- 1UMR 8067 BOREA (MNHN, CNRS, IRD, SU, UCN, UA), Laboratoire de Biologie des Organismes et Ecosystèmes Aquatiques, Paris, France
- 2MNHN, Station Marine de Dinard, CRESCO, Dinard, France
- 3School of Life Sciences, University of Liverpool, Liverpool, United Kingdom
- 4UMR DECOD (Ecosystem Dynamics and Sustainability), INRAE, Institut Agro, IFREMER, Rennes, France
- 5MIAME, Gestion des migrateurs amphihalins dans leur environnement, OFB, INRAE, Institut Agro, Université Pau et Pays de l’Adour, Rennes, France
- 6Centre d’expertise et données sur le patrimoine naturel, PatriNat OFB-MNHN-CNRS IRD, Station marine de Dinard, Dinard, France
- 7CGEL (EPHE-PSL), Dinard, France
- 8Université de Rennes, Rennes, France
Competition arises when species share a limited resource, but this can be avoided through niche partitioning. Despite the large body of literature on diadromous fishes, very few studies have focused on niche partitioning when competing for resources. Diadromous fishes are suffering a global decline throughout their range in part due to their peculiar life history traits as they migrate from the sea to freshwater to spawn or the reverse. They are particularly sensitive to river fragmentation induced by barriers. Dams for instance are expected to alter the spatial distribution and resource exploitation of diadromous fishes as well as other organisms. Here, we studied the ecological niche of six taxa of diadromous fishes, temporally co-occurring in the same land–sea continuum obstructed by two river dams. We used Bayesian mixing models run on C and N isotopes to infer the various habitats used and the origin of the trophic carbon in muscle and scale tissues of diadromous fishes. Results showed that the sub-adults of Anguilla anguilla, juveniles of Petromyzon marinus, and Salmo trutta exploit mainly the freshwater, or marine part for adults of Lampetra fluviatilis, whereas juveniles of Platichthys flesus and Mugilidae use all the habitats of the land–sea continuum. In the freshwater habitat, the microphytobenthos and biofilm are the main sources of carbon used, while in the marine habitat, the basal carbon sources are more diverse. The analyses of niche overlaps between diadromous fishes demonstrate that the widest isotopic niches, observed for A. anguilla and P. flesus, also have more impact within the community. Results are discussed in terms of intra- and interspecific competition between these diadromous fishes and their generalist/specialist strategies in an obstructed catchment.
1 Introduction
Species, populations, or individuals that co-exist locally potentially compete with each other for resources, notably when limited (Hutchinson, 1957; Chase, 2011). In this sense, resources can include any ecological features and their interactions such as diet and habitat (Chase, 2011). One way to limit intra- and interspecific competition is niche partitioning (Chase, 2011). Niche partitioning can occur spatially and/or temporally, following the variability of resource distribution through space and time. Specialization/generalization strategies theoretically respond to several environmental forces, including population size (Sargent and Otto, 2006, reviewed in Sexton et al., 2017), resource abundance, diversity, and accessibility (MacArthur and Pianka, 1966; Charnov, 1976; Svanbäck and Bolnick, 2007). In the context of interspecific competition and considering population size, models predict that specialization is expected to be promoted in locally rare populations/species, while generalization will be favoured when population/species are locally abundant (Sargent and Otto, 2006, reviewed in Sexton et al., 2017). Specialists are also expected to maximize their fitness in one environment, while generalist fitness will be maximized in multiple ones (Sexton et al., 2017). According to the niche theory, the smaller the local community (individuals and species), the greater the community will be impacted by stochastic ecological processes (Chase, 2003; Fukami, 2004). This is the case for instance when the type and abundance of preys vary in heterogeneous environments. In an environment where resources are patchily distributed, the optimal foraging theory predicts that the niche width of a consumer depends on habitat and prey availabilities as well as their distribution (MacArthur and Pianka, 1966; Charnov, 1976; Svanbäck and Bolnick, 2007). The range of preys is expected to be greater in generalist consumers than in specialist consumers. Therefore, determining niche width can reveal spatial and temporal patterns in resource use and interspecific resource competition (Bolnick et al., 2011), which is particularly important for species conservation. This conceptual framework is particularly relevant for diadromous species sharing common life history traits and resources, whose populations have declined worldwide for many reasons including the fragmentation of freshwater habitats (e.g., Costa-Dias et al., 2009; Lassalle et al., 2009; Duarte et al., 2021; Waldman and Quinn, 2022).
The diadromous fishes have peculiar life history traits as they migrate from the sea to freshwater to spawn (anadromous) or the reverse (catadromous) (Myers, 1949; Harden-Jones, 1968). Many temperate watersheds host multiple diadromous species with varied life histories both within and between species. In France, the potential richness in diadromous taxa is higher in the largest rivers, with, for instance, a maximum of seven taxa observed for the French Atlantic and the English Channel River systems (Seine, Loire, Adour, Dordogne, and Garonne) (Merg et al., 2020). They all share a life history that makes them particularly sensitive to river fragmentation induced by the construction of dams, weirs, and sluices (Pringle et al., 2000; Limburg and Waldman, 2009; Waldman and Quinn, 2022). In Europe, there are more than one million river barriers (Belletti et al., 2020). For diadromous fish, river barriers hamper connectivity and can lead to the confinement of populations and accumulation of individuals down to the barriers (Katano et al., 2006; Drouineau et al., 2018; Forget et al., 2018; Trancart et al., 2020; Verhelst et al., 2021; Teichert et al., 2022a). Over-density can increase intra- and interspecific competition, which in turn can hamper species diversity and individual growth, survival, and/or reproduction (Laffaille et al., 2003; Machut et al., 2007; Costa et al., 2008). Dams have been shown to decrease diadromous species diversity (Merg et al., 2020) and alter growth and resident/migrant strategies in the white-spotted char (Salvelinus leucomaenis) (Morita et al., 2000). It may also bias the sex determination of eels (Laffaille et al., 2006; Costa et al., 2008). Despite the large body of literature on diadromous fishes, very few studies have focused on sympatric diadromous fishes and their potential competition in a catchment obstructed by dams (but see Katano et al., 2006). Indeed, an accumulation of the different life stages of diadromous fish downstream to the dams is likely to enhance intra- and interspecific competition for food and habitats and promote generalization for abundant species and specialization for rare ones.
A diadromous niche can be studied through stable isotope analysis, as such analysis provides quantitative measures of both resource and habitat, generally represented in a multivariate space. This is particularly useful in the context of resource partitioning among sympatric species (Newsome et al., 2007); it informs on spatial and temporal variations of resources and consumers (Hobson, 1999). Due to isotope fractionation between prey and consumer, N stable isotope ratios (δ15N) of fish inform on their trophic position, while C stable isotope ratios (δ13C) indicate which type of C fuels their food chains, primary producers such as plant or algae, or decomposers. Both C and N stable isotope ratios are commonly used in combination to depict energy sources and transfers within food webs (e.g., Kostecki et al., 2010; Kostecki et al., 2012; Teichert et al., 2022a; Teichert et al., 2022b). In aquatic systems, δ13C indirectly informs on salinity variations, with marine ecosystems being 13C enriched compared to freshwater ones (Peterson and Fry, 1987; Hobson, 1999; Herzka, 2005; Reis-Santos et al., 2015). Thus, a diadromous fish entering a river after a significant feeding period in the ocean has the typical 13C enriched signature of the marine environment (Hobson, 1999). Comparatively, freshwater river resident fish can display a large range of 13C-depleted values compared to marine fish, depending on the origin of items they feed on, from the riparian ecosystem (leaf litter, terrestrial plants, or invertebrates) to aquatic primary producers (e.g., algae, plants, and microphytobenthos), invertebrates or fish. When studying the isotopic niche of a consumer, one can sample all the preys and alimentary items and then run an isotopic analysis to decipher which preys and items are more specifically consumed (e.g., Kostecki et al., 2010). This is nevertheless subjected to individual variations in life stage, size, and sexes and also to previously encountered habitats for migrating individuals (Saboret and Ingram, 2019). Therefore, instead of using the exact prey and alimentary items consumed by fishes, one can focus on the basal carbon and nitrogen sources of the isotopic ratio of the consumer (e.g., Lafage et al., 2021). This approach is particularly adapted to diadromous fish species with complex life histories, as they use different trophic strategies and habitats in terms of salinity.
In this study, we analysed the interspecific competition through niche partitioning for trophic and habitat resources between diadromous species in the sea–estuary–freshwater continuum on the Sélune River (Normandy, France). This continuum is obstructed by two large electric dams, which reduce the watercourse accessibility of diadromous fish to 12 km in the downstream part of the river. We hypothesize that an accumulation of different life stages of diadromous fish below a dam is likely to enhance intra- and interspecific competition for food and habitats, increasing niche overlaps and promoting generalization for abundant species and specialization for rare ones. We measured stable C and N isotope values of basal sources (particular organic matter, microphytobenthos and biofilm, and plants) along the continuum and compared the values with those of juveniles to adults of European eels (Anguilla anguilla), salmonids (Salmo salar and Salmo trutta), lampreys (Lampetra fluviatilis and Petromyzon marinus), European flounders (Platichthys flesus), and Mugilidae caught in the same study site. We ran Bayesian simulations (mixing models and ellipse areas) on isotopic data to infer feeding habitat, trophic position, niche width, and overlaps between the species by life stages.
