- 1Behavioural and Chemical Ecology Unit (BCEU), International Centre of Insect Physiology and Ecology (icipe), Nairobi, Kenya
- 2Arthropod Pathology Unit (APU), International Centre of Insect Physiology and Ecology (icipe), Nairobi, Kenya
- 3Department of Zoology and Entomology, University of Pretoria, Hatfield, South Africa
- 4Forestry and Agricultural Biotechnology Institute (FABI), University of Pretoria, Hatfield, South Africa
Intraguild interactions are known to trigger competition among phytophagous insects that depend on the same food source, which may have implications on natural enemy performance. Here, we tested the hypothesis that intraguild interactions between the greenhouse whitefly, Trialeurodes vaporariorum, and the South American tomato pinworm, Phthorimaea absoluta on the host plant tomato would affect performance of the pinworm ectoparasitoid Bracon nigricans. In oviposition bioassays, P. absoluta females significantly laid more eggs and were attracted to tomato plants infested with T. vaporariorum than uninfested plants. Additionally, T. vaporariorum infestation decreased B. nigricans parasitism on P. absoluta larvae and attraction to tomato plants. In coupled gas chromatography-electroantennographic detection (GC-EAD) recordings, antennae of P. absoluta and B. nigricans females detected 18 and 15 components, respectively from the herbivore-induced volatiles, which were identified by GC-mass spectrometry (GC-MS) as green leaf volatiles (GLVs) and terpenes. In dose-response behavioral assays, P. absoluta and B. nigricans responses varied with the composition and concentration of blends and individual compounds tested. Whereas P. absoluta was attracted to GLVs and terpenes, B. nigricans avoided most of these chemicals, especially the GLV (E)-2-hexenal and terpenes δ-3-carene, and (E)-caryophyllene. The results suggest that herbivore intraguild interactions can influence the behavioral ecology and performance of natural enemies which may have evolutionary significance. Also, these specific GLVs and terpenes can be exploited in the management of P. absoluta.
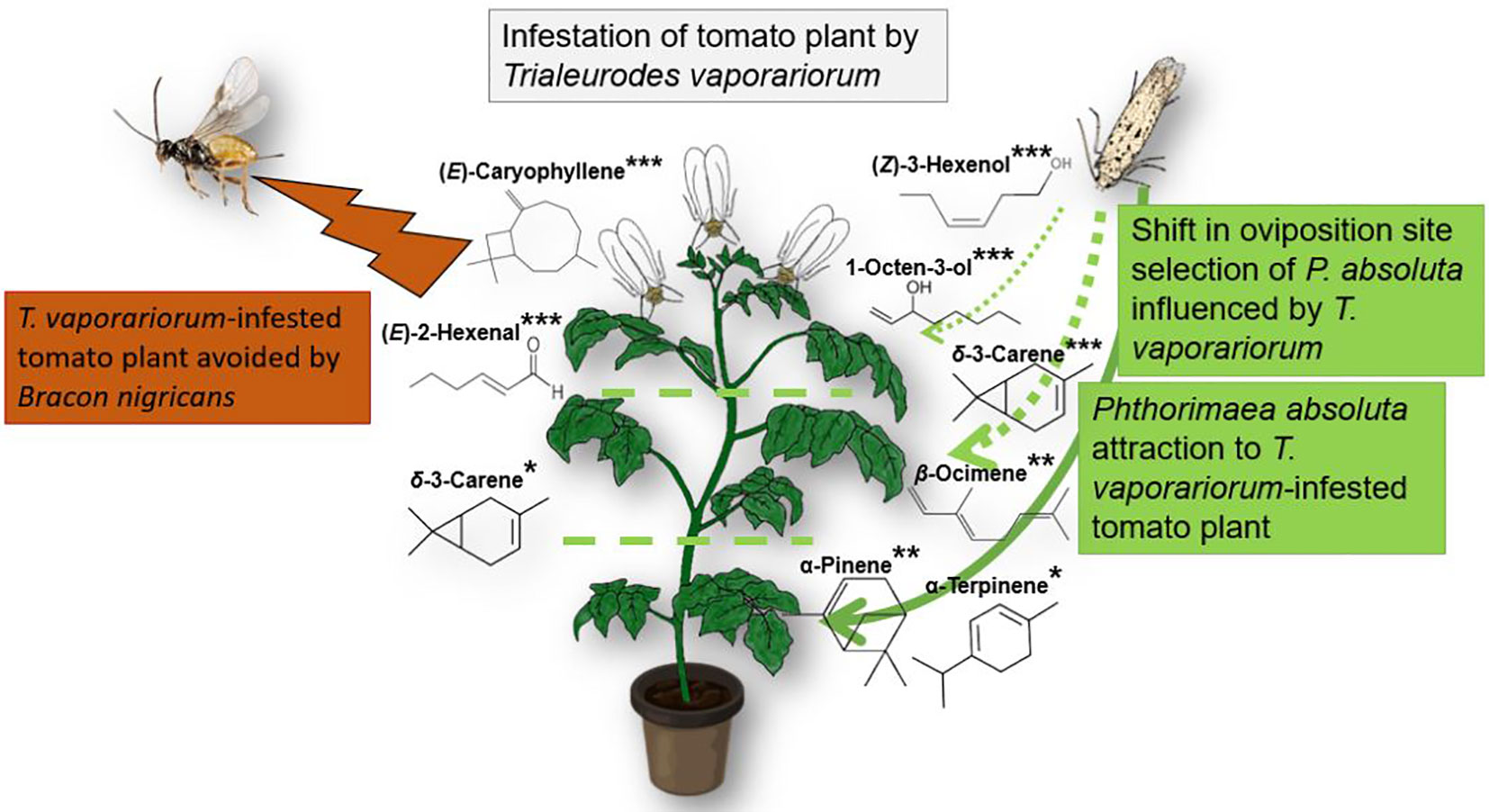
Graphical Abstract Trialeurodes vaporariorum infestation increases Phthorimaea absoluta attraction and oviposition. Trialeurodes vaporariorum constraints P. absoluta to shift its preferred oviposition site from apical and median plant strata to basal. Trialeurodes vaporariorum-infested tomato plants were avoided by the ectoparasitoid Bracon nigricans. (Z)-3-hexenol, 1-octen-3-ol, δ-3-carene, β-ocimene (mixture of isomers E and Z), α-pinene, and α-terpinene mediate the attraction of P. absoluta to T. vaporariorum-infested tomato plants. (E)-2-hexenal, (E)-caryophyllene and δ-3-carene mediate the avoidance of T. vaporariorum-infested tomato plants by B. nigricans.
Introduction
Arthropods live in complex networks shaped by different types of interactions with other forms of life, particularly plants and microbes (Thompson et al., 2012; Poelman and Dicke, 2014). These interactions are grouped into two; vertical interactions where energy flows from primary to higher trophic levels (Thompson et al., 2012; Wang et al., 2019) and horizontal interactions among species belonging to the same feeding guild (Zhang et al., 2009; Bird et al., 2019; Zhang et al., 2019; Zhao et al., 2019). Of the two feeding guilds, horizontal interactions (intraguild interaction) among arthropod species are described as “competitive” or “life-threatening” (Kaplan and Denno, 2007; Bird et al., 2019; Liu et al., 2021). Recent research has revealed that intraguild interactions can be mutually beneficial (Zhang et al., 2009; Soler et al., 2012; Zhang et al., 2019; Hu et al., 2020; Liu et al., 2021). For instance, a collaborative partnership has been reported between the brown planthopper, Nilaparvata lugens (Hemiptera: Delphacidae), and the rice striped stemborer, Chilo suppressalis (Lepidoptera: Crambidae) (Hu et al., 2020; Liu et al., 2021), which affects the feeding and oviposition performances of both species. Furthermore, it has been demonstrated that feeding by the stemborer induced repellency in the parasitoid of the planthopper (Hu et al., 2020). Additionally, herbivory of the silverleaf whitefly, Bemisia tabaci (Hemiptera: Aleyrodidae), and the red spider mite, Tetranychus urticae (Arachnida: Tetranychidae) in lima bean, Phaseolus lunatus L. (Fabaceae) increased defense against the predatory mites, Phytoseiulus persimilis (Arachnida: Phytoseiidae) (Zhang et al., 2009).
The expression of defense genes and the emission of volatile organic compounds (VOCs) by the host plant reflect the nature of the intraguild herbivore interaction, which can be competitive or friendly (Rodriguez-Saona et al., 2003; Rodriguez-Saona et al., 2005; Soler et al., 2012; Zhang et al., 2019). Herbivore-induced plant volatiles (HIPVs) play crucial roles in these ecological interactions. They influence the host plant location of intraguild herbivores or conspecific species by acting as attractants or repellents (De Moraes et al., 2001; Dicke and Baldwin, 2010; Soler et al., 2012; Ameye et al., 2018; Turlings and Erb, 2018; Hu et al., 2020; Zu et al., 2020). HIPVs can also function as oviposition deterrents or boosters (De Moraes et al., 2001; War et al., 2011; Hu et al., 2020; Liu et al., 2021) or serve as host searching cues for natural enemies and pollinators (Dicke and Baldwin, 2010; Ameye et al., 2018; Turlings and Erb, 2018). HIPVs play an important role in priming and alerting neighboring plants to an impending herbivore attack by triggering host defenses (Thaler et al., 2002; Engelberth et al., 2004; Sugimoto et al., 2014; Sugimoto et al., 2021). Green leaf volatiles (GLVs) and plant defense elicitors (PDEs) are chemical signals released from plants, especially in response to stresses such as herbivory (Rodriguez-Saona et al., 2003; War et al., 2011; Soler et al., 2012), mechanical wounding (Piesik et al., 2010; Ameye et al., 2018), pathogenic infection (Piesik et al., 2011; Ameye et al., 2018), adverse climatic conditions (drought and heat) (Piesik et al., 2011; Ameye et al., 2018), and edaphic factors (Effah et al., 2021). However, when herbivory triggers the release of GLVs and PDEs, the amount and quality of these compounds might vary depending on the feeding mode of the insect (piercing/sucking vs. chewing) (Soler et al., 2012; Rowen and Kaplan, 2016), and this may define the type of interactions between conspecific or heterospecific species (Kaplan and Denno, 2007; Liu et al., 2021).
Greenhouse whitefly (GWF), Trialeurodes vaporariorum (Hemiptera: Aleyrodidae), and the South American tomato pinworm (TPW), Phthorimaea (Tuta) absoluta (Lepidoptera: Gelechiidae) are two of the most damaging pests of tomatoes, Solanum lycopersicum L. (Solanaceae) worldwide (Biondi et al., 2018; Khamis et al., 2021). The two species are endemic to various ecozones, where they co-occur not only on tomato but also on other solanaceous crops (Biondi et al., 2018; Khamis et al., 2021). Trialeurodes vaporariorum and P. absoluta can cause different forms of damage to their commonly shared host plants, based on their distinctive feeding behaviors (Biondi et al., 2018; Milenovic et al., 2019). Whiteflies directly harm plants by sucking plant sap and withdrawing nutrients, resulting in chlorosis and plant weakness (Inbar and Gerling, 2008; Milenovic et al., 2019; Khamis et al., 2021). Adult whiteflies are known to transmit viruses from damaged to healthy plants, hence indirectly causing insect-borne plant diseases (Ghosh et al., 2019; Khamis et al., 2021; Ayelo et al., 2022). Molasses (honeydew) released by the nymphs of whiteflies forms a sticky coating on foliage, allowing fungus such as sooty mold to proliferate and cause the leaves to appear black and dirty, compromising the photosynthetic activity of the plant (Ghosh et al., 2019). On the other hand, P. absoluta causes injury through larval feeding on leaves and other aboveground plant organs (Torres et al., 2001; Biondi et al., 2018). Following egg hatching, the neonate larvae penetrate the epidermis of adjacent plant tissues to develop inside, producing expanding mines on mesophyll (Biondi et al., 2018). Females of the two herbivore species display similar within-plant eggs distribution patterns with both having preferences for apical oviposition on the young leaflets of the host plant (Cocco et al., 2014; Ullah and Lim, 2016).
Even though T. vaporariorum and P. absoluta co-occur in a number of agroecologies, very little research has examined the nature of the trophic relationship between the two and their environment. However, Ayelo et al. (2021a) recently demonstrated that P. absoluta unlike T. vaporariorum herbivory of tomato plants, attracted the mirid predator, Nesidiocoris tenuis (Hemiptera: Miridae), a commonly-shared predator of both pests (Pappas et al., 2015; Hassanpour et al., 2016; Aigbedion-Atalor et al., 2021). In a related study, T. vaporariorum herbivory of tomato plant and the honeydew produced attracted its parasitoid Encarsia formosa (Hymenoptera: Aphelinidae) (Ayelo et al., 2021b; Ayelo et al., 2022). Meanwhile, the ectoparasitoid Bracon nigricans (Hymenoptera: Braconidae) has been documented as a very promising biocontrol agent for P. absoluta (Biondi et al., 2013; Sambo et al., 2022). For instance, the parasitoid has been found to rapidly arrest the development of P. absoluta larvae by injecting venom that immobilizes the pest and enhances parasitism by the female wasp through oviposition on the cuticle of the host larvae (Biondi et al., 2013). Although both pests share the same host plant and a common natural enemy, little is known about their interactions within the same tomato ecosystem. However, studies have shown that insect herbivory can modulate plant volatile responses, which may have implications for the bionomics of higher trophic level species (Turlings et al., 1995; Liu et al., 2021; Ayelo et al., 2022).
In the current study, we tested the hypothesis that intraguild interactions on tomato by two of its key pests, the greenhouse whitefly, T. vaporariorum, and the South American tomato pinworm, P. absoluta with different feeding behaviors, would affect interactions with the pinworm ectoparasitoid B. nigricans. To achieve this, we first studied the oviposition responses of P. absoluta to tomato plants infested with T. vaporariorum. Secondly, we assessed parasitism of P. absoluta larvae by B. nigricans in tomato plants infested with T. vaporariorum. Thirdly, we investigated the exploitation of T. vaporariorum-induced tomato volatiles by P. absoluta and B. nigricans. Lastly, we used gas chromatography coupled to electroantennographic detection (GC-EAD) and GC-mass spectrometry (GC-MS) to compare the chemistry of the volatiles of intact and herbivore-induced tomato plants and behavioral assays to identify the mediating semiochemicals.
