- Department of Biology, Maynooth University, Maynooth, Ireland
Photorhabdus spp. are the only known terrestrial bioluminescent bacteria. We show that the bioluminescence produced by these bacteria reduces scavenging activity on the insect cadavers they colonize. Photorhabdus spp. are the symbiont of the insect pathogenic nematodes Heterorhabditis spp. Together they kill insects and colonize the cadaver. The function of their bioluminescence has been the subject of debate, but here for the first time we demonstrate an ecological benefit. In our experiments, fewer Photorhabdus temperata -infected cadavers than uninfected cadavers were scavenged, but only in dark conditions where their bioluminescence would be visible. This was the case both in the field and in laboratory experiments with Lehmannia valentiana slugs (the primary scavengers found in our field tests). We also show that L. valentiana is innately deterred from scavenging on uninfected cadavers in proximity to light imitating the bioluminescence of Photorhabdus, indicating that luminescence can be a deterrent independent of chemical cues. We propose a multimodal defence where bioluminescence works together with the chemical defences also produced by Photorhabdus to deter scavengers, such as slugs, from feeding on the host cadaver, with the potential for aposematism.
1 Introduction
Bioluminescence is the biochemical production and emission of light by a living organism (Widder, 1999), usually by the oxidation of a light-emitting molecule -a luciferin - by a luciferase enzyme (Haddock et al., 2010). Bioluminescence has evolved many times (Haddock et al., 2010; Lau and Oakley, 2021) in prokaryotes and eukaryotes including bacteria, fungi, dinoflagellates, arthropods, and fish (Herring, 1994; Dunlap and Kita-Tsukamoto, 2006; Forey and Patterson, 2006; Baker et al., 2008). The original function of luciferin-luciferase systems may have been for detoxification of reactive oxygen species (McElroy and Seliger, 1962; Seliger, 1975; Rees et al., 1998; Labas et al., 2001), but bioluminescence now serves diverse ecological functions including defence, attraction of prey, and intraspecific communication (Herring, 1989; Buck and Case, 2002; Lewis and Cratsley, 2008). Distraction or deterrence of nocturnal predators and scavengers has been proposed as the function of bioluminescence in Dinoflagellata, Copepoda, Cnidaria and Coleoptera (Morin, 1983; Buskey and Swift, 1985; Latz et al., 1988; Branham and Wenzel, 2001; Herring and Widder, 2004).
In bacteria, while the mechanism of luminescence is well characterised (Meighen and Szittner, 1992; Forst and Nealson, 1996; Zavilgelsky and Shakulov, 2018), its functions are less well understood than in higher organisms (Nealson and Hastings, 1979; Timsit et al., 2021). Amongst the biochemical benefits that have been suggested are the detoxification of reactive oxygen species (Timmins et al., 2001) or the promotion of DNA repair (Czyz et al., 2000), but the likely importance of ecological functions involving perception by some other organisms is increasingly acknowledged (Nealson and Hastings, 1979; Zavilgelsky and Shakulov, 2018; Timsit et al., 2021). Many marine species of bioluminescent bacteria reside in specialised light organs of higher organisms (fish and squid) where they clearly provide light in return for nutrients from their host, whilst other marine species are postulated to use light to attract consumers that provide a medium for growth and a means of dispersal (Nealson and Hastings, 1979; Widder, 2010; Tanet et al., 2020; Ramesh and Bessho-Uehara, 2021). Photorhabdus spp. are the only known terrestrial bioluminescent bacteria; the function of the bioluminescence in this genus is debated (Peat and Adams, 2008; Waterfield et al., 2009; Zavilgelsky and Shakulov, 2018). Photorhabdus spp. are insect pathogens that occur as obligate symbionts of the Heterorhabditis spp. of nematodes. (Boemare, 2002; Clarke, 2008; Waterfield et al., 2009). The stress resistant, free-living infective juvenile stage of the nematode travels through soil and enters a living insect, releasing the bacteria from its gut. When the bacteria have grown to a certain density, they emit light at a wavelength of around 490 nm (Poinar et al., 1980; Maher et al., 2021). Pigmentation of the cadaver can shift the light emitted by 20-30 nm depending on the species of Photorhabdus and its characteristic colouration (Maher et al., 2021). Luminescence can start as early as 20 hours post infection and is visible to the human eye by 72 hrs (Poinar et al., 1980), but only in darkness. Buried in the soil, and/or at night, these luminescent insect cadavers would stand out as a source of light. Within 2-3 days of infection the bacteria help to kill the insect and enzymatically break down the cadaver, resulting in a bacteria-rich nutrient soup on which the nematodes feed (Waterfield et al., 2009; Dillman and Sternberg, 2012). The nematodes then develop to adulthood and reproduce within the host. Several generations may be produced over 2-3 weeks, until resources are depleted, after which the next generation of infective juveniles with their symbiont leave the cadaver in search of new hosts (Adams and Nguyen, 2002). Only the infective juvenile stage is capable of survival in soil conditions (Poinar, 1979).
Functions that have been ascribed to bioluminescence in Photorhabdus can be divided into: general biochemical functions, roles specialised to its ecological niche, and redundancy (Poinar et al., 1980; Akhurst and Boemare, 1990; Peat and Adams, 2008; Waterfield et al., 2009; Peat et al., 2010; Zavilgelsky and Shakulov, 2018; Timsit et al., 2021). The lux operon containing the genes responsible for bacterial bioluminescence had a single evolutionary origin and spread by horizontal gene transfer (Kasai et al., 2006); many of the common functions that are ascribed to the cellular processes that result in bacterial bioluminescence, including protection against free radicals and stimulation of DNA repair, have also been ascribed to Photorhabdus (Nealson and Hastings, 1979; Peat and Adams, 2008; Zavilgelsky and Shakulov, 2018). Amongst the adaptive functions related to Photorhabdus’s specialised niche that have been proposed are: signalling to the nematode to synchronise symbiosis (Waterfield et al., 2009); cell-to-cell communication with other bacteria (Timsit et al., 2021); attracting new insect hosts to the cadaver (Poinar et al., 1980; Patterson et al., 2015) or deterring scavengers from it (Akhurst and Boemare, 1990; Baur et al., 1998; Jones et al., 2015; Maher et al., 2021). It has also been suggested that since the acquisition by Photorhabdus of the lux operon from a marine bacterium, bioluminescence is a nonfunctional trait in the genus that is declining over time, due to lack of selection pressure in the terrestrial environment (Peat and Adams, 2008). The most widely hypothesised possible ecological function proposed for bioluminescence in Photorhabdus is deterring scavengers from damaging the host cadaver, but to date there is no evidence supporting this or any other proposed function (Poinar et al., 1980; Akhurst and Boemare, 1990; Peat and Adams, 2008; Waterfield et al., 2009; Peat et al., 2010; Zavilgelsky and Shakulov, 2018; Timsit et al., 2021; Cimen, 2023).
There is ample evidence that Photorhabdus spp. invest heavily in defence of the cadaver against both microbes and animals (reviewed by Raja et al., 2021), protecting both themselves and their essential nematode mutualist. They produce a wide variety of molecules (Bode, 2009; Cimen, 2023), some of which have antimicrobial activity and suppress competing bacteria and fungi - including agents of putrefaction (Li et al., 1995; Eleftherianos et al., 2007; Ullah et al., 2015; Tobias et al., 2018). Insect cadavers infected by Heterorhabditis and their symbionts are also protected against feeding by a variety of scavengers including ants, wasps, crickets and beetles, and this is attributed to the production of an as yet unidentified chemical called “scavenger deterrent factor” by Photorhabdus (Baur et al., 1998; Zhou et al., 2002; Gulcu et al., 2012; Ulug et al., 2014; Gulcu et al., 2018; Raja et al., 2021). There is some evidence that olfactory cues contribute to scavenger deterrence (Jones et al., 2015), and that the distinct red colouration typical of many Photorhabdus-infected cadavers can serve as a warning signal of unpalatability for daytime scavengers such as birds (Fenton et al., 2011; Jones et al., 2017). Jones et al. (2017) proposed that a multimodal defence – chemical and visual – due to Photorhabdus may protect the cadaver, either by acting in concert and/or by being effective against different scavengers.
Here, we test the hypothesis that bioluminescence contributes to the protection of Photorhabdus-infected cadavers against nocturnal scavengers (Akhurst and Boemare, 1990), using Photorhabdus temperata Fischer-Lesaux, Villard, Brunnel, Normand & Boemare, carried by the nematode Heterorhabditis downesi Stock, Griffin & Burnell. Photorhabdus temperata emits light at a high intensity relative to other species of Photorhabdus tested (Hyrsl et al., 2004; Maher et al., 2021). We tracked the luminescence profile produced by P. temperata infected cadavers over the course of the infection to determine at what stage the luminescence would have its highest impact. In field trials at dusk, we compare scavenging on infected and uninfected insect cadavers under conditions either of ambient light, where only chemical defence is expected, or in darkness, where bioluminescence may be visible and contribute to cadaver defence. We conduct similar experiments in the laboratory using the scavenger encountered most commonly in the field trials, the slug Lehmannia valentiana Férussac. Most slugs are omnivorous, nocturnal scavengers that feed on dead vegetation, fungi, dead animals, and other detritus (Barnes and Weil, 1945; Jennings and Barkham, 1975; Keller and Snell, 2002). While they possess simple eyes (and certain light sensitive areas of the brain) that can detect light intensity, they do not form clear images (Zieger et al., 2009; Nishiyama et al., 2019) and seek food using olfactory cues (Gelperin, 1974; Gelperin, 1975; Kiss, 2017). Where organisms are protected in more than one modality, it can be difficult to disentangle the contribution of each modality to defence; artificial light sources are an important tool in demonstrating a defensive role of light where chemical defences are also effective (Underwood et al., 1997; Marek et al., 2011). We use light emitting diodes (LEDs) producing artificial light simulating that of a Photorhabdus-infected cadaver at peak luminescence in a choice experiment to test the effect of light alone on L. valentiana orientation and scavenging of freeze killed insects.
