- 1Department of Wildland Resources and Ecology Center, Utah State University, Logan, UT, United States
- 2Richfield Field Office, Henry Mountains Field Station, Bureau of Land Management, Hanksville, UT, United States
- 3Institute of Zoology, Zoological Society of London, London, United Kingdom
Rangeland management has traditionally focused on the grazing effects of livestock, or on wild ungulates because they are potential competitors with livestock. However, herbivory by smaller herbivores, such as lagomorphs, could play a much greater role in shaping the plant community than is commonly thought. Being selective feeders with high mass-specific metabolic rates, we hypothesized that lagomorphs impose an herbivory regime on the rangeland that differs from that of ungulates and drives the plant community toward a different composition. We used an 8-year exclosure experiment with three plot types (complete exclosure, partial exclosure, open rangeland), each 5.95 m2, to separate the effects of grazing by lagomorphs (jackrabbits and cottontails) from the effects of the large grazers (mainly cattle and bison) on vegetation structure and plant community composition. We replicated the experiment across 20 sites in a semiarid rangeland in the western US. The plant community in the complete exclosure plots (lagomorphs and ungulates excluded) developed a fivefold greater standing crop of grass than the open rangeland but supported the lowest biomass, density, and aerial cover of forbs. Partial exclosure plots (ungulates excluded, lagomorphs included) developed a community with higher forb phytomass (33.6% increase relative to open rangeland) and lower forb species richness (16.4% decrease relative to open rangeland). This effect was mainly caused by the encroachment of noxious weeds, including Salsola tragus (prickly Russian thistle/tumbleweed), which covered up to 37% of the ground in plots that lagomorphs had exclusive access to. We infer that selective grazing by lagomorphs facilitates noxious weeds that are elsewhere controlled to some extent by (a) the coarse grazing and trampling effects of large ungulates and (b) competition with native herbaceous species. For semiarid rangelands that have been degraded by livestock grazing, our complete and partial exclosures show that a reduction in stocking rate might not return the rangeland to its former state due to a hysteresis effect resulting from the facilitation of noxious weeds by lagomorphs. Rangeland management should be informed by an understanding of the entire food web, which includes the direct and indirect grazing effects of small herbivores such as lagomorphs.
1 Introduction
The effects of herbivory on a plant community are expected to vary according to the composition of the herbivore guild but, in rangelands, the larger members of this guild, i.e., ungulates, have been studied far more extensively than the smaller members, such as lagomorphs (Augustine and McNaughton, 1998; Díaz et al., 2007; Gao and Carmel, 2020). Shrub-steppe ecosystems are generally managed as rangelands for livestock production and livestock species have dietary overlap with native ungulates and other smaller herbivores, such as lagomorphs (Ranglack et al., 2015a). Public lands of the western US are extensive, and their rangelands are primarily stocked with cattle that often comingle with native ungulates. Together, they consume a large proportion of the annual production of herbaceous phytomass (Bakker et al., 2006; Rebollo et al., 2013). However, lagomorphs are often overlooked, or at least underestimated, as consumers even though they selectively remove nutrient-dense plant parts from the forage resources shared by the entire herbivore guild.
Compared with ungulates, lagomorphs are very small-bodied and consequently have much higher mass-specific metabolic rates, thus requiring higher selectivity for higher quality forage. This implies that lagomorphs impose an herbivory regime on the rangeland that is qualitatively different from that of ungulates and we hypothesize that this drives the plant community toward a different composition. Any lagomorph effect is obscured, however, when ungulates and lagomorphs are foraging together, and so we report here on an exclosure experiment that separated the grazing effect of lagomorphs from that of the entire grazing guild.
In western US rangelands, lagomorphs are represented mainly by black-tailed jackrabbits (Lepus californicus) and desert cottontails (Sylvilagus audubonii). They remain underrepresented in the literature with respect to their impacts on rangeland resources, perhaps due to their small body size, nocturnal activity, and absence of any economic value (Rebollo et al., 2013; Simes et al., 2015; Schlater et al., 2021). Nevertheless, previous studies have shown that lagomorphs selectively consume high-quality plant parts (Hudson et al., 2005). When their population cycles reach high densities every 7–11 years, they can substantially reduce the standing crop of herbaceous vegetation (Rebollo et al., 2013; Ranglack et al., 2015a; Simes et al., 2015) and limit recruitment of certain plant species, especially native perennials (Bakker et al., 2006). Conversely, evidence from a long-term exclosure experiment in a mesic grassland indicates that rabbit grazing cannot control invasive weeds that have allelopathic properties (Crawley, 1990; Scherber et al., 2003). Accordingly, we predict that selective herbivory on palatable plants by lagomorphs should indirectly facilitate noxious weeds in a rangeland by reducing interspecific competition from palatable grasses and forbs. We used an 8-year experiment to test for evidence of such an effect in a semiarid rangeland managed for mixed-species grazing. Through the use of complete and semipermeable grazing exclosures, our experimental design separated the effects of herbivory by lagomorphs (jackrabbits and cottontails) from those of the entire grazing guild that includes large ungulates (mainly cattle and bison, Bison bison; Figure 1).
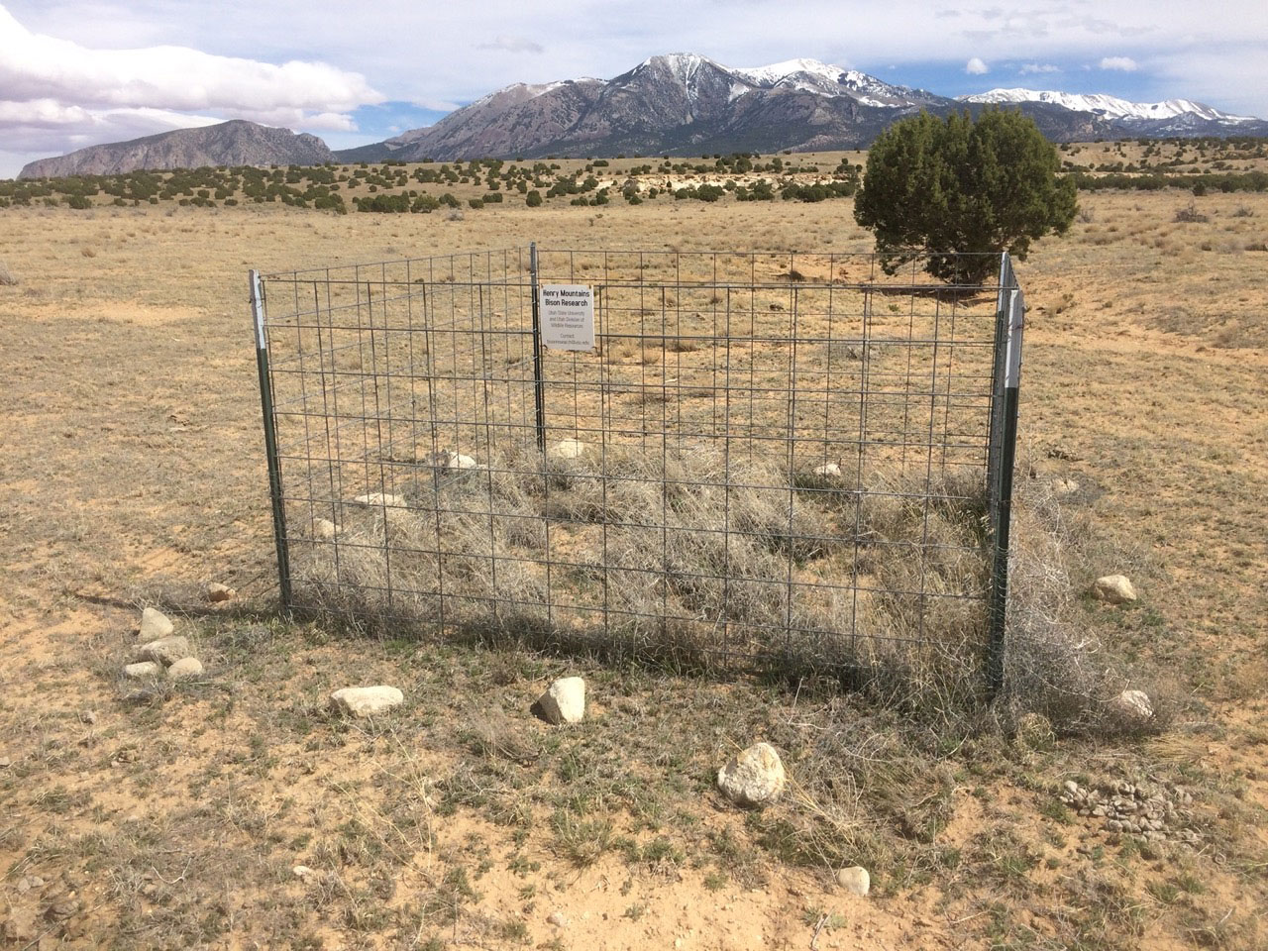
Figure 1 An “all out” (AO) exclosure pictured 5 years into the experiment. It is constructed of cattle panels with a chicken wire mesh apron around the lower half, to exclude all ungulates and lagomorphs (Photo: J.T. du Toit).