Materials and methods
Plants and insects
Tomato plants (Solanum lycopercicum L., cv. Terminator F1) were grown in a greenhouse on autoclaved substrate (sand: manure mixture at 5:2) in 8-cm diameter and 7.5-cm high plastic pots at 24–26°C, 65–70% R.H. and 12:12 (Light: Dark) photoperiod. All the insects were obtained from the Animal Rearing and Quarantine Unit (ARQU), icipe-Kenya, reared using optimized insect breeding protocols (Ayelo et al., 2021a; Ayelo et al., 2021b) under controlled environmental conditions of 24–26°C, 65–70% R.H., and 12L:12D. On tomato plants, P. absoluta (larvae and adults) and T. vaporariorum (nymphs and adults) were separately confined in netted Plexiglass cages measuring 65 × 65 × 75 cm. The parasitoid wasp, B. nigricans was maintained on 3rd–4th instar larvae of P. absoluta-carrying tomato plants in sleeved Plexiglass cages (40 × 40 × 50 cm). Adults of the insects were fed on 10% honey solution.
No-choice and choice oviposition experiments with Phthorimaea absoluta and Trialeurodes vaporariorum
Oviposition experiments were carried out in three ways to evaluate the within-plant egg distribution patterns of T. vaporariorum and P. absoluta. The experiments were conducted in 45 × 45 × 65 cm netted Plexiglass cages with six-week-old (with 10–12 fully expanded compound leaves), healthy potted tomato plants at 24–26°C, 65% R.H., and 12L:12D.
Experiment I
Tomato plants were individually infested in separate cages with varying densities (1:1 sex ratio) of two-day-old adults of T. vaporariorum (50, 100, 200, and 300) and P. absoluta (10, 20, and 40). Hereinafter, T. vaporariorum-infested plants are referred to as GWF50, GWF100, GWF200, and GWF300; while P. absoluta-infested plants are referred to as TPW10, TPW20, and TPW40. Using different infestation densities reduced bias (Ayelo et al., 2021b), and these densities indicate moderate to severe damage produced by the two species in the field (Cocco et al., 2014; Lima et al., 2018). The insects were fed ad libitum with honey solution (10%) and allowed to oviposit for three days. For this oviposition experiment, ten tomato plants were employed as replicates.
Experiment II
The oviposition behavior of P. absoluta on T. vaporariorum-infested tomato plants (sequential oviposition) was studied to assess the effect of prior infestation by T. vaporariorum on the within-plant oviposition pattern of P. absoluta. The study was a no-choice experiment where individual tomato plants were separately infested with densities of 50, 100, 200, and 300 (1:1 sex ratio) T. vaporariorum for three days. The adult whiteflies were then removed with a hand aspirator, and different densities of P. absoluta (TPW10, TPW20, and TPW40) were used to infest each T. vaporariorum-infested tomato plant in separate oviposition cages for three days. This resulted in twelve different combinations of infestation densities, along with control groups consisting of T. vaporariorum-free tomato plants and tomato plants infested simultaneously with 200 T. vaporariorum and 20 P. absoluta (GWF | TPW). Seven tomato plants per treatment group were employed as biological replicates.
Experiment III
This is a choice experiment designed to determine the factors that influence the oviposition site selection behavior of female P. absoluta. The study specifically aims to determine whether the presence of intraguild eggs occupying the oviposition site or the induced defenses of tomato plants by heterospecifics have a stronger influence on the oviposition behavior of P. absoluta females. Treatments included T. vaporariorum-free plants (intact plants), T. vaporariorum-partially-infested plants (selected leaves per stratum were protected), and tomato plants infested with adults T. vaporariorum without protection (for clarity, to be referred to henceforth as T. vaporariorum-fully-infested plants). The treatment plants were prepared by infesting individual tomato plants with 200 T. vaporariorum (1:1 sex ratio) for three days in Plexiglass cages. The use of GWF200 was determined based on the results of Experiment II, in which P. absoluta oviposition pattern was altered on tomato plants with this density of T. vaporariorum (Figure 1A). To achieve the partial infestation (Rodriguez-Saona et al., 2005) of tomato plants by adults T. vaporariorum, 1–3 selected leaves from each plant stratum were bagged in non-woven aerated drawstring bags (27 × 36 cm; Shenzhen Technology Co., China) during the exposure. The T. vaporariorum-partially-infested plants resulted in exposed leaves (hereinafter, GWF-exposed leaves) and leaves protected from infestation by T. vaporariorum (hereinafter, GWF-non-exposed leaves). Before offering T. vaporariorum-infested plants to P. absoluta, the protective bags and T. vaporariorum adults were removed from the cages. Thus, T. vaporariorum-eggs-carrying and intact tomato plants were offered to TPW40 for three days in a dual-choice oviposition assay (Ayelo et al., 2021a). The combinations were: (1) intact tomato plant versus T. vaporariorum-partially-infested tomato plant; (2) intact tomato plant versus T. vaporariorum-fully-infested tomato plant; and (3) T. vaporariorum-partially-infested tomato plant versus T. vaporariorum-fully-infested tomato plant. Each combination was tested with seven tomato plants of each treatment (replicates).
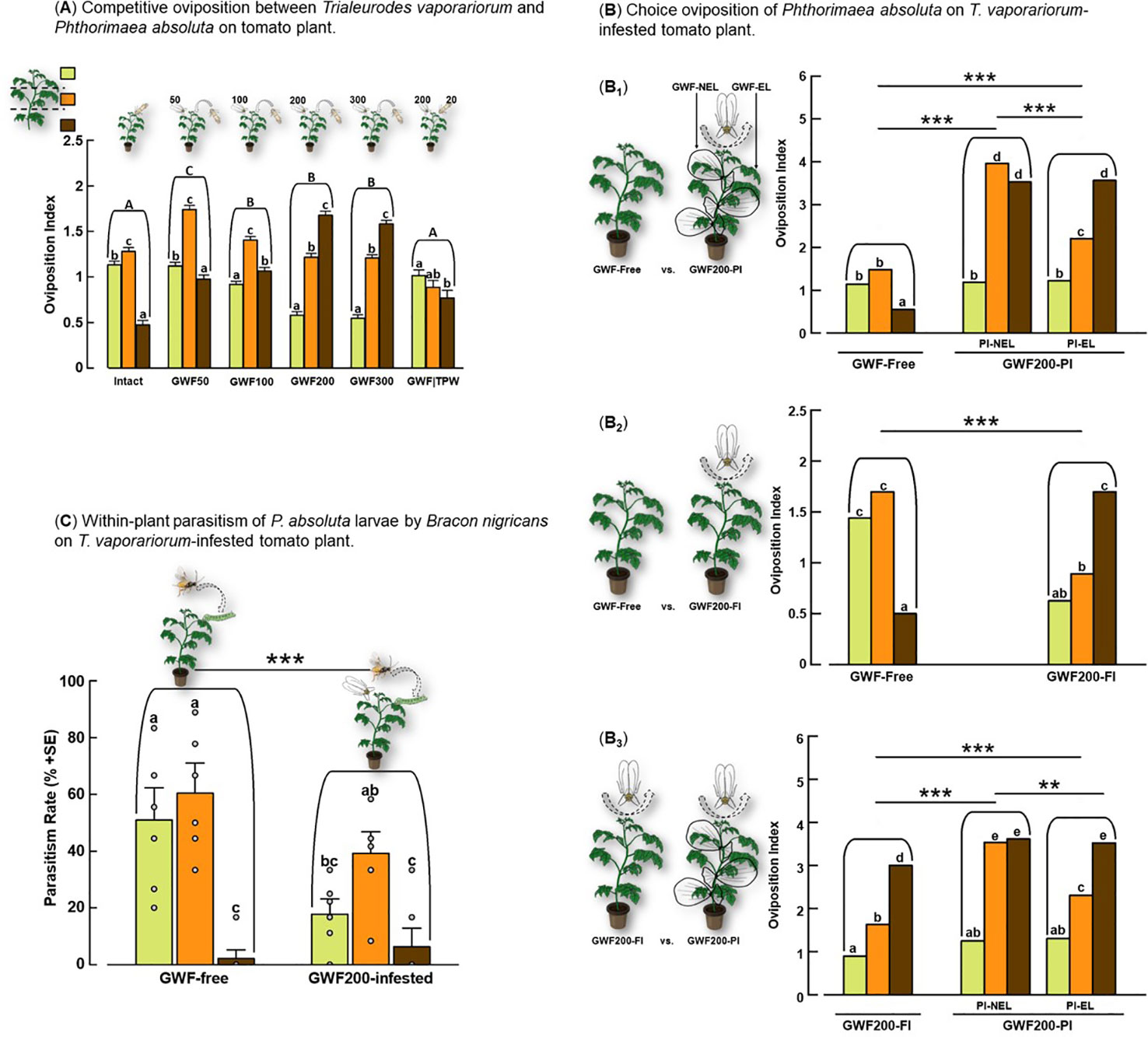
Figure 1 No-choice oviposition (A) and choice oviposition (B) patterns of Phthorimaea absoluta and within-plant parasitism by Bracon nigricans (C) on intact versus Trialeurodes vaporariorum-infested tomato plants. Oviposition experiments were conducted using intact tomato plants exposed to different treatments for 3 days (A). Tomato plants were subjected to different treatments: Intact, GWF200-partially-infested (GWF200-PI), and GWF200-fully-infested (GWF-FI). GWF200-PI resulted in non-exposed leaves (PI-NEL) and exposed leaves (PI-EL) (B). The oviposition indices of P. absoluta were recorded and presented as the mean number of eggs per 3-cm-diameter tomato leaf for each plant stratum. Significant differences between treatments and plant strata were determined using a log link GLM and likelihood ratio test (LR test). Different lowercase letters above each bar indicate significant differences between mean oviposition indices per tomato plant stratum (Tukey’s HSD test, P ≤ 0.05; N = 7). Uppercase letters (A) and asterisk (*) (B) indicate significance difference between the treatment plants at **P< 0.01, and ***P< 0.001. The within-plant parasitism performance of B. nigricans was tested using two treatment plants: GWF-free versus GWF200-infested (C). Each bar shows parasitism rates per plant stratum. Significance was determined using one-way ANOVA (P< 0.05; N = 8). Different letters above bars indicate significant differences between treatments, and asterisk above the bars imply treatment significant difference.
In all the oviposition experiments, eggs were counted from 3 cm diameter circle of tomato leaf (counting section) in order to eliminate bias caused by variation in leaf count and area per plant (Arnó et al., 2006). A steel pipe (3 cm diameter) was used to delimit the counting sections on the abaxial and adaxial faces of the leaves. While the counting of P. absoluta eggs was done directly, that of T. vaporariorum was done under a dissecting microscope (35×; Leica EZ4 HD, Leica Microsystems Ltd., Germany). Depending on the leaf size and the number of available leaves per plant, 5–7 counting sections on 2–4 compound leaves were examined from each of the apical, middle, and basal thirds of the plant (hence referred to as plant stratum) (Gonring et al., 2020) to calculate the insects’ oviposition indices per plant stratum. Plants were discarded after the egg counting exercise was completed and not utilized in any additional experiments to prevent the introduction of potential sources of bias owing to physical stress. The oviposition index of the insects was calculated as follows:
Olfactory responses of Phthorimaea absoluta to Trialeurodes vaporariorum-infested tomato plants
A Y-tube olfactometer was used to test responses of P. absoluta gravid females to intact and herbivore-induced tomato plant odors following procedures as described previously from our laboratory (Agbessenou et al., 2022). Briefly, the olfactometer was oriented vertically, and Teflon tubing was used to connect the two arms of the apparatus to the test odor sources. These sources consisted of individual tomato plant, with foliage enclosed in clean Kitchen Craft oven bags (59 × 53.5 cm; Thomas Plant Birmingham Ltd, England). The control arm of the olfactometer was an empty oven bag. The olfactometer was positioned in the bioassay arena made of a black cardboard box (40 × 40 × 55 cm), to eliminate visual cues. The arena was illuminated uniformly with 20 W red fluorescent lamps from the top of the box. A constant flow of 150 mL min−1 of air was produced by a vacuum pump, which was then filtered through activated charcoal, passed through each odor source and discharged through the base tube, where individual test insects were released and allowed to make a choice. The odor sources included: (i) intact plants (uninfested), (ii) GWF-infested plants (individual tomato plant infested with two-day-old 200 adults of GWF for 3 days), (iii) TPW-infested plants (individual tomato plant infested with 20 first instar larvae of P. absoluta for three days), and (iv) GWF|TPW-infested plants (individual tomato plant infested with 200 adults of T. vaporariorum for seven days, and 20 first instar of P. absoluta introduced from the fourth day). These odor sources and charcoal-purified air (control) were assessed against each other in dual choice combinations (Table 1). An individual insect is recorded as having made a choice when it walked to the end of an arm towards the odor sources and remained there for at least 30 sec. Twenty individuals per day for five days were tested individually for each dual-choice.
Olfactory responses of parasitoid Bracon nigricans to Trialeurodes vaporariorum-infested tomato plants
Olfactometer assays were conducted as described in section 2.3 for P. absoluta to assess the response of two-day-old mated parasitoid B. nigricans to T. vaporariorum-infested tomato plants odors. However, the bioassay arena was illuminated uniformly with a 220–240 V cool white fluorescent lamps. The odor sources consisted of: (i) intact plants, (ii) GWF-infested plants, (iii) TPW-infested plants, and GWF|TPW-infested plants. These odor sources and charcoal-purified air (control) were assessed against each other in dual combinations (Table 1). Twenty parasitoids per day for four days were tested for each dual choice combination by releasing individual parasitoid in the base tube of the Y-tube.
