- Department of Biology, College of Science, Princess Nourah bint Abdulrahman University, Riyadh, Saudi Arabia
Background: Soils contaminated with heavy metals (HMs) pose a risk to human health via the food chain, as many edible plants absorb these metals. In turn, some of these plants could be used as phytoremediators for such soils. Calligonum comosum is an indigenous medicinal shrub that grows naturally in wide swaths of sandy soil in Saudi Arabia and has many advantages that render it a promising candidate for the treatment of HM-contaminated areas. But the impact of HM on this plant remains unknown, especially in the early stages of its development.
Objective: This investigation aimed to study the effects of lead (Pb) and cadmium (Cd) on the germination of C. comosum seeds under laboratory conditions, and assess the seeds’ response to these metals.
Methods: The C. comosum seed germination was monitored in Petri dishes containing Pb and Cd at increasing concentrations (25, 50, 75, and 100 µM) for up to 3 weeks. SDS-PAGE was used to examine the protein profile of germinated seeds and the western blot was used to assess the influence of HMs on the activities of the catalase enzyme and the beta subunit of ATP synthase (AtpB).
Results: The germination rate and speed of C. comosum seeds were delayed by increasing concentrations of either Pb or Cd, but this effect was dose-dependent. SDS-PAGE analysis results revealed that exposure to both metals led to altered protein profiles as indicated by the resulting band intensities and disappearance of some proteins compared with the untreated controls. Further, the western blot analysis detected greater activity of catalase enzyme as well as AtpB in the Cd- and Pb-treated seeds.
Conclusion: C. comosum seeds treated with Cd or Pb enhance protein degradation and denaturation beside oxidative stress, leading to reduced seed viability. These results suggest oxidoreduction proteins and those involved in ATP synthesis are enhanced in C. comosum seeds in response to Cd and Pb stressors, which is a probable mechanism by which seeds may tolerate heavy metal stress.
1 Introduction
Environmental contamination from either natural or anthropogenic activity can affect an ecosystem and its components, and ultimately human health. One of the ecosystem components is the soil which is highly considered especially when it becomes contaminated by heavy metals (HMs) (Benavides et al., 2005). Heavy metals are naturally present in various ecosystems in a wide range of concentrations (Nobi et al., 2010). However, the general increase in the concentration of HMs worldwide is arguably the consequence of increasing human activities and industrial wastes (Singh et al., 2015). The pollution of air and soil with HMs would accumulate in plants, either directly or indirectly, and thus, find their way into animals and humans through the food chain (Pál et al., 2006). Accordingly, the real threat of an over-bioaccumulation of HMs is ultimately its negative impact on human health; they are the most dangerous contaminants because of their persistence and long-term non-degradability (Borges et al., 2016).
Plants absorb several metallic elements from the soil; some of these are known to be toxic even at low concentrations while others have unknown biological function (Peralta-Videa et al., 2009). For plants, particular HMs, such as manganese and copper, are essential micronutrients whereas others, such as mercury and cadmium (Cd), are toxic compounds (Polle and Schützendübel, 2003). Many studies have investigated the toxicity of HMs in soil or water, as well as their effects on the local environment and plants. Such as a recent review by Zhu et al. (2021) who reported negative impact of Cd on different physiological behaviors in plants, starting from the germination of seed, growth of seedling, beside the photosynthesis, and antioxidation system. Further, about 10% of the total HM pollution is as consequences to lead (Pb) that affect to the plant metabolic functions, photosynthesis and overall plant growth and development (Collin et al., 2022).
Nevertheless, there are many gaps left to address, especially those concerning pollution reduction in specific HM-contaminated areas. Some recent approaches to protecting the environment by restoring degraded or polluted areas fall under bioremediation technology. Vijayaraghavan and Balasubramanian (2015) defined bioremediation as a “treatment that uses naturally occurring organisms to break down hazardous substances into less toxic or non-toxic substances,” which includes bioaccumulation, biosorption, and phytoremediation techniques. Phytoremediation is a type of “green technology,” that is, an environmental-friendly approach, which is safe and relatively cheap to implement (Gratão et al., 2005; Sharma et al., 2015). In phytoremediation, plants are introduced into the polluted environment to absorb the resident pollutants; these pollutants will eventually accumulate in plant tissues. In the last decade, phytoremediation has been moved from a conceptual to a practical and viable technology in general for environmental cleanups of organic and inorganic contaminants (Devi and Kumar, 2020).
Many researchers have started to study various plants and their specific behavior in contaminated environments with HMs. They found that the absorption and accumulation of HMs in the plant tissues varies depending on the plant species, the particular metal and its concentration, and the plant organ (Iqbal et al., 2012; Amer et al., 2017). A recent study indicated that, the movement of individual HM in water and a porous medium was not temperature dependent (Bai et al., 2021).
Heavy metals toxicity may affect the morphology and molecular structure of the plants used for remediation. Hence, morphological and molecular analyses could enhance our understanding of how plants respond to HM toxicity and likewise reflect the potential of that plant’s use as a phytoremediator. Plant molecular responses to metal pollution have been reported (Alotaibi et al., 2021); for example, protein responses to environmental variables. Some authors used proteomics (e.g., Ahsan et al., 2009; Lequeux et al., 2010; Hego et al., 2016), a powerful tool for describing protein responses in living organisms that reveals the variation in protein profiles at the cellular level of organelles under conditions of various environmental stresses, including HM stress in certain plant species. Plant responses to HMs could be detected through augmented protein levels (Zhao and Chengcai, 2011; Sharmin et al., 2012). Recently, Alotaibi et al. (2021) pointed out that HMs bind to the functional site in some bioactive compounds of the cell, leading to toxicity, as well as cellular imbalance, by altering the DNA, proteins, and some other vital cell components.
Germination plays a major role in the life cycle of plants and its potential is influenced by many factors. These factors are highly correlated with biological traits of a given species. An impact of HMs on seed germination and early seedling development is expected (Li et al., 2005) because the former is very sensitive to the environment, especially unfavorable conditions. These effects include reductions in seed germination and development (Ilić et al., 2015). For example, Bhushan and Gupta (2008) reported that HMs suppressed the total amylolytic activity of amyloidosis in the endosperm or embryonic axis.
Calligonum comosum L’Her is an indigenous shrub species that grows wildly in sandy deserts of the Kingdom of Saudi Arabia. Furthermore, C. comosum is characterized by a fast growth rate in sand dunes, and extensive and deep root system (Ranjbarfordoei et al., 2013), suggesting it may tolerate a wide spectrum of environmental conditions. These traits also make it a potential candidate for use in the treatment of HM-contaminated areas. However, no data are yet available regarding the tolerance of this plant and its response to HMs.
In the present study, to evaluate the ability of C. comosum as a phytoremediator for the removal of HMs, the in vitro germination of its seeds was tested using increasing concentrations of lead (Pb) and Cd. The physiological response of these seeds to HM stress was also evaluated.
2 Materials and methods
All experimental work described here was conducted at the College of Science, Princess Nourah bint Abdulrahman University (located at 24.83680 latitude and 46.72436 longitude), Riyadh, Saudi Arabia. The experiments were done in summer where the mean temperature was 45°C with little precipitation.
2.1 Heavy metal treatments and seed germination
The seeds of C. comosum L’Her (Polygonaceae) used in this study were freshly collected, in May 2019, from the Al-Barrak Reserve in Al-Shehiya, in the Al-Qassim region, Saudi Arabia. The seeds were soaked in sterile distilled water for 24 h to break the dormancy, as a preparatory step for the experiment. The seeds were distributed across 36 plastic Petri dishes (Petri dishes with lid, 90mm Dia x 15mm Deep); each was prepared with a filter paper (Whattmann No. 1) placed at the bottom on which 10 seeds were placed. The dishes were divided into three groups: two treatments and one control. The control group consisted of four dishes, and each treatment group was divided further into four subgroups, each containing four dishes (i.e., replicates). Cadmium sulfate Hydrate (CdSO4,H2O, Sigma-Aldrich) and lead sulfate (PbSO4,Sigma-Aldrich) solutions were prepared at concentrations of 25, 50, 75, and 100 μM in distilled/tap water for either treatment; only distilled/pure tap water was used for the control group. Each HM concentration was used for wetting the filter papers of one subgroup. All the dishes were then incubated at constant temperature (20°C) dark conditions in a germination incubator. Germination was monitored for 3 weeks; a seed was considered to have sprouted if the root was at least 2 mm in length. The germination data were recorded daily and retained until end of the experiment.
The germination rate (G%) was calculated as follows: G% = (number of sprouted seeds/number of initial seeds) × 100 (Onofri et al., 2018).
Clear description to the experimental design is illustrated at Figure 1.
