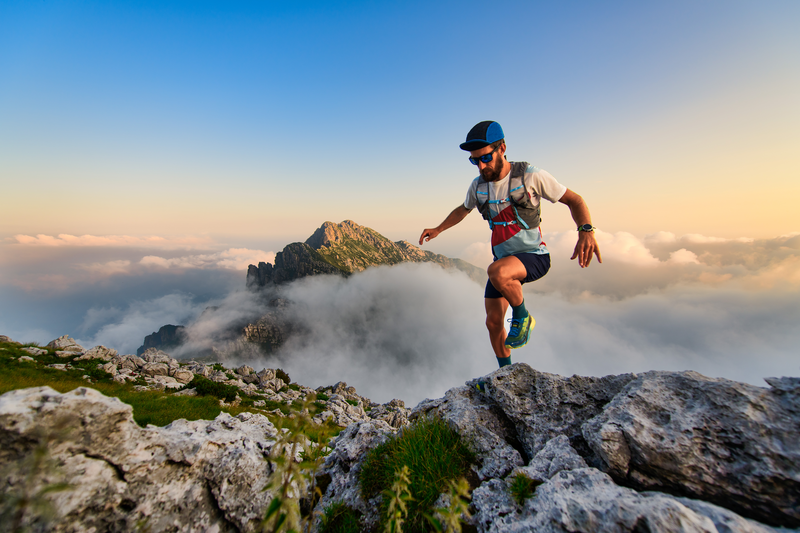
94% of researchers rate our articles as excellent or good
Learn more about the work of our research integrity team to safeguard the quality of each article we publish.
Find out more
ORIGINAL RESEARCH article
Front. Ecol. Evol. , 27 October 2023
Sec. Environmental Informatics and Remote Sensing
Volume 11 - 2023 | https://doi.org/10.3389/fevo.2023.1296111
This article is part of the Research Topic Monitoring, Early Warning and Mitigation of Natural and Engineered Slopes, volume III View all 17 articles
Introduction: As a vital component within glacier systems, the occurrences of glacial lake disasters in high mountain regions have progressively emerged as one of the most destructive natural calamities amid the backdrop of global warming. The swift advancement of glacial lake identification techniques offers a pivotal perspective for forecasting and mitigating the perils of glacial lake outburst disasters.
Methods: To evaluate the thematic evolution, research focal points, and forthcoming directions within the glacier identification domain, a comprehensive bibliometric analysis was conducted on glacial lake identification-related literature from 1991 to 2023 in the Web of Science Core Collection database.
Results: 1) The United States, the United Kingdom, and China stand as principal nations propelling the field's advancement. The Chinese Academy of Sciences demonstrates the highest activity in terms of article publications and international collaborations. 2) Climate change, compilation of glacial lake inventories, methodologies for risk assessment, glacial lake outburst floods, comprehensive disaster management strategies, and hydrodynamic models constitute the domain's research hotspots. It is a typical multidisciplinary field. 3) Persistently high-impact topics over an extended period include “hazard”, “Late Pleistocene”, “environmental change”, “ice sheet”, and “lake sediments”. Keywords indicating the present cutting-edge research encompass “inventory”, “glacial lake outburst flood”, “risk”, “dynamics”, “Tibetan Plateau”, “evolution”, and “high mountain Asia”.
Discussion: This paper delves into the current status and pivotal concerns of glacial lake identification techniques, methodologies, and the scale of identification research themes. Further Research should concentrate on avenues like “recognition methods grounded in machine learning and deep learning”, “multisource data fusion datasets”, “novel algorithms and technologies adaptable to scale transformation and data expansion”, as well as “enhancing spatiotemporal data resolution”. This will ultimately enable precise, prolonged, and multiscalar identification of glacial lakes. his study provides valuable guidance and reference for future research in the field of glacial lake identification.
As glaciers melt and retreat, the resulting depressed terrains gradually fill with meltwater, giving rise to glacial lakes. These lakes, considered one of the most sensitive indicators of global climate change, are widely distributed in regions featuring extensive glaciers and active glacial processes, such as the Himalayas, the Andes, and the Alps (Motschmann et al., 2020; Thompson et al., 2020). Similar to the predisposing environments and consequences of debris flow disasters, these areas often possess favorable conditions for glacial lake formation (Yang et al., 2023a), including low latitudes, high altitudes, steep slopes, and rugged terrain (Yang et al., 2023b; Allen et al., 2019). In recent years, with the intensification of global warming and glacier melting, glacial lakes have become increasingly prevalent features within natural landscapes.
Glacial lakes primarily derive their water from the meltwater of surrounding glaciers, often characterized by lower temperatures. Many glacial lakes form at the moraine margins comprised of ice-deposited sediments and fragments, potentially containing suspended sediments that contribute to the unique natural landscapes of these lakes (Frydrych and Rdzany, 2022). When the structural integrity of an ice dam weakens or is compromised, ice dam failure can lead to landslides, debris flows, or even glacial lake outburst floods (GLOFs) (Pei et al., 2023). Over time, the size, shape, and water levels of glacial lakes may fluctuate due to local geological conditions, climate factors, and dynamic changes within the glacier system (Begam and Sen, 2019). Glacial lakes pose significant potential hazards, especially when they become unstable and lead to downstream flooding. The rapid release of a substantial volume of lake water during such events can have catastrophic consequences for nearby infrastructure and human safety (Cook et al., 2018; Emmer et al., 2020). GLOFs typically involve a complex sequence of processes, including initial triggering mechanisms, influences and feedback from surrounding terrain and topography, variations in lake surface or snow cover, dam overtopping, erosion and sediment deposition along the flood path, and the spreading of floods or debris flows in the affected area (Sattar et al., 2021; Sattar et al., 2023). In Nepal’s Kanchenjunga region, at least six severe GLOF events have occurred historically. Among these, remote sensing analysis of the Nangama GLOF event suggests that approximately 800,000 cubic meters of ice debris and avalanches might have triggered an outburst of about 11.2 million cubic meters ±1.4 million cubic meters of water. Debris from the flood formed a moraine-dammed lake called Chheche Pokhari Lake on the Pabuk Khola River, around 2 kilometers downstream from the lake (Byers et al., 2020). Statistics show about 80% of GLOF events are triggered by glacier collapse or rockfall-induced ice avalanches (Awal et al., 2011). In recent years, human activities have intensified land resource development, and global warming has amplified hydrological cycles. As one of the most notable regions affected by global climate change, glacier-covered areas are experiencing rapid changes in retreat and melting, an increase in the frequency of extreme climate events. The frequency of extreme climate events is increasing. In particular, the frequency of GLOF outbreaks, the extent of disasters, and the scope of disasters are on the rise (Yang et al., 2022; Dømgaard et al., 2023; Emmer, 2023). Therefore, assessing the historical and future risks associated with glacial lakes is of paramount importance.
Traditionally, much of the research on GLOF risks has focused on large glacial lakes and climate change-triggering factors. However, there remains a gap in understanding mechanisms related to wave overflow, erosion-induced dam failure, and empirical data. The spatial and temporal distribution of GLOF disasters is uneven (Dou et al., 2023). Spatially, due to specific geological and glaciodynamic conditions, different regions might exhibit localized hotspots, demonstrating significant spatial heterogeneity (Yang et al., 2023c; Dahlquist and West, 2022; Qi et al., 2022). Temporally, the probability of disaster occurrence increases during certain seasons or specific climate events, and interannual variability suggests fluctuations in the frequency of disasters each year (Taylor et al., 2022; Emmer, 2023). Long-term trends describe changes in disaster frequency, scale, and timing, often attributed to climate change and glacier retreats (Ahmed et al., 2022a; Emmer et al., 2020; Zhao et al., 2022). It’s important to note that GLOF disasters are sudden and are limited by the lack of monitoring techniques and incomplete data resources, making it challenging to directly observe their mechanisms and processes (Worni et al., 2014; Williams et al., 2022; Ali et al., 2023). This involves comprehensive analysis of multi-source data across multiple time and spatial scales, making it difficult to accurately identify or predict the location and timing of disaster occurrences (Hata et al., 2022). In contrast to mudslides and landslide disasters, the prediction and monitoring of these events typically require consideration of factors such as rainfall and mountain stability. Various methods, including experimental research, erosion monitoring, and numerical simulations, can be employed (Qiu et al., 2022; Wang X. et al., 2022c; Ma et al., 2023; Yang et al., 2023d; Zhao et al., 2023). On the other hand, the prediction and monitoring of glacial lakes necessitate a focus on factors such as the water level of the glacial lake and glacier meltwater volume. These tasks often rely more on remote sensing technologies and sensor monitoring methods. In recent years, with the advancement of remote sensing and Geographic Information Systems (GIS) techniques, various glacial lake identification methods have been increasingly applied in identifying glacier lakes. By interpreting and analyzing remote sensing images, researchers can identify information about the number, location, morphology, and area of glacial lakes, aiding in identifying potential risk areas (Begam and Sen, 2019; Ahmed et al., 2022b). Additionally, GIS technology can be used to analyze issues related to the spatial distribution, trends, and potential risks of glacial lakes (Rawat et al., 2023; Ahmed et al., 2022c). As a critical component of warning systems, glacial lake identification techniques have gradually evolved into important ways of identifying potential risk areas and predicting disaster situations. They offer scientific support for glacial lake management, monitoring, ecological conservation, and biodiversity protection, ultimately safeguarding people living in potentially risky areas and creating a more stable environment for sustainable development (Zhou et al., 2023; Wang L. Y. et al., 2022). Despite the achievements in glacial lake identification, challenges still exist. Such as the accuracy of remote sensing image interpretation, long-term monitoring of glacial lake changes, and the identification and risk assessment of potentially dangerous glacial lakes (Kaushik et al., 2022; Gao et al., 2023; Zhang et al., 2023).
Bibliometrics is a crucial method for evaluating the current status, forefront trends, and developmental trajectories of scientific research. In the context of glacial lakes, bibliometrics finds applications primarily in glacier mass balance research identification and monitoring, evolution of glacial lake outburst processes, and assessment of disaster risks based on remote sensing techniques (Liu et al., 2023). Through bibliometric and data visualization analysis of glacial lake literature related to remote sensing technology in the Web of Science (WOS) database from 1990 to 2021. China and the United States are major countries in the application of remote sensing technology to glacial lake research. The Chinese Academy of Sciences stands out as the most productive research institution. Current research hotspots include keywords such as “Climate change,” “Inventory,” and “Dynamics” (Yu et al., 2023). Bibliometric methods have played a significant role in the overview studies of glacial lake-related fields, particularly in studies regarding glacier retreat and glacial lake outbursts. For instance, bibliometric analysis of literature on GLOFs has helped to identify research characteristics, geographical distribution, and trends on a global scale (Emmer, 2018). The intrinsic connection between dynamic changes in glacial lakes and climate change, as well as natural disasters such as glacial lake outbursts, have a huge impact on the environment and society. This makes the study of glacial lakes a hot topic that arouses strong interest among scientific researchers and society. Despite the substantial progress made by numerous researchers in this field, there is still a lack of comprehensive statistics and analysis of the literature. Therefore, it’s essential to systematically review and summarize the current state of glacial lake identification research. This approach will provide a deeper understanding of the developmental trajectory of this research. At the same time, we will enhance comprehension of the mechanisms behind glacial lake outburst disasters and predictive warning technologies, identify pressing scientific issues, and grasp current research hotspots and future trends. Such a review study will contribute to guiding and referencing further research in the field of glacial lake identification.
This study employs a research approach that combines literature review, knowledge graph analysis, and bibliometrics. The primary analytical methods utilized include citation analysis, co-citation analysis, and bibliographic coupling analysis. Techniques such as clustering analysis, visualization analysis, and change-point detection analysis are employed. Through these methods, the study aims to comprehensively analyze the main achievements, research hotspots, cutting-edge directions, and future research trends in the field of glacial lake identification based on authoritative literature data. Diverging from previous research, this study integrates traditional literature reading methods with bibliometric visualization analysis (Hengst et al., 2021; Veettil and Kamp, 2021). This integration offers an objective and systematic overview of research based on glacial lake identification. Focusing on literature in the field of glacial lake identification published on the WOS database, the study summarizes and analyzes research accomplishments over the past 32 years. It specifically emphasizes the application of identification techniques in the early detection of glacial lake outburst disasters, extending beyond the realms of GIS and remote sensing technology. The research objectives encompass: (1) Identifying representative countries, institutions, and collaboration patterns within the field. (2) Uncovering valuable information contained in core literature. (3) Highlighting current research hotspot themes. (4) Revealing trends in glacial lake identification literature globally, encompassing the past, present, and future. (5) Summarizing the developmental trajectory of the field, pointing out shortcomings, challenges, and frontiers of research. Through the integration of diverse analytical methods, this study provides a holistic understanding of the glacial lake identification field, shedding light on its evolution, challenges, and promising research directions.