2 Material and methods
2.1 Study site and sampled diadromous species
The Sélune River is 91 km long from its source to the Mont- Saint-Michel Bay (Normandy, France). Two large hydropower dams, La Roche-qui-Boit (height = 16 m) and Vezins (36 m), were constructed in the early 20th century, constraining the diadromous fish community to use the downstream most 12 km of the river (Figure 1). This community is composed of catadromous species (i.e., reproduction at sea), which grow in the estuary and/or the river, such as the European eel (A. anguilla), a complex of species belonging to the Mugilidae family (Chelon ramada, Chelon auratus, and Chelon labrosus). The European flounder (P. flesus), a facultative catadromous species (some individuals reproduce in brackish or freshwater areas), is found in the downstream part of the river and the estuary. Finally, anadromous species (i.e., reproduction in the river) are also observed below the dams: two species of lamprey (L. fluviatilis and P. marinus) and two species of salmonids (S. salar and S. trutta, the latter being facultative anadromous). These seven diadromous species use the Mont-Saint-Michel Bay, the estuary, and the Sélune River as adults, sub-adults, or juveniles throughout the year (Table 1). Elvers of A. anguilla arrive from March to May and then grow for several years until metamorphosing in the silver stage (Acou et al., 2005; Teichert et al., 2022a). Departure for migration of silver eels occurs from August to December. Adults of lamprey P. marinus and L. fluviatilis enter the river in winter and spring to spawn, while the juveniles grow in the river for several years before moving to sea from early fall to early spring. Adults of S. salar and S. trutta arrive from spring to autumn, enter the river, and reproduce in December and January, and then juveniles stay 1 or 2 years before migrating to the ocean as smolts. Juveniles of P. flesus and Mugilidae also grow in the Sélune River after their respective arrival from June to August and April, May, and July (Table 1). Sporadically, shads (Alosa alosa and Alosa fallax, not included in this study) can also be observed in the Sélune River.
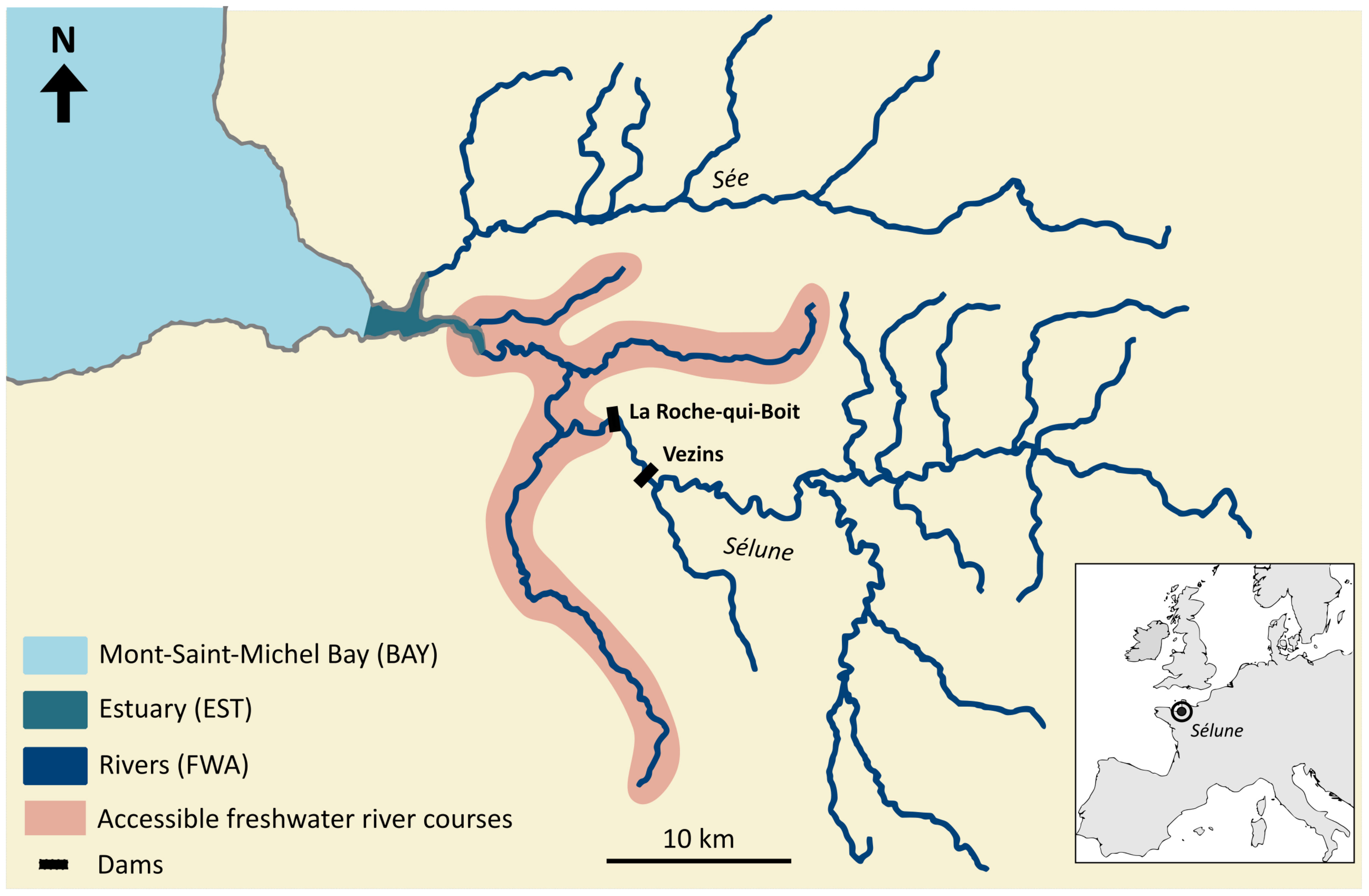
Figure 1 Map of the Mont-Saint-Michel Bay (BAY), estuary (EST), and rivers of the Sélune and surrounding ones (FWA). Dams of La Roche-qui-Boit and Vezins are represented as black rectangles. The accessible freshwater river courses are depicted in red.
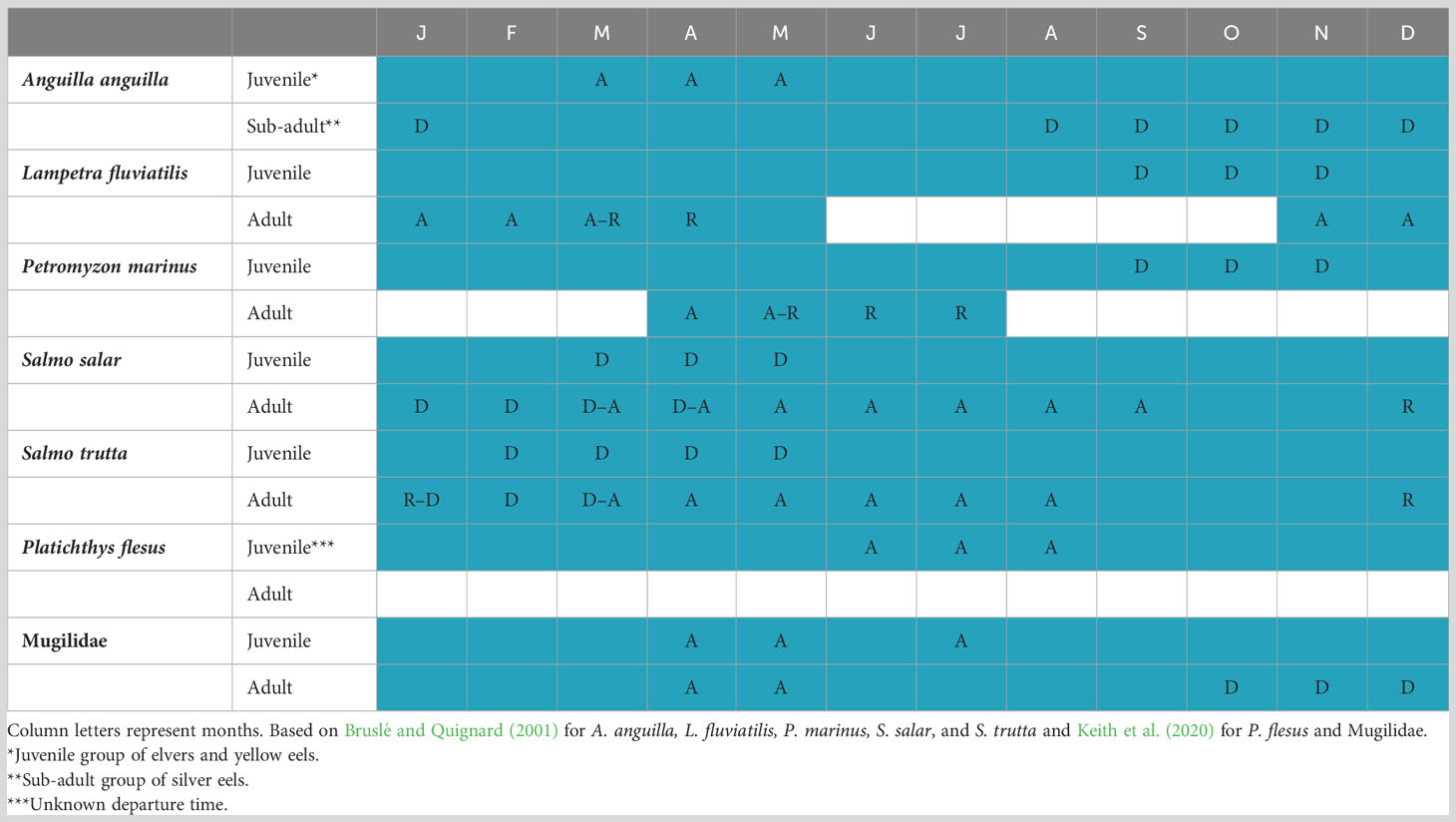
Table 1 Diadromous species temporal presence (in blue) in the Sélune River with months of arrival (A), reproduction (R), and departure from (D).
