- 1Tropical Biodiversity and Bioresource Utilization Laboratory, Qiongtai Normal University, Haikou, China
- 2Faculty of Criminal Science & Technology, Nanjing Police University, Nanjing, China
- 3Department of Social Sciences and Policy Studies, Education University of Hong Kong, Hong Kong, Hong Kong SAR, China
Urban green roofs have emerged as an innovative nature-based solution for enhancing urban sustainability and resilience. Based on the Web of Science Core Collection database, the scholarly papers on green roofs published in the past two decades were analyzed quantitatively and visualized using bibliometric techniques. The study scope covered research countries, institutions, main journals, crucial authors, highly cited documents, hot topics, and research frontiers. The 3210 publications mined from the bibliographic database on green roofs have increased progressively in the study period, with a significantly faster pace in recent years. The research subjects changed gradually over time, extending into more disciplines and becoming decidedly multidisciplinary. The notable bibliometric features registered a pronounced spatial concentration of research outputs in a small number of countries, regions, research institutions, journals, and researchers, mainly associated with developed metropolises. The number of publications and citation impacts identified the world’s top ten researchers and journals. Well-defined clusters depicted by knowledge graphs indicated a diverse range in the number and strength of inter-node connections for countries, institutions, researchers, and research topics defined by keywords. The research scope focuses on quantifying benefits and costs and optimizing designs to maximize biodiversity, hydrologic, thermal, and energy benefits as urban sustainability and climate-adaptation solutions. Emerging research should enhance biodiversity through optimal plant selection, integrate green roofs with renewable energy systems for sustainability, apply computational tools to enhance designs, evaluate low-cost and locally relevant design options for developing cities, and quantify co-benefits like stormwater reduction and energy savings. Multidisciplinary efforts leveraging simulations, ecosystem services valuation, and participatory community partnerships can advance context-appropriate green roof innovation and evidence-based policymaking to expand adoption in cities worldwide. Quantifying and communicating co-benefits are critical measures to drive wider implementation.
1 Introduction
Urbanization has accelerated globally since the 1950s, fundamentally transforming landscapes, nature contents and abiotic and biotic processes in urbanized areas (Xu et al., 2011; Taşkın and Saban, 2023). Urban sprawl often brings the proliferation of impervious surfaces like concrete and asphalt to seal previously natural areas. Impermeable roads, sidewalks, parking lots, recreational grounds and buildings prevent rainfall infiltration into the soil, causing most precipitation to runoff (Frazer, 2005). This extensive land sealing significantly alters the urban hydrological cycle, inducing undesirable consequences. The extensive replacement of natural vegetation and soil with impervious cover severely diminishes the ability of urban areas to absorb rainwater (Coutts et al., 2012). This degradation is compounded by climate change, which has brought more frequent and extreme precipitation events in many regions (Mendonça, 2023). The rapid rainwater runoff also curtails the key hydrological function of evaporative cooling, thus contribution to the intensification of the urban heat island effect and associated heat stress impact on human comfort and health (Jim, 2015a). In China, flooding disasters in recent decades have incurred massive financial losses in cities beset by the exigencies of tackling explosive population growth and development (Wang et al., 2021; Peng and Zhang, 2022). Urban green infrastructure presents a promising nature-based solution to offset the negative hydrological effects of urbanization. However, its widespread implementation has encountered various obstacles in highly urbanized areas (Aparicio Uribe et al., 2022). It is necessary to find innovative solutions to realize the water-smart city goal.
Green roofs have been increasingly recognized as a feasible alternative to ground-level urban greenery (Figure 1). They present effective mitigation measures for stormwater management in the built environment (Berardi et al., 2014; Wang et al., 2023). Retrofitted on existing rooftops, they increase water infiltration and retention, reduce and delay runoff, and improve water quality (Wong and Jim, 2014; Wong and Jim, 2015). In developed cities where rooftops may account for 60% of impervious cover (Li and Yeung, 2014), expanding the rooftop green infrastructure across underutilized roof spaces provides immense potential to alleviate urban flooding with minimal land requirements (McKenzie, 2020). The ecological rehabilitation of roofs through green infrastructure techniques like living roofs and rain gardens offers cities a practical nature-based solution to reverse the hydrological disruptions of urbanization and mitigate climate change impacts (Adem Esmail and Suleiman, 2020). An emerging strategy is integrating nature into the three-dimensional built fabric through innovative and eclectic green space planning (James et al., 2009). With a global rooftop area estimated at over 32 billion m2, repurposing roofs as living landscapes could transform cities into natural-cum-cultural entities (Cortinovis et al., 2022).
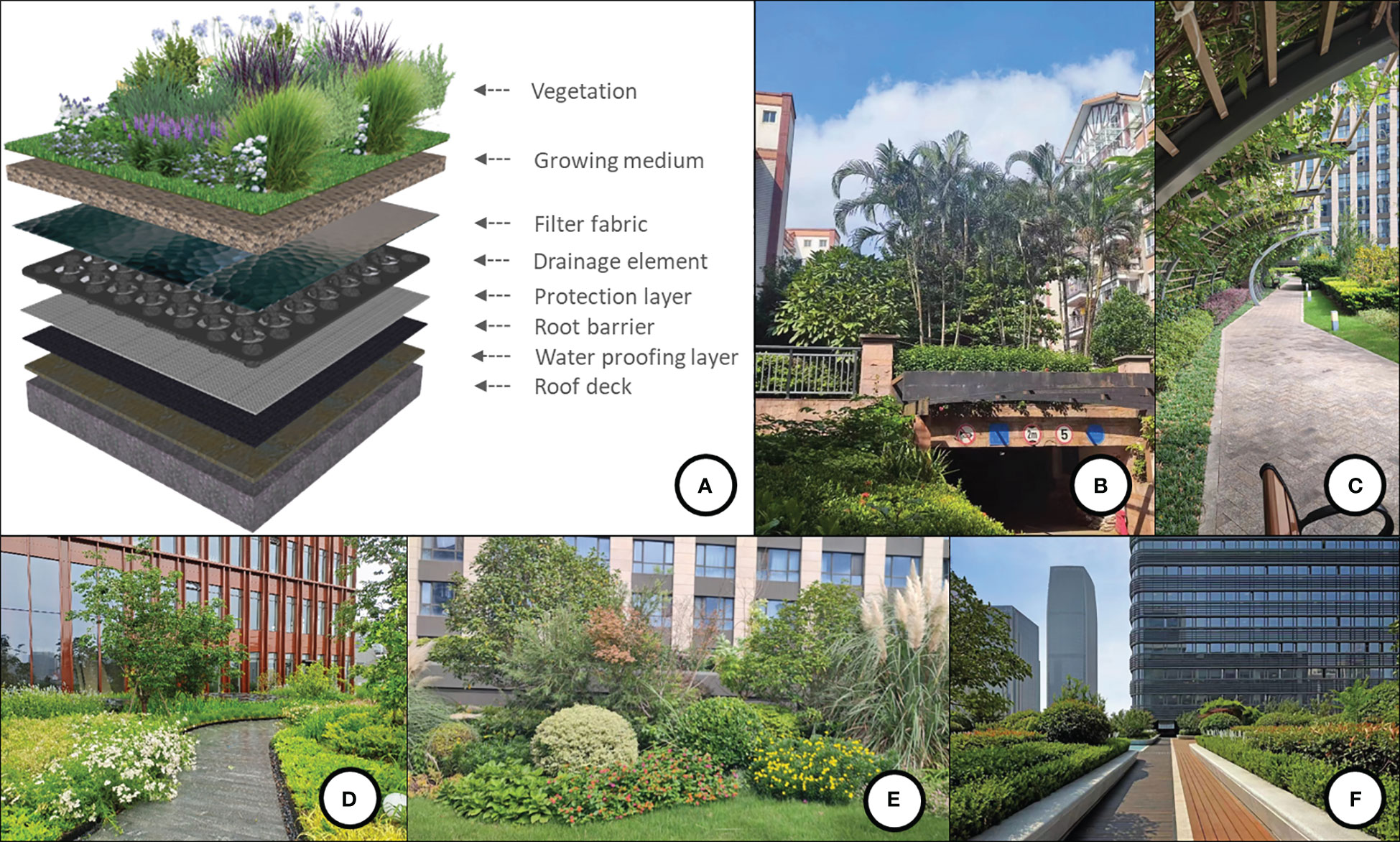
Figure 1 Structural layers of a modern green roof system and selected examples in China: (A) A typical cross-section of green roof; (B) A residential area in Haikou; (C–E). Office buildings in Shanghai; (F) A government office area in Jinan.
Rooftop greening provides multifunctional environmental and ecological benefits (Jim et al., 2022). Green roofs can reinforce resilience to climate change impacts (Peng and Jim, 2015). They mitigate the urban heat island effect by cooling the substrate, surface and ambient air temperatures (Lee et al., 2013). Rooftop vegetation traps and filters harmful suspended particulate matter, absorbs gaseous air pollutants, and sequesters carbon. They improve air quality and remove greenhouse gases (Zaid et al., 2018). The diverse services qualify green roofs as an integral component of green infrastructure for climate-adaptive and sustainable cities. Beyond environmental benefits, green roofs fulfill aesthetic and psychological functions due to landscape-visual appeals and salubrious venues for outdoor recreation and relaxation (Mesimäki et al., 2017). Some rooftops have been transformed into productive urban farms, blending green space with urban agriculture to yield fresh produce with a minimal transport carbon footprint (Saha and Eckelman, 2017). Recognizing the immense potential of green roofs, many governments have instituted enabling policies, regulations, and incentives to promote adoption (Jim and Hui, 2022; Essuman-Quainoo and Jim, 2023). Such initiatives can render communities greener, healthier, happier, and more resilient (Li and Yeung, 2014; Dobrzańska et al., 2022).
The looming climate change impacts call for enlightened ecological design strategies like green roofs to climate-proof cities (James et al., 2009; Cortinovis et al., 2022). In the past few decades, scientific research on green roofs has flourished, aiming to quantify their multiple functions and benefits across environmental, economic, and social realms (Berardi et al., 2014; Al Sayah et al., 2023). It is an opportune time to synthesize the literature to gain insights into the state of the art, research trends and future directions. Recent advances in sophisticated quantitative software provide powerful and suitable tools to conduct a detailed and systematic bibliometric review. The quantitative data-driven approach can decipher and discover underlying knowledge structures, research hotspots, and emerging directions by mapping the intricate networks between publications, authors, institutions, countries, and concepts (Li et al., 2022). With intensifying challenges to urban sustainability globally (Wachsmuth and Angelo, 2018), applying bibliometric analysis to map the extensive green roof literature can synthesize current understanding and shed light on unanswered questions to advance the research field. The findings will inform strategic priorities for the scientific community, policymakers, and practitioners to expedite green infrastructure implementation in cities worldwide.
