- 1Department of Evolutionary Animal Ecology, University of Bayreuth, Bayreuth, Germany
- 2Department of Developmental Biology and Genetics, Indian Institute of Science, Bengaluru, India
Social behaviors and lifestyles have evolved as successful strategies to cope with adverse and challenging living conditions, often by manipulating the immediate environment. These manipulations can extend to the surrounding microbiome, both in terms of combating harmful agents such as pathogens but also by facilitating the growth of beneficial microbes. In contrast to the largely antagonistic role traditionally assigned to microbes in social systems, these host–microbe interactions are receiving increasing attention as potential facilitators of social evolution. Here, we explore this perspective using Nicrophorus burying beetles, a group of insect carrion breeders which offer insights into the evolutionary interactions between sociality and microbial mutualists in a relatively simple family model. Recent studies have demonstrated the constant microbial challenges faced by Nicrophorus nurseries and the costly consequences they entail. Here, we provide an overview of these challenges and then explore the role of microbial mutualists in this social endeavor, focusing on the advantages they confer in terms of development and immunity. Additionally, we discuss how these mutualistic associations may select for committed parental care and more obligate forms of social life by promoting prolonged social associations through vertical transmission. Our review highlights the hypothesis that microbial mutualists not only provide immediate benefits but may also encourage social interactions in their hosts. However, the occurrence, degree, and underlying mechanisms of this phenomenon remain largely theoretical, as do the evolutionary feedbacks on microbes. Empirical evidence in this area is currently limited, emphasizing the need for further research. Nicrophorus burying beetles represent an ideal system to investigate the interplay between microbial mutualists and social evolution, offering a promising avenue for future studies. Overall, this review underscores the importance of understanding the complex interactions between microbial mutualists and social behaviors in challenging environments, and beyond.
Introduction
Social life is a ubiquitous and successful strategy across many life forms, ranging from temporary facultative associations to obligatory and highly complex associations (Box 1). Among the many benefits contributing to the success of social lifestyles, several derive from reshaping or manipulating the immediate surroundings of participating individuals. These include sophisticated burrows or nests alleviating adverse conditions such as unfavorable climate (Kinlaw, 1999; Bautista et al., 2003; Körner et al., 2018), predation pressure (Ebensperger and Bozinovic, 2000) or the increased prevalence of pathogenic microbes inherent to high organismic densities (Cremer et al., 2007; Van Meyel et al., 2018). However, with increasing attention towards the importance of microbiomes in animal adaptation (McFall-Ngai et al., 2013; Colston and Jackson, 2016), several studies have proposed that social modifications of the environment including its microbial components may not be limited to external immunity and sanitary behaviors aimed at mitigating the increased risk of pathogen transmission but may also extend to nutritional or defensive benefits facilitated by the careful management, exchange, and spread of microorganisms (Archie and Tung, 2015; Biedermann and Rohlfs, 2017).
The extent and importance of host–microbe interactions in social systems may have long been underestimated (Lombardo, 2008) but could represent key facilitators of social evolution. Recent decades have seen increasing numbers of studies revealing various roles of microbiota across different levels of social complexity. In the gregarious German cockroach Blattella germanica, for instance, gut bacteria have been shown to greatly contribute to production of aggregation pheromones (Wada-Katsumata et al., 2015). A famous example from more derived social systems can be found in attine ants which employ elaborate farming of a fungal symbiont as a food source (Quinlan and Cherret, 1977) and whose nests serve as microenvironments carefully designed to suit the needs for both ant and fungus (Roces and Kleineidam, 2000). While this particular phenomenon has been well studied for nearly half a century, the existence and role of antibiotic-producing microbiota in maintaining this symbiosis (Currie, 2001) and the process of their selection (Barke et al., 2011) were elucidated much more recently. Microbial management aided by microbiota can also be found in the bumblebee Bombus terrestris where newly emerged workers gain resistance against a lethal trypanosomatid parasite by acquiring parts of their nestmates’ microbiota from their feces (Koch and Schmid-Hempel, 2011). Further, implications for microbial mutualists as possible drivers of social evolution have been found in wood-feeding termites using proctodeal (fecal–oral) trophallaxis to transmit hindgut symbionts to freshly eclosed individuals or to provide nitrogen supplementation to adults, enabling multiple generations to thrive and maintain complex nest structures on a nitrogen-poor diet (Brune and Ohkuma, 2011).
Box 1. On the topic and terminology of sociality.
Finding a concise definition on what comprises “social life”, or “sociality”, is no easy task. There is no single, ubiquitously useful framework describing the term “social”, likely because it carries different meanings in different fields of research. An extreme form of sociality is seen in some eusocial Hymenoptera, where insect societies show overlap of generations, cooperative brood care, and reproductive division of labor. However, not all "social" insects exhibit all these features. Rather, on the continuum of sociality, social interactions may be classified based on how group members share space and time for shorter durations sometimes as members of the same family or as mere nestmates. Other frameworks include “cooperative group living” and refer to cooperative breeding in addition to eusociality (Rubenstein and Abbot, 2017). To investigate the evolution of complex sociality, we must also consider simple, presumably non-derived systems defined by Lin and Michener (1972) as “communal”, “semisocial”, and “subsocial”. These are stages of group living which likely facilitate the selection for social traits and behaviors, as well as the transfer of mutualist microbes. “Subsociality”, meaning a group of a mother and her juvenile offspring (i.e., a family group), is often considered a critical stepping stone towards the consolidation of complex social systems (Kramer and Meunier, 2019). Here, we outline the importance of host–microbe interactions in such a “subsocial” framework, but also aim to highlight the role of these mechanisms in social evolution in general, and thus are interested in a broader, less restrictive understanding of sociality. As a result, we employ a broad definition of sociality here, after Costa (2018), who defines sociality as the “association of individuals in space and time for reasons other than mutual attraction to feeding or nesting sites, or arenas for courtship or mate competition”.
Challenging diets and environments may be of particular importance in revealing and understanding the mechanisms and importance of host–microbe interactions, for instance in taxa inhabiting volatile and dynamic microenvironments, such as ephemeral resources. These patchy resource sites (e.g., carrion, dung, and fruit) are subject to intense competition and represent prime targets for microorganisms, invertebrates, and vertebrates evolved to monopolize nutrients by reducing competition (Janzen, 1977). As a result, any exploitation of ephemeral resources must either be done rapidly or employ substantial physical and biochemical alterations to the microenvironment to preserve and provision the resource for a prolonged duration. While moderately challenging or optional for opportunists such as facultative scavengers, these requirements may pose significant challenges for species obligately relying on carrion such as carrion breeders, resulting in intense selection pressures (Fialho et al., 2018; Charabidze et al., 2021). These selective processes have facilitated a wide variety of adaptations and strategies to thrive even in hazardous circumstances, including exhibiting parental care, which are expected to shape interactions between macro- and microbial colonizers of patchy resources.
Integrating perspectives on microbial interactions in the context of evolutionary ecology and social evolution offer a prime opportunity to better understand not only the evolution of specialist exploiters such as carrion breeders, but also the possible role of microbial associates in facilitating social strategies of animals in hostile and adverse habitats. Here, we look into the association between microbes and carrion breeders, highlighting the need to manage carrion microbial communities as a possible facilitator for the evolution of social interactions and parental care. To this end, we review strategies employed by insect carrion breeders in general and burying beetles of the genus Nicrophorus in particular. Nicrophorus species are not only well-studied in the context of subsocial systems and social evolution but also receive increasing attention in terms of collective external (social) immunity (sensu Cotter and Kilner, 2010a; Otti et al., 2014; Van Meyel et al., 2018) and microbe interactions, representing an ideal candidate for synthesis.