2 Materials and methods
2.1 Study area
The exclosure experiment was conducted in the northern foothills of the Henry Mountains (HM) of south-central Utah (Figure 2), where the study area was further described by Ranglack et al. (2015a). In 2011, 20 replicate sites were semi-randomly positioned across the Steele Butte North grazing allotment (33,436 ha, centered on 38° 6' 29' N; 110° 55' 1' W), which has generally low elevation (1,545 m–2,334 m), mild temperatures (mean annual range 2.22°C–21.7°C), and low mean annual precipitation. Data from the nearest weather station (Hanksville, 22 km away) show annual precipitation to be 151 mm ± 11.1 mm (30-year mean ± SE, 1992–2021) and the coefficient of variation to be 37% (Utah Climate Center, Utah State University). The study area is an arid and semiarid shrub-steppe region positioned on the borders of the Great Basin Desert with vegetation that consists mainly of halophytic native perennial grasses and forbs, such as galleta grass (Pleuraphis jamesii) and desert globemallow (Sphaeralcea spp.). The soils are typically entisols on alluvial terraces, fans, and mesas. This public land is managed by the Bureau of Land Management (BLM) and constitutes the largest conservation priority area within all roadless BLM lands in the US (Dickson et al., 2014). This rangeland is used by cattle ranchers with permits to public grazing allotments. It is also valued by the public for hunting and other recreational purposes. The cattle on the HM rangeland comingle with the only free-ranging, genetically pure, disease-free, and hunted population of bison in North America (Ranglack et al., 2015b). The mule deer (Odocoileus hemionus) population in this area is renowned for its trophy antlers, which motivates state and federal agencies to conduct control operations (aerial gunning and a state-sponsored bounty program) on coyotes (Canis latrans) with the objective of protecting mule deer fawns from predation. The grazing permittees support the coyote control program because of their concerns over possible depredation of newborn cattle calves, even though coyotes are major predators of lagomorphs in this area (Ranglack et al., 2015a).
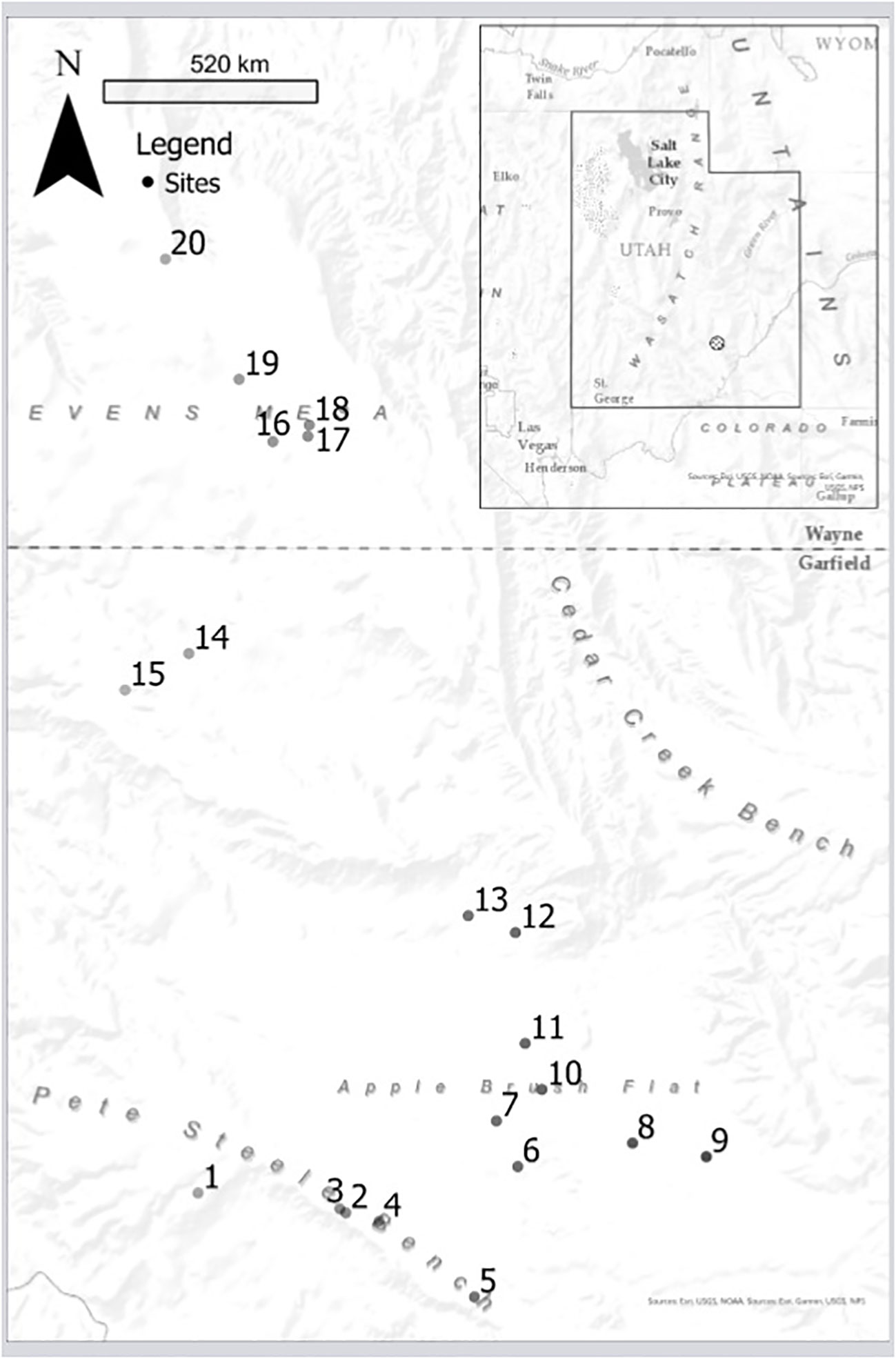
Figure 2 The 20 sites within the study area, each shown as a dot with its corresponding site number. The inset shows where the study area is in relation to the state of Utah, within the western US.
Our study used 40 small grazing exclosures (each 5.95 m2, Figure 3) at 20 replicate sites (two exclosures per site) in a randomized block design. We used multiple small exclosures rather than fewer larger ones to minimize the risk of damage by bison and they all remained intact throughout the study period. The two types of exclosure plot were (1) a complete exclosure constructed of coarse-meshed steel cattle panels with a chicken wire mesh apron that excluded lagomorphs, livestock, and all large wildlife species (all out, AO; Figure 1) and (2) a semipermeable exclosure constructed of only cattle panels (without the chicken wire mesh apron) that allowed access to lagomorphs but excluded livestock and large wildlife species (lagomorphs in, ungulates out, LIUO). Each site encompassing the three types of plot covered ~100 m2. The experiment was left to run undisturbed for eight years from October 2012 until we began our vegetation measurements in October 2020, by which time the vegetation in the exclosures was visibly very different from the surrounding rangeland (Figure 1).
