- 1Department of Comparative, Diagnostic, and Population Medicine, College of Veterinary Medicine, University of Florida, Gainesville, FL, United States
- 2Loggerhead Marinelife Center, Juno Beach, FL, United States
- 3National Save The Sea Turtle Foundation, Ft. Lauderdale, FL, United States
Assessments of health variables in wild animal populations have evolved into important tools for characterizing spatiotemporal population trends and fitness, effects of stressors, diseases, and ecosystem health. Blood as a sample matrix can be obtained fairly non-invasively in the field, with preservation and sample processing techniques that allow for readily available routine and advanced diagnostic testing of blood. For wild-caught hawksbill sea turtles (Eretmochelys imbricata) foraging in southeastern Florida, USA, the objectives of this study were to (1) establish reference intervals for hematological and 24 plasma biochemical analytes, (2) determine length-and body condition-specific relationships with blood analytes, and (3) determine how water temperature influenced plasma biochemical analytes. Reference intervals were established for clinically normal juvenile (n = 26) and subadult (n = 39) hawksbills, with descriptive data reported for adult turtles (n = 3). Although subadults (mainly captured at Palm Beach County) were heavier and larger with greater body depth, juveniles (mainly captured at Monroe County) had a higher body condition index. Positive length-specific correlations were identified for packed cell volume, eosinophils, aspartate aminotransferase, phosphorus, cholesterol, glutamate dehydrogenase, total protein, albumin, and globulins, with negative correlations including alkaline phosphatase, creatine kinase, calcium, calcium to phosphorus ratio, and glucose. Subadults had less frequent morphological features of red blood cell regeneration compared to juveniles. These findings provide insight into life-stage class differences regarding hematopoiesis, antigenic stimulation, somatic growth, dietary shifts, nutritional status, osmoregulation, metabolism, physical activity or stress levels, and possible habitat differences. Life-stage class is the likely driver for the observed blood analyte differences, in addition to influences from water temperature. The data herein offer baseline information for a snapshot in time for critically endangered hawksbills inhabiting the Florida reef system and for answering individual-and population-relevant questions of relevance to conservation and population management.
1. Introduction
Six sea turtle species are known to occur in waters off the east and west coasts of Florida, USA (Foley et al., 2003; Eaton et al., 2008). After olive ridleys (Lepidochelys olivacea), the critically endangered (Mortimer and Donnelly, 2008) hawksbill sea turtle (Eretmochelys imbricata) is the rarest species to occur in Florida (Meylan and Redlow, 2006; Mortimer and Donnelly, 2008), with this aggregation being the second most northern of the Atlantic Ocean population (Meylan et al., 2011; Wood et al., 2013). The Atlantic hawksbill population appears to be genetically distinct from the Pacific population (Wood et al., 2013). Hawksbills are believed to occupy the Florida reef system due to warm water transport by the Florida current from the Gulf of Mexico and the Caribbean (Meylan and Redlow, 2006; Blumenthal et al., 2009). Although hawksbill nesting in Florida is uncommon (Meylan and Redlow, 2006), juvenile and subadult life-stage classes are known to inhabit Florida’s waters year-round (Wood et al., 2013, 2017). The Florida Continental Reef Tract provides refuge for hawksbills and is home to numerous sponges and octocoral species on which these animals forage (Moyer et al., 2003; Banks et al., 2008; Wood et al., 2017). Hawksbill turtles in Florida waters originate from Mexico and the Caribbean via the Florida Current and/or Gulf Stream, recruiting from oceanic to neritic habitats predominantly in the Florida Keys (i.e., Monroe County) as small juveniles. It is apparent that some move along the Southeast Florida Continental Reef Tract to eventually reach their northern terminus in Palm Beach County, Florida, as they advance through their subadult life-stage (Wood et al., 2013). Then, upon reaching sexual maturity, the young adults undertake reproductive migrations out of Florida, and are only seen transiently thereafter.
Despite the known occurrence of these animals in Florida, no assessment of overall health using blood analytes has occurred for this aggregation to date. Health assessments have been conducted for wild-caught hawksbills from Oman (nesting adults) (Alkindi et al., 2002), Brazil (nesting adults) (Goldberg et al., 2013), the Persian Gulf (nesting adults) (Ehsanpour et al., 2015), Australia (immature foraging) (Whiting et al., 2014), the Galápagos Archipelago (immature and adult foraging) (Muñoz-Pérez et al., 2017), Mexico (nesting adults) (Salvarani et al., 2018), Belize (immature) (Crooks et al., 2023) and in rehabilitating hawksbills from the United Arab Emirates (juvenile) (Hampel et al., 2009; Caliendo et al., 2010). Reference intervals using American Society for Veterinary Clinical Pathology (ASVCP) guidelines (Friedrichs et al., 2012) have only been established for one wild-caught hawksbill population in Australia (Whiting et al., 2014); however, several other hawksbill studies report measures of central tendency and full range of values (Goldberg et al., 2013; Ehsanpour et al., 2015; Salvarani et al., 2018). Establishment of blood analyte reference intervals in sea turtles is important, as various stressors that sea turtles face continue to intensify; therefore, health assessments are becoming increasingly valuable to conservation efforts as they provide baseline data for future population health and fitness comparisons in the face of environmental changes, increasing stressors, and potential disease outbreaks (Deem et al., 2001; Aguirre and Lutz, 2004; Wikelski and Cooke, 2006; Cooke and O’Connor, 2010; Deem and Harris, 2017; Reséndiz and Lara-Uc, 2018; Mashkour et al., 2020; Page-Karjian et al., 2020; Perrault et al., 2020), in addition to improving clinical decision-making in individual animals undergoing veterinary care (Delgado et al., 2011).
Coral cover in the Florida Continental Reef Tract, where hawksbills are known to inhabit, is declining at alarming rates due to climate change, disease, and deteriorating water quality (Ruzicka et al., 2013; Voosen, 2019; Neely et al., 2021); therefore, establishing baselines of hawksbill health variables is necessary to understand physiological responses of animals and future population and ecosystem challenges that may result from habitat degradation or loss. For wild-caught juvenile and subadult hawksbills foraging in southeastern Florida, USA, the objectives of this study were to (1) establish reference intervals for hematological and 24 plasma biochemical analytes, (2) determine length-and body condition-specific relationships with blood analytes, and (3) determine how water temperature influenced plasma biochemical analytes.
2. Materials and methods
2.1. Ethical procedures
This study was reviewed and authorized by the National Marine Fisheries Service (NMFS) [Permit #22988], Florida Fish and Wildlife Conservation Commission (FWC) [Marine Turtle Permits #021 and #077], Florida Keys National Marine Sanctuary [Research Permit #175], and University of Florida’s Institutional Animal Care and Use Committee (IACUC) [#201706823]. All handling and sampling procedures of sea turtles were performed according to NMFS and FWC regulations (NMFS SEFSC, 2008).
2.2. Capture technique, morphometrics, and sample collection, processing, and analysis
Hawksbill turtles were hand-captured during snorkel and/or scuba surveys between Jun 2017 and Oct 2020 along the 2–30 m deep nearshore reefs of the Southeast Florida Continental Reef Tract from Jupiter, Florida, USA (26.987°N, −80.033°W) to Key West, Florida, USA (23.673°N, −82.981°W) (Figure 1). The water temperature was recorded at the time and depth of each capture with a Sherwood Wisdom 2 (Sherwood Scuba®, Santa Ana, CA) dive computer while on scuba, and/or the vessel’s onboard surface water temperature gauge when snorkeling in water less than 9 m deep. Primarily, two counties in Florida were utilized for this study including Palm Beach (towns of Jupiter and West Palm Beach) and Monroe (towns of Islamorada and Key West), which are known foraging areas of this species in Florida (Meylan and Redlow, 2006; Wood et al., 2013, 2017). One turtle was captured in Miami, Florida, USA (Miami-Dade County) (25.690°N, −80.085°W). This individual was included in analyses of the Monroe County turtles due to the close proximity of the two locations. Turtles captured at depths exceeding 10 m (>1 atm) were brought to the surface at a rate of ≤9 m/min. Turtles were transferred to a research vessel within 20 min of capture and examined for the presence of internal passive integrated transponder (PIT) tags.
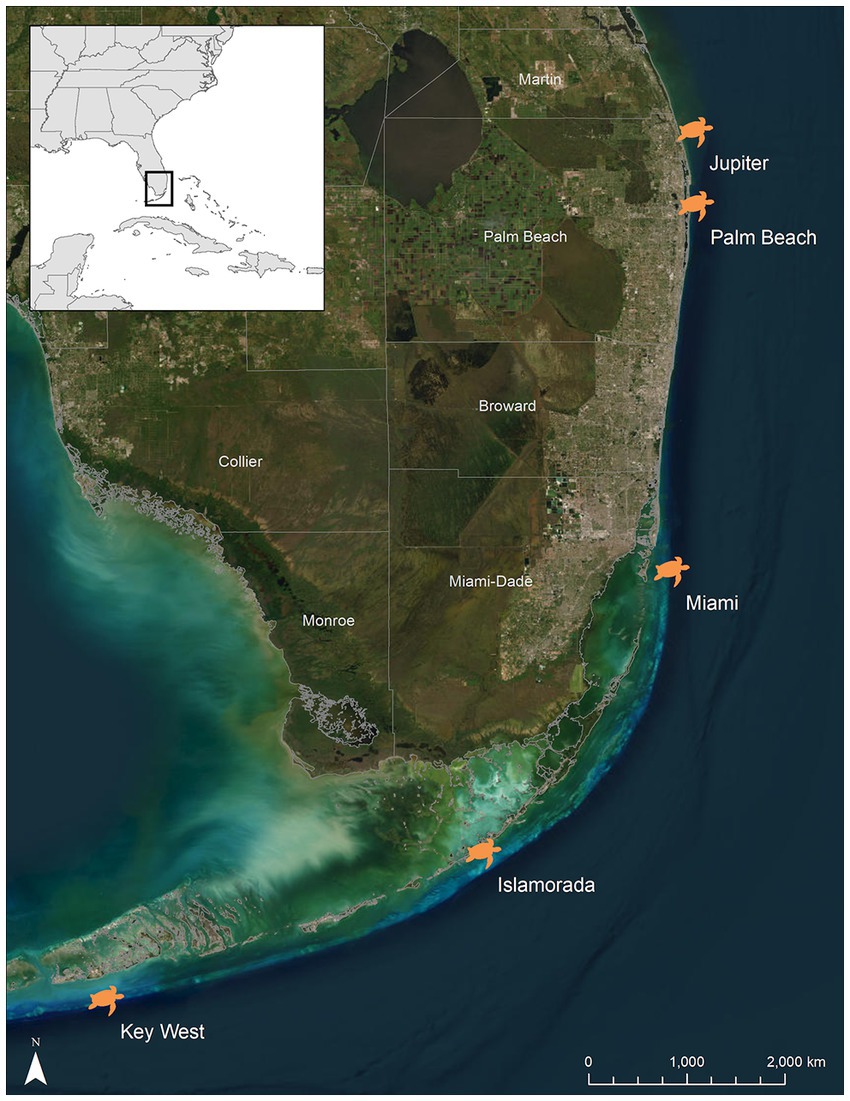
Figure 1. Locations of the five capture sites of hawksbill sea turtles (Eretmochelys imbricata) across Florida’s, USA reef tract. Jupiter and Palm Beach are located in Palm Beach County, while Islamorada and Key West are located in Monroe County. One individual was captured off of a reef in Miami (Miami-Dade County). This individual was included in the Monroe County group for statistical analyses.