Carrion as a breeding resource
Carrion, i.e., the flesh of a dead or decaying animal, represents a highly rewarding resource that resembles the high nutrient content of fresh prey without the energy expenditure, risk of injury, and probability of failure that are inevitably linked with predation (Yang et al., 2008; Wilson and Wolkovich, 2011). Exploited by a vast number of consumers, energy transfer between organisms via carrion consumption can exceed that of predation within an ecosystem (Wilson and Wolkovich, 2011), leading to intense competition between decomposers, arthropods, and scavengers to locate and monopolize carrion resources (DeVault et al., 2003). Unlike seeds or fruits, which as products of selective processes affect – and are affected by – the if and when of their consumption, carrion is not an active participant in the mediation of interactions between animals and microbes (Buchholz and Levey, 1990), precluding any possibility of coevolution between carrion and its consumers. In terms of food and breeding resources, carrion is more akin to dung, albeit less abundant and more ephemeral (Englmeier et al., 2023). As a result, carrion exploitation is associated with significant challenges mainly driven by the rapid colonization and subsequent decomposition by microorganisms. In this first section, we explore the mechanisms and effects of carrion decomposition in the context of utilizing carrion as a food source as well as critical resource for sustaining and rearing offspring.
Overall nutrient loss
While not immediately apparent, carrion decay commences quickly, driven by the bodies’ own enzymes (autolysis) and by colonization of both native and foreign microbes resulting in putrefaction and disintegration. Typically, initial stages of carrion decomposition are accompanied by rapid growth of the cadaver’s native microbial community (Pechal et al., 2013), subsequently leading to a depletion of oxygen concentrations that supports the growth of anaerobic microorganisms from the intestinal tract, e.g., Clostridium and Bacteriodes (Forbes and Carter, 2016). These changes lead to the breakdown of carbohydrates, lipids, and proteins which is expected to greatly diminish the nutritional value of the prized carrion for animal consumers (DeVault et al., 2003). However, microbial communities proliferating on the carrion can also increase the nutritional value of their substrate by increasing the value of the food through predigestion or serving as food themselves (Bärlocher, 1985), an aspect that can be exploited by opportunistic and specialist carrion feeders, as we discuss later.
Proliferation of pathogenic microbes
In addition to nutrient loss, microbial colonizers of carrion often include obligate or facultative pathogens. Microbes thriving on decaying tissue can turn not only the resource itself but also surrounding soil into a hazardous area (Fialho et al., 2018). This pathogen pressure represents a significant obstacle for animals looking to breed on this rare resource since a prolonged stay represents increased infection risk to the brood arising from the brood’s vulnerability to disease due to their often-close proximity, high relatedness (Shykoff and Schmid-Hempel, 1991; Altizer and Nunn, 2006), and potentially undeveloped immunity (Tallamy, 1984; Kaltenpoth and Engl, 2014). Furthermore, pathogen exposure during early life can alter offspring response to parental care (Körner et al., 2020) and shape offspring long-term immunity investment (Vogelweith et al., 2017) on top of the costs associated with overcoming the infection itself. Breeding on carrion can thus result in life-long fitness detriments for offspring, and caring parents attending the brood may succumb to infection themselves which could result in a total fitness loss. Pathogen pressure has long been considered a major selective force for social and family life overall (Hamilton, 1987; Cremer et al., 2007; Korb and Heinze, 2015; Meunier, 2015) and may represent a particularly tall obstacle for carrion breeders.
Release of toxic metabolites
Given the temporary and strongly localized nature of carrion, intense selection pressures have resulted in the evolution of strong competitive abilities for microbial colonizers. Even if competing microorganisms are not pathogenic to scavengers, decomposers, or breeding competitors, they are frequently able to ward off unwanted visitors by producing a range of potentially harmful secondary metabolites (Janzen, 1977). These toxic emissions are produced in a variety of ways, with the carcass’ own gut biomass likely contributing the most to decomposition and colonization by anaerobic microbes, which often leads to the accumulation of organic acids (propionic acid, lactic acid), toxic and volatile polyamines (putrescine and cadaverine), and gases (methane, hydrogen sulfide, ammonia) causing bloating (Gill-King, 1997; Forbes, 2008). Further ingestion of endo- and exo-toxins produced by carrion-colonizing bacteria (e.g., Clostridium, Staphylococcus) and fungi (e.g., Aspergillus, Fusarium) by scavenging animals can damage host tissue, affect the nervous or immune system, potentially causing death. Regardless of whether or not the release of toxic metabolites has been primarily selected for by improving competitive ability against animals or other microbial competitors (Sherratt et al., 2006), they represent effective means to make carrion unattractive, unpalatable, or outright hazardous for any exploiting parties.
Production of microbial volatile organic compounds
To take advantage of high-value carrion resources, interested animals must first detect their prized carcasses in a timely manner. While visual cues can be of use to vertebrate scavengers, e.g., birds (Buckley, 1996; DeVault et al., 2003), necrophilous insects are thought to heavily rely on olfactory detection of volatile organic compounds (LeBlanc and Logan, 2010). Some of these cues likely originate endogenously during tissue autolysis, but evidence suggests that a vast array of different microbial volatile organic compounds (MVOCs) may be the major driver of olfactory detection of carrion by decomposers and scavengers (Stotzky and Schenck, 1971; Lowery et al., 2008; Tomberlin et al., 2012; von Hoermann et al., 2022). These substances are mostly derived from the chemical breakdown of protein-rich tissues and, just like the microbe-driven decay from which they originate, progress through stages of different composition and intensity (Pechal et al., 2013; Pechal et al., 2014) and thus reveal carrion state and location over long distances (e.g., Gram and Huss, 1996; Tomberlin et al., 2012). The succession of arriving insects, typically led by dipteran blow flies (Calliphoridae) and flesh flies (Sarcophagidae), further modifies and speeds up decomposition (Anderson and Cervenka, 2002; Kreitlow, 2010; Pechal et al., 2013). Progressive stages of decay then attract progressively diverse competitors and threats, such as predatory rove beetles (Coleoptera: Staphylinidae) attracted by products of putrefaction (Forbes and Carter, 2016). To prospective breeders attempting to monopolize a carcass for their offspring, the emission of MVOCs could be considered overall undesirable – even though they serve as important cues for the breeders themselves. Furthermore, vertebrate scavengers are not necessarily attracted by microbial evidence of decay and may even be deterred by it (DeVault et al., 2003). Therefore, the emission of MVOCs is somewhat of a double-edged sword as carrion breeders initially rely on them for localizing carrion, but once colonized, they must regulate its microbial communities to suppress the emission of MVOCs to successfully monopolize the carrion.
Quick or thorough? Overcoming the hazards of decay
Insects that specialize in rearing offspring on or around carrion show several adaptations that improve their competitive ability to discover, retain and/or quickly consume carcasses while reducing associated costs (Hanski, 1987). These include relocation or burying of resources, fast colonization, ovoviviparity, fast larval growth rates, group feeding, and pupating away from the carcass in safer microhabitats. Although reliance on carrion selects for rapid completion of feeding/larval stages – a phenomenon unmistakably evident and prevalent within necrophagous insects – we find contrasting life history strategies through which they achieve it. On one hand, blow flies and bottle flies have evolved to grow in large groups that reach pupal stages in less than 4 days (Greenberg, 1991; Scanvion et al., 2018). Adult females typically deposit the eggs and leave them unattended with virtually no parental care. Smaller carrion may be detected, colonized and consumed before vertebrate scavengers are able to even detect it (Putman, 1978), with negligible effort in resource manipulation or relocation. This hit-and-run strategy prevails by minimizing the costs of the current reproductive effort in favor of individual survival and prospects of future reproduction while still taking advantage of a high-value resource. Alternatively, larvae of some silphid carrion beetles (Silphinae, e.g., Oiceoptoma) invest in chitinous armor and high mobility early which may greatly delay development but could increase their survival on contested carrion or allow them to leave to find new resources. Importantly, the carrion resources used by both carrion flies and Silphinae include large carcasses, upwards of e.g., rabbit-sized vertebrates, which are likely impossible to monopolize but are far less likely to deplete as rapidly as smaller bodies such as mice or birds.