This study applied bibliometric analysis to examine thoroughly the green roof research field. The quantitative approach pursues several objectives: (1) assess output and citation profiles to gauge the literature’s impact; (2) identify influential publications through literature co-citation network analysis; (3) determine key contributing authors, institutions, countries, and journals; (4) elucidate collaboration patterns and their evolution; and (5) to delineate hot topics and research frontiers over the study period. This bibliometric study seeks to uncover publication and authorship trends and emerging research priorities by quantifying research outputs, mapping citation networks, and elucidating collaboration linkages. The goal is to assess green roof literature objectively to highlight influential works, leading contributors, productive collaborations, and critical knowledge gaps to inform future research directions. Findings will synthesize our current understanding and provide strategic insights to continue advancing the study of green roofs.
2 Data and methods
2.1 Data source
The global literature about green roofs published from 2000 to 2022, held in the core database of the Web of Science (WoS), was exhaustively scanned. The search terms “green roof”, “eco-roof”, “living roof”, and “vegetated roof”, used in the paper title, abstract and keyword list, were used to identify the matching publications. The WoS database supplied comprehensive bibliographic information meeting the research requirements: publication year, language, journal name, paper title, author name, author affiliation, keyword, document type, abstract, citation count, impact factor, and citation impact index. The data harvested on 21 May 2023 were exported in CSV format to facilitate analysis by the bibliometric software. In total, 3210 items were retrieved, which were grouped into four categories: article (reporting original research findings), review, conference proceeding paper, and other type. VOSviewer (version 1.6.19) analyzed the essential attributes and relationships: Co-authorship, Co-occurrence, Citation, Bibliographic coupling, Co-citation, and Theme (van Eck and Waltman, 2010).
2.2 Data analysis and visualization
VOSviewer is a versatile and popular software used for bibliometric network analysis (van Eck and Waltman, 2010). It allows users to construct and visualize networks of various entities (Oyewola and Dada, 2022). The software’s quantitative and relationship-focused analysis facilitates understanding the evolution and emerging trends within a research domain (Diwanji, 2022). It can be combined with traditional literature review methods to enhance research analysis (Zare et al., 2017).
The literature data retrieved from WoS was saved in text format for VOSviewer analysis. Knowledge graphs of co-authorship were drawn between authors, institutions, and countries. A node’s circle size represented the number of publications, and the inter-node distance indicated the strength of collaboration. Similarly, knowledge graphs were drawn to depict co-occurrence keyword clustering based on the frequency of keyword co-occurrences (Orduña-Malea and Costas, 2021). Each node represented a keyword, and a node’s circle size indicated the keyword occurrence frequency (Toledo et al., 2019). The inter-node distance indicated the frequency of keyword co-occurrence, with a shorter distance indicating a higher co-occurrence frequency (Li et al., 2022). Microsoft Excel 2019 analyzed the distribution of publications by years, journals, author institutions, etc., of the retrieved papers and generated charts.
3 Results and discussion
3.1 Temporal distribution of publications
The 3210 publications on green roofs issued between 2000 and 2022 were mined from the WoS database. They included 2831 (88.19%) original research articles, 228 (7.10%) review articles, 65 (2.02%) conference proceeding papers, and 86 (2.67%) other forms of publications such as meeting abstracts, editorial materials, letters, book chapters, etc. (Figure 2).
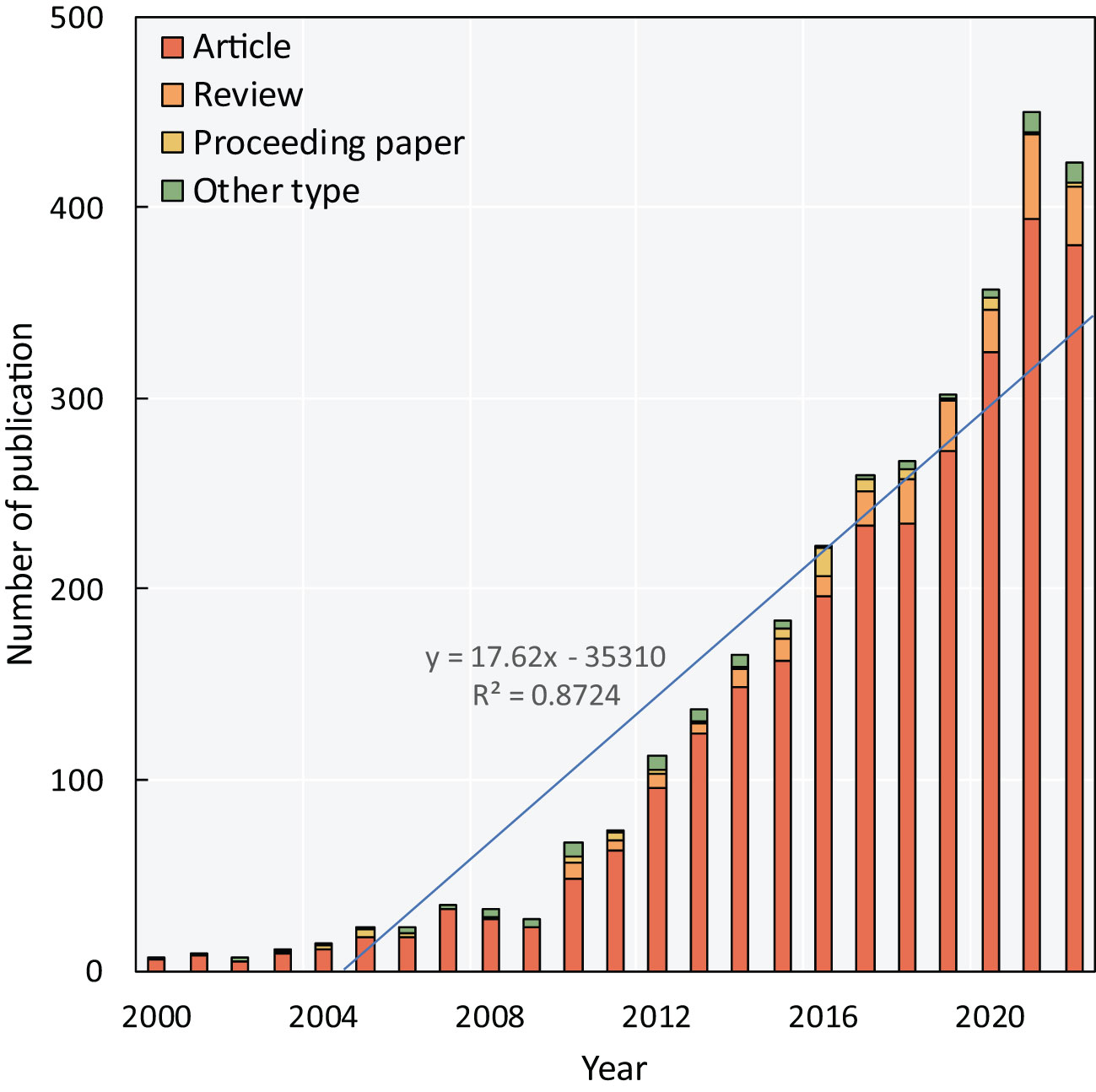
Figure 2 The temporal distribution of publications on green roofs from 2000 to 2022. The regression trend line indicates the rate of change in the study period. In the legend, “Article” refers to papers reporting original research on green roofs.
The temporal distribution of publications can be divided into three phases. (1) The initial incubation phase in 2000−2009 experienced few publications due to the incipient development of the research field characterized by limited understanding of the subject matter. Less than 30 publications were delivered per year, with an average of merely 15.6 per year. (2) The early developmental phase in 2010−2016 witnessed an incremental annual increase, reaching an average of nearly 120 per year, rising from 48 in 2010 to 196 in 2016. The 2015 Paris Climate Agreement (Clémençon, 2016) could have provided an impetus to studies on green roof benefits for urban temperature regulation, energy savings, and stormwater management. (3) The fast expansion phase in 2017−2022 signified substantial growth to 2000 papers in six years. Against the backdrop of intensifying global climate change, green roof research is increasingly regarded as an important contributor to environmental and human well-being. Recent green roof studies have concentrated on urban biodiversity, microclimate mitigation, policy development, optimized materials and technology, urban and landscape design tools, real-time environmental monitoring, and mental health benefits (Lee et al., 2013; Li and Yeung, 2014; Liberalesso et al., 2020).
3.2 Bibliometric features
3.2.1 Subject categories
The most researched subject categories reflect the evolution of the green roof field over time (Table 1). Early studies in 2000−2009 focused narrowly on environmental sciences (50, 17.0%), civil engineering (40, 13.6%), construction/building technology (36, 12.2%), and energy (31, 10.5%). In 2010−2016, the inquiries expanded substantially into broader sustainability fields like environmental studies (114, 5.8%), urban studies (110, 5.6%), and green/sustainable science and technology (107, 5.5%). The last few years (2017−2022) witnessed notable and further broadening, with environmental sciences (779, 26.7%) and engineering (281, 9.5%) remaining dominant, but ecology (142, 4.8%) and water resources (249, 8.4%) rising in prominence. The overall trend indicates a maturing field with growing interdisciplinary breadth through diverse lenses spanning the built environment, ecology, hydrology, climate resilience, and sustainability realms (Peng and Jim, 2015). The importance recently accorded to urban studies signals recognition of green roofs’ potential to address sustainability challenges in cities (James et al., 2009; Dorst et al., 2019). As knowledge accumulates across domains, a more holistic systems perspective will be critical to leverage fully green roofs for regenerative and climate-adaptive urban design.
3.2.2 Main research countries, regions and institutions
The United States and China generate the most green roof publications, jointly accounting for over one-third of the total (Table 2). Italy ranks third, followed by major English-speaking countries: UK, Australia, and Canada. Western European nations known for green technology, like Germany and France, also make it to the top ten. Regarding research impact, the USA and UK have the highest total citations, mean citations per article, and h-index. However, normalizing for publication volume, Australia and the UK have the highest field-weighted citation impacts. This result indicates that Australia is proportionally more influential in green roof research (Irga et al., 2017). Beyond quantity, the data reflects a global distribution of top green roof knowledge clusters extending from Western Europe to North America and Asia-Pacific. With urbanization and climate change challenges becoming more acute worldwide, international collaboration will be essential to advance green roof research. Context-specific studies are needed to understand how regional differences in climate, building types, policies, and culture shape green infrastructure adoption (Chen and Gou, 2022).