2.2 Sampling and sample preparation
Samples were collected by INRAE (UMR DECOD) and MNHN (Marine Station of Dinard) between 2009 and 2020. Such a large temporal scale lessens the risk of a bias coming from a single specific year or season and allows for depicting a broad view of the isotopic niche of species and their interactions. All samples originated from the Sélune River watershed and Mont-Saint-Michel Bay, downstream to the lowest dam in the river continuum to the sea (i.e., La Roche-qui-Boit) (Figure 1 and see Appendix A for locations). The use of already collected tissues aimed at avoiding an additional sampling on natural populations, in accordance with the 3 R principle (replacement, reduction, and refinement) in animal studies (Russell and Burch, 1959). For adult S. salar, scales collected from 2000 to 2014 were used on fish that stayed one winter at sea (1SW, n = 69, size range 450–700 mm) and two winters at sea (2SW, n = 75, size range 700–850 mm) while returning to the Sélune River to spawn. As they do not feed on the studied catchment, they were not included in the models. Muscle samples of juveniles of S. trutta (n = 38, size range 60–80 mm), P. marinus (n = 4, size range 130–150 mm), P. flesus (n = 115, size range 20–205 mm), Mugilidae (undetermined genus and species, n = 15, size range 20–90 mm), sub-adults of A. anguilla (n = 90, size range 510–860 mm), and adults of L. fluviatilis (n = 7, size range 250–350 mm) were obtained. Three basal sources were collected from the Mont-Saint-Michel Bay (BAY), the estuary (EST), and the freshwater part of the Sélune River (FWA) downstream of the dam of La Roche-qui-Boit (Table S1, Appendix A) (Figure 1). Photosynthetic organisms (PLA) consisted of aquatic vascular plants in FWA, halophytes in EST, and algae in BAY; they were collected by hand and carefully cleaned out to remove invertebrates. Particulate organic matter (POM) came from 1 l of water filtered on decarbonated (4 h at 550°C) GF-F filters (47 mm diameter, Whatman) at the laboratory. Freshwater samples of POM were also taken upstream to the dams, as they are susceptible to influencing the trophic network downstream (Figure 1; Appendix A). Microphytobenthos and photosynthetic biofilm (MPBB) were sampled in the marine and freshwater environments, respectively (downstream to the lowest dam). The microphytobenthos were extracted from the superficial layer (5 mm) of scraped muddy sediment by exposing them to light for 2 h. The sediment was then covered with a 100-μm nylon filter and sand previously sieved (63 and 250 μm) and decarbonated (5 h, 550°C). After waiting several hours for the microphytobenthos to migrate to the surface through the nylon filter, the superficial layer (2 mm) was scraped and sieved through seawater on a 45-μm filter. The content of the filter was then filtered again under decarbonated GF-F filters (4 h, 550°C). In the river, biofilms were collected on the bottom substratum. Large stones (10 to 20 cm) were picked up, and the biofilm was retrieved by gently brushing until a concentrated solution in a 5-ml vial before filtration on decarbonated GF-F filters (4 h, 550°C) at the laboratory. All samples were kept in a cooler at the field before being frozen (except for the GF-F filters), lyophilized, and ground into a fine powder. Samples were weighed to the nearest 0.01 mg into tin cups in anticipation of stable isotope analyses.
2.3 Stable isotope analyses
C and N isotope analyses were performed at the Stable Isotopes in Nature Laboratory (Fredericton, New Brunswick, Canada) using Carlo Erba NC2500 elemental analyser and a Finnigan Mat Delta XP IRMS. The carbon and nitrogen isotope ratios were expressed in the delta notation δ13C and δ15N, where δX = [(RSample/RReference) − 1] × 1000, where X = δ13C or δ15N, and R is the ratio 13C:12C or 15N:14N in the sample and the reference material. Results are referred to Vienna Peedee Belemnite Carbonate (VPDB) for C and to atmospheric nitrogen for N and expressed in units of ‰ ± standard deviation (sd). Data were corrected using working standards (bass muscle, bovine liver, nicotinamide, and acetanilide) that were previously calibrated against International Atomic Energy Agency (IAEA) standards CH6, CH7, N1, and N2. Repeated analyses of IAEA standards and working standards showed that maximum standard deviations for δ13C and δ15N values were 0.26‰ and 0.37‰, respectively. Standard deviations of samples were analysed in duplicate (n = 10) and averaged 0.11‰ and 0.19‰ for δ13C and δ15N, respectively; single measurements were then carried out on all other samples. The analytical precision (standard deviation for repeated measurements of internal standards) was ±0.2‰ and ±0.3‰ for δ13C or δ15N, respectively.
2.4 Statistical analyses
C:N ratios were checked to identify possible bias in δ13C values due to high lipid content in the tissue (Post et al., 2007). When C:N ≥ 3.5, δ13C values were corrected following Post et al. (2007). Similarly, δ13C values obtained from scale tissues were corrected for high concentrations of glycine and proline according to Satterfield and Finey (2001). Analyses of isotopic data obtained for basal sources and diadromous fish were performed in R 4.2.1 (R Core Team, 2022) using Bayesian mixing models and analytical tool suite proposed by MixSIAR 3.1.12 (Su and Yajima, 2012; Stock and Semmens, 2016; Stock et al., 2018). δ13C and δ15N values were used to estimate the contributions of basal sources (PLA, MPBB, and POM) to diadromous species for habitats (FWA, EST, and BAY) whose contribution was above the 0.30 threshold. An exception was made for P. marinus for which the small sample size did not allow to have more than three basal sources for the model to converge. For this species, basal sources were studied only in FWA, as this habitat is more likely used (Quintella et al., 2003). For each diadromous species, models were set up with the following characteristics: three chains with 300,000 iterations and a burn-in of 200,000 with a thin of 100 to allow for adequate model convergence. Trophic enrichment factor values were derived from Kostecki et al. (2012) and Selleslagh et al. (2015). Values for aquatic habitat (FWA, EST, and BAY) and carbon origin (POM, MPBB, and plants for FWA and BAY) models were 2‰ ± 0.1‰ and 5.8‰ ± 0.1‰ for δ13C and δ15N, respectively. All MixSIAR models were run with residual and process errors set as TRUE. Model convergence was checked using Gelman–Rubin and Geweke’s diagnostics. Isotopic niche widths (δ13C and δ15N) of each diadromous species and overlaps were analysed using the R package, SIBER 2.1.7 (Jackson et al., 2011; Rossman et al., 2016). Posterior ellipsoids were calculated after 2 × 104 iterations, a burn-in of 1 × 103, over two chains; Bayesian standard ellipse areas (SEA_B) were calculated, and the 95% predicted Bayesian confidence interval (SEA_B CI95) was used to estimate the proportion of overlapping between species. Details of all the R script, raw data, and model results used in this study are available in Appendix B.
3 Results
3.1 Species position along the river–sea isotopic continuum
The trophic position of diadromous species/life stage and basal sources are based on their mean values of δ13C and δ15N, which were added to trophic enrichment factors for diadromous species. Figure 2 shows the overall organization of the food web of the different species making up the diadromous community. In particular, we see their relative position and the relative influence of basal sources according to their origin: freshwater, estuarine, and marine habitats (Figure 2). From the position of each diadromous species within the trophic network (Figure 2) and the results of the mixing models (Figure 3), we can define the relative contributions of basal sources (MPBB, POM, and PLA) according to habitats (FWA, EST, and BAY).