2 Methods
2.1 Species, storage and conditioning
Late instar Galleria mellonella L. larvae were supplied by Peregrine Live Foods (Chipping Ongar, UK) and Live Foods Direct (North Anston, UK). They were infected with H. downesi K122 (a native Irish strain carrying Photorhabdus temperata that imparts a yellow-orange colour to the infected cadaver) by applying 100 infective juveniles to each larva. The insects were incubated at 20°C and died 3 days later, and these freshly dead cadavers were used in experiments. Relative light intensity of the cadavers was recorded using 5-minute exposure in a Syngene G:Box Chemi HR16 BioImaging System using GeneSnap 7.12 software (SynGene, Cambridge, UK). Images (16 bit) were exported to Image J (v1.5) and analysed for mean grey value/pixel. To determine peak luminescence over the course of infection, the luminescence produced by two sets of infected G. mellonella (N = 13, 19) were measured at alternating 2 hr intervals from 1-19 days post infection.
Freshly dead cadavers at day 3 of infection with relative light intensity 24,723 ± 643.58 (mean ± SE) mean grey scale units/pixel were used in experiments. As controls, G. mellonella were frozen for 24 hours and thawed 2 hours prior to use.
Slugs (Lehmannia valentiana; synonym Limax valentianus) were collected from mature deciduous woodland. Identification of the collected slugs was confirmed by dissection and examination of genitalia (Forsyth, 2004) of a proportion (~10%) of the population. The slugs were maintained in plastic containers with a thin layer of damp soil at 15°C and 16:8 Light: Dark for 1 week, during which time they were fed fresh vegetables and dog food kibble (Bakers Small Dog, Purina, UK). Slugs used in experiments were 5-6 cm in length (fully extended).
2.2 Does bioluminescence deter nocturnal scavenging (field study)
Field trials were conducted at dusk (starting 2 hrs before sunset), in open deciduous woodland with ivy/grass ground cover. Ambient light levels were <200 lux at soil level (recorded using an RT MT30 digital lux meter). A single H. downesi-infected or freeze-killed cadaver was placed in a Petri dish (3.5 cm) with a hole (4 mm diam.) in the base to allow drainage. Each dish was placed in a shallow (2-3 cm deep) hole in the soil which was covered with a rigid polypropylene sheet (5 x 6 cm) resting on the soil surface. The cover was either translucent or opaque (black) to allow or prevent, respectively, light from entering the test arena below. There were thus four treatments, infected or freeze-killed cadavers in light or dark conditions (Figure 1A). Dishes were arranged in groups of 4 (1 dish of each treatment), with all dishes and all groups 1 m apart. After 2 hrs the number and identity of invertebrates in the dishes were recorded and the dishes were returned to the laboratory. Signs of scavenging were recorded and classed as: cadaver fully consumed, partly consumed, or small punctures in the cuticle. In addition, each cadaver was weighed at the start and end of the experiment and the proportion of weight lost was recorded.
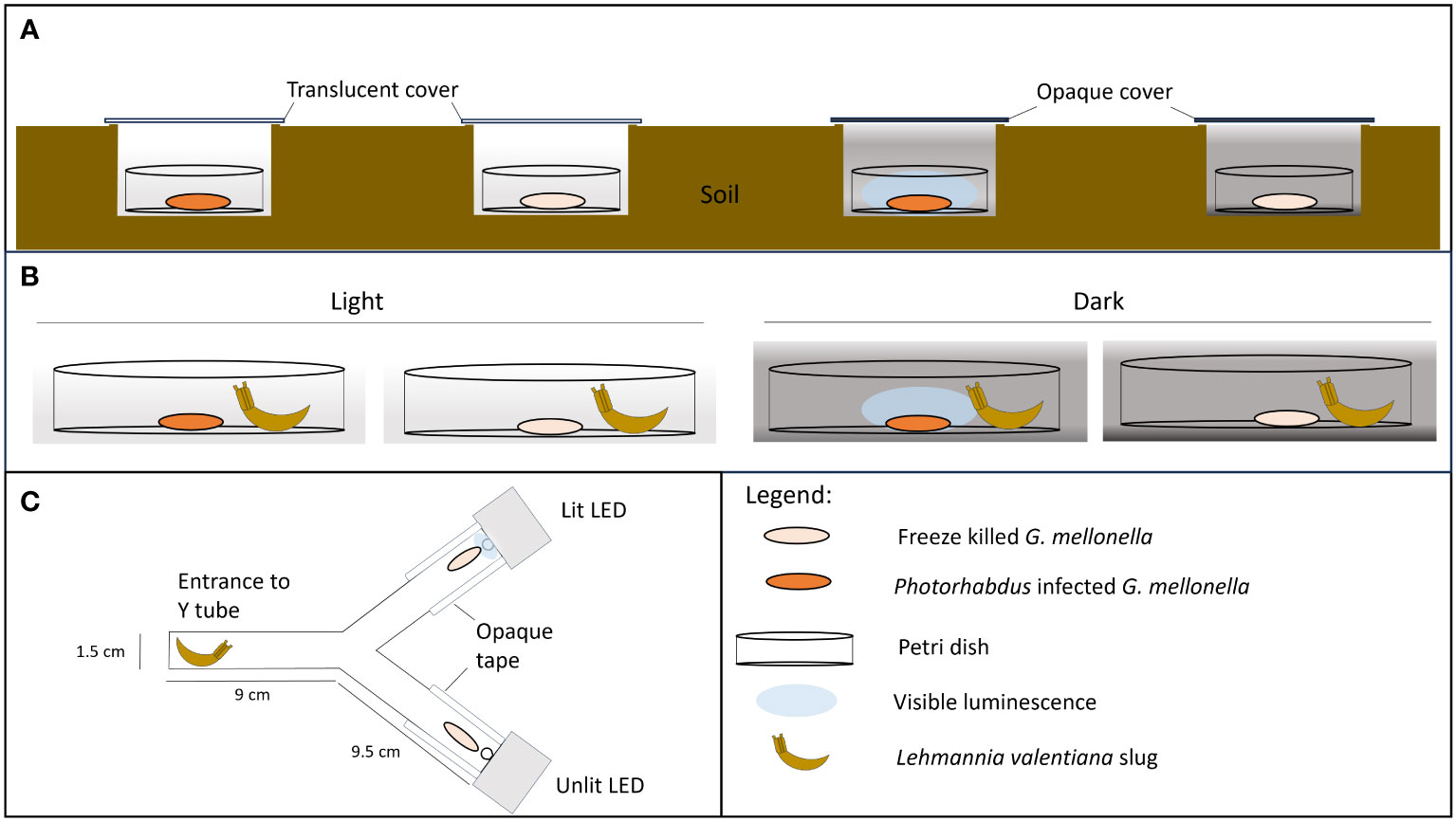
Figure 1 Experimental set up. (A, B) No-choice trials in which scavenging is recorded on Photorhabdus temperata-infected insect cadavers under conditions where bioluminescence is visible or not. Freeze-killed insects are included as controls. (A) Field trial: Insect cadavers are in soil pits covered with either translucent or black polypropylene squares, to allow or block light from entering the pit. (B) Lab trial: Insect cadavers are exposed to a scavenger slug (L. valentiana) under conditions of either light or dark. (C) Two-choice apparatus with either a lit LED (emitting at 470 nm) or unlit LED light positioned behind a freeze-killed insect at the end of each arm. A slug is placed in the entrance chamber at the start of the experiment.
The were 6 trials for this field study, from June to November 2021, at two woodland locations in County Kildare: Maynooth University Campus and Carton Demesne (Supplementary Table 1). Overall, a total of 426 cadavers were tested, 103-108 per treatment.
2.3 Does bioluminescence deter slugs (L. valentiana) from feeding? (lab study)
Based on the findings of the field trials, the slug L. valentiana was used as a model scavenger in lab trials. The slugs were allowed to feed on either a H. downesi-infected or freeze killed cadaver under conditions in which the bioluminescence would either be visible (i.e., in darkness) or not visible (i.e. in low level ambient light (8-20 lux) provided by an LED bench lamp. Slugs which had been starved for 24 hrs before the experiment were placed individually in 9 cm Petri dishes, 2 cm from the centre of the dish. A cadaver (either infected or freeze-killed) was then introduced to the centre of each dish. As in the field trial, there were thus four treatments, infected or freeze-killed cadavers in light or dark conditions (Figure 1B). Slugs were observed for 2 hours, and their behaviour was recorded using the following categories: moving (within or outside a 2 cm radius of the cadaver), feeding (rasping the cadaver), tasting (touching the cadaver with mouth parts), searching (rotation of the tentacles), or none of the above. Red light was used for observations in the dark condition. The experiment was conducted at 16°C. After 5 hrs the cadavers were removed, and signs of scavenging were noted. The weight of each cadaver was recorded before and after the trial and the proportion weight loss was calculated. There were 18 trials for this lab study. Overall, a total of 569 slugs were tested, 141-144 slugs per treatment.
