- 1School of Biosciences, Cardiff University, Cardiff, United Kingdom
- 2Wildlife Ecology & Health Group (WE&H) and Departament de Ciència Animal, Escola Tècnica Superior d’Enginyeria Agroalimentaria i Forestal i de Vetarinària (ETSEAFIV), Universitat de Lleida (UdL), Lleida, Spain
- 3Innovación en Gestión y Conservación de Ungulados S.L. (INGULADOS), Cáceres, Spain
- 4Departamento de Medicina Animal, Faculta de Veterinaria, Universidad de Extremadura, Cáceres, Spain
- 5Centre de Recerca en Sanitat Animal (CReSA, IRTA-UAB), Campus de la Universitat Autònoma de Barcelona, Barcelona, Spain
- 6Departamento de Sanitat i d’Anatomia Animals, Universitat Autònoma de Barcelona, Barcelona, Spain
- 7Wildlife Ecology and Health Group (WE&H), and Servei d’ Ecopatologia de Fauna Salvatge (SEFaS), Departament de Medicina i Cirurgia Animals, Facultat de Veterinària, Universitat Autònoma de Barcelona (UAB), Barcelona, Spain
Introduction: Tuberculosis (TB), caused by the Mycobacterium tuberculosis complex (MTC), is an important disease in both human and animal systems. Helminths are commonly found in coinfection with MTC and TB is often exacerbated in such coinfections. Long-term anthelmintic administration, to control helminths, can improve a host’s ability to control MTC infection. Mass drug administration programmes, in which anthelmintics are given only once or twice a year, leaving periods where helminth reinfection can occur, are common in both human and domestic animal populations. To date, the effect of such intermittent control programmes on MTC infection and severity has not been explored.
Methods: Here we investigate the consequences of a ten-day, annual, mass ivermectin administration on TB prevalence and severity in free-ranging juvenile and yearling (<2 years) wild boar (Sus scrofa).
Results: This single annual anthelmintic treatment administered over six years reduced TB severity. Further, the proportion of wild boar with severe TB continued to decrease with successive treatments. TB prevalence, however, did not decrease significantly over the course of the study.
Discussion: While ivermectin has direct anti-mycobacterial effects in vitro, the short duration of treatment means that the reduction in TB severity we observe in wild boar is unlikely to be accounted for by such a direct mechanism. Disruption of the helminth community and subsequent modification or enhancement of the host immune response is a potential mechanism. Future work should examine the consequences of annual anthelmintic drug administration on helminth community composition and structure and on the host immunological responses through time.
1 Introduction
Tuberculosis (TB), caused by bacteria of the Mycobacterium tuberculosis complex (MTC), is classed, in humans, as the second (after Covid-19) leading cause of death from a single infectious agent (World Health Organisation, 2021) and is also a significant health and economic threat to domestic animals (Pesciaroli et al., 2014; WOAH, 2022). Wildlife can act as reservoir hosts for MTC (e.g., European badger, Meles meles, in United Kingdom, brush-tailed possum, Trichosurus vulpecula, in New Zealand, African buffalo, Syncerus caffer, in South Africa or wild boar, Sus scrofa, in Spain; Fitzgerald and Kaneene, 2013). In humans, MTC frequently occurs in coinfection with helminths, particularly in low and middle-income countries (Salgame et al., 2013) and will regularly occur in coinfection in wildlife, given the ubiquity of helminths in these systems (Sepulveda and Kinsella, 2013). Controversy on the consequences of MTC and helminth coinfections exists, and while the species of coinfecting helminths and the outcome measure may affect the conclusions of these studies, most suggest that coinfection is detrimental to the host and that control of MTC is reduced (Potian et al., 2011; Li and Zhou, 2013; Babu and Nutman, 2016).
Immune control of MTC infections is complex but is largely mediated by a type 1 T-cell response (Th1) response (Babu and Nutman, 2016). Poorer control of MTC infection is observed in hosts with immune responses skewed towards a regulatory T-cell (Treg) response and production of alternatively activated macrophages (Babu and Nutman, 2016). Most helminth infections are characterised by a type 2 T-cell response. As a Th2 response is co-downregulatory with the Th1 response, this may reduce the effectiveness of MTC control by the host. Helminth infections tend to be chronic and as they progress, they also stimulate both Treg responses and the production of alternatively activated macrophages. Helminth coinfection, therefore, would be expected to decrease control of MTC, and infection with helminths has been associated with a shift from latent MTC infection (LTBI) to an active disease state (George et al., 2014; George et al., 2015; Kiflie et al., 2021). Helminth coinfection is also reported to reduce the efficacy of BCG vaccination programmes and the efficacy of TB testing (Elias et al., 2006; Badawy et al., 2013). The Th1 response is, however, pro-inflammatory and this inflammation can cause significant damage to host tissues. The downregulation of the Th1 response, due to the effects of Th2 and T-reg responses may therefore limit damage to the host caused by MTC infection (Garrido-Amaro et al., 2021).
Anthelmintic drug administration programmes to control helminths could help to control coinfecting microparasites (Elias et al., 2001; Hotez et al., 2006). Where anthelmintic was given to MTC-infected individuals, under conditions where reinfection was unlikely, treatment was shown to reduce Treg cells (Toulza et al., 2016) with the likely consequence of improved MTC control by the host. In an experimental study in wild African buffalo, long-term clearance of helminths was achieved via a slow-release bolus of fenbendazole with the consequence of reduced TB severity (Ezenwa and Jolles, 2015). Concurrently, TB increased in prevalence through increased transmission duration due to the MTC-infected buffalo living longer following treatment. In wild boar, the administration of ivermectin as an antiparasitic treatment enhanced host immunocompetence (López-Olvera et al., 2006), although whether this was due to the parasiticidal action of the drug (Uhlíř and Volf, 1992; Njoo et al., 1994; Savanur et al., 1996; Cooper et al., 2002) or to the activation of the immune response of the host (Wildenburg et al., 1998; Ali et al., 2002; Ali et al., 2003) remains unclear. Collectively, these studies suggest that the outcome of helminth reduction may be positive at the individual level but may have more complex outcomes at population scale.
Mass anthelmintic drug administration programmes are common in human populations (Humphries et al., 2012; Vercruysse et al., 2012) and in veterinary practice (Coles, 1997; Charlier et al., 2012). Such control programmes, however, usually administer the drugs at six or twelve-month intervals and helminth reinfection occurs over the intervening period. In-feed ivermectin administration is gaining importance for endo- and ectoparasite control in wildlife populations, including both intensively managed enclosures and free-ranging populations (Fernandez-De-Mera et al., 2004; López-Olvera et al., 2006; Moroni et al., 2022). While long-term control of helminths affects TB, the effects of punctuated anthelmintic administrations is not well understood. Here we evaluated the effects of a ten day per year mass administration of oral ivermectin on the dynamics of Mycobacterium bovis, the causative agent of tuberculosis, in a naturally infected wild boar population from central Spain. Wild boar are the main wildlife reservoir for MTC in the Mediterranean basin (Naranjo et al., 2008).
Wild boar are exploited for commercial hunting purposes and hence, wild boar populations receiving supplementary food in fenced estates are common in the Centre and South of the Iberian Peninsula (Gortazar et al., 2006). These enclosures enable assessment of the effect of short-term annual anthelmintic treatment on TB in wild boar. We hypothesise that even a short-term annual drug administration of anthelmintic will be sufficient to reduce TB severity and prevalence, due to the perturbation of the helminth community resulting in a likely immunological shift away from the Th2 and Treg responses associated with the majority of helminth infections.
