- 1Doctoral School of Animal Science, University of Debrecen, Debrecen, Hungary
- 2Arctic Centre, University of Lapland, Rovaniemi, Finland
- 3Department of Nature Conservation Zoology and Game Management, University of Debrecen, Debrecen, Hungary
- 4Department of Tisza Research, Institute of Aquatic Ecology, Debrecen, Hungary
- 5Institute of Metagenomics, University of Debrecen, Debrecen, Hungary
Introduction: Urbanization has led to a recent surge of interest in urban biodiversity, and wildlife responses to urban environments have become a hot topic in environmental sciences and biodiversity conservation. However, adaptations to urbanization and the factors driving them are less understood.
Methodology: We studied possible morphological adaptations to urban environments by comparing body size and condition of adult and post-fledging juvenile Hooded Crows (Corvus cornix), a bird species that has recently colonised cities in eastern Europe, between two urban and two rural populations, between two different-sized cities and between locations within one city in Hungary.
Results: Adult crows from the rural cropland-woodland area were lighter and in poorer condition than crows from the rural grassland area or those from the medium-sized city. There were no differences in morphological traits of adults or juveniles between the large and the medium-sized cities. The comparison of multiple trapping locations within the city of Debrecen showed that juveniles in the Zoo area were larger, heavier, and in better condition than individuals in the other locations and that adults in the Zoo had longer wings and tarsi than adults in other locations. Our results indicated that urbanized Hooded Crows showed some morphological changes to live in urban environments, but we did not find large-scale, consistent differences between urban and rural areas. However, we found significant variation within one city.
Discussion: Our results suggest that urban-rural environmental differences may be mediated by local factors, of which the year-round availability of anthropogenic food is fundamental. Such food is widely available in cities, although its quality may be suboptimal for bird development. Our study suggests that the variation in body size variables is probably more site-dependent than gradient-dependent and shows that documenting wildlife adaptations to urban environments requires multiple spatial scales ranging from regional to local (within-city) scales.
1 Introduction
Urbanization is an ever-increasing anthropogenic environmental change, rapidly expanding at the expense of natural ecosystems (Sih et al., 2011; Gil and Brumm, 2014). Urbanization creates environments that are unfavourable to most of the plant and animal species that once occupied the areas where cities are now present. These novel urban environments are characterized by high levels of human disturbances, pollution, and landscape changes (Shochat et al., 2006; Chamberlain et al., 2009). Urban environments expose wildlife to novel challenges representing selective constraints that are changing the composition of animal communities, and the overall response of wildlife is a strong decrease in biodiversity in cities (Adams, 2016; McDonald et al., 2020; Lepczyk et al., 2023). While many wild species avoid urban areas, some species seem to be adapting to or even preferring such environments (Xu et al., 2020; Spotswood et al., 2021; Lepczyk et al., 2023; Neate-Clegg et al., 2023). Yet, recent research has shown that urban areas can also provide essential ecological systems to support and protect biodiversity (Spotswood et al., 2021; Lepczyk et al., 2023). Nonetheless, numerous studies have indicated that urban areas differ from rural ones in species composition and that urban areas are mostly dominated by a few generalist species (Shochat et al., 2006; Evans et al., 2009; Morelli et al., 2016; Evans et al., 2018). There are many factors to which animals living in urban areas should adapt to, including warmer microclimates, permanent anthropogenic food supplies (Heiss et al., 2009; Baltensperger et al., 2013; Fidino et al., 2021), novel hiding places, and decreased predation pressure (Krausman et al., 2014; Eötvös et al., 2019). While the effects of urbanization on community-level structure are well documented, morphological adaptations to urbanization are still inadequately understood. Moreover, the ultimate driving factors behind the persistence of some species in cities are still debated, and their assessment is considered a top priority in environmental studies (Shochat et al., 2006; Lowry et al., 2013; Spotswood et al., 2021; Fidino et al., 2021). Although some studies have analysed morphological and physical differences (Evans et al., 2009; Meillère et al., 2015; Amiot et al., 2022), as well as phenotypic adaptations of animals living in urban environments (Shochat, 2004; Bókony et al., 2012; Meillère et al., 2017), it is not clear what precisely makes a successful urban adapter or what changes result in urban populations of wild species.
Earlier studies have indicated that some species (e.g., house sparrows Passer domesticus; magpies Pica spp., crows Corvus spp.; and rooks Corvus frugilegus) do better in urban settings than others (Zimaroyeva et al., 2016; Xu et al., 2020; Jokimäki et al., 2021; Jokimäki et al., 2022). Especially resident, as opposed to migratory, and omnivorous, as opposed to food-specialist, bird species thrive in cities (Chace and Walsh, 2006; Benmazouz et al., 2021; Hahs et al., 2023). These species might benefit, e.g., from the warmer winter microclimate and a wide range of anthropogenic food sources in cities (Withey and Marzluff, 2009; Seress and Liker, 2015). In particular, the ability to use anthropogenic food sources may be fundamental for species to adapt to city life (Møller, 2009; Lowry et al., 2013; Sol et al., 2013). As urban habitats provide more anthropogenic food and widely different food types than natural habitats (Shochat et al., 2006; Chamberlain et al., 2009), successful urban animals may need behavioural and/or morphological adaptations to be able to use these resources (Shochat et al., 2006; Partecke, 2014). For instance, Shochat (2004) suggested that high food availability and low mortality characterizing urban environments might explain the changes observed in urban individuals, especially in foraging behaviour, which may subsequently influence bill morphology (Kulemeyer et al., 2009). Additionally, easily accessible food in urban areas seems to influence habitat choice because synanthropic birds such as corvid species generally increase in number with increased food availability (Preininger et al., 2019; García-Arroyo et al., 2023). However, the low quality of widely available food may have an adverse impact on urban birds (Seress et al., 2020). For instance, Shochat (2004) suggested that urban food resources might be inadequate and lead to poor body condition in urban wildlife, ultimately resulting in a decrease in the average body mass of individuals. In addition to low-quality food resources, chemical pollution and increased anthropogenic noise and light pollution can also have detrimental effects on bird populations during different phases of the life cycle (Chace and Walsh, 2006; Bonier, 2012; Gil and Brumm, 2014).
Furthermore, urban environments can be warmer than rural areas (Evans et al., 2009), and the body size of birds has been found to decrease in response to a warmer climate (Yom-Tov et al., 2006), and individuals of the same species living in colder environments can be larger than conspecifics living in warmer conditions (“Bergmann’s rule”; Meiri and Dayan, 2003; McCollin et al., 2015; Lövei and Magura, 2022). Järvinen et al. (1988) have noticed that the same average decrease in House Sparrow size detected in a 700 km north-south gradient in Finland (smaller sparrows in the south) can be detected even within an urbanization gradient (the capital city of Finland, Helsinki), when one moves from the suburban areas to the center of the city (smaller sparrows in the center of the city). Also, some studies have reported morphological adaptations in birds at a local scale (Hostetler, 2001 ;Husby et al., 2011; Teplitsky et al., 2008; Brommer et al., 2014; Kaiser et al., 2016; Baldwin et al., 2023). In addition, a recent study demonstrated that certain taxa, such as birds, displayed smaller body size and smaller mobility in more urbanized areas (Hahs et al., 2023).
Urbanization may also affect wing length and shape. For instance, birds in warmer climates can have longer wings than birds of the same species in colder climates (“Allen’s rule”; McCollin et al., 2015), and sedentary birds have shorter and more pointed wings than migratory ones (“Seebohm’s rule”; Fitzpatrick, 1998; Evans et al., 2009). Previous studies found that urbanization may favour a resident way of life (Pulliainen, 1963; Yeh and Price, 2004; Partecke et al., 2005; Hahs et al., 2023) or may even reduce migratory tendencies in some species (e.g., Partecke et al., 2005; Møller et al., 2014; Bonnet-Lebrun et al., 2020). Also, multiple anthropogenic effects (e.g., anthropogenic food, a warmer microclimate, and less predation; Liker, 2020) may act in synergy to accelerate morphological changes, e.g., in bill size and shape and tarsus length, as reported in bird species such as the Blackbird (Turdus merula) (Evans et al., 2009) and the New Zealand Fantail (Rhipidura fuliginosa) (Amiot et al., 2022).
However, because urbanized birds need to adapt to diverse elements of their environment, it is often challenging to unravel the overall impact of urbanization on individuals. In fact, the results of earlier studies related to morphological and behavioural adaptations appear to vary substantially between areas and/or species (Evans et al., 2009; Seress and Liker, 2015; Sepp et al., 2018). Some researchers have not detected intra-species differences in morphological or body condition traits between different habitat types (Sol et al., 2013) or in indices of locomotory morphology (Amiot et al., 2022). However, Caizergues et al. (2018) as well as Liker et al. (2008) reported morphological differences among habitats.
In general, results from a single broad-scale comparison study between urban and rural areas may give information about which species may occur in urban areas, but the results might change over the years, giving a misleading picture of the situation (Chamberlain et al., 2009). For instance, anthropogenic factors such as temperature, pollution, food availability, and nest sites can be driven by unique processes and thus influence birds in a scale-dependent manner (Clergeau et al., 2006; Merckx et al., 2018). Therefore, it may be appropriate to analyse the potential effect of local urbanization on Hooded Crows since such bird species may respond to similar biophysical features but within an entirely different landscape (Jackson and Fahrig, 2015).
In our study, we used the Hooded Crow (Corvus cornix) as a model species to study morphological variation at four different study sites: two cities with different sizes and two rural sites. Because urban areas might differ in temperature and food availability from rural ones, these resources might also differ between large and small cities. Urbanization creates different environments depending on the extent to which human activities may influence wildlife at different levels (Hostetler, 2001; McCaffrey and Mannan, 2012; Pennington and Blair, 2011). Moreover, the complexity of the urban environment calls for the study of the effects of various anthropogenic factors at a local level (Melles et al., 2003; Fidino et al., 2021). Thus, a more restricted investigation within one city might highlight the effect of different environmental factors acting locally.
A recent review (Benmazouz et al., 2021) found that 23% of all corvid species (n = 133) regularly occur in urban environments globally and suggested that corvids, especially crows (Corvus spp.), may thus be good model species in understanding the effects of urbanization on animal behaviour and morphology, along with other species such as Great Tits (Parus major), House Sparrows, and Blackbirds (Turdus merula). Assuming that urban-induced changes in environmental conditions, such as temperature and food availability, will cause changes in the morphology of crows, we predicted that due to the urban heat island phenomenon urban crows would be smaller and less heavy than their rural conspecifics, in correspondence with Bergmann’s rule. Due to the great anthropogenic food availability in cities, we predicted that urban crows would be larger than rural ones. However, if the quality of urban food is low, then we can predict that urban crows would smaller and worse condition than in crows living in less urbanized environments (Shochat, 2004; Seress et al., 2020). Also, assuming that urban crows will be more sedentary than rural ones (Yeh and Price, 2004; Partecke et al., 2005; Bonnet-Lebrun et al., 2020) and that migratory birds will have more pointed wings (Seebohm’s rule), we predicted that urban crows will have shorter wings than rural ones. Contrary to Allen’s rule on longer wings in warmer climates, we presume that sedentary Hooded Crows in Hungary are more likely to conform to Seebohm’s rule of shorter wings than to Allen’s rule of larger extremities, mostly because it is still uncertain whether wing length conforms to Allen’s rule (McCollin et al., 2015). Finally, by assuming higher availability of anthropogenic food sources in urban than rural environments (Tryjanowski et al., 2015; Cereghetti et al., 2019), we predicted that morphological and body mass differences exist between urban and rural areas or even within the same city if food availability varies within the city.
In this study, we compared the morphology of Hooded Crows between four distinct sites: two rural ones and two urban ones of different sizes, as well as between multiple capture sites with different infrastructure within a single city. Thus, if the food factor is important, we predicted that crows from Budapest (a larger city) would be larger than crows from Debrecen (a smaller city). Moreover, over the last decades, the urbanization process of the Hooded Crow population in Debrecen has been monitored, and the Zoo area seemed to be the starting place of the urban colonization by the Hooded Crow (Kövér et al., 2015; Kövér et al., 2019). It is highly possible that the great, continuous, and predictable artificial food sources have attracted crows to settle there first. However, urban environments are not homogeneous, and (anthropogenic) food availability might differ a lot even within a city border, highlighting the importance of the local-scale urban studies (Melles et al., 2003). Additionally, several studies have demonstrated morphological adaptations in urban birds at local levels (Järvinen et al., 1988; Husby et al., 2011; Teplitsky et al., 2008; Brommer et al., 2014; Kaiser et al., 2016; Baldwin et al., 2023; Hahs et al., 2023). The five trapping locations in the city of Debrecen differ mainly in their built-up infrastructure, availability of anthropogenic food and level of human presence (more details in Study areas). We thus predicted that these small-scale differences between urban locations may also explain some variation in body size variables of adult and juvenile Hooded Crows. More specifically, we expected higher values of body size measurements in the case of the Zoo than in other areas because the availability of anthropogenic food was highest in this location.
