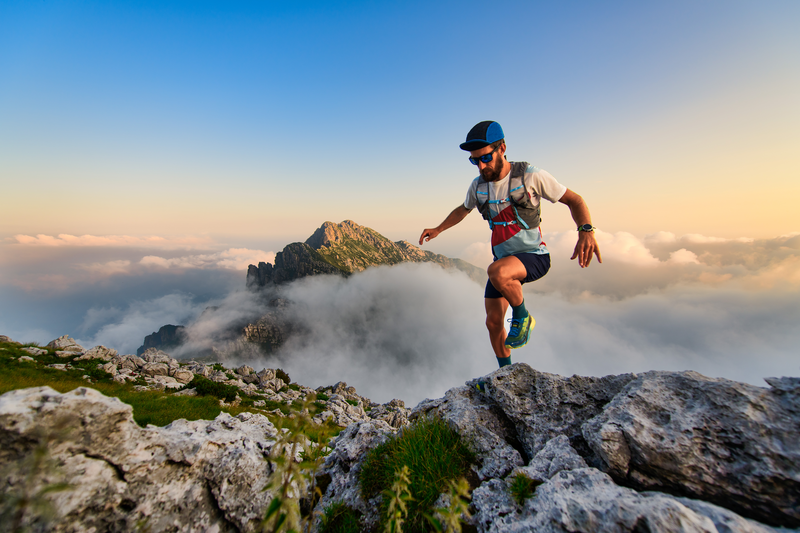
94% of researchers rate our articles as excellent or good
Learn more about the work of our research integrity team to safeguard the quality of each article we publish.
Find out more
ORIGINAL RESEARCH article
Front. Ecol. Evol. , 03 August 2023
Sec. Urban Ecology
Volume 11 - 2023 | https://doi.org/10.3389/fevo.2023.1248515
The rapid expansion and implementation of digital technologies are profoundly changing our lifestyles, production methods, and modes of exchange. However, whether or not such change can help promote the sustainability of urban socio-environmental systems has not been fully investigated. In this paper, we propose a comprehensive framework that utilizes the spatial Durbin model to investigate whether and how the digital economy contributes to low-carbon urban development, using the panel data of 276 Chinese cities from 2011 to 2018. This framework systematically identifies triple dimensions of low-carbon transition taking place throughout the whole value chain system, i.e. green innovation, production reshaping, and consumption upgrading. The finding reveals that the digital economy in a city exerts a significant mitigating effect on carbon emissions in both local and neighboring cities. It is also observed that digital economy activities play a significant role in alleviating urban carbon emissions due to their capacity to stimulate green innovation and promote sustainable patterns of production and consumption. The robustness of these findings is assessed through the consideration of endogeneity and substitution of the explained and explanatory variables. This paper contributes to a systematic understanding of the linkage between the revolution of digitalization within economic systems and the transition towards sustainability within environmental systems. It also provides valuable insights to policymakers on strategies to promote and accelerate low-carbon development.
The extensive economic activities driven by the Industrial Revolution have approved to be the primary driving force for the global warming as they are the main contributing factors for the increased greenhouse gas emissions (Li and Lin, 2015; Liu and Bae, 2018; IPCC, 2021). Therefore, it is an urgent to accelerate the transition of human economic activities from a high-carbon to a low-carbon growth trajectory, which includes resource-efficient manufacturing, business innovation with a focus on sustainability, and the exploration of renewable energy sources (Bi et al., 2016; Geels et al., 2016; Velenturf and Purnell, 2021). With the onset of the Fourth Industrial Revolution, contemporary society is entering into a novel era propelled by diverse digitalization activities, such as artificial intelligence and cloud computing. The digitalization revolution has been extensively emphasized and recognized as a highly effective measure for mitigating carbon emissions (de Sousa Jabbour et al., 2018; Cheng et al., 2021; Elheddad et al., 2021; Lyu et al., 2023). It possesses the potential to transform the processes of production, consumption, and transportation within the entire economic system, which is widely acknowledged as the primary source of CO2 emissions. However, the opposing voices argue that the utilization of digital technologies not only fails to reduce carbon emissions but also leads to increased environmental pollution, electronic waste, and energy crises (Avom et al., 2020). This controversy gives rise to two research questions (RQ) that we aim to address. RQ.1 What is the real underlying correlation between the concurrent developments of digital economy and the low-carbon economy? RQ.2 How does the digital economy within a specific city affect local and adjacent low-carbon development? The investigation of these questions is advantageous for comprehending the intricate interconnections among the ongoing digitalization revolution in regional production, consumption, and energy systems, and regional transition toward low-carbon development. This holds particular significance for China, as its economy undergoes a pivotal shift from high-speed and rapid economic expansion to a focus on high-quality and sustainable development, spurred by the objectives of attaining carbon peak by 2030 and carbon neutrality by 2060.
The impact of digitalization transition on carbon dioxide emissions has garnered substantial attention in the literature, given the increasing integration of digital economy activities such as artificial intelligence, internet utilization, and online enterprises (Lange et al., 2020; Dong et al., 2022a; Wang et al., 2022). There are three points of views in the literature. The initial literature branch shows that the emergence of digital economy appears to exert a mitigating impact on carbon emissions through various mechanisms, including the reduction of innovation externalities and the enhancement of energy efficiency. According to Meng and Zhao (2022), the expansion of digital economy activities facilitates low-carbon development by improving energy efficiency and total factor productivity, leading to a subsequent decrease in carbon emissions. Similarly, Wang et al. (2022) contend that the reduction in carbon emissions can be ascribed to the rise in information and communication technology (ICT) penetration, facilitated through the modification and optimization of sectoral production structure. It is also found that the expansion of digital application is negatively correlated with energy intensity and carbon intensity, particularly when it comes to the Internet and online businesses, such as e-finance, e-commerce, and e-production (Asongu et al., 2018; Lange et al., 2020). The second branch of existing literature holds an opposite viewpoint arguing that the development of ICT may not always be environmentally-friendly but could result in a rise in carbon emissions (Usman et al., 2021). This is further supported by by Nguyen et al. (2020), who utilized data from Argentina, Canada, China, France, Germany, Italy, Japan, Mexico, Republic of Korea, Russian Federation, Turkey, United Kingdom, United States. Moyer and Hughes (2012) posited that the overall influence of ICT on the reduction of carbon emissions is constrained by the gradual decline in energy prices facilitated by the widespread application of ICT. This decline consequently fuels an escalation in individuals’ energy consumption, thereby resulting in increased CO2 emissions. The third branch of literature posits that the correlation between digital activities in an economic system and carbon emissions cannot be simply characterized as a binary relationship (i.e., positive or negative). Instead, it exhibits a complex pattern, resembling an inverted U-shaped or N-shaped curve. For example, Li et al. (2021a) undertook an empirical investigation employing global panel data encompassing 190 countries, in which they ascertained an inverse U-shaped correlation between the digitalization transition and carbon emissions. This finding is further corroborated by Li and Wang (2022), whereas Dong et al. (2022a) put forth the proposition that the advancement of digital economy has the potential to decrease carbon emission intensity; however, it may concurrently lead to an increase in per capita carbon emissions. It has also been observed that the development of digital economy probably results in consuming more energy and resources. For instance, enhanced accessibility to online shopping is likely to amplify household consumption as a whole. Moreover, the planned obsolescence of electronic devices may engender supplementary and heightened demands for factors such as labor, energy, and physical capital (Van den Bergh, 2011; Kostakis et al., 2016).
