- 1Wankara Laboratory, Cape Horn International Center, Universidad de Magallanes, Puerto Williams, Chile
- 2Millennium Institute of Biodiversity of Antarctic and Subantarctic Ecosystems, Universidad de Chile, Santiago, Chile
- 3Millennium Nucleus of Austral Invasive Salmonids (INVASAL), Universidad de Concepción, Concepción, Chile
- 4Department of Biology, Ecology and Environmental Sciences, University of Barcelona, Barcelona, Spain
- 5Institut de Recerca de la Biodiversitat, University of Barcelona, Barcelona, Spain
- 6Entomology and Plant Pathology Department, The University of Tennessee, Knoxville, TN, United States
- 7British Antarctic Survey, Natural Environment Research Council (NERC), Cambridge, United Kingdom
- 8Department of Zoology, University of Johannesburg, Johannesburg, South Africa
Introduction: Ecogeographical patterns in body size have been described across a wide range of vertebrate species. However, insects have shown inconsistent patterns in studies to date. Aquatic insects, particularly those from remote regions, have barely been explored.
Methods: The Magellanic sub-Antarctic ecoregion offers an ideal natural laboratory with near pristine environments, limiting the potential influence of confounding variables. In this study, we evaluated the influence of elevation on body and wing size and aspect ratio patterns for 10 species of mayfly (Ephemeroptera) and stonefly (Plecoptera) along a steep coastal elevation gradient (~0–600 m a.s.l.).
Results: We detected significant relationships between altitude and morphological features for the studied species. Additionally, we found that in females, morphological changes were slightly more pronounced than in males, probably due to their role as dispersers. While body size showed an increase along the elevation gradient, there was a notable decrease in some species' wing length over a relatively short geographic distance.
Discussion: Our data suggest that morphological plasticity might be promoted in response to the harsh environmental conditions that typify the steep coastal Magellanic sub-Antarctic mountain ranges.
1 Introduction
Morphological plasticity along environmental gradients has long interested naturalists and ecologists. Body size is considered a significant organism trait, correlated with various aspects of ecology and biology (Blackburn and Gaston, 2001; Chown and Klok, 2003; Chown and Gaston, 2010). Bergmann’s rule (Bergmann, 1847) remains one of the most debated ecogeographical patterns, addressing body size variation in response to decreasing environmental temperature (e.g., latitudinal, altitudinal). Originally, Bergmann’s rule referred to endothermic animals, but patterns have been also explored in ectotherms. In insects, reported patterns are inconsistent (conventional, converse, or no pattern at all), and mechanisms are unclear, which has led to the generality of the rule being questioned (Blackburn et al., 1999; Blanckenhorn and Demont, 2004; McCulloch and Waters, 2017; Pallarés et al., 2019). While very few studies have addressed aquatic insect taxa, some have reported similar inconsistencies (McCulloch et al., 2017; Pallarés et al., 2019). Conversely to Bergmann’s rule, the temperature–size rule refers to developmental rates in colder environments, such as at higher latitudes or elevations (Atkinson and Sibly, 1997; Angilletta and Dunham, 2003). Cold temperatures result in slow growth rates in ectotherms, leading to increased adult body size, and in turn also to increased fecundity. Increased body size is often considered as a proxy indicator of fitness, potentially as a trade-off in adverse conditions. Wing length and wing shape, through their direct influence on flight characteristics, have been suggested to be directly related to dispersal ability, influencing species distributions and flight activity (Malmqvist, 2000; McCulloch et al., 2017). Insects inhabiting higher elevations are expected to show a greater incidence of wing reduction (brachyptery) or wing loss (aptery). However, wing morphological patterns also show inconsistent correlations with environmental parameters, varying from strong (Plecoptera, McCulloch and Waters, 2017), moderate (Ephemeroptera, Malmqvist, 2000), weak (Plecoptera, Malmqvist, 2000) to the absence of such patterns (Orthoptera, Levy and Nufio, 2015).
The Cape Horn Biosphere Reserve (CHBR) is in the southern part of the Magellanic sub-Antarctic ecoregion, lying between 54 and 56°S. This region is considered unique due to the absence of a biogeographic replicate globally, its unpolluted water courses and bodies, the world’s southernmost rainforests and wetlands, and minimal human-associated development, enabling the opportunity to study species and communities under pre-industrial conditions with minimal confounding influences (Contador et al., 2015). Embedded in the CHBR lies Navarino Island (55°S), whose mountain ranges span steep elevation gradients (~1000 m a.s.l.) over short geographic distances, with clear changes in air and water temperatures and biotic assemblages (Contador et al., 2015; Rendoll-Cárcamo et al., 2019). Additionally, these acute elevation gradients have strong influence on aquatic insect diversity, shaping community structure, composition, function, life cycles and thermal physiology (Contador and Kennedy, 2016; Rendoll-Cárcamo et al., 2020). Navarino Island is also the global southern-most distributional limit of some major aquatic insect lineages, such as Ephemeroptera and Plecoptera (henceforth mayflies and stoneflies, respectively). In these southern catchments mayflies are represented three families: Baetidae, Leptophlebiidae and Nesameletidae, while stoneflies are represented by two: Gripopterygidae and Notonemouridae. Species representing these families are present throughout the elevation gradient, while their life history and phenology have been studied (Contador and Kennedy, 2016), little is known about their morphology.
These remote and pristine mountainous landscapes offer a unique opportunity to assess morphological patterns in a close-to-natural state, serving as a baseline for mid- and long-term monitoring of climate-change-derived effects. Our objective is to investigate the changes in wing and body size through the elevation gradient. We hypothesize that the steep elevation gradients prevalent in Magellanic sub-Antarctic catchments will promote larger body sizes across all mayfly and stonefly species at higher elevations, while also resulting in wing reduction as a plastic response to harsh environmental conditions. Additionally, we predict that female individuals will exhibit greater morphological variation due to their role as the dispersing sex.
