
Triassic–Jurassic plant-arthropod interactions in the Sichuan Basin, South China, have not yet been comprehensively surveyed, despite an individual case of insect damage having been investigated (Feng et al., 2022). Only a few records of fossil insects have been documented (Lin, 1978; Li et al., 2007; Fang et al., 2013). Identification of the insect groups that caused the DTs observed in the current study remains unresolved owing to scarce occurrences of body fossils belonging only to Blattoidea (Zhang et al., 2021) recovered from the succession and the generalized feeding strategies represented by many of the DTs identified. The current study provides preliminary data on the distribution of DTs caused by insect herbivores from the latest Triassic Xujiahe Formation and the earliest Jurassic Zhenzhuchong Formation. This study provides a foundation for further research on plant-animal interactions in the region to determine whether the similar feeding strategies, diversities and distributions on plants before and after the end-Triassic extinction event are a consistent feature of the East Asian subtropical biome. We did not detect any clear patterns of change in insect damage types across the Triassic–Jurassic transition in the Qilixia section, and we contend that the several minor changes in representation are primarily the result of sampling biases.
Many measures have been adopted by plants for resisting insect herbivory, such as adjusting growth time, developing specialized morphologically features for physical defense, and investment in chemical substances as deterrents (Qin, 1996). Foliar size and morphology are greatly influenced by the environment in which the plant lives. Certain foliar characters, such as marginal teeth on leaves and surface ridges on rachises are very common on modern leaves and can serve to deter herbivores or disrupt their feeding on vulnerable portions of the leaf. However, such features may also be evolutionary responses to various other biotic and abiotic factors (Maiti et al., 2016; McCoy et al., 2021).
The prominent marginal teeth on pinnules of Pterophyllum ctenoides Ôishi (Figures 9A, B), represents an unusual morphological feature for this genus. Based on their position on the apices of leaflets, we interpret these structures as possible defenses against margin-feeding insects. Such cusps are too small to represent defenses against vertebrate herbivores but they may have provided benefits in disrupting movement and tissue consumption by margin/apex-feeding insects. The vast majority of margin feeding damage on Pterophyllum is effectively restricted to the distal parts of the pinnules (Figure 5B), suggesting that marginal teeth had selective advantages in hindering herbivory. Prominent transverse surface ridges are also evident on the rachises of Pterophyllum species (Figure 9C). These thickenings probably served a similar function to robust hairs along the rachis of Jurassic Anomozamites (Pott et al., 2012), in providing benefits for disrupting herbivore movement and protecting the rachis from specific feeding (e.g., piercing and sucking) and egg-laying (oviposition) behaviors by roughening the surface texture and impeding access to the vascular supply.
Figure 9 Examples of physical defenses on some leaf fragments. (A, B) Prominent teeth on the apices of pinnules of Pterophyllum ctenoides Ôishi. (C) Obvious thickening ridges on the rachis of Pterophyllum ctenoides Ôishi. All scale bars = 5 mm, except for B1 = 2 mm.
There is considerable potential for investigations tracking plant-insect interactions across the Triassic–Jurassic transition globally. To date, most research within this time interval has focused on isolated plant assemblages either of Late Triassic (Pott et al., 2008; Feng et al., 2014; Gnaedinger et al., 2014; Lara et al., 2017; Labandeira et al., 2018; Cariglino et al., 2022; Feng et al., 2022) or Early Jurassic (van Konijnenburg-van Cittert and Schmeißner, 1999; Popa and Zaharia, 2011; Edirisooriya and Dharmagunawardhane, 2013) age. Ideally, successions employed to track herbivory through the ETE should have rich palaeofloras preceding and succeeding the event; and the floras should have well-resolved taxonomic compositions with abundant and well-preserved specimens. To meet standards for regional or global comparison and yielding more accurate results, a series of researchers have proposed approaches and protocols calling for sampling routines (Schachat et al., 2018; Schachat et al., 2020) or at least five identifiable plant taxa and a minimum of 50 collected hand specimens (Cariglino et al., 2021). Relatively few continuous successions through the ETE have assemblages deemed to fulfill these requirements which may have led to a dearth of past studies of this topic.