The aforementioned conclusion pertaining to the influence of digital economy activities on carbon emissions seems to be controversial. However, when considering specific conditions or contexts, it likely retains validity. This assertion can be supported by three fundamental reasons. The primary reason is rooted in the complex interplay between the revolution of digitalization and the pursuit of low-carbon development. This correlation is influenced by multiple factors that have been thoroughly investigated from diverse perspectives, encompassing technological innovation and energy conservation (Dong et al., 2022a; Ma et al., 2022; Lyu et al., 2023), as well as population density, industrial structure optimization and environmental regulations (Luan et al., 2022; Ma et al., 2023). The second reason is attributed to the divergence of methodologies employed in quantifying the digital economy within the existing literature. Some scholars treat the ICT as a key metric for assessing the digital economy (Lange et al., 2020), whereas others concentrate solely on the levels of Internet usage or online business (Li and Wang, 2022). Finally, it is important to acknowledge that the heterogeneity in sample selection may exert an influence on the empirical findings. For instance, Ulucak and Khan (2020) analyze data exclusively from the BRICs countries (i.e. Brazil, Russia, India, China, and South Africa), while Li et al. (2021a) conduct their study using global panel data encompassing 190 countries. The integration of these disparate viewpoints within a comprehensive framework capable of effectively capturing the intricate interplay between digital economy activities and the promotion of low-carbon development indeed poses a significant and daunting challenge.
Previous research has made significant contributions to our understanding of environmental effects of digital economy activities, yet there are still notable knowledge gaps that need to be addressed. On the one hand, although several studies have noted the carbon effect associated with the rise of digital economy activities, these studies are still in their infancy. Most of them only explore the impact from regional production system perspective, such as industry structure or energy structure, while the potential mechanism triggered by regional innovation and consumption systems remains underexplored. The digitalization revolution is altering the entire value chain system, whether in terms of innovation direction, production process, or consumption orientation. However, such mechanisms are not fully understood. On the other hand, the spatial spillover effect resulting from the development of digital economy activities has received limited attention, despite extensive discussions on the associated environmental externalities. In light of China’s development landscape, characterized by political centralization and economic decentralization, local governments often engage in strategic competitive endeavors aimed at fostering economic growth and implementing measures for environmental preservation (Xu, 2011; Wu et al., 2020). The manifestation of these effects is expected in both regional digital economy activities and environmental regulatory strategies.
In this paper, we attempt to empirically examine whether and how the rise of digital economy activities affects urban sustainability transition by utilizing panel data collected from 267 Chinese cities spanning the period from 2011 to 2018. First, we extend previous work by establishing a more comprehensive and systematic framework that documents triple trajectories of sustainability transition within the realm of digital economy, namely green innovation, production reshaping, and consumption upgrading. This exploration, to our best acknowledge, is the first step to integrate the mechanisms of green innovation as well as sustainable production and consumption in a holistic framework. Second, instead of relying on the general regression model, we adopt a spatial model to assess the environmental externalities associated with the development of digital economy. This approach offers a clearer understanding of the pivotal role played by digital economy activities in shaping regional or urban transition toward a low-carbon development. Third, we endeavor to establish an indicator that quantifies the degree of development in urban digital economy activities by integrating three fundamental dimensions, namely digital technology, digital infrastructure, and digital applications. This approach diverges from previous studies that solely relied on a single dimension, such as ICT or internet use, to capture the environmental impacts of digital economy activities.
In the era of digitalization, the development strategy oriented by digital transformation presents a novel approach to reconciling economic growth with environmental preservation. The implementation of digital technologies, such as the Internet of Things, artificial intelligence, and big data analytics, serves to fortify conventional production methods while concurrently diminishing their energy consumption. This digital shift also engenders novel sectors characterized by enhanced sustainability, including digital trade, digital finance, and digital delivery (Wu et al., 2021). Second, the current process of digital transformation is conducive to establishing an intellectualized environment governance system that can boost sustainable development. For example, both central or local governments can employ digital tools or platforms, such as digital environmental monitoring systems, intelligent energy tracking systems, and environment-focused big data analysis systems. This utilization aims to enhance ecological and environmental governance, conserve energy, and elevate information transparency, environmental accountability, and service coordination while minimizing expenses (Zampou and Katerina, 2011). Third, public environmental supervision seems to be more effective and efficient in the digital era. Many studies conducted by Jiang et al. (2021); Ren et al. (2021), and Huang et al. (2023) suggest that social media platforms such as Twitter and WeChat offer the general public a novel avenue to participate in activities pertaining to cleaner production and consumption. Based the aforementioned analysis, it can be inferred that the potential low-carbon effects arising from digital economy activities likely are more likely to manifest within the context of urban development. Hence, a basic hypothesis is proposed.
Hypothesis 1a: The development of digital economy in a city exhibits a mitigating effect on local carbon emissions.
Moreover, the digital economy serves as a novel paradigm of economic progress, where unique incentive policies, usage patterns, and development models are introduced, offering valuable lessons and opportunities for neighboring regions to assimilate and emulate. Particularly under the Chinese system of “benchmark competition” derived from decentralized decision-making, local governments often exhibit strategic interactions and imitation behaviors in the development of new economy activities, with a focus on GDP performance (Song et al., 2021; Hao et al., 2023). Consequently, it is probable that there exists a spatial correlation between digital economy activities and carbon emissions, giving rise to the following hypothesis.
Hypothesis 1b: The development of digital economy in a city exerts a dynamic spatial spillover effect that leads to a reduction in carbon emissions in neighboring cities.