This research is based on the fundamental theories of bibliometrics. It utilizes a literature knowledge graph generated from a sample of literature, employing quantitative statistics, co-occurrence analysis, and visualization methods. The objective is to reveal the progress and hot topics in the field of glacial lake identification-related research and predict future research trends and focal points. For quantitative analysis of data, various software tools were used, including VOSviewer (version 1.6.19, Centre for Science and Technology Studies, Leiden University), CiteSpace (6.1.R6 Advanced), Pajek (version 2008), and Origin, among others. Using VOSviewer and CiteSpace, all essential functions required for literature data visualization were realized. These tools offer powerful capabilities in terms of literature data statistics, co-occurrence word clouds, network clustering, density analysis, and interactive relationships, along with efficient graphical user interfaces (Zhang et al., 2022a). Through different algorithms for standardization, mapping, and clustering, the study constructs and visualizes keyword cluster analyses of glacial lake identification, relationships among countries’ publications, as well as co-occurrence analyses in the field. This generates interactive visualizations of the structural and temporal patterns and trends in the scientific field, allowing for a comprehensive overview of the glacial lake identification domain. This approach enables researchers to closely and widely track the development of the field, discern prominent shifts in knowledge, and identify focal topics (Li et al., 2022a). The analysis in this paper includes various aspects: (1) Research progress and hot directions across different periods and countries. (2) Clustering of collaborative relationships among countries and institutions. (3) Evolution of thematic paths. (4) Co-occurrence clustering of keywords. (5) Keyword emergence graphs, among others.
We employed the Web of Science Core Collection (WOSCC) database for literature retrieval and data collection to obtain a sample of literature related to the field of glacial lake identification. WOS is one of the earliest and most widely used databases globally, renowned for its reputable and widely recognized literature data, along with its rich literature organization and statistical features (Liu, 2019; Singh et al., 2021). Precisely defining the search formula is a key prerequisite for successfully collecting sample literature data. We used the following search formula to filter relevant literature: (TS = (Glacial Lake) and TS = (Identify) or TS = (Glacial Lake) and TS = (Identification) or (TS = (Glacier Lake) and TS = (Identify) or TS = (Glacier Lake) and (Identification)). The retrieval date was May 20, 2023, resulting in 2,143 relevant documents. After refining the data by literature type, we retained three types of articles: research papers, conference papers (meetings), and review papers. Subsequently, we exported the data in plain text format. Using the built-in deduplication and merging functions in the CiteSpace software, we further processed the data by removing duplicates and eliminating literature unrelated to the main topic terms. This resulted in a final dataset of 2,121 sample documents relevant to the topic of glacial lake identification.
Global annual publication counts can directly reflect the research activity and scientific development trends in a particular academic field (Wang et al., 2021). By utilizing the citation analysis report from the WOS, we can gather key data indicators for analyzing the development of the glacial lake identification research domain. According to the provided citation analysis report: There are 46,472 citing documents, reduced to 45,296 after excluding self-citations; the total citations count is 66,326, reduced to 62,537 after excluding self-citations; the average citations per paper is 31.27, and the h-index is 105. Considering that data for the year 2023 is incomplete, it’s not included in Figure 1. To gain a comprehensive understanding of the glacial lake identification research field, a slice analysis of retrieval data from 1991 to 2022 was performed with a time interval of 1 year. This analysis reflects the patterns of both the total number of articles (TP) and annual article impact (H) changing over time (where H represents the number of citations received by the most cited article in a year, reflecting its influence) (Mansur et al., 2021). Figures 1, 2 illustrate the global volume of publications and their citation impact, respectively.
Figure 1 Temporal variation in the number of published literature and citations on glacial lakes from 1991 to 2022. (A) Quantity of published literature. (B) Citation count. Curves represent exponential fits based on the data.
During the period from 1991 to 2023, despite fluctuations in the number of published papers related to glacial lake identification (n = 2121) in certain years, the overall trend of global publication volume and citation count has been on the rise (Figure 2). The quantity of publications and citations is mainly concentrated after 1998, with the highest number of articles published after 2013, showing the most significant growth. Through statistical analysis, it was found that the total number of published articles in the 9 years after 2014 is approximately 1.4 times that of the 23 years before 2014. This suggests that research in this field has garnered widespread attention from the academic community in recent years, which might also reflect the severity of GLOF risks. The citation impact of articles in the field of glacial lake hazards has exhibited an exponential growth trend since 2000. This indicates, on one hand, that new technologies like remote sensing, GIS, and deep learning have provided more data sources and analytical tools for glacial lake identification research (Lu et al., 2020; Sawi et al., 2022). On the other hand, in recent years, topics such as the interaction between glacial lakes and climate change, glacial lake water resources management, and glacial lake-related disasters have increased in frequency. Disaster early warning, glacial lake change monitoring and evolution mechanism, glacial lake flood and outburst risk assessment, glacial lake and ecological environment impact, glacial lake disaster prevention and risk management urgently need to carry out in-depth and detailed research (Mulsow et al., 2015; Viani et al., 2022).
From 1991 to 2023, the number of cited papers in glacial lake identification research has shown two significant leaps. Based on the growth trends of publications and their citations, the publication history in this field can be divided into three phases. The first phase (1991-1997) was constrained by limited remote sensing technology and data accessibility, focusing on preliminary exploration primarily in specific glacier regions and types of glacial lakes (Knox, 1996). The second phase (1998-2013) witnessed noticeable progress compared to the first phase, with a steady increase in annual publications and citations. During this phase, a focus was placed on processing multi-source remote sensing data, improving feature extraction algorithms, establishing diverse datasets, and monitoring glacial lake evolution and trends (McKillop and Clague, 2007; Bolch et al., 2011; Wang et al., 2012). The third phase (2014 to present) saw rapid developments in numerical models and machine learning. The number of publications and citations increased from 103 and 3,163 in 2014 to 168 and 7,838 in 2022, respectively. As of May 20, 2023, the publication count and citation count for the year 2023 are 54 and 3,467, respectively. Additionally, the rate of citation growth has outpaced the growth of publications, indicating a significant lag effect and the increasing interest of researchers in the glacial lake identification field over the past three decades. This can be attributed to interdisciplinary technology integration and application, the launch of more satellites, the establishment of hydrological models, faster computing platforms, and more accurate artificial intelligence models. It provides abundant high-precision, large-scale data support for glacial lake identification research (Thakur et al., 2016; Motschmann et al., 2020). Furthermore, international peer communication and knowledge sharing have played a crucial role in the rapid advancement of research in this field (as discussed further below) (Zdorovennova et al., 2021).
Academic journals are essential platforms for the public dissemination of research findings and serve as crucial channels for researchers to gain new knowledge, theories, and discoveries in specific fields or topics (Bondi and Cacchiani, 2021). Research outcomes in the field of glacial lake identification are distributed across 621 different journals, with 344 of them having published only one paper each. The relevant information for the top 10 journals in terms of publication count is provided in Table 1. Upon analysis, it becomes evident that most of these journals belong to the category of Earth Sciences, covering various disciplines such as natural sciences, environmental sciences, and paleobiology. These journals encompass fields including disaster science, geography, remote sensing, and environmental science, demonstrating the diversity and interdisciplinary nature of glacial lake research topics. From Table 1, it can be observed that the 5-year impact factors of the top 10 journals range from 2.1 to 5.6, showing a significant span. However, the number of high-impact factor journals is relatively small, indicating that there is a scarcity of high-quality articles in the field of glacial lake identification research. The quality of papers varies, and there is a need to further enhance the overall research quality. Over 32 years, the journal “Quaternary science reviews” has published the highest number of papers (178), followed by “Boreas” (84), “Quaternary international” (73), and “Geomorphology” (69). “Quaternary science reviews” is recognized as one of the most authoritative and academically influential journals in the field of earth sciences. It has a high 5-year impact factor and H-index. Although “Frontiers in earth science” has a high publication count and impact factor, its relatively low H-index suggests that there is still room for improvement in terms of academic influence for the papers it publishes. When considering the locations of journal publishers, the top 10 journals are all based in developed countries. Among them, three are based in the United Kingdom and the Netherlands, indicating that these developed countries hold a leading position in glacial lake identification research.
This study aims to provide a comprehensive overview of the publication status of countries or regions that rank highly in terms of the number of published articles, revealing the distribution of the global glacial lake identification field. The paper quantity from the top 10 ranked countries is summarized (Figure 2 and Table 2), encompassing all countries mentioned in the literature. The spatial distribution of literature publication reflects the academic research activity level within a particular field for countries or regions, offering insights into regional strengths, and influence, as well as international exchange and collaboration. The collaboration network graph (Figure 2) displays the level of cooperation among countries, with the thickness of connections reflecting the frequency of collaboration—thicker lines signify closer cooperation. Over 100 countries or regions have published papers related to glacial lake identification.
Table 2 Top 10 countries by quantity of published literature on glacial lake identification and relevant data.
From Figure 2, it can be observed that the United States engages in the most frequent international collaboration, followed closely by the United Kingdom, Germany, France, and China. China maintains significant exchanges with countries like the United States, Canada, Australia, and Japan. Sweden, Norway, Belgium, France, the Netherlands, and Germany also exhibit close collaboration. The combined publication count of the top ten countries is 2,727 papers, accounting for 128.57% of the global total, the top 5 countries collectively contribute to 99.67%. Despite variations in publication volume among different countries, it reflects wide attention from researchers across multiple nations to the field of glacial lake identification. This underscores the vital role of international collaboration in advancing this field’s academic progress, with global cooperation and exchanges exerting a positive influence. In terms of publication count by country, the United States holds a leading position in the global glacial lake identification field. According to statistics from the WOSCC database, the United States has published a total of 649 articles, constituting 30.60% of the overall total (2,121 articles), the United Kingdom has published 510 articles, China has published 406 articles, Canada has published 283 articles, and Germany has published 266 articles. Considering citation counts, the United States has accumulated a total of 20,677 citations, averaging 38.94 citations per paper, the United Kingdom has 20,241 citations, averaging 41.82 citations per paper, China has 10,174 citations, averaging 25.06 citations per paper, Canada has 21,758 citations, averaging 29.39 citations per paper, and Germany has 9,142 citations, averaging 34.37 citations per paper. Notably, China’s average publication year is 2017, in stark contrast to other countries whose average publication years fall around 2013. This indicates China’s rapid increase in attention to this field in recent years, suggesting a potential ongoing growth in scientific influence. Among the top 10 countries for article publication, only China and Russia are developing countries, with relatively lower average citation rates per article. In summary, research in the glacial lake identification field centers around the United States, the United Kingdom, and China, with significantly higher publication quantities and citation counts compared to other countries. This highlights their substantial contributions in the research domain, potentially linked to their advantages in education, research investment, and technological innovation. Other developed nations also play important roles in the field, while developing countries complement these efforts, making crucial contributions to progress through their strengths in science, technology, talent cultivation, and socio-economic development.
Inter-institutional collaboration relationships provide insights and evaluations of collaboration patterns, effectiveness, and innovation capabilities among institutions, serving as references and guidance for the development of institutional partnerships. We considered the clarity of the institutional collaboration network diagram, setting a minimum publication threshold of 20 to select and focus on active and influential institutions within the research domain. Figure 3 illustrates the collaboration network diagram of these institutions. Based on calculations from VOSviewer software, the Chinese Academy of Sciences has collaborated with other institutions to publish the most articles in glacial lake identification research (154 papers). It is followed by the University of Chinese Academy of Sciences (52 papers), Russian Academy of Sciences (48 papers), University of Bergen (48 papers), and United States Geological Survey (42 papers)—these 5 institutions have the highest publication volume. In terms of citation counts, the Chinese Academy of Sciences has the highest number of citations in the WOS, totaling 5,369. It is followed by the University of Zurich (1,966 citations), University of Sheffield (1,785 citations), US Geological Survey (1,700 citations), and University of Colorado (1,640 citations). Looking at the total link strength, the top 5 institutions are the Chinese Academy of Sciences (115), the University of Chinese Academy of Sciences (62), the University of Bern (42), the University of Oxford (39), and University of Colorado (38). It’s evident that the Chinese Academy of Sciences not only leads in terms of article publications but also possesses an extensive collaborative network in international peer relationships (highest total link strength). The Chinese Academy of Sciences, the University of Chinese Academy of Sciences, and the University of Colorado serve as key institutions in the glacial lake identification field, playing significant roles in research within the domain. It’s worth noting that although institutions like the Russian Academy of Sciences, the University of Copenhagen, and the Australian National University demonstrate impressive research achievements, cross-national or cross-regional institutional collaboration appears relatively low. Therefore, enhancing knowledge sharing, collaboration, resource sharing, complementarity, and expanding the impact and sustainability of research are aspects that require strengthening in the future international cooperation within the glacial lake identification field.