Within 5–10 min of returning to the vessel, up to 5 mL of blood were collected from the dorsal cervical sinus using 20-gauge, 1.5″ needles and 7 mL lithium heparin vacutainer tubes (Becton Dickinson, Franklin Lakes, New Jersey, USA), following standardized safe blood volume withdrawal guidelines for sea turtles (NMFS SEFSC, 2008). The sampling site was disinfected with alternating applications of povidone iodine and 70% isopropyl alcohol both before and after venipuncture. The vacutainers were then transferred to an insulated sleeve (i.e., bubble wrap) and placed on wet ice for up to 8 h until processing.
Following blood collection, standard (i.e., notch to tip) straight carapace length (SCL) and the maximum body depth (BD) of each subject were measured with aluminum calipers. Mass was estimated to the nearest kg using a 300 kg digital scale. Body condition index (BCI) was calculated (Bjorndal et al., 2000), applying a correction factor of 10,000. Physical examination included assessment of the presence and location of conspicuous epibiota (e.g., macroalgae, coral, barnacles, etc.; Figure 2) and/or physical abnormalities, which were diagrammed and photographed. Prior to release, Inconel tags (National Band and Tag Co., Newport, Kentucky, USA) were placed on the trailing edge of the second distal scale of each front flipper, and/or one PIT tag (Biomark, Inc., Boise, Idaho, USA) was injected into the right front shoulder in all turtles of sufficient size (>30 cm SCL). All turtles were released ~20 min after sample collection, at or near the capture site.
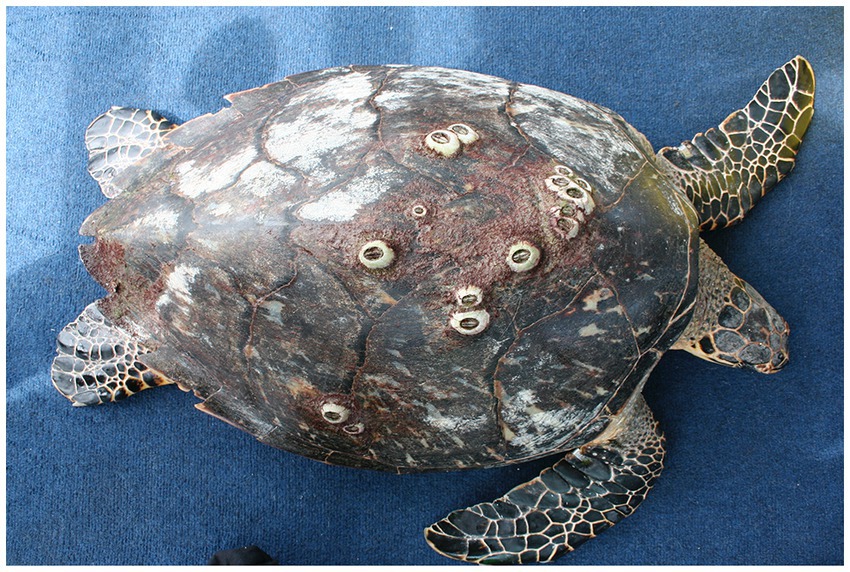
Figure 2. Photograph of a subadult hawksbill sea turtle (Eretmochelys imbricata) from Palm Beach County representative of the study turtles with associated normal epibiota coverage.
Upon return to the laboratory, whole blood was well-mixed and packed cell volume (PCV) was determined using the average of two microhematocrit tubes after centrifugation for 5 min at 7,500 g (12,000 rpm) using a ZipCombo microhematocrit centrifuge (LW Scientific, Lawrenceville, Georgia, USA). Blood films were prepared from well-mixed whole blood, air-dried, and stained with Wright-Giemsa (Harleco®, EMD Millipore, Billerica, Massachusetts, USA). Blood film evaluation included white blood cell (WBC) estimate (Weiss, 1984) using an eyepiece of 18 mm diameter, a 200 white blood cell differential including heterophils, lymphocytes, monocytes, eosinophils, and basophils, and morphological evaluation of red blood cells (RBC), WBCs, and thrombocytes. The heterophil:lymphocyte ratio was calculated.
The remaining whole blood was spun at 1350 g (3,300 rpm) in a Champian E33 centrifuge (Ample Scientific, LLC, Norcross, Georgia, USA) for 6–8 min. Plasma was separated from the RBC and WBC, placed in cryovials, and stored in a standard freezer (−20°C) until shipment on dry ice after 48 h to the University of Florida for storage in an ultralow freezer. After 8–434 d (mean ± SD = 139 ± 102 d), samples were then shipped to the University of Miami Avian and Wildlife Laboratory for biochemical analyses using an Ortho 250XR (Ortho Clinical Diagnostics, Rochester, New York, USA) dry slide chemistry analyzer. Biochemical analytes of interest included alkaline phosphatase (ALP), amylase, aspartate aminotransferase (AST), bile acids, blood urea nitrogen (BUN), calcium, phosphorus, chloride, cholesterol, creatine phosphokinase (CK), gamma-glutamyl transferase, glucose, glutamate dehydrogenase (GLDH), lipase, magnesium, potassium, sodium, triglycerides, and uric acid. The calcium:phosphorus ratio was calculated.
Plasma protein electrophoresis was conducted using the SPIFE 3000 system (Helena Laboratories, Inc., Beaumont, Texas, USA). Fractions of interest included albumin and total globulins. The albumin:globulin ratio was calculated.
3. Statistical analyses
Statistical analyses were performed using MedCalc® statistical software (version 19.6, Ostend, Belgium) and SPSS for Windows (version 27; Chicago, Illinois, USA). Measures of central tendency and range are reported for all blood analytes in Standard International (SI) units. Reference intervals (95% with associated 90% confidence intervals) were determined for sea turtles of juvenile and subadult life-stage classes that fit the inclusion criteria for the study (Moore et al., 2020), using parametric methods based on recommendations by Friedrichs et al. (2012) for sample sizes ≥20, but <40. Normality was assessed using the Shapiro-Wilk test, while outliers were detected using the Dixon-Reed test. Outliers were subsequently removed and when necessary, logarithmic or Box-Cox transformations were employed to generate accurate reference intervals. Several blood analytes could not be normalized to fit a Gaussian distribution; therefore, reference intervals for those analytes were calculated using the robust method.
Spearman correlations were used to determine relationships between individual hematological and plasma biochemical analytes. Differences in SCL by capture location (Palm Beach v. Monroe) and BCI in juveniles and subadults were assessed using independent samples t-tests. Linear regression analysis was used to assess the relationship of SCL to mass (using log-transformed data). Stepwise backward multiple regression was used to determine the impacts of SCL, BCI, water temperature (independent variables) upon capture on blood analytes (dependent variables). Outliers were determined using Tukey’s method and subsequently removed from analyses. Transformations were employed as necessary. To determine if polychromasia, anisocytosis, basophilic stippling, and number of immature RBCs/100 mature RBCs were influenced by life-stage class, Kruskal-Wallis with Dunn’s post-hoc comparisons were used.
4. Results
4.1. Physical examination and morphometrics
A total of 68 hawksbills were captured from 5 Jun 2017–30 Oct 2020. Water temperatures were similar for both sites from May–October, when turtles were typically captured: 23.3–29.4°C (mean 27.4°C) in Palm Beach County and 26.7–29.4°C (mean 28.1°C) in Monroe County, respectively. Inclusion criteria for study animals included the absence of overt acute or debilitating external injuries, normal behavior, visibly adequate body condition, and absence of difficulties during blood withdrawal (Figure 2). Morphometric results by sampling location are reported in Table 1. Turtles of <50 cm SCL were considered juveniles (n = 26), those of 50–78 cm SCL were considered subadults (n = 39), and turtles >78 cm were considered adults (n = 3) (Boulon, 1994; Wood et al., 2017). Hawksbills from Palm Beach County were significantly heavier, had a significantly longer SCL (Figure 3), and a greater body depth than turtles from Monroe County (p < 0.001 in all cases; Table 1); turtles from Monroe County had significantly larger BCI (p < 0.001; Table 1). Log-transformed mass and SCL strongly correlated (r2 = 0.989; p < 0.001; Figure 4). Using a t-test (t (60) = −2.378; p = 0.021), we found that juveniles had a significantly higher BCI (mean ± SD: 1.16 ± 0.10; range: 0.97–1.39) than subadults (mean ± SD: 1.10 ± 0.10; range: 0.94–1.32), and overall that BCI tended to decrease with SCL (y = −0.002x + 1.24; r2 = 0.079; p = 0.027; n = 62).
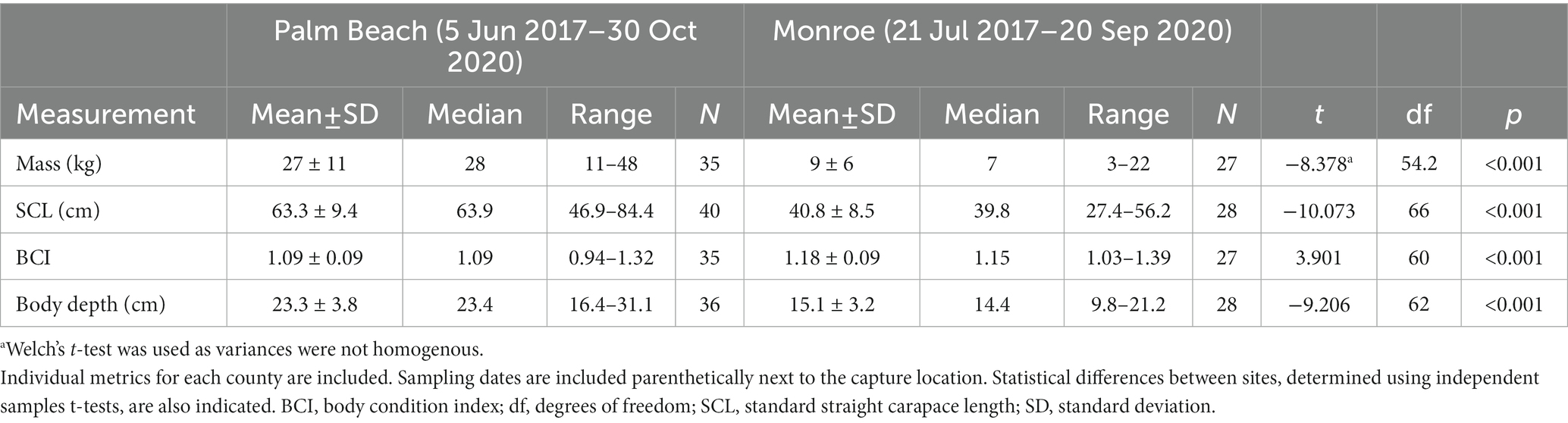
Table 1. Morphometric data of in-water assessed hawksbill sea turtles (Eretmochelys imbricata) from Florida, USA.