Drawing upon the perspective of value chain system, we present a comprehensive analysis of the mechanisms through which the development of digital economy influences CO2 reduction, identifying three pathways: innovation transition, production reshaping, and consumption upgrading (see Figure 1). From the innovation transition perspective, the process of digitalizing has the potential to stimulate green technologies and inclusive innovation. Traditional industries are often associated with high levels of pollution as they face difficulties in obtaining funds and resources for green innovation and technology (De Vita et al., 2011). However, the process of digitalization possesses the capacity to enhance the efficiency of traditional production factors, such as land, labor, and capital. This transformation facilitates profound alterations in established production methods and lifestyles by making knowledge and technology more adaptable, portable, and environmentally sustainable (Murphree & Anderson, 2018; Banalieva & Dhanaraj, 2019; Ma et al., 2022). As a result, it catalyzes the development of green technologies. Liu et al. (2022) confirm that the ongoing digital revolution within the finance sector yields a favorable influence on corporate green innovation. Wu et al. (2021) contend that the progression of ICT facilitates the dissemination of energy-saving technological innovations. Green innovation is widely acknowledged as a pivotal catalyst for facilitating the transition of society from high-emission to low-emission practices. This ultimately leads to the hypothesis stated below.
Hypothesis 2a: The development of digital economy in a city has the potential to reduce urban carbon emissions through the channel of green innovation.
From the production reshaping perspective, the digital revolution leads to a transition in the production process, causing a shift from the traditional value chain of network, which is primarily oriented towards economic efficiency, to the current digital and sustainable manufacturing system. This shift offers an efficient solution for decreasing regional carbon emissions. On the one hand, digital technologies can effectively integrate horizontal and vertical production systems, unlocking environmentally sustainable manufacturing and paving the way towards a more sustainable industry system (de Sousa Jabbour et al., 2018). Ciliberto et al. (2021) argue that the implementation of Industry 4.0, which encompasses production systems, information technology, and big data analytics, exerts a positive impact on both sustainable production and circular economy capabilities. On the other hand, in the realm of digital production, it is possible to incorporate a distinctive electronic identification into products. This facilitates the gathering of data pertaining to product utilization throughout its entire carbon emissions cycle, thereby contributing to the mitigation of individual carbon footprints (Martínez et al., 2022). Hence, we posit the following hypothesis.
Hypothesis 2b: The development of digital economy in a city can lower urban carbon emissions through the channel of production reshaping.
From the consumption upgrading perspective, the digital economy is regarded to be a powerful driving force that can bring about a significant transformation in the public’s way of life. On one hand, the utilization of digital technologies, such as big data, cloud computing, and blockchain, has the potential to disrupt the prevailing information asymmetry between consumers and producers, thereby enabling consumers to make more informed and sustainable purchasing decisions. It is argued that online purchasing and home delivery are more environmentally friendly and cleaner than conventional business activities (Palsson et al., 2017). On the other hand, the digital platform, such as e-commerce, e-finance and e-delivery, provides an alternative low-carbon lifestyle and behavior for residents. The evidence estimated by Tehrani et al. (2009) shows that e-shopping can reduce air pollution by 39% and energy consumption by 71%, by substituting delivery vans for personal trips to the supermarket. Briefly, people’s consumption structure can be optimized in the digital age, thus the following hypothesis is proposed.
Hypothesis 2c: The development of digital economy in a city can decrease urban carbon emissions through the channel of consumption upgrading.
We build a comprehensive framework to summarize five hypotheses mentioned above (see Figure 1). The expansion of digital economy possesses the potential to propel the socioeconomic system towards a transition characterized by low-carbon principles. With regards to the innovation transition, the revolution of digitalization offers promising prospects for fostering green product design and green process innovation. Another transition occurring in the manufacturing module, such as an increase in digital production and robotics, reshapes the production mode and leads to the optimization of production structure. The digitalization revolution also has brought about the transformation of the consumption module and has given rise to new forms of consumption, such as green purchases and sustainable online services. Consequently, the consumption structure has been optimized and upgraded. In this case, we suggest that the transition of the entire value chain system, including three crucial and systematic components, i.e green innovation, production reshaping, and consumption upgrading, acts as a moderating role in how the expansion of digital economy activities influences low-carbon urban development.
In order to test Hypothesis 1a, we establish a regression which is based on the model developed by Dietz and Rosa (1994).
Where Carb is the level of carbon emissions; Dig denotes the level of digital economy; λ1 represents the coefficient of digital economy impacting on carbon emissions; X denotes a series of control variables which includes population, technology, GDP as well as FDI; δ1 denotes the coefficient of the controls; i and t denotes city and year, respectively; μi is the city-specific fixed effect, ηt is the time-specific fixed effect; it is the stochastic error term.
In order to test Hypothesis 1b, we develop Equation (1) into the spatial Durbin model (SDM). It can be explicitly formulated as follows:
where wij is spatial weight matrix. ρ denotes the spatial auto-regressive coefficient, when ρ≥0, it indicates that carbon emissions in a city has a positive spatial correlation. Similarly, θ represents the coefficient of spatial spillover effect of the explanatory variable, when θ≦0, it means that the development of digital economy in a city has a spillover effect on reducing adjacent city’s carbon emissions, meaning that Hypothesis 1b can be supported.
Two intermediary models are introduced to investigate the mechanisms through which the development of digital economy activities in a city influences urban carbon emissions.
where the item of M denotes the mechanism variable which has three options. The first mechanism, green innovation, is introduced to test Hypothesis 2a. In this circumstance, if the coefficient λ3 is positive in Equation 3 and the coefficient γ is negative in Equation 4, then Hypothesis 2a is supported. The second one represents production reshaping which is applied to test Hypothesis 2b, while consumption upgrading is chosen as the third mechanism to test Hypothesis 2c. Similarly, Hypothesis 2b and 2c would be supported only if the coefficients λ3 is positive and the coefficient γ is negative.
The dependent variable, carbon emissions, is quantified by the quantity of urban carbon emissions. The source of carbon emission data applied in this paper is derived from Chen et al. (2020). However, the data obtained from Chen’s study is at the county level, not at the city level. Therefore, we applied the method presented in previous studies (Dong et al., 2022b; Zeng et al., 2022) to transform the county-level carbon emission data into city-level data.
Prior studies usually employ a single indicator to measure the development of digital economy, such as internet penetration, ICT development, or digital trade (Salahuddin et al., 2016; Asongu et al., 2018; Li et al., 2021a). However, this method may overlook the broader societal implications associated with the development of various digital economy activities. In this case, we employ a comprehensive indicator to assess the independent variable. Our measurement of this variable relies on five distinct indicators, encompassing the levels of internet penetration, mobile phone penetration, employment in the internet-related sector, output of the internet-related sector, and the development of digital finance. The principal component analysis technique is used to process the multi-dimensional indication. We standardize the data first, then reduce it to a single dimension. Following that, a comprehensive index measuring the development of the digital economy at the city level is derived. This assessment differs from previous research in that it takes a complete approach that is specifically designed to capture the multiple characteristics of digital economy activities.