Figure 3 Visualization of institutional publication quantity and its collaborative relationships network.
Literature co-citation clustering analysis is a method of literature analysis aimed at identifying and analyzing groups of literature that share similar citation patterns and citation networks. Using the co-citation network analysis method, a total of 33 clusters were identified in the field of glacial lake identification. This study primarily focuses on the 4 most representative clusters among these 33, as illustrated in Figure 4. Modularity Q and silhouette values represent the quality of the clustering results. In this study, the network modularity (Q=0.9006) is greater than 0.3, and the silhouette value (0.9618) is greater than 0.7. This indicates that the co-citation clusters exhibit characteristics of large clusters and that the performance of the network clustering is favorable (Chen, 2006). The study primarily delves into the top 4 major clusters. Tables 3, 4 provide information about these clusters, including silhouette, size, representative terms, and exemplary literature. Based on the clustering analysis, the top 4 clusters are as follows: Cluster #0 - Debris-Covered Glaciers, Cluster #1 - Glacial Lake Outburst Floods, Cluster #2 - Climate, and Cluster #3 - Glacial Lakes.
Figure 4 Knowledge graph of co-citation network clustering analysis. Here, the Link Retaining Factor (LRF) is 3.0, the maximum Links per Node (L/N) is 10, the Look Back Years (LBY) is 5, the minimum citation number (e) is 1.0, the notes is 904, and the nodal links is 1,747.
As shown in Figure 4, the most prominent cluster topic is #0 “Debris-Covered Glaciers”. This cluster comprises 68 articles with an average publication year of 2011 and a Silhouette value of 0.943. The cluster theme is related to the formation of glacial lakes. The most representative article within this cluster is “response of debris-covered glaciers in the Mount Everest region to recent warming and implications for outburst flood hazards”. This article highlights that climate warming may intensify the melting of debris-covered glaciers in the Everest region, potentially leading to severe GLOF disasters (Benn et al., 2012). The main thematic terms within this cluster include “surface ponds”, “hazard assessment”, and “glaciological natural hazards”. “Surface ponds” refer to surface water bodies on debris-covered glaciers, playing a critical role in the field of glacial lake identification. These ponds are a result of accumulated meltwater on the debris-covered ice surface and significantly impact glacier dynamics, such as melt rates and the formation of surface water pools (Miles et al., 2017). “Hazard assessment” and “glaciological natural hazards” emphasize the natural disasters related to glacier dynamics, particularly in cases where the glacier surface is covered with debris. These natural hazards encompass a variety of phenomena, including glacier floods and ice avalanches. In such contexts, conducting hazard assessments is a crucial step in eval-uating the likelihood of global glacier meltwater disasters. Understanding the hazards posed by debris-covered glaciers is essential for assessing risks associated with glacial lakes and implementing disaster mitigation strategies (Ye et al., 2022). Within this cluster, the themes of important articles predominantly cover the impacts of global warming on the current state and prospects of glaciers in the Himalayan region, compiling comprehensive inventories of glacial lakes and risk assessment methods, as well as integrated strategies for managing glacial lake disasters (Bolch et al., 2012; Lesi et al., 2022; Viani et al., 2022; Rinzin et al., 2023; Singh et al., 2023).
The second major cluster topic is #1 “Glacial Lake Outburst Flood” with 56 articles. The average publication year is 2019, and the silhouette value is 0.917. This cluster emphasizes an understanding of GLOF disasters. The key thematic terms within this cluster include “glacier eruption floods”, “GLOF hazard”, and “ice thickness glacier velocity”. This underscores that GLOF disasters are frequently discussed topics in the field of glacial lake identification. In this cluster, considerable attention is given to the frequency and severity of glacial lake outburst events, especially detailed studies concerning factors driving erosion and scouring along their paths. Scholars employ techniques like remote sensing interpretation and historical record analysis to study the frequency and severity of GLOFs (Shan et al., 2021; Dømgaard et al., 2023). This analysis helps identify regions prone to frequent global climate change-induced occurrences, assess potential hazards of GLOFs to human communities, infrastructure, and the environment, and identify environmentally vulnerable areas. This, in turn, prioritizes monitoring, disaster prevention, and mitigation efforts in these regions for decision-makers and stakeholders. Additionally, some researchers explore the significant role of erosion in the formation and evolution of glacier lakes. They study various erosion processes, including glacial erosion, water flow scouring, and sediment transport (Tomczyk and Ewertowski, 2021). Furthermore, certain scholars investigate variables such as glacial lake flow velocity, glacier morphology, and potential topographic conditions to determine how these driving factors impact the formation and expansion of glacial lakes (Wang W. C. et al., 2022). These studies provide crucial insights into understanding the mechanisms and influencing factors of GLOF disasters in greater depth.
The third major cluster topic is #2 “Climate,” comprising 52 articles. The average publication year for this cluster is 2010, and its silhouette value is 0.996. The main thematic terms within this cluster include “Last Glacial Maximum”, “glacial geomorphology”, and “Switzerland.” This indicates that climate change as a significant driving factor for glacial lake formation is frequently mentioned in the field of glacial lake identification, particularly when exploring sensitive areas highly influenced by climate. Some scholars conduct research on glacial lakes within their study areas at long-term climate scales, including interannual and decadal scales (Morriss et al., 2013; Harrison et al., 2019). Research findings suggest that rising temperatures and changes in precipitation patterns contribute to glacier retreat and glacier meltwater, providing a water source for glacial lakes and resulting in changes in glacial lake dynamics (Allen et al., 2016; Gao et al., 2023). Certain researchers simulate future climate scenarios to assess potential impacts on glacial lakes (Allen et al., 2022). These studies offer insights into understanding how glacial lakes respond to climate change. Future research will likely focus on quantifying the effects of these interactions on glacial lake formation, development, and outburst. This cluster highlights the importance of investigating how climate change influences glacial lake dynamics and how glacial lakes respond to changing climate conditions. Such research is crucial for understanding the broader implications of climate change on glacial landscapes and associated hazards.
The fourth major cluster topic is #3 “Glacial Lake” encompassing 49 articles. The average publication year for this cluster is 2014, and its silhouette value is 0.982. The key thematic terms within this cluster include “lake mapping”, “Eastern Himalayas”, and “hydrodynamic modeling”. This cluster emphasizes the significance of glacial lake monitoring, remote sensing, and hydrological modeling as crucial technical approaches in the field of glacial lake identification. This is particularly notable for specific regions like the Eastern Himalayas. In terms of lake mapping, some researchers employ techniques such as satellite imagery, aerial photography, and LiDAR scanning to identify and delineate glacial lakes (Dhote et al., 2022). They analyze the spectral and spatial characteristics of these lakes to distinguish their natural features from other bodies of water. Lake mapping studies also focus on detecting and monitoring temporal changes in glacier lakes. By comparing historical imagery with current data, researchers track fluctuations in lake area, volume, and extent (Kellerer-Pirklbauer et al., 2021). This information aids in understanding how glacial lakes respond to climate change, glacier dynamics, and other environmental factors. Furthermore, some scholars have developed comprehensive databases for glacial lakes, including their locations, areas, and other relevant information. These inventories serve as valuable resources for monitoring changes, assessing hazards, and managing glacier lakes (Shugar et al., 2020). In the cluster with the theme “hydrodynamic modeling” mathematical models are primarily applied to simulate and understand the hydrodynamic behavior of glacial lakes. Research in this area includes model development and validation, assessment of lake stability and potential hazards, and predictions of future changes in glacial lake behavior. The focus is on the physical processes and dynamics of glacial lakes, such as water flow, sediment transport, and lake level fluctuations. This cluster underscores the importance of employing advanced techniques like remote sensing and mathematical modeling to comprehensively study glacial lakes’ physical characteristics, behavior, and responses to changing environmental conditions (Sattar et al., 2021; Duan et al., 2023).
The WOSCC database categorizes papers from various journals precisely and integrates them based on specific scientific fields. This categorization assists researchers in gaining in-depth insights into the unique contributions of various subfields within a specific research area, aiding academic assessment, research orientation, and identifying collaboration opportunities. By mapping these scientific categories onto the comprehensive dataset of glacial lake identification literature, a more targeted and comprehensive research perspective is provided to analyze the subfields and research hotspots involved in this research domain. To reveal the disciplinary characteristics of the research field more visually, this study has created an overlay map of the research domain (Figure 5) based on the Global Science Map, which is derived from the summarized literature data of the WOS database (Leydesdorff et al., 2013; Leydesdorff et al., 2019).
Figure 5 Research categories in the field of glacial lake identification on the global science category map.
The collection divides all WOS research fields into 5 clusters: #1 Biology & Medicine, #2 Chemistry & Physics, #3 Ecology and Environmental Science & Technology, #4 Engineering & Mathematics, and #5 Psychology & Social Sciences. According to these results, the majority of literature on glacial lake identification research falls under the “Chemistry & Physics” scientific field (cluster #2). Within this, the “spectroscopy” scientific category stands out, indicating the pivotal role spectroscopic techniques play in glacial lake identification and research. Spectroscopic techniques, predominantly employed in remote sensing and satellite image analysis, play a critical role in accurately identifying and monitoring glacial lakes. By studying unique spectral features like glacial lakes and shape and texture features, researchers can differentiate glacial lakes from other substances. This wide application of spectroscopy enables large-scale detection and mapping of glacial lakes, providing valuable data for inventorying and monitoring (He et al., 2021). Spectroscopy is also crucial for assessing the temporal evolution of glacier lakes, including expansion or retreat, causes of GLOFs, climate change impacts, and potential hazards. These pieces of information are vital for an in-depth understanding of dynamic changes within glacial systems (Ahmed et al., 2022b; Kumar et al., 2021). In this scientific field, the second most significant scientific category is “green & sustainable science & technology” which plays a vital role in glacial lake identification through various technological applications. One important application is powering glacial lake monitoring systems using renewable energy sources. By utilizing solar energy and other renewables, researchers can reduce carbon emissions and ensure continuous monitoring of glacial lakes without relying on fossil fuels. Furthermore, green and sustainable technologies aid in comprehending the environmental impacts of GLOFs and the role of climate change. Through sustainable monitoring techniques, researchers have been able to gather data on global warming and its consequences, such as downstream flooding and infrastructure damage, providing support for risk mitigation strategies related to GLOFs (Viani et al., 2022). Moreover, green and sustainable technologies underscore the importance of preserving glacier ecosystems and their biodiversity (Viani et al., 2020). With sustainable methods, researchers can minimize disturbances to these fragile ecosystems, promoting long-term protection and conservation of glacial lakes.
The second most prominent scientific field is “Ecology and Environmental Science & Technology” (cluster #3). Within this field, the most representative scientific categories are “environmental sciences” and “ecology” which have important applications in glacial lake identification. One significant application is the study of the physical and chemical characteristics of glacial lakes. Researchers analyze factors such as water temperature, pH, and sediment composition to understand the characteristics of the glacial lake itself and its surrounding environment. This information aids in identifying and categorizing different types of glacial lakes, allowing for the assessment of their vulnerability to climate change (Qu et al., 2022; Steffen et al., 2022). This understanding of the interaction between glacial lakes and their environment contributes to the scientific basis for protecting and managing these fragile ecosystems. Another application of environmental science in glacial lake identification is the study of glacial lake dynamics. Researchers utilize hydrological models and field measurements to explore the processes of glacial lake formation, expansion, and retreat. These applications are crucial for predicting the future changes in glacial lakes and their potential impacts on downstream communities’ safety, ecology, and economy (Ogier et al., 2021).