Parasitism of Phthorimaea absoluta larvae by the parasitoid Bracon nigricans on Trialeurodes vaporariorum-infested tomato plants
We investigated the parasitism pattern of B. nigricans against P. absoluta in T. vaporariorum infested tomato plants. To achieve this, 30–42 P. absoluta third instar larvae were distributed on T. vaporariorum-free and T. vaporariorum-infested tomato plants. The T. vaporariorum-infested tomato plants were prepared as described for olfactory bioassays, by exposing individual tomato plants to 200 adults of T. vaporariorum for three days. Three P. absoluta larvae were permitted to mine and penetrate the epidermis of each compound leaf from each plant stratum. This was accomplished by placing third instar larvae of P. absoluta starved for 3 h on the surface of tomato leaflets with a small paintbrush and tracking them until they penetrated the epidermis. The two types of plants were placed in adjacent corners of the oviposition cage (45 × 45 × 65 cm). To access parasitism, 30 pairs of two-day-old parasitoid were released into the cage for 24-h. After which the leaves were sampled according to their position on the plant (plant strata) and incubated along with fresh tomato leaves in aerated clear plastic cylinder jars (18 × 8 cm) for three days. Then, the exoskeleton of the larvae per leaf were individually examined under a stereomicroscope (200×; Leica DM500, Leica Microsystems Ltd., Germany) with the help of a small paintbrush to determine their parasitization status (presence or absence of eggs of B. nigricans). The parasitism was recorded per plant stratum. The experiment was repeated with eight dual combinations of the treatment plants (replicates), i.e., involving 240 pairs of parasitoids in total.
Collection of tomato plant volatiles
Headspace volatiles released by intact and herbivore-infested tomato plants were trapped using a dynamic push–pull airflow system (Analytical Research System, Florida, US). Individual plants were bagged in Kitchen Craft oven bags (59 × 53.5 cm; Thomas Plant Birmingham Ltd, England) pre-conditioned in an oven at ~100°C for 24 h before use. The bagged plants were connected to the airflow system and charcoal purified air passed through at 350 mL/min with the volatiles collected onto Super Q filter traps (30-mg adsorbent, Sigma Scientific, Florida, USA) for 24 h (5:00 pm–5:00 pm the next day). The adsorbed volatiles were eluted under a gentle stream of nitrogen with 150 µL of dichloromethane (DCM) and stored at –80°C until required for chemical analyses.
One µL of each extract was analyzed using coupled gas chromatography/mass spectrometry (GC/MS) on an Agilent Technologies 7890B GC linked to a 5795C MS (inert XL/EI/CI MSD) triple axis mass detector, equipped with a HP5-MSI low bleed capillary column (30 m × 0.25 mm i.d, 0.25 μm) (Agilent Technologies) set in a splitless mode. Helium was used as the carrier gas at a flow rate of 1.2 mL per min. The oven temperature was programmed as follows: 35°C for 5 min and increased (at a rate of 10°C per min) to 280°C where it was held for 10.5 min. The temperatures of ion source, and quadrupoles were 230°C, and 150°C, respectively. Spectra were captured at a multiplier voltage of 70 eV. In the full scan mode, fragment ions were examined over the mass range of 40–550 m/z (Adams et al., 2023). To identify the compounds, mass spectra, and Kovats retention indices (RIs) were compared to those of previously published databases by the National Institute of Standards and Technology (NIST) and Wiley library (Wiley Registry 11th Edition/NIST2014). The concentration of compounds was determined by creating external calibration curves using stock solutions of the monoterpene α-pinene and the sesquiterpene (E)-caryophyllene, with a concentration range of 0.1 to 1,000 ng/µL. To quantify the compounds, the peak area of the unknown compound was compared against the known concentration curves through extrapolation. Concentrations were expressed in ng/plant/h.
Electrophysiology
Couple GC-electroantennographic detection (EAD) with an HP-5MS column (30 m × 0.25 mm i.d. × 0.25 µm film thickness, Agilent Technologies, Palo Alto, California, USA) was used for the GC-EAD recordings. Two drawn-out glass capillaries (1.5 mm i.d.) were filled with Ringer saline solution (7.5 g NaCl, 0.7 g KCl, 0.2 g CaCl2, and 0.2 g MgCl2 dissolved in 1L of distilled water). Individual antennae cut from ice-chilled insects were mounted between the glass capillary tubes attached to silver-coated electrode wires of the probe (INR-II, Syntech, Hilversum, the Netherlands), with the base of the head antenna connected to the reference electrode. Two-microlitres (2-µL) aliquot of T. vaporariorum-infested tomato plant volatile was injected into the entry port of the GC-FID at an injection temperature of 280°C and a split valve delay of 5 min. The oven temperature was programmed from 35°C (3 min hold) to 280°C at 10°C/min. The column effluent was split 1:1 for simultaneous detection by FID and EAD. Data were visualized and analyzed with GC-EAD software (EAG 2015 Version 1.2.6, Syntech, Hilversum, The Netherlands). EAD responses to FID peaks were defined as repeatable deflections of the baseline; and a compound is only confirmed EAD-active if it elicited at least six consistent EAG responses, i.e., compounds detected by at least six antennae. The identities of EAD-active components were confirmed with synthetic authentic standards were available.
Olfactory responses of Phthorimaea absoluta and its parasitoid Bracon nigricans to synthetic compounds
Eleven synthetic compounds were tested per insect species in this experiment (Tables 2, 3). Three concentrations of each compound were evaluated at their natural release concentration (ng/plant/h), as well as at double and half the natural concentration. The compounds were diluted in DCM and applied to filter paper (30 mm diameter), which was then evaluated against a control (filter paper loaded with 10-μL DCM). After evaporation, the treated filter papers were placed in Pasteur pipettes connected to a Y-tube olfactometer. Each choice had 75 insects and a 10-min observation time limit.
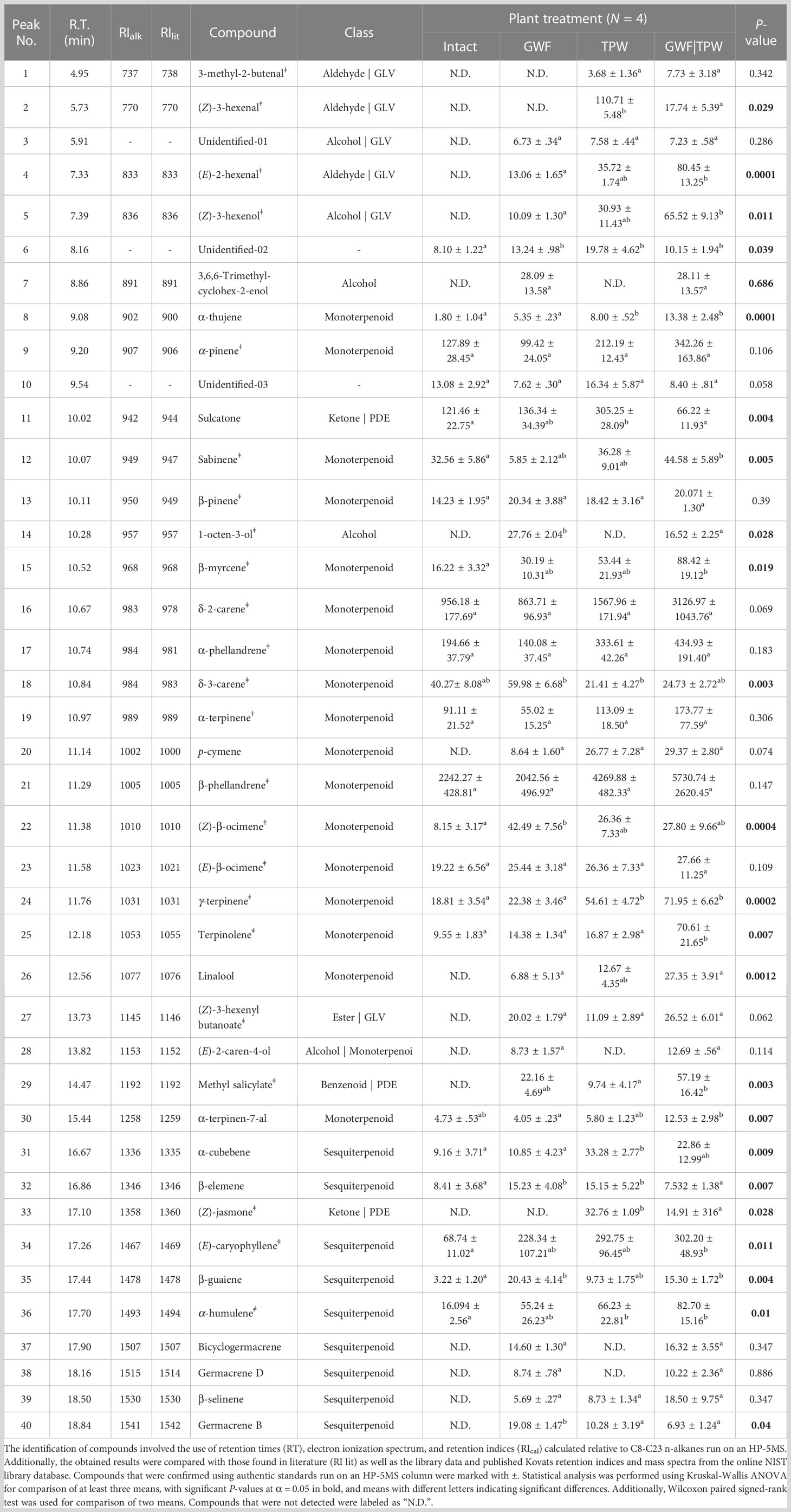
Table 3 Amount (ng/plant/h) of volatile compounds identified in the headspace of intact, and herbivore-infested tomato plants.
To confirm the behavior elicited by the compounds, blends containing natural release rate concentrations, double and half the natural concentrations were prepared using the ratios of individually tested compounds for each insect species (Table 3). Two blends were prepared based on the results from tests with individual compounds: one with attractive compounds (for P. absoluta) and one with repellent compounds (for B. nigricans). These blends were tested against the initial blends to confirm the compound mediating the insect behavior towards T. vaporariorum-infested tomato plant volatile.
Chemicals
Authentic standards (>95% purity) of the compounds used in this study were purchased from Sigma-Aldrich, Inc., United States and Sigma-Aldrich Chemie GmbH, Germany. Dichloromethane (99.9% purity) used for volatile elution and as control during the olfactory guided bioassays was purchased from Merck (Merck KGaA, Germany).
Statistical analyses
The data were checked for normality using Shapiro–Wilk test and equal variances using Levene test before selecting the appropriate statistical analyses. Data from the Y-tube olfactometer were analyzed using a binomial test (two-tailed test); individuals that did not make a choice were excluded from the analysis. The within-plant oviposition data were analyzed using a generalized linear model (GLM) with likelihood radio test (LR test) in the log link function. One-way ANOVA was used to compare the within-plant parasitism of B. nigricans on P. absoluta. Means were compared using the Tukey’s HSD test (P ≤ 0.05). Due to the non-normal distribution of the GC-MS data, the Kruskal-Wallis test was used for the analysis, using Dunn’s multiple pairwise comparison of the means. All the statistics were conducted in R Studio (R Core Team, 2022).
Results
Oviposition patterns of Phthorimaea absoluta and Trialeurodes vaporariorum on intact tomato foliage
Phthorimaea absoluta (TPW) females significantly laid more eggs, reflecting a higher oviposition index on leaves in the apical and middle strata of intact plants than on the basal stratum (LR test with GLM, χ2 = 676.03, df = 2, P< 0.0001, Figure 2A1–3). However, when infested with TPW20, P. absoluta females significantly preferred to lay their eggs on leaves in the middle stratum over apical and basal strata, respectively (LR test with GLM, χ2 = 252.18, df = 2, P< 0.0001, Figure 2A2). On the other hand, females of T. vaporariorum (GWF) laid significantly more eggs on leaves in the apical stratum than on leaves in the middle and basal strata (LR test with GLM, df = 2, P< 0.0001, Figure 2B1–4) of tomato plants irrespective of the infestation density.
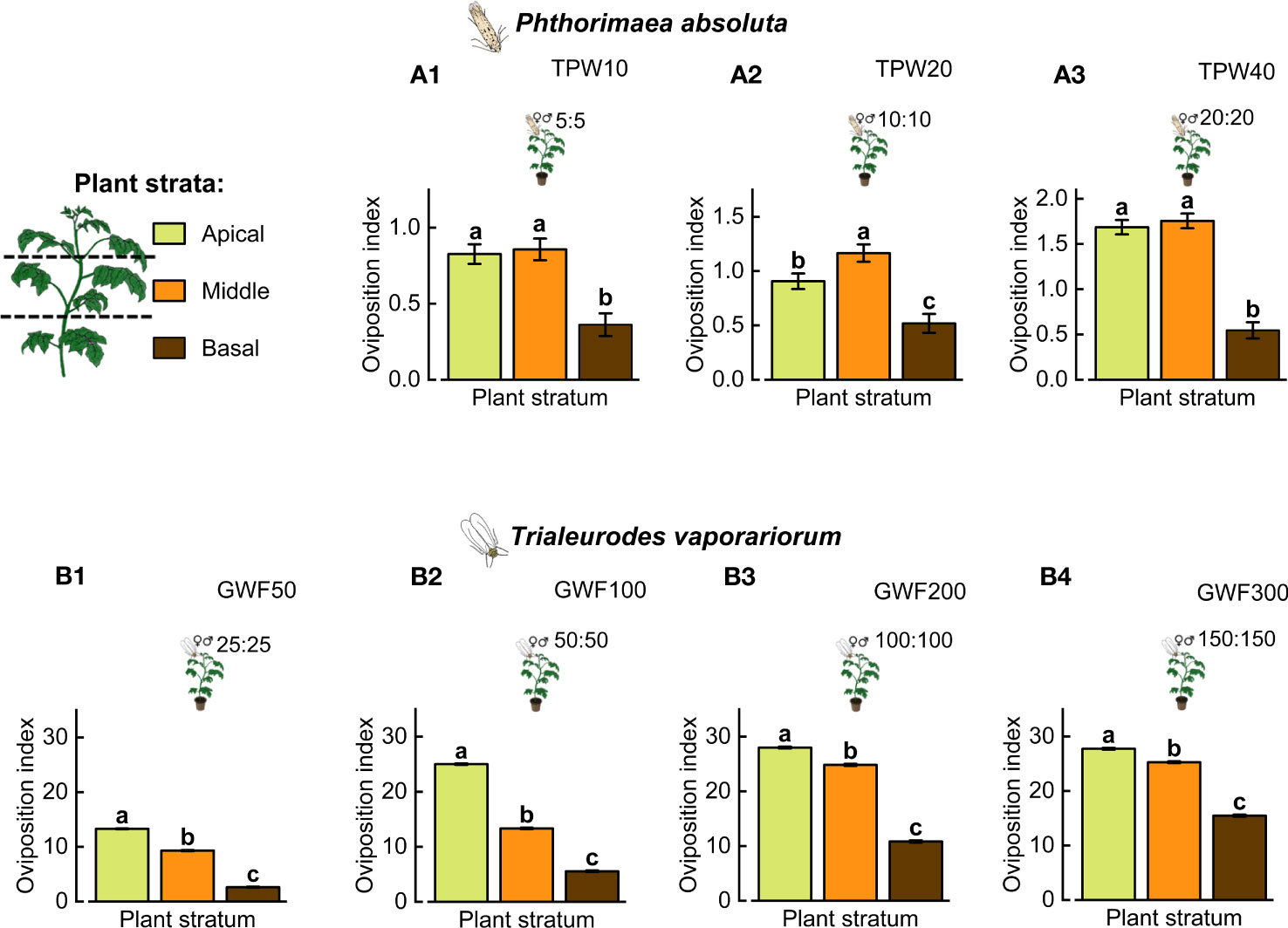
Figure 2 Within-plant oviposition index of Phthorimaea absoluta and Trialeurodes vaporariorum. Different densities (1:1 sex ratio) of (A1–3) P. absoluta (10, 20, and 40) and (B1–4) T. vaporariorum (50, 10, 200, and 300) were tested in a 3-day no-choice oviposition experiment using intact potted tomato plants. The oviposition indices of the insects were recorded for the apical, middle, and basal plant strata. Significant differences between treatments were determined using a likelihood ratio test (LR test) with a generalized linear model (GLM) in the log link function (P< 0.05; N = 10). Different letters above each bar indicate significant differences between mean oviposition indices per tomato plant stratum (Tukey’s HSD test, P ≤ 0.05; N = 10).