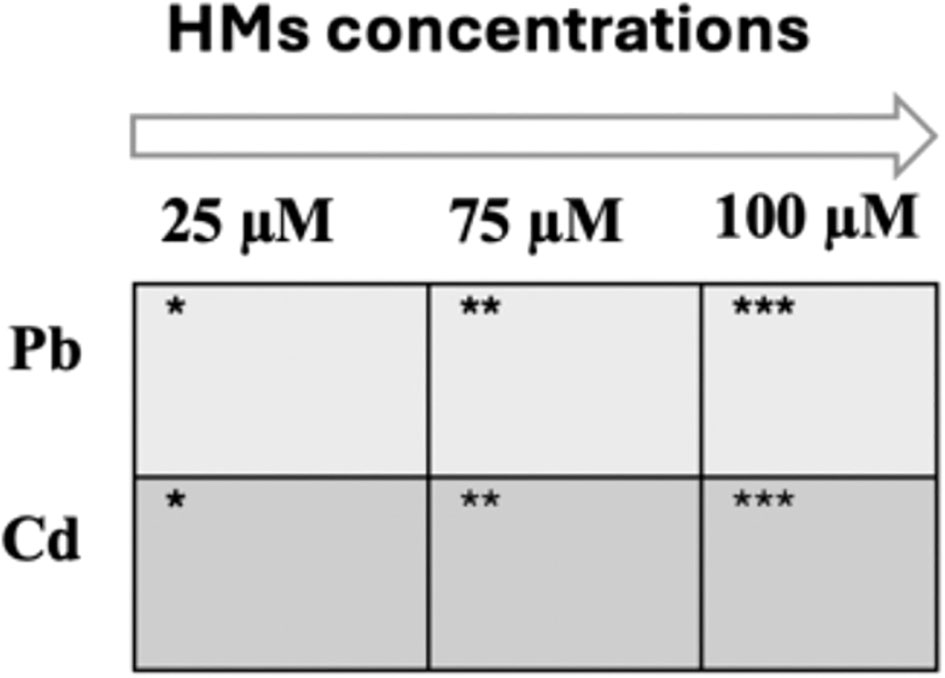
Figure 1 The expected effects of Pb and Cd at three different levels: 25, 75, and 100 µM on seed germination, where *, ** and *** refer to strong, stronger and the strongest effect, respectively.
2.2 Protein profile analysis
2.2.1 Total protein extraction
A volume of 10 mL liquid nitrogen was added to o.8 g seeds in mortar then grinded and lysis buffer (800 μl) was added (0.02 g of CHAPS, 0.6 g of Urea, 0.05% bromophenol blue) and ground thoroughly. Then the lysate was transferred from the mortar to fresh eppendorf tubes. 20 μl of lysis buffer was added to the tube containing the lysate and vortexed to mix the contents uniformly then incubation at -20°C for 1 hour. The proteins at the end of this incubation, get precipitated in the buffered solution. Tubes were taken to -20°C freezer for 30 minutes then centrifuged at 14000 rpm, 4°C and 30 minutes. Supernatants were discarded carefully without disturbing the pellet. Acetone was added for washing then tubes were vortexed and incubate at -20°C for 30 minutes then centrifuged at 14000 rpm, 4°C for 15 min. Supernatant was discarded carefully without disturbing the pellet. Thereafter, 400 μl rehydration buffer was added to the air-dried pellet and vortexed then incubated overnight at 4°C for complete solubilization of proteins in the rehydration buffer. Sample is then ready for SDS PAGE analysis.
2.2.2 Quantification of total protein and SDS-PAGE
Obtained protein extracts were quantified with the 2D Quant Kit (GE Healthcare Life Sciences), by following the manufacturer’s recommendations. Before protein loading onto the gel, 5% β-mercaptoethanol to 2x Laemmli sample buffer was added to each sample, vortexed and spend down then heated on thermomixer at 99 °C for 5 minutes. For protein separation by gel electrophoresis, equal amount of protein (20ul) was loaded into the well of SDS-PAGE, along with molecular weight markers for 5 min at 50 V and increase the voltage to 100-150 V to finish the run in about 45 min. Then gel was fixed in 40% ethanol and 10% acetic acid for 15 minutes then rinsed with 100 mL of water. Stain (QC colloidal coomassie blue) was added to the gel overnight with shaking then the gel was de-stained in 100 mL water for one hour to be ready for gel image.
2.3 Western blot
Approximately, 30 μL of protein from each sample was loaded onto SDS-PAGE at the same above-mentioned conditions. After the run, the proteins were transferred to the membrane by using Trans blot turbo transfer system then blocked in 5% powdered skimmed milk in Tris-buffered saline with 0.1% tween-20 (TBST) at room temperature for one hour. Then, blot was washed two times by washing buffer for 5 minutes then with Tris-buffered saline followed by incubation with the primary antibodies (Anti-ATP synthase beta and anti-CAT) for 1 hour under constant stirring. Conjugation was achieved by incubating the membranes with the secondary antibody (anti-rabbit IgG alkaline phosphatase conjugate) at room temperature for one hour on shaker. The relative accumulation of secondar antibody was visualized using enhanced chemiluminescent detection procedure using charge-coupled device camera.
2.4 Statistical analysis
The numbers of the germinated seeds were analyzed by the MS EXCEL 2019 for Windows for mean and standard deviation as well as the figures. Graphpad Prism Software version 9.1 (San Diego, California, USA) was used for One-Way ANOVA for comparing the germination rate between the control and treated seeds.
3 Results
3.1 Seed morphology and germination rate after treatment with CD and Pb
At the end of the experiment, the morphology of seeds was examined and their germination percentage tallied. The germinated seeds displayed various morphological changes under the HM treatments compared with the control (Figure 2). Germination was evidently delayed in response to HM exposure, and although germination occurred in all treatments, its rate and speed varied according to the type and concentration of the HM tested (Table 1). Generally, the germination rate decreased in dose-dependent style manner, in that seeds treated with the highest HM concentrations had the lowest germination rate.
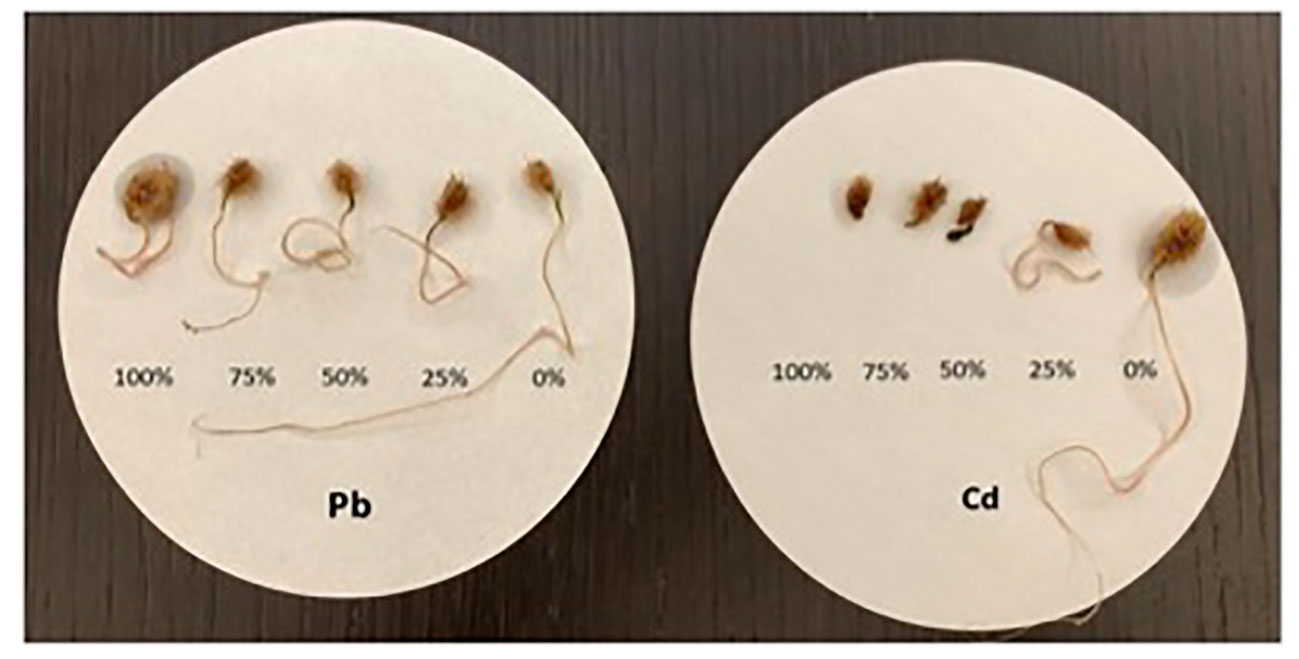
Figure 2 Morphology of germinated C. comosum seeds after treating them with increasing concentrations of cadmium (Cd) and lead (Pb).
