- 1Department of Biology, Trent University, Peterborough, ON, Canada
- 2School of the Environment, Washington State University, Pullman, WA, United States
Interspecific competition is notoriously difficult to detect and quantify, especially in species that are wide-ranging or otherwise difficult to track in the wild. Research investigating interspecific competition usually relies on niche overlap measurements despite that this approach alone does not yield rigorous inference. As an illustration, we review published research assessing interspecific competition in mid-sized carnivores in North America (bobcat – Lynx rufus; Canada lynx – Lynx canadensis; coyote – Canis latrans), and report on shortcomings associated with commonly used study designs and types of inference. Niche overlap measurements typically focus on one or two resources (e.g., food, space, habitat), often using non-independent sampling units and inadequate replication. Few studies measure overlap variation through space, time, or resource variability, which is crucial for robust assessment. Niche overlap (or lack thereof) is used as evidence both for and against interspecific competition, reflecting a weak link between competition theory, predicted responses, and observations. Overall, challenges associated with conducting competition research in the field promote over-reliance on simple measurements, flawed study designs and weak inference. Minimally, niche overlap studies should include assessment across multiple niche dimensions and spatial or temporal variation in competitor density or resource availability. Dynamic investigative approaches should include new technologies for tracking inter-individual interactions, study designs that leverage quasi-experiments (e.g., decline in shared resources, biological control of one competitor), and synthetic analyses (e.g., meta-regression). Ultimately, better understanding of competition theory vis-à-vis study design and data needs will promote improved understanding of the role of interspecific competition in nature.
Introduction
Interspecific competition, defined here as the shared use of a limited resource (Abrams, 2022), is widely recognized as a primary force shaping ecological communities. Competitive interactions can be exploitative, involving indirect interactions over resources (usually food, space, or habitat), causing limitation in per capita resource availability and potential physical or niche-related displacement or death (Schoener, 1983). Alternatively, agonistic or interference competition arises when individuals interact directly through strife and exert physical displacement, avoidance, injury, or death (Case and Gilpin, 1974).
Conclusively demonstrating competitive relationships in nature has been a longstanding challenge (Connor and Simberloff, 1986; Keddy, 1989). For example, if two species have overlapping food, habitat, or space use, does this mean that they actively compete or that they do not compete but rather co-exist on abundant resources? Can interspecific competition be detected using data from a single set of observations in space and time, or is replication or other ancillary information necessary for conclusive assessment? These questions have spurred much discussion over the prevalence and intensity of competition in natural systems, and how best to test for it (Diamond, 1986; Gurevitch et al., 1992; Abrams, 2022). Currently, the prevailing viewpoint that interspecific competition is common and important in shaping communities and ecosystems (e.g., Denno et al., 1995; Kaplan and Denno, 2007; Cusumano et al., 2016) is supported by evidence ranging in quality, quantity, and relevance, depending on the species and system under investigation and the type of study design and data collected in the assessment (Blanchet et al., 2020; Salinas-Ramos et al., 2020; Watz and Nyqvist, 2022). At one end of the spectrum, plants and sessile invertebrate animals are well-suited for interspecific competition research and can support rigorous laboratory or field tests, including being amenable to controlled field experiments and evaluating the broader role of competition on population dynamics and community structure (Denno et al., 1995; Aschehoug et al., 2016). Indeed, much foundational theory and empirical testing for interspecific competition occurred decades ago, during the golden age of ecological field experiments involving a variety of (mostly sessile) model organisms (Connell, 1983; Schoener, 1983; Chesson, 2000). Since then developments in competition theory and corresponding field-based assessment have languished, including a lack of research involving realistic species interactions in organisms that naturally occur at low density, are wide-ranging, or are otherwise difficult to study because of logistical or ethical challenges (Abrams, 2022). These gaps limit our understanding of the prevalence, magnitude, and drivers of interspecific competition in nature.