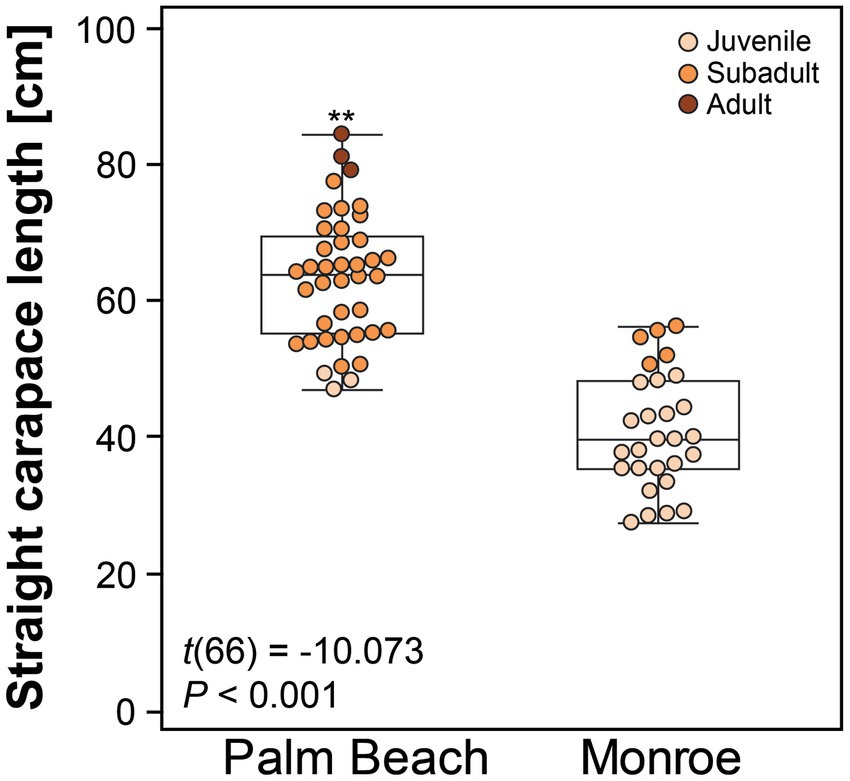
Figure 3. Straight carapace length of hawksbill sea turtles (Eretmochelys imbricata) from Palm Beach and Monroe Counties, Florida, USA. The central box represents the lower to upper quartiles, with the middle line representing the median. The vertical lines extend from the minimum to maximum values. Asterisks above the Palm Beach box-plot indicate a significant difference between the two groups at p < 0.050.
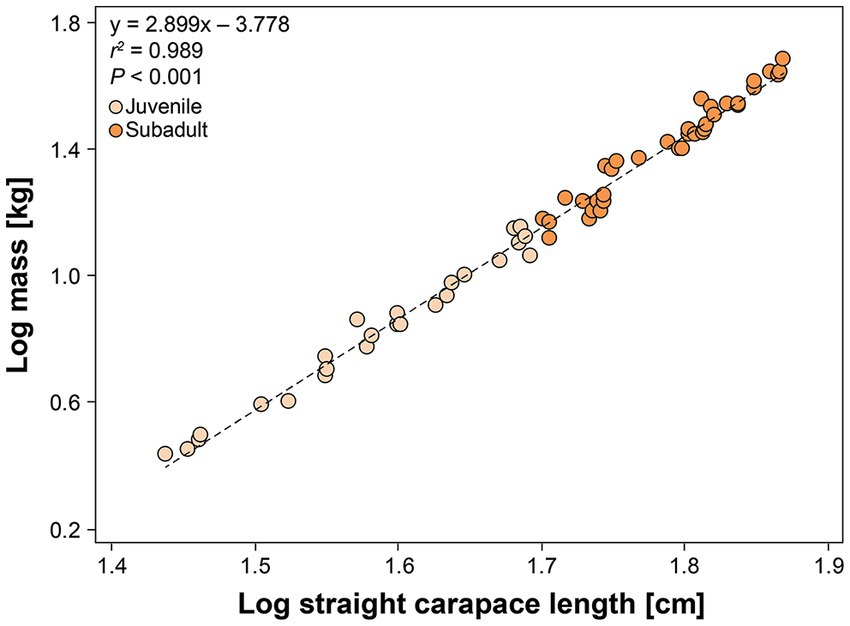
Figure 4. Relationship between log-transformed mass (kg) and standard straight carapace length (cm) in in-water assessed hawksbill sea turtles (Eretmochelys imbricata) from Florida, USA. Adults are not shown as they were too large to weigh. The equation for the line-of-best-fit is shown.
Small patches of red and/or brown algae (Figure 2), particularly on the posterior carapace, were ubiquitous, while small patches of calcareous algae were less common. Fire coral colonies (Millepora sp.) were occasionally found on larger individuals. Fourteen turtles (20%) had dented or chipped marginal scutes; seven (10%) had at least one scalloped hind limb; one (1%) had a partially amputated (~50%) hind limb; and two (3%) had minor front flipper damage. All described injuries/abnormalities were completely healed and were not expected to impact blood analytes. All turtles appeared to be in robust condition as observed by the thickset nature of the soft tissues surrounding the neck and flippers and BCI scores. No fibropapilloma tumors or acute or healed injuries specific to shark predation were observed.
All turtles were considered to be clinically normal and fit the inclusion criteria for this study as they were all active and alert, had robust subjective body condition scores (Tristan and Norton, 2017), minimal epibiota, and minor injuries or shell abnormalities that most likely did not impact blood analytes.
4.2. Reference intervals
Mild (1+) hemolysis was present in seven samples, while mild (1+) lipemia was present in one sample. Neither degree of interferences is thought to affect dry chemistry analyses (Andreasen et al., 1997; Stacy and Innis, 2017; Stacy et al., 2019). Measures of central tendency and range of the measured blood analytes are reported in SI units for all three life-stage classes (juvenile, subadult, and adult; Tables 2–4, respectively; conventional units are reported in Supplementary Tables S1–S3), while reference intervals, due to the sufficient number of data points, are described for juveniles and subadults only (Tables 2, 3). Results of morphological evaluation of RBC, WBC, and thrombocytes are shown in Table 5.
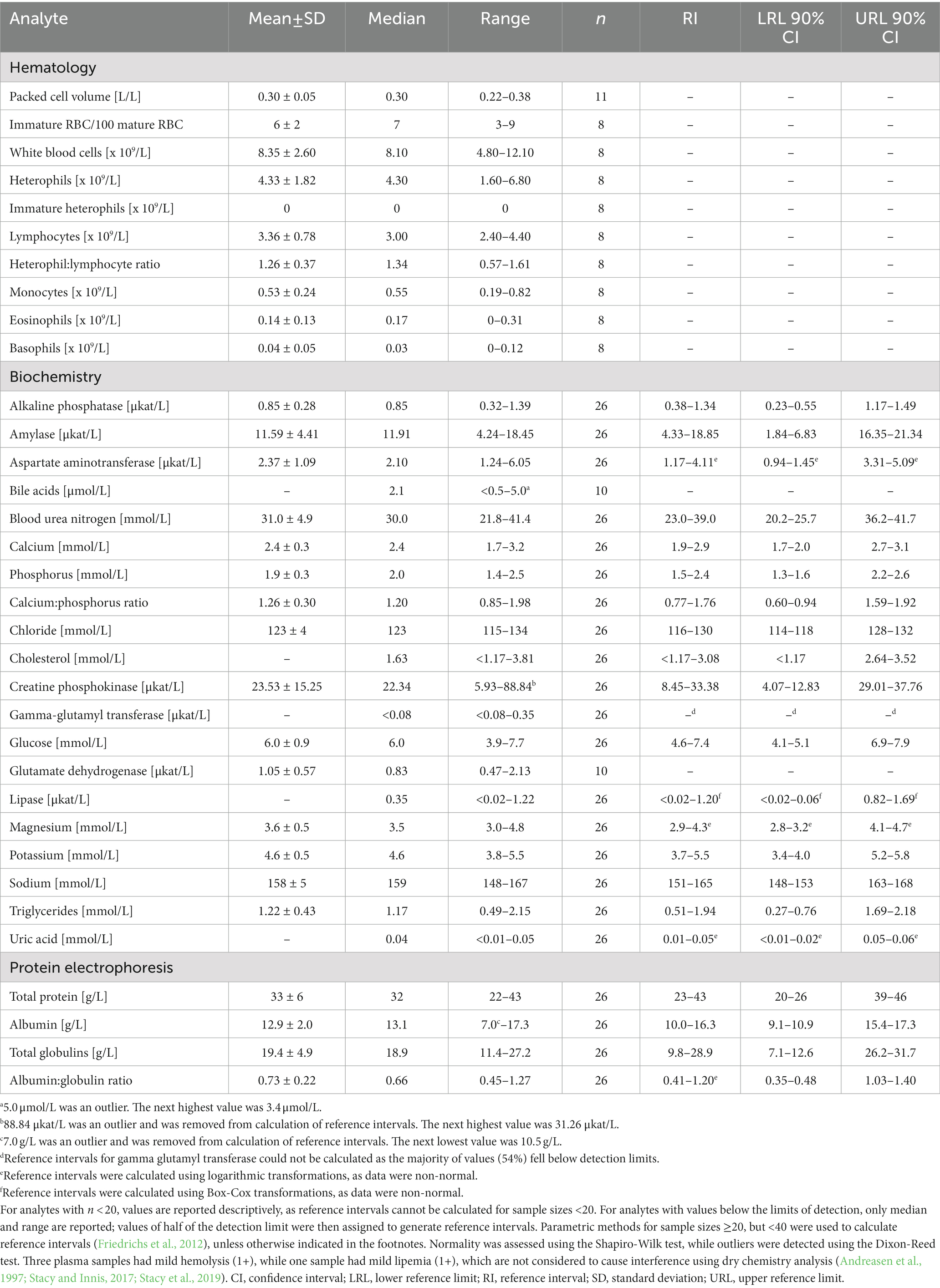
Table 2. Measures of central tendency, range, and reference intervals (with 90% confidence intervals for upper and lower limits) for hematological and plasma biochemical data (including protein electrophoresis) in Standard International units for in-water, juvenile (25–49.9 cm standard straight carapace length) hawksbill sea turtles (Eretmochelys imbricata) from Florida, USA.
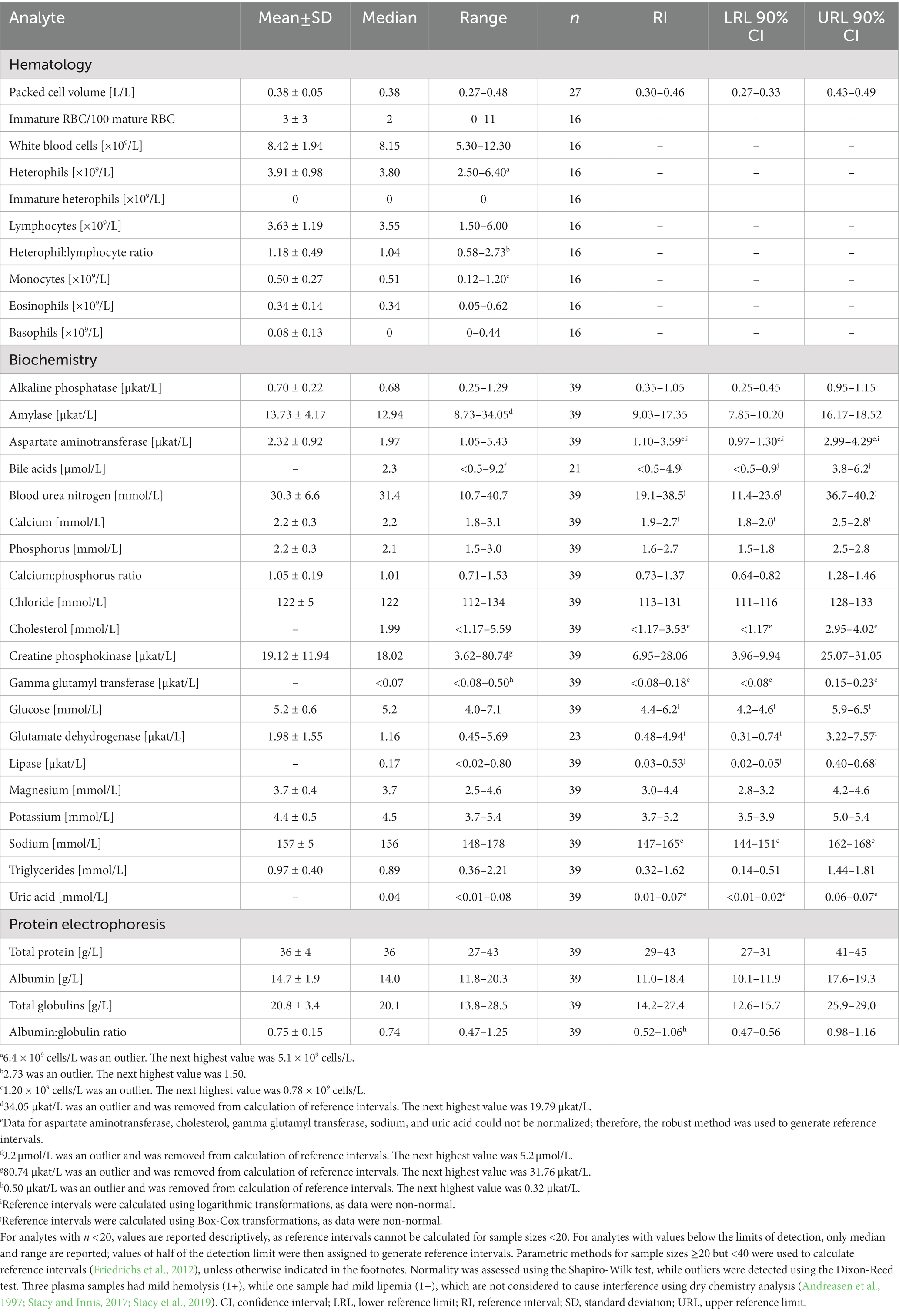
Table 3. Measures of central tendency, range, and reference intervals (with 90% confidence intervals for upper and lower limits) for hematological and plasma biochemical data (including protein electrophoresis) in Standard International units for in-water, subadult (50–78 cm standard straight carapace length) hawksbill sea turtles (Eretmochelys imbricata) from Florida, USA.