2 Materials and methods
2.1 Study site, wild boar population and helminth community
Sampling was carried out in a private hunting estate of 2000 ha in Toledo, central Spain (39° 55′ 15.55″ N, 5°10′ 32.33″ E). The natural vegetation in the area is typically Mediterranean, dominated by scattered holm oaks (Quercus ilex) and shrubs of Cistus ladanifer, Erica spp. and Genista anglica. The study area was fenced and divided into two different and isolated enclosures used as the treatment area and control area. At the start of the study, wild boar density was 45 individuals/km2 and 25 individuals/km2 in the treatment and control areas respectively. The helminth community of these boar was assessed in 66 juvenile (> 6 ≤ 12 months) and yearling (≥ 12 to 24 months) boars following Gassó et al. (2015), lung nematodes were classified using Gassó et al. (2014) and Frontera et al. (2009) for gastrointestinal helminths. The helminth community included Metastrongylus spp., Ascarops strongylina, Physiocephalus sexalatus, Hyostrongylus rubidus, Globocephalus urosubulatus, and Oesophagostomum dentatum and there is one known acanthocephalan, Macracanthorhynchus hirudinaceus (see Supplementary Table 1 for further details). In both treatment and control areas supplementary food (1 kg of barley per individual) was provided daily from April to October, when there is natural food shortage. The supplementation was provided in five feeders specially designed to avoid access by non-target ungulates such as red deer, Cervus elaphus (Figure 1).
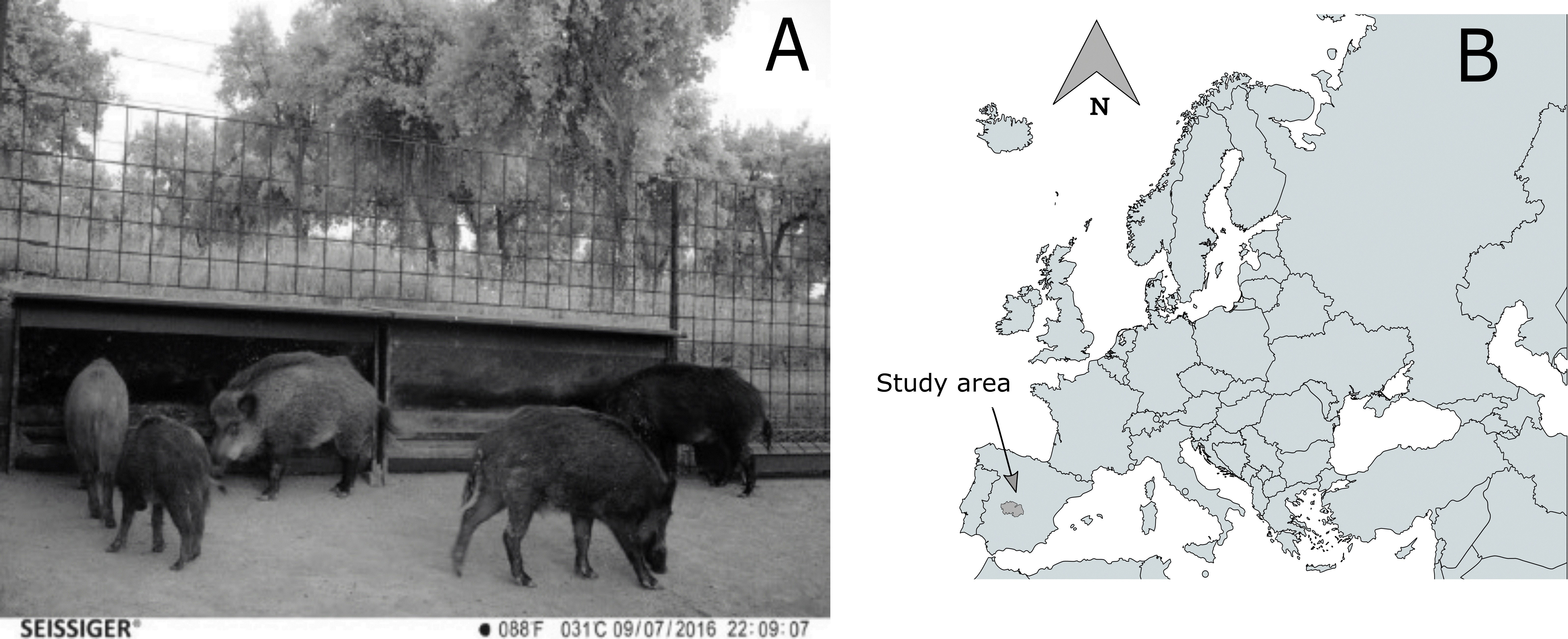
Figure 1 Wild boar herd feeding on ivermectin medicated feed (A) aimed at controlling helminth infections in experimental game states in Central Spain (B). Animals were fed at night to avoid the heat of summer. (Photo by Ingulados S.L.).
2.2 Experimental design
In the treatment enclosure, feed was coated in ivermectin at a concentration of 6.19 mg/g of feed (Ivomec Premix®, Merial) and administered for ten consecutive days (Campbell, 1989; Borgsteede et al., 2007). Since natural food is scarce during the summer, most wild boar feed on the supplement and hence receive an ivermectin dose sufficient to deworm them, at least partially and in most cases totally. In swine, oral ivermectin is effective at a single dose of 0.3 mg/kg (Vanhaecke et al., 2014) and thus a medium size boar of 50 kg would need 15 mg of drug (2.4 g of medicated feed) to be efficiently treated. Since our dosage is estimated to be thousands of times higher than the minimum required to deworm all boar should be dewormed. The high dose is assumed to be safe as ivermectin toxicity has only been observed in neonatal pigs and not in adults (Sanford et al., 1988).
2.3 Sampling procedure and processing
From winter 2012–13 to winter 2016–17, MTC infection status was systematically evaluated in 302 wild boar hunter-harvested in winter. As boar were not sacrificed for research purposes but legally hunter-harvested, within the framework of the regular annual hunting plan approved by the regional authority in charge of livestock and wildlife management, hence no ethics committee approvals were needed.
After the wild boar were culled, gender was assigned visually by inspecting genitalia (192 female and 102 male, 8 unrecorded), and age was estimated (83 adult, 89 juvenile, 123 yearling, with 7 unrecorded) by dental biometry (Gonçalves et al., 2015). A post-mortem examination of each boar was performed to assess the presence of TB-like macroscopic lesions affecting submandibular, retropharyngeal, mediastinal and/or mesenteric lymph nodes, as well as thoracic and/or abdominal organs (Martín-Hernando et al., 2007). Respiratory (trachea and lungs) and digestive (stomach, small and large intestine) tracts were removed and stored in individual plastic bags. Submandibular and/or retropharyngeal lymph nodes were dissected and stored in sterile containers for microbiological analyses.
2.4 Bovine tuberculosis status
The combination of microbiological culture and histopathology increases the sensitivity and specificity of TB surveys in wild boar (Santos et al., 2010). Diagnosis of TB was, therefore, based on the isolation of M. bovis in microbiological cultures from submandibular or retropharyngeal lymph nodes and from a piece of caudal lung lobe, as well as on the presence of microscopic granulomatous TB lesions. For bacterial culture, two grams of clean tissue were homogenized in 10 ml of sterile water with 0.2% albumin (Albumin from bovine serum Sigma, St Louis, MO, USA) for four minutes in a mechanical homogenizer (Smasher; AES Laboratories, Montreal, QC, Canada). The homogenized material was then decontaminated by the hexadecyl pyridinium chloride method (Corner and Trajstman, 1988). Finally, two Lowenstein–Jensen slants, with pyruvate and without glycerol, were inoculated in parallel and incubated for 6–8 weeks. Suspicious colonies obtained in microbiological cultures were identified as M. tuberculosis complex by PCR and Spoligotyped following standard methods (Kamerbeek et al., 1997), allowing their identification as M. bovis.
Animals were classified into three groups: (i) TB-free boars (negative culture and no signs of TB lesions), (ii) positive culture and/or localized gross lesions in submandibular or retropharyngeal lymph nodes (mild TB), and (iii) positive culture and disseminated gross lesions in lymph nodes and any other organ, e.g. lung, liver, mesenteric lymph nodes and/or spleen (severe TB).
