- 1Biological, Earth, and Environmental Sciences, University of New South Wales, Sydney, NSW, Australia
- 2Coral Conservation and Research Group, Trace and Environmental DNA Laboratory, School of Molecular and Life Sciences, Curtin University, Bentley, WA, Australia
- 3Collections and Research, Western Australian Museum, Welshpool, WA, Australia
- 4School of Environmental and Life Sciences, University of Newcastle, Callaghan, NSW, Australia
Ocean warming has been driving mortality events across the world’s coral reef ecosystems and is resulting in multifaceted ecosystem restructuring. With the rapid shifts occurring across ecosystems, questions arise of which species, in which locations, have the capacity to persevere under climate change. Environmental generalism refers to species with the biological traits that support environmental flexibility, enabling the organism to occupy a broad range of environmental conditions. Some Scleractinia have been categorised as environmental generalists and proposed as likely winners under changing climate conditions, as environmental generalists have been considered less susceptible to environmental disturbance than specialist species. Given the complexity of the holobiont structure of corals, which includes photoendosymbiosis and diverse microbial consortia, understanding the complexity of the coral holobiont–environment interaction for the generalist corals will be an important factor in accurately predicting the success of these species into the future. Here we conduct a literature search to compile topics and concepts of environmental generalism for Australia’s warming coral reef ecosystems and the breadth of holobiont responses to ecosystem restructuring. We synthesise these findings in the context of the latitudinal expanse of Australia’s coral reefs to highlight how it is necessary to understand the biological underpinnings of generalist corals.
1 Introduction
Scleractinian, or hard (stony) corals are most widely known for their role as the trophic and structural foundation of coral reefs, particularly for reefs in warm, shallow, tropical waters (Hamylton et al., 2022). While corals dominate tropical reefs and provide the foundation for the most diverse ecosystems on the planet, corals are also found across a breadth of light and temperature regimes outside of reef forming structures, including within sandy bays, caves, coastal waterways, mangroves, seagrass beds, temperate waters and the deep sea (Perry and Larcombe, 2003; Cairns, 2007; Richards et al., 2016; Camp et al., 2019; Watanabe and Nakamura, 2019; Burt et al., 2020). In addition to being found across a range of marine ecosystems, this highly diverse group of organisms exhibits a wide variety of life history traits, growth forms, and adaptations to the array of environmental regimes in which they are found (Jackson, 1991; Connell et al., 2004; Todd, 2008; Zawada et al., 2019; Bairos-Novak et al., 2021). This diversity of coral life highlights the challenges that arise when forecasting the role this taxa will play in ecosystem restructuring under environmental change.
Global coral reef ecosystems, dominated by reef-forming scleractinia (Pandolfi et al., 2011; Vermeij et al., 2011; Hughes et al., 2018; Cornwall et al., 2021; Eddy et al., 2021), are undergoing significant changes due to anthropogenic climate change. Record warm temperatures and rates of warming are disrupting coral photoendosymbiosis and causing widespread whole organism heat-induced and bleaching-induced mortality across the world’s coral reefs (Donovan et al., 2021; Hughes et al., 2021). As bleaching events increase in frequency, the impact of these disturbance events accumulates and affects the capacity of the ecosystem to recover (Dietzel et al., 2021). In one well-documented study undertaken between 1968 and 2004, comprising 6001 benthic surveys of 2,667 Indo-Pacific reefs, a 1–2% yearly decline in coral cover was demonstrated (Bruno and Selig, 2007). Similarly, in the Seychelles, a 17-year data set demonstrated mass bleaching events reduced live coral cover by > 90% in the reefs surveyed, leading to a shift towards a macroalgae-dominated ecosystem in nine out of 21 reefs (Graham et al., 2006; Graham et al., 2015). A time-series analysis of coral reefs in Moorea, French Polynesia found extensive coral loss and change in abundance of the major coral genera on the reef between 1979 and 2009 due to the cumulative impacts of Acanthaster planci outbreaks, cyclones, and bleaching (Pratchett et al., 2011). A similar trajectory has been reported for coral reefs in Okinawa, Japan, where net coral area declined 42–72% and changes in abundance of the dominant scleractinian taxa followed bleaching events, typhoons, and increased sedimentation (Harii et al., 2014). The recent coral cover report for the Great Barrier Reef by the Australian Institute of Marine Science (AIMS, 2022) also suggested that short-term gains in coral cover following severe bleaching and mortality events in 2021/2022 highlighted long-term impacts of shifting population structure to fast growing but thermally susceptible coral species. Fast-growing Acropora species, which drive recovery on damaged reefs, are also the most vulnerable to the common disturbances affecting the GBR (AIMS, 2022). These examples underscore widespread coral reef degradation from biological and anthropogenic stressors (Eddy et al., 2021). As such, coral reefs are now being altered in historically unprecedented ways (van Woesik et al., 2012).
The vast ecosystem restructure occurring in marine ecosystems has also been shown to include the replacement of historically dominant coral species with corals exhibiting more persistent, weedy, and opportunistic traits (Knowlton, 2001; McClanahan et al., 2007; de Bakker et al., 2016; Caballero Aragón et al., 2019; McWilliam et al., 2020; Cornwall et al., 2022). Generalist coral species, or those that thrive across a breadth of environmental conditions and are considered more tolerant of sub-optimal conditions than species within a narrow environmental niche (Richmond et al., 2005; Clavel et al., 2011; Darling et al., 2012), are hypothesised to play an important role in this population restructure (Courtney et al., 2020). In contrast, specialist species may be more susceptible to metapopulation dynamics under climate change, with potential extinction risks due to decreased effective population size and genetic variation in already small populations; this has been seen in butterflyfish (Lawton et al., 2011), marine teleosts (Smith and Fujio, 1982), and marine molluscs (Lavm and Nevo, 1981). Generalist coral species may be more successful than specialist coral species on reef ecosystems as the climate continues to change (Clavel et al., 2011; Chichorro et al., 2019), offering critical ecological opportunities for the reconfiguration of coral reefs. As such, addressing knowledge gaps in the potential for these species to expand into new habitats as ecosystem restructuring occurs is crucial for predicting both future reef structure and coral extinction risk (Bridge et al., 2020).
1.1 Terminology used in environmental generalism research for corals and coral reef ecosystems
Generalist species have been defined in ecology as those that exploit multiple habitat types or food sources, while a specialist is limited to only one or a few (van Tienderen, 1991). There are several ecological predictions that have been linked to both generalist and specialist species that hypothesise various uses of resources determine the range size of the species (Slatyer et al., 2013).This hypothesis states generalist species are more likely to have a higher tolerance towards habitat loss and climate change due to their adaptabilities and large range sizes through positive correlations between niche breadth and geographic range. Generalist species with a broad resource breadth are thought to be more persistent because they are less sensitive to stochastic fluctuations of any given resource. This concept is also the foundation of the generalism–specialism debate, where generalism in resource use and niche breadth has been suggested to create an ongoing cycle where the success of generalists drive speciation and species extinction (Dennis et al., 2011). However, there is clearly a wide range in how generalist species and their role in their environment is defined.
The terms “generalist species”, “generalism” and “generalist coral” appear to be underutilized and inconsistently applied in coral reef research. For example, a search of primary research publications for the terms <generalist AND coral> between 2010 and 2022 identified 187 articles, of which only 72 studies related to specifically to Scleractinian coral species (opposed to other species within coral reef ecosystems). Of these studies, 2 species, Montastrea cavernosa and Stylophora pistillata, were the primary study species in the research conducted (Table 1). While definitions for generalist species and use of generalist terminology has varied in the primary literature, the research that has used these terms to-date has classified generalist species by the taxa within-reef distribution, with 68% of studies identified here referring to depth generalists (Figure 1). Generalist corals have also been defined through:
1) flexibility of their relationship with Symbiodiniaceae and the bacterial microbiome (16% of the identified studies),
2) variation in life history traits (11% of identified studies),
3) a broad environmental distribution (4% of identified studies), and
4) plastic morphology (1% of identified studies).

Table 1 72 publications returned in a Google Scholar search for the terms <generalist AND coral> between 2010 and 2022, sorted into five primary categories used to define generalists in the literature: depth, environmental, life history, morphology, and symbiont.
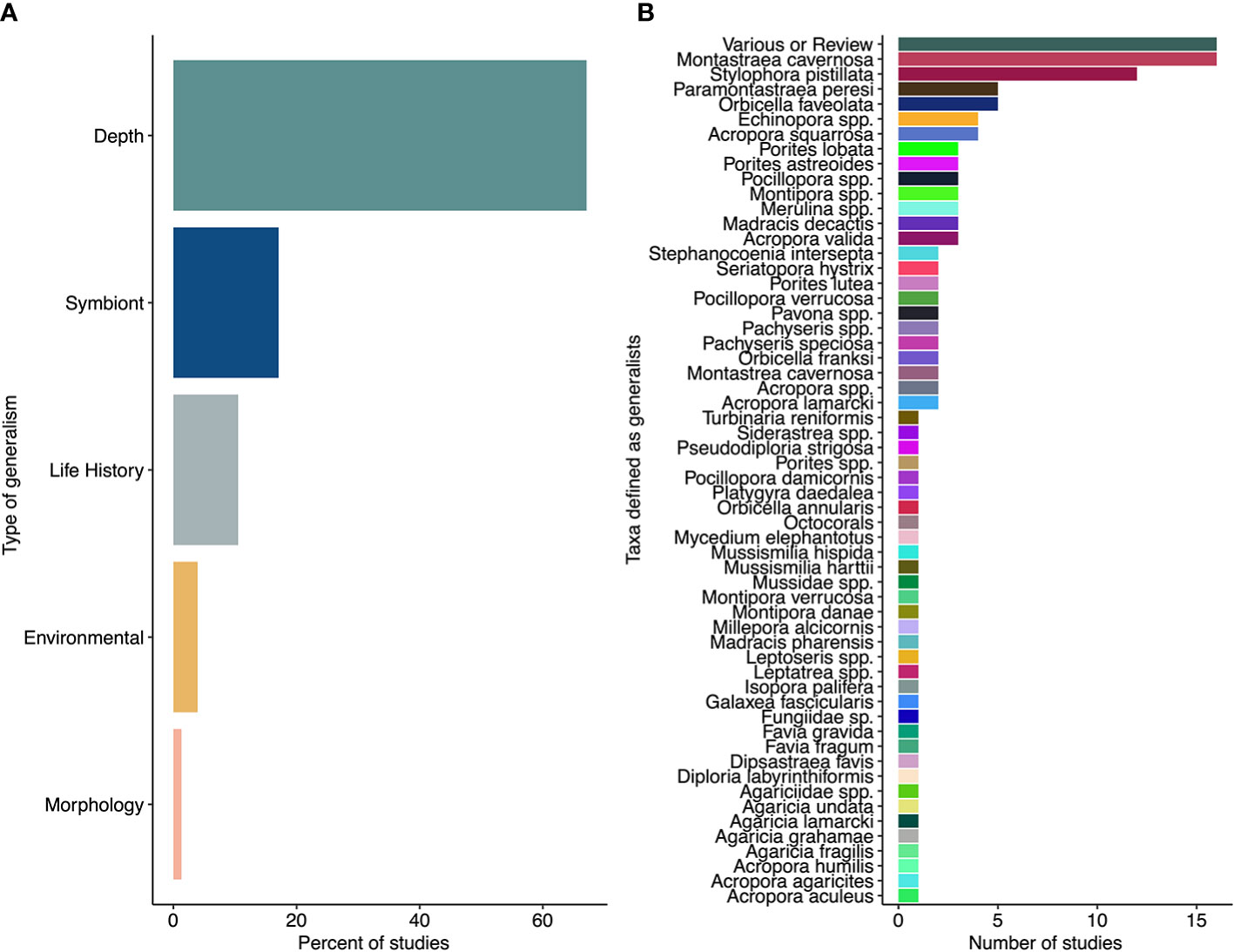
Figure 1 (A) Percentage of the 72 studies included in the present manuscript that are focused around each category of generalism. (B) Number of studies per taxa considered “generalists” in the 72 studies considered herein. “Various or Review” refers to studies that didn’t specify taxa or that contained mention of the terms <generalist AND coral> in a review, respectively.
Two studies have conducted the most extensive categorisation of coral species within generalist and specialists groups:
Firstly, Darling et al. (2012) applied a trait-based classification approach for 143 species of coral, defining generalist species as those having life history traits of competitive, weedy, and stress-tolerant corals. Competitive species have traits that align with specialist species, such as efficiency in using resources and dominance in highly populated, ideal environments. Weedy corals have fast reproduction times and diverse species traits, which allows them to opportunistically colonise a variety of disturbed environments. Stress-tolerant corals are defined as those with slow growth, long generation times, large corallites, and high fecundity that help them to proliferate in chronically harsh environments. Generalist corals have overlapping traits with both weedy and stress tolerant corals, as defined by Darling et al. (2012), and we refer to corals with this set of traits as “generalists” from here on out. Sommer et al. (2014) also utilised trait-based filtering for corals along a latitudinal gradient in high-latitude eastern Australia, finding high-latitude coastal reefs to be mainly dominated by generalist coral species of massive morphologies, which persist in conditions of high environmental stress but also contribute to a low structural complexity and have limited recovery potential following disturbance due to slow-growing trait of massive morphotypes. However, consistent with Darling et al. (2012), the authors also found that species with similar functional characteristics have higher co-occurrence. This raises the question of how abiotic filtering (e.g. environmental parameters) will influence the structure of coral communities when corals with different life-history strategies (e.g. different growth rates or reproductive modes) share similar traits.