2 Materials and methods
2.1 Study area and site characterization
Navarino Island is located south of Tierra del Fuego and the Beagle Channel within the CHBR (Figure 1). Sampling surveys took place during austral summer of 2021 (January) to ensure the collection of emerging adults. Twelve malaise traps (Watkins and Doncaster; dimensions: 1.7m long x 1.2m wide, and 1.7m highest point, 0.95m) were installed for 80–90 h adjacent to three streams (one per elevation belt, and four elevations) draining to the north coast of Navarino Island, the Róbalo, Bronces and Ukika rivers, each in different catchments. Each catchment was sampled at a time with four malaise traps installed within the same day. After retrieval, it took a 24 h lapse to install traps in the following catchment. All sampling occurs in the span of two weeks. The selection of these was based on their marked elevation belts, faunal composition (Contador et al., 2015; Rendoll-Cárcamo et al., 2019; Rendoll-Cárcamo et al., 2020), vegetation types, and practical accessibility. The four elevation belts were identified based on biotic (southern beech forests composition) and abiotic features and based on previous assessments: 130–180 m a.s.l., evergreen forests (Nothofagus betuloides) and Sphagnum peatlands; 230–280 m a.s.l., mixed forests (N. betuloides dominant) and shrubs; 350–380 m a.s.l., mixed forests (N. pumilio dominant) and Sphagnum and Astelia-Donatia peatlands; 450–500 m a.s.l., krummholz (treeline of N. antarctica and N. pumilio) and cushion peatlands. A key consideration when identifying elevation belts was to sample away from the presence of invasive salmonids in the lower parts of the streams (Maldonado-Márquez et al., 2020). Invasive fishes can drastically influence the ecology and biology of insect species by predation and, without proper delimitation, could mask any natural patterns present (Koch et al., 2020).
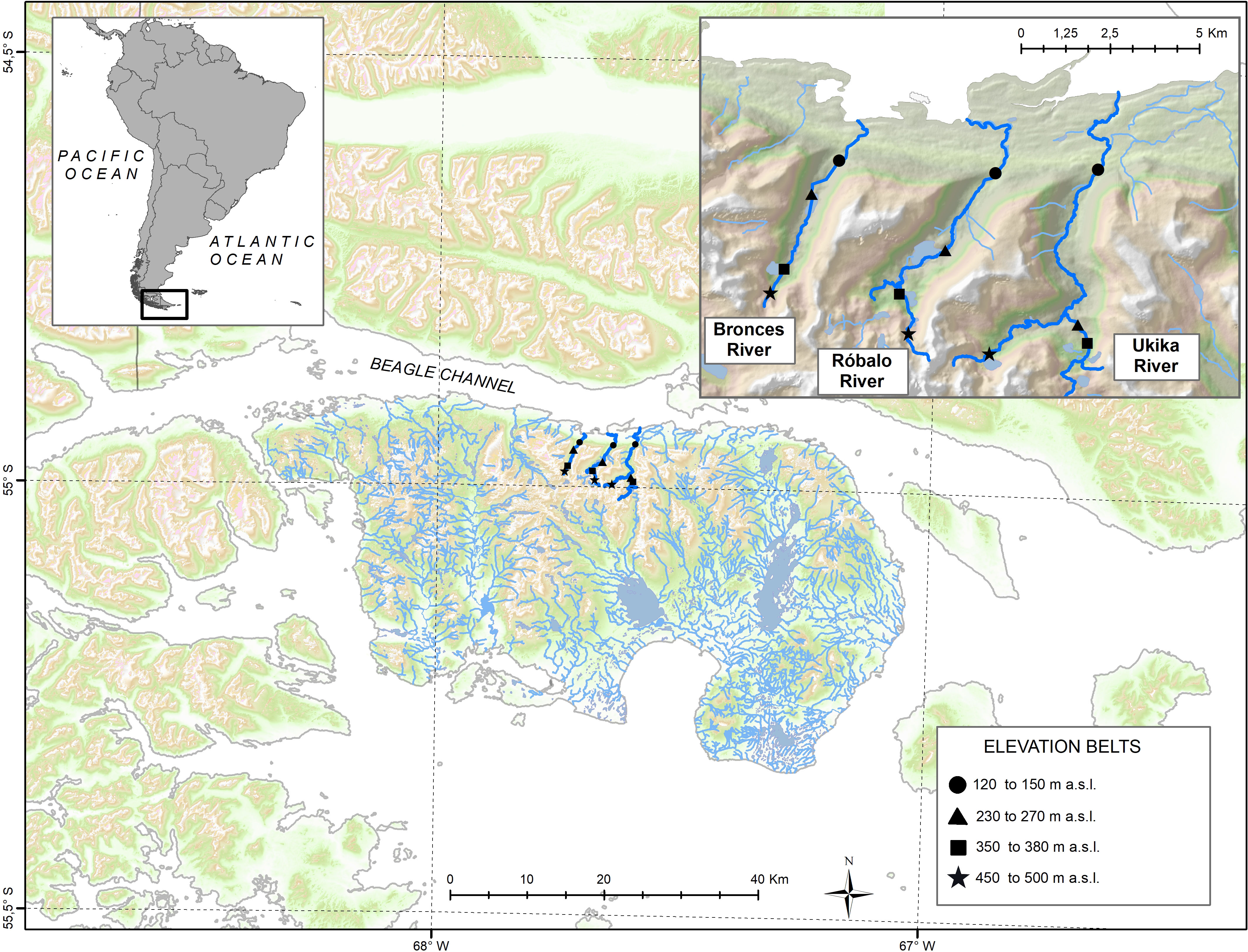
Figure 1 Location of Navarino Island in Southern South America, with surveyed river catchments (Bronces, Róbalo and Ukika rivers) highlighted. Each symbol represents a different elevation belt as shown in legend.
2.2 Specimen processing and measurements
A total of 553 mayfly (n = 332) and stonefly (n = 221) imagos were collected from the malaise traps and stored in containers in ethanol (95%). All individuals were identified to species level following pertinent literature (Nieto, 2004; Mclellan and Zwick, 2007; Domínguez and Fernández, 2009), and sex was determined to assess a priori if sexual dimorphism was present within species. Nevertheless, all specimens were included in the analyses regardless of sex due to low male abundances. Forewings were dissected from the body as close to the thorax as possible, then mounted and fixated onto microscope slides. Photographs were taken using a Leica S6D stereoscope with attached camera (M-SHOT MD90), and measurements of body length and wing length were performed on ImageJ 1.53e (Schneider et al., 2012). Body length was measured from the anterior margin of the head (clypeus) to the tip of the last abdominal segment (excluding cerci), while wing length was measured as the distance between the base and the apical margin. Relative wing length (wing length/body length) was used along wing length as body size varies between species, as in McCulloch et al. (2017).