The color patterns and proximity of country nodes indicate the strength and direction of their relationships (Figure 3). The diagram excludes countries with less than five publications and limited research capacity. Seven clusters are differentiated, indicating distinct regional research clusters in green roof technology and knowledge sharing. The Asia-Pacific region forms a prominent yellow cluster, indicating close collaborations between China, South Korea, Taiwan (China), and Southeast Asian countries. This pattern reflects rapid urbanization and the emerging green building initiatives in the region. North and South America also show tight integration, with the USA playing the bridging role to connect research across the Americas. Western Europe constitutes a densely interconnected red cluster, with countries like Italy, France, Spain, Belgium, and Sweden benefiting from proximity and shared policies and standards under the European Union umbrella. The British Isles represent a somewhat insular but highly productive light-blue cluster, while Germany anchors research in Northern Europe. The Middle East, South Asia, and Africa form a loosely connected green cluster marked by geographic dispersion, relatively weak links, and fewer specialized research institutes. Japan and Singapore in orange have strong research capabilities but less regional integration. Figure 3 illustrates how geographic, cultural, and political factors exert lingering impacts on knowledge generation, clustering, and diffusion patterns. Targeted cooperation between hubs can strengthen the universal dissemination of green roof expertise while tailoring applications to local contexts (Appio et al., 2019).
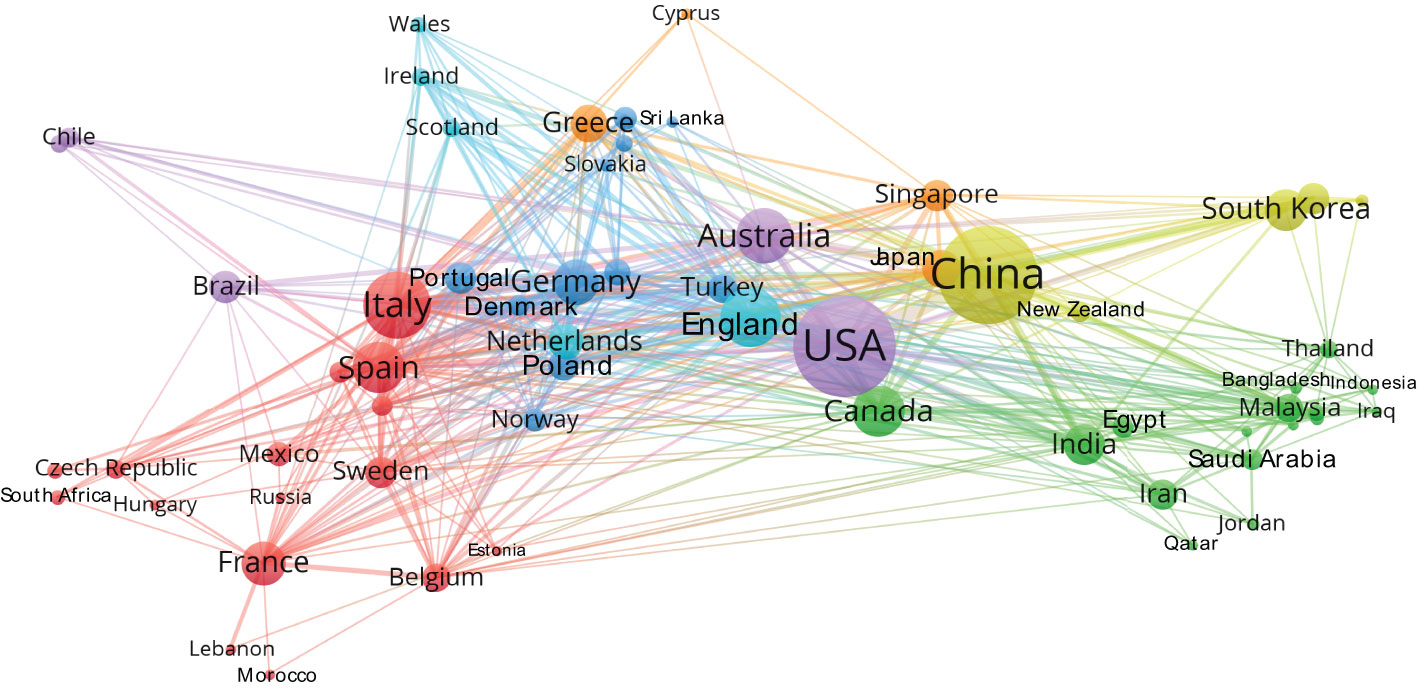
Figure 3 Knowledge graph depicting the research collaboration network of countries with reference to green roof research publications from 2000 to 2022. Only countries with more than five publications have been included.
The distribution of research forte in green roofs may be echoed by the inter-institution cooperative network, with frequent activities signifying strong research capabilities (Table 3; Figure 4). The Chinese Academy of Sciences published the most papers with 59, followed by the University of Hong Kong with 52. However, the University of Sheffield had the highest total citations (4,308) and mean citations per paper (86.16). Table 3 displays the percentage of total publications, h-index, and countries of the institutions. The data denote the global distribution of research strength, with high output from China and major impacts from the UK, France, USA, and Australia.
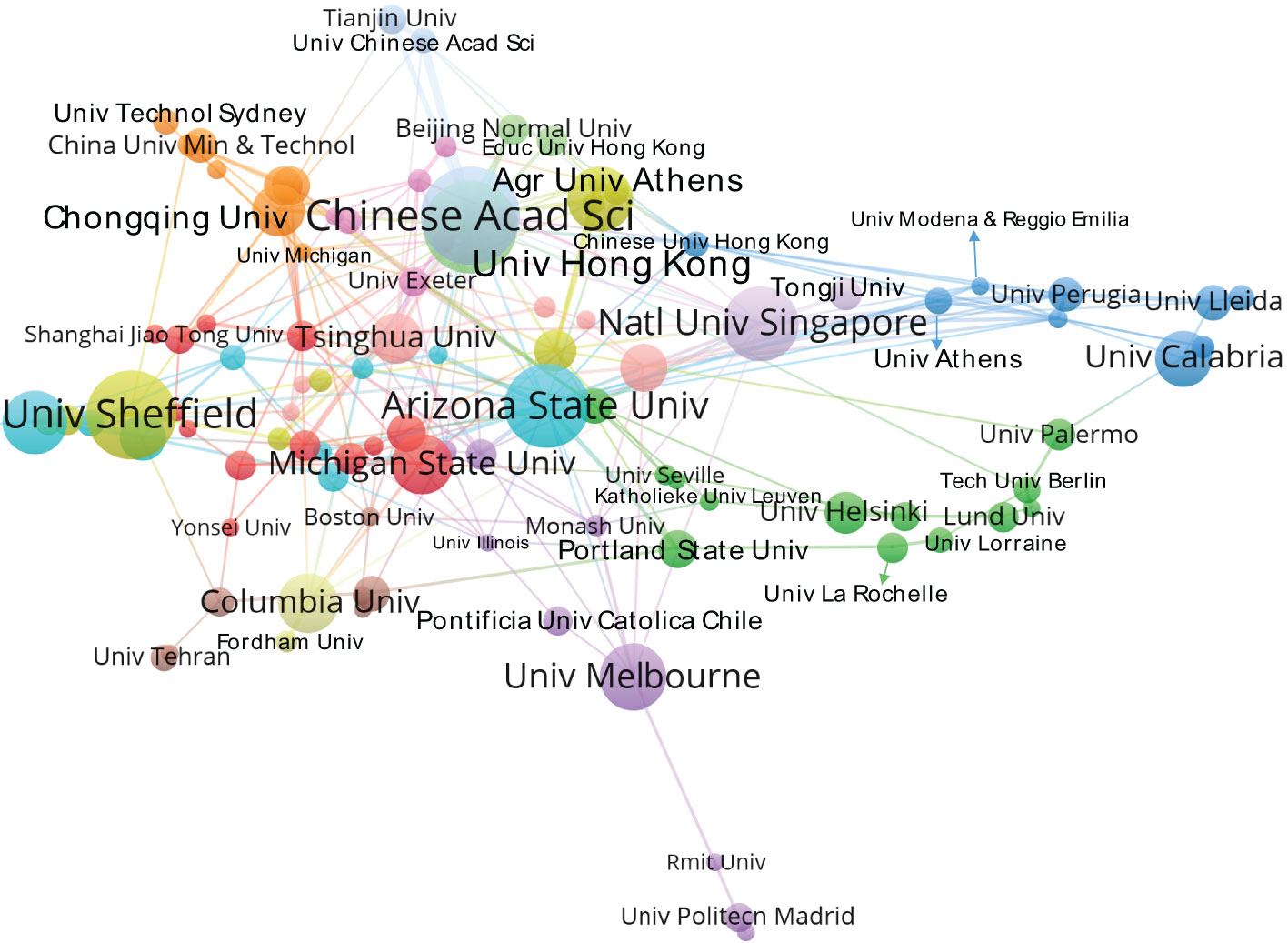
Figure 4 Knowledge graph depicting institutional cooperations involving more than ten publications on green roofs from 2000 to 2022.
No one institution dominates green roof research (Figure 4). Rather, the expertise is distributed across top global cities like Hong Kong, Paris, London, Singapore, and Melbourne, which are pioneers of green roof study (Shmelev and Shmeleva, 2019). The research institutions are grouped based on geographical proximity and shared regional expertise in green roofs. The red and light-blue clusters reflect the strong North American hubs, with major US universities at their core. The green and blue clusters represent European centers of excellence, with the green one anchored in Northern Europe and the blue in Southern Europe (Mediterranean region). The strength of Asia-Pacific is seen in the red and orange clusters, which are associated with leading Chinese, Korean, Hong Kong, and Australian universities. The yellow cluster bridges Western Europe and North America, reflecting the lingering trans-Atlantic association. While geography is the main grouping criterion, some universities are prominent connectors between regions. The Swedish University of Agricultural Sciences, Arizona State University, and the University of Hong Kong are present in multiple clusters. The clustering patterns demonstrate time-honored geographic coherence, with universities leveraging regional knowledge sharing while a few globalized institutions cross-pollinate and hybridize ideas between hubs. Identifying and learning the modus operandi of these networking universities could help improve global collaboration.
Therefore, international collaboration is essential to advancing green roof research in the face of urbanization and climate change challenges. Researchers can enhance the growth and dissemination of green roof expertise worldwide by leveraging regional knowledge sharing and fostering cooperation between clusters.
3.2.3 Journal citation analysis
The top 10 journals publishing green roof research extend across a broad interdisciplinary territory and signify an applied focus on sustainable buildings and infrastructure (Table 4). The journal Energy and Buildings has published the most green roof articles (179, 5.7%), which is logical given green roofs’ coupling with the building envelope and abilities to reduce building energy use and improve indoor thermal comfort. Sustainability and Building and Environment rank next, indicating the technology’s role in urban sustainable development. Ecological Engineering, and Urban Forestry & Urban Greening emphasize green roofs’ ecological benefits, while Water highlights the stormwater management applications. Major international companies like Elsevier and MDPI publish the leading journals. Most publishers originate from Europe (Switzerland, Netherlands, Germany), followed by North America.
