- Ministry of Education Key Laboratory of Ecology and Resource Use of the Mongolia Plateau, Collaborative Innovation Center for Grassland Ecological Security, School of Ecology and Environment, Inner Mongolia University, Hohhot, China
Ecological integrity can satisfactorily reflect the comprehensive quality of ecosystems and has become a useful tool for evaluating the ecological environment. Ecological integrity evaluation has been widely applied in various ecosystems. Conducted in the Alxa League, the study established an ecological integrity index based on ecosystem structure, function and resilience and evaluated the ecological integrity of the study area in 1990, 2000, 2010 and 2020. Using hotspots spatial analyses, we analyzed the temporal and spatial variation of ecological integrity index during the study period. The main contributing factors affecting ecological integrity were identified with the help of the geographical detector model. Our results showed that: (1) Ecosystem structure, function and resilience in the Alxa League had obvious spatial heterogeneity and barely changed from 1990 to 2020. (2) Half of the area had a poor ecological integrity index, and the decrease in ecological integrity mainly occurred in the Alxa Left Banner. (3) Among the factors affecting the ecological integrity index, land use intensity was the major driving factor, and desertification was a key reason leading to the decrease. Ecological integrity evaluation can increase public awareness of desert conditions and guide policy makers to make reasonable and sustainable policies or strategies to protect and restore desert ecosystems.
1 Introduction
Natural ecosystems play a vital role in providing products and services for human survival and development (Costanza et al., 1997; Peng et al., 2017). For centuries, human activities and global change have had considerable influence on ecosystem structure and function (Hughes et al., 2003; Foley et al., 2005; Hughes et al., 2018). Although ecological restoration measures have been implemented to reduce the negative impacts on ecosystems (Peng et al., 2018; Fischer et al., 2021; Li et al., 2021), many ecological problems still limit human social and economic development and even threaten human health (Qu and Fan, 2010; Law and Annual, 2017; Chase et al., 2020). Thus, it has become necessary to conduct ecological valuations to diagnose the condition of the ecological environment. As an important concept in natural resource management and environmental protection, ecological integrity has been widely used in ecological condition evaluations (Huang et al., 2006; Borja et al., 2008).
Ecological integrity, also known as ecosystem integrity, was first noted by Leopold in 1949 (Leopold, 1949). Initially, ecological integrity was designed as an objective reflection of the ecosystem composition, which included physical, chemical and biological integrity (Karr and Dudley, 1981; Karr, 1996). Later, scholars argued that ecological integrity should not only focus on the ecosystem structure, but also consider other characteristics of natural ecosystems, such as ecosystem function, resilience and self-organization (Andreasen et al., 2001; Pan et al., 2021b; Ma et al., 2022). In addition, some studies began to keep a watchful eye on the involvement of humans, not only considered the condition of natural ecosystem itself, the ability of meeting human demand also should be adopted during the construction of ecological integrity (Kay, 1991; Angermeier and Karr, 1994; Parrish et al., 2003). With the development of ecological integrity theory, the concept of ecological integrity was widely expected to reflect both the comprehensive quality of ecosystem composition, structure, function, and resilience and the ability to achieve sustainable development (Karr and Dudley, 1981; Barbour et al., 2000; Parrish et al., 2003; Wurtzebach and Schultz, 2016; Roche and Campagne, 2017).
The high practicability and effective ability reflect the comprehensive condition of ecosystems have led to ecological integrity being widely used in ecological evaluations. For example, Tierney et al. (2009) evaluated the forest ecological integrity in Acadia National Park and provided effective management suggestions and scientific guidance related to potential problems. Ecological integrity was also regarded as an indicator reflecting the condition of agroecosystems in southern South America, to scientifically guide agricultural production (Blumetto et al., 2019). In addition, the ecological significance behind ecological integrity made it sever well in reflecting the local ecological environment, and ecological integrity assessments have been broadly applied to various ecosystem types, such as oceans (Maloney, 2019), rivers (Stevens et al., 2021), wetlands (Jiang et al., 2015), lakes (Ozkundakci et al., 2014), grasslands (Kaiser et al., 2009) and urban area (MacGregor-Fors et al., 2022). In addition, sustaining sufficient levels of ecological integrity also became the fundamental basis for the implementation of natural resource protection. The Clean Water Act (CWA) in 1972 regarded maintaining high-quality ecological integrity as the basic and durative goal for protecting water resources (Barbour et al., 2000), and similar requirements appeared in the Austrian Water Act in 1990 (Moog and Chovanec, 2000). National parks in China also took maintaining high-level ecological integrity as the standard during conservation management (Zhao and Yang, 2021).
Typically, the method used to evaluate ecological integrity depends on the type of research area, which can be divided into small-scale and large-scale areas (Coppedge et al., 2006; Mora, 2017; Zeleny et al., 2021). Small-scale research has been usually conducted using field observational data, such as species data and soil physicochemical index data (Ordóñez and Duinker, 2012; Blumetto et al., 2019). Large-scale research has used spatial data produced by remote sensing techniques or geographic information systems (Revenga, 2005; Lausch et al., 2017) to evaluate the ecological integrity of large-scale regions. In large-scale studies, these indicators mainly included the landscape index (Pan et al., 2021b) and ecosystem services (Han et al., 2022), which can reflect the condition of ecosystem structure and function.
Desert ecosystems are the dominant ecosystem type, covering approximately one-third of the global terrestrial surface area (Bidak et al., 2015; Liang et al., 2019). Deserts can provide ecosystem services associated with windbreaks and sand fixation, soil conservation, biodiversity conservation and culture (Warren, 1981; Khan et al., 2017). Moreover, deserts suffer from various kinds of ecological problems, such as dust storms (Middleton, 2017), land desertification (Shao et al., 2023) and water scarcity (Fang et al., 2015). Scholars currently focused on desert ecosystems have focused mainly on one part of ecological integrity, such as desert ecosystem structure (Durant et al., 2012; Wang et al., 2022b) or desert ecosystem function (Slemnev and Gunin, 2000; Taylor et al., 2017; Alrawaf et al., 2023). However, few studies have focused on evaluating the overall ecological integrity condition in arid regions.
In China, desert ecosystems and desertification-prone regions occupy 44% of the land area, especially in northern China (Wang et al., 2022a), where had our study area, Alxa League. As a typical arid desert region, the Alxa League has harsh natural conditions, sparse precipitation, strong evaporation and a vulnerable ecological environment. Although ecological governance projects, such as the “Three-North” Shelter Forest Program (Zhang et al., 2016), have been applied, the ecological environment is still fragile. In addition, how the quality of the natural environment of the Alxa League has changed in recent decades and the factors that have influenced the environment are still unclear. An evaluation of ecological integrity of the Alxa League not only provides an effective tool for decision-makers to identify the integrity status of regional ecosystems but also has guiding significance for the conservation and restoration of ecological environments.
In this study, we established a framework that included structure, function and resilience of the ecosystem to evaluate the ecological integrity of the Alxa League. We aimed to: (1) evaluate the ecological integrity of the Alxa League and analyze the spatiotemporal variation in ecological integrity from 1990 to 2020 and (2) explore the factors influencing the ecological integrity of the Alxa League.
2 Materials and methods
2.1 Study area
The Alxa League is in western Inner Mongolia of China and covers an area of 270,000 km2 (37°24′–42°47′N, 97°10′–106°53′E) (Figure 1). The Alxa League has a temperate continental climate, and the annual average temperature is approximately 7.9–10.0°C. The annual precipitation is only 30–300 mm, and approximately 80% of rainfall is concentrated between June and September. The main land use types in Alxa are desert and barren, accounting for approximately 71.6% and 16.3%, respectively (referring to the land use data in 2020). The vegetation coverage in most areas is less than 15%, and the main vegetation types are degraded shrubbery and grassland, including typical desert psammophytes (He et al., 2007). Alxa has three of China’s eight deserts, including the Badain Jaran, Tengger and Ulan Buh Deserts. Three banners, Ejin Banner, Alxa Right Banner and Alxa Left Banner (henceforth simply Ejin, Alxa right and Alxa left) make up the Alxa League. The population density of Alxa is low, and the total population was approximately 0.26 million in 2020.