2.3 Data analyses
Due to the mixed linear and non-linear nature of our data we tested morphological variation in body, wing and relative wing length by fitting a generalized additive model (GAM) with elevation, species and sex as predictors. GAM analyses captures linear and non-linear relationships between predictors and the response variables, and also provides an adjusted r2 value and deviance as measures of the proportion of variability in the response variable that is explained by the model. Additionally, we explored the interaction between elevation and species for all morphological features. All analyses were performed in R Studio 1.4.1717 (R Studio, 2022) using the packages mgcv.
3 Results
In general terms, we found that elevation as a negative effect on wing length on both orders, Ephemeroptera and Plecoptera (Figure 2), suggesting that with increasing elevation, wings decrease in size. For mayflies this negative effect of elevation on wing length was significant (p = 0.066), although for stoneflies was not (p = 0.372). When the pattern is explored with the interaction of elevation and each species it is noticeable that wing length reduction varies among species, and particularly for mayflies, it occurs for larger sized species (Figure 3). In stoneflies, the pattern is species dependant, some species show a decrease on wing length, while others show an increase, even cases in which the direction of the effect is sex related (Figure 3). A particular case of increase wing length towards higher elevations was found in the mayfly species Massartelopsis irarrazavali and the stonefly Senzilloides panguipulli (Figure 3, Table S1), showing the variability of patterns in sub-Antarctic mayflies. Furthermore, our analysis also indicated a negative wing length coefficient between sexes, suggesting that males have, on average, shorter wings than females, regardless of elevation (mayflies, p = 0.031; stoneflies, p < 0.001). The model on wing length explained a relatively high percentage of variability for both insect orders (mayflies, r2 = 0.874; stoneflies, r2 = 0.858, for details see Table 1).
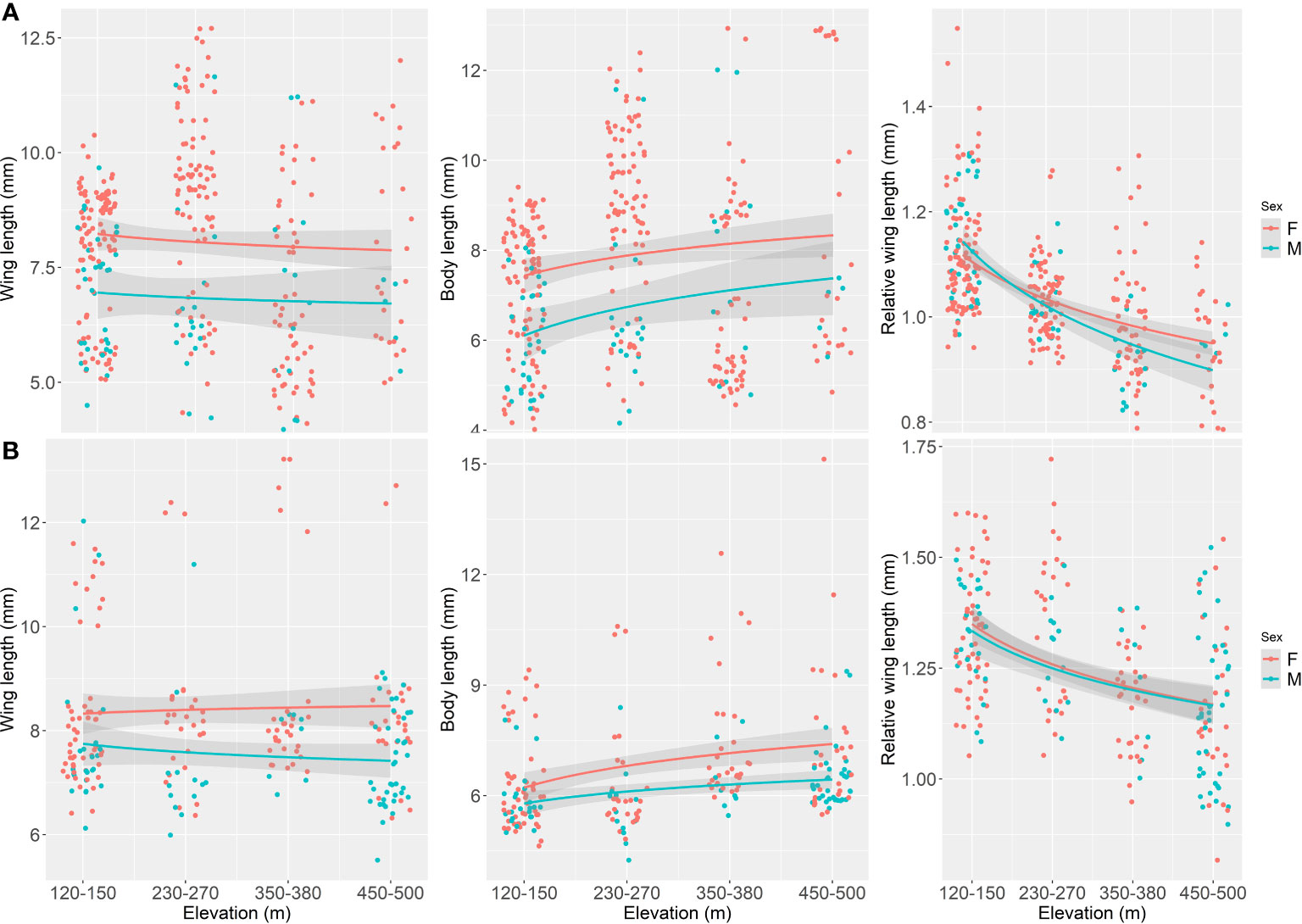
Figure 2 Elevation (m a.s.l.) versus wing length (mm), body length (mm) and relative wing length (mm) for Magellanic sub-Antarctic (A) mayflies (Ephemeroptera) and (B) stoneflies (Plecoptera). Females and males are represented by red and light blue circles and lines respectively.