Regarding the three-year impact, Landscape and Urban Planning and Urban Forestry & Urban Greening have the highest journal citation indicator (JCI) at around 2, reflecting green roofs’ expansion beyond engineering into urban ecology and planning. However, engineering-focused journals like Energy and Buildings still account for a large share of citations. As green roof research integrates concepts and insights across scientific domains, the publications have decidedly diversified beyond applied engineering into environmental, social, and economic sustainability fields (Berardi et al., 2014; Peng and Jim, 2015; Irga et al., 2017; Wachsmuth and Angelo, 2018). However, knowledge translation and collaboration between cognate disciplines covering engineering, ecology, climatology, and urban planning remain essential to advancing green roof understanding and deployment in cities worldwide.
3.2.4 Key authors
A visual review found 9091 authors associated with 3210 articles. The most frequently published author was C.Y. Jim from the Education University of Hong Kong (previously affiliated with the University of Hong Kong), with 52 total publications (Table 5). Applying Price’s Law (Li et al., 2022), the key contributors in green roof research were determined by the formula mp=0.749 , where mp is the minimum number of papers published by core authors during the period, and npmax is the number of papers by the author with the highest output. The calculation yielded mp= 5.30, identifying 1549 core authors with ≥ 6 publications. Table 5 lists the top ten authors with > 20 publications. These researchers are affiliated with institutions in China, Canada, the USA, UK, and Australia. Researchers from China have published extensively with high citation impact, including C.Y. Jim from Hong Kong. Other prolific authors are from Canada, the USA, UK, and Australia, including Jeremy Lundholm, Bradley D. Rowe, Virginia Stovin, and Claire Farrell. These researchers have collectively published over 150 articles, each with an h-index ranging from 15 to 27, indicating significant global expertise and influence in this research area.
VOSviewer generated the authors’ co-citation network knowledge graph, visualized in Figure 5, with each node representing one author. This diagram depicts the interconnected authorship in the research field. It shows the tangled and complicated linkages between authors, forming four prominent author clusters, each with a single or a few lead authors.
The red cluster includes scholars such as C.Y. Jim, D.J. Sailor, and U. Berardi. They are mainly committed to research on thermal performance, energy impacts, hydrology, microclimate effects, ecosystem services, cost-benefit analysis, policy development, and optimized integration of green roofs in urban environments (Sailor, 2008; Peng and Jim, 2013; Berardi et al., 2014). The authors have contributed significant insights into the multifunctional benefits of green roofs in varied climate-building-green roof permutations through field studies, modeling, and interdisciplinary analysis.
The green cluster includes J.C. Berndtsson, V. Stovin, K. Vijayaraghavan, N.D. Vanwoert, M. Shafique, and others. Their research focuses on green roof water and plant relationships, including stormwater and hydrology functions, moisture retention, drought tolerance, water quality improvements, plant dynamics, rooftop agriculture, thermal performance, and energy modeling (Berndtsson et al., 2008; Castleton et al., 2010; Vijayaraghavan and Raja, 2014). Their findings provide important insights into optimizing green roof performance through plant selection, substrate design, irrigation strategies, and integration with stormwater systems.
The blue cluster includes K.L. Getter, E. Oberndorfer, D.B. Rowe, A. Nagase, JS Macivor, and others. Their studies concentrate on biodiversity, habitat creation, plant dynamics, animal populations, thermal performance, energy modeling, moisture retention, and ecosystem services (Lundholm et al., 2010; Nagase and Dunnett, 2010; Rowe et al., 2012). Their results offer useful hints into optimizing green roof vegetation, substrates, and strategies to maximize ecological and energy benefits in urban areas.
The yellow cluster includes M. Santamouris, H. Akbari, T.R. Oke, H. Takebayashi, A. Synnefa, and others. They focus mainly on thermal performance and energy impacts of green roofs, including mitigating urban heat islands, energy savings, microclimate effects, heat flux, evapotranspiration, and cooling potential (Takebayashi and Moriyama, 2007; Santamouris, 2014; Akbari et al., 2016). Their work provides important quantitative insights into optimizing green roof design for maximum energy efficiency and climate change mitigation in urban areas.
3.2.5 Highly cited publications
Urban green roof research has expanded rapidly in the past 15 years, led by teams in North America, Europe, Asia, and Australia (Table 6). Highly cited studies in journals like Landscape and Urban Planning, BioScience, and Solar Energy demonstrate the crucial green roof benefits of mitigating the urban heat island through evaporative cooling, increased albedo, and shading (Santamouris, 2014), reducing building energy use by lowering cooling demands (Sadineni et al., 2011), retaining stormwater through absorption by vegetation and substrates (Mentens et al., 2006; Dietz, 2007; Czemiel Berndtsson, 2010), and providing wildlife habitat to support biodiversity (Oberndorfer et al., 2007). Additional studies show that integrating green roofs into urban planning can optimize sustainability benefits (Norton et al., 2015). This growing literature establishes that thoughtfully designed green roofs can help cities mitigate climate change impacts, manage stormwater, reduce energy use, support biodiversity, and advance resilience goals (Su et al., 2023). Further research can continue optimizing green infrastructure performance and integration.
3.3 Research hotspots
The growing body of green roof research has demonstrated the versatile abilities to provide sustainability benefits in urban areas (Berardi et al., 2014; Peng and Jim, 2015; Cortinovis et al., 2022), including mainly stormwater management, urban heat island mitigation, energy savings, and biodiversity enhancement (Figure 6). As cities worldwide increasingly recognize green roofs and walls as critical components of resilience and climate adaptation strategies, targeted research will be essential to augment adoption and ensure the realization of full green roof potential to create livable, sustainable, and regenerative urban environments. The following paragraphs discuss four key research hotspots.
(1) The red cluster is centered around the hydrologic functions of green roofs. The five top keywords are: green roof, green infrastructure, low-impact development, stormwater management, and sustainability (Figure 6). Green roofs are increasingly recognized as a critical green infrastructure and low-impact development solution to mitigate the harmful hydrologic impacts of urbanization (Mentens et al., 2006; Raimondi and Becciu, 2021). Many studies using field experiments, laboratory tests, and computational models have demonstrated green roofs’ significant hydrologic functions: stormwater retention, peak flow reduction, and water quality improvement through enhanced infiltration, evapotranspiration, and filtration (Alves et al., 2023). Field studies also quantified relationships between vegetation type, substrate depth, drainage layer design and water retention (Nagase and Dunnett, 2010; Sims et al., 2016). While most studies focused on individual green roofs, larger-scale modeling showed that widespread green roof installation combined with rainwater harvesting and detention practices could significantly reduce urban flooding and meet “sponge city” objectives in China (Liao et al., 2017). However, fulfilling such benefits is beset with challenges, including the lack of cost-benefit analysis, financial incentives, and difficulties in retrofitting existing buildings, resulting in limited green roof adoption (Peng and Jim, 2015).
(2) The green cluster highlights the cooling functions of green and cool roofs. The five top keywords are: urban heat island, cool roof, climate change, Envi-met, and urban greening. Green and cool roofs can mitigate urban heat islands (Figure 6) (Singh et al., 2020). Researchers have increasingly adopted the cooling effects of green roofs and high albedo of “cool roofs” to mitigate the urban heat island (Takebayashi and Moriyama, 2007; Lee et al., 2013; Santamouris, 2014). Many studies have used field measurements, remote sensing data, and microclimate models to assess green and cool roofs’ air-cooling benefits (Jim, 2015b; Vahmani et al., 2016). Extensive green roof installation can increase carbon stocks in Thessaloniki by 2.5 times based on high spatial resolution remote sensing data. The dynamic energy simulations found up to 5% heating and 16% cooling energy consumption reduction in some blocks (Karteris et al., 2016). However, widespread green and cool roof adoption is hindered by high costs and difficulties in retrofitting existing buildings (Shafique et al., 2018). Some studies combined green and cool roofs to tap their synergistic effects (Tewari et al., 2019). Several studies emphasized integrating urban heat island mitigation strategies with city planning using geospatial tools to strategically locate green and cool roofs to optimize cost-benefit (Norton et al., 2015). Future research can provide stronger economic incentives, develop lower-cost solutions for existing buildings, and leverage GIS analysis to identify priority areas for green and cool roof implementation to maximize urban heat island mitigation.
(3) The blue cluster concentrates on green wall functions of energy conservation and thermal comfort. The five top keywords are: green wall, energy saving, thermal performance, thermal comfort, green building, and energy efficiency (Figure 6). Green walls, also known as living walls or vertical gardens, can furnish energy-saving and thermal comfort benefits in buildings (Irga et al., 2017; Liberalesso et al., 2020). Green walls can reduce heat flux through building envelopes and lower energy consumption for cooling due to added thermal insulation properties (Perini et al., 2011). For example, heat flux through a green wall can reduce internal heat gain and electricity consumption compared to a blank wall (Andrić et al., 2019). Additionally, green walls can passively cool indoor environments through evaporation from plants and growing media (Perini et al., 2011; Norton et al., 2015). This cooling effect improves occupants’ thermal comfort by moderating indoor temperatures (Daghigh, 2015). Furthermore, green walls can reduce life-cycle energy consumption and carbon emissions compared to conventional walls by factoring in operational energy savings (Ottelé et al., 2011). However, some studies note that green walls may increase environmental impacts due to material use and maintenance (Liberalesso et al., 2020). Therefore, potential tradeoffs with other impacts highlight the need for holistic sustainability assessments (Manso et al., 2021).
(4) The yellow cluster is associated with the cost-effectiveness of extensive green roofs. The top five keywords are: extensive green roof, vegetative roof, urban ecology, biodiversity, and Sedum (Figure 6). Green roofs offer an effective nature-based solution in urban areas (Dorst et al., 2019). Extensive green roofs with shallow substrate depth are well-suited for retrofitting existing buildings. Hardy, low-maintenance succulent species such as Sedum were often planted (Rowe et al., 2012; Vijayaraghavan and Raja, 2014). Even in small or modular applications, extensive green roofs can enhance urban biodiversity by providing habitats for plants, insects, birds and other wildlife not typically found in built-up areas (Lundholm et al., 2010). Modeling studies of large-scale green roof deployment indicate that increased connectivity between patches can significantly improve habitat availability and species dispersal across metropolitan regions (Braaker et al., 2014). While upfront costs are rather high, life-cycle assessments (LCA) show that extensive green roofs’ energy savings and ecological benefits can render them competitive over their lifespan vis-a-vis conventional roofs (Clark et al., 2008; Tighnavard Balasbaneh et al., 2024). Green roofs exemplify ecological design innovations that can increase biodiversity, restore critical ecosystem functions, and foster more sustainable and resilient cities.