These strategies are in stark contrast to necrophagous beetles of the genus Nicrophorus (Coleoptera: Silphidae, Figure 1). These beetles specialize in exploiting small animal carcasses, on which they engage in elaborate biparental care: after arriving at a carcass, the mating pair physically prepares the carrion into an edible nursery for their soon-to-emerge larvae by burying the carcass, removing hair or feathers, opening a feeding cavity, and applying exudates to control microbial colonization, thereby warding off decomposition and volatile smells. The pair mates repeatedly until emergence of the first larvae while the female continuously lays eggs near the buried carcass (Pukowski, 1933; Scott, 1998). As the larvae hatch after a few days, they migrate inside the carcass nursery where one or both parents continuously attend to them for several days – guarding against intruders, feeding the offspring with regurgitates (Pukowski, 1933; Eggert and Müller, 1997; Scott, 1998), and continually applying antimicrobial exudates (Hoback et al., 2004; Rozen et al., 2008; Cotter and Kilner, 2010b; Degenkolb et al., 2011; Palmer et al., 2016). As outlined above, dedicating to prolonged residence while caring for vulnerable offspring is expected to be associated with severe risks, including fitness penalties such as parental death and/or failure of the brood. On the flipside, successful carcass maintenance can result in a monopoly over the treated carcass from close competitors such as carrion flies or other carrion beetles. Prominent and abundant carcass breeders such as blow flies and Silphine beetles, which, in evolutionary terms, precede Nicrophorus as carrion specialists by a large margin, are much better adapted to endure on decomposing carrion, and their competition may have facilitated the transition of Nicrophorus to small carrion, microbial management, and parental care (Trumbo et al., 2016). It has been hypothesized that the specialization on monopolizing and maintaining fresh, small carcasses by visual concealment and control of MVOCs – instead of enduring microbial pressure and competition on larger and/or older carcasses as seen in Silphinae or Dipterans – is what steered the evolutionary trajectory of Nicrophorus towards nesting behavior, sociality, and parental care (Trumbo and Sikes, 2021).
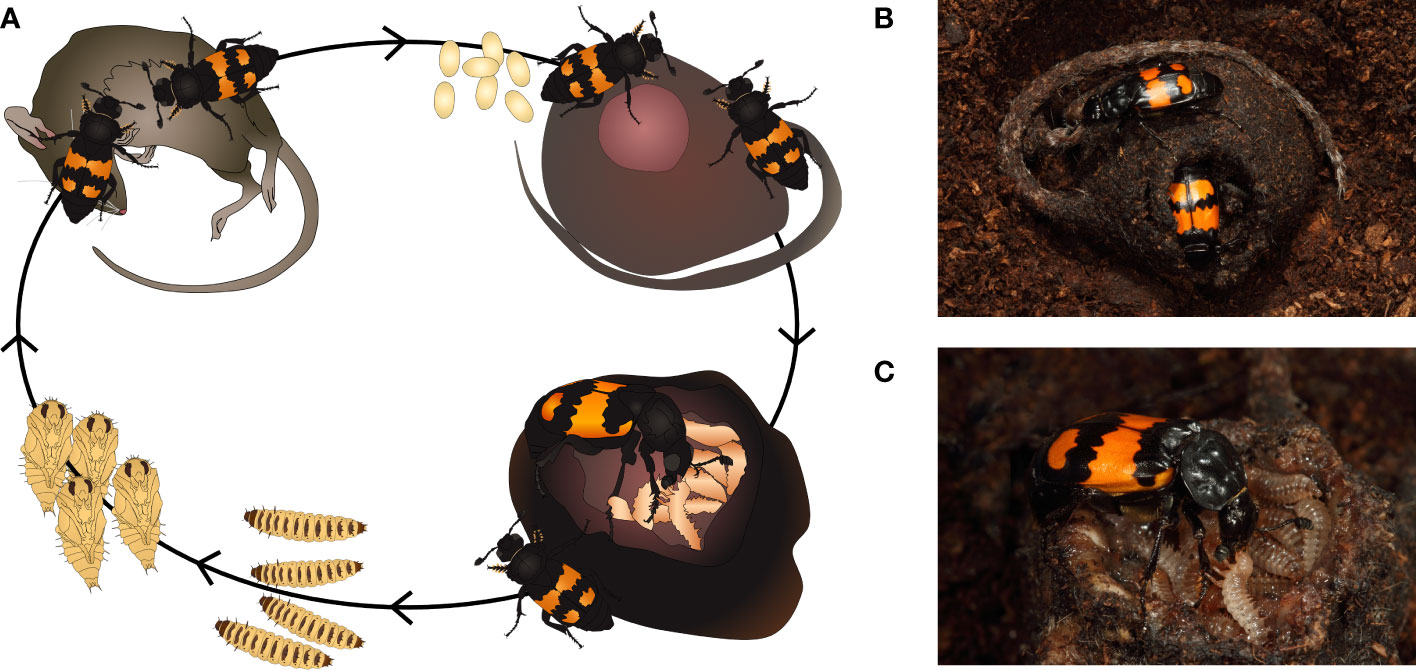
Figure 1 Nicrophorus burying beetles (here, N. vespilloides). (A) Typical Nicrophorus life cycle. After carcass discovery, males can advertise the resource to potential mates. After assembly, pairs mate repeatedly while preparing the carcass by removing fur or feathers and altering the carcass microbiome via application of oral and anal exudates. This prevents putrefaction and decay in addition to providing further benefits to larvae, likely via a biofilm of mutualistic bacteria, Yarrowia fungi, and their extracellular metabolites. (B) Mating pair on a carcass with early-stage larvae. Carcasses are continuously maintained during larval development by both sexes, but males primarily perform guard duty. Larvae also contribute to carcass maintenance with their own exudate excretions. (C) N. vespilloides female provisioning a begging larva. Parents provision via regurgitation after signaling their readiness chemically, triggering larval begging. However, larvae in several Nicrophorus species can feed completely independently, and in all Nicrophorus species, larvae shed their dependency on care after a few days. Both direct feeding as well as parental exudate application are likely candidates for the transfer of mutualist microbial symbionts. Photos by Heiko Bellmann. Graphics by Madlen Prang.
As a result, several facets of Nicrophorus’ unique breeding behaviors deal directly with vertebrate and invertebrate threats, such as burial of the carcass to avoid visual detection by scavengers or guard duty to prevent unwelcome arthropod intrusions. However, recent years have seen a surge of studies beginning to elucidate the selective pressures, mechanisms, and consequences associated with the beetles’ habit of subduing and/or modifying the microbial colonization of their otherwise rotting nursery (Vogel et al., 2017; Duarte et al., 2018; Shukla et al, 2018a; Shukla et al., 2018a). In addition to direct defense of the carrion against macroscopic and microscopic competitors, fostering of mutualistic symbionts during pre- and post-hatching care represent key mediators of the benefits of parental care in these species, making the burying beetles excellent model systems to understand these interlinkages. The substantial behavioral and physiological investments in face of harsh competition have been well-studied in the context of parental care evolution, which is traditionally expected to be promoted under restricting ecological conditions (Tallamy and Wood, 1986, but see Kramer et al., 2017). We now discuss these ecological conditions in detail to understand the role of resource management in driving social interactions and familial aggregations in carrion beetles.
Grave consequences: costs of microbial competitors to Nicrophorus species
Unregulated microbial growth can dramatically reduce the feasibility of carrion as food source and brood site over time. Left unchecked, it can have detrimental and sometimes catastrophic effects on Nicrophorus’ reproductive attempts. For instance, N. vespilloides and N. orbicollis parents reproducing on old, decomposing carcasses with a high load of microbial decomposers were more likely to experience brood failure and produce significantly smaller and fewer larvae (McLean et al., 2014; Trumbo et al., 2016; Delclos et al., 2021), thus leading to consistent fitness loss. Furthermore, in aged, deteriorated carcasses, larvae beg more for oral regurgitations from parents, and grow significantly slower than larvae reared on fresh carcasses (Rozen et al., 2008). While some of these detrimental effects may be mediated by adjustments to parental care behaviors in response to a poorer environment, there is also evidence for direct harm done by microbes. Adult females provided with aged carcasses not only produced fewer eggs, but egg survival and larval body mass are negatively affected after exposure to antagonistic grave-soil microbes or pathogens (Jacobs et al., 2014; Wang and Rozen, 2017). On the other hand, inhibition of microbe-driven decomposition prevents putrefactive gases used by scavengers and fellow invertebrates to detect carcasses (Madea et al., 2010). Prolonging early stages of decay and suppressing microbial volatiles may also inadvertently discourage conspecific competitors searching for food (as opposed to breeding grounds) to visit the nursery carcass, in which case they prefer carcasses in active decay (von Hoermann et al., 2016). Indeed, burying beetles preserve carrion by preventing accumulation of metabolites typically associated with carrion decomposition to support a more conducive environment that optimizes larval development (Shukla et al., 2018a; Trumbo et al., 2021). Thus, it is safe to conclude that governing the microbial surroundings represents a key aspect of burying beetle family life, and very well may be of particular importance in early social evolution. However, if we are to understand whether the challenge of regulating the surrounding microbiome represents a hindrance to (Cremer et al., 2007; Korb and Heinze, 2015) or driver (Jackson and Hart, 2009; Otti et al., 2014) of family life, it is necessary to delve into the mechanisms of antimicrobial defenses during parental care, how they are mediated by interactions between family members, as well as the role of beneficial microbial mutualists.