2.2 Data sources and processing
The data we used in this study can be classified into two categories: spatial data and statistical data, and the time nodes of the data we used were 1990, 2000, 2010 and 2020. Spatial data included the following: (1) The land-cover dataset (1 km resolution), digital elevation model (DEM) data (90 m resolution) and distance to highway data were supported by the Data Center for Resources and Environmental Sciences, Chinese Academy of Sciences (RESDC) (http://www.resdc.cn). Slop and aspect data (90 m resolution) were calculated from DEM data and were used as driving factors. (2) The meteorological datasets (1 km resolution), including annual precipitation, mean annual temperature and annual potential evapotranspiration, were used as driving factors, which came from the National Tibetan Plateau Data Center (National Tibetan Plateau Data Center, tpdc.ac.cn). (3) The NDVI data (100 m resolution). Using the Google Earth Engine (GEE) platform, we obtained the NDVI data of the growing period (June-October) based on the red and near infrared bands of the surface reflectance collections for Landsat 5 and 8 (USGS Landsat Surface Reflectance Tier 1). Based on the NDVI data, we obtained the fractional vegetation cover data of Alxa Left. Statistical data included GDP and population data, were mostly collected from the Alxa Statistical Yearbook and Inner Mongolia Statistical Yearbook.
To show the features of the classical desert ecosystem region, we divided the unused land into desert and barren parts, and forestland was divided into forest and shrub land (Wang et al., 2022b). The remaining land use types included dryland, grassland, water and construction land.
Typically, the spatial scale of grid included 1 km, 3 km, 5 km and 10 km (Ou et al., 2018). In our study, we conducted the ecological integrity in the different scales and found that the spatial distribution regularity of ecosystem integrity index was quite similar. Referencing the previous study (Gao et al., 2011; Liu et al., 2023) and considering the area of the study area, where covered great area of dune and desert, the 5 km × 5 km grid was less than the average area of typical land use type in the Alxa League, which means the 5 km × 5 km grid was small enough to represent the ecological integrity condition of the Alxa League. Thus, we decided use 5 km × 5 km grid as the final scale. Using the Create Fishnet tools in ArcGIS 10.7, we divided the Alxa League into 9916 5 km × 5 km grids. These grids were chosen as the basic research area, and analysis of the spatial temporal change in ecological integrity was also based on them.
2.3 Ecological integrity index
We established an ecological integrity assessment framework based on ecosystem structure, ecosystem function and ecosystem resilience (Figure 2). From these three parts, the assessment not only reflected the comprehensive quality of the ecosystem, but also ensured the ability of sustainable development under human demand. Referring to the assessment method of ecosystem health of Costanza (2012), we established the calculation of the ecological integrity index (EII):
where EII is the regional ecological integrity index, ESI is the ecosystem structure index, and EFI and ERI are the ecosystem function index and ecosystem resilience index, respectively. These indexes (i.e., EII, ESI, EFI and ERI) were normalized to [0,1], and the processing method was as follows:
where Xij is the actual value of the indicator in the i-th year, min (Xi) is the minimum value, max (Xi) is the maximum value, and Yij is the normalized value.
2.3.1 Ecosystem structure index
The ecosystem structure index reflects the stability of the ecosystem structure, which is mostly measured by the landscape pattern index in terms of landscape heterogeneity and connectivity (Howell et al., 2018). Landscape heterogeneity is a combination of land use and landscape features (Seidl and Golobic, 2020), and landscape connectivity represents the connectivity of the overall landscape and important ecosystems, such as forest, water and grassland, which are highly related to soil conservation, windbreaks and sand fixation (Styers et al., 2010; Lavorel et al., 2017). We calculated several landscape indexes (Table 1) from class metrics based on ArcGIS 10.7 and Fragstats 4.2 software, and the ecosystem structure index (ESI) was measured as follows:
where Ski is the area of landscape i in research grid k; Sk is the total area of landscape in grid k, and the following indexes were normalized and explained in Table 1.
2.3.2 Ecosystem function index
Ecosystem services represent the products and services provided by natural ecosystems for humans (Costanza et al., 1997; de Groot et al., 2002). Ecosystem service value reflects the quality of regional ecosystems and shows the ability of basic ecosystem functions, such as grain production and climate comfort (Groot, 1992; Egoh et al., 2007). Referencing the Millennium Ecosystem Assessment (Finlayson et al., 2005), we measured the ecosystem function index from provisioning, regulating, supporting and cultural services, and used the equivalence factor to calculate the value of these services (Costanza et al., 1997; Xie et al., 2003). The following method was used:
where Ai is the different land use areas, and Vsi is the monetary value unit of the service type for different land use types. Based on the improved method of Xie et al. (2015), we obtained the Vsi for Alxa, and then normalized the ESV to the ecosystem function index (EFI). The value of Vsiis given in Table S1.
2.3.3 Ecosystem resilience index
Ecosystem resilience is designed to describe the ability of an ecosystem to resist the disturbance and restore stability after disturbance; hence, resilience can be characterized through resistance and resilience to external disturbances (Holling, 1973; Whitford et al., 1999). Referring to previous studies on ecosystem resilience models (Peng et al., 2017; Pan et al., 2021b) and considering the ecological environment of the study area, we obtained a method of ecosystem resilience index in the Alxa League. Ecosystem resistance is the ability to avoid change, we chose the NDVI to represent the Resist. The NDVI can reflect the condition of vegetation coverage, which helps to show where these is a good situation of ecosystem resistance. In addition, the value trend of Resist (NDVI) in each land use type used in our research was similar to that in previous research (Xiao et al., 2019; Zhou et al., 2022). For ecosystem resilience, which is the ability of an ecosystem to restore itself to its original state, we obtained the value of Resil based on the study of Gao (2001).
where ERI is the ecosystem resilience index, Resisit represents ecosystem resistance, and Resil represents ecosystem resilience; Ai and A are the area of different land use types and the total area in each grid, respectively; Pi refers to the ecological resilience score, the value of Pi were referred the study of Gao (2001) and adjusted to suitable for the condition of the Alxa League. The specific value of Pi is given in Table S2.
2.3.4 Levels of each index
To better describe each level of ecological indexes and show the regional differences and temporal changes in the Alxa League, we used the natural breaks (Jenks) method to divide all indexes into five levels, and the same index from 1990 to 2020 had the same standard (Table 2).
2.4 Hotspots and coldspots spatial analyses of the EII
Hotspots spatial analyses have been widely used in ecology to help determine the spatial locations of hotspots and coldspots, namely, spatial clusters of high and low values (Li et al., 2016; Zhao et al., 2020). In our study, we used the hotspots analysis tool in ArcGIS 10.7 (Getis-Ord Gi*) to explore the spatial changes in ecological integrity from 1990 to 2020 in the Alxa League, and the increasing EII changes represented hotspots, while the decreasing EII changes represented coldspots.
2.5 Analysis of driving factors
2.5.1 Geographical detector model
Spatial autocorrelation and spatial heterogeneity are two major features of ecological and geographical phenomena (Goodchild and Haining, 2004; Wang et al., 2016). Based on the feature of spatial heterogeneity, the geographical detector model (GDM) can be used to indicate the relationship between a geographical feature and a factor (Zhao et al., 2020; Zhu et al., 2020). In this study, we used GDM to explore the relationship between ecological integrity and driving factors in the remaining 3378 grids in Alxa Left, and found the change in each factor from 1990 to 2020. Its calculation formula is as follows:
where q represents the explanatory power of the driving force X to Y, and the range is 0–1; i is the number of stratifications (classes) of dependent variable Y or factor X; ∂i2 and ∂2 represent the variance in the Y value of layer i and the whole area respectively; and n and ni are the number of units in the whole area and layer i, respectively.