Table 2 summarises trait-based classifications used in the studies of Darling et al. (2012), Darling et al. (2019) and Sommer et al. (2014) to further elaborate on how generalist species can encompass a combination of various life history strategies or be composed of a subset of competitive taxa (as defined by Darling et al., 2012). The ambiguity in defining generalist species highlights a knowledge gap crucial for predicting and managing the form and function of future reefs and optimising conservation planning. Pocilloporid corals (Gray, 1840) are a widespread taxonomic group of scleractinian corals that have been reported on coral reef ecosystems worldwide across vast geographic, environmental, and depth ranges (Figure 2). Known to be a fast-growing and opportunistic genus (Brustolin et al., 2019), Pocilloporids serve as an example of generalist traits due to both high levels of phenotypic plasticity and a broad environmental distribution (Todd, 2008; Schmidt-Roach et al., 2014; Putnam et al., 2020; Burgess et al., 2021). The capacity for plasticity in the group contributes to the challenge of identifying species, species complexes and species ranges in addition to having substantial genetic variability amongst visually similar species (Todd, 2008). For example, Pocillopora damicornis has traditionally been described as four distinct ecomorphs – elongate, semi-disturbed, compact, and branching (Veron and Pichon, 1976). Recent studies have therefore used genetic and morphometric evidence to differentiate species within the Pocilloporids, with Schmidt-Roach et al. (2014) describing eight species within the P. damicornis species complex (including a novel taxon Pocillopora bairdi). Johnston et al. (2022) genetically identified 673 colonies in the Pocillopora species complex around Mo’orea, French Polynesia, and found that the 4 most abundant species were often visually indistinguishable yet exhibited clear physiological differences in response to light and water temperature variance. These studies indicate that while many Pocilloporid species are visually indistinguishable, genetic differences may be associated with distinct traits that affect their success in a changing environment. The misinterpretation of taxonomical units within species, e.g. for Pocillopora damicornis (Schmidt-Roach et al., 2012), contributes to challenges of disentangling morphological and life history variation with environment. This further highlights the complexity and plasticity of the Pocilloporid family. The wide variety of responses recorded between coral host, algal symbionts, bacterial microbiome (“holobiont”), and the holobiont–environment interaction, suggests that generalist corals such as the Pocilloporidae may have the breadth of plastic traits necessary to survive in a changing world. Here we review (1) the traits of the Pocilloporid holobiont associated with environmental generalism; (2) the aspects of holobiont–environment interaction that would support the assumption of environmental generalism in this genera; and (3) the intersection of environmental generalism, holobiont structure, and the restructuring of a diverse range of coral habitats across Australia’s east coast.
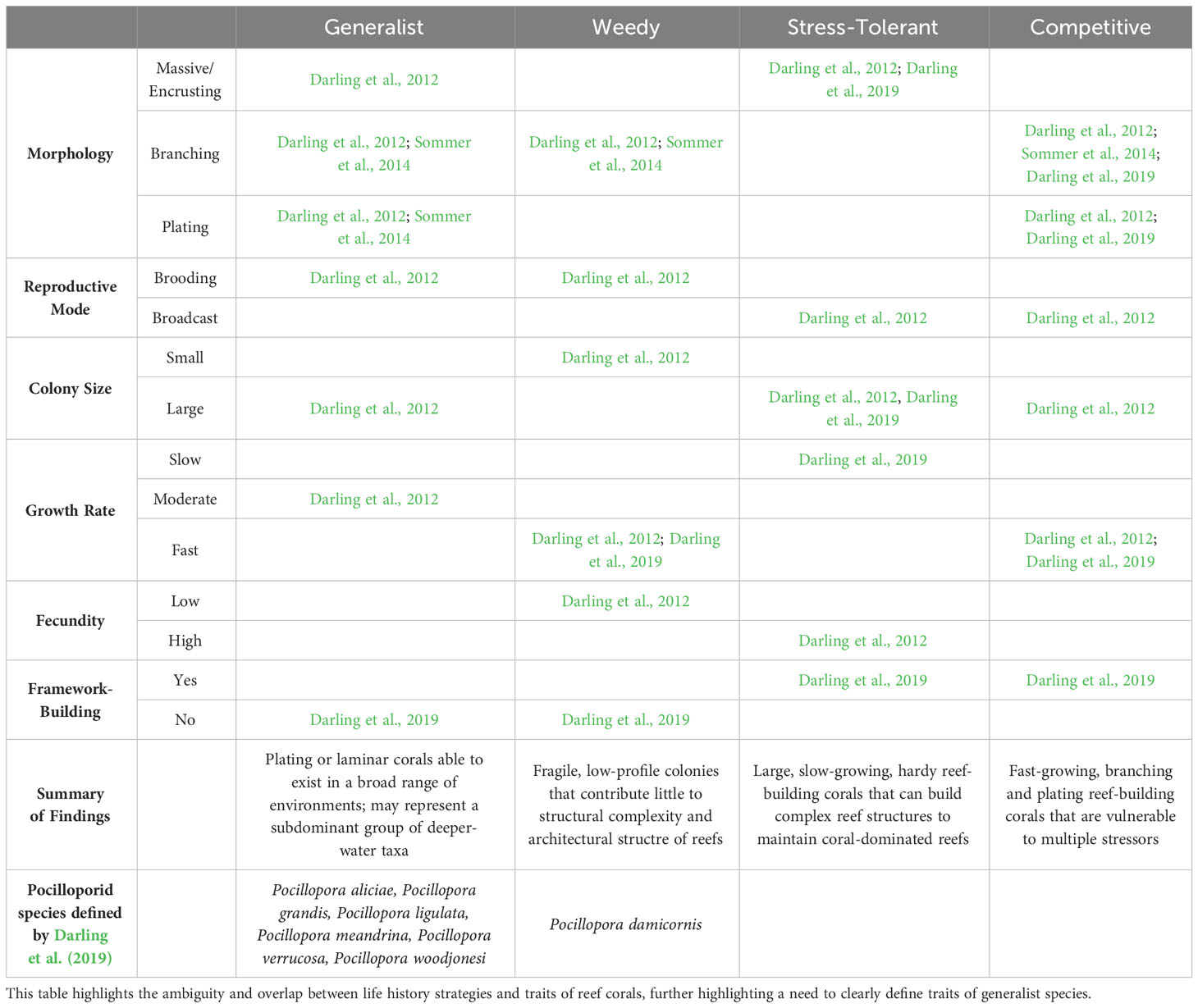
Table 2 A summary of trait-based classifications used by Darling et al. (2012), Darling et al. (2019), and Sommer et al. (2014) to define generalist species and three life-history forms contained within: weedy, stress-tolerant, and competitive.
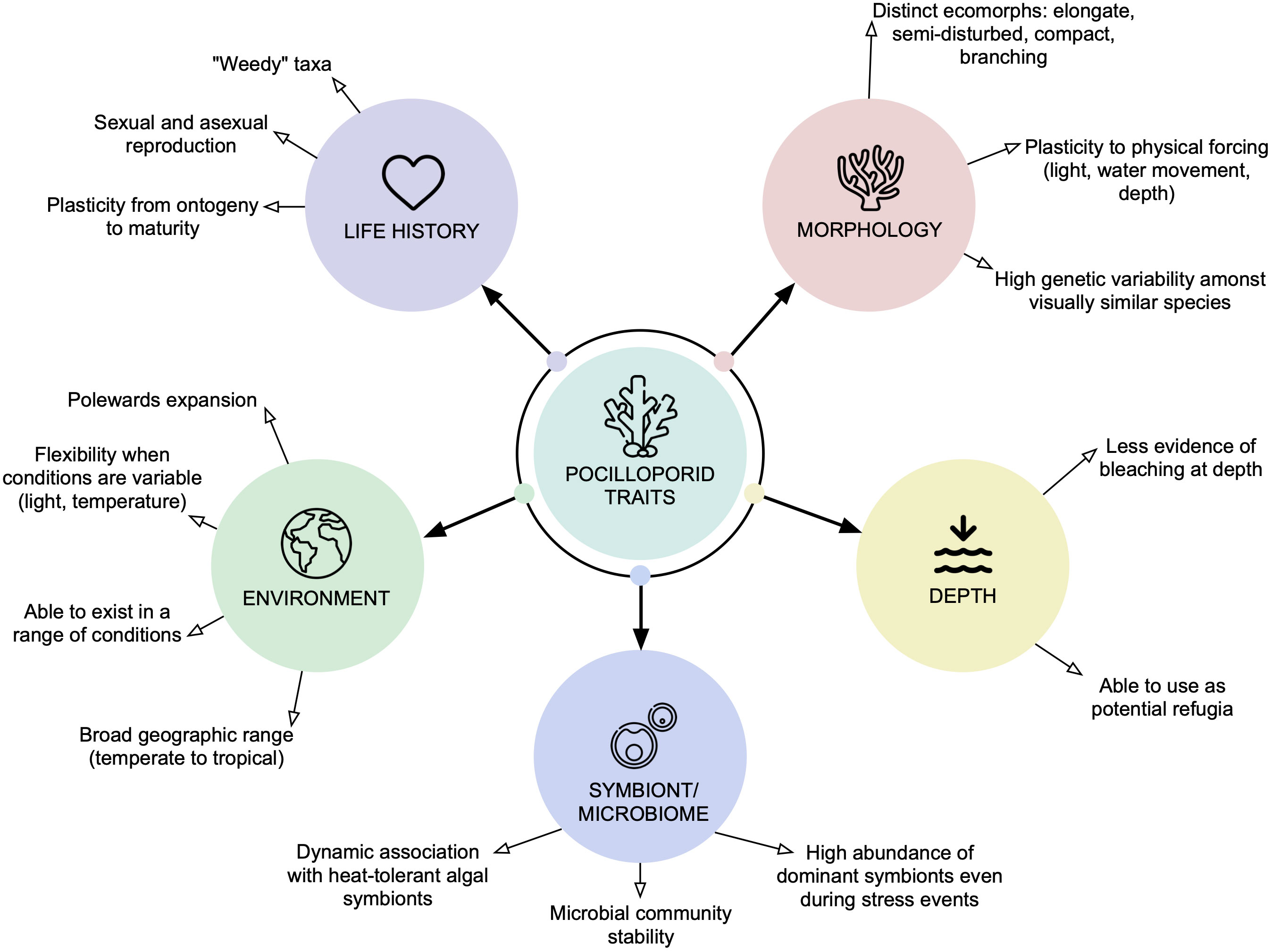
Figure 2 A schematic illustrating the five domains of generalist species defined in the literature search conducted herein, and how traits common to Pocilloporids apply to each domain. Black text arrows indicate how Pocilloporids may respond to influence their success when environmental pressures (e.g. increased light, rising temperatures, physical forcing) are applied.
2 Generalist traits and the Pocilloporid holobiont
2.1 Morphological plasticity
Pocilloporid corals have a high degree of plasticity in overall skeletal morphology across environments (Todd, 2008; Johnston et al., 2017). Pocilloporids increase in compactness with increasing water motion (Kaandorp, 1999), vary in branch structure concurrent with degree of reef exposure (Lesser et al., 1994), and can appear elongate in subtropical regions while being robust in tropical regions (Schmidt-Roach et al., 2014). Furthermore, water flow and wave motion were found to systematically ordinate Pocillopora colony structure in Mo’orea, French Polynesia (Corso et al., 2022), due to physical forcing that likely aligns colonies with the dominant direction of the wave energy. Pocilloporids can also exhibit microstructural plasticity in response to light, water movement, and depth. Pocilloporid microstructure is influenced by environmental parameters, for example significant variation in 17 micro- and two macrostructural characteristics was observed in Pocillopora verrucosa colonies found between 7 and 38–45m depth (Gélin et al., 2018).
2.2 Tissue structure and within-colony symbiont distribution
Biological traits of tissue structure and its influence on symbiont distribution within a colony are believed to support vast environmental tolerance, including depth and light tolerance. In a study by Terraneo et al., examining symbiont types in shallow and mesophotic corals in the Red Sea, Durusdinium spp., known to assist with stress and thermal tolerance, was found predominately in shallow water corals compared to mesophotic corals (Terraneo et al., 2023). This suggests that symbiont distribution may be host and environment specific. Pocilloporids, characterised by an imperforate tissue structure that lacks intercalating tissues in a skeletal matrix (Yost et al., 2013), exhibit adaptability to diverse environmental conditions due to their shallow tissue depth. This tissue structure that predominantly extends across the surface of the skeleton contrasts with the tissue structure within the matrix of the colonial skeleton that is seen in perforate corals. Another potential contributing factor to the flexibility of Pocilloporids across different depths is their highly variable symbiont distribution related to light penetration and amplification within the colonies (Terán et al., 2010). Interestingly, Pocilloporids are the only genera that have been linked to polyp bail out responses (Fordyce et al., 2017; Chuang and Mitarai, 2020; Gösser et al., 2021), wherein individual polyps disassociate from a colony under stress and re-settle on the benthic substrate. It is important to note that to date the role of polyp bailout responses in Pocilloporid recovery of coral cover (Fordyce et al., 2017) following severe bleaching events has not been well investigated.
2.3 Bacterial microbiome stability
Several thousand microbes have been discovered within the coral microbiome (Rohwer et al., 2002). These are comprised of archaea, bacteria, viruses and Symbiodiniaceae (Symbiodinium) which inhabit the coral tissues, skeleton, mucus and gastrovascular cavity (Osman et al., 2018). These microbial interactions can either be mutualistic, parasitic or symbiotic interactions (Aranda et al., 2016). The ubiquitous distribution of Pocilloporid corals across environments have made them a well-studied target in coral bacterial microbiome research since 2018, characterising the Pocillopora microbiome across the Indo-Pacific and Red Sea. There also evidence that the coral microbial associations with P. damicornis may be a factor influencing bleaching response in these corals (Gilbert et al., 2012); namely, widely observed microbial patterns in response to bleaching may contribute to Pocillopora’s classification as a generalist. These include microbial stability during bleaching and across different environmental conditions (Ziegler et al., 2019; Brener-Raffalli et al., 2018; Pogoreutz et al., 2018; Epstein et al., 2019; Maher et al., 2020; Bergman et al., 2021). Some studies have found that despite severe bleaching in P. damicornis (e.g. loss of symbionts, but no mortality), bacterial communities did not increase in diversity, change, or become pathogen dominated; rather, the community structure resembled that of healthy P. damicornis colonies (Bergman et al., 2021). Similar findings have been observed in P. damicornis larvae, where Symbiodinium cell densities of larvae decreased under high temperature conditions, but no reduced survivorship was observed (Haryanti et al., 2015). Bacterial shifts in response to bleaching have been observed, for example shifts in Symbiodinium-associated partner bacteria, such as Lactococcus and Bacillus, in Pocillopora verrucosa colonies during a natural bleaching event in the South China Sea (Yang et al., 2021). Interestingly, in a study of P. acuta, the microbiome was found to respond rapidly and flexibly to transplantation in Singapore, becoming similar to that of the local colonies at the transplanted reef within 1–2 days of transplantation (Deignan and McDougald, 2022). Even in stable communities, Endozoicomonas is a bacterial associate consistently found in the microbiome of Pocilloporid corals (Pogoreutz et al., 2018; Epstein et al., 2019; Voolstra and Ziegler, 2020; Ricci et al., 2022), with Maher et al. (2019) reporting Endozoicomonas dominate Pocillopora microbiomes that have declined in overall diversity. Microbial stability in Pocillopora in response to warming may contribute to its role as a generalist species on reefs.