2 Materials and methods
2.1 Study areas
We collected morphological and body condition data from four sites in Hungary, from two urban areas (Budapest and Debrecen) and two rural areas (Szakoly and Balmazújváros; Figure 1). In addition, more detailed data were collected from five locations within the city of Debrecen (Figure 1). In total, we sampled individuals in eight trapping sites. These study areas and sites were chosen because they hosted wildlife management programs that included the trapping of Hooded Crows, which enabled us to measure the body size of large numbers of individuals caught in the traps by the wildlife managers.
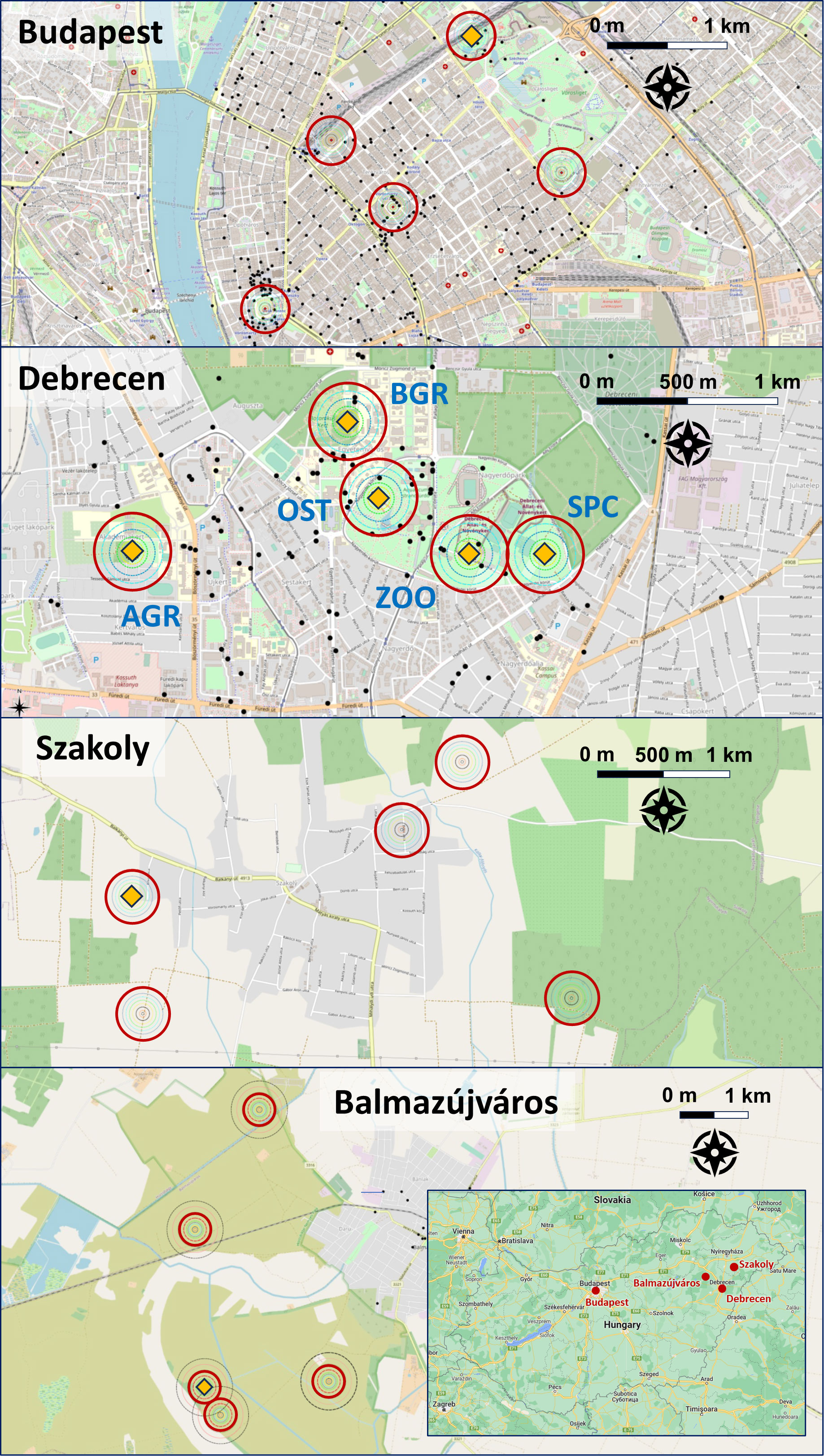
Figure 1 Trapping sites (orange diamonds), their 200-m-radius circular buffers (red circles, n = 5 in each of the four area) and potential anthropogenic food sources (dark dots) in Budapest (urban), Debrecen (urban), Szakoly (rural) and Balmazújváros (rural). For geographic location of the four study areas within Hungary, please see inset. Locations within Debrecen, AGR, Agricultural Campus of University of Debrecen; OST, Open Stage Theater; SPC, Sports Centre; ZOO, Zoo. Colour codes, dark green: forest, light green: city park, olive green: grassland, grey: built-up area. Source of map: OpenStreetMap project, openstreetmap.org.
Hungary has a typical continental climate with warm summers and cold, snowy winters, with a long-term mean annual temperature of 8-11°C. The city of Budapest (47°29’33”N; 19°03’05”E) is the capital and most populous city of Hungary, with 1.706.851 inhabitants (3250.3 inhabitants/km2, Hungarian Central Statistical Office, 2022b). Hooded Crows in Budapest were first reported in the 1970s, and the settlement of the species has occurred in the early 1980s (Tapfer, 1978; Tapfer, 1985, as cited in Kövér et al., 2015). In Budapest, all data were collected in the Zoo because of the general importance of Zoos in the establishment and colonization of crows in urban areas (Kövér et al., 2019) and because there was an active trapping programme in the Zoo.
The city of Debrecen (47°52’99.7”N; 21°63’91.6”E) is an urban area with 199,725 inhabitants (human population density 432.6 inhabitants/km2, Hungarian Central Statistical office, 2022a), 195 km east of Budapest. The built-up core areas of the city are surrounded mostly by the streets of the old agricultural town, which are bordered by tree lines, urban parks, and residential areas. From the north, the city of Debrecen is bordered by Nagyerdő or the Great Forest, the first nationally protected area in Hungary (declared in 1939), whereas suburban residential areas, gardens, and industrial sites cover the peripheries of the city. Hooded Crows started to breed in Debrecen in the 1980s, and between 2006 and 2013, their urban population more than doubled, and exceeded 100 nesting pairs, with a density of more than 4 nests/km2 (Kövér et al., 2015; Kövér et al., 2019). We selected five trapping locations in Debrecen in the northern part of the city (Figure 1), where Hooded Crows first established as nesting species. These five trapping locations differ mainly in the proportion of built-up infrastructure, availability of anthropogenic food and level of human presence (disturbance). The city Zoo (ZOO) has year-round high availability of artificial food in the open-top animal enclosures, animal food storage areas, and open garbage cans, and seasonally varying human presence (Kövér et al., 2019). The Sports Centre (SPC) has a high human presence throughout the year, mostly natural food availability (large open grassy athletic fields) and no anthropogenic food (Kövér et al., 2019). The agricultural campus of the University of Debrecen at Böszörményi Street (AGR) has moderate human presence and food availability, with smaller extents of open grassy areas. The OST location is a small grassy park surrounded by a park forest, with high human presence and some food availability in open-air restaurants and garbage cans. Finally, the Botanical Garden of the University of Debrecen (BGR) is a collection of native and non-native plants with intermediate availability of anthropogenic food and low presence of humans. Because we captured only four individuals at the BGR location, we have excluded data from this location from all analyses presented. Trapping locations at the Zoo and the SPC were c. 400 m from one another, whereas the distance between any other pair of locations was higher than 500 m (Figure 1). While we observed that some adults can fly several km to feeding sites (garbage dump in the S part of the city; P. Paládi, unpubl.data), adults are highly territorial during breeding and stay on their territory with their young for several weeks or months after the young leave the nest, to which they often return within this period. Most of our data were collected in the breeding period or soon after, but in all cases before Hooded Crows started to aggregate and formed flocks before the winter (in November). Thus, there was little chance that individuals moved extensively between the trapping locations.
The rural areas were near Szakoly, a village 35 km north-east of Debrecen (218 km from Budapest), and near Balmazújváros, a town 25 km west of Debrecen (178 km from Budapest) (Figure 1). Szakoly lies in the Nyírség sand hill area, historically rich in oak and birch forests (Tóber and Kiss, 2014), but now mostly covered by black locust and poplar forests. The Szakoly area covers 41.44 km2 and has 2563 inhabitants (61.8 inhabitants/km2, Hungarian Central Statistical office, 2022c). The site of data collection was in the hunting area outside the village (Figure 1). Balmazújváros lies on loess and alkali soils near Hortobágy National Park and has an area of 205.45 km2 and a population of 16.954 people (82.5 inhabitants/km2, Hungarian Central Statistical Office, 2022d). Balmazújváros is a typical rural town, with a central park surrounded by historic buildings. Trapping was conducted inside Hortobágy National Park, an area of vast grasslands interspersed with wetlands and small patches of wooded areas (Figure 1).
2.2 Study species
The Hooded Crow is a large passerine commonly found throughout northern, eastern, and south-eastern Europe and parts of the Middle East (Keller, 2020). Depending on the part of its range, the Hooded Crow is either a sedentary (Kövér et al., 2015; Szala et al., 2020) or a partly migratory species (Suhonen and Jokimäki, 2019). Generalist by nature and resident in Hungary, the Hooded Crow is a typical scavenger feeding on a wide variety of food such as grains, seeds, nuts, fruits, a variety of worms, mice, insects, fish, turtles, frogs, clams, birds, bird eggs and nestlings, carrion, litter, and garbage (Cornell Lab of Ornithology, 2015). Traditionally a rural species of agricultural areas, the Hooded Crow was first reported to nest in a city in Hungary in 1959 (Juhász, 1983). In Hungary and several other parts of eastern Europe, the increasing prevalence of Hooded Crows in urban areas is a reason for human concern. Crows are considered nuisance because they scatter garbage, disturb humans and pets with aggressive vocalisations or attacks during the breeding season, and can transmit diseases through faecal droppings (Vuorisalo et al., 2003; Kövér et al., 2022). The Hooded Crow is a game or quarry species and is frequently culled by hunting associations outside human settlements. The Hooded Crow population in Hungary is estimated at c. 50,000 pairs (Nagy, 2021). While most pairs breed in lowland rural agricultural areas, crows have become abundant in larger cities in Hungary (Nagy, 2021). There are no estimates on the size of the breeding population in Budapest or in the two rural areas studied. In Debrecen, the number of breeding pairs exceeded 150 pairs in 2018-2019 and is likely approaching 200 pairs currently (Kövér L., unpubl. data).
According to our non-published observations of wing-tagged birds in Debrecen, the median annual home range size of Hooded Crows is 41 ha (interquartile range 14 to 84 ha, n = 21 birds). Correspondingly, the closely related Carrion Crow has been reported to have a home range of 22-29 ha (Nakamura, 1998), and the House Crow has a home range of 0.2-22.3 ha (Lim and Sodhi, 2009). According to Møller (1983), in Denmark, resident Hooded Crows are year-long sedentary birds that stay in or near their territory, similar to Hooded Crows residing in Debrecen. Møller (1983) reported that these resident crows were commonly found near their feeding grounds; they did not use energy to fly to the feeding grounds as they knew the location of food sources. Interestingly, several studies have demonstrated the increasing abundance of crows in developed areas with easily accessible anthropogenic food sources (Preininger et al., 2019; García-Arroyo et al., 2023). It seems that these crows prefer to reside near easily accessible food sources. Moreover, American Crow populations were observed to increase in areas with more anthropogenic resources, reduce their home range size, and use less space for breeding in urban areas (Marzluff et al., 2001; Withey and Marzluff, 2005; Marzluff and Neatherlin, 2006; Withey and Marzluff, 2009). Also, in a recent study, Hahs et al. (2023) demonstrated that wild birds displayed smaller mobility in more urbanised areas and thus had reduced home ranges in areas where they could exploit local resources.
2.3 Data collection on hooded crow body size
We captured Hooded Crows by using ladder entrance traps between April and November 2020 in all urban and rural areas (Figure 1) (Kövér et al., 2018). This period corresponds to the nesting and chick-rearing and post-fledging periods of crows in Hungary. In urban areas, trapping was carried out twice a week, whereas in rural areas, trapping was conducted during two visits (Bálmazújváros) or four visits (Szakoly) between April and June 2020. All birds were caught by IB and LK, with the assistance of a corresponding person from the local wildlife management units in rural areas. Traps had a fixed structure and were open continuously during the trapping period. In Debrecen, traps were visited only after sunset to avoid the recognition of the researchers by the crows, which may influence data collection in other ongoing studies of urban crows. In the three other study areas, this was not a concern and traps were visited during the daytime. To minimize the problem that our sample of captured crows was not a random sample of the local populations and to minimize the chances that trap-happy, trap-shy, weak, or less intelligent individuals are caught, we repositioned the traps c. once a month by moving them 10-30 m away within one trapping location to prevent birds from getting used to the traps.