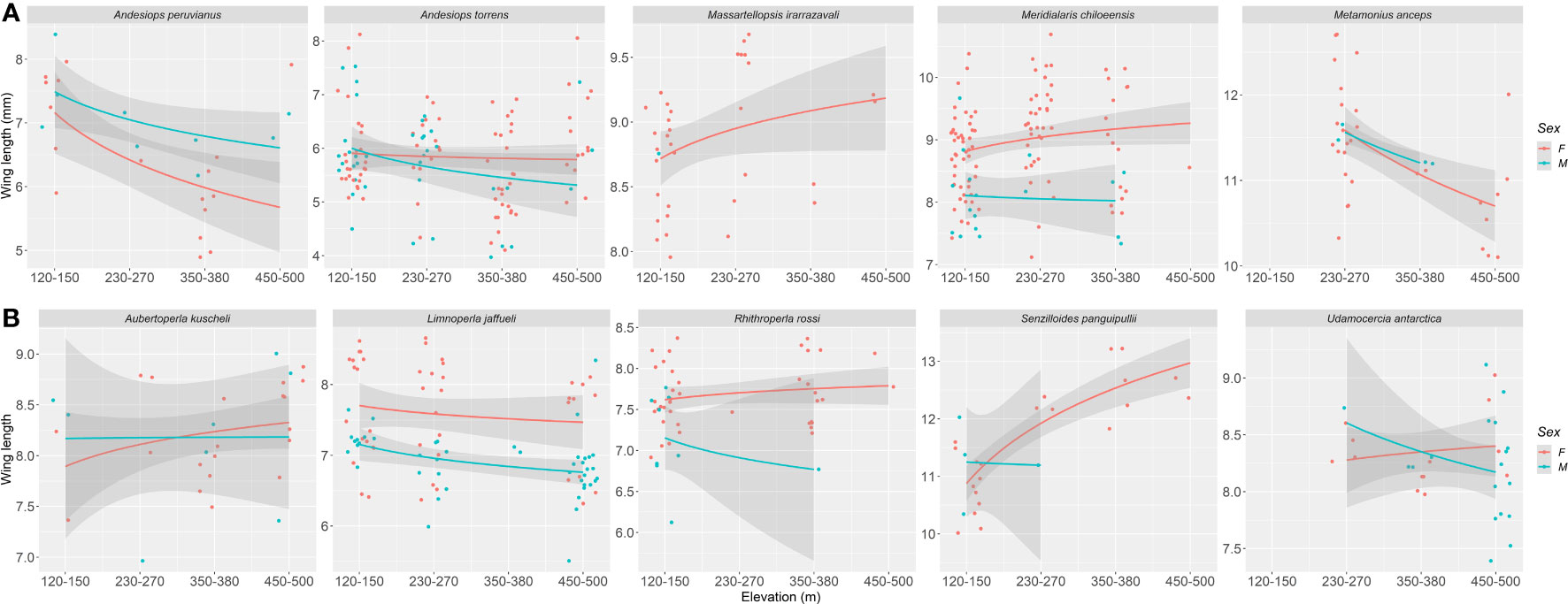
Figure 3 Elevation (m a.s.l.) versus wing length (mm) for Magellanic sub-Antarctic (A) mayfly species (Ephemeroptera) and (B) stonefly species (Plecoptera). Females and males are represented by red and light blue circles and lines respectively.
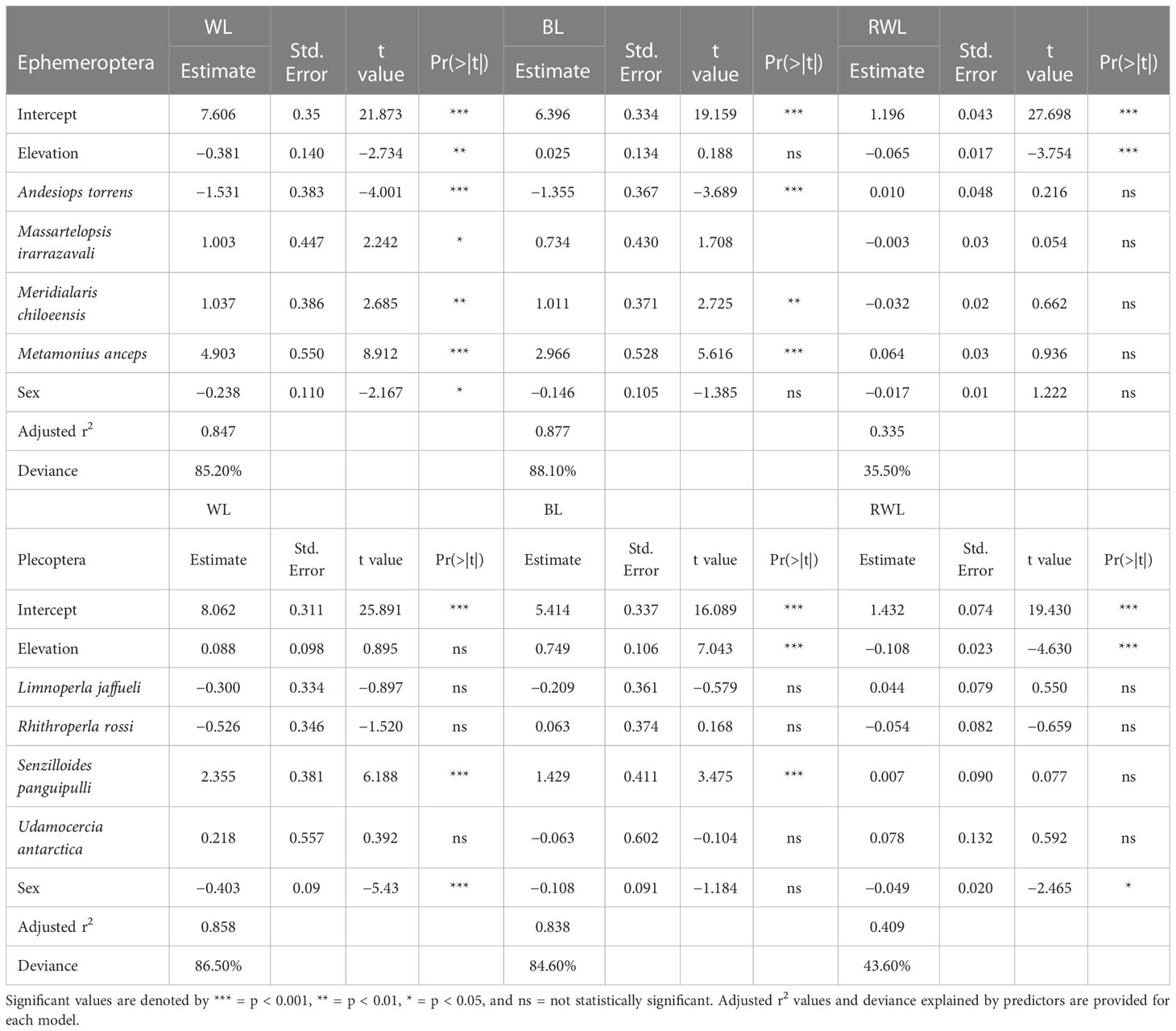
Table 1 Predictor estimates obtained by generalized additive models (GAMs) for morphological features (WL = wing length, BL = body length, RWL = relative wing length) of sub-Antarctic mayfly and stonefly species.