2.5.2 Selection of driving factors
Through hotspots analysis, we found that the change in EII mainly occurred in Alxa Left. To determine how nature and humans influence the ecological integrity in Alxa Left, we selected eleven driving factors and explored their influence on the EII (Table 3). These driving factors mainly refer to the local condition of the research area and the related studies (He et al., 2019; Wang et al., 2022c), and can be categorized into five groups of driving forces. The calculation of land use intensity referenced the study of Zhuang and Liu (1997).
3 Results
3.1 Spatial and temporal characteristics of ecological indexes
3.1.1 Ecosystem structure, ecosystem function and ecosystem resilience
In 2020, the ecosystem structure in the Alxa League was dominated by the excellent level, accounting for 71.2% of the Alxa League. The regions with poor and fair structure indexes were particularly distributed in the middle of Ejin and the east of Alxa Left, which accounted for only 2.8% of the Alxa area. Different from the ecosystem structure index, the poor level was the primary degree of the ecosystem function index, and 85.4% of the regions of the Alxa League were categorized as having a poor function index in 2020. Only 400 km2 had a high ecosystem function index value in the Alxa League (Table S3), and these regions were mainly in the water around the area of Ejin and Alxa Left. This contrast of spatial distribution between ecosystem structure and function index was especially embodied in Alxa Right, where 88.3% of the area had an excellent structure index, while no region had a good or excellent function index. For the ecosystem resilience index in 2020, half of the area of the Alxa League was at a poor level. Of the regions with a poor resilience index in the Alxa League, 42.8% were located in Alxa Right and 40.1% were Ejin. However, Alxa Left had the highest proportion of fair and moderate resilience indexes in the Alxa League, with values of 56.6% and 59.9%, respectively.
From 1990 to 2020, ecosystem structure and function showed similar temporal changes, and the proportion of each degree of both indexes barely changed (Table S3). The area classified by the poor and fair ecosystem resilience indexes slightly changed during the study period (Table S3). Although the area of each ecosystem index type changed slightly, the spatial distribution pattern of these index levels barely changed from 1990 to 2020 (Figure S1). Thus, we selected only 2020 as the example to analyze the spatial distribution characteristics and area proportions of these indexes (Figure 3).
3.1.2 Ecological integrity index
A similar spatial distribution pattern was observed between the ecosystem resilience index and EII (Figure 4), and the primary degree of EII was also poor, similar to the ecosystem resilience index. The area with a poor EII were mainly located in Alxa Right and western Ejin, especially in Alxa Right, and the poor EII regions showed a contiguous distribution pattern. Except in 1990, the percent of poor EII in Alxa League was always over 60% from 1990 to 2020. The second main degree of EII was the fair level, and fair EII regions were mostly distributed in the east of Alxa Left and in eastern Ejin. The fair EII covered 34.9% of the area in the Alxa League in 1990, while this number changed to only 22.2%, on average, in the last three decades. The area with a moderate EII accounted for 11.8%–14.6% of the Alxa League, and half of the moderate level areas were located in Alxa Left. The sum of the good and excellent levels accounted for only 2.3% of the Alxa League on average during the study period, and these high-value regions were mainly in eastern Alxa Left and the middle of Ejin. The turning point of the EII occurred in 2000, when the number of low EII value areas showed an obvious expansion trend from 1990 to 2000; 46.4% of fair level area and 15.8% of moderate level area in 1990 changed to poor level area and fair level area in 2000 (Figure 5), respectively. The changed area mostly emerged in the middle of Alxa Right and the south of Alxa Left. In the following years, although the area of each EII level transformed, the proportion of each degree was maintained at a stable value (Figure 5).
3.2 Hotspots and coldspots of EII changes
In the 1990–2000, 2000–2010 and 2010–2020 periods, the change in the EII mainly occurred in Alxa Left and Ejin (Figure 6). From 1990 to 2000 (Figure 6A), the EII coldspots area accounted for 8.1% of the Alxa League, and 79.2% of the coldspots regions were located in Alxa left, covering 15,500 km2. The hotspots regions accounted for 6.7% of the Alxa League, and they were mainly clustered in northern Ejin, with a total hotspots area of 12,900 km2. In the following decade (Figure 6B), the EII of the Alxa League mainly showed decreasing trend, and the coldspots area accounted for 8.5% of the Alxa League, which was the highest among the three periods. The coldspots regions of the EII were mainly distributed in northern Ejin, where 20.5% of the area showed significant negative changes. In addition, the EII in Alxa Left and Alxa Right had small regions with an improving trend from 2000 to 2010, and the hotspots area accounted for 6.3% of the Alxa League, which was the lowest among the three periods. From 2010 to 2020 (Figure 6C), the distribution of EII change was more scattered than that in other periods, and 53.0% of the change in the Alxa League was located in Alxa Left. In this period, the areas of coldspots and hotspots were similar, and both accounted for approximately 7.2% of the Alxa League. We also explored how the EII changed between 1990 and 2020 (Figure 6D), and the percentages of coldspots and hotspots in the Alxa League were 12.4% and 7.3%, respectively. From 1990 to 2020, we found that in each period, the dominate change trend of the EII in the Alxa League was a decrease. Especially in eastern Alxa Left (Figure S2), the EII significantly decreased in the 1990–2000 period, while in the 1990–2020 period, this area still showed a negative change, which means that the EII in this region has not recovered since 2000. To determine the factors influencing the EII and the reasons for the EII changes in Alxa Left, we analyzed the driving factors in the next section.
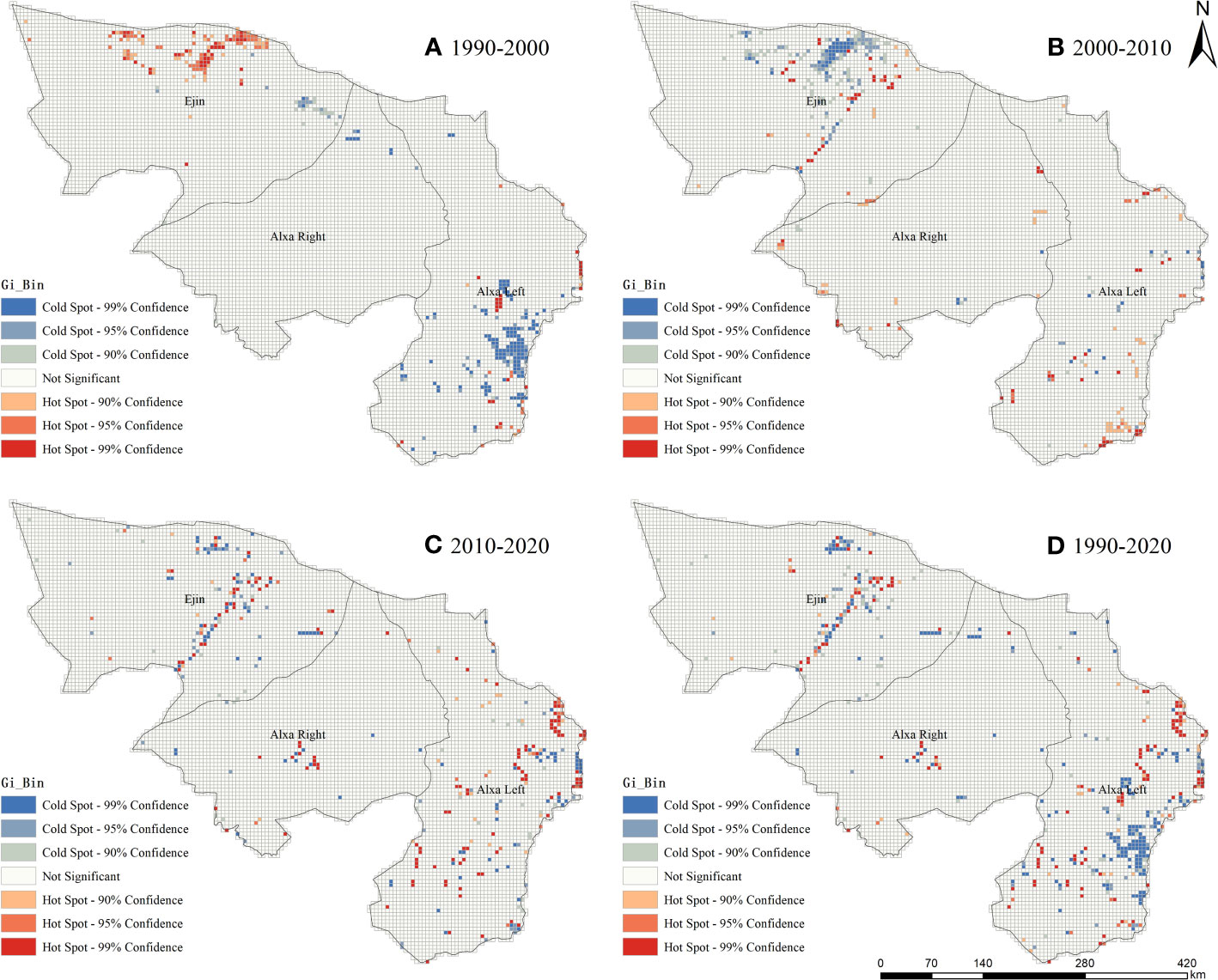
Figure 6 Spatial clustering of EII change between 1990 and 2000 (A), 2000 and 2010 (B), 2010 and 2020 (C), and 1990 and 2020 (D).