The Qilixia section in the northern Sichuan Basin represents one of the best-exposed Upper Triassic–Lower Jurassic successions in South China and more than fulfills these criteria, yielding diverse and abundant plant fossils (Wang et al., 2010) that have been dated securely by macro/micro-floral (Ye et al., 1986; Li et al., 2020; Zhou et al., 2021) and magnetostratigraphic (Li et al., 2017; Lu et al., 2023) studies. Other sections in the Sichuan Basin have the potential to verify the results obtained from Qilixia. These sections need to be sampled systematically to avoid collection biases towards the more complete and better-preserved fragments, and to guarantee optimal reflection of plant-insect interactions during the studied interval. We trust that the detailed observations of insect feeding damage types across all plant fragments in the Rhaetian and Hettangian–Sinemurian assemblages from the Qilixa section will inspire further studies on this topic in South China and elsewhere.
Two principal sources of error are recognized for this study. Firstly, we note that markedly fewer specimens were available from the Lower Jurassic (176 fragments) compared to the Rhaetian (564 fragments) sequence in the Qilixia section. This may have contributed to the lower observed levels of plant and herbivory diversity in the Lower Jurassic. Secondly, some fossils from the Qilixia section are heavily coated by mineral staining or disrupted by minute mineral concretions. These may obfuscate the identification of some herbivore damage, especially concealing minute traces of piercing and sucking or galling damage. Large collections of more pristine fossils from the region have the potential to clarify the full range of damage types in this succession. Internationally, numerous well-studied Upper Triassic to Jurassic floras from Greenland (Harris, 1937), Europe (Lundblad, 1950; Pott and McLoughlin, 2009; Kustatscher et al., 2018), Iran (Sadovnikov, 2020) and Australia (Jones and de Jersey, 1947; Jansson et al., 2008) offer great potential for clarifying the global patterns of vegetation and herbivory change in terrestrial ecosystems through the ETE.
Plant-arthropod interactions were systematically recorded for the first time from leaf impression/compression fossils recovered from upper Rhaetian and the Lower Jurassic strata in the Qilixia section, Sichuan Basin. Herbivory features from the latest Rhaetian Xujiahe flora are assigned to 28 DTs referable to seven principle FFGs on 13 genera of plants. Twenty-one DTs assigned to six FFGs were recorded on seven genera of plants recovered from the earliest Jurassic Zhenzhuchong flora. Most FFGs (with the exception of skeletonization), and generalized damage types, persisted from the latest Triassic to the earliest Jurassic without obvious major proportional change, despite substantial turnover in the vegetation through the ETE, signifying an ability of insects to transfer feeding to alternative plant groups. We are aware of several potential sources of error in our study (e.g., differential recovery of Triassic versus Jurassic samples; mineral masking of simple damage features on some specimens). However, we regard the results of this study as the first step in addressing the changing character of autotroph-arthropod interactions across the fourth of the ‘big five’ extinctions of the Phanerozoic. This preliminary assessment of herbivory across the Triassic–Jurassic boundary requires complementary studies within the Sichuan Basin, South China, to verify the regional patterns of vegetation and herbivory change in the subtropics of East Asia. We also encourage equivalent investigations from other parts of the world to clarify the global patterns of plant-insect interactions and floristic change across this major event in Earth’s history.
The original contributions presented in the study are included in the article/Supplementary Material. Further inquiries can be directed to the corresponding authors.
YX: Conceptualization, Data curation, Formal analysis, Investigation, Methodology, Resources, Software, Visualization, Writing – original draft, Validation. YW: Conceptualization, Funding acquisition, Project administration, Resources, Supervision, Validation, Writing – review & editing. LL: Funding acquisition, Resources, Validation, Writing – review & editing. NL: Funding acquisition, Resources, Validation, Writing – review & editing. YZ: Resources, Validation, Visualization, Writing – review & editing. ZH: Resources, Validation, Visualization, Writing – review & editing. SM: Conceptualization, Data curation, Funding acquisition, Investigation, Methodology, Project administration, Supervision, Validation, Writing – review & editing.
The author(s) declare financial support was received for the research, authorship, and/or publication of this article. This study is financially supported by the National Natural Science Foundation of China (grant numbers 42330208, 42072009, 41972120, 42172129, 42202020), the Stratigraphic Priority Program (B) of the Chinese Academy of Sciences (grant number XDB 2610302), the Programs from State Key Lab of Palaeobiology and Stratigraphy (20191103, 173131, 213112), and CSC (grant number 202204910396). SM is funded by grants from the Swedish Research Council (VR grant numbers 2018-04527 and 2022-03920).
Especially thank Ms. Ting Li from the Nanjing Institute of Geology and Paleontology, CAS for helpful discussions.
