- 1College of Forestry, Northwest A&F University, Xianyang, Shaanxi, China
- 2College of Resources and Environmental Engineering, Tianshui Normal University, Tianshui, Gansu, China
Black locust (Robinia pseudoacacia L.), a common woody afforestation species, may threaten native biodiversity and ecosystems. Its invasive nature has not been examined in Asia, and links between aboveground and underground ecosystems have been inadequately established. Here, to figure out its effect on native ecosystems along the afforestation chronosequence, we selected three age class mixed forests of Robinia pseudoacacia/Platycladus orientalis stands (M7, M13, and M23) and a pure native forest of Platycladus orientalis stands (P0) as the control group on the Loess Plateau in China. The results exhibited that Black locust significantly increased the shrub diversity by 23.24%, herb richness by 10.79%, total carbon by 6.14%, organic carbon by 16.05%, and total nitrogen by 36.24% in 13 years of introduction. While the decrease in the diversity and richness of shrubs and herbs, soil pH, and total phosphorus emerged after the introduction of 13 years. Despite the increasing richness and diversity of soil bacteria, total phosphorus was inferred to be a limiting factor for microbes after 23 years. The partial least squares path models showed strong correlations and remarkable aboveground–underground associations. Moreover, vegetation, the main driving force, had the most decisive influence on soil properties and microbial communities. Taken together, these results will contribute to assessments and management of black locust plantations and provide insights into the biological invasion and aboveground–underground theories.
1. Introduction
Black locust originated in the Appalachian and Ozark regions of North America (Kleinbauer et al., 2010). Due to its light-demanding, vegetative reproduction through root and stem sprouts, wide ecological ranges, and fast growth, this species has always been planted widely in the world, especially for ecological erosion control, vegetation restoration, and reconstruction in fragile ecological zones (Laiolo et al., 2003), which has led to “black locust mania” since the 18th century (Vítková et al., 2016). In the 1950s, as a major woody afforestation species, black locust was also introduced into the Loess Plateau, China, and now its distribution area is more than 70,000 ha (Liang et al., 2018). Recently, it has been proven to have obvious negative impacts on native vegetation communities, animal communities, and ecosystems (Essl et al., 2011; Vilà et al., 2011; Vítková et al., 2016, 2017; Lazzaro et al., 2017) and is considered as an invasive species in several European countries, such as Norway, the Czech Republic, Germany, and Italy (Vítková et al., 2017). However, there are fewer reports of the ecological effects of the introduction of the alien black locust on the native ecosystems in Asia (Chang-Seok et al., 2004), and its invasive nature has not been finalized. Therefore, a compelling and scientific evaluation of the effects of this species on native ecosystems is necessary for local forest management.
Black locusts greatly enhance nitrogen contents in soil (Macedo et al., 2008), improve forest stand conditions, and alter the biodiversity of vegetation communities (Vítková et al., 2017) and microbial communities in native ecosystems (Kleinbauer et al., 2010). For soil properties, an increasing number of studies provide evidence that black locust plantations markedly raised organic carbon and nitrogen content on degraded agricultural lands (Zeng et al., 2015; Papaioannou et al., 2016; Vítková et al., 2017; Liu et al., 2018b; Zhang et al., 2019a,b), but many other studies find no any increase in nitrogen stocks in the mineral soil (Marco et al., 2013) or even the species loss in nutrient-poor stands (Rice and Federici, 2004). Moreover, the proliferation of this particular species in the central regions of Italy has resulted in a gradual decline in the richness and diversity of native species (Benesperi et al., 2012). However, Motta et al. (2009) assumed that native plant species are likely to outcompete black locusts and dominate in the absence of disturbance stand over several decades. The understory flora caused by black locust invasion were different from those dominated by native trees (Vítková et al., 2017), and their effects on biodiversity are still equivocal. In short, there is no consensus on whether the introduction of black locusts will bring about drastic positive or negative ecological effects on the native vegetation communities, soil properties, and soil microbial communities.
Recently, a number of studies have focused on the aboveground–underground associations, especially the drivers of the relative abundance and diversity of the soil microbial community (Bardgett and Wardle, 2013). Many studies considered that plant and soil properties co-shape the richness of soil microbial communities (Zhang C. et al., 2016; Yang et al., 2017b, 2019a; Xu et al., 2020). Some studies indicated that plant richness and coverage were predicted to play key roles in the abundance of soil microbial communities (Goodness et al., 2016; Jiang et al., 2017), while other studies showed that soil properties such as soil nutrients and humidity made more contributions to the composition and diversity of microorganisms (Reinhart et al., 2016). Yet it remains uncertain whether there are still any aboveground–underground links or associations among vegetation, soil properties, and soil microbial communities in the new ecosystem after the introduction of the alien black locust, and if so, how they interact with each other. Which one has the biggest contribution to the ecosystem component?
Given that the black locust has been introduced around the world for long history and could have produced far-reaching ecological effects on the local ecosystem through a long succession process, most studies chose only a certain age class of black locust stands to compare with native stands whether in Europe or Asia and did not study the effect of introduction time (Vítková et al., 2017; Lazzaro et al., 2018). Compared with the unintroduced area, plant communities, soil properties, and microbial communities under pure black locust forest showed significant changes during the afforestation years (Liu et al., 2018a; Xu et al., 2020). Thus, we hypothesized that the introduction of alien black locust had effects on native ecosystems along the introduction chronosequence. To verify this hypothesis, we adopted the method of replacing the introduction chronosequence with space and selected three age-class artificial mixed forests of R. pseudoacacia/P. orientalis stands (7a, 13a, and 23a) and pure native forests of P. orientalis stands (CK, 23a) with approximately similar site conditions. The aims of this study were to (1) evaluate qualitatively and quantitatively the effects on vegetation, soil properties, and soil fungal and bacterial communities caused by the introduction of alien black locust following afforestation; (2) explore the aboveground and belowground associations among vegetation, soil properties, and soil microbial communities; and (3) if the relationships do exist, clarify how these ecosystem components affect each other.
2. Materials and methods
2.1. Experimental area
Yongshou County, Shaanxi province, is the ideal area for studying the introduction of black locust as its an area with more than 70 years of afforestation history and is the largest area of black locust forest in China. It belongs to the southern Loess Plateau gully region (34°47′–34°51′ N, 108°05′–108°10′ E). The annual mean temperature is 10.8°C, and the annual mean precipitation is 605 mm, mainly concentrated in August and September every year (Liu et al., 2018a). There is an annual solar radiation hour of 2166.2 h and a frost-free period of 210 days per year. The dominant soil type is loessial (Liu et al., 2018b).
In this region, the original vegetation had been destroyed completely by the 1950s, and only a small area of natural secondary broad-leaved forests such as Quercus wutaishanica Mayr and Populus davidiana Dode remain (Ma, 1991; Yu et al., 2014). In the 1970s to 1980s, large-scale vegetation afforestation projects were carried out, and the main tree species was exotic black locust, as well as native trees, such as Pinus tabulaeformis Carr. and Platycladus orientalis (L.) Franco (Yu et al., 2014). However, there was the only mixed forest of Robinia pseudoacacia/Platycladus orientalis stands with native tree species, a large studied area and a different age class. Moreover, to exclude the influence of stand density on the experiment, we chose the mixed forest of R. pseudoacacia/P. orientalis stands with a mixed ratio of 6:4 as the research object.
2.2. Experiment design
In July 2021, adopting the method of substituting space for introduction chronosequence, we selected three age classes (7, 13, and 23 years) of the artificial mixed forest of R. pseudoacacia/P. orientalis stands and pure native forest of P. orientalis stands (23a) with approximately similar elevation, slope aspect, soil type, etc. (Supplementary Table S1 and Figure 1). Among them, the pure native P. orientalis forest was 23 years age class, which represented the stage of native stands and no introduced black locust, so it was marked as P0. In the three age classes of mixed forest, P. orientalis was the same age class of 23 years, but R. pseudoacacia were three age classes of 7, 13, and 23 years. They represented three introduction times of 7, 13, and 23 years and were recorded as M7, M13, and M23, respectively.
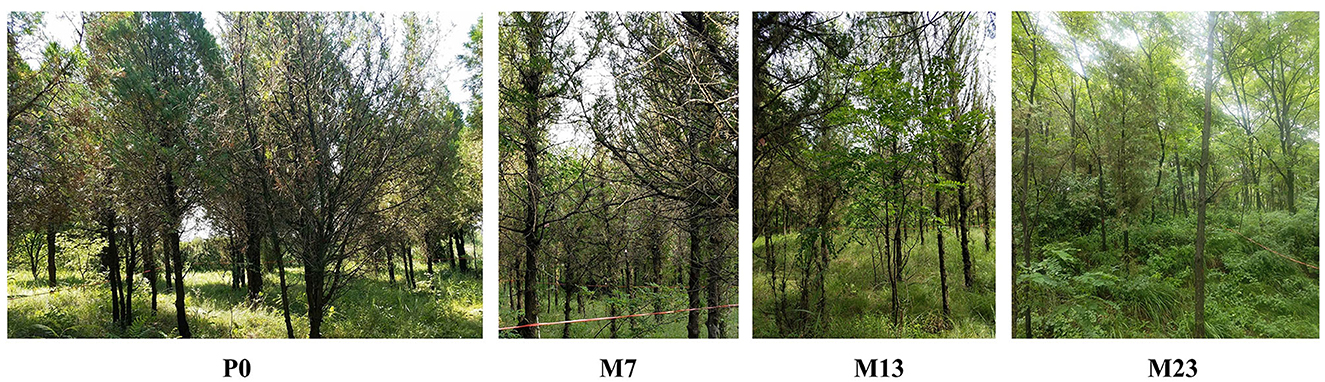
Figure 1. Photographs of the partial study sites for different stand types. P0, the pure native P. orientalis forest without introduced black locust (CK, 0 years). M7, M13, and M23 are the three age classes of the mixed forest of R. pseudoacacia/P. orientalis stands, which represent three introduction chronosequence of 7, 13, and 23 years.
Three 20 m × 20 m representative sample plots were established for each stand type (Supplementary Table S1). The distance between replications plots of each age class stand was >1 km, and the distance between plots of different age class stands was more than 2 km. In total, 12 plots were surveyed. In each plot, three 2 m × 2 m subplots and five 1 m × 1 m subplots were arranged along the diagonal of the plot for investigating shrubs and herbaceous composition. For mixed forest stands, the mixed proportion of R. pseudoacacia/P. orientalis was 6:4. Numerous ecosystem component indices were chosen (Table 1).
2.3. Plant investigation, soil sampling, and analysis
In each plot, we measured the diameter at breast height (DBH), height, crown diameter, and canopy density (CD) of trees. We also surveyed each species with the name, the number of stems, coverage, frequency, and height using a similar sampling approach that Liu et al. (2018b) described and separated into native or non-native species to calculate the diversity and richness index of shrub, herb, and understory vegetation. The ecotype of vegetation is the most direct and sensitive indicator to reflect the change in the ecological environment. To indicate the changing understory microenvironment, we classified the ecological types of plants by referring to Flora of Loess Plateau (Chen et al., 2007; Li, 2009) and Flora of China (http://www.iplant.cn/frps). According to the needs of water, vegetation ecotypes were divided into xerophytes, mesophytes, and xero-mesophyte. Xerophytes were adapted to dry habitats, while mesophytes were adapted to an average supply of water and temperature environment (Li, 2009). Detailed information about shrubs and herbs is listed in Supplementary Tables S2, S3.