Once a bird was captured, we measured ten morphological traits. Body mass (g) was measured using a Pesola spring balance, and the fat reserve score was estimated on a scale of 1 to 3, as in standard biometric measurements, by feeling the keel and the breast muscles (Gregory and Robins, 1998; Roloff, 2003). We used a metal calliper to measure body length (from tip of bill to tip of tail), wing length (from carpal joint to wingtip), tarsus length, tail length, skull length (from base of bill to back of skull), skull width, bill length (from tip to skull along the culmen), and bill thickness (maximum vertical extent of the bill) (accuracy ± 0.1 mm). We determined the age of the crows (juvenile vs. adult) by the colour of the inside of the upper mandible (O’Donoghue et al., 1998). Crows that hatched and fledged in the study year (2020) were considered juveniles in this study. Fledged juveniles are not fed by the parents (except while still at the nest); thus, juvenile crows do not rely on adults for food. To avoid inter-observer bias in measurements, all morphological measurements were taken by IB. In all study areas, individuals were disposed of once captured as part of an ongoing wildlife population management program at that time. Thus, each individual was measured only once and there was no pseudo-replication arising from repeated measurements of the same individual.
2.4 Data on anthropogenic food sources and temperature
To evaluate possible explanations for the morphological differences observed, we also compared the availability of anthropogenic food sources and the microclimate of the study areas. To this end, we surveyed the immediate surroundings of the traps for anthropogenic food sources in circular buffers of 200 m radius (an area of 12.6 ha) around each urban and rural trap. Observations of wing-tagged birds suggested that the median annual home range size of Hooded Crows in Debrecen is 41 ha (interquartile range 14 to 84 ha, n = 21 birds), but also that it shows large variation because some crows nesting in the studied northern locations occasionally fly 6-7 km to the garbage dump in the south-western part of the city for feeding (P. Paládi, unpubl. data). Interestingly, several studies have demonstrated the increasing abundance of crows in developed areas with easily accessible anthropogenic food sources (Preininger et al., 2019; García-Arroyo et al., 2023), suggesting that crows prefer to nest near easily accessible food sources. American Crows, for example, were observed to increase in numbers in areas with more anthropogenic resources, to reduce their home range size, and to use less space for breeding in urban areas (Marzluff et al., 2001; Marzluff and Neatherlin, 2006; Withey and Marzluff, 2009). In Debrecen, we had five traps with five buffers around them. In Budapest and both rural areas, where we had only one trap per site, we also surveyed four additional, randomly selected 200-m-radius buffers to estimate the availability of anthropogenic food sources, to obtain estimates from an equal number of circles (n = 5) at each study area (Figure 1). We mapped food availability by systematically walking the study areas between September and October 2022 and recording the location of potential food sources such as open-air restaurants, Zoo feeding areas, trashcans, garbage piles, etc. (García-Arroyo et al., 2023) using a hand-held GPS unit in the study areas. There were no known places of crow concentrations, such as landfills or garbage dumps, in the studied areas. We then stored, processed, and visualized these data using QGIS 2.6.3.1 (QGIS Development Team, 2022) and created buffers of radius of 200 m around each trap location to characterise the availability of anthropogenic food sources.
Temperature data were obtained from the Visual Crossing online weather database (VisualCrossing Cooperation, 2022). We extracted the annual average temperature of the last 10 years for the urban study areas (Budapest, i.d.: E3429, 6 km from the nearest trap site; and Debrecen, i.d.: 12882099999, 5 km from the nearest trap site) and the weather station closest to the rural study areas (for Szakoly, Nyíregyháza station, i.d.: 12892099999, 29 km away from the nearest trap site; and for Balmazújváros, the Poroszló station, i.d.: 12866099999, 53 km from the nearest trap site) (Visual Crossing Corporation, 2022).
2.5 Statistical analysis
We performed three sets of statistical analyses to test differences in crow morphology. First, we analysed the body size differences of adults between the two urban and the two rural populations (Analysis 1). Only three juveniles were captured in the two rural areas total, thus, the joint analysis of data from the four areas was not performed for juveniles. Second, we analysed differences between juveniles of the two cities of different sizes, i.e. between Budapest (large) and Debrecen (medium-sized city) (Analysis 2). Finally, we analysed differences in juvenile and adult body size between four trapping locations within the Debrecen population only (Analysis 3). In one location, we only captured adults, thus, Analysis 3 is limited to three locations in the case of juveniles. Data from juveniles and adults were analysed separately because juvenile body size was expected to vary more than adult body size throughout the study period.
In all analyses, the response variables were the ten original variables (body mass, fat reserve score, body length, wing length, tarsus length, tail length, skull length, skull width, bill length, and bill thickness) plus body condition, estimated by the residuals from a simple linear regression of body mass over tarsus length as in previous studies (Chamberlain et al., 2009; Labocha and Hayes, 2012; Dulisz et al., 2021) (11 response variables total).
We analysed the differences in body size variables by building general linear models using the ‘lm’ function of the ‘stats’ package of R (R Core Team, 2022) with the four areas (Analysis 1), the two cities (Analysis 2), or the four (adults) or three (juveniles) locations (Analysis 3) as main effects. Because this study involved body size measurements taken between the months of April and November, we controlled for possible seasonal differences by using the Julian week of capture (week 1 as the first week of the year) as a covariate in linear models. We first fit the models with the main effect, Julian week, and their interaction. When the interaction term was not significant (p < 0.05), we removed it from further analyses. When the interaction was significant, we prepared interaction plots to evaluate the main effect as a function of season (Julian week). For post-hoc testing when the main effect was significant, we used the ‘emmeans’ function of the ‘emmeans’ package in R to calculate adjusted means and their standard errors (S.E.) and report the t-statistic and its associated p-value.
Before fitting linear models, we checked the assumption of normality by the Shapiro-Wilk test and the assumption of homoscedasticity by the Bartlett test. In cases where assumptions were not met, we log-transformed the variables for the linear models, these are indicated as log (variable name) in the tables. Because not all measurements were available for all birds, sample sizes may differ between statistical tests. Finally, because we conducted several statistical tests on the same dataset, we calculated and report p-values adjusted by the False Discovery Rate method (Benjamini and Hochberg, 1995) in all tests of main effects, obtained by using the ‘p.adjust’ function of the ‘stats’ package.
To compare data on mean annual temperature between the four study areas, we used one-way analysis of variance and used contrast values and associated p-values to infer significance. Furthermore, we compared the number of potential anthropogenic food sources in 200-radius circles around trapping locations in Budapest and Debrecen by using Wilcoxon rank sum test. All calculations and statistical testing were conducted in R version 4.2.2 (R Core Team, 2022; RStudio Team, 2022).
3 Results
We captured and measured a total of 248 individuals of Hooded Crows. Our sample size included 43 birds from rural areas (Balmazújváros: 2 juveniles, 26 adults; Szakoly: 1 vs. 14) and 205 birds from urban areas (Budapest: 28 vs. 10; Debrecen: 128 vs. 39).
3.1 Morphological differences in adult body size between four study areas
The body mass and condition of the adult Hooded Crows differed between the study areas (Figure 2). Crows in the rural Szakoly area were lighter and in poorer body condition than rural individuals from Balmazújváros (body mass, F3,84 = 4.079, padj = 0.049, contrast estimate: 50 ± 15.3, t84 = 3.272, padj = 0.008; body condition, F3,84 = 9.154, padj = 0.001, 60 ± 13.3, t84 = 4.558, padj = 0.0001) or urban individuals from Debrecen (body mass, contrast: 41 ± 14.5, t84 = 2.832, padj = 0.029; body condition, 61 ± 12.5, t84 = 4.895, padj < 0.0001) (Figure 2). There were no differences in adult body size between the two urban areas (adjusted p > 0.56).
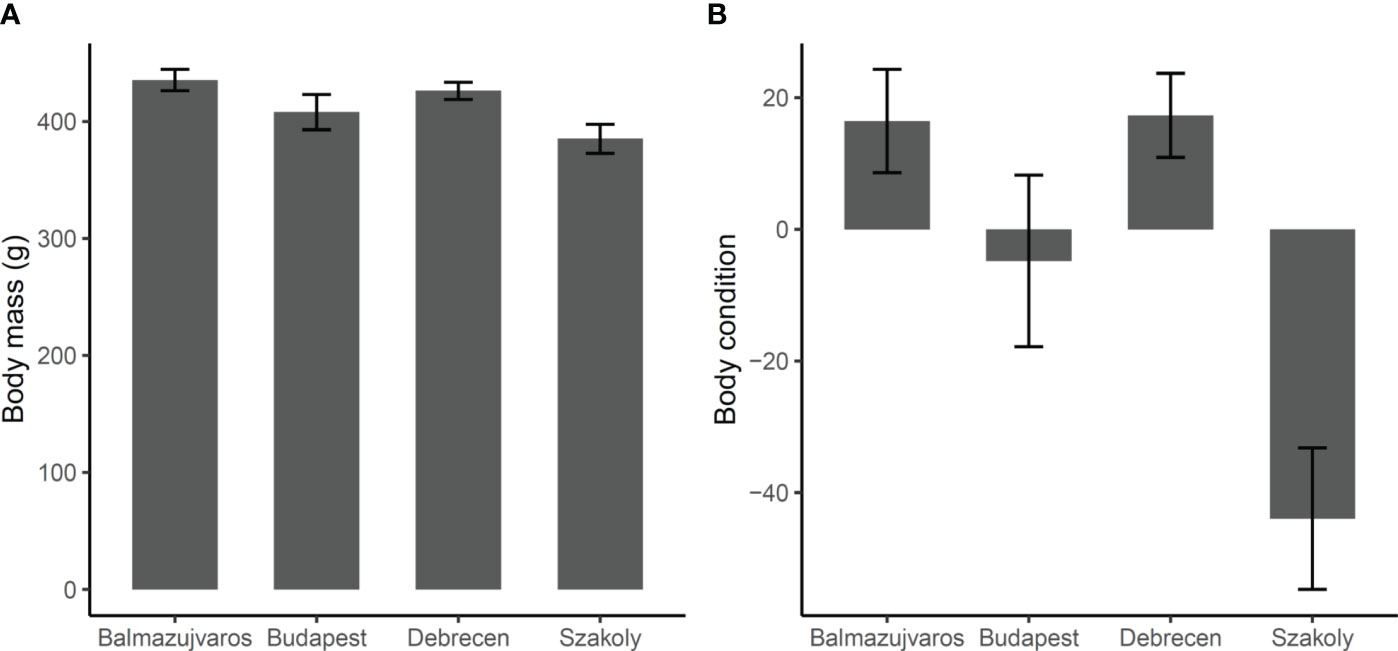
Figure 2 Mean ± S.E. values adjusted for seasonal differences of body mass (A) and body condition (B) of adult Hooded Crows in two rural (Balmazújváros, Szakoly) and two urban (Budapest, Debrecen) populations in Hungary. Statistics are in Table 1.
3.2 Morphological differences in juvenile body size between cities
In the comparison of juveniles from the large city (Budapest) to those from a medium-sized city (Debrecen), we found that crows in Budapest had better body condition and longer and thicker bills than crows in Debrecen (adjusted means ± S.E.s; body condition, Budapest: 46.9 ± 17.30 units, Debrecen: -4.1 ± 4.20 units, F152 = 6.891, padj = 0.037; bill length, Budapest: 52.9 ± 0.682 mm, Debrecen: 50.9 ± 0.29 mm, F154 = 22.247, padj = 0.0006; bill thickness, Budapest: 19.9 ± 0.48 mm, Debrecen: 18.1 ± 0.12 mm, F153 = 22.430, padj = 0.0006). The Julian week effect was significant for body mass (slope B = –6.32 ± 2.864, F154 = 8.574, p = 0.004), body condition (–4.74 ± 2.397, F152 = 9.640, p = 0.002), bill length (–0.001 ± 0.003, F = 9.278, p = 0.003) and bill thickness (–0.11 ± 0.067, F = 18.884, p < 0.0001). We also detected significant interactions between Julian week of capture and four body size variables, namely body mass (interaction coefficient estimate 9.60 ± 2.995, F154 = 10.283, p = 0.002), body condition (7.53± 2.504, F152 = 9.032, p = 0.003), skull width (0.14 ± 0.070, F153 = 4.005, p = 0.047) and bill thickness (0.21 ± 0.070, F153 = 9.346, p = 0.003) (Figure S1). The interactions suggested increasing trends in these four body size variables in Debrecen and decreasing trends in Budapest as the season progressed (Figure S1). However, juveniles were caught on average c. 8 weeks earlier in Debrecen than in Budapest, as we could not catch crows in Budapest Zoo, which was closed in the early part of the breeding season due to the COVID-19 pandemic (mean ± S.E. Julian week of capture, Debrecen: week 30.2 ± 0.467, Budapest: week 37.8 ± 0.953, t69 = 9.292, p < 0.0001).