Antimicrobial defenses in individuals and social groups of carrion beetles
Burying beetles have evolved an elaborate and complex arsenal of antimicrobial defenses to overcome microbial competitors and threats. It has long been known that Nicrophorus pre- and post-hatching care involves smearing the carcass with oral and anal secretions, a behavior originally hypothesized to serve to retain moisture or deter other carrion visitors by advertising the beetles’ presence (Pukowski, 1933). More recent studies revealed that the anal exudates are characterized by antimicrobial activity, affecting the microbial community of the carcass by inhibiting several Gram-positive and Gram-negative bacteria, yeasts, and molds (Suzuki, 2001; Hoback et al., 2004; Cotter and Kilner, 2010a; Cotter and Kilner, 2010b; Hall et al., 2011; Steiger et al., 2011; Arce et al., 2012; Hwang and Lin, 2013). Exudates are produced outside of care but antimicrobial activity of the exudates is upregulated in the presence of a carcass (Cotter and Kilner, 2010b; Cotter et al., 2010; Steiger et al., 2011) whereas the volume of anal and oral secretions produced by the parents is thought to increase during breeding (Steiger S, personal obs.). The secretions, constituents of external immune defenses, (Otti et al., 2014) are costly, directly trading off with parts of the internal immunity (Cotter et al., 2013; Reavey et al., 2014b) and lifetime reproductive success of Nicrophorus parents (Cotter et al., 2010).
Similar external immunity mechanisms are also known to occur in other carrion breeders and feeders, such as blowfly maggots (Kerridge et al., 2005; Pöppel et al., 2015) which can facilitate the growth of a parentally derived, beneficial microbiome around them in absence of parental attendance (Crooks et al., 2016; Junkins et al., 2019). In burying beetles, however, externalized immune defense protects both parents and larvae due to extensive parental care, larval contributions, and overlapping generations, thus facilitating a form of social immunity unusual for subsocial insects (Cotter and Kilner, 2010a; Cotter and Kilner, 2010b; Meunier, 2015; Van Meyel et al., 2018). Indeed, Arce et al. (2012) found that the application of parental (anal) exudates or lysozyme equivalents significantly increased larval survival in the absence of parents. Although Nicrophorus larvae can also produce antimicrobial secretions (Arce et al., 2013; Reavey et al., 2014a), the exudates of the parents appear to be more potent, presumably due to a higher volume and better timing since parents apply their exudates before the larvae hatch and thus influence microbial growth earlier than their offspring. Additionally, parental social immunity can reduce the costs of larval defense mechanisms that would trade-off against investment in larval development time and biomass. In fact, in the presence of parents, the larvae downregulate genes associated with immune defenses (Ziadie et al., 2019), suggesting a highly context-dependent expression of immune-related genes and a potential trade-off between individual and social immunity. These findings overall indicate that harnessing control of the surrounding microbiome is at least as, if not more important, than provisioning or more physical defense of the larvae.
As a result of their apparent importance, the chemical composition of Nicrophorus secretions has drawn significant attention in recent years. So far, transcriptomics and proteomics suggest that a lysozyme (c-lysozyme-2; Jacobs et al., 2016; Palmer et al., 2016) and an antimicrobial peptide (thaumatin-4), which are also present in their anal exudates (Jacobs et al., 2016), are strongly induced during the period of parental care. A diverse array of 27 putative antimicrobial peptides, 13 lysozymes and other low molecular weight secondary metabolites present in the anal secretions (Degenkolb et al., 2011; Jacobs et al., 2016; Vogel et al., 2017) might also be used by parents to regulate the microbial communities of carcasses. Intriguingly, key parts of this antimicrobial recipe likely originate from microbes: several of the bacteria isolated from burying beetles are known to produce antimicrobial compounds (Heise et al., 2019), highlighting the important role of mutualist or commensalist microbes in regulating the carrion microbiome and shaping the larval environment.
Keeping friends close, enemies closer: social aggregations regulate beetle and carrion microbiota
Since breeding N. vespilloides beetles themselves produce a highly potent antimicrobial cocktail and additionally harbor microbes that produce antimicrobial compounds, one would imagine that the prepared carcass is a highly sanitized resource free of any characteristic microbiota. On the contrary, carcasses with breeding Nicrophorus rather it is teeming with a conserved and abundant bacterial and fungal community that the beetles deploy and carefully manage (Shukla et al., 2018a; Shukla et al., 2018b). For instance, parental secretions not only suppress competitive and parasitic bacteria but simultaneously serve to inoculate gut symbionts onto the carcass. Secretions produced by larval aggregations and those applied by parent beetles on the carcass surface have been hypothesized to create an interface between the carrion tissue and the developing larvae (Shukla et al., 2018b). This interface is likely facilitated by a biofilm-like carcass matrix consisting of mutualistic bacteria, Yarrowia fungi, and their extracellular metabolites that promote larval development. The fungal taxon assigned to Yarrowia sp. is consistently present in high relative proportions across the two subfamilies Nicrophorinae and Silphinae and was detected in all the eight genera of burying beetles investigated across the two subfamilies in a comparative study (Kaltenpoth and Steiger, 2014). Once on the carcass, Yarrowia is highly metabolically active and transcriptomic analysis of beetle-prepared carcasses indicates a potential role for the yeast in carrion digestion and detoxification, in maintaining carrion quality and promoting larval growth (Shukla et al., 2018a). Experimental removal of the carcass matrix (while leaving the carcass itself unaffected) leads to lower larval weight gain and biomass conversion for the same amount of carcass tissue consumed, strongly indicating that the matrix containing the parental secretions, symbionts, and predigested carrion nutrients acts as a source of additional nutritional benefits to the developing larvae (Shukla et al., 2018a). This matrix also produces heat, which could indirectly benefit larvae by increasing carcass processing by symbionts, and directly by also improving larval metabolic rate and development (Matuszewski and Mądra-Bielewicz, 2021).
Caring is sharing? Microbe transfer as a major factor of family life benefits
There is a correlation between similarities in microbiome compositions and social cohesion between group members in many animals including insects (Archie and Tung, 2015). Sharing of mutualistic microbiota promoting larval development may indeed be a key driver of familial aggregations in general. Overlap of generations and prolonged parental care is one way to maximize the transmission and spread of symbiotic microorganisms within family members – parental care and extended familial aggregations on carcasses can be selected to ensure a steady and assured prevalence of symbionts on carcasses. Beneficial symbiotic microbes can be effective in spreading across aggregated conspecifics – a factor which may represent a distinct advantage of carrion breeders exhibiting post-hatching care over their less attentive co-tenants. Given the apparent importance of successful symbiont transmission, the stochasticity in carrion’s spatial distribution and its susceptibility to be colonized by decomposers, pathogens, and parasites, one may very well predict that Nicrophorus parents host and vertically transmit a core set of digestive and defensive symbionts to their offspring, rather than relying on acquiring beneficial microorganisms horizontally every generation.