The litter was collected from the soil surface using five 1 m × 1 m quadrats randomly arranged in each plot for calculating litter biomass (LB). We also collected the top 0–20 cm soil at 10 points in each 20 m × 20 m plot according to an S-shaped pattern and mixed them evenly into one sample. The visible plant roots, stones, and litter were removed, and then, soil samples were sifted through the 2-mm sieve, divided into two parts, and transported to the laboratory immediately. Two parts of soil samples were stored in a −80°C refrigerator and at room temperature, respectively, for DNA analysis and nutrient analysis. The analytical methodology of soil physicochemical properties was determined as previously reported (Bao, 2000), including pH, SM, BD, SOC, TC, TP, TN, -N, -N, and MBC (Xu et al., 2020).
2.4. Fungal and bacterial community analysis
2.4.1. DNA extraction, PCR, and high-throughput sequencing
Using the MP FastDNA spin kit for soil (MP Biomedicals, Solon, OH, USA), we extracted fungal and bacterial DNA from 0.5 g of soil samples. The V4 region of the bacterial 16S rRNA gene and the ITS1 region of the fungal rRNA gene were amplified using the primers 515F (GTGCCAGCMGCCGCGGTAA) and 806R (GGACTACHVGGGTWTCTAAT) for 16S rRNA and the primers ITS5-1737F (GGAAGTAAAAGTCGTAACAAGG) and ITS2-2043R (GCTGCGTTCTTCATCGATGC) for ITS (Bokulich and Mills, 2013; Mori et al., 2014). PCR products were detected using electrophoresis with 2% agarose gel. Then, a TruSeq® DNA PCR-free Sample Preparation Kit was used for library construction, and the target bands were recovered. Finally, qualified sequencing was performed on an Illumina NovaSeq6000 platform (Majorbio Bio-Pharm Technology Co., Ltd. Shanghai, China).
2.4.2. Sequence data processing
First, the raw tags were demultiplexed and quality filtered to obtain effective tags. Then, Uparse v7.0.1001 (http://www.drive5.com/uparse/) was operated for effective tags clustering. By default, sequence clustering became OTUs with 97% identity. After clustering, species annotation analysis and taxonomic information at each classification level were performed using the Mothur method and the SSU rRNA database of SILVA138 (http://www.arb-silva.de/) (Wang et al., 2007; Edgar, 2013).
2.5. Statistical analyses
We used a one-way analysis of variance (one-way ANOVA) and a least significant difference (LSD) to estimate the significant differences in soil properties, vegetation properties, and microbial communities among different stands using SPSS 20.0 (Chicago, USA). Hbacteria, Sbacteria, Hfungi, and Sfungi indices were calculated as the diversity and abundance of the microbial community at the OTU level using QIIME software (Version 1.9.1). Figures displaying microbial community assemblages were produced using OriginPro2018 software (OriginLab, USA). Non-metrical multidimensional scaling (NMDS) was used for reflecting the inter-group and intra-group differences of samples at different stands. To know the relationship between ecological indices and introduction chronosequence, principal component analysis (PCA) was used first to select the most significant influential indices from all ecological indices and then further regression analysis was performed using the “basicTrendline” package in R-4.0.5 (R Core Team, 2021). The data were normalized using log transformation. Pearson's correlation analysis was used to understand the relationships among soil properties, vegetation properties, and microbial communities. Furthermore, we used the partial least squares path models (PLS-PM) with the “plspm” package in R to assess the interaction of vegetation, soil, and microorganisms.
3. Results
3.1. The characteristics of understory flora along the introduction chronosequence
The canopy density (CD), litter biomass (LB), and richness of understory vegetation (Splant) were significantly affected by the introduction chronosequence, except for the diversity of understory vegetation (Hplant) (Figure 2). Compared with P0, CD, LB, and Splant significantly increased by 16.67–45.83%, 1.44–7.27%, and 27.43–47.79%, respectively after the introduction of black locust (p < 0.05). The richness and diversity of shrubs and herbs (Sshrub, Hshrub, Hherb, and Sherb), also changed after the introduction of the black locust. Hshrub and Sherb increased by 23.24% and 10.79% first and then decreased by 29.46% and 25.52%, with the highest values in M13, but Hherb varied later than Hshrub with decreasing trends from M13 to M23. In addition, from P0 to M23, the dominant species of shrub changed gradually from Lespedeza bicolor to Rubus parvifolius, and the dominant species of herb changed from Tripogon chinensis and Carex lanceolata to Aster tataricus and Patrinia scabiosifolia, as well as the ecotypes transformed xerophyte into mesophyte (Supplementary Tables S2, S3).
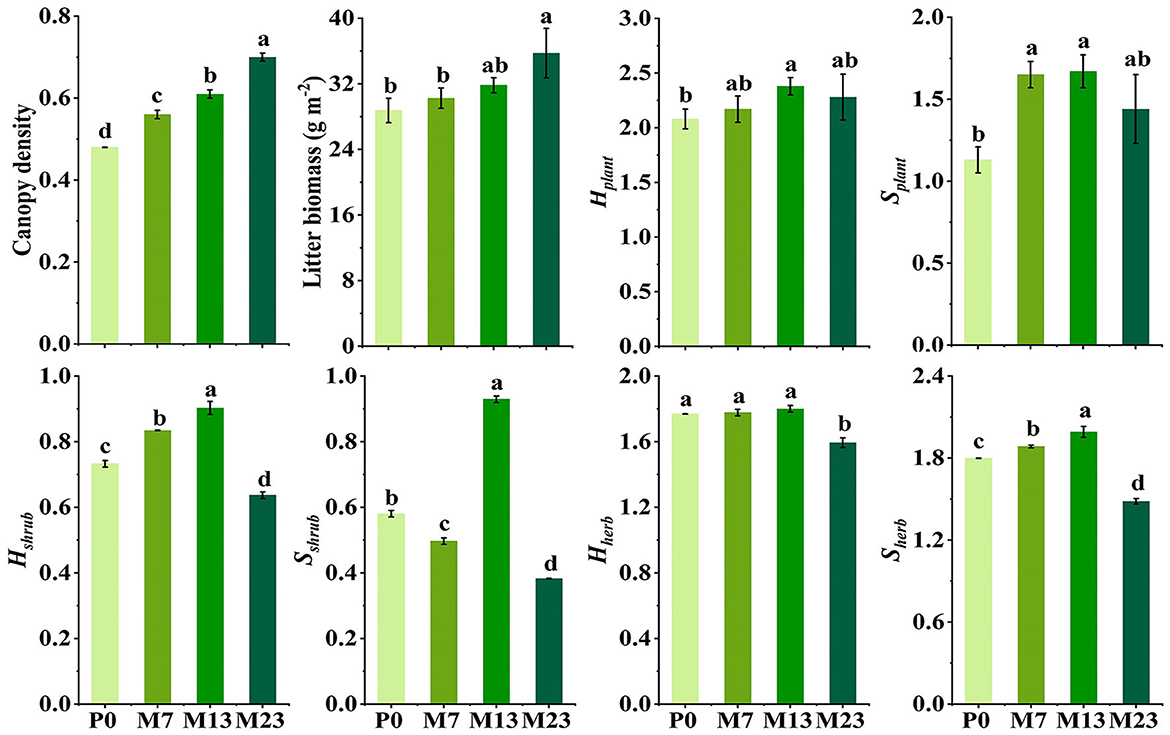
Figure 2. The mean (±standard error) of characteristics of understory vegetation with introduction chronosequence (n = 3). a–dDifferent letters indicate that variables were significantly different at p < 0.05 in each stand type. P0 is the pure native P. orientalis forest without the introduced black locust (CK, 0 years). M7, M13, and M23 are the three age classes of the mixed forest of R. pseudoacacia/P. orientalis stands, which represent three introduction chronosequences of 7, 13, and 23 years.
3.2. Soil properties along the introduction chronosequence
Statistically significant differences were found for almost all soil properties except for BD and SM after the introduction of the black locust (Table 2). Carbon-related variables, such as SOC and MBC, increased quickly by more than 79.32% over the introduction chronosequence. The content of TC changed fast, with the decreasing trends from P0 to M7, and increasing trends after the introduction of 7 years. Nitrogen-related variables and pH changed more slowly, with TN, -N, and pH not showing significant differences relative to pure native P. orientalis forest stands until 23 years of the black locust introduction. -N showed differences after 13 years of black locust introduction. TP changed with the increasing trends initially in the early introduction years relative to pure native stands, then decreasing along the introduction chronosequence. On the whole, the introduction of black locust significantly increased the content of -N, -N, TN, TC, SOC, and MBC by 9.76, 24.94%, 69.05%, 15.56%, 79.32%, and 1.27, respectively, while decreased pH, TP, and C/N by 2.65%, 8.93%, and 32.56%.
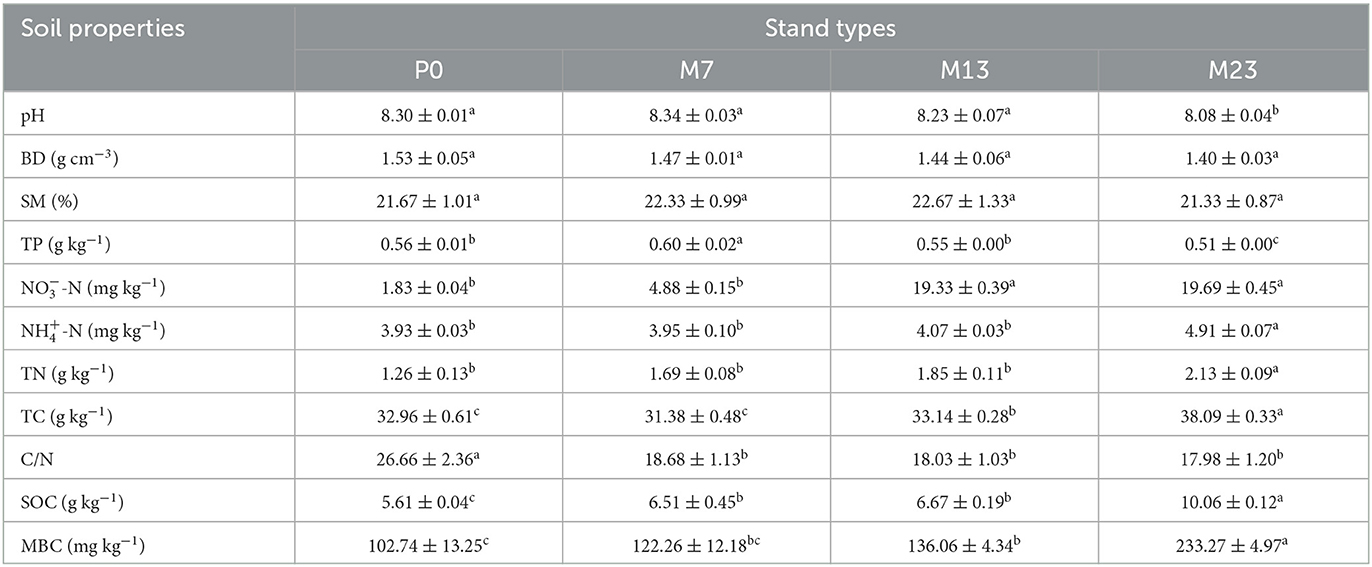
Table 2. Soil properties with introduction chronosequence. The values were mean ± standard error (n = 3). Different letters indicated that variables significantly differed from stand types at p < 0.05.