Here, we review study designs used to assess interspecific competition in free-ranging animals and evaluate levels of inference provided by niche overlap measurement, the predominant investigative approach. As a model system, we use published research on mid-sized North American carnivores; bobcat (Lynx rufus), Canada lynx (Lynx canadensis), and coyote (Canis latrans), which are sympatric across small (bobcat–lynx) and large (bobcat–coyote, lynx–coyote) portions of their geographic range (Anderson and Lovallo, 2003; Bekoff and Gese, 2003). Bobcats occur across most of southern and central North America where they occupy a variety of habitats and rely on diverse prey types. Lynx occur almost exclusively in the northern portion of North America where they rely on snowshoe hares (Lepus americanus) as primary prey. Over the last two centuries, coyotes expanded their historical range from the North American prairies to now encompass most of the continent, where they exhibit broad dietary and habitat tolerance (Bekoff and Gese, 2003). There is a general sense that coyotes compete directly with bobcats and lynx (Litvaitis, 1992; Buskirk et al., 2000; Anderson and Lovallo, 2003) and that bobcats may displace lynx (Parker et al., 1983; Hoving et al., 2003). However, reliable evidence of interspecific competition in these species is scant (Murray et al., 2008), leaving open the possibility that weak study design and supporting data contribute to this uncertainty. Thus, our understanding of competition among mid-sized North American carnivores, and species with low tractability in general, is likely on weak footing.
Study designs to assess competition
Interspecific competition research falls into in 3 broad categories: descriptive, experimental, and comparative (Keddy, 1989). Descriptive studies are mainly comprised of point-in-time observations of species interactions, including evidence of mortality, strife, or active displacement. Such studies are typically opportunistic and supported by anecdotal evidence, with limited emphasis on study design. Experimental studies involve direct manipulation of competitor numbers or variation in abundance of a shared limiting resource (Connor and Simberloff, 1986), although the latter approach is rare. Field experiments measure numerical responses or niche shifts in relation to manipulation, and are conducted as academic exercises or else can be by-products of human intervention like commercial harvest or biological control of a competitor species. Experimental manipulations offer a powerful, direct measure of interspecific competition albeit possibly with limited generalizability of their findings. Because field experiments can disturb natural ecosystems and face substantive logistical, financial, and ethical challenges, they are increasingly rare (Diamond, 1986; Rockwood, 2006).
Most contemporary efforts to study interspecific competition in free-ranging animals are comparative studies that assess niche dimensions and/or overlap (e.g., food, space, and habitat) for co-occurring species in regions of sympatry. Using niche overlap to assess competition usually rests on the assumption of competitive exclusion, where species with marked overlap in one or more niche dimension (i.e., resource type) actively compete when the resource in question is limiting (Abrams, 2022). In theory, niche overlap should be transitory, with long-term co-existence on the same resource not being possible and the inferior competitor eventually succumbing to niche displacement or extirpation. However, niche overlap may be longstanding if competition is weak or if the species are in a competitive stalemate (Chesson, 2000; Adler et al., 2007), as may arise if resources are abundant or if intraspecific competition imposes especially strong population regulation (Connell, 1983; Adler et al., 2018). Further, species may exhibit niche overlap without competition simply by virtue of living in a shared environment (e.g., Holt and Bonsall, 2017; Blanchet et al., 2020). This means that species with overlapping niches may: i) actively compete but be in transition to a state with less/no competition, ii) compete in a stalemate, or iii) co-exist without competition (Figure 1). Likewise, species without niche overlap may not compete owing to different resource use, or else may: i) compete exploitatively in an unmeasured niche dimension or ii) compete agonistically rather than exploitatively (Figure 1). It is also possible that species co-exist without niche overlap, owing to past competition (i.e., ghost of competition past, sensu Connell, 1980). Accordingly, competition theory predicts a variety of possible levels of niche overlap depending on the context of the interspecific interaction, meaning that appropriate study designs and careful inference are needed to distinguish between these complex and subtle possibilities.