Three mechanism variables are introduced, i.e. green innovation, production reshaping, consumption upgrading. The mechanism of green innovation is quantified by the per capita number of urban green patents granted, while the evaluation of the mechanism of consumption upgrading is determined through the calculation of Engel’s coefficient, which is measured by the ratio of non-food expenditure to total expenditure. It is essential to note that the measurement of production reshaping is derived from an index of industrial structure rationalization, borrowed from Thayer’s coefficient.
The determinants of economic development, technology innovation and population outlined in the classical model are considered as three control variables in this paper. The control variable of economic development is measured by the per capita real GDP at the city level, where the GDP deflator is computed using 1978 as the base year. Technology innovation is measured by the per capita urban patents granted, while the control of population is measured by the quantity of urban population per unit of land area. According to the model generated by Dietz and Rosa (1994), economic development and population are expected to have a positive effect on carbon emissions, whereas technology innovation is expected to have a negative effect. Additionally, we also control the potential effect of foreign direct investment (FDI) on urban carbon emissions, which is measured by the quantity of foreign investment at the city level.
The carbon emission data is derived from Chen et al. (2020). It is important to acknowledge that, in contrast to conventional CO2 data obtained from energy consumption, Chen et al. (2020) creatively utilize the original DMSP/OLS and night light data as indicators of energy consumption. The utilization of satellite data is advocated for its wider coverage, higher precision, and superior fitting accuracy (Sun et al., 2020). Chen’s methodology has garnered broad acceptance in the literature (Dong et al., 2022a; Zeng et al., 2022). The data concerning internet penetration ratio, mobile phone penetration ratio, employment of internet related sector, output of Internet related sector, total employment, employment as well as output in different sector, GDP per capita, FDI, population size, non-food expenditure, total expenditure at the city level are obtained from the China City Statistical Yearbook (2012-2019). The data pertaining to the development of digital finance is sourced from the Digital Research Center of Peking University. The data concerning the number of patents granted and green patents granted is sourced from China National Intellectual Property Administration. Due to data availability, we select the panel data of 267 Chinese prefecture-level cities during the period from 2011 to 2018 as our research samples. Notably, few missing data in our sample is converted through the utilization of the moving average method. Table 1 shows the definition and descriptive statistics for the primary variables.
Figure 2 illustrates the temporal and spatial variations in the development of China’s digital economy at the city level. The map on the right exhibits a notably deeper shade in comparison to the left, demonstrating a significant overall increase in digital economy activities across both the eastern and western regions of China. However, the distribution of digital economy activities across regions is markedly uneven. On average, cities situated in China’s coastal regions exhibit a higher development level of digital economy activities compared to those in the inland regions. Notably, some cities, such as Chengdu, Xi’an, and Kunming in the inland region, still have relatively high level of the development of digital economy activities. In contrast, the development of digital economy in some coastal cities such as Huizhou, Suqian, and Nanping still falls behind. This implies that the spatial factor, which must not be overlooked, potentially plays a crucial role in shaping the advancement of digital economy activities within a given region. The map further reveals periodic darkening of the digital economy index, suggesting an upward trajectory in the growth of the digital economy.
Figure 3 depicts the geographical distribution of carbon emissions during two periods in China, i.e. from 2011 to 2014 and from 2015 to 2018. It shows that the carbon emissions, as a whole, trends to decrease from the East to West areas as well as from the North to South areas. Additionally, there is a slight decrease in carbon emissions from the period of 2011-2014 to 2015-2018, indicating that the carbon emissions has been somewhat controlled. However, it is worth noting that cities with the highest carbon emissions are still concentrated in specific areas, such as the Beijing-Tianjin-Hebei region, the Yangtze River Delta. Intuitionally, it can be observed that the dynamism of digital economy activities in China exhibits a roughly spatial correlation with the distribution of carbon emissions.
Table 2 presents the findings of the Moran’s index analysis conducted on carbon emissions and the index of digital economy development between 2011 and 2018. The results indicate a statistically significant positive relationship between all Moran’s indexes at the 1% significance level. This suggests a spatial connection between the development of digital economy activities and carbon emissions among neighboring cities. Furthermore, the analysis reveals an increasing trend in the Moran’s index value for digital economy development over time, while the Moran’s index value for carbon emissions exhibits a slight decline. In other words, the spatial correlation of digital economy development strengthens over time, whereas the correlation between carbon emissions and neighboring cities weakens. These findings basically align with the observations presented in Figures 2 and 3.
Table 3 reports the regression results of the development of digital economy in a city impacting on urban carbon emissions. Column (1) presents the fixed effect model, while the spatial Durbin models are shown in Column (2). It shows that the coefficient of digital economy, whether in the fixed effect model or spatial Durbin model, both exhibits a negative sign at the significance of 1%, indicating that digital economy activities in a city indeed exert a significant and negative impact on urban carbon emissions. This empirical result is supportive of Hypothesis 1a, meaning that carbon dioxide emissions in a city can be significantly reduced by developing urban digital economy. This finding confirms the significance of enhancing digital economy activities as a means to mitigate carbon emissions and attain a state of low-carbon development. This conclusion is also consistent with the evidences provided by Zhang et al. (2022) and Yi et al. (2022). The result from Column (2) indicates that the coefficient of spatial weighted Dig is significantly negative, meaning that carbon emissions in a city can be reduced by its adjacent cities’ digital economy development. To increase the consistence, we also conduct two regressions by substituting the SDM with the dynamic SDM (see Column 3 in Table 3) as well as by replacing the spatial distance weighted matrix with the economic matrix (see Column 4 and 5 in Table 3). The findings indicate that there indeed remains a notable spatial spillover regarding the correlation between the promotion of digital economy activities and the reduction of carbon emissions. This conclusion strongly supports the opinion illustrated in Hypothesis 1b.
Moreover, the results from Table 3 also show that the lagged and spatial lagged items of explained variable are both positive at the significance of 1%. It demonstrates that carbon emissions, on the one hand, has obvious “snowball” or path dependence effect, that is, a city would release more and more carbon dioxide as time goes by. On the other hand, it confirms the characteristics of “race to the bottom” occurring in the process of carbon emissions among neighbor cities. This conclusion is also confirmed by Li and Wang (2022) as well as by Zhang et al. (2022). It implies that the policy in a city focusing on reducing carbon emissions not only required to be enhanced and carried out continuously but also need to cooperate with the adjacent cities. The coefficient of the control of Technology turns out to be significantly negative at the 1% level in all models, indicating that technology innovation, expectedly, has a reducing effect on carbon emissions, whereas the coefficients of other controls of population, FDI, and GDP per capita lack of statistical significance.