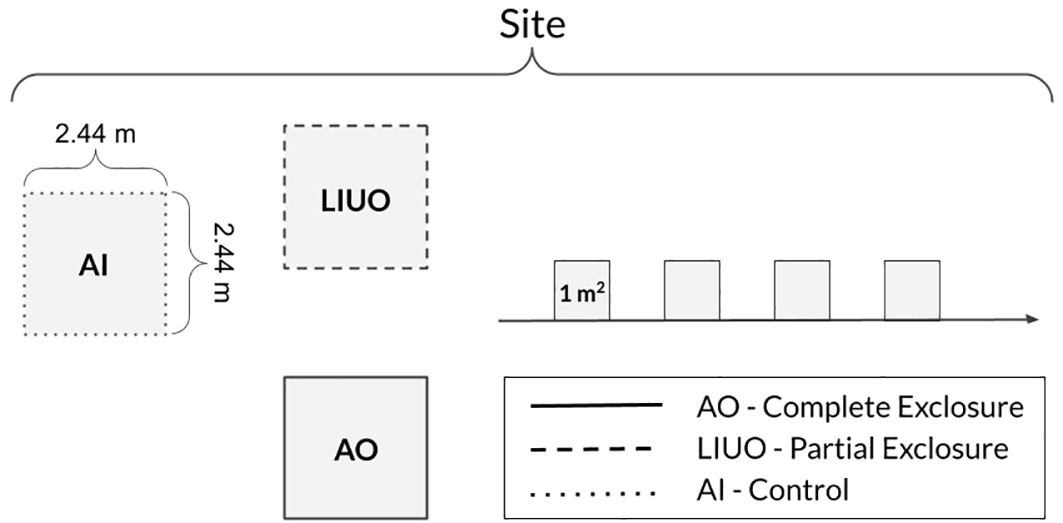
Figure 3 The layout at each experimental site: the solid-lined box is an exclosure plot that keeps all ungulates and lagomorphs out (AO); the dashed box is a semipermeable exclosure plot that allows lagomorphs in but keeps ungulates out (LIUO); the unlined shaded box is an open plot that allows all grazers in (AI). The 1-m2 quadrats are on an adjacent transect in open rangeland. Each side of an exclosure is one cattle panel 2.44 m (8') long and 1.27 m (50”) high.
2.2 Plant community composition
Four 1-m2 quadrats were sampled within each of the exclosures (AO, LIUO) at all 20 sites. Each quadrat was laid next to the other with corners joining in the middle of each plot to avoid any edge effects. To enhance accuracy in quadrat sampling, each 1-m2 quadrat was subdivided with wire into four quadrants (0.25 m2 each) during data collection and the data were combined for each 1-m2 quadrat. Then, the data from the four 1-m2 quadrats were averaged for each plot because they were not independent samples. Within each quadrant, we counted the number of individually rooted plants of each species and visually estimated the percent aerial cover of each species and plant functional type (grass, forb, shrub, or cactus). Visual estimates of percent coverage of bare ground and cryptobiotic soil crust were also recorded; together with the aerial cover of the plants, the total for each quadrant was 100%. Percentages were converted to proportions for statistical analysis. Grasses were counted by the number of bunches (each crown) or by free-standing new shoots. For rhizomatous species such as galleta grass, individuals were counted by each free-standing plant (ramet) or connected bunch (genet). For the open rangeland, which we called the “all in” (AI) or “control” community, we collected the same data by quadrat sampling every other meter (four times) along a transect starting 5 m from the center of the experimental site (Figure 3). The direction of the transect was determined by moving sequentially through cardinal directions from one site to the next, starting with North. Multiple separate quadrats (4 × 1 m2) along a transect were used rather than one plot (1 × 4 m2) so as to maximize the capture of spatial variability at each site for enhanced accuracy in quantifying “control” rangeland conditions.
At the end of the 2020 growing season (late October), all vegetation within the exclosures (AO, LIUO) and in one 4-m2 open rangeland plot (AI; Figure 3) was clipped to ground level, loosely packed in paper bags, and labeled by plot number and plant functional type (grass, forb, cactus, shrub). Only living parts were collected for shrubs. The clipped vegetation was mostly dry upon collection, but to achieve complete dehydration without mold, the bags were left open to air-dry in a temperature-controlled laboratory for 3 months. The dry phytomass was then sieved to remove any soil before weighing.
2.3 Lagomorph densities
During vegetation sampling within each plot type, we also counted the total number of lagomorph pellets in each quadrat. In addition, spotlight counts were conducted at least monthly for 2 years along a 16-km route through the study area in a vehicle driven at speeds of 16–24 km/h with two observers (see Simes et al., 2015). To estimate transect width, two measurements were taken after dark (one on each side of the road) at 10 different points approximately 1.6 km apart along the route, and the distance from roadside to the first visual obstruction (e.g., tree) to the spotlight beam was recorded. Where there was no obstruction, the maximum distance of detecting a lagomorph-sized object was measured at 70 m. From the mean detection distance (27.6 m on each side), plus the mean width of the road (4.02 m), we estimated a total transect width of 59.3 m and so the total transect area was 94.9 ha. Lagomorphs were either designated as cottontails, jackrabbits, or unknown if their species was not identifiable. Assuming the cottontail:jackrabbit ratio was constant across the unidentifiable lagomorphs, we observed means of 0.1 jackrabbits ha−1 and 0.05 cottontails ha−1.
2.4 Ungulate densities
The bison population in the Henry Mountains is controlled with recreational hunts that are adjusted annually with quotas to bring the population down to an escapement threshold objective of 325 animals. Bison use the rangeland around the study area mainly in mid-to-late-summer when calving and breeding (Ranglack and du Toit, 2015). If the typical pre-hunt population size was 400 animals during the 8 years of the exclosure study, and if they all used that grazing allotment, then the summer density would have been 0.01 animals ha−1 as a maximum estimate. By using the numbers in the cattle grazing permits issued to private ranchers by the Bureau of Land Management throughout the 8 years of the exclosure study, we estimated the density of cattle to have been 0.03–0.27 animals ha−1 from October to May in each seasonal cycle. No cattle are permitted in the area of our study during the months of June to September. Elk (Cervus canadensis) occur in the Henry Mountains but are managed to a minimal density and were never sighted during our study. Although mule deer are common in the uplands, they were infrequently sighted in the lower-elevation rangeland where our study was conducted.
2.5 Statistical analysis
Two methods were used for analyzing plant community data: first, we compared the absolute values of each measured variable across plot types and sites; second, we calculated the relative effect size (response) of each exclosure type relative to the control at each site and then compared the effects of the exclosure types pairwise across all 20 sites. Absolute values were analyzed using generalized linear mixed models (GLMM). Percent cover, species density (number of species m−2), and aboveground dry phytomass of each plant functional type (grass, forb, shrub, cactus) were the response variables, plot type (AO, LIUO, AI) was the explanatory variable, and site was the random effect for analyses. Each GLMM was created using R-packages glmmTMB, emmeans, and DHARMa in RStudio (Version 1.3.1093© 2009-2020 RStudio, PBC). Species density was analyzed in the model with both Gaussian and Poisson distributions. Percent cover and phytomass data were log-transformed in the model if the original data showed deviations from normality. In those cases, the log transformation removed or reduced skewness in the data to make it a better fit for linear modeling (Feng et al., 2014). P-values generated by the GLMM were adjusted using the Tukey method for a family of three estimates.
For aboveground phytomass and cover within each plant functional type, and for the whole plant community combined, the effect size of each exclosure type (E) was quantified relative to the control (C) using the log-transformed response ratio (RR). In this method, RRx = (E/C), where E is the measured value of the response variable in exclosure treatment x, and C is its value in the control plot. RR is the most robust effect-size metric in ecological exclosure experiments (Lajeunesse and Forbes, 2003) because it quantifies change relative to the control at the same site. With herbivory being constant at the site level, pairwise comparisons of exclosure types at each site are robust despite variability in herbivory between sites. Thus, for each treatment x, an analysis can include multiple values of RR from replicated sites without the need for an across-site measure of variability around means in the response variable. In this way, each response variable (aboveground phytomass or cover within each plant functional type) in both exclosure treatments (AO and LIUO) yielded a pair of RR values for each site, which were log-transformed and compared across sites using the pairwise t-test.