Oviposition patterns of Phthorimaea absoluta on Trialeurodes vaporariorum-adult-infested plant
On intact tomato plants, female P. absoluta had a significantly lower oviposition index on the basal stratum of the plant than on the apical and middle strata (LR test with GLM, χ2 = 676.03, df = 2, P< 0.0001, Figure 1A). On GWF50 tomato plants, the oviposition indices from the different plant strata differed significantly (LR with GLM, χ2 = 297.26, df = 2, P< 0.0001, Figure 1A), with the oviposition index recorded in the middle stratum being the highest, successively followed by apical and basal strata. Likewise, on GWF100 tomato plants, the oviposition index of P. absoluta on leaves in the middle stratum was significantly the highest, while the index in the basal stratum was higher than on the apical stratum of the plants (LR with GLM, χ2 = 677.33, df = 2, P< 0.0001, Figure 1A). In contrast, at the highest infestation densities (GWF200 and GWF300), the oviposition indices in basal stratum of the plants were significantly higher than on leaves in the apical and middle strata (LR with GLM, χ2 (GWF200) = 743.39, χ2 (GWF300) = 960.91, df = 2, P< 0.0001, Figure 1A). Yet, on tomato plants co-infested with adults T. vaporariorum and P. absoluta (GWF|TPW), the lowest oviposition index of P. absoluta occurred in the basal plant stratum than in the apical stratum (LR with GLM, χ2 = 400.51, df = 2, P< 0.0001, Figure 1A). Overall, oviposition indices of P. absoluta on intact and herbivore-infested tomato plants were significantly different (LR with GLM, χ2 = 8648.21, df = 5, P< 0.0001, Figure 1A), with the highest oviposition index being associated with GWF50.
Within-plant choice oviposition with Phthorimaea absoluta on Trialeurodes vaporariorum-adult-infested plant
Figure 1B shows the difference in the oviposition preferences of P. absoluta on intact and GWF200-partially-infested and fully-infested tomato plants. The oviposition index was significantly higher on GWF200-partially-infested tomato plants than on intact plants (LR with GLM, χ2 = 336.75, df = 1, P< 0.0001, Figure 1B1). Additionally, higher oviposition responses by P. absoluta were recorded on tomato leaves uninfested with T. vaporariorum over infested tomato leaves (LR with GLM, χ2 = 381.26, df = 2, P< 0.0001, Figure 1B1). Further, a significantly higher oviposition index was recorded on intact than GWF200-fully-infested tomato plants (LR with GLM, χ2 = 21.79, df = 1, P< 0.0001, Figure 1B2), with a significantly higher index found in the basal stratum of GWF200-fully-infested tomato plants (LR with GLM, χ2 = 200.66, df = 5, P< 0.0001, Figure 1B2). In paired assays with GWF200-partially-infested and GWF200-fully-infested tomato plants, a relatively higher oviposition index was recorded on GWF200-partially-infested tomato plants (LR with GLM, χ2 = 72.17, df = 1, P< 0.0001, Figure 1B3). Overall, significantly highest oviposition indices were recorded in the basal stratum of both treatment plants than apical and middle strata (LR with GLM, χ2 = 462.28, df = 2, P< 0.0001, Figure 1B3).
Parasitism rate of Phthorimaea absoluta larvae by Bracon nigricans female wasp
The parasitism rate of B. nigricans wasps on P. absoluta larvae differed significantly across the plant strata (ANOVA, F = 20.85, df = 5, P< 0.0001, Figure 1C). Overall, the parasitism rate was highest in middle and apical strata than basal stratum (ANOVA, F = 38.19, df = 2, P< 0.0001, Figure 1C). The lowest parasitism rate of P. absoluta larvae was observed on plants infested with T. vaporariorum (GWF200-infested) plants than on GWF-free plants (ANOVA, F = 14.92, df = 1, P< 0.0001, Figure 1C).
Olfactory responses of Phthorimaea absoluta to Trialeurodes vaporariorum-infested tomato plants
Females of P. absoluta significantly preferred odors from intact tomato plant than the air (control) (Combination A; Binomial test, N = 56, P< 0.001; Figure 3A). Likewise, female P. absoluta significantly preferred odors from intact tomato plants over tomato plants infested with conspecific larvae (TPW-infested) (Combination G; Binomial test, N = 86, P< 0.05, Figure 3A). Contrastingly, no discernible preference was found for plants co-infested with adults of T. vaporariorum and first instar larvae of P. absoluta (GWF|TPW-infested) when these treatments were compared to air control (Combination D; Binomial test, N = 86, P > 0.05, Figure 3A), and intact plant odor (Combination F; Binomial test, N = 94, P > 0.05, Figure 3A). Interestingly, odors released by plants infested with T. vaporariorum (GWF-infested) were more attractive to P. asboluta females than to the odors from tomato plants infested with conspecific larvae (Combination I; Binomial test, N = 95, P< 0.001, Figure 3A), and tomato plants co-infested with T. vaporariorum and P. absoluta (Combination J; Binomial test, N = 92, P< 0.01, Figure 3A).
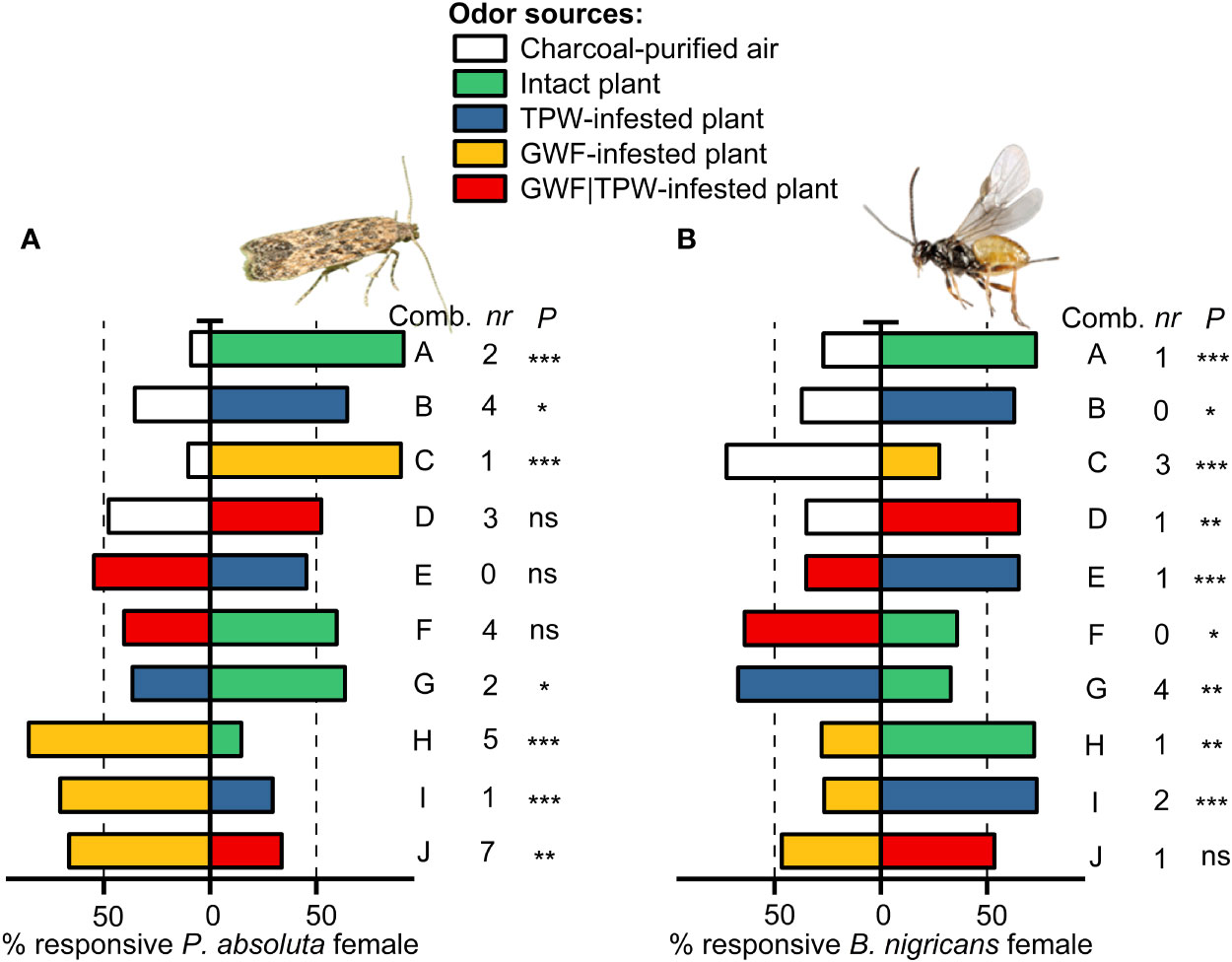
Figure 3 Dual olfactory choice of Phthorimaea absoluta and Bracon nigricans. The olfactory responses of P. absoluta and B. nigricans to odors of intact tomato plants or plants infested with adults T. vaporariorum or larvae T. absoluta or with both insects were evaluated in a Y-tube olfactometer. The percentages of females that chose either of the odor sources are shown in bars with asterisks indicating significant differences from an exact binomial test at: *P< 0.05, **P< 0.01, ***P< 0.001. ns = not significant (P >0.05). Non-respondents (nr) were excluded from the analysis. (A) Olfactory preferences of female P. absoluta towards intact and herbivore-infested tomato plant odor sources. (B) Olfactory preferences of female B. nigricans towards intact and different herbivore-treated tomato plant odor sources. Refer to Table 1 for details regarding the combinations tested.
Olfactory responses of parasitoid Bracon nigricans to Trialeurodes vaporariorum-infested tomato plants
Female B. nigricans preferred intact plant odors over air (Combination A; Binomial test, N = 74, P< 0.001; Figure 3B). They also showed a significant response to P. asboluta (TPW)-infested tomato plant odors compared to air (Combination B; Binomial test, N = 78, P< 0.05; Figure 3B). Interestingly, the proportion of responses to T. vaporariorum (GWF)-infested tomato plant odors was significantly lower than the proportion of responses to purified air (Combination C; Binomial test, N = 80, P< 0.001; Figure 3B). The wasps responded significantly to co-infested tomato plant odors, with a higher response rate to GWF | TPW co-infested tomato plant odors than purified air (Combination D; Binomial test, N = 80, P< 0.05; Figure 3B). Furthermore, B. nigricans showed a higher response to TPW-infested tomato plant odors than to odors from co-infested (Combination E; Binomial test, N = 74, P< 0.05) tomato plants, intact (Combination G; Binomial test, N = 76, P< 0.05), and GWF-infested tomato plants (Combination I; Binomial test, N = 75, P< 0.0001; Figure 3B). However, they were more attracted to co-infested tomato plant odors than intact tomato plant odors (Combination F; Binomial test, N = 78, P< 0.001; Figure 3B), and were more attracted to intact tomato plant odors than GWF-infested tomato plant odors (Combination H; Binomial test, N = 79, P< 0.001; Figure 3B). The parasitoid responded similarly to GWF-infested tomato plant odors and co-infested tomato plant odors (Combination J; Binomial test, N = 73, P > 0.05; Figure 3B).
GC-MS analysis of intact and herbivore-infested tomato plant volatiles
A total of 40 compounds were detected in intact and herbivore-infested tomato plants (Table 3). There were six green leaf volatiles (GLVs), three plant defense elicitors (PDEs), three alcohols, 17 monoterpenes, nine sesquiterpenes and two unidentified compounds (Table 3). Overall, GLVs and PDEs were upregulated in herbivore-infested tomato plants compared with intact tomato plant odors (Table 3, Figure 4). However, the levels of specific compounds including unidentified compound 2, (E)-2-hexenal, (Z)-3-hexenol, 3,6,6-trimethyl-cyclohex-2-enol, 1-octen-3-ol, and the terpenes β-myrcene, δ-3-carene, (Z)-β-ocimene, methyl salicylate, β-elemene, (E)-caryophyllene, β-guaiene, α-humulene, bicyclogermacrene, germacrene D, and germacrene B were also upregulated by herbivory caused by T. vaporariorum. (Table 1).