The effect of elevation on body length was found to be negligible for mayflies (p = 0.851), although for stoneflies we found a significant positive effect (p < 0.0001), indicating that as elevation increases, body length tends to increase as well (Figure 2). Similar to the trends on wing length, we found that body length varies within species (Figure 4, Table S1). Four of the five mayfly species showed an increase of body size with increasing elevation, and in the case of Andesiops peruvianus female body length decrease (Table 1). All stonefly species tend to have longer body length in higher elevations. The effects of sex on body length resulted to be non-significant for both, mayflies and stoneflies, yet particular cases such as the described A. peruvianus exists. In the case of body length, predictors of the model explained a relatively high percentage of variability for both insect orders (mayflies, r2 = 0.877; stoneflies, r2 = 0.838, for details see Table 1).
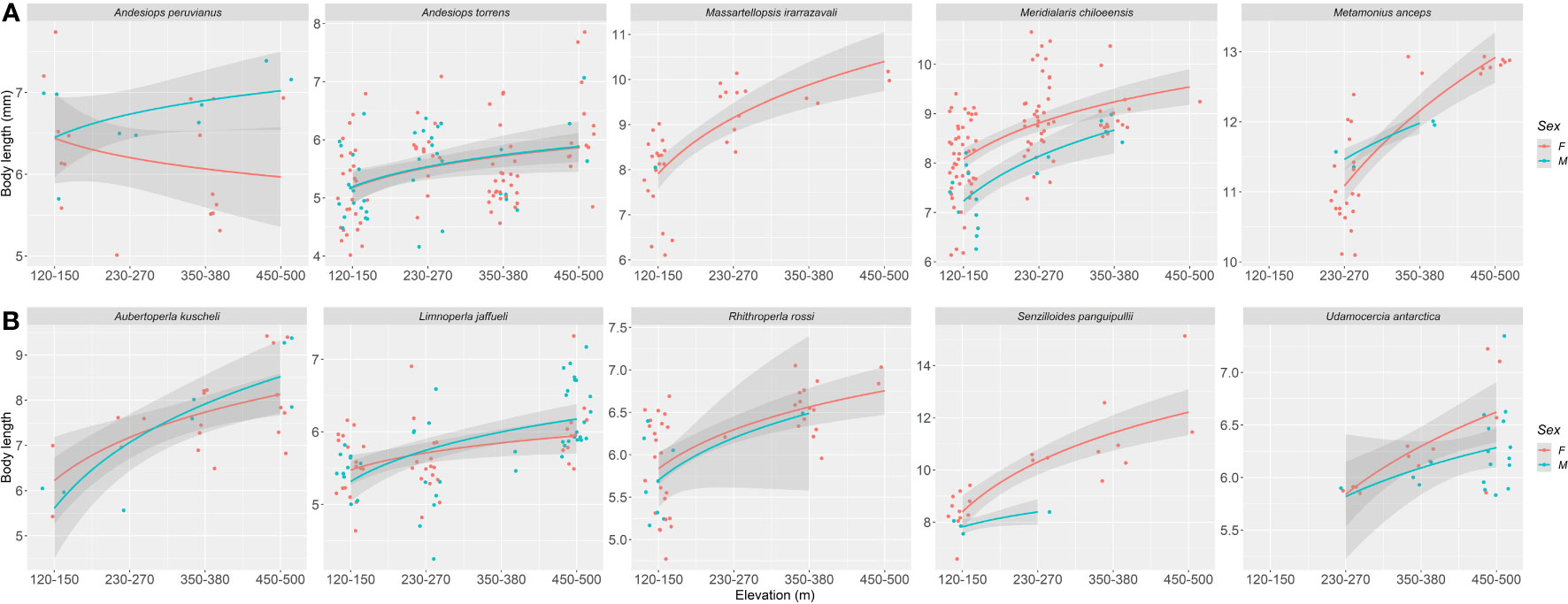
Figure 4 Elevation (m a.s.l.) versus body length (mm) for Magellanic sub-Antarctic (A) mayfly species (Ephemeroptera) and (B) stonefly species (Plecoptera). Females and males are represented by red and light blue circles and lines respectively.
Relative wing length changes in elevation (which represents the ratio of wing length to body length) was statistically significant for mayflies (p < 0.0001) and stoneflies (p < 0.0001) (Figure 2). All studied species of mayflies and stoneflies showed a decrease in relative wing length along the elevation gradient (Figure 5, Table S1). Since this measure standardizes sizes when species have different wing or body lengths, elevation is suggested to be the only predictor that accounts for variability (mayflies, r2 = 0.335; stoneflies, r2 = 0.409). Interestingly, males have lower relative wing length ratios than females, an effect only found to be significant within stoneflies (p = 0.0145, for details see Table 1).