2.4 Photoendosymbiont and holobiont bleaching responses
In Pocilloporid corals Symbiodinium are transferred maternally, leading to co-evolution with the host and species-specific associations of symbionts irrespective of geography (Pinzón and LaJeunesse, 2011; Schmidt-Roach et al., 2013a; Brener-Raffalli et al., 2018). Stability in patterns and composition of P. damicornis-associated Symbiodiniaceae has been found between adults and juveniles in the South China Sea irrespective of exposure to stress (i.e. ocean acidification), with a consistent dominance of the thermally tolerant endosymbiont Durusdinium spp. (Zhou et al., 2021). Endosymbiont population stability has also been observed in adult Pocillopora, with the stress-tolerant Durusdinium largely dominating the Symbiodiniaceae population in P. acuta throughout bleaching response of the host coral (Poquita-Du et al., 2020a; Poquita-Du et al., 2020b). In the Red Sea, where there are strong environmental gradients from north to south, Symbiodinium microadriaticum (type A1) has been found to dominate across a latitutinal gradient (Sawall et al., 2014). While the dominant resident Symbiodinium in Pocillopora corals often remain at a high abundance, even during stress events (e.g. cold-water bleaching, (McGinley et al., 2012), there is also some evidence of flexibility in host–symbiont combinations with variability across sites (Cunning et al., 2013) or environments (Ros et al., 2021; Botté et al., 2022). Observed interactions between physiological performance, host genotype, and symbiont communities in P. damicornis, as observed between flat or slope reef habitats of Australia’s Heron Island in Marhoefer et al. (2021), emphasise that local adaptation of colonies as a product of environmental factors is also possible and should be accounted for.
3 The generalist holobiont–environment interaction and a changing climate
The largest influences on corals is the availability of photosynthetically available radiation (PAR), which causes variations in morphology, skeletal structures, photosynthetic traits, heterotrophic feeding, and Symbiondinaceae genera (Martinez et al., 2020). Generalist species are regarded as likely to be more successful than specialists as the climate continues to change (Clavel et al., 2011; Chichorro et al., 2019) due to their ability to adjust to these influences in their environment. Understanding the holobiont traits that contribute to environmental generalism is critical to predicting the structure of reefs under future scenarios. As global climate change continues to drive ecosystem change and novel environmental conditions become increasingly prevalent, the hypothesised increased role of environmental generalists in ecosystem stabilisation is evident (Graham et al., 2014; Williams and Graham, 2019). A recent study conducted on the reefs of French Polynesia investigating a 26-year monitoring database found that corals in the genus Pocillopora now make up 84% of the total recovery rate on the reef following disturbance (Pérez-Rosales et al., 2021). While the success of some genera on disturbed reefs is beneficial for overall coral coverage, a convergence towards post-disturbance communities less diverse and dominated by a single genera, morphology or phenotype (as shown by Pérez-Rosales et al. (2021) for 5 out of the 7 reefs) is also discussed as a key risk of sudden ecosystem declines under further disturbance events (Dalin et al., 2009; Palumbi et al., 2009; Lin, 2011). Palumbi et al. (2009) emphasise the importance of preserving biodiversity on reefs, as communities dominated by a single genus are likely have a more limited range of functions in response to disturbance than reefs composed of a diverse range of genera. Dominance of a single genera, especially one that is visually indistinguishable from other species within its genera, may be due to niche differences in co-occurring cryptic species. For example, Johnston et al. (2022) found greater differential abundances of Pocilloporid species across depths than amongst sites separated by several kilometers. The four most abundant species observed in this study were visibly indistinguishable at the gross colony level, yet exhibited differences in their associations and response to light irradiance and water temperature that may further promote their proliferation across reef environments with potentially varying degrees of generalism by species (Johnston et al., 2022).
Recent monitoring on the GBR following severe bleaching events of 2016–20 have also found an increase in dominance of Pocillopora species over the previously dominant specialist Acropora species (AIMS, 2021), noting that an increase in cover of a single genera had the potential to put the reefs at greater risk in future events. Therefore, the role of environmental generalist species (those with greater plasticity for a range of some conditions) will be uncertain in highly dynamic marine environments, particularly those environments with severe and frequent extreme conditions (Munday, 2004; Camp et al., 2018).
As warming on temperate, subtropical, and tropical reefs continues to drive the emergence of novel ecosystems, efforts in conservation and ecosystem rehabilitation, repair, and restoration are now based around supporting (repair and rehabilitation) or actively undertaking (restoration) reconstruction of altered and degraded environments (Fox et al., 2019; Vardi et al., 2021; Shaver et al., 2022). Successful ecosystem repair, reconstruction or restoration involves identifying the target organisms that can acclimate and proliferate in both current and predicted future environmental conditions (Prober et al., 2015; van Oppen et al., 2017; Vardi et al., 2021; Shaver et al., 2022). Understanding the physiological range of the target organism in a dynamic environment for these types of conservation efforts will therefore be the cornerstone of the science of conservation ecology on coral reefs. Detailed understanding of the plasticity of the biological traits which underpin an organism’s success across a range of environment parameters will be key for conservation efforts, particularly those focused on organisms that show resilience and environmental plasticity (Kimball et al., 2016; Boström-Einarsson et al., 2020; Shaver et al., 2022). The broad similarities amongst Pocilloporid species in the relationship between morphology, microbiome, and plasticity across sites or environmental conditions are therefore potentially key factors contributing to the success of this genera.
4 Australia as a case study: are there locations in eastern Australia where coral reefs are resistant to environmental change?
Australia’s reefs have been subject to the impacts of climate change in recent years, and we focus specifically on several examples where reefs have been impacted by warming. We continue to build on the aforementioned point that generalist species may be better suited to acclimate to current environmental conditions (e.g. warming), but that it is critical to understand what role they will play in future reef conditions or in the highly dynamic marine environments mentioned herein. The majority of Australia’s coral reef ecosystems extend across approximately 344,400 km2 of the continent’s east coast, which includes the ecosystems of tropical coral reefs, subtropical coral reefs, and high-latitude coral reefs (Great Barrier Reef Marine Park Authority, 2012; Figure 3). Most tropical corals are found 30–200 km offshore along the shallow inshore and lagoonal reefs, submerged reefs, mesophotic and deep-water coral reefs of the Great Barrier Reef/Coral Sea (Olsson et al., 2008). Subtropical continental shelf coral populations are also found along approximately 900 km of continental shelf from the southern Great Barrier Reef to the Solitary Islands, with temperate populations now being reported as far south as Sydney Harbour (Harriott and Banks, 1995; Harriott and Smith, 2002; Linklater et al., 2016; Roelfsema et al., 2016; Linklater et al., 2018; Linklater et al., 2019). Coral populations within the subtropical-to-temperate transition zone of Eastern Australia include marine habitats with unique assemblages of corals, sea grasses, kelps and turfs (Wilson and Harrison, 2003; Vergés et al., 2016; Sommer et al., 2017; Vergés et al., 2019). Further south, merging temperate and subtropical waters supports the development of new species, unique species interactions, and distinct habitats on the world’s most southern coral reef, World Heritage-listed Lord Howe Island (Harriott et al., 1995; Noreen et al., 2009; Edgar et al., 2010).
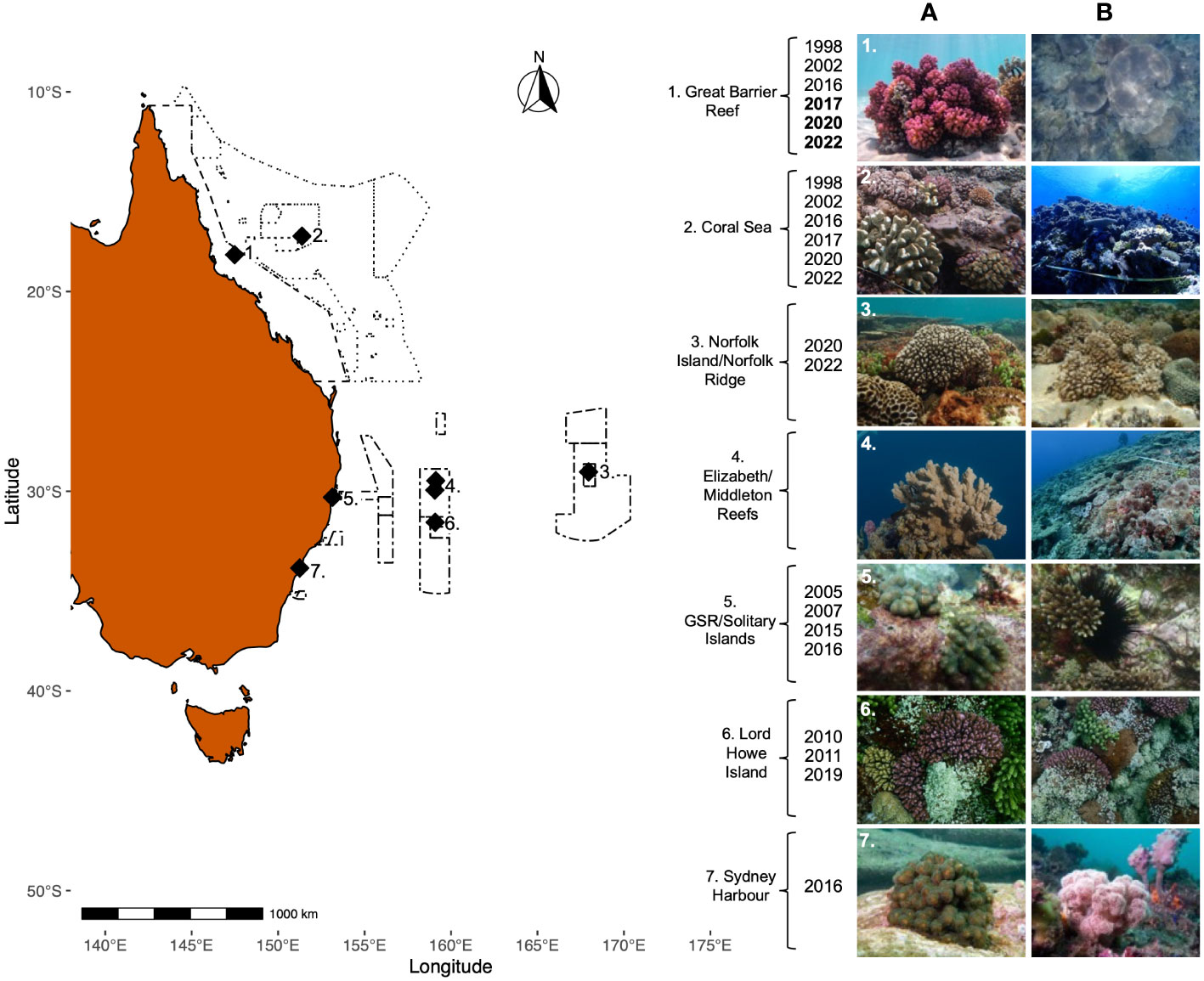
Figure 3 A map of reef locations included along Australia’s east coast. Long dashes border the Great Barrier Reef Marine Park, dotted lines border the Coral Sea Marine Park, and dot-dash lines border the Temperate East Australian Marine Park Network. Sites are: 1). Great Barrier Reef, 2). Coral Sea, 3). Norfolk Island and Norfolk Ridge, 4). Elizabeth and Middleton Reefs, 5). Solitary Islands Marine Park, 6). The Lord Howe Seamount Chain, Lord Howe Island, and Ball’s Pyramid, and 7). Sydney Harbour. For images on the right-hand side, numbers correspond to reef location and letters depict (A) an example of a Pocilloporid from each location and (B) a whole-reef image from each location. All pictures taken by Jessica Bergman, with the exception of images reproduced with permission: 2A, 2B: Parks Australia Coral Sea Marine Park Coral Reef Health Report; 4A, 4B: Antonia Cooper; 7A, 7B: John Turnbull. Bleaching events recorded at each location are listed along the left-hand side of the photos. For 1). Great Barrier Reef: Bolded dates are years when both the N. GBR and S. GBR bleached, and dates not in bold are years when only the N. GBR bleached.
Coral reefs within Australia’s southern Great Barrier Reef and Great Southern Reef, particularly those at the convergence of sub-tropical and temperate zones, are as such experiencing climate mediated changes to species distribution and abundance, benthic habitat change, and the range extension of tropical species (Pandolfi et al., 2003; Brandl et al., 2019; Eddy et al., 2021). Warming oceans are driving high coral mortality, changes to population structure, emergence of new diseases, reduced growth, and reduced recruitment even on high-latitude reefs (generally outside of 30° N or S). Most evident of this problem is the severity and frequency of mass coral bleaching events now increasingly recorded in corals within Australia’s understudied high-latitude reefs. For example, bleaching was recorded at Lord Howe Island in 1998, 2005, 2010, 2011 and 2019, one of the highest frequencies of coral bleaching events reported in Australia (Harrison et al., 2011; Dalton et al., 2020; Steinberg et al., 2022). Additionally, mass bleaching affected 22 high-latitude coral assemblages found south of the GBR in 2016 (Kim et al., 2019). Genetic evidence shows that connectivity to larger metapopulations is limited for corals growing at the southern edge of their species range (Noreen et al., 2015; Mizerek et al., 2021), resulting in high levels of evolutionary novelty (Miller and Ayre, 2008; Noreen et al., 2009; Schmidt-Roach et al., 2013a; Noreen et al., 2015) in these locations.