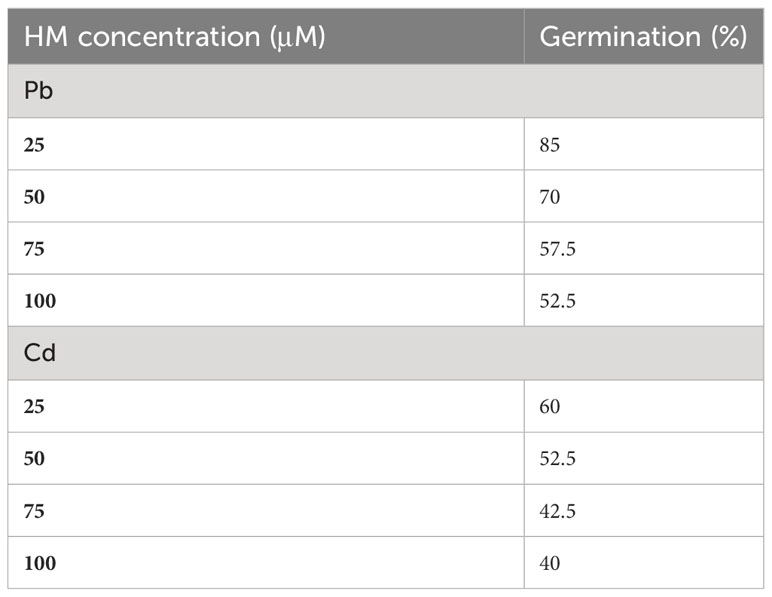
Table 1 Seed germination rate (percentage) of C. comosum after treating them with cadmium (Cd) and lead (Pb) at different concentrations.
3.2 Effects of lead and cadmium treatments on seed germination speed
Pronounced differences in the trends for seed germination rate were found between the control and those seeds soaked in various Pb concentrations (Figure 3). A similar dose-dependent trend was also observed for those soaked in Cd (Figure 4). One way ANOVA was done for comparing the seed germination in treated seeds with the control where Fisher’s LSD identified no significant variations between the control and Pb treated seeds except for seeds treated with 100 µM (p < 0.0051). However, all treatments with Cd showed significant variations in relation to untreated control seeds (Figures 2, 3).
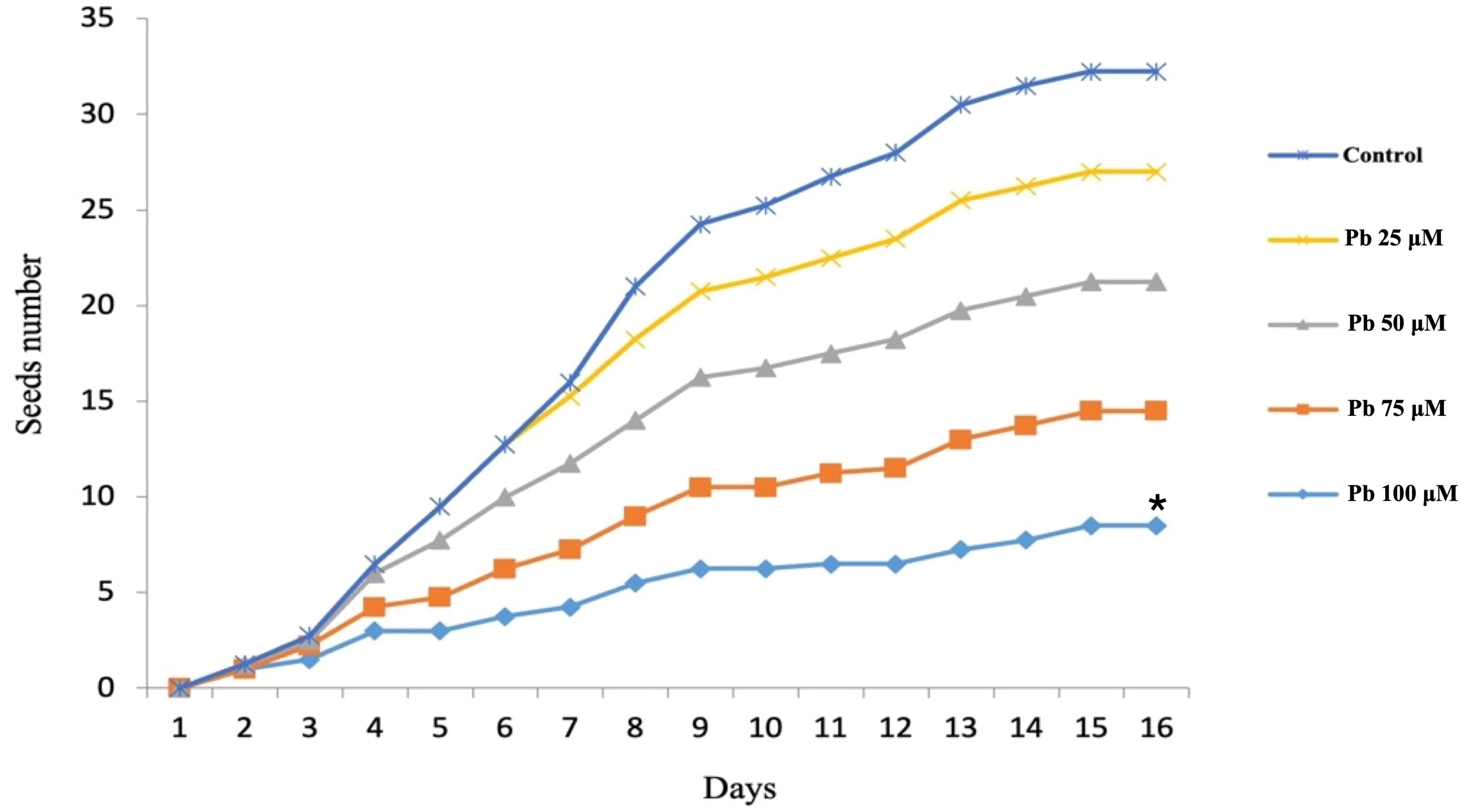
Figure 3 Seed germination rate after treatment with lead at different concentrations. * indicate significant variation in the seed number as consequences to treatment in relation to control.
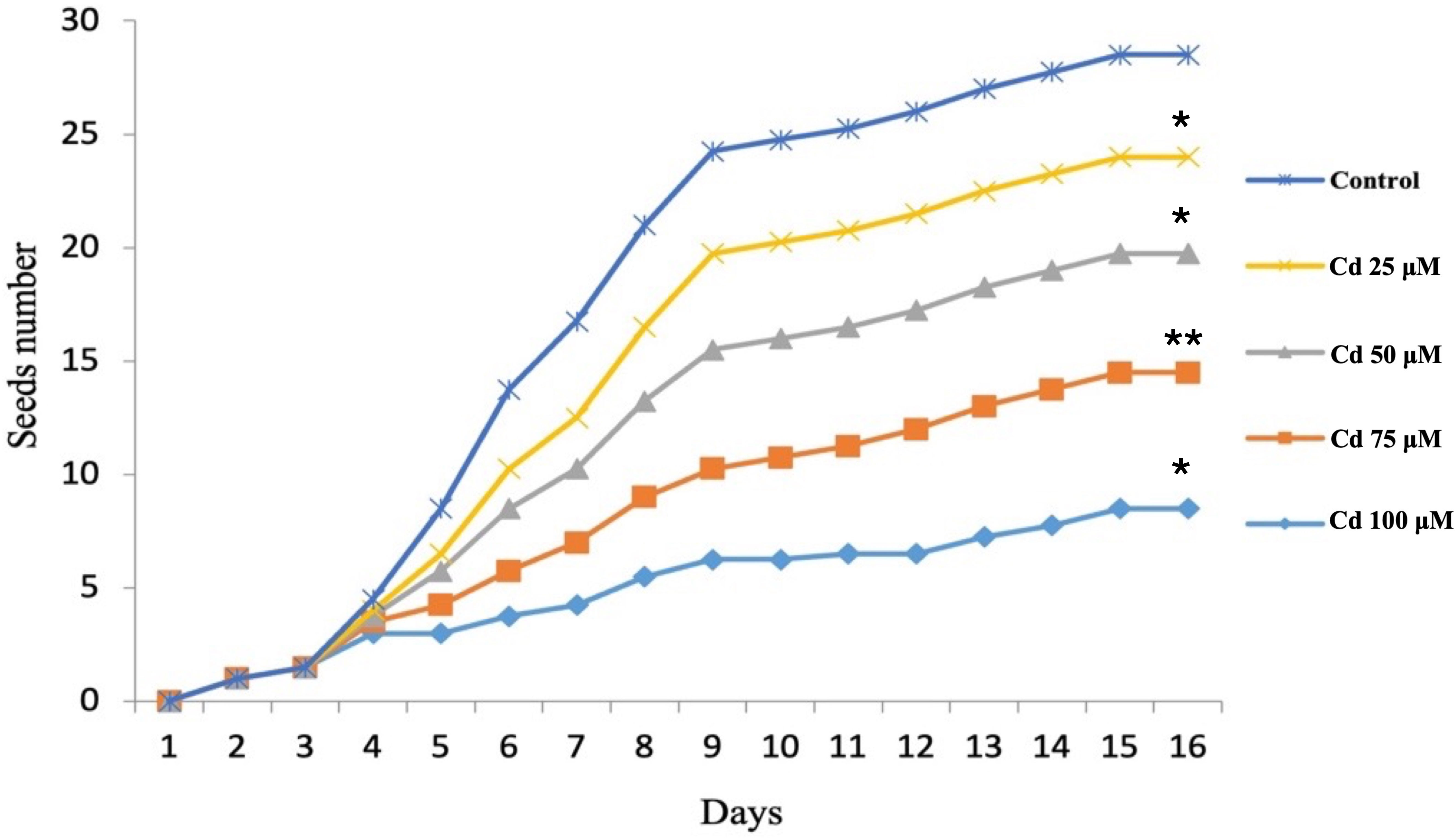
Figure 4 Seed germination rate after treatment with cadmium at different concentrations. *, ** indicate significant variations in the seed number as consequences to treatment in relation to control.
