- 1Department of Biology, Hampden-Sydney College, Hampden-Sydney, VA, United States
- 2College of Osteopathic Medicine, New York Institute of Technology, Old Westbury, NY, United States
Teeth are often the first structures that anatomists and paleontologists examine to understand the ecology and morphology of feeding, both because teeth are highly specialized structures that provide precise information, and because they are among the best and most commonly preserved fossils. Unfortunately, many fragmentary fossil and recent specimens lack teeth, and some come from edentulous individuals and taxa, as in mysticete (baleen) whales. In our broad comparative review, we survey non-dental osteological features that, due to size, shape, arrangement, and surface features reflecting muscle attachments, provide useful clues to general or specific aspects of prey capture, intraoral transport, processing, or swallowing. We focus on hyoid, palatal, and pterygoid bones, mandibular symphyses and processes such as the coronoid, and the temporal fossa and zygomatic arch, as well as adjacent cranial bones relating to oral and pharyngeal anatomy. These bones relate to muscles of five general locations especially indicative of feeding: mandibular, hyoid, tongue, pharyngeal, and facial regions. Together these bones and muscles affect feeding and related activities including suckling and breathing. We discuss osteological correlates that provide special relevance to key transitions in cetacean evolutionary history, such as the shift from predominantly terrestrial to aquatic feeding, the shift from typical mammalian mastication to swallowing prey entirely or nearly whole, and the separation of respiratory and digestive passages. We also point to examples of modern specialists in these anatomical optima for different modes of prey capture, intraoral transport, processing, and swallowing. Although we focus on cetaceans, our approach is broadly relevant to all vertebrates, notably other marine tetrapods.
Introduction
Georges Cuvier, widely recognized as the father of vertebrate paleontology, famously stated: “Show me your teeth and I will tell you who you are” (Rudwick, 1998). This statement demonstrates Cuvier’s bold confidence, but also his recognition of the undeniable value that teeth offer in providing direct information regarding the lifestyle of any vertebrate, from fishes to mammals (Bergqvist, 2003; Ungar, 2010). This prominent ecological role, along with their morphological diversity (at gross and ultrastructural levels) and high level of mineralized tissues that often allow teeth to be preserved and to persist for long durations in the fossil record, together combine to make dentition one of the most important and reliable sources of information for paleontologists and neomorphologists, to the point that they are typically the first source that scientists logically turn to in studies of feeding (Lucas, 2004; Hillson, 2005).
There are, nonetheless, serious liabilities in over-reliance on dental information (von Koenigswald, 1997), starting with the fact that many fragmentary fossils and Recent specimens do not include teeth. In other cases, some animals are edentulous to begin with, either due to advanced age or pathological conditions, but also because of systematic considerations wherein entire taxa have lost teeth. Prominent among such cases is the mammalian Parvorder Mysticeti, filter-feeding whales which lack any teeth (apart from occasional embryonic anlagen), and which instead bear hundreds of keratinous plates of baleen (Werth, 2000). Baleen occasionally fossilizes under favorable conditions (Esperante et al., 2008), but this occurs much less frequently than preservation of teeth.
Fortunately, teeth (or baleen or other analogous structures to capture and process food) are not the sole morphological indicators of feeding mechanisms. Many bones, particularly of the skull, provide useful clues relevant to general or specific aspects of prey capture, ingestion, transport, processing, or swallowing. Such bones often divulge information through their size, shape, arrangement, and surface structures that reveal muscle attachments and aspects of their function due to the moment arms they produce (Maughan et al., 1983; Brand et al., 1986). With regard to feeding, muscle groups of at least five general regions are of particular importance:
(1) Jaw adductors or other mandibular/masticatory muscles
(2) Hyoid and related interramal muscles
(3) Tongue muscles
(4) Pharyngeal muscles
(5) Superficial, facial, and neck muscles
Numerous muscles of these five regions are distinct yet, unsurprisingly, often relate to muscles of other regions both directly and indirectly.
With few exceptions (such as intrinsic muscles of the tongue’s muscular hydrostat), skeletal muscles attach to bones or other skeletal tissues via tendinous cords, sheaths, or aponeuroses at either fibrous or fibrocartilaginous entheses (Alexander, 1985). Therefore, study of bones’ surface structures also may provide valuable macro- and microanatomical details concerning musculature and related soft tissue arrangements, particularly those associated with dynamic activities such as feeding (Lanyon and Rubin, 1985).