It has been shown that N. vespilloides larvae that receive parentally transmitted microbiota produce larger broods, and that the mutualistic bacteria perform better in colonizing the larval gut than environmental and entomopathogenic bacteria, providing an important buffer against infection (Wang and Rozen, 2017). In turn, experimental broad removal of bacterial and/or fungal denizens of a carcass nursery did not yield larger broods, suggesting that either the prevention of microbial deterioration is not the primary selective force for manipulating the microbial environment, or that indiscriminate removal of the microbiome on the carcass is inadvertently costly due to the removal of important beneficial interactions with some of its parts (Trumbo et al., 2016). While parental application of gut symbionts on the carcass likely facilitates a route for symbiont acquisition by the offspring, a recent study suggests that transmission of beneficial microbiota from parents to larvae may occur through direct feeding contact, i.e., regurgitation, rather than inoculation of the carcass (Miller et al., 2021).
Regardless of the mode of transmission of the beneficial microbes, it is becoming more apparent that host–microbe interactions are key to the larval health and growth benefits of Nicrophorus family aggregations. Having received attention only relatively recently, the importance of beneficial host–microbe interactions may turn out to be a critical piece of the puzzle of family life evolution in general and Nicrophorus systems in particular, as it may very well be central to negotiating hazardous carrion breeding. An important aspect here is that the beetles externalize their hindgut microbiota (or at least key microbes; Miller et al., 2019) on to the carcass surface. There, the bacteria and the yeast are metabolically active and involved in breaking down the carcass and possibly making available nutrients to the developing larvae, detoxifying toxic metabolites, and providing defense against bacteria, eukaryotic antagonists, and even nematodes (Heise et al., 2019; Wang and Rozen, 2019) – all of which very likely provides key fitness benefits to the beetles (Shukla et al., 2018a).
In many cases where insect–symbiont interactions have been studied, transmission of symbionts to the offspring occurs without elaborate parental care or social interactions – often transovarially, through egg surfaces, or through special glands or mycangia. However, in several cases the symbiont is also externalized on a dietary resource, a place often associated with social interactions of varying degrees, as seen in ambrosia beetles, fungus-growing termites, and ants (Quinlan and Cherret, 1977; Mueller et al., 2005). In burying beetles, this dietary resource is the carcass, and application of microbes represents both externalization of the symbiont as well as a (albeit perhaps less prominent) form of vertical transmission from parents to offspring. The ephemeral, microbe-prone and highly competitive nature of the resource selects for larval aggregations, biparental care, and overlap of generations. Further, parental secretions and reorganization of the carcass into a nest support the growth of a mutualistic microbial community. The constant threat of microbial challenges from the surrounding soil, the need to replenish antimicrobial defenses on the carrion to maintain control over volatiles, the necessity to maintain appropriate densities of mutualistic yeast, and a shared resource whose health dictates the survival of the entire brood that benefit from the growth of the microbiota, all are thus important determinants for selection favoring enhanced parental care by the beetles and prolonged familial aggregations. Intriguingly, very similar selective conditions and strategies can be found in other exploiters of ephemeral resources: for instance, Onthophagus taurus dung beetles also provide their larvae with an edible nursery, provide biparental pre-hatching care by concealing their prize below ground, and transfer crucial microbial mutualists via maternal “gifts” in the brood ball (Hunt and Simmons, 2002; Estes et al., 2013; Shukla et al., 2016) – but do not attend their brood post hatching, nor can the larvae aggregate or invest into a mutually beneficial manipulation of the surrounding microbiome. This difference in strategies further highlights how the extreme ephemerality of carrion, even compared to dung (Englmeier et al., 2023), and associated need for microbial management, may have driven the evolution of social behaviors and parental care in Nicrophorus beetles.
Conclusion
Adverse and difficult living conditions are considered key drivers of the evolution of social behaviors and lifestyles. Using the increasingly important model of Nicrophorus burying beetles, recent studies have explored this special evolutionary interaction by outlining the constant onslaught of microbes on Nicrophorus nurseries and its costly consequences, and how caring parents and cooperating offspring tackle this threat. This review highlights the special role of microbial mutualists in this social endeavor. We examine how a relatively simple, non-derived form of sociality benefits from these microbial mutualists by receiving developmental and immunity advantages, and how this may select for committed parental care and more obligate social life down the road by encouraging prolonged social associations through vertical transfer. In turn, we emphasize that many of aspects, details, and forms of these important host–microbe interactions remain largely unknown, or opaque. Importantly, it has been hypothesized that microbial mutualists not only grant benefits by providing immediate benefits, but may even encourage social interactions in their hosts (Lewin-Epstein et al., 2017). The occurrence, degree, and mechanisms of such a phenomenon, however, remain largely theoretical, as do the evolutionary feedbacks of such an interaction on microbes (Biedermann and Rohlfs, 2017). So far, empirical evidence is severely lacking, and we argue that Nicrophorus offers an interesting and ideally suitable system to explore this exciting new avenue into social evolution.
Author contributions
MK and SPS took the lead in writing the manuscript. All authors contributed equally to the content of the manuscript and approved the submitted version.
Funding
Funded by the Deutsche Forschungsgemeinschaft (DFG, German Research Foundation) – 491183248. Funded by the Open Access Publishing Fund of the University of Bayreuth. SPS acknowledges funds from the Department of Biotechnology and Science and Engineering Research Board, Government of India.
Acknowledgments
We thank Jos Kramer for his comments on the manuscript.
Conflict of interest
The authors declare that the research was conducted in the absence of any commercial or financial relationships that could be construed as a potential conflict of interest.
Publisher’s note
All claims expressed in this article are solely those of the authors and do not necessarily represent those of their affiliated organizations, or those of the publisher, the editors and the reviewers. Any product that may be evaluated in this article, or claim that may be made by its manufacturer, is not guaranteed or endorsed by the publisher.
References
Altizer S., Nunn C. L. (2006). Infectious disease in primates (Oxford, UK: Oxford University Press).
Anderson G., Cervenka V. (2002). “Insects associated with the body,” in Advances in forensic taphonomy: method, theory, and archaeological perspectives. Eds. Haglund W., Sorg M. (Boca Raton, FL: CRC Press).
Arce A. N., Johnston P. R., Smiseth P. T., Rozen D. E. (2012). Mechanisms and fitness effects of antibacterial defences in a carrion beetle. J. Evol. Biol. 25, 930–937. doi: 10.1111/j.1420-9101.2012.02486.x
Arce A. N., Smiseth P. T., Rozen D. E. (2013). Antimicrobial secretions and social immunity in larval burying beetles, Nicrophorus vespilloides. Anim. Behav. 86, 741–745. doi: 10.1016/j.anbehav.2013.07.008
Archie E. A., Tung J. (2015). Social behavior and the microbiome. Curr. Opin. Behav. Sci. 6, 28–34. doi: 10.1016/j.cobeha.2015.07.008
Barke J., Seipke R. F., Yu D. W., Hutchings M. I. (2011). A mutualistic microbiome. Commun. Integr. Biol. 4, 41–43. doi: 10.4161/cib.13552
Bärlocher F. (1985). The role of fungi in the nutrition of stream invertebrates. Bot. J. Linn. Soc 91, 83–94. doi: 10.1111/j.1095-8339.1985.tb01137.x
Bautista A., Drummond H., Martínez-Gómez M., Hudson R. (2003). Thermal benefit of sibling presence in the newborn rabbit. Dev. Psychobiol. 43, 208–215. doi: 10.1002/dev.10134
Biedermann P. H. W., Rohlfs M. (2017). Evolutionary feedbacks between insect sociality and microbial management. Curr. Opin. Insect Sci. 22, 92–100. doi: 10.1016/j.cois.2017.06.003
Brune A., Ohkuma M. (2011). “Role of the termite gut microbiota in symbiotic digestion,” in Biology of termites: A modern synthesis. Eds. Bignell D. E., Roisin Y., Lo N. (Berlin, Germany: Springer), vol. pp. , 439–475.