3.3 SDS-PAGE analysis
Protein profiles of C. comosum seeds treated with Pb or Cd were assessed by SDS-PAGE analysis, as presented in Figures 5, 6, respectively. Compared with untreated seeds (control), the protein profile of treated seeds displayed thinner protein bands with a higher molecular weight (MW). Increment in protein MW was noted in dose-dependent manner, in that a higher MW was found for bands from treated seeds with 100 µM of HMs. Although not constantly the same for both metals and concentrations, this was the overall trend that appeared. Higher doses of both Cd and Pb resulted in seeds that contained higher MW proteins vis-à-vis lower doses. Furthermore, treated seeds showed more bands than did the untreated control, where only five bands were noted (Tables 2, 3).
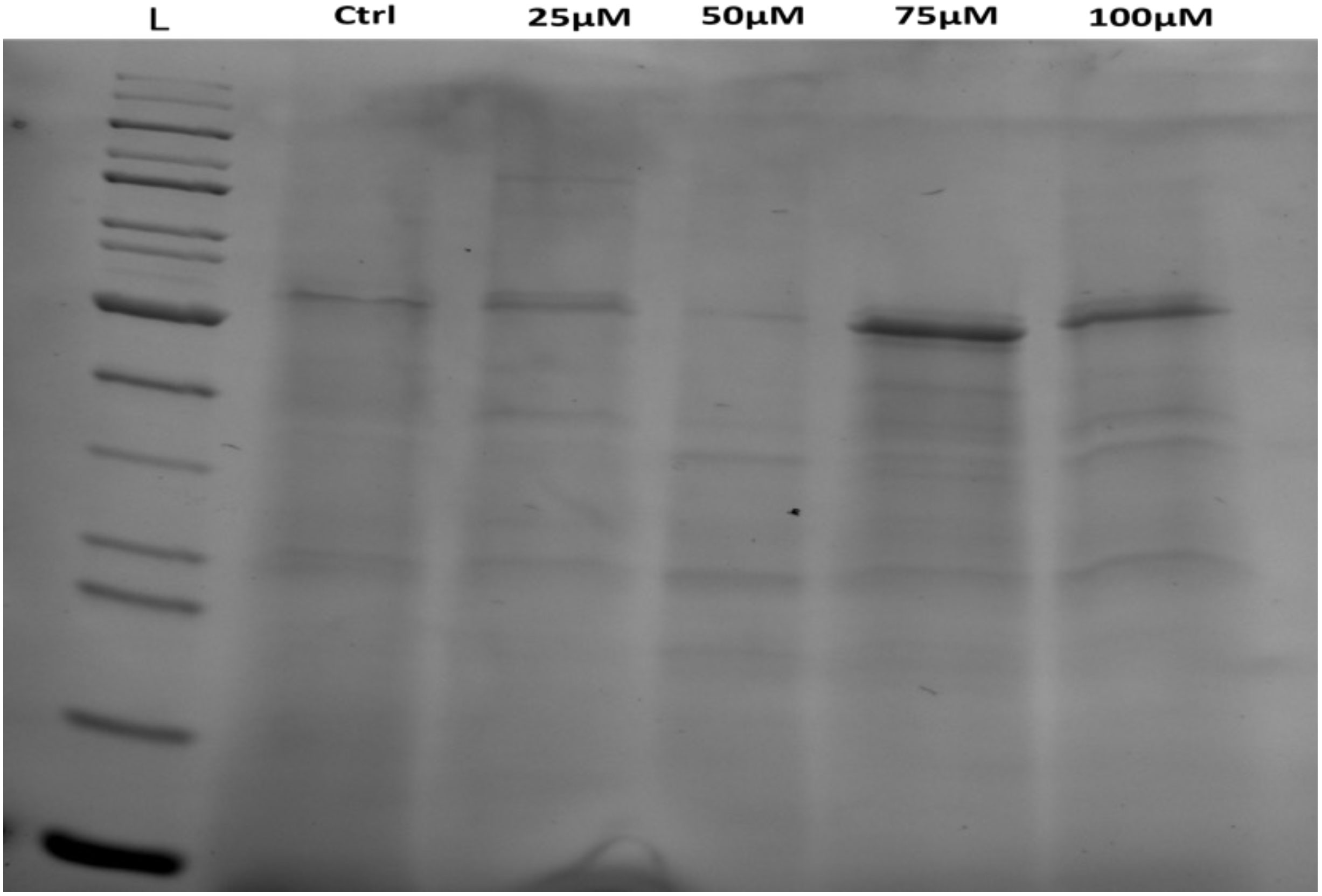
Figure 5 SDS-PAGE analysis of total protein content from C. comosum seeds before and after treating them with different concentrations of Pb. Lane L is the ladder; lane 1, control and lane 2,3,4 and 5 represent the protein profile from seeds treated with 25, 50, 75, and 100 µM of Pb respectively.
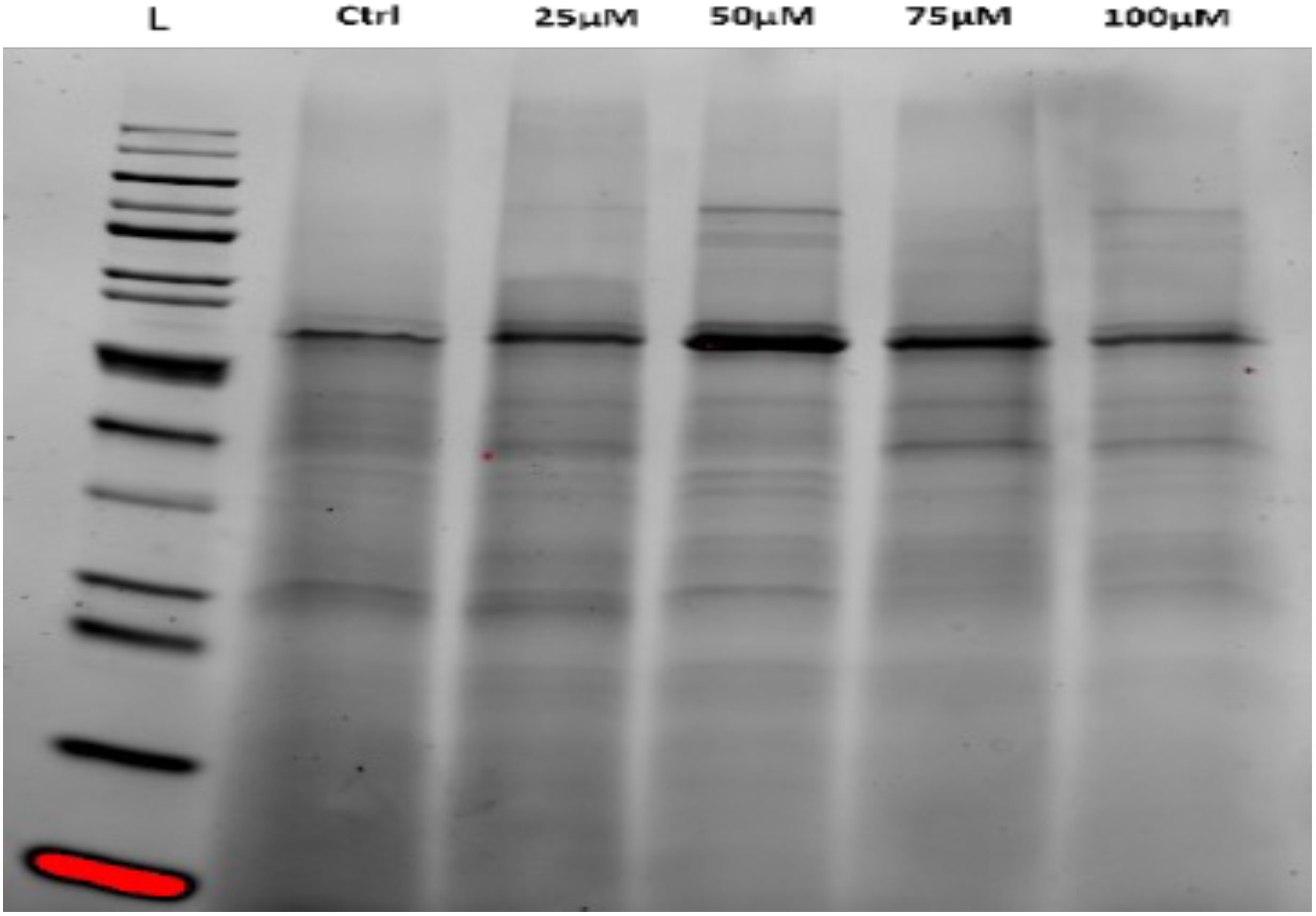
Figure 6 SDS-PAGE analysis of total protein content from C. comosum seeds before and after treating them with different concentrations of Cd. Lane L is the ladder; lane 1, control and lane 2,3,4 and 5 represent the protein profile from seeds treated with 25, 50, 75, and 100 µM of Cd respectively.