Two different indices were used to measure diversity within the plant community: Hill–Shannon diversity is preferred (Roswell et al., 2021) because it includes both the number of species and their relative abundance; Menhinick’s richness was also used because it is a conventional metric allowing comparisons across studies (Whittaker, 1977). The absolute values of both indices were used in comparisons across plot types and sites in GLMMs, and their relative values (RR) for each exclosure type relative to the control were also compared across sites using the pairwise t-test.
Finally, we used an unconstrained ordination method to map community structure. This method collapsed multiple dimensions (treatment communities and sites) by each functional group and the community as a whole to measure dissimilarity. Specifically, we used non-metric multidimensional scaling (NMDS) in R (R-packages Vegan, Tibble, and Tidyverse) to visualize potential dissimilarities among communities in the “control” and in each exclosure type across all 20 sites.
3 Results
3.1 Aboveground phytomass comparisons
During clipping, it was discovered that the complete exclosure (AO) at one site (#17, Figure 2) was occupied by a nest of harvester ants (Pogonomyrmex spp.) that had completely denuded the grass cover. That site was consequently removed from the analyses leaving N = 19 sites. The GLMM analyses (Figure 4) for the entire community showed that the control plots (AI) had less aboveground phytomass (88.9 ± 30 g m−2; mean ± SE) than both the complete (AO; 116 ± 12.4 g m−2) and partial exclosures (LIUO; 128.2 ± 12.9 g m−2). Within forbs, there was more aboveground phytomass in the partial exclosure (LIUO; 30.6 ± 5.74 g m−2) when directly compared with the complete exclosure (AO; 15.4 ± 4.18 g m−2). Within grasses, the control plot (AI, 12.4 ± 1.31 g m−2) had much less phytomass than both the LIUO (66.6 ± 8.71 g m−2) and AO (75.5 ± 8.07 g m−2) exclosures, but there was no significant difference between LIUO and AO exclosures.
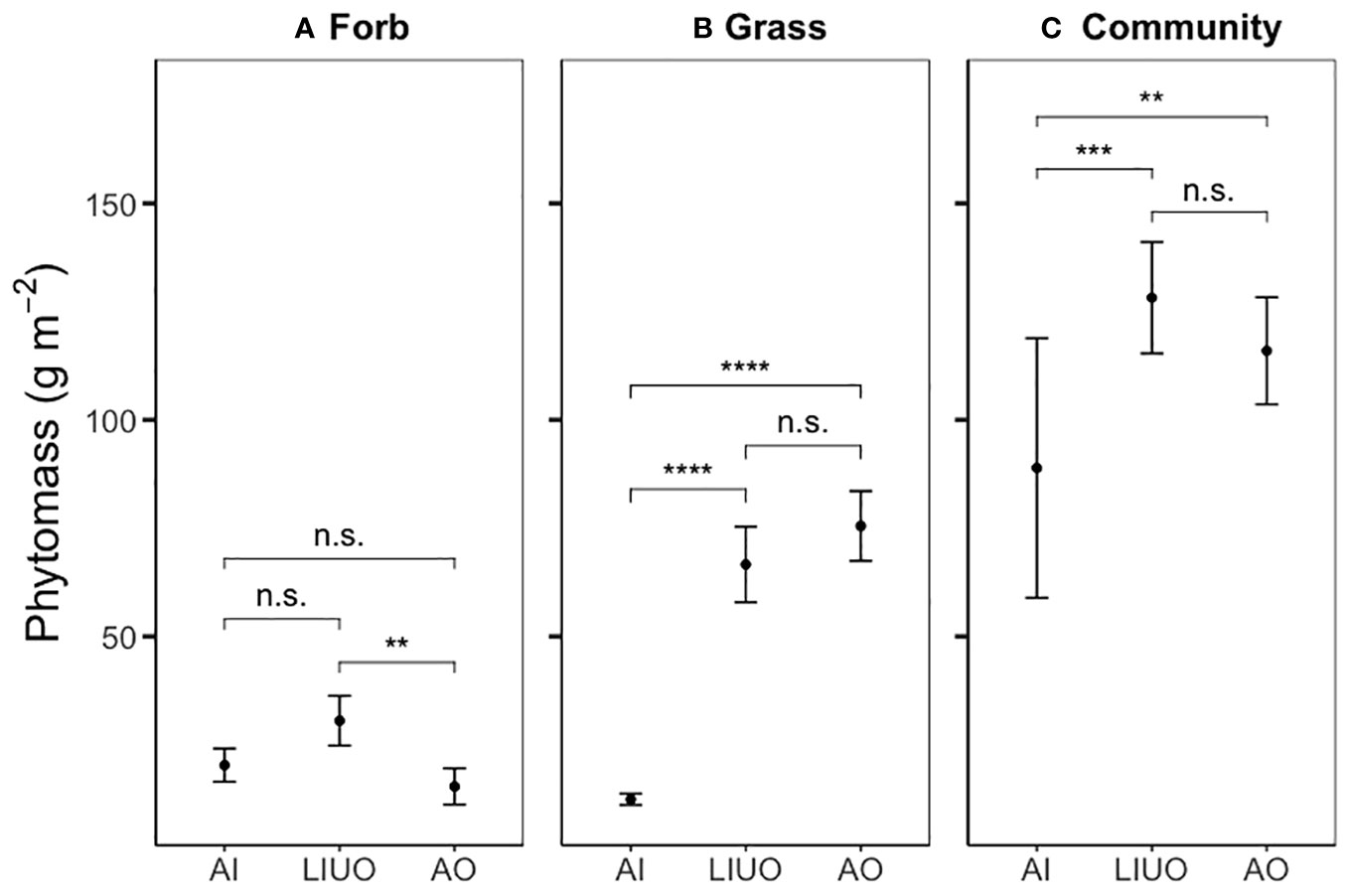
Figure 4 Absolute values for aboveground phytomass (mean ± SE) for the three plot types separated by forb (A), grass (B) and the whole community (C = grass + forb +cactus + shrub). Horizontal comparator bars indicate significant differences between two treatments (**p ≤ 0.01; ***p ≤ 0.001; ****p ≤ 0.0001) as determined by GLMM analyses, whereas nonsignificant differences (p > 0.05) are denoted “n.s.”.
Pairwise response ratio (log RR) comparisons of phytomass between exclosure types found a significant difference for forbs only (p < 0.005), with the exclosure effect being higher in the partial exclosure (LIUO) than the complete exclosure (AO). This result indicates that, relative to the control, forb phytomass was higher where lagomorphs had exclusive access compared with where neither lagomorphs nor ungulates had access.
For shrubs and cactuses, we found no significant variation in either absolute phytomass or relative effect (RR) of exclosure treatment across sites.
3.2 Aerial cover comparisons
The GLMM analysis revealed that the percent aerial cover of the entire plant community varied significantly between only the AI (control) and AO exclosure treatment (p < 0.05; Figure 5), with less aerial cover overall in the AI plots (18.7% ± 1.86%, mean ± SE) than in the AO exclosures (23.4% ± 2.3%). Thus, the total grazing pressure significantly reduced the total aerial cover of the community, as expected. Nevertheless, for forbs, aerial cover in the AI plots (8.85% ± 1.34%) was greater (p < 0.05) than in the AO exclosures (6.7% ± 1.28%), whereas grass cover was less in the AI plots (7.8% ± 1.22%) than both the LIUO (11.8% ± 1.49%, p < 0.01) and AO (13.5% ± 1.9%, p < 0.001) exclosures.