The observed species composition changes observed on temperate to subtropical reefs have the potential to profoundly alter temperate marine ecosystems within the coming decades. The question arises as to whether generalist corals will be a feature of Australia’s changing east coast coral reef ecosystems as climate change continues to impact these regions. The response of generalist species, specifically Pocilloporids, to climate change and ocean warming in Australia has varied by region. For example, bleaching events occurring on the tropical reefs of the Great Barrier Reef have impacted almost all species (Stuart-Smith et al., 2018), with over 43% of reefs bleaching in 1998, 56% bleaching in 2002, and 85% in 2016 (Hughes et al., 2017) and region-wide coral bleaching occurring from February–April 2020 and December–March 2022 (Ainsworth et al., 2021, GBRMPA Reef Snapshot, 2022). Pocillopora spp. has been categorised as bleaching-sensitive on the GBR, showing declines in small colonies of up to 28% on the reef crest and 30.2% on the reef slope following mass coral bleaching in 2016/2017 (Dietzel et al., 2020). In the Keppel Island archipelago, situated on the southern inshore GBR, 21% of living corals were affected by bleaching, with Pocillopora and branching Acropora the most affected (Kennedy et al., 2017). Botté et al. (2022) also found a degree of mild, moderate, or severe bleaching in all Pocillopora acuta colonies sampled between 3.5–5.6°C-weeks on Pandora and Havannah Island reefs within the Palm Island group on the central GBR. However, in the Coral Sea, partial mortality was lower in Pocillopora species during bleaching in 2020 than in 2018/2019 prior to bleaching, and overall incidence of partial mortality in all species surveyed remained below 5% (Burn et al., 2022). Outside of Australia, co-evolved mutualisms between Pocillopora corals and heat-tolerant symbionts have been found with few observable tradeoffs, suggesting that increased prevalence of these mutualisms will contribute to reef growth and the proliferation of Pocillopora spp. in warming environments (Mexico; Turnham et al., 2023). An additional study in Panama suggests that Pocillopora will be selected over other coral genera in warming regions, due to associations with thermotolerant symbionts, the potential acquisition of thermally tolerant symbionts during heat stress, and generalist traits such as a high growth rate and capacity for asexual reproduction through fragmentation (Palacio-Castro et al., 2023). However, in an Australian context, dominance of Pocilloporids on Australia’s reefs will come with a reduction in non-Pocilloporid species in a formerly highly diverse region. This reduction in non-Pocilloporid species diversity may impact ecological functions and reef resilience to other stressors in highly variable, marginal environments.
In subtropical or marginal reefs, such as those around Norfolk Island and Lord Howe Island, the reefs are dominated by subtropical and temperate species and presumed to be isolated from surrounding bioregions. This creates a unique environment with a high presence of endemic fauna and peripheral populations vulnerable to extinction (van der Meer et al., 2015; Edgar et al., 2017). However, there has been limited research to date focused on coral populations in these subtropical environments, and how generalist species and the overall coral populations will be affected by warming oceans is largely unknown. At Lord Howe Island between 2005 and 2007 up to 95% of the coral community in the lagoon bleached, with 41% mortality occurring in Pocillopora damicornis as well as evidence of minor bleaching in Poritidae and Acroporidae (Dalton et al., 2011). In 2010, 2011 and 2019, bleaching was also observed in lagoonal sites at Lord Howe Island, with up to 99% of colonies bleached at shallow lagoon sites and Pocillopora, Stylophora, Seriatopora, and Porites as the most affected species (Dalton et al., 2020). The responses of Pocilloporids to warming are clearly variable across environments.
In temperate reefs, such as the continental coral reefs of the eastern extent of the Great Southern Reef (GSR) including the Solitary Islands Marine Park (SIMP) and the associated reefs of Australia’s southeast coast, ocean warming is contributing to range contractions, habitat losses, changes in energy pathways, and the facilitation of species (Wernberg et al., 2013; Vergés et al., 2014; Tuckett et al., 2017; Smith et al., 2021). In surveys of a bleaching event extending along the eastern coast of Australia from Flinders Reef to Southwest Rocks (including the SIMP), Pocillopora bleached more at sites where temperatures were historically cooler and overall, Pocillopora and Porites were more susceptible to bleaching than Acropora, Goniastrea, and Turbinaria (Kim et al., 2019). Bleaching severity also increased for Pocillopora spp. with latitude without a significant change in relative abundance (Kim et al., 2019). Abrupt changes to the size structure of the endemic Pocillopora aliciae were also noted following a bleaching event in 2016, demonstrating a strong association between heat stress and declining P. aliciae population density (Lachs et al., 2021). P. aliciae is a subtropical endemic branching coral (Schmidt-Roach et al., 2013a; Schmidt-Roach et al., 2013b; Schmidt-Roach et al., 2014), which is hypothesised to have extended its range into temperate (18.8–24.7°C 2012–2022 monthly average) habitats of Sydney NSW (Booth and Sear, 2018). Establishment of P. aliciae in temperate waterways has been suggested to accelerate tropicalization of coastal Sydney and facilitate benthic habitat complexity for fish communities, although further examination is needed to determine the limits of coral range extension into high-latitude regions (Abrego et al., 2021). However, despite generally exhibiting an enhanced stress tolerance characteristic of corals in a fluctuating environment (Oliver and Palumbi, 2011), P. aliciae has been deemed the least viable coral species under thermal stress at the SIMP (Cant et al., 2021) and is categorised as susceptible to thermal stress.
This is further evident with the 2016 El Niño and a corresponding increase in seawater temperatures of > 2°C above the long-term mean summer maxima causing bleaching in up to 60% of all corals in Sydney Harbour (Goyen et al., 2019). Incorporating the response of Pocilloporid species under thermal stress into the response of the benthic coral community as a whole, there is evidence of an overall decline in total coral cover at the SIMP between 1990 and 2014 (Mizerek et al., 2021). Taken together, these studies suggest that the response of Pocilloporid species to thermal stress within the reefs of eastern Australia are still equivocal and highly variable by region, and likely depend on location-specific adaptations.
5 Conclusion
Community reassembly, altered dispersal patterns, and novel species interactions, including novel holobiont–environment interactions, are hypothesised to be involved in the formation of entirely novel ecosystems (Spalding et al., 2007; Sommer et al., 2014; Sommer et al., 2017; Sommer et al., 2018). Ocean warming is hypothesised to warm regions considered marginal environments for coral growth and expand coral dominance in these locations (Beger et al., 2014; Tuckett et al., 2017; Booth and Sear, 2018; Nakabayashi et al., 2019; González-Pech et al., 2022). As such, recent studies have focused on how ocean warming may drive the development of novel coral ecosystems in high-latitude reef habitats, described as “changes in species configurations, interactions, and functions, within the parameter space of calcifying coral-dominated reefs” (Graham et al., 2014).
However, warming is also driving marine heatwaves and bleaching events, as seen in the past decades across Australia’s subtropical and temperate reef systems and coral populations. In Australia alone, coral bleaching has been observed in high-latitude locations within the last two decades with a frequency and severity comparable to that of tropical locations. In Australia bleaching events have been reported at Lord Howe Island (Harrison et al., 2011; Dalton et al., 2020), Rottnest Island (Thomson et al., 2011; le Nohaïc et al., 2017), eastern New South Wales (Kim et al., 2019), Sydney Harbour (Goyen et al., 2019), and the Houtman Abrolhos Islands (Abdo et al., 2012; Smale et al., 2012). One of the most extreme examples of changing distribution is the expansion of the coral Pocillopora aliciae into temperate marine environments close to Sydney Harbour (González-Pech et al., 2022). It is therefore critical to understand which coral species, in which locations and by which means, are functioning as generalists as these species are predicted to be better able to withstand a changing environment. Generalist species may provide new ecological opportunities and play critical functional roles as coral reefs reconfigure under climate change. More research is warranted to define generalist traits and which species display them.
Author contributions
JB reviewed the literature and wrote the manuscript with contribution from TA, PS, and ZR. All authors contributed to the article and approved the submitted version.
Funding
This project was supported by a UNSW Scientia Scholarship, Australian Government Research Training Program Scholarship, and the American Australian Association’s Graduate Education Fund Scholarship to JB. In addition, TA was supported by UNSW Scientia Funding and research conducted with Parks Australia.
Conflict of interest
The authors declare that the research was conducted in the absence of any commercial or financial relationships that could be construed as a potential conflict of interest.
Publisher’s note
All claims expressed in this article are solely those of the authors and do not necessarily represent those of their affiliated organizations, or those of the publisher, the editors and the reviewers. Any product that may be evaluated in this article, or claim that may be made by its manufacturer, is not guaranteed or endorsed by the publisher.
References
Abdo D. A., Bellchambers L. M., Evans S. N. (2012). Turning up the heat: increasing temperature and coral bleaching at the high latitude coral reefs of the Houtman Abrolhos Islands. PloS One 7, e43878. doi: 10.1371/journal.pone.0043878
Abrego D., Howells E. J., Smith S. D. A., Madin J. S., Sommer B., Schmidt-Roach S., et al. (2021). Factors limiting the range extension of corals into high-latitude reef regions. Diversity 13, 632. doi: 10.3390/d13120632
AIMS (2021). Annual summary report of coral reef condition 2020/2021. Townsville, QLD: Australian Institute of Marine Science.
AIMS (2022). Annual summary report of coral reef condition 2021/2022. Townsville, QLD: Australian Institute of Marine Science.
Ainsworth T. D., Leggat W., Silliman B. R., Lantz C. A., Bergman J. L., Fordyce A. J., et al. (2021). Rebuilding relationships on coral reefs: Coral bleaching knowledge-sharing to aid adaptation planning for reef users. BioEssays 43, 1–9. doi: 10.1002/bies.202100048
Aranda M., Li Y., Liew Y. J., Baumgarten S., Simakov O., Wilson M. C., et al. (2016). Genomes of coral dinoflagellate symbionts highlight evolutionary adaptations conducive to a symbiotic lifestyle. Sci. Rep. 6, 39734. doi: 10.1038/srep39734
Bairos-Novak K. R., Hoogenboom M. O., van Oppen M. J. H., Connolly S. R. (2021). Coral adaptation to climate change: Meta-analysis reveals high heritability across multiple traits. Glob Chang Biol. 27, 5694–5710. doi: 10.1111/gcb.15829
Beger M., Sommer B., Harrison P. L., Smith S. D. A., Pandolfi J. M. (2014). Conserving potential coral reef refuges at high latitudes. Divers. Distrib 20, 245–257. doi: 10.1111/ddi.12140
Benayahu Y., Bridge T. C. L., Colin P. L., Liberman R., McFadden C. S., Pizarro O., et al. (2019). Octocorals of the Indo-Pacific. Mesophotic Coral Ecosyst., 709–728. doi: 10.1007/978-3-319-92735-0_38
Bergman J. L., Leggat W., Ainsworth T. D. (2021). The meta-organism response of the environmental generalist Pocillopora damicornis exposed to differential accumulation of heat stress. Front. Mar. Sci. 8. doi: 10.3389/fmars.2021.664063/
Bloomberg J., Holstein D. M. (2021). Mesophotic coral refuges following multiple disturbances. Coral Reefs 40, 821–834. doi: 10.1007/S00338-021-02087-W
Bollati E., Plimmer D., D’Angelo C., Wiedenmann J. (2017). FRET-mediated long-range wavelength transformation by photoconvertible fluorescent proteins as an efficient mechanism to generate orange-red light in symbiotic deep water corals. Int. J. Mol. Sci. 18, 1174. doi: 10.3390/IJMS18071174
Bongaerts P., Carmichael M., Hay K. B., Tonk L., Frade P. R., Hoegh-Guldberg O. (2015a). Prevalent endosymbiont zonation shapes the depth distributions of scleractinian coral species. R Soc. Open Sci. 2, 140297. doi: 10.1098/rsos.140297
Bongaerts P., Frade P. R., Hay K. B., Englebert N., Latijnhouwers K. R. W., Bak R. P. M., et al. (2015b). Deep down on a Caribbean reef: lower mesophotic depths harbor a specialized coral-endosymbiont community. Sci. Rep. 5, 1–9. doi: 10.1038/srep07652
Bongaerts P., Frade P. R., Ogier J. J., Hay K. B., van Bleijswijk J., Englebert N., et al. (2013). Sharing the slope: Depth partitioning of agariciid corals and associated Symbiodinium across shallow and mesophotic habitats (2-60 m) on a Caribbean reef. BMC Evol. Biol. 13, 1–15. doi: 10.1186/1471-2148-13-205
Bongaerts P., Ridgway T., Sampayo E. M., Hoegh-Guldberg O. (2010). Assessing the “deep reef refugia” hypothesis: focus on Caribbean reefs. Coral Reefs 29, 309–327. doi: 10.1007/S00338-009-0581-X
Bongaerts P., Riginos C., Brunner R., Englebert N., Smith S. R., Hoegh-Guldberg O. (2017). Deep reefs are not universal refuges: Reseeding potential varies among coral species. Sci. Adv. 3, e1602373. doi: 10.1126/sciadv.1602373
Bongaerts P., Smith T. B. (2019). Beyond the “Deep Reef Refuge” hypothesis: a conceptual framework to characterize persistence at depth. Coral Reefs World 12, 881–895. doi: 10.1007/978-3-319-92735-0_45
Booth D. J., Sear J. (2018). Coral expansion in Sydney and associated coral-reef. fishes. Coral Reefs 37, 995. doi: 10.1007/S00338-018-1727-5
Boström-Einarsson L., Babcock R. C., Bayraktarov E., Ceccarelli D., Cook N., Ferse S. C. A., et al. (2020). Coral restoration – A systematic review of current methods, successes, failures and future directions. PloS One 15, e0226631. doi: 10.1371/journal.pone.0226631
Botté E. S., Cantin N. E., Mocellin V. J., O’Brien P. A., Rocker M. M., Frade P. R., et al. (2022). Reef location has a greater impact than coral bleaching severity on the microbiome of Pocillopora acuta. Coral Reefs 41, 63–79. doi: 10.1007/s00338-021-02201-y
Brandl S., Rasher D., Côté I., Casey J., Darling E., Lefcheck J., et al. (2019). Coral reef ecosystem functioning: eight core processes and the role of biodiversity. Front. Ecol. Environ. 17, 445–454. doi: 10.1002/fee.2088
Brandtneris V. W., Brandt M. E., Glynn P. W., Gyory J., Smith T. B. (2016). Seasonal variability in calorimetric energy content of two caribbean mesophotic corals. PloS One 11, e0151953. doi: 10.1371/journal.pone.0151953
Brener-Raffalli K., Clerissi C., Vidal-Dupiol J., Adjeroud M., Bonhomme F., Pratlong M., et al. (2018). Thermal regime and host clade, rather than geography, drive Symbiodinium and bacterial assemblages in the scleractinian coral Pocillopora damicornis sensu lato. Microbiome 6, 39. doi: 10.1186/s40168-018-0423-6
Bridge T. C. L., Luiz O. J., Kuo C. Y., Precoda K., Madin E. M. P., Madin J. S., et al. (2020). Incongruence between life-history traits and conservation status in reef corals. Coral Reefs 39, 271–279. doi: 10.1007/S00338-019-01885-7
Bruno J. F., Selig E. R. (2007). Regional decline of coral cover in the Indo-Pacific: Timing, extent, and subregional comparisons. PloS One 2, e711. doi: 10.1371/journal.pone.0000711
Brustolin M. C., Nagelkerken I., Ferreira C. M., Goldenberg S. U., Ullah H., Fonseca G. (2019). Future ocean climate homogenizes communities across habitats through diversity loss and rise of generalist species. Glob Chang Biol. 25, 3539–3548. doi: 10.1111/gcb.14745
Burgess S. C., Johnston E. C., J Wyatt A. S., Leichter J. J., Edmunds P. J., Johnston E. C., et al. (2021). Response diversity in corals: hidden differences in bleaching mortality among cryptic Pocillopora species. Ecology 102, e03324. doi: 10.1002/ecy.3324
Burn D., Matthews S., Pisapia C., Hoey A., Pratchett M. (2022). Changes in the incidence of coral injuries during mass bleaching across Australia’s Coral Sea Marine Park. Mar. Ecol. Prog. Ser. 682, 97–109. doi: 10.3354/meps13935
Burt J. A., Camp E. F., Enochs I. C., Johansen J. L., Morgan K. M., Riegl B., et al. (2020). Insights from extreme coral reefs in a changing world. Coral Reefs 39, 495–507. doi: 10.1007/S00338-020-01966-Y
Caballero Aragón H., Armenteros M., Perera Valderrama S., Rey Villiers N., Cobián Rojas D., Campos Verdecia K., et al. (2019). Ecological condition of coral reef assemblages in the Cuban Archipelago. Mar. Biol. Rsrch 15, 61–73. doi: 10.1080/17451000.2019.1577557
Cacciapaglia C., van Woesik R. (2015). Reef-coral refugia in a rapidly changing ocean. Glob Chang Biol. 21, 2272–2282. doi: 10.1111/gcb.12851
Cairns S. D. (2007). Deep-water corals: an overview with special reference to diversity and distribution of deep-water scleractinian corals. B Mar. Sci. 81, 311–322.