3.3 Driving factors of the EII in Alxa Left
Based on the geographical detector model, we explored the explanatory power (q values) of driving factors to EII in Alxa Left and all eleven factors (Table 3) passed the 0.01 significance test. Figure 7 shows the results of single factor geographic explanatory and the interaction between the factors.
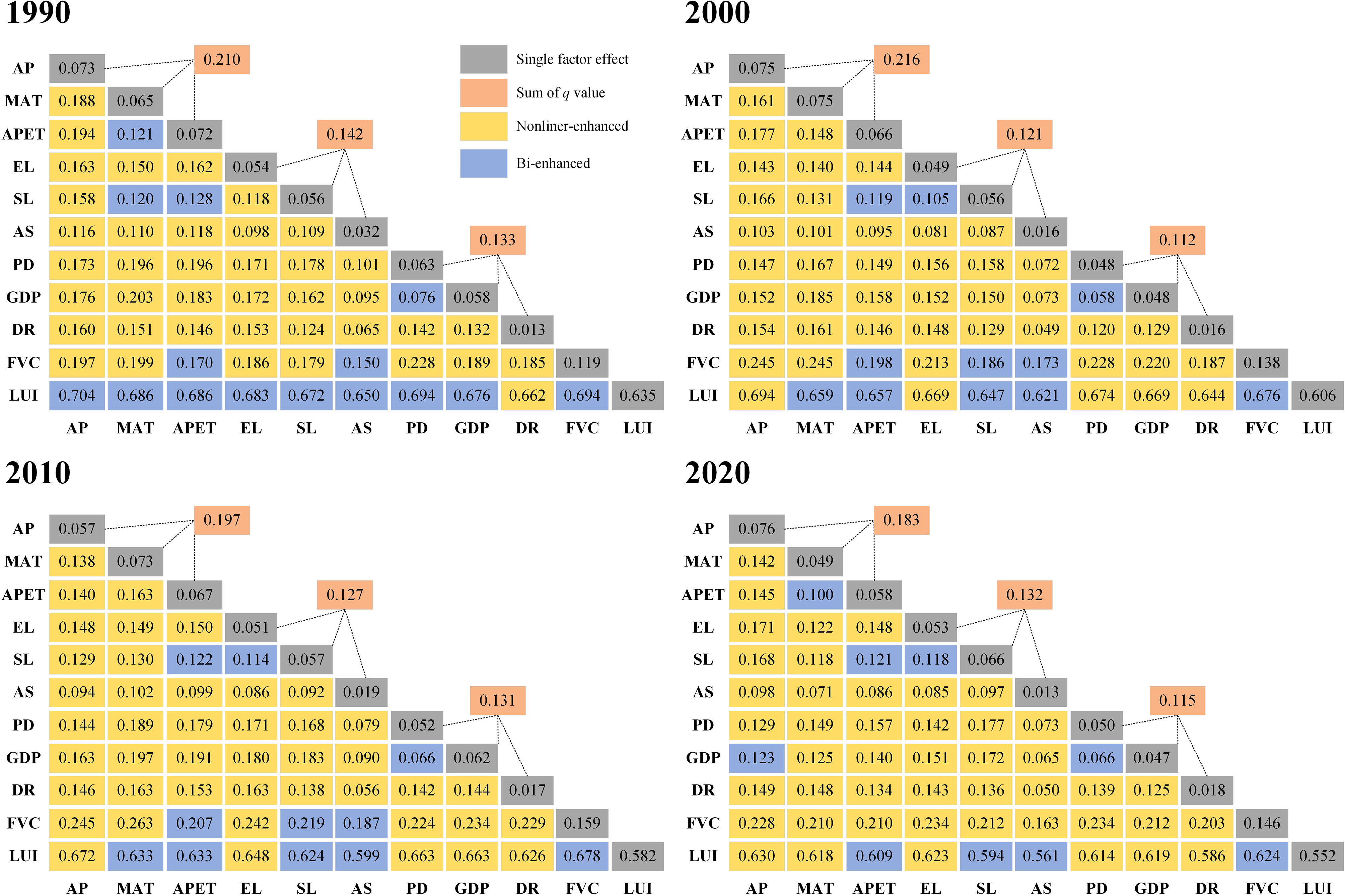
Figure 7 Interactive influence coefficients of EII in Alxa Left from 1990 to 2020. From the top to bottom, the sum of q value represents climate, topography and economic factor.
The land use intensity had the highest q value among these selected factors, and it could contribute more than 0.550 to the EII in each year. However, the explanatory power of land use intensity showed weakening trend, and the value in 2020 was 0.552, 13.1% lower than that in 1990. The following driving factor was fractional vegetation cover, which could 0.141 to the EII of Alxa Left, on average, and the explanatory power has continued to increase since 1990. The sum q value of climate was 0.202 on average over four years, and the annual precipitation had a slightly higher q value among the climate parts, with an average of 0.070. The mean annual temperature and annual potential evapotranspiration had similar explanatory power for the EII, with average q values of 0.066. The q value of the remaining driving factors was less than 0.06, and these factors mainly came from topography and economic factors. The sum of the q values of the economic factors contributed only 0.123 on average to the EII.
The interaction detection results showed that the interactions between pairs of the selected factors exhibited more highly significant effects than did any single factor, and the interaction type was mainly manifested as a nonlinear enhancement of both factors (Figure 7). Land use intensity and fractional vegetation cover exerted strong influences on the factor interactions, and the q values of the interactions of other factors with land use intensity and fractional vegetation cover were several times higher than the value for the independent actions of these factors. In addition, the pair of land use intensity and fractional vegetation cover had significant bilateral enhancement in each year, and the greatest enhancement was 0.694 in 1990. From 1990 to 2020, the highest q value was 0.704 in 1990, which was produced by the combination of land use intensity and annual precipitation (Figure 7).
4 Discussion
4.1 Driving factors of ecological integrity
In our study, we found that land use intensity was the dominant driving factor affecting desert ecosystem integrity. This result was similar to the study of Pan et al. (2021a), who found that land use intensity had a great influence on ecosystem services in arid regions. Land use intensity also influenced the ecological integrity condition of different ecosystem types, such as wetland (Jiang et al., 2015) and island zones (Jiang et al., 2018). However, compared with desert ecosystems, the explanatory power of land use intensity in other ecosystems was much weaker, and economic factors had more weight in terms of affecting ecosystem integrity (Xiao et al., 2022; Xu et al., 2022). Typically, the economy has a complicated influence on the local natural ecological environment (Kijima et al., 2010), while our study showed that the economic factor had a lower influence on ecological integrity. Because land use intensity was highly related to economic acuities (Zhuang and Liu, 1997), the explanatory power between economic factors and land use intensity would not have such a great magnitude difference in Alxa Left. However, in Alxa Left, limited human activities influenced the land use intensity in the oasis regions, it is difficult to affect the original natural environment in the dune regions. In addition, both the low population and the large dune area limited the economic growth of Alxa Left. Thus, this background in Alxa Left made land use intensity the primary driving factor, and economic factors had less explanatory power for ecological integrity.