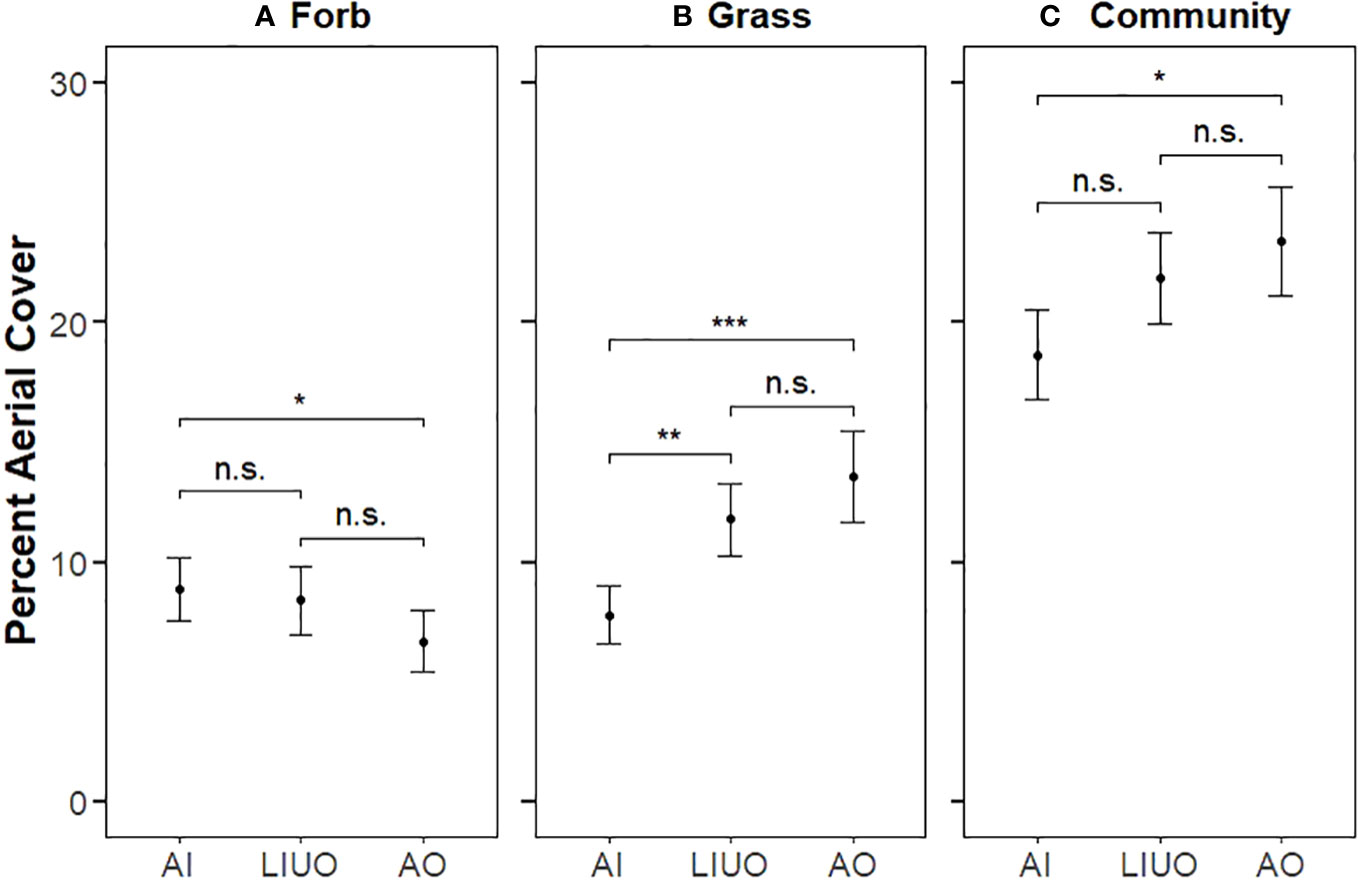
Figure 5 Percent aerial cover (mean ± SE) for the three plot types separated by forb (A), grass (B), and the whole community (C = grass + forb + cactus + shrub).. Horizontal comparator bars indicate significant differences between two treatments (*p ≤ 0.05; **p ≤ 0.01; ***p ≤ 0.001) as determined by GLMM analyses, whereas non-significant differences (p > 0.05) are denoted “n.s.”.
In pairwise comparisons of the exclosure effects relative to control conditions (RR), we found that it was only with forb aerial cover that there was a significant exclosure effect (p < 0.05), with forb cover being relatively higher in LIUO than in AO exclosures. There was no significant treatment effect for any other single functional group or the community grouped together as a whole was compared.
3.3 Species density, diversity and richness, and community dissimilarity
The mean index for Mehinick’s richness for the entire vegetation community in the AI (0.77) plots was higher than both the AO (0.58) and LIUO (0.56) plots when all three were compared directly in the GLMM (p < 0.05); however, the Kolmogorov–Smirnov test for goodness of fit showed significant deviation (p < 0.05), so these results may not be reliable. The GLMM showed that the density of forbs (individuals m−2) was lower (p < 0.05) in the AI (control) plots (39.6 ± 6.7 indiv. m−2) than the LIUO exclosures (48.9 ± 4.97 indiv. m−2); however, there were no significant variations in Hill–Shannon diversity or Mehinick’s richness indices for forbs specifically.
The only ordination of interest from the non-metric multidimensional scaling analyses was for grass, in which a stress level—the value that represents the difference between observed and fitted distances in a two-dimensional space—of 0.132 was reached using a Bray–Curtis calculation method. This indicates dissimilarity among the control and the two exclosure communities for grass, as would be expected in a grazing exclosure experiment.
3.4 Evidence of lagomorph facilitation of noxious weeds
We further explored the findings above that (a) absolute forb phytomass benefitted most from exclusion of ungulates but exposure to lagomorphs and (b), relative to the control, forb phytomass and aerial cover were both higher in the LIUO exclosures than in the AO exclosures. We found that, for forbs specifically, there was a negative correlation between phytomass and species richness in LIUO exclosures only (r = −0.55, p < 0.05), where richness declined as phytomass increased. One invasive forb species, Russian thistle (Salsola tragus), was dominant in the LIUO exclosures (Table 1), with the highest mean cover of any forb across the study area (even with the exclusion site #17) for all plot types combined at 2.17% (SE = 0.008), and a mean of 2.47% (SE = 0.009) in LIUO exclosures specifically. At site #17 where the AO exclosure had lost all of its grass to harvester ants, the LIUO exclosure was 37% covered by Russian thistle. In LIUO exclosures and AI plots combined, Russian thistle plant counts were positively correlated with lagomorph pellet counts (r = 0.54, p < 0.005). The mean (± SE) density of lagomorph pellets in AO exclosures was 5.98 m−2 (± 3.32), but those were all bleached, probably remaining from before the exclosures were erected or washed in during rainstorms. There was no significant difference (p > 0.05) in pellet density between AI plots (17.0 ± 2.94 pellets m−2) and LIUO plots (18.0 ± 8.00 pellets m−2), indicating that lagomorphs were entering the semipermeable exclosures without fear or favor. The two most commonly occurring forbs across sites were Russian thistle and threadleaf snakeweed (Gutierrezia microcephala), both of which are noxious weeds (Table 1). In terms of the mean (± SE) frequency of individual plants m−2, across all sites Russian thistle was almost twice as frequent in LIUO (3.25 ± 1.29 plants m−2) than in AI plots (1.71 ± 0.66 plants m−2), whereas the frequency in LIUO plots was not significantly greater (p > 0.05) than in AO plots (2.70 ± 1.02 plants m−2). Snakeweed is clearly facilitated by grazing, achieving the highest frequencies of occurrence in AI plots (2.89 ± 0.76 plants m−2) where exposed to both ungulate and lagomorph grazing, less in LIUO plots (1.95 ± 0.51 plants m−2) where exposed to lagomorph grazing only, and less again in AO plots (0.980 ± 0.260 plants m−2) where exposed to no grazing by either ungulates or lagomorphs.