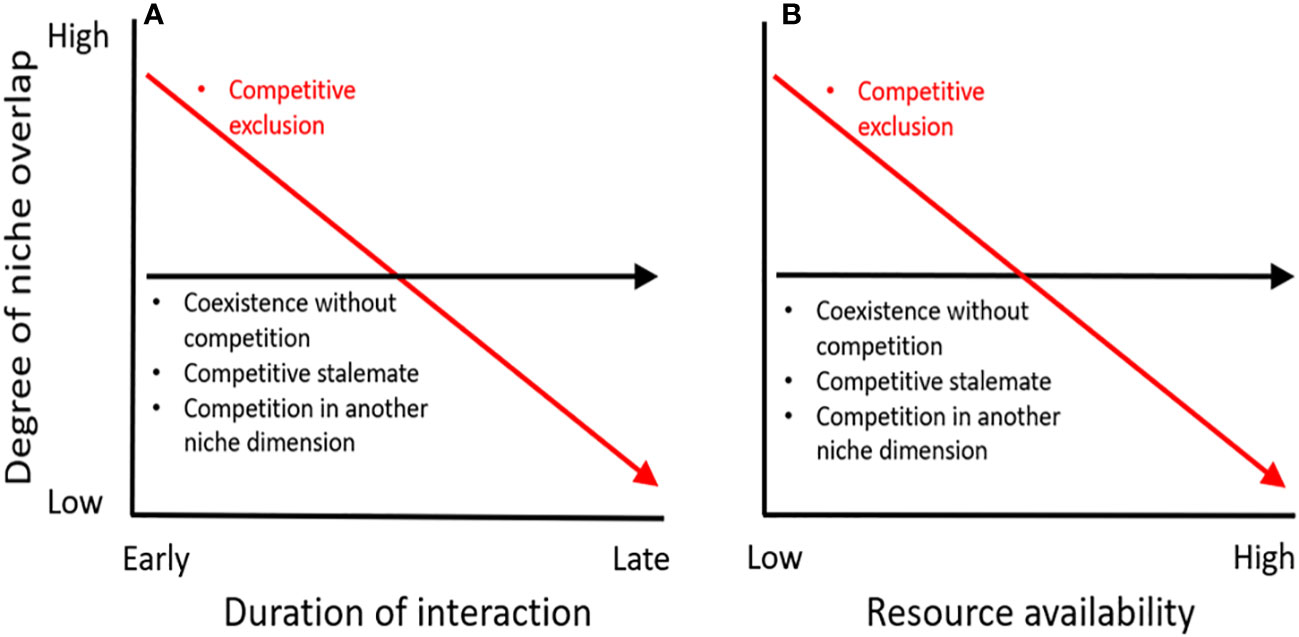
Figure 1 Conceptual model of the degree of niche overlap expected between 2 putatively competing species, depending on the duration of the interaction (A) and availability of a limiting resource, per capita (B). Under competitive exclusion (red), niche overlap should decline through time (character displacement) and with increasing resource availability (transition from realized to fundamental niche). Alternatively (black), coexistence without competition should exhibit invariant niche overlap across interaction timeline and resource availability, as should also be the case if species reach a competitive stalemate or if competition takes place in another (unmeasured) niche dimension. The shape, slope and relative levels of niche overlap relationships are arbitrary, and the model assumes that all other environmental conditions are held constant.
The merits of different study designs in ecological research can be indexed according to two primary metrics: i) strength of the inference (i.e., paucity of likely alternate hypotheses); and ii) generality of the findings (i.e., diversity of populations where the inference applies) (see Garton et al., 2005). If niche overlap studies are to contribute tangibly to interspecific competition research, they must be designed to provide robust inference and generality. First, the sampling unit should constitute an independent observation that is adequately replicated through space or time (Hurlburt, 1984). For example, competition experiments usually consider a manipulated site as the sampling unit, with responses being evaluated at multiple sites (i.e., replicated samples) and individuals at the same site considered as non-independent subunits exposed to a common manipulation (i.e., subsamples). However, comparative studies are conducted with less control over study design, meaning that non-independent sampling and pseudoreplication are more common, leading to weaker inference (see Garton et al., 2005). On the other hand, the added realism and larger spatial scale of many comparative studies compared to experiments may provide added generality of the findings, especially if the study spans conditions (e.g., time, resource levels, competitor density) that could influence competitive interactions.
Case study: mid-sized carnivores in North America
Our review comprised published papers (1986–2023) assessing whether bobcats, Canada lynx, and coyotes compete or partition resources in the wild. In Web of Science, we searched abstracts for the terms ‘competiti*’ or ‘partition*’ along with each pairwise combination of ‘bobcat’, ‘lynx’, and ‘coyote’ in all fields, yielding 111 results. We further screened references and those cited in papers that met our criteria for inclusion. We focused our review on studies assessing niche overlap between species (Supplementary Table 1) and only included papers stating either that the study was designed to assess interspecific competition or where competition was considered when interpreting the results. For each study, we recorded type of study, species examined, measurements and sampling units, spatial and temporal scale, ancillary data informing the assessment, and whether competition or related interactions (e.g., partitioning, exclusion, avoidance) were detected or speculated (Supplementary Table 1). We limited our analysis to a qualitative review because our primary interest was study design and derivable inference rather than prevalence or magnitude of competitive interactions. Although we used mid-sized North American carnivores as an illustration, our findings should apply more broadly to other systems where niche overlap is used in similar assessment (see Salinas-Ramos et al., 2020, Watz and Nyqvist, 2022).