Buchholz R., Levey D. J. (1990). The evolutionary triad of microbes, fruits, and seed dispersers: an experiment in fruit choice by cedar waxwings, bombycilla cedrorum. Oikos 59, 200. doi: 10.2307/3545535
Buckley N. J. (1996). Food finding and the influence of information, local enhancement, and communal roosting on foraging success of north american vultures. Auk 113, 473–488. doi: 10.2307/4088913
Charabidze D., Trumbo S., Grzywacz A., Costa J. T., Benbow M. E., Barton P. S., et al. (2021). Convergence of social strategies in carrion breeding insects. Bioscience 71, 1028–1037. doi: 10.1093/biosci/biab068
Colston T. J., Jackson C. R. (2016). Microbiome evolution along divergent branches of the vertebrate tree of life: what is known and unknown. Mol. Ecol. 25, 3776–3800. doi: 10.1111/mec.13730
Costa J. T. (2018). The other insect societies: overview and new directions. Curr. Opin. Insect Sci. 28, 40–49. doi: 10.1016/j.cois.2018.04.008
Cotter S. C., Kilner R. M. (2010a). Personal immunity versus social immunity. Behav. Ecol. 21, 663–668. doi: 10.1093/beheco/arq070
Cotter S. C., Kilner R. M. (2010b). Sexual division of antibacterial resource defence in breeding burying beetles, Nicrophorus vespilloides. J. Anim. Ecol. 79, 35–43. doi: 10.1111/j.1365-2656.2009.01593.x
Cotter S. C., Littlefair J. E., Grantham P. J., Kilner R. M. (2013). A direct physiological trade-off between personal and social immunity. J. Anim. Ecol. 82, 846–853. doi: 10.1111/1365-2656.12047
Cotter S. C., Topham E., Price A. J. P., Kilner R. M. (2010). Fitness costs associated with mounting a social immune response. Ecol. Lett. 13, 1114–1123. doi: 10.1111/j.1461-0248.2010.01500.x
Cremer S., Armitage S., Schmid-Hempel P. (2007). Social immunity. Curr. Biol. 17, R693–R702. doi: 10.1016/j.cub.2007.06.008
Crooks E. R., Bulling M. T., Barnes K. M. (2016). Microbial effects on the development of forensically important blow fly species. Forensic Sci. Int. 266, 185–190. doi: 10.1016/j.forsciint.2016.05.026
Currie C. R. (2001). A community of ants, fungi, and bacteria: A multilateral approach to studying symbiosis. Annu. Rev. Microbiol. 55, 357–380. doi: 10.1146/annurev.micro.55.1.357
Degenkolb T., Düring R. A., Vilcinskas A. (2011). Secondary metabolites released by the burying beetle nicrophorus vespilloides: chemical analyses and possible ecological functions. J. Chem. Ecol. 37, 724–735. doi: 10.1007/s10886-011-9978-4
Delclos P. J., Bouldin T. L., Tomberlin J. K. (2021). Olfactory choice for decomposition stage in the burying beetle Nicrophorus vespilloides: Preference or aversion? Insects 11, 1–12. doi: 10.3390/insects12010011
DeVault T. L., Rhodes O. E., Shivik J. A. (2003). Scavenging by vertebrates: Behavioral, ecological, and evolutionary perspectives on an important energy transfer pathway in terrestrial ecosystems. Oikos 102, 225–234. doi: 10.1034/j.1600-0706.2003.12378.x
Duarte A., Welch M., Swannack C., Wagner J., Kilner R. M. (2018). Strategies for managing rival bacterial communities: Lessons from burying beetles. J. Anim. Ecol. 87, 414–427. doi: 10.1111/1365-2656.12725
Ebensperger L. A., Bozinovic F. (2000). Communal burrowing in the hystricognath rodent, Octodon degus: a benefit of sociality? Behav. Ecol. Sociobiol. 47, 365–369. doi: 10.1007/s002650050678
Eggert A.-K., Müller J. K. (1997). “Biparental care and social evolution in burying beetles: lessons from the larder,” in The evolution of social behavior in insects and arachnids. Eds. Choe J. C., Crespi B. J. (Cambridge, UK: Cambridge University Press), 216–236
Englmeier J., Mitesser O., Benbow M. E., Hothorn T., von Hoermann C., Benjamin C., et al. (2023). Diverse effects of climate, land use, and insects on dung and carrion decomposition. Ecosystems 26, 397–411. doi: 10.1007/s10021-022-00764-7
Estes A. M., Hearn D. J., Snell-Rood E. C., Feindler M., Feeser K., Abebe T., et al. (2013). Brood ball-mediated transmission of microbiome members in the dung beetle, Onthophagus taurus (Coleoptera: Scarabaeidae). PloS One 8, 1–15. doi: 10.1371/journal.pone.0079061
Fialho V. S., Rodrigues V. B., Elliot S. L. (2018). Nesting strategies and disease risk in necrophagous beetles. Ecol. Evol. 8, 3296–3310. doi: 10.1002/ece3.3919
Forbes S. (2008). “Decomposition chemistry in a burial environment,” in Soil analysis in forensic taphonomy. Eds. Tibbett M., Carter D. O. (Boca Raton, FL: CRC Press), 203–223.
Forbes S. L., Carter D. O. (2016). “Processes and mechanisms of death and decomposition of vertebrate carrion,” in Carrion ecology, evolution, and their applications. Eds. Benbow M. E., Tomberlin J. K., Tarone A. M. (Boca Raton, FL: CRC Press).
Gill-King H. (1997). “Chemical and ultrastructural aspects of decomposition,” in Forensic taphonomy: the postmortem fate of human remains. Eds. Haglund W., Sorg M. (Boca Raton, FL: CRC Press), 93–108.
Gram L., Huss H. H. (1996). Microbiological spoilage of fish and fish products. Int. J. Food Microbiol. 33, 121–137. doi: 10.1016/0168-1605(96)01134-8
Greenberg B. (1991). Flies as forensic indicators. J. Med. Entomol. 28, 565–577. doi: 10.1093/jmedent/28.5.565
Hall C. L., Wadsworth N. K., Howard D. R., Jennings E. M., Farrell L. D., Magnuson T. S., et al. (2011). Inhibition of microorganisms on a carrion breeding resource: The antimicrobial peptide activity of burying beetle (Coleoptera: Silphidae) oral and anal secretions. Environ. Entomol. 40, 669–678. doi: 10.1603/EN10137
Hamilton W. D. (1987). “Kinship, recognition, disease and intelligence,” in Animal societies: theories and facts. Eds. Brown L., Kikkawa J. (Tokyo: Japan Scientific Societies Press).