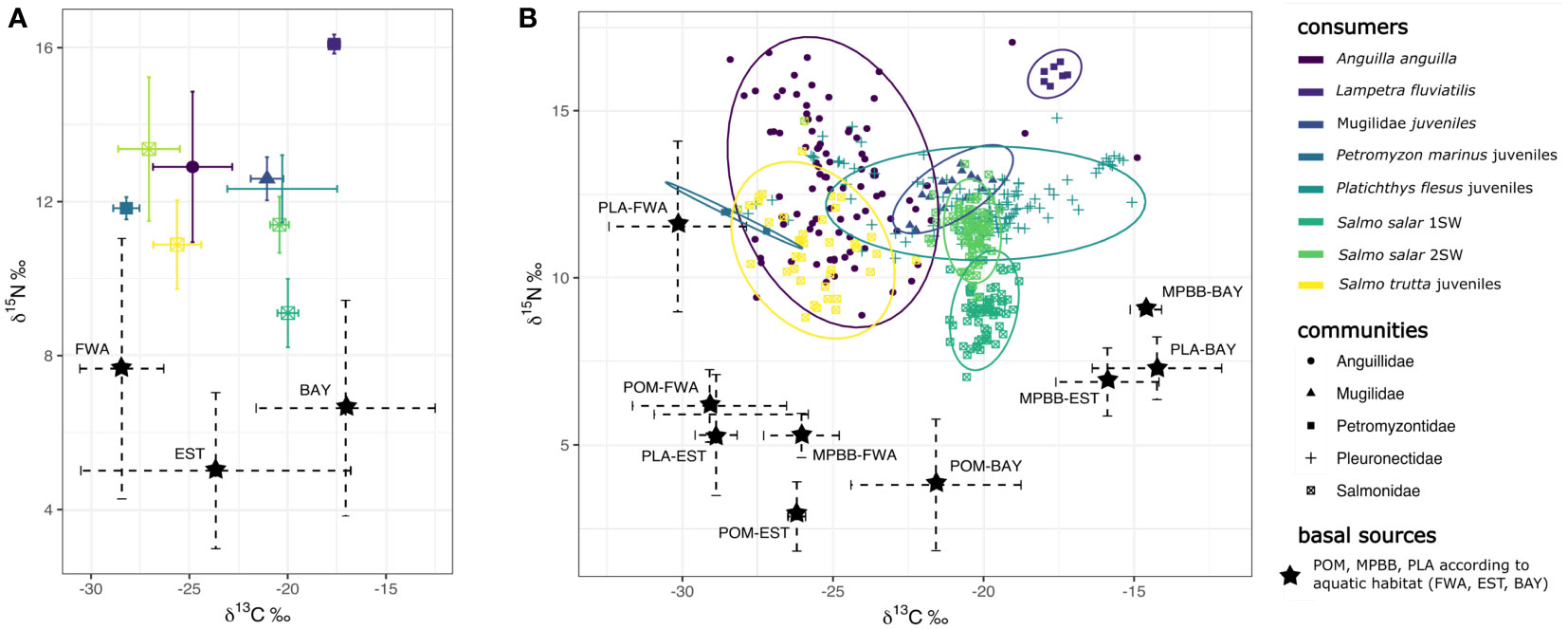
Figure 2 (A) Biplot of the means and standard deviations of δ13C (x-axis) and δ15N values (y-axis) of basal sources and diadromous studied along the Mont- Saint-Michel Bay to Sélune River continuum. (B) Biplot of the means and standard deviations of δ13C (x-axis) and δ15N values (y-axis) of basal sources and δ13C and δ15N values and their 95% ellipses for each of the diadromous studied. Basal sources are displayed according to aquatic habitat (estuary (EST), freshwater (FWA), and marine (BAY)) and sample type (particular organic matter (POM), microphytobenthos-biofilm (MPBB), and aquatic plants (PLA)). Different colours represent the various diadromous species (family for Mugilidae) studied, which include sub-adults of Anguilla anguilla, adults of Lampetra fluviatilis and Salmo salar 1SW and 2SW, and juveniles of Mugilidae, Petromyzon marinus, Platichthys flesus, and Salmo trutta. The same shapes correspond to diadromous belonging to the same family. S. salar 1SW and 2SW spend respectively one and two winters at sea to grow before migrating to the Sélune for reproduction.
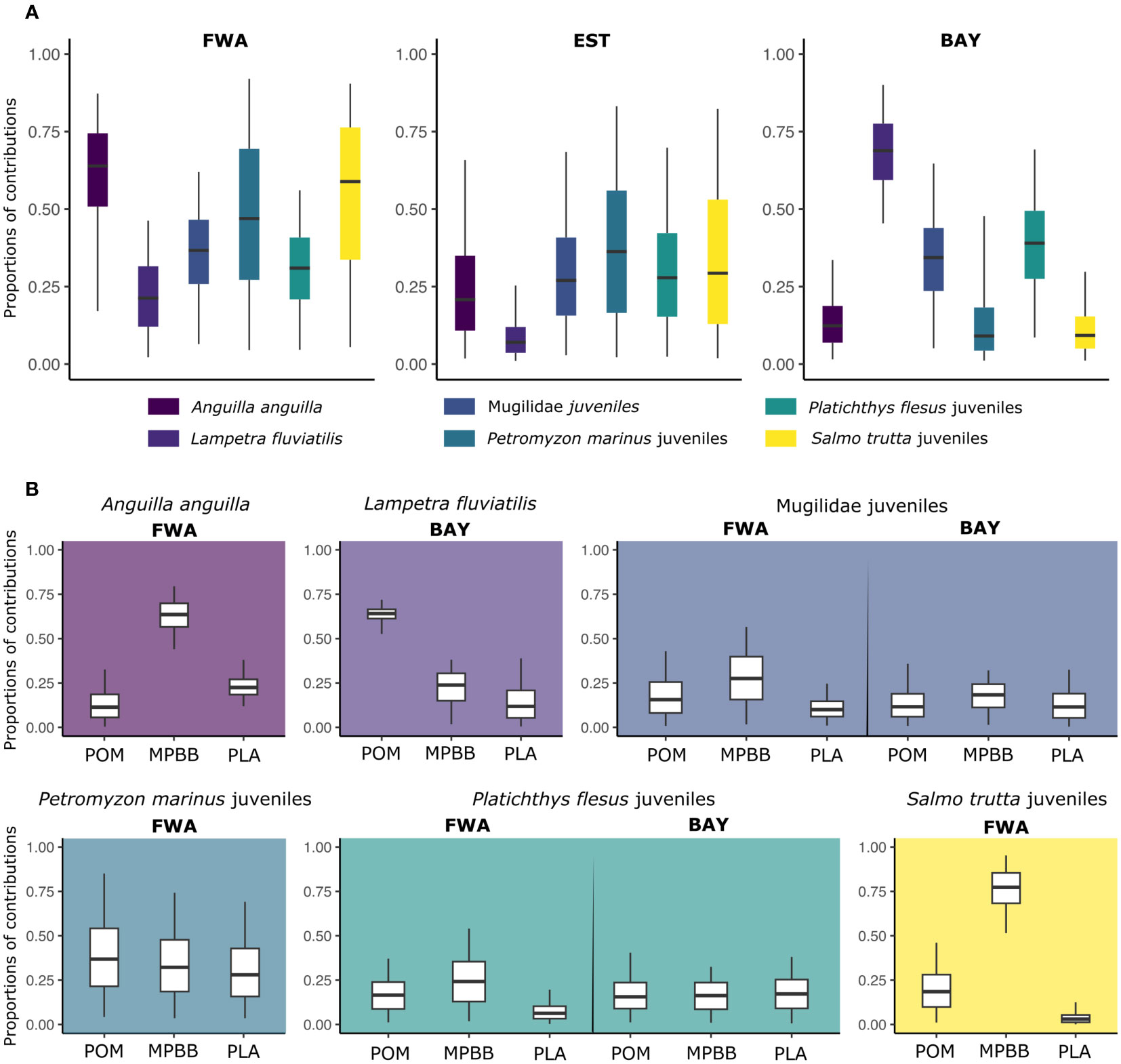
Figure 3 Boxplots of median contributions (with 50% and 95% Bayesian confidence intervals) of each basal source to diadromous fish consumers. (A) Contribution of each habitat type (freshwater (FWA), estuary (EST), and marine (BAY)). (B) Contribution of each sample type (particular organic matter (POM), microphytobenthos and biofilm (MPBB), and aquatic plants (PLA)) within the most dominant habitat type. Between three and six basal sources and a maximum of two different habitats were included in each model to maximize model convergence. A summary of the contribution proportions is presented in Table S2.
FWA basal source was the major contributor to the isotopic signatures of sub-adult A. anguilla and juveniles of P. marinus and S. trutta (Figures 2, 3A, Table S2). MPBB were the most likely basal source for sub-adult A. anguilla and juvenile S. trutta, while POM and MPBB were for juvenile P. marinus Figure 3B). Conversely, equal average contributions of FWA, EST, and BAY were found for juveniles of P. flesus and Mugilidae, suggesting that individuals could exploit trophic resources from different habitats along the land–sea continuum (Figures 2, 3A, Table S2). Juveniles of these species found their carbon origin mainly on MPBB in FWA and BAY, except for P. flesus in BAY, where individuals relied equally on all three basal sources (Figure 3B). Adults of L. fluviatilis had more typical marine signatures (Figures 2, 3A, Table S2), with marine POM being their most likely basal source (Figure 3B). Adults S. salar also had typical marine signatures, with higher δ15N values for 2SW compared to 1SW fish (Figure 2). Mixing models using basal sources from the three habitats were not run for S. salar since the species do not feed when migrating back to freshwater.
3.2 Isotopic niches of diadromous fishes
Isotopic niche widths of diadromous species varied between species and life stages. Mean SEA_B [CI95] was maximum for A. anguilla (12.314 [9.853–15.039]) and then decreased for P. flesus juveniles (7.675 [6.251–9.225]), S. trutta juveniles (4.250 [3.063–5.869]), Mugilidae juveniles (1.037 [0.614–1.793]), P. marinus juveniles (0.334 [0.104–1.211]), and L. fluviatilis (0.203 [0.094–0.480]) (Figure 4, Table S3). Correction for sample size applied to SEA_B did not significantly alter the niche widths of diadromous species, but marginally for P. marinus (SEA_B = 0.334, SEAc = 0.086) (Table S3).