We found 60 papers assessing competition between bobcat–coyote (n = 51), lynx–bobcat (n = 6) and lynx–coyote (n = 5) pairs; one study investigated both lynx–bobcat and lynx–coyote interactions. A single, exclusively descriptive paper (Gipson and Kamler, 2002) reported agonistic interactions between bobcats and coyotes, and a single experimental study (Henke and Bryant, 1999) leveraged a coyote control programme to assess bobcat numerical responses. The remaining papers (n = 58) evaluated interspecific competition via a comparative study design. Of these comparative studies, all but five (91.4%) measured some form of niche overlap between species, with the remainder assessing correlation between harvest statistics (Lovell et al., 1998; Guillaumet et al., 2015) and other measures of relative abundance (Main et al., 1999), trends in annual survival and cause-specific mortality (Kamler and Gipson, 2004), and outcomes of competitive encounters (Allen et al., 2016). Focusing on niche overlap studies from hereon, over half (54.7%, n = 29) measured overlap in a single type of resource or niche dimension (mostly space use n = 14; diet n = 9; habitat n = 3; time n = 3) (Supplementary Table 1). Most studies were conducted at a single site (62.3%, n = 33 studies), or spanned a relatively small spatial scale (< 500 km2) (52.8%, n = 28) given space requirements of the carnivore species in question. Studies were sometimes temporally disjunct (24.5%, n = 13), and often conducted over short timespans (≤ 2 years 49.1%, n = 26), reflecting the challenges of field research but likely compromising inference on temporal variability in competition or resource availability. Most studies (69.8%, n = 37) did not track individuals directly, meaning that the actual number of study animals was not known. Often the sampling unit used in the study was non-independent, as would arise with point locations for cameras, watering holes, tracks, or scats (Supplementary Table 1). Relatively few studies sought to address lack of independence statistically via mixed effects models or related adjustments (e.g., Atwood et al., 2011; Gese et al., 2013, Ochoa et al., 2021). Only 4 studies did not use some ancillary data to provide a more comprehensive assessment of interspecific competition or assess whether other factors might better explain patterns in niche overlap. Nonetheless, we surmised that very few studies measured resource availability and competitor abundance at the level needed to confirm resource limitation or estimate resource availability per capita. Overall, 43.4% of studies drew firm conclusions (Y = 8; N = 17) about competition and related interactions (e.g., partitioning, exclusion, avoidance), but in most studies inference was deemed equivocal (Supplementary Table 1).
Discussion
Most research studies claiming to evaluate interspecific competition in mid-sized North American carnivores lack the design and data collection needed for robust assessment. Niche overlap studies rarely have the spatial and temporal scale and ancillary data needed to measure whether variation conforms to predictions from theory. While some studies acknowledge these limitations, it remains that by design virtually all niche overlap studies lack the ability to substantively advance our understanding of interspecific competition. Because the limitations we observed extend to other species and systems where field investigations are similarly challenged (see Salinas-Ramos et al., 2020; Watz and Nyqvist, 2022), we infer that our current understanding of the role of interspecific competition in ecological communities is on weak footing.
Comparative studies are vital in community ecology research, and in the absence of experimental manipulation niche overlap measurement is understandably a tempting line of investigation for assessing exploitative competition between sympatric species. However in many studies the statistical unit of measure (i.e., camera locations, watering holes, scats) is unlikely to be appropriate, independent, and properly replicated (see Supplementary Table 1). For example, individual animals may visit watering holes multiple times or deposit many scats during the study, and without attention to data hierarchy, sampling fails to account for expected individual variation. To some extent, lack of independence can be addressed a priori as part of study design, for example by adopting a probability sampling framework (e.g., Garton et al., 2005). Post-hoc statistical remedies for non-independence include nesting variables or accounting for random effects, but these approaches are unhelpful when data are not suitable for constructing representative data hierarchies (Arnqvist, 2020; Silk et al., 2020). Consequently, statistical models using non-independent sampling units often will be mis-specified. Even using individuals as the sampling unit can be problematic if their ranges overlap and they have covarying responses to local resources. Ultimately, we consider the problem of sample independence in niche overlap research as mostly related to a mismatch between the study scale vs. data needs for robust inference (see also Davies and Gray, 2015). Most comparative studies are simply too restricted in time, space and sampling extent to use niche overlap measurement as a robust assessment of interspecific competition, and peer reviewers and journal editors should be more vigilant of these limitations (see Rogers et al., 2021).