Hanski I. (1987). Carrion fly community dynamics: patchiness, seasonality and coexistence. Ecol. Entomol. 12, 257–266. doi: 10.1111/j.1365-2311.1987.tb01004.x
Heise P., Liu Y., Degenkolb T., Vogel H., Schäberle T. F., Vilcinskas A. (2019). Antibiotic-producing beneficial bacteria in the gut of the burying beetle Nicrophorus vespilloides. Front. Microbiol. 10, 1–11. doi: 10.3389/fmicb.2019.01178
Hoback W. W., Bishop A. A., Kroemer J., Scalzitti J., Shaffer J. J. (2004). Differences among antimicrobial properties of carrion beetle secretions reflect phylogeny and ecology. J. Chem. Ecol. 30, 719–729. doi: 10.1023/B:JOEC.0000028427.53141.41
Hunt J., Simmons L. W. (2002). Behavioural dynamics of biparental care in the dung beetle Onthophagus taurus. Anim. Behav. 64, 65–75. doi: 10.1006/anbe.2002.3036
Hwang W., Lin H.-M. (2013). Carcass fungistasis of the burying beetle Nicrophorus nepalensis hope (Coleoptera: Silphidae) in A Journal of Entomology. (Psyche (London), 2013. doi: 10.1155/2013/162964
Jackson D. E., Hart A. G. (2009). Does sanitation facilitate sociality? Anim. Behav. 77, e1–e5. doi: 10.1016/j.anbehav.2008.09.013
Jacobs C. G., Steiger S., Heckel D. G., Wielsch N., Vilcinskas A., Vogel H. (2016). Sex, offspring and carcass determine antimicrobial peptide expression. Sci. Rep. 6, 2–9. doi: 10.1038/srep25409
Jacobs C. G. C., Wang Y., Vogel H., Vilcinskas A., van der Zee M., Rozen D. E. (2014). Egg survival is reduced by grave-soil microbes in the carrion beetle, Nicrophorus vespilloides. BMC Evol. Biol. 14, 1–8. doi: 10.1186/s12862-014-0208-x
Janzen D. H. (1977). Why fruits rot, seeds mold, and meat spoils. Am. Nat. 111, 691–713. doi: 10.1086/283200
Junkins E. N., Speck M., Carter D. O. (2019). The microbiology, pH, and oxidation reduction potential of larval masses in decomposing carcasses on Oahu, Hawaii. J. Forensic Leg. Med. 67, 37–48. doi: 10.1016/j.jflm.2019.08.001
Kaltenpoth M., Engl T. (2014). Defensive microbial symbionts in Hymenoptera. Funct. Ecol. 28, 315–327. doi: 10.1111/1365-2435.12089
Kaltenpoth M., Steiger S. (2014). Unearthing carrion beetles’ microbiome: Characterization of bacterial and fungal hindgut communities across the Silphidae. Mol. Ecol. 23, 1251–1267. doi: 10.1111/mec.12469
Kerridge A., Lappin-Scott H., Stevens J. R. (2005). Antibacterial properties of larval secretions of the blowfly, Lucilia sericata. Med. Vet. Entomol. 19, 333–337. doi: 10.1111/j.1365-2915.2005.00577.x
Kinlaw A. (1999). A review of burrowing by semi-fossorial vertebrates in arid environments. J. Arid Environ. 41, 127–145. doi: 10.1006/jare.1998.0476
Koch H., Schmid-Hempel P. (2011). Socially transmitted gut microbiota protect bumble bees against an intestinal parasite. Proc. Natl. Acad. Sci. U.S.A. 108, 19288–19292. doi: 10.1073/pnas.1110474108
Korb J., Heinze J. (2015). Major hurdles for the evolution of sociality. Annu. Rev. Entomol. 61, 297–316. doi: 10.1146/annurev-ento-010715-023711
Körner M., Foitzik S., Meunier J. (2018). Extended winters entail long-term costs for insect offspring reared in an overwinter burrow. J. Therm. Biol. 74, 116–122. doi: 10.1016/j.jtherbio.2018.03.021
Körner M., Vogelweith F., Libbrecht R., Foitzik S., Feldmeyer B., Meunier J. (2020). Offspring reverse transcriptome responses to maternal deprivation when reared with pathogens in an insect with facultative family life. Proc. R. Soc B Biol. Sci. 287. doi: 10.1098/rspb.2020.0440
Kramer J., Körner M., Diehl J. M. C., Scheiner C., Yüksel-Dadak A., Christl T., et al. (2017). When earwig mothers do not care to share: Parent-offspring competition and the evolution of family life. Funct. Ecol. 31, 2098–2107. doi: 10.1111/1365-2435.12915
Kramer J., Meunier J. (2019). The other facets of family life and their role in the evolution of animal sociality. Biol. Rev. 94, 199–215. doi: 10.1111/brv.12443
Kreitlow K. L. (2010). “Insect succession in a natural environment,” in Forensic entomology: the utility of arthropods in legal investigations. Eds. Byrd J., Castner J. (Boca Raton, FL: CRC Press), 251–270.
LeBlanc H. N., Logan J. G. (2010). “Current concepts in forensic entomology,” in Current concepts in forensic entomology. Eds. Amendt J., Goff M. L., Campobasso C. P., Grassberger M. (Dordrecht: Springer), 205–221.
Lewin-Epstein O., Aharonov R., Hadany L. (2017). Microbes can help explain the evolution of host altruism. Nat. Commun. 8, 1–7. doi: 10.1038/ncomms14040
Lin N., Michener C. D. (1972). Evolution of sociality in insects. Q. Rev. Biol. 47, 131–159. doi: 10.1086/407216
Lombardo M. P. (2008). Access to mutualistic endosymbiotic microbes: An underappreciated benefit of group living. Behav. Ecol. Sociobiol. 62, 479–497. doi: 10.1007/s00265-007-0428-9
Lowery C. A., Dickerson T. J., Janda K. D. (2008). Interspecies and interkingdom communication mediated by bacterial quorum sensing. Chem. Soc Rev. 37, 1337–1346. doi: 10.1039/b702781h
Madea B., Preuss J., Musshoff F. (2010). “From flourishing life to dust—The natural cycle of growth and decay,” in Mummies of the world. Eds. Wieczorek A., Rosendahl W. (Munich: Prestel Verlag), 14–29.
Matuszewski S., Mądra-Bielewicz A. (2021). Heat production in a feeding matrix formed on carrion by communally breeding beetles. Front. Zool. 18, 1–12. doi: 10.1186/s12983-020-00385-7
McFall-Ngai M., Hadfield M. G., Bosch T. C. G., Carey H. V., Domazet-Lošo T., Douglas A. E., et al. (2013). Animals in a bacterial world, a new imperative for the life sciences. Proc. Natl. Acad. Sci. 110, 3229–3236. doi: 10.1073/pnas.1218525110
McLean A. H. C., Arce A. N., Smiseth P. T., Rozen D. E. (2014). Late-life and intergenerational effects of larval exposure to microbial competitors in the burying beetle Nicrophorus vespilloides. J. Evol. Biol. 27, 1205–1216. doi: 10.1111/jeb.12394
Meunier J. (2015). Social immunity and the evolution of group living in insects. Philos. Trans. B 370, 19–21. doi: 10.1098/rstb.2014.0102
Miller C., Bates S. T., Gielda L. M., Creighton J. C. (2021). The role of parental care in the establishment of the offspring digestive tract microbiome in Nicrophorus defodiens.. Anim Behav. 172, 35–44. doi: 10.1016/j.anbehav.2020.11.006
Miller C. J., Bates S. T., Gielda L. M., Curtis Creighton J. (2019). Examining transmission of gut bacteria to preserved carcass via anal secretions in Nicrophorus defodiens. PloS One 14, 1–13. doi: 10.1371/journal.pone.0225711
Mueller U. G., Gerardo N. M., Aanen D. K., Six D. L., Schultz T. R. (2005). The evolution of agriculture in insects. Annu. Rev. Ecol. Evol. Syst. 36, 563–595. doi: 10.1146/annurev.ecolsys.36.102003.152626
Otti O., Tragust S., Feldhaar H. (2014). Unifying external and internal immune defences. Trends Ecol. Evol. 29, 625–634. doi: 10.1016/j.tree.2014.09.002
Palmer W. J., Duarte A., Schrader M., Day J. P., Kilner R. M., Jiggins F. M. (2016). A gene associated with social immunity in the burying beetle Nicrophorus vespilloides. Proc. R. Soc B Biol. Sci. 283, 20152733. doi: 10.1098/rspb.2015.2733
Pechal J. L., Benbow M. E., Crippen T. L., Tarone A. M., Tomberlin J. K. (2014). Delayed insect access alters carrion decomposition and necrophagous insect community assembly. Ecosphere 5, 1–21. doi: 10.1890/ES14-00022.1
Pechal J. L., Crippen T. L., Tarone A. M., Lewis A. J., Tomberlin J. K., Benbow M. E. (2013). Microbial community functional change during vertebrate carrion decomposition. PloS One 8, 1–11. doi: 10.1371/journal.pone.0079035
Pöppel A. K., Vogel H., Wiesner J., Vilcinskas A. (2015). Antimicrobial peptides expressed in medicinal maggots of the blow fly Lucilia sericata show combinatorial activity against bacteria. Antimicrob. Agents Chemother. 59, 2508–2514. doi: 10.1128/AAC.05180-14
Pukowski E. (1933). Ökologische untersuchungen an necrophorus F. Zeitschrift für morphol. und Ökologie der Tiere 27, 518–586. doi: 10.1007/BF00403155
Putman R. J. (1978). Flow of energy and organic matter from a carcase during decomposition: decomposition of small mammal carrion in temperate systems 2. Oikos 31, 58. doi: 10.2307/3543384
Quinlan R. J., Cherret J. M. (1977). The role of substrate preparation in the symbiosis between the leaf-cutting ant Acromyrmex octospinosus (Reich) and its food fungus. Ecol. Entomol. 2, 161–170. doi: 10.1111/j.1365-2311.1977.tb00877.x
Reavey C. E., Beare L., Cotter S. C. (2014a). Parental care influences social immunity in burying beetle larvae. Ecol. Entomol. 39, 395–398. doi: 10.1111/een.12099
Reavey C. E., Warnock N. D., Vogel H., Cotter S. C. (2014b). Trade-offs between personal immunity and reproduction in the burying beetle, Nicrophorus vespilloides. Behav. Ecol. 25, 415–423. doi: 10.1093/beheco/art127
Roces F., Kleineidam C. (2000). Humidity preference for fungus culturing by workers of the leaf-cutting ant Atta sexdens rubropilosa. Insectes Soc 47, 348–350. doi: 10.1007/PL00001728
Rozen D. E., Engelmoer D. J. P., Smiseth P. T. (2008). Antimicrobial strategies in burying beetles breeding on carrion. Proc. Natl. Acad. Sci. U.S.A. 105, 17890–17895. doi: 10.1073/pnas.0805403105
Rubenstein D. R., Abbot P. (2017). “The evolution of social evolution,” in Comparative social evolution. Eds. Rubenstein D. R., Abbot P. (Cambridge, MA: Cambridge University Press), 1–18.