2.5 Statistical analyses
We assessed the effect of the annual ten day anthelmintic ivermectin treatment on TB prevalence and subsequently TB severity in juvenile (<1 year) and wild yearling (between 1 and 2 years) boar using two generalised linear models with binomial error structures and logit link functions (GLM) within the R statistical package (R Core Team, 2023). We used GLM, as initial data assessment, using the ASReml-R v4 mixed modeling package (VSN International Ltd, Hemel Hempstead, United Kingdom), did not support the need to include either boar age or sampling year as non-linear terms (assessed by the change in Log Likelihood). To assess the effect of the anthelmintic treatment on TB prevalence, TB presence/absence data was included as the dependent variable (n = 209). To assess the effect of the treatment on TB severity we used only the infected subset of juvenile and yearling wild boar, as adult boar could have significant pathology from prior TB infection and this could obscure any effect of treatment on severity. Severity was included as the dependent variable with mild TB graded as 0 and severe TB graded as 1 (n = 83). In both models the initial terms were the main effects of sampling year, boar age, enclosure (treated or untreated), and second order interactions between treatment and each of the other main effects. The models were subsequently reduced in a stepwise manner using the Chi-squared statistics and associated p values, with p = 0.05 considered as the cut-off for significance. Differences between levels of factors and between timepoints were assessed using the ‘contrast’ package (Kuhn et al., 2022).
3 Results
Of the 209 juvenile and yearling wild boar considered in this study 83 (39.7%) were infected with MTC, of these, 37 (44.6%) suffered mild TB and the remainder (55.4%) were graded as severe infections. The initial model assessment showed that both binomial models were slightly overdispersed (1.35 and 1.18 respectively for the TB infection and TB severity models). Replacing the binomial with a quasibinomial error structure did not improve the dispersion, and, as the level of overdispersion was relatively small, the logit model was retained. TB prevalence did not differ significantly between treated and control enclosures nor with boar age, sex, or year of sampling (Tables 1, 2a). TB severity, however, was significantly associated with enclosure in interaction with time (X2 = 13.84, residual d.f. = 1, p<0.001, Table 2b), such that severe TB prevalence reduced with time in the treatment enclosure and rose substantially with time in the control enclosure (Figure 2; Table 3). Contrasts revealed that significant differences between enclosures were observed from the third year of treatment (i.e., from winter of 2014–15 on, Table 2).
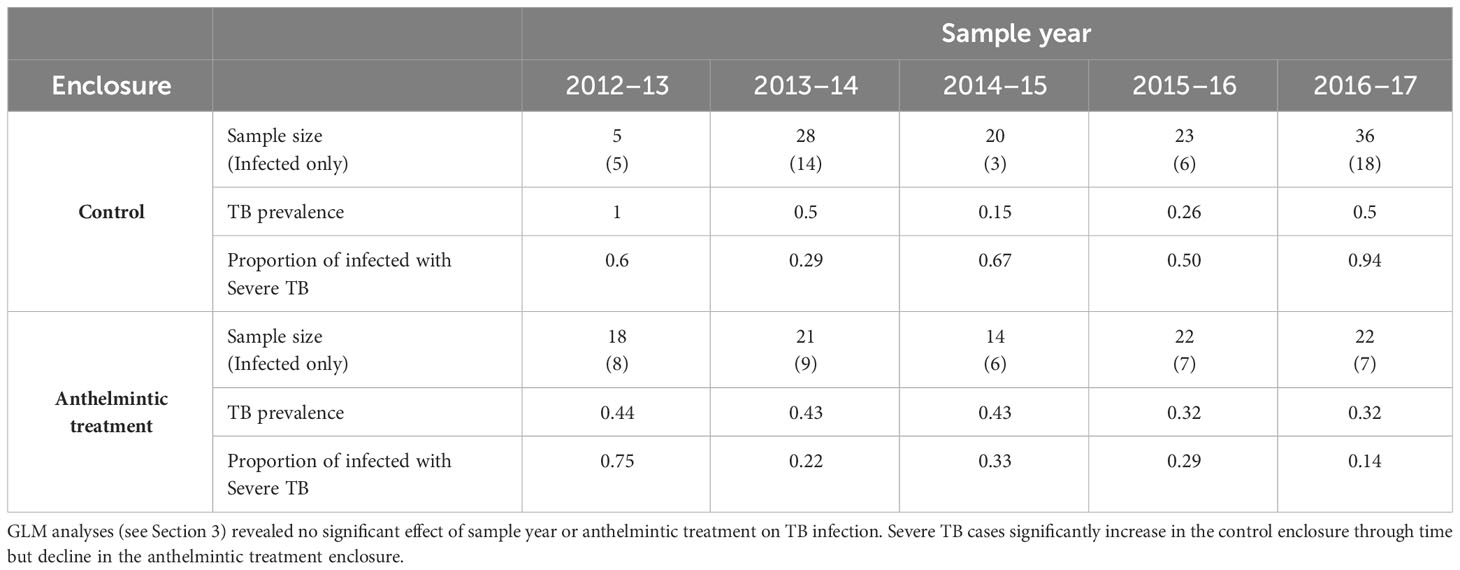
Table 1 Tuberculosis prevalence in all boar and proportion of young and juvenile boar with severe tuberculosis, through time within the control and anthelmintic treatment enclosures.
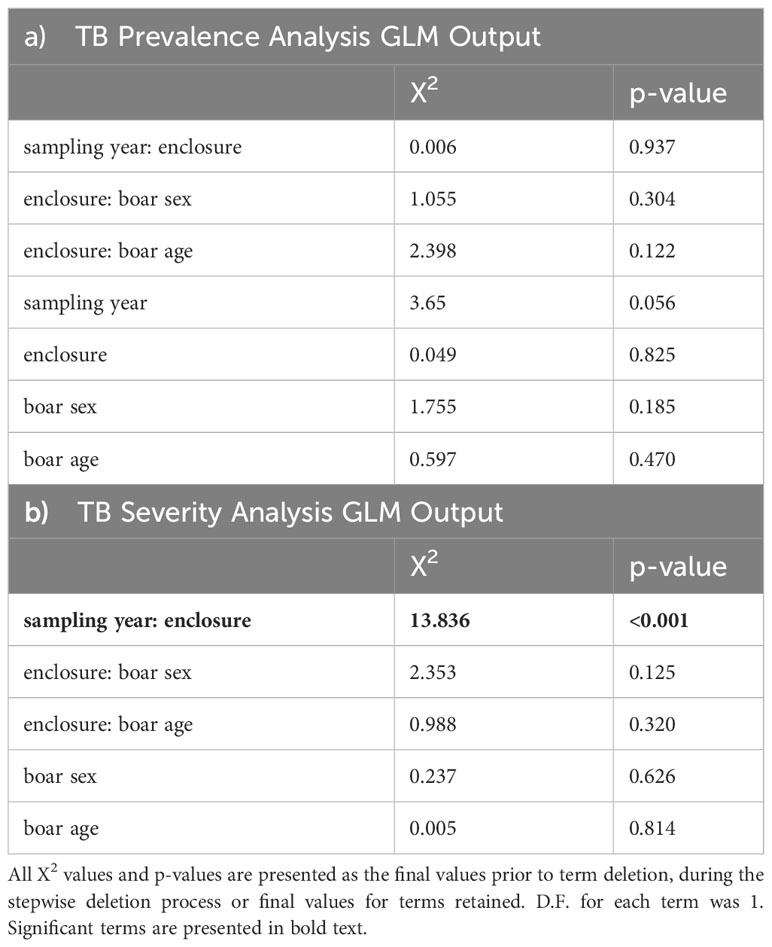
Table 2 Statistics and p-values from the GLM analyses of TB a) prevalence and b) severity in wild boar.