The third most active scientific field is “Engineering & Mathematics” (cluster #4), with the most representative scientific categories being “computer science, interdisciplinary applications” and “engineering, multidisciplinary”. Computer science has multiple important applications in glacial lake identification. One primary application is remote sensing and image processing techniques. Computer scientists develop algorithms and models to analyze satellite images and aerial photographs of glacial lakes, determining and mapping features such as their location, size, shape, and depth (Li et al., 2022b). Furthermore, GIS play a crucial role in glacial lake identification within the realm of computer science. Researchers use GIS software to integrate and analyze various spatial data, such as topographic maps, elevation models, and hydrological data (Viani et al., 2016). This helps understand the spatial relationships between glacial lakes and their surrounding environment, as well as predict potential risks of GLOFs. Another important application of computer science in glacial lake identification is the development of automated and efficient recognition methods. Leveraging machine learning and artificial intelligence technologies, researchers have been able to train algorithms to identify and classify glacial lakes from large datasets. This significantly enhances the speed and accuracy of glacial lake identification, thereby improving the monitoring and management of these dynamic environments (Bazilova and Kääb, 2022). Additionally, computer science plays a critical role in the development of decision support systems for glacial lake management. By integrating diverse data sources and utilizing modeling techniques, valuable insights and technical support are provided for glacial lake management decisions, aiming to mitigate risks associated with GLOFs and climate change (Rinzin et al., 2023). Glacial lake identification research exhibits typical interdisciplinary characteristics. Through the integration of various scientific categories such as environmental science, computer science, geology, hydrology, and climatology, researchers can gain a more comprehensive understanding of glacial lakes and their dynamics. This multidisciplinary approach highlights the complex interactions and feedback mechanisms between glacial lakes and their surrounding environment. By considering factors such as climate change, glacier dynamics, hydrological processes, and human activities, researchers can better comprehend the driving factors and impacts of glacial lake formation and changes (Prakash and Nagarajan, 2018). Moreover, interdisciplinary approaches aid in the development of early warning systems for GLOFs and decision support tools, contributing to the improved management and protection of these fragile ecosystems (Gu et al., 2023).
The fourth most active scientific field is “Biology & Medicine” (cluster #1), with a significant and representative scientific category being “mathematical & computational biology”. The application of mathematical and computational biology in this field includes: (1) Quantitative modeling of glacial lake dynamics: Mathematical modeling is employed to describe the physical, chemical, and biological processes that control the formation, growth, and decay of glacial lakes. These models integrate various parameters such as glacier melting rates, precipitation, and temperature (Lencioni, 2018; Ogier et al., 2021). (2) Species distribution models: Computational biology techniques are used to establish species distribution models for biological entities within glacial lakes. These models are built based on environmental and ecological data, predicting the spatial distribution and potential habitat suitability for different species within glacial lake ecosystems (Medeiros et al., 2022). By simulating the response of biological communities and habitats to changing environmental conditions, these models help predict the impact of climate change on glacial lake ecosystems. This aids in assessing potential changes in species ranges and ecological disruptions, enabling the development of effective strategies for the protection and management of glacial lake ecosystems. It also facilitates the identification of priority conservation areas within glacial lake ecosystems, allowing targeted conservation efforts to maintain crucial habitats and species. The application of mathematical and computational biology enhances our understanding of the complex interactions between glacial lakes and their biological components, and it contributes to informed decision-making for the conservation and sustainable management of these unique ecosystems.
In our study, we have discovered that the field of “Psychology & Social Sciences” (cluster #5) exhibits the lowest level of activity among scientific domains. All the scientific categories within this field have fewer than 70 documents each, with “social sciences, interdisciplinary” (62 documents), “social issues” (57 documents), and “environmental studies” (53 documents) being the most significant ones.
By employing the clustering analysis technique within the CiteSpace software, researchers can visually depict the evolutionary process of various research topics over different periods, thereby unveiling research hotspots and future trends in the field’s development (Abbas et al., 2018). In this study, we harnessed CiteSpace’s distinctive functionalities, namely Timeline and Burstness, to process the foundational data of the literature. The time range was set from 1991 to 2023, with a time slice length of 1, enabling the detection of burst keywords within each time slice. The research outcomes are presented in Figures 6, 7. Figure 6 illustrates ten timelines, while Figure 7 showcases 25 keywords, with high-frequency keywords predominantly appearing before 2010. The forefront of glacier lake identification research manifests significantly in three distinct research stages, each characterized as follows.
Figure 6 Visual timeline clustering of research topic evolution from 1991 to 2023. Curves indicate literature citation relationships, with warmer colors indicating closer research time frames. Larger keyword clusters signify research hotspots for a specific period.
(1) The period from 1991 to 2010 marked the primary stage of research in the field of glacier lake identification. Emerging keywords during this phase include “Canada”, “British Columbia”, “age”, “record”, “Younger Dryas”, “island”, “Antarctica”, “vegetation history”, “Lake Vostok”, and “calibration”. Additionally, high-frequency keywords associated with this period encompass “climate change”, “Last Glacial Maximum”, “lake sediment”, “environmental change”, and “ice sheet” among others. The timeline chart and burst keywords of this period exhibit a substantial number of terms, indicating a strong academic interest in the glacier lake identification domain and the emergence of numerous new research concepts. The research direction is relatively diverse, encompassing a wide range of scientific inquiries, warranting continuous and profound attention. The primary research themes during this phase pertain to glacial lake formation and evolution, exploration, and research of paleoclimate and paleoenvironment, and lake sediment analysis (Bajracharya and Mool, 2009; Corbett and Munroe, 2010; Sionneau et al., 2010).
(2) The period from 2011 to 2018 witnessed the emergence of keywords such as “catastrophic drainage”; “Pleistocene”, “glacial lake”, “subglacial lake”, “flood”, “stream”, “volume”, and “conservation”. High-frequency keywords closely related to this era also encompassed “hazard”, “glacial geomorphology”, and “mass balance” among others. Building upon the foundation of 1991 to 2010, this stage led to the emergence of several new specific research directions within the GLOF domain, contributing to the enrichment and further development of glacier lake identification research. The primary research themes during this phase encompass glacial lake hazards and risk assessment and glacial lake dynamics. The theme of glacial lake hazards and risk assessment primarily involves the evaluation of potential risks associated with glacial lake disasters, as well as the development of warning systems and disaster management strategies (Petrakov et al., 2012; Aggarwal et al., 2016). On the other hand, the glacial lake dynamics theme focuses on the study of glacier lake volume and dynamics, involving keywords such as “volume”, “glacial geomorphology”, and “mass balance”. This research content encompasses measurements and monitoring of glacier lakes, along with investigations into the mechanisms underlying interactions between glacier lakes and factors such as climate and topography (Li and Sheng, 2012; Emmer et al., 2015).
(3) The period from 2019 to 2023 brought forth keywords such as “inventory”, “glacial lake outburst flood”, “risk”, “dynamics”, “Tibetan Plateau”, “evolution”, and “high mountain Asia”. Concurrently, high-frequency keywords included “Himalaya”, “moraine dammed lake”, “evolution”, “permafrost”, and “precipitation” among others. During this phase, the glacier lake identification research domain exhibited a rapid increase in annual publications, entering a phase of heightened research activity. Various research branches gradually evolved into more specific directions, yet fewer new research directions emerged. Instead, researchers focused on in-depth investigations building upon the foundation of the preceding phase. Notable research themes in the hotspot literature included the drawing of inventories for lakes in the Qinghai-Tibet Plateau using remote sensing techniques, GLOF risk assessment, precipitation variation and its impact on glacial lake response, and the evolution of glacial lakes in high mountain Asia (Allen et al., 2019; Dwivedi et al., 2022; Zhang et al., 2022b; Compagno et al., 2022).
In recent years, the field of glacier lake identification has made significant progress, yet it still faces a range of critical technical challenges. These challenges encompass limitations in spectral resolution, objective factors related to climate change, interference from shadows and ice, as well as constraints on the accuracy of automated algorithms. These factors restrict the depth and breadth of research and also impede the accuracy and applicability of relevant applications (Wangchuk et al., 2019; Hao et al., 2023). In the domain of glacier lake identification, accurate identification of glacier lakes holds extensive academic and practical value in reducing the environmental impact of these lakes. Therefore, this study delves into two research themes—identification techniques and methods, and identification scales—providing insight into the future directions of glacier lake identification research.
(1) In the realm of glacier lake identification techniques and methods, significant strides have been made, giving rise to various remote sensing technologies, GIS, and machine learning approaches (Kaushik et al., 2022; Wangchuk et al., 2022). These include feature extraction and classification methods based on synthetic aperture radar (SAR) and satellite multispectral data, as well as applications of deep learning models. These methods not only accurately identify the spatial distribution of glacier lakes but also distinguish between different types of glacier lakes, allowing researchers to determine the morphology of glacier lakes with unprecedented precision. This offers an effective means for studying glacier lake characteristics. However, despite these advancements, challenges persist. One of the most crucial challenges is the environmental complexity of glacial lakes. Factors like distinct topographic features, spectral differences due to regional water forms, and the transient nature of glacier lakes introduce complexities that existing algorithms struggle to address. This complexity results in potential ambiguities in satellite-derived datasets (Jiang et al., 2023). For instance, small and shallow glacier lakes are often obscured by surrounding vegetation or debris, posing a considerable obstacle. Additionally, while higher-resolution datasets enhance the accuracy of lake identification, they are also susceptible to increased noise, necessitating more intricate processing algorithms to extract accurate information. Existing models and algorithms still simplify the intricate interactions between glacier lakes and their surroundings to a certain extent. To achieve more accurate assessment results, further integration of multidisciplinary data from ecology, meteorology, geology, and more is needed to account for the complex interactions (Emmer and Curin, 2021). Lastly, while many existing models excel in identifying glacier lakes in specific geographical locations or under certain conditions, their applicability across different times and spaces remains a challenge. Investigating the applicability and scalability of these models requires deeper research to ensure their utility across diverse temporal and spatial contexts.
Drawing upon the comprehensive overview presented earlier, we proffer 5 pivotal research topics to steer the trajectory of future glacier lake identification research. To begin, in light of the burgeoning influx and diversification of remote sensing data, researchers must prioritize the advancement of more potent, adaptable, and efficient algorithms. In forthcoming investigations, precedence should be granted to the development of adaptive algorithms capable of dynamic adjustments based on the distinctive characteristics of diverse study areas. Such an approach would markedly enhance the accuracy of models across varying terrains and conditions. Moreover, the exploration of advanced algorithms rooted in machine learning and deep learning offers a propitious avenue to seamlessly identify and further refine glacier lake features within intricate backdrops. Illustratively, Convolutional Neural Networks (CNNs) hold the promise to discern nuanced spectral differentials through meticulous training. Secondly, cognizant of the intricate tapestry characterizing glacier lake regions, it becomes apparent that reliance on a solitary data source might prove inadequate to satiate the exigencies of accurate identification. Prospective inquiries could envisage the assimilation of data originating from optical, Synthetic Aperture Radar (SAR), and even on-site sensors, coalescing into an amalgamated multi-source data fusion dataset. This dataset, by amalgamating diverse streams of data, engenders a holistic image of the glacier lake landscape. Thirdly, the establishment of a systematic approach to trace the dynamic trajectory of glacier lake’s evolution assumes paramount significance. Such a methodological construct facilitates a comprehensive analysis of the evolution of glacier lakes, their latent perils, and the broader repercussions associated with climate fluctuations. Fourthly, the construction of integrative models, harnessing the unique strengths of each constituent model, offers a prudent stratagem to counterbalance the inherent limitations of individual models. Collaborative algorithms across models present the promise of engendering outcomes of heightened applicability, thereby impelling the ubiquity of glacier lake assessment models across a global expanse. Concludingly, notwithstanding the vantage point proffered by remote sensing techniques, the role of field research remains indispensable in the schema of validation and calibration. Future research endeavors should, in particular, be attuned to the exigency of on-site measurements, employing tools such as unmanned aerial vehicles or unmanned boats for the procurement of on-site data. This pivotal validation of remote sensing outcomes bolsters the credence of glacier lake identification.
(2) Concerning the issue of glacier lake identification scales, researchers have explored various scales, encompassing the microscale (such as glacier lake morphology and internal structure), mesoscale (such as geomorphic features of glacier lakes), and macroscale (such as regional distribution of glacier lakes), to delve into the complexity of glacier lake systems (Dahlquist and West, 2022; Li et al., 2022c; Zhang et al., 2022c). Nevertheless, despite headway being made, several outstanding challenges persist. Firstly, the challenge of data matching and fusion across disparate scales remains pivotal. How to organically integrate microscale glacier lake features with the macroscale spatial distribution poses a question that necessitates further inquiry. Secondly, scale conversion and data extrapolation stand as pivotal technical challenges. The translation of microscale data into analyses on a larger scale, as well as effective scale expansion when data is limited, demands comprehensive exploration. Moreover, the potential incongruities across various data sources and interpretations introduce complexity into comparative analyses and global assessments. Factors such as spatial resolution, the diverse geomorphic features of glacial environments, and the manifold physical attributes of glacier lakes themselves, collectively compound the difficulty in interpreting data.