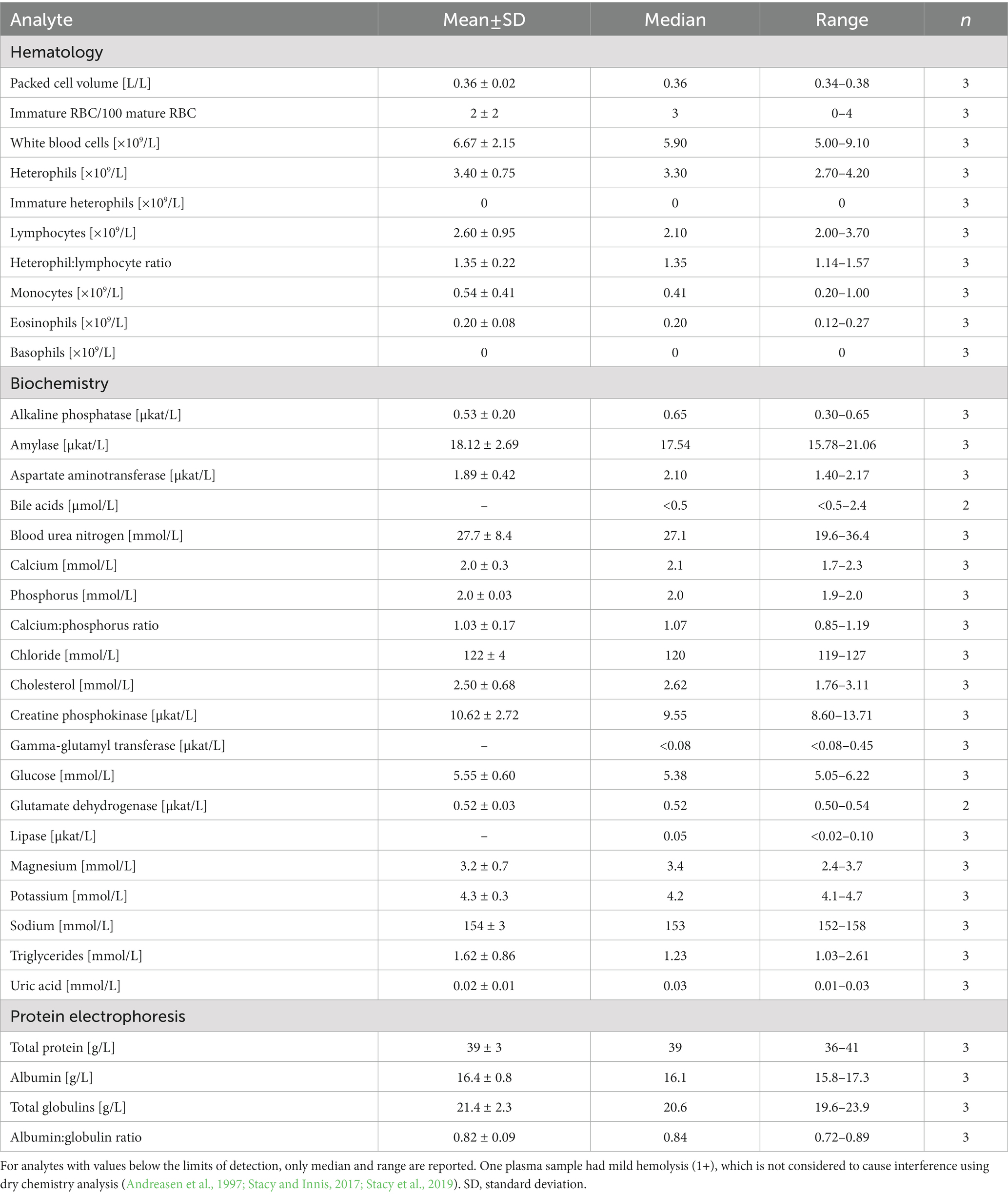
Table 4. Measures of central tendency and range for hematological and plasma biochemical data (including protein electrophoresis) in Standard International units for in-water, adult (>78 cm straight carapace length) hawksbill sea turtles (Eretmochelys imbricata) from Florida, USA.
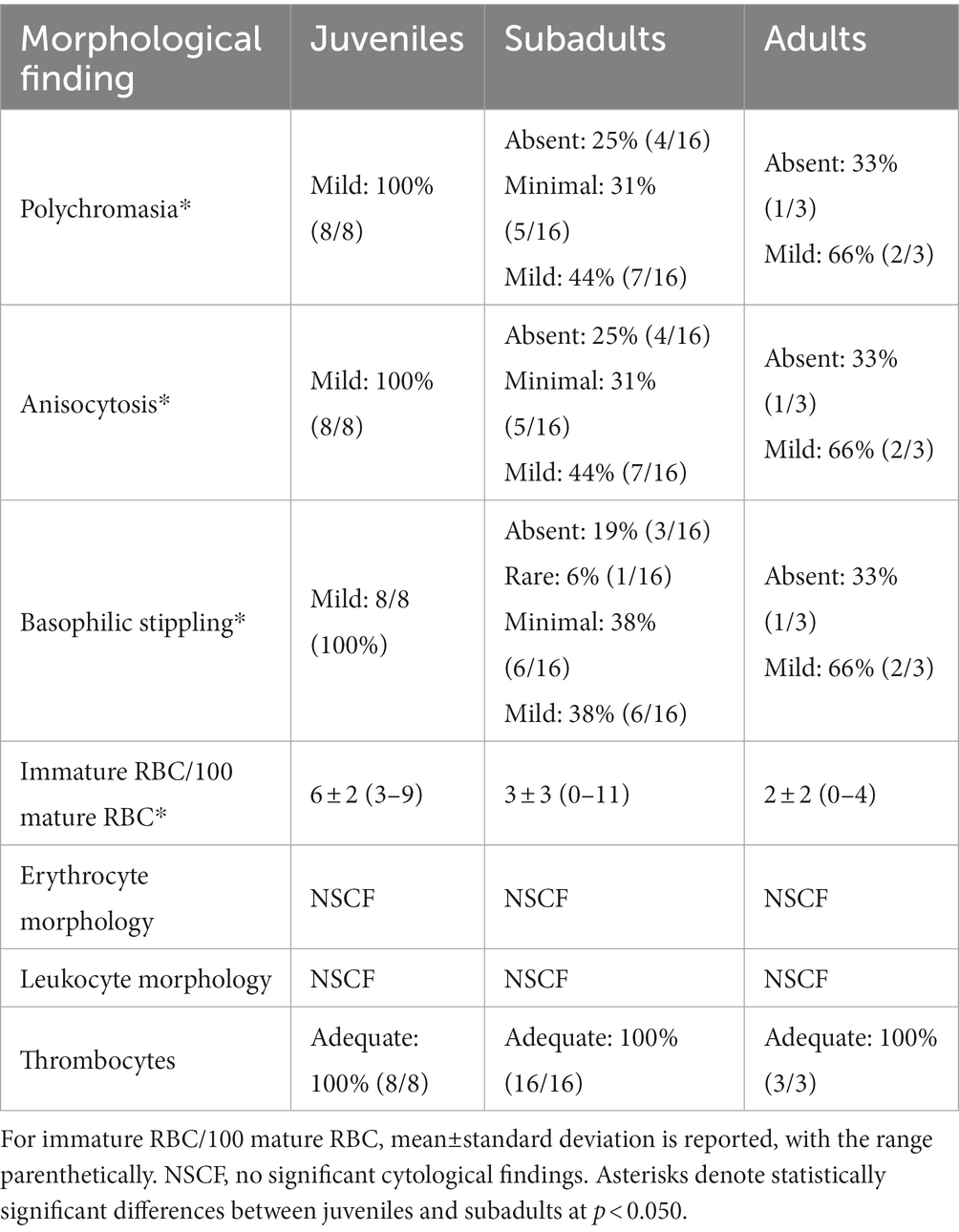
Table 5. Morphological evaluation of red blood cells (RBC), white blood cells, and thrombocytes for in-water, hawksbill sea turtles (Eretmochelys imbricata) of three life-stage classes from Florida, USA.
Spearman correlations of hematological and biochemical analytes from all study turtles identified several statistically significant correlations of biological relevance: bile acids and AST (rs = 0.363; p = 0.032; n = 35), GGT and AST (rs = 0.310; p = 0.010; n = 68), GGT and cholesterol (rs = 0.288; p = 0.017; n = 68), GLDH and AST (rs = 0.709; p < 0.001; n = 35), magnesium and phosphorus (rs = 0.369; p = 0.002; n = 68), uric acid and phosphorus (rs = 0.369; p = 0.002; n = 68), uric acid and magnesium (rs = 0.325; p = 0.007; n = 68), uric acid and sodium (rs = 0.328; p = 0.007; n = 68), triglycerides and GGT (rs = 0.318; p = 0.008; n = 68), and triglycerides and glucose (rs = 0.359; p = 0.003; n = 68). There were no correlations between CK and AST (rs = 0.117; p = 0.340; n = 68) or CK and phosphorus (rs = 0.158; p = 0.197; n = 68).
Blood film review data (Table 5) showed that polychromasia and anisocytosis significantly differed by life-stage class (H(2) = 6.347; p = 0.042 for both categories), with subadults having a lower occurrence of both in comparison to juveniles (p = 0.035 for both categories). Basophilic stippling also significantly differed by life-stage class (H(2) = 7.406; p = 0.025), with subadults having a lower occurrence in comparison to juveniles (p = 0.020). Lastly, number of immature RBCs/100 mature RBCs significantly differed by life-stage class (H(2) = 7.209; p = 0.027), with subadults having a lower occurrence of immature RBCs in comparison to juveniles (p = 0.033).