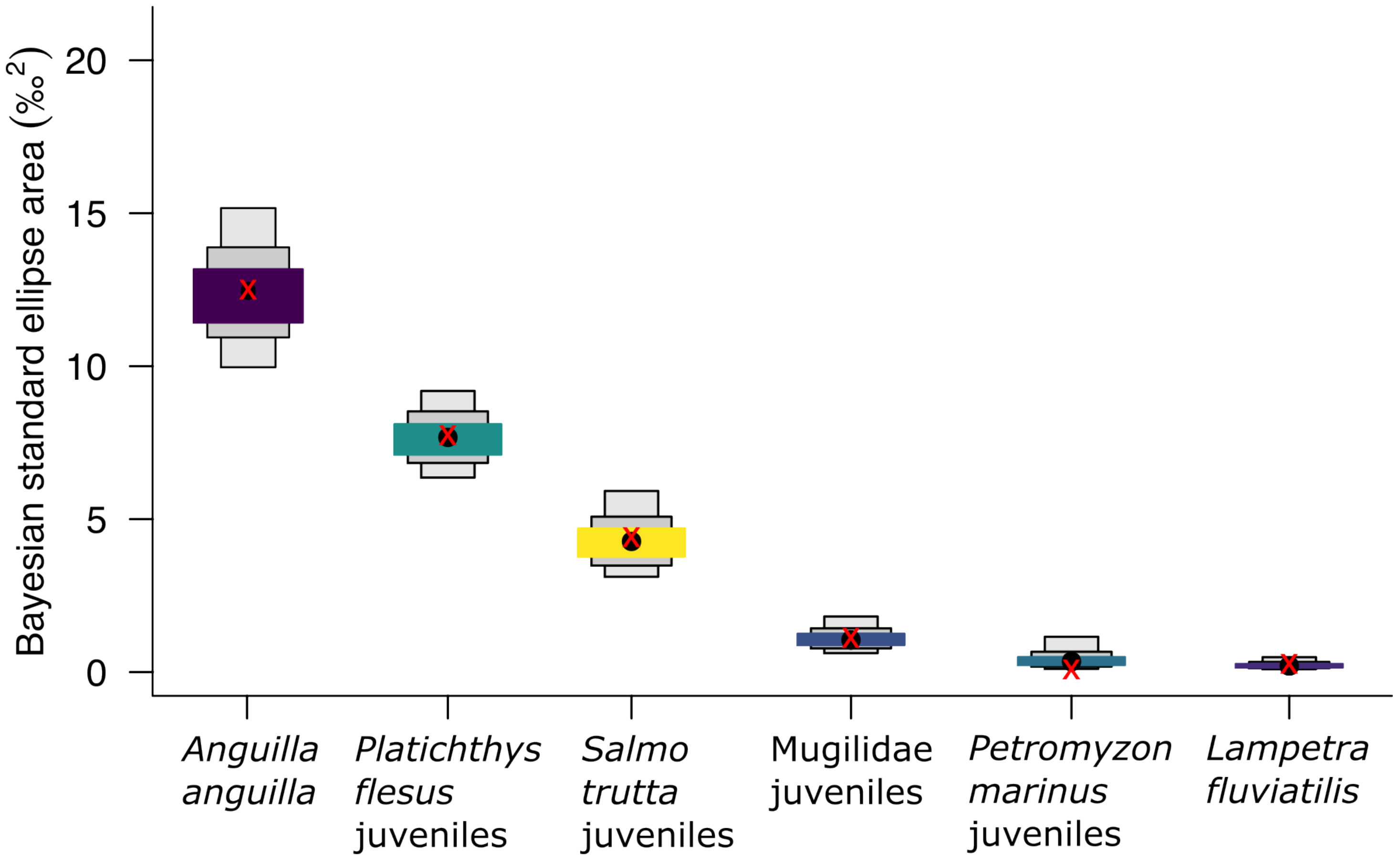
Figure 4 Diadromous isotopic niche widths as Bayesian standard ellipse areas (SEA_B) for δ13C and δ15N of diadromous species. Box width and colour denote credible intervals (colours: 50%, medium; grey, 75%, narrow; light grey, 95%); black dots, median; red crosses, predicted standard ellipse area of 95% (2.5% to 97.5%) corrected by sample size (SEAc). See also Tables S3 and S4.
The isotopic niche of A. anguilla, which is the widest niche observed in this dataset (Figure 4, Table S4), overlapped with several other diadromous species as juveniles, such as P. flesus, S. trutta, P. marinus, and Mugilidae (Figure 5, Table S5). Juveniles of P. flesus, the second widest isotopic niche observed in this dataset, overlapped with sub-adults of A. anguilla and juveniles of S. trutta and Mugilidae (Figure 5, Table S5). The isotopic niche of S. trutta as juveniles overlapped with sub-adults of A. anguilla and juveniles of P. flesus and P. marinus. The isotopic niche of juveniles of Mugilidae overlapped with sub-adults of A. anguilla and juveniles of P. flesus.
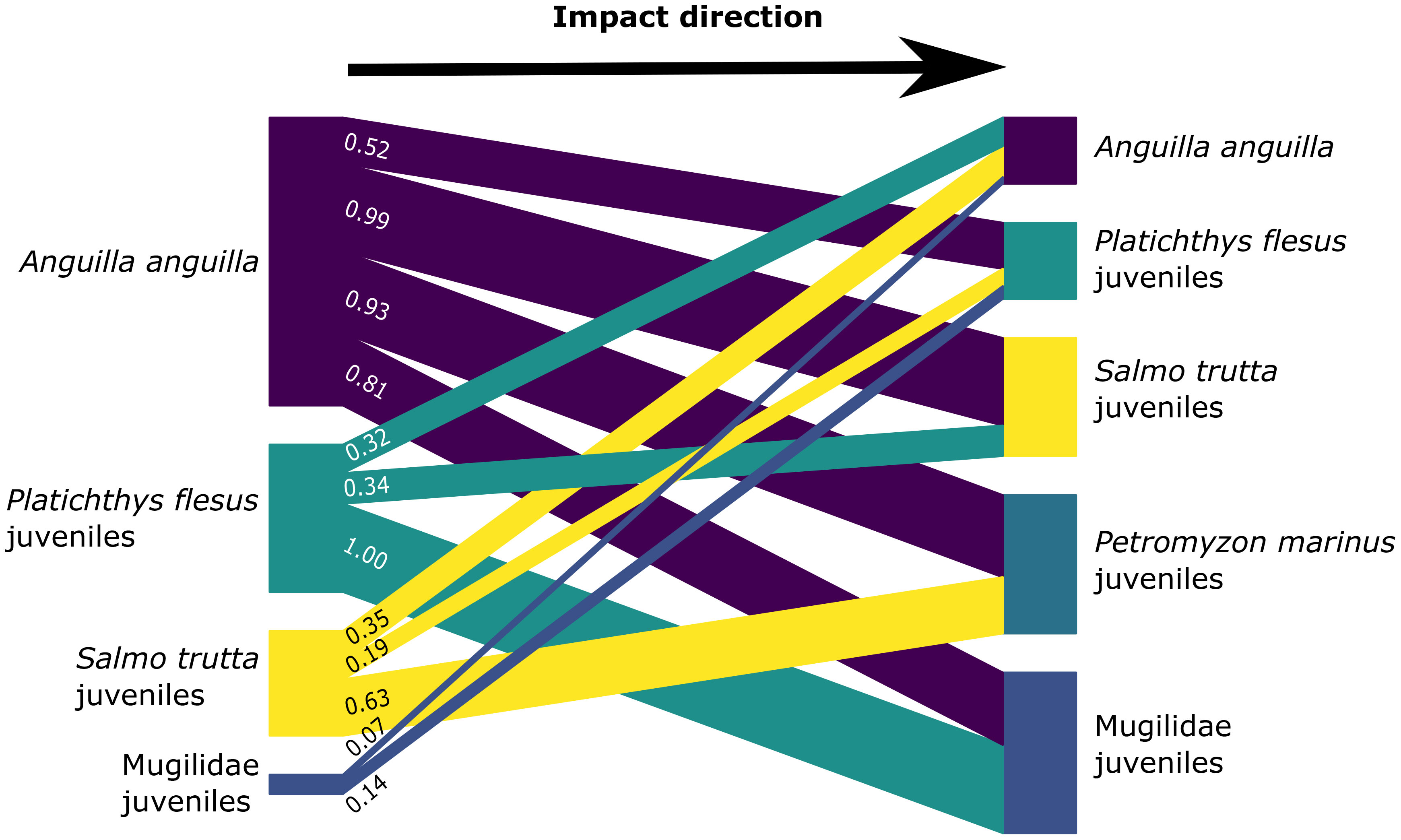
Figure 5 Bipartite graph of the overlap impacts of diadromous species/life stage (left group) on the isotopic niche width of others (right group). Niche width is retrieved as Bayesian standard ellipse areas (SEA_B) for δ13C and δ15N of diadromous species. The width of the bars between the left and right groups represents the proportion (see numbers) of impact from the left diadromous species/life stage on the right ones, calculated as the overlap between the two species divided by the niche width of the right one. Petromyzon marinus is absent from the left group because its impact on the right diadromous species was below 0.05 or null. Lampetra fluviatilis is absent from the left diadromous species for the same reason, and in the right group because it is not impacted. See also Table S4.
The isotopic niches of A. anguilla and juveniles of P. flesus overlapped more with other diadromous niches than they were impacted. Indeed, their niche width impact is greater on the left panels compared to the right panels (Figure 5, Table S4). On the contrary, the isotopic niches of juveniles of S. trutta, Mugilidae, and P. marinus were more impacted by other diadromous niches that they overlapped with (Figure 5, Table S4). The isotopic niche of adult L. fluviatilis, characterized by a typical marine signature, did not overlap with other diadromous species niches and was not impacted by them [not represented in Figure 5, as overlap values were below 0.05 (Table S5)].