3.3. Soil microbial community along the introduction chronosequence
In total, 647,370 bacterial sequences and 766,194 fungi sequences were used for cluster analysis at a 97% similarity level. Based on the results of our analyses, soil fungal and bacterial communities were affected by black locust introduction (Figure 3).
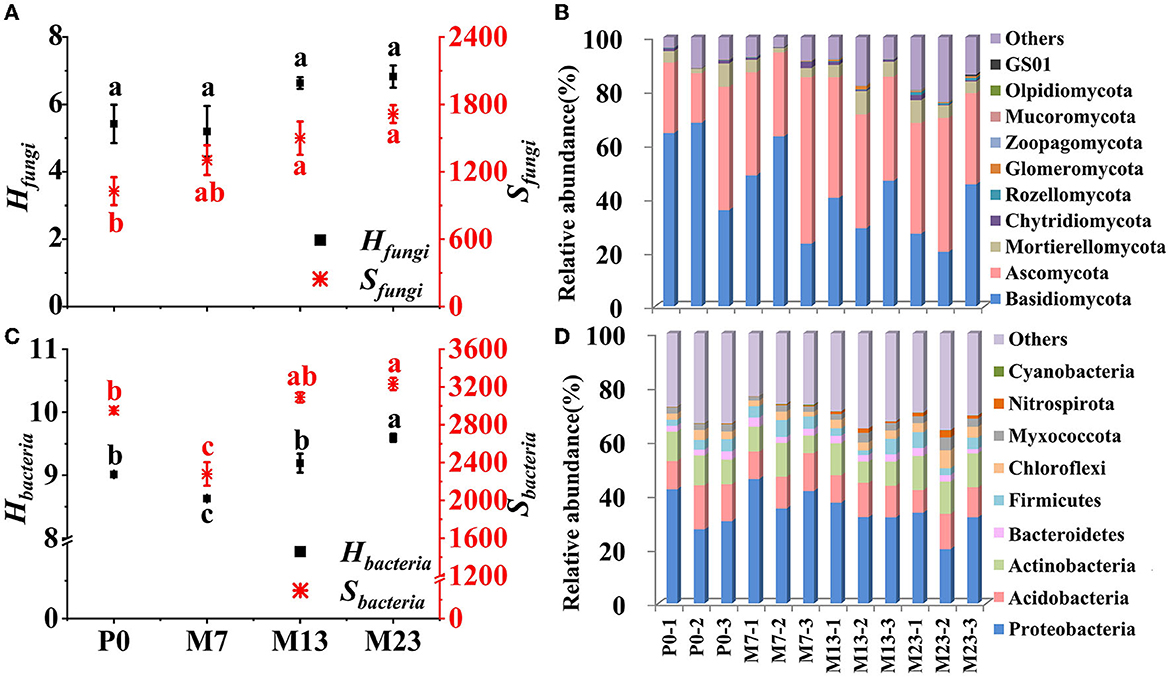
Figure 3. Indices of fungal (A) and bacterial (C) communities of different forest stands. The mean ± standard error is shown, and different letters indicate that variables are significant differences at p < 0.05 among different stand types using the LSD test. Relative abundance of dominant fungal (B) and bacterial (D) species at the phylum level. P0 is the pure native P. orientalis forest without the introduced black locust (CK, 0 years). M7, M13, and M23 are the three age classes of the mixed forest of R. pseudoacacia/P. orientalis stands, which represent three introduction chronosequence of 7, 13, and 23 years.
For the fungal community, Sfungi increased along the introduction chronosequence, whereas Hfungi did not vary significantly after the introduction of the black locust (Figure 3A). For the relative abundance of soil microorganisms in each stand, fungal communities were dominated by Basidiomycota (42.67%), Ascomycota (39.37%), and Mortierellomycota (5.04%) (relative abundance >1%) (Figure 3B). Along the introduction chronosequence, the relative abundance of Ascomycota increased, but Basidiomycota revealed a reverse trend (Supplementary Table S4). Additionally, the dominant genera were Hygrocybe and Lysurus at all forest stands, and the abundance of Hygrocybe decreased from P0 to M23. Fusarium was the dominant genus in M13 and M23 (Supplementary Figure S1a).
Sbacteria and Hbacteria of soil bacterial community were affected significantly by the introduction of black locust, with the trends decreasing initially in the early introduction years then increasing following introduction chronosequence with the lowest values in M7 (Figure 3C). The dominant phylums of bacterial community were Proteobacteria, Acidobacteria, Actinobacteria, Firmicutes, Chloroflexi, Myxococcota, and Bacteroidetes, with average proportions of 34.14%, 11.97%, 10.42%, 4.03%, 3.24%, 2.66%, and 2.52%, respectively (Figure 3D). The dominant phyla were affected by the introduction of black locust, except for Myxococcota and Bacteroidetes (Supplementary Table S4). Along the introduction chronosequence, the relative abundance of Proteobacteria, Actinobacteria, and Chloroflexi had increasing trends from M7 to M23. Conversely, the abundance of Acidobacteria decreased with the introduction of chronosequence. Pseudomonas, Lysobacter, Ramlibacter, MND1, Sphingomonas, Dongia, RB41, and Bacillus were the dominant genera in all forest stands (Supplementary Figure S1b).
NMDS analysis showed that the soil fungal and bacterial communities composition of P0 were distinct from those of M7, M13, and M23 (Figure 4). Furthermore, the soil fungal community of P0 much differed from those of M7, M13, and M23 than the bacterial community.
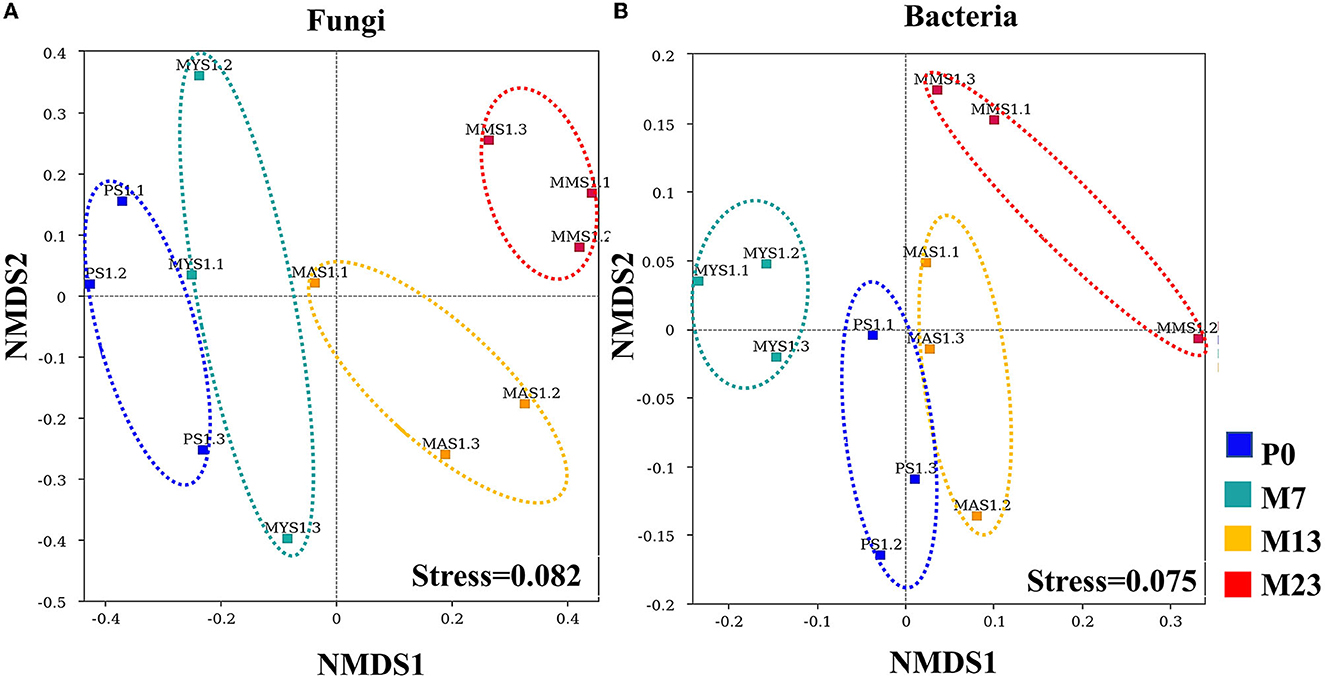
Figure 4. Plots of NMDS representation analysis of soil fungal communities (A) and bacterial communities (B) colored by different stand types. P0 is the pure native P. orientalis forest without the introduced black locust (CK, 0 years). M7, M13, and M23 are the three age classes of the mixed forest of R. pseudoacacia/P. orientalis stands, which represent three introduction chronosequence of 7, 13, and 23 years.
3.4. Ecological indices response to introduction chronosequence
Through PCA, all ecological indices were divided into four dimensions (Supplementary Table S5). The first axis explained 56% of the total proportion variance, with several variables having loadings of more than 0.8 (MBC, SOC, -N, CD, -N, TC, Hbacteria, C/N, and TP). In addition to these variables, TN, Sfungi, Hfungi, and pH were also loaded on the first axis. The second axis, third axis, and fourth axis accounted for 15%, 8%, and 6% of the total proportion variance, respectively. After further regression analysis, the results showed that CD, -N, SOC, MBC, TC, and TN were significantly affected by introduction years, with regression coefficients R2 ≥ 0.80 (Figure 5). Meanwhile, SOC, MBC, -N, TC, TN, TP, Sfungi, pH, Hbacteria, Splant, and -N varied quadratically non-linearly along the introduction chronosequence, but CD, C/N, and Hfungi varied one-dimensional linearly (Supplementary Table S6). Variables such as TP, pH, and Splant increased initially and then decreased along the introduction chronosequence, while TC and Hbacteriarevealed reverse trends. CD and Hfungiincreased with the introduction years, but C/N decreased (Figure 6).