Based on the preceding synthesis, we posit three pivotal research topics for the next phase. Firstly, the development and adoption of a unified standard framework assume paramount significance. Pioneering novel algorithms and techniques applicable to scale conversion and data extrapolation is indispensable to enhancing the precision and reliability of data translation across disparate scales. Such an endeavor would engender a standardized perspective for the observation, analysis, and comparison of glacier lakes, thereby ensuring the congruence of research outcomes. Secondly, concomitant with the escalating demand for heightened glacier lake identification accuracy, the requisition for data of elevated spatial resolution is concurrently mounting. Strategic investment and integration of these monitoring tools will contribute to refining the spectrum of identification scales and bolstering accuracy. Thirdly, cross-scale data fusion constitutes the linchpin in resolving the quandary of multi-scale glacier lake identification. Effectively amalgamating microscale and macroscale data through cross-scale data fusion empowers a more comprehensive apprehension of the complexity underpinning glacier lake systems. Such an approach holds the potential to furnish a more precise depiction and analysis of glacier lake changes by holistically integrating data across scales.
Against the backdrop of accelerated glacier retreat and intensified global warming, the identification and study of glacier lakes geomorphic features characterized by rapid change and pivotal environmental significance have assumed an increasingly urgent tenor. In such a context, bibliometrics emerges as a potent tool to assess the development of literature and research trends, furnishing invaluable insights for the glacier lake identification domain. Admittedly, the bibliometric approach employed in this study is not devoid of limitations. By unveiling these limitations, we can more accurately appraise the current research landscape within glacier lake identification and glean valuable insights to inform the design and methodologies of future research endeavors.
(1) We acknowledge that the utilization of WOSCC in this study, while a widely employed literature repository with abundant scholarly information, may still fall short in comprehensively encompassing all publications within the glacier lake identification domain. This could result in our analysis not fully encapsulating important literature from other databases or non-academic channels, such as CNKI and Scopus databases, thereby raising concerns about data incompleteness. Additionally, specific types of literature like books, doctoral theses, and patents, among others, might not be entirely incorporated in these databases, potentially influencing our grasp of the field’s entirety. Such gaps may emanate from the chosen literature search strategies and a subset of studies confined to English-language publications. Furthermore, errors or omissions could potentially arise during data retrieval and indexing processes within the WOS. Despite deploying well-formulated strategies for data retrieval and indexing using the WOS, we cannot guarantee the accurate classification and indexing of all relevant literature. Moreover, it is pertinent to recognize that the WOS primarily encompasses literature in the natural sciences, which could introduce a certain degree of bias in the glacier lake identification field, especially in interdisciplinary studies. This might lead to a partial interpretation of research within the glacier lake identification domain due to the potential omission of research from diverse disciplines.
(2) The analysis of countries and institutions carries inherent limitations. The countries and institutions contributing to the glacier lake identification field indicate that the United States, the United Kingdom, and China have the highest publication and citation counts, with each producing over 300 papers. From the perspective of the threat posed by glacial lake hazards, scientists from New Zealand and the UK have emphasized the significant challenges that global socio-economics faces due to GLOFs. Notably, in the high mountain Asia region alone, approximately one million people reside within a 10-kilometer radius of glacial lakes. The Himalayan region in Asia (including countries like India, Pakistan, and China) and the Andes region in South America (encompassing Peru and Bolivia) face even greater threats (Taylor et al., 2023). Regarding international collaboration, for instance, collaboration between the United States, the United Kingdom, and China has shown deeper engagement, primarily spanning Europe, North America, and East Asia. However, the level of international collaboration among South American and South Asian nations remains relatively low, exemplified by countries such as India, Pakistan, Peru, and Bolivia. This phenomenon underscores the close connection between research activities in the glacier lake identification field and the economic capabilities of each country. Developed nations, owing to their resource and technological advantages, are more inclined to invest in specialized fields like glacier lake identification within the realm of scientific research. On the other hand, developing countries may allocate resources to more pressing issues. In light of these considerations, it is essential to recognize that the aforementioned analysis, while informative, should be interpreted with the understanding that the distribution of research activities is influenced by complex economic and regional dynamics.
(3) There are certain limitations to the co-citation analysis employed in this study. Co-citation clustering, as a quantitative analytical tool, holds significant advantages in revealing citation relationships and trends in research fields. However, it is important to acknowledge the following challenges: Firstly, co-citation clustering heavily relies on citation relationships between documents, but these relationships may not always accurately reflect the actual knowledge structure within the research field. Citation relationships can be influenced by various factors such as citation practices and the evolution of citation networks. Consequently, this method may suffer from citation bias, potentially affecting the accuracy and reliability of clustering outcomes. Secondly, co-citation clustering primarily utilizes citation data for analysis, often overlooking the semantic content of the documents. This oversight could lead to the erroneous aggregation of documents from different topics or research areas due to citation relationships, resulting in misleading clustering results. Furthermore, the co-citation clustering method typically neglects the temporal factor’s impact on citation relationships. Research fields evolve and develop over time, yet this method may not fully account for the dynamic changes in citation relationships across different periods. As a result, the method might provide an incomplete understanding of research trends and evolution. In conclusion, while co-citation analysis offers valuable insights, its limitations highlight the need for a comprehensive approach that integrates multiple analytical methods to obtain a more accurate and nuanced perspective on the development and trends within the glacier lake identification field.
This study leveraged the strengths of software tools such as VOSviewer 1.6.19 and CiteSpace 6.1.R6 to employ bibliometric and quantitative analysis methods. Focusing on glacier lake identification as the research theme, an in-depth visual analysis was conducted on 2,121 publications from the WOSCC database spanning the years 1991 to 2023. This analysis encompassed research progress and trends across different periods, national collaboration networks, thematic evolution pathways, and more, providing a comprehensive overview of the field’s developmental trajectory, shortcomings, and prospective research directions. Several significant research findings were derived from this investigation.
(1) Regarding the annual distribution of publications and citation counts, both indicators exhibit a clear trend of rapid growth, signaling the increasing scholarly attention toward the field of glacier lake identification. From a national or regional perspective, the involvement of countries, journals, and institutional affiliations in the glacier lake identification domain is predominantly concentrated in Europe and North America. Notably, the United States, the United Kingdom, and China stand at the forefront in terms of publication volume, citation impact, and international collaboration efforts.
(2) Literature co-citation analysis focuses on the citation relationships between documents, serving as a means to assess and analyze the influence, significance, and collaborative networks of research literature within the academic community. Research findings indicate that significant clusters of research topics in the glacier lake identification domain include “debris-covered glaciers”, “glacial lake outburst flood”, “climate”, and “glacial lake”. Based on the analysis of the most frequently co-cited documents, research hotspots in the field encompass climate change, compilation of glacier lake inventories, methodologies for risk assessment, GLOFs, strategies for comprehensive disaster management, and hydrodynamic modeling. Notably, Switzerland and the Eastern Himalayas emerged as prominent focal areas in the realm of glacier lake identification.
(3) A detailed analysis of scientific categories and thematic clusters unveils the diversity and interdisciplinary nature of the glacier lake identification domain. Research in this field spans multiple scientific categories, primarily stemming from “Chemistry & Physics” and “Ecology and Environmental Science & Technology.” Noteworthy specific disciplines encompassed are “spectroscopy”, “environmental sciences”, “ecology,” “computer science, interdisciplinary applications”, “engineering, multidisciplinary”, and “mathematical & computational biology”.
(4) The analysis of keyword timelines and burst terms has unveiled the developmental trajectory of the glacier lake identification field over the past 32 years. Themes such as “hazard”, “Late Pleistocene”, “environmental change”, “ice sheet”, and “lake sediments” have sustained heightened interest over an extended temporal span. The evolution of research themes can be distinctly categorized into three phases: Firstly, during the period from 1991 to 2010, which marks the nascent stage of glacier lake identification research, numerous novel concepts emerged. Research orientations exhibited a degree of dispersion, accompanied by a prevalence of scientific queries warranting long-term investigation. Key emergent terms encompassed “Canada”, “record”, “Younger Drya”, “vegetation history”, “Lake Vostok”, and “calibration” among others. Research themes centered on “glacial lake formation and evolution”, “exploration and research of paleoclimate and paleoenvironment”, and “lake sediment analysis”. Secondly, spanning from 2011 to 2018, this phase witnessed a deeper enrichment and expansion of the glacier lake identification domain. Bursting keywords included “catastrophic drainage”, “Pleistocene”, “glacial lake”, “subglacial lake”, “flood”, “stream”, “volume”, and “conservation”. Research themes predominantly encompassed “glacial lake hazards and risk assessment” as well as “glacial lake dynamics”. Thirdly, the phase from 2019 to the present has seen the glacier lake identification field assume a progressively specialized trajectory, with a rapid escalation in annual publications and citations. Eminent keywords during this period comprise “inventory”, “glacial lake outburst flood”, “risk”, “dynamics”, “Tibetan Plateau”, “evolution”, and “high mountain Asia”. Noteworthy appearances also include “Himalaya”, “moraine dammed lake”, “evolution”, “permafrost”, and “precipitation”. The focal literature gravitates toward research avenues such as “utilizing remote sensing techniques for the compilation of Qinghai-Tibet Plateau lake inventories”, “glacial lake outburst flood risk”, “precipitation variation and glacial lake response”, and “evolution of glacial lakes in high mountain Asia”.
(5) Building upon the synthesis of preceding research accomplishments, further exploration into the challenges inherent in glacier lake identification methodologies and techniques, as well as the dimensionality of recognition scales, has been undertaken. This endeavor is then complemented by a prospective delineation of forthcoming research trajectories within the glacier lake identification domain. Noteworthy among these trajectories are the “recognition methods based on machine learning and deep learning”, the “integration of multi-source data fusion datasets”, the “emergence of novel algorithms and techniques tailored for scale transformation and data expansion”, alongside the “advancement of remote sensing technologies with elevated spatial resolution”.
The original contributions presented in the study are included in the article/supplementary material. Further inquiries can be directed to the corresponding author.
ZL: Methodology, Software, Visualization, Writing – review & editing. ZY: Funding acquisition, Project administration, Supervision, Writing – original draft. NH: Writing – original draft, Data curation. LW: Writing – original draft. YZ: Writing – original draft. WJ: Writing – original draft. ZW: Writing – original draft. TZ: Writing – original draft. JZ: Writing – original draft. XZ: Writing – original draft.
The author(s) declare financial support was received for the research, authorship, and/or publication of this article. This research was funded by the National Natural Science Foundation of China (Grant No. 41861134008), the Muhammad Asif Khan academician workstation of Yunnan Province (Grant No. 202105AF150076), the Key R&D Program of Yunnan Province (Grant No. 202003AC100002), and the General Program of basic research plan of Yunnan Province (Grant No. 202001AT070043).
The authors declare that the research was conducted in the absence of any commercial or financial relationships that could be construed as a potential conflict of interest.
All claims expressed in this article are solely those of the authors and do not necessarily represent those of their affiliated organizations, or those of the publisher, the editors and the reviewers. Any product that may be evaluated in this article, or claim that may be made by its manufacturer, is not guaranteed or endorsed by the publisher.