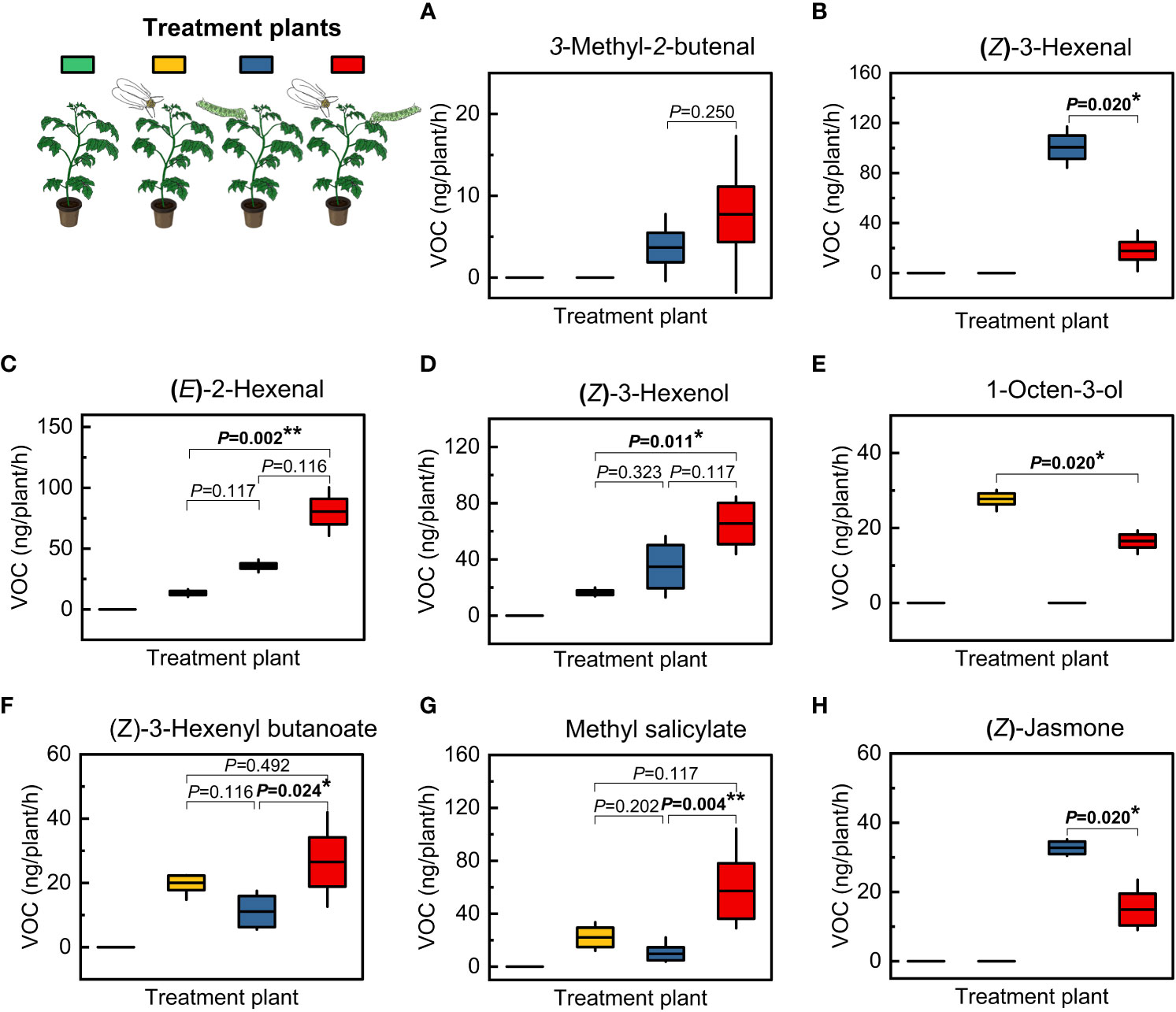
Figure 4 Amount (ng/plant/h) of GLVs and PDEs released by intact and herbivore-infested tomato plants. Treatment plants included intact plants, T. vaporariorum (GWF)-infested plants, P. absoluta (TPW)-infested plants, and GWF|TPW-infested plants. Each box represents the amount (ng/plant/h) of individual GLVs and PDEs per treatment plant. These compounds included: (A) 3-Methyl-2-butenal; (B) (Z)-3-Hexenal; (C) (E)-2-Hexenal; (D) (Z)-3-Hexenol; (E) 1-Octen-3-ol; (F) (Z)-Hexenyl butanoate; (G) Methyl salicylate; (H) (Z)-Jasmone. Treatment plants that did not emit a VOC were excluded from the statistics. The mean is represented by the line within the boxes, and the standard deviations are shown by whiskers (plus–minus). Bold numbers above the boxes indicate a significant difference in the volatile compounds emitted by the treatment plants (Kruskal-Wallis test at *, P<0.05; **, P<0.01; N = 4).
GC-EAD responses of Phthorimaea absoluta and Bracon nigricans to Trialeurodes vaporariorum-induced volatile compounds
In GC-EAD recordings, P. absoluta and B. nigricans detected 18 and 15 components, respectively (Figure 5). The EAG-active components were identified as (Z)-3-hexenol (5), α-pinene (9), 6-methy-5-hepten-2-one (sulcatone) (11), β-myrcene (15), δ-2-carene (16), δ-3-carene (18), α-terpinene (19), (Z)-β-ocimene (22), methyl salicylate (29), germacrene D (38), However, P. absoluta detected additional components including: unidentified (3), 1-octen-3-ol (14), (Z)-β-ocimene (23), γ-terpinene (24), terpinolene (25), linalool (26), (Z)-3-hexenyl butanoate (27), and germacrene B (40), whereas B. nigricans detected (E)-2-hexenal (4), β-phellandrene (21), and β-elemene (32), (E)-caryophyllene (34), β-selinene (39), and (Figure 5; Table 1). Except for the unidentified (3), sulcatone (11), linalool (26), β-elemene (32), germacrene D (38), β-selinene (39), and germacrene B (40), the EAD-active components were all confirmed with their synthetic authentic standards.
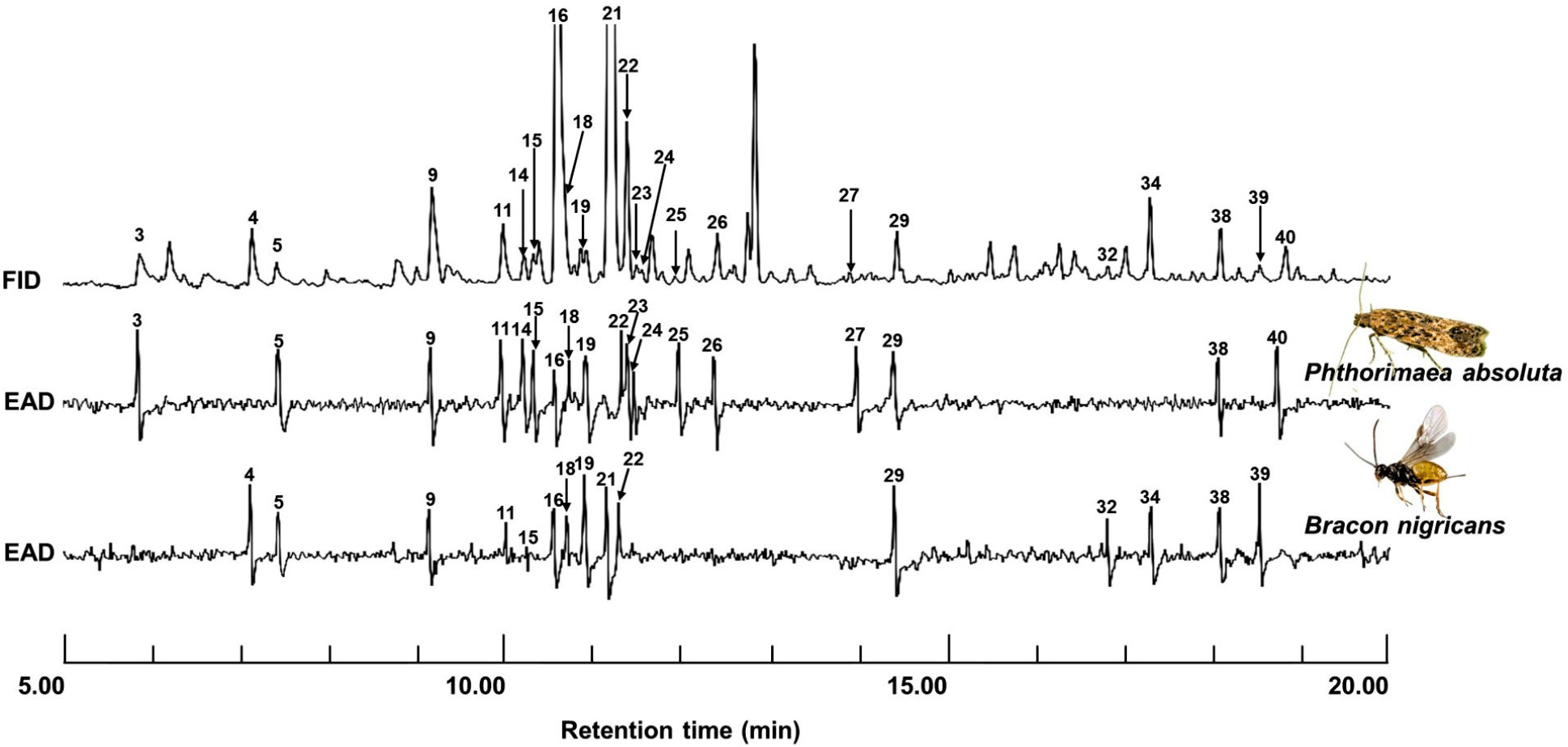
Figure 5 GC-EAG-active compounds from Trialeurodes vaporariorum-induced tomato volatile. The flame ionization detector (FID) and female P. absoluta and B. nigricans antennae (EAD) were used to detect volatile compounds from T. vaporariorum-infested tomato plants. The following EAD-active compounds were identified for P. absoluta: unidentified (3), (Z)-3-hexenol (5), α-pinene (9), sulcatone (11), 1-octen-3-ol (14), β-myrcene (15), δ-2-carene (16), δ-3-carene (18), α-terpinene (19), (Z)-β-ocimene (22), (Z)-β-ocimene (23), γ-terpinene (24), terpinolene (25), linalool (26), (Z)-3-hexenyl butanoate (27), methyl salicylate (29), germacrene D (38), and germacrene B (40). Bracon nigricans detected: (E)-2-hexenal (4), (Z)-3-hexenol (5), α-pinene (9), sulcatone (11), β-myrcene (15), δ-2-carene (16), δ-3-carene (18), α-terpinene (19), β-phellandrene (21), (Z)-β-ocimene (22), methyl salicylate (29), and β-elemene (32), (E)-caryophyllene (34), germacrene B (38), and β-selinene (39).
Olfactory responses of Phthorimaea absoluta and Bracon nigricans to synthetic compounds
Phthorimaea absoluta responses to synthetic compounds
All the concentrations tested for the GLVs (Z)-3-hexenol (20, 10, 5 ng/µL; Figure 6A) and 1-octen-3-ol (56, 28, and 14 ng/µL; Figure 6C) were attractive to females of P. absoluta (Binomial test, N = 69, P< 0.0001; Figure 6). However, methyl salicylate elicited attraction of P. absoluta females at half the natural concentration (11 ng/µL) (Binomial test, N = 75, P< 0.001, Figure 6F). In contrast, (Z)-3-hexenyl butanoate (40, 20, 10 ng/µL; Figure 6J) was avoided by P. absoluta at all tested concentrations (Binomial test, P< 0.05, Figure 6). Additionally, P. absoluta was indifferent to the natural concentration (22 ng/L) of methyl salicylate (Binomial test, N = 74, P >0.05, Figure 6F), all the concentrations (15, 30, 60 ng/L) of myrcene (Binomial test, N = 68–72, P >0.05, Figure 6D) tested, and to the natural and half the natural concentration (11, 22 ng/L) of γ-terpinene (Binomial test, N = 73, P >0.05, Figure 6I). However, P. absoluta avoided double the natural concentration of γ-terpinene (44 ng/L) (Binomial test, N = 75, P <0.001, Figure 6I). Phthorimaea absoluta female was significantly attracted to the terpenes: α-pinene at natural and half the natural concentration (99, and 45.5 ng/µL; Figure 6B); δ-2-carene at half concentration of (432 ng/µL; Figure 6E); δ-3-carene at the natural and double the natural concentration (60, and 120 ng/µL; Figure 6F); α-terpinene at half and double the natural concentration (27.5 ng/µL; Figure 6G); and β-ocimene (34, and 68 ng/µL; Figure 6H) at the natural and double the natural concentration (Binomial test, N = 71–75, P< 0.05; Figure 6H. In contrast, both δ-2-carene and methyl salicylate were avoided at double their natural concentrations; 1728 ng/µL (Figure 6C) and 44 ng/µL (Figure 6K), respectively by females of P. absoluta (Binomial test, N = 71–75, P< 0.05, Figure 6J).