Here we review and discuss several osteological correlates of feeding in Cetacea, of interest and utility to paleontologists and anatomists alike, that provide special relevance to important transitions in cetacean evolutionary history (Werth, 2000; Bisconti and Carnevale, 2022). These include the shift from predominantly terrestrial to aquatic prey capture and ingestion, the shift from typical mammalian mastication to swallowing prey entirely or almost entirely whole, and the complete separation of respiratory and digestive passages, such that the cetacean pharynx no longer has a free soft palate dividing naso- and oropharyngeal sections. These osteological correlates include but are not limited to hyoid, palatal, and pterygoid bones, mandibular symphyses and processes such as the coronoid, and the temporal fossa and zygomatic arch, as well as adjacent cranial bones relating to oral and pharyngeal anatomy. Such structures affect not just feeding but also have corresponding functional effects on other activities including swallowing, suckling, and breathing. Throughout, our aim is to focus on examples of osteological correlates of key anatomical changes reflecting evolutionary transitions rather than to provide an exhaustive list of such bony structures. Although we focus on cetaceans, our approach is broadly relevant to all vertebrates, notably other marine tetrapods, particularly in the context of the overall shapes and proportions of the feeding apparatus, not necessarily the homologous bony structures themselves (Lanyon and Rubin, 1985).
The bony landmarks we highlight are generally presumed to be indicative of feeding function (Hiiemae and Crompton, 1985; Herring, 1993). However, some features clearly relate to multiple functions. For example, cranial telescoping (Buono and Vlachos, 2022), which occurred in both mysticete and odontocete evolution (albeit differently), is frequently and properly cited as relating to migration of the external nares more superiorly, as in crocodilians, and caudally along the rostrum for easier breathing underwater. However, this shift simultaneously had the effect of lengthening the rostrum, with obvious implications for feeding (e.g., allowing for a larger oral filter or expanded dentition), and in odontocetes certain bones, notably the maxillae, are extended along with the development of echolocation specializations (Churchill et al., 2016, 2018).
We do not pretend to offer these landmarks as novel or surprising. Rather, our aim is to highlight a list of known osteological (non-dental) features that can serve as a preliminary checklist for researchers describing new fossil taxa or otherwise considering the feeding habits of a taxon (Helgason et al., 2008).
We have organized our treatment according to sequential stages of the “process model” of feeding outlined by Hiiemae (2000) by which food is acquired, moved into and within the oral cavity, processed, and swallowed. Obviously, many anatomical structures, such as the tongue, play crucial roles in multiple (or even all) stages of feeding; here we introduce and describe structures according to when they first become involved in feeding.
Prey acquisition
Aquatic feeding differs notably from terrestrial feeding because of water’s much greater density (80x) relative to air (Nybakken and Bertness, 2005), and thus water’s subsequent buoyancy, with items frequently suspended in a truly three-dimensional medium. Like terrestrial vertebrates, aquatic vertebrates can simply grasp items with teeth and jaws. This basic grasping feeding method is commonly classified as raptorial (“seizing”) feeding, or simply as biting (Marshall and Goldbogen, 2015; Marshall and Pyenson, 2019). Because the predator’s body or its jaws normally simply overcome prey by moving faster, this typically involves ram ingestion (Werth, 2000).
A disadvantage of raptorial feeding in water is that the density of both predator and prey bodies is similar to that of the surrounding water. This means that when a predator moves its head, neck, jaws, or entire body, it typically displaces a similarly-sized volume of water, which in turn pushes prey away. For a predator moving toward prey, the result is a compressive bow wave that must somehow be avoided or obviated. In short, water’s incompressibility means that items in water, whether buoyant or not, are readily repelled by most motions of a predator’s head and jaws. Pinnipeds often rely on flexible necks that “uncoil” during feeding strikes (Marshall et al., 2015). Because of the risk of pushing items away during prey acquisition, thinner, elongate jaws and rostra with less volume and surface area (e.g., “pincer jaws”) are prevalent, especially in lineages with shorter, less flexible necks. Thus in Cetacea, longirostrine forms are common; odontocete skulls frequently bear many teeth, with a total tooth count (up to 240) often exceeding the typical eutherian maximum of 44 teeth (Loch et al., 2015; Werth et al., 2019). In this and many other ways, the ecology and skeletal morphology of feeding changed dramatically when mammalian and other tetrapod predators, such as diverse marine reptiles of the Mesozoic, secondarily returned to the sea.
Despite its potential disadvantages, a clear benefit of raptorial feeding is that it does not require substantial modification of feeding behavior or morphology from terrestrial ancestors, apart from jaw modifications just mentioned. Accordingly, the mechanism by which most dolphins and pinnipeds acquire food, by snapping their jaws at and around prey items, is essentially the same process used by most terrestrial carnivorans. A further benefit of raptorial biting is that once acquired, prey are generally held immobile by the jaws and teeth. Prey items need not be transported to the dentition; they can be easily crushed, torn, punctured, or otherwise processed for easier swallowing and quicker assimilation of nutrients and energy.