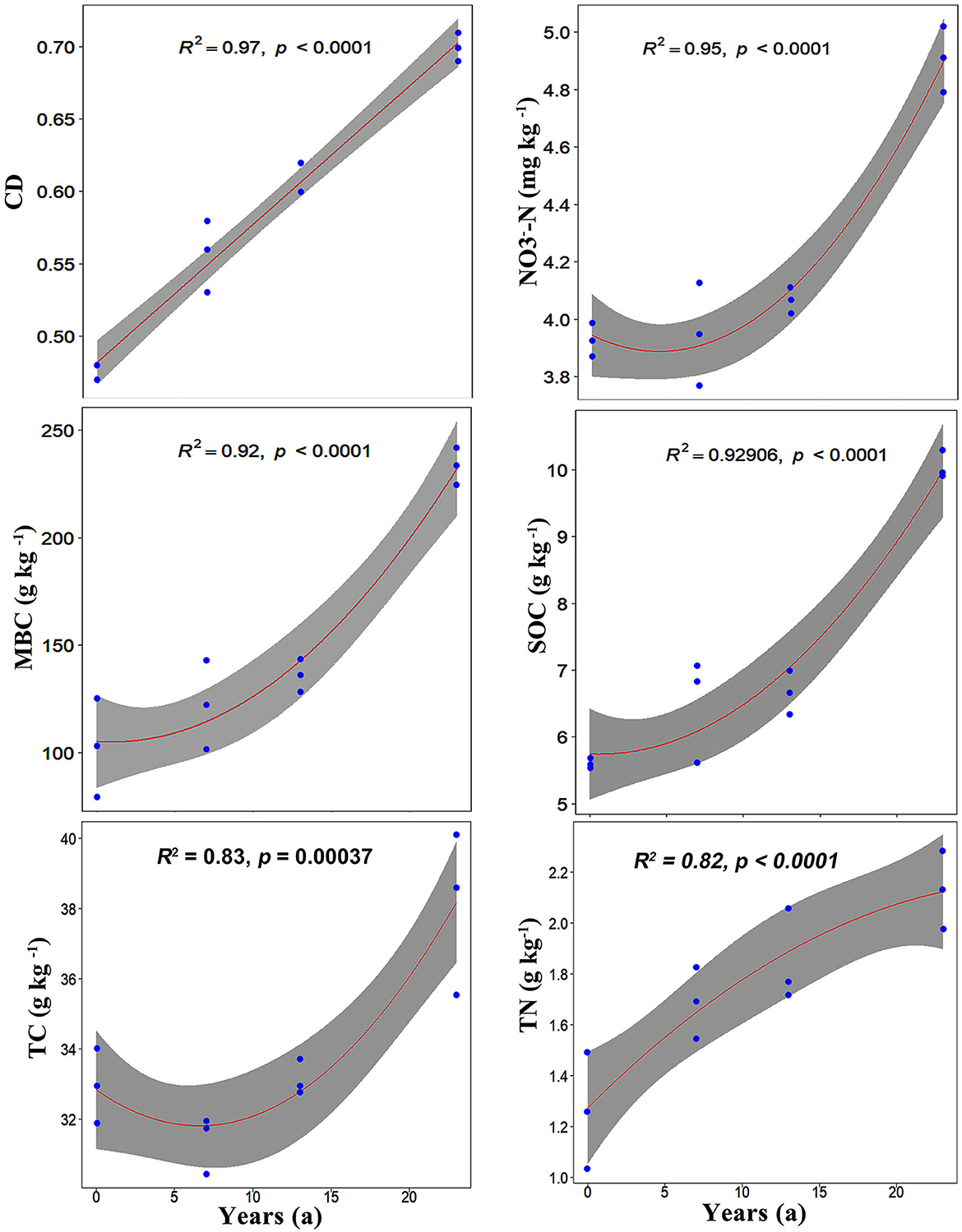
Figure 5. The relationships between ecological indices and introduction chronosequence (R2 ≥ 0.8). For all plots, the x-axis is the introduction chronosequence from 0 years to 23 years. The line in each plot represents regression model fit, and the shaded area represents its 95% confidence limits.
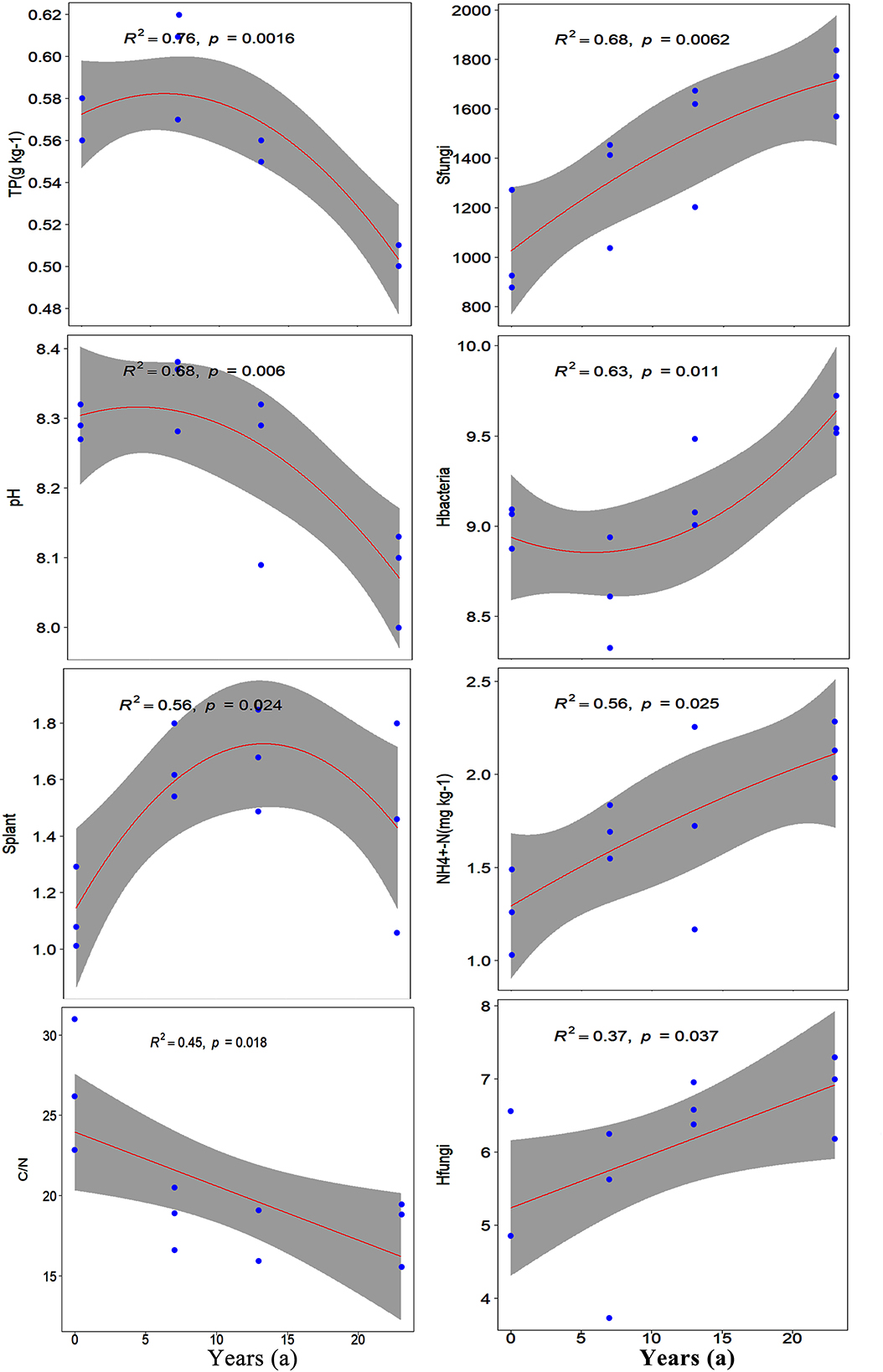
Figure 6. The relationships between ecological indices and introduction chronosequence (R2 < 0.8). For all plots, the x-axis is the introduction chronosequence from 0 years to 23 years. The line in each plot represents regression model fit, and the shaded area represents its 95% confidence limits.
3.5. The relationship among understory vegetation, soil, and microbial community
The network graph was built to explore the correlation of different variables of ecosystem components (Figure 7A). Vegetation is closely related to soil properties, such as the significant positive correlation between LB and -N, TP, -N, and SOC. Similarly, vegetation characteristics appeared to be markedly correlated to microbial communities, with Hplant being positively correlated with the Hfungi, as well as LB being positively correlated with Hfungi, Sfungi, and Hbacteria. In addition, -N and SOC were markedly positively correlated with Sfungi, but pH and TP were significantly negatively correlated with the Hbacteria, Hfungi, and Sfungi, which revealed that vegetation had important effects on soil properties and microbial communities.
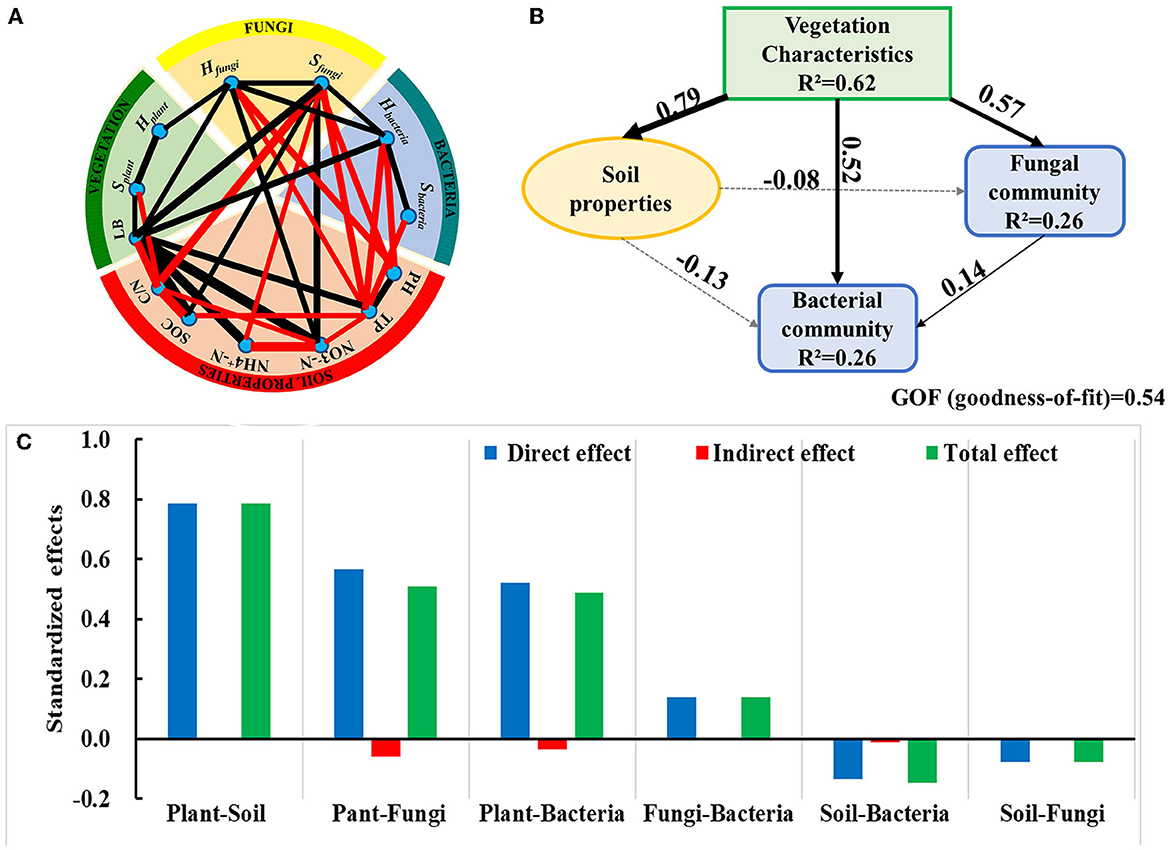
Figure 7. Network graph of the significant Pearson's correlation of different variables of ecosystem components (A) and the result of PLS-PM among the indices of ecosystem components (B). (C) Standardized direct and indirect effects obtained from the PLS-PM are presented. Positive correlations are colored by black lines, whereas negative correlations are colored by red lines, and the thicker the line, the stronger the correlation. The arrow width reflects the path coefficients. Solid lines indicate that the path coefficients are positive, and the dashed lines indicate that the path coefficients are negative. R2 is the coefficient of determination of endogenous latent variable.