In addition to human activists, natural factors influenced the ecological integrity condition of Alxa Left. Related studies have shown that the ecological environment of desert ecosystems is deeply influenced by climate factors (Clarke and Rendell, 1998; Ran et al., 2021). In our study, climate factors, including precipitation, temperature and potential evapotranspiration had a great effect on the ecological integrity of Alxa Left. He et al. (2019) found that temperature and precipitation had a significant influence on ecosystem health in the northwest region of China, which also supported our results regarding the influence of climate factors. Vegetation also plays an important role in influencing ecological conditions. The vegetation in Alxa Left was composed of natural vegetation and artificial vegetation (Zhang et al., 2021), and the condition of fractional vegetation cover had a significant effect on ecosystem resilience, which indirectly influenced the ecological integrity. In addition, studies have found that fractional vegetation cover could influence the quality of ecosystem health and services in arid regions (Halmy, 2019; Lu et al., 2022). Moreover, suitable natural environmental factors such as climate and topography could improve the growth of vegetation (He et al., 2007; Jiang et al., 2017), which was reflected by the result in which climate and topography could enhance the q value of fractional vegetation cover. We found that the explanatory power of fractional vegetation cover showed an increasing trend from 1990 to 2020. Due to afforestation projects in recent years (Zhang et al., 2021), the condition of vegetation in Alxa Left has improved since 2005, and these ecological restoration projects have led to the increasing weight of fractional vegetation cover.
4.2 Toward a new understanding of deserts
In this study, we established a framework, including ecosystem structure, function and resilience, to evaluate the ecological integrity of the Alxa League, where the main ecosystem type is desert. Although the assessment results showed that the dune region was dominated by low ecological integrity value, compared with other ecosystem, it is hard to say that these places have poor ecosystem health. From 1990 to 2020, the EII changes mainly occurred around rivers and lakes in Ejin or in the grassland and forest in Alxa Left. For example, in eastern Alxa Left, the EII significantly decreased during the study period. In contrast, the great area of dune-covered regions, such as Badain Jaran Desert, is in the middle of Alxa Right, where ecological integrity was stable and barely changed during the study period. A related study also proved that the central regions of dunes were more healthy and stable than the border regions of deserts and grasslands because the central regions had a lower distribution of frequent human activities or natural influences (Cheng et al., 2020).
The pressure from ecological problems, such as land desertification, made the desert situation unpleasant. In our study, we found that desertification caused a negative change in the EII in Alxa Left, by combining land use data between 1990 and 2020. The ecological integrity evaluation could well identify the regions where the ecological environment had worsened. Combined with the results of the factors influencing ecological integrity, our study provides significant guidance for ecological restoration. Moreover, through long-term monitoring and analysis of spatial changes in ecological integrity, we can obtain the regularity of how the ecological integrity state has worsened, which can be applied to other similar regions and provide a reference for ecological protection. At present, hundreds of millions of people inhabit regions classified as desert ecosystems or desertification-prone regions in China (Wang X. M. et al., 2022). Empowering these people under combating desertification also remains a challenge in China (Wang et al., 2023). A previous study showed that positive ecological restoration is the dominant driver of the recent reversal of desertification (Liu et al., 2020). An ecological integrity evaluation and the related analysis could generate information needed for developing suitable and scientific measures for ecological environmental governance in desert regions.
4.3 Limitations and further research
In this study, considering the accessibility of data and the condition of administrative divisions of the Alxa League, we showed the results of ecological integrity based on 5-km grid scales. Taking administrative divisions as the evaluation unit is more suitable for macroscale decision-making and management, but some important information might be lost during the evaluation process, while some details could be obtained well at the grid scale (Peng et al., 2015; Li et al., 2022). Thus, the scale effect could not be ignored during the ecological integrity evaluation. Second, this paper analyzed the driving factors of ecological integrity of the Alxa Left, the further research should force on what measures could protect the ecological integrity and how to improve the ecological integrity, which could provide more significant guidance for local ecological governance.
5 Conclusions
In this study, we established a framework using ecosystem structure, ecosystem function and ecosystem resilience, and evaluated the ecological integrity of the Alxa League from 1990 to 2020.
The ecosystem structure, function and resilience had obvious spatial heterogeneity and barely changed during the study period. In the Alxa League, the ecological intensity degree was always dominated by poor levels during the study periods, and the high value regions were mainly distributed in the middle of the Ejin Banner and the Alxa Left Banner, while low value regions were clustered in the Alxa Right Banner. From 1990 to 2020, the ecological integrity of the Alxa Left Banner had a significant deceasing trend, and we found that compared with dune regions, other ecosystems, such as grassland and water in desert regions, had a much greater possibility of having a negative change in ecological integrity. In the Alxa Left Banner, the ecological integrity was influenced mainly by the land use intensity, and the next most influential factors were fractional vegetation cover and precipitation. The economic factors showed quiet weak influence on the ecological integrity.
Through the evaluation of ecological integrity and the analysis of spatiotemporal variation, we could monitor the fluctuation in the ecological environmental quality of the Alxa League. In addition, this study provides references for ecological restoration based on the results of driving factors.
Data availability statement
The raw data supporting the conclusions of this article will be made available by the authors, without undue reservation.
Author contributions
HS: Data curation, Methodology, Writing – original draft. WC: Methodology, Writing – review & editing. HL: Methodology, Writing – review & editing. XZ: Supervision, Writing – review & editing. LxW : Supervision, Funding acquisition, Writing – review & editing. LW: Supervision, Funding acquisition, Writing – review & editing.
Funding
This work was supported by grants from the Second Tibetan Plateau Scientific Expedition and Research Program (2019QZKK0307), the National Natural Science Foundation of China (31960249, 32160279) and the Science and Technology Major Project of Inner Mongolia (2022YFHH0024, 2022YFHH0017, 2021ZD0011).
Acknowledgments
We acknowledge all the producers of the datasets used in this study. We are also grateful to the reviewers for their helpful comments on the manuscript.
Conflict of interest
The authors declare that the research was conducted in the absence of any commercial or financial relationships that could be construed as a potential conflict of interest.
Publisher’s note
All claims expressed in this article are solely those of the authors and do not necessarily represent those of their affiliated organizations, or those of the publisher, the editors and the reviewers. Any product that may be evaluated in this article, or claim that may be made by its manufacturer, is not guaranteed or endorsed by the publisher.