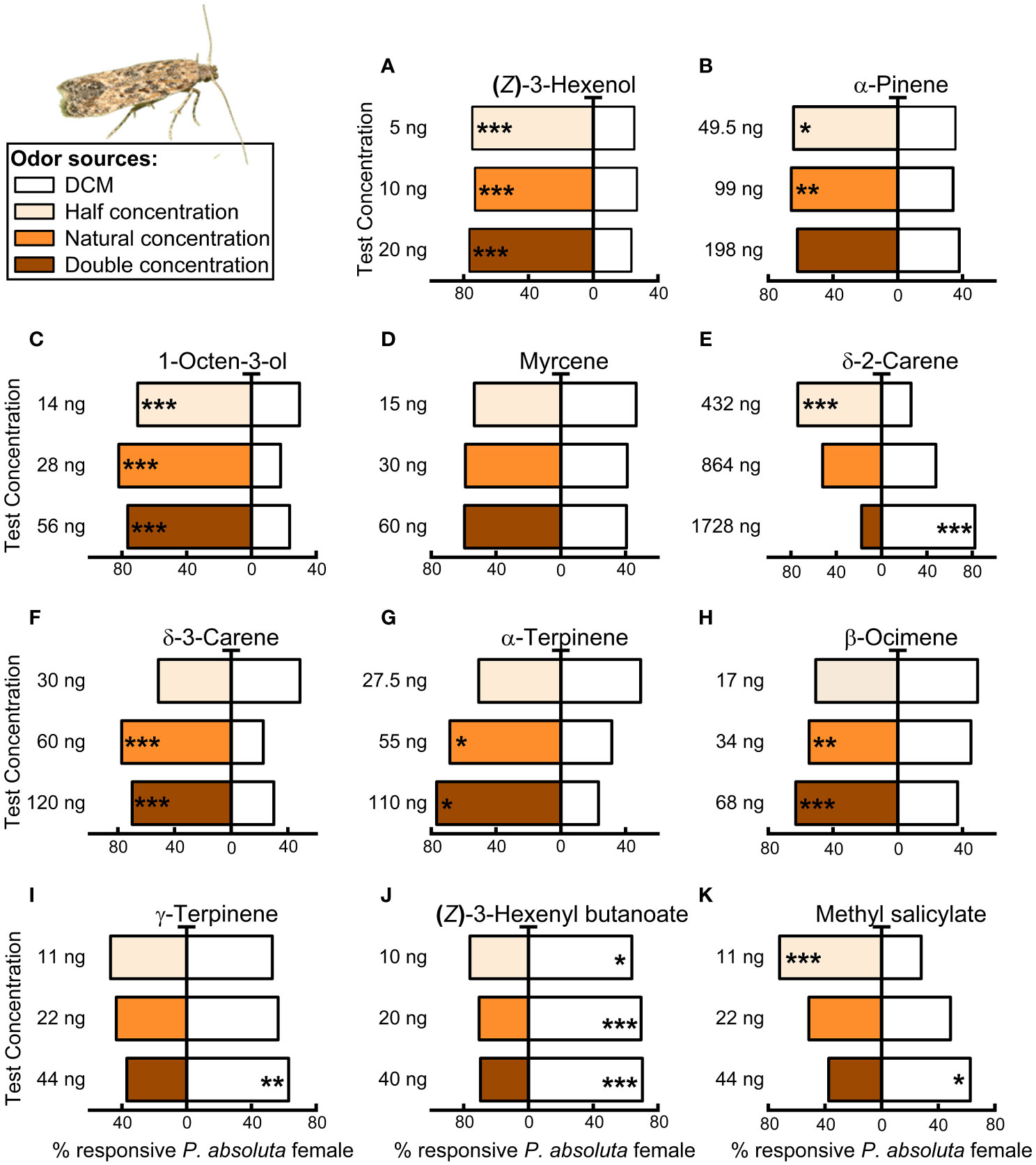
Figure 6 Olfactory responses of female Phthorimaea absoluta to synthetic compounds in a Y-tube olfactometer. These compounds included: (A) (Z)-3-Hexenol; (B) α-Pinene; (C) 1-Octen-3-ol; (D) Myrcene; (E) δ-2-Carene; (F) δ-3-Carene; (G) α-Terpinene; (H) β-Ocimene; (I) γ-Terpinene; (J) (Z)-3-hexenyl butanoate; (K) Methyl salicylate. Bars represent the percentages of insect choosing either of the odor sources. Asterisks indicate significant differences from a binomial test (*P< 0.05, **P< 0.01, ***P< 0.001; N = 69–75 moths and wasps).
Phthorimaea absoluta females were significantly attracted to a blend of all 11 compounds at their natural concentrations (Blend TPW2), and to with half the natural concentration of Blend TPW1 and double the natural concentration of Blend TPW3 (Binomial test, N = 75, P< 0.05, Figure 7A). However, when compared to a blend of exclusively attractive compounds (Blend Atr.; see Table 3), females showed no significant preference to the blends (Binomial test, N = 75, P< 0.05, as depicted in Figure 7B).
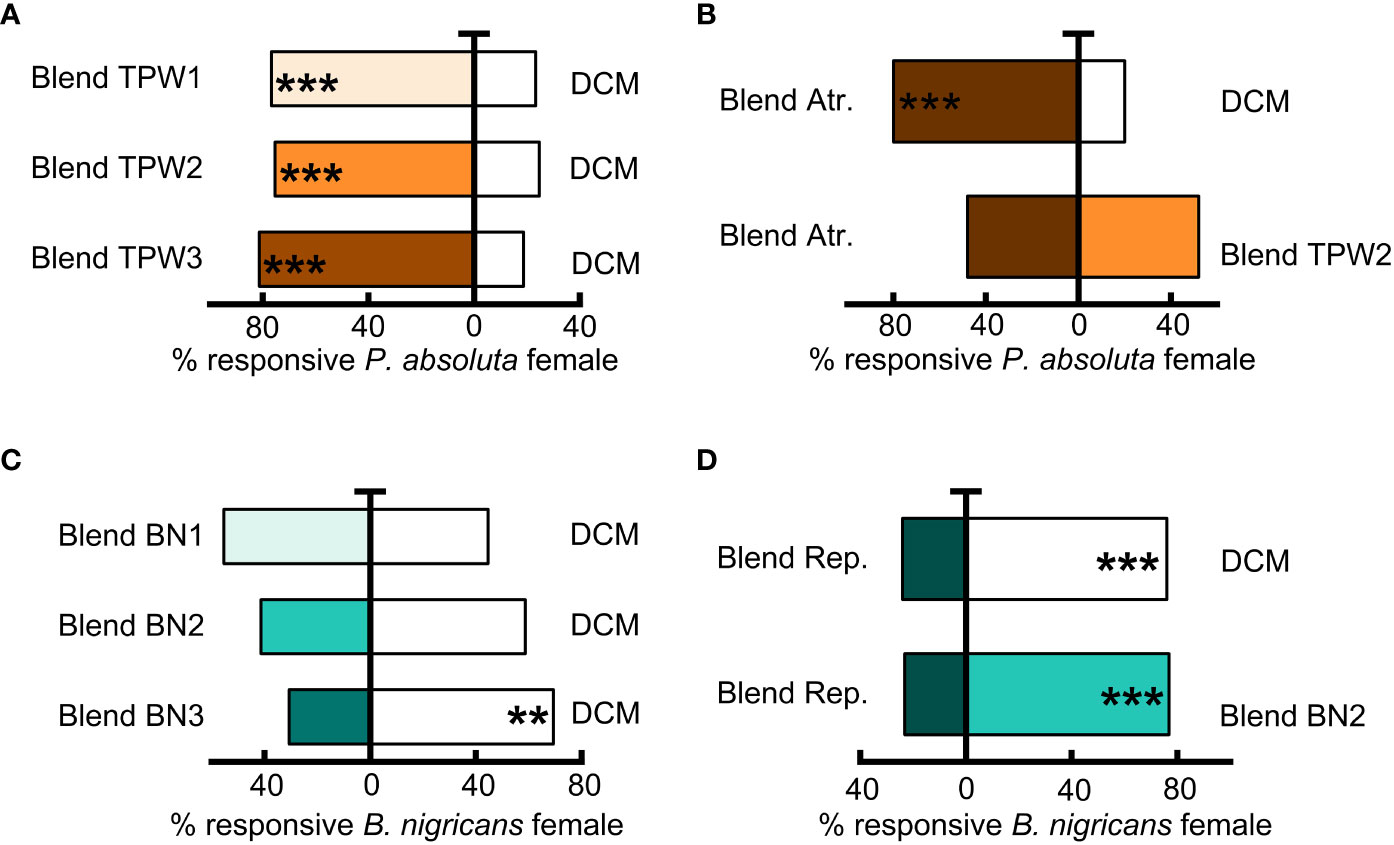
Figure 7 Olfactory responses of females Phthorimaea absoluta and Bracon nigricans to synthetic compounds in a Y-tube olfactometer. The components of each blend are list in Table 2. (A) Testing of Blend TPW1, Blend TPW1, and Blend TPW1 for P. absoluta responses. (B) Testing of Blend Atr. P. absoluta responses. (C) Testing of Blend BN1, Blend BN2, and Blend BN3 for Bracon nigricans responses. (D) Testing of Blend Rep. for B. nigricans responses.
Bracon nigricans responses to synthetic compounds
The females of B. nigricans showed varied responses to different VOCs and concentrations (Figures 8A–K). The parasitoid was attracted to (Z)-3-hexenol (15.5, 31, 62 ng/µL; Figure 8B), α-pinene (49.5, 99, 198 ng/µL; Figure 8C) at all the concentrations tested (Binomial test, N = 74 –75, P< 0.05; Figure 8). However, only double the natural concentrations of δ-2-carene, β-ocimene and methyl salicylate, were attractive to the parasitoid (Binomial test, N = 71–75, P< 0.05, Figures 8E, I, J). On the other hand, (E)-2-hexenal and (E)-caryophyllene were significantly avoided by B. nigricans females at all concentrations tested (Binomial test, P< 0.001, N = 75, Figures 8A, K). However, the female wasp avoided δ-3-carene at the natural and double the natural concentration (60, and 120 ng/μL; Binomial test, P< 0.05, N = 73–75, Figure 8F). Only the double of the natural concentrations of myrcene (60 ng/µL, Figure 8D), α-terpinene (110 ng/µL, Figure 8G), and β-phellandrene (4086 ng/µL, Figure 8H) were significantly avoided by B. nigricans females (Binomial test, P< 0.01, N = 73–75, Figures 8).
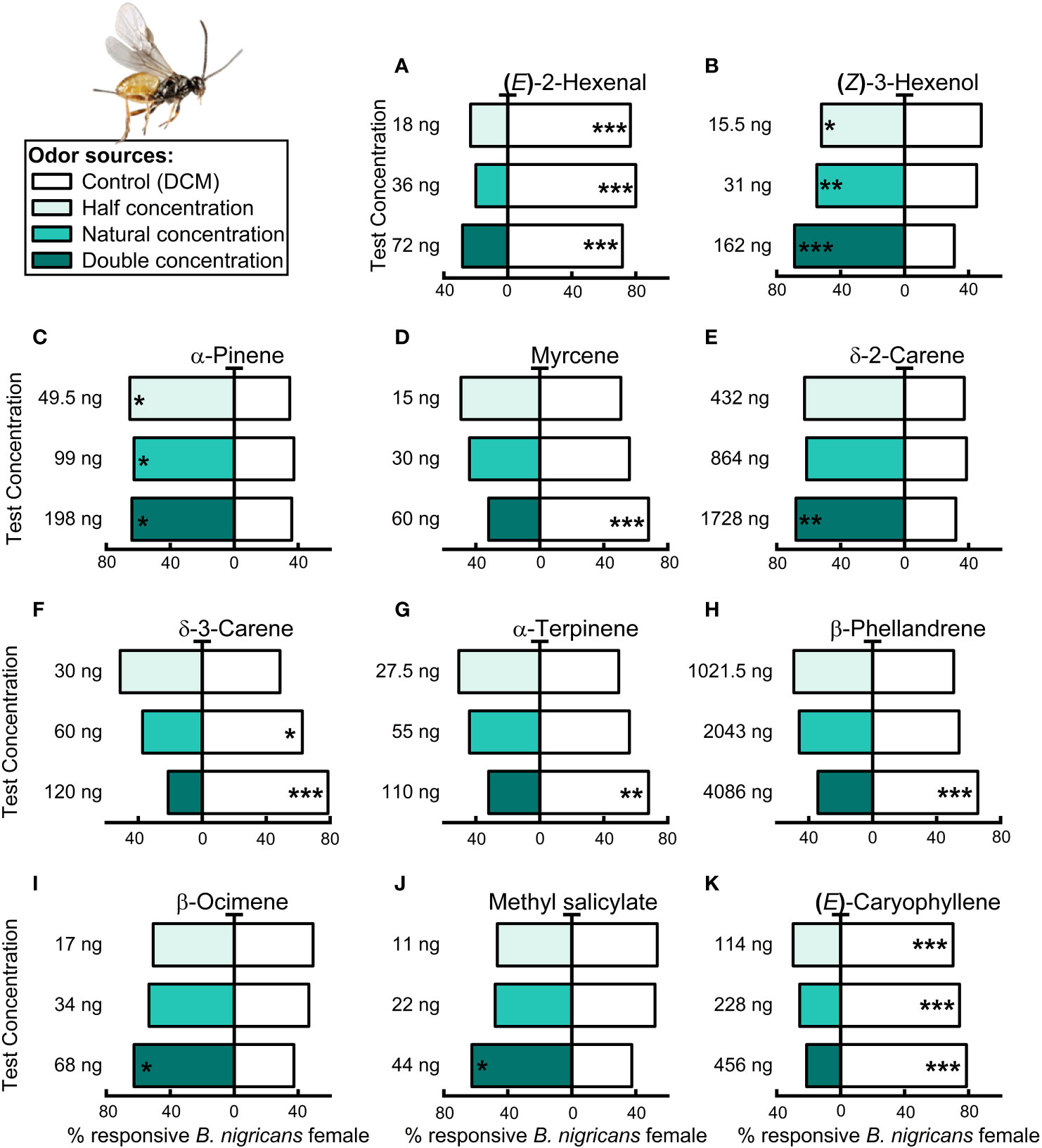
Figure 8 Olfactory responses of female parasitoid Bracon nigricans to synthetic compounds in a Y-tube olfactometer. These compounds included: (A) (E)-2-Hexenal; (B) (Z)-3-Hexenol; (C) α-Pinene; (D) Myrcene; (E) γ-2-Carene; (F) γ-3-Carene; (G) α-Terpinene; (H) β-Phellandrene; (I) β-Ocimene; (J) Methyl salicylate; (K) (E)-Caryophyllene. Bars represent the percentages of insect choosing either of the odor sources. Asterisks indicate significant differences from a binomial test (*P< 0.05, **P< 0.01, ***P< 0.001; N = 69–75 moths and wasps).