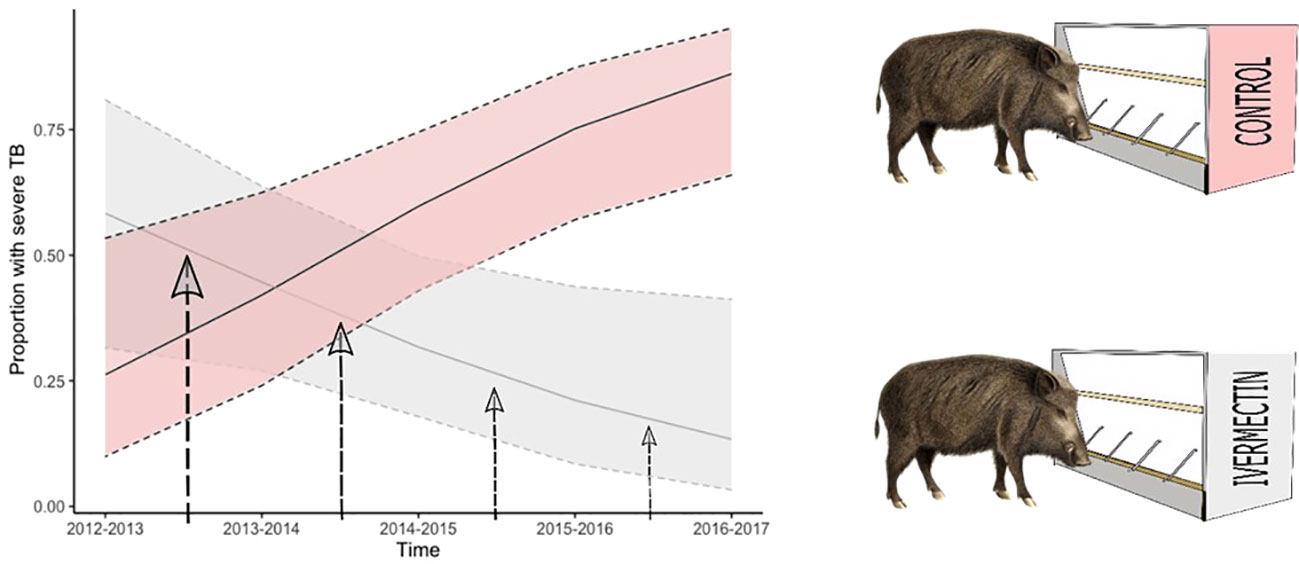
Figure 2 Relationship between sample year and enclosure treatment group and the proportion of infected animals that were graded as having severe tuberculosis (TB). Lines represent the GLM predicted proportion of infected juvenile and yearling boar with severe TB. Shading and outer dotted lines represent the 95% confidence intervals of the prediction. Light red shading represents the control enclosure and the anthelmintic treated enclosure (solid line and grey shading for 95% confidence intervals of the prediction). Vertical arrows represent the yearly two-week treatments during summer.
4 Discussion
As hypothesised the ten day annual anthelmintic treatment was associated with a reduced proportion of severe TB but no change in TB prevalence in yearling or juvenile wild boar. Our findings on severity agree with the response observed in a long-term anthelmintic treatment in African buffalo (Ezenwa and Jolles, 2015), where TB severity decreased in anthelmintic treated animals. Conversely, prevalence in these wild buffalo increased, because the dewormed buffalo lived longer and hence transmitted MTC for a longer period. In the boar, anthelmintic dose was limited to ten days per year and as even ultra-low doses of MTC (1–3 bacteria) have been shown to result in infection (Plumlee et al., 2021) contact among boar at feeders is likely sufficient to maintain infection rates, even if overall MTC excretion is reduced after treatment (Navarro-Gonzalez et al., 2013). TB severity in our two enclosures only diverges significantly from the third year of treatment. Our work therefore highlights that any effect of punctuated mass drug administration programmes may not appear in short term studies and that there is a need for more long-term monitoring in both human and animal populations.
The reduction of the Th2 and Treg responses, that are likely to follow deworming, and the subsequent increase in the protective Th1 response, may have enabled the boar to better control MTC. An immune enhancement effect of ivermectin has previously been observed in dewormed wild boar (López-Olvera et al., 2006). Such effects of ivermectin treatment have also been observed in studies on other hosts (Wildenburg et al., 1998; Ali et al., 2002; Ali et al., 2003; López-Olvera et al., 2006). It may seem counterintuitive then, that TB prevalence is not lower in the treatment enclosure. Our experimental protocol, however, does not give us a measure of the number of MTC bacteria within the host. It may be that while transmission was sufficient to maintain prevalence, MTC bacterial numbers were reduced in treated hosts, explaining the reduced TB severity. Further, our severity analysis only includes yearling and juvenile boar, hence, the fact that significant divergence in severity between the enclosures only occurs after two years, and is cumulative over time, is not a simple consequence of pathology increasing in infected individuals over time. We propose that the observed difference may result from differences in infectious dose at the population level. If MTC bacterial numbers were reduced in treated hosts, then the dose passed from boar to boar in the treatment enclosure would be lower, compared to those in the control enclosure. Work in other species suggests that TB pathology is related to the received infectious dose (Kong et al., 2009; Saini et al., 2012; Tsenova et al., 2020). Onward transmission of increasingly lower doses in the treatment enclosure and increasingly high infectious doses in the control enclosure, is one mechanism that could explain our observations.
Deworming could also have direct beneficial effects allowing boar to better resist and/or repair damage from MTC infection. Fattening pigs showed mass gain and increased body condition, after removal of Ascaris suum (Boes et al., 2010). Improved ability to repair damage may also explain why we see no evidence that removing helminths increases inflammatory damage from MTC. A further possibility for the response to helminth removal is a direct effect of the anthelmintic on the mycobacterium. Ivermectin and other avermectins have in vitro activity against Mycobacterium spp. (Lim et al., 2013). Given the transient nature of our treatment, the high transmission rate of TB and the fact that no change in TB prevalence was observed in our populations, such a direct effect appears unlikely. Overall, the alteration of the boar immune response resulting from helminth removal is the more likely mechanism by which this helminth-MTC relationship is mediated, potentially bolstered by the improved health of the host.
There is considerable variation in the immune response induced by helminths depending on the species and the stage of infection (Anthony et al., 2007; Allen and Maizels, 2011) (e.g., schistosome cercaria and adults worms bias toward a Th1 response whilst their eggs stimulate the Th2 pathway; Caldas et al., 2008). In our study, helminths are only likely to be removed for around a single month each year, with recolonization occurring after this period. This perturbation may, however, be sufficient to keep helminth numbers low for a more prolonged period or might cause successional changes in the helminth community, in turn impacting the precise strength and form of the immune response in the boar. While we know the species of helminths found in the boar, there is a lack of knowledge about the immune response stimulated by each species and stage. In interpreting this study, we must therefore be cautious, as similar perturbations with a different group of helminths could produce different outcomes.
The majority of emerging and re-emerging infectious diseases of humans and domestic animals originate in wildlife reservoirs (Jones et al., 2008; Morse et al., 2012), where coinfection with helminths is ubiquitous. To date, anthelmintic administration in wildlife populations has largely been undertaken for experimental purposes (e.g., Ferrari et al., 2009; Knowles et al., 2013; Pedersen and Antonovics, 2013; Pedersen and Fenton, 2015; Moroni et al., 2022). Antiparasitic drug administration (including ivermectin) is, however, being considered for disease management in captive, intensively managed, and free-ranging wildlife populations (López-Olvera et al., 2006; Sánchez-Isarria, 2008b; Sánchez-Isarria et al., 2008a; Espinosa et al., 2017; Old et al., 2021; Wilkinson et al., 2021; Bains et al., 2022; Wilkinson et al., 2023). Only a few studies have attempted to assess the consequences of the removal of non-target pathogens (e.g., deworming) on the dynamics of concurrent infectious diseases, and these have yielded contrasting results (Pedersen and Antonovics, 2013; Ezenwa and Jolles, 2015; Sweeny et al., 2020). Overall, these studies suggest that parasite communities are robust to the perturbations caused by punctuated deworming, since the effect of anthelmintic treatment on nematodes is usually short-lived (Knowles et al., 2013). Subtle effects on community assemblage and recolonization order might, however, have longer term consequences for the relationship between the helminths and the coinfecting microparasite communities, not least, by altering the host immune response, which may drive the interactions between them.