There probably exists an endogenous problem within the linkage between the comprehensive development of digital economy and carbon emissions abatement. In this regard, we take three measures to solve potential endogeneity. First of all, the explanatory variable (Dig) is replaced by China’s Digital Financial Inclusion Index. It is believed that this index, to some extent, can reflect the development of digital economy activities (Elheddad et al., 2021; Yi et al., 2022). The results demonstrate a significant and negative correlation between the digital economy development and urban carbon emissions (see Column 1 to 3 in Table 4). Moreover, the coefficient of the weighted digital economy also exhibits a significant negative effect. It indeed supports hypotheses 1a and 1b.
Second, we apply another alternative measurement for the explained variable in the models (see Column 4 to 6 in Table 4), where the level of carbon emissions is replaced by the index of environment pollution which incorporates both urban wastewater discharge and sulfur dioxide emissions. The finding shows that the coefficients still exhibit similarities with the baseline estimations. This results reconfirm that the benchmark results are robust.
Lastly, the 2SLS (two stage least square) approach is used to figure out potential endogeneity problem. It is necessary to explain the way of how to choose instrumental variable (IV). The IV is ideally required to be related with the endogenous independent variable but unrelated with the dependent variable. According to previous studies (Huang et al., 2019; Wu et al., 2021; Yi et al., 2022), the number of historical posts and telecommunications in 1984 is proven to meet the requirements of IV. In this case, the number of post and telecommunication offices per 10,000 individuals in 1984 is employed as the first IV. The statistic value concerning the first stage regression and the F test (see Column 1 in Table 5) shows that this IV basically meet two requirements. The result of 2SLS approach reveals a notable and statistically significant negative coefficient for the digital economy index (see Column 2 in Table 5). Additionally, referring to the work provided by Zhang et al. (2022), we still select the geographic location as another IV, which is determined by calculating the distance between each prefecture-level city and its nearest coastal port based on their respective longitude and latitude coordinates. It is necessary to note that the distance, which remains unchanged over time, is added into a temporal dimension by multiplying it with the number of internet users for each respective year. The first stage regression in Column (3) of Table 5 displays that the endogenous independent variable (i.e. Dig) is strongly correlated with the IV. The F-statistic test retains a value exceeding 10 and retains statistical significance at the 1 percent level, thereby indicating that the second IV is less likely to be affected by the problem of endogeneity. The second stage regression demonstrates that the development of digital economy within a city can indeed diminish urban carbon emissions (see Column 4 in Table 5). In brief, the estimation results above are indeed robust and reliable.
Table 6 reports the findings regarding the mechanism of the development of digital economy activities associated with the abatement of urban carbon emissions. The process of mediating effect test follows the procedure illustrated in Equation (3) and (4). First, for checking the mechanism of green innovation, the coefficient of digital economy listed in Column (1) turns out to be positive and significant, that is, λ3 in Equation (3) is significantly positive, implying that the development of digital economy in a city is associated with urban green innovation. It also can be seen from Column (2) in Table 6 that the coefficient of the mechanism variable is significantly negative, that is, γ is significant, indicating that green innovation can significantly reduce urban carbon emissions. The results of Column (1) combined with Column (2) confirm that the green innovation transition indeed plays a mediating role in linking the digital economy with the abatement of urban carbon emissions. It means that Hypothesis 2a is supported.
Second, in order to test the mechanism of production reshaping, we report the mediating effect results in Column (3) and (4). The coefficient of digital economy appears to be both significant and positive, suggesting that the development of digital economy activities contributes to the transition of urban industries from heavily-polluting production to sustainable production. At the same time, such transition turns out to significantly reduce urban carbon emissions (see Column 4 in Table 6). It implies that the mechanism of production reshaping also plays a pivotal role in influencing the relationship between the rise of digital economy and the abatement of urban carbon emissions. Thus, hypothesis 2b is confirmed.
Third, we examine the mechanism of consumption upgrading by conducting two regressions according to Equation (3) and (4) in Table 6. In Column (5), the coefficient of digital economy exhibits a significantly positive sign, whereas in Column (6), both the coefficients of digital economy and mechanism variable display negative and significant effects. These findings strongly support hypothesis 2c, thereby confirming that the development of digital economy activities within an urban setting significantly contributes to the mitigation of urban carbon emissions through the channel of consumption upgrading.
Traditional manufacturing or service systems, whether for manufacturers or consumers, are generally characterized by high levels of energy consumption, high levels of pollution, and high carbon emissions (Li and Lin, 2015; Liu and Bae, 2018). However, with the advent of the digitalization revolution, a more sustainable consumption and production system is likely constructed, which is essential to reducing traditional environmental damage. Our empirical evidence supports the assertion that the expansion of digital economy activities in a city has a considerable and mitigating effect on urban low-carbon economy. This conclusion is also basically supported by previous studies (Wang et al., 2022; Yi et al., 2022; Zhang et al., 2022). However, earlier studies identify a range of impacting mechanisms, such as energy efficiency, environmental regulation, and technology innovation, that must borrow from various theories rather than a systematic framework. When various mechanisms are simultaneously applied to explain the connection between the digital economy and low-carbon development, it appears difficult to achieve a unanimous conclusion. Our work endeavors to ascertain a comprehensive mechanism that elucidates why the digital economy engenders a mitigating, rather than exacerbating, impact on carbon emissions. We argue that the rapid development of diverse digital economy activities in urban China is fundamentally transforming the entire value chain system. This transformation can be categorized into three interdependent and complementary systems: the innovation system, the production system, and the consumption system. Based on our evidence, it has been confirmed that the innovation system is undergoing a shift towards environmental friendliness in response to the digitalization revolution. Moreover, both the production and consumption systems are progressively adopting more sustainable practices. In this scenario, carbon emissions across the whole socioeconomic system in urban China have experienced a significant decrease. It further implies that the value creation system, in the digital age, is not only becoming increasingly intellectualized and digitalized, but also becoming more and more sustainable.