3.3 Morphological differences within the city of Debrecen
Juvenile Hooded Crows differed between locations in body mass, fat reserve score, body condition, body length, tarsus length, skull width, and bill thickness (Figure 3; Table 1). Post-hoc tests showed several significant differences between SPC and ZOO, with higher values for ZOO than for SPC (body mass: contrast = 42.1 ± 10.8 g, t121 = 3.904, p = 0.0005; fat reserve score: 0.2 ± 0.07 units, t122 = 2.672, p = 0.023; body condition: 28.3 ± 10.0 units, t121 = 2.821, p = 0.015). In addition, tarsus length was higher in AGR than in SPC (contrast: 2.4 ± 0.99 mm, t119 = 2.466, p = 0.040) (Figure 3). The Julian week effect was significant for body mass (slope ± S.E.: 2.2 ± 0.90 g, F = 5.802, p = 0.018), body condition (2.0 ± 0.84 units, F = 5.933, p = 0.016), bill length (0.2 ± 0.06 mm, F = 10.527 p = 0.002) and bill thickness (0.005 ± 0.001, F = 3.999, p = 0.0001), whereas the week*location interaction was significant for tarsus length (F = 3.382, p = 0.037; Figure 3F). The latter interaction showed that tarsus length increased in the ZOO and SPC locations and decreased in the AGR location (Figure 3F).
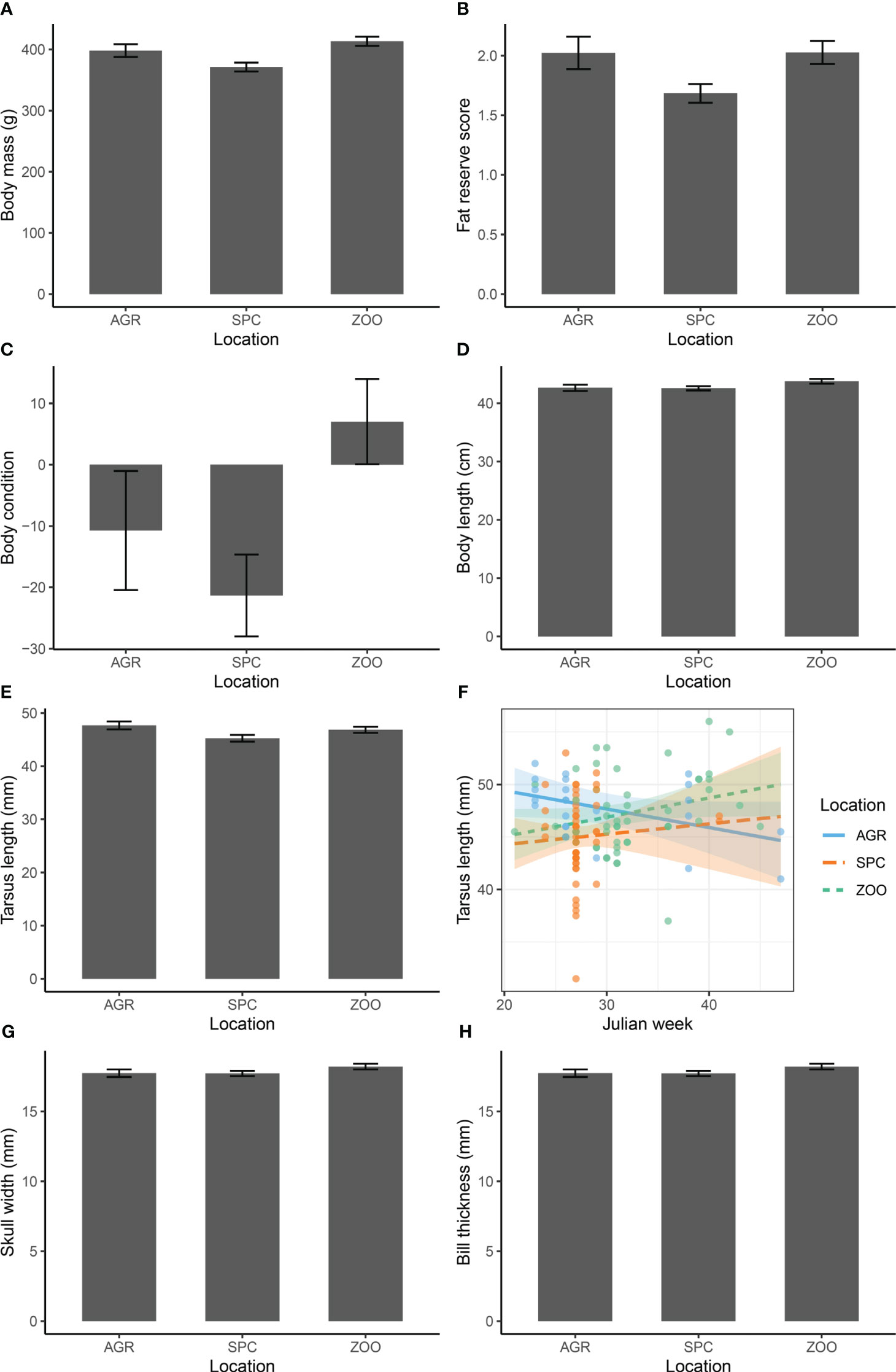
Figure 3 Mean ± S.E. values adjusted for seasonal differences of body size variables (A-E, G, H) of juvenile Hooded Crows captured in three locations within the city of Debrecen (locations are in Figure 1) and the interaction in tarsus length between season and locations (F).
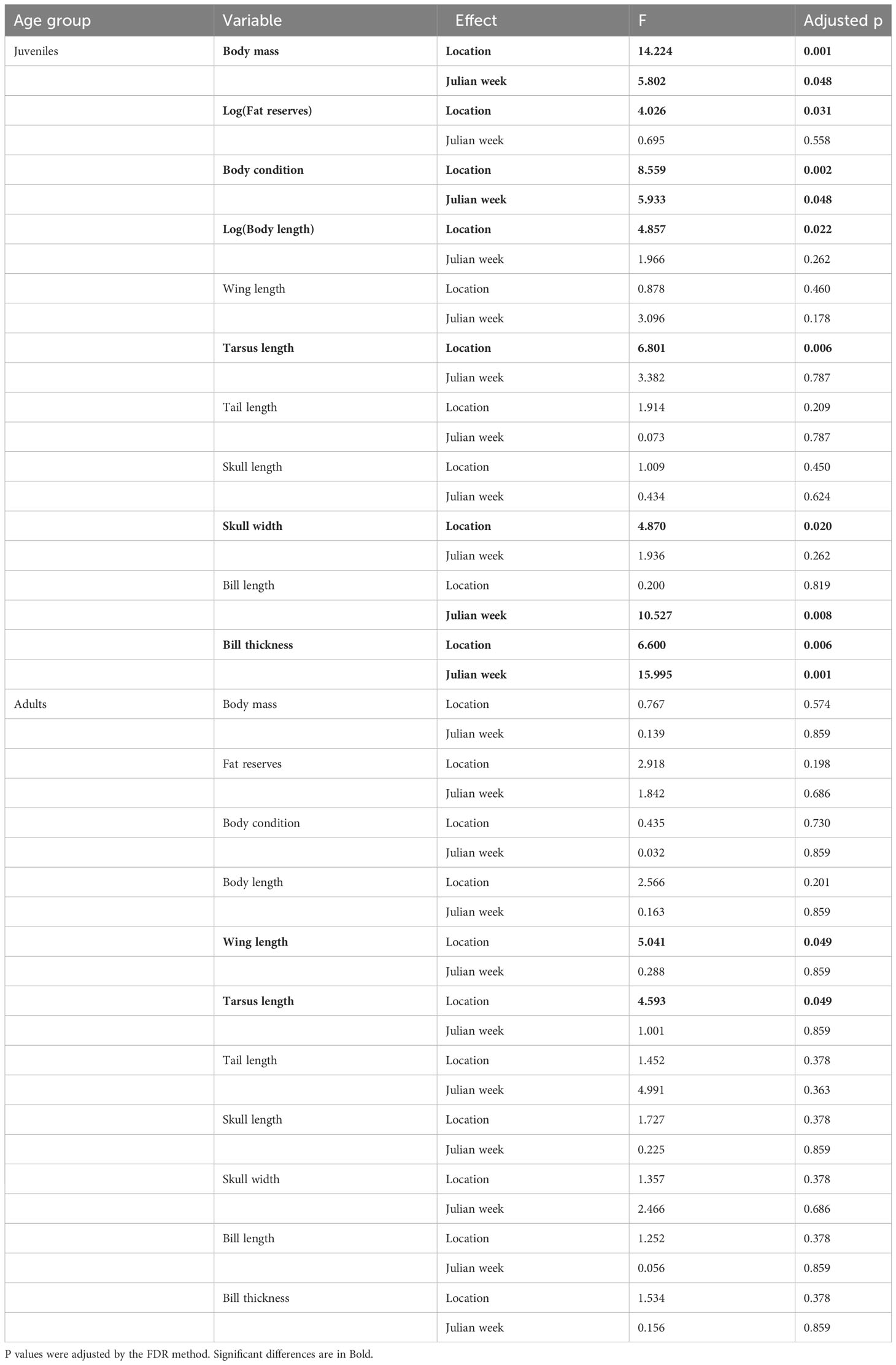
Table 1 Results of linear models testing the effects of location and season (Julian week) on body size variables of Hooded Crows captured in three locations (juveniles) or four locations (adults) in the city of Debrecen.
Adult Hooded Crows differed in wing length and tarsus length between locations in Debrecen, mainly because individuals caught in the ZOO had longer wings than those caught in OST (contrast ± S.E. 2.3 ± 0.68 cm, t30 = 0.011) and longer tarsi than those caught in AGR (6.2 ± 2.11, t30 = 2.939, p = 0.030) (Figure 4; Table 1).
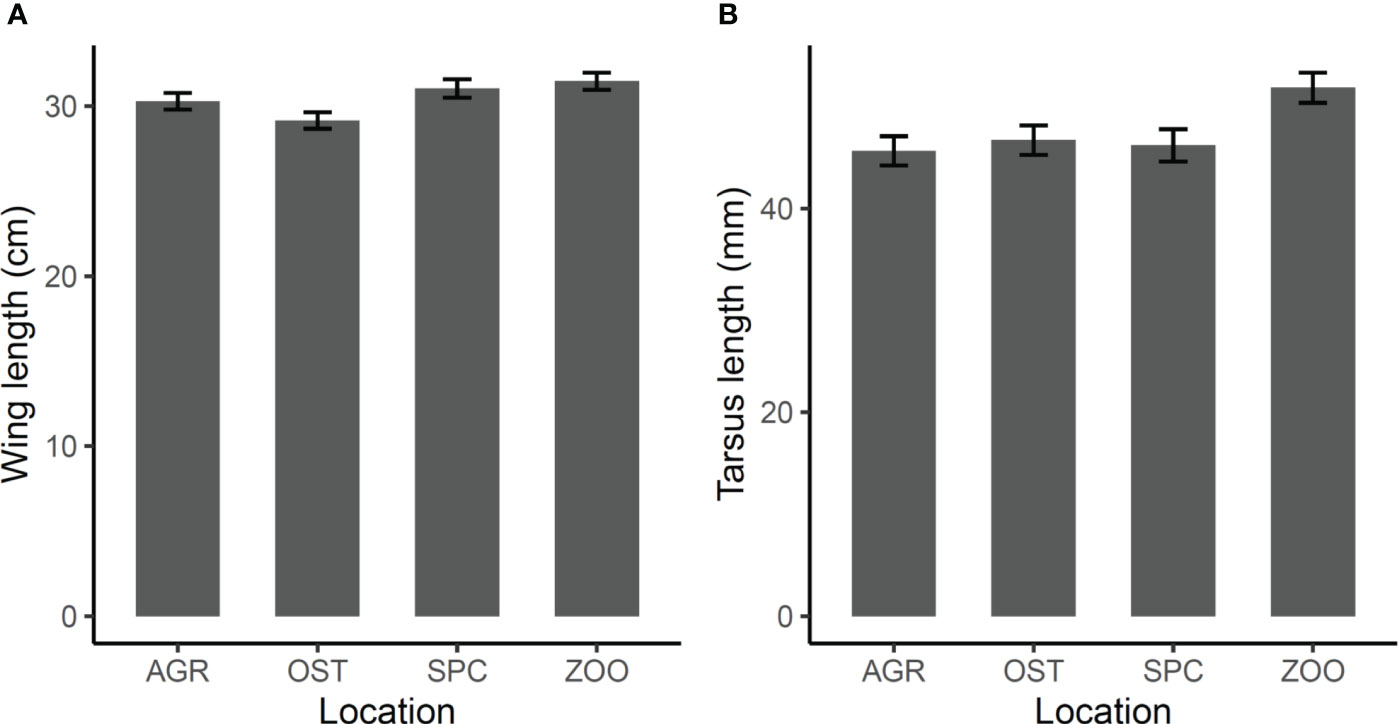
Figure 4 Mean ± S.E. values adjusted for seasonal differences of wing length (A) and tarsus length (B) of adult Hooded Crows captured in four locations within the city of Debrecen (locations are in Figure 1).