While our study adds weight to the argument that intermittent mass anthelmintic drug administration can be beneficial to wild hosts, there needs to be careful consideration before oral administration of such drugs to free-ranging wildlife populations. Utility, efficacy, and potential risks to target and non-target host species, and even to human health should be carefully considered (Rowe et al., 2019; Moroni et al., 2020; Valldeperes et al., 2022). In our study the amount of medicated feed required for deworming was 2.4 g, and individual over-dosing is therefore likely to have occurred. Such over-dosing has been reported for topical applications of macrocyclic lactones in wombats (Old et al., 2021; Mounsey et al., 2022). Overdosing of some drugs may be harmful to the host and/or result in release of the drug into the environment while underdosing may result in incomplete removal. In both forms of inappropriate dosing the probability of the development of drug resistance increases (Currie et al., 2004; Bliss et al., 2008; Terada et al., 2010; Vermunt et al., 2011). Detrimental environment consequences are also possible, in particular, for coprophagous insects and other non-target invertebrates (Sommer et al., 1992; Herd et al., 1996; Verdú et al., 2015; Verdú et al., 2018). The benefits for individual hosts must therefore be carefully weighed against the risk to other hosts and to the environment.
TB eradication programmes in Spain are based on skin-testing and slaughter of livestock (Napp et al., 2019) and/or hunting to control wild reservoir hosts (Boadella et al., 2012; García-Jiménez et al., 2013; Mentaberre et al., 2014). Pharmacological interventions using vaccines with heat-inactivated Mycobacterium bovis have also been demonstrated to be efficient for TB control in wild boar farms (Díez-Delgado et al., 2017), and free-ranging populations (Díez-Delgado et al., 2018). To our knowledge, our study is the first attempt to control TB in wild boar with a helminth targeted intervention.
Overall, our study provides robust evidence for the utility of helminth removal from wild hosts where they are managed and where they pose a potential threat for spillover of MTC infection to domestic animal or human populations. Nevertheless, there were limitations to our study. The treatment enclosure had a larger number of boar than the control enclosure at the start of the experiment. As higher densities generally lead to higher transmission, for directly transmitted pathogens, it is possible that any subtle reduction in TB transmission resulting from helminth removal, could have been masked by higher transmission resulting from higher host density. We are also limited by having no immunological information from our boar, hence we can only hypothesise regarding the mechanism by which helminth removal affected MTC/TB. Nevertheless, our work clearly demonstrates that TB is affected by coinfection with helminths and that their removal improves the outcome for individual hosts. More work will be required to explore the complex consequences at the population level. Our study also lends support to the growing body of research demonstrating that helminths cannot be ignored if we are to understand and successfully control important microparasitic diseases.
Data availability statement
The raw data supporting the conclusions of this article will be made available by the authors, without undue reservation.
Ethics statement
Ethical approval was not required for the studies involving animals in accordance with the local legislation and institutional requirements because animals are game species legally hunted during the game season. Written informed consent was obtained from the owners for the participation of their animals in this study.
Author contributions
Study conception and design: JL, DG, JS, PF-L and ES. Data collection: DG, PG, DR, GM, RV, PF-L and JR-O. TB diagnosis: PG, RV and WG. Analysis and interpretation of results: JL, DG, RV, PG and ES. Drafted manuscript preparation: JL, DG, JS, CG-A, IT-B, JR-O, and ES. All authors contributed to the article and approved the submitted version.
Funding
ES is supported by the Spanish Ministerio de Ciencia Innovación y Universidades (MICINN) through a Ramon y Cajal agreement (RYC-2016-21120). GM is a Serra Hunter Fellow.
Acknowledgments
The authors would like to thank the gamekeepers involved in the treatment and sampling campaigns.
Conflict of interest
The authors declare that the research was conducted in the absence of any commercial or financial relationships that could be construed as a potential conflict of interest.
Publisher’s note
All claims expressed in this article are solely those of the authors and do not necessarily represent those of their affiliated organizations, or those of the publisher, the editors and the reviewers. Any product that may be evaluated in this article, or claim that may be made by its manufacturer, is not guaranteed or endorsed by the publisher.
Supplementary material
The Supplementary Material for this article can be found online at: https://www.frontiersin.org/articles/10.3389/fevo.2023.1186295/full#supplementary-material
References
Ali M. M., Baraka O. Z., AbdelRahman S. I., Sulaiman S. M., Williams J. F., Homeida M. M., et al. (2003). Immune responses directed against microfilariae correlate with severity of clinical onchodermatitis and treatment history. J. Infect. Dis. 187, 714–717. doi: 10.1086/367709
Ali M. M. M., Mukhtar M. M., Baraka O. Z., Homeida M. M. A., Kheir M. M., Mackenzie C. D. (2002). Immunocompetence may be important in the effectiveness of Mectizan® (ivermectin) in the treatment of human onchocerciasis. Acta Trop. 84, 49–53. doi: 10.1016/S0001-706X(02)00117-1
Allen J. E., Maizels R. M. (2011). Diversity and dialogue in immunity to helminths. Nat. Rev. Immunol. 116, 375–388. doi: 10.1038/nri2992
Anthony R. M., Rutitzky L. I., Urban J. F., Stadecker M. J., Gause W. C. (2007). Protective immune mechanisms in helminth infection. Nat. Rev. Immunol. 712, 975–987. doi: 10.1038/nri2199
Babu S., Nutman T. B. (2016). Helminth-tuberculosis co-infection: an immunologic perspective. Trends Immunol. 37, 597–607.
Badawy A. A., Yahya R. S., Awad S. I., Al-Sawah G. A., Kizilbash N. A. (2013). Relationship between NRAMP1 gene polymorphism and efficacy of BCG vaccine in a helminth-infected population. Genet. Mol. Res. 12, 3048–3056. doi: 10.4238/2013.January.30.4
Bains J., Carver S., Hua S. (2022). Pathophysiological and pharmaceutical considerations for enhancing the control of Sarcoptes scabiei in wombats through improved transdermal drug delivery. Front. Vet. Sci. 9. doi: 10.3389/FVETS.2022.944578/BIBTEX
Bliss D. H., Moore R. D., Kvasnicka W. G. (2008). Parasite resistance in US cattle. Am. Assoc. Bov Pract. Conf. Proc. 41, 109–114. doi: 10.21423/AABPPRO20084377
Boadella M., Vicente J., Ruiz-Fons F., de la Fuente J., Gortázar C. (2012). Effects of culling Eurasian wild boar on the prevalence of Mycobacterium bovis and Aujeszky’s disease virus. Prev. Vet. Med. 107, 214–221. doi: 10.1016/J.PREVETMED.2012.06.001
Boes J., Kanora A., Havn K. T., Christiansen S., Vestergaard-Nielsen K., Jacobs J., et al. (2010). Effect of Ascaris suum infection on performance of fattening pigs. Vet. Parasitol. 172, 269–276. doi: 10.1016/J.VETPAR.2010.05.007
Borgsteede F. H. M., Gaasenbeek C. P. H., Nicoll S., Domangue R. J., Abbott E. M. (2007). A comparison of the efficacy of two ivermectin formulations against larval and adult Ascaris suum and Oesophagostomum dentatum in experimentally infected pigs. Vet. Parasitol. 146, 288–293. doi: 10.1016/j.vetpar.2007.02.027