Supplementary material
The Supplementary Material for this article can be found online at: https://www.frontiersin.org/articles/10.3389/fevo.2023.1266736/full#supplementary-material
References
Alrawaf T. I., Abubakar I. R., Alshabibi N. M., Al-Matar K. M., Dano U. L., Elhadi E. M. A., et al. (2023). The distribution of ecotourism activities and potential consequences for the Saudi desert ecosystem. J. Arid Environ. 213, 1–11. doi: 10.1016/j.jaridenv.2023.104950
Andreasen J. K., O’Neill R. V., Noss R., Slosser N. C. (2001). Considerations for the development of a terrestrial index of ecological integrity. Ecol. Indic. 1, 21–35. doi: 10.1016/S1470-160X(01)00007-3
Angermeier P. L., Karr J. R. (1994). Biological integrity versus biological diversity as policy directives: Protecting biotic resources. Bioscience 44, 690–697. doi: 10.2307/1312512
Barbour M. T., Swietlik W. F., Jackson S. K., Courtemanch D. L., Davies S. P., Yoder C. O. (2000). Measuring the attainment of biological integrity in the USA: a critical element of ecological integrity. Hydrobiologia 422, 453–464. doi: 10.1023/A:1017095003609
Bidak L. M., Kamal S. A., Halmy M. W. A., Heneidy S. Z. (2015). Goods and services provided by native plants in desert ecosystems: Examples from the northwestern coastal desert of Egypt. Global Ecol. Conserv. 3, 433–447. doi: 10.1016/j.gecco.2015.02.001
Blumetto O., Castagna A., Cardozo G., Garcia F., Tiscornia G., Ruggia A., et al. (2019). Ecosystem Integrity Index, an innovative environmental evaluation tool for agricultural production systems. Ecol. Indic. 101, 725–733. doi: 10.1016/j.ecolind.2019.01.077
Borja A., Bricker S. B., Dauer D. M., Demetriades N. T., Ferreira J. G., Forbes A. T., et al. (2008). Overview of integrative tools and methods in assessing ecological integrity in estuarine and coastal systems worldwide. Mar. pollut. Bull. 56, 1519–1537. doi: 10.1016/j.marpolbul.2008.07.005
Chase J. M., Blowes S. A., Knight T. M., Gerstner K., May F. (2020). Ecosystem decay exacerbates biodiversity loss with habitat loss. Nature 584, 238–23+. doi: 10.1038/s41586-020-2531-2
Cheng W. J., Xi H. Y., Sindikubwabo C., Si J. H., Zhao C. G., Yu T. F., et al. (2020). Ecosystem health assessment of desert nature reserve with entropy weight and fuzzy mathematics methods: A case study of Badain Jaran Desert. Ecol. Indic. 119. doi: 10.1016/j.ecolind.2020.106843
Clarke M. L., Rendell H. M. (1998). Climate change impacts on sand supply and the formation of desert sand dunes in the south-west USA. J. Arid Environ. 39, 517–531. doi: 10.1006/jare.1997.0372
Coppedge B. R., Engle D. M., Masters R. E., Gregory M. S. (2006). Development of a grassland integrity index based on breeding bird assemblages. Environ. Monit. Assess. 118, 125–145. doi: 10.1007/s10661-006-1237-8
Costanza R. (2012). Ecosystem health and ecological engineering. Ecol. Eng. 45, 24–29. doi: 10.1016/j.ecoleng.2012.03.023
Costanza R., D’Arge R., De Groot R., Farber S., Grasso M., Hannon B., et al. (1997). The value of the world’s ecosystem services and natural capital. Nature 387, 253–260. doi: 10.1038/387253a0
de Groot R. S., Wilson M. A., Boumans R. M. J. (2002). A typology for the classification, description and valuation of ecosystem functions, goods and services. Ecol. Econ. 41, 393–408. doi: 10.1016/S0921-8009(02)00089-7
Durant S. M., Pettorelli N., Bashir S., Woodroffe R., Wacher T., De Ornellas P., et al. (2012). Forgotten biodiversity in desert ecosystems. Science 336, 1379–1380. doi: 10.1126/science.336.6087.1379
Egoh B., Rouget M., Reyers B., Knight A. T., Cowling R. M., Van Jaarsveld A. S., et al. (2007). Integrating ecosystem services into conservation assessments: A review. Ecol. Econ. 63, 714–721. doi: 10.1016/j.ecolecon.2007.04.007
Fang G. H., Yang J., Chen Y. N., Zammit C. (2015). Comparing bias correction methods in downscaling meteorological variables for a hydrologic impact study in an arid area in China. Hydrol. Earth Syst. Sci. 19, 2547–2559. doi: 10.5194/hess-19-2547-2015
Finlayson M., Cruz R. D., Davidson N., Alder J., Cork S., Groot, Lévêque C., et al. (2005). Millennium Ecosystem Assessment: Ecosystems and human well-being: wetlands and water synthesis. (Washington, D.C: Island Press).
Fischer J., Riechers M., Loos J., Martin-Lopez B., Temperton V. M. (2021). Making the UN decade on ecosystem restoration a social-ecological endeavour. Trends Ecol. Evol. 36, 20–28. doi: 10.1016/j.tree.2020.08.018
Foley J. A., Defries R., Asner G. P., Barford C., bonan G., Carpenter S. R., et al. (2005). Global consequences of land use. Science 309, 570–574. doi: 10.1126/science.1111772
Gao J. X. (2001). Exploration of sustainable development theory: ecological carrying capacity theory, methods and application (Beijing: China Environmental Press).
Gao B., Li X., Li Z., Chen W., He X., Qi S. (2011). Assessment of ecological risk of coastal economic developing zone in Jinzhou Bay based on landscape pattern. Shengtai Xuebao/Acta Ecol. Sin. 31, 3441–3450.
Goodchild M. F., Haining R. P. (2004). GIS and spatial data analysis: Converging perspectives. Papers Regional Sci. 83, 363–385. doi: 10.1007/s10110-003-0190-y
Groot D. D. (1992). Functions of Nature: Evaluation of Nature in Environmental Planning, Management and Decision Making (Groningen,Netherlands: Wolters-Noordhoff B.V.).
Halmy M. W. A. (2019). Assessing the impact of anthropogenic activities on the ecological quality of arid Mediterranean ecosystems (case study from the northwestern coast of Egypt). Ecol. Indic. 101, 992–1003. doi: 10.1016/j.ecolind.2019.02.005
Han J. Q., Wang D. Y., Zhang S. W. (2022). Momoge internationally important wetland: ecosystem integrity remote assessment and spatial pattern optimization study. Land 11. doi: 10.3390/land11081344
He J. H., Pan Z. Z., Liu D. F., Guo X. N. (2019). Exploring the regional differences of ecosystem health and its driving factors in China. Sci. Total Environ. 673, 553–564. doi: 10.1016/j.scitotenv.2019.03.465
He M. Z., Zheng J. G., Li X. R., Qian Y. L. (2007). Environmental factors affecting vegetation composition in the Alxa Plateau, China. J. Arid Environ. 69, 473–489. doi: 10.1016/j.jaridenv.2006.10.005
Holling C. S. (1973). Resilience and stability of ecological systems. Annu. Rev. Ecol. Evol. System. 4, 1–23. doi: 10.1146/annurev.es.04.110173.000245
Howell P. E., Muths E., Hossack B. R., Sigafus B. H., Chandler R. B. (2018). Increasing connectivity between metapopulation ecology and landscape ecology. Ecology 99, 1119–1128. doi: 10.1002/ecy.2189
Huang B., Ouyang Z., Zheng H., Wang X., Miao H. (2006). Connotation of ecological integrity and its assessment methods: A review. Yingyong Shengtai Xuebao 17, 2196–2202.
Hughes T. P., Baird A. H., Bellwood D. R., Card M., Connolly S. R., Folke C., et al. (2003). Climate change, human impacts, and the resilience of coral reefs. Science 301, 929–933. doi: 10.1126/science.1085046
Hughes T. P., Kerry J. T., Baird A. H., Connolly S. R., Dietzel A., Eakin C. M., et al. (2018). Global warming transforms coral reef assemblages. Nature 556, 492–49+. doi: 10.1038/s41586-018-0041-2
Jiang M. Z., Chen H. Y., Chen Q. H., Wu H. Y., Chen P. (2015). Wetland ecosystem integrity and its variation in an estuary using the EBLE index. Ecol. Indic. 48, 252–262. doi: 10.1016/j.ecolind.2014.08.008
Jiang L. L., Jiapaer G., Bao A. M., Guo H., Ndayisaba F. (2017). Vegetation dynamics and responses to climate change and human activities in Central Asia. Sci. Total Environ. 599, 967–980. doi: 10.1016/j.scitotenv.2017.05.012
Jiang M. Z., Pang X. P., Wang J. J., Cao C. (2018). Islands ecological integrity evaluation using multi sources data. Ocean Coast. Manage. 158, 134–143. doi: 10.1016/j.ocecoaman.2018.03.033
Kaiser W., Avenant N. L., Haddad C. R. (2009). Assessing the ecological integrity of a grassland ecosystem: the applicability and rapidity of the SAGraSS method. Afr. J. Ecol. 47, 308–317. doi: 10.1111/j.1365-2028.2008.00962.x
Karr J. R. (1996). Ecological Integrity and Ecological Health Are Not the Same. National Academy of Engineering. (Engineering Within Ecological Constraints. Washington, DC: The National Academies Press). doi: 10.17226/4919
Karr J. R., Dudley D. R. (1981). Ecological perspective on water quality goals. Environ. Manage. 5, 55–68. doi: 10.1007/BF01866609
Kay J. J. (1991). A nonequilibrium thermodynamic framework for discussing ecosystem integrity. Environ. Manage. 15, 483–495. doi: 10.1007/BF02394739
Khan A. A., Ruby T., Naz N., Rafay M. (2017). “Desert ecosystem management: A sustainable and wise use,” in Ecosystem Functions and Management: Theory and Practice. Ed. Sandhu H. (Cham: Springer International Publishing).