3.4 Temperature and artificial food abundance in study areas
Mean annual temperature between 2011 and 2021 differed significantly between the study areas (Figure S2, one-way ANOVA, F3,40 = 3.145, p = 0.040), mainly because Budapest, the largest city in Hungary, had a significantly higher mean annual temperature than Szakoly, the smallest of the studied settlements (contrast: 0.83 ± 0.30, padj = 0.042). When the two urban and two rural areas were pooled, the difference was also significant, with urban areas being significantly warmer than rural areas (Welch two-sample t-test, t40.708 = 2.792, p = 0.008).
Potential anthropogenic food sources were most abundant in 200-m-radius circular buffers around trapping locations in Budapest, followed by Debrecen, whereas they were completely missing from the two rural study areas in Balmazújváros and Szakoly (Figure 1). The mean number of such food sources was 12.6 ± 11.95 in Budapest (n = 5), 3.6 ± 3.78 in Debrecen (n = 5), and 0 in the two rural areas. Although the difference between Budapest and Debrecen was not significant (Wilcoxon rank sum test, W = 19.5, p = 0.172), the mean number of food sources (area: 12.56 ha) was thus three times higher (1.0 units/ha) in Budapest than in Debrecen (0.3 units/ha).
In the city of Debrecen, nine anthropogenic food sources (food service establishments) were found at the Zoo, five at the SPC, and five near the AGR, however, all were outside of the 200-m buffer zone around the trap location.
4 Discussion
4.1 Morphological differences across spatial scales and age categories
Our study provided three key results. First, we found that adults differed in body mass and body condition between the study areas studied, mainly because individuals near Szakoly had lower body mass and condition than individuals caught near Balmazújváros or in Debrecen. These differences can be explained by three possible reasons. First, the trapping location near Balmazújváros was deep inside Hortobágy National Park, an extensive grassland area, which provided ample availability of natural food sources. In contrast, the trapping location near Szakoly was surrounded by arable lands and forests and with very little grassland, which provided few or poor opportunities for feeding. Second, individuals were caught and measured on average 3 weeks earlier in Szakoly (mean ± S.D. Julian week, 18 ± 4.1 weeks) than in Balmazújváros (21 ± 3.0) or Debrecen (21 ± 7.8); F2,88 = 3.134, p = 0.048), and it is also possible that body mass and condition were lower earlier than later in the breeding season. The start of breeding is normally associated with high levels of stress and energetic demands, resulting in lower body mass and condition since breeders tend to restrict their movements away from their nest and thus have less time available for feeding (Harding et al., 2011). Finally, it is also possible that Hooded Crows in Szakoly, a wooded area with more predators than in grasslands, minimised their fat reserves and body mass to increase their ability to escape from predators. Body mass is a highly plastic trait, and passerines readily accumulate fat reserves and can rapidly reduce it (Brommer et al., 2015) e.g. in response to possible predation pressure (MacLeod et al., 2006).
Second, we did not detect differences in juvenile body size between the two urban areas, probably because our data were from different parts of the post-fledging period. Despite the substantial overlap in Julian weeks of capture between Budapest and Debrecen, juvenile birds were caught on average 8 weeks later in Budapest than in Debrecen. If the body size of juveniles still grows after fledging, this temporal difference may explain why birds in Budapest had larger bills and better body condition at the time of capture. Similarly, we found no difference in adult body size between the two cities or between the two cities and the rural area near Balmazújváros. In addition to the abundance of natural food sources in the grasslands near Balmazújváros (see above), the absence of body mass differences between the Balmazújváros rural and the two urban areas could also be the result of a balanced trade-off between food predictability and prediction risk (Amiot et al., 2022). This is consistent with the low predictability of food sources in urban areas leading to reduced fat reserves (Cuthill et al., 2000; Shochat, 2004; Brodin, 2007), which would be counter-balanced by the low levels of predation pressures and/or the sedentary way of life observed in urban environments.
Perhaps most interestingly, we found differences in body size between trapping locations within the city of Debrecen. Juvenile Hooded Crows captured in the Zoo location were generally larger, i.e., had higher body mass and better body condition, had more fat reserves, were lengthier, and had wider skulls and bills than juveniles caught elsewhere, especially in the SPC. Even more interesting is that the Zoo and the SPC are close (< 500 m) to each other (Figure 1, Figure S3). Because juvenile crows were captured on average 4 weeks earlier in the SPC location (28 ± 2.9 weeks) than the Zoo location (32 ± 5.2), F = 0.313, p < 0.001), differences in capture time might partly explain some morhpological differences observed between these birds, since it is possible that body size variables were lower earlier than later in the season. Nonetheless, the Zoo has been the centre of Hooded Crow colonisation in Debrecen (Kövér et al., 2015), which implies that it may provide better conditions for breeding and/or survival than other areas. The primary attractant of Hooded Crows to the Zoo is likely the year-round high availability of easily accessible food in open-top enclosures of Zoo animals, food storage areas and garbage cans near enclosures and open-air restaurants. Each of these are regularly visited by crows several times a day (Kövér et al., 2019). Natural (grassland) food sources are limited as most of the Zoo area is forested (Figure S3). In contrast, artificial, regularly mowed lawns of sport fields in the SPC (Figure S3) represent natural food sources and also attract crows, but likely provide less energy-rich food compared to the Zoo.
Some of the differences found in juveniles, namely, in tarsus length, could also be detected in adults, in that the Zoo birds had longer tarsi and wings than birds from other locations within the city. This result confirms that the Zoo probably has better or more sources for feeding and/or that larger, more dominant birds occupy the Zoo area rich in food sources. Because locomotion-related traits such as tarsus and wing length usually have lower values for urban than rural birds because urban birds move less (Liker et al., 2008; Bókony et al., 2012; Meillère et al., 2015; Dulisz et al., 2016; Caizergues et al., 2018), birds with longer tarsi and wings in the Zoo are probably more dominant or of higher individual quality than birds from other parts of the city. Such findings can be explained by the founder effect, which implies that the observed differences are mainly due to the morphological traits of the birds that were the first to colonise the area (Evans et al., 2009). These results also support the view that local conditions within a city may also modify patterns in body size along the rural-urban gradient (Evans et al., 2009; Seress and Liker, 2015; Sepp et al., 2018), likely due to the local ecological variation involved (Teplitsky et al., 2008; Husby et al., 2011; Brommer et al., 2014).
Earlier studies of the morphology of adult birds have reported inconsistent responses to urbanization, ranging from negative effects (Giraudeau et al., 2014) through no effects (Bókony et al., 2012; Grunst et al., 2014; Meillère et al., 2017; Amiot et al., 2022) to positive effects (Meillère et al., 2015). A recent comprehensive study of six animal groups found that birds in general have smaller body sizes in more urbanised areas than in less urbanised areas (Hahs et al., 2023). In addition, feeding strategy and body size were more important traits in explaining success in urban environments than reproductive traits, and omnivory was very frequent in the most urbanised areas in birds (Hahs et al., 2023). Our results obtained in the highly omnivorous Hooded Crows suggest that of the local conditions, the availability of anthropogenic food sources emerges in importance. Anthropogenic food sources often show an aggregated pattern (e.g. Figure 1), which can lead to the emergence of urban areas with stable and reliable food sources, which may be worth defending in territories (e.g. by larger adults in the breeding season, as suggested in this study) or may be worth visiting in groups outside the breeding season (e.g. by flocks of up to 300 birds in the Zoo, as is regularly observed in the winter). These “islands” of stable food sources can provide better conditions for chick-rearing and allow better conditions for development, resulting in larger fledglings. As such, these islands can be worth defending by larger, more dominant adults. These mechanisms could explain the local-scale patterns observed in our study, i.e., larger juveniles and adults in the Zoo area than in other locations within the city.
However, greater food availability in cities does not necessarily result in a larger body size or better body condition because most reports have indicated that birds in cities are often smaller and/or in worse body condition than their rural conspecifics (Evans et al., 2009; Meillère et al., 2015; Hahs et al., 2023), while others indicated no morphological differences along the urban-rural gradient (Bókony et al., 2012; Meillère et al., 2017; Amiot et al., 2022). It is plausible that not only the quantity, but also the quality of anthropogenic food influences the body size and condition of the birds. Compared to natural food, anthropogenic food is often low in proteins and vitamins and rich in fats, carbohydrates, and salt (García-Arroyo et al., 2023), which may lead to lower breeding success (Chamberlain et al., 2009; Meyrier et al., 2017; Caizergues et al., 2018) and slower young development (Peach et al., 2008; Seress et al., 2012) in birds. In addition, the lack of certain nutrients can impose direct costs on bird development. For example, vitamin B12 is central to carbohydrate and fat metabolism and affects weight gain, food intake, and feather development (Van Grouw, 2018). Poor developmental conditions are often responsible for shorter structural body sizes in birds (Peach et al., 2008; Seress et al., 2012). Our results on shorter tarsi and structural size in juvenile Hooded Crows from different trapping locations in Debrecen agree with this mechanism and support the idea that the local environment may slow down or limit bird development. In the closely related Carrion Crow (Corvus corone), urban birds produced smaller fledglings, and these differences could be linked experimentally to food availability (Richner et al., 1989). Alternatively, smaller body sizes can also reflect ongoing adaptations to novel environments at several levels (Liker, 2020). For example, birds can change their food preferences and feeding strategies (Shochat, 2004), but they can also exhibit morphological changes (Badyaev et al., 2008; Bosse et al., 2017). The quantity and quality of anthropogenic food available may therefore be a driving factor in wild bird adaptation to novel environments. The adaptation process is expected to accelerate if there is no gene flow between urban and rural populations. Some earlier studies have detected gene flow between urban and rural populations (Björklund et al., 2010; Carlen and Munshi-South, 2021), whereas others have not (Zhang et al., 2013; Tang et al., 2016; Markowski et al., 2021). We have no molecular data to evaluate this possibility. However, gene flow cannot be ruled out in Hooded Crows, where the winter density of crows increases greatly in cities (Nagy, 2021), suggesting an influx of individuals from nearby rural areas.
Because temperature is not likely to vary much at the local, within-city scale (few hundred meters to few kilometers), our results do not provide support for Bergmann’s rule. Our findings are in line with previous studies that reported lower conformity to Bergmann’s rule in passerines than in non-passerines (Meiri and Dayan, 2003). Conformity to Bergmann’s rule can be difficult to detect due to various biological and ecological factors (He et al., 2023). For instance, individuals may adopt strategies other than increasing body size to cope with colder environments. Additionally, variation in body size may be mediated by the individuals’ unique environment and biogeographic history (Mainwaring and Street, 2021). While global warming is generally expected to lead to smaller body sizes, it may not be universal (Tian and Benton, 2020) because individuals can change their activity or behaviour (Beever et al., 2017) or their geographic ranges (Rushing et al., 2020) to adapt to the changing climate. These results call for more extensive, wide-scale studies when one tries to analyse possible morphological changes in relation to Bergmann’s rule or to global warming.
Furthermore, we found no differences in wing length between individuals from urban vs. rural areas, or between cities in either age group. Thus, we found no evidence supporting either Allen’s rule (longer wings in colder environments) or Seebohm’s rule (shorter wings in more sedentary lifestyle). However, it is also possible that our findings may support both rules at the same time. For instance, because Hooded Crows are year-long residents in urban areas, they may display shorter wings, as per Seebohm’s rule. However, the warmer climate in urban areas can induce longer wings in these birds, as per Allen’s rule. Thus, the combined result of both mechanisms, i.e., no variation in wing length between crows from different areas, operating at the same time cannot be distinguished from the absence of both mechanisms.
Additional explanations are also possible for the lack of large-scale urban-rural differences in the morphology of Hooded Crows. Based on their omnivorous feeding strategy, Hooded Crows are more likely to show variation in body mass and body condition due to their consumption of a wide variety of foods, which explains the large variation in measurements of body mass (min – max values for adults in this study: 300 – 530 g) and possibly of other variables. It is also possible that the effect of anthropogenic food sources availability on crow morphological traits is likely to differ with development stages as well as with the availability and relative quality of natural food. Finally, the en masse appearance of Hooded Crows in cities in Hungary has started relatively recently (1980s and 1990s), and it is possible that the few decades since then have been too short for morphological variation to arise.