The authors declare that the research was conducted in the absence of any commercial or financial relationships that could be construed as a potential conflict of interest.
All claims expressed in this article are solely those of the authors and do not necessarily represent those of their affiliated organizations, or those of the publisher, the editors and the reviewers. Any product that may be evaluated in this article, or claim that may be made by its manufacturer, is not guaranteed or endorsed by the publisher.
The Supplementary Material for this article can be found online at: https://www.frontiersin.org/articles/10.3389/fevo.2023.1338865/full#supplementary-material
Supplementary Appendix 1 | Records of damage types (DTs) on individual specimens through the uppermost Xujiahe Formation and lowermost Zhenzhuchong Formation.
Barbacka M., Pacyna G., Kocsis A., Jarzynka A., Ziaja J., Bodor E. (2017). Changes in terrestrial floras at the Triassic–Jurassic Boundary in Europe. Palaeogeogr. Palaeoclimatol. Palaeoecol. 480, 80–93. doi: 10.1016/j.palaeo.2017.05.024
Benton M. J. (1995). Diversification and extinction in the history of life. Science 268 (5207), 52–58. doi: 10.1126/science.7701342
Calatayud P. A., Sauvion N., Thiéry D. (2018). Plant–insect interactions. Oxford Bibliographies. doi: 10.1093/OBO/9780199830060-0193
Cariglino B., Moisan P., Lara M. B. (2021). The fossil record of plant-insect interactions and associated entomofaunas in Permian and Triassic floras from southwestern Gondwana: A review and future prospects. J. South Am. Earth Sci. 111, 103512. doi: 10.1016/j.jsames.2021.103512
Cariglino B., Prevec R., Lara M. B., Zavattieri A. M. (2022). Evidence of insect leaf mining on Late Triassic (Carnian) gymnosperms from Argentina. Rev. Palaeobot. Palynol. 305, 104749. doi: 10.1016/j.revpalbo.2022.104749
Clapham M. E., Karr J. A., Nicholson D. B., Ross A. J., Mayhew P. J. (2016). Ancient origin of high taxonomic richness among insects. Proc. R. Soc B. 283, 20152476. doi: 10.1098/rspb.2015.2476
Condamine F. L., Clapham M. E., Kergoat G. J. (2016). Global patterns of insect diversification: towards a reconciliation of fossil and molecular evidence? Sci. Rep. 6, 19208. doi: 10.1038/srep19208
Currano E. D., Wilf P., Wing S. L., Labandeira C. C., Lovelock E. C., Royer D. L. (2008). Sharply increased insect herbivory during the Paleocene-Eocene Thermal Maximum. Proc. Natl. Acad. Sci. U. S. A. 105, 1960–1964. doi: 10.1073/pnas.0708646105
Donovan M. P., Iglesias A., Wilf P., Labandeira C. C., Cúneo N. R. (2016). Rapid recovery of Patagonian plant–insect associations after the end-Cretaceous extinction. Nat. Ecol. Evol. 1, 12. doi: 10.1038/s41559-016-0012
Donovan M. P., Iglesias A., Wilf P., Labandeira C. C., Cúneo N. R. (2018). Diverse plant-insect associations from the latest Cretaceous and early Paleocene of Patagonia, Argentina. Ameghiniana 55, 303–338. doi: 10.5710/AMGH.15.02.2018.3181
Edirisooriya G., Dharmagunawardhane H. A. (2013). Plant insect-interactions in Jurassic fossil flora from Sri Lanka. Int. J. Sci. Res. Pub. 3, 1.