4.3. Influence of straight carapace length, body condition, and water temperature upon capture on blood analytes
SCL showed significant positive relationships with PCV, eosinophils, AST, phosphorus, cholesterol, GLDH, total protein, albumin, and globulins and significant negative relationships with ALP, CK, calcium, calcium:phosphorus ratio, and glucose. BCI showed a significant negative relationship with PCV. Water temperature showed significant positive relationships with AST, cholesterol, GGT, glucose, lipase, triglycerides, and albumin. Complete statistical results are shown in Table 6.
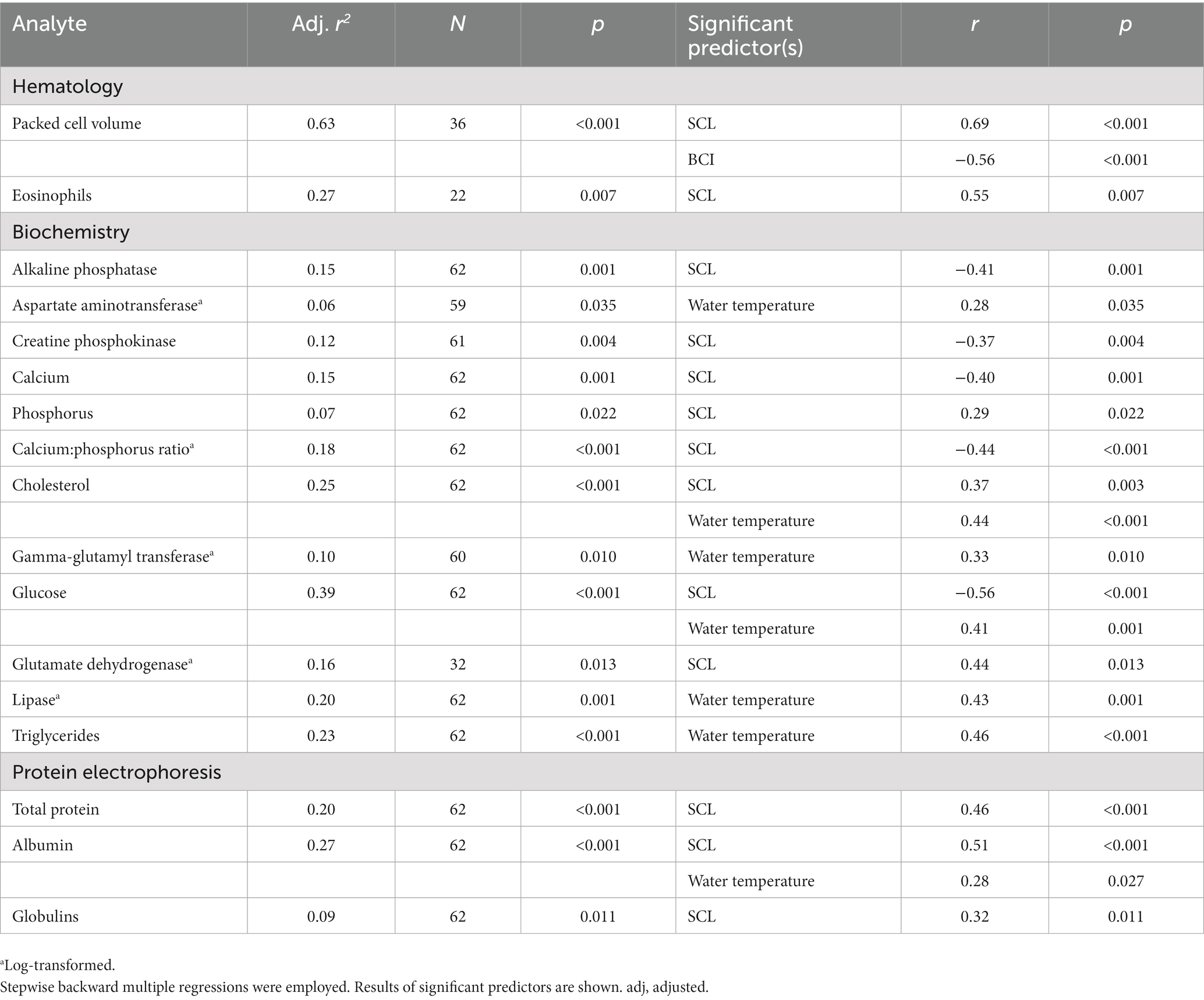
Table 6. Relationship of blood analytes to straight carapace length (SCL), body condition index (BCI), and water temperature upon capture of in-water, hawksbill sea turtles (Eretmochelys imbricata) from Florida, USA.
5. Discussion
This study reports hematology and plasma biochemical blood analyte data for wild-caught hawksbills during four years of field work in the Atlantic Ocean, and evaluated influences of intrinsic (length, body depth, BCI) and extrinsic factors (water temperature), with life-stage class being identified as the most relevant factor for the observed blood analyte differences. The data herein provide an important baseline framework and insight into the physiology of the critically endangered hawksbill sea turtle from the reef system of southeastern Florida during a defined time frame. This work will provide a springboard for future assessments on fitness and stressor effects on this population and allows for comparisons to other geographical populations.
5.1. Physical examination and morphometrics
A thorough external physical examination is an essential component of sea turtle health assessment studies as it provides important information on body condition, activity level, mentation, physical abnormalities, and evidence of trauma or disease (Deem and Harris, 2017; Tristan and Norton, 2017; Page-Karjian and Perrault, 2021). These findings are endpoints needed for defining inclusion criteria in wild animal studies. In fact, some health assessment studies are solely based on physical examination and morphometric measurements (Maulida et al., 2017). All hawksbills captured during the study time frame fit the inclusion criteria for the study. The lack of overt injuries in hawksbills leading to exclusion of some of the study animals contrasts with other sea turtle species. For example, two of 36 immature Kemp’s ridleys (Lepidochelys kempii) from Georgia, USA were excluded due to abnormal shell formation or monofilament entanglement (Perrault et al., 2020).
BCI of all hawksbills in this study ranged from 0.94–1.39 (mean ± SD: 1.13 ± 0.10), which is similar to two aggregations of hawksbills from Puerto Rico (means of 1.16 and 1.18) (Diez and van Dam, 2002) and the Bahamas (mean ± SD: 1.17 ± 0.08; range: 1.05–1.41) (Bjorndal and Bolten, 2010), but higher than hawksbills from Indonesia (mean ± SD: 1.06 ± 0.07; range: 0.92–1.14) (Maulida et al., 2017), and lower than hawksbills from the Monito cliff wall in Puerto Rico (mean: 1.24) (Diez and van Dam, 2002) and the Cayman Islands (Little Cayman mean ± SD: 1.25 ± 0.17; Grand Cayman mean ± SD: 1.24 ± 0.18) (Blumenthal et al., 2009). Norton and Wyneken (2015) suggest that BCI scores of <1.00 are indicative of emaciation in sea turtles, and since all turtles in the present study were considered robust based on visual evaluation, BCI scores should be specifically developed for individual sea turtle species, geographical locations/populations, and life-stage classes (Nishizawa and Joseph, 2022).
There is evidence that hawksbill turtles migrate during their development and growth from small juveniles in the Florida Keys along the Southeast Florida Continental Reef Tract to subadults in their northern range in Palm Beach County, Florida (Wood et al., 2013). The observed statistically significant difference between capture sites based on SCL and thus differentiating juveniles mainly captured in Monroe County vs. subadult and adult turtles mainly captured at Palm Beach County supports this further. The very small overlap of SCL (Figure 3) between juveniles and subadults at both capture sites also substantiates that secondary recruitment of juveniles from the Keys to the north occurs in this population.
BCI was significantly higher in hawksbills from Monroe County compared to those from Palm Beach County, despite turtles from Palm Beach County having higher SCL, mass, and body depth. These trends are driven by life-stage class distribution between the two sites, as juveniles had a significantly higher BCI than subadults, and more juveniles (23 of 28 total turtle captures; 82%) were caught in Monroe compared to Palm Beach County (3 of 40 total turtle captures; 8%). These morphometrical data trends in hawksbills suggest fast somatic growth rates in juvenile hawksbills since smaller juveniles had higher BCI potentially indicating greater food intake. A comparison of forage items of the two Florida hawksbill aggregations from this study has not been reported, therefore it is unknown if dietary or habitat-related differences between the Palm Beach County and Monroe County sites have influenced the results. Differences in BCI by capture locations and by carapace length have previously been reported in green turtles (Chelonia mydas) (Diez and van Dam, 2002; Peig and Green, 2010; Lamont and Johnson, 2021). It is likely that dissimilarities in BCI of sea turtles are mainly driven by differences in environmental conditions, population density, disease prevalence, and/or forage availability (Bjorndal et al., 2000; Diez and van Dam, 2002; Labrada-Martagón et al., 2010; Rossi et al., 2019; Lamont and Johnson, 2021).
5.2. Hematology
PCV is considered an indicator of overall fitness (Stamper et al., 2005). Based on data from captive animals, Frair (1977) suggested that hawksbills have an overall lower PCV than other sea turtle species (range: 0.17–0.42 L/L) and attributed this to dissimilarities in size and growth. In foraging hawksbills from Australia and nesting hawksbills from Brazil, PCV ranged from 0.12–0.41 L/L and 0.34–0.40, respectively (Goldberg et al., 2013; Whiting et al., 2014). The PCV of all hawksbills in the current study ranged from 0.22–0.48 L/L. Mild anemia in sea turtles is defined as PCV ranging from 0.19–0.25 L/L, with severe anemia occurring in patients with PCV ≤0.12 L/L (Stacy and Innis, 2017); therefore, it seems that wild-caught juvenile hawksbills may have naturally occurring lower PCV (Frair, 1977; Whiting et al., 2014), under due consideration that lymph contamination during blood sampling cannot always be reliably excluded in sea turtles (Stacy and Innis, 2017). Of note, the methodology of obtaining PCV data should be considered, as some studies report discrepancies between hematocrit (Hct) data (using automated analyzers) and the manually obtained PCV (via spun capillary tubes), with Hct data being considered inaccurate (i.e., lower) via automated methods in sea turtles (Muñoz-Pérez et al., 2017; Stacy and Innis, 2017).
While the ranges of PCV established in this and other studies overlap, it also appears that there may be life-stage class differences in this species. For example, we observed a strong positive relationship between PCV and SCL in Florida hawksbills, indicating that RBCs presumably increase in length, width, and volume as turtles mature and oxygen demands increase with longer dive times as described in other studies (Frair, 1977; Stamper et al., 2005; Perrault et al., 2016; Stacy et al., 2018). This trend of increasing PCV with turtle size has been documented in six of the seven sea turtle species (Frair, 1977; Wood and Ebanks, 1984; Casal et al., 2009; Rousselet et al., 2013; Perrault et al., 2016; Stacy et al., 2018).
In comparison to subadults, juvenile hawksbills had higher incidences of RBC polychromasia (i.e., increased basophilic color), anisocytosis (i.e., variation in RBC size), and basophilic stippling (i.e., punctate basophilic cytoplasmic inclusions), and a greater proportion of immature RBC in relation to total mature RBC. These morphological features of RBC are often associated with active erythroid production (Stacy et al., 2011), an observation also described in younger turtles of other sea turtle species and reptiles in general (Fleming et al., 2020; Perrault et al., 2020; Stacy and Harr, 2020; Perrault et al., 2022).
Eosinophils in this study made up a small proportion (range: 0–8%) of the overall leukogram (<0.70 × 109 cells/L), which is much lower than in similarly sized wild-caught hawksbills from the Galápagos (Muñoz-Pérez et al., 2017). We also observed a significant positive relationship between SCL and absolute eosinophils. While the exact function of reptilian eosinophils has not been established, they are thought to aid in immune stimulation and phagocytosis of parasites (Stacy et al., 2011; Rousselet et al., 2013; Stacy and Innis, 2017; Stacy and Harr, 2020). Therefore, the observed correlation may be due to antigenic stimulation or influences from environmental factors (Stacy et al., 2011), with increasing antigenic stimulation as turtles grow and age (e.g., increased incidence of spirorchid infection in larger turtles) (Deem et al., 2006; Casal et al., 2009; Innis et al., 2010).