4 Discussion
The various diadromous species studied here are all simultaneously present in the Sélune River where they are confined to the 12-km downstream reach below the dam and estuary. Although they do not arrive, colonize, and reproduce at the same time, they all co-occur at some point for their development, growth, or migration for reproduction. The Sélune River and estuarine system is thus a peculiar aquatic ecosystem, where several diadromous species reside for some times: one is critically endangered (A. anguilla), another is vulnerable (S. salar), and others are least concern (S. trutta, P. flesus, Mugilidae, P. marinus, and L. fluviatilis), as classified by the International Union for Conservation of Nature (IUCN) red list of threatened species in Europe (IUCN, 2023). This study provides the first isotopic analysis by means of basal carbon sources to infer the trophic ecology and habitat use of several diadromous species living in the same river. Basal carbon sources are not directly assimilated by the diadromous species studied here, but they are transferred along the food chains from primary consumers to fish. Focusing on the basal carbon sources instead of the diet allowed us to infer and compare the niche partitioning of these species, although they feed on different sources of prey.
Results confirm that sub-adults of A. anguilla (Cucherousset et al., 2011), juveniles of P. marinus (Quintella et al., 2003), and S. trutta (Michel and Oberdorff, 1995) exploit mainly the freshwater, or the marine part for adults of L. fluviatilis (Kelly and King, 2001), while some others, such as juveniles of P. flesus and Mugilidae (Lafage et al., 2021; Teichert et al., 2022b), use all habitats of the land–sea continuum. Several diadromous adults do not feed when they migrate in the Sélune aquatic system. This is the case of P. marinus and L. fluviatilis, which feed as external parasites of marine fishes and cetaceans, but also of S. salar 1SW and 2SW, spending respectively 1 and 2 years at sea to grow before migrating in the Sélune for reproduction. S. salar 1SW and 2SW were not included in the Bayesian models because they do not feed near the environment of the Sélune aquatic system (Keith et al., 2020), as opposed to L. fluviatilis, whose hosts are thought to live in marine habitats close to the Sélune River (Elliott et al., 2021). Indeed, S. salar 1SW feed and grow in Greenland seas, and S. salar 2SW in North Ireland and England seas (MacKenzie et al., 2012). In the same way, A. anguilla fast on their spawning migration from freshwater to the Sargasso Sea (Wright et al., 2022). However, the δ13C and δ15N isotopic signatures remain stable for approximately 1 month in their muscles (Buchheister and Latour, 2010) and therefore reflect the eel diet in the freshwater growth habitat they came from.
Niche partitioning between these diadromous fishes also occurs through the distinct use of basal carbon sources, but this depends on the habitat used. Indeed, sub-adults of A. anguilla and juveniles of S. trutta, P. flesus, and Mugilidae all use MPBB as their primary source of carbon in freshwater. In this habitat, juveniles of P. marinus equally relied on MPBB and POM as basal sources of carbon, but the small sample size for this species did not allow us to clearly infer their basal carbon source. In the marine habitat, diadromous basal carbon sources are more diverse, ranging from POM for adult L. fluviatilis, to POM, MPBB, and PLA for juveniles of P. flesus and Mugilidae even though MPBB is the main source of basal carbon for the later. Competition over basal carbon sources is thus more likely in the freshwater part of the land–sea continuum, and from our results, we identified three factors that could exacerbate this competition. Firstly, we found that diadromous species in the Sélune River watershed and Mont-Saint-Michel Bay depend more on the freshwater than on the marine habitats, and secondly, they all rely on the same basal carbon source (i.e., MPBB) in the freshwater habitat. Thirdly, these diadromous species, exploiting the freshwater habitat of the land–sea continuum, are physically coerced by the dams, particularly species that would normally colonize upper parts of rivers (i.e., A. anguilla, L. fluviatilis, P. marinus, S. salar, and S. trutta). These factors certainly play a role in increasing both intra- and inter-species competition between these diadromous when co-occurring in the same river. By contrast, we found that juveniles of P. flesus and Mugilidae exploit both the marine and freshwater parts of the land–sea continuum of the Sélune River watershed and Mont-Saint-Michel Bay, and they developed different strategies to exploit different sources of basal carbon. This could be a strategy to relax the likely competition both within and between these species. Niche partitioning through space is widely described in the literature, and strategies to minimize competition take place not only between but also within species (Sexton et al., 2017).
Nonetheless, the wider picture of potential competition within and between these diadromous fishes is not easily retrieved from their use of habitats (freshwater, estuarine, and marine) and carbon sources (POM, MPBB, and plants). Niche overlap assessment is thus essential to estimate the competition between diadromous species. Our results showed that niche overlaps occurred between diadromous, despite the use of indirect sources of carbon (we concentrated on basal primary producers as basal sources). This means that any basal source compartment alteration could impact diadromous fish and possibly the competition between each other. Some diadromous species such as A. anguilla (sub-adults) and P. flesus (juveniles) overlapped other diadromous species’ niches, more than they were overlapped by P. marinus and Mugilidae, both as juveniles. This is mainly because they have a wider niche than the impacted species. The dominance of A. anguilla in this system, in terms of both niche use and width, is congruent with other studies (Cucherousset et al., 2011; Denis et al., 2022) and explains why this euryecious and opportunistic species was classified, in France until 1984, as a pest in salmonid producing rivers before their decline (CSP, 1998). In A. anguilla, some individuals are more specialized in fish and others in invertebrates (Cucherousset et al., 2011). This translates not only in morphological differences but also in distinct habitat niche use in a river, with individuals of the former being located further from the riverbank (Cucherousset et al., 2011). A similar pattern of specialization is observed for P. flesus where some individuals exploit different habitats of the land–sea continuum (Teichert et al., 2022b) and different sources of basal carbon, albeit no morphological differences have been described in this species. Globally at the scale of the studied area, A. anguilla and P. flesus appeared to be more generalist than other diadromous species, as individuals strategically exploit different sources of carbon and/or habitats within the same watershed. The other diadromous fishes; juveniles of S. trutta, P. marinus, and Mugilidae; and adults of L. fluviatilis appeared more specialized in their habitat use and basal source of carbon.
Intraspecific competition is one of the main drivers of inter-individual variation (individual specialization; Bolnick et al., 2003), with theory predicting an increase in phenotypic variation leading to population niche expansion as competition increases (Van Valen, 1965; Bolnick, 2001; Svanbäck and Bolnick, 2005; Sexton et al., 2017). Phenotypic variation in the head morphology of A. anguilla has been demonstrated according to their specialization degree in fishes or invertebrates (Cucherousset et al., 2011). This suggests that A. anguilla presents some level of intraspecific competition in the Sélune watershed, as demonstrated by their wide niche. This idea is reinforced by the presence of dams obstructing the Sélune watershed over more than a century; the La-Roche-qui-Boit and Vezins dams were built in 1914 and 1927, respectively. The concentration of different diadromous species on an obstructed catchment is expected to lead to co-occurrence of species that do not co-occur in an open watershed and vice versa because of alteration/delay of the species-specific patterns of migration. Patterns of migration depend on the local environmental conditions experienced by individuals, and intraspecific competition will decrease the availability of individual resources. This will in turn increase interspecific competition over the same resources exploited by different species.
In the presence of interspecific competition, specialization and therefore niche width reduction are favoured when species are locally rare, while generalization is favoured when species are locally abundant (relative to other species) (Sargent and Otto, 2006; Sexton et al., 2017). How niches are partitioned, how intraspecific and interspecific competitions impact them, and whether abundant and rare species differ in their niche width are critical questions, as these will determine their ability to cope with changes (global change and/or anthropogenic pressures) (Colles et al., 2009; Sexton et al., 2017). There are concerns and signals of the global decline of specialist species (Clavel et al., 2011), and some generalist species such as A. anguilla are already critically endangered. In the Sélune River, dam removal is expected to relax the likely competition within and between diadromous species as densities downstream of the dams will decrease, rendering novel habitats upstream of the dams accessible (Merg et al., 2020). This may increase the niche width of diadromous species relaxing their local densities and potentially altering their generalist/specialist feeding strategy. Thus, restoration actions of the watersheds used by diadromous fish, such as dam removal, improved connectivity, and promotion of habitat diversity, are evidently needed for their conservation.
Data availability statement
The original contributions presented in the study are included in the article/Supplementary Material. Further inquiries can be directed to the corresponding authors.
Ethics statement
Ethical approval was not required for the study involving animals in accordance with the local legislation and institutional requirements because tissue samples were retrieved from collections.
Author contributions
AL: conceptualization, data curation, validation, formal analysis, methodology, and writing—original draft, review and editing. AC: conceptualization, methodology, funding acquisition, supervision, and writing—review and editing. J-MR: conceptualization, validation, formal analysis, methodology, and writing—review and editing. NT: methodology, formal analysis, and writing—review and editing. EF: conceptualization, funding acquisition, and writing—review and editing. AA: conceptualization and writing—review and editing. All authors contributed to the article and approved the submitted version.
Funding
This work was funded by the Atlantic Area INTERREG Project DiadES (https://diades.eu/) to EF, AC, and AL. The funding body played no role in the design of the study and collection, analysis, interpretation of data, and writing of the manuscript.