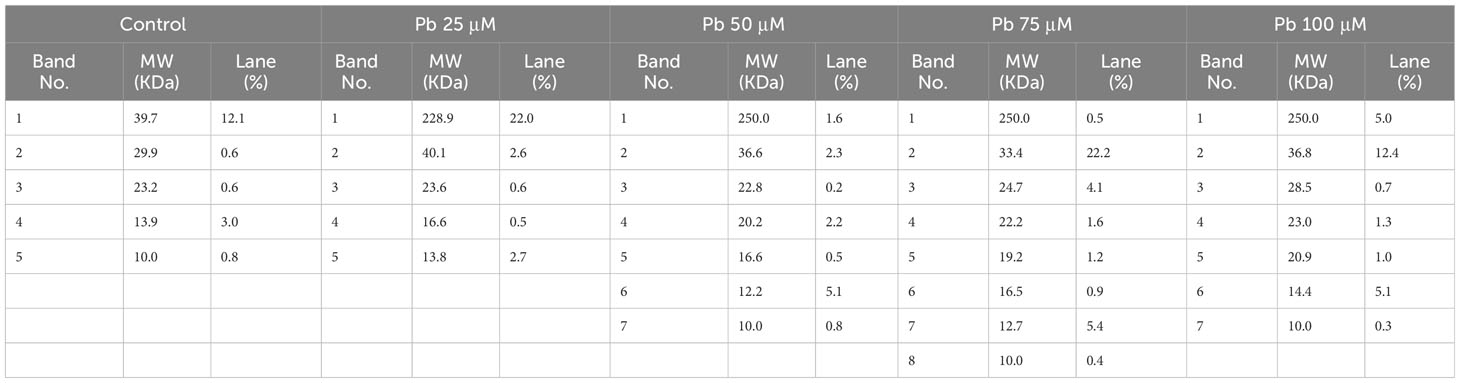
Table 2 Molecular weight, lane, and band number of the proteins extracted from seeds of C. comosum treated with different concentrations of Pb (0, 25, 50, 75, and 100 μM).
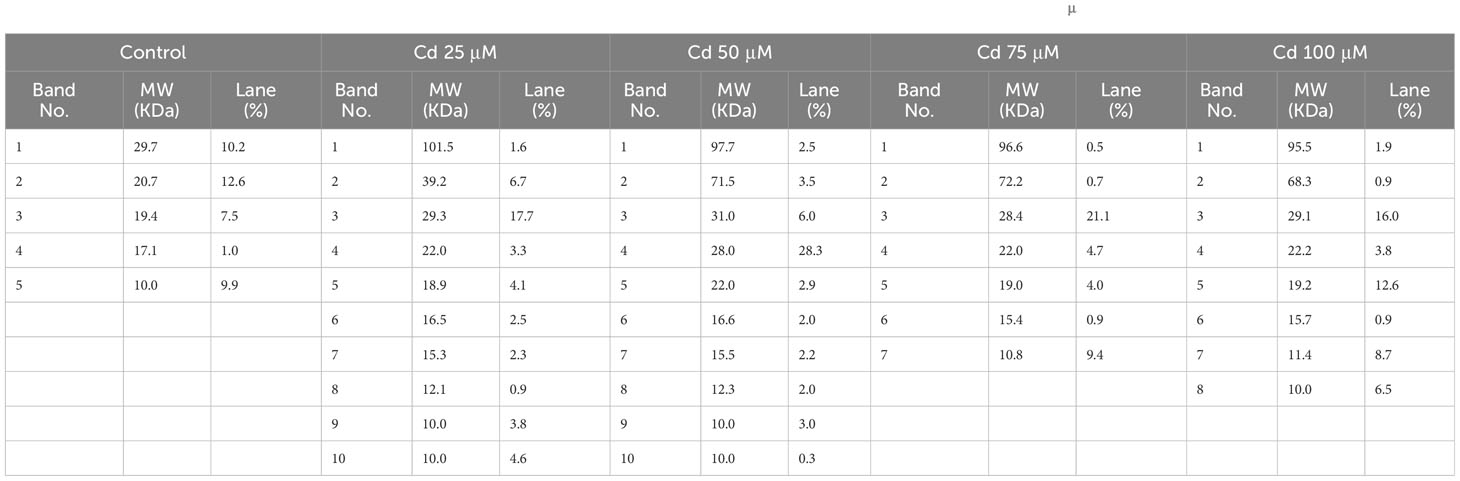
Table 3 Molecular weight, lane, and band number of the protein extracted from seeds treated with different concentrations of Cd (0, 25, 50, 75, and 100 μM).
3.4 Western blot
Accumulation of some proteins in the seeds of C. comosum subjected to different concentrations of Cd and Pb was inferred from their western blot analysis. Clear bands of catalase protein were only discernable in the seeds treated with higher concentrations of Pb, that is, 75 or 100 µM (Figure 7). In general, the catalase enzyme accumulated more in seeds incurring high concentrations of Cd and Pb. Yet the bands were clearer for Pb-treated seeds than Cd-treated ones. Additionally, the catalase enzyme was detected at low concentrations in both treatments as well as in the control.
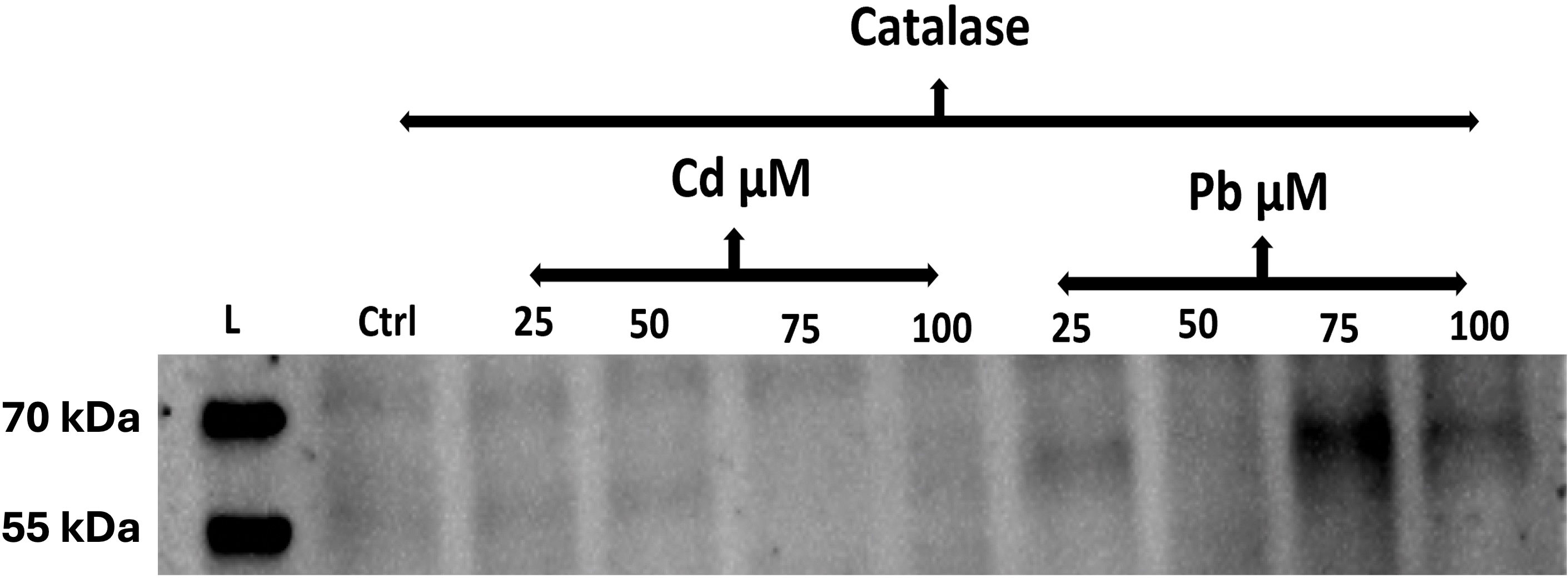
Figure 7 Western blot analysis using anti-CAT antibody of the total protein extracted from the seeds of C. comosum after treatment with Cd and Pb. Ctrl, control. Lanes are the treatment groups with Cd and Pb at 25, 50, 75, and 100 µM for 15 days. kDa, molecular mass.