2.4 Does cadaver-mimicking light attract or repel slugs, or affect their feeding?
In order to separate the effects of light from those of chemical defences, a L. valentiana slug was given a choice between two freeze-killed insects; one of which was paired with artificial light of a similar wavelength and intensity to that of a Photorhabdus infected insect, and the other was not. The experiment was carried out in the dark, at 16°C. A freeze-killed G. mellonella was placed at the end of each arm of a Y shaped tube directly in front of a 5 mm-diam. LED (HLMP-AB64/65, Avago Technologies Inc., USA) which was either lit or unlit (Figure 1C). The lit diodes emitted light at 470 nm and the relative light intensity (measured as described for cadavers) was adjusted to fall within the range 15,000-35,000 (mean 24303) mean grey scale units/pixel to mimic the intensity of light emitted by bioluminescent cadavers at days 3-6 of infection (Figure 2). The sides of each arm were covered with opaque tape and tubes were orientated in different directions to avoid positional effects. A single L. valentiana slug that had been starved for 24-48 hrs was placed in the entrance chamber of the Y-tube, and its behaviour was observed for 2 hrs under red light. The time spent in each of the following behavioural categories was recorded for each arm: moving, feeding, tasting, searching or none of the above. After 5 hrs the cadavers were removed and examined for signs of scavenging. The proportion of weight loss of each cadaver was calculated. The experiment was conducted across 13 trials with a total of 98 slugs tested.
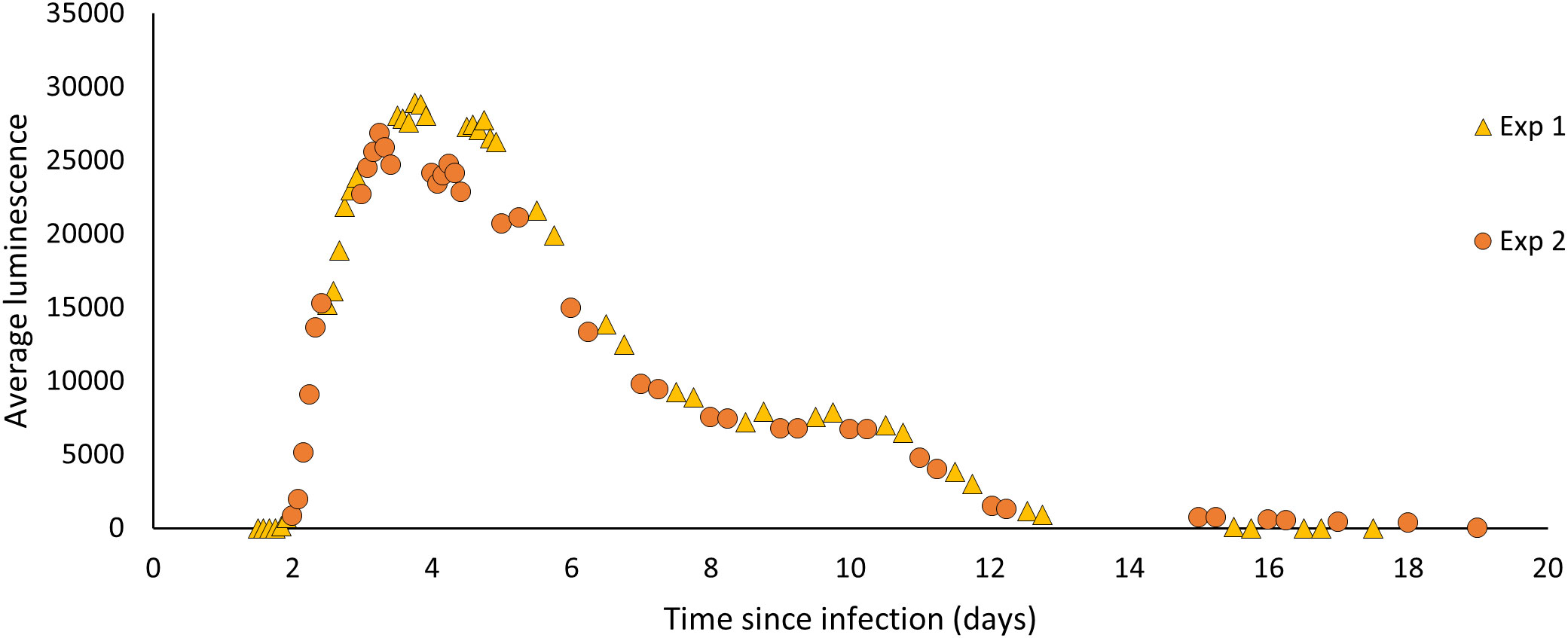
Figure 2 The average luminescence (mean grey scale units/pixel) produced by P. temperata-infected G. mellonella from time of exposure to time of emergence. Larvae infected with 100 IJs of H. downesi were measured at 2-4 hr intervals for two sets of infections (Experiment 1 and 2).
2.5 Data analysis
For each experiment, the results for repeated trials were pooled prior to analysis. The incidence of scavenging was analysed using Pearson’s Chi square with significance set at P < 0.05, and with Bonferroni correction for multiple post hoc comparisons. Weight loss of cadavers in field and lab trials, and proportion of time spent feeding, moving, tasting, and searching in the non-choice lab test were compared between treatments using Kruskal-Wallis tests with significance at P < 0.05. Where significance was detected, this was followed by Mann-Whitney U tests with Bonferroni correction applied for multiple comparisons. For the choice experiment, slugs that did not move into either arm were excluded from the analysis, resulting in 69 replications. A Wilcoxon signed rank test was used to compare time spent in each arm of the Y tube, with a hypothesised proportion of 50%. The proportion weight loss of the cadavers and slug behaviours for this experiment were analysed using a two-sample t-test and Mann-Whitney U tests, respectively. All data analysis was performed using Minitab v. 20.3 statistical software or R studio v. 2022.12.0 + 353.
3 Results
The average luminescence of H. downesi infected G. mellonella increased from day 2 of infection, peaked at day 3 at 28924.3 ± 1132.482 (mean ± SE, N = 19) mean grey scale units/pixel and decreased from then, ceasing completely by day 19 (Figure 2).
3.1 Does bioluminescence deter nocturnal scavenging (field study)?
Slugs were the most frequently recorded potential scavengers, accounting for 70% (68/97 individuals) of all invertebrates found in the dishes, with L. valentiana being the most common slug species, accounting for 23% (22/97) of all invertebrates found. Other potential invertebrate scavengers recorded in the dishes included earwigs (Dermaptera) and beetles (Coleoptera) (Supplementary Table 4).
There were significant differences between treatments in scavenging rate (the proportion of cadavers showing signs of biting or feeding) by nocturnal scavengers (Chi square: X23 = 10.39, P = 0.0155, N = 103-108) (Figure 3A; Supplementary Table 2) and in the weight loss of cadavers, used as a proxy for amount consumed (Kruskal-Wallis: H3 = 18.09, P < 0.001, N = 103-108) (Figure 3B; Supplementary Table 3). Scavenging rate and consumption were lower for P. temperata-infected than for freeze-killed cadavers, but the differences were significant only for those under dark covers, where luminescence would be apparent, but not for cadavers under translucent covers, indicating that bioluminescence is effective in reducing scavenging (Figures 3A, B). The majority of those cadavers that had evidence of scavenging had only small puncture wounds in both the infected treatments (Dark: 10/15; 66.7%, Light: 23/28; 82.1%) and the controls (Dark: 25/37; 67.6%, Light: 17/31; 54.8%). Of the cadavers that were attacked, the proportion that were fully consumed was approximately 3.6 times lower in the infected treatments than in the controls both in the dark (1/15; 6.7% vs 9/37; 24.3%) and in the light (3/28; 10.7% vs 12/31; 38.7%).