Camp E., Edmondson J., Doheny A., Rumney J., Grima A., Huete A., et al. (2019). Mangrove lagoons of the Great Barrier Reef support coral populations persisting under extreme environmental conditions. Mar. Ecol. Prog. Ser. 625, 1–14. doi: 10.3354/meps13073
Camp E. F., Schoepf V., Mumby P. J., Hardtke L. A., Rodolfo-Metalpa R., Smith D. J., et al. (2018). The future of coral reefs subject to rapid climate change: lessons from natural extreme environments. Front. Mar. Sci. 5. doi: 10.3389/fmars.2018.00004
Cant J., Salguero-Gómez R., Kim S. W., Sims C. A., Sommer B., Brooks M., et al. (2021). The projected degradation of subtropical coral assemblages by recurrent thermal stress. J. Anim. Ecol. 90, 233–247. doi: 10.1111/1365-2656.13340
Claar D. C., Fabina N. S., Putnam H. M., Cunning R., Sogin E., Baum J. K., et al. (2017). Embracing complexity in coral–algal symbioses. Algal Cyanobacteria Symbioses, 467–492. doi: 10.1142/9781786340580_0015
Chichorro F., Juslén A., Cardoso P. (2019). A review of the relation between species traits and extinction risk. Biol. Conserv. 237, 220–229. doi: 10.1016/j.biocon.2019.07.001
Chuang P. S., Mitarai S. (2020). Signaling pathways in the coral polyp bail-out response. Coral Reefs 39, 1535–1548. doi: 10.1007/S00338-020-01983-X
Clavel J., Julliard R., Devictor V. (2011). Worldwide decline of specialist species: toward a global functional homogenization? Front. Ecol. Environ. 9, 222–228. doi: 10.1890/080216
Connell J. H., Hughes T. P., Wallace C. C., Tanner J. E., Harms K. E., Kerr A. M. (2004). A long-term study of competition and diversity of corals. Ecol. Monogr. 74, 179–210. doi: 10.1890/02-4043
Cornwall C. E., Comeau S., Kornder N. A., Perry C. T., van Hooidonk R., DeCarlo T. M., et al. (2021). Global declines in coral reef calcium carbonate production under ocean acidification and warming. P Natl. Acad. Sci. 118, e2015265118. doi: 10.1073/pnas.2015265118
Cornwall C. E., Comeau S., Putnam H., Schoepf V. (2022). Impacts of ocean warming and acidification on calcifying coral reef taxa: mechanisms responsible and adaptive capacity. Emerg. Top. Life Sci. 6, 1–9. doi: 10.1042/etls20210226
Corso J., French B., Edwards C., Pedersen N., Zgliczynski B., Planes S., et al. (2022). Non-random orientation of Pocillopora colonies on forereefs of Moorea, French Polynesia. Mar. Ecol. Prog. Ser. 693, 177-182. doi: 10.3354/meps14078
Courtney T. A., Barnes B. B., Chollett I., Elahi R., Gross K., Guest J. R., et al. (2020). Disturbances drive changes in coral community assemblages and coral calcification capacity. Ecosphere 11, e03066. doi: 10.1002/ecs2.3066
Courtney T. A., Lebrato M., Bates N. R., Collins A., de Putron S. J., Garley R., et al. (2017). Environmental controls on modern scleractinian coral and reef-scale calcification. Sci. Adv. 3. doi: 10.1126/sciadv.1701356
Cunning R., Glynn P. W., Baker A. C. (2013). Flexible associations between Pocillopora corals and Symbiodinium limit utility of symbiosis ecology in defining species. Coral Reefs 32, 795–801. doi: 10.1007/S00338-013-1036-Y
Dalin P., Kindvall O., Björkman C. (2009). Reduced population control of an insect pest in managed willow monocultures. PloS One 4, e5487. doi: 10.1371/journal.pone.0005487
Dalton S. J., Carroll A. G., Dalton S. J., Carroll A. G. (2011). Monitoring coral health to determine coral bleaching response at high latitude eastern Australian reefs: an applied model for a changing climate. Diversity 3, 592–610. doi: 10.3390/d3040592
Dalton S. J., Carroll A. G., Sampayo E., Roff G., Harrison P. L., Entwistle K., et al. (2020). Successive marine heatwaves cause disproportionate coral bleaching during a fast phase transition from El Niño to La Niña. Sci. Total Environ. 715, 136951. doi: 10.1016/j.scitotenv.2020.136951
Darling E. S., Alvarez-Filip L., Oliver T. A., McClanahan T. R., Côté I. M. (2012). Evaluating life-history strategies of reef corals from species traits. Ecol. Lett. 15, 1378–1386. doi: 10.1111/j.1461-0248.2012.01861.x
Darling E. S., McClanahan T. R., Maina J., Gurney G. G., Graham N. A. J., Januchowski-Hartley F., et al. (2019). Social–environmental drivers inform strategic management of coral reefs in the Anthropocene. Nat. Ecol. Evo. 3, 1341–1350. doi: 10.1038/s41559-019-0953-8
de Bakker D. M., Meesters E. H., Bak R. P. M., Nieuwland G., van Duyl F. C. (2016). Long-term shifts in coral communities on shallow to deep reef slopes of Curaçao and Bonaire: Are there any winners? Front. Mar. Sci. 3. doi: 10.3389/fmars.2016.00247
Deignan L. K., McDougald D. (2022). Differential response of the microbiome of Pocillopora acuta to reciprocal transplantation within Singapore. Microb. Ecol. 83, 608–618. doi: 10.1007/s00248-021-01793-w
Dennis R. L. H., Dapporto L., Fattorini S., Cook L. M. (2011). The generalism–specialism debate: the role of generalists in the life and death of species. Biol. J. Linn Soc. 104, 725–737. doi: 10.1111/J.1095-8312.2011.01789.X
Dietzel A., Bode M., Connolly S. R., Hughes T. P. (2020). Long-term shifts in the colony size structure of coral populations along the Great Barrier Reef. P Roy Soc. B-Biol Sci. 287, 20201432. doi: 10.1098/rspb.2020.1432
Dietzel A., Connolly S. R., Hughes T. P., Bode M. (2021). The spatial footprint and patchiness of large-scale disturbances on coral reefs. Glob Chang Biol. 27, 4825–4838. doi: 10.1111/gcb.15805
Donovan M. K., Burkepile D. E., Kratochwill C., Shlesinger T., Sully S., Oliver T. A., et al. (2021). Local conditions magnify coral loss after marine heatwaves. Science 372, 977–980. doi: 10.1126/science.abd9464
Eckert R. J., Studivan M. S., Voss J. D. (2019). Populations of the coral species Montastraea cavernosa on the Belize Barrier Reef lack vertical connectivity. Sci. Rep. 9, 1–11. doi: 10.1038/s41598-019-43479-x
Eddy T. D., Lam V. W. Y., Reygondeau G., Cisneros-Montemayor A. M., Greer K., Palomares M. L. D., et al. (2021). Global decline in capacity of coral reefs to provide ecosystem services. One Earth 4, 1278–1285. doi: 10.1016/j.oneear.2021.08.016
Edgar C. J., Ceccarelli G. J., Stuart-Smith D. D., Cooper R. D. (2017). Biodiversity survey of the temperate east coast commonwealth marine reserve network: Elizabeth & Middleton reefs, Lord Howe island & Norfolk island. Reef Life Survey Foundation Incorporated.
Edgar G. J., Davey A., Kelly G., Mawbey R. B., Parsons K. (2010). Biogeographical and ecological context for managing threats to coral and rocky reef communities in the Lord Howe Island Marine Park, South-Western Pacific. Aquat Conserv. 20, 378–396. doi: 10.1002/aqc.1075
Epstein H. E., Torda G., van Oppen M. J. H. (2019). Relative stability of the Pocillopora acuta microbiome throughout a thermal stress event. Coral Reefs 38, 373–386. doi: 10.1007/S00338-019-01783-Y
Eyal G., Cohen I., Eyal-Shaham L., Ben-Zvi O., Tikochinski Y., Loya Y. (2019a). Photoacclimation and induction of light-enhanced calcification in the mesophotic coral Euphyllia paradivisa. R Soc. Open Sci. 6. doi: 10.1098/rsos.180527
Eyal G., Eyal-Shaham L., Loya Y. (2021). Symbiodiniaceae conduct under natural bleaching stress during advanced gametogenesis stages of a mesophotic coral. Coral Reefs 40, 959–964. doi: 10.1007/S00338-021-02082-1
Eyal G., Tamir R., Kramer N., Eyal-Shaham L., Loya Y. (2019b). The Red Sea: Israel. Coral Reefs World 12, 199–214. doi: 10.1007/978-3-319-92735-0_11
Fabina N. S., Putnam H. M., Franklin E. C., Stat M., Gates R. D. (2012). Transmission mode predicts specificity and interaction patterns in coral-symbiodinium networks. PloS One 7, e44970. doi: 10.1371/journal.pone.0044970
Fabina N. S., Putnam H. M., Franklin E. C., Stat M., Gates R. D. (2013). Symbiotic specificity, association patterns, and function determine community responses to global changes: defining critical research areas for coral-Symbiodinium symbioses. Glob Chang Biol. 19, 3306–3316. doi: 10.1111/gcb.12320
Feldman B., Shlesinger T., Loya Y. (2018). Mesophotic coral-reef environments depress the reproduction of the coral Paramontastraea peresi in the Red Sea. Coral Reefs 37, 201–214. doi: 10.1007/S00338-017-1648-8
Fordyce A., Camp E., Ainsworth T. D. (2017). Polyp bailout in Pocillopora damicornis following thermal stress. F1000 Res. 6, 687. doi: 10.12688/f1000research.11522.2
Fox H. E., Harris J. L., Darling E. S., Ahmadia G. N., Estradivari, Razak T. B. (2019). Rebuilding coral reefs: success (and failure) 16 years after low-cost, low-tech restoration. Restor. Ecol. 27, 862–869. doi: 10.1111/rec.12935
GBRMPA. (2017). Final report: 2016 coral bleaching event on the Great Barrier Reef. Townsville, QLD: Great Barrier Reef Marine Park Authority.
Garavelli L., Studivan M. S., Voss J. D., Kuba A., Figueiredo J., Chérubin L. M. (2018). Assessment of mesophotic coral ecosystem connectivity for proposed expansion of a marine sanctuary in the Northwest Gulf of Mexico: Larval dynamics. Front. Mar. Sci. 5. doi: 10.3389/fmars.2018.00174
Gélin P., Fauvelot C., Bigot L., Baly J., Magalon H. (2018). From population connectivity to the art of striping Russian dolls: the lessons from Pocillopora corals. Ecol. Evol. 8, 1411–1426. doi: 10.1002/ece3.3747
Gilbert J. A., Hill R., Doblin M. A., Ralph P. J. (2012). Microbial consortia increase thermal tolerance of corals. Mar. Biol. 159, 1763–1771. doi: 10.1007/S00227-012-1967-9
González-Pech R., Hughes D., Strudwick P., Lewis B., Booth D., Figueira W., et al. (2022). Physiological factors facilitating the persistence of Pocillopora aliciae and Plesiastrea versipora in temperate reefs of south-eastern Australia under ocean warming. Coral Reefs 41, 1239–1253. doi: 10.1007/S00338-022-02277-0
Gösser F., Raulf A., Mosig A., Tollrian R., Schweinsberg M. (2021). Signaling pathways of heat- and hypersalinity-induced polyp bailout in Pocillopora acuta. Coral Reefs 40, 1713–1728. doi: 10.1007/S00338-021-02191-X
Goodbody-Gringley G., Waletich J. (2018). Morphological plasticity of the depth generalist coral, Montastraea cavernosa, on mesophotic reefs in Bermuda. Ecology 99, 1688–1690. Available at: https://www.jstor.org/stable/26625782.