Bands of higher intensities were recorded for β-ATP synthase subunit proteins from seeds exposed to high concentrations of the Cd and Pb treatments relative to those of the control (Figure 8). Clearer bands were also observed for Pb-treated seeds vis-à-vis the Cd-treated ones.
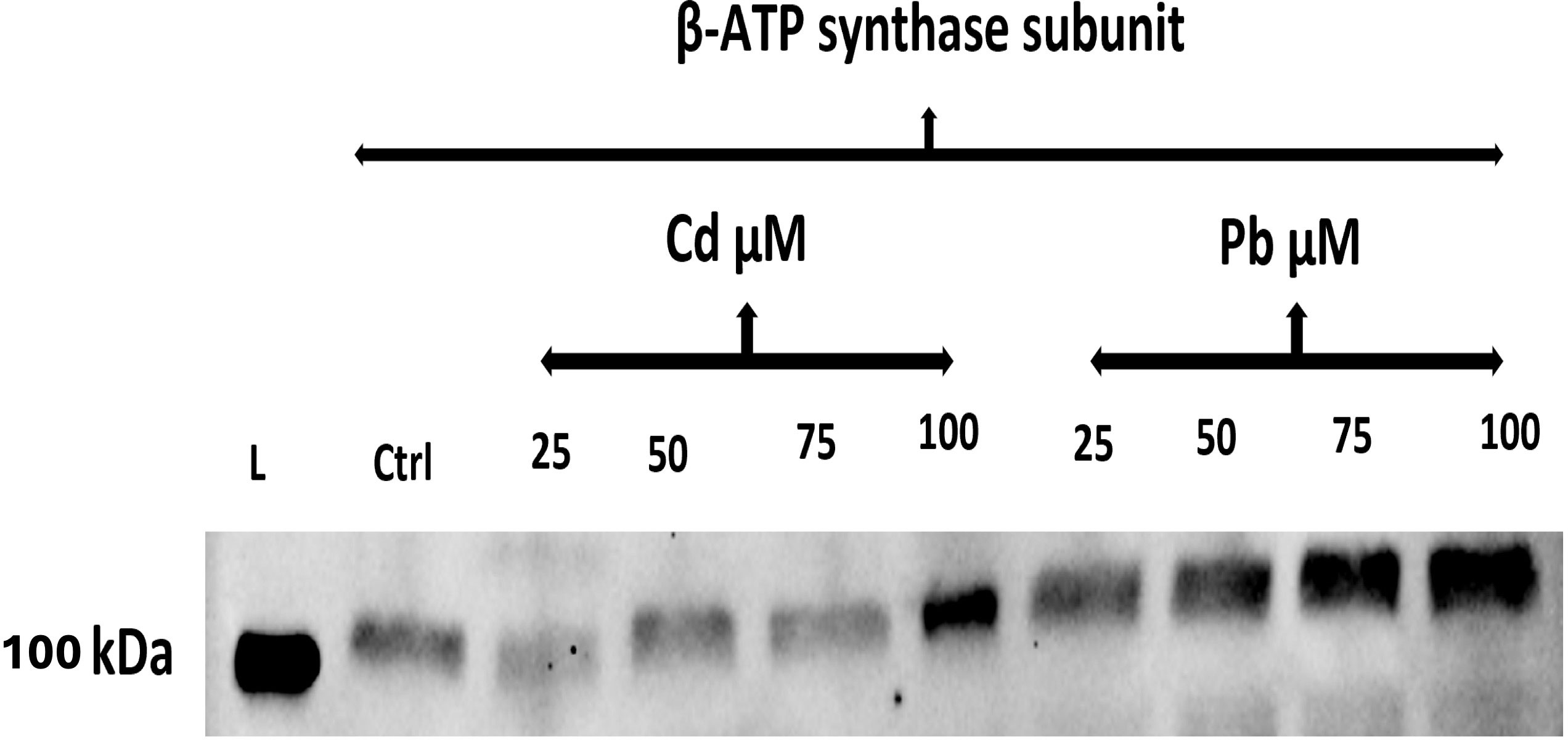
Figure 8 Western blot analysis using the anti-β-ATP synthase subunit antibody of total protein extracted from the seeds of C. comosum after their treatment with Cd or Pb. Ctrl, control. Treatment groups with Cd and Pb at 25, 50, 75, and 100 µM for 15 days. kDa, molecular mass.
4 Discussion
HMs play a significant role in plant growth and development, but when they exceed the prerequisite limit for normal plant growth, this exposure can lead to adverse morphological, metabolic, physiological, and molecular effects (Singhal et al., 2023). In this context, the current study investigated the possible effects of HMs on the germination and physiology of C. comosum seeds.
Seed germination is an important stage in the life history of plants and understanding the factors influencing its tolerance limits are necessary when selecting and using a plant species in phytoremediation application for soils with HM contamination. Here C. comosum was studied because it is expected to tolerate and mitigate soil contamination because of its special characteristics and abundance in sandy deserts, as well areas in which people picnic and camp where pollution due to human activities is highly expected. Some HMs, such as Cd, chromium, and Pb, among others, are toxic even at very low exposure concentrations (Gill, 2014; Pastor-Jáuregui et al., 2020). Several studies indicated that HMs reduce or inhibit the germination of seeds by lowering their water uptake, leading to death by damaging the embryo (El Rasafi et al., 2016) depending on the concentration of the HM (Kranner and Colville, 2011; Saderi and Zarinkamar, 2012). In the present study, some C. comosum seeds were capable of germinating even when exposed to high concentrations of Cd or Pb, and the results showed clear differences in the shape and length of the rootstock according to the type of HM applied. According to Siddiqui et al. (2014), the inhibitory effect of HMs is more pronounced on seedling growth than on seed germination.
Pb is not an essential element for plant growth (Peralta-Videa et al., 2009). Although some species, such as Allium cepa, H. vulgare and Zea mays, can tolerate Pb via complexation and inactivation, others, such as Brassica napus, Phaseolus vulgaris, and three sorghum cultivars incur toxicity because Pb impairs certain metabolic pathways (Wierzbicka, 1999; Osman and Fadhlallah, 2023).
In consequence, exposure to excessive Pb may include inhibition of seed germination, plant growth, and chlorophyll synthesis (Begonia et al., 2004; Zulfiqar et al., 2019). Results of the current study showed divergence in the seed germination between the control and seeds soaked in a low Pb concentration. Negative effects of Pb on seed germination and seedling growth, as well as hinder later growth of some tree species have been reported before (Shafiq and Iqbal, 2005; Baruah et al., 2019; Diaconu et al., 2020; Yáñez-Espinosa et al., 2020).
Cadmium (Cd) is known as one of the most highly toxic metals affecting many plant species (Li et al., 2005; Di Salvatore et al., 2008). Some studies have demonstrated an inhibitory effect of Cd on seed germination and plant growth, in addition to greater mitotic abnormalities. Reductions in seed germination, growth, or enzymatic activity have been correlated with mounting Cd concentrations; however, an increase in total chlorophyll content has been reported in tomato plants treated with Cd (Baruah et al., 2019).
Reduced seed germination and impaired plant development could be due to the fact that HMs may prevent water absorption by seeds, leading to their suppressed germination or facilitating the metal’s entry, resulting in embryo toxicity (Ilić et al., 2015), by repressing the total amylolytic activity of amyloidosis (Bhushan and Gupta, 2008).
Germination speed can serve as a reliable indicator to evaluate seedling vigor (Yáñez-Espinosa et al., 2020). Given that germination is a potentially vulnerable stage in the life cycle of plants, the tissues that cover the seed embryo have the ability to protect it, to some extent, against toxicity from HMs. Such protection could be secured by selective absorption; however, once the seed coat begins to break, growth is reduced according to the concentration of the HM (Saderi and Zarinkamar, 2012).
The seeds in this study were adversely affected by Cd as well as Pb because neither element is essential for plant growth and development. Accordingly, specific defensive proteins might accumulate in seeds in response to high concentrations of HMs. Many bands detected in treated C. comosum seeds could be related the formation of novel proteins in them as a defense mechanism against prominent HM stress. Ten bands were detected in seeds treated with 25 or 50 µM of Pb; however, this number decreased after exposure to a higher concentration (100 µM). This result suggests the seeds were trying to resist Pb-induced stress, but a higher Pb concentration degraded the defensive proteins and the seeds could not tolerate that. However such findings were not observed for Cd treated seeds.