5.3. Electrolytes and minerals
Although plasma electrolyte and mineral concentrations in hawksbill turtles from this study fell within normal ranges reported for other sea turtle species (Stacy and Innis, 2017), several physiologically relevant relationships with SCL were observed. Similar to juvenile loggerheads (Caretta caretta) in North Carolina (Kelly et al., 2015), phosphorus concentrations positively correlated with SCL in hawksbills, suggesting bone and/or somatic growth, given that there were no positive size-relevant correlations with CK, the main enzyme of muscle tissues (Stacy and Innis, 2017). The observed negative correlations of calcium and calcium to phosphorus ratio with SCL suggest differences in somatic growth rates as turtles grow and age (Bolten and Bjomdal, 1992), bone metabolism, or possibly osmoregulation (i.e., associated with changes in salt gland regulation with somatic growth). Hawksbill turtles have an oceanic-neritic development pattern and recruit back to nearshore environments at a small size (20–35 cm carapace length) (Bolten, 2003). Additionally, regional differences are a known major driver for somatic growth in the species (Bjorndal et al., 2016). In contrast to hawksbill SCL, phosphorus in oceanic-juvenile loggerheads correlated negatively (Stacy et al., 2018) and calcium to phosphorus ratio in immature Kemp’s ridleys (Perrault et al., 2020) correlated positively with carapace length. Possible considerations for these differences include species, life stage-class, and dietary or habitat variations. The observed positive correlations of magnesium with phosphorus and uric acid with magnesium, phosphorus, and sodium suggest potential clinical utility of these analytes in the assessment of renal function in sea turtles, particularly in the presence of normal CK activities (Stacy and Innis, 2017).
5.4. Tissue enzyme activities
Tissue enzyme activities showed several metabolically important correlations with SCL and water temperature in this study. The positive correlations of AST and GLDH with SCL, with the absence of CK associations that would indicate release from muscle tissue, suggest that larger turtles with higher hepatic volume likely have greater tissue enzyme release into plasma, similar to hawksbills in the Persian Gulf and Brazil (Goldberg et al., 2013; Ehsanpour et al., 2015). The negative correlation of SCL with CK further supports the conclusions on mineral correlations, in that the observed correlations with SCL and calcium and phosphorus suggest bone growth differences in smaller turtles rather than growth of muscle tissue.
The negative correlation of ALP with SCL was unexpected, since ALP activities are known to be higher in younger, growing mammals (Allison, 2022); however, this tissue enzyme is widely distributed in sea turtle tissues and isoenzymatic activities of ALP in bone tissue have not been assessed to date in reptiles (Anderson et al., 2013; Petrosky et al., 2015; Adamovicz et al., 2019). The tissue enzymes AST, GGT, and lipase correlated positively with water temperature, which could be driven by higher physical activity or somatic growth rate in smaller turtles.
Activities of GLDH have rarely been reported in sea turtles (March et al., 2018) and the clinical utility of this enzyme is currently unknown (Stacy and Innis, 2017). Green turtles undergoing rehabilitation in Australia showed strong correlations between plasma GLDH and AST activities, suggesting the potential use as indicators for hepatocellular injury (March et al., 2018). This assumption is further supported by the observed correlations between GLDH and AST, but not CK in hawksbill turtles from this study. Additionally, in eastern box turtles (Terrapene carolina carolina), GLDH activities were highest in liver, followed by kidney and gall bladder, with activities in skeletal muscle being among the lowest of the ten tissues examined (Adamovicz et al., 2019). The observed positive correlations of GGT with AST and cholesterol, bile acids with AST, and triglycerides with GGT and glucose highlight the potential for the clinical utility of these analytes for the diagnosis of liver disorders in hawksbill turtles. As such, concurrently increased GLDH, GGT, and/or AST activities, increased triglycerides, cholesterol, and/or bile acids, along with normal CK could support the clinical diagnosis of liver abnormalities, as supported by general assumptions in clinical chemistry interpretations in sea turtles (Stacy and Innis, 2017).
5.5. Lipids
Plasma lipid concentrations in sea turtles often differ by life-stage class. Similar to green turtles and loggerheads (Hasbún et al., 1998; Labrada-Martagón et al., 2010; Delgado et al., 2011; Prieto-Torres et al., 2013; Rousselet et al., 2013), positive correlations of plasma cholesterol and SCL were also observed in hawksbill turtles from this study. These observations suggest BCI differences due to life-stage class as observed in leatherbacks (Dermochelys coriacea), whereby foraging individuals had higher plasma lipids compared to nesting turtles (Harris et al., 2011), likely due to little or lack of foraging during the nesting season (Perrault et al., 2014).
5.6. Glucose
Glucose concentrations in hawksbills from Florida were similar in range to juveniles after successful rehabilitation and in adult nesting hawksbills (Caliendo et al., 2010; Goldberg et al., 2013), and were negatively correlated with SCL, a trend that has also been observed in loggerheads in captive care (Kakizoe et al., 2007; Rousselet et al., 2013). With the additional positive correlation with water temperature, considerations for higher plasma glucose in smaller turtles include higher activity (i.e., in warmer waters), comparatively increased stress level during capture and handling (i.e., smaller turtles often require a short duration chase before capture that is not typically required in larger turtles), or, given similar trends in captive loggerheads, differences in metabolic rates between life-stage classes (Goldberg et al., 2013; Rousselet et al., 2013). Specifically for water temperature, possible higher carbohydrate consumption during periods of higher temperatures is presumed to result in higher metabolic rate, as described in green turtles from Mexico and loggerheads from North Carolina, USA (Stamper et al., 2005; Labrada-Martagón et al., 2010; Kelly et al., 2015).
5.7. Proteins
Similar to other sea turtles, we found that SCL positively correlated with total protein, albumin, and globulins (Hasbún et al., 1998; Osborne et al., 2010; Delgado et al., 2011; Whiting et al., 2014; Kelly et al., 2015; Stacy et al., 2018; Perrault et al., 2020), trends that can be explained by nutritional, seasonal, and/or environmental differences (Frair and Shah, 1982), or as a result of immune system maturation due to antigenic stimulation as turtles grow and age (Innis et al., 2010; Osborne et al., 2010; Perrault et al., 2014). Larger hawksbills foraging in Honduras preferred poriferan prey (e.g., Melophlus ruber), with smaller individuals foraging mainly on algae (Berube et al., 2012). Forage items of the two hawksbill aggregations in Florida from this study have not been reported and could also influence size-relevant correlations with plasma protein concentrations. Lastly, we found that temperature and albumin were positively correlated in hawksbills from this study possibly due to higher activity levels and increased food consumption during warming periods, a trend that has also been observed in Blanding’s turtles (Emydoidea blandingii) from Illinois (Andersson et al., 2021).
6. Conclusion
This study reports blood analyte data from the critically endangered hawksbill sea turtle encompassing three life-stage classes inhabiting the Florida reef system and gives insight into basic physiological metrics for this population during the study period of 2017–2020. With the defined inclusion criteria used herein, the population was assessed as “clinically normal,” thus offering to identify various aspects of sea turtle biology and physiology that are influenced by extrinsic and intrinsic factors. These data will support answering population-driven questions (e.g., future spatio-temporal comparisons, conservation management) in addition to their utility for individual animals (e.g., stranding, rehabilitation).
Data availability statement
The original contributions presented in the study are included in the article/Supplementary materials. Further inquiries can be directed to the corresponding author.
Ethics statement
This study was reviewed and authorized by the National Marine Fisheries Service (NMFS) [Permit #22988], Florida Fish and Wildlife Conservation Commission (FWC) [Marine Turtle Permits #021 and #077], Florida Keys National Marine Sanctuary [Research Permit #175], and University of Florida’s Institutional Animal Care and Use Committee (IACUC) [#201706823]. All handling and sampling procedures of sea turtles were performed according to NMFS and FWC regulations.
Author contributions
NIS, JRP, and LDW conceptualized the study and wrote the original manuscript. LDW led all fieldwork and sample collection. JRP analyzed the data. All authors contributed to the article and approved the submitted version.
Funding
We gratefully acknowledge the National Save The Sea Turtle Foundation (Ft. Lauderdale, Florida) for generously providing funding for this research.
Acknowledgments
The authors thank biological scientists Laurie Adler and Brittany Barbeau for sample archiving, logistical help, and technical support, Sarah Hirsch for assistance with the map, and Meghan Koperski for assistance with permitting. The authors thank all dive boat operators and their staff for providing access to reefs in Palm Beach County, and the captains and crew from the National Save The Sea Turtle Foundation for access to sites in Broward, Miami-Dade, and Monroe Counties. The authors also thank all of the dedicated volunteers who assisted with turtle surveys, captures, and water safety protocols.
Conflict of interest
The authors declare that the research was conducted in the absence of any commercial or financial relationships that could be construed as a potential conflict of interest.
Publisher’s note
All claims expressed in this article are solely those of the authors and do not necessarily represent those of their affiliated organizations, or those of the publisher, the editors and the reviewers. Any product that may be evaluated in this article, or claim that may be made by its manufacturer, is not guaranteed or endorsed by the publisher.
Supplementary material
The Supplementary material for this article can be found online at: https://www.frontiersin.org/articles/10.3389/fevo.2023.1199688/full#supplementary-material
References
Adamovicz, L., Griffioen, J., Cerreta, A., Lewbart, G. A., and Allender, M. C. (2019). Tissue enzyme activities in free-living eastern box turtles (Terrapene carolina carolina). J. Zoo Wildl. Med. 50, 45–54. doi: 10.1638/2018-0079
Aguirre, A. A., and Lutz, P. L. (2004). Marine turtles as sentinels of ecosystem health: is fibropapillomatosis an indicator? EcoHealth 1, 275–283. doi: 10.1007/s10393-004-0097-3
Alkindi, A. Y. A., Mahmoud, I. Y., and Plude, J. L. (2002). Comparative study of plasma parameters in olive ridley (Lepidochelys olivacea) and hawksbill (Eretmochelys imbricata) during nesting. Sultan Qaboos Univ. J. Sci. 7, 231–239. doi: 10.24200/squjs.vol7iss2pp231-239
Allison, R. W. (2022). “Laboratory evaluation of the liver” in Veterinary hematology, clinical chemistry, and cytology. eds. M. A. Thrall, G. Weiser, R. W. Allison, and T. W. Campbell. 3rd ed (Hoboken, NJ: John Wiley & Sons), 430–433.
Anderson, E. T., Socha, V. L., Gardner, J., Byrd, L., and Manire, C. A. (2013). Tissue enzyme activities in the loggerhead sea turtle (Caretta caretta). J. Zoo Wildl. Med. 44, 62–69. doi: 10.1638/1042-7260-44.1.62
Andersson, K. E., Adamovicz, L., Mumm, L. E., Bradley, S. E., Winter, J. M., Glowacki, G., et al. (2021). Plasma electrophoresis profiles of Blanding’s turtles (Emydoidea blandingii) and influences of month, age, sex, health status, and location. PLoS One 16:e0258397. doi: 10.1371/journal.pone.0258397
Andreasen, C. B., Andreasen, J. R., and Thomas, J. S. (1997). Effects of hemolysis on serum chemistry analytes in ratites. Vet. Clin. Pathol. 26, 165–171. doi: 10.1111/j.1939-165X.1997.tb00729.x
Banks, K. W., Riegl, B. M., Richards, V. P., Walker, B. K., Helmle, K. P., Jordan, L. K., et al. (2008). “The reef tract of continental Southeast Florida (Miami-Dade, Broward and Palm Beach Counties, USA)” in Coral reefs of the USA. eds. B. Riegl and R. Dodge (Dordrecht: Springer), 175–220.