We found inconsistent interpretation of niche overlap as evidence either for or against interspecific competition, and likewise lack of overlap is interpreted as evidence for or against competition (see Supplementary Table 1). This alarming inconsistency reflects a weak correspondence between competition theory, predicted outcomes, collected observations, and data interpretation. This confusion highlights the mistake in trying to infer competitive relationships using point measures of niche overlap and without broader understanding of the timeline of interactions, resource limitation, or interactions in other niche dimensions (Figure 1). For example, studies conducted along 1–2 niche axes ignore potential fine-scale niche partitioning given that diet overlap may indicate either active competition or lack of competition if species forage in different areas or at different times (e.g., see Hood et al., 2021). Species may exhibit skewed levels of niche overlap when studied at narrow spatial scales or during disjunct and restricted seasonal windows (e.g., Marrotte et al., 2020; Morin et al., 2020). Likewise, lack of ancillary data to assess numerical changes in competitor abundance or resource availability per capita make it virtually impossible to link niche overlap results to expected outcomes predicted by theory (Salinas-Ramos et al., 2020). Collectively, these shortcomings highlight the unsuitability of traditional niche overlap measurement as a solitary test of interspecific competition.
We highlight that virtually all studies we reviewed were designed to report on phenomenological patterns without measuring: i) magnitude of competition for parameterizing mathematical models, ii) primary drivers and constraints of competition, to support a mechanistic understanding, or iii) competition impacts to populations and communities. The lack of a broader understanding of competition remains a longstanding weakness in ecology (Tilman, 1987; Keddy, 1989; Abrams, 2022), and improvements require strategic study design and reinforced links between theory, predicted outcomes, data collection and statistical inference.
Future directions
For many animals, emerging biologging tools may help advance insights into agonistic interactions by measuring direct contact between instrumented individuals and related outcomes like spatial displacement or avoidance (Drewe et al., 2012; Broekhuis et al., 2019). In some cases, lack of sample independence can be addressed via probability sampling (Garton et al., 2005) or by adopting analytical techniques to support appropriate data hierarchies (e.g., Ruell and Crooks, 2007). Niche overlap analysis conducted in regions of species sympatry/allopatry may facilitate contrast while also reinforcing generality and large-scale inference (Peers et al., 2013; Gooliaf et al., 2018). However, in general study designs need to be considerably more dynamic and multi-faceted to yield contextualized niche overlap assessment. Although traditional field experiments will not experience a resurgence in competition research, natural experiments or quasi-experiments (Diamond, 1986) can be leveraged to provide additional insight. Ongoing range shifts, arrival of invasive species, decline in shared resources, or systemic biological control can each serve as a baseline for comparing niche overlap across different conditions. For example, ongoing shifts in distribution and abundance of bobcats, lynx and coyotes (Anderson and Lovallo, 2003; Bekoff and Gese, 2003) and active coyote depopulation efforts (Henke and Bryant, 1999) may provide a stronger framework for investigating niche displacement (see also Watz and Nyqvist, 2022). When combined with measures of competitor density or resource availability per capita, these approaches hold promise for robust assessment. Finally, the numerous given sampling design challenges facing many contemporary field studies, standardized data collection across studies would support synthetic approaches like meta-regression (Nakagawa et al. 2023), which could further inform on the magnitude, mechanistic underpinnings, and generality of competition. Thus, solutions exist to address many shortcomings in contemporary interspecific competition research, but ultimately they require stronger links to theory and innovative approaches to study design, data collection, and statistical inference.
Data availability statement
The original contributions presented in the study are included in the article/Supplementary Material. Further inquiries can be directed to the corresponding author.
Author contributions
DM: Conceptualization, Supervision, Writing – original draft, Writing – review & editing. JG: Conceptualization, Data curation, Formal Analysis, Investigation, Visualization, Writing – review & editing. AS: Conceptualization, Data curation, Investigation, Writing – review & editing. DT: Conceptualization, Investigation, Writing – review & editing.
Funding
The author(s) declare financial support was received for the research, authorship, and/or publication of this article. The publication of this article was supported by the Canada Research Chairs program.
Acknowledgments
We are grateful to Jonathan Gilbert for constructive comments on an earlier draft and to Stan Boutin for lessons learned many years ago when we (DLM) sought to assess interspecific competition via niche overlap measurement.
Conflict of interest
The authors declare that the research was conducted in the absence of any commercial or financial relationships that could be construed as a potential conflict of interest.