Nonetheless, many cetaceans have, like other aquatic animals, moved beyond raptorial feeding. Two simple solutions to the problem of pushing prey away and potentially out of reach, both of which have been widely and independently adopted by diverse aquatic vertebrates and invertebrates in lieu of raptorial grasping/biting, are suction and filter feeding. In suction feeding, a predator expands its oral cavity to generate subambient pressure, drawing in a volume of water holding individual or multiple prey items (Wainwright et al., 2015). After prey are trapped by teeth or other holding structures (such as gill rakers in fish), the water can be discarded, with excess water flowing unidirectionally through a pharyngeal opening such as the operculum of fishes, or by reversing flow so that the water flows back out through the oral aperture through which it entered. Suction feeding requires a means to quickly and effectively draw a volume of water into the mouth (typically via rapid depression and retraction of the hyoid skeleton and consequent piston-like withdrawal of the tongue). It also demands the ability to accommodate ingested water (typically via extensible pharyngeal or adjacent gular tissues, facilitated by expansible grooves or pleats on the exterior of the throat).
The second alternative to the problems associated with feeding in a dense aquatic medium is filter feeding: essentially, casting a net with lower drag (flow resistance) than moving the head or entire body. In many invertebrates, an external filter (i.e., outside the body proper, as on a lophophore or similar antenna- or tentacle-like arm) is moved through a prey field to capture food, often by physically snagging it with stinging cells or mucus strands. Most vertebrates instead rely on internal filters within the body (usually within the pharynx or oral cavity), such that water-borne prey are captured intraorally while water is allowed to pass through excurrent pores and escape. Particle capture occurs by various mechanisms from simple sieving and direct interception (Rubenstein and Koehl, 1977) to more complex cross-flow filtration (Sanderson et al., 2016).
It has long been understood that baleen whales are filter feeders (Pivorunas, 1979). Less well appreciated and understood are the diverse ways in which mysticetes acquire and filter prey, whether by ram lunging in rorquals (Balaenopteridae; Goldbogen et al., 2017), skimming and cross-flow filtration in bowhead and right whales (Balaenidae; Werth and Potvin, 2016), or benthic suction ingestion, the primary feeding mode of gray whales, Eschrichtiidae (Ray and Schevill, 1974). Thus suction and filtration are not mutually exclusive but can be combined to capture and retain prey, respectively.
Many publications rightly focus on the crucial roles played by dentition or analogous oral structures, especially baleen, in capturing and retaining prey (Werth, 2000; Marshall and Goldbogen, 2015; Marshall and Pyenson, 2019). This makes sense because such structures generally play principal roles in feeding; further, they are (particularly in the case of teeth) among the most commonly preserved and found indicators of feeding. Here we focus instead, as noted previously, solely on osteological features. Of course, scrutiny solely of bones reveals valuable information regarding dentition when examining tooth-bearing bones such as the maxillary and dentary. The number of alveolar sockets for teeth, along with such considerations as the alveolar width, depth, and type (e.g., single or multiple-rooted), can provide useful dental information even in the absence of teeth themselves.
The HYOID skeleton (Figure 1) plays a pivotal role in cetacean feeding, particularly when prey are acquired via intraoral suction generation, which depends on rapid and forceful hyolingual retraction and depression. The hyoid skeletons of the earliest cetaceans probably differed little from those of their terrestrial ancestors, and those of cetaceans’ closest living relatives within Artiodactyla. As in most mammals, the hyoid consists of two arches (cornua) comprising several bony elements. There is a central, median basihyal to which the bilaterally paired cornua attach. Directly projecting laterally from the basihyal are paired thyrohyal bones, which make up the greater cornu. Also connected to the basihyal, via synovial joints, are the paired ceratohyal, stylohyal, and possibly tympanohyal elements (although the presence of the latter is questioned; Lawrence and Schevill, 1965; Oelschlager, 1986). Together these constitute the lesser cornu, which attach to the skull’s tympanic and periotic bones near the stylomastoid foramen, and by means of ligamentous attachment to the paroccipital process of the exoccipital (Reidenberg and Laitman, 1994; Werth, 2007). Numerous muscles connect the hyoid skeleton to the larynx, sternum, and tongue (Lawrence and Schevill, 1965; Werth, 2007); these include the mylohyoid, geniohyoid, sternohyoid, thyrohyoid, occipitohyoid, omohyoid, and ceratohyoid (AKA interhyoid) muscles.