Kijima M., Nishide K., Ohyama A. (2010). Economic models for the environmental Kuznets curve: A survey. J. Econ. Dyn. Control 34, 1187–1201. doi: 10.1016/j.jedc.2010.03.010
Lausch A., Erasmi S., King D. J., Magdon P., Heurich M. (2017). Understanding forest health with remote sensing-part II—A review of approaches and data models. Remote Sens. 9, 129. doi: 10.3390/rs9020129
Lavorel S., Grigulis K., Leitinger G., Kohler M., Schirpke U., Tappeiner U. (2017). Historical trajectories in land use pattern and grassland ecosystem services in two European alpine landscapes. Regional Environ. Change 17, 2251–2264. doi: 10.1007/s10113-017-1207-4
Law K. L., Annual R. (2017). Plastics in the marine environment. Annu. Rev. Mar. Sci. 9, 205–229. doi: 10.1146/annurev-marine-010816-060409
Li G. D., Fang C. L., Wang S. J. (2016). Exploring spatiotemporal changes in ecosystem-service values and hotspots in China. Sci. Total Environ. 545, 609–620. doi: 10.1016/j.scitotenv.2015.12.067
Li Q. W., Jin T. T., Peng Q. D., Lin J. Q., Zhang D., Huang J. T., et al. (2022). Identifying the extent of the spatial expression of landscape fragmentation based on scale effect analysis in Southwest China. Ecol. Indic. 141. doi: 10.1016/j.ecolind.2022.109120
Li Y. R., Zhang X. C., Cao Z., Liu Z. J., Lu Z., Liu Y. S. (2021). Towards the progress of ecological restoration and economic development in China’s Loess Plateau and strategy for more sustainable development. Sci. Total Environ. 756. doi: 10.1016/j.scitotenv.2020.143676
Liang M. W., Chen J. Q., Smith N. G., Bai X., Jia C. Z., Li Z. Y., et al. (2019). Changes and regulations of net ecosystem CO2 exchange across temporal scales in the Alxa Desert. J. Arid Environ. 164, 78–84. doi: 10.1016/j.jaridenv.2019.01.016
Liu H., Wang R. Z., Sun H. Y., Cao W. J., Song J., Zhang X. F., et al. (2023). Spatiotemporal evolution and driving forces of ecosystem service value and ecological risk in the Ulan Buh Desert. Front. Environ. Sci. 10. doi: 10.3389/fenvs.2022.1053797
Liu Q. F., Zhang Q., Yan Y. Z., Zhang X. F., Niu J. M., Svenning J. C. (2020). Ecological restoration is the dominant driver of the recent reversal of desertification in the Mu Us Desert (China). J. Clean. Prod. 268. doi: 10.1016/j.jclepro.2020.122241
Lu Y. Y., Xu X. L., Zhao J. H., Han F. (2022). Spatiotemporal evolution of mountainous ecosystem services in an arid region and its influencing factors: A case study of the Tianshan mountains in Xinjiang. Land 11. doi: 10.3390/land11122164
Ma X., Xu X., Li J. (2022). Changes of terrestrial ecosystem integrity index and driving factors in the Yangtze River Delta from 2000 to 2018. Acta Ecol. Sin. 42, 3738–3748. doi: 10.5846/stxb202011263035
MacGregor-Fors I., Falfan I., Garcia-Arroyo M., Lemoine-Rodriguez R., Gomez-Martinez M. A., Marin-Gomez O. H., et al. (2022). A novel approach for the assessment of cities through ecosystem integrity. Land 11. doi: 10.3390/land11010003
Maloney E. M. (2019). How do we take the pulse of an aquatic ecosystem? Current and historical approaches to measuring ecosystem integrity. Environ. Toxicol. Chem. 38, 289–301. doi: 10.1002/etc.4308
Middleton N. J. (2017). Desert dust hazards: A global review. Aeolian Res. 24, 53–63. doi: 10.1016/j.aeolia.2016.12.001
Moog O., Chovanec A. (2000). Assessing the ecological integrity of rivers: walking the line among ecological, political and administrative interests(*). Hydrobiologia 422, 99–109. doi: 10.1023/A:1017053829050
Mora F. (2017). A structural equation modeling approach for formalizing and evaluating ecological integrity in terrestrial ecosystems. Ecol. Inf. 41, 74–90. doi: 10.1016/j.ecoinf.2017.05.002
Ordóñez C., Duinker P. N. (2012). Ecological integrity in urban forests. Urban Ecosyst. 15, 863–877. doi: 10.1007/s11252-012-0235-6
Ou W., Wang H., Tao Y. (2018). A land cover-based assessment of ecosystem services supply and demand dynamics in the Yangtze River Delta region. Acta Ecol. Sin. 38, 6337–6347. doi: 10.5846/stxb201707061225
Ozkundakci D., Hamilton D. P., Kelly D., Schallenberg M., De Winton M., Verburg P., et al. (2014). Ecological integrity of deep lakes in New Zealand across anthropogenic pressure gradients. Ecol. Indic. 37, 45–57. doi: 10.1016/j.ecolind.2013.10.005
Pan N. H., Guan Q. Y., Wang Q. Z., Sun Y. F., Li H. C., Ma Y. R. (2021a). Spatial Differentiation and Driving Mechanisms in Ecosystem Service Value of Arid Region:A case study in the middle and lower reaches of Shule River Basin, NW China. J. Clean. Prod. 319. doi: 10.1016/j.jclepro.2021.128718
Pan Z. Z., He J. H., Liu D. F., Wang J. W., Guo X. A. (2021b). Ecosystem health assessment based on ecological integrity and ecosystem services demand in the Middle Reaches of the Yangtze River Economic Belt, China. Sci. Total Environ. 774. doi: 10.1016/j.scitotenv.2020.144837
Parrish J. D., Braun D. P., Unnasch R. S. (2003). Are we conserving what we say we are? Measuring ecological integrity within protected areas. Bioscience 53, 851–860. doi: 10.1641/0006-3568(2003)053[0851:AWCWWS]2.0.CO;2
Peng J., Liu Y. X., Li T. Y., Wu J. S. (2017). Regional ecosystem health response to rural land use change: A case study in Lijiang City, China. Ecol. Indic. 72, 399–410. doi: 10.1016/j.ecolind.2016.08.024
Peng J., Liu Y. X., Wu J. S., Lv H. L., Hu X. X. (2015). Linking ecosystem services and landscape patterns to assess urban ecosystem health: A case study in Shenzhen City, China. Landscape Urban Plann. 143, 56–68. doi: 10.1016/j.landurbplan.2015.06.007
Peng J., Pan Y. J., Liu Y. X., Zhao H. J., Wang Y. L. (2018). Linking ecological degradation risk to identify ecological security patterns in a rapidly urbanizing landscape. Habitat Int. 71, 110–124. doi: 10.1016/j.habitatint.2017.11.010
Qu J. H., Fan M. H. (2010). The current state of water quality and technology development for water pollution control in China. Crit. Rev. Environ. Sci. Technol. 40, 519–560. doi: 10.1080/10643380802451953
Ran C., Wang S. J., Bai X. Y., Tan Q., wu L. H., Luo X. L., et al. (2021). Evaluation of temporal and spatial changes of global ecosystem health. Land Degrad. Dev. 32, 1500–1512. doi: 10.1002/ldr.3813
Revenga C. (2005). Developing indicators of ecosystem condition using geographic information systems and remote sensing. Regional Environ. Change 5, 205–214. doi: 10.1007/s10113-004-0085-8
Roche P. K., Campagne C. S. (2017). From ecosystem integrity to ecosystem condition: a continuity of concepts supporting different aspects of ecosystem sustainability. Curr. Opin. Environ. Sustain. 29, 63–68. doi: 10.1016/j.cosust.2017.12.009
Seidl N. P., Golobic M. (2020). Quantitative assessment of agricultural landscape heterogeneity. Ecol. Indic. 112. doi: 10.1016/j.ecolind.2020.106115
Shao W. Y., Wang Q. Z., Guan Q. Y., Zhang J., Yang X. Y., Liu Z. (2023). Environmental sensitivity assessment of land desertification in the Hexi Corridor, China. Catena 220. doi: 10.1016/j.catena.2022.106728
Slemnev N. N., Gunin P. D. (2000). Functioning of desert ecosystems of Gobi in Mongolia. Rastitel’nye Resursy 36, 24–43.