Goyen S., Camp E. F., Fujise L., Lloyd A., Nitschke M. R., LaJeunensse T., et al. (2019). Mass coral bleaching of P. versipora in Sydney Harbour driven by the 2015–2016 heatwave. Coral Reefs 38, 815–830. doi: 10.1007/S00338-019-01797-6
Graham N. A., Cinner J. E., Norström A. V., Nyström M. (2014). Coral reefs as novel ecosystems: embracing new futures. Curr. Opin. Environ. Sustain 7, 9–14. doi: 10.1016/j.cosust.2013.11.023
Graham N. A. J., Jennings S., MacNeil M. A., Mouillot D., Wilson S. K. (2015). Predicting climate-driven regime shifts versus rebound potential in coral reefs. Nature 518, 94–97. doi: 10.1038/nature14140
Graham N. A. J., Wilson S. K., Jennings S., Polunin N. V. C., Bijoux J. P., Robinson J. (2006). Dynamic fragility of oceanic coral reef ecosystems. P Natl. Acad. Sci. 103, 8425–8429. doi: 10.1073/pnas.0600693103
Great Barrier Reef Marine Park Authority. (2012). Great Barrier Reef general reference map. Available at: http://hdl.handle.net/11017/869.
Grimsditch G., Pisapia C., Huck M., Karisa J., Obura D., Sweet M. (2017). Variation in size frequency distribution of coral populations under different fishing pressures in two contrasting locations in the Indian Ocean. Mar. Environ. Res. 131, 146–155. doi: 10.1016/j.marenvres.2017.09.017
Guest J. R., Tun K., Low J., Vergés A., Marzinelli E. M., Campbell A. H., et al. (2016). 27 years of benthic and coral community dynamics on turbid, highly urbanised reefs off Singapore. Sci. Rep. 6, 1–10. doi: 10.1038/srep36260
Hamylton S. M., Hutchings P., Hoegh-Guldberg (Eds.) (2022). Coral reefs of Australia: perspectives from beyond the water’s edge. (Australia: CSIRO Publishing).
Harii S., Hongo C., Ishihara M., Ide Y., Kayanne H., Kayanne H. (2014). Impacts of multiple disturbances on coral communities at Ishigaki Island, Okinawa, Japan, during a 15 year survey. Mar. Ecol. Prog. Ser. 509, 171–180. doi: 10.3354/meps10890
Harriott V. J., Banks S. A. (1995). Recruitment of scleractinian corals in the Solitary Islands Marine Reserve, a high latitude coral-dominated community in Eastern Australia. Mar. Ecol. Prog. Ser. 123, 155–161. doi: 10.3354/meps123155
Harriott V. J., Harrison P. L., Banks S. A. (1995). The coral communities of Lord Howe Island. Mar. Freshw. Res. 46, 457–465. doi: 10.1071/mf9950457
Harriott V., Smith S. (2002). Coral population dynamics in a subtropical coral community, Solitary Islands Marine Park, Australia. Proc. 9th Int. Coral Reef Symposium 1, 573–581.
Harrison P., Dalton S., Carroll A. (2011). Extensive coral bleaching on the world’s southernmost coral reef at Lord Howe Island, Australia. Coral Reefs 30, 775. doi: 10.1007/s00338-011-0778-7
Haryanti D., Yasuda N., Harii S., Hidaka M. (2015). High tolerance of symbiotic larvae of Pocillopora damicornis to thermal stress. Zool Stud. 54, 52. doi: 10.1186/s40555-015-0134-7
Hernandez-Agreda A., Leggat W., Ainsworth T. D. (2018). A comparative analysis of microbial DNA preparation methods for use with massive and branching coral growth forms. Front. Microbiol. 9. doi: 10.3389/fmicb.2018.02146
Hernandez-Agreda A., Leggat W., Bongaerts P., Ainsworth T. D. (2016). The microbial signature provides insight into the mechanistic basis of coral success across reef habitats. MBio 4, e00560–e00516. doi: 10.1128/mBio.00560-16
Holstein D. M., Paris C. B., Vaz A. C., Smith T. B. (2016). Modeling vertical coral connectivity and mesophotic refugia. Coral Reefs 35, 23–37. doi: 10.1007/S00338-015-1339-2
Holstein D. M., Smith T. B., Gyory J., Paris C. B. (2015). Fertile fathoms: Deep reproductive refugia for threatened shallow corals. Sci. Rep. 5, 1–12. doi: 10.1038/srep12407
Hughes T. P., Kerry J. T., Álvarez-Noriega M., Álvarez-Romero J. G., Anderson K. D., Baird A. H., et al. (2017). Global warming and recurrent mass bleaching of corals. Nature 543, 373–377. doi: 10.1038/nature21707
Hughes T. P., Kerry J. T., Baird A. H., Connolly S. R., Dietzel A., Eakin C. M., et al. (2018). Global warming transforms coral reef assemblages. Nature 556, 492–496. doi: 10.1038/s41586-018-0041-2
Hughes T., Kerry J., Connolly S., Álvarez-Romero J. (2021). Emergent properties in the responses of tropical corals to recurrent climate extremes. Curr. Biol. 23, 5393–5399. doi: 10.1016/j.cub.2021.10.046
Januchowski-Hartley F. A., Bauman A. G., Morgan K. M., Seah J. C. L., Huang D., Todd P. A. (anda2020a). Accreting coral reefs in a highly urbanized environment. Coral Reefs 39, 717–731. doi: 10.1007/S00338-020-01953-3/
Jackson J. B. C. (1991). Adaptation and diversity of reef corals. Bioscience 41, 475–482. doi: 10.2307/1311805
Johnston E. C., Forsman Z. H., Flot J. F., Schmidt-Roach S., Pinzón J. H., Knapp I. S. S., et al. (2017). A genomic glance through the fog of plasticity and diversification in Pocillopora. Sci. Rep. 7, 1–11. doi: 10.1038/s41598-017-06085-3
Johnston E. C., Wyatt A. S. J., Leichter J. J., Burgess S. C. (2022). Niche differences in co-occurring cryptic coral species (Pocillopora spp.). Coral Reefs 41, 767–778. doi: 10.1007/S00338-021-02107-9
Kaandorp J. A. (1999). Morphological analysis of growth forms of branching marine sessile organisms along environmental gradients. Mar. Biol. 134, 295–306. doi: 10.1007/S002270050547
Kahng S. E., Akkaynak D., Shlesinger T., Hochberg E. J., Wiedenmann J., Tamir R., et al. (2019). Light, temperature, photosynthesis, heterotrophy, and the lower depth limits of mesophotic coral ecosystems. Mesophotic Coral Ecosyst. (Cham), 801–828. doi: 10.1007/978-3-319-92735-0_42
Kahng S., Copus J., Wagner D. (2017). Mesophotic coral ecosystems. Mar. Anim. Forests 10, 978. doi: 10.1007/978-3-319-17001-5_4-1
Karisa J. F., Obura D. O., Chen C. A. (2020). Spatial heterogeneity of coral reef benthic communities in Kenya. PloS One 15, e0237397. doi: 10.1371/journal.pone.0237397
Kennedy E., Ordoñez A., Diaz-Pulido G. (2017). Coral bleaching in the southern inshore Great Barrier Reef: a case study from the Keppel Islands. Mar. Freshw. Res. 69, 191–197. doi: 10.1071/MF16317
Kim S. W., Sampayo E. M., Sommer B., Sims C. A., Gómez-Cabrera M., del C., et al. (2019). Refugia under threat: Mass bleaching of coral assemblages in high-latitude eastern Australia. Glob Chang Biol. 25, 3918–3931. doi: 10.1111/gcb.14772
Kimball S., Funk J. L., Sandquist D. R., Ehleringer J. R. (2016). “Ecophysiological considerations for restoration,” in Foundations of restoration ecology (Washington, DC: Island Press/Center for Resource Economics), 153–181. doi: 10.5822/978-1-61091-698-16
Knowlton N. (2001). The future of coral reefs. Proc. Natl. Acad. Sci. U.S.A. 98, 5419–5425. doi: 10.1073/pnas.091092998
Kramer N., Guan J., Chen S., Wangpraseurt D., Loya Y. (2021a). Characterization of morpho-functional traits in mesophotic corals reveals optimized light capture and photosynthesis. bioRxiv. doi: 10.1101/2021.09.29.462347
Kramer N., Tamir R., Ben-Zvi O., Jacques S. L., Loya Y., Wangpraseurt D. (2021b). Efficient light-harvesting of mesophotic corals is facilitated by coral optical traits. Funct. Ecol. 36, 406–418. doi: 10.1111/1365-2435.13948
Kramer N., Tamir R., Eyal G., Loya Y. (2020). Coral morphology portrays the spatial distribution and population size-structure along a 5–100 m depth gradient. Front. Mar. Sci. 7. doi: 10.3389/fmars.2020.00615
Lachs L., Sommer B., Cant J., Hodge J. M., Malcolm H. A., Pandolfi J. M., et al. (2021). Linking population size structure, heat stress and bleaching responses in a subtropical endemic coral. Coral Reefs 40, 777–790. doi: 10.1007/S00338-021-02081-2
Lavm B., Nevo E. (1981). Genetic diversity in marine molluscs: a test of the niche-width variation hypothesis. Mar. Ecol. 2, 335–342. doi: 10.1111/J.1439-0485.1981.TB00275.X
Lawton R. J., Messmer V., Pratchett M. S., Bay L. K. (2011). High gene flow across large geographic scales reduces extinction risk for a highly specialised coral feeding butterflyfish. Mol. Ecol. 20, 3584–3598. doi: 10.1111/J.1365-294X.2011.05207.X
Laverick J. H., Green T. K., Burdett H. L., Newton J., Rogers A. D. (2019). Depth alone is an inappropriate proxy for physiological change in the mesophotic coral Agaricia lamarcki. J. Mar. Biol. Assoc. United Kingdom 99, 1535–1546. doi: 10.1017/S0025315419000547
le Nohaïc M., Ross C. L., Cornwall C. E., Comeau S., Lowe R., McCulloch M. T., et al. (2017). Marine heatwave causes unprecedented regional mass bleaching of thermally resistant corals in northwestern Australia. Sci. Rep. 7, 1–11. doi: 10.1038/s41598-017-14794-y
Lesser M., Weis V., Patterson M., Jokiel P. (1994). Effects of morphology and water motion on carbon delivery and productivity in the reef coral, Pocillopora damicornis (Linnaeus): diffusion barriers, inorganic carbon. J. Exp. Mar. Biol. Ecol. 178, 153–179. doi: 10.1016/0022-0981(94)90034-5
Lesser M. P., Slattery M., Laverick J. H., Macartney K. J., Bridge T. C. (2019). Global community breaks at 60 m on mesophotic coral reefs. Global Ecol. Biogeography 28, 1403–1416. doi: 10.1111/geb.12940
Lin B. (2011). Resilience in agriculture through crop diversification: adaptive management for environmental change. Bioscience 61, 183–193. doi: 10.1525/bio.2011.61.3.4
Linklater M., Carroll A. G., Hamylton S. M., Jordan A. R., Brooke B. P., Nichol S. L., et al. (2016). High coral cover on a mesophotic, subtropical island platform at the limits of coral reef growth. Cont Shelf Res. 130, 34–46. doi: 10.1016/j.csr.2016.10.003
Linklater M., Ingleton T. C., Kinsela M. A., Morris B. D., Allen K. M., Sutherland M. D., et al. (2019). Techniques for classifying seabed morphology and composition on a subtropical-temperate continental shelf. Geosci J. 9, 141. doi: 10.3390/geosciences9030141
Linklater M., Jordan B A. R., Carroll A. G., Neilson J., Gudge B S., Brooke B. P., et al. (2018). Mesophotic corals on the subtropical shelves of Lord Howe Island and Balls Pyramid, south-western Pacific Ocean. Mar. Freshw. Res. 70, 43–61. doi: 10.1071/mf18151
Loya Y., Eyal G., Treibitz T., Lesser M. P., Appeldoorn R. (2016). Theme section on mesophotic coral ecosystems: advances in knowledge and future perspectives. Coral Reefs 35, 1–9. doi: 10.1007/S00338-016-1410-7
Mies M., Francini-Filho R. B., Zilberberg C., Garrido A. G., Longo G. O., Laurentino E., et al. (2020). South Atlantic coral reefs are major global warming refugia and less susceptible to bleaching. Front. Mar. Sci. 7. doi: 10.3389/fmars.2020.00514
Maher R. L., Rice M. M., McMinds R., Burkepile D. E., Vega Thurber R. (2019). Multiple stressors interact primarily through antagonism to drive changes in the coral microbiome. Sci. Rep. 9, 1–12. doi: 10.1038/s41598-019-43274-8
Maher R. L., Schmeltzer E. R., Meiling S., McMinds R., Ezzat L., Shantz A. A., et al. (2020). Coral microbiomes demonstrate flexibility and resilience through a reduction in community diversity following a thermal stress event. Front. Ecol. Evol. 8. doi: 10.3389/fevo.2020.555698
Marhoefer S. R., Zenger K. R., Strugnell J. M., Logan M., van Oppen M. J., Kenkel C. D., et al. (2021). Signatures of adaptation and acclimatization to reef flat and slope habitats in the coral Pocillopora damicornis. Front. Mar. Sci. 8. doi: 10.3389/fmars.2021.704709
Martinez S., Kolodny Y., Shemesh E., Scucchia F., Nevo R., Levin-Zaidman S., et al. (2020). Energy sources of the depth-generalist mixotrophic coral Stylophora pistillata. Front. Mar. Sci. 7, 566663. doi: 10.3389/fmars.2020.566663
McClanahan T. R., Ateweberhan M., Graham N. A. J., Wilson S. K., Sebastián C. R., Guillaume M. M., et al. (2007). Western Indian Ocean coral communities: bleaching responses and susceptibility to extinction. Mar. Ecol. Prog. Ser. 337, 1–13. doi: 10.3354/meps337001