Caldas I. R., Campi-Azevedo A. C., Oliveira L. F. A., Silveira A. M. S., Oliveira R. C., Gazzinelli G. (2008). Human Schistosomiasis mansoni: Immune responses during acute and chronic phases of the infection. Acta. Trop. 108, 109–117. doi: 10.1016/J.ACTATROPICA.2008.05.027
Charlier J., Levecke B., Devleesschauwer B., Vercruysse J., Hogeveen H. (2012). The economic effects of whole-herd versus selective anthelmintic treatment strategies in dairy cows. J. Dairy Sci. 95, 2977–2987. doi: 10.3168/JDS.2011-4719
Coles G. C. (1997). Nematode control practices and anthelmintic resistance on British sheep farms. Vet. Rec. 141, 91–93. doi: 10.1136/VR.141.4.91
Cooper P. J., Schwartz L. B., Irani A. M., Awadzi K., Guderian R. H., Nutman T. B. (2002). Association of transient dermal mastocytosis and elevated plasma tryptase levels with development of adverse reactions after treatment of onchocerciasis with ivermectin. J. Infect. Dis. 186, 1307–1313. doi: 10.1086/344318
Corner L. A., Trajstman A. C. (1988). An evaluation of 1-hexadecylpyridinium chloride as a decontaminant in the primary isolation of Mycobacterium bovis from bovine lesions. Vet. Microbiol. 18, 127–134. doi: 10.1016/0378-1135(88)90058-2
Currie B. J., Harumal P., McKinnon M., Walton S. F. (2004). First documentation of in vivo and in vitro ivermectin resistance in Sarcoptes scabiei. Clin. Infect. Dis. 39, 8–12. doi: 10.1086/421776/2/39-1-E8-FIG003.GIF
Díez-Delgado I., Rodríguez O., Boadella M., Garrido J. M., Sevilla I. A., Bezos J., et al. (2017). Parenteral vaccination with heat-inactivated Mycobacterium bovis reduces the prevalence of tuberculosis-compatible lesions in farmed wild boar. Transbound Emerg. Dis. 64, 18–21. doi: 10.1111/TBED.12526
Díez-Delgado I., Sevilla I. A., Romero B., Tanner E., Barasona J. A., White A. R., et al. (2018). Impact of piglet oral vaccination against tuberculosis in endemic free-ranging wild boar populations. Prev. Vet. Med. 155, 11–20. doi: 10.1016/J.PREVETMED.2018.04.002
Elias D., Mengistu G., Akuffo H., Britton S. (2006). Are intestinal helminths risk factors for developing active tuberculosis? Trop. Med. Int. Heal. 11, 551–558. doi: 10.1111/j.1365-3156.2006.01578.x
Elias D., Wolday D., Akuffo H., Petros B., Bronner U., Britton S., et al. (2001). Effect of deworming on human T cell responses to mycobacterial antigens in helminth-exposed individuals before and after bacille Calmette-Guérin (BCG) vaccination. Clin. Exp. Immunol. 123, 219–225. doi: 10.1046/j.1365-2249.2001.01446.x
Espinosa J., López-Olvera J. R., Cano-Manuel F. J., Fandos P., Pérez J. M., López-Graells C., et al. (2017). Guidelines for managing captive Iberian ibex herds for conservation purposes. J. Nat. Conserv. 40, 24–32. doi: 10.1016/J.JNC.2017.09.002
Ezenwa V. O., Jolles A. E. (2015). Opposite effects of anthelmintic treatment on microbial infection at individual versus population scales. Science. 347, 175–177. doi: 10.1126/science.1261714
Fernandez-De-Mera I. G., Vicente J., Gortazar C., Höfle U., Fierro Y. (2004). Efficacy of an in-feed preparation of ivermectin against helminths in the European wild boar. Parasitol. Res. 92, 133–136. doi: 10.1007/S00436-003-0976-5/METRICS
Ferrari N., Cattadori I. M., Rizzoli A., Hudson P. J. (2009). Heligmosomoides polygyrus reduces infestation of Ixodes ricinus in free-living yellow-necked mice, Apodemus flavicollis. Parasitology. 136, 305–316. doi: 10.1017/S0031182008005404
Fitzgerald S. D., Kaneene J. B. (2013). Wildlife reservoirs of bovine tuberculosis worldwide: hosts, pathology, surveillance, and control. Vet. Pathol. 50 (3), 488–499. doi: 10.1177/0300985812467472
García-Jiménez W. L., Benítez-Medina J. M., Fernández-Llario P., Abecia J. A., García-Sánchez A., Martínez R., et al. (2013). Comparative Pathology of the Natural infections by Mycobacterium bovis and by Mycobacterium caprae in Wild Boar (Sus scrofa). Transbound Emerg. Dis. 60, 102–109. doi: 10.1111/j.1865-1682.2012.01321.x
Garrido-Amaro C., Cardona P., Gassó D., Arias L., Velarde R., Tvarijonativiciute A., et al. (2021). Protective effect of intestinal helminthiasis against tuberculosis progression is abrogated by intermittent food deprivation. Front. Immunol. 12. doi: 10.3389/fimmu.2021.627638
Gassó D., Feliu C., Ferrer D., Mentaberre G., Casas-Díaz E., Velarde R., et al. (2015). Uses and limitations of faecal egg count for assessing worm burden in wild boars. Vet. Par. 209 (1-2), 133–137. doi: 10.1016/j.vetpar.2015.02.006
Gassó D., Rossi L., Mentaberre G., Casas E., Velarde R., Nosal P., et al. (2014). An identification key for the five more common species of Metastrongylus. Parasitol. Res. 113, 3495–3500. doi: 10.1007/s00436-014-4001-y
George P. J., Kumar N. P., Sridhar R., Hanna L. E., Nair D., Banurekha V. V., et al. (2014). Coincident helminth infection modulates systemic inflammation and immune activation in active pulmonary tuberculosis. PloS Negl. Trop. Dis. 8. doi: 10.1371/journal.pntd.0003289
George P. J., Pavan Kumar N., Jaganathan J., Dolla C., Kumaran P., Nair D., et al. (2015). Modulation of pro- and anti-inflammatory cytokines in active and latent tuberculosis by coexistent Strongyloides stercoralis infection. Tuberculosis. 95, 822–828. doi: 10.1016/J.TUBE.2015.09.009
Gonçalves P., Risco D., Fernández-Llario P., Barquero-Pérez O., Serrano E., Hermoso-de-Mendoza J., et al. (2015). A new method for ageing wild boar using dental measures. Ecol. Indic. 62, 328–332. doi: 10.1016/j.ecolind.2015.11.013
Gortazar C., Acevedo P., Ruiz-Fons F., Vicente J. (2006). Disease risks and overabundance of game species. Eur. J. Wildl. Res. 52, 81–87. doi: 10.1007/s10344-005-0022-2
Herd R. P., Sams R. A., Ashcraft S. M. (1996). Persistence of ivermectin in plasma and faeces following treatment of cows with ivermectin sustained-release, pour-on or injectable formulations. Int. J. Parasitol. 26, 1087–1093. doi: 10.1016/S0020-7519(96)80007-5
Hotez P. J., Molyneux D. H., Fenwick A., Ottesen E., Sachs S. E., Sachs J. D. (2006). Incorporating a rapid-impact package for neglected tropical diseases with programs for HIV/AIDS, tuberculosis, and malaria: A comprehensive pro-poor health policy and strategy for the developing world. PloS Med. 3, 576–584. doi: 10.1371/journal.pmed.0030102
Humphries D., Nguyen S., Boakye D., Wilson M., Cappello M. (2012). The promise and pitfalls of mass drug administration to control intestinal helminth infections. Curr. Opin. Infect. Dis. 25, 584–589. doi: 10.1097/QCO.0B013E328357E4CF
Jones K. E., Patel N. G., Levy M. A., Storeygard A., Balk D., Gittleman J. L., et al. (2008). Global trends in emerging infectious diseases. Nature. 451, 990–993. doi: 10.1038/nature06536
Kamerbeek J., Schouls L., Kolk A., Van Agterveld M., Van Soolingen D., Kuijper S., et al. (1997). Simultaneous detection and strain differentiation of Mycobacterium tuberculosis for diagnosis and epidemiology. J. Clin. Microbiol. 35, 907–914. doi: 10.1128/JCM.35.4.907-914.1997
Kiflie A., Bewket G., Abate E., Schön T., Blomgran R. (2021). Differential effects of asymptomatic Ascaris lumbricoides, Schistosoma mansoni or hook worm infection on the frequency and TGF-beta-producing capacity of regulatory T cells during active tuberculosis. Tuberculosis. 131, 102126. doi: 10.1016/J.TUBE.2021.102126
Knowles S. C. L., Fenton A., Petchey O. L., Jones T. R., Barber R., Pedersen A. B. (2013). Stability of within-host-parasite communities in a wild mammal system. Proc. Biol. Sci. 280, 20130598. doi: 10.1098/rspb.2013.0598
Kong Y., Subbian S., Cirillo S. L. G., Cirillo J. D. (2009). Application of optical imaging to study of extrapulmonary spread by tuberculosis. Tuberculosis. 89, S15–S17. doi: 10.1016/S1472-9792(09)70006-X
Kuhn M., Weston S., Wing J., Forester J., Thaler T. (2022). contrast: A Collection of Contrast Methods (R package version 0.24.2).