The impacting mechanism systematically identified from the perspective of value chain in our exploration offers a more comprehensive framework to explain the nexus between the ongoing digitalization revolution occurring in the socioeconomic system and the transition to sustainability in the eco-environmental system. The existing mechanisms documented in the literature can be largely generalized and encompassed within the scope of our framework. For example, the mechanisms, such as energy consumption and industrial structure, discussed by Zhang et al. (2022), can indeed be broadly generalized as sustainable production and consumption in our discussion. Similarly, the moderating role of investments in research and development, as identified by Ma et al. (2022), can be actually attributed to the transition of innovation system in response to the digitalization revolution. Abukhader (2008) proposes two concepts that precisely capture the nature of how e-commerce (a typical activity of digital economy) affects the environmental system. The first is eco-effectiveness, which means “doing the right things”, while the second is eco-efficiency, which means “doing things right”. By integrating these two complementary concepts, our framework proves highly beneficial in explaining why the digital economy is important for reducing carbon emissions. The process of digitalization in the entire value chain system involves a diverse range of complementary actors, including inventors, manufacturers, and consumers. These actors are collectively motivated by the principles of both eco-effectiveness and eco-efficiency. To be specific, digitalization facilitates inventors in designing environmentally friendly and low-carbon products rather than highly polluting ones. Additionally, it encourages consumers to choose low-carbon goods over energy-intensive alternatives. Similarly, the digitalization process in the production process helps manufacturers to increase eco-efficiency and decrease carbon emissions. In general, the digitalization revolution in urban China is undeniably driving its local economic system towards low-carbon development. Besides, it also generates a spillover effect that contributes to the decrease of carbon emissions in neighboring cities. This conclusion indicates that when a region strives for low-carbon development, it is vital not only to recognize digitalization as a driving force in reshaping local value chain system, but also to take into account the spillovers from neighboring regions.
Digitalization revolution and sustainability transition initially appear to be two distinct concepts, but they could be increasingly integrated and synergistic in pursuit of a shared objective (i.e. low-carbon development). This article tries to shed light on the linkage between the digitalization revolution and the transition to sustainability, adopting a perspective focusing on the transformative effects of digital economy across the entirety of value chain system. To this end, we construct theoretical and empirical models to comprehensively detect the mechanism by which the development of digital economy activities reduces urban carbon emissions, based on China’s prefecture-level city data from 2011 to 2018. The result shows that the development of digital economy can significantly reduce urban carbon emissions by the way of driving the entire value creation system transformation, including green innovation, production reshaping, and consumption upgrading. The result also confirms that the rise of digital economy has a significant spatial spillover effect on reducing urban carbon emissions. The findings persist as robust even when accounting for endogeneity, alternative measures of explanatory and explained variables. This paper provides a complementary perspective on the nature of assessing the impact of digital economy on carbon emissions abatement at the city level, contributing to a better understanding of the complex relationship between the digitalization revolution and sustainability transition.
The findings, in this paper, have important policy implications for China and other developing countries. First, our conclusion inspires that the value creation system can make progress towards more advanced and sustainable development by harnessing the potential of the digitalization wave. Compared with traditional environment regulation, it is necessary to pay attention to the type of digitalization for environmental governance. The policy focus should not merely support the digitalization infrastructure, ICT technology, artificial intelligence, or internet penetration. Instead, it should consider the economy system as a whole, undergoing a dual transformations of digitalization and sustainability. This is particularly important in China, where the consumer digital economy experiences fast growth while the industry digital economy lags behind. It is necessary to drive the innovation and production systems towards digitalization and low-carbonization with the help of the Industry 4.0. Second, since digitalization and carbon emissions both have spatial spillover effects, local governments that support the value chain system in the direction of digitalization and sustainability should coordinate with their neighboring regions. More important, the supporting policies from the central government which aims at boosting the growth of digital economy and the decrease of carbon emissions should not be limited to a few cities. Instead, they should focus on more typical cities across China. Third, due to the carbon lock-in effect in particular sectors and regions, policies for low-carbon development should be continuous and industry-specific. For instance, in the post-COVID-19 pandemic era, stimulating economic recovery for each country is crucial. However, such recovery and development must not come at the expense of environmental pollution.
Although the nexus between the digital economy and low-carbon urban development has been theoretically and empirically detected in our study, there may be still some limitations that require further attention. First, the paper sheds light on the aforementioned nexus by examining the transformation of the value chain system led by digitalization, but we have not explored the broader macro mechanisms including political, economic, and cultural activities, nor have we delved into the micro mechanisms that involve individual consciousness and its influence on individual behaviors. Future research should aim to build a more comprehensive framework to thoroughly explore the nexus between the digitalization trend and the transition to a low-carbon economy. Second, due to limitations in data availability, this paper does not undertake any heterogeneity tests. The question of whether the effect of digitalization on low-carbon urban development varies across different contexts remains uncertain. It is imperative to consider a more comprehensive analysis of heterogeneity in terms of various regions, digitalization activities, or firm characteristics in future studies. Third, it is worth to note that the dynamic relationship between digital economy activities and carbon emissions may vary at different stages of growth. Additional research can investigate the development phase when digital economy activities have a greater mitigating effect on carbon emissions, as well as the moment when they generate more spatial spillover effects to adjacent regions.
The original contributions presented in the study are included in the article/supplementary material. Further inquiries can be directed to the corresponding authors.
WY and XT: conceptualization, formal analysis, and writing–original draft, funding acquisition, resources, and supervision. NL and JC: investigation, data curation, project administration, software, and visualization. SZ: methodology, validation and writing–review and editing. All authors contributed to the article and approved the submitted version.
This work was supported by National Natural Science Foundation of China (Grant No. 72273031), Major Project Funding for social science research base in Fujian province social science planning (Grant No. FJ2022MJDZ013; FJ2020MJDZ018), and the China Scholarship Council (Grant No. 202006655010).
The authors declare that the research was conducted in the absence of any commercial or financial relationships that could be construed as a potential conflict of interest.
All claims expressed in this article are solely those of the authors and do not necessarily represent those of their affiliated organizations, or those of the publisher, the editors and the reviewers. Any product that may be evaluated in this article, or claim that may be made by its manufacturer, is not guaranteed or endorsed by the publisher.