Fang Y., Zhang H. C., Wang B., Zheng D. R. (2013). A new cockroach (Blattaria: Caloblattinidae) from the Upper Triassic Xujiahe Formation of Sichuan Province, southwestern China. Insect Syst. Evol. 44, 167–174. doi: 10.1163/1876312X-44022101
Feng Z., Su T., Yang J. Y., Chen Y. X., Wei H. B., Dai J., et al. (2014). Evidence for insect-mediated skeletonization on an extant fern family from the Upper Triassic of China. Geology. 42, 407–410. doi: 10.1130/G35369.1
Feng Z., Wan S., Sui Q., Labandeira C., Guo Y., Chen J. B. (2022). A Triassic tritrophic triad documents an early food-web cascade. Curr. Biol. 32, 5165–5171. doi: 10.1016/j.cub.2022.10.031
Gandolfo M. A., Zamaloa M. C. (2021). Southern high-latitude plant-insect interactions from the miocene of Tierra Del Fuego, Argentina. Int. J. Plant Sci. 182, 523–532. doi: 10.1086/714285
Gnaedinger S. C., Adami-Rodrigues K., Gallego O. F. (2014). Endophytic oviposition on leaves from the Late Triassic of northern Chile: Ichnotaxonomic, palaeobiogeographic and palaeoenvironment considerations. Geobios. 47, 221–236. doi: 10.1016/j.geobios.2014.06.003
Greene S. E., Martindale R. C., Ritterbush K. A., Bottjer D. J., Corsetti F. A., Berelson W. M. (2012). Recognising ocean acidification in deep time: An evaluation of the evidence for acidification across the Triassic–Jurassic boundary. Earth-Sci. Rev. 113, 72–93. doi: 10.1016/j.earscirev.2012.03.009
Hagström J., Mehlqvist K. (2012). The dawn of terrestrial ecosystems on Baltica: First report on land plant remains and arthropod coprolites from the Upper Silurian of Gotland, Sweden. Palaeogeog. Palaeoclimatol. Palaeoecol. 317–318, 162–170. doi: 10.1016/j.palaeo.2012.01.001
Harris T. M. (1937). The fossil flora of Scoresby Sound, East Greenland. 5. Stratigraphic relations of the plant beds. Medd Grøn. 112, 1–176.
He T., Dal Corso J., Newton R. J., Wignall P. B., Mills B. J. W., Todaro S., et al. (2020). An enormous sulfur isotope excursion indicates marine anoxia during the end-Triassic mass extinction. Sci. Adv. 6, eabb6704. doi: 10.1126/sciadv.abb6704
Huang Q. S. (1992). “Plants,” in The Triassic of Qinling Mountains and Neighboring Areas. Eds. Yin H. F., Yang F. Q., Huang Q. S., Yang H. S., Lai X. L. (Wuhan: China University of Geosciences Press), 174–179.
Jansson I.-M., McLoughlin S., Vajda V., Pole M. (2008). An Early Jurassic flora from the Clarence-Moreton Basin, Australia. Rev. Palaeobot. Palynol. 150, 5–21. doi: 10.1016/j.revpalbo.2008.01.002
Jones O. A., de Jersey N. J. (1947). The flora of the Ipswich Coal Measures: morphology and succession. Pap. Dep. Geol. Univ. Queensl. 3, 1–88.
Krassilov V., Karasev E. (2008). First evidence of plant–arthropod interaction at the Permian–Triassic boundary in the Volga Basin, European Russia. Alavesia. 2, 247–252.
Kustatscher E., Ash S. R., Karasev E., Pott C., Vajda V., Yu J. X., et al. (2018). “Flora of the Late Triassic,” in The Late Triassic World: earth in a time of transition. Ed. Tanner L. H. (Heidelberg: Springer International Publishing AG), 545–622.
Labandeira C. C. (2002). “The history of associations between plants and animals,” in Plant–Animal Interactions: An Evolutionary Approach, vol. 26–74 . Eds. Herrera C. M., Pellmyr O. (Oxford, UK: Blackwell), 248–261.
Labandeira C. C. (2006). The four phases of plant-arthropod associations in deep time. Geol. Acta. 4, 409–438.