Li X. X., Zhou X. N. (2013). Co-infection of tuberculosis and parasitic diseases in humans: a systematic review. Parasit. Vectors. 6, 79. doi: 10.1186/1756-3305-6-79
Lim L. E., Vilchèze C., Ng C., Jacobs W. R., Ramón-García S., Thompson C. J. (2013). Anthelmintic avermectins kill Mycobacterium tuberculosis, including multidrug-resistant clinical strains. Antimicrob. Agents Chemother. 57, 1040–1046. doi: 10.1128/AAC.01696-12/ASSET/B2796A73-CD3E-473D-AD20-E7EDA6C72B64/ASSETS/GRAPHIC/ZAC9991015560003.JPEG
López-Olvera J. R., Höfle U., Vicente J., Fernández-de-Mera I. G., Gortázar C. (2006). Effects of parasitic helminths and ivermectin treatment on clinical parameters in the European wild boar (Sus scrofa). Parasitol. Res. 98, 582–587. doi: 10.1007/s00436-005-0099-2
Martín-Hernando M. P., Höfle U., Vicente J., Ruiz-Fons F., Vidal D., Barral M., et al. (2007). Lesions associated with Mycobacterium tuberculosis complex infection in the European wild boar. Tuberculosis. 87, 360–367. doi: 10.1016/j.tube.2007.02.003
Mentaberre G., Romero B., De Juan L., Navarro-González N., Velarde R., Mateos A., et al. (2014). Long-term assessment of wild boar harvesting and cattle removal for bovine tuberculosis control in free ranging populations. PLoS ONE 9, e88824. doi: 10.1371/JOURNAL.PONE.0088824
Moroni B., Granados Torres J. E., López-Olvera J. R., Espinosa C. J., Ráez-Bravo A., Mentaberre G., et al. (2022). Ivermectin plasma concentration in iberian ibex (Capra pyrenaica) following oral administration: A pilot study. Front. Vet. Sci. 9. doi: 10.3389/FVETS.2022.830157/BIBTEX
Moroni B., Valldeperes M., Serrano E., López-Olvera J. R., Lavín S., Rossi L. (2020). Comment on: “The treatment of sarcoptic mange in wildlife: A systematic review”. Parasit. Vectors. 13, 1–4. doi: 10.1186/S13071-020-04347-0/METRICS
Morse S. S., Mazet J. A. K., Woolhouse M., Parrish C. R., Carroll D., Karesh W. B., et al. (2012). Prediction and prevention of the next pandemic zoonosis. Lancet. 380, 1956–1965. doi: 10.1016/S0140-6736(12)61684-5
Mounsey K., Harvey R. J., Wilkinson V., Takano K., Old J., Stannard H., et al. (2022). Drug dose and animal welfare: important considerations in the treatment of wildlife. Parasitol. Res. 121, 1065–1071. doi: 10.1007/S00436-022-07460-4/FIGURES/1
Napp S., Ciaravino G., Pérez de Val B., Casal J., Saéz J. L., Alba A. (2019). Evaluation of the effectiveness of the surveillance system for tuberculosis in cattle in Spain. Prev. Vet. Med. 173, 104805. doi: 10.1016/J.PREVETMED.2019.104805
Naranjo V., Gortazar C., Vicente J., de la Fuente J. (2008). Evidence of the role of European wild boar as a reservoir of Mycobacterium tuberculosis complex. Vet. Microbiol. 127, 1–9. doi: 10.1016/j.vetmic.2007.10.002
Navarro-Gonzalez N., Fernandez-Llario P., Perez-Martin J. E., Mentaberre G., Lopez-Martin J. M., Lavin S., et al. (2013). Supplemental feeding drives endoparasite infection in wild boar in Western Spain. Vet. Parasitol. 196, 114–123. doi: 10.1016/j.vetpar.2013.02.019
Njoo F. L., Hack C. E., Oosting J., Luyendijk L., Stilma J. S., Kijlstra A. (1994). C-reactive protein and interleukin-6 are elevated in onchocerciasis patients after ivermectin treatment. J. Infect. Dis. 170, 663–668. doi: 10.1093/INFDIS/170.3.663
Old J. M., Skelton C. J. A., Stannard H. J. (2021). The use of Cydectin® by wildlife carers to treat sarcoptic mange in free-ranging bare-nosed wombats (Vombatus ursinus). Parasitol. Res. 120, 1077–1090. doi: 10.1007/S00436-020-07012-8/FIGURES/10
Pedersen A. B., Antonovics J. (2013). Anthelmintic treatment alters the parasite community in a wild mouse host. Biol. Lett. 9, 20130205. doi: 10.1098/rsbl.2013.0205
Pedersen A. B., Fenton A. (2015). The role of antiparasite treatment experiments in assessing the impact of parasites on wildlife. Trends Parasitol. 31, 200–211. doi: 10.1016/j.pt.2015.02.004
Pesciaroli M., Alvarez J., Boniotti M. B., Cagiola M., Di Marco V., Marianelli C., et al. (2014). Tuberculosis in domestic animal species. Res. Vet. Sci. 97, S78–S85. doi: 10.1016/j.rvsc.2014.05.015
Plumlee C. R., Duffy F. J., Gern B. H., Delahaye J. L., Cohen S. B., Stoltzfus C. R., et al. (2021). Ultra-low dose aerosol infection of mice with Mycobacterium tuberculosis more closely models human tuberculosis. Cell Host Microbe. 29, 68–82.e5. doi: 10.1016/J.CHOM.2020.10.003
Potian J., Rafi W., Bhatt K., McBride A., Gause W. C., Salgame P. (2011). Preexisting helminth infection induces inhibition of innate pulmonary anti-tuberculosis defense by engaging the IL-4 receptor pathway. J. Exp. Med. 208, 1863–1874. doi: 10.1084/jem.20091473
R Core Team (2023). R: A Language and Environment for Statistical Computing. R Foundation for Statistical Computing, Vienna, Austria. https://www.R-project.org.
Rowe M. L., Whiteley P. L., Carver S. (2019). The treatment of sarcoptic mange in wildlife: A systematic review. Parasit. Vectors. 12, 1–14. doi: 10.1186/S13071-019-3340-Z/TABLES/5
Saini D., Hopkins G. W., Seay S. A., Chen C. J., Perley C. C., Click E. M., et al. (2012). Ultra-low dose of Mycobacterium tuberculosis aerosol creates partial infection in mice. Tuberculosis. 92, 160–165. doi: 10.1016/j.tube.2011.11.007
Salgame P., Yap G. S., Gause W. C. (2013). Effect of helminth-induced immunity on infections with microbial pathogens. Nat. Immunol. 14, 1118–1126. doi: 10.1038/ni.2736
Sánchez-Isarria M. A., Hermoso J., Theureau de la Peña J., Casanova G., Burgui J. M., Sanchís G. (2008a). “Metodología empleada en la estrategia del control de la sarna sarcóptica en la cabra montés de la Reserva Valenciana de Caza de la Muela de Cortes entre los años 2002-2007 (Valencia),” in Tendencias Actuales En El Estudio y Conservación de Los Caprinos Europeos. Eds. Granados J. E., Cano-Manuel F. J., Fandos P., Cadenas de Llano R. (Granada, Spain: Junta de Andalucía), 255–268.