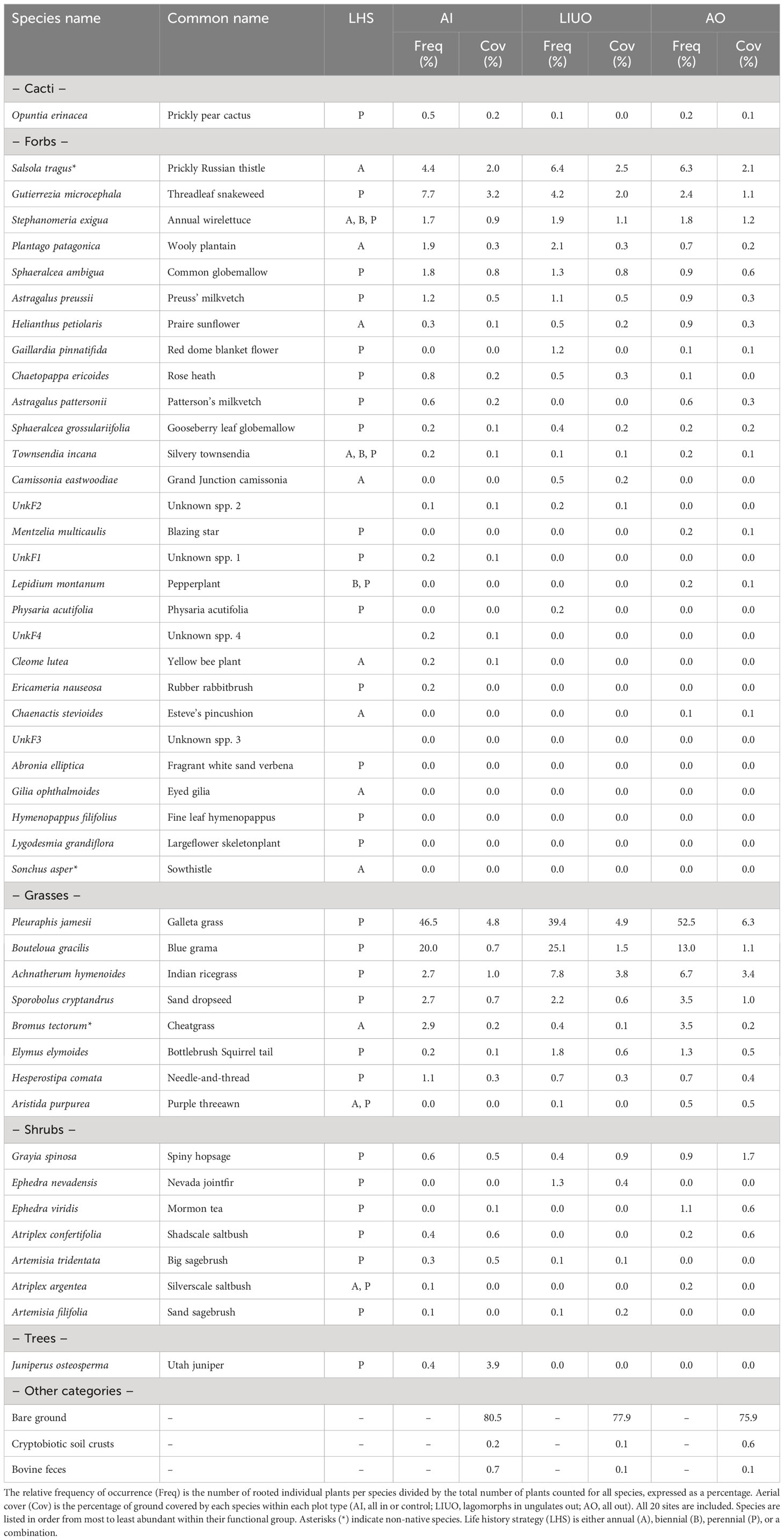
Table 1 All plant species identified in the study are listed by functional group (cactus, forb, grass, shrub, tree).
4 Discussion
The results of our experiment constitute an analysis of the effects of 8 years of differential herbivore exclusion on a rangeland plant community. It was impossible to conduct continuous annual sampling of phytomass throughout the study period because of disturbance to each treatment and so we used point-in-time comparisons at the end of the experimental period. This approach assumes that differences measured between treatments at the end of the study represent differences that developed consistently throughout. The caveat is that interannual variation in precipitation is high in semiarid rangelands such as our study area and so it might be argued that the standing crop (aboveground phytomass) was influenced more by the recent precipitation pattern than by longer-term effects of the experimental design. However, Knapp and Smith (2001) have shown that across North American biomes, including drylands, there is no relationship between interannual variations in precipitation and aboveground net primary production. Also, the effect sizes of the exclosure treatments in our spatially replicated experiment are overwhelming in comparison with the between-site variations in standing crop. We thus offer our results as evidence of how differential exclusions of ungulates and lagomorphs over 8 years led to the assembly of plant communities that were quantitatively and qualitatively different, especially with respect to the herbaceous layer.
The main effect was obviously that excluding ungulate grazers resulted in a plant community with a substantially larger standing crop of grass (fivefold larger in phytomass) than the open rangeland (Figures 1, 4). This explains why the NMDS ordination found the grass component of the community to generate the greatest dissimilarity among control and treatment plots. Less intuitive but of greater interest, however, was the way in which the forb component of the community responded to the differential exclosure experiment. The species density of forbs (species count m−2) was higher in the open rangeland plots (AI) than in the exclosures. However, while complete grazing exclosure (AO) did not increase the standing crop of forbs, partial exclosure (LIUO) doubled it relative to complete exclosure. Species richness in the partial exclosures decreased as phytomass increased, and the forb community became dominated by Russian thistle. This result is of particular interest because it is counter to our initial expectation for this species, with its tumbleweed dispersal system being blocked by the exclosures. Furthermore, if an artefactual effect of the exclosures had a strong influence on Russian thistle establishment, then it would have applied equally to LIUO and AO exclosures, but that was not the case.
Russian thistle is a C4 invasive annual forb introduced to the United States from Eurasia in the late 19th century and now occurs widely as a ruderal weed throughout western US rangelands. In our study system, it was one of the top four most abundant species, with the other three being grasses (P. jamesii, B. gracilis, and A. hymenoides; Table 1), with these four species together contributing 71.2% of the rooted individual plants found across plots and sites. In terms of commonness (frequency of occurrence), the top two forbs across the study area were Russian thistle and snakeweed, both of which are noxious weeds. Russian thistle is classified as a noxious weed mainly because of its prickly growth form and the common problem of tumbleweeds piling up against fence lines and buildings. It is consumed by cattle, having fair forage quality when young, but it can develop potentially toxic concentrations of soluble oxalates and nitrates (Hageman et al., 1988). Snakeweed is a short-lived perennial shrub/forb native to US rangelands. It is notoriously problematic due to its volatile secondary metabolites, among which saponins are the greatest threat to livestock due to reproductive toxicity and subsequent abortion (McDaniel and Ross, 2002).
We infer from the results of our experiment that lagomorph herbivory facilitates the proliferation of noxious weeds, especially Russian thistle, which became most abundant in the LIUO exclosures that kept ungulates out and allowed lagomorphs in. There was also a positive correlation between the numbers of Russian thistle plants and lagomorph pellets across all plots that lagomorphs had access to. Snakeweed became less common where vertebrate grazers were fully excluded, but in the partial exclosures where lagomorphs had access it remained common. These results can only be explained by lagomorphs concentrating their feeding on plants other than noxious weeds, presumably because of their prickly, fibrous, and potentially toxic properties. By selectively feeding on palatable herbaceous plants, lagomorphs would ultimately reduce interspecific competition for water in the rhizosphere of this semiarid ecosystem, thereby facilitating noxious weeds through competitive release. This advantage was neither gained in the complete exclosures where native plants were not grazed at all, nor on the open rangeland where coarse-feeding cattle and bison grazed and trampled Russian thistle together with the rest of the herbaceous layer (Figure 1). Conversely, at site #17 (Figure 2), where harvester ants removed all grass and almost all forbs other than Russian thistle—suggesting its unpalatability to ants as well as lagomorphs—the extensive cover by Russian thistle (37%) demonstrates the success of this species in sites where interspecific competition is suppressed.