We also note that we found more differences in morphological traits of juveniles and fewer differences for adults. Adult body size thus appears to be more stable than juvenile body size, which probably varies because juveniles still grow after they leave the nest. This was partially supported by positive relationships between Julian week and several juvenile body size variables (Table 1). These results also suggest that urban crows may be subject to site-specific ecological pressures during their development, and that such constraints seem to disappear during adulthood, as birds start to move around.
Finally, we found that urban environments were warmer and had a higher availability of anthropogenic food sources than rural ones. Urban environments are generally warmer than rural areas (Heiss et al., 2009; Baltensperger et al., 2013; Krausman et al., 2014), mostly due to built-up areas, which effectively absorb heat (Terjung and Louie, 1973; Oke and McCaughey, 1983; EU Science Hub, 2022). Higher temperatures have previously been associated with variability in animal morphology (Brommer et al., 2015; Caizergues et al., 2018; Mainwaring and Street, 2021) and a series of trade-offs affecting feeding, reproduction, nest site selection, offspring provisioning, and migration (Van de Ven et al., 2019). Our results, however, did not agree with these observations or with Bergmann’s rule suggesting that individuals living in warmer conditions would have a smaller body size than their conspecifics living in colder conditions.
Taken together, our results suggest no universal differences in body size of Hooded Crows between urban and rural areas. However, our observations of local differences in juvenile and adult body size at least partly indicated that the variation in body size is probably more site-dependent than gradient-dependent. Such dependence was previously reported in the European Blackbird (Turdus merula; Evans et al., 2009). While there may be substantial morphological variation between adjacent urban (Zoo vs. other trapping locations) and rural crow populations (e.g. Szakoly vs. other areas), such differentiation may not operate across the full range of the species. Thus, site-specific, locally condition-dependent variation in body size between Hooded Crow populations may appear if the selective pressure acting on crow morphology varies inconsistently between study sites.
4.2 Study limitations
First, we collected data for only one year, thus, we cannot evaluate the robustness of our findings for longer time periods. We restricted data collection to one year only to minimise the variance in morphological traits attributable to between-year differences in weather, food availability, and other possible factors. Second, we had data from only three rural juveniles, which hindered us from conducting an urban-rural comparison of juveniles. While we made every attempt to increase the sample size of juvenile crows living in rural areas, Hooded Crows are highly intelligent and were often too difficult to catch with the traps used (Kövér et al., 2018). While adults in both urban and rural areas can be caught near their active nests, they can hardly be caught outside the nesting period as they are wary of traps. Third, we measured anthropogenic food availability in our study areas rather than the use of anthropogenic food by crows. Although direct monitoring of the use food would have been highly informative, this was not possible as our data were collected as part of ongoing crow management programmes. Nonetheless, recent studies have provided evidence on the use of anthropogenic food by crows (Cereghetti et al., 2019; García-Arroyo et al., 2023) and that anthropogenic food is important for urban birds (Tryjanowski et al., 2015; Preininger et al., 2019). Finally, we did not collect data during the winter period, which is characterised by low food availability and is likely to be a bottleneck in crow survival. Beginning in late November, Hooded Crows from Debrecen usually aggregate and move together in one or a few big flocks, which prevented us from allocating crows to trapping locations within the city. We thus ended the trapping effort in early November.
4.3 Conclusions
In conclusion, the comparison of the morphological traits of birds from the four study areas (two rural and two urban areas) showed that urban adult crows did not differ from their rural conspecifics. Nonetheless, birds from Szakoly (one of the rural areas) were smaller than birds from Bálmazújváros (the second rural area) and the city of Debrecen. We did not detect any differences in the morphology of crows, neither in adults nor in juveniles, between the two different-sized cities. Finally, the local-scale comparison of crows from several locations within the city of Debrecen showed that juveniles had significantly larger body sizes and adults had longer wings and tarsi in the Zoo area than in other locations. Taken together, these findings suggest that divergences in morphological traits are more likely site-specific than gradient-specific and have likely resulted from rapid local responses to spatially differing factors. Further studies involving multiple scales and sites and factors other than food availability and microclimate will be fruitful to explore inter-site variability in morphology and to better understand the rapid responses of wild birds’ phenotypic traits to urbanization.
Data availability statement
The raw data supporting the conclusions of this article will be made available by the authors, without undue reservation.
Ethics statement
Ethical approval was not required for the study involving animals in accordance with the local legislation and institutional requirements because this study presents non-experimental research on wild birds, and it was carried out in accordance with institutional guidelines. Additionally, birds were captured for the purpose of wildlife management in accordance with local and national regulations. These birds were measured in the least invasive manner, and did not suffer unnecessarily.
Author contributions
IB and LK contributed to conception and design of the study. IB, PP and LK organized the database. IB and SL performed the statistical analysis. IB wrote the first draft of the manuscript. IB, LK, JJ, M-LK-J and SL wrote sections of the manuscript. JJ, LJ, GK, LK and SL supervised the study. All authors contributed to the article and approved the submitted version.
Funding
This research was funded by the Tempus Public Foundation - Stipendium Hungaricum Program, grant number: SH-00355-004/2019 and by a grant from the National Research, Development and Innovation Office of Hungary (NKFIH-OTKA K 134931). Project no. TKP2021-NKTA-32 has been implemented with the support provided from the National Research, Development and Innovation Fund of Hungary, financed under the TKP2021-NKTA funding scheme.
Acknowledgments
We thank the staff of the local wildlife management units in Szakoly and Balmazújváros for their assistance in the field. We are also grateful to Mr. Gergely Sándor Nagy and Mr. Miklós Persányi, the directors of Debrecen and Budapest Zoo for their help. We thank for the cooperation to Mrs. Orsolya Hamecz, the head of the Department of Green Area Management of Debrecen; to Mr. László Papp, the director of the Botanical Garden of the University of Debrecen and to all of the gardeners at the Agricultural Campus at the University of Debrecen.
Conflict of interest
The authors declare that the research was conducted in the absence of any commercial or financial relationships that could be construed as a potential conflict of interest.
Publisher’s note
All claims expressed in this article are solely those of the authors and do not necessarily represent those of their affiliated organizations, or those of the publisher, the editors and the reviewers. Any product that may be evaluated in this article, or claim that may be made by its manufacturer, is not guaranteed or endorsed by the publisher.
Supplementary material
The Supplementary Material for this article can be found online at: https://www.frontiersin.org/articles/10.3389/fevo.2023.1196075/full#supplementary-material
References
Amiot C., Harmange C., Ji W. (2022). Morphological differences along a chronological gradient of urbanisation in an endemic insectivorous bird of New Zealand. Urban. Ecosyst. 25 (2), 465–475. doi: 10.1007/s11252-021-01156-w
Badyaev A. V., Young R. L., Oh K. P., Addison C. (2008). Evolution on a local scale: developmental, functional, and genetic bases of divergence in bill form and associated changes in song structure between adjacent habitats. Evol.: Int. J. Org. Evol. 62 (8), 1951–1964. doi: 10.1111/j.1558-5646.2008.00428.x
Baldwin J. W., Garcia-Porta J., Botero C. A. (2023). Complementarity in Allen’s and Bergmann’s rules among birds. Nature Communications 14 (1), 4240. doi: 10.1038/s41467-023-39954-9
Baltensperger A. P., Mullet T. C., Schmid M. S., Humphries G. R. W., Kövér L., Huettmann F. (2013). Seasonal observations and machine-learning-based spatial model predictions for the common raven (Corvus corax) in the urban, sub-arctic environment of Fairbanks, Alaska. Polar. Biol. 36 (11), 1587–1599. doi: 10.1007/s00300-013-1376-7
Beever E. A., Hall L. E., Varner J., Loosen A. E., Dunham J. B., Gahl M. K., et al. (2017). Behavioral flexibility as a mechanism for coping with climate change. Front. Ecol. Environ. 15 (6), 299–308. doi: 10.1002/fee.1502
Benjamini Y., Hochberg Y. (1995). Controlling the false discovery rate: a practical and powerful approach to multiple testing. J. R. Stat. Soc.: Ser. B. (Methodological). 57 (1), 289–300. doi: 10.1111/j.2517-6161.1995.tb02031.x
Benmazouz I., Jokimäki J., Lengyel S., Juhász L., Kaisanlahti-Jokimäki M. L., Kardos G., et al. (2021). Corvids in urban environments: A systematic global literature review. Animals 11 (11), 3226. doi: 10.3390/ani11113226
Björklund M., Ruiz I., Senar J. C. (2010). Genetic differentiation in the urban habitat: the great tits (Parus major) of the parks of Barcelona city. Biol. J. Linn. Soc. 99 (1), 9–19. doi: 10.1111/j.1095-8312.2009.01335.x
Bókony V., Seress G., Nagy S., Lendvai Á.Z., Liker A. (2012). Multiple indices of body condition reveal no negative effect of urbanization in adult house sparrows. Landscape Urban. Plann. 104 (1), 75–84. doi: 10.1016/j.landurbplan.2011.10.006
Bonier F. (2012). Hormones in the city: Endocrine ecology of urban birds. Hormones. Behav. 61 (5), 763–772. doi: 10.1016/j.yhbeh.2012.03.016
Bonnet-Lebrun A. S., Manica A., Rodrigues A. S. (2020). Effects of urbanization on bird migration. Biol. Conserv. 244, 108423. doi: 10.1128/AAC.48.1.1-14.2004
Bosse M., Spurgin L. G., Laine V. N., Cole E. F., Firth J. A., Gienapp P., et al. (2017). Recent natural selection causes adaptive evolution of an avian polygenic trait. Science 358 (6361), 365–368. doi: 10.1126/science.aal3298
Brodin A. (2007). Theoretical models of adaptive energy management in small wintering birds. Philos. Trans. R. Soc. B.: Biol. Sci. 362 (1486), 1857–1871. doi: 10.1098/rstb.2006.1812
Brommer J. E., Hanski I. K., Kekkonen J., Väisänen R. A. (2014). Size differentiation in F innish house sparrows follows B ergmann’s rule with evidence of local adaptation. J. Evol. Biol. 27 (4), 737–747. doi: 10.1111/jeb.12342
Brommer J. E., Hanski I. K., Kekkonen J., Väisänen R. A. (2015). Bergmann on the move: a temporal change in the latitudinal gradient in body mass of a wild passerine. J. Ornithol. 156 (4), 1105–1112. doi: 10.1007/s10336-015-1211-8
Caizergues A. E., Grégoire A., Charmantier A. (2018). Urban versus forest ecotypes are not explained by divergent reproductive selection. Proc. R. Soc. B.: Biol. Sci. 285 (1882), 20180261. doi: 10.1098/rspb.2018.0261
Carlen E., Munshi-South J. (2021). Widespread genetic connectivity of feral pigeons across the Northeastern megacity. Evol. Appl. 14 (1), 150–162. doi: 10.1111/eva.12972
Cereghetti E., Scherler P., Fattebert J., Grüebler M. U. (2019). Quantification of anthropogenic food subsidies to an avian facultative scavenger in urban and rural habitats. Landscape Urban. Plann. 190, 103606. doi: 10.1016/j.landurbplan.2019.103606
Chace J. F., Walsh J. J. (2006). Urban effects on native avifauna: A review. Landscape Urban. Plann. 74 (1), 46–69. doi: 10.1016/j.landurbplan.2004.08.007
Chamberlain D. E., Cannon A. R., Toms M. P., Leech D. I., Hatchwell B. J., Gaston K. J. (2009). Avian productivity in urban landscapes: a review and meta-analysis. Ibis 151 (1), 1–18. doi: 10.1111/j.1474-919X.2008.00899.x
Clergeau P., Croci S., Jokimäki J., Kaisanlahti-Jokimäki M. L., Dinetti M. (2006). Avifauna homogenisation by urbanisation: analysis at different European latitudes. Biol. Conserv. 127 (3), 336–344. doi: 10.1016/j.biocon.2005.06.035
Cornell Lab of Ornithology (2015) All about birds: american crows. Available at: https://www.allaboutbirds.org/guide/american_crow/id.
Cuthill I. C., Maddocks S. A., Weall C. V., Jones E. K. (2000). Body mass regulation in response to changes in feeding predictability and overnight energy expenditure. Behav. Ecol. 11 (2), 189–195. doi: 10.1093/beheco/11.2.189
Dulisz B., Nowakowski J. J., Górnik J. (2016). Differences in biometry and body condition of the House Sparrow (Passer domesticus) in urban and rural population during breeding season. Urban Ecosystems. 19 (3), 1307–1324. doi: 10.1007/s11252-016-0546-0
Dulisz B., Dynowska M., Nowakowski J. J. (2021). Body condition and colonization by fungi of House Sparrows Passer domesticus in the urban and rural environment. Eur. Zool. J. 88 (1), 152–164. doi: 10.1080/24750263.2020.1857447
Eötvös C. B., Magura T., Lövei G. L. (2019). A meta-analysis indicates reduced predation pressure with increasing urbanization. Landscape Urban. Plann. 180, 54–59. doi: 10.1016/j.landurbplan.2018.08.010
EU Science Hub (2022) Cities are often 10-15°C hotter than their rural surroundings. Available at: https://joint-research-centre.ec.europa.eu/jrc-news/cities-are-often-10-15-degc-hotter-their-rural-surroundings-2022-07-25_en.