Sánchez-Isarria M. A., Hermoso J., Theureau de la Peña J., Casanova G., Burgui J. M., Sanchís G. (2008b). “La ivermectina como tratamiento de la sarna sarcóptica en el medio natural: vías de aplicación,” in Tendencias Actuales En El Estudio y Conservación de Los Caprinos Europeos. Eds. Granados J. E., Cano-Manuel F. J., Fandos P., Cadenas de Llano R. (Granada, Spain: Junta de Andalucía Consejería de Medio Ambiente), 269–276.
Sanford S. E., Rehmtulla A. J., Josephson G. K. (1988). Ivermectin overdose and toxicosis in neonatal pigs. Can. Vet. J. 29, 735–736. doi: 10.1079/cabicompendium.7829
Santos N., Geraldes M., Afonso A., Almeida V., Correia-Neves M. (2010). Diagnosis of tuberculosis in the wild boar (Sus scrofa): A comparison of methods applicable to hunter-harvested animals. PLoS ONE 5, e12663. doi: 10.1371/JOURNAL.PONE.0012663
Savanur N. H., Krishnappa G., Sastry K. N. V., Narayana K. (1996). Effect of ivermectin on lymphocyte status in rabbits. Indian Vet. J. 73, 501–503.
Sepulveda M. S., Kinsella J. M. (2013). Helminth collection and identification from wildlife. J. Vis. Exp. 82, e51000. doi: 10.3791/51000
Sommer C., Steffansen B., Nielsen B. O., Grønvold J., Vagn Jensen K. M., Brøchner Jespersen J., et al. (1992). Ivermectin excreted in cattle dung after subcutaneous injection or pour-on treatment: concentrations and impact on dung fauna. Bull. Entomol. Res. 82, 257–264. doi: 10.1017/S0007485300051804
Sweeny A. R., Thomason C. A., Carbajal E. A., Hansen C. B., Graham A. L., Pedersen A. B. (2020). Experimental parasite community perturbation reveals associations between Sin Nombre virus and gastrointestinal nematodes in a rodent reservoir host. Biol. Lett. 16, 20200604. doi: 10.1098/RSBL.2020.0604
Terada Y., Murayama N., Ikemura H., Morita T., Nagata M. (2010). Sarcoptes scabiei var. canis refractory to ivermectin treatment in two dogs. Vet. Dermatol. 21, 608–612. doi: 10.1111/J.1365-3164.2010.00895.X
Toulza F., Tsang L., Ottenhoff T. H. M., Brown M., Dockrell H. M. (2016). Mycobacterium tuberculosis-specific CD4+ T-cell response is increased, and Treg cells decreased, in anthelmintic-treated patients with latent TB. Eur. J. Immunol. 46, 752–761. doi: 10.1002/eji.201545843
Tsenova L., Fallows D., Kolloli A., P. O’Brien P., Kushner N., et al. (2020). Inoculum size and traits of the infecting clinical strain define the protection level against Mycobacterium tuberculosis infection in a rabbit model. Eur. J. Immunol. 50, 858–872. doi: 10.1002/eji.201948448
Uhlíř J., Volf P. (1992). Ivermectin: its effect on the immune system of rabbits and rats infested with ectoparasites. Vet. Immunol. Immunopathol. 34, 325–336. doi: 10.1016/0165-2427(92)90173-N
Valldeperes M., Rossi L., Mentaberre G., Moroni B., Gardini G., Odore R., et al. (2022). Un comensal inesperado: detección de ivermectina en jabalíes (Sus scrofa) simpátricos con una población de cabra montés (Capra pyrenaica) afectada por sarna sarcóptica y tratada con pienso medicado, in: 39èmes Rencontres Du Groupe d’Étude Sur l’Écopathologie de La Faune Sauvage de Montagne (GEEFSM) (Córdoba: Libro de Resúmenes. Universidad de Córdoba), 44.
Vanhaecke C., Perignon A., Monsel G., Regnier S., Bricaire F., Caumes E. (2014). The efficacy of single dose ivermectin in the treatment of hookworm related cutaneous larva migrans varies depending on the clinical presentation. J. Eur. Acad. Dermatol. Venerol. 28, 655–657. doi: 10.1111/jdv.12097
Vercruysse J., Levecke B., Prichard R. (2012). Human soil-transmitted helminths: Implications of mass drug administration. Curr. Opin. Infect. Dis. 25, 703–708. doi: 10.1097/QCO.0B013E328358993A
Verdú J. R., Cortez V., Martinez-Pinna J., Ortiz A. J., Lumaret J. P., Lobo J. M., et al. (2018). First assessment of the comparative toxicity of ivermectin and moxidectin in adult dung beetles: Sub-lethal symptoms and pre-lethal consequences. Sci. Rep. 81 (8), 1–9. doi: 10.1038/s41598-018-33241-0
Verdú J. R., Cortez V., Ortiz A. J., González-Rodríguez E., Martinez-Pinna J., Lumaret J. P., et al. (2015). Low doses of ivermectin cause sensory and locomotor disorders in dung beetles. Sci. Rep. 51, 1–10. doi: 10.1038/srep13912
Vermunt J. J., West D. M., Pomroy W. E. (2011). Inefficacy of moxidectin and doramectin against ivermectin-resistant Cooperia spp. of cattle in New Zealand. N. Z. Vet. J. 44, 188–193. doi: 10.1080/00480169.1996.35971
Wildenburg G., Korten S., Mainuka P., Büttner D. W. (1998). Ivermectin influence on the mast cell activity in nodules of onchocerciasis patients. Trop. Med. Int. Health. 3, 918–925. doi: 10.1046/J.1365-3156.1998.00326.X
Wilkinson V., Richards S. A., Næsborg-Nielsen C., Carver S. (2023). Time to consider pharmacological interventions against infectious disease in wildlife. J. Appl. Ecol. 60, 229–236. doi: 10.1111/1365-2664.14327
Wilkinson V., Tokano K., Nichols D., Martin A., Holme R., Phalen D., et al. (2021). Fluralaner as a novel treatment for sarcoptic mange in the bare-nosed wombat (Vombatus ursinus): safety, pharmacokinetics, efficacy and practicable use. Parasit. Vectors. 14, 1–21. doi: 10.1186/S13071-020-04500-9/TABLES/7
WOAH (2022). “Mammalian tuberculosis (infection with Mycobacterium tuberculosis complex),” in Manual of Diagnostic Tests and Vaccines for Terrestrial Animals, 1–22.
World Health Organisation (2021) Global Tuberculosis Report 2021. Available at: https://www.who.int/publications/i/item/9789240037021 (Accessed March, 2023).
Keywords: bovine tuberculosis, ivermectin, helminths, coinfection, Mycobacterium bovis, Sus scrofa
Citation: Lello J, Gassó D, Gonçalves P, Risco D, García WL, Segalés J, Garrido-Amaro C, Mentaberre G, Torres-Blas I, Velarde R, López-Olvera JR, Fernández-Llario P and Serrano E (2023) Annual short-burst mass anthelmintic administration reduces tuberculosis severity but not prevalence in a wildlife reservoir. Front. Ecol. Evol. 11:1186295. doi: 10.3389/fevo.2023.1186295
Received: 14 March 2023; Accepted: 25 September 2023;
Published: 23 October 2023.
Edited by:
Dennis Murray, Trent University, CanadaReviewed by:
Brianna R. Beechler, Oregon State University, United StatesAniruddha V. Belsare, Auburn University, United States
Copyright © 2023 Lello, Gassó, Gonçalves, Risco, García, Segalés, Garrido-Amaro, Mentaberre, Torres-Blas, Velarde, López-Olvera, Fernández-Llario and Serrano. This is an open-access article distributed under the terms of the Creative Commons Attribution License (CC BY). The use, distribution or reproduction in other forums is permitted, provided the original author(s) and the copyright owner(s) are credited and that the original publication in this journal is cited, in accordance with accepted academic practice. No use, distribution or reproduction is permitted which does not comply with these terms.
*Correspondence: Joanne Lello, lelloj@cardiff.ac.uk; Emmanuel Serrano, emmanuel.serrano@uab.cat
†These authors have contributed equally to this work