The female wasp did not show a significant preference for a blend of naturally-released concentrations or half the natural concentrations (Blend BN2 and Blend BN1, respectively) (Binomial test, P> 0.05, N = 75, Figure 7C). However, it significantly avoided a blend with double the natural concentration (Blend BN3) (Binomial test, P< 0.01, N = 75, Figure 7C). A blend of all avoided compounds at their natural released concentrations (Blend Rep.) was also significantly avoided (Binomial test, P< 0.001, N = 72, 75, Figure 7D) when compared to the DCM control and the initial blend of the 11 compounds (Blend BN2).
Discussion
The biological responses of P. absoluta and its associated parasitoid B. nigricans to T. vaporariorum infestation of tomato plants were investigated. Our findings revealed that, unlike intact plants, tomato plants infested with adults T. vaporariorum influenced the oviposition site selection of P. absoluta, causing a shift from the middle and apical strata to the basal stratum. This change in the oviposition pattern of P. absoluta corresponded with the rise in T. vaporariorum infestation density. Moreover, olfactory assays show that tomato plants with T. vaporariorum infestations were more attractive to gravid P. absoluta than intact plants, conspecifically-infested plants, and co-infested T. vaporariorum plants. Intriguingly, B. nigricans, the ectoparasitoid of P. absoluta, avoids T. vaporariorum-infested tomato plants. Specific compounds from green leaf volatiles and terpenoid groups were identified through GC-EAD recordings and GC-MS and olfactory bioassays as mediators of these interactions.
In community ecology, the concept of the struggle for existence is well-documented. For instance, according to Gause’s competitive exclusion principle, intraguild competition is inevitable between herbivore insects sharing the same niche (Kaplan and Denno, 2007; Bird et al., 2019). Our results suggest that P. absoluta and T. vaporariorum responses align with this theory. Indeed, in the absence of any intraguild species, females of the two herbivores lay their eggs on young leaves of tomato plants for fitness benefits for their progeny, including access to a nutrient-rich food source and protection from predators and parasites during early development stages when their eggs are laid on young tomato leaves in the absence of intraguild species (Bernays and Chapman, 1994; Collatz and Dorn, 2013). We found this egg-laying pattern as vulnerable to intraguild competition. For instance, the co-occurrence of P. absoluta and T. vaporariorum on tomato plant created competition for niche partitioning, whereby P. absoluta shifted from its preferred oviposition site, the middle and apical plant strata to basal plant stratum in the presence of the intraguild competitor, T. vaporariorum to assure survival of their progenies, as previously observed for certain insects (Dixon, 1985; Bernays and Chapman, 1994; Collatz and Dorn, 2013).
These findings suggest that the within-host shift of P. absoluta could be beneficial to the herbivore. First, T. vaporariorum infestation not only prompted the within-plant oviposition shift in P. absoluta, but also improved its general oviposition performance. This lends credence to earlier evidence indicating that phloem-feeding insects may boost insect pest reproduction (Rodriguez-Saona et al., 2005; Zhang et al., 2009; Soler et al., 2012; Liu et al., 2021). Rodriguez-Saona et al. (2005) observed that the beet armyworm Spodoptera exigua preferred tomato plants injured by the aphid Macrosiphum euphorbiae for oviposition. Likewise, Soler et al. (2012) found that earlier phloem feeder infestation improved secondary feeder (i.e., leaf-chewers) bionomics. Despite the increased oviposition observed for P. absoluta, it would be interesting to study the survival of these eggs and the feeding performance of the larvae on T. vaporariorum-infested tomato plants. Such a study would help determine whether tomato infestation by T. vaporariorum has a positive effect on P. absoluta. Furthermore, the within-plant parasitism pattern of B. nigricans suggests that a change in P. absoluta oviposition may be an evolutionary strategy to avoid parasitism (Bernays and Chapman, 1994).
Our olfactory bioassays showed that female P. absoluta was more attracted to T. vaporariorum-infested tomato plants than to intact ones. This implies that semiochemicals play a crucial role in shaping the oviposition choice and performance of P. absoluta on T. vaporariorum-infested tomato plants. Similar results were observed by Liu et al. (2021) in C. suppressalis after N. lugens infestation of rice plants, which they attributed to semiochemical emission. However, we suggest that these infochemicals not only affect the overall appeal of P. absoluta to T. vaporariorum-infested tomato plants but also their within-plant dispersion and the activities of B. nigricans. In choice-oviposition experiments, for instance, P. absoluta preferred T. vaporariorum-infested plants to intact ones and T. vaporariorum-protected leaves to T. vaporariorum-exposed ones, unlike its parasitoid B. nigricans. This finding provides evidence for the semiochemical mediation of not only intraguild interactions but also implications for higher trophic levels. The lower preference of B. nigricans for odors from tomato plants infested with T. vaporariorum compared to intact tomato plant odors and those infested with P. absoluta larvae may be due to several factors. One possible explanation is that the parasitoids are adapted to recognize specific cues or a “volatile signature” emitted by their preferred host, P. absoluta larvae-infested plants (Soler et al., 2012; Conchou et al., 2019). Hence, they may not be as receptive to the odors from other pests like T. vaporariorum. Additionally, the volatile signature of T. vaporariorum may signal to B. nigricans that there is a non-host present, which may attract other competitive parasitoids or hyperparasitoid species targeting whiteflies. Furthermore, our results show that the parasitoids responded more strongly to co-infested tomato plant odors than to charcoal-purified air, and intact plants, suggesting that they can differentiate between the odors of tomato plants infested with different pests using chemical cues.
In the present study, we identified specific compounds through GC-EAD recordings as mediators of these interesting tritrophic interactions. Indeed, both the herbivore P. absoluta and its parasitoid B. nigricans responded to a panoply of VOCs, including GLVs such as (Z)-3-hexanal, (Z)-3-hexenol, and (Z)-3-hexenyl butanoate, as well as methyl salicylate and terpenes including α-pinene, δ-2-carene, δ-3-carene, and (E)-caryophyllene, among others. Interestingly, these compounds were not identified as EAD-active for P. absoluta in a previous study by Miano et al. (2022), which compared wild tomato (var. cerasiforme) and cultivated tomato (var. Rambo F1). This highlights the importance of variations in the qualitative and quantitative compositions of headspace volatile blends and individual compounds, which can greatly influence insect responses. These variables are influenced by a range of environmental circumstances, the plant variety, herbivory or physical damage, or lack thereof, and herbivore feeding style (Zhang et al., 2009; Ayelo et al., 2021a; Ayelo et al., 2022). Additional findings from the olfactory bioassays indicate that certain compounds are accountable for the response of female P. absoluta towards tomato plants infested with T. vaporariorum, including (Z)-3-hexenol, 1-octen-3-ol, α-pinene, δ-2-carene, δ-3-carene, α-terpinene, and β-ocimene. Although there is extensive research on plant volatiles and their impact on insect behavior, there is limited research specifically exploring the attractiveness of P. absoluta to the aforementioned volatile compounds. Nonetheless, Agbessenou et al. (2022) demonstrated that P. absoluta was attracted to α-pinene, δ-2-carene, and β-phellandrene when tested individually. Our findings strongly support the notion that these compounds may play a critical role in attracting P. absoluta, as evidenced by the attraction of the female moth of the pest to a combination of these compounds compared to a blend of most of the compounds initially detected by the antennae of the pest. Additionally, the fact that (Z)-3-hexenol, 1-octen-3-ol, δ-2-carene, and β-ocimene are upregulated in response to T. vaporariorum herbivory highlights their importance in shaping the intraguild interactions between T. vaporariorum and P. absoluta. Zhang et al. (2009) showed similar results of upregulation of these compounds due to phloem feeding insects in their study on interactions between spider mites and silverleaf whiteflies in Lima beans, which resulted in a reduced attraction of predatory mites. However, our findings suggest that the upregulation of these compounds does not directly affect the performance of the parasitoid B. nigricans. Instead, we found (E)-2-hexenal, δ-3-carene, and (E)-caryophyllene as compounds contributing to the avoidance of B. nigricans to T. vaporariorum-infested tomato plants.
The parasitoid B. nigricans exhibits distinct olfactory preferences when it comes to volatile compounds emitted by tomato plants infested with T. vaporariorum. These preferences shed light on how the parasitoid locates hosts amidst a variety of different odors. Specifically, B. nigricans is strongly attracted to (Z)-3-hexenol, δ-2-carene, methyl salicylate, and β-ocimene at higher concentrations, indicating that these compounds likely function as host location cues, as suggested by earlier studies (Turlings and Wäckers, 2004; Ayelo et al., 2021b). However, these compounds are unable to counteract the avoidance/repellence effect of (E)-2-hexenal, (E)-caryophyllene, and δ-3-carene on B. nigricans. Moreover, even naturally-released concentrations of these and other compounds, such as myrcene, α-terpinene, and β-phellandrene, at higher concentrations cause the female wasp to avoid them. As such, these volatiles are unlikely to serve as suitable cues for host location for B. nigricans. Olfactory bioassays reveal that B. nigricans can distinguish between various volatile compounds and blend concentrations released by host-infested tomato plants. Interestingly, B. nigricans did not show any significant preference for blends with natural or half concentrations, instead, actively avoided blends with double the natural concentrations. This finding underscores the importance of concentration in influencing parasitoid behavior. Thus, the upregulation of these compounds by T. vaporariorum herbivory compared to intact tomato plants suggest that T. vaporariorum provide enemy-free space for P. absoluta on tomato plant by manipulating the emission of infochemicals (Soler et al., 2012; Liu et al., 2021).
Conclusions
The present study sheds light on the intricate interactions among herbivores and their associated parasitoids, and their impacts on plant fitness and pest management. Our findings suggest that phloem-feeding insects, such as T. vaporariorum, can alter the oviposition behavior of herbivores like P. absoluta, leading to niche partitioning and the redistribution of herbivore populations within plants. This has important implications for pest management, as it suggests that the presence of one herbivore species can attract another, leading to increased pest pressure on crops. Additionally, the observed olfactory responses of the parasitoid B. nigricans to specific volatile compounds can inform the development of pest management strategies that utilize semiochemicals to disrupt the oviposition and feeding behaviors of herbivores and their associated natural enemies. Overall, our study highlights the complex nature of intraguild interactions and their potential impacts on higher trophic levels, underscoring the need for further research to better understand and manage these ecological interactions in agricultural systems.
Data availability statement
The raw data supporting the conclusions of this article will be made available by the authors, without undue reservation.
Author contributions
All authors designed the study. KF performed the experiments, analyzed the data, and drafted the manuscript. FK secured the funding. BT, AY, and FK supervised the study and contributed substantially to the revisions. All authors contributed to the article and approved the submitted version.
Funding
This research was funded by the African Union (AU) (Tuta IPM Project, Contract number: AURG II-2-123-2018) through the International Centre of Insect Physiology and Ecology (icipe).
Acknowledgments
The authors gratefully acknowledge the financial support for this research by the following organizations and agencies: the Swedish International Development Cooperation Agency (Sida); the Swiss Agency for Development and Cooperation (SDC); the Australian Centre for International Agricultural Research (ACIAR); the Federal Democratic Republic of Ethiopia; and the Government of the Republic of Kenya. This study was in partial fulfillment for the PhD degree of the first author and was supported by DAAD In-Region Postgraduate Scholarships.
Conflict of interest
The authors declare that the research was conducted in the absence of any commercial or financial relationships that could be construed as a potential conflict of interest.
Publisher’s note
All claims expressed in this article are solely those of the authors and do not necessarily represent those of their affiliated organizations, or those of the publisher, the editors and the reviewers. Any product that may be evaluated in this article, or claim that may be made by its manufacturer, is not guaranteed or endorsed by the publisher.
Author disclaimer
The views expressed herein do not necessarily reflect the official opinion of the donors.
References
Adams B., Yusuf A. A., Torto B., Khamis F. M. (2023). Non-host plant odors influence the tritrophic interaction between tomato, its foliar herbivore Tuta absoluta and mirid predator Nesidiocoris tenuis. Front. Plant Sci. 14. doi: 10.3389/fpls.2023.1014865
Agbessenou A., Akutse K. S., Yusuf A. A., Khamis F. M. (2022). The endophyte Trichoderma asperellum M2RT4 induces the systemic release of methyl salicylate and (Z)-jasmone in tomato plant affecting host location and herbivory of Tuta absoluta. Front. Plant Sci. 13. doi: 10.3389/fpls.2022.860309
Aigbedion-Atalor P. O., Hill M. P., Ayelo P. M., Ndlela S., Zalucki M. P., Mohamed S. A. (2021). Can the combined use of the mirid predator Nesidiocoris tenuis and a braconid larval endoparasitoid Dolichogenidea gelechiidivoris improve the biological control of Tuta absoluta? Insects 12. doi: 10.3390/INSECTS12111004
Ameye M., Allmann S., Verwaeren J., Smagghe G., Haesaert G., Schuurink R. C., et al. (2018). Green leaf volatile production by plants: a meta-analysis. New Phytol. 220, 666–683. doi: 10.1111/nph.14671
Arnó J., Albajes R., Gabarra R. (2006). Within-plant distribution and sampling of single and mixed infestations of Bemisia tabaci and Trialeurodes vaporariorum (Homoptera: aleyrodidae) in winter tomato crops. J. Econ. Entomol. 99, 331–340. doi: 10.1093/jee/99.2.331
Ayelo P. M., Yusuf A. A., Chailleux A., Mohamed S. A., Pirk C. W. W., Deletre E. (2022). Chemical cues from honeydew and cuticular extracts of Trialeurodes vaporariorum serve as kairomones for the parasitoid Encarsia formosa. J. Chem. Ecol. 48, 370–383. doi: 10.1007/s10886-022-01354-6
Ayelo P. M., Yusuf A. A., Pirk C. W. W., Chailleux A., Mohamed S. A., Deletre E. (2021a). Terpenes from herbivore-induced tomato plant volatiles attract Nesidiocoris tenuis (Hemiptera: miridae), a predator of major tomato pests. Pest Manage. Sci. 77, 5255–5267. doi: 10.1002/ps.6568
Ayelo P. M., Yusuf A. A., Pirk C. W. W., Mohamed S. A., Chailleux A., Deletre E. (2021b). The role of Trialeurodes vaporariorum-infested tomato plant volatiles in the attraction of Encarsia formosa (Hymenoptera: aphelinidae). J. Chem. Ecol. 47, 192–203. doi: 10.1007/s10886-021-01245-2
Bernays E. A., Chapman R. F. (1994). Host-plant selection by phytophagous insects (New York: Chapman & Hall, Inc.).