The author(s) declared that they were an editorial board member of Frontiers, at the time of submission. This had no impact on the peer review process and the final decision.
Publisher’s note
All claims expressed in this article are solely those of the authors and do not necessarily represent those of their affiliated organizations, or those of the publisher, the editors and the reviewers. Any product that may be evaluated in this article, or claim that may be made by its manufacturer, is not guaranteed or endorsed by the publisher.
Supplementary material
The Supplementary Material for this article can be found online at: https://www.frontiersin.org/articles/10.3389/fevo.2023.1281108/full#supplementary-material
References
Abrams P. (2022). Competition Theory in Ecology (Oxford: Oxford University Press). doi: 10.1093/oso/9780192895523.001.0001
Adler P. B., HilleRisLambers J., Levine J. M. (2007). A niche for neutrality. Ecol. Lett. 10, 95–104. doi: 10.1111/j.1461-0248.2006.00996.x
Adler P. B., Smull D., Choi R. T., Furniss T., Kulmatiski A., Meiners J. M., et al. (2018). Competition and coexistence in plant communities: intraspecific competition us stronger than interspecific competition. Ecol. Let 21, 1319–1329. doi: 10.1111/ele.13098
Allen M. L., Wilmers C. C., Elbroch L. M., Golla J. M., Wittmer H. U. (2016). The importance of motivation, weapons, and foul odors in driving encounter competition in carnivores. Ecology 97, 1905–1912. doi: 10.1002/ecy.1462
Anderson E. M., Lovallo M. J. (2003). “Bobcat and lynx (Lynx rufus and Lynx canadensis),” in Wild Mammals of North America – Biology, Management, and Conservation. Eds. Feldhamer G. A., Thompson B. C., Chapman J. A. (Baltimore, MD: The Johns Hopkins University Press), 758–786.
Arnqvist G. (2020). Mixed models offer no freedom from degrees of freedom. Trends Ecol. Evol. 35, 329–335. doi: 10.1016/j.tree.2019.12.004
Aschehoug E. T., Brooker R., Atwater D. Z., Maron J. L., Callaway R. M. (2016). The mechanisms and consequences of interspecific competition among plants. Annu. Rev. Ecol. Systematics 47, 263–281. doi: 10.1146/annurev-ecolsys-121415-032123
Atwood T. C., Fry T. L., Leland B. R. (2011). Partitioning of anthropogenic watering sites by desert carnivores. J. Wildl. Manage. 75, 1609–1615. doi: 10.1002/jwmg.225
Bekoff M., Gese E. M. (2003). “Coyote (Canis latrans),” in Wild Mammals of North America – Biology, Management, and Conservation. Eds. Feldhamer G. A., Thompson B. C., Chapman J. A. (Baltimore, MD: The Johns Hopkins University Press), 467–481.
Blanchet F. G., Cazelles K., Gravel. D. (2020). Co-occurrence is not evidence of ecological interactions. Ecol. Let. 23, 1050–1063. doi: 10.1111/ele.13525
Broekhuis F., Madsen E. K., Keiwua K., Macdonald D. W. (2019). Using GPS collars to investigate the frequency and behavioural outcomes of intraspecific interactions among carnivores: A case study of male cheetahs in the Maasai Mara, Kenya. PloS One 14 (4), e0213910. doi: 10.1371/journal.pone.0213910
Buskirk S. W., Ruggiero L. F., Krebs C. J. (2000). “Habitat fragmentation and interspecific competition: Implications for lynx conservation,” in Ecology and conservation of lynx in the United States. Eds. Ruggiero L. F., Aubry K. B., Buskirk S. W., Koehler G. M., Krebs C. J., McKelvey K. S., Squires J. R. (Boulder, CO: University Press of Colorado), 83–100.
Case T. J., Gilpin M. E. (1974). Interference competition and niche theory. Proc. Nat. Acad. Sci. 71, 3073–3077. doi: 10.1073/pnas.71.8.3073
Chesson P. (2000). Mechanisms of maintenance of species diversity. Ann. Rev. Ecol. Syst. 31, 343–366. doi: 10.1146/annurev.ecolsys.31.1.343
Connell J. H. (1980). Diversity and the coevolution of competitors, or the ghost of competition past. Oikos 35, 131–138. doi: 10.2307/3544421
Connell J. H. (1983). On the prevalence and relative importance of interspecific competition: Evidence from field experiments. Am. Nat. 122, 661–696. doi: 10.1086/284165
Connor E. F., Simberloff D. (1986). Competition, scientific method, and null models in ecology: Because field experiments are difficult to perform, ecologists often rely on evidence that is nonexperimental and that therefore needs to be rigorously evaluated. Am. Sci. 74, 155–162. Available at: https://www.jstor.org/stable/27854031.