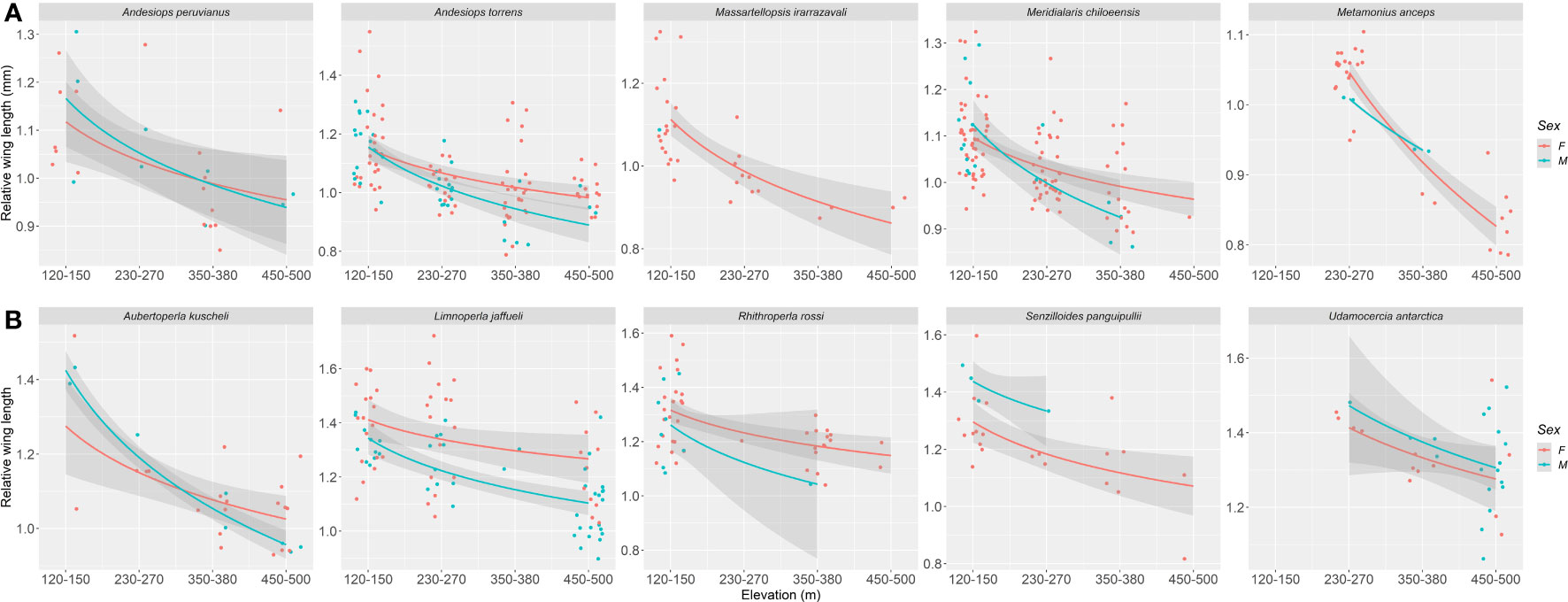
Figure 5 Elevation (m a.s.l.) versus relative wing length (mm) for Magellanic sub-Antarctic (A) mayfly species (Ephemeroptera) and (B) stonefly species (Plecoptera). Females and males are represented by red and light blue circles and lines respectively.
4 Discussion
Elevation, as a proxy for various environmental variables, has a significant association with body size and wing length. In remote areas, such as the Magellanic sub-Antarctic ecoregion, elevation gradients are short and steep, influencing the biology and ecology of the species that inhabit these pristine environments.
Trends of wing length variation are mixed; showing decrease pattern and in some case an increase. Wings features are commonly associated with dispersal ability, and wing reduction is suggested to influence conformity to Bergmann’s rule (Levy and Nufio, 2015). In harsher high elevation habitats, wing reduction may imply a trade-off with body size and overall fitness (Roff, 1977). Such wing reduction has been described in various stoneflies (McCulloch and Waters, 2017), and specifically in the southern South American genus Notoperla (Vera, 2011). Additionally, it has been suggested that conditions in high elevation habitats might influence wing reduction by selecting against fully winged individuals (Roff, 1990; McCulloch et al., 2017). Moreover, we found that taking sexual dimorphism into account is crucial. In general, females (mayflies and stoneflies) as dispersers, have significantly larger wings than males. Due to the low male abundances for certain species in our samples, species-specific sexual dimorphism driven differences as yet to be explored. Previous studies have also taken into account the impact of sexual dimorphism, selecting only females for their analyses (McCulloch et al., 2017). Few studies have documented species with reduced or absent wings inhabiting above the treeline (~500 m) in these sub-Antarctic environments. However, these cases may not be as uncommon, examples of such species include Andiperla willinki (Gripopterygidae), limoniid craneflies, mycetophilids, among others (RIM pers. obs.).
Our study detected a body size increase for studied mayfly and stonefly species towards higher elevations, following the ecogeographical pattern known as Bergmann’s Rule. Studies examining the application of Bergmann’s Rule to insects have generated widely varying outcomes, with findings in conformity (McCulloch and Waters, 2017; this study), converse (Pallarés et al., 2019), or detecting no discernible pattern (Levy and Nufio, 2015). For example, results consistent with Bergmann’s Rule have been reported more commonly than the inverse for stoneflies, while inverse clines have been reported for mayflies (Shelomi, 2012; McCulloch and Waters, 2017). Several mechanisms have been proposed to underly Bergmann’s Rule (see Blackburn et al., 1999), however the phylogenetic coherence of the study group remains key, as well as study design (Shelomi, 2012). Body size clines are, to some extent, better explained by the temperature–size rule (Atkinson and Sibly, 1997; Angilletta and Dunham, 2003). Insects and other ectotherms inhabiting cooler environments grow more slowly but as a result gain more mass over longer periods of time, achieving larger adult sizes (Atkinson, 1994). The elevation gradient of catchments on Navarino Island is characterized by marked changes in water temperature in short distances, influencing the ecology and biology of freshwater taxa (Contador et al., 2015; Rendoll-Cárcamo et al., 2020). The relatively steep decline in temperature towards higher elevation allows for longer periods of ice and snow duration, with some habitats remaining totally or partially frozen for up to half the year (Rendoll-Cárcamo et al., 2019). While bodies of water are completely frozen during this period, streams still flow bellow layers of ice. The increase in body size detected for all studied species (except for Andesiops peruvianus females which body length decrease towards high elevations), might be linked to thermal resources promoting longer life cycles (Chown and Klok, 2003).