Evans K. L., Gaston K. J., Sharp S. P., McGowan A., Hatchwell B. J. (2009). The effect of urbanisation on avian morphology and latitudinal gradients in body size. Oikos 118 (2), 251–259. doi: 10.1111/j.1474-919X.2008.00898.x
Evans B. S., Reitsma R., Hurlbert A. H., Marra P. P. (2018). Environmental filtering of avian communities along a rural-to-urban gradient in Greater Washington, D.C., USA. Ecosphere 9, e02402. doi: 10.1002/ecs2.2402
Fidino M., Gallo T., Lehrer E. W., Murray M. H., Kay C. A. M., Sander H. A., et al. (2021). Landscape-scale differences among cities alter common species’ responses to urbanization. Ecol. Appl. 31 (2), e02253. doi: 10.1002/eap.2253
Fitzpatrick S. (1998). Intraspecific variation in wing length and male plumage coloration with migratory behaviour in continental and island populations. J. Avian Biol. 29 (3), 248–256. doi: 10.2307/3677107
García-Arroyo M., Gómez-Martínez M. A., MacGregor-Fors I. (2023). Litter buffet: On the use of trash bins by birds in six boreal urban settlements. Avian Res. 14, 100094. doi: 10.1016/j.avrs.2023.100094
Gil D., Brumm H. (2014). Acoustic communication in the urban environment: patterns, mechanisms, and potential consequences of avian song adjustments. Avian Urban. Ecol., 69–83. doi: 10.1093/acprof:osobl/9780199661572.001.0001
Giraudeau M., Mousel M., Earl S., McGraw K. (2014). Parasites in the city: Degree of urbanization predicts poxvirus and coccidian infections in house finches (Haemorhous mexicanus). PloS One 9 (2), e86747. doi: 10.1371/journal.pone.0086747
Gregory N. G., Robins J. K. (1998). A body condition scoring system for layer hens. New Z. J. Agric. Res. 41 (4), 555–559. doi: 10.1080/00288233.1998.9513338
Grunst M. L., Rotenberry J. T., Grunst A. S. (2014). Variation in adrenocortical stress physiology and condition metrics within a heterogeneous urban environment in the song sparrow Melospiza melodia. J. Avian Biol. 45 (6), 574–583. doi: 10.1111/jav.00459
Hahs A. K., Fournier B., Aronson M. F., Nilon C. H., Herrera-Montes A., Salisbury A. B., et al. (2023). Urbanisation generates multiple trait syndromes for terrestrial animal taxa worldwide. Nat. Commun. 14 (1), 4751. doi: 10.1038/s41467-023-39746-1
Harding A. M. A., Welcker J., Steen H., Hamer K. C., Kitaysky A. S., Fort J., et al. (2011). Adverse foraging conditions may impact body mass and survival of a high Arctic seabird. Oecologia 167, 49–59. doi: 10.1007/s00442-011-1971-7
He J., Tu J., Yu J., Jiang H. (2023). A global assessment of Bergmann’s rule in mammals and birds. Global Change Biol. 29 (18), 5199–5210. doi: 10.1111/gcb.16860
Heiss R. S., Clark A. B., McGowan K. J. (2009). Growth and nutritional state of American crow nestlings vary between urban and rural habitats. Ecol. Appl. 19 (4), 829–839. doi: 10.1890/08-0140.1
Hostetler M. (2001). “The importance of multi-scale analyses in avian habitat selection studies in urban environments,” in Avian ecology and conservation in an urbanizing world. Eds. Marzluff J. M., Bowman R., Donnelly R. (Boston: Kluwer Academic Publishers), 139–154. doi: 10.1007/978-1-4615-1531-9_7
Hungarian Central Statistical office (2022a) Debrecen. Available at: https://www.ksh.hu/apps/hntr.telepules?p_lang=EN&p_id=15130.
Hungarian Central Statistical Office (2022b) Budapest. Available at: https://www.ksh.hu/apps/hntr.telepules?p_lang=EN&p_id=13578.
Hungarian Central Statistical office (2022c) Szakoly. Available at: https://www.ksh.hu/apps/hntr.telepules?p_lang=EN&p_id=04774.
Hungarian Central Statistical Office (2022d) Balmazújváros. Available at: https://www.ksh.hu/apps/hntr.telepules?p_lang=EN&p_id=02918.
Husby A., Hille S. M., Visser M. E. (2011). Testing mechanisms of Bergmann’s rule: phenotypic decline but no genetic change in body size in three passerine bird populations. Am. Nat. 178 (2), 202–213. doi: 10.1086/660834
Jackson H. B., Fahrig L. (2015). Are ecologists conducting research at the optimal scale? Global Ecol. Biogeogr. 24 (1), 52–63. doi: 10.1111/geb.12233
Järvinen O., Väisänen R. A., Ranta E. (1988). Eläimistö. Suomen. Kartasto/Atlas. Over. Finland. Fol. 142, 11–18.
Jokimäki J., Kaisanlahti-Jokimäki M. L., Suhonen J. (2022). Long-term winter population trends of corvids in relation to urbanization and climate at northern latitudes. Animals 12 (14), 1820. doi: 10.3390/ani12141820
Jokimäki J., Suhonen J., Kaisanlahti-Jokimäki M. L. (2021). Differential long-term population responses of two closely related human-associated sparrow species with respect to urbanization. Birds 2 (3), 230–249. doi: 10.3390/birds2030017
Juhász L. (1983). Faunistic and synecological survey of Debrecen’s ornithofauna (Doxtiram dissertation, University of Debrecen).
Kaiser A., Merckx T., Van Dyck H. (2016). The Urban Heat Island and its spatial scale dependent impact on survival and development in butterflies of different thermal sensitivity. Ecology and Evolution. 6 (12), 4129–4140. doi: 10.1002/ece3.2166
Keller V. (2020). “Carrion Crow,” in European breeding bird atlas 2: distribution, abundance and change. Eds. Keller V., Herrando S., Voríšek P., Franch M., Kipson M., Milanesi P., Martí D., Anton M., Klvanová A., Kalyakin M. V. (Barcelona, Spain: Lynx Edicions/European Bird Census Council (EBCC), 562–563.
Kövér L., Gyüre P., Balogh P., Huettmann F., Lengyel S., Juhász L. (2015). Recent colonization and nest site selection of the Hooded Crow (Corvus corone cornix L.) in an urban environment. Landscape Urban. Plann. 133, 78–86. doi: 10.1016/j.landurbplan.2014.09.008
Kövér L., Lengyel S., Takenaka M., Kirchmeir A., Uhl F., Miller R., et al. (2019). Why do Zoos attract crows? A comparative study from Europe and Asia. Ecol. Evol. 9 (24), 14465–14475. doi: 10.1002/ece3.5881
Kövér L., Paládi P., Benmazouz I., Šorgo A., Špur N., Juhász L., et al. (2022). Is the Hitchcock story really true? Public opinion on hooded crows in cities as input to management. Animals 12, 1207. doi: 10.3390/ani12091207
Kövér L., Tóth N., Lengyel S., Juhász L. (2018). Corvid control in urban environments: a comparison of trap types. North-Western. J. Zool. 14 (1), 85–90.
Krausman P. R., Christensen S. A., Mcdonald J. E., Leopold B. D. (2014). Dynamics and social issues of overpopulated deer ranges in the United States: a long term assessment. California. Fish. Game. 100 (3), 436–450.
Kulemeyer C., Asbahr K., Gunz P., Frahnert S., Bairlein F. (2009). Functional morphology and integration of corvid skulls–a 3D geometric morphometric approach. Front. Zool. 6 (1), 1–14. doi: 10.1186/1742-9994-6-2
Labocha M. K., Hayes J. P. (2012). Morphometric indices of body condition in birds: a review. J. Ornithol. 153 (1), 1–22. doi: 10.1007/s10336-011-0706-1
Lepczyk C. A., Aronson M. F., La Sorte F. A. (2023). Cities as sanctuaries. Front. Ecol. Environ. 21 (5), 251–259. doi: 10.1002/fee.2637
Liker A. (2020). Biologia Futura: adaptive changes in urban populations. Biol. Futura. 71 (1), 1–8. doi: 10.1007/s42977-020-00005-9
Liker A., Papp Z., Bókony V., Lendvai A. Z. (2008). Lean birds in the city: body size and condition of house sparrows along the urbanization gradient. J. Anim. Ecol. 77 (4), 789–795. doi: 10.1111/j.1365-2656.2008.01402.x
Lim H. C., Sodhi N. S. (2009). Space use and habitat selection of house crows in a tropical urban environment: A radio-tracking study. Raffles. Bull. Zool. 57 (2), 561–568.
Lövei G. L., Magura T. (2022). Body size and the urban heat island effect modulate the temperature–size relationship in ground beetles. J. Biogeogr. 49 (9), 1618–1628. doi: 10.1111/jbi.14458
Lowry H., Lill A., Wong B. B. (2013). Behavioural responses of wildlife to urban environments. Biol. Rev. 88 (3), 537–549. doi: 10.1111/brv.12012
MacLeod R., Barnett P., Clark J., Cresswell W. (2006). Mass-dependent predation risk as a mechanism for house sparrow declines? Biol. Lett. 2 (1), 43–46. doi: 10.1098/rsbl.2005.0421
Mainwaring M. C., Street S. E. (2021). Conformity to Bergmann’s rule in birds depends on nest design and migration. Ecol. Evol. 11 (19), 13118–13127. doi: 10.1002/ece3.8034
Markowski M., Minias P., Bańbura M., Glądalski M., Kaliński A., Skwarska J., et al. (2021). Genetic structure of urban and non?urban populations differs between two common parid species. Sci. Rep. 11 (1), 1–10. doi: 10.1038/s41598-021-89847-4
Marzluff J. M., Bowman R., Donnelly R. (2001). “A historical perspective on urban bird research: trends, terms, and approaches,” in Avian ecology in an urbanizing world. Eds. Marzluff J. M., Bowman R., Donnelly R. (Boston, Massachusetts: Kluwer Academic Publishers), 1–17.
Marzluff J. M., Neatherlin E. (2006). Corvid response to human settlements and campgrounds: causes, consequences, and challenges for conservation. Biol. Conserv. 130 (2), 301–314. doi: 10.1016/j.biocon.2005.12.026
McCaffrey R. E., Mannan R. W. (2012). How scale influences birds’ responses to habitat features in urban residential areas. Landscape and Urban Planning. 105 (3), 274–280. doi: 10.1016/j.landurbplan.2011.12.022
McCollin D., Hodgson J., Crockett R. (2015). Do British birds conform to Bergmann’s and Allen’s rules? An analysis of body size variation with latitude for four species. Bird. Study. 62 (3), 404–410. doi: 10.1080/00063657.2015.1061476
McDonald R. I., Mansur A. V., Ascensão F., Colbert M., Crossman K., Elmqvist T., et al. (2020). Research gaps in knowledge of the impact of urban growth on biodiversity. Nat. Sustainabil. 3 (1), 16–24. doi: 10.1038/s41893-019-0436-6
Meillère A., Brischoux F., Henry P. Y., Michaud B., Garcin R., Angelier F. (2017). Growing in a city: Consequences on body size and plumage quality in an urban dweller, the house sparrow (Passer domesticus). Landscape Urban. Plann. 160, 127–138. doi: 10.1016/j.landurbplan.2016.12.014
Meillère A., Brischoux F., Parenteau C., Angelier F. (2015). Influence of urbanization on body size, condition, and physiology in an urban exploiter: a multi-component approach. PloS One 10 (8), e0135685. doi: 10.1371/journal.pone.0135685
Meiri S., Dayan T. (2003). On the validity of Bergmann’s rule. J. Biogeogr. 30 (3), 331–351. doi: 10.1046/j.1365-2699.2003.00837.x
Melles S., Glenn S., Martin K. (2003). Urban bird diversity and landscape complexity: species–environment associations along a multiscale habitat gradient. Conserv. Ecol. 7 (1), 1–22. doi: 10.5751/ES-00478-070105
Merckx T., Souffreau C., Kaiser A., Baardsen L. F., Backeljau T., Bonte D., et al. (2018). Body-size shifts in aquatic and terrestrial urban communities. Nature 558 (7708), 113–116. doi: 10.1038/s41586-018-0140-0
Meyrier E., Jenni L., Bötsch Y., Strebel S., Erne B., Tablado Z. (2017). Happy to breed in the city? Urban food resources limit reproductive output in Western Jackdaws. Ecol. Evol. 7 (5), 1363–1374. doi: 10.1002/ece3.2733
Møller A. P. (1983). Habitat selection, flocking and feeding behaviour of hooded crows Corvus corone. Ornis. Fennica. 60 (4), 105–111.