Abbas G., Murtaza B., Bibi I., Shahid M., Niazi N. K., Khan M. I., et al. (2018). Arsenic uptake, toxicity, detoxification, and speciation in plants: physiological, biochemical, and molecular aspects. Int. J. Environ. Res. Public Health 15 (1), 59. doi: 10.3390/ijerph15010059
Aggarwal A., Jain S. K., Lohani A. K., Jain N. (2016). Glacial lake outburst flood risk assessment using combined approaches of remote sensing, GIS and dam break modelling. Geomat. Nat. Haz. Risk. 7 (1), 18–36. doi: 10.1080/19475705.2013.862573
Aggarwal S., Rai S. C., Thakur P. K., Emmer A. (2017). Inventory and recently increasing GLOF susceptibility of glacial lakes in Sikkim, Eastern Himalaya. Geomorphology. 295, 39–54. doi: 10.1016/j.geomorph.2017.06.014
Ahmed R., Ahmad S. T., Wani G. F., Mir R. A., Ahmed P., Jain S. K. (2022c). High resolution inventory and hazard assessment of potentially dangerous glacial lakes in upper Jhelum basin, Kashmir Himalaya, India. Geocarto Int. 37, 10681–10712. doi: 10.1080/10106049.2022.2038693
Ahmed R., Ahmad S. T., Wani G. F., Mir R. A., Almazroui M., Bansal J. K., et al. (2022b). Glacial lake changes and the identification of potentially dangerous glacial lakes (PDGLs) under warming climate in the Dibang River Basin, Eastern Himalaya, India. Geocarto Int. 37 (27), 17659–17685. doi: 10.1080/10106049.2022.2134461
Ahmed R., Rawat M., Wani G. F., Ahmad S. T., Ahmed P., Jain S. K., et al. (2022a). Glacial lake outburst flood hazard and risk assessment of gangabal lake in the upper jhelum basin of kashmir himalaya using geospatial technology and hydrodynamic modeling. Remote Sens. 14, 5957. doi: 10.3390/rs14235957
Ali S. N., Pandey P., Singh P., Mishra S., Shekhar M., Misra K. G., et al. (2023). Intimidating evidences of climate change from the higher himalaya: A case study from lahaul, himachal pradesh, India. J. Indian Soc Remote Sens. 51 (5), 1099–1112. doi: 10.1007/s12524-023-01686-0
Allen S. K., Rastner P., Arora M., Huggel C., Stoffel M. (2016). Lake outburst and debris flow disaster at Kedarnath, June 2013: hydrometeorological triggering and topographic predisposition. Landslides. 13 (6), 1479–1491. doi: 10.1007/s10346-015-0584-3
Allen S. K., Sattar A., King O., Zhang G., Bhattacharya A., Yao T., et al. (2022). Glacial lake outburst flood hazard under current and future conditions: worst-case scenarios in a transboundary Himalayan basin. Nat. Hazards Earth Syst. Sci. 22 (11), 3765–3785. doi: 10.5194/nhess-22-3765-2022
Allen S. K., Zhang G., Wang W., Yao T., Bolch T. (2019). Potentially dangerous glacial lakes across the Tibetan Plateau revealed using a large-scale automated assessment approach. Sci. Bull. 64, 435–445. doi: 10.1016/j.scib.2019.03.011
Aranbarri J. K., Gonzalez-samperiz P., Valero-garces B., Moreno A., Gil-Romera G., Sevilla-Callejo M., et al. (2014). Rapid climatic changes and resilient vegetation during the Lateglacial and Holocene in a continental region of south-western Europe. Global Planet. Change. 114, 50–65. doi: 10.1016/j.gloplacha.2014.01.003
Awal R., Nakagawa H., Kawaike K., Baba Y., Zhang H. (2011). Study on moraine dam failure and resulting flood/debris flow hydrograph due to waves overtopping and erosion. Ital. J. Eng. Geol. Env. 2011, 3–12. doi: 10.4408/IJEGE.2011-03.B-001
Bajracharya S. R., Mool P. (2009). Glaciers, glacial lakes and glacial lake outburst floods in the Mount Everest region, Nepal. Ann. Glaciol. 50 (53), 81–86. doi: 10.3189/172756410790595895
Barrell D. J., Almond P. C., Vandergoes M., Lowe D. J., Newnham R. M. (2013). A composite pollen-based stratotype for inter-regional evaluation of climatic events in New Zealand over the past 30,000 years (NZ-INTIMATE project). Quat. Sci. Rev. 74, 4–20. doi: 10.1016/j.quascirev.2013.04.002
Bazilova V., Kääb A. (2022). Mapping area changes of glacial lakes using stacks of optical satellite images. Remote Sens. 14 (23), 5973–5973. doi: 10.3390/rs14235973
Begam S., Sen D. (2019). Mapping of moraine dammed glacial lakes and assessment of their areal changes in the central and eastern himalayas using satellite data. J. Mt. Sci. 16, 77–94. doi: 10.1007/s11629-018-5023-1
Benn D. I., Bolch T., Hands K. A., Gulley J. D., Luckman A. J., Nicholson L. I., et al. (2012). Response of debris-covered glaciers in the Mount Everest region to recent warming, and implications for outburst flood hazards. Earth Sci. Rev. 114 (1-2), 156–174. doi: 10.1016/j.earscirev.2012.03.008
Blaauw M. (2010). Methods and code for 'classical' age-modelling of radiocarbon sequences. Quat. Geochronol. 5 (5), 512–518. doi: 10.1016/j.quageo.2010.01.002
Blockley S. P. E., Lane C. S., Hardiman M., Rasmussen S. O., Seierstad I. K., Steffensen J. P., et al. (2012). Synchronisation of palaeoenvironmental records over the last 60,000 years, and an extended INTIMATE(1) event stratigraphy to 48,000 b2k. Quat. Sci. Rev. 36, 2–10. doi: 10.1016/j.quascirev.2011.09.017
Bolch T., Bolch T., Kulkarni A. V., Kääb A., Huggel C., Huggel C., et al. (2012). The state and fate of himalayan glaciers. Science. 336 (6079), 310–314. doi: 10.1126/science.1215828
Bolch T., Peters J., Yegorov A., Pradhan B., Buchroithner M. F., Blagoveshchensky V. A. (2011). Identification of potentially dangerous glacial lakes in the northern Tien Shan. Nat. Hazard. 59 (3), 1691–1714. doi: 10.1007/s11069-011-9860-2
Bondi M., Cacchiani S. (2021). Knowledge communication and knowledge dissemination in a digital world. J. Pragmat. 186, 117–123. doi: 10.1016/j.pragma.2021.10.003
Byers A. C., Chand M. B., Lala J., Shrestha M., Byers E. A., Watanabe T. (2020). Reconstructing the history of glacial lake outburst floods (GLOF) in the kanchenjunga conservation area, east Nepal: an interdisciplinary approach. Sustainability. 12, 5407. doi: 10.3390/su12135407
Carey M., Huggel C., Bury J. T., Portocarrero C., Haeberli W. (2012). An integrated socio-environmental framework for glacier hazard management and climate change adaptation: lessons from Lake 513, Cordillera Blanca, Peru. Clim. Change. 112 (3-4), 733–767. doi: 10.1007/s10584-011-0249-8
Carrivick J. L., Tweed F. S. (2013). Proglacial lakes: character, behaviour and geological importance. Quat. Sci. Rev. 78, 34–52. doi: 10.1016/j.quascirev.2013.07.028
Carrivick J. L., Tweed F. S. (2016). A global assessment of the societal impacts of glacier outburst floods. Global Planet. Change. 144, 1–16. doi: 10.1016/j.gloplacha.2016.07.001
Chen C. (2006). CiteSpace II: Detecting and visualizing emerging trends and transient patterns in scientific literature. J. Am. Soc Inf. Sci. Technol. 57 (3), 359–377. doi: 10.1002/asi.20317
Clark P. U., Dyke A. S., Shakun J. D., Carlson A. E., Clark J., Wohlfarth B., et al. (2009). The last glacial maximum. Science. 325 (5941), 710–714. doi: 10.1126/science.1172873
Compagno L., Huss M., Zekollari H., Miles E. S., Farinotti D. (2022). Future growth and decline of high mountain Asia's ice-dammed lakes and associated risk. Commun. Earth Environ. 3 (1), 191. doi: 10.1038/s43247-022-00520-8
Cook K. L., Andermann C., Gimbert F., Adhikari B. R., Hovius N. (2018). Glacial lake outburst floods as drivers of fluvial erosion in the Himalaya. Science. 362, 53–57. doi: 10.1126/science.aat4981
Cook S. J., Quincey D. J. (2015). Estimating the volume of Alpine glacial lakes. Earth Surf. Dyn. 3 (4), 559–575. doi: 10.5194/esurf-3-559-2015
Corbett L. B., Munroe J. S. (2010). Investigating the influence of hydrogeomorphic setting on the response of lake sedimentation to climatic changes in the Uinta Mountains, Utah, USA. J. Paleolimnol. 44 (1), 311–325. doi: 10.1007/s10933-009-9405-9
Dahlquist M. P., West A. J. (2022). The imprint of erosion by glacial lake outburst floods in the topography of central Himalayan rivers. Earth Surf. Dyn. 10 (4), 705–722. doi: 10.5194/esurf-10-705-2022
Dhote P. R., Thakur P. K., Chouksey A., Srivastav S. K., Raghvendra S., Rautela P., et al. (2022). Synergistic analysis of satellite, unmanned aerial vehicle, terrestrial laser scanner data and process-based modelling for understanding the dynamics and morphological changes around the snout of Gangotri Glacier, India. Geomorphology. 396, 108005. doi: 10.1016/j.geomorph.2021.108005
Dømgaard M., Kjeldsen K. K., Huiban F., Carrivick J. L., Khan S. A., Bjørk A. A. (2023). Recent changes in drainage route and outburst magnitude of the Russell Glacier ice-dammed lake, West Greenland. Cryosphere. 17, 1373. doi: 10.5194/tc-17-1373-2023
Dou X., Fan X., Wang X., Yunus A. P., Xiong J., Tang R., et al. (2023). Spatio-temporal evolution of glacial lakes in the tibetan plateau over the past 30 years. Remote Sens. 15, 416. doi: 10.3390/rs15020416
Duan H. Y., Yao X. J., Zhang Y., Wang Q., Du Z. S., Hu J. Y., et al. (2023). Lake volume and potential hazards of moraine-dammed glacial lakes-a case study of Bienong Co, southeastern Tibetan Plateau. Cryosphere. 17 (2), 591–616. doi: 10.5194/tc-17-591-2023
Dwivedi S. K., Chandra N., Bahuguna S., Pandey A., Khanduri S., Lingwal S., et al. (2022). Hydrometeorological disaster risk assessment in upper Gori-Ramganga catchment, Uttarakhand, India. Geocarto Int. 37 (26), 11998–12013. doi: 10.1080/10106049.2022.2063403
Emmer A. (2018). GLOFs in the WOS: bibliometrics, geographies and global trends of research on glacial lake outburst floods (Web of Science 1979-2016). Nat. Hazards Earth Syst. Sci. 18 (3), 813–827. doi: 10.5194/nhess-18-813-2018
Emmer A. (2023). Vanishing evidence? On the longevity of geomorphic GLOF diagnostic features in the Tropical Andes. Geomorphology. 422, 108552. doi: 10.1016/j.geomorph.2022.108552
Emmer A., Curin V. (2021). . Can a dam type of an alpine lake be derived from lake geometry? A negative result. J. Mountain Sci. 18 (3), 614–621. doi: 10.1007/s11629-020-6003-9
Emmer A., Harrison S., Mergili M., Allen S., Frey H., Huggel C. (2020). 70 years of lake evolution and glacial lake outburst floods in the Cordillera Blanca (Peru) and implications for the future. Geomorphology. 365, 107178. doi: 10.1016/j.geomorph.2020.107178
Emmer A., Merkl S., Mergili M. (2015). Spatiotemporal patterns of high-mountain lakes and related hazards in western Austria. Geomorphology. 246, 602–616. doi: 10.1016/j.geomorph.2015.06.032
Frydrych M., Rdzany Z. (2022). Glacial outburst flood in the marginal zone of the Wartanian Glaciation: An example from Adamów, central Poland. Quat. Int. 617, 21–39. doi: 10.1016/j.quaint.2021.08.014
Gao Y. P., Yang W. G., Guo R., Jiang L. M. (2023). Remote sensing monitoring and analysis of jinwuco lateral moraine landslide-glacial lake outburst in southeast tibet. Remote. Sens. 15, 1475. doi: 10.3390/rs15061475
Gardelle J., Arnaud Y., Berthier E. (2011). Contrasted evolution of glacial lakes along the Hindu Kush Himalaya mountain range between 1990 and 2009. Global Planet. Change. 75 (1-2), 47–55. doi: 10.1016/j.gloplacha.2010.10.003
Gu C. J., Li S. J., Liu M., Hu K. L., Wang P. (2023). Monitoring glacier lake outburst flood (GLOF) of lake merzbacher using dense chinese high-resolution satellite images. Remote Sens. 15 (7), 1941. doi: 10.3390/rs15071941