Scanvion Q., Hédouin V., Charabidzé D. (2018). Collective exodigestion favours blow fly colonization and development on fresh carcasses. Anim. Behav. 141, 221–232. doi: 10.1016/j.anbehav.2018.05.012
Scott M. P. (1998). The ecology and behavior of burying beetles. Annu. Rev. Entomol. 43, 595–618. doi: 10.1146/annurev.ento.43.1.595
Sherratt T. N., Wilkinson D. M., Bain R. S. (2006). Why fruits rot, seeds mold and meat spoils: A reappraisal. Ecol. Modell. 192, 618–626. doi: 10.1016/j.ecolmodel.2005.07.030
Shukla S. P., Plata C., Reichelt M., Steiger S., Heckel D. G., Kaltenpoth M., et al. (2018a). Microbiome-assisted carrion preservation aids larval development in a burying beetle. Proc. Natl. Acad. Sci. U.S.A. 115, 11274–11279. doi: 10.1073/pnas.1812808115
Shukla S. P., Sanders J. G., Byrne M. J., Pierce N. E. (2016). Gut microbiota of dung beetles correspond to dietary specializations of adults and larvae. Mol. Ecol. 25, 6092–6106. doi: 10.1111/mec.13901
Shukla S. P., Vogel H., Heckel D. G., Vilcinskas A., Kaltenpoth M. (2018b). Burying beetles regulate the microbiome of carcasses and use it to transmit a core microbiota to their offspring. Mol. Ecol. 27, 1980–1991. doi: 10.1111/mec.14269
Shykoff J. A., Schmid-Hempel P. (1991). Parasites and the advantage of genetic variability within social insect colonies. Proc. R. Soc B Biol. Sci. 243, 55–58. doi: 10.1098/rspb.1991.0009
Steiger S., Gershman S. N., Pettinger A. M., Eggert A.-K., Sakaluk S. K. (2011). Sex differences in immunity and rapid upregulation of immune defence during parental care in the burying beetle, Nicrophorus orbicollis. Funct. Ecol. 25, 1368–1378. doi: 10.1111/j.1365-2435.2011.01895.x
Stotzky G., Schenck S. (1971). Volatile organic compounds and microorganisms. CRC Crit. Rev. Microbiol. 4, 333–382. doi: 10.3109/10408417609102303
Suzuki S. (2001). Suppression of dungal development on carcasses by the burying beetle Nicrophorus quadripunctatus (Coleoptera: Silphidae). Entomol. Sci. 4, 403–405.
Tallamy D. W., Wood T. K. (1986). Convergence patterns in subsocial insects. Annu. Rev. Entomol. 31, 369–390. doi: 10.1146/annurev.en.31.010186.002101
Tomberlin J. K., Crippen T. L., Tarone A. M., Singh B., Adams K., Rezenom Y. H., et al. (2012). Interkingdom responses of flies to bacteria mediated by fly physiology and bacterial quorum sensing. Anim. Behav. 84, 1449–1456. doi: 10.1016/j.anbehav.2012.09.013
Trumbo S. T., Philbrick P. K. B., Stökl J., Steiger S. (2021). Burying beetle parents adaptively manipulate information broadcast from a microbial community. Am. Nat. 197, 366–378. doi: 10.1086/712602
Trumbo S. T., Sikes D. S. (2021). Resource concealment and the evolution of parental care in burying beetles. J. Zool. 1, 1–8. doi: 10.1111/jzo.12916
Trumbo S. T., Sikes D. S., Philbrick P. K. B. (2016). Parental care and competition with microbes in carrion beetles: a study of ecological adaptation. Anim. Behav. 118, 47–54. doi: 10.1016/j.anbehav.2016.06.001
Van Meyel S., Körner M., Meunier J. (2018). Social immunity: why we should study its nature, evolution and functions across all social systems. Curr. Opin. Insect Sci. 28, 1–7. doi: 10.1016/j.cois.2018.03.004
Vogel H., Shukla S. P., Engl T., Weiss B., Fischer R., Steiger S., et al. (2017). The digestive and defensive basis of carcass utilization by the burying beetle and its microbiota. Nat. Commun. 8, 1–15. doi: 10.1038/ncomms15186
Vogelweith F., Körner M., Foitzik S., Meunier J. (2017). Age, pathogen exposure, but not maternal care shape offspring immunity in an insect with facultative family life. BMC Evol. Biol. 17. doi: 10.1186/s12862-017-0926-y
von Hoermann C., Ruther J., Ayasse M. (2016). Volatile organic compounds of decaying piglet cadavers perceived by nicrophorus vespilloides. J. Chem. Ecol. 42, 756–767. doi: 10.1007/s10886-016-0719-6
von Hoermann C., Weithmann S., Sikorski J., Nevo O., Szpila K., Grzywacz A., et al. (2022). Linking bacteria, volatiles and insects on carrion: the role of temporal and spatial factors regulating inter-kingdom communication via volatiles. R. Soc Open Sci. 9. doi: 10.1098/rsos.220555
Wada-Katsumata A., Zurek L., Nalyanya G., Roelofs W. L., Zhang A., Schal C. (2015). Gut bacteria mediate aggregation in the German cockroach. Proc. Natl. Acad. Sci. U.S.A. 112, 15678–15683. doi: 10.1073/pnas.1504031112
Wang Y., Rozen D. E. (2017). Gut microbiota in the burying beetle, Nicrophorus vespilloides, provide colonization resistance against larval bacterial pathogens. Ecol. Evol. 8, 1646–1654. doi: 10.1002/ece3.3589
Wang Y., Rozen D. E. (2019). Fitness costs of phoretic nematodes in the burying beetle, Nicrophorus vespilloides. Ecol. Evol. 9, 26–35. doi: 10.1002/ece3.4570
Wilson E. E., Wolkovich E. M. (2011). Scavenging: How carnivores and carrion structure communities. Trends Ecol. Evol. 26, 129–135. doi: 10.1016/j.tree.2010.12.011
Yang L. H., Bastow J. L., Spence K. O., Wright A. N. (2008). What can we learn from resource pulses? Ecology 89, 621–634. doi: 10.1890/07-0175.1
Keywords: Nicrophorus, social evolution, carrion breeder, microbe associations, microbial mutualists
Citation: Körner M, Steiger S and Shukla SP (2023) Microbial management as a driver of parental care and family aggregations in carrion feeding insects. Front. Ecol. Evol. 11:1252876. doi: 10.3389/fevo.2023.1252876
Received: 04 July 2023; Accepted: 04 August 2023;
Published: 18 August 2023.
Edited by:
Silvio Erler, Julius Kühn-Institut – Braunschweig, GermanyReviewed by:
Sheena Cotter, University of Lincoln, United KingdomTobias Engl, Max Planck Institute for Chemical Ecology, Germany
Copyright © 2023 Körner, Steiger and Shukla. This is an open-access article distributed under the terms of the Creative Commons Attribution License (CC BY). The use, distribution or reproduction in other forums is permitted, provided the original author(s) and the copyright owner(s) are credited and that the original publication in this journal is cited, in accordance with accepted academic practice. No use, distribution or reproduction is permitted which does not comply with these terms.
*Correspondence: Maximilian Körner, bWF4a29lcm5lckBnbXgubmV0