The path analysis was used to further analyze the relationships among the ecosystem components, such as vegetation, soil chemistry, and microbial phyla, and showed positive response relationships except for the negative responses of soil properties on fungi and bacteria communities (Figure 7B). It also revealed that vegetation had the strongest direct influence on soil chemical properties, as well as a slightly stronger direct and indirect influence on the fungal community than on the bacterial community (Figure 7C).
4. Discussion
Our results showed black locust had effects on native ecosystems along the introduction chronosequence, as well as the strong associations between aboveground and underground on the Loess Plateau. It suggested native ecosystems were affected by afforestation efforts in China, at least from a flora, soil, and microbial perspective.
4.1. Effects on understory flora after the introduction of alien black locust
The introduction of black locust significantly increased canopy density, litter biomass, and the richness but not the diversity of the understory in our study area (Figure 2). Shrub diversity and herb richness increased to the highest values in M13, then decreased along the introduction chronosequence. However, the diversity of herbs took longer than shrubs to reach the levels where it significantly differed from pure stands with lower values in M23. Other studies also found that black locust formed markedly specific understory vegetation communities, which differ from the native dominant species (Vítková and Kolbek, 2010; Sitzia et al., 2012). The shifts in understory species composition may be from changes in moisture, temperature, and light conditions by the growth of black locust (Liu et al., 2018b; Dyderski and Jagodziński, 2019b). In the early stages of introduction, superior living conditions, such as adequate light conditions and less competition, led to the better growth of vegetation, which caused a higher richness of herbs and higher diversity of shrubs (Xu et al., 2020). Subsequently, the highest Hshrub, Sshrub, and Sherbvalues were found in M13, which is due to the moderate light intensity and the best forest microhabitat created by the vertical laminated structure of mixed forest stands. However, the growth of black locust and canopy density gradually increased over time, causing lower light conditions and intense interspecific competition over growing space for herbaceous and shrub layers. As a result, in the later stages of introduction, some sun-loving and drought-tolerant undergrowth (such as Agropyron cristatum and Lespedeza cuneata) were replaced gradually by shade-tolerant, moist-loving species (such as Aster tataricus, Fragaria vesca, and Rubus innominatus), leading to the decrease in Hshrub, Sshrub, Hherb,and Sherb (Wei et al., 2013; Kou and Jiao, 2016). The dominant herbaceous species changed gradually from Tripogon chinensis and Carex lanceolata to Aster tataricus and Patrinia scabiosifolia, and shrubs species from Lespedeza cuneata to Rubus parvifolius. Additionally, the ecotypes transformed xerophytes into mesophytes from P0 to M23 (Kirkham, 2005; Li, 2009), which also indicated that the microclimate was changed with the introduction time. In addition, Dyderski and Jagodziński (2019a) showed that black locust forests favored the occurrence of alien species in the undergrowth, but we did not find non-native species of understory that invaded this area, which may be due to the differences in forest age class, afforestation history, natural environment, and so on.
4.2. Effects on soil properties after the introduction of alien black locust
Soil properties were affected in our study area after the introduction of alien black locust except for BD and SM (Table 2). Changes in soil properties associated with carbon appeared significantly higher in mixed stands forests compared with pure stands after 7 years of introduction. Nitrogen-related soil properties and pH might take longer to change, with levels not showing significant differences from pure stands until the introduction year of 13 (-N) or 23 (TN, -N, pH). As the forest age class increased, the content of soil carbon and nitrogen increased after the introduction of black locust, but pH decreased (Figures 5, 6), which is in line with the findings by Khan et al. (2010), who found that the leaves of black locust improved the content of soil carbon and nitrogen and decreased soil pH. The higher total nitrogen content under black locust forest was related to litter input and nitrogen-fixing bacteria (Medina-Villar et al., 2016; Anna et al., 2023). Moreover, rhizobia secrete some organic nitrogen into the soil during their lifetime, and the root nodules fall off, thus greatly improving the soil fertility (Babu et al., 2023). It is generally accepted that a nitrogen-fixing species has immediate effects on the N pool in the soils. However, this effect seems to have been delayed for a long time based on our research. This may be because the black locust could not only maintain its needs by nitrogen fixation but also retain most of the soil nitrogen for its root and plant growth of the associated tree species P. orientalis (Zhai et al., 1991a; Zhang and Chang, 2006). In the initial introduction stage, black locust was younger with lower nitrogen fixation capacity, and the fixed nitrogen may be used for the growth of R. pseudoacacia and P. orientalis. With the increase in the introduction chronosequence, the nitrogen fixation capacity of R. pseudoacacia increased gradually, as well as the amount of nitrogen (Jiang et al., 2015). In the later stage of the black locust introduction, there was a surplus of soil nitrogen after being utilized by the growth of associated tree species P. orientalis, so the soil nitrogen content increased significantly. In addition, the significantly higher -N than -N may be because of the high rate of nitrification and soil organisms ability to easily absorb -N (Hou et al., 2015). Leaves of black locust contain more phosphorus than that of P. orientalis (Zhai et al., 1991b), and higher phosphorus leaf litter decomposition resulted in a higher TP content of M7 than that of P0. However, the growing root system with more nitrogen-fixing rhizobia of R. pseudoacacia and the survival and reproduction of plentiful microbes consumed more phosphorus (Carl et al., 2018), leading to the decreasing trends of TP after 13 years of black locust introduction. Liu et al. (2018b) found the decreasing trends of soil available phosphorus was related to Hbacteria in the pure over-mature forest of black locust and inferred phosphorus may be the limit for the diversity of soil bacterial groups in mixed over-mature black locust forest.
4.3. Effects on soil microbial community after the introduction of alien black locust
Similarly, the introduction of black locust affected the properties of the soil microbial community in our study, such as Sfungi, Sbacteria, and Hbacteria except for Hfungi (Figures 3A, C). The increasing trends of Sfungi and Hbacteria, indices after 7 years of introduction were correlated to litter biomass (Figure 7A). The undergrowth of vegetation and accumulation of litter biomass always lead to more inputs of nutrients to accelerate microbial reproduction (Wang et al., 2010; Vilà et al., 2011). The lowest Hbacteria and Sbacteria in M7 suggested that in the early stages of introduction, some bacteria species fail to adapt to the new changing environment (Bani et al., 2018). Different patterns of species diversity and richness between fungal and bacterial communities changed along the introduction chronosequence suggesting an incongruous process between fungi and bacteria succession.
The dominant fungal and bacterial groups were affected significantly after the introduction of black locust, especially Basidiomycota, Ascomycota, Proteobacteria, and Acidobacteria (Supplementary Table S4). This result was consistent with previous studies in pure black locust forests during the afforestation time (Liu et al., 2018b; Yang et al., 2019b). Compared with the native forest of P. orientalis stands, Ascomycota were higher in mixed forest stands, but Basidiomycota showed a reverse trend (Supplementary Table S4). The transition of dominant phyla from oligotrophic Basidiomycota-dominant to copiotrophic Ascomycota-dominant community means that soil nutrients and resource availability have been greatly improved after the introduction of alien black locust (Yang et al., 2019b). Proteobacteria are commonly considered copiotrophic bacteria, and their abundance increase in nutrient-rich conditions, while Acidobacteria belong to an oligotrophic group, and their abundance decrease in nutrient-rich conditions (Jangid et al., 2008; Zhang C. et al., 2016). In addition, the relative abundance of Proteobacteria has increased gradually as introduction time increased, whereas that of Acidobacteria showed the opposite trend (Supplementary Table S4). These results further indicated that the introduction of black locust increased the soil nutrient conditions. Moreover, light could affect the metabolism of Acidobacteria all the time (Xu et al., 2020), so increased understory growth may negatively affect them. This may be the reason why Acidobacteria reduced after the introduction of black locust. Due to the abundance of Actinobacteria being positively related to soil carbon and nitrogen contents, they were considered copiotrophic bacteria in some forest soils (Eilers et al., 2010; Nemergut et al., 2011; Ramirez et al., 2012). We also found an increased abundance of Actinobacteria after the introduction of black locust (Supplementary Table S4), which was paralleled by the content of soil carbon and nitrogen. Actinobacteria may have a potential function in carbon and nitrogen cycling in forest soils (Navarrete et al., 2015). Some Chloroflexi have been found to participate in the process of cellulose degradation (Cole et al., 2013; Podosokorskaya et al., 2013) and in the oxidation of (Sorokin et al., 2012, 2014), so the increasing abundance of Chloroflexi may be related to litter decomposition and nitrification in our study area (Figure 3D). In addition, Lysobacter, Ramlibacter, and Bacillus were found in the black locust forest of the Yellow River Delta; Sphingomonas was related to nitrogen fixation; Pseudomonas and Bacillus were related to phosphate-solubilizing microorganisms (Ding et al., 2017).
4.4. Aboveground–underground associations
Ecosystem structure and function strongly relied on key associations between aboveground and underground ecological indices (Kardol and Wardle, 2010). Our results showed significant correlations among the ecosystem indices of vegetation, soil, fungi, and bacteria communities in our study area (Figure 8).