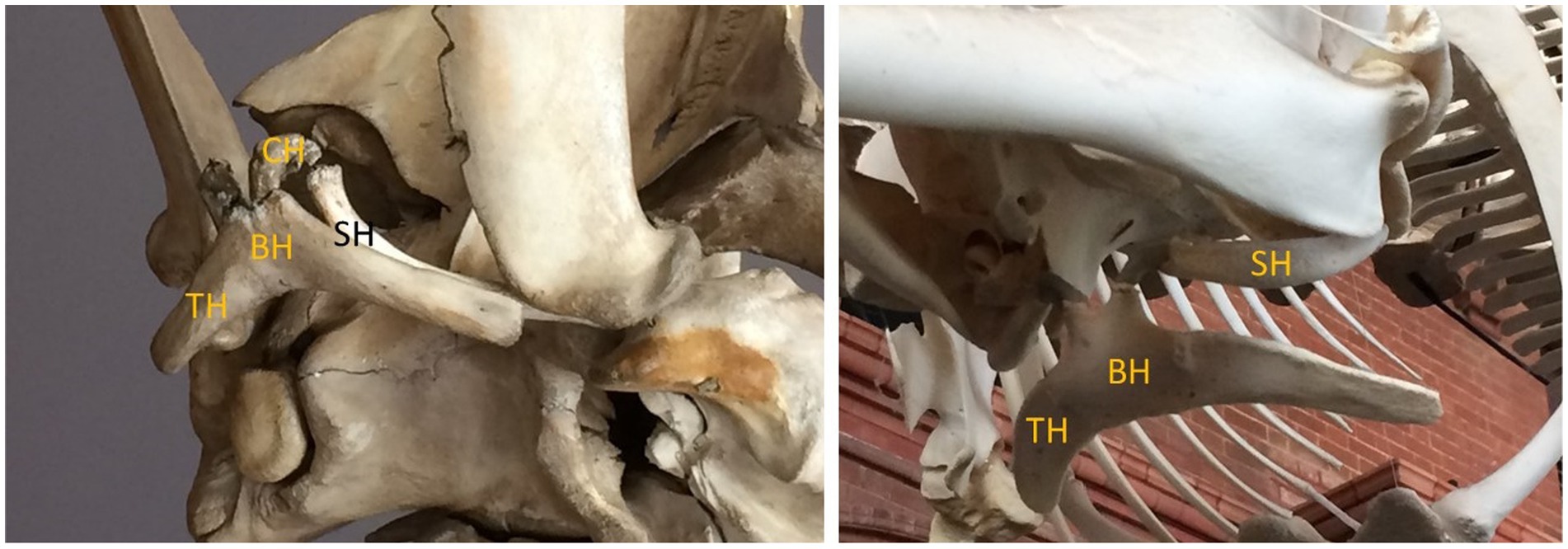
Figure 1. Cetacean hyoid skeletons of a mysticete (left, pygmy right whale, Caperea marginata) and odontocete (right, killer whale, Orcinus orca) in ventral view showing two cornua (horns) with fused elements: BH, basihyal; TH, thyrohyal; CH, ceratohyal; SH, stylohyal.
The hyoid skeleton and its constituent bones are key indicators of prey acquisition (Lauder, 1985; Wilga, 2008), and feeding more generally, in Cetacea, particularly as they relate to lingual movement. This is important because the tongue is a muscular hydrostat which lacks internal bony support. Tongue movements depend on extrinsic lingual muscles originating from the hyoid (e.g., the hyoglossus and styloglossus muscles, from the greater and lesser cornua, respectively) as well as from the mandible (genioglossus) and palate (palatoglossus). Although the tongue and hyoid therefore play key roles in feeding of all mammals, they took on important new roles and new importance with the shift during cetacean evolution to feeding in the aquatic realm (Reidenberg and Laitman, 1994; Werth, 2006a).
Specifically, with the adoption of intraorally generated suction for prey acquisition, the hyoid took on new importance. A large, robust hyoid apparatus, with large surface area for greater muscle attachment, as well as rugose surfaces indicative of muscular origins and insertion, is a telltale sign of suction feeding, especially when the hyoid appears disproportionately large. Such robust hyoids characterize modern sperm whales, Physeter macrocephalus (Werth, 2004), and their sister group Kogiidae (Bloodworth and Marshall, 2007), which are known to be suction feeders (Werth, 2000). The hyoid apparatus is also notably enlarged in beaked whales (Ziphiidae; Heyning and Mead, 1996) and Monodontidae (Werth, 2000)--again, documented suction feeders. Among mysticetes, gray whales are likewise distinctive for the unusually prominent size of the hyoid, with considerable surface area for muscle attachment on the basi-, thyro-, and stylohyal elements (Werth, 2007) Not merely the size alone, but the roughness of these bones and the profusion of ridges or other surface structures also argue for a central role in anchoring strong tongue musculature (Putz and Kroyer, 1999; Werth, 2000).