Acknowledgments
The authors would like to thank the UMR DECOD (INRAE) for providing some samples used in this study. These samples were collected and analysed (stable isotope analyses) as part of the Atlantic Area INTERREG Project DiadES (https://diades.eu/) and of the Sélune River restoration programme (https://programme-selune.com), which is supported by funding from the Seine-Normandy Water Agency (AESN) and the French Biodiversity Agency (OFB).
Conflict of interest
The authors declare that the research was conducted in the absence of any commercial or financial relationships that could be construed as a potential conflict of interest.
Publisher’s note
All claims expressed in this article are solely those of the authors and do not necessarily represent those of their affiliated organizations, or those of the publisher, the editors and the reviewers. Any product that may be evaluated in this article, or claim that may be made by its manufacturer, is not guaranteed or endorsed by the publisher.
Supplementary material
The Supplementary Material for this article can be found online at: https://www.frontiersin.org/articles/10.3389/fevo.2023.1242452/full#supplementary-material
Appendix A | All raw data organised per source and species/life stage of diadromous, and details of the mixing models.
Appendix B | R scripts and packages used in the analysis of this article.
References
Acou A., Boury P., Laffaille P., Crivelli A. J., Feunteun E. (2005). Towards a standardized characterization of the potentially migrating silver European eel (Anguilla Anguilla, L.). Archiv für Hydrobiol. 164, 237–255. doi: 10.1127/0003-9136/2005/0164-0237
Belletti B., de Leaniz C. G., Jones J., Bizzi S., Börger L., Segura G., et al. (2020). More than one million barriers fragment Europe’s rivers. Nature 588, 436–441. doi: 10.1038/s41586-020-3005-2
Bolnick D. I. (2001). Intraspecific competition favours niche width expansion in. Drosophila melanogaster. Nat. 410, 463–466. doi: 10.1038/35068555
Bolnick D. I., Ingram T., Stutz W. E., Snowberg L. K., Lau O. L., Paull J. S. (2011). Ecological release form interspecific competition leads to decoupled changes in population and individual niche width. Proc. R. Soc. London B 277, 1789–1797. doi: 10.1098/rspb.2010.0018
Bolnick D. I., Svanbäck R., Fordyce J. A., Yang L. H., Davis J. M. (2003). The ecology of individuals: incidence and implications of individual specialization. Am. Nat. 161, 1–28. doi: 10.1086/343878
Bruslé J., Quignard J.-P. (2001). Biologie des poisons d’eau douce européens (France: Editions TEC & DOC). 625p.
Buchheister A., Latour R. J. (2010). Turnover and fractionation of carbon and nitrogen stable isotopes in tissues of a migratory coastal predator, summer flounder (Paralichthys dentatus). Can. J. Fish. Aquat. Sci. 67, 445–461. doi: 10.1139/F09-19
Charnov E. L. (1976). Optimal foraging, the marginal value theorem. Theor. Pop. Biol. 9, 129–136. doi: 10.1016/0040-5809(76)90040-X
Chase J. M. (2003). Community assembly: When does history matter? Oecologia 136, 489–498. doi: 10.1007/s00442-003-1311-7
Chase J. M. (2011). “Ecological Niche Theory,” in The Theory of Ecology. Eds. Scheiner S. M., Willig M. R. (Chicago and London: The University of Chicago Press), 93–108. doi: 10.7208/9780226736877-006
Clavel J., Julliard R., Devictor V. (2011). Worldwide decline of specialist species: toward a global functional homogenization? Front. Ecol. Environ. 9, 222–228. doi: 10.1890/080216
Colles A., Liow L. H., Prinzing A. (2009). Are specialists at risk under environmental change? Neoecological, paleoecological and phylogenetic approaches. Ecol. Lett. 12, 849–863. doi: 10.1111/j.1461-0248.2009.01336.x
Costa J. L., Domingos I., Assis C. A., Almeida P. R., Moreira F., Feunteun E., et al. (2008). Comparative ecology of the European eel, Anguilla Anguilla (L. 1758), in a large Iberian river. Environ. Biol. Fishes 81, 421. doi: 10.1007/s10641-007-9229-2
Costa-Dias S., Sousa R., Lobón-Cerviá J., Laffaille P. (2009). “The decline of diadromous fish in Western Europe inland waters: mains causes and consequence,” in Fisheries: Management, economics and perspectives. Eds. McManus N. F., Bellinghouse D. S. (Nova Science Publishers Inc), 67–92.
Cucherousset J., Acou A., Blanchet S., Britton J. R., Beaumont W. R. C., Gozlan R. E. (2011). Fitness consequences of individual specialisation in resource use and trophic morphology in European eels. Oecologia 167, 75–84. doi: 10.1007/s00442-011-1974-4
Denis J., Rabhi K., Le Loc’h F., Ben Rais Lasram F., Boutin K., Kazour M., et al. (2022). Role of estuarine habitats for the feeding ecology of the European eel (Anguilla Anguilla L.). PloS One 17, e0270348. doi: 10.1371/journal.pone.0270348
Drouineau H., Carter C., Rambonilaza M., Beaufaron G., Bouleau G., Gassiat A., et al. (2018). River continuity restoration and diadromous fishes: much more than an ecological issue. Environ. Manage. 61, 671–686. doi: 10.1007/s00267-017-0992-3
Duarte G., Segurado P., Haidvogl G., Pont D., Ferreira M. T., Branco P. (2021). Damn those damn dams: Fluvial longitudinal connectivity impairment for European diadromous fish throughout the 20th century. Sci. Total Environ. 761, 143293. doi: 10.1016/j.scitotenv.2020.143293
Elliott S. A. M., Deleys N., Rivot E., Acou A., Réveillac E., Beaulaton L. (2021). Shedding light on the river and sea lamprey in western European marine waters. Endangered Species Res. 44, 409–419. doi: 10.3354/esr01113
Forget G., Baglinière J.-L., Marchand F., Richard A., Nevoux M. (2018). A new method to estimate habitat potential for Atlantic salmon (Salmo salar): predicting the influence of dam removal on the Sélune River (France) as a case study. ICES J. Mar. Sci. 75, 2172–2181. doi: 10.1093/icesjms/fsy089
Fukami T. (2004). Assemble history interacts with ecosystem size to influence species diversity. Ecology 85, 3234–3242. doi: 10.1890/04-0340
Herzka S. Z. (2005). Assessing connectivity of estuarine fishes based on stable isotope ratio analysis. Estuar. Coast. Shelf Sci. 64, 58–69. doi: 10.1016/j.ecss.2005.02.006
Hobson K. A. (1999). Tracing origins and migration of wildlife using stable isotopes: a review. Oecologia 120, 314–326. doi: 10.1007/s004420050865
Hutchinson G. E. (1957). Concluding remarks. Cold Spring Harbor Symp. Quantative Biol. 22, 415–427. doi: 10.1101/SQB.1957.022.01.039
IUCN. (2023). The IUCN Red List of Threatened Species. Version 2022-2. Available at: https://www.iucnredlist.org.
Jackson A. L., Parnell A. C., Inger R., Bearhop S. (2011). Comparing isotopic niche widths among and within communities: SIBER - Stable Isotope Bayesian Ellipses in R. J. Anim. Ecol. 80, 595–602. doi: 10.1111/j.1365-2656.2011.01806.x
Katano O., Nakamura T., Abe S., Yamamoto S., Baba Y. (2006). Comparison of fish communities between above- and below-dam sections of small streams; barrier effect to diadromous fishes. J. Fish Biol. 63, 767–782. doi: 10.1111/j.0022-1112.2006.00964.x
Keith P., Poulet N., Denys G., Changeux T., Feunteun E., Persat H. (2020). Les poisons d’eau douce de France. 2nd Edition (Paris: Biotope Editions, Mèze, Muséum national d’Histoire naturelle). (collections Inventaires et biodiversité), 704p.
Kelly F. L., King J. J. (2001). A review of the ecology and distribution of three lamprey species, Lampetra fluviatilis (L.), Lampetra planeri (Bloch) and Petromyzon marinus (L.): a context for conservation and biodiversity considerations in Ireland. Biol. Environ.: Proc. R. Irish Acad. 101, 165–185.