Møller A. P. (2009). Successful city dwellers: a comparative study of the ecological characteristics of urban birds in the Western Palearctic. Oecologia 159 (4), 849–858. doi: 10.1007/s00442-008-1259-8
Møller A. P., Jokimäki J., Skorka P., Tryjanowski P. (2014). Loss of migration and urbanization in birds: a case study of the blackbird (Turdus merula). Oecologia 175 (3), 1019–1027. doi: 10.1007/s00442-014-2953-3
Morelli F., Benedetti Z., Ibáñez-Álamo J. D., Jokimäki J., Mänd R., Tryjanowski P., et al. (2016). Evidence of evolutionary homogenization of bird communities in urban environments across Europe. Global Ecol. Biogeogr. 25 (11), 1284–1293. doi: 10.1111/geb.12486
Nagy G. G. (2021). “Hooded crow (Corvus cornix linnaeus 1758),” in Bird atlas of Hungary. Eds. Szép T., Csörgő T., Halmos G., Lovászi P., Nagy K., Schmidt A. (Budapest: Ministry for Agriculture and BirdLife Hungary), 480–482, ISBN: ISBN: 978-615-5673-67-2.
Nakamura S. (1998). The territorial behaviour of the Carrion Crows Corvus corone corone in Japan. Japanese. J. Ornithol. 46 (4), 213–223. doi: 10.3838/jjo.46.213
Neate-Clegg M. H., Tonelli B. A., Youngflesh C., Wu J. X., Montgomery G. A., Şekercioğlu Ç.H., et al. (2023). Traits shaping urban tolerance in birds differ around the world. Curr. Biol. 33 (9), 1677–1688. doi: 10.1016/j.cub.2023.03.024
O’Donoghue P. D., Cross T. F., O’Halloran J. (1998). Bill colour as an ageing technique in the Hooded Crow Corvus corone cornix: A cautionary note. Ringing & Migration 19 (1), 1–4. doi: 10.1080/03078698.1998.9674153
Oke T. R., McCaughey J. H. (1983). Suburban-rural energy balance comparisons for Vancouver, BC: an extreme case? Boundary-Layer. Meteorol. 26 (4), 337–354. doi: 10.1007/BF00119532
Partecke J. (2014). “Mechanisms of phenotypic responses following colonization of urban areas: From plastic to genetic adaptation,” in Avian urban ecology: Behavioural and physiological adaptations. Eds. Partecke J., Gil D., Brumm H. (Oxford: Oxford University Press), 131–142.
Partecke J., Van’t Hof, T J., Gwinner E. (2005). Underlying physiological control of reproduction in urban and forest-dwelling European blackbirds Turdus merula. J. Avian Biol. 36 (4), 295–305. doi: 10.1111/j.0908-8857.2005.03344.x
Peach W. J., Vincent K. E., Fowler J. A., Grice P. V. (2008). Reproductive success of house sparrows along an urban gradient. Anim. Conserv. 11 (6), 493–503. doi: 10.1111/j.1469-1795.2008.00209.x
Pennington D. N., Blair R. B. (2011). Habitat selection of breeding riparian birds in an urban environment: untangling the relative importance of biophysical elements and spatial scale. Diversity Distributions. 17 (3), 506–518. doi: 10.1111/j.1472-4642.2011.00750.x
Preininger D., Schoas B., Kramer D., Boeckle M. (2019). Waste disposal sites as all-you-can eat buffets for carrion crows (Corvus corone). Animals 9 (5), 215. doi: 10.3390/ani9050215
Pulliainen E. (1963). On the history, ecology and ethology of the mallards (Anas platyrhynchos) overwintering in Finland. Ornis. Fenn. 40, 45–66.
QGIS Development Team (2022). QGIS geographic information system (Open Source Geospatial Foundation Project). Available at: http://qgis.osgeo.org.
R Core Team (2022). R: A language and environment for statistical computing (Vienna, Austria: R Foundation for Statistical Computing). Available at: https://www.R-project.org/.
Richner H., Schneiter P., Stirnimann H. (1989). Life-history consequences of growth rate depression: an experimental study on carrion crows (Corvus corone corone L.). Funct. Ecol. 3 (5), 617–624. doi: 10.2307/2389577
Roloff G. (2003). Blood chemistry, cytology, and body condition in adult northern goshawks (Accipiter gentilis). J. Raptor. Res. 37 (4), 299–306.
RStudio Team (2022). RStudio: integrated development for R (Boston, MA: RStudio, PBC). Available at: http://www.rstudio.com/.
Rushing C. S., Royle J. A., Ziolkowski D. J. Jr., Pardieck K. L. (2020). Migratory behavior and winter geography drive differential range shifts of eastern birds in response to recent climate change. Proc. Natl. Acad. Sci. United. States America 117 (23), 12897–12903. doi: 10.1073/pnas.2000299117
Sepp T., McGraw K. J., Kaasik A., Giraudeau M. (2018). A review of urban impacts on avian life-history evolution: Does city living lead to slower pace of life? Global Change Biol. 24 (4), 1452–1469. doi: 10.1111/gcb.13969
Seress G., Bókony V., Pipoly I., Szép T., Nagy K., Liker A. (2012). Urbanization, nestling growth and reproductive success in a moderately declining house sparrow population. J. Avian Biol. 43 (5), 403–414. doi: 10.1111/j.1600-048X.2012.05527.x
Seress G., Liker A. (2015). Habitat urbanization and its effects on birds. Acta Zool. Acad. Sci. Hungaricae. 61 (4), 373–408. doi: 10.17109/AZH.61.4.373.2015
Seress G., Sándor K., Evans K. L., Liker A. (2020). Food availability limits avian reproduction in the city: An experimental study on great tits Parus major. J. Anim. Ecol. 89 (7), 1570–1580. doi: 10.1111/1365-2656.13211
Shochat E. (2004). Credit or debit? Resource input changes population dynamics of city-slicker birds. Oikos 106 (3), 622–626. doi: 10.1111/j.0030-1299.2004.13159.x
Shochat E., Warren P. S., Faeth S. H., McIntyre N. E., Hope D. (2006). From patterns to emerging processes in mechanistic urban ecology. Trends Ecol. Evol. 21 (4), 186–191. doi: 10.1016/j.tree.2005.11.019
Sih A., Ferrari M. C., Harris D. J. (2011). Evolution and behavioural responses to human-induced rapid environmental change. Evol. Appl. 4 (2), 367–387. doi: 10.1111/j.1752-4571.2010.00166.x
Sol D., Lapiedra O., González-Lagos C. (2013). Behavioural adjustments for a life in the city? Anim. Behav. 85 (5), 1101–1112. doi: 10.1016/j.anbehav.2013.01.023
Spotswood E. N., Beller E. E., Grossinger R., Grenier J. L., Heller N. E., Aronson M. F. (2021). The biological deserts fallacy: cities in their landscapes contribute more than we think to regional biodiversity. Bioscience. 71 (2), 148–160. doi: 10.1093/biosci/biaa155
Suhonen J., Jokimäki J. (2019). Temporally stable species occupancy frequency distribution and abundance–occupancy relationship patterns in urban wintering bird assemblages. Front. Ecol. Evol. 7. doi: 10.3389/fevo.2019.00129
Szala K., Dylewski Ł., Tobolka M. (2020). Winter habitat selection of Corvids in an urban ecosystem. Urban. Ecosyst. 23 (3), 483–493. doi: 10.1007/s11252-020-00942-2
Tang G. S., Sadanandan K. R., Rheindt F. E. (2016). Population genetics of the olive-winged bulbul (Pycnonotus plumosus) in a tropical urban-fragmented landscape. Ecol. Evol. 6 (1), 78–90. doi: 10.1002/ece3.1832
Tapfer D. (1978). A dolmányos varjú (Corvus cornix) további és rendszeres fészkelése Budapest VIII. kerületében. Madártani. tájékoztató., 39–41.
Tapfer D. (1985). A dolmányos varjak (Corvus cornix) fészkelése Budapest belső kerületeiben. Madártani. tájékoztató., 55–56.
Teplitsky C., Mills J. A., Alho J. S., Yarrall J. W., Merilä J. (2008). Bergmann’s rule and climate change revisited: Disentangling environmental and genetic responses in a wild bird population. Proc. Natl. Acad. Sci. 105 (36), 13492–13496. doi: 10.1073/pnas.080099910
Terjung W. H., Louie S. S. (1973). Solar radiation and urban heat islands. Ann. Assoc. Am. Geographers. 63 (2), 181–207. doi: 10.1111/j.1467-8306.1973.tb00918.x
Tian L., Benton M. J. (2020). Predicting biotic responses to future climate warming with classic ecogeographic rules. Curr. Biol. 30 (13), R744–R749. doi: 10.1016/j.cub.2020.06.003
Tóber M., Kiss A. (2014). Landscape history of the medieval Sand Ridge area in Central Hungary: examples of sand and arboreal vegetation in medieval documentation compared to the results of natural scientific and archaeological investigations. Siedlungsforschung 31, 247–269.
Tryjanowski P., Skórka P., Sparks T. H., Biaduń W., Brauze T., Hetmański T., et al. (2015). Urban and rural habitats differ in number and type of bird feeders and in bird species consuming supplementary food. Environ. Sci. pollut. Res. 22 (19), 15097–15103. doi: 10.1007/s11356-015-4723-0
Van de Ven T. M., McKechnie A. E., Cunningham S. (2019). The costs of keeping cool: behavioural trade-offs between foraging and thermoregulation are associated with significant mass losses in an arid-zone bird. Oecologia 191 (1), 205–215. doi: 10.1007/s00442-019-04486-x
VisualCrossing Cooperation (2022) Weather query builder. Available at: https://www.visualcrossing.com/weather/weather-data-services/Budapest/metric/2020-01-01/2020-12-31.
Vuorisalo T., Andersson H., Hugg T., Lahtinen R., Laaksonen H., Lehikoinen E. (2003). Urban development from an avian perspective: causes of Hooded Crow (Corvus corone cornix) urbanisation in two Finnish cities. Landscape Urban. Plann. 62 (2), 69–87. doi: 10.1016/S0169-2046(02)00124-X
Withey J. C., Marzluff J. M. (2005). Dispersal by juvenile American crows (Corvus brachyrhynchos) influences population dynamics across a gradient of urbanization. The Auk. 122 (1), 205–221. doi: 10.1093/auk/122.1.205
Withey J. C., Marzluff J. M. (2009). Multi-scale use of lands providing anthropogenic resources by American Crows in an urbanizing landscape. Landscape Ecol. 24 (2), 281–293. doi: 10.1007/s10980-008-9305-9
Xu Y., Cao Z., Wang B. (2020). Effect of urbanization intensity on nest-site selection by Eurasian Magpies (Pica pica). Urban. Ecosyst. 23 (5), 1099–1105. doi: 10.1007/s11252-020-00996-2
Yeh P. J., Price T. D. (2004). Adaptive phenotypic plasticity and the successful colonization of a novel environment. Am. Nat. 164 (4), 531–542. doi: 10.1086/423825
Yom-Tov Y., Yom-Tov S., Wright J., JR Thorne C., Du Feu R. (2006). Recent changes in body weight and wing length among some British passerine birds. Oikos 112 (1), 91–101. doi: 10.1111/j.0030-1299.2006.14183.x
Zhang S., Suo M., Liu S., Liang W. (2013). Do major roads reduce gene flow in urban bird populations? PloS One 8 (10), e77026. doi: 10.1371/journal.pone.0077026
Keywords: adaptation, anthropogenic food, body condition, city, corvid, heat island, human-wildlife conflict, urban bird
Citation: Benmazouz I, Jokimäki J, Juhász L, Kaisanlahti-Jokimäki M-L, Paládi P, Kardos G, Lengyel S and Kövér L (2023) Morphological changes in hooded crows (Corvus cornix) related to urbanization. Front. Ecol. Evol. 11:1196075. doi: 10.3389/fevo.2023.1196075
Received: 29 March 2023; Accepted: 26 September 2023;
Published: 10 October 2023.
Edited by:
Salvador García-Ayllón Veintimilla, Polytechnic University of Cartagena, SpainReviewed by:
Bree Putman, California State University, San Bernardino, United StatesFranco Leandro de Souza, Federal University of Mato Grosso do Sul, Brazil
Copyright © 2023 Benmazouz, Jokimäki, Juhász, Kaisanlahti-Jokimäki, Paládi, Kardos, Lengyel and Kövér. This is an open-access article distributed under the terms of the Creative Commons Attribution License (CC BY). The use, distribution or reproduction in other forums is permitted, provided the original author(s) and the copyright owner(s) are credited and that the original publication in this journal is cited, in accordance with accepted academic practice. No use, distribution or reproduction is permitted which does not comply with these terms.
*Correspondence: Isma Benmazouz, benmazouz.isma@agr.unideb.hu