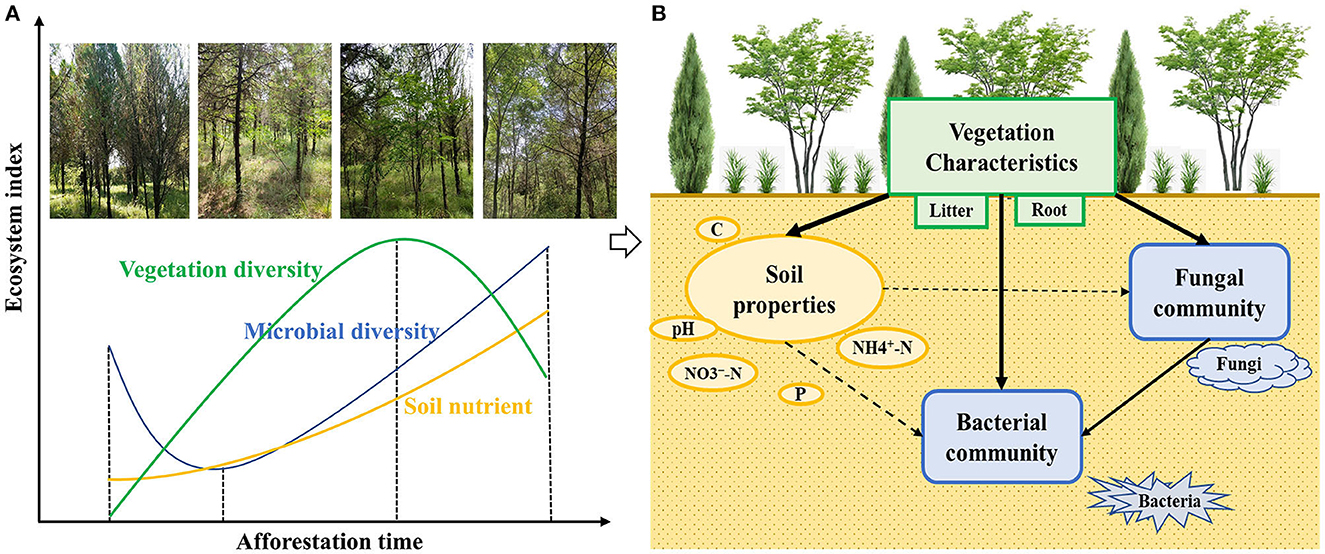
Figure 8. Multiple effects were caused by alien black locust (A) and conceptual model of aboveground–underground associations (B).
Vegetation appeared to have the strongest influence on soil properties after the introduction of black locust and the significant correlations between litter biomass and soil carbon and nitrogen content in this study (Figure 7B). It may be the introduction of black locust that led to the changes of the microenvironment and understory composition initially, and later had strong far-reaching effects on soil properties through the multiple litters and plant roots (Santos et al., 2016; Reynolds et al., 2017). Black locust, as a leguminous species, has N2-fixing capacity and could considerably improve the nitrogen content in soil through the action of root and rhizobia. Additionally, the increase in vegetation communities would affect the microorganisms by accelerating litter decomposition and root biomass accumulation and providing enough energy and nutrients for microbial growth (Zhang Y. et al., 2016).
The vegetation community had stronger effects on the soil microbial community (Figure 7B), which suggested the close associations between aboveground and underground ecosystems (Xu et al., 2020). Vegetation diversity was correlated to fungi diversity rather than bacterial diversity (Figure 7A), suggesting that the diversity of vegetation may have more effects on the diversity of fungi than bacteria, which was consistent with the result of Liu et al. (2018b) and Xu et al. (2020). The reason may be that fungal communities comprise various groups such as pathogens and reciprocal symbiosis fungi (such as ectomycorrhizal and endophytic mycorrhizal fungi) and interact more with the host plant species than bacterial communities under the same environmental factors (Yang et al., 2017a). Moreover, soil fungal pathogens could recruit dominant species and adjust their survival rate to maintain the diversity of the vegetation communities, while fungal reciprocal symbiosis could offer nutrients and protection to benefit the host plants (Lei et al., 2020). Significant correlation between LB and Hfungi, Sfungi, and Hbacteria further indicated that litter is one of the nutrient resources of microbial communities and has significant effects on the richness and diversity of fungi and bacteria communities (Yang et al., 2019b; Zeng et al., 2019).
Soil properties had effects on the richness and diversity of soil fungi and bacterial communities, such as significant positive relationships between SOC and Sfungi, -N, and Sfungi and Hfungi (Figure 7A). The reason is that SOC and -N provided more available nutrition resources for fungal communities during the litter decomposition (Zhang Y. et al., 2016). Labile carbon is an energy source for soil microfauna and a key factor in maintaining soil microbial biodiversity (Babu et al., 2020; Singh et al., 2020). Merrick et al. (1995) and Marzluf (1997) reported soil nitrate N was the preferable N resource for microbes and influenced microbial community richness. The availability of N forms and more nutrition resources activate the fungi community, leading to the increasing diversity of fungi. Furthermore, pH was significantly negatively correlated with bacterial diversity, fungal diversity, and fungal abundance (Figure 7A), suggesting that the environment with the decrease of pH over time of black locust introduction appeared to benefit microbial communities, so there were increasing trends of bacterial diversity, fungal diversity, and richness in mixed forest stands. Xu et al. (2020) thought black locust would provide a more optimal living environment for copiotrophs. Phosphorus is a key chemical element and is related to rRNA synthesis with the growth of soil microbes. Liu et al. (2018b) found available phosphorus had more potent effects on the composition of bacterial phyla, especially for α-proteobacteria. However, TP was found to be significantly negatively correlated with bacterial diversity, fungal diversity, and fungal abundance in this study (Figure 7A). An explanation for this result is that the increasing microbial communities demanded lots of soil available phosphorus for their growth and development, as well as their metabolisms for producing phosphatases as litter decomposition progresses (Manzoni et al., 2012; Hacker and Oelmann, 2015; Fatemi et al., 2016; Luo et al., 2017). Moreover, the lower abundance of phosphorus-solubilizing microorganisms, such as Pseudomonas, Bacillus, and Fusarium, indicated there was lower available phosphorus in M23 (Supplementary Figure S1). Consequently, we inferred that after the introduction of black locust for many years, the progressively decreasing phosphorus content may restrict the growth and development of soil microorganisms.
In short, the introduction of the black locust after 13 years had negative effects on shrub diversity, herb diversity, and herb richness on the Loess Plateau (Figure 8). Even though there were positive effects on soil nutrients associated with carbon and nitrogen except for phosphorus, as well as microbial diversity and richness, it has been proved that in pure black locust forest with age class from mature to over-mature, soil nutrients and microbial diversity declined (Liu et al., 2018b). The inconsistent results were probably due to the different age classes of black locust forests. Therefore, whether the effects of the introduction of black locust on soil nutrients and microorganisms are positive or negative remains to be further studied in the future.
Additionally, the stronger correlations and influences among vegetation, soil properties, and microbial communities indicated stronger aboveground and underground associations in this study area. Vegetation, the main driving force, affects the structure and composition of soil microbial communities by changing species composition, litter biomass, soil properties, root-soil system components, and other factors (Hacker and Oelmann, 2015; Zhang C. et al., 2016). Soil microorganisms are the link between aboveground and underground components of the ecosystem and play an important role in forest succession and nutrient cycle (Li et al., 2013; Kou and Jiao, 2016). This kind of association among vegetation–soil–microbes can be used for other ecosystems (Reynolds et al., 2017), and the root is also a key and non-negligible component for studying aboveground and underground associations in the forest ecosystem.
5. Conclusion
These results clarified that the introduction of black locust had significant effects on plant communities, soil properties, and soil microbial communities in native forest stands, such as the non-linear trends of the richness and diversity indices of understory vegetation and soil microbial communities along the afforestation chronosequence. Furthermore, the strong correlations among vegetation, soil properties, and soil microbiome suggested that there were remarkable aboveground–underground associations and that vegetation, as the main driving force, had far-reaching effects on soil properties and soil microbial communities. In short, these results will contribute to the assessments and management of black locust plantations around the world and provide insights into biological invasion and its conservation theories.
Data availability statement
The datasets presented in this study can be found in online repositories. The name of the repository and accession number can be found below: NCBI; PRJNA979340.
Author contributions
YK and LZ conceived this research and designed all experiments. LZ, CW, HL, GL, and HZ performed the experiments. KK, ML, XZ, and GM helped with the investigation work and collection of samples. LZ analyzed experimental data and drafted the manuscript. YK gave comments and suggestions for this article. All authors contributed to the article and approved the submitted version.
Funding
The authors are grateful for the funds of Technology and Demonstration of Ecological Protection for Gully Building and Protection and Quality and Efficiency Improvement of Apple Industry in Gully Region of Loess Plateau (2016YFC0501706-01).
Conflict of interest
The authors declare that the research was conducted in the absence of any commercial or financial relationships that could be construed as a potential conflict of interest.
Publisher's note
All claims expressed in this article are solely those of the authors and do not necessarily represent those of their affiliated organizations, or those of the publisher, the editors and the reviewers. Any product that may be evaluated in this article, or claim that may be made by its manufacturer, is not guaranteed or endorsed by the publisher.
Supplementary material
The Supplementary Material for this article can be found online at: https://www.frontiersin.org/articles/10.3389/fevo.2023.1210498/full#supplementary-material
References
Anna, D. M., Pasquale, N., Speranza, C. P., Valeria, M., Lucia, S., Anna, G. R., et al. (2023). Combined effect of black locust invasion and fire on soils of Mediterranean shrublands and pine forests. CATENA, 220, 6656. doi: 10.1016/j.catena.2022.106656
Babu, S., Mohapatra, K. P., Yadav, G. S., Lal, R., and Kumar, A. (2020). Soil carbon dynamics in diverse organic land use systems in North Eastern Himalayan ecosystem of India. Catena 194, 104785. doi: 10.1016/j.catena.2020.104785
Babu, S., Singh, R., Avasthe, R., Kumar, S., Rathore, S. S., Singh, V. K., et al. (2023). Soil carbon dynamics under organic farming: Impact of tillage and cropping diversity. Ecol. Indic. 147, 109940. doi: 10.1016/j.ecolind.2023.109940
Bani, A., Pioli, S., Ventura, M., Panzacchi, P., Borruso, L., Tognetti, R., et al. (2018). The role of microbial community in the decomposition of leaf litter and deadwood. Appl. Soil Ecol. S0929139317311204. doi: 10.1016/j.apsoil.2018.02.017
Bardgett, R., and Wardle, D. A. (2013). Aboveground-belowground linkages: biotic interactions, ecosystem processes, and global change. Eos Transact. Am. Geophys. Union 92, 222–222. doi: 10.1029/2011EO260011
Benesperi, R., Giuliani, C., Zanetti, S., Gennai, M., Lippi, M. M., Guidi, T., et al. (2012). Forest plant diversity is threatened by Robinia pseudoacacia (black-locust) invasion. Biodiv. Conserv. 21, 3555–3568. doi: 10.1007/s10531-012-0380-5
Bokulich, N. A., and Mills, D. A. (2013). Improved selection of internal transcribed spacer-specific primers enables quantitative, ultrahigh-throughput profiling of fungal communities. Appl. Environ. Microbiol. 79, 2519–2526. doi: 10.1128/Aem.03870-12
Carl, C., Peter, B., Maik, V., Dirk, L., and Hans, P. (2018). Key drivers of competition and growth partitioning among Robinia pseudoacacia L. trees. For. Ecol. Manage. 430, 86–93. doi: 10.1016/j.foreco.2018.08.002
Chang-Seok L. Hyun-Je C. and Hoonbok, Y. (2004). Stand dynamics of introduced black locust (Robinia pseudoacacia L.) plantation under different disturbance regimes in Korea—sciencedirect. For. Ecol. Manage. 189, 281–293. doi: 10.1016/j.foreco.2003.08.012
Chen, C. G., Fischer, A., Herrmann, W., and Yang, P. H. (2007). Flora of the Loess Plateau in Central China. IHW Verlag.