Berube, M. D., Dunbar, S. G., Rtzler, K., and Hayes, W. K. (2012). Home range and foraging ecology of juvenile hawksbill sea turtles (Eretmochelys imbricata) on inshore reefs of Honduras. Chelonian Conserv. Biol. 11, 33–43. doi: 10.2744/CCB-0898.1
Bjorndal, K. A., Bolten, A. B., and Milani, Y. C. (2000). Green turtle somatic growth odel: evidence for density dependence. Ecol. Appl. 10, 269–282. doi: 10.1890/1051-0761(2000)010[0269:GTSGME]2.0.CO;2
Bjorndal, K. A., and Bolten, A. B. (2010). Hawksbill sea turtles in seagrass pastures: success in a peripheral habitat. Mar. Biol. 157, 135–145. doi: 10.1007/s00227-009-1304-0
Bjorndal, K. A., Chaloupka, M., Saba, V. S., Diez, C. E., Van Dam, R. P., Krueger, B. H., et al. (2016). Somatic growth dynamics of West Atlantic hawksbill sea turtles: a spatio-temporal perspective. Ecosphere 7:e01279. doi: 10.1002/ecs2.1279
Blumenthal, J. M., Austin, T. J., Bell, C. D., Bothwell, J. B., Broderick, A. C., Ebanks-Petrie, G., et al. (2009). Ecology of hawksbill turtles, Eretmochelys imbricata, on a western Caribbean foraging ground. Chelonian Conserv. Biol. 8, 1–10. doi: 10.2744/CCB-0758.1
Bolten, A. B. (2003). “Variation in sea turtle life history patterns: neritic vs. oceanic developmental stages” in The biology of sea turtles, Volume II. eds. P. L. Lutz, J. C. Musick, and J. Wyneken (Boca Raton, FL: CRC Press), 243–257.
Bolten, A. B., and Bjorndal, A. (1992). Blood profiles for a wild population of green turtles (Chelonia mydas) in the southern Bahamas: size-specific and sex-specific relationships. J. Wildl. Dis. 28, 407–413. doi: 10.7589/0090-3558-28.3.407
Boulon, R. H. Jr. (1994). Growth rates of wild juvenile hawksbill turtles, Eretmochelys imbricata, in St. Thomas, United States Virgin Islands. Copeia. 1994, 811–814. doi: 10.2307/1447200
Caliendo, V., McKinney, P., Robinson, D., Baverstock, W., and Hyland, K. (2010). Plasma biochemistry and hematology values in juvenile hawksbill turtles (Eretmochelys imbricata) undergoing rehabilitation. J. Herpetol. Med. Surg. 20, 117–121. doi: 10.5818/1529-9651-20.4.117
Casal, A. B., Camacho, M., López-Jurado, L. F., Juste, C., and Orós, J. (2009). Comparative study of hematologic and plasma biochemical variables in eastern Atlantic juvenile and adult nesting loggerhead sea turtles (Caretta caretta). Vet. Clin. Pathol. 38, 213–218. doi: 10.1111/j.1939-165X.2008.00106.x
Cooke, S., and O’Connor, C. (2010). Making conservation physiology relevant to policy makers and conservation practitioners. Conserv. Lett. 3, 159–166. doi: 10.1111/j.1755-263X.2010.00109.x
Crooks, G. C., Calle, P. P., Moore, R. P., McClave, C., Toledo, P., Gomez, N. A., et al. (2023). Hematologic and biochemical values of free-ranging hawksbill sea turtles (Eretmochelys imbricata) in Glover’s reef, Belize. J. Zoo Wildl. Med. 54, 49–55. doi: 10.1638/2021-0086
Deem, S. L., Dierenfeld, E. S., Sounguet, G. P., Alleman, A. R., Cray, C., Poppenga, R. H., et al. (2006). Blood values in free-ranging nesting leatherback sea turtles (Dermochelys coriacea) on the coast of the Republic of Gabon. J. Zoo Wildl. Med. 37, 464–471. doi: 10.1638/05-102.1
Deem, S. L., and Harris, H. S. (2017). “Health assessments” in Sea turtle health and rehabilitation. eds. C. A. Manire, T. M. Norton, B. A. Stacy, C. J. Innis, and C. A. Harms (Plantation, FL: J Ross Publishing), 945–957.
Deem, S. L., Karesh, W. B., and Weisman, W. (2001). Putting theory into practice: wildlife health in conservation. Conserv. Biol. 15, 1224–1233. doi: 10.1046/j.1523-1739.2001.00336.x
Delgado, C., Valente, A., Quaresma, I., Costa, M., and Dellinger, T. (2011). Blood biochemistry reference values for wild juvenile loggerhead sea turtles (Caretta caretta) from Madeira archipelago. J. Wildl. Dis. 47, 523–529. doi: 10.7589/0090-3558-47.3.523
Diez, C., and van Dam, R. P. (2002). Habitat effect on hawksbill turtle growth rates on feeding grounds at Mona and Monito Islands, Puerto Rico. Mar. Ecol. Prog. Ser. 234, 301–309. doi: 10.3354/meps234301
Eaton, C., McMichael, E., Witherington, B. E., Foley, A., Hardy, R., and Meylan, A. (2008). In-water sea turtle research in Florida: review and recommendations. US Department of Commerce, NOAA Technical Memorandum NMFS-OPR-38, 233.
Ehsanpour, M., Ahmadi, M. R., Bahri, A. H., Afkhami, M., and Reich, K. J. (2015). Plasma biochemistry values in wild female hawksbill turtles (Eretmochelys imbricata), during nesting and foraging seasons in Qeshm Island, Persian Gulf. Comp. Clin. Path. 24, 561–566. doi: 10.1007/s00580-014-1945-3
Fleming, K. A., Perrault, J. R., Stacy, N. I., Coppenrath, C. M., and Gainsbury, A. M. (2020). Heat, health and hatchlings: associations of in situ nest temperatures with morphological and physiological characteristics of loggerhead sea turtle hatchlings from Florida. Conserv. Physiol. 8:coaa046. doi: 10.1093/conphys/coaa046
Foley, A., Dutton, P., Redlow, A., and Teas, W. (2003). The first records of olive ridleys in Florida, USA. Mar. Turt. Newsl. 101, 23–25.
Frair, W. (1977). Sea turtle red blood cell parameters correlated with carapace lengths. Comp. Biochem. Physiol. A Physiol. 56, 467–472. doi: 10.1016/0300-9629(77)90269-9
Frair, W., and Shah, B. K. (1982). Sea turtle blood serum protein concentrations correlated with carapace lengths. Comp. Biochem. Physiol. A Physiol. 73, 337–339. doi: 10.1016/0300-9629(82)90164-5
Friedrichs, K. R., Harr, K. E., Freeman, K. P., Szladovits, B., Walton, R. M., Barnhart, K. F., et al. (2012). ASVCP reference interval guidelines: determination of de novo reference intervals in veterinary species and other related topics. Vet. Clin. Pathol. 41, 441–453. doi: 10.1111/vcp.12006
Goldberg, D. W., Leitão, S. A. T., Godfrey, M. H., Lopez, G. G., Santos, A. J. B., Neves, F. A., et al. (2013). Ghrelin and leptin modulate the feeding behaviour of the hawksbill turtle Eretmochelys imbricata during nesting season. Conserv. Physiol. 1:cot016. doi: 10.1093/conphys/cot016
Hampel, M., Robinson, D., Baverstock, W., and Hyland, K. (2009). Haematology and biochemistry blood parameters of juvenile hawksbill turtles (Eretmochelys imbricata). Wildlife Middle East 4:8237.
Harris, H. S., Benson, S. R., Gilardi, K. V., Poppenga, R. H., Work, T. M., Dutton, P. H., et al. (2011). Comparative health assessment of western Pacific leatherback turtles (Dermochelys coriacea) foraging off the coast of California, 2005-2007. J. Wildl. Dis. 47, 321–337. doi: 10.7589/0090-3558-47.2.321
Hasbún, C. R., Lawrence, A. J., Samour, J. H., and Al-Ghais, S. (1998). Duodenal volvulus in free-living green turtles from coastal United Arab Emirates. J. Wildl. Dis. 34, 797–800. doi: 10.7589/0090-3558-34.4.797
Innis, C., Merigo, C., Dodge, K., Tlusty, M., Dodge, M., Sharp, B., et al. (2010). Health evaluation of leatherback turtles (Dermochelys coriacea) in the northwestern Atlantic during direct capture and fisheries gear disentanglement. Chelonian Conserv. Biol. 9, 205–222. doi: 10.2744/CCB-0838.1
Kakizoe, Y., Sakaoka, K., Kakizoe, F., Yoshii, M., Nakamura, H., Kanou, Y., et al. (2007). Successive changes of hematologic characteristics and plasma chemistry values of juvenile loggerhead turtles (Caretta caretta). J. Zoo Wildl. Med. 38, 77–84. doi: 10.1638/05-096.1
Kelly, T. R., McNeill, J. B., Avens, L., Hall, A. G., Goshe, L. R., Hohn, A. A., et al. (2015). Clinical pathology reference intervals for an in-water population of juvenile loggerhead sea turtles (Caretta caretta) in Core Sound, North Carolina, USA. PLoS One 10:e0115739. doi: 10.1371/journal.pone.0115739
Labrada-Martagón, V., Méndez-Rodríguez, L. C., Gardner, S. C., Cruz-Escalona, V. H., and Zenteno-Saívn, T. (2010). Health indices of the green turtle (Chelonia mydas) along the Pacific coast of Baja California Sur, Mexico. II. Body condition index. Chelonian Conserv. Biol. 9, 173–183. doi: 10.2744/CCB-0807.1
Lamont, M. M., and Johnson, D. (2021). Variation in species composition, size and fitness of two multi-species sea turtle assemblages using different neritic habitats. Front. Mar. Sci. 7:608740. doi: 10.3389/fmars.2020.608740
March, D. T., Vinette-Herrin, K., Peters, A., Ariel, E., Blyde, D., Hayward, D., et al. (2018). Hematologic and biochemical characteristics of stranded green sea turtles. J. Vet. Diagn. Investig. 30, 423–429. doi: 10.1177/1040638718757819
Mashkour, N., Jones, K., Kophamel, S., Hipolito, T., Ahasan, S., Walker, G., et al. (2020). Disease risk analysis in sea turtles: a baseline study to inform conservation efforts. PLoS One 15:e0230760. doi: 10.1371/journal.pone.0230760
Maulida, F. F., Hadia, S., Imron, M. A., and Reischig, T. (2017). Geometry morphometry and health status of hawksbill turtle (Eretmochelys imbricata Linnaeus, 1766) in Maratua Island, East Kalimantan-Indonesia. KnE. Life Sci. 3, 100–110. doi: 10.18502/kls.v3i4.693
Meylan, P. A., Meylan, A. B., and Gray, J. A. (2011). The ecology and migrations of sea turtles 8. Tests of the developmental habitat hypothesis. Bull. Am. Mus. Nat. Hist. 357, 1–70. doi: 10.1206/357.1
Meylan, P., and Redlow, A. (2006). Eretmochelys imbricata - hawksbill turtle. Chelonian Res. Monogr. 3, 105–127.