Cusumano E. P., Peri E., Colazza S. (2016). Interspecific competition/facilitation among insect parasitoids. Curr. Opin. Insect Sci. 14, 12–16. doi: 10.1016/j.cois.2015.11.006
Davies G. M., Gray A. (2015). Don’t let spurious accusations of pseudoreplication limit our ability to learn from natural experiments (and other messy kinds of ecological monitoring). Ecol. Evol. 5, 5295–5304. doi: 10.1002/ece3.1782
Denno R. F., McClure M. S., Ott J. R. (1995). Interspecific interactions in phytophagous insects: Competition re-examined and resurrected. Annu. Rev. Entomology 40, 297–331. doi: 10.1146/annurev.en.40.010195.001501
Diamond J. (1986). “Overview: Laboratory experiments, Field experiments, and Natural Experiments,” in Community Ecology. Eds. Diamond J., Case T. (New York, NY: Harper & Row), 3–22.
Drewe J. A., Weber N., Carter S. P., Bearhop S., Harrison X. A., Dall S. R. X., et al. (2012). Performance of proximity loggers in recording intra- and inter-species interactions: A laboratory and field-based validation study. PloS One 7 (6), e39068. doi: 10.1371/journal.pone.0039068
Garton E. O., Ratti J. T., Giudice J. H. (2005). “Research and experimental design,” in Techniques for Wildlife Investigations and Management. Ed. Braun C. E. (Bethesda, MD: The Wildlife Society).
Gese E. M., Dowd J. L. B., Aubry L. M. (2013). The influence of snowmobile trails on coyote movements during winter in high-elevation landscapes. PloS One 8, e82862. doi: 10.1371/journal.pone.0082862
Gipson P. S., Kamler J. F. (2002). Bobcat killed by a coyote. Southwest Nat. 47, 511–513. doi: 10.2307/3672519
Gooliaf T. J., Weir R. D., Hodges K. E. (2018). Estimating bobcat and Canada lynx distributions in British Columbia. J. Wildl. Manage. 82, 810–820. doi: 10.2307/3672519
Guillaumet A., Bowman J., Thornton D., Murray D. L. (2015). The influence of coyote on Canada lynx populations assessed at two different spatial scales. Community Ecol. 16, 135–146. doi: 10.1556/168.2015.16.2.1
Gurevitch J., Morrow L. L., Wallace A., Walsh J. S. (1992). A meta-analysis of competition in field experiments. Am. Nat. 111, 1169–1194. doi: 10.2307/3078927
Henke S. E., Bryant F. C. (1999). Effects of coyote removal on the faunal community in western Texas. J. Wildl. Manage. 63, 1066–1081. doi: 10.2307/3802826
Holt R.D., Bonsall M.B. (2017). Apparent competition. Ann. Rev. Ecol. Evol. Syst. 48: 447–471. doi: 10.1146/annurev-ecolsys-110316-022628
Hood G. R., Blankenship D., Doellman M. M., Feder J. L. (2021). Temporal resource partitioning mitigates interspecific competition and promotes coexistence among insect parasites. Biol. Rev. 96, 1969–1988. doi: 10.1111/brv.12735
Hoving C. L., Joseph R. A., Krohn W. B. (2003). Recent and historical distributions of Canada lynx in Maine and the northeast. Northeast. Nat. 10, 363–382. doi: 10.2307/3858654
Hurlburt S. H. (1984). Pseudoreplication and the design of field experiments. Ecology 54, 187–211. doi: 10.2307/1942661
Kamler J. F., Gipson P. S. (2004). Survival and cause-specific mortality among furbearers in a protected area. Am. Midland Nat. 151, 27–34. doi: 10.1674/0003-0031(2004)151[0027:SACMAF]2.0.CO,2
Kaplan I., Denno R. F. (2007). Interspecific interactions in phytophagous insects revisited: A quantitative assessment of competition theory. Ecol. Let. 10, 977–994. doi: 10.1111/j.1461-0248.2007.01093.x
Litvaitis J. A. (1992). “Niche relations between coyotes and sympatric carnivora,” in Ecology and Management of the Eastern coyote. Ed. Boer A. H. (Frederickton, NB: University of New Brunswick), 73–85.