McGinley M., Aschaffenburg M., Pettay D., Smith R., LaJeunesse T., Warner M. (2012). Symbiodinium spp. in colonies of eastern Pacific Pocillopora spp. are highly stable despite the prevalence of low-abundance background populations. Mar. Ecol. Prog. Ser. 462, 1–7. doi: 10.3354/meps09914
McWilliam M., Pratchett M. S., Hoogenboom M. O., Hughes T. P. (2020). Deficits in functional trait diversity following recovery on coral reefs. P Roy Soc. B-Biol Sci. 287, 20192628. doi: 10.1098/rspb.2019.2628
Miller K., Ayre D. (2008). Protection of genetic diversity and maintenance of connectivity among reef corals within marine protected areas. Conserv. Biol. 22, 1245–1254. doi: 10.1111/j.1523-1739.2008.00985.x
Mizerek T. L., Madin J. S., Benzoni F., Huang D., Luiz O. J., Mera H., et al. (2021). No evidence for tropicalization of coral assemblages in a subtropical climate change hot spot. Coral Reefs 40, 1451–1461. doi: 10.1007/S00338-021-02167-X
Muir P. R., Pichon M. (2019). Biodiversity of reef-building, scleractinian corals. Coral Reefs World (Cham), 589–620. doi: 10.1007/978-3-319-92735-0_33
Munday P. (2004). Habitat loss, resource specialization, and extinction on coral reefs. Glob Chang Biol. 10, 1642–1647. doi: 10.1111/j.1365-2486.2004.00839.x
Nakabayashi A., Yamakita T., Nakamura T., Aizawa H., Kitano Y. F., Iguchi A., et al. (2019). The potential role of temperate Japanese regions as refugia for the coral Acropora hyacinthus in the face of climate change. Sci. Rep. 9, 1–12. doi: 10.1038/s41598-018-38333-5
Noreen A. M. E., Harrison P. L., van Oppen M. J. H. (2009). Genetic diversity and connectivity in a brooding reef coral at the limit of its distribution. P Roy Soc. B-Biol Sci. 276, 3927–3935. doi: 10.1098/RSPB.2009.1050
Noreen A. M. E., Schmidt-Roach S., Harrison P. L., van Oppen M. J. H. (2015). Diverse associations among coral host haplotypes and algal endosymbionts may drive adaptation at geographically peripheral and ecologically marginal locations. J. Biogeogr 42, 1639–1650. doi: 10.1111/jbi.12536
Oliver T. A., Palumbi S. R. (2011). Do fluctuating temperature environments elevate coral thermal tolerance? Coral Reefs 30, 429–440. doi: 10.1007/s00338-011-0721-y
Olsson P., Folke C., Hughes T. P. (2008). Navigating the transition to ecosystem-based management of the Great Barrier Reef, Australia. P Natl. Acad. Sci. 105, 9489–9494. doi: 10.1073/PNAS.0706905105
Osman E. O., Smith D. J., Ziegler M., Kürten B., Conrad C., El-Haddad K. M., et al. (2018). Thermal refugia against coral bleaching throughout the northern Red Sea. Global Change Biol. 24, e474–e484. doi: 10.1111/gcb.13895
Palacio-Castro A. M., Smith T. B., Brandtneris V., Baker A. C. (2023). Increased dominance of heat-tolerant symbionts creates resilient coral reefs in near-term ocean warming. Proc Natl Acad Sci 120, e2202388120. doi: 10.1073/pnas.2202388120
Palumbi S., Sandifer P., Allan J., Beck M., Fautin D., Fogarty M., et al. (2009). Managing for ocean biodiversity to sustain marine ecosystem services. Front. Ecol. Environ. 7, 204–211. doi: 10.1890/070135
Pandolfi J. M., Bradbury R. H., Sala E., Hughes T. P., Bjorndal K. A., Cooke R. G., et al. (2003). Global trajectories of the long-term decline of coral reef ecosystems. Science 301, 955–958. doi: 10.1126/science.1085706
Pandolfi J. M., Connolly S. R., Marshall D. J., Cohen A. L. (2011). Projecting coral reef futures under global warming and ocean acidification. Science 333, 418–422. doi: 10.1126/science.1204794
Parasharya D., Padate G. (2013). Status of scleractinian corals of Narara reef in the Gulf of Kachchh, western India. Jalplavit 4, 49–59.
Pérez-Rosales G., Brandl S. J., Chancerelle Y., Siu G., Martinez E., Parravicini V., et al. (2021). Documenting decadal disturbance dynamics reveals archipelago-specific recovery and compositional change on Polynesian reefs. Mar. pollut. Bull. 170, 112659. doi: 10.1016/j.marpolbul.2021.112659
Perry C. T., Larcombe P. (2003). Marginal and non-reef-building coral environments. Coral Reefs 22, 427–432. doi: 10.1007/S00338-003-0330-5
Pinzón J. H., LaJeunesse T. C. (2011). Species delimitation of common reef corals in the genus Pocillopora using nucleotide sequence phylogenies, population genetics and symbiosis ecology. Mol. Ecol. 20, 311–325. doi: 10.1111/j.1365-294X.2010.04939.x
Pogoreutz C., Rädecker N., Cárdenas A., Gärdes A., Wild C., Voolstra C. R. (2018). Dominance of Endozoicomonas bacteria throughout coral bleaching and mortality suggests structural inflexibility of the Pocillopora verrucosa microbiome. Ecol. Evol. 8, 2240–2252. doi: 10.1002/ece3.3830
Polinski J. M., Voss J. D. (2018). Evidence of photoacclimatization at mesophotic depths in the coral-Symbiodinium symbiosis at Flower Garden Banks National Marine Sanctuary and McGrail Bank. Coral Reefs 37, 779–789. doi: 10.1007/S00338-018-1701-2
Poquita-Du R., Goh Y., Huang D., Chou L., Todd P. (2020a). Gene expression and photophysiological changes in Pocillopora acuta coral holobiont following heat stress and recovery. Microorganisms 8, 1227. doi: 10.3390/microorganisms8081227
Poquita-Du R., Huang D., Chou L., Todd P. (2020b). The contribution of stress-tolerant endosymbiotic dinoflagellate Durusdinium to Pocillopora acuta survival in a highly urbanized reef system. Coral Reefs 39, 745–755. doi: 10.1007/s00338-020-01902-0
Pratchett M. S., Trapon M., Berumen M. L., Chong-Seng K. (2011). Recent disturbances augment community shifts in coral assemblages in Moorea, French Polynesia. Coral Reefs 30, 183–193. doi: 10.1007/s00338-010-0678-2
Prober S. M., Byrne M., McLean E. H., Steane D. A., Potts B. M., Vaillancourt R. E., et al. (2015). Climate-adjusted provenancing: a strategy for climate-resilient ecological restoration. Front. Ecol. Evol. 3. doi: 10.3389/fevo.2015.00065
Putnam H. M., Ritson-Williams R., Cruz J. A., Davidson J. M., Gates R. D. (2020). Environmentally-induced parental or developmental conditioning influences coral offspring ecological performance. Sci. Rep. 10, 1–14. doi: 10.1038/s41598-020-70605-x
Putnam H. M., Stat M., Pochon X., Gates R. D. (2012). Endosymbiotic flexibility associates with environmental sensitivity in scleractinian corals. P Roy Soc. B-Biol Sci. 279, 4352–4361. doi: 10.1098/rspb.2012.1454
Ricci F., Tandon K., Black J. R., Cao K.-A. L., Blackall L. L., Verbruggen H. (2022). Host traits and phylogeny contribute to shaping coral-bacterial symbioses. mSystems 7, e00044–e00022. doi: 10.1128/msystems.00044-22
Richards Z., Kirkendale L., Moore G., Hosie A., Huisman J., Bryce M., et al. (2016). Marine biodiversity in temperate Western Australia: multi-taxon surveys of Minden and Roe reefs. Diversity 8, 7. doi: 10.3390/D8020007
Richmond C. E., Breitburg D. L., Rose K. A. (2005). The role of environmental generalist species in ecosystem function. Ecol. Modell 188, 279–295. doi: 10.1016/j.ecolmodel.2005.03.002
Roelfsema C., Thurstan R., Beger M., Dudgeon C., Loder J., Kovacs E., et al. (2016). A citizen science approach: A detailed ecological assessment of subtropical reefs at point lookout, Australia. PloS One 11, e0163407. doi: 10.1371/journal.pone.0163407
Rohwer F., Seguritan V., Azam F., Knowlton N. (2002). Diversity and distribution of coral-associated bacteria. Mar. Ecol. Prog. Ser. 243, 1–10. doi: 10.3354/meps243001
Ros M., Suggett D. J., Edmondson J., Haydon T., Hughes D. J., Kim M., et al. (2021). Symbiont shuffling across environmental gradients aligns with changes in carbon uptake and translocation in the reef-building coral Pocillopora acuta. Coral Reefs 40, 595–607. doi: 10.1007/S00338-021-02066-1
Saad O. S., Lin X., Ng T. Y., Li L., Ang P., Lin S. (2022). Species richness and generalists–specialists mosaicism of symbiodiniacean symbionts in corals from Hong Kong revealed by high-throughput ITS sequencing. Coral Reefs 41, 1–12. doi: 10.1007/S00338-021-02196-6
Sawall Y., Al-Sofyani A., Banguera-Hinestroza E., Voolstra C. R. (2014). Spatio-temporal analyses of Symbiodinium physiology of the coral Pocillopora verrucosa along large-scale nutrient and temperature gradients in the Red Sea. PloS One 9, e103179. doi: 10.1371/journal.pone.0103179
Schmidt-Roach S., Lundgren P., Miller K. J., Gerlach G., Noreen A. M. E., Andreakis N. (2012). Assessing hidden species diversity in the coral Pocillopora damicornis from Eastern Australia. Coral Reefs 32, 161–172. doi: 10.1007/s00338-012-0959-z
Schmidt-Roach S., Lundgren P., Miller K. J., Gerlach G., Noreen A. M. E., Andreakis N. (2013a). Assessing hidden species diversity in the coral Pocillopora damicornis from Eastern Australia. Coral Reefs 32, 161–172. doi: 10.1007/s00338-012-0959-z
Schmidt-Roach S., Miller K., Andreakis N. (2013b). Pocillopora aliciae: A new species of scleractinian coral (Scleractinia, Pocilloporidae) from subtropical Eastern Australia. Zootaxa 3626, 576–582. doi: 10.11646/zootaxa.3626.4.11
Schmidt-Roach S., Miller K. J., Lundgren P., Andreakis N. (2014). With eyes wide open: a revision of species within and closely related to the Pocillopora damicornis species complex (Scleractinia; Pocilloporidae) using morphology and genetics. Zoo J. Linn Soc. 170, 1–33. doi: 10.1111/zoj12092
Scucchia F., Malik A., Putnam H. M., Mass T. (2021). Genetic and physiological traits conferring tolerance to ocean acidification in mesophotic corals. Glob Chang Biol. 27, 5276–5294. doi: 10.1111/gcb.15812
Scucchia F., Nativ H., Neder M., Goodbody-Gringley G., Mass T. (2020). Physiological characteristics of Stylophora pistillata larvae across a depth gradient. Front. Mar. Sci. 7. doi: 10.3389/fmars.2020.00013
Serrano X. M., Baums I. B., O’Reilly K., Smith T. B., Jones R. J., Shearer T. L., et al. (2014). Geographic differences in vertical connectivity in the Caribbean coral Montastraea cavernosa despite high levels of horizontal connectivity at shallow depths. Mol. Ecol. 23, 4226–4240. doi: 10.1111/mec.12861
Shaver E. C., McLeod E., Hein M. Y., Palumbi S. R., Quigley K., Vardi T., et al. (2022). A roadmap to integrating resilience into the practice of coral reef restoration. Glob Chang Biol. 28, 4752–4764. doi: 10.1111/gcb.16212
Shlesinger T., Grinblat M., Rapuano H., Amit T., Loya Y. (2018). Can mesophotic reefs replenish shallow reefs? Reduced coral reproductive performance casts a doubt. Ecology 99, 421–437. doi: 10.1002/ecy.2098
Shlesinger T., Loya Y. (2019). Sexual reproduction of scleractinian corals in mesophotic coral ecosystems vs. shallow reefs. Coral Reefs World (Cham), 653–666. doi: 10.1007/978-3-319-92735-0_35
Silveira C. B., Gregoracci G. B., Coutinho F. H., Silva G. G. Z., Haggerty J. M., de Oliveira L. S., et al. (2017). Bacterial community associated with the reef coral Mussismilia braziliensis’s momentum boundary layer over a diel cycle. Front. Microbiol. 8. doi: 10.3389/fmicb.2017.00784
Silverstein R. N., Correa A. M. S., Baker A. C. (2012). Specificity is rarely absolute in coral–algal symbiosis: implications for coral response to climate change. P Roy Soc. B-Biol Sci. 279, 2609–2618. doi: 10.1098/rspb.2012.0055
Slatyer R. A., Hirst M., Sexton J. P. (2013). Niche breadth predicts geographical range size: a general ecological pattern. Ecol. Lett. 16, 1104–1114. doi: 10.1111/ele.12140
Smale D., Reefs T. W.-C. (2012). Ecological observations associated with an anomalous warming event at the Houtman Abrolhos Islands, Western Australia. Coral Reefs 31, 441. doi: 10.1007/s00338-012-0873-4. 2012, Undefined.