Labandeira C. C. (2007). The origin of herbivory on land: initial patterns of plant tissue consumption by arthropods. Insect Sci. 14, 259–275. doi: 10.1111/j.1744-7917.2007.00141.x-i1
Labandeira C. C. (2013). Deep-time patterns of tissue consumption by terrestrial arthropod herbivores. Naturwissenschaften. 100, 355–364. doi: 10.1007/s00114-013-1035-4
Labandeira C. C. (2021). Ecology and evolution of gall-inducing arthropods: the pattern from the terrestrial fossil record. Front. Ecol. Evol. 9. doi: 10.3389/fevo.2021.632449
Labandeira C. C., Sepkoski J. J. Jr. (1993). Insect diversity in the fossil record. Science. 261, 310–315. doi: 10.1126/science.11536548
Labandeira C. C., Wappler T. (2023). Arthropod and pathogen damage on fossil and modern plants: exploring the origins and evolution of herbivory on land. Annu. Rev. Entomol. 68, 341–361. doi: 10.1146/annurev-ento-120120-102849
Labandeira C. C., Anderson J. M., Anderson H. M. (2018). “Expansion of arthropod herbivory in Late Triassic South Africa: the Molteno biota, Aasvoëlberg 411 site and developmental biology of a gall,” in The Late Triassic World: Earth in a time of transition. Ed. Tanner L. H. (Cham: Springer International Publishing), 623–719. doi: 10.1007/978-3-319-68009-5_14
Labandeira C. C., Johnson K. R., Lang P. (2002a). Preliminary assessment of insect herbivory across the Cretaceous–Tertiary boundary: Major extinction and minimum rebound. Geol. Soc Am. Spec. Pap. 361, 297–327. doi: 10.1130/0-8137-2361-2.297
Labandeira C. C., Johnson K. R., Wilf P. (2002b). Impact of the terminal Cretaceous event on plant–insect associations. Proc. Natl. Acad. Sci. U. S. A. 99, 2061–2066. doi: 10.1073/pnas.042492999
Labandeira C. C., Kustatscher E., Wappler T. (2016). Floral assemblages and patterns of insect herbivory during the Permian to Triassic of Northeastern Italy. PloS One 11, e0165205. doi: 10.1371/journal.pone.0165205
Labandeira C. C., Wilf P., Johnson K. R., Marsh F. (2007). Guide to insect (and other) damage types on compressed plant fossils, Version 3.0 (Washington D C: Smithsonian Institution), 1–25.
Lara M. B., Cariglino B. C., Zavattieri A. M. (2017). Palaeoenvironmental interpretation of an Upper Triassic deposit in southwestern Gondwana (Argentina) based on an insect fauna, plant assemblage, and their interactions. Palaeogeogr. Palaeoclimatol. Palaeoecol. 476, 163–180. doi: 10.1016/j.palaeo.2017.03.029
Lathuilière B., Marchal D. (2009). Extinction, survival and recovery of corals from the Triassic to Middle Jurassic time. Terra Nova. 21, 57–66. doi: 10.1111/j.1365-3121.2008.00856.x
Li Z., Hong Y. C., Yang D. (2007). A new Middle Triassic genus and species of Mylacridae (Blattodea) from China. Zootaxa 1660, 53–59. doi: 10.11646/zootaxa.1660.1.4
Li L., Wang Y., Kürschner W. M., Ruhl M., Vajda V. (2020). Palaeovegetation and palaeoclimate changes across the Triassic–Jurassic transition in the Sichuan Basin, China. Palaeogeogr. Palaeoclimatol. Palaeoecol. 556, 109891. doi: 10.1016/j.palaeo.2020.109891
Li M. S., Zhang Y., Huang C. J., Ogg J., Hinnov L., Wang Y. D., et al. (2017). Astronomical tuning and magnetostratigraphy of the Upper Triassic Xujiahe Formation of South China and Newark supergroup of North America: implications for the Late Triassic time scale. Earth Planet. Sci. Lett. 475, 207–223. doi: 10.1016/j.epsl.2017.07.015
Lindström S., Sanei H., van de Schootbrugge B., Pedersen G. K., Lesher C. E., Tegner C., et al. (2019). Volcanic mercury and mutagenesis in land plants during the end-Triassic mass extinction. Sci. Adv. 5, eaaw4018. doi: 10.1126/sciadv.aaw4018
Lindström S., van de Schootbrugge B., Dybkjar K., Pedersen G. K., Fiebig J., Nielsen L. H., et al. (2012). No causal link between terrestrial ecosystem change and methane release during the end-Triassic mass extinction. Geology 40, 531–534. doi: 10.1130/G32928.1
Liu W. H., Dai X. H., Xu J. S. (2015). Influences of leaf-mining insects on their host plants: a review. Collect. Bot. 34, e005. doi: 10.3989/collectbot.2015.v34.005
Lu N., Wang Y. D., Xu Y. Y., Li L. Q., Xie X. P., Popa M. E., et al. (2023). Oscillations of a fluvial-lacustrine system and its ecological response prior to the end-Triassic: Evidence from the eastern Tethys region. Geol. Mag. 58, 1239–1255. doi: 10.1002/gj.4658
Lucas S. G., Tanner L. H. (2015). End-Triassic nonmarine biotic events. J. Palaeogeogr. 4, 331–348. doi: 10.1016/j.jop.2015.08.010
Lundblad A. B. (1950). Studies in the Rhaeto-Liassic floras of Sweden. I. Pteridophyta, Pteridospermae and Cycadophyta from the mining district of NW Scania. Kungl. Svenska Vetensk Akad. Handl. 1, 1–82.