Kostecki C., Le Loc’h F., Roussel J.-M., Desroy N., Huteau D., Riera P., et al. (2010). Dynamics of an estuarine nursery ground: the spatio-temporal relationship between the river flow and the food web of the juvenile common sole (Solea solea, L.) as revealed by stable isotopes analysis. J. Sea Res. 64, 54–60. doi: 10.1016/j.seares.2009.07.006
Kostecki C., Roussel J.-M., Desroy N., Roussel G., Lanshere J., Le Bris H., et al. (2012). Trophic ecology of juvenile flatfish in a coastal nursery ground: contributions of intertidal primary production and freshwater particulate organic matter. Mar. Ecol. Prog. Ser. 449, 221–232. doi: 10.3354/meps09563
Lafage D., Carpentier A., Duhamel S., Dupuy C., Feunteun E., Lesourd S., et al. (2021). Site characteristics more than vegetation type influence food web structure of intertidal salt marshes. Front. Mar. Sci. 8. doi: 10.3389/fmars.2021.669759
Laffaille P., Acou A., Guillouët J., Mounaix B., Legault A. (2006). Patterns of silver eel (Anguilla Anguilla L.) sex ratio in a catchment. Ecol. Freshw. Fish 15, 583–588. doi: 10.1111/j.1600-0633.2006.00195.x
Laffaille P., Feunteun E., Baisez A., Robinet T., Acou A., Legault A., et al. (2003). Spatial organisation of European eel (Anguilla Anguilla L.) in a small catchment. Ecol. Freshw. Fish 12, 254–264. doi: 10.1046/j.1600-0633.2003.00021.x
Lassalle G., Crouzet P., Rochard E. (2009). Modelling the current distribution of European diadromous fishes: an approach integrating regional anthropogenic pressures. Freshw. Biol. 54, 587–606. doi: 10.1111/j.1365-2427.2008.02135.x
Limburg K. E., Waldman J. R. (2009). Dramatic declines in North Atlantic diadromous fishes. Bioscience 59, 955–965. doi: 10.1525/bio.2009.59.11.7
MacArthur R. H., Pianka E. R. (1966). On optimal use of a patchy environment. Am. Nat. 100, 603–609. doi: 10.1086/282454
Machut L. S., Limburg K. E., Schmidt R. E., Dittman D. (2007). Anthropogenic impacts on American eel demographics in Hudson River Tributaries, New York. Trans. Am. Fish. Soc. 136, 1699–1713. doi: 10.1577/T06-140.1
MacKenzie K. M., Trueman C. N., Palmer M. R., Moore A., Ibbotson A. T., Beaumont W. R. C., et al. (2012). Stable isotopes reveal age-dependent trophic level and spatial segregation during adult marine feeding in populations of salmon from the UK. ICES J. Mar. Sci. 69, 1637–1645. doi: 10.1093/icesjms/fss074
Merg M.-L., Dézerald O., Kreutzenberger K., Demski S., Reyjol Y., Usseglio-Polatera P., et al. (2020). Modeling diadromous fish loss from historical data: Identification of anthropogenic drivers and testing of mitigation scenarios. PloS One 15, e0236575. doi: 10.1371/journal.pone.0236575
Michel P., Oberdorff T. (1995). Feeding habits of fourteen European freshwater fish species. Cybium 19, 5–46. doi: 10.26028/cybium/1995-191-001
Morita K., Yamamoto S., Hoshino N. (2000). Extreme life history change of white-spotted char (Salvelinus leucomaenis) after damming. Can. J. Fish. Aquat. Sci. 57, 1300–1306. doi: 10.1139/f00-050
Myers G. S. (1949). Usage of anadromous, catadromous and allied terms for migratory fishes. Copeia 1949, 89–97. doi: 10.2307/1438482
Newsome S. D., Martinez del Rio C., Bearhop S., Phillips D. L. (2007). A niche for isotopic ecology. Front. Ecol. Environ. 5, 429–436. doi: 10.1890/060150.1
Peterson B. J., Fry B. (1987). Stable isotopes in ecosystem studies. Annu. Rev. Ecol. System. 18, 293–320. doi: 10.1146/annurev.es.18.110187.001453
Post D. M., Layman C. A., Arrington D. A., Takimoto G., Quattrochi J., Montana C. G. (2007). Getting to the fat of the matter: models, methods and assumptions for dealing with lipids in stable isotope analyses. Oecologia 152, 179–189. doi: 10.1007/s00442-006-0630-x
Pringle C. M., Freeman M. C., Freeman B. J. (2000). Regional effects of hydrologic alterations on riverine macrobiota in the New World: tropical-temperate comparisons. BioScience 50, 807–823. doi: 10.1641/0006-3568(2000)050[0807:REOHAO]2.0.CO;2
Quintella B. R., Andrade N. O., Almeida P. R. (2003). Distribution, larval stage duration and growth of the sea lamprey ammocoetes, Petromyzon marinus L., in a highly modified river basin. Ecol. Freshw. Fish 12, 286–293. doi: 10.1046/j.1600-0633.2002.00030.x
R Core Team (2022). R: A language and environment for statistical computing (Vienna, Austria: R Foundation for Statistical Computing). Available at: https://www.R-project.org/.
Reis-Santos P., Tanner S. E., França S., Vasconcelos R. P., Gillanders B. M., Cabral H. N. (2015). Connectivity within estuaries: an otolith chemistry and muscle stable isotope approach. Ocean Coast. Manage. 118, 51–59. doi: 10.1016/j.ocecoaman.2015.04.012
Rossman S., Ostrom P. H., Forest G., Zipkin E. F. (2016). Beyond carbon and nitrogen: guidelines for estimating three-dimensional isotopic niche space. Ecol. Evol. 6, 2405–2513. doi: 10.1002/ece3.2013
Russell W. M. S., Burch R. L. (1959). The principles of Humane Experimental Technique (Springfield: Charles C. Thomas Publishing).
Saboret G., Ingram T. (2019). Carryover effects of larval environment on individual variation in a facultatively diadromous fish. Ecol. Evol. 9, 10630–10640. doi: 10.1002/ece3.5582
Sargent R. D., Otto S. P. (2006). The role of local species abundance in the evolution of pollinator attraction in flowering plants. Am. Nat. 167, 67–80. doi: 10.1086/498433
Satterfield F. R. I. V., Finey B. P. (2001). Stable isotope analysis of Pacific salmon: insight into trophic status and oceanographic conditions over the last 30 years. Prog. Oceanogr. 53, 231–246. doi: 10.1016/S0079-6611(02)00032-0
Selleslagh J., Blanchet H., Bachelet G., Lobry J. (2015). Feeding habitats, connectivity and origin of organic matter supporting fish populations in an estuary with a reduced intertidal area assessed by stable isotope analysis. Estuaries Coasts 38, 1431–1447. doi: 10.1007/s12237-014-9911-5
Sexton J. P., Montiel J., Shay J. E., Stephens M. R., Slatyer R. A. (2017). Evolution of ecological Niche breadth. Annu. Rev. Ecol. Evol. System. 48, 183–206. doi: 10.1146/annurev-ecolsys-110316-023003
Stock B. C., Jackson A. L., Ward E. J., Parnell A. C., Phillips D. L., Semmens B. X. (2018). Analyzing mixing systems using a new generation of Bayesian tracer mixing models. PeerJ 6, e5096. doi: 10.7717/peerj.5096
Su Y. S., Yajima M. (2012). R2jags: A Package for Running jags from R. R package version 0.7-1. Available at: http://CRAN.R-project.org/package=R2jags.
Svanbäck R., Bolnick D. I. (2005). Intraspecific competition affects the strength of individual specialization: an optimal diet theory method. Evolution. Ecol. Res. 7, 993–1012.
Svanbäck R., Bolnick D. I. (2007). Intraspecific competition drives increased resource use diversity within a natural population. Proc. R. Soc. London B 274, 839–844. doi: 10.1098/rspb.2006.0198
Teichert N., Lizé A., Tabouret H., Roussel J.-M., Bareille G., Trancart T., et al. (2022b). European flounder foraging movements in an estuarine nursery seascape inferred from otolith microchemistry and stable isotopes. Mar. Environ. Res. 182, 105797. doi: 10.1016/j.marenvres.2022.105797
Teichert N., Lizé A., Tabouret H., Gérard C., Bareille G., Acou A., et al. (2022a). A multi-approach study to reveal eel life-history traits in an obstructed catchment before dam removal. Hydrobiologia 849, 1885–1903. doi: 10.1007/s10750-022-04833-9
Trancart T., Carpentier A., Acou A., Charrier F., Mazel V., Danet V., et al. (2020). When “Safe” Dams kill: analyzing combination of impacts of overflow dams on the migration of silver eels. Ecol. Eng. 145, 105741. doi: 10.1016/j.ecoleng.2020.105741
Van Valen L. (1965). Morphological variation and width of ecological niche. Am. Nat. 99, 377–390. doi: 10.1086/282379
Verhelst P., Reubens J., Buysse D., Goethals P., Van Wichelen J., Moens T. (2021). Toward a roadmap for diadromous fish conservation: the Big Five considerations. Front. Ecol. Environ. 19, 396–403. doi: 10.1002/fee.2361
Waldman J. R., Quinn T. P. (2022). North American diadromous fishes: Drivers of decline and potential for recovery in the Anthropocene. Sci. Adv. 8, eabl5486. doi: 10.1126/sciadv.abl5486
Keywords: diadromous fishes, stable isotopes, trophic niche, competition, dam, catchment
Citation: Lizé A, Teichert N, Roussel J-M, Acou A, Feunteun E and Carpentier A (2023) Isotopic niches of diadromous fishes inform on interspecific competition in an obstructed catchment. Front. Ecol. Evol. 11:1242452. doi: 10.3389/fevo.2023.1242452
Received: 19 June 2023; Accepted: 06 November 2023;
Published: 28 November 2023.
Edited by:
Pedro Segurado, University of Lisbon, PortugalReviewed by:
Gonçalo Duarte, University of Lisbon, PortugalSusana França, Center for Marine and Environmental Sciences (MARE), Portugal
Copyright © 2023 Lizé, Teichert, Roussel, Acou, Feunteun and Carpentier. This is an open-access article distributed under the terms of the Creative Commons Attribution License (CC BY). The use, distribution or reproduction in other forums is permitted, provided the original author(s) and the copyright owner(s) are credited and that the original publication in this journal is cited, in accordance with accepted academic practice. No use, distribution or reproduction is permitted which does not comply with these terms.
*Correspondence: Anne Lizé, anne.lize@mnhn.fr; Alexandre Carpentier, alexandre.carpentier@univ-rennes.fr