Smith P. J., Fujio Y. (1982). Genetic variation in marine teleosts: High variability in habitat specialists and low variability in habitat generalists. Mar. Biol. 69, 7–20. doi: 10.1007/BF003969551 69,
Smith S. M., Malcolm H. A., Marzinelli E. M., Schultz A. L., Steinberg P. D., Vergés A. (2021). Tropicalization and kelp loss shift trophic composition and lead to more winners than losers in fish communities. Glob Chang Biol. 27, 2537–2548. doi: 10.1111/gcb.15592
Smith T. B., Holstein D. M., Ennis R. S. (2019). Disturbance in mesophotic coral ecosystems and linkages to conservation and management. Coral Reefs World 12, 911–929. doi: 10.1007/978-3-319-92735-0_47
Sommer B., Beger M., Harrison P. L., Babcock R. C., Pandolfi J. M. (2018). Differential response to abiotic stress controls species distributions at biogeographic transition zones. Ecography 41, 478–490. doi: 10.1111/ecog.02986
Sommer B., Harrison P. L., Beger M., Pandolfi J. M. (2014). Trait-mediated environmental filtering drives assembly at biogeographic transition zones. Ecology 95, 1000–1009. doi: 10.1890/13-1445.1
Sommer B., Sampayo E. M., Beger M., Harrison P. L., Babcock R. C., Pandolfi J. M. (2017). Local and regional controls of phylogenetic structure at the high-latitude range limits of corals. P Roy Soc. B-Biol Sci. 284, 20170915. doi: 10.1098/rspb.2017.0915
Soto D., de Palmas S., Ho M. J., Denis V., Chen C. A. (2018). Spatial variation in the morphological traits of Pocillopora verrucosa along a depth gradient in Taiwan. PloS One 13, e0202586. doi: 10.1371/journal.pone.0202586
Spalding M., Fox H., Allen G., Davidson N., Ferdaña Z., Finlayson M., et al. (2007). Marine ecoregions of the world: a bioregionalization of coastal and shelf areas. Bioscience 57, 573–583. doi: 10.1641/B570707
Steinberg R. K., Ainsworth T. D., Moriarty T., Bednarek T., Dafforn K. A., Johnston E. L. (2022). Bleaching susceptibility and resistance of octocorals and anemones at the world’s southern-most coral reef. Front. Physiol. 13. doi: 10.3389/fphys.2022.804193
Stuart-Smith R. D., Brown C. J., Ceccarelli D. M., Edgar G. J. (2018). Ecosystem restructuring along the Great Barrier Reef following mass coral bleaching. Nature 560, 92–96. doi: 10.1038/s41586-018-0359-9
Studivan M. S., Milstein G., Voss J. D. (2019). Montastraea cavernosa corallite structure demonstrates distinct morphotypes across shallow and mesophotic depth zones in the Gulf of Mexico. PloS One 14, e0203732. doi: 10.1371/journal.pone.0203732
Studivan M. S., Voss J. D. (2018a). Assessment of mesophotic coral ecosystem connectivity for proposed expansion of a Marine sanctuary in the Northwest gulf of Mexico: Population genetics. Front. Mar. Sci. 5. doi: 10.3389/fmars.2018.00152
Studivan M. S., Voss J. D. (2018b). Population connectivity among shallow and mesophotic Montastraea cavernosa corals in the Gulf of Mexico identifies potential for refugia. Coral Reefs 37, 1183–1196. doi: 10.1007/S00338-018-1733-7
Studivan M. S., Voss J. D. (2020). Transcriptomic plasticity of mesophotic corals among natural populations and transplants of Montastraea cavernosa in the Gulf of Mexico and Belize. Mol. Ecol. 29, 2399–2415. doi: 10.1111/mec.15495
Swain T. D., Lax S., Gilbert J., Backman V., Marcelino L. A. (2021). ). A phylogeny-informed analysis of the global coral-symbiodiniaceae interaction network reveals that traits correlated with thermal bleaching are specific to symbiont transmission mode. mSystems 6. doi: 10.1128/msystems.00266-21
Tamir R., Ben-Zvi O., Eyal G., Kramer N., Loya Y. (2020). Reciprocal-transplantation between shallow and mesophotic stony corals. Mar. Environ. Res. 161, 105035. doi: 10.1016/j.marenvres.2020.105035
Tamir R., Eyal G., Kramer N., Laverick J. H., Loya Y. (2019). Light environment drives the shallow-to-mesophotic coral community transition. Ecosphere 10, e02839. doi: 10.1002/ecs2.2839
Thomas C. J., Bridge T. C. L., Figueiredo J., Deleersnijder E., Hanert E. (2015). Connectivity between submerged and near-sea-surface coral reefs: can submerged reef populations act as refuges? Divers. Distrib 21, 1254–1266. doi: 10.1111/ddi.12360
Terán E., Méndez E. R., Enríquez S., Iglesias-Prieto R. (2010). Multiple light scattering and absorption in reef-building corals. Appl. Opt 49, 5032–5042. doi: 10.1364/AO.49.005032
Terraneo T. I., Ouhssain M., Castano C. B., Aranda M., Hume B. C., Marchese F., et al. (2023). From the shallow to the mesophotic: a characterization of Symbiodiniaceae diversity in the Red Sea NEOM region. Front. Mar. Sci. 10, 1077805. doi: 10.3389/fmars.2023.1077805
Thomson D. P., Bearham D., Graham F., Eagle J. V. (2011). High latitude, deeper water coral bleaching at Rottnest Island, Western Australia. Coral Reefs 30, 1107. doi: 10.1007/S00338-011-0811-X
Todd P. A. (2008). Morphological plasticity in scleractinian corals. Biol. Rev. 83, 315–337. doi: 10.1111/j.1469-185X.2008.00045.x
Tuckett C. A., de Bettignies T., Fromont J., Wernberg T. (2017). Expansion of corals on temperate reefs: direct and indirect effects of marine heatwaves. Coral Reefs 36, 947–956. doi: 10.1007/s00338-017-1586-5
Turnham K. E., Aschaffenburg D. M., Tye Pettay D., Paz-García D. A., Reyes-Bonilla H., Pinzón J., et al. (2023). High physiological function for corals with thermally tolerant, host-adapted symbionts. P Roy Soc. B-Biol Sci. 290, 20231021. doi: 10.1098/rspb.2023.1021
van der Meer M. H., Berumen M. L., Hobbs J. P. A., van Herwerden L. (2015). Population connectivity and the effectiveness of marine protected areas to protect vulnerable, exploited and endemic coral reef fishes at an endemic hotspot. Coral Reefs 34, 393–402. doi: 10.1007/S00338-014-1242-2
van Oppen M. J. H., Gates R. D., Blackall L. L., Cantin N., Chakravarti L. J., Chan W. Y., et al. (2017). Shifting paradigms in restoration of the world’s coral reefs. Glob Chang Biol. 23, 3437–3448. doi: 10.1111/gcb.13647
van Oppen M. J. H., Bongaerts P., Underwood J. N., Peplow L. M., Cooper T. F. (2011). The role of deep reefs in shallow reef recovery: an assessment of vertical connectivity in a brooding coral from west and east Australia. Mol. Ecol. 20, 1647–1660. doi: 10.1111/J.1365-294X.2011.05050.X
van Tienderen P. H. (1991). Evolution of generalists and specialists in spatially heterogeneous environments. Evolution 45, 1317–1331. doi: 10.1111/J.1558-5646.1991.TB02638.X
van Woesik R., Franklin E. C., O’Leary J., McClanahan T. R., Klaus J. S., Budd A. F. (2012). Hosts of the plio-pleistocene past reflect modern-day coral vulnerability. P Roy Soc. B-Biol Sci. 279, 2448–2456. doi: 10.1098/rspb.2011.2621
Vardi T., Hoot W. C., Levy J., Shaver E., Winters R. S., Banaszak A. T., et al. (2021). Six priorities to advance the science and practice of coral reef restoration worldwide. Restor. Ecol. 29, e13498. doi: 10.1111/REC.13498
Varasteh T., Salazar V., Tschoeke D., Francini-Filho R. B., Swings J., Garcia G., et al. (2021). Breviolum and Cladocopium are dominant among Symbiodiniaceae of the coral holobiont Madracis decactis. Microb. Ecol. 84, 325–335. doi: 10.1007/S00248-021-01868-8
Vergés A., Doropoulos C., Malcolm H. A., Skye M., Garcia-Pizá M., Marzinelli E. M., et al. (2016). Long-term empirical evidence of ocean warming leading to tropicalization of fish communities, increased herbivory, and loss of kelp. Proc. Natl. Acad. Sci. 113, 13791–13796. doi: 10.1073/pnas.1610725113
Vergés A., Mccosker E., Mayer-Pinto M., Coleman M. A., Wernberg T., Ainsworth T., et al. (2019). Tropicalisation of temperate reefs: Implications for ecosystem functions and management actions. Funct. Ecol., 1–14. doi: 10.1111/1365-2435.13310
Vergés A., Steinberg P. D., Hay M. E., Poore A. G. B., Campbell A. H., Ballesteros E., et al. (2014). The tropicalization of temperate marine ecosystems: climate-mediated changes in herbivory and community phase shifts. P Roy Soc. B-Biol Sci. 281, 1–10. doi: 10.1098/rspb.2014.0846
Vermeij M., Bakker J., van der Hal N., Bak R. (2011). Juvenile coral abundance has decreased by more than 50% in only three decades on a small Caribbean island. Diversity 3, 296–307. doi: 10.3390/d3030296
Veron J., Pichon M. (1976). “Scleractinia of eastern Australia. Part I: families thamnasteriidae, astrocoeniidae, pocilloporidae,” in Corals of the world (Canberra, Australia: Australian Institute of Marine Science & Australian National University Press).
Voolstra C. R., Ziegler M. (2020). Adapting with microbial help: microbiome flexibility facilitates rapid responses to environmental change. BioEssays 42, 2000004. doi: 10.1002/bies.202000004
Watanabe A., Nakamura T. (2019). Carbon dynamics in coral reefs. Blue Carbon Shallow Coast. Ecosyst., 273–293. doi: 10.1007/978-981-13-1295-3_10
Wang J. T., Chen Y. Y., Tew K. S., Meng P. J., Chen C. A. (2012). Physiological and biochemical performances of menthol-induced aposymbiotic corals. PloS One 7, e46406. doi: 10.1371/journal.pone.0046406
Wernberg T., Smale D., Tuya F., Thomsen M., Langlois T., de Bettignies T., et al. (2013). An extreme climatic event alters marine ecosystem structure in a global biodiversity hotspot. Nat. Clim Chang 3, 78–82. doi: 10.1038/nclimate1627
Wepfer P. H., Nakajima Y., Hui F. K. C., Mitarai S., Economo E. P. (2020). Metacommunity ecology of Symbiodiniaceae hosted by the coral Galaxea fascicularis. Mar. Ecol. Prog. Ser. 633, 71–87. doi: 10.3354/meps13177
Williams G. J., Graham N. A. J. (2019). Rethinking coral reef functional futures. Funct. Ecol. 33, 942–947. doi: 10.1111/1365-2435.13374
Wilson J., Harrison P. (2003). Spawning patterns of scleractinian corals at the Solitary Islands - a high latitude coral community in eastern Australia. Mar. Ecol. Prog. Ser. 260, 115–123. doi: 10.3354/meps260115
Yang Q., Zhang Y., Ahmad M., Ling J., Zhou W., Zhang Y., et al. (2021). Microbial community structure shifts and potential Symbiodinium partner bacterial groups of bleaching coral Pocillopora verrucosa in South China Sea. Ecotoxicology 30, 966–974. doi: 10.1007/S10646-021-02380-Y/
Yost D. M., Wang L.-H., Fan T.-Y., Chen C.-S., Lee R. W., Sogin E., et al. (2013). Diversity in skeletal architecture influences biological heterogeneity and Symbiodinium habitat in corals. Zoology 116, 262–269. doi: 10.1016/j.zool.2013.06.001
Zawada K. J. A., Madin J. S., Baird A. H., Bridge T. C. L., Dornelas M. (2019). Morphological traits can track coral reef responses to the Anthropocene. Funct. Ecol. 33, 962–975. doi: 10.1111/1365-2435.13358
Zhou G., Tong H., Cai L., Huang H. (2021). Transgenerational effects on the coral Pocillopora damicornis microbiome under ocean acidification. Microb. Ecol. 82, 572–580. doi: 10.1007/S00248-021-01690-2
Ziegler M., Grupstra C. G. B., Barreto M. M., Eaton M., BaOmar J., Zubier K., et al. (2019). Coral bacterial community structure responds to environmental change in a host-specific manner. Nat. Commun. 10, 1–11. doi: 10.1038/s41467-019-10969-5
Ziegler M., Roder C., Bchel C., Voolstra C. R. (2015A). Niche acclimatization in Red Sea corals is dependent on flexibility of host-symbiont association. Mar. Ecol. Prog. Ser. 533, 149–161. doi: 10.3354/meps11365
Ziegler M., Roder C. M., Büchel C., Voolstra C. R. (2015B). Mesophotic coral depth acclimatization is a function of host-specific symbiont physiology. Front. Mar. Sci. 2. doi: 10.3389/fmars.2015.00004
Keywords: generalist, coral reefs, climate change, Pocilloporid, Australia
Citation: Bergman JL, Richards ZT, Sawyers P and Ainsworth TD (2023) Environmental generalism, holobiont interactions, and Pocilloporid corals in the warming oceans of the eastern coast of Australia. Front. Ecol. Evol. 11:1190455. doi: 10.3389/fevo.2023.1190455
Received: 20 March 2023; Accepted: 28 November 2023;
Published: 18 December 2023.
Edited by:
Jennifer L. Matthews, University of Technology Sydney, AustraliaReviewed by:
Alexandra Dempsey, Khaled bin Sultan Living Oceans Foundation, United StatesYvonne Sawall, Bermuda Institute of Ocean Sciences, Bermuda
Copyright © 2023 Bergman, Richards, Sawyers and Ainsworth. This is an open-access article distributed under the terms of the Creative Commons Attribution License (CC BY). The use, distribution or reproduction in other forums is permitted, provided the original author(s) and the copyright owner(s) are credited and that the original publication in this journal is cited, in accordance with accepted academic practice. No use, distribution or reproduction is permitted which does not comply with these terms.
*Correspondence: Jessica L. Bergman, ai5iZXJnbWFuQHVuc3cuZWR1LmF1