Maiti R., Rodríguez H. G., Ivanova N. S. (2016). “Leaf traits,” in Autoecology and Ecophysiology of Woody Shrubs and Trees. Eds. Maiti R., Rodríguez H. G., Ivanova N. S. (Oxford: John Wiley & Sons, Ltd), 74–82.
Mander L., Kürschner W. M., McElwain J. C. (2010). An explanation for conflicting records of Triassic–Jurassic plant diversity. Proc. Natl. Acad. Sci. U. S. A. 107, 15351–15356. doi: 10.1073/pnas.1004207107
Mander L., Kürschner W. M., McElwain J. C. (2013). Palynostratigraphy and vegetation history of the Triassic–Jurassic transition in East Greenland. J. Geol. Soc 170, 37–46. doi: 10.1144/jgs2012-018
Marzoli A., Bertrand H., Knight K. B., Cirilli S., Buratti N., Vérati C., et al. (2004). Synchrony of the Central Atlantic magmatic province and the Triassic–Jurassic boundary climatic and biotic crisis. Geology. 32, 973. doi: 10.1130/g20652.1
McCoy V. E., Wappler T., Labandeira C. C. (2021). “Exceptional fossilization of ecological interactions: Plant defenses during the four major expansions of arthropod herbivory in the fossil record,” in Fossilization: Understanding the Material Nature of Ancient Plants and Animals. Eds. Gee C. T., McCoy V. E., Sander P. M. (Baltimore: Johns Hopkins University Press), 187–220.
McElwain J. C., Beerling D. J., Woodward F. I. (1999). Fossil plants and global warming at the Triassic–Jurassic boundary. Science. 285, 1386–1390. doi: 10.1126/science.285.5432.1386
McElwain J. C., Popa M. E., Hesselbo S. P., Haworth M., Surlyk F. (2007). Macroecological responses of terrestrial vegetation to climatic and atmospheric change across the Triassic/Jurassic boundary in East Greenland. Paleobiology 33, 547–573. doi: 10.1666/06026.1
McGhee G. R. J.r, Clapham M. E., Sheehan P. M., Bottjer D. J., Droser M. L. (2013). A new ecological-severity ranking of major Phanerozoic biodiversity crises. Palaeogeogr. Palaeoclimatol. Palaeoecol. 370, 26–270. doi: 10.1016/j.palaeo.2012.12.019
McLoughlin S., Martin S. K., Beattie R. (2015). The record of Australian Jurassic plant–arthropod interactions. Gondwana Res. 27, 940–959. doi: 10.1016/j.gr.2013.11.009
Olsen P. E., Kent D. V., Sues H. D., Koeberl C., Huber H., Montanari A., et al. (2002). Ascent of dinosaurs linked to an iridium anomaly at the Triassic–Jurassic boundary. Science. 296, 1305–1307. doi: 10.1126/science.1065522
Olsen P. E., Shubin N. H., Anders M. H. (1987). New Early Jurassic tetrapod assemblages constrain Triassic–Jurassic tetrapod extinction event. Science. 237, 1025–1029. doi: 10.1126/science.3616622
Panfili G., Cirilli S., Dal Corso J., Bertrand H., Medina F., Youbi N., et al. (2019). New biostratigraphic constraints show rapid emplacement of the Central Atlantic Magmatic Province (CAMP) during the end-Triassic mass extinction interval. Glob. Planet. Change. 172, 60–68. doi: 10.1016/j.gloplacha.2018.09.009
Peng J., Li J., Li W., Slater S. M., Zhu H., Vajda V. (2018). The Triassic to Early Jurassic palynological record of the Tarim Basin, China. Palaeobio. Palaeoenv. 98, 7–28. doi: 10.1007/s12549-017-0279-y
Percival L. M. E., Ruhl M., Hesselbo S. P., Jenkyns H. C., Mather T. A., Whiteside J. H. (2017). Mercury evidence for pulsed volcanism during the end-Triassic mass extinction. Proc. Natl. Acad. Sci. U. S. A. 114, 7929–7934. doi: 10.1073/pnas.1705378114
Popa M. E., Zaharia A. (2011). Early Jurassic oviposition on Bennettitalean leaves from Romania. Acta Palaeontol. Romaniae. 7, 285–290.