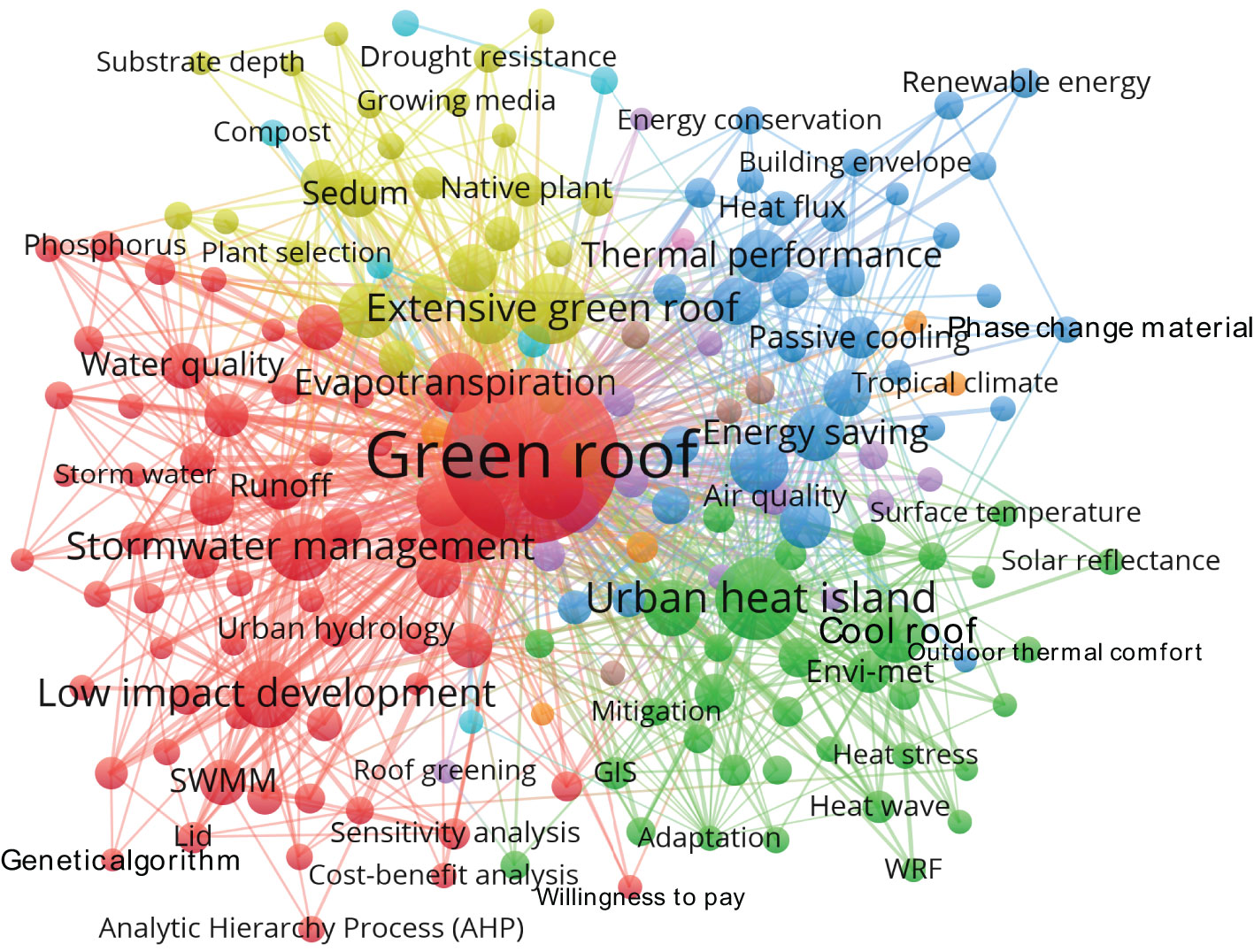
Figure 6 Knowledge graph depicting keyword co-occurrence and clustering of green roof research publications from 2000 to 2022.
3.4 Research frontiers
As green roofs continue to gain popularity as a nature-based solution in cities, ongoing research is pushing the frontier to improve understanding and unlock their full potential. The data analysis identified the following five notable research frontiers.
(1) Enhancing biodiversity and habitat provision. Some studies explore optimal plant selection and traits to support more diverse flora, fauna, and pollinators. An emerging trend investigates leveraging green roofs to enhance urban biodiversity and habitat provision. Thoughtful plant selection and trait-based design can transform rooftops into thriving ecosystems for birds, bees, butterflies and other wildlife (Williams et al., 2014). Strategically selecting native plants, sedums, alliums, and other taxa that provide critical food and nesting resources, ensuring flowering and fruiting times can spread across seasons to optimize foraging opportunities (MacIvor and Lundholm, 2011). Modeling tools and databases with plant trait details can enable more systematic selection for adaptive characteristics like drought tolerance and heat resistance, which will become increasingly important under climate change (Lundholm et al., 2010; Butler et al., 2012).
Critical research gaps remain regarding detailed plant-pollinator interactions, competition dynamics between native and invasive species, and reconciling aesthetic preferences with biodiversity goals (Williams et al., 2014). Green roofs can be integrated with green walls, parks, and other urban green infrastructure through ecological network modeling and spatial optimization techniques. The outcomes can provide exciting opportunities to augment biodiversity benefits across cities or metropolitan regions.
(2) Integrating green roofs with renewable energy systems. Incorporating solar photovoltaic panels, geothermal heat pumps, rainwater harvesting, and other systems with green roofs can maximize sustainability. An emerging research focus integrates green roofs with renewable energy technologies to maximize urban sustainability benefits (Manso et al., 2021; Liu et al., 2023). For example, green roofs are increasingly combined with building-applied photovoltaics (BAPVs) to form a symbiotic system that can enhance solar panel efficiency while mitigating the urban heat island effect (Santamouris, 2014). (Cruz Torres et al., 2023). Green roof-based rainwater harvesting systems can capture water for irrigation or non-potable building uses while reducing stormwater runoff volumes (Vijayaraghavan and Raja, 2014). Looking ahead, integrated optimization methodologies leveraging computational modeling can develop complementary configurations and sizing of green roof-renewable energy systems tailored to specific climates and building types (Daghigh, 2015; Sims et al., 2016; Morakinyo et al., 2017). More work is needed on long-term performance characterization, life-cycle cost analysis, and interactions between vegetation and renewable systems from an energy standpoint (Ottelé et al., 2011). Another research opportunity lies in policy and incentive structures to encourage joint adoption and cover incremental costs of integration (Irga et al., 2017; Wachsmuth and Angelo, 2018). Innovative studies can investigate how cities increasingly adopted zero-carbon goals, and climate resilience measures can foster combined green roof-renewable energy systems.
(3) Using computational tools and simulations. Applying machine learning, 3D modeling, and tools like EnergyPlus can optimize green roof design and forecast benefits. This development signifies a growing role for advanced computational tools and simulations in green roof design and performance optimization (Tsang and Jim, 2011; Wei et al., 2022). For example, machine learning techniques can improve plant species combination and trait selection and enhance stormwater retention, urban cooling, and biodiversity in different climates (Olivieri et al., 2013; Aparicio Uribe et al., 2022). 3D modeling can enable virtual prototyping to refine factors like substrate composition and depths, drainage layers, and layouts to balance green roof benefits and engineering constraints (Appio et al., 2019; Shmelev and Shmeleva, 2019). Whole building energy modeling platforms like EnergyPlus can integrate green roof modules to raise the simulation accuracy of impacts on building heating and cooling loads (Sailor, 2008; Cortinovis et al., 2022). As green roofs are increasingly adopted as urban resilience solutions, computational and simulation tools can integrate evaluation of performance, economics, and synergies with other technologies like solar PV (Cruz Torres et al., 2023). However, some knowledge gaps need attention, such as improving the model representation of complex soil-plant-atmosphere interactions, integrating site-specific parameters, and validating against field data (Zare et al., 2017). Multidisciplinary datasets can be enlisted to develop computational co-design frameworks to optimize green roof configurations. The findings can fulfill multiple objectives like energy savings, stormwater reduction, and urban heat island mitigation (James et al., 2009; Zare et al., 2017). Advanced simulations and digital design tools can pump-prime innovations and fine-tune green roof performance.
(4) Focus on appropriate technology for developing countries. Low-cost local materials like compressed earth blocks and passive cooling techniques can be evaluated for social and climate resilience. An important emerging research focus involves evaluating and optimizing green roof design for developing countries. Due to constraints in resources and technical capacity, recent work explores low-cost, locally available materials such as compressed earth blocks and recycled aggregates rather than importing components (Molineux et al., 2009). Green roofs requiring little maintenance for developing regions can be designed using native, drought-tolerant plants (Nagase and Dunnett, 2010; Butler et al., 2012). Innovative passive cooling techniques can integrate wind towers and solar chimneys with green roofs to enhance natural ventilation and indoor thermal comfort (Daghigh, 2015; Morakinyo et al., 2017). Beyond conventional rooftops, community-based greening of dwellings in informal settlements can place green sheets on corrugated metal sheet roofs. Such socially-relevant methods can offer social and environmental co-benefits and climate resilience for vulnerable urban populations (Kimemia et al., 2020). However, ensuring local community participation and addressing structural barriers related to the lack of incentives or supporting policies is equally important. Costs and technical capacity can be reduced through locally available materials and plants. Thus, green roofs combined with down-to-earth passive cooling techniques could provide much-needed indoor cooling. More applied research partnerships between universities and communities can focus on context-appropriate solutions and multiple co-benefits for social inclusion and environmental justice.
(5) Quantifying green roof co-benefits in cities. This field has attracted increasing research attention. For example, LCA studies have estimated cumulative energy savings and carbon sequestration benefits from reduced building heating and cooling loads (Peng and Jim, 2015; Cruz Torres et al., 2023). Economic valuations have reckoned cost savings from avoided stormwater infrastructure expansion and substituting it with retention and detention functions of green roofs (Alim et al., 2022). The air quality improvement brought by green roofs can generate public health benefits, as indicated by reduced asthma attacks. This important function of removing particulate matter and gaseous pollutants can be quantified (Singh et al., 2020). Social science methods can measure the mental health value of green roof access for urban residents and workers (Lee et al., 2015). Emerging co-optimization tools can explain tradeoffs between maximizing green roof outcomes like energy savings versus stormwater reduction and biodiversity enhancement (Lundholm et al., 2010; Ma et al., 2023). However, variability in monetization techniques and inconsistent valuation assumptions remain challenging, and demand continued research. Developing widely accepted metrics and standardized methods is crucial for green roof applications. Overall, comprehensive performance quantification and benefit monetization can strengthen the economic case for policy incentives like green roof mandates. It also opens innovative funding opportunities to subsidize or promote green roof installation, such as payment for ecosystem services schemes (Calderón-Argelich et al., 2021).