The accumulation of particular proteins in C. comosum seeds in response to different concentrations of Cd and Pb was indicated by the western blot analysis. The catalase enzyme accumulated more in the seeds exposed to high concentrations of Cd and Pb. Catalase is an important enzyme in the metabolism of reactive oxygen species, with significant potential to control their negative impact on cell functioning, along with its ability to reduce H2O2 toxicity in cells (Apel and Hirt, 2004; Anjum et al., 2016). High catalase accumulation can indicate a high oxidative stress condition in the seeds caused by the presence of Cd and Pb, and the associated accumulation of ROS not eliminate by the seeds’ anti-oxidative systems. Consistent with that, the catalase enzyme had low activity at low-concentration treatments of HMs as well as in the control group. This suggests low-stress conditions for seeds are imposed by those concentrations. Bolstered levels of the antioxidant enzyme (catalase) in treated seeds could constitute a cellular protection mechanism against ROS produced in response to Cd stress (Hossain et al., 2012).
Furthermore, the ATP synthase subunits are principal enzymes in the photosynthesis and biosynthetic pathway of ATP. Here, the antibody against the β-ATP synthase subunit was used to identify and gauge its presence in proteins of seeds treated with HMs. ATP synthase functions in the aerobic pathway to strengthen the proton gradient in order to generate ATP in the electron transport chain. High production of this protein as indicated by high band intensities indicates a high level of ATP synthase unit enzyme in seeds treated with high concentrations of Pb or Cd. This result suggests ATP increases significantly in stressed plants, and that seeds facing high-stress conditions may require more energy to survive their exposure to Cd- and Pb-induced stresses. A similar observation was reported for plants treated with Cd or Cu for 6 days, in which an increased PM H+-ATPase activity was recorded (Janicka-Russak et al., 2012). Therefore, it could be clearly observed that AtpB activity increased in a dose-dependent manner, which implies the C. comosum seeds had the ability in reducing the inhibitory action of HMs. Another similar finding was that of Oliveira et al. (2020), who concluded that ATPases and catalase were enhanced in CCN 51 genotype cacao plants under Cd stress.
5 Conclusions
Based on the present findings, it is evident that HMs affect germination of C. comosum seeds with variation in sensitivity to the tested metal and its concentration. Low toxicity was demonstrated for Pb compared to Cd in the seed germination process. Furthermore, the accumulation of some proteins, as indicated by high band intensities in response to higher HM concentrations, as well as accumulation of catalase and the β-ATP synthase subunit, suggests a coordinated protein response of C. comosum seeds to stress conditions imposed by HMs. Development of C. comosum seeds in the presence of HM may suggest their ability as potential phytoremediators however, it is important to understand the HM–tolerance mechanisms in a given plant species across all of its developmental stages.
Data availability statement
The raw data supporting the conclusions of this article will be made available by the authors, without undue reservation.
Author contributions
MA: Conceptualization, Data curation, Investigation, Methodology, Project administration, Writing – original draft.
Funding
The author(s) declare financial support was received for the research, authorship, and/or publication of this article. The authors are grateful to Princess Nourah bint Abdulrahman University for supporting this research through the Sabbatical Leave Program.
Acknowledgments
Author acknowledges the significant contributions from Dr. Afrah E. Mohammed, Department of Biology/College of Science/Princess Nourah bint Abdulrahman university.
Conflict of interest
The authors declares that the research was conducted in the absence of any commercial or financial relationships that could be construed as a potential conflict of interest.
Publisher’s note
All claims expressed in this article are solely those of the authors and do not necessarily represent those of their affiliated organizations, or those of the publisher, the editors and the reviewers. Any product that may be evaluated in this article, or claim that may be made by its manufacturer, is not guaranteed or endorsed by the publisher.
Abbreviations
Pb, Lead; Cd, Cadmium; HMs, Heavy metals; AtpB, ATP synthase; ANOVA, Analysis of Variance; SDS-PAGE, Sodium dodecyl sulfate–polyacrylamide gel electrophoresis; Analysis of Variance (ANOVA), Explanation, Formula, and Applications; MW, Molecular weight; IgG, Immunoglobulin G; LSD, Least significant differences; ROS, Reactive oxygen species; ATP, Adenosine triphosphate; H2O2, Hydrogen peroxid.
References
Ahsan N., Renaut J., Komatsu S. (2009). Recent developments in the application of proteomics to the analysis of plant responses to heavy metals. Proteomics 9, 2602–2621. doi: 10.1002/pmic.200800935
Alotaibi M. O., Mohammed A. E., Almutairi T. A., Shinwari Z., Alaiya A., Elobeid M. M. (2021). Physio-morphology and proteomic attitude of Ziziphus spina-christi in copper-contaminated sites in Saudi Arabia: Is it a candidate bioremediator? Curr. Proteomics 18, 279–292. doi: 10.2174/1570164617999200612114626
Amer M., Tyler A., Fouda T., Hunter P., Elmetwalli A., Wilson C., et al. (2017). Spectral characteristics for estimation heavy metals accumulation in wheat plants and grain. Sci. Pap. S. Manage. Econ. Eng. Agric. Rural Dev. 17, 47–56. doi: 10.21608/jpd.2019.41905
Anjum N. A., Sharma P., Gill S. S., Hasanuzzaman M., Khan E. A., Kachhap K., et al. (2016). Catalase and ascorbate peroxidase representative H2O2-detoxifying heme enzymes in plants. Environ. Sci. pollut. Res. Int. 23, 19002–19029. doi: 10.1007/s11356-016-7309-6
Apel K., Hirt H. (2004). Reactive oxygen species: Metabolism, oxidative stress, and signal transduction. Annu. Rev. Plant Biol. 55, 373–399. doi: 10.1146/annurev.arplant.55.031903.141701
Bai B., Nie Q., Zhang Y., Wang X., Hu W. (2021). Cotransport of heavy metals and SiO2 particles at different temperatures by seepage. J. Hydrol. 597, 125771. doi: 10.1016/j.jhydrol.2020.125771
Baruah N., Mondal S. C., Farooq M., Gogoi N. (2019). Influence of heavy metals on seed germination and seedling growth of wheat, pea, and tomato. Water Air. Soil pollut. 230, 273. doi: 10.1007/s11270-019-4329-0
Begonia M. T., Begonia G. B., Miller G. S., Gilliard D. (2004). Effects of chelate application time on the phytoextraction of lead-contaminated soils. Bull. Environ. Contam. Toxicol. 73, 1033–1040. doi: 10.1007/s00128-004-0529-3
Benavides M. P., Gallego S. M., Tomaro M. L. (2005). Cadmium toxicity in plants. Braz. J. Plant Physiol. 17, 21–34. doi: 10.1590/S1677-04202005000100003
Bhushan B., Gupta K. (2008). Effect of lead on carbohydrate mobilization in oat seeds during germination. J. Appl. Sci. Environ. Manage. 12, 29–33. doi: 10.4314/jasem.v12i2.55523
Borges K. S. C., D’Avila R. C., Campos M. L., Coelho C. M. M., Miquelluti D. J., Galvan, Nd. S. (2016). Germination and initial development of Brachiaria brizantha and Brachiaria decumbens on exposure to cadmium, lead and copper. J. Seed. Sci. 38, 335–343. doi: 10.1590/2317-1545v38n4165265
Collin S., Baskar A., Geevarghese D. M., Ali M. N. V. S., Bahubali P., Choudhary R., et al. (2022). Bioaccumulation of lead (Pb) and its effects in plants: A review. J. Hazardous. Mater. Lett. 3, 100064. doi: 10.1016/j.hazl.2022.100064
Devi P., Kumar P. (2020). Concept and application of phytoremediation in the fight of heavy metal toxicity. J. Pharm. Sci. Res. 12, 795–804.
Diaconu M., Pavel L. V., Hlihor R. M., Rosca M., Fertu D. I., Lenz M., et al. (2020). Characterization of heavy metal toxicity in some plants and microorganisms—A preliminary approach for environmental bioremediation. N. Biotechnol. 56, 130–139. doi: 10.1016/j.nbt.2020.01.003
Di Salvatore M., Carafa A. M., Carratù G. (2008). Assessment of heavy metals phytotoxicity using seed germination and root elongation tests: A comparison of two growth substrates. Chemosphere 73, 1461–1464. doi: 10.1016/j.chemosphere.2008.07.061
El Rasafi T., Nouri M., Bouda S., Haddioui A. (2016). The effect of Cd, Zn and Fe on seed germination and early seedling growth of Wheat and Bean. Ekológia 35, 213–223. doi: 10.1515/eko-2016-0017
Gratão P. L., Polle A., Lea P. J., Azevedo R. A. (2005). Making the life of heavy metal-stressed plants a little easier. Funct. Plant Biol. 32, 481–494. doi: 10.1071/FP05016
Hego E., Vilain S., Barré A., Claverol S., Dupuy J. W., Lalanne C., et al. (2016). Copper stress-induced changes in leaf soluble proteome of Cu-sensitive and tolerant Agrostis capillaris L. populations. Proteomics 16, 1386–1397. doi: 10.1002/pmic.201500083
Hossain Z., Hajika M., Komatsu S. (2012). Comparative proteome analysis of high and low cadmium accumulating soybeans under cadmium stress. Amino Acids 43, 2393–2416. doi: 10.1007/s00726-012-1319-6
Ilić S., Mirecki N., Trajković R., Kapoulas N., Milenković L., Šunić L. (2015). Effect of Pb on germination of different seed and his translocation in Bean seed tissues during sprouting. Fresenius. Environ. Bull. 24, 2a.