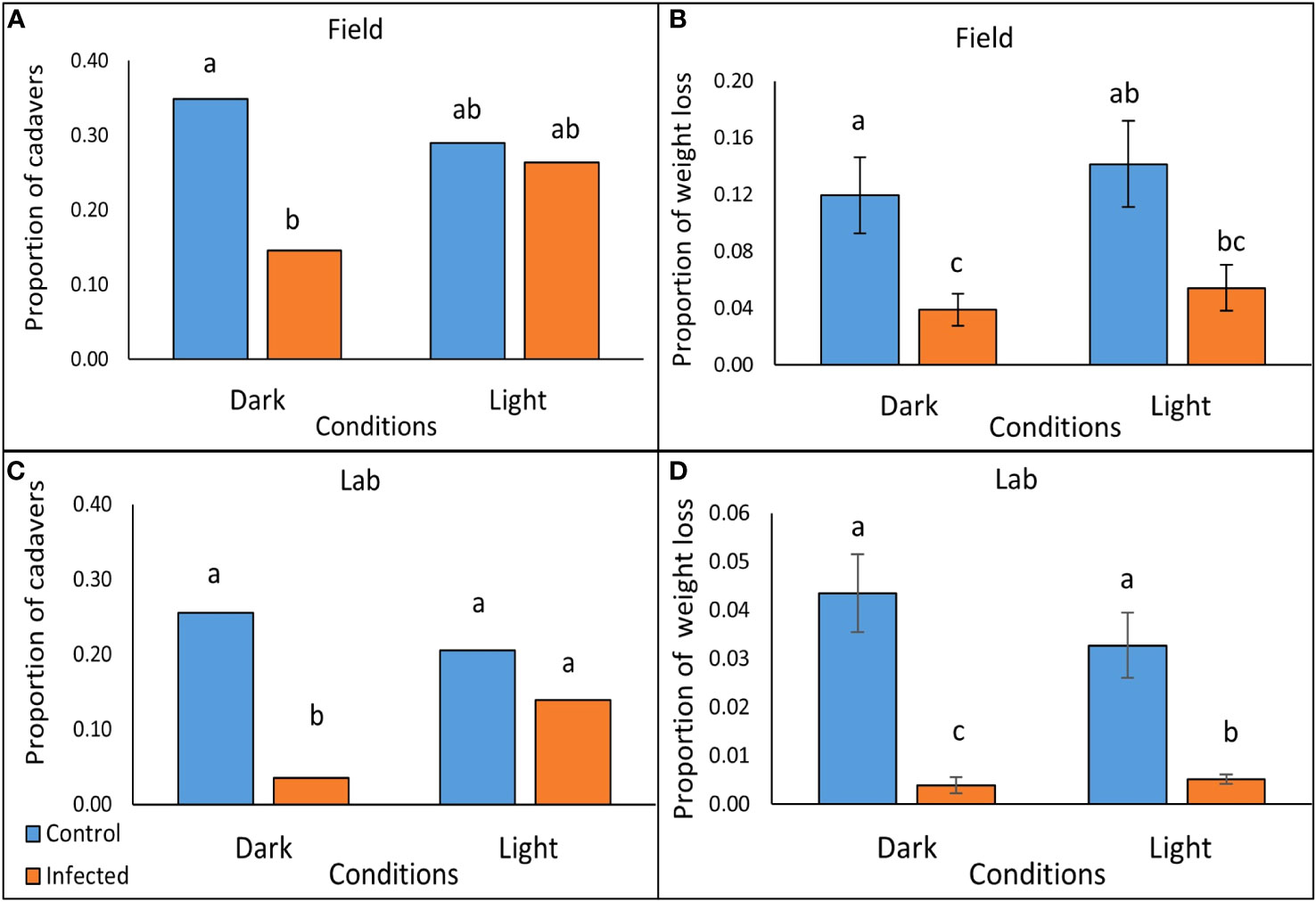
Figure 3 Scavenging on P. temperata- infected and freeze-killed (control) G. mellonella cadavers under conditions of either light or dark, after 2 hrs in the field (A, B), and after 5 hrs exposure to slugs (L. valentiana) in the laboratory (C, D). (A, C) The proportion of cadavers tested that showed incidence of scavenging in the field and laboratory trials respectively (columns accompanied by the same letters are not significantly different at P < 0.05, Chi square with Bonferroni correction). (B, D) Weight loss as a proportion of the cadaver (mean +/- SE) of G. mellonella tested in the field and laboratory respectively (Columns accompanied by the same letters are not significantly different at P < 0.05, Mann Whitney with Bonferroni correction).
There was no difference between treatments in their association with invertebrates, either in incidence (the proportion of dishes containing at least one invertebrate along with the cadaver) (Pearsons’s chi square: X23 = 1.382, P = 0.710, N = 103-108) or in numbers of individuals recorded (Kruskal Wallis: H3 = 0.85, P = 0.838, N = 103-108).
3.2 Does bioluminescence deter slugs (L. valentiana) from feeding (lab study)?
In laboratory tests with L. valentiana there were significant differences between treatments in the proportion of cadavers showing signs of scavenging (Pearsons’s chi square: X23 = 33.968, P < 0.0001, N = 141-144) (Figure 3C; Supplementary Table 5), in weight loss of cadavers- a proxy for amount consumed (Kruskal-Wallis: H3 = 65.71, P < 0.001, N = 141-144)(Figure 3D; Supplementary Table 6), and in the amount of time the L. valentiana slugs spent feeding on the cadavers during the 2 hr observation (Kruskal-Wallis: H3 = 10.26, P = 0.016, N = 141-144) (Figure 4; Supplementary Table 7). There was less scavenging (attack and consumption) by L. valentiana on P. temperata-infected than on control cadavers. The difference between infected and control cadavers in the incidence of scavenging (Figure 3C) and in time spent feeding (Figure 4) was significant only in the dark when luminescence would be visible, and not in the light, when luminescence would not be apparent, indicating a defensive role for bioluminescence. In the light conditions, the amount of cadaver consumed was lower for infected than for freeze-killed, indicating the operation of chemical defences. The amount of infected cadaver consumed in the dark was lower than in ambient light, clearly showing the additive effect of both defence modalities (Figure 3D). Time spent on behaviours other than feeding (moving close to the cadaver, moving at a distance from the cadaver, searching, tasting) did not differ between treatments (Supplementary Table 8).
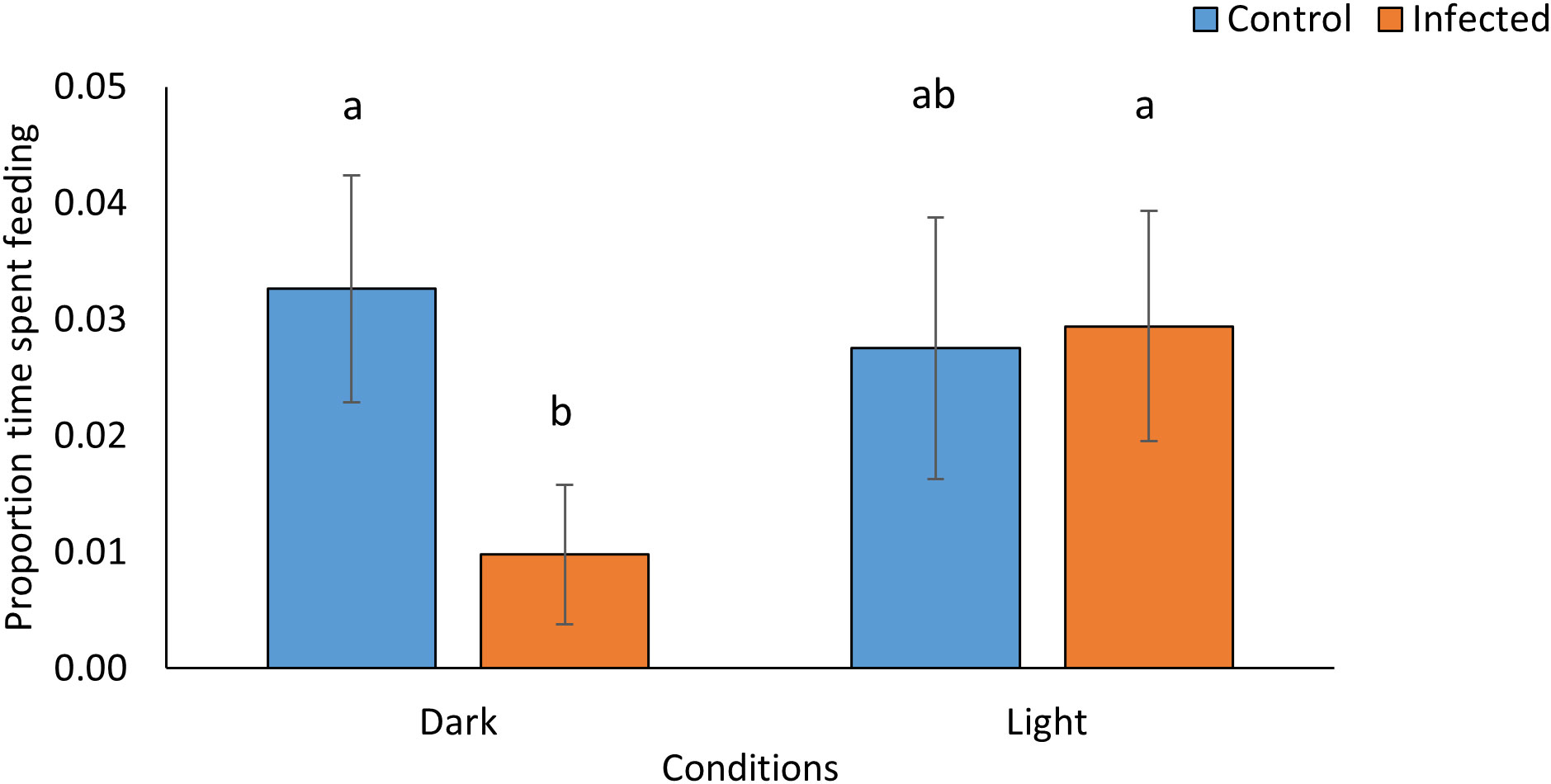
Figure 4 Proportion of time L. valentiana slugs spent feeding on P. temperata- infected or freeze killed (control) G. mellonella cadavers under conditions of either light or dark in no-choice laboratory experiments. Values accompanied by the same letter are not significantly different (Mann Whitney tests with Bonferroni correction, P < 0.05).
3.3 Does cadaver-mimicking light attract, repel, or affect feeding of slugs?
There was no evidence that the slug L. valentiana was either repelled or attracted by artificial light of similar wavelength and intensity to a nematode-infected insect. When given a choice of two arms each with a freeze-killed G. mellonella as bait either paired or not paired with a light source, they spent an equal proportion of time in each arm (Wilcoxon signed rank test: Z = 1152.50, P = 0.980; N = 69), and there was no difference in the amount of time spent moving, searching, tasting, or feeding in the two arms (Supplementary Table 7). However, there was a lower incidence of scavenging on the bait insect paired with a light than on the bait insect without a light (Pearson’s Chi square: X21 = 3.881, P = 0.049; N = 69) (Figure 5). There was no difference in the percentage weight loss of cadavers in the two conditions (Mann Whitney W= 4989, P = 0.411; N = 69).