In addition, a major challenge in green roof research is the lack of accurate quantification and accounting of carbon reduction and sink enhancement benefits. In the complex urban carbon cycle, Green roofs involve natural carbon sequestration through plants and substrates and artificial pathways related to artificial materials, components, installation, and maintenance (Zaid et al., 2018; Dong and He, 2023). This “natural-artificial” duality leads to difficulties in measuring green roofs’ net carbon footprint (Dorst et al., 2019; Cortinovis et al., 2022; Liu et al., 2023). The complex interactions between biotic and abiotic urban carbon fluxes of green roofs require detailed assessment, monitoring and modeling to systematically map carbon pools and flows (Karteris et al., 2016; Zare et al., 2017; Aparicio Uribe et al., 2022). However, limitations in current LCA methods have generated uncertainties about the magnitude of green roof carbon impacts. To enable robust accounting, future efforts must improve LCA protocols tailored to urban ecosystems with a hybrid natural-artificial carbon cycling regime. It is necessary to develop integrated measurement and modeling frameworks to capture the holistic carbon footprint of green roofs across biotic and abiotic components. A more accurate quantification can offer stronger evidence of carbon benefits to promote adoption.
3.5 Implications and limitations of the study
This study on green roofs has identified several areas for future study, policymaking, and practical applications to enhance urban sustainability. Longitudinal studies covering a certain period can assess the long-term sustainability and resilience of green roof ecosystems. Additionally, comparative studies that evaluate different green roof configurations under diverse climatic conditions can offer valuable insights for optimizing design strategies. Exploring the socioeconomic benefits of green roofs can permit a more comprehensive understanding of their overall value and contributions to the community. Policymakers can leverage our findings to develop supportive policies, incentives, and regulations, highlighting the potential of green roofs as a sustainable urban solution. However, our study indicates that further research is required to assess their economic feasibility and scalability. Professionals involved in green roof design, installation, and maintenance can use our results to make informed decisions regarding plant selection, substrate composition, and maintenance practices. Our findings can guide practitioners in tailoring green roof designs to match site conditions and maximize their environmental benefits in specific urban contexts. Lastly, sharing case studies and best practices from successful green roof projects can facilitate knowledge transfer, foster collaboration among practitioners, and advance professional practice standard.
The reliance on bibliometric data as the primary source of analysis is a limitation of our study. Although bibliometric analysis provides valuable insights into the trends, patterns, partnerships, and scholarly impact of research in the field, it is important to note that it does not capture the full scope of green roof research. The exclusion of non-indexed or non-academic literature, such as gray literatures or reports from industry practitioners, may have resulted in an incomplete representation of the field. Future studies could incorporate a broader range of sources, including non-traditional forms of literature, to gain a more comprehensive understanding. Another limitation is related to potential language biases. Our study focused primarily on English-language publications and excluded relevant research in other languages. This language bias could affect the generalizability and inclusiveness of our findings. Future studies could overcome this constraint by conducting multilingual searches and incorporating research published in various languages to provide a more globally representative view of green roof research. Furthermore, it is important to acknowledge that our study is based on a specific time frame, covering two decades of research. As such, our findings may not have captured the latest developments in the field. Green roof research is a rapidly evolving field characterized by constantly emerging new study directions, technologies, and methodologies. To address this omission, future research could consider conducting more frequent systematic reviews or employing real-time data collection methods to capture the most up-to-date trends and advancements.
4 Conclusion
Green roofs have become an important nature-based solution for enhancing urban sustainability and resilience. However, research gaps remain in quantifying their full carbon impacts and optimizing their design for the developing world context. Recent studies show that urban vegetation can provide notable carbon sequestration benefits. However, accurately measuring the net carbon footprint of green roofs and walls has remained challenging due to the diverse emission sources, pathways, and quantities associated with varied materials, installation, and maintenance. Computational tools using LCA and process-based biogeochemical modeling show promise for holistically assessing carbon fluxes. However, improved sensor data collection and model parameterization are needed.
Some evidence suggests green roofs may confer cooling benefits in some developing world cities. More research is required to improve the design and selection of plant species assemblages, including more native members, composition and properties of growing mediums, and irrigation practices for cost-effectiveness, climate suitability, and local relevance to natural and socioeconomic conditions in different regions. As cities grapple with converging sustainability crises, nature-based solutions like green roofs and walls are increasingly prioritized in climate adaptation and ecosystem service strategies. However, design tradeoffs related to biodiversity, energy use, and microclimate regulation are complex. Continued research can develop multi-criteria sustainability frameworks and scenario modeling to evaluate ecosystem service bundles and improve decision support.
Additionally, connecting green infrastructure into conjoined landscape-scale green networks demonstrates the potential to enhance and synergize associated ecological benefits. In summary, key research directions are proposed to advance green roofs and walls as innovative and enlightened urban sustainability solutions. They include improving biogeochemical models and sensor networks to strengthen carbon accounting; optimizing designs for the developing world context through climate-smart plant selection and optimized resource-use efficiency; leveraging emerging computational tools to evaluate ecosystem service tradeoffs; and integrating green roofs and walls into interconnected urban green networks to maximize biodiversity and resilience functions. Addressing these research gaps through interdisciplinary approaches and international collaboration can provide a stronger foundation to harness the full potential of urban green infrastructure.
Author contributions
CX: Data curation, Formal Analysis, Investigation, Methodology, Software, Writing – original draft. DL: Data curation, Formal Analysis, Investigation, Software, Writing – review & editing. CJ: Formal Analysis, Validation, Writing – review & editing.
Funding
The author(s) declare financial support was received for the research, authorship, and/or publication of this article. This research was funded by the National Natural Science Foundation of China (grant number: 32360417), the Natural Science Foundation of Hainan Province (grant number: 423MS061, 623RC519), and the Education Department of Hainan Province (grant number: Hnky2023ZD-17).
Conflict of interest
The authors declare that the research was conducted in the absence of any commercial or financial relationships that could be construed as a potential conflict of interest.
Publisher’s note
All claims expressed in this article are solely those of the authors and do not necessarily represent those of their affiliated organizations, or those of the publisher, the editors and the reviewers. Any product that may be evaluated in this article, or claim that may be made by its manufacturer, is not guaranteed or endorsed by the publisher.
References
Adem Esmail B., Suleiman L. (2020). Analyzing evidence of sustainable urban water management systems: A review through the lenses of sociotechnical transitions. Sustainability 12 (11), 4481. doi: 10.3390/su12114481
Akbari H., Cartalis C., Kolokotsa D., Muscio A., Pisello A. L., Rossi F., et al. (2016). Local climate change and urban heat island mitigation techniques - The state of the art. J. Civil Eng. Manage. 22 (1), 1–16. doi: 10.3846/13923730.2015.1111934
Alexandri E., Jones P. (2008). Temperature decreases in an urban canyon due to green walls and green roofs in diverse climates. Building and environment 43 (4), 480–493. doi: 10.1016/j.buildenv.2006.10.055
Al Sayah M. J., Versini P.-A., Schertzer D. (2023). “Chapter 8 - Exploring nature-based adaptation solutions for urban ecohydrology: Definitions, concepts, institutional framework, and demonstration,” in Handbook of Hydroinformatics. Eds. Eslamian S., Eslamian F. (Amsterdam, Netherlands: Elsevier), 117–135.