Iqbal M., Bakht J., Shafi M., Ullah R. (2012). Effect of heavy metal and EDTA application on heavy metal uptake and gene expression in different Brassica species. Afr. J. Biotechnol. 11, 7649–7658. doi: 10.5897/AJB11.3223
Janicka-Russak M., Kabała K., Burzyński M. (2012). Different effect of cadmium and copper on H+-ATPase activity in plasma membrane vesicles from Cucumis sativus roots. J. Exp. Bot. 63, 4133–4142. doi: 10.1093/jxb/ers097
Kranner I., Colville L. (2011). Metals and seeds: Biochemical and molecular implications and their significance for seed germination. Environ. Exp. Bot. 72, 93–105. doi: 10.1016/j.envexpbot.2010.05.005
Lequeux H., Hermans C., Lutts S., Verbruggen N. (2010). Response to copper excess in Arabidopsis thaliana: Impact on the root system architecture, hormone distribution, lignin accumulation and mineral profile. Plant Physiol. Biochem. 48, 673–682. doi: 10.1016/j.plaphy.2010.05.005
Li W., Khan M. A., Yamaguchi S., Kamiya Y. (2005). Effects of heavy metals on seed germination and early seedling growth of Arabidopsis thaliana. Plant Growth Regul. 46, 45–50. doi: 10.1007/s10725-005-6324-2
Nobi E. P., Dilipan E., Thangaradjou T., Sivakumar K., Kannan L. (2010). Geochemical and geo-statistical assessment of heavy metal concentration in the sediments of different coastal ecosystems of Andaman Islands, India. Estuar. Coast. Shelf. Sci. 87, 253–264. doi: 10.1016/j.ecss.2009.12.019
Oliveira B. R. M., de Almeida A. F., Pirovani C. P., Barroso J. P., Neto C. H. D. C., Santos N. A., et al. (2020). Mitigation of Cd toxicity by Mn in young plants of cacao, evaluated by the proteomic profiles of leaves and roots. Ecotoxicology 29, 340–358. doi: 10.1007/s10646-020-02178-4
Onofri A., Benincasa P., Mesgaran M. B., Ritz C. (2018). Hydrothermal-time-to-event models for seed germination. Eur. J. Agron. 101, 129–139. doi: 10.1016/j.eja.2018.08.011
Osman H. E., Fadhlallah R. S. (2023). Impact of lead on seed germination, seedling growth, chemical composition, and forage quality of different varieties of Sorghum. J. Umm. Al-Qura. Univ. Appl. Sci. 9 (1), 77–86. doi: 10.1007/s43994-022-00022-5
Pál M., Horváth E., Janda T., Páldi E., Szalai G. (2006). Physiological changes and defense mechanisms induced by cadmium stress in maize. J. Plant Nutr. Soil Sci. 169, 239–246. doi: 10.1002/jpln.200520573
Pastor-Jáuregui R., Paniagua-López M., Martínez-Garzón J., Martín-Peinado F., Sierra-Aragón M. (2020). Evolution of the residual pollution in soils after bioremediation treatments. Appl. Sci. 10, 1006. doi: 10.3390/app10031006
Peralta-Videa J. R., Lopez M. L., Narayan M., Saupe G., Gardea-Torresdey J. (2009). The biochemistry of environmental heavy metal uptake by plants: Implications for the food chain. Int. J. Biochem. Cell Biol. 41, 1665–1677. doi: 10.1016/j.biocel.2009.03.005
Polle A., Schützendübel A. (2003). “Heavy metal signalling in plants: Linking cellular and organismic responses. Topics in current genetics,” in Plant stress responses, vol. 4) . Eds. Hirt H., Shinozaki K. (Berlin, Heidelberg: Springer-Verlag), 1–29. doi: 10.1007/978-3-540-39402-0_8
Ranjbarfordoei A., Vandamme P., Samson R. (2013). Some ecophysiological characteristics of artà (Calligonum comosum Ľ Hérit) in response to drought stress. For. Sci. Pract. 15, 114–120. doi: 10.1007/s11632-013-0208-8
Saderi S. Z., Zarinkamar F. (2012). The effect of different Pb and Cd concentrations on seed germination and seedling growth of Matricaria Chamomilla. Adv. Environ. Biol. 6, 1940–1943.
Shafiq M., Iqbal M. Z. (2005). The toxicity effects of heavy metals on germination and seedling growth of cassia siamea Lamk. J. New Seeds. 7, 95–105. doi: 10.1300/J153v07n04_07
Sharma S., Singh B., Manchanda V. K. (2015). Phytoremediation: Role of terrestrial plants and aquatic macrophytes in the remediation of radionuclides and heavy metal contaminated soil and water. Environ. Sci. pollut. Res. Int. 22, 946–962. doi: 10.1007/s11356-014-3635-8
Sharmin S. A., Alam I., Kim K. H., Kim Y. G., Kim P. J., Bahk J. D., et al. (2012). Chromium-induced physiological and proteomic alterations in roots of Miscanthus sinensis. Plant Sci. 187, 113–126. doi: 10.1016/j.plantsci.2012.02.002
Siddiqui M. M., Abbasi B. H., Ahmad N., Ali M., Mahmood T. (2014). Toxic effects of heavy metals (Cd, Cr and Pb) on seed germination and growth and DPPH-scavenging activity in Brassica rapa var. turnip. Toxicol. Ind. Health 30, 238–249. doi: 10.1177/0748233712452605
Singh S., Parihar P., Singh R., Singh V. P., Prasad S. M. (2015). Heavy metal tolerance in plants: Role of transcriptomics, proteomics, metabolomics, and ionomics. Front. Plant Sci. 6. doi: 10.3389/fpls.2015.01143
Singhal R. K., Kumar M., Bose B., Mondal S., Srivastava S., Dhankher O. P., et al. (2023). Heavy metal (loid)s phytotoxicity in crops and its mitigation through seed priming technology. Int. J. Phytoremediation. 25, 187–206. doi: 10.1080/15226514.2022.2068502
Vijayaraghavan K., Balasubramanian R. (2015). Is biosorption suitable for decontamination of metal-bearing wastewaters? A critical review on the state-of-the-art of biosorption processes and future directions. J. Environ. Manage. 160, 283–296. doi: 10.1016/j.jenvman.2015.06.030
Wierzbicka M. (1999). Comparison of lead tolerance in Allium cepa with other plant species. Environ. pollut. 104, 41–52. doi: 10.1016/S0269-7491(98)00156-0
Yáñez-Espinosa L., Briones-Gallardo R., Flores J., Castill E. (2020). Effect of heavy metals on seed germination and seedling development of Nama aff. Stenophylla collected on the slope of a mine tailing dump. Int. J. Phytoremediation. 22, 1448–1461. doi: 10.1080/15226514.2020.1781782
Zhao Y., Chengcai C. (2011). Towards understanding plant response to heavy metal stress, abiotic stress in plants - mechanisms and adaptations. InTech, 59–78.
Zhu T., Li L., Duan Q., Liu X., Chen M. (2021). Progress in our understanding of plant responses to the stress of heavy metal cadmium. Plant Signal Behav. 16 (1), 1836884. doi: 10.1080/15592324.2020.1836884
Keywords: seed germination, lead, cadmium, protein, phytoremediation, Saudi Arabia
Citation: Alotaibi MO (2024) Effect of cadmium and lead on the morphology and protein profile of Calligonum comosum seeds. Front. Ecol. Evol. 11:1308943. doi: 10.3389/fevo.2023.1308943
Received: 07 October 2023; Accepted: 08 December 2023;
Published: 11 January 2024.
Edited by:
Peijian Shi, Nanjing Forestry University, ChinaReviewed by:
Abolghassem Emamverdian, Nanjing Forestry University, ChinaJayani J. Wewalwela, University of Colombo, Sri Lanka
Copyright © 2024 Alotaibi. This is an open-access article distributed under the terms of the Creative Commons Attribution License (CC BY). The use, distribution or reproduction in other forums is permitted, provided the original author(s) and the copyright owner(s) are credited and that the original publication in this journal is cited, in accordance with accepted academic practice. No use, distribution or reproduction is permitted which does not comply with these terms.
*Correspondence: Modhi O. Alotaibi, mouotaebe@pnu.edu.sa