Cole, J. K., Gieler, B. A., Heisler, D. L., Palisoc, M. M., Williams, A. J., Dohnalkova, A. C., et al. (2013). Kallotenue papyrolyticum gen. nov., sp. nov., a cellulolytic and filamentous thermophile that represents a novel lineage (Kallotenuales ord. nov., Kallotenuaceae fam. nov.) within the class Chloroflexia. Int. J. Sys. Evolut. Microbiol., 63(Pt 12), 4675–4682. doi: 10.1099/ijs.0.053348-0
Ding, X., Jing, R., Huang, Y., Chen, B., and Fengyun, M. A. (2017). Bacterial structure and diversity of rhizosphere and bulk soil of robinia pseudoacacia Forests in Yellow river delta. Acta Pedol. Sinica 54, 1293–1302. doi: 10.11766/trxb201703230510
Dyderski, M. K., and Jagodziński, A. (2019a). Context-dependence of urban forest vegetation invasion level and alien species' ecological success. Forests 10, 26. doi: 10.3390/f10010026
Dyderski, M. K., and Jagodziński, A. (2019b). similar impacts of alien and native tree species on understory light availability in a temperate forest. Forests 10, 951. doi: 10.3390/f10110951
Edgar, R. (2013). UPARSE: highly accurate OTU sequences from microbial amplicon reads. Nat. Methods 10, 996–998. doi: 10.1038/nmeth.2604
Eilers, K. G., Lauber, C. L., Knight, R., and Fierer, N. (2010). Shifts in bacterial community structure associated with inputs of low molecular weight carbon compounds to soil. Soil Biol. Biochem. 42, 896–903. doi: 10.1016/j.soilbio.2010.02.003
Essl, F., Milasowszky, N., and Dirnb, C. k. T. (2011). Plant invasions in temperate forests: Resistance or ephemeral phenomenon? Basic Appl. Ecol. 12, 1–9. doi: 10.1016/j.baae.2010.10.003
Fatemi, F. R., Fernandez, I. J., Simon, K. S., and Dail, D. B. (2016). Nitrogen and phosphorus regulation of soil enzyme activities in acid forest soils. Soil Biol. Biochem. 98, 171–179. doi: 10.1016/j.soilbio.2016.02.017
Goodness, J., Andersson, E., Anderson, P., and Elmqvist, T. (2016). Exploring the links between functional traits and cultural ecosystem services to enhance urban ecosystem management. Ecol. Indic. 70, 597–605. doi: 10.1016/j.ecolind.2016.02.031
Hacker, N., and Oelmann, Y. (2015). Plant diversity shapes microbe-rhizosphere effects on P mobilisation from organic matter in soil. Ecol. Lett. 18, 1356–1365. doi: 10.1111/ele.12530
Hou, Y., Liu, L., Chu, H., Ma, S. J., Zhao, D., and Liang, R. (2015). Effects of exotic plant Rhus typhina invasion on soil properties in different forest types. Acta Ecol. Sinica 35, 5324–5330. doi: 10.5846/stxb201404160740
Jangid, K., Williams, M. A., Franzluebbers, A. J., Sanderlin, J. S., Reeves, J. H., Jenkins, M. B., et al. (2008). Relative impacts of land-use, management intensity and fertilization upon soil microbial community structure in agricultural systems. Soil Biol. Biochem. 40, 2843–2853. doi: 10.1016/j.soilbio.2008.07.030
Jiang, L., Xun, S., Sun, J., and University, S. A. (2015). Measurement and usage of Robinia pseudoacacia's nitrogen fixation ability. J. Shandong Fores. Sci. Technol. 45, 4–7.
Jiang, Y., Wang, W., Xie, Q., Liu, N., Liu, L., Wang, D., et al. (2017). Plants transfer lipids to sustain colonization by mutualistic mycorrhizal and parasitic fungi. Science 2017, 356. doi: 10.1126/science.aam9970
Kardol, P., and Wardle, D. A. (2010). How understanding aboveground-belowground linkages can assist restoration ecology RID A-2600-2010. Trends Ecol. Evol. 25, 670–679. doi: 10.1016/j.tree.2010.09.001
Khan, B., Ablimit, A., Mahmood, R., and Qasim, M. (2010). Robinia pseudoacacia leaves improve soil physical and chemical properties. J. Arid. Land, 2, 266–271. doi: 10.3724/SP.J.1227.2010.00266
Kirkham, M. B. (2005). Leaf anatomy and leaf elasticity. Princ. Soil Plant Water Relat. 13, 357–378. doi: 10.1016/B978-012409751-3/50021-9
Kleinbauer, I., Dullinger, S., Peterseil, J., and Essl, F. (2010). Climate change might drive the invasive tree Robinia pseudacacia into nature reserves and endangered habitats. Biol. Conserv. 143, 382–390. doi: 10.1016/j.biocon.2009.10.024
Kou, G., and Jiao, J. Y. (2016). The effect of Robinia pseudoacacia afforestation on soil and vegetation properties in the Loess Plateau (China): a chronosequence approach. Forest Ecol. Manag. 375, 146–158. doi: 10.1016/j.foreco.2016.05.025
Laiolo, P., Caprio, E., and Rolando, A. (2003). Effects of logging and non-native tree proliferation on the birds overwintering in the upland forests of north-western Italy. For. Ecol. Manage. 179, 441–454. doi: 10.1016/S0378-1127(02)00542-X
Lazzaro, L., Mazza, G., D'Errico, G., Fabiani, A., Giuliani, C., Inghilesi, A. F., et al. (2017). How ecosystems change following invasion by Robinia pseudoacacia: insights from soil chemical properties and soil microbial, nematode, microarthropod and plant communities. Sci. Total Environ. 622-623, 1509–1518. doi: 10.1016/j.scitotenv.2017.10.017
Lazzaro, M., d'Errico, F., Giuliani, I. A. F, and Lagomarsino, L. L. (2018). How ecosystems change following invasion by Robinia pseudoacacia: Insights from soil chemical properties and soil microbial, nematode, microarthropod and plant communities. Sci. Total Environ. 4, 17.
Lei, C., Swenson, N. G., Niuniu, J., Xiangcheng, M., Haibao, R., Liangdong, G., et al. (2020). Differential soil fungus accumulation and density dependence of trees in a subtropical forest. Science 366, 124–128. doi: 10.1126/science.aau1361
Li, D. W. (2009). Floristic Geography of the Loess Plateau in Northern Shaanxi. Translated from Chinese by. Yangling: Northwest University of agriculture and Forestry Press.
Li, J. J., Zheng, Y. M., Yan, J. X., Li, H. J., and He, J. Z. (2013). Succession of plant and soil microbial communities with restoration of abandoned land in the Loess Plateau, China. J. Soils Sedi. 13, 760–769. doi: 10.1007/s11368-013-0652-z
Liang, H., Xue, Y., Li, Z., Shuai, W., and Fu, B. (2018). Soil moisture decline following the plantation of Robinia pseudoacacia forests: Evidence from the Loess Plateau. For. Ecol. Manage. 412, 62–69. doi: 10.1016/j.foreco.2018.01.041
Liu, J. L., Dang, P., Gao, Y., Zhu, H. L., and Zhao, Z. (2018a). Effects of tree species and soil properties on the composition and diversity of the soil bacterial community following afforestation. For. Ecol. Manage. 427, 342–349. doi: 10.1016/j.foreco.2018.06.017
Liu, J. L., Yang, Z. L., Dang, P., Zhu, H. L., and Zhao, Z. (2018b). Response of soil microbial community dynamics to Robinia pseudoacacia L. afforestation in the loess plateau: a chronosequence approach. Plant Soil 423, 327–338. doi: 10.1007/s11104-017-3516-2
Luo, Y., Zang, H., Yu, Z., Chen, Z., Gunina, A., Kuzyakov, Y., et al. (2017). Priming effects in biochar enriched soils using a three-source-partitioning approach: 14C labelling and 13C natural abundance. Soil Biol. Biochem. 4, 6. doi: 10.1016/j.soilbio.2016.12.006
Macedo, M. O., Resende, A. S., Garcia, P. C., Boddey, R. M., Jantalia, C. P., Urquiaga, S., et al. (2008). Changes in soil C and N stocks and nutrient dynamics 13 years after recovery of degraded land using leguminous nitrogen-fixing trees. For. Ecol. Manage. 255, 1516–1524. doi: 10.1016/j.foreco.2007.11.007
Manzoni, S., Taylor, P., Richter, A., Porporato, A., and Gren, G. I. (2012). Environmental and stoichiometric controls on microbial carbon-use efficiency in soils. New Phytol. 196, 79. doi: 10.1111/j.1469-8137.2012.04225.x
Marco, A. D., Arena, C., Giordano, M., and Santo, A. (2013). Impact of the invasive tree black locust on soil properties of Mediterranean stone pine-holm oak forests. Plant Soil 372, 473–486. doi: 10.1007/s11104-013-1753-6
Marzluf, G. A. (1997). Genetic regulation of nitrogen metabolism in the fungi. Microbiol. Mol. Biol. Rev. 61, 17–32. doi: 10.1128/.61.1.17-32.1997
Medina-Villar, S., Rodríguez-Echeverría, S., Lorenzo, P., Alonso, A., Pérez-Corona, E., and Castro-Díez, P. (2016). Impacts of the alien trees Ailanthus altissima (Mill.) Swingle and Robinia pseudoacacia L. on soil nutrients and microbial communities. Soil Biol. Biochem. 96, 65–73. doi: 10.1016/j.soilbio.2016.01.015
Merrick, M., and Edwards, J. R. A. (1995). Nitrogen Control in Bacteria. Microbiol. Rev. 5, 622. doi: 10.1128/mr.59.4.604-622.1995
Mori, H., Maruyama, F., Kato, H., Toyoda, A., Dozono, A., Ohtsubo, Y., et al. (2014). Design and experimental application of a novel non-generate universal primer set that amplifies prokaryotic 16S rRNA genes with a low possibility to amplify eukaryotic rRNA genes. DNA Res. 21, 217–227. doi: 10.1093/dnares/dst052
Motta, R., Nola, P., and Berretti, R. (2009). The rise and fall of the black locust (Robinia pseudoacaciaL.) in the “Siro Negri” Forest Reserve (Lombardy, Italy): lessons learned and future uncertainties. Ann. For. Sci. 66, 410–410. doi: 10.1051/forest/2009012
Navarrete, A. A., Tsai, S. M., Mendes, L. W., Faust, K., Hollander, M., Cassman, N. A., et al. (2015). Soil microbiome responses to the short-term effects of Amazonian deforestation. Mol. Ecol. 45, 13172. doi: 10.1111/mec.13172
Nemergut, D. R., Cleveland, C. C., Wieder, W. R., Washenberger, C. L., and Townsend, A. R. (2011). Plot-scale manipulations of organic matter inputs to soils correlate with shifts in microbial community composition in a lowland tropical rain forest. Soil Biol. Biochem. 42, 2153–2160. doi: 10.1016/j.soilbio.2010.08.011
Papaioannou, A., Chatzistathis, T., Papaioannou, E., and Papadopoulos, G. (2016). Robinia pseudoacacia as a valuable invasive species for the restoration of degraded croplands. Catena 137, 310–317. doi: 10.1016/j.catena.2015.09.019