Pott C., Labandeira C. C., Krings M., Kerp H. (2008). Fossil insect eggs and ovipositional damage on bennettitalean leaf cuticles from the Carnian (Upper Triassic) of Austria. J. Paleontol. 82, 778–789. doi: 10.1666/06-094.1
Pott C., McLoughlin S. (2009). Bennettitalean foliage from the Rhaetian-Bajocian (latest Triassic-Middle Jurassic) floras of Scania, southern Sweden. Rev. Palaeobot. Palynol. 158, 117–166. doi: 10.1016/j.revpalbo.2009.08.004
Pott C., McLoughlin S., Wu S. Q., Friis E. M. (2012). Trichomes on the leaves of Anomozamites villosus sp. nov. (Bennettitales) from the Daohugou beds (Middle Jurassic), Inner Mongolia, China: Mechanical defence against herbivorous arthropods. Rev. Palaeobot. Palynol. 169, 48–60. doi: 10.1016/j.revpalbo.2011.10.005
Qin E. J. (1996). Adaptive and coordinated evolution of insects and host plants. Bull. Bio. 31, 1–3.
Rampino M. R., Caldeira K., Rodriguez S. (2023). Cycles of ~32.5 My and ~26.2 My in correlated episodes of continental flood basalts (CFBs), hyper-thermal climate pulses, anoxic oceans, and mass extinctions over the last 260 My: Connections between geological and astronomical cycles. Earth–Sci. Rev. 246, 104548. doi: 10.1016/j.earscirev.2023.104548
Rees P. K., Ziegler A. M., Vades P. J. (2000). “Jurassic phytogeography and climates: new data and model comparisons,” in Warm Climate in Earth History. Eds. Huber B. T., MacLeod K. G., Wing S. L. (New York: Cambridge University Press), 297–318.
Ruhl M., Bonis N. R., Reichart G. J., Damste J. S. S., Kürschner W. M. (2011). Atmospheric carbon injection linked to end-Triassic mass extinction. Science 333, 430–434. doi: 10.1126/science.1204255
Ruhl M., Hesselbo S. P., Al-Suwaidi A., Jenkyns H. C., Damborenea S. E., Manceñido M. O., et al. (2020). On the onset of Central Atlantic Magmatic Province (CAMP) volcanism and environmental and carbon-cycle change at the Triassic–Jurassic transition (Neuquén Basin, Argentina). Earth–Sci. Rev. 208, 103229. doi: 10.1016/j.earscirev.2020.103229
Ruhl M., Veld H., Kürschner W. M. (2010). Sedimentary organic matter characterization of the Triassic–Jurassic boundary GSSP at Kuhjoch (Austria). Earth Planet. Sci. Lett. 292, 17–26. doi: 10.1016/j.epsl.2009.12.046
Sadovnikov G. N. (2020). Plant communities and ecostratigraphy of the Upper Triassic of Alborez (Northern Iran). Stratigr. Geol. Correl. 28, 781–815. doi: 10.1134/S0869593820050093
Schachat S. R., Labandeira C. C., Maccracken S. A. (2018). The importance of sampling standardization for comparisons of insect herbivory in deep time: a case study from the Late Palaeozoic. R. Soc Open Sci. 5, 171991. doi: 10.1098/rsos.171991
Schachat S. R., Maccracken S. A., Labandeira C. C. (2020). Sampling fossil floras for the study of insect herbivory: how many leaves is enough? Fossil Rec. 23, 15–32. doi: 10.5194/fr-23-15-2020
Schoepfer S. D., Algeo T. J., van de Schootbrugge B., Whiteside J. H. (2022). The Triassic–Jurassic transition–A review of environmental change at the dawn of modern life. Earth–Sci. Rev. 232, 104099. doi: 10.1016/j.earscirev.2022.104099
Schoonhoven L. M., van Loon J. J. A., Dicke M. (2005). Insect-plant biology. 2nd ed. (New York: Oxford University Press), 421.