4.1 The hysteresis effect
Our experiment provides a case study for the hysteresis effect and its influence on alternative stable states in ecosystems (Beisner et al., 2003), a concept that long-term studies are now confirming to have real and problematic manifestations in semiarid rangelands (Cipriotti et al., 2019). In such ecosystems, due to the effects of chronic grazing by livestock, it is to be expected that ruderal forbs (including noxious weeds) will reduce the grass:forb ratio of ground cover (Zhong et al., 2022). This reduction is observed to be the current state on the Henry Mountains rangeland as measured in our control (AI) plots. Now, if reduced stocking were implemented with the aim of returning the rangeland from its current state A to some former hypothetical state H (with equilibrium levels of grazing by wild ungulates and lagomorphs), then hysteresis should be expected and the community would drift toward an alternative state B (Figure 6). This is expected because the previously absent invasive plants would remain, and the uncontrolled lagomorph populations would maintain their selective feeding on palatable herbaceous plants and thereby allow unpalatable forbs, such as Russian thistle and snakeweed, to proliferate as we observed. Our experiment demonstrates the inherent problem with any objective to manage a transition from the present state A along a higher trajectory toward a more desirable state with a higher grass:forb ratio. The extreme case of complete (AO) exclusion for 8 years did elevate the grass:forb ratio to state C, but that would be impractical for rangeland management because it would require both substantial destocking and continuously intensive lagomorph control.
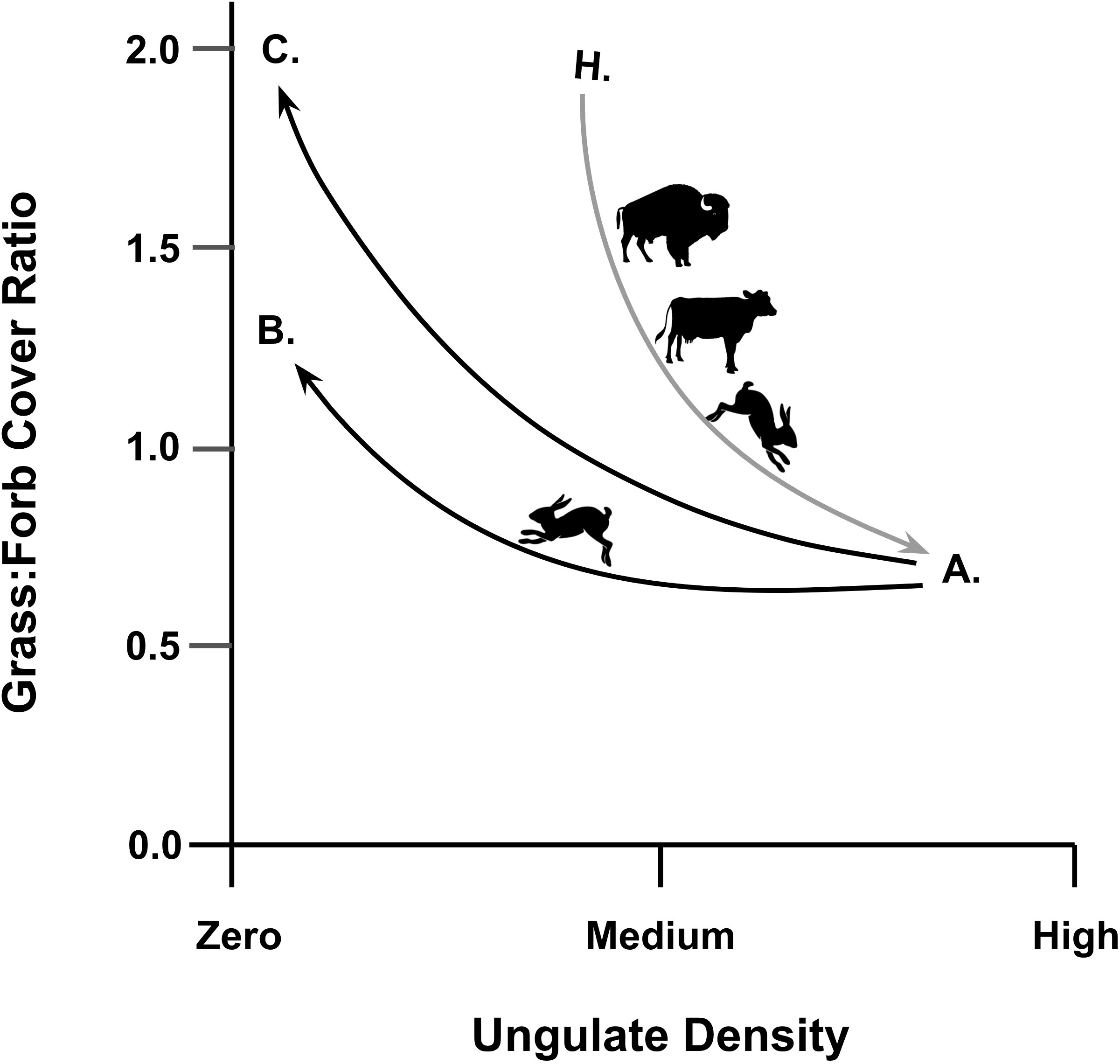
Figure 6 Our experimental exclosure study illustrates the hysteresis effect in rangeland plant community dynamics. The transition from some hypothetical benchmark state H prior to cattle stocking to the present state A is driven by ungulate and lagomorph herbivory, represented by our AI “control” plots (grass:forb cover ratio = 0.88). Even complete destocking of ungulates would not return the rangeland to its benchmark state H because selective herbivory by lagomorphs favors invasive ruderal forbs, which drive toward an alternative state B represented by our LIUO plots (grass:forb cover ratio = 1.40). Complete removal of all vertebrate grazers could result in a transition to a more grassy alternative state C, represented by our AO plots (grass:forb cover ratio = 2.02), but that would be impracticable at the landscape scale.
4.2 Management implications
With ecosystem service provision from the world’s rangelands being progressively challenged by climatic warming, aridification, CO2 enrichment (Polley et al., 2017), and invasive plants (DiTomaso et al., 2017), there is an increasing need to consider new approaches to rangeland management. Noxious weeds, both native and invasive, impose a multibillion-dollar cost on rangeland management in the US (DiTomaso et al., 2017). Thus, the dual effects of grass consumption and noxious weed facilitation by lagomorphs combine into a substantial ecosystem disservice at the landscape scale. Jackrabbits and cottontails are the main prey of coyotes on the rangelands of the southwestern US (Bartel and Knowlton, 2005), yet government-administered predator control programs specifically target coyotes in these landscapes. The objectives of coyote control, which include reducing predation on livestock and juvenile mule deer, have been questioned by research on their economical (Berger, 2006) and ecological (Mahoney et al., 2018) outcomes. Now, our differential exclosure experiment indicates that any alleviation of predation on lagomorphs could have unintended consequences for plant community dynamics, with costly implications for rangeland management. Our findings point toward a more inclusive approach to rangeland management that takes the food web into consideration, and also recognizes that a livestock-driven degraded state might be irreversible due to the hysteresis effect.
Data availability statement
The raw data supporting the conclusions of this article will be made available by the authors, without undue reservation.
Author contributions
JdT conceived and designed the study with contributions from SG, who also conducted most of the fieldwork, data collection, and analysis. DC facilitated agency support and contributed information on grazing history. All authors contributed to the article and approved the submitted version.
Funding
Funding was granted by the Utah Public Lands Initiative through the Utah Agricultural Experiment Station at Utah State University (PLI grant # 1497 to JdT) and by the US Department of the Interior’s Bureau of Land Management (GRANT13354229 to JdT).
Acknowledgments
Permission for the research was granted by the US Department of the Interior’s Bureau of Land Management. Field and logistical assistance was provided by Sue Fivecoat and Sarah Baldwin. Valuable assistance with statistical analyses was provided by Susan Durham. Helpful comments on a previous draft were contributed by Kari Veblen and Julie Young. We acknowledge that our study area is located in the ancestral, traditional, and contemporary lands of the Southern Paiute and Ute Tribes. In offering this land acknowledgment, we affirm Indigenous sovereignty, history, and experiences.
Conflict of interest
The authors declare that the research was conducted in the absence of any commercial or financial relationships that could be construed as a potential conflict of interest.
Publisher’s note
All claims expressed in this article are solely those of the authors and do not necessarily represent those of their affiliated organizations, or those of the publisher, the editors and the reviewers. Any product that may be evaluated in this article, or claim that may be made by its manufacturer, is not guaranteed or endorsed by the publisher.