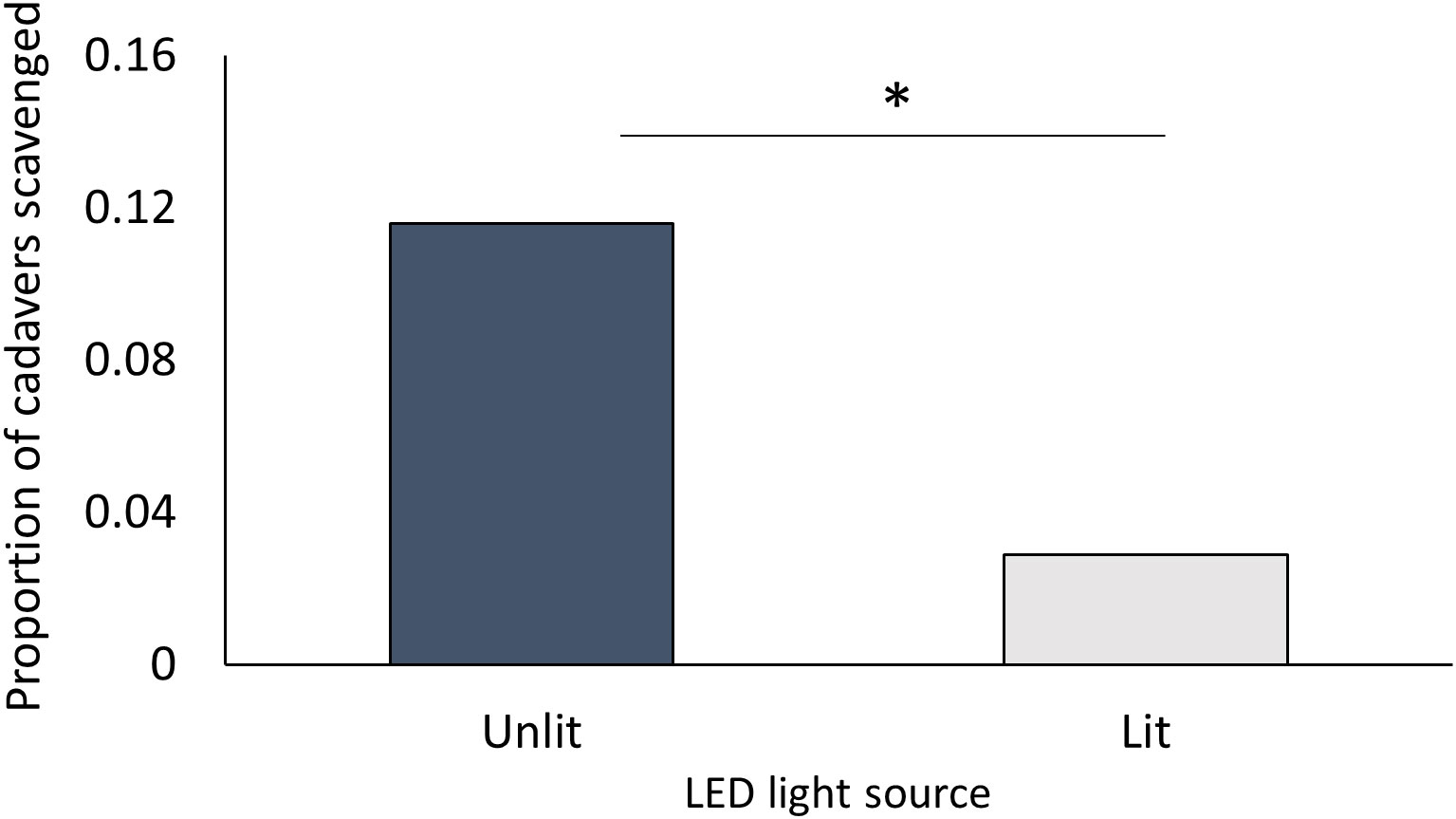
Figure 5 Proportion of freeze-killed G. mellonella cadavers scavenged by L. valentiana when close to either a lit or an unlit LED in a choice test. Slugs that did not move into either arm of the Y-tube were excluded from analysis. Significant differences between treatments in scavenging rate is indicated by * (Chi square, P < 0.05).
4 Discussion
Our findings support the scavenger-deterrence hypothesis (Akhurst and Boemare, 1990) for bioluminescence in Photorhabdus. In both our field and laboratory trials, a lower rate of scavenging on Photorhabdus-infected cadavers compared to controls was significant only under dark conditions, where bioluminescence would be visible, providing strong evidence that bioluminescence contributes to scavenger deterrence, while the experiment with an artificial light source indicates that bioluminescence alone could protect cadavers. Cadavers were not completely undefended in ambient light conditions, since the amount of cadaver consumed was reduced in the light as well as the dark, indicating the operation of a chemical “scavenger deterrent factor” (Zhou et al., 2002; Gulcu et al., 2012). We suggest that the two defences operate slightly differently, with chemical factors reducing the amount of cadaver consumed while light serves to reduce the probability of a cadaver being damaged in the first place. Preventing breaches of the cadaver cuticle is important; even a small lesion without further consumption may completely compromise the success of the nematode-bacterial complex developing inside, by increasing vulnerability to desiccation or competing organisms (Koppenhöfer et al., 1997; Baur et al., 1998; Serwe-Rodriguez et al., 2004).
The scavenger-deterrent hypothesis contrasts with the earlier suggestion by Poinar et al. (1980) that light could attract insects which could then be infected by, or serve to transport, the next generation of nematodes as they emerge from the cadaver into the soil. While there is some evidence that insects are attracted by the light emitted by P. luminescens (Patterson et al., 2015), attraction of new hosts is implausible as an ecological function of bioluminescence [as indeed was recognised by Poinar et al. (1980)]. As we show, it peaks within days of infection, long before the new infective stage nematodes have been produced and are ready to emerge from the host. In H. downesi, for example, the first infective juveniles do not emerge until at least 15 days after infection, by which time luminescence has declined to a low level. In contrast, peak luminescence occurs at a time when its role in defence against scavengers would be important: the nematodes developing inside are at a vulnerable stage (Poinar, 1979) and cadavers are otherwise poorly defended (Baur et al., 1998; Fenton et al., 2011; Gulcu et al., 2012; Ulug et al., 2014; Jones et al., 2015, Jones et al., 2017). Bioluminescence is produced once Photorhabdus reaches a critical population density (Meighen, 1999), while defences based on unpalatable chemicals and/or pigmentation intensify over time (Baur et al., 1998; Gulcu et al., 2012; Ulug et al., 2014; Jones et al., 2015; Jones et al., 2017), presumably as the products responsible for them accumulate within the cadaver. Although not tested here, it may be that bioluminescence as an independent defence is particularly important early in the infection, helping during a period of vulnerability while chemical defence is less efficient.
Variation amongst scavenger species in the extent to which they are deterred from Photorhabdus-infected cadavers (Gulcu et al., 2012; Ulug et al., 2014; Gulcu et al., 2018) may explain why trends in the field, with a diverse assemblage of scavengers, were less clearcut than in the laboratory trials with just a single species of photophobic nocturnal scavenger - the slug L. valentiana. Slugs move away from areas of light and are particularly sensitive to blue light in the 400 - 520 nm range (Suzuki et al., 1979; Nishiyama et al., 2019) which spans the range in which Photorhabdus emits luminescence (Peat et al., 2010). Their negative response to diffuse light is mainly associated with habitat selection (South, 1992; Zieger et al., 2009). In our experiments, there was no evidence that a point source of light deterred the approach of L. valentiana – there was no difference in the category “moving close” to a cadaver in the no-choice experiment, or in the frequency of entry into lit or unlit arms containing a cadaver-mimicking LED in the choice experiment. Slugs seek and recognise food using olfaction (Gelperin, 1974; Gelperin, 1975; Kiss, 2017); again, the lack of effect on “moving close” may indicate that slugs were not deterred at a distance by the odour of infected cadavers. There is evidence that the odour of P. luminescens-infected cadavers deters attack by beetle and avian scavengers (Jones et al., 2015; Jones et al., 2017), but it would appear that for L. valentiana, both chemical and light defences operate at close contact, serving to deter feeding, but neither modality deters their approach.
The unpalatability of Photorhabdus-infected cadavers to scavengers appears to be an innate (unlearned) response, as it is displayed in laboratory tests by untrained animals (Baur et al., 1998; Zhou et al., 2002; Gulcu et al., 2012; Lordan et al., 2014; Ulug et al., 2014; Raja et al., 2017; Gulcu et al., 2018; Cimen, 2023). Similarly, the aversive response of slugs to the luminescence of Photorhabdus shown here seems also to be innate, as it was demonstrated by naïve (untrained) individuals at first exposure. Bioluminescence is produced by Photorhabdus as a constant glow. In general, while sudden flashes of bioluminescence are repellent, bioluminescent glows are thought to function as attractant signals (Haddock et al., 2010). It is argued that without additional chemical defences, light would only serve to make the emitting organism more obvious to predators (De Cock and Matthysen, 1999). Slugs may be unusual in having an innate dislike of feeding on glowing food, though few terrestrial animals have been tested for this response. For toads and small mammals, the possibility of an innate deterrent response to the bioluminescence of glow worms or millipedes could not be excluded (De Cock and Matthysen, 1999; De Cock and Matthysen, 2003; Marek et al., 2011).