Our findings highlight the significance of the short and steep elevation gradient that characterize sub-Antarctic Navarino Island. Higher elevation belts (350–380 m and 450–500 m) can be considered a transitional environments due to its proximity to both scrubby forests or krummholz and the tree-line at higher elevations and evergreen-dominated forests below. Additionally, it is one of the most aquatic insect species-rich environments in the Magellanic sub-Antarctic ecoregion (Contador et al., 2015), and also a ecophysiological standpoint where thermal breadth influences species (Rendoll-Cárcamo et al., 2020). Our results emphasize the importance of studying and monitoring these ecosystems since the ice-thawing, snow-melt, and permanent and temporal ponds in this elevation are starting to dry out earlier in summer, with shrubs and dwarf trees colonizing the once hyper-humid peatbogs (JR-C pers. obs.). This is similar to the first stages of shrubification found in Arctic tundra (Zhang et al., 2013; Mekonnen et al., 2021). Moreover, the recent declaration of the Magallanes region as a drought-prone area calls for proper focus, characterization, and monitoring of these environments and their biota. It is worth noting that this and other studies dealing with high-elevation or mountaintop insect species could be indicators of the escalator effect (Wilson et al., 2005). Climate change and its associated warming are expected to influence organism’s thermal associations, resulting in range contractions and in long term extirpation of populations and/or extinction of species (Urban, 2018). Due to the short metric distances of sub-Antarctic Magellanic elevation ranges and its strong influence on freshwater biota, the escalator effect should be taken in consideration when exploring new hypotheses related to morphological variation and insect dispersal.
Body size and wing length are key morphological features of insect species that help in understanding how the environment selects for such features, and which potential trade-offs and/or mechanisms are involved. Our data illustrate how elevation, even over a short geographic range, can have an important influence on insect morphology. Further population connectivity studies are now required to assess the extent to which the observed changes influence insect dispersal ability in these remote southern environments in these and other insect orders.
Data availability statement
The original contributions presented in the study are included in the article/Supplementary Material. Further inquiries can be directed to the corresponding author.
Author contributions
JR-C was lead author. MG, TC, PC, RIM contributed equally to the work. JR-C conceived and designed the research, collected field data, identified the species, and curated the data. JR-C and TC performed statistical analyses, and with MG produced the figures. JR-C wrote the initial draft, with significant contributions from TC, RIM, and PC. All authors contributed to the article and approved the submitted version.
Funding
JR-C is supported by the National Agency for Research and Development (ANID)/Scholarship Program/DOCTORADO BECAS CHILE/2021 – 21212048. PC is supported by NERC core funding to the BAS “Biodiversity, Evolution and Adaptation” Team. JR-C, MG and TC are supported by BASE Millennium Science Initiative Program – ICN2021_002, ANID/BASAL FB210018 and INVASAL ANID – Millennium Science Initiative Program – NCN2021-056. Additional support for RIM was provided by a grant from the US National Science Foundation [DEB-1949813].
Acknowledgments
The authors thank Miguel Troncoso and Javiera and Sophia Troncoso Villar for their help in the field. This is a contribution of the Wankara Laboratory. We also thank the three reviewers for their comments and suggestions, which greatly improved this manuscript.
Conflict of interest
The authors declare that the research was conducted in the absence of any commercial or financial relationships that could be construed as a potential conflict of interest.
Publisher’s note
All claims expressed in this article are solely those of the authors and do not necessarily represent those of their affiliated organizations, or those of the publisher, the editors and the reviewers. Any product that may be evaluated in this article, or claim that may be made by its manufacturer, is not guaranteed or endorsed by the publisher.
Supplementary material
The Supplementary Material for this article can be found online at: https://www.frontiersin.org/articles/10.3389/fevo.2023.1188889/full#supplementary-material
References
Angilletta M. J. J. R., Dunham A. E. (2003). The temperature-size rule in ectotherms: simple evolutionary explanations may not be general. Am. Nat. 162 (3), 332–342. doi: 10.1086/377187
Atkinson D. (1994). Temperature and organism size: a biological law for ectotherms? Adv. Ecol. Res. 25, 1–58. doi: 10.1016/S0065-2504(08)60212-3
Atkinson D., Sibly R. M. (1997). Why are organisms usually bigger in colder environments? Making sense of a life history puzzle. TREE. 12, 235–239. doi: 10.1016/S0169-5347(97)01058-6
Bergmann C. (1847). Über die verhältnisse der wärmeökonomie der thiere zu ihrer grösse. Gottinger Studien. 3, 595–708.
Blackburn T. M., Gaston K. J. (2001). Linking patterns in macroecology. J. Anim. Ecol. 70, 338–352. doi: 10.1046/j.1365-2656.2001.00484.x
Blackburn T. M., Gaston K. J., Loder N. (1999). Geographic gradients in body size: a clarification of Bergmann’s rule. Divers. Distrib. 5, 165–174. doi: 10.1046/j.1472-4642.1999.00046.x
Blanckenhorn W., Demont M. (2004). Bergmann and converse Bergmann latitudinal clines in arthropods: two ends of a continuum? ICB. 44, 413–424. doi: 10.1093/icb/44.6.413
Chown S. L., Gaston K. J. (2010). Body size variation in insects: a macroecological perspective. Biol. Rev. 85, 139–169. doi: 10.1111/j.1469-185X.2009.00097.x
Chown S. L., Klok C. J. (2003). Altitudinal body size clines: latitudinal effects associated with changing seasonality. Ecography. 26 (4), 445–455. doi: 10.1034/j.1600-0587.2003.03479.x
Contador T., Kennedy J. H., Rozzi R., Ojeda J. (2015). Sharp altitudinal gradients in Magellanic Sub-Antarctic streams: patterns along a fluvial system in the Cape Horn biosphere reserve (55° S). Polar Biol. 38 (11), 1853–1866. doi: 10.1007/s00300-015-1746-4
Contador T., Kennedy J. (2016). The life histories of Meridialaris chiloeensis (Demoulin, 1955) (Ephemeroptera: Leptophlebiidae) and Gigantodax rufescens (Edwards, 1931) (Diptera: Simuliidae) on a Magellanic sub-Antarctic island (55° S). Aquat. Insects 37 (2), 1–14. doi: 10.1080/01650424.2015.1134799
Domínguez E., Fernández H. R. (2009). Macroinvertebrados bentónicos sudamericanos. Sistemática y Biología. (Tucumán, Argentina: Fundación Miguel Lillo).