Hao T., Jing L. W., Liu J. S., Wang D. L., Feng T. T., Zhao A. G., et al. (2023). Automatic detection of subglacial water bodies in the AGAP region, east Antarctica, based on short-time fourier transform. Remote Sens. 15 (2), 363. doi: 10.3390/rs15020363
Harrison S., Smith D. E., Glasser N. F. (2019). Late Quaternary meltwater pulses and sea level change. J. Quat. Sci. 34 (1), 1–15. doi: 10.1002/jqs.3070
Hata S., Sugiyama S., Heki K. (2022). Abrupt drainage of Lago Greve, a large proglacial lake in Chilean Patagonia, observed by satellite in 2020. Commun. Earth Environ. 3 (1), 190. doi: 10.1038/s43247-022-00531-5
He Y., Yao S., Yang W., Yan H. W., Zhang L. F., Wen Z. Q., et al. (2021). An extraction method for glacial lakes based on landsat-8 imagery using an improved U-net network. IEEE J. Sel. Top. Appl. Earth Obs. Remote Sens. 14, 6544–6558. doi: 10.1109/jstars.2021.3085397
Hengst A. M., Armstrong W. H., Rick B., McGrath D. (2021). Short-term variability in alaska ice-marginal lake area: implications for long-term studies. Remote Sens. 13 (19), 3955. doi: 10.3390/rs13193955
Ivy-Ochs S., Kerschner H., Maisch M., Christl M., Kubik P. W., Schlüchter C. (2009). Latest Pleistocene and Holocene glacier variations in the European Alps. Quat. Sci. Rev. 28 (21-22), 2137–2149. doi: 10.1016/j.quascirev.2009.03.009
Jiang L. Y., Fan X. M., Deng Y., Zou C. B., Feng Z. T., Djukem D. L. W., et al. (2023). Combining geophysics, remote sensing and numerical simulation to assess GLOFs: Case study of the Namulacuo Lake in the Southeastern Tibetan Plateau. Sci. Total Environ. 880, 163262. doi: 10.1016/j.scitotenv.2023.163262
Kaushik S., Singh T. S., Joshi P. K., Dietz A. J. (2022). Automated mapping of glacial lakes using multisource remote sensing data and deep convolutional neural network. Int. J. Appl. Earth Obs. Geoinf. 115, 103085. doi: 10.1016/j.jag.2022.103085
Kellerer-Pirklbauer A., Avian M., Benn D. I., Bernsteiner F., Krisch P., Ziesler C. (2021). Buoyant calving and ice-contact lake evolution at Pasterze Glacier (Austria) in the period 1998-2019. Cryosphere. 15 (3), 1237–1258. doi: 10.5194/tc-15-1237-2021
King O., Dehecq A., Quincey D. J., Carrivick J. L. (2018). Contrasting geometric and dynamic evolution of lake and land-terminating glaciers in the central Himalaya. Global Planet. Change. 167, 46–60. doi: 10.1016/j.gloplacha.2018.05.006
Knox J. C. (1996). Late quaternary Upper Mississippi River alluvial episodes and their significance to the Lower Mississippi River system. Eng. Geol. 45 (1-4), 263–285. doi: 10.1016/s0013-7952(96)00017-8
Kumar M., Al-quraishi A. M. F., Mondal I. (2021). . Glacier changes monitoring in Bhutan High Himalaya using remote sensing technology. Environ. Eng. Res. 26 (1), 38–50. doi: 10.4491/eer.2019.255
Lencioni V. (2018). Glacial influence and stream macroinvertebrate biodiversity under climate change: Lessons from the Southern Alps. Sci. Total Environ. 622, 563–575. doi: 10.1016/j.scitotenv.2017.11.266
Lesi M., Nie Y., Shugar D. H., Wang J. D., Deng Q. H., Chen H. Y. (2022). Landsat- and Sentinel-derived glacial lake dataset in the China-Pakistan Economic Corridor from 1990 to 2020. Earth Syst. Sci. Data. 14 (12), 5489–5512. doi: 10.5194/essd-14-5489-2022
Leydesdorff L., Carley S., Rafols I. (2013). Global maps of science based on the new Web-of-Science categories. Scientometrics. 94 (2), 589–593. doi: 10.1007/s11192-012-0784-8
Leydesdorff L., Wagner C. S., Bornmann L. (2019). Interdisciplinarity as diversity in citation patterns among journals: Rao-Stirling diversity, relative variety, and the Gini coefficient. J. Informetr. 13 (1), 255–269. doi: 10.1016/j.joi.2018.12.006
Li J., Gu Y. Y., Wu L. X., Guo L., Xu H. D., Miao Z. L. (2022b). Changes in Glaciers and Glacial Lakes in the Bosula Mountain Range, Southeast Tibet, over the past Two Decades. Remote Sens. 14 (15), 3792. doi: 10.3390/rs14153792
Li J. J., Mao Y., Ouyang J. Y., Zheng S. N. (2022a). A review of urban microclimate research based on citeSpace and VOSviewer analysis. Int. J. Environ. Res. Public Health 19 (8), 4741. doi: 10.3390/ijerph19084741
Li J. L., Sheng Y. W. (2012). An automated scheme for glacial lake dynamics mapping using Landsat imagery and digital elevation models: a case study in the Himalayas. Int. J. Remote Sens. 33 (16), 5194–5213. doi: 10.1080/01431161.2012.657370
Li W. P., Wang W., Gao X., Wang X. C., Wang R. H. (2022c). Inventory and spatiotemporal patterns of glacial lakes in the HKH-TMHA region from 1990 to 2020. Remote Sens. 14 (6), 1351. doi: 10.3390/rs14061351
Liu W. S. (2019). The data source of this study is Web of Science Core Collection? Not enough. Scientometrics. 121 (3), 1815–1824. doi: 10.1007/s11192-019-03238-1
Liu Z. Q., Yang Z. Q., Chen M., Xu H. H., Yang Y., Zhang J., et al. (2023). Research hotspots and frontiers of mountain flood disaster: bibliometric and visual analysis. Water. 15 (4), 673. doi: 10.3390/w15040673
Lu H., Zheng D., Yang K., Yang F. (2020). Last-decade progress in understanding and modeling the land surface processes on the Tibetan Plateau. Hydrol. Earth Syst. Sci. 24 (12), 5745–5758. doi: 10.5194/hess-24-5745-2020
Ma S. Y., Qiu H. J., Zhu Y. R., Yang D. D., Tang B. Z., Wang D. Z., et al. (2023). Topographic changes, surface deformation and movement process before, during and after a rotational landslide. Remote Sens. 15 (3), 662. doi: 10.3390/rs15030662
Mansur V., Guimarães C., Carvalho M. S., Lima L. D., Coeli C. M. (2021). From academic publication to science dissemination. Cad. Saude Public. 37 (7), 140821. doi: 10.1590/0102-311x00140821
McKillop R. J., Clague J. J. (2007). Statistical, remote sensing-based approach for estimating the probability of catastrophic drainage from moraine-dammed lakes in Southwestern British Columbia. Global Planet. Change. 56 (1-2), 153–171. doi: 10.1016/j.gloplacha.2006.07.004
Medeiros A. S., Chipman M. L., Francis D. R., Hamerlík L., Langdon P., Puleo P. J. K., et al. (2022). A continental-scale chironomid training set for reconstructing Arctic temperatures. Quat. Sci. Rev. 294, 107728. doi: 10.1016/j.quascirev.2022.107728
Miles E. S., Willis I. C., Arnold N. S., Steiner J. F., Pellicciotti F. (2017). Spatial, seasonal and interannual variability of supraglacial ponds in the Langtang Valley of Nepal 1999-2013. J. Glaciol. 63 (237), 88–105. doi: 10.1017/jog.2016.120
Morriss B. F., Hawley R. L., Chipman J. W., Andrews L. C., Catania G. A., Hoffman M. J., et al. (2013). A ten-year record of supraglacial lake evolution and rapid drainage in West Greenland using an automated processing algorithm for multispectral imagery. Cryosphere. 7 (6), 1869–1877. doi: 10.5194/tc-7-1869-2013
Motschmann A., Huggel C., Carey M., Moulton H., Walker-Crawford N., Muñoz R. (2020). Losses and damages connected to glacier retreat in the Cordillera Blanca, Peru. Clim. Change. 162, 837–858. doi: 10.1007/s10584-020-02770-x
Mulsow C., Koschitzki R., Maas H. (2015). Photogrammetric monitoring of glacier margin lakes. Geomatics Nat. Hazards Risk. 6 (5-7), 600–613. doi: 10.1080/19475705.2014.939232
Nie Y., Liu Q., Wang J., Zhang Y., Sheng Y., Liu S. (2018). An inventory of historical glacial lake outburst floods in the Himalayas based on remote sensing observations and geomorphological analysis. Geomorphology. 308, 91–106. doi: 10.1016/j.geomorph.2018.02.002
Nie Y., Sheng Y. W., Liu Q., Liu L. S., Liu S. Y., Zhang Y. L., et al. (2017). A regional-scale assessment of Himalayan glacial lake changes using satellite observations from 1990 to 2015. Remote Sens. Environ. 189, 1–13. doi: 10.1016/j.rse.2016.11.008
Ogier C., Werder M. A., Huss M., Kull I., Hodel D., Farinotti D. (2021). Drainage of an ice-dammed lake through a supraglacial stream: hydraulics and thermodynamics. Cryosphere. 15 (11), 5133–5150. doi: 10.5194/tc-15-5133-2021
Pei Y. Q., Qiu H. J., Zhu Y. R., Wang J. D., Yang D. D., Tang B. Z., et al. (2023). Elevation dependence of landslide activity induced by climate change in the eastern Pamirs. Landslides. 20 (6), 1115–1133. doi: 10.1007/s10346-023-02030-w
Petrakov D. A., Tutubalina O. V., Aleinikov A. A., Chernomorets S. S., Evans S. G., Kidyaeva V. M., et al. (2012). . Monitoring of Bashkara Glacier lakes (Central Caucasus, Russia) and modelling of their potential outburst. Nat. Hazard. 61 (3), 1293–1316. doi: 10.1007/s11069-011-9983-5
Prakash C., Nagarajan R. (2018). Glacial lake changes and outburst flood hazard in Chandra basin, North-Western Indian Himalaya. Geomat. Nat. haz. risk. 9 (1), 337–355. doi: 10.1080/19475705.2018.1445663
Qi M., Liu S. Y., Wu K. P., Zhu Y., Xie F., Jin H., et al. (2022). Improving the accuracy of glacial lake volume estimation: A case study in the Poiqu basin, central Himalayas. J. Hydrol. 610, 127973. doi: 10.1016/j.jhydrol.2022.127973
Qiu H. J., Zhu Y. R., Zhou W. Q., Sun H. S., He J. Y., Liu Z. J. (2022). Influence of DEM resolution on landslide simulation performance based on the Scoops3D model. Geomat. Nat. Haz. Risk. 13 (1), 1663–1681. doi: 10.1080/19475705.2022.2097451
Qu G., Dai X. A., Cheng J. Y., Li W. L., Wang M. L., Liu W. X., et al. (2022). Characterization of long-time series variation of glacial lakes in southwestern tibet: A case study in the nyalam county. Remote Sens. 14 (19), 4688. doi: 10.3390/rs14194688
Rawat M., Jain S. K., Ahmed R., Lohani A. K. (2023). Glacial lake outburst flood risk assessment using remote sensing and hydrodynamic modeling: a case study of Satluj basin, Western Himalayas, India. Environ. Sci. pollut. Res. 30, 41591–41608. doi: 10.1007/s11356-023-25134-1
Rinzin S., Zhang G. Q., Sattar A., Wangchuk S., Allen S. K., Dunning S. A., et al. (2023). GLOF hazard, exposure, vulnerability, and risk assessment of potentially dangerous glacial lakes in the Bhutan Himalaya. J. Hydrol. 619, 129311. doi: 10.1016/j.jhydrol.2023.129311
Sattar A., Allen S. K., Mergili M., Haeberli W., Frey H., Kulkarni A. V., et al. (2023). Modeling potential glacial lake outburst flood process chains and effects from artificial lake-level lowering at gepang gath lake, Indian himalaya. J. Geophys. Res. Earth. Surf. 128, 3. doi: 10.1029/2022jf006826
Sattar A., Goswami A., Kulkarni A. V., Emmer A., Haritashya U. K., Allen S., et al. (2021). Future glacial lake outburst flood (GLOF) hazard of the south lhonak lake, sikkim himalaya. Geomorphology. 388, 107783. doi: 10.1016/j.geomorph.2021.107783
Sawi T., Holtzman B. K., Walter F., Paisley J. W. (2022). An unsupervised machine-learning approach to understanding seismicity at an alpine glacier. J. Geophys. Res.: Earth Surf. 127 (12), e2022JF006909. doi: 10.1029/2022jf006909
Shan Z., Li Z. D., Dong X. Y. (2021). Impact of glacier changes in the Himalayan Plateau disaster. Ecol. Inf. 63, 101316. doi: 10.1016/j.ecoinf.2021.101316
Shugar D. H., Burr A., Haritashya U. K., Kargel J. S., Watson C. S., Kennedy M. C., et al. (2020). Rapid worldwide growth of glacial lakes since 1990. Nat. Clim. Change. 10, 939–945. doi: 10.1038/s41558-020-0855-4
Singh V. K., Singh P., Karmakar M., Leta J., Mayr P. (2021). The journal coverage of Web of Science, Scopus and Dimensions: A comparative analysis. Scientometrics. 126 (6), 5113–5142. doi: 10.1007/s11192-021-03948-5