Biondi A., Desneux N., Amiens-Desneux E., Siscaro G., Zappalà L. (2013). Biology and developmental strategies of the palaearctic parasitoid Bracon nigricans (Hymenoptera: braconidae) on the neotropical moth Tuta absoluta (Lepidoptera: gelechiidae). J. Econ. Entomol. 106, 1638–1647. doi: 10.1603/EC12518
Biondi A., Guedes R. N. C., Wan F. H., Desneux N. (2018). Ecology, worldwide spread, and management of the invasive south American tomato pinworm, Tuta absoluta: past, present, and future. Annu. Rev. Entomol. 63, 239–258. doi: 10.1146/annurev-ento-031616-034933
Bird G., Kaczvinsky C., Wilson A. E., Hardy N. B. (2019). When do herbivorous insects compete? a phylogeneticmeta-analysis. Ecol. Lett. 22, 875–883. doi: 10.1111/ele.13245
Cocco A., Serra G., Lentini A., Deliperi S., Delrio G. (2014). Spatial distribution and sequential sampling plans for Tuta absoluta (Lepidoptera: gelechiidae) in greenhouse tomato crops. Pest Manage. Sci. 71, 1311–1323. doi: 10.1002/ps.3931
Collatz J., Dorn S. (2013). Tritrophic consequences arising from a host shift between apple and walnut in an oligophagous herbivore. Biol. Control 65, 330–337. doi: 10.1016/j.biocontrol.2013.03.011
Conchou L., Lucas P., Meslin C., Proffit M., Staudt M., Renou M. (2019). Insect odorscapes: from plant volatiles to natural olfactory scenes. Front. Physiol. 10. doi: 10.3389/fphys.2019.00972
De Moraes C. M., Mescher M. C., Tumlinson J. H. (2001). Caterpillar-induced nocturnal plant volatiles repel conspecific females. Nature 410, 577–579. doi: 10.1038/35069058
Dicke M., Baldwin I. T. (2010). The evolutionary context for herbivore-induced plant volatiles: beyond the “cry for help.” Trends Plant Sci. 15, 167–175. doi: 10.1016/j.tplants.2009.12.002
Dixon A. F. G. (1985). Aphid ecology an optimization approach. 2nd ed. (New York: Springer Science+Business Media).
Effah E., Barrett D. P., Peterson P. G., Potter M. A., Holopainen J. K., Clavijo McCormick A. (2021). Seasonal volatile emission patterns of the endemic new Zealand shrub Dracophyllum subulatum on the north island central plateau. Front. Plant Sci. 12. doi: 10.3389/fpls.2021.734531
Engelberth J., Alborn H. T., Schmelz E. A., Tumlinson J. H. (2004). Airborne signals prime plants against insect herbivore attack. Proc. Natl. Acad. Sci. U S A 101, 1781–1785. doi: 10.1073/pnas.0308037100
Ghosh S., Kanakala S., Lebedev G., Kontsedalov S., Silverman D., Alon T., et al. (2019). Transmission of a new polerovirus infecting pepper by the whitefly Bemisia tabaci. J. Virol. 93, 1–14. doi: 10.1128/jvi.00488-19
Gonring A. H. R., Walerius A. H., Picanço M. M., Bacci L., Martins J. C., Picanço M. C. (2020). Feasible sampling plan for Tuta absoluta egg densities evaluation in commercial field tomato. Crop Prot. 136, 105239. doi: 10.1016/j.cropro.2020.105239
Hassanpour M., Bagheri M., Golizadeh A., Farrokhi S. (2016). Functional response of Nesidiocoris tenuis (Hemiptera: miridae) to Trialeurodes vaporariorum (Hemiptera: aleyrodidae): effect of different host plants. Biocontrol Sci. Technol. 26, 1489–1503. doi: 10.1080/09583157.2016.1216521
Hu X., Su S., Liu Q., Jiao Y., Peng Y., Li Y., et al. (2020). Caterpillar-induced rice volatiles provide enemy-free space for the offspring of the brown planthopper. Elife 9, 1–19. doi: 10.7554/eLife.55421
Inbar M., Gerling D. (2008). Plant-mediated interactions between whiteflies, herbivores, and natural enemies. Annu. Rev. Entomol. 53, 431–448. doi: 10.1146/annurev.ento.53.032107.122456
Kaplan I., Denno R. F. (2007). Interspecific interactions in phytophagous insects revisited: a quantitative assessment of competition theory. Ecol. Lett. 10, 977–994. doi: 10.1111/j.1461-0248.2007.01093.x
Khamis F. M., Ombura F. L. O., Ajene I. J., Akutse K. S., Subramanian S., Mohamed S. A., et al. (2021). Mitogenomic analysis of diversity of key whitefly pests in Kenya and its implication to their sustainable management. Sci. Rep. 11, 1–11. doi: 10.1038/s41598-021-85902-2
Lima C. H. O., Sarmento R. A., Galdino T. V. S., Pereira P. S., Silva J., Souza D. J., et al. (2018). Spatiotemporal dynamics of whitefly Bemisia tabaci (Hemiptera: aleyrodidae) in commercial watermelon crops. J. Econ. Entomol. 111, 1895–1903. doi: 10.1093/jee/toy110
Liu Q., Hu X., Su S., Ning Y., Peng Y., Ye G., et al. (2021). Cooperative herbivory between two important pests of rice. Nat. Commun. 12, 1–13. doi: 10.1038/s41467-021-27021-0
Miano R. N., Ayelo P. M., Musau R., Hassanali A., Mohamed S. A. (2022). Electroantennogram and machine learning reveal a volatile blend mediating avoidance behavior by tuta absoluta females to a wild tomato plant. Sci. Rep. 12, 1–16. doi: 10.1038/s41598-022-13125-0
Milenovic M., Wosula E. N., Rapisarda C., Legg J. P. (2019). Impact of host plant species and whitefly species on feeding behavior of Bemisia tabaci. Front. Plant Sci. 10. doi: 10.3389/fpls.2019.00001
Pappas M. L., Steppuhn A., Geuss D., Topalidou N., Zografou A., Sabelis M. W., et al. (2015). Beyond predation: the zoophytophagous predator macrolophus pygmaeus induces tomato resistance against spider mites. PloS One 10. doi: 10.1371/journal.pone.0127251
Piesik D., Lemńczyk G., Skoczek A., Lamparski R., Bocianowski J., Kotwica K., et al. (2011). Fusarium infection in maize: volatile induction of infected and neighboring uninfected plants has the potential to attract a pest cereal leaf beetle, Oulema melanopus. J. Plant Physiol. 168, 1534–1542. doi: 10.1016/j.jplph.2011.01.032
Piesik D., Wenda-Piesik A., Lamparski R., Tabaka P., Ligor T., Buszewski B. (2010). Effects of mechanical injury and insect feeding on volatiles emitted by wheat plants. Entomol. Fenn. 21, 117–128. doi: 10.33338/ef.84521
Poelman E. H., Dicke M. (2014). “Plant-mediated interactions among insects within a community ecological perspective,” in Ecology and evolution of insect-plant interactions. Eds. Voelckel C., Jander G. (Wiley-Blackwell Chichester, United Kingdom: Annual Plant Reviews), 309–337. doi: 10.1002/9781118472507.ch9
R Core Team (2022). R: a language and environment for statistical computing. (R Foundation for Statistical Computing, Vienna, Austria). Available at: https://www.r-project.org/.
Rodriguez-Saona C., Chalmers J. A., Raj S., Thaler J. S. (2005). Induced plant responses to multiple damagers: differential effects on an herbivore and its parasitoid. Oecologia 143, 566–577. doi: 10.1007/s00442-005-0006-7
Rodriguez-Saona C., Crafts-Brandner S. J., Cañas L. A. (2003). Volatile emissions triggered by multiple herbivore damage: beet armyworm and whitefly feeding on cotton plants. J. Chem. Ecol. 29, 2539–2550. doi: 10.1023/A:1026314102866
Rowen E., Kaplan I. (2016). Eco-evolutionary factors drive induced plant volatiles: a meta-analysis. New Phytol. 210, 284–294. doi: 10.1111/nph.13804
Sambo S. M., Ndlela S., du Plessis H., Obala F., Mohamed S. A. (2022). Identification, microhabitat, and ecological niche prediction of two promising native parasitoids of Tuta absoluta in Kenya. Insects 13, 496. doi: 10.3390/insects13060496
Soler R., Badenes-Pérez F. R., Broekgaarden C., Zheng S. J., David A., Boland W., et al. (2012). Plant-mediated facilitation between a leaf-feeding and a phloem-feeding insect in a brassicaceous plant: from insect performance to gene transcription. Funct. Ecol. 26, 156–166. doi: 10.1111/j.1365-2435.2011.01902.x
Sugimoto K., Iijima Y., Takabayashi J., Matsui K. (2021). Processing of airborne green leaf volatiles for their glycosylation in the exposed plants. Front. Plant Sci. 12. doi: 10.3389/fpls.2021.721572
Sugimoto K., Matsui K., Iijima Y., Akakabe Y., Muramoto S., Ozawa R., et al. (2014). Intake and transformation to a glycoside of (Z)-3-hexenol from infested neighbors reveals a mode of plant odor reception and defense. Proc. Natl. Acad. Sci. U S A 111, 7144–7149. doi: 10.1073/pnas.1320660111
Thaler J. S., Karban R., Ullman D. E., Boege K., Bostock R. M. (2002). Cross-talk between jasmonate and salicylate plant defense pathways: effects on several plant parasites. Oecologia 131, 227–235. doi: 10.1007/s00442-002-0885-9
Thompson R. M., Brose U., Dunne J. A., Hall R. O., Hladyz S., Kitching R. L., et al. (2012). Food webs: reconciling the structure and function of biodiversity. Trends Ecol. Evol. 27, 689–697. doi: 10.1016/j.tree.2012.08.005
Torres J. B., Faria C. A., Evangelista W. S. J., Pratissoli D. (2001). Within-plant distribution of the leaf miner Tuta absoluta (Meyrick) immatures in processing tomatoes, with notes on plant phenology. Int. J. Pest Manage. 47, 173–178. doi: 10.1080/02670870010011091
Turlings T. C. J., Erb M. (2018). Tritrophic interactions mediated by herbivore-induced plant volatiles: mechanisms, ecological relevance, and application potential. Annu. Rev. Entomol. 63, 433–452. doi: 10.1146/annurev-ento-020117-043507
Turlings T. C. J., Loughrin J. H., Mccall P. J., Röse U. S. R., Lewis W. J., Tumlinson J. H. (1995). How caterpillar-damaged plants protect themselves by attracting parasitic wasps. Proc. Natl. Acad. Sci. U S A 92, 4169–4174. doi: 10.1073/pnas.92.10.4169
Turlings T. C. J., Wäckers F. (2004). Recruitment of predators and parasitoids by herbivore-injured plants. Eds. Carde R. T., Millar J. G. (United Kingdom: Cambridge University Press). doi: 10.1017/cbo9780511542664.003
Ullah M. S., Lim U. T. (2016). Within-greenhouse and within-plant distribution of greenhouse whitefly, Trialeurodes vaporariorum (Hemiptera: aleyrodidae), in strawberry greenhouses. Appl. Entomol. Zool. 51, 333–339. doi: 10.1007/s13355-016-0394-7
Wang S., Brose U., Gravel D. (2019). Intraguild predation enhances biodiversity and functioning in complex food webs. Ecology 100, e02616. doi: 10.1002/ecy.2616
War A. R., Sharma H. C., Paulraj M. G., War M. Y., Ignacimuthu S. (2011). Herbivore induced plant volatiles:Their role in plant defense for pest management. Plant Signal. Behav. 6, 1973–1978. doi: 10.4161/psb.6.12.18053
Zhang P. J., Wei J. N., Zhao C., Zhang Y. F., Li C. Y., Liu S. S., et al. (2019). Airborne host–plant manipulation by whiteflies via an inducible blend of plant volatiles. Proc. Natl. Acad. Sci. U S A 116, 7387–7396. doi: 10.1073/pnas.1818599116
Zhang P. J., Zheng S. J., Van Loon J. J. A., Boland W., David A., Mumm R., et al. (2009). Whiteflies interfere with indirect plant defense against spider mites in Lima bean. Proc. Natl. Acad. Sci. U S A 106, 21202–21207. doi: 10.1073/pnas.0907890106
Zhao Q., Van den Brink P. J., Carpentier C., Wang Y. X. G., Rodríguez-Sánchez P., Xu C., et al. (2019). Horizontal and vertical diversity jointly shape food web stability against small and large perturbations. Ecol. Lett. 22, 1152–1162. doi: 10.1111/ele.13282
Keywords: intraguild interactions, competition, niche, green leaf volatiles, Trialeurodes vaporariorum
Citation: Fiaboe KR, Yusuf AA, Torto B and Khamis FM (2023) Herbivore intraguild interactions decrease ectoparasitoid Bracon nigricans parasitism of Phthorimaea absoluta and responses to tomato volatiles. Front. Ecol. Evol. 11:1200040. doi: 10.3389/fevo.2023.1200040
Received: 04 April 2023; Accepted: 05 June 2023;
Published: 04 July 2023.
Edited by:
Christopher M. Ranger, United States Department of Agriculture, United StatesReviewed by:
Radhika Venkatesan, Indian Institute of Science Education and Research (Kolkata), IndiaQi Su, Yangtze University, China
Copyright © 2023 Fiaboe, Yusuf, Torto and Khamis. This is an open-access article distributed under the terms of the Creative Commons Attribution License (CC BY). The use, distribution or reproduction in other forums is permitted, provided the original author(s) and the copyright owner(s) are credited and that the original publication in this journal is cited, in accordance with accepted academic practice. No use, distribution or reproduction is permitted which does not comply with these terms.
*Correspondence: Fathiya Mbarak Khamis, ZmtoYW1pc0BpY2lwZS5vcmc=; Baldwyn Torto, YnRvcnRvQGljaXBlLm9yZw==