Because an enlarged hyoid is an obvious indicator of suction feeding, its presence or absence in fossil cetaceans provides a clear signal of the feeding mode of extinct taxa, and a gauge of the extent to which archaeocetes or crown cetacean lineages had made the transition from terrestrial to fully aquatic feeding, and of the feeding mechanisms they employed. Given that there is an extensive fossil record of physeterids with apparent ecological diversity, including many macroraptorial forms (Lambert et al., 2010, 2014, 2017), any evidence of hyoid enlargement would, even in the absence of dentition, provide useful information as to feeding methods. Unfortunately, the hyoid skeleton is not always recovered with cranial and postcranial fossils, just as it is not always recovered from carcasses by neomorphologists, and it is often missing from skeletal specimens and mounted displays (Werth, 2006b). The tongue of cetaceans is often a target of predators (Werth, 2000), perhaps because it lacks bones yet is a rich source of soft muscle and fat; this may in part explain why hyoid bones are often missing from fossils.
The bony PALATE is another key indicator of feeding methods in Cetacea, and again especially of suction ingestion. An arched or vaulted palate is found in several suction feeding marine mammals, notably the walrus, Odobenus rosmarus (Gordon, 1984). A similar palate is likewise present in Odobenocetops (de Muizon, 1993; de Muizon and Domning, 2002), a Pliocene odontocete that all evidence indicates was also a suction feeder; indeed, the ecomorphological convergence of these taxa is striking. Although the palate is not so prominently vaulted in Monodontidae, including extant narwhals, Monodon monoceros, and belugas, Delphinapterus leucas, the palate is somewhat arched in both species, likely as an adaptation for suction feeding (to create a larger space into which water and prey are drawn, and to generate greater subambient suction pressures when the tongue is depressed; Werth, 2006a). Interestingly, although beaked whales are clear suction feeders, the palate is not vaulted or indeed even concave along its ventral surface. The ziphiid palate is typically ridged or rough (Heyning and Mead, 1996) rather than smooth and elevated, as in many suction feeders. Heyning and Mead (1996) concluded that the ridged palate of ziphiids may, in the absence of functional dentition, serve to retain captured prey, particularly the slippery cephalopods on which many beaked whales specialize. Hence the palate often indicates factors of prey type as well as prey acquisition method (Table 1).
Sperm whales are also documented suction experts that frequently feed on cephalopods. Although the bony palate of sperm whales is not vaulted, the palate into which the lower jaw neatly fits exhibits a pronounced ventral concavity. This is likely less related to suction generation–especially since there is no true oral “cavity,” with the space around the rod-like mandible completely open on the sides, and thus with suction apparently primarily generated at the circular oropharyngeal opening rather than at a circular mouth aperture, as in most non-cetacean suction feeders (Werth, 2004)–and instead probably relates simply to the soft tissues that enclose the mandible when the jaw is closed. Nonetheless, this provides another important reminder that bones alone, although often valuably instructive, often produce a misleading or at least incomplete picture. When present, soft tissues provide invaluable information to “flesh out” the morphology and ecology of feeding, such that neomorphologists are advised to dissect whole specimens rather than rely solely on osteological museum specimens (Werth, 2006a).
The MANDIBLE (dentary bone) forms the lower jaw (Figure 2), so that apart from bearing lower teeth, and even in functionally edentulous taxa, it remains a crucial structure for feeding, and particularly for prey acquisition (Barroso et al., 2012). However, the mandible is equally important for all other aspects of feeding, especially mastication or other processing of food. Relative to other mammals, including the earliest cetaceans, the mandible of odontocetes is notably thin and gracile, unlike the thick, robust lower jaw of archaeocetes. Although this evolutionary trend undoubtedly reflects a diminution or total absence of prey processing in modern odontocetes, it also signifies other structural and functional changes. For instance, the odontocete mandible notably takes on special significance for its non-feeding sensory role in directing sound waves to the ear via the thin “pan bone” and mandibular fat body which runs along the mandibular ramus. The upshot is that major and minor alterations alike to “feeding” morphology may reflect other changes unrelated to actual feeding per se, as distinguished by the four main sequential events we outline. Alternatively, such changes may relate indirectly to feeding. Jaw snapping and clapping probably play roles in displaying threat behavior, but possibly also play roles in debilitating prey (Norris and Møhl, 1983).