Singh H., Varade D. M., de Vries M. V., Adhikari K., Rawat M., Awasthi S., et al. (2023). Assessment of potential present and future glacial lake outburst flood hazard in the Hunza valley: A case study of Shisper and Mochowar glacier. Sci. Total Environ. 868, 161717. doi: 10.1016/j.scitotenv.2023.161717
Sionneau T., Bout-Roumazeilles V., Flower B. P., Bory A., Tribovillard N., Kissel C., et al. (2010). Provenance of freshwater pulses in the Gulf of Mexico during the last deglaciation. Quat. Res. 74 (2), 235–245. doi: 10.1016/j.yqres.2010.07.002
Steffen T., Huss M., Estermann R., Hodel E., Farinotti D. (2022). Volume, evolution, and sedimentation of future glacier lakes in Switzerland over the 21st century. Earth Surf. Dyn. 10 (4), 723–741. doi: 10.5194/asurf-10-723-2022
Taylor C. J., Carr J. R., Rounce D. R. (2022). Spatiotemporal supraglacial pond and ice cliff changes in the Bhutan–Tibet border region from 2016 to 2018. J. Glaciol. 68, 101–113. doi: 10.1017/jog.2021.76
Taylor C., Robinson T. R., Dunning S., Rachel C. J., Westoby M. (2023). Glacial lake outburst floods threaten millions globally. Nat. Commun. 14 (1), 487. doi: 10.1038/s41467-023-36033-x
Thakur P. K., Dixit A., Chouksey A., Aggarwal S. P., Kumar A. S. (2016). Ice sheet features identification, glacier velocity estimation and glacier zones classification using high resolution optical and SAR data. Asia-Pacific Remote. Sens. 9877, 19. doi: 10.1117/12.2224027
Thompson I., Shrestha M., Chhetri N., Agusdinata D. B. (2020). An institutional analysis of glacial floods and disaster risk management in the Nepal Himalaya. Int. J. Disaster Risk Reduct. 47, 101567. doi: 10.1016/j.ijdrr.2020.101567
Tomczyk A. M., Ewertowski M. W. (2021). Baseline data for monitoring geomorphological effects of glacier lake outburst flood: a very-high-resolution image and GIS datasets of the distal part of the Zackenberg River, northeast Greenland. Earth Syst. Sci. Data. 13 (11), 5293–5309. doi: 10.5194/essd-13-5293-2021
Veettil B. K., Kamp U. (2021). Glacial lakes in the andes under a changing climate: A review. J. Earth Sci. 32, 1–19. doi: 10.1007/s12583-020-1118-z
Veh G., Korup O., von Specht S., Roessner S., Walz A. (2019). Unchanged frequency of moraine-dammed glacial lake outburst floods in the Himalaya. Nat. Clim. Change. 9 (5), 379–383. doi: 10.1038/s41558-019-0437-5
Viani C., Colombo N., Bollati I. M., Mortara G., Perotti L. E., Giardino M. (2022). Socio-environmental value of glacier lakes: assessment in the Aosta Valley (Western Italian Alps). Reg. Environ. Change. 22 (1), 1–21. doi: 10.1007/s10113-021-01860-5
Viani C., Giardino M., Huggel C., Perotti L., Mortara G. (2016). An Overview of Glacier Lakes in the Western Italian Alps from 1927 to 2014 Based on Multiple Data Sources (historical maps, orthophotos and reports of the glaciological surveys). Geogr. Fis. Din. Quat. 39 (2), 203–214. doi: 10.4461/gfdq2016.39.19
Viani C., Machguth H., Huggel C., Godio A., Franco D., Perotti L., et al. (2020). Potential future lakes from continued glacier shrinkage in the Aosta Valley Region (Western Alps, Italy). Geomorphology 355:107068. doi: 10.1016/j.geomorph.2020.107068
Wang L. Y., Qiu H. J., Zhou W. Q., Zhu Y. R., Liu Z. J., Ma S. Y., et al. (2022). The post-failure spatiotemporal deformation of certain translational landslides may follow the pre-failure pattern. Remote Sens. 14 (10), 2333. doi: 10.3390/rs14102333
Wang W., Bai J. H., Zhang L., Jia J., Yu L., Zhang S. Y. (2021). Biochar modulation of the soil nitrogen cycle: a CiteSpace-based bibliometric study. J. Beijing Norm. Univ. Nat. Sci. 57 (1), 76–85. doi: 10.12202/j.0476-0301.2020438
Wang W. C., Yao T., Yang W., Joswiak D. R., Zhu M. L. (2012). Methods for assessing regional glacial lake variation and hazard in the southeastern Tibetan Plateau: a case study from the Boshula mountain range, China. Environ. Earth Sci. 67 (5), 1441–1450. doi: 10.1007/s12665-012-1589-z
Wang W. C., Zhang T. G., Yao T. D., An B. S. (2022). Monitoring and early warning system of Cirenmaco glacial lake in the central Himalayas. Int. J. Disaster Risk Reduct. 73, 102914. doi: 10.1016/j.ijdrr.2022.102914
Wang X., Chen G., Dai X. A., Zhao J. J., Liu X., Gao Y., et al. (2022). Improved process management of glacial lake outburst flood hazards by integrating modular monitoring, assessment, and simulation. Water Resour. Manage. 36 (7), 2343–2358. doi: 10.1007/s11269-022-03146-9
Wang X., Guo X. Y., Yang C. D., Liu Q. H., Wei J. F., Zhang Y., et al. (2020). Glacial lake inventory of high-mountain Asia in 1990 and 2018 derived from Landsat images. Earth Syst. Sci. Data. 12 (3), 2169–2182. doi: 10.5194/essd-12-2169-2020
Wangchuk S., Bolch T., Robson B. A. (2022). Monitoring glacial lake outburst flood susceptibility using Sentinel-1 SAR data, Google Earth Engine, and persistent scatterer interferometry. Remote Sens. Environ. 271, 112910. doi: 10.1016/j.rse.2022.112910
Wangchuk S., Bolch T., Zawadzki J. (2019). Towards automated mapping and monitoring of potentially dangerous glacial lakes in Bhutan Himalaya using Sentinel-1 Synthetic Aperture Radar data. Int. J. Remote Sens. 40 (12), 4642–4667. doi: 10.1080/01431161.2019.1569789
Williams R. D., Griffiths H. M., Carr J. R., Hepburn A. J., Gibson M., Williams J. J., et al. (2022). Integrating historical, geomorphological and sedimentological insights to reconstruct past floods: Insights from Kea Point, Mt. Cook Village, Aotearoa New Zealand. Geomorphology. 398, 108028. doi: 10.1016/j.geomorph.2021.108028
Worni R., Huggel C., Clague J. J., Schaub Y., Stoffel M. (2014). Coupling glacial lake impact, dam breach, and flood processes: A modeling perspective. Geomorphology. 224, 161–176. doi: 10.1016/j.geomorph.2014.06.031
Worni R., Huggel C., Stoffel M. (2013). Glacial lakes in the Indian Himalayas-From an area-wide glacial lake inventory to on-site and modeling based risk assessment of critical glacial lakes. Sci. Total Environ. 468-469, S71–S84. doi: 10.1016/j.scitotenv.2012.11.043
Yang L. Y., Lu Z., Zhao C. Y., Kim J., Yang C. S., Wang B. H., et al. (2022). Analyzing the triggering factors of glacial lake outburst floods with SAR and optical images: A case study in Jinweng Co, Tibet, China. Landslides. 19, 855–864. doi: 10.1007/s10346-021-01831-1
Yang Z. Q., Chen M., Zhang J., Ding P., He N., Yang Y. (2023a). Effect of initial water content on soil failure mechanism of loess mudflow disasters. Front. Ecol. Evol. 11. doi: 10.3389/fevo.2023.1141155
Yang Z. Q., Wei L., Liu Y. Q., He N., Zhang J., Xu H. H. (2023c). Discussion on the relationship between debris flow provenance particle characteristics, gully slope, and debris flow types along the karakoram highway. Sustainability. 15, 5998. doi: 10.3390/su15075998
Yang Z. Q., Xiong J. F., Zhao X. G., Meng X. R., Wang S. B., Li R., et al. (2023d). Column-hemispherical penetration grouting mechanism for newtonian fluid considering the tortuosity of porous media. Processes. 11, 1737. doi: 10.3390/pr11061737
Yang Z. Q., Zhao X. G., Chen M., Zhang J., Yang Y., Chen W. T., et al. (2023b). Characteristics, dynamic analyses and hazard assessment of debris flows in Niumiangou Valley of Wenchuan County. Appl. Sci. 13, 1161. doi: 10.3390/app13021161
Ye Q., Zhang X. Q., Wang Y. Z., Zhang Z., Hu Y. F., Zhu L. P. (2022). Monitoring glacier thinning rate in Rongbuk Catchment on the northern slope of Mt. Qomolangma from 1974 to 2021. Ecol. Indic. 144, 109418. doi: 10.1016/j.ecolind.2022.109418
Yu A. J., Shi H. L., Wang Y. F., Yang J., Gao C. C., Lu Y. (2023). A bibliometric and visualized analysis of remote sensing methods for glacier mass balance research. Remote Sens. 15 (5), 1425. doi: 10.3390/rs15051425
Zdorovennova G., Palshin N., Golosov S., Efremova T., Belashev B. Z., Bogdanov S., et al. (2021). Dissolved oxygen in a shallow ice-covered lake in winter: effect of changes in light, thermal and ice regimes. Water. 13 (17), 2435. doi: 10.3390/w13172435
Zhang D. H., Zhou G., Li W. D., Han L., Zhang S. Q., Yao X., et al. (2023). A robust glacial lake outburst susceptibility assessment approach validated by GLOF event in 2020 in the Nidu Zangbo Basin, Tibetan Plateau. Catena. 220, 106734. doi: 10.1016/j.catena.2022.106734
Zhang G. Q., Yao T. D., Xie H. J., Wang W. C., Yang W. (2015). An inventory of glacial lakes in the Third Pole region and their changes in response to global warming. Global Planet. Change. 131, 148–157. doi: 10.1016/j.gloplacha.2015.05.013
Zhang M. M., Chen F., Guo H. D., Yi L., Zeng J. Y., Li B. (2022c). Glacial lake area changes in high mountain asia during 1990-2020 using satellite remote sensing. Research. 2022, 9821275. doi: 10.34133/2022/9821275
Zhang T. G., Wang W. C., Gao T. G., An B. S., Yao T. D. (2022b). An integrative method for identifying potentially dangerous glacial lakes in the Himalayas. Sci. Total Environ. 806, 150442. doi: 10.1016/j.scitotenv.2021.150442
Zhang Y. Q., You X. L., Huang S., Wang M. H., Dong J. W. (2022a). Knowledge atlas on the relationship between water management and constructed wetlands-A bibliometric analysis based on CiteSpace. Sustainability. 14 (14), 8288. doi: 10.3390/su14148288
Zhao F. Y., Long D., Li X. D., Huang Q., Han P. (2022). Rapid glacier mass loss in the Southeastern Tibetan Plateau since the year 2000 from satellite observations. Remote Sens. Environ. 270, 112853. doi: 10.1016/j.rse.2021.112853
Zhao X. G., Yang Z. Q., Meng X. R., Wang S. B., Li R., Xu H. H., et al. (2023). Study on mechanism and verification of columnar penetration grouting of time-varying newtonian fluids. Processes. 11, 1151. doi: 10.3390/pr11041151
Keywords: glacial lake identification, bibliometric analysis, research focal points, climate change, remote sensing, GLOF
Citation: Liu Z, Yang Z, He N, Wei L, Zhu Y, Jiao W, Wang Z, Zhang T, Zhang J and Zou X (2023) Three decades of glacial lake research: a bibliometric and visual analysis of glacial lake identification. Front. Ecol. Evol. 11:1296111. doi: 10.3389/fevo.2023.1296111
Received: 18 September 2023; Accepted: 13 October 2023;
Published: 27 October 2023.
Edited by:
Haijun Qiu, Northwest University, ChinaCopyright © 2023 Liu, Yang, He, Wei, Zhu, Jiao, Wang, Zhang, Zhang and Zou. This is an open-access article distributed under the terms of the Creative Commons Attribution License (CC BY). The use, distribution or reproduction in other forums is permitted, provided the original author(s) and the copyright owner(s) are credited and that the original publication in this journal is cited, in accordance with accepted academic practice. No use, distribution or reproduction is permitted which does not comply with these terms.
*Correspondence: Zhiquan Yang, eXpxMTk4MzgxNkBrdXN0LmVkdS5jbg==; Na He, aG42MTg4NkAxNjMuY29t; Yingyan Zhu, emhfeV95QGltZGUuYWMuY24=
Disclaimer: All claims expressed in this article are solely those of the authors and do not necessarily represent those of their affiliated organizations, or those of the publisher, the editors and the reviewers. Any product that may be evaluated in this article or claim that may be made by its manufacturer is not guaranteed or endorsed by the publisher.
Research integrity at Frontiers
Learn more about the work of our research integrity team to safeguard the quality of each article we publish.