Koch B. J., Hall R. O. Jr., Peckarsky B. L. (2020). Nonconsumptive effects of brook trout predators reduce secondary production of mayfly prey. Freshw. Sci. 39 (3), 549–558. doi: 10.1086/710236
Levy R. A., Nufio C. R. (2015). Dispersal potential impacts size clines of grasshoppers across an elevation gradient. Oikos. 124, 610–619. doi: 10.1111/oik.01615
Maldonado-Márquez A., Contador T., Rendoll-Cárcamo J., Moore S., Pérez-Troncoso C., Gomez-Uchida D., et al. (2020). Southernmost distribution limit for endangered Peladillas (Aplochiton taeniatus) and non-native coho salmon (Oncorhynchus kisutch) coexisting within the Cape Horn biosphere reserve. J. Fish Biol. 96 (6), 1495–1500. doi: 10.1111/jfb.14309
Malmqvist B. (2000). How does wing length relate to distribution patterns of stoneflies (Plecoptera) and mayflies (Ephemeroptera)? Biol. Conserv. 93 (2), 271–276. doi: 10.1016/S0006-3207(99)00139-1
McCulloch G. A., Wallis G. P., Waters J. M. (2017). Does wing size shape insect biogeography? Evidence from a diverse regional stonefly assemblage. Glob. Ecol. Biogeogr. 26 (1), 93–101. doi: 10.1111/geb.12529
McCulloch G. A., Waters J. M. (2017). Does wing reduction influence the relationship between altitude and insect body size? A case study using New Zealand’s diverse stonefly fauna. Ecol. Evol. 00, 1–8. doi: 10.1002/ece3.3713
McLellan I. D., Zwick P. (2007). New species of and keys to South American Gripopterygidae (Plecoptera). Illiesia 3 (4), 20.
Mekonnen Z. A., Riley W. J., Berner L. T., Bouskill N. J., Torn M. S., Iwahana G., et al. (2021). Arctic tundra shrubification: a review of mechanisms and impacts on ecosystem carbon balance. Environ. Res. Lett. 16 (5), 053001. doi: 10.1088/1748-9326/abf28b
Nieto C. (2004). South American baetidae (Ephemeroptera): a new generic synonymy. Stud. Neotrop. Fauna Environ. 39 (2), 95–101. doi: 10.1080/01650520412331333747a
Pallarés S., Lai M., Abellán P., Ribera I., Sánchez-Fernández D. (2019). An interspecific test of Bergmann's rule reveals inconsistent body size patterns across several lineages of water beetles (Coleoptera: Dytiscidae). Ecol. Entomol. 44 (2), 249–254. doi: 10.1111/een.12701
Rendoll-Cárcamo J., Contador T., Convey P., Kennedy J. (2020). Sub-Antarctic freshwater invertebrate thermal tolerances: an assessment of critical thermal limits and behavioral responses. Insects. 11, 102. doi: 10.3390/insects11020102
Rendoll-Cárcamo J., Contador T., Gañán M., Troncoso C. P., Márquez A. M., Convey P., et al. (2019). Altitudinal gradients in Magellanic sub-Antarctic lagoons: the effect of elevation on freshwater macroinvertebrate diversity and distribution. PeerJ. 7, e7128. doi: 10.7717/peerj.7128
Roff D. (1977). Dispersal in dipterans: its costs and consequences. J. Anim. Ecol. 46 (2), 443–456. doi: 10.2307/3822
Roff D. (1990). The evolution of flightlessness in insects. Ecol. Monogr. 60 (4), 389–421. doi: 10.2307/1943013
R Studio (2022) R studio: integrated development environment for r (Version 1.4.1717). Available at: https://www.rstudio.com/.
Schneider C. A., Rasband W. S., Eliceiri K. W. (2012). NIH Image to ImageJ: 25 years of image analysis. Nat. Methods 9 (7), 671–675. doi: 10.1038/nmeth.2089
Shelomi M. (2012). Where are we now? Bergmann’s rule sensu lato in insects. Am. Nat. 180, 511–519. doi: 10.1086/667595
Urban M. C. (2018). Escalator to extinction. PNAS 115 (47), 11871–11873. doi: 10.1073/pnas.1817416115
Vera A. (2011). Nuevos registros distribucionales de Notoperla enderlein (Insecta: plecoptera), con comentarios taxonómicos y biológicos. Ans. Ins. Patagon. 39 (1), 109–112. doi: 10.4067/S0718-686X2011000100010
Wilson R. J., Gutiérrez D., Gutiérrez J., Martínez D., Agudo R., Monserrat V. J. (2005). Changes to the elevational limits and extent of species ranges associated with climate change. Ecol. Lett. 8 (11), 1138–1146. doi: 10.1111/j.1461-0248.2005.00824.x
Keywords: body size, ecogeographical patterns, Ephemeroptera, Plecoptera, sub-Antarctic, wing reduction
Citation: Rendoll-Cárcamo J, Gañán M, Madriz RI, Convey P and Contador T (2023) Wing reduction and body size variation along a steep elevation gradient: a case study with Magellanic sub-Antarctic mayflies and stoneflies. Front. Ecol. Evol. 11:1188889. doi: 10.3389/fevo.2023.1188889
Received: 18 March 2023; Accepted: 12 June 2023;
Published: 07 July 2023.
Edited by:
David Houghton, Hillsdale College, United StatesReviewed by:
Luke Jacobus, Indiana University Columbus, United StatesRobert Haack, Northern Research Station, Forest Service (USDA), United States
Angelica Pytel, Hillsdale College, United States
Copyright © 2023 Rendoll-Cárcamo, Gañán, Madriz, Convey and Contador. This is an open-access article distributed under the terms of the Creative Commons Attribution License (CC BY). The use, distribution or reproduction in other forums is permitted, provided the original author(s) and the copyright owner(s) are credited and that the original publication in this journal is cited, in accordance with accepted academic practice. No use, distribution or reproduction is permitted which does not comply with these terms.
*Correspondence: Javier Rendoll-Cárcamo, javier.rendoll@umag.cl