Abukhader S. M. (2008). Eco-efficiency in the era of electronic commerce-should ‘Eco-Effectiveness’ approach be adopted? J. Cleaner Production 16 (7), 801–808. doi: 10.1016/j.jclepro.2007.04.001
Asongu S. A., Le Roux S., Biekpe N. (2018). Enhancing ICT for environmental sustainability in sub-Saharan Africa. Technological Forecasting Soc. Change 127 (2), 209–216. doi: 10.1016/j.techfore.2017.09.022
Avom D., Nkengfack H., Fotio H. K., Totouom A. (2020). ICT and environmental quality in Sub-Saharan Africa: Effects and transmission channels. Technological Forecasting Soc. Change 155 (6), 120028. doi: 10.1016/j.techfore.2020.120028
Banalieva E. R., Dhanaraj C. (2019). Internalization theory for the digital economy. J. Int. Business Stud. 50 (5), 1372–1387. doi: 10.1057/s41267-019-00243-7
Bi K., Huang P., Wang X. (2016). Innovation performance and influencing factors of low-carbon technological innovation under the global value chain: A case of Chinese manufacturing industry. Technological Forecasting Soc. Change 111, 275–284. doi: 10.1016/j.techfore.2016.07.024
Chen J., Gao M., Cheng S., Hou W., Song M., Liu X., et al. (2020). County-level CO2 emissions and sequestration in China during 1997-2017. Sci. Data 7 (1), 1–12. doi: 10.1038/s41597-020-00736-3
Cheng Y., Awan U., Ahmad S., Tan Z. (2021). How do technological innovation and fiscal decentralization affect the environment? A story of the fourth industrial revolution and sustainable growth. Technological Forecasting Soc. Change 162, 120398. doi: 10.1016/j.techfore.2020.120398
Ciliberto C., Szopik-Depczyńska K., Tarczyńska-Łuniewska M., Ruggieri A., Loppolo G. (2021). Enabling the Circular Economy transition: A sustainable lean manufacturing recipe for Industry 4.0. Business Strategy Environ. 30 (7), 3255–3272. doi: 10.1002/bse.2801
de Sousa Jabbour A. B. L., Jabbour C. J. C., Foropon C., Filho M. G. (2018). When titans meet–Can industry 4.0 revolutionise the environmentally-sustainable manufacturing wave? The role of critical success factors. Technological Forecasting Soc. Change 132, 18–25. doi: 10.1016/j.techfore.2018.01.017
De Vita G., Tekaya A., Wang C. L. (2011). The many faces of asset specificity: A critical review of key theoretical perspectives. Int. J. Manage. Rev. 13 (4), 329–348. doi: 10.1111/j.1468-2370.2010.00294.x
Dietz T., Rosa E. A. (1994). Rethinking the environmental impacts of population, affluence and technology. Hum. Ecol. Rev. 1 (2), 277–300. Available at: https://www.jstor.org/stable/24706840.
Dong F., Hu M., Gao Y., Liu Y., Zhu J., Pan Y. (2022a). How does digital economy affect carbon emissions? Evidence from global 60 countries. Sci. Total Environ. 852, 158401. doi: 10.1016/j.scitotenv.2022.158401
Dong Z., Xia C., Fang K., Zhang W. (2022b). Effect of the carbon emissions trading policy on the co-benefits of carbon emissions reduction and air pollution control. Energy Policy 165, 112998. doi: 10.1016/j.enpol.2022.112998
Elheddad M., Benjasak C., Deljavan R., Alharthi M., Almabrok J. M. (2021). The effect of the Fourth Industrial Revolution on the environment: The relationship between electronic finance and pollution in OECD countries. Technological Forecasting Soc. Change 163, 120485. doi: 10.1016/j.techfore.2020.120485
Geels F. W., Berkhout F., Van Vuuren D. P. (2016). Bridging analytical approaches for low-carbon transitions. Nat. Climate Change 6 (6), 576–583. doi: 10.1038/nclimate2980
Hao X., Li Y., Ren S., Wu H, Hao Yu. (2023). The role of digitalization on green economic growth: Does industrial structure optimization and green innovation matter? J. Environ. Manage. 325, 116504. doi: 10.1016/j.jenvman.2022.116504
Huang H., Long R., Chen H., Sun Q., Sun K., Ma W., et al. (2023). Do stakeholders have the same concerns about anti-food waste law in China? Using big data from social media. Environ. Impact Assess. Rev. 100, 107071. doi: 10.1016/j.eiar.2023.107071
Huang Q., Yu Y., Zhang S. (2019). Internet development and productivity growth in manufacturing industry: internal mechanism and China experiences. China Ind. Economics 36 (8), 5–23. doi: 10.19581/j.cnki.ciejournal.2019.08.001
IPCC (2021). “The intergovernmental panel on climate change (IPCC),” in Climate change 2021: the physical science basis (Cambridge, United Kingdom:Cambridge University Press).
Jiang P., Zhou J., Van Fan Y., Klemeš J. J., Zheng M., Varbanov P. S. (2021). Data analysis of resident engagement and sentiments in social media enables better household waste segregation and recycling. J. Cleaner Production 319, 128809. doi: 10.1016/j.jclepro.2021.128809
Kostakis V., Roos A., Bauwens M. (2016). Towards a political ecology of the digital economy: Socio-environmental implications of two competing value models. Environ. Innovation Societal Transitions 18 (2), 82–100. doi: 10.1016/j.eist.2015.08.002
Lange S., Pohl J., Santarius T. (2020). Digitalization and energy consumption. Does ICT reduce energy demand? Ecol. Economics 176, 106760. doi: 10.1016/j.ecolecon.2020.106760
Li K., Lin B. (2015). Impacts of urbanization and industrialization on energy consumption/CO2 emissions: does the level of development matter? Renewable Sustain. Energy Rev. 52 (8), 1107–1122. doi: 10.1016/j.rser.2015.07.185
Li X., Liu J., Ni P. (2021a). The Impact of the digital economy on CO2 emissions: a theoretical and empirical analysis. Sustainability 13 (13), 7267. doi: 10.3390/su13137267
Li Z., Wang J. (2022). The dynamic impact of digital economy on carbon emission reduction: evidence city-level empirical data in China. J. Cleaner Production 351, 131570. doi: 10.1016/j.jclepro.2022.131570
Liu X., Bae J. (2018). Urbanization and industrialization impact of CO2 emissions in China. J. Cleaner Production 172 (1), 178–186. doi: 10.1016/j.jclepro.2017.10.156
Liu J., Jiang Y., Gan S., He L., Zhang Q. (2022). Can digital finance promote corporate green innovation? Environ. Sci. Pollut. Res. 29 (24), 35828–35840. doi: 10.1007/s11356-022-18667-4
Luan F., Yang X., Chen Y., Regis P. J. (2022). Industrial robots and air environment: A moderated mediation model of population density and energy consumption. Sustain. Production Consumption 30 (3), 870–888. doi: 10.1016/j.spc.2022.01.015
Lyu Y., Zhang L., Wang D. (2023). Does digital economy development reduce carbon emission intensity? Front. Ecol. Evol. 11, 1176388.
Ma L., Hong Y., He S., Luo H., Liu G., Zheng J., et al. (2023). The influence of digital economy development on urban carbon emission intensity in the Yangtze River Economic Belt: Mediating mechanism and spatial effect. Front. Ecol. Evol. 11, 1148505. doi: 10.3389/fevo.2023.1148505
Ma Q., Tariq M., Mahmood H., Zeeshan K. (2022). The nexus between digital economy and carbon dioxide emissions in China: The moderating role of investments in research and development. Technol. Soc. 68, 101910. doi: 10.1016/j.techsoc.2022.101910
Martínez J. M. G., Puertas R., Martín J. M. M., Domingo R. (2022). Digitalization, innovation and environmental policies aimed at achieving sustainable production. Sustain. Production Consumption 32 (2), 92–100. doi: 10.1016/j.spc.2022.03.035
Meng F., Zhao Y. (2022). How does digital economy affect green total factor productivity at the industry level in China: From a perspective of global value chain. Environ. Sci. pollut. Res. 29 (52), 79497–79515. doi: 10.1007/s11356-022-21434-0
Moran P. A. P. (1950). Notes on continuous stochastic phenomena. Biometrika 37 (1/2), 17–23. doi: 10.1093/biomet/37.1-2.17
Moyer J. D., Hughes B. B. (2012). ICTs: do they contribute to increased carbon emissions? Technological Forecasting Soc. Change 79 (5), 919–931.