Stevens L. E., Aly A. A., Arpin S. M., Apostolova I., Ashley G. M., Barba P. Q., et al. (2021). Imperiled: The Encyclopedia of Conservation. Reference Module Earth Syst. Environ. Sci. (Oxford: Elsevier), 436–451. doi: 10.1016/B978-0-12-821139-7.00111-2
Styers D. M., Chappelka A. H., Marzen L. J., Somers G. L. (2010). Developing a land-cover classification to select indicators of forest ecosystem health in a rapidly urbanizing landscape. Landscape Urban Plann. 94, 158–165. doi: 10.1016/j.landurbplan.2009.09.006
Taylor N. T., Davis K. M., Abad H., Mcclung M. R., Moran M. D. (2017). Ecosystem services of the Big Bend region of the Chihuahuan Desert. Ecosyst. Serv. 27, 48–57. doi: 10.1016/j.ecoser.2017.07.017
Tierney G. L., Faber-Langendoen D., Mitchell B. R., Shriver W. G., Gibbs J. P. (2009). Monitoring and evaluating the ecological integrity of forest ecosystems. Front. Ecol. Environ. 7, 308–316. doi: 10.1890/070176
Wang X., Ge Q., Geng X., Wang Z., Gao L., Bryan B. A., et al. (2023). Unintended consequences of combating desertification in China. Nat. Commun. 14, 1139. doi: 10.1038/s41467-023-36835-z
Wang X. M., Geng X., Liu B., Cai D. W., Li D. F., Xiao F. Y., et al. (2022a). Desert ecosystems in China: Past, present, and future. Earth Sci. Rev. 234. doi: 10.1016/j.earscirev.2022.104206
Wang X., Geng X., Li D., Xiao F., Cai D. (2022b). Atlas of Desert Ecosystems in China (Beijing: Sino Maps Press).
Wang X. Z., Wu J. Z., Liu Y. L., Hai X. Y., Shanguan Z. P., Deng L. (2022c). Driving factors of ecosystem services and their spatiotemporal change assessment based on land use types in the Loess Plateau. J. Environ. Manage. 311. doi: 10.1016/j.jenvman.2022.114835
Wang J. F., Zhang T. L., Fu B. J. (2016). A measure of spatial stratified heterogeneity. Ecol. Indic. 67, 250–256. doi: 10.1016/j.ecolind.2016.02.052
Warren A. (1981). Research on desert ecology: review. Geograph. J. 147, 215–217. doi: 10.2307/634538
Whitford W. G., Rapport D. J., Desoyza A. G. (1999). Using resistance and resilience measurements for “fitness” tests in ecosystem health. J. Environ. Manage. 57, 21–29. doi: 10.1006/jema.1999.0287
Wurtzebach Z., Schultz C. (2016). Measuring ecological integrity: history, practical applications, and research opportunities. Bioscience 66, 446–457. doi: 10.1093/biosci/biw037
Xiao R., Liu Y., Fei X. F., Yu W. X., Zhang Z. H., Meng Q. X. (2019). Ecosystem health assessment: A comprehensive and detailed analysis of the case study in coastal metropolitan region, eastern China. Ecol. Indic. 98, 363–376. doi: 10.1016/j.ecolind.2018.11.010
Xiao Z. L., Liu R., Gao Y. H., Yang Q. Y., Chen J. L. (2022). Spatiotemporal variation characteristics of ecosystem health and its driving mechanism in the mountains of southwest China. J. Clean. Prod. 345. doi: 10.1016/j.jclepro.2022.131138
Xie G. D., Lu C. X., Leng Y. F., Zheng D., Li S. C. (2003). Ecological assets valuation of the Tibetan Plateau. J. Natural Resour. 18, 189–196. doi: 10.11849/zrzyxb.2003.02.010
Xie G. D., Zhang C. X., Zhang L. M., Chen W. H., Li S. M. (2015). Improvement of the evaluation method for ecosystem service value based on per unit area. J. Of Natural Resour. 30, 1243–1254. doi: 10.11849/zrzyxb.2015.08.001
Xu D., Cai Z., Xu D., Lin W. P., Gao J., Li L. B. (2022). Land use change and ecosystem health assessment on Shanghai-Hangzhou bay, Eastern China. Land 11. doi: 10.3390/land11060867
Zeleny J., Mercado-Bettin D., Muller F. (2021). Towards the evaluation of regional ecosystem integrity using NDVI, brightness temperature and surface heterogeneity. Sci. Total Environ. 796. doi: 10.1016/j.scitotenv.2021.148994
Zhang X., Huang T., Zhang L., Shen Y., Zhao Y., Gao H., et al. (2016). Three-North Shelter Forest Program contribution to long-term increasing trends of biogenic isoprene emissions in northern China. Atmos. Chem. Phys. 16, 6949–6960. doi: 10.5194/acp-16-6949-2016
Zhang X. Y., Xu D. Y., Wang Z. Y. (2021). Optimizing spatial layout of afforestation to realize the maximum benefit of water resources in arid regions: A case study of Alxa, China. J. Clean. Prod. 320. doi: 10.1016/j.jclepro.2021.128827
Zhao Z., Yang R. (2021). The concept of national park authenticity and integrity in China and its evaluation framework. Biodivers. Sci. 29, 1271–1278. doi: 10.17520/biods.2021287
Zhao R., Zhan L. P., Yao M. X., Yang L. C. (2020). A geographically weighted regression model augmented by Geodetector analysis and principal component analysis for the spatial distribution of PM2.5. Sustain. Cities Soc. 56. doi: 10.1016/j.scs.2020.102106
Zhou Y. Y., Yue D. X., Li S. Y., Liang G., Chao Z. Z., Zhao Y., et al. (2022). Ecosystem health assessment in debris flow-prone areas: A case study of Bailong River Basin in China. J. Clean. Prod. 357. doi: 10.1016/j.jclepro.2022.131887
Zhu L. J., Meng J. J., Zhu L. K. (2020). Applying Geodetector to disentangle the contributions of natural and anthropogenic factors to NDVI variations in the middle reaches of the Heihe River Basin. Ecol. Indic. 117. doi: 10.1016/j.ecolind.2020.106545
Keywords: the Alxa League, ecological integrity, desert ecosystem, ecosystem evaluation, driving factors
Citation: Sun H, Cao W, Liu H, Zhang X, Wang L and Wen L (2023) Evaluation and driving factors of ecological integrity in the Alxa League from 1990 to 2020. Front. Ecol. Evol. 11:1266736. doi: 10.3389/fevo.2023.1266736
Received: 25 July 2023; Accepted: 13 September 2023;
Published: 27 September 2023.
Edited by:
Changchun Huang, Nanjing Normal University, ChinaReviewed by:
Winfried Voigt, Friedrich Schiller University Jena, GermanyQinghe Zhao, Henan University, China
Copyright © 2023 Sun, Cao, Liu, Zhang, Wang and Wen. This is an open-access article distributed under the terms of the Creative Commons Attribution License (CC BY). The use, distribution or reproduction in other forums is permitted, provided the original author(s) and the copyright owner(s) are credited and that the original publication in this journal is cited, in accordance with accepted academic practice. No use, distribution or reproduction is permitted which does not comply with these terms.
*Correspondence: Lu Wen, d2VubHVAaW11LmVkdS5jbg==