While not demonstrated here, the bioluminescence produced by Photorhabdus-infected cadavers could act more generally as an aposematic signal for nocturnal scavengers, as has been suggested for the red colour of P. luminescens-infected cadavers for diurnal scavengers (Fenton et al., 2011). Aposematism is the use of warning signals to advertise unprofitability (Breed and Moore, 2016) epitomised by the conspicuous colours used by insects and frogs to advertise toxicity or unpalatability (Guilford, 1990; Ruxton et al., 2018). Like conspicuous colours, bioluminescence is frequently associated with unpalatability, leading to the suggestion that it functions as an aposematic signal in a range of organisms, including algae (Dinoflagellata), annelids, brittle stars (Ophiuroidea), millipedes, glow worm larvae (Coleoptera) and fish (lanternsharks: Etmopteridae) (Grober, 1988a; Grober, 1988b; De Cock and Matthysen, 1999; Marek et al., 2011; Verdes and Gruber, 2017; Duchatelet et al., 2019; Cusick and Widder, 2020). This suggestion is not always supported by empirical data, but there is good experimental evidence both for brittle stars (Grober, 1988a; Grober, 1988b; Jones and Mallefet, 2013) and for glow worms (De Cock and Matthysen, 1999; De Cock and Matthysen, 2003). Toads (Bufo bufo) were deterred by the bioluminescence of the common glow worm, after learning to associate the glow with the noxious smell/taste of the larvae (De Cock and Matthysen, 1999; De Cock and Matthysen, 2003). Naïve wild-caught toads demonstrated some aversion to the bioluminescence, which was interpreted as evidence either of prior learning in the field or of neophobia (rejection of novel food), but the aversion was strengthened by the associative learning (De Cock and Matthysen, 2003). Similarly, when paired with chemical defences, the bioluminescence of Photorhabdus may strengthen the innate aversion of slugs to feeding on luminous food, and in addition deter other nocturnal scavengers from feeding, even those that are initially indifferent to the light or even attracted by it. Associative learning - required for aposematism to work- is well documented in insects (Dethier, 1980; Duerr and Quinn, 1982; Matsumoto and Mizunami, 2000; Giurfa, 2007; Giurfa, 2015) and in gastropods (Delaney and Gelperin, 1986; Balaban, 1993; Farley et al., 2004) including L. valentiana which can form a long-term association between chemical and visual stimuli (Fujisaki and Matsuo, 2017).
In conclusion, our experiments provide support for an ecological function for the bioluminescence of Photorhabdus in line with the scavenger deterrence hypothesis (Akhurst and Boemare, 1990), and show that light acts in concert with chemical defences, as previously shown for colour and chemical defences in daylight (Fenton et al., 2011; Jones et al., 2017). Deterrence is unlikely to have been the function of bioluminescence in the bacteria from which Photorhabdus acquired its lux operon, as there is evidence that in the marine environment, a constant glow is attractive (Zavilgelsky and Shakulov, 2018). A plausible scenario is that the gene transfer occurred within a crustacean; bioluminescent marine bacterial species occur as pathogens of many crustacea (Nealson and Hastings, 1979; Ramesh and Bessho-Uehara, 2021), and Photorhabdus can infect littoral amphipods and isopods (Mauléon et al., 2006). Bioluminescence may have been maintained by in the terrestrial environment by protecting infected hosts against innately photophobic scavengers such as slugs, and/or by aposematically reinforcing pre-existing chemical defences of Photorhabdus. Support for the scavenger deterrence in P. temperata does not rule out the possibility that bioluminescence is maintained in Photorhabdus by more than one selection pressure, either in the same or different species, or is being lost in some species in which it offers less advantage (Peat et al., 2010).
Data availability statement
The raw data supporting the conclusions of this article will be made available by the authors, without undue reservation.
Ethics statement
The species used in this study did not require formal ethical approval. The methodology used for these experiments was in keeping with recommendations for the ethical treatment of invertebrates (Drinkwater et al., 2019). Slugs collected for use in lab trials were monitored for 3 weeks after experimentation and healthy slugs were released at their collection point. Ecological impact during field trials was minimised by recording invertebrate activity in situ without removal.
Author contributions
MC: Data curation, Formal analysis, Investigation, Methodology, Validation, Visualization, Writing – original draft, Writing – review & editing. SL: Data curation, Investigation, Methodology, Writing – review & editing. CG: Conceptualization, Supervision, Writing – original draft, Writing – review & editing.
Funding
The authors declare financial support was received for the research, authorship, and/or publication of this article. This work was funded by the Maynooth University Doctoral Incentivisation scheme and the European Union Seventh Framework Programme: Grant agreement no 612713—BIOCOMES Project.
Acknowledgments
We would like to acknowledge Carton House and Maynooth University for allowing us access to their grounds for the purpose of this study, with special thanks to John Plummer and Ivan Griffin for facilitating this. We thank Robyn Travers, Niamh Treanor, Peter Lillis, Noel Gavin, Nick Irani and Tracey Cassells for their assistance at various stages throughout this project. This research was funded by the Maynooth University Doctoral Incentivisation scheme.
Conflict of interest
The authors declare that the research was conducted in the absence of any commercial or financial relationships that could be construed as a potential conflict of interest.
Publisher’s note
All claims expressed in this article are solely those of the authors and do not necessarily represent those of their affiliated organizations, or those of the publisher, the editors and the reviewers. Any product that may be evaluated in this article, or claim that may be made by its manufacturer, is not guaranteed or endorsed by the publisher.
Supplementary material
The Supplementary Material for this article can be found online at: https://www.frontiersin.org/articles/10.3389/fevo.2023.1264251/full#supplementary-material
References
Adams B., Nguyen K. (2002). “Taxonomy and systematics,” in Entomopathogenic nematology. Ed. Gaugler R. (New York, NY: CABI publishing), 2–3.
Akhurst R. J., Boemare N. E. (1990). “Biology and taxonomy of xenorhabdus,” in Entomopathogenic nematodes in biological control. Eds. Gaugler R., Kaya H. K. (Boca Raton, FL: CRC Press), 75–90.
Baker A., Robbins I., Moline M. A., Iglesias-Rodriguez M. D. (2008). Oligonucleotide primers for the detection of bioluminescent dinoflagellates reveal novel luciferase sequences and information on the molecular evolution of this gene. J. Phycol. 44, 419–428. doi: 10.1111/j.1529-8817.2008.00474.x
Balaban P. (1993). Behavioral neurobiology of learning in terrestrial snails. Prog. Neurobiol. 41, 1–19. doi: 10.1016/0301-0082(93)90038-T
Barnes H. F., Weil J. W. (1945). Slugs in gardens: Their numbers, activities, and distribution (II). J. Anim. Ecol. 14, 71–105. doi: 10.2307/1386
Baur M. E., Kaya H. K., Strong D. R. (1998). Foraging ants as scavengers on entomopathogenic nematode-killed insects. Biol. Control. 12, 231–236. doi: 10.1006/bcon.1998.0635
Bode H. B. (2009). Entomopathogenic bacteria as a source of secondary metabolites. Curr. Opin. Chem. Biol. 13, 224–230. doi: 10.1016/j.cbpa.2009.02.037
Boemare N. (2002). Interactions between the partners of the entomopathogenic bacterium nematode complexes, Steinernema-Xenorhabdus and Heterorhabditis-Photorhabdus. Nematology 4, 601–603. doi: 10.1163/15685410260438863
Branham M. A., Wenzel J. W. (2001). The evolution of bioluminescence in cantharoids (Coleoptera: Elateroidea). Florida Entomol. 84, 565–586. doi: 10.2307/3496389
Buck J., Case J. F. (2002). Physiological links in firefly flash code evolution. J. Insect Behav. 15, 51–68. doi: 10.1023/A:1014480028731
Buskey E. J., Swift E. (1985). Behavioral responses of oceanic zooplankton to simulated bioluminescence. Biol. Bull. 168, 263–275. doi: 10.2307/1541239
Cimen H. (2023). The role of Photorhabdus-induced bioluminescence and red cadaver coloration on the deterrence of insect scavengers from entomopathogenic nematode-infected cadavers. J. Invertebrate. Pathol. 196, 107871. doi: 10.1016/j.jip.2022.107871
Clarke D. J. (2008). Photorhabdus: a model for the analysis of pathogenicity and mutualism. Cell. Microbiol. 10, 2159–2167. doi: 10.1111/j.1462-5822.2008.01209.x
Cusick K. D., Widder E. A. (2020). Bioluminescence and toxicity as driving factors in harmful algal blooms: Ecological functions and genetic variability. Harmful. Algae 98, 101850. doi: 10.1016/j.hal.2020.101850
Czyz A., Wróbel B., Wegrzyn G. (2000). Vibrio harveyi bioluminescence plays a role in stimulation of DNA repair. Microbiology 146, 283–288. doi: 10.1099/00221287-146-2-283
De Cock R., Matthysen E. (1999). Aposematism and bioluminescence: experimental evidence from glow-worm larvae (Coleoptera: Lampyridae). Evol. Ecol. 13, 619–639. doi: 10.1023/A:1011090017949
De Cock R., Matthysen E. (2003). Glow-worm larvae bioluminescence (Coleoptera: Lampyridae) operates as an aposematic signal upon toads (Bufo bufo). Behav. Ecol. 14, 103–108. doi: 10.1093/beheco/14.1.103
Delaney K., Gelperin A. (1986). Post-ingestive food-aversion learning to amino acid deficient diets by the terrestrial slug Limax maximus. J. Comp. Physiol. 159, 281–295. doi: 10.1007/BF00603975
Dethier V. G. (1980). Food-aversion learning in two polyphagous caterpillars, Diacrisia virginica and Estigmene congrua. Physiol. Entomol. 5, 321–325. doi: 10.1111/j.1365-3032.1980.tb00242.x
Dillman A. R., Sternberg P. W. (2012). Entomopathogenic nematodes. Curr. Biol. 22, R430–R431. doi: 10.1016/j.cub.2012.03.047
Drinkwater E., Robinson E. J., Hart A. G. (2019). Keeping invertebrate research ethical in a landscape of shifting public opinion. Methods Ecol. Evol. 10, 1265–1273. doi: 10.1111/2041-210X.13208
Duchatelet L., Pinte N., Tomita T., Sato K., Mallefet J. (2019). Etmopteridae bioluminescence: dorsal pattern specificity and aposematic use. Zool. Lett. 5, 9. doi: 10.1186/s40851-019-0126-2
Duerr J. S., Quinn W. G. (1982). Three Drosophila mutations that block associative learning also affect habituation and sensitization. Proc. Natl. Acad. Sci. 79, 3646–3650. doi: 10.1073/pnas.79.11.3646
Dunlap P. V., Kita-Tsukamoto K. (2006). “Luminous bacteria,” in The prokaryotes: volume 2: ecophysiology and biochemistry. Eds. Dworkin M., Falkow S., Rosenberg E., Schleifer K.-H., Stackebrandt E. (New York, NY: Springer New York), 863–892.