Lovell C. D., Leopold B. D., Shropshire C. C. (1998). Trends in Mississippi predator populations 1980-1995. Wildlife Soc. Bull. 26, 552–556.
Main M. B., Walsh P. B., Portier K. M., Coates S. F. (1999). Monitoring the expanding range of coyotes in Florida: Results of the 1997-98 statewide scent station surveys. Florida Field Nat. 27, 150–162.
Marrotte R. R., Bowman J., Morin S. J. (2020). Spatial segregation and habitat partitioning between bobcat and Canada lynx. Facets 5, 503–522. doi: 10.1139/facets-2019-0019
Morin S. J., Bowman J., Marrotte R. R. (2020). Fine-scale habitat selection by sympatric Canada lynx and bobcat. Ecol. Evol. 10, 9396–9409. doi: 10.1002/ece3.6626
Murray D. L., Steury T. D., Roth J. D. (2008). Assessment of Canada lynx research and conservation needs in the southern range: another kick at the cat. J. Wildl. Manage. 72, 1463–1472. doi: 10.2193/2007-389
Nakagawa S., Yang Y., Macartney E. L., Spake L., Lagisz M. (2023). Quantitative evidence synthesis: a practical guide on meta-analysis, meta-regression, and publication bias tests for environmental sciences. Environ. Evid. 12, 8. doi: 10.1186/s13750-023-00301-6
Ochoa G. V., Chou P. P., Hall L. K., Knight R. N., Larsen R. T., McMillan B. R. (2021). Spatial and temporal interactions between top carnivores at water sources in two deserts of western North America. J. Arid Env. 184, 104303. doi: 10.1016/j.jaridenv.2020.104303
Parker G. R. M., Maxwell J. W., Morton L. D., Smith G. E. J. (1983). The ecology of the lynx (Lynx canadensis) on Cape Breton Island. Can. J. Zool. 61, 770–786. doi: 10.1139/z83-102
Peers M. J. L., Thornton D. H., Murray D. L. (2013). Evidence for large-scale effects of competition: Niche displacement in Canada lynx and bobcat. Proc. R. Soc B. 280, 20132495. doi: 10.1098/rspb.2013.2495
Rogers A., Dietz K.-J., Gifford M. L., Lunn J. E. (2021). The importance of independent replication of treatments in plant science. J. Exp. Bot. 15, 5270–5274. doi: 10.1093/jxb/erab268
Ruell E. W., Crooks K. R. (2007). Evaluation of noninvasive genetic sampling methods for felid and canid populations. J. Wildl. Manage. 71, 1690–1694. doi: 10.2193/2006-061
Salinas-Ramos V. B., Ancillotto L., Bosso L., Sánchez-Cordero V. (2020). Interspecific competition in bats: state of knowledge and research challenges. Mammal Rev. 50, 68–81. doi: 10.1111/mam.12180
Schoener T. (1983). Field experiments on interspecific competition. Am. Nat. 122, 240–285. doi: 10.1086/284133
Silk M. J., Harrison X. A., Hodgson D. J. (2020). Perils and pitfalls of mixed effects regression models in biology. PeerJ 8, 20. doi: 10.7717/peerj.9522
Tilman D. (1987). The importance of the mechanisms of interspecific competition. Am. Nat. 139, 769–774. doi: 10.1146/annurev-ecolsys-121415-032123
Keywords: interspecific competition, study design, niche overlap, Canada lynx, bobcat, coyote
Citation: Murray DL, Gobin J, Scully A and Thornton DH (2023) Conventional niche overlap measurements are not effective for assessing interspecific competition. Front. Ecol. Evol. 11:1281108. doi: 10.3389/fevo.2023.1281108
Received: 21 August 2023; Accepted: 23 October 2023;
Published: 13 November 2023.
Edited by:
Pavel Kindlmann, Charles University, CzechiaReviewed by:
Jonathan H. Gilbert, Independent researcher, United StatesCopyright © 2023 Murray, Gobin, Scully and Thornton. This is an open-access article distributed under the terms of the Creative Commons Attribution License (CC BY). The use, distribution or reproduction in other forums is permitted, provided the original author(s) and the copyright owner(s) are credited and that the original publication in this journal is cited, in accordance with accepted academic practice. No use, distribution or reproduction is permitted which does not comply with these terms.
*Correspondence: Dennis L. Murray, ZGVubmlzbXVycmF5QHRyZW50dS5jYQ==