Alim M. A., Rahman A., Tao Z., Garner B., Griffith R., Liebman M. (2022). Green roof as an effective tool for sustainable urban development: An Australian perspective in relation to stormwater and building energy management. J. Cleaner Production 362, 132561. doi: 10.1016/j.jclepro.2022.132561
Alves R. A., Rudke A. P., Martins L. D., de Melo Souza S. T., Bovo A. B., Goroski Rambalducci M. J., et al. (2023). Urban stormwater management from the perspective of nature-based solutions: a bibliometric review. J. Ecohydraulics, 1–21. doi: 10.1080/24705357.2023.2284392
Andrić I., Koc M., Al-Ghamdi S. G. (2019). A review of climate change implications for built environment: Impacts, mitigation measures and associated challenges in developed and developing countries. J. Cleaner Production 211, 83–102. doi: 10.1016/j.jclepro.2018.11.128
Aparicio Uribe C. H., Bonilla Brenes R., Hack J. (2022). Potential of retrofitted urban green infrastructure to reduce runoff - A model implementation with site-specific constraints at neighborhood scale. Urban Forestry Urban Greening 69, 127499. doi: 10.1016/j.ufug.2022.127499
Appio F. P., Lima M., Paroutis S. (2019). Understanding Smart Cities: Innovation ecosystems, technological advancements, and societal challenges. Technological Forecasting Soc. Change 142, 1–14. doi: 10.1016/j.techfore.2018.12.018
Berardi U., GhaffarianHoseini A., GhaffarianHoseini A. (2014). State-of-the-art analysis of the environmental benefits of green roofs. Appl. Energy 115, 411–428. doi: 10.1016/j.apenergy.2013.10.047
Berndtsson J. C., Bengtsson L., Jinno K. (2008). First flush effect from vegetated roofs during simulated rain events. Hydrology Res. 39 (3), 171–179. doi: 10.2166/nh.2008.044
Berndtsson J. C. (2010). Green roof performance towards management of runoff water quantity and quality: A review. Ecological engineering 36 (1), 351–360. doi: 10.1016/j.ecoleng.2009.12.014
Bowler D. E., Buyung-Ali L., Knight T. M., Pullin A. S. (2010). Urban greening to cool towns and cities: A systematic review of the empirical evidence. Landscape and urban planning 97 (3), 147–155. doi: 10.1016/j.landurbplan.2010.05.006
Braaker S., Ghazoul J., Obrist M. K., Moretti M. (2014). Habitat connectivity shapes urban arthropod communities: the key role of green roofs. Ecology 95 (4), 1010–1021. doi: 10.1890/13-0705.1
Butler C., Butler E., Orians C. M. (2012). Native plant enthusiasm reaches new heights: Perceptions, evidence, and the future of green roofs. Urban Forestry Urban Greening 11 (1), 1–10. doi: 10.1016/j.ufug.2011.11.002
Calderón-Argelich A., Benetti S., Anguelovski I., Connolly J. J. T., Langemeyer J., Baró F. (2021). Tracing and building up environmental justice considerations in the urban ecosystem service literature: A systematic review. Landscape Urban Plann. 214, 104130. doi: 10.1016/j.landurbplan.2021.104130
Castleton H. F., Stovin V., Beck S. B. M., Davison J. B. (2010). Green roofs; building energy savings and the potential for retrofit. Energy Buildings 42 (10), 1582–1591. doi: 10.1016/j.enbuild.2010.05.004
Chen S., Gou Z. (2022). An investigation of green roof spatial distribution and incentive policies using green buildings as a benchmark. Land 11 (11), 2067. doi: 10.3390/land11112067
Clark C., Adriaens P., Talbot F. B. (2008). Green roof valuation: A probabilistic economic analysis of environmental benefits. Environ. Sci. Technol. 42 (6), 2155–2161. doi: 10.1021/es0706652
Clémençon R. (2016). The two sides of the Paris Climate Agreement: Dismal failure or historic breakthrough? J. Environ. Dev. 25 (1), 3–24. doi: 10.1177/1070496516631362
Cortinovis C., Olsson P., Boke-Olén N., Hedlund K. (2022). Scaling up nature-based solutions for climate-change adaptation: Potential and benefits in three European cities. Urban Forestry Urban Greening 67, 127450. doi: 10.1016/j.ufug.2021.127450
Coutts A. M., Tapper N. J., Beringer J., Loughnan M., Demuzere M. (2012). Watering our cities: The capacity for water sensitive urban design to support urban cooling and improve human thermal comfort in the Australian context. Prog. Phys. Geography: Earth Environ. 37 (1), 2–28. doi: 10.1177/0309133312461032
Cruz Torres F., Babí Almenar J., Rugani B. (2023). Photovoltaic-green roof energy communities can uphold the European Green Deal: Probabilistic cost-benefit analyses help discern economically convenient scenarios. J. Cleaner Production 414, 137428. doi: 10.1016/j.jclepro.2023.137428
Czemiel Berndtsson J. (2010). Green roof performance towards management of runoff water quantity and quality: A review. Ecol. Eng. 36 (4), 351–360. doi: 10.1016/j.ecoleng.2009.12.014
Daghigh R. (2015). Assessing the thermal comfort and ventilation in Malaysia and the surrounding regions. Renewable Sustain. Energy Rev. 48, 681–691. doi: 10.1016/j.rser.2015.04.017
Dietz M. E. (2007). Low Impact development practices: A review of current research and recommendations for future directions. Water Air Soil pollut. 186 (1), 351–363. doi: 10.1007/s11270-007-9484-z
Diwanji V. S. (2022). Fuzzy-set qualitative comparative analysis in consumer research: A systematic literature review. Int. J. Consumer Stud. 47 (6), 2767–2789. doi: 10.1111/ijcs.12889
Dobrzańska J., Nadolny A., Kalbarczyk R., Ziemiańska M. (2022). Urban resilience and residential greenery & mdash: The evidence from Poland. Sustainability 14 (18), 11317. doi: 10.3390/su141811317
Dong X., He B.-J. (2023). A standardized assessment framework for green roof decarbonization: A review of embodied carbon, carbon sequestration, bioenergy supply, and operational carbon scenarios. Renewable Sustain. Energy Rev. 182, 113376. doi: 10.1016/j.rser.2023.113376
Dorst H., van der Jagt A., Raven R., Runhaar H. (2019). Urban greening through nature-based solutions – Key characteristics of an emerging concept. Sustain. Cities Soc. 49, 101620. doi: 10.1016/j.scs.2019.101620
Essuman-Quainoo B., Jim C. Y. (2023). Understanding the drivers of green roofs and green walls adoption in Global South cities: Analysis of Accra, Ghana. Urban Forestry Urban Greening 89, 128106. doi: 10.1016/j.ufug.2023.128106
Frazer L. (2005). Paving Paradise: The peril of impervious surfaces. Environ. Health Perspect. 113 (7), A456–A462. doi: 10.1289/ehp.113-a456
Hirsch J. E. (2005). An index to quantify an individual's scientific research output. Proc. Natl. Acad. Sci. 102 (46), 16569–16572. doi: 10.1073/pnas.0507655102
Irga P. J., Braun J. T., Douglas A. N. J., Pettit T., Fujiwara S., Burchett M. D., et al. (2017). The distribution of green walls and green roofs throughout Australia: Do policy instruments influence the frequency of projects? Urban Forestry Urban Greening 24, 164–174. doi: 10.1016/j.ufug.2017.03.026
James P., Tzoulas K., Adams M. D., Barber A., Box J., Breuste J., et al. (2009). Towards an integrated understanding of green space in the European built environment. Urban Forestry Urban Greening 8 (2), 65–75. doi: 10.1016/j.ufug.2009.02.001
Jim C. Y. (2015a). Assessing climate-adaptation effect of extensive tropical green roofs in cities. Landscape Urban Plann. 138, 54–70. doi: 10.1016/j.landurbplan.2015.02.014
Jim C. Y. (2015b). Thermal performance of climber greenwalls: Effects of solar irradiance and orientation. Appl. Energy 154, 631–643. doi: 10.1016/j.apenergy.2015.05.077
Jim C. Y., Hui L. C. (2022). Offering green roofs in a compact city: Benefits and landscape preferences of socio-demographic cohorts. Appl. Geogr. 145, 102733. doi: 10.1016/j.apgeog.2022.102733
Jim C. Y., Hui L. C., Rupprecht C. D. D. (2022). Public perceptions of green roofs and green walls in Tokyo, Japan: A call to heighten awareness. Environ. Manage. 70 (1), 35–53. doi: 10.1007/s00267-022-01625-8
Karteris M., Theodoridou I., Mallinis G., Tsiros E., Karteris A. (2016). Towards a green sustainable strategy for Mediterranean cities: Assessing the benefits of large-scale green roofs implementation in Thessaloniki, Northern Greece, using environmental modelling, GIS and very high spatial resolution remote sensing data. Renewable Sustain. Energy Rev. 58, 510–525. doi: 10.1016/j.rser.2015.11.098
Kimemia D., Van Niekerk A., Annegarn H., Seedat M. (2020). Passive cooling for thermal comfort in informal housing. J. Energy South. Afr. 31, 28–39. doi: 10.17159/2413-3051/2020/v31i1a7689
Lee J. S., Kim J. T., Lee M. G. (2013). Mitigation of urban heat island effect and greenroofs. Indoor Built Environ. 23 (1), 62–69. doi: 10.1177/1420326X12474483
Lee K. E., Williams K. J. H., Sargent L. D., Williams N. S. G., Johnson K. A. (2015). 40-second green roof views sustain attention: The role of micro-breaks in attention restoration. J. Environ. Psychol. 42, 182–189. doi: 10.1016/j.jenvp.2015.04.003
Li J., Mao Y., Ouyang J., Zheng S. (2022). A review of urban microclimate research based on CiteSpace and VOSviewer analysis. Int. J. Environ. Res. Public Health 19 (8), 4741. doi: 10.3390/ijerph19084741
Li W. C., Yeung K. K. A. (2014). A comprehensive study of green roof performance from environmental perspective. Int. J. Sustain. Built Environ. 3 (1), 127–134. doi: 10.1016/j.ijsbe.2014.05.001
Liao K.-H., Deng S., Tan P. Y. (2017). “Blue-Green infrastructure: New frontier for sustainable urban stormwater management,” in Greening Cities: Forms and Functions. Eds. Tan P. Y., Jim C. Y. (Singapore: Springer Singapore), 203–226.
Liberalesso T., Oliveira Cruz C., Matos Silva C., Manso M. (2020). Green infrastructure and public policies: An international review of green roofs and green walls incentives. Land Use Policy 96, 104693. doi: 10.1016/j.landusepol.2020.104693
Liu H.-Y., Skandalos N., Braslina L., Kapsalis V., Karamanis D. (2023). Integrating solar energy and nature-based solutions for climate-neutral urban environments. Solar 3, 382–415. doi: 10.3390/solar3030022
Lundholm J., MacIvor J. S., MacDougall Z., Ranalli M. (2010). Plant species and functional group combinations affect green roof ecosystem functions. PLoS One 5 (3). doi: 10.1371/journal.pone.0009677
Ma N., Zhang Y., Zhang R., Zhang W., Li X. (2023). Comprehensive review of food-energy-water nexus at the community scale. J. Cleaner Production 420, 138311. doi: 10.1016/j.jclepro.2023.138311
MacIvor J. S., Lundholm J. (2011). Insect species composition and diversity on intensive green roofs and adjacent level-ground habitats. Urban Ecosyst. 14 (2), 225–241. doi: 10.1007/s11252-010-0149-0
Manso M., Teotónio I., Silva C. M., Cruz C. O. (2021). Green roof and green wall benefits and costs: A review of the quantitative evidence. Renewable Sustain. Energy Rev. 135, 110111. doi: 10.1016/j.rser.2020.110111
McKenzie T. (2020). “Environmental innovations in urban ecosystems,” in Introduction to Agroecology. Eds. Caldwell C. D., Wang S. (Singapore: Springer Singapore), 237–258.
Mendonça F. (2023). “Urban floodings: Conceptions and challenges in the scope of global climate change – A look at the city of São Paulo, Brazil,” in Urban Flooding in Brazil. Eds. Mendonça F., Farias A., Buffon E. (Cham: Springer International Publishing), 3–20.