Eleftherianos I., Boundy S., Joyce S. A., Aslam S., Marshall J. W., Cox R. J., et al. (2007). An antibiotic produced by an insect-pathogenic bacterium suppresses host defenses through phenoloxidase inhibition. Proc. Natl. Acad. Sci. 104, 2419–2424. doi: 10.1073/pnas.0610525104
Farley J., Jin I., Huang H., Kim J. I. (2004). Chemosensory conditioning in molluscs: II. A critical review. Learn. Behav. 32, 277–288. doi: 10.3758/BF03196027
Fenton A., Magoolagan L., Kennedy Z., Spencer K. A. (2011). Parasite-induced warning coloration: A novel form of host manipulation. Anim. Behav. 81, 417–422. doi: 10.1016/j.anbehav.2010.11.010
Forey P. L., Patterson C. (2006). Description and systematic relationships of Tomognathus, an enigmatic fish from the English Chalk. J. Syst. Palaeontol. 4, 157–184. doi: 10.1017/S1477201905001719
Forst S., Nealson K. (1996). Molecular biology of the symbiotic-pathogenic bacteria Xenorhabdus spp. and Photorhabdus spp. Microbiol. Rev. 60, 21–43. doi: 10.1128/mr.60.1.21-43.1996
Fujisaki Y., Matsuo R. (2017). Context-dependent passive avoidance learning in the terrestrial slug Limax. Zool. Sci. 34, 532–537. doi: 10.2108/zs170071
Gelperin A. (1974). Olfactory basis of homing behavior in the giant garden slug, Limax maximus. Proc. Natl. Acad. Sci. 71, 966–970. doi: 10.1073/pnas.71.3.966
Gelperin A. (1975). Rapid food-aversion learning by a terrestrial mollusk. Science 189, 567–570. doi: 10.1126/science.1145215
Giurfa M. (2007). Behavioral and neural analysis of associative learning in the honeybee: a taste from the magic well. J. Comp. Physiol. 193, 801–824. doi: 10.1007/s00359-007-0235-9
Giurfa M. (2015). Learning and cognition in insects. WIREs. Cogn. Sci. 6, 383–395. doi: 10.1002/wcs.1348
Grober M. S. (1988a). Brittle-star bioluminescence functions as an aposematic signal to deter crustacean predators. Anim. Behav. 36, 493–501. doi: 10.1016/S0003-3472(88)80020-4
Grober M. S. (1988b). Responses of tropical reef fauna to brittle-star luminescence (Echinodermata: Ophiuroidea). J. Exp. Mar. Biol. Ecol. 115, 157–168. doi: 10.1016/0022-0981(88)90100-1
Guilford T. (1990). The secrets of aposematism: Unlearned responses to specific colours and patterns. Trends Ecol. Evol. 5, 323–348. doi: 10.1016/0169-5347(90)90177-F
Gulcu B., Hazir S., Kaya H. (2012). Scavenger deterrent factor (SDF) from symbiotic bacteria of entomopathogenic nematodes. J. Invertebrate. Pathol. 110, 326–333. doi: 10.1016/j.jip.2012.03.014
Gulcu B., Hazir S., Lewis E. E., Kaya K. H. (2018). Evaluation of responses of different ant species (Formicidae) to the scavenger deterrent factor associated with the entomopathogenic nematode-bacterium complex. Eur. J. Entomol. 115, 312–317. doi: 10.14411/eje.2018.030
Haddock S. H. D., Moline M. A., Case J. F. (2010). Bioluminescence in the sea. Annu. Rev. Mar. Sci. 2, 443–493. doi: 10.1146/annurev-marine-120308-081028
Herring P. J. (1989). “Bioluminescent communication in the sea,” in In light and life in the sea, vol. 16 . Eds. Herring P. J., Campbell A. K., Whitfield M., Maddock L. (Cambridge: Cambridge University Press), 245–265.
Herring P. J., Widder E. A. (2004). Bioluminescence of deep-sea coronate medusae (Cnidaria: Scyphozoa). Mar. Biol. 146, 39–51. doi: 10.1007/s00227-004-1430-7
Hyrsl P., Ciz M., Lojek A. (2004). Comparison of the bioluminescence of Photorhabdus species and subspecies type strains. Folia Microbiol. (Praha). 49, 539–542. doi: 10.1007/BF02931530
Jennings T. J., Barkham J. P. (1975). Food of slugs in mixed deciduous woodland. Oikos 26, 211–221. doi: 10.2307/3543711
Jones A., Mallefet J. (2013). Why do brittle stars emit light? Behavioural and evolutionary approaches of bioluminescence. Cahiers. Biol. Mar. 54, 729–734.
Jones R. S., Fenton A., Speed M. P. (2015). “Parasite-induced aposematism” protects entomopathogenic nematode parasites against invertebrate enemies. Behav. Ecol. 27, 645–651. doi: 10.1093/beheco/arv202
Jones R. S., Fenton A., Speed M. P., Mappes J. (2017). Investment in multiple defences protects a nematode-bacterium symbiosis from predation. Anim. Behav. 129, 1–8. doi: 10.1016/j.anbehav.2017.03.016
Kasai S., Okada K., Hoshino A., Iida T., Honda T. (2006). Lateral transfer of the lux gene cluster. J. Biochem. 141, 231–237. doi: 10.1093/jb/mvm023
Keller H. W., Snell K. L. (2002). Feeding activities of slugs on myxomycetes and macrofungi. Mycologia 94, 757–760. doi: 10.1080/15572536.2003.11833169
Kiss T. (2017). Do terrestrial gastropods use olfactory cues to locate and select food actively? Invertebrate. Neurosci. 17, 9. doi: 10.1007/s10158-017-0202-2
Koppenhöfer A. M., Baur M. E., Stock S. P., Choo H. Y., Chinnasri B., Kaya H. K. (1997). Survival of entomopathogenic nematodes within host cadavers in dry soil. Appl. Soil Ecol. 6, 231–240. doi: 10.1016/S0929-1393(97)00018-8
Labas Y. A., Matz M. V., Zakhartchenko V. A. (2001). “On the origin of bioluminescent systems,” in Bioluminescence and chemiluminescence. Eds. Case J. F., Herring P. J., Robison B. H., Haddock S. H. D., Kricka L. J., Stanley P. E. (Singapore: World Scientific), 91–94.
Latz M. I., Frank T. M., Case J. F. (1988). Spectral composition of bioluminescence of epipelagic organisms from the Sargasso Sea. Mar. Biol. 98, 441–446. doi: 10.1007/BF00391120
Lau E. S., Oakley T. H. (2021). Multi-level convergence of complex traits and the evolution of bioluminescence. Biol. Rev. 96, 673–691. doi: 10.1111/brv.12672
Lewis S. M., Cratsley C. K. (2008). Flash signal evolution, mate choice, and predation in fireflies. Annu. Rev. Entomol. 53, 293–321. doi: 10.1146/annurev.ento.53.103106.093346
Li J., Chen G., Wu H., Webster J. M. (1995). Identification of two pigments and a hydroxystilbene antibiotic from Photorhabdus luminescens. Appl. Environ. Microbiol. 61, 4329–4333. doi: 10.1128/aem.61.12.4329-4333.1995
Lordan J., Alegre S., Alins G., Sarasúa M. J., Morton A., García del Pino F. (2014). Compatibility between Forficula auricularia and entomopathogenic nematodes to be used in pome fruit pest management. J. Appl. Entomol. 138, 635–643. doi: 10.1111/jen.12118
Maher A. M. D., Asaiyah M., Quinn S., Burke R., Wolff H., Bode H. B., et al. (2021). Competition and co-existence of two Photorhabdus symbionts with a nematode host. Microbial. Ecol. 81, 223–239. doi: 10.1007/s00248-020-01573-y
Marek P., Papaj D., Yeager J., Molina S., Moore W. (2011). Bioluminescent aposematism in millipedes. Curr. Biol. 21, R680–R681. doi: 10.1016/j.cub.2011.08.012
Matsumoto Y., Mizunami M. (2000). Olfactory learning in the cricket Gryllus bimaculatus. J. Exp. Biol. 203, 2581–2588. doi: 10.1242/jeb.203.17.2581
Mauléon H., Denon D., Briand S. (2006). Spatial and temporal distribution of Heterorhabditis indica in their natural habitats of Guadeloupe. Nematology 8, 603–617. doi: 10.1163/156854106778614083
McElroy W. D., Seliger H. H. (1962). “Origins and evolution of bioluminescence,” in Horizons in biochemistry. Eds. Kasha M., Pullman B. (New York: Academic Press), 91–102.