Scott A. C., Anderson J. M., Anderson H. M. (2004). Evidence of plant–insect interactions in the Upper Triassic Molteno Formation of South Africa. J. Geol. Soc 161, 401–410. doi: 10.1144/0016-764903-118
Sepkoski J. J. Jr (1996). “Patterns of Phanerozoic extinction: A perspective from global data bases,” in Global events and event stratigraphy. Ed. Walliser O. H. (Berlin: Springer, Heidelberg University), 35–51. doi: 10.1007/978-3-642-79634-0_4
Steinthorsdottir M., Tosolini A. M. P., McElwain J. C. (2015). Evidence for insect and annelid activity across the Triassic–Jurassic transition of east Greenland. Palaios. 30, 597–607. doi: 10.2110/palo.2014.093
Vajda V., Bercovici A. (2014). The global vegetation pattern across the Cretaceous–Paleogene mass extinction interval: A template for other extinction events. Glob. Planet. Change. 122, 29–49. doi: 10.1016/j.gloplacha.2014.07.014
Vajda V., McLoughlin S., Slater S. M., Gustafsson O., Rasmusson A. G. (2023). The ‘seed-fern’ Lepidopteris mass-produced the abnormal pollen Ricciisporites during the end-Triassic biotic crisis. Palaeogeogr. Palaeoclimatol. Palaeoecol 627, 111723. doi: 10.1016/j.palaeo.2023.111723
van de Schootbrugge B., Quan T. M., Lindström S., Püttmann W., Heunisch C., Pross J., et al. (2009). Floral changes across the Triassic/Jurassic boundary linked to flood basalt volcanism. Nat. Geosci. 2, 589–594. doi: 10.1038/ngeo577
van Konijnenburg-van Cittert J. H. A., Schmeißner S. (1999). Fossil insect eggs on Lower Jurassic plant remains from Bavaria (Germany). Palaeogeogr. Palaeoclimatol. Palaeoecol. 152, 215–223. doi: 10.1016/S0031-0182(99)00059-0
Wang Y. D., Fu B. H., Xie X. P., Huang Q. S., Li K., Li G., et al. (2010). The Terrestrial Triassic and Jurassic systems in the Sichuan Basin, China (Hefei: University of Science and Technology of China Press), 216.
Ye M. N., Liu X. Y., Huang G. Q., Chen L. X., Peng S. J., Xu A. F., et al. (1986). Late Triassic and Early–Middle Jurassic Fossil Plants from Northeastern Sichuan (Hefei: Anhui Science and Technology Publishing House), 141.
Zambon R., Denayer J., Prestianni C. (2023). Plant-insect interactions in the Selandian (Early Paleocene) Gelinden Fossil Flora (Belgium) and what they mean for the ecosystems after the Cretaceous–Paleogene mass extinction. Palaeogeogr. Palaeoclimatol. Palaeoecol. 619, 111524. doi: 10.1016/j.palaeo.2023.111524
Zhang Q. Q., Zheng D. R., Wang B., Zhang H. C. (2021). Review of Triassic insects in China. Geol. Soc Spec. Publ. 521, 45–60. doi: 10.1144/sp521-2021-121
Keywords: herbivory, insect damage types, functional feeding groups, end-Triassic mass extinction, vegetation turnover
Citation: Xu Y, Wang Y, Li L, Lu N, Zhu Y, Huang Z and McLoughlin S (2024) Plant-insect interactions across the Triassic–Jurassic boundary in the Sichuan Basin, South China. Front. Ecol. Evol. 11:1338865. doi: 10.3389/fevo.2023.1338865
Received: 15 November 2023; Accepted: 18 December 2023;
Published: 09 January 2024.
Edited by:
Juan Manuel Robledo, Centro de Ecología Aplicada del Litoral (CONICET), ArgentinaReviewed by:
Torsten Wappler, Hessisches Landesmuseum Darmstadt, GermanyCopyright © 2024 Xu, Wang, Li, Lu, Zhu, Huang and McLoughlin. This is an open-access article distributed under the terms of the Creative Commons Attribution License (CC BY). The use, distribution or reproduction in other forums is permitted, provided the original author(s) and the copyright owner(s) are credited and that the original publication in this journal is cited, in accordance with accepted academic practice. No use, distribution or reproduction is permitted which does not comply with these terms.
*Correspondence: Yongdong Wang, eWR3YW5nQG5pZ3Bhcy5hYy5jbg==; Stephen McLoughlin, c3RldmUubWNsb3VnaGxpbkBucm0uc2U=
Disclaimer: All claims expressed in this article are solely those of the authors and do not necessarily represent those of their affiliated organizations, or those of the publisher, the editors and the reviewers. Any product that may be evaluated in this article or claim that may be made by its manufacturer is not guaranteed or endorsed by the publisher.
Research integrity at Frontiers
Learn more about the work of our research integrity team to safeguard the quality of each article we publish.