Podosokorskaya, O. A., Bonch-Osmolovskaya, E. A., Novikov, A. A., Kolganova, T. V., and Kublanov, I. V. (2013). Ornatilinea apprima gen. nov., sp. nov., a cellulolytic representative of the class Anaerolineae. Int. J. Sys. Evolut. Microbiol. 63(Pt 1), 86–92. doi: 10.1099/ijs.0.041012-0
R Core Team. (2021). R: A Language and Environment for Statistical Computing. R Foundation for Statistical Computing, Vienna, Austria. Available online at: https://www.R-project.org/
Ramirez, K. S., Craine, J. M., and Fierer, N. (2012). Consistent effects of nitrogen amendments on soil microbial communities and processes across biomes. Glob. Chang. Biol. 18, 1918–1927. doi: 10.1111/j.1365-2486.2012.02639.x
Reinhart, K. O., Dangi, S. R., and Vermeire, L. T. (2016). The effect of fire intensity, nutrients, soil microbes, and spatial distance on grassland productivity. Plant Soil 409, 203–216. doi: 10.1007/s11104-016-2957-3
Reynolds, H. L., Miller, A. J., Ferguson, J. K., and Blumenthal (2017). Effects of between-site variation in soil microbial communities and plant-soil feedbacks on the productivity and composition of plant communities. J. Appl. Ecol. 54, 1028–1039. doi: 10.1111/1365-2664.12937
Rice, S. K., and Federici, W. R. (2004). Impacts of the exotic, nitrogen-fixing black locust (Robinia pseudoacacia) on nitrogen-cycling in a pine–oak ecosystem. Plant Ecol. 174, 97–107. doi: 10.1023/B:VEGE.0000046049.21900.5a
Santos, F., Nadelhoffer, K., and Bird, J. A. (2016). Rapid fine root C and N mineralization in a northern temperate forest soil. Biogeochemistry 128, 187–200. doi: 10.1007/s10533-016-0202-z
Singh, R., Babu, S., Avasthe, R. K., Yadav, G. S., and Chandra, P. (2020). Crop productivity, soil health, and energy dynamics of Indian Himalayan intensified organic maize-based systems. Int. Soil Water Conserv. Res. 4, 3. doi: 10.1016/j.iswcr.2020.11.003
Sitzia, T., Campagnaro, T., Dainese, M., and Cierjacks, A. (2012). Plant species diversity in alien black locust stands: a paired comparison with native stands across a north-Mediterranean range expansion. For. Ecol. Manage. 285, 85–91. doi: 10.1016/j.foreco.2012.08.016
Sorokin, D. Y., Lücker, S., Vejmelkova, D., Kostrikina, N. A., Kleerebezem, R., Rijpstra, W. I. C., et al. (2012). Nitrification expanded: discovery, physiology and genomics of a nitrite-oxidizing bacterium from the phylum Chloroflexi. Isme. J. 6, 2245. doi: 10.1038/ismej.2012.70
Sorokin, D. Y., Vejmelkova, D., Lucker, S., Streshinskaya, G. M., Rijpstra, W., Damste, J. S., et al. (2014). Nitrolancea hollandica gen. nov., sp. nov., a chemolithoautotrophic nitrite-oxidizing bacterium isolated from a bioreactor belonging to the phylum Chloroflexi. Int. J. Sys. Evolut. Microbiol. 64(Pt 6), 2232. doi: 10.1099/ijs.0.062232-0
Vilà, M., Espinar, J. L., Hejda, M., Hulme, P. E., and Pyek, P. (2011). Ecological impacts of invasive alien plants: A meta-analysis of their effects on species, communities and ecosystems. Ecol. Lett. 14, 702–708. doi: 10.1111/j.1461-0248.2011.01628.x
Vítková, M., and Kolbek, J. (2010). Vegetation classification and synecology of Bohemian Robinia pseudacacia stands in a Central European context. Phytocoenologia 40, 205–241. doi: 10.1127/0340-269X/2010/0040-0425
Vítková, M., Müllerová, J., Sádlo, J., Pergl, J., and Pyšek, P. (2017). Black locust (Robinia pseudoacacia) beloved and despised: a story of an invasive tree in Central Europe. For. Ecol. Manage. 384, 287–302. doi: 10.1016/j.foreco.2016.10.057
Vítková, M., Pergl, J., and Sádlo, J. (2016). Black locust: from global ecology to local management – a case study from the Czech RepublicIntroduced tree species in European forests. Opport. Challen. 2016, 306.
Wang, Q., Garrity, G. M., Tiedje, J. M., and Cole, J. R. (2007). Naive Bayesian classifier for rapid assignment of rRNA sequences into the new bacterial taxonomy. Appl. Environ. Microbiol. 73, 5261–5267. doi: 10.1128/AEM.00062-07
Wang, Y., Shao, M., and Liu, Z. (2010). Large-scale spatial variability of dried soil layers and related factors across the entire Loess Plateau of China. Geoderma 159, 99–108. doi: 10.1016/j.geoderma.2010.07.001
Wei, X. H., Liu, Q. L., Guo, X. M., and Zhang, N. (2013). Restoring ecosystem carbon sequestration through afforestation: a sub-tropic restoration case study. Forest Ecol. Manag. 300, 60–67. doi: 10.1016/j.foreco.2012.06.018
Xu, M., Gao, D., Fu, S., Lu, X., and Feng, Y. (2020). Long-term effects of vegetation and soil on the microbial communities following afforestation of farmland with Robinia pseudoacacia plantations. Geoderma 15, 4263. doi: 10.1016/j.geoderma.2020.114263
Yang, T., Adams Jonathan, M., Shi, Y., Jin-Sheng, J., and Xin, C. (2017a). Soil fungal diversity in natural grasslands of the Tibetan Plateau: associations with plant diversity and productivity. New Phytol. 215, 756–765. doi: 10.1111/nph.14606
Yang, Y., Cheng, H., Dou, Y., and An, S. (2019a). Plant and soil traits driving soil fungal community due to tree plantation on the Loess Plateau. Sci. Total Environ. 708, 134560. doi: 10.1016/j.scitotenv.2019.134560
Yang, Y., Cheng, H., Gao, Y., and An, S. (2019b). Plant and soil traits driving soil fungal community due to tree plantation on the Loess Plateau. Sci. Total Environ. 708, 134560.
Yang, Y., Dou, Y., Huang, Y., and An, S. (2017b). Links between soil fungal diversity and plant and soil properties on the loess plateau. Front. Microbiol. 8, 2198. doi: 10.3389/fmicb.2017.02198
Yu, Z. Q., Liu, J. L., Yang, T. T., Zhang, S. X., Zhao, Z., and Wang, D. H. (2014). Seed rain and soil seed bank of Platycladus orientalis plantation in Weibei Loess Plateau. J. Northwest A and F Univ. (Nat. Sci. Ed.) 42, 85–92. doi: 10.13207/J.cnki.jnwafu.2014.06.004
Zeng, Q., An, S., Liu, Y., Wang, H., and Wang, Y. (2019). Biogeography and the driving factors affecting forest soil bacteria in an arid area. Sci. Total Environ. 680, 124–131. doi: 10.1016/j.scitotenv.2019.04.184
Zeng, Q., Xin, L. I., Dong, Y., Yayun, L. I., and Shaoshan, A. N. (2015). Soil microbial biomass nitrogen and carbon, water soluble nitrogen and carbon under different arbors forests on the Loess Plateau. Acta Ecol. Sinica 35, 3598–3605. doi: 10.5846/stxb201404030633
Zhai, M. P., Yin, W. L., and Zhang, G. M. (1991a). Studies on the mixed forest of Platycladus orientalis and Robinia pseudoacacia in Xishan area of Beijing (I)- growth, root system and water status. J. Beijing Forestry Univ. S2, 121–128.
Zhai, M. P., Yin, W. L., and Zhang, G. M. (1991b). Studies on the mixed forest of Platycladus orientalis and Robinia pseudoacacia in Xishan area of Beijing (II)- the nutritional status of the mixed forest and the chlorophyll content of Platycladus orientalis leaves. J.Beijing Fores. Univ. 45, 129–134.
Zhang, C., Liu, G., Xue, S., and Wang, G. (2016). Soil bacterial community dynamics reflect changes in plant community and soil properties during the secondary succession of abandoned farmland in the Loess Plateau. Soil Biol. Biochem. 97, 40–49. doi: 10.1016/j.soilbio.2016.02.013
Zhang, J., and Chang, Q. R. (2006). Effects on soil fertility in different types of forest on loess plateau. Bullet. Soil Water Conserv. 26, 26–28. doi: 10.13961/j.cnki.stbctb.2006.03.008
Zhang, W., Liu, W., Xu, M., Deng, J., Han, X., Yang, G., et al. (2019a). Response of forest growth to C:N:P stoichiometry in plants and soils during Robinia pseudoacacia afforestation on the Loess Plateau, China. Geoderma, 337, 280–289. doi: 10.1016/j.geoderma.2018.09.042
Zhang, W., Xu, Y., Gao, D., Wang, X., Liu, W., Deng, J., et al. (2019b). Ecoenzymatic stoichiometry and nutrient dynamics along a revegetation chronosequence in the soils of abandoned land and Robinia pseudoacacia plantation on the Loess Plateau, China. Soil Biol. Biochem. 134, 1–14. doi: 10.1016/j.soilbio.2019.03.017
Zhang, Y., Dong, S., Gao, Q., Liu, S., Zhou, H., Ganjurjav, H., et al. (2016). Climate change and human activities altered the diversity and composition of soil microbial community in alpine grasslands of the Qinghai-Tibetan Plateau. Sci. Total Environ. 562, 353–363. doi: 10.1016/j.scitotenv.2016.03.221
Keywords: Robinia pseudoacacia (black locust), alien species, native ecosystems, soil bacteria and fungi, chronosequence, aboveground–underground
Citation: Zhang L, Li H, Wu C, Linghu G, Zhu H, Khamphilavong K, Li M, Zhou X, Ma G and Kang Y (2023) Far-reaching effects on soil properties and underground microbial ecosystem after the introduction of black locusts in forest. Front. Ecol. Evol. 11:1210498. doi: 10.3389/fevo.2023.1210498
Received: 22 April 2023; Accepted: 29 May 2023;
Published: 14 July 2023.
Edited by:
Subhash Babu, Indian Agricultural Research Institute (ICAR), IndiaReviewed by:
Xiangwei Gong, Shenyang Agricultural University, ChinaVivek Yadav, Xinjiang Academy of Agricultural Sciences, China
Muhammad Qudrat Ullah Farooqi, University of Western Australia, Australia
Copyright © 2023 Zhang, Li, Wu, Linghu, Zhu, Khamphilavong, Li, Zhou, Ma and Kang. This is an open-access article distributed under the terms of the Creative Commons Attribution License (CC BY). The use, distribution or reproduction in other forums is permitted, provided the original author(s) and the copyright owner(s) are credited and that the original publication in this journal is cited, in accordance with accepted academic practice. No use, distribution or reproduction is permitted which does not comply with these terms.
*Correspondence: Yongxiang Kang, yxkang@nwsuaf.edu.cn