References
Augustine D. J., McNaughton S. J. (1998). Ungulate effects on the functional species composition of plant communities: herbivore selectivity and plant tolerance. J. Wildlife Manage. 62 (4), 1165–1183. doi: 10.2307/3801981
Bakker E. S., Ritchie M. E., Olff H., Milchunas D. G., Knops J. M. H. (2006). Herbivore impact on grassland plant diversity depends on habitat productivity and herbivore size. Ecol. Lett. 9, 780–788. doi: 10.1111/j.1461-0248.2006.00925.x
Bartel R. A., Knowlton F. F. (2005). Functional feeding responses of coyotes, Canis latrans, to fluctuating prey abundance in the Curlew Valley, Utah 1977-1993. Can. J. Zoology 83, 569–578. doi: 10.1139/Z05-039
Beisner B. E., Haydon D. T., Cuddington K. (2003). Alternative stable states in ecology. Front. Ecol. Environ. 1 (7), 376–382. doi: 10.1890/1540-9295(2003)001[0376:ASSIE]2.0.CO;2
Berger K. M. (2006). Carnivore-livestock conflicts: Effects of subsidized predator control and economic correlates on the sheep industry. Conserv. Biol. 20 (3), 751–761. doi: 10.1111/j
Cipriotti P. A., Aguiar M. R., Wiegand T., Paruelo J. M. (2019). Combined effects of grazing management and climate on semi-arid steppes: Hysteresis dynamics prevent recovery of degraded rangelands. J. Appl. Ecol. 56 (9), 2155–2165. doi: 10.1111/1365-2664.13471
Crawley M. J. (1990). Rabbit grazing, plant competition and seedling recruitment in acid grassland. J. Appl. Ecol. 27 (3), 803–820. doi: 10.2307/2404378
Díaz S., Lavorel S., McIntyre S., Falczuk V., Casanoves F., Milchunas D. G., et al. (2007). Plant trait responses to grazing - a global synthesis. Global Change Biol. 13, 313–341. doi: 10.1111/j.1365-2486.2006.01288.x
Dickson B. G., Zachmann L. J., Albano C. M. (2014). Systematic identification of potential conservation priority areas on roadless Bureau of Land Management lands in the western United States. Biol. Conserv. 178, 117–127. doi: 10.1016/j.biocon.2014.08.001
DiTomaso J. M., Monaco T. A., James J. J., Firn J. (2017). “Invasive plant species and novel rangeland systems,” in Rangeland systems: processes, management and challenges. Ed. Briske D. D. (Switzerland: Springer, Cham), 429–465. doi: 10.1007/978-3-319-46709-2_13
Feng C., Wang H., Lu N., Chen T., He H., Lu Y., et al. (2014). Log-transformation and its implications for data analysis. Shanghai Arch. Psychiatry 26 (2), 105–109. doi: 10.3969/j.issn.1002-0829.2014.02
Gao J., Carmel Y. (2020). Can the intermediate disturbance hypothesis explain grazing–diversity relations at a global scale? Oikos 00, 1–10. doi: 10.1111/oik.06338
Hageman J. H., Fowler J. L., Suzukida M., Salas V., Lecaptain R. (1988). Analysis of Russian thistle (Salsola species) selections for factors affecting forage nutritional value. J. Range Manage. 41 (2), 155–158. doi: 10.2307/3898953
Hudson R., Rodríguez-Martínez L., Distel H., Cordero C., Altbäcker V., Martínez-Gómez M. (2005). A comparison between vegetation and diet records from the wet and dry season in the cottontail rabbit Sylvilagus floridanus at Ixtacuixtla, central Mexico. Acta Theriologica 50 (3), 377–389. doi: 10.1007/BF03192633
Knapp A. K., Smith M. D. (2001). Variation among biomes in temporal dynamics of aboveground primary production. Science 291, 481–484. doi: 10.1126/science.291.5503.481
Lajeunesse M. J., Forbes M. R. (2003). Variable reporting and quantitative reviews: A comparison of three meta-analytical techniques. Ecol. Lett. 6, 448–454. doi: 10.1046/j.1461-0248.2003.00448.x
Mahoney P. J., Young J. K., Hersey K. R., Larsen R. T., McMillan B. R., Stoner D. C. (2018). Spatial processes decouple management from objectives in a heterogeneous landscape: predator control as a case study. Ecol. Appl. 28 (3), 786–797. doi: 10.1002/eap.1686
McDaniel K. C., Ross T. T. (2002). Snakeweed: Poisonous properties, livestock losses, and management considerations. J. Range Manage. 55, 277–284. doi: 10.2307/4003135
Polley H. W., Bailey D. W., Nowak R. S., Stafford-Smith M. (2017). “Ecological consequences of climate change on rangelands,” in Rangeland systems: processes, management and challenges. Ed. Briske D. D. (Switzerland: Springer, Cham), 229–260.
Ranglack D. H., Dobson L. K., du Toit J. T., Derr J. (2015b). Genetic analysis of the Henry Mountains bison herd. PloS One 10 (12), e0144239. doi: 10.1371/journal.pone.0144239
Ranglack D. H., Durham S., du Toit J. T. (2015a). Competition on the range: Science vs. perception in a bison-cattle conflict in the western USA. J. Appl. Ecol. 52, 467–474. doi: 10.1111/1365-2664.12386
Ranglack D. H., du Toit J. T. (2015). Habitat selection by free-ranging bison in a mixed grazing system on public land. Rangeland Ecol. Manage. 68, 349–353. doi: 10.1016/j.rama.2015.05.008
Rebollo S., Milchunas D. G., Stapp P., Augustine D. J., Derner J. D., Rebollo S., et al. (2013). Disproportionate effects of non-colonial small herbivores on structure and diversity of grassland dominated by large herbivores. Oikos 122 (12), 1757–1767. doi: 10.1111/j.l600-0706.2013.00403.x
Roswell M., Dushoff J., Winfree R. (2021). A conceptual guide to measuring species diversity. Oikos 130, 321–338. doi: 10.1111/oik.05876
Scherber C., Crawley M. J., Porembski S. (2003). The effects of herbivory and competition on the invasive alien plant Senecio inaequidens (Asteraceae). Diversity Distributions 9, 415–426. doi: 10.1046/j.1472-4642.2003.00049.x
Schlater S. M., Ringenberg J. M., Bickford N., Ranglack D. H. (2021). White-tailed jackrabbits: a review and call for research. Southwestern Nat. 65 (2), 161–172. doi: 10.1894/0038-4909-65.2.161
Simes M. T., Longshore K. M., Nussear K. E., Beatty G. L., Brown D. E., Esque T. C. (2015). Black-tailed and white-tailed jackrabbits in the American West: History, ecology, ecological significance, and survey methods. Western North Am. Nat. 75 (4), 491–519. doi: 10.3398/064.075.0406
Whittaker R. H. (1977). Evolution of species diversity in land communities. Evolutionary Biol. 10, 1–67. doi: 10.1007/978-1-4615-6953-4_1
Keywords: ecological hysteresis, jackrabbits, mixed-species grazing management, ruderal forbs, dryland ecosystems, total grazing pressure
Citation: Garrett SM, Chigbrow D and du Toit JT (2023) Native lagomorphs facilitate noxious weeds in a semi-arid rangeland. Front. Ecol. Evol. 11:1237755. doi: 10.3389/fevo.2023.1237755
Received: 09 June 2023; Accepted: 27 September 2023;
Published: 13 October 2023.
Edited by:
A. Joshua Leffler, South Dakota State University, United StatesReviewed by:
Jameson Brennan, South Dakota State University, United StatesLaura Godó, Hungarian Academy of Sciences, Hungary
Benjamin Geaumont, North Dakota State University, United States
Copyright © 2023 Garrett, Chigbrow and du Toit. This is an open-access article distributed under the terms of the Creative Commons Attribution License (CC BY). The use, distribution or reproduction in other forums is permitted, provided the original author(s) and the copyright owner(s) are credited and that the original publication in this journal is cited, in accordance with accepted academic practice. No use, distribution or reproduction is permitted which does not comply with these terms.
*Correspondence: Johan T. du Toit, johan.dutoit@usu.edu