Mentens J., Raes D., Hermy M. (2006). Green roofs as a tool for solving the rainwater runoff problem in the urbanized 21st century? Landscape Urban Plann. 77 (3), 217–226. doi: 10.1016/j.landurbplan.2005.02.010
Mesimäki M., Hauru K., Kotze D. J., Lehvävirta S. (2017). Neo-spaces for urban livability? Urbanites’ versatile mental images of green roofs in the Helsinki metropolitan area, Finland. Land Use Policy 61, 587–600. doi: 10.1016/j.landusepol.2016.11.021
Molineux C. J., Fentiman C. H., Gange A. C. (2009). Characterising alternative recycled waste materials for use as green roof growing media in the U.K. Ecol. Eng. 35 (10), 1507–1513. doi: 10.1016/j.ecoleng.2009.06.010
Morakinyo T. E., Dahanayake K.W.D.K.C., Ng E., Chow C. L. (2017). Temperature and cooling demand reduction by green-roof types in different climates and urban densities: A co-simulation parametric study. Energy Buildings 145, 226–237. doi: 10.1016/j.enbuild.2017.03.066
Nagase A., Dunnett N. (2010). Drought tolerance in different vegetation types for extensive green roofs: Effects of watering and diversity. Landscape Urban Plann. 97 (4), 318–327. doi: 10.1016/j.landurbplan.2010.07.005
Norton B. A., Coutts A. M., Livesley S. J., Harris R. J., Hunter A. M., Williams N. S. G. (2015). Planning for cooler cities: A framework to prioritise green infrastructure to mitigate high temperatures in urban landscapes. Landscape Urban Plann. 134, 127–138. doi: 10.1016/j.landurbplan.2014.10.018
Oberndorfer E., Lundholm J., Bass B., Coffman R. R., Doshi H., Dunnett N., et al. (2007). Green roofs as urban ecosystems: Ecological structures, functions, and services. BioScience 57 (10), 823–833. doi: 10.1641/B571005
Olivieri F., Di Perna C., D’Orazio M., Olivieri L., Neila J. (2013). Experimental measurements and numerical model for the summer performance assessment of extensive green roofs in a Mediterranean coastal climate. Energy Buildings 63, 1–14. doi: 10.1016/j.enbuild.2013.03.054
Orduña-Malea E., Costas R. (2021). Link-based approach to study scientific software usage: the case of VOSviewer. Scientometrics 126 (9), 8153–8186. doi: 10.1007/s11192-021-04082-y
Ottelé M., Perini K., Fraaij A. L. A., Haas E. M., Raiteri R. (2011). Comparative life cycle analysis for green façades and living wall systems. Energy Buildings 43 (12), 3419–3429. doi: 10.1016/j.enbuild.2011.09.010
Oyewola D. O., Dada E. G. (2022). Exploring machine learning: a scientometrics approach using bibliometrix and VOSviewer. SN Appl. Sci. 4 (5), 143. doi: 10.1007/s42452-022-05027-7
Peng L. L. H., Jim C. Y. (2013). Green-roof effects on neighborhood microclimate and human thermal sensation. Energies 6 (2), 598–618. doi: 10.3390/en6020598
Peng L. L. H., Jim C. Y. (2015). Economic evaluation of green-roof environmental benefits in the context of climate change: The case of Hong Kong. Urban Forestry Urban Greening 14 (3), 554–561. doi: 10.1016/j.ufug.2015.05.006
Peng J., Zhang J. (2022). Urban flooding risk assessment based on GIS- game theory combination weight: A case study of Zhengzhou City. Int. J. Disaster Risk Reduction 77, 103080. doi: 10.1016/j.ijdrr.2022.103080
Perini K., Ottelé M., Fraaij A. L. A., Haas E. M., Raiteri R. (2011). Vertical greening systems and the effect on air flow and temperature on the building envelope. Building Environ. 46 (11), 2287–2294. doi: 10.1016/j.buildenv.2011.05.009
Raimondi A., Becciu G. (2021). Performance of green roofs for rainwater control. Water Resour. Manage. 35 (1), 99–111. doi: 10.1007/s11269-020-02712-3
Rowe D. B., Getter K. L., Durhman A. K. (2012). Effect of green roof media depth on Crassulacean plant succession over seven years. Landscape Urban Plann. 104 (3-4), 310–319. doi: 10.1016/j.landurbplan.2011.11.010
Sadineni S. B., Madala S., Boehm R. F. (2011). Passive building energy savings: A review of building envelope components. Renewable Sustain. Energy Rev. 15 (8), 3617–3631. doi: 10.1016/j.rser.2011.07.014
Saha M., Eckelman M. J. (2017). Growing fresh fruits and vegetables in an urban landscape: A geospatial assessment of ground level and rooftop urban agriculture potential in Boston, USA. Landscape Urban Plann. 165, 130–141. doi: 10.1016/j.landurbplan.2017.04.015
Sailor D. J. (2008). A green roof model for building energy simulation programs. Energy Buildings 40 (8), 1466–1478. doi: 10.1016/j.enbuild.2008.02.001
Santamouris M. (2014). Cooling the cities - A review of reflective and green roof mitigation technologies to fight heat island and improve comfort in urban environments. Solar Energy 103, 682–703. doi: 10.1016/j.solener.2012.07.003
Shafique M., Kim R., Rafiq M. (2018). Green roof benefits, opportunities and challenges – A review. Renewable Sustain. Energy Rev. 90, 757–773. doi: 10.1016/j.rser.2018.04.006
Shmelev S. E., Shmeleva I. A. (2019). Multidimensional sustainability benchmarking for smart megacities. Cities 92, 134–163. doi: 10.1016/j.cities.2019.03.015
Sims A. W., Robinson C. E., Smart C. C., Voogt J. A., Hay G. J., Lundholm J. T., et al. (2016). Retention performance of green roofs in three different climate regions. J. Hydrology 542, 115–124. doi: 10.1016/j.jhydrol.2016.08.055
Singh N., Singh S., Mall R. K. (2020). “Chapter 17 - Urban ecology and human health: implications of urban heat island, air pollution and climate change nexus,” in Urban Ecology. Eds. Verma P., Singh P., Singh R., Raghubanshi A. S. (Amsterdam, the Netherlands: Elsevier), 317–334.
Su J., Wang M., Razi M. A., Dom N. M., Sulaiman N., Tan L.-W. (2023). A bibliometric review of nature-based solutions on urban stormwater management. Sustainability 15 (9), 7281. doi: 10.3390/su15097281
Takebayashi H., Moriyama M. (2007). Surface heat budget on green roof and high reflection roof for mitigation of urban heat island. Building Environ. 42 (8), 2971–2979. doi: 10.1016/j.buildenv.2006.06.017
Taşkın A., Saban F. D. (2023). Swallowed by the urbanization: Spatial evolution of Adana Bağlarbaşı District. J. Design Resilience Architecture Plann. 4 (2), 57–71. doi: 10.47818/DRArch.2023.v4i2093
Tewari M., Yang J., Kusaka H., Salamanca F., Watson C., Treinish L. (2019). Interaction of urban heat islands and heat waves under current and future climate conditions and their mitigation using green and cool roofs in New York City and Phoenix, Arizona. Environ. Res. Lett. 14 (3), 034002. doi: 10.1088/1748-9326/aaf431
Tighnavard Balasbaneh A., Sher W., Madun A., Ashour A. (2024). Life cycle sustainability assessment of alternative green roofs – A systematic literature review. Building Environ. 248, 111064. doi: 10.1016/j.buildenv.2023.111064
Toledo R., Miranda Junior H. L., Farias Filho J. R., Costa H. G. (2019). A scientometric review of global research on sustainability and project management dataset. Data Brief 25, 104312. doi: 10.1016/j.dib.2019.104312
Tsang S. W., Jim C. Y. (2011). Theoretical evaluation of thermal and energy performance of tropical green roofs. Energy 36 (5), 3590–3598. doi: 10.1016/j.energy.2011.03.072
Vahmani P., Sun F., Hall A., Ban-Weiss G. (2016). Investigating the climate impacts of urbanization and the potential for cool roofs to counter future climate change in Southern California. Environ. Res. Lett. 11 (12), 124027. doi: 10.1088/1748-9326/11/12/124027
van Eck N., Waltman L. (2010). Software survey: VOSviewer, a computer program for bibliometric mapping. Scientometrics 84 (2), 523–538. doi: 10.1007/s11192-009-0146-3
Vijayaraghavan K., Raja F. D. (2014). Design and development of green roof substrate to improve runoff water quality: Plant growth experiments and adsorption. Water Res. 63, 94–101. doi: 10.1016/j.watres.2014.06.012
Wachsmuth D., Angelo H. (2018). Green and gray: New ideologies of nature in urban sustainability policy. Ann. Am. Assoc. Geographers 108 (4), 1038–1056. doi: 10.1080/24694452.2017.1417819
Wang P., Li Y., Zhang Y. (2021). An urban system perspective on urban flood resilience using SEM: evidence from Nanjing city, China. Natural Hazards 109 (3), 2575–2599. doi: 10.1007/s11069-021-04933-0
Wang M., Sun C., Zhang D. (2023). Opportunities and challenges in green stormwater infrastructure (GSI): A comprehensive and bibliometric review of ecosystem services from 2000 to 2021. Environ. Res. 236, 116701. doi: 10.1016/j.envres.2023.116701
Wei T., Jim C. Y., Chen Y., Chen A., Li X. (2022). Complementary influence of green-roof and roof-slab thermal conductivity on winter indoor warming assessed by finite element analysis. Energy Rep. 8, 14852–14864. doi: 10.1016/j.egyr.2022.11.019
Williams N. S. G., Lundholm J., Scott MacIvor J. (2014). Forum: Do green roofs help urban biodiversity conservation? J. Appl. Ecol. 51 (6), 1643–1649. doi: 10.1111/1365-2664.12333
Wolch J. R., Byrne J., Newell J. P. (2014). Urban green space, public health, and environmental justice: The challenge of making cities `just green enough'. Landscape and urban planning 135, 234–244. doi: 10.1016/j.landurbplan.2014.01.017
Wong G. K. L., Jim C. Y. (2014). Quantitative hydrologic performance of extensive green roof under humid-tropical rainfall regime. Ecol. Eng. 70, 366–378. doi: 10.1016/j.ecoleng.2014.06.025
Wong G. K. L., Jim C. Y. (2015). Identifying keystone meteorological factors of green-roof stormwater retention to inform design and planning. Landscape Urban Plann. 143, 173–182. doi: 10.1016/j.landurbplan.2015.07.001
Xu Y., Tang B.-s., Chan E. H. W. (2011). State-led land requisition and transformation of rural villages in transitional China. Habitat Int. 35 (1), 57–65. doi: 10.1016/j.habitatint.2010.03.002
Zaid S. M., Perisamy E., Hussein H., Myeda N. E., Zainon N. (2018). Vertical greenery system in urban tropical climate and its carbon sequestration potential: A review. Ecol. Indic. 91, 57–70. doi: 10.1016/j.ecolind.2018.03.086
Keywords: green roof, bibliometric analysis, research hotspot, research frontier, nature-based solution, urban sustainability
Citation: Xie C, Liu D and Jim CY (2024) Vicissitudes and prospects of green roof research: a two-decade systematic bibliometric review. Front. Ecol. Evol. 11:1331930. doi: 10.3389/fevo.2023.1331930
Received: 02 November 2023; Accepted: 18 December 2023;
Published: 08 January 2024.
Edited by:
Ümüt Halik, Xinjiang University, ChinaReviewed by:
Mo Wang, Guangzhou University, ChinaAliya Baidourela, Xinjiang Agricultural University, China
Copyright © 2024 Xie, Liu and Jim. This is an open-access article distributed under the terms of the Creative Commons Attribution License (CC BY). The use, distribution or reproduction in other forums is permitted, provided the original author(s) and the copyright owner(s) are credited and that the original publication in this journal is cited, in accordance with accepted academic practice. No use, distribution or reproduction is permitted which does not comply with these terms.
*Correspondence: C. Y. Jim, Y3lqaW1AZWR1aGsuaGs=