Murphree M., Anderson J. A. (2018). Countering overseas power in global value chains: Information asymmetries and subcontracting in the plastics industry. J. Int. Manage. 24 (2), 123–136. doi: 10.1016/j.intman.2017.09.007
Nguyen T. T., Pham T. A. T., Tram H. T. X. (2020). Role of information and communication technologies and innovation in driving carbon emissions and economic growth in selected G-20 countries. J. Environ. Manage. 261, 110162. doi: 10.1016/j.jenvman.2020.110162
Palsson H., Pettersson F., Hiselius L. W. (2017). Energy consumption in e-commerce versus conventional trade channels-Insights into packaging, the last mile, unsold products and product returns. J. Cleaner Production 164 (10), 765–778. doi: 10.1016/j.jclepro.2017.06.242
Ren S., Hao Y., Xu L., Wu H., Ba N. (2021). Digitalization and energy: How does internet development affect China's energy consumption? Energy Economics 98, 105220. doi: 10.1016/j.eneco.2021.105220
Salahuddin M., Alam K., Ozturk I. (2016). The effects of Internet usage and economic growth on CO2 emissions in OECD countries: A panel investigation. Renewable Sustain. Energy Rev. 62, 1226–1235. doi: 10.1016/j.rser.2016.04.018
Song Y., Zhang X., Zhang M. (2021). The influence of environmental regulation on industrial structure upgrading: Based on the strategic interaction behavior of environmental regulation among local governments. Technological Forecasting Soc. Change 170, 120930. doi: 10.1016/j.techfore.2021.120930
Sun Y., Zheng S., Wu Y., Schlink U., Singh R. P. (2020). Spatiotemporal variations of city-level carbon emissions in China during 2000–2017 using nighttime light data. Remote Sens. 12 (18), 2916. doi: 10.3390/rs12182916
Tehrani S. M., Karbassi A. R., Ghoddosi J., Monavari S. M. (2009). Prediction of energy consumption and urban air pollution reduction in e-shopping adoption. J. Food Agric. Environ. 7 (3-4), 898–903. Available at: https://www.researchgate.net/publication/233794816.
Ulucak R., Khan S. U. D. (2020). Does information and communication technology affect CO2 mitigation under the pathway of sustainable development during the mode of globalization? Sustain. Dev. 28 (4), 857–867. doi: 10.1002/sd.2041
Usman A., Ozturk I., Ullah S., Hassan A. (2021). Does ICT have symmetric or asymmetric effects on CO2 emissions? Evidence from selected Asian economies. Technol. Soc. 67, 101692. doi: 10.1016/j.techsoc.2021.101692
Van den Bergh J. C. J. M. (2011). Energy conservation more effective with rebound policy. Environ. resource economics 48 (1), 43–58. doi: 10.1007/s10640-010-9396-z
Velenturf A. P. M., Purnell P. (2021). Principles for a sustainable circular economy. Sustain. Production Consumption 27, 1437–1457. doi: 10.1016/j.spc.2021.02.018
Wang J., Dong X., Dong K. (2022). How digital industries affect China's carbon emissions? Analysis of the direct and indirect structural effects. Technol. Soc. 68, 101911. doi: 10.1016/j.techsoc.2022.101911
Wu H., Hao Y., Ren S., Yang X., Xie G. (2021). Does internet development improve green total factor energy efficiency? Evidence from China. Energy Policy 153, 112247. doi: 10.1016/j.enpol.2021.112247
Wu H., Li Y., Hao Y., Ren S., Zhang P. (2020). Environmental decentralization, local government competition, and regional green development: Evidence from China. Sci. Total Environ. 708, 135085. doi: 10.1016/j.scitotenv.2019.135085
Xu C. (2011). The fundamental institutions of China's reforms and development. J. Economic Literature 49 (4), 1076–1151. doi: 10.1257/jel.49.4.1076
Yi M., Liu Y., Sheng M. S., Wen L. (2022). Effects of digital economy on carbon emission reduction: New evidence from China. Energy Policy 171, 113271. doi: 10.1016/j.enpol.2022.113271
Zampou E., Pramatari K. (2011). “An approach to the assessment of the environmental impact of e-government services,” in ECIS 2011 Proceedings. 213. Available at: https://aisel.aisnet.org/ecis2011/213.
Zeng P., Wei X., Duan Z. (2022). Coupling and coordination analysis in urban agglomerations of China: Urbanization and ecological security perspectives. J. Cleaner Production 365, 132730. doi: 10.1016/j.jclepro.2022.132730
Keywords: digital economy, carbon emissions, sustainable production, sustainable consumption, low-carbon development, urban transition
Citation: Yu W, Lan N, Tan X, Zhang S and Chen J (2023) Does the digital economy drive low-carbon urban development? The role of transition to sustainability. Front. Ecol. Evol. 11:1248515. doi: 10.3389/fevo.2023.1248515
Received: 27 June 2023; Accepted: 20 July 2023;
Published: 03 August 2023.
Edited by:
Guochang Fang, Nanjing University of Finance and Economics, ChinaCopyright © 2023 Yu, Lan, Tan, Zhang and Chen. This is an open-access article distributed under the terms of the Creative Commons Attribution License (CC BY). The use, distribution or reproduction in other forums is permitted, provided the original author(s) and the copyright owner(s) are credited and that the original publication in this journal is cited, in accordance with accepted academic practice. No use, distribution or reproduction is permitted which does not comply with these terms.
*Correspondence: Xiaolan Tan, dGFueGlhb2xhbkBoaHUuZWR1LmNu; Shanshan Zhang, emhhbmdzc0B6anV0LmVkdS5jbg==
Disclaimer: All claims expressed in this article are solely those of the authors and do not necessarily represent those of their affiliated organizations, or those of the publisher, the editors and the reviewers. Any product that may be evaluated in this article or claim that may be made by its manufacturer is not guaranteed or endorsed by the publisher.
Research integrity at Frontiers
Learn more about the work of our research integrity team to safeguard the quality of each article we publish.