Meighen E. A. (1999). Autoinduction of light emission in different species of bioluminescent bacteria. Luminescence 14, 3–9. doi: 10.1002/(SICI)1522-7243(199901/02)14:1<3::AID-BIO507>3.0.CO;2-4
Meighen E. A., Szittner R. B. (1992). Multiple repetitive elements and organization of the lux operons of luminescent terrestrial bacteria. J. Bacteriol. 174, 5371–5381. doi: 10.1128/jb.174.16.5371-5381.1992
Nealson K. H., Hastings J. W. (1979). Bacterial bioluminescence: its control and ecological significance. Microbiol. Rev. 43, 496–518. doi: 10.1128/mr.43.4.496-518.1979
Nishiyama H., Nagata A., Matsou Y., Matsuo R. (2019). Light avoidance by a non-ocular photosensing system in the terrestrial slug Limax valentianus. J. Exp. Biol. 222, jeb208595. doi: 10.1242/jeb.208595
Patterson W., Upadhyay D., Mandjiny S., Bullard-Dillard R., Storms M., Menefee M., et al. (2015). Attractant role of bacterial bioluminescence of Photorhabdus luminescens on a Galleria mellonella model. Am. J. Life Sci. 3, 290–294. doi: 10.11648/j.ajls.20150304.16
Peat S., Adams B. (2008). Natural selection on the luxA gene of bioluminescent bacteria. Symbiosis 46, 101–108.
Peat S. M., French-Constant R. H., Waterfield N. R., Marokházi J., Fodor A., Adams B. J. (2010). A robust phylogenetic framework for the bacterial genus Photorhabdus and its use in studying the evolution and maintenance of bioluminescence: A case for 16S, gyrB, and glnA. Mol. Phylogenet. Evol. 57, 728–740. doi: 10.1016/j.ympev.2010.08.012
Poinar G. O., Thomas G., Haygood M., Nealson K. H. (1980). Growth and luminescence of the symbolic bacteria associated with the terrestrial nematode Heterorhabditis bacteriophora. Soil Biol. Biochem. 12, 5–10. doi: 10.1016/0038-0717(80)90095-4
Raja R. K., Aiswarya D., Gulcu B., Raja M., Perumal P., Sivaramakrishnan S., et al. (2017). Response of three cyprinid fish species to the Scavenger Deterrent Factor produced by the mutualistic bacteria associated with entomopathogenic nematodes. J. Invertebrate. Pathol. 143, 40–49. doi: 10.1016/j.jip.2016.11.013
Raja R. K., Arun A., Touray M., Gulsen S. H., Cimen H., Gulcu B., et al. (2021). Antagonists and defense mechanisms of entomopathogenic nematodes and their mutualistic bacteria. Biol. Control. 152, 104452. doi: 10.1016/j.biocontrol.2020.104452
Ramesh C., Bessho-Uehara M. (2021). Acquisition of bioluminescent trait by non-luminous organisms from luminous organisms through various origins. Photochem. Photobiol. Sci. 20, 1547–1562. doi: 10.1007/s43630-021-00124-9
Rees J. F., De Wergifosse B., Noiset O., Dubuisson M., Janssens B., Thompson E. M. (1998). The origins of marine bioluminescence: Turning oxygen defence mechanisms into deep-sea communication tools. J. Exp. Biol. 201, 1211–1221. doi: 10.1242/jeb.201.8.1211
Ruxton G. D., Allen W. L., Sherratt T. N., Speed M. P. (2018). Avoiding attack: The evolutionary ecology of crypsis, aposematism, and mimicry. 2nd ed (Oxford: Oxford University Press).
Seliger H. H. (1975). The origin of bioluminescence. Photochem. Photobiol. 21, 355–361. doi: 10.1111/j.1751-1097.1975.tb06684.x
Serwe-Rodriguez J., Sonnenberg K., Appleman B., Bornstein-Forst S. (2004). Effects of host desiccation on development, survival, and infectivity of entomopathogenic nematode Steinernema carpocapsae. J. Invertebrate. Pathol. 85, 175–181. doi: 10.1016/j.jip.2004.03.003
Suzuki H., Watanabe M., Tsukahara Y., Tasaki K. (1979). Duplex system in the simple retina of a gastropod mollusc, Limax flavus. J. Comp. Physiol. 133, 125–130. doi: 10.1007/BF00657527
Tanet L., Martini S., Casalot L., Tamburini C. (2020). Reviews and syntheses: Bacterial bioluminescence – ecology and impact in the biological carbon pump. Biogeosciences 17, 3757–3778. doi: 10.5194/bg-17-3757-2020
Timmins G. S., Jackson S. K., Swartz H. M. (2001). The evolution of bioluminescent oxygen consumption as an ancient oxygen detoxification mechanism. J. Mol. Evol. 52, 321–332. doi: 10.1007/s002390010162
Timsit Y., Lescot M., Valiadi M., Not F. (2021). Bioluminescence and photoreception in unicellular organisms: Light-signalling in a bio-communication perspective. Int. J. Mol. Sci. 22, 11311. doi: 10.3390/ijms222111311
Tobias N. J., Shi Y.-M., Bode H. B. (2018). Refining the natural product repertoire in entomopathogenic bacteria. Trends Microbiol. 26, 833–840. doi: 10.1016/j.tim.2018.04.007
Ullah I., Khan A. L., Ali L., Khan A. R., Waqas M., Hussain J., et al. (2015). Benzaldehyde as an insecticidal, antimicrobial, and antioxidant compound produced by Photorhabdus temperata M1021. J. Microbiol. 53, 127–133. doi: 10.1007/s12275-015-4632-4
Ulug D., Hazir S., Kaya H. K., Lewis E. (2014). Natural enemies of natural enemies: the potential top-down impact of predators on entomopathogenic nematode populations. Ecol. Entomol. 39, 462–469. doi: 10.1111/een.12121
Underwood T. J., Tallamy D. W., Pesek J. D. (1997). Bioluminescence in firefly larvae: a test of the aposematic display hypothesis (Coleoptera: Lampyridae). J. Insect Behav. 10, 365–370. doi: 10.1007/BF02765604
Verdes A., Gruber D. F. (2017). Glowing Worms: Biological, chemical, and functional diversity of bioluminescent annelids. Integr. Comp. Biol. 57, 18–32. doi: 10.1093/icb/icx017
Waterfield N. R., Ciche T., Clarke D. (2009). Photorhabdus and a host of hosts. Annu. Rev. Microbiol. 63, 557–574. doi: 10.1146/annurev.micro.091208.073507
Widder E. A. (1999). “Bioluminescence,” in Adaptive mechanisms in the ecology of vision. Eds. Archer S. N., Djamgoz M. B. A., Loew E. R., Partridge J. C., Vallerga S. (Boston: Kluwer Academic Publishers), 555–581.
Widder E. A. (2010). Bioluminescence in the ocean: origins of biological, chemical, and ecological diversity. Science 328, 704–708. doi: 10.1126/science.1174269
Zavilgelsky G. B., Shakulov R. S. (2018). Mechanisms and origin of bacterial bioluminescence. Mol. Biol. 52, 812–822. doi: 10.1134/S0026893318060183
Zhou X., Kaya H. K., Heungens K., Goodrich-Blair H. (2002). Response of ants to a deterrent factor(s) produced by the symbiotic bacteria of entomopathogenic nematodes. Appl. Environ. Microbiol. 68, 6202–6209. doi: 10.1128/AEM.68.12.6202-6209.2002
Zieger M. V., Vakoliuk I. A., TuChina O. P., Zhukov V. V., Meyer-Rochow V. B. (2009). Eyes and vision in Arion rufus and Deroceras agreste (Mollusca; Gastropoda; Pulmonata): What role does photoreception play in the orientation of these terrestrial slugs? Acta Zool. 90, 189–204. doi: 10.1111/j.1463-6395.2008.00369.x
Keywords: bioluminescent bacteria, aposematism, Heterorhabditis downesi, Lehmannia valentiana, invertebrates
Citation: Cassells MD, Labaude S and Griffin CT (2024) Beware glowing cadavers: bioluminescence of nematode symbiont Photorhabdus protects nematode-infected host cadavers from nocturnal scavengers. Front. Ecol. Evol. 11:1264251. doi: 10.3389/fevo.2023.1264251
Received: 20 July 2023; Accepted: 13 December 2023;
Published: 05 January 2024.
Edited by:
Stephen T. Trumbo, University of Connecticut, United StatesReviewed by:
Harun Cimen, Adnan Menderes University, TürkiyeRamesh Chatragadda, Council of Scientific and Industrial Research (CSIR), India
Copyright © 2024 Cassells, Labaude and Griffin. This is an open-access article distributed under the terms of the Creative Commons Attribution License (CC BY). The use, distribution or reproduction in other forums is permitted, provided the original author(s) and the copyright owner(s) are credited and that the original publication in this journal is cited, in accordance with accepted academic practice. No use, distribution or reproduction is permitted which does not comply with these terms.
*Correspondence: Maria D. Cassells, maria.cassells@mu.ie
†Present address: Sophie Labaude, Caribaea Initiative, Université des Antilles, Guadeloupe, France