Moore, R. A., Camus, M. S., Harr, K., Kjielgaard-Hansen, M., Korchia, J., Jeffery, U., et al. (2020). Systematic evaluation of 106 laboratory reference data articles from nondomestic species published 2014 to 2016: assessing compliance with reference interval guidelines. J. Zoo Wildl. Med. 51, 469–477. doi: 10.1638/2019-0186
Mortimer, J. A., and Donnelly, M., IUCN SSC Marine Turtle Specialist Group. (2008) Eretmochelys imbricata. The IUCN Red List of Threatened Species 2008: e.T8005A12881238 doi: 10.2305/IUCN.UK.2008.RLTS.T8005A12881238.en
Moyer, R. P., Riegl, B., Banks, K., and Dodge, R. E. (2003). Spatial patterns and ecology of benthic communities on a high-latitude South Florida (Broward County, USA) reef system. Coral Reefs 22, 447–464. doi: 10.1007/s00338-003-0334-1
Muñoz-Pérez, J. P., Lewbart, G. A., Hirschfeld, M., Alarcón-Ruales, D., Denkinger, J., Castañeda, J. G., et al. (2017). Blood gases, biochemistry and haematology of Galápagos hawksbill turtles (Eretmochelys imbricata). Conserv. Physiol. 5:cox028. doi: 10.1093/conphys/cox028
National Marine Fisheries Service Southeast Fisheries Science Center (NMFS SEFSC). (2008). Sea turtle research techniques manual. US Department of Commerce, NOAA Technical Memorandum NMFS-SEFSC-579, 92.
Neely, K. L., Lewis, C. L., Lunz, K. S., and Kabay, L. (2021). Rapid population decline of the pillar coral Dendrogyra cylindrus along the Florida reef tract. Front. Mar. Sci. 8:656515. doi: 10.3389/fmars.2021.656515
Nishizawa, H., and Joseph, J. (2022). Differences in the morphological body condition index of sea turtles between species and size classes. J. Mar. Biol. Assoc. U. K. 102, 479–485. doi: 10.1017/S0025315422000765
Norton, T., and Wyneken, J. Body condition scoring the sea turtle. (2015). Available at: https://lafeber.com/vet/body-condition-scoring-the-sea-turtle/
Osborne, A. G., Jacobson, E. R., Bresette, M. J., Singewald, D. A., Scarpino, R. A., and Bolten, A. B. (2010). Reference intervals and relationships between health status, carapace length, body mass, and water temperature and concentrations of plasma total protein and protein electrophoretogram fractions in Atlantic loggerhead sea turtles and green turtles. J. Am. Vet. Med. Assoc. 237, 561–567. doi: 10.2460/javma.237.5.561
Page-Karjian, A., Chabot, R., Stacy, N. I., Morgan, A. S., Valverde, R. A., Stewart, S., et al. (2020). Comprehensive health assessment of green turtles Chelonia mydas nesting in southeastern Florida, USA. Endanger. Species Res. 42, 21–35. doi: 10.3354/esr01036
Page-Karjian, A., and Perrault, J. R. (2021). “Sea turtle health assessments: maximizing turtle encounters to better understand health” in Sea turtle research and conservation: Lessons learned from working in the field. ed. B. Nahill (St. Louis, MO: Academic Press), 31–44.
Peig, J., and Green, A. (2010). The paradigm of body condition: a critical reappraisal of current methods based on mass and length. Funct. Ecol. 24, 1323–1332. doi: 10.1111/j.1365-2435.2010.01751.x
Perrault, J. R., Arendt, M. D., Schwenter, J. A., Byrd, J. L., Harms, C. A., Cray, C., et al. (2020). Blood analytes of immature Kemp’s ridley sea turtles (Lepidochelys kempii) from Georgia, USA: reference intervals and body size correlations. Conserv. Physiol. 8:coaa091. doi: 10.1093/conphys/coaa091
Perrault, J. R., Page-Karjian, A., and Miller, D. L. (2016). Nesting leatherback sea turtle (Dermochelys coriacea) packed cell volumes indicate decreased foraging during reproduction. Mar. Biol. 163:232. doi: 10.1007/s00227-016-3007-7
Perrault, J. R., Page-Karjian, A., Morgan, A. N., Burns, L. K., and Stacy, N. I. (2022). Morphometrics and blood analytes of leatherback sea turtle hatchlings (Dermochelys coriacea) from Florida: reference intervals, temporal trends with clutch deposition date, and body size correlations. J. Comp. Physiol. B 192, 313–324. Available from:. doi: 10.1007/s00360-021-01422-5
Perrault, J. R., Wyneken, J., Page-Karjian, A., Merrill, A., and Miller, D. L. (2014). Seasonal trends in nesting leatherback turtle (Dermochelys coriacea) serum proteins further verify capital breeding hypothesis. Conserv. Physiol. 2:cou002. doi: 10.1093/conphys/cou002
Petrosky, K. Y., Knoll, J. S., and Innis, C. (2015). Tissue enzyme activities in Kemp’s ridley turtles (Lepidochelys kempii). J. Zoo Wildl. Med. 46, 637–640. doi: 10.1638/2015-0014.1
Prieto-Torres, D. A., Hernández, J. L., Henríquez, A. R. B., Alvarado, M. C., and Dávila, M. J. (2013). Blood biochemistry of the breeding population of green turtles (Chelonia mydas) in the Aves Island Wildlife Refuge, Venezuela. South Am. J. Herpetol. 8, 147–154. doi: 10.2994/SAJH-D-13-00010.1
Reséndiz, E., and Lara-Uc, M. M. (2018). Health assessments in free-ranging sea turtles: perspective of animal welfare in wildlife. Anim. Welfare 29, 794–799. doi: 10.5772/intechopen.76111
Rossi, S., Sánchez-Sarmiento, A. M., Dos, S. R. G., Zamana, R. R., Prioste, F. E. S., Gattamorta, M. A., et al. (2019). Monitoring green sea turtles in Brazilian feeding areas: relating body condition index to fibropapillomatosis prevalence. J. Mar. Biol. Assoc. UK. 99, 1879–1887. doi: 10.1017/S0025315419000730
Rousselet, E., Stacy, N. I., Lavictoire, K., Higgins, B. M., Tocidlowski, M. E., Flanagan, J. P., et al. (2013). Hematology and plasma biochemistry analytes in five age groups of immature, captive-reared loggerhead sea turtles (Caretta caretta). J. Zoo Wildl. Med. 44, 859–874. doi: 10.1638/2012-0162R1.1
Ruzicka, R. R., Colella, M. A., Porter, J. W., Morrison, J. M., Kidney, J. A., Brinkhuis, V., et al. (2013). Temporal changes in benthic assemblages on Florida Keys reefs 11 years after the 1997/1998 El Niño. Mar. Ecol. Prog. Ser. 489, 125–141. doi: 10.3354/meps10427
Salvarani, P. I., von Osten, J. R., and Morgado, F. (2018). Plasma biochemistry values in wild female hawksbill turtle (Eretmochelys imbricata) during nesting in Mexican coast. Braz. J. Vet. Res. Anim. Sci. 55:e134727. doi: 10.11606/issn.1678-4456.bjvras.2018.134727
Stacy, N. I., Alleman, A. R., and Sayler, K. A. (2011). Diagnostic hematology of reptiles. Clin. Lab. Med. 31, 87–108. doi: 10.1016/j.cll.2010.10.006
Stacy, N. I., Bjorndal, K. A., Perrault, J. R., Martins, H. R., and Bolten, A. B. (2018). Blood analytes of oceanic-juvenile loggerhead sea turtles (Caretta caretta) from Azorean waters: reference intervals, size-relevant correlations and comparisons to neritic loggerheads from western Atlantic coastal waters. Conserv. Physiol. 6:coy006. doi: 10.1093/conphys/coy006
Stacy, N. I., Chabot, R. M., Innis, C. J., Cray, C., Fraser, K. M., Rigano, K. S., et al. (2019). Plasma chemistry in nesting leatherback sea turtles (Dermochelys coriacea) from Florida: understanding the importance of sample hemolysis effects on blood analytes. PLoS One 14:e0222426. doi: 10.1371/journal.pone.0222426
Stacy, N. I., and Harr, K. E. (2020). “Hematology of reptiles with a focus on circulating inflammatory cells” in Infectious disease and pathology of reptiles: a color atlas and text. eds. E. R. Jacobson and M. M. Garner. 2nd ed (Boca Raton, FL: CRC Press), 267–330.
Stacy, N., and Innis, C. (2017). “Clinical pathology” in Sea turtle health and rehabilitation. eds. C. A. Manire, T. M. Norton, B. A. Stacy, C. J. Innis, and C. A. Harms (Plantation, FL: J. Ross Publishing), 147–207.
Stamper, M. A., Harms, C., Epperly, S. P., Braun-McNeill, J., Avens, L., and Stoskopf, M. K. (2005). Relationship between barnacle epibiotic load and hematologic parameters in loggerhead sea turtles (Caretta caretta), a comparison between migratory and residential animals in Pamlico Sound, North Carolina. J. Zoo Wildl. Med. 36, 635–641. doi: 10.1638/04-074.1
Tristan, T., and Norton, T. (2017). “Physical examination” in Sea turtle health and rehabilitation. eds. C. A. Manire, T. M. Norton, B. A. Stacy, C. J. Innis, and C. A. Harms (Plantation, FL: J. Ross Publishing), 99–121.
Voosen, P. (2019). Scientists track Florida’s vanishing barrier reef. Science 364:319. doi: 10.1126/science.364.6438.319
Weiss, D. (1984). Uniform evaluation and semiquantitative reporting of hematologic data in veterinary laboratories. Vet. Clin. Pathol. 13, 27–31. doi: 10.1111/j.1939-165X.1984.tb00836.x
Whiting, S. D., Guinea, M. L., Fomiatti, K., Flint, M., and Limpus, C. J. (2014). Plasma biochemical and PCV ranges for healthy, wild, immature hawksbill (Eretmochelys imbricata) sea turtles. Vet. Rec. 174:608. doi: 10.1136/vr.101396
Wikelski, M., and Cooke, S. J. (2006). Conservation physiology. Trends Ecol. Evol. 21, 38–46. doi: 10.1016/j.tree.2005.10.018
Wood, L. D., Brunnick, B., and Milton, S. L. (2017). Home range and movement patterns of subadult hawksbill sea turtles in Southeast Florida. J. Herpetol. 51, 58–67. doi: 10.1670/15-133
Wood, F. E., and Ebanks, G. K. (1984). Blood cytology and hematology of the green sea turtle. Herpetologica 40, 331–336.
Keywords: health assessment, hematology, marine turtle, plasma biochemistry, physiology, protein electrophoresis, somatic growth
Citation: Stacy NI, Perrault JR and Wood LD (2023) Blood analytes of hawksbill sea turtles (Eretmochelys imbricata) from Florida waters: reference intervals and size-relevant correlations. Front. Ecol. Evol. 11:1199688. doi: 10.3389/fevo.2023.1199688
Edited by:
Mary Ann Ottinger, University of Houston, United StatesReviewed by:
Edward Narayan, The University of Queensland, AustraliaLuis Gerardo Herrera M, Universidad Nacional Autónoma de México, Mexico
Fernando Manuel Raposo Morgado, University of Aveiro, Portugal
Copyright © 2023 Stacy, Perrault and Wood. This is an open-access article distributed under the terms of the Creative Commons Attribution License (CC BY). The use, distribution or reproduction in other forums is permitted, provided the original author(s) and the copyright owner(s) are credited and that the original publication in this journal is cited, in accordance with accepted academic practice. No use, distribution or reproduction is permitted which does not comply with these terms.
*Correspondence: Nicole I. Stacy, c3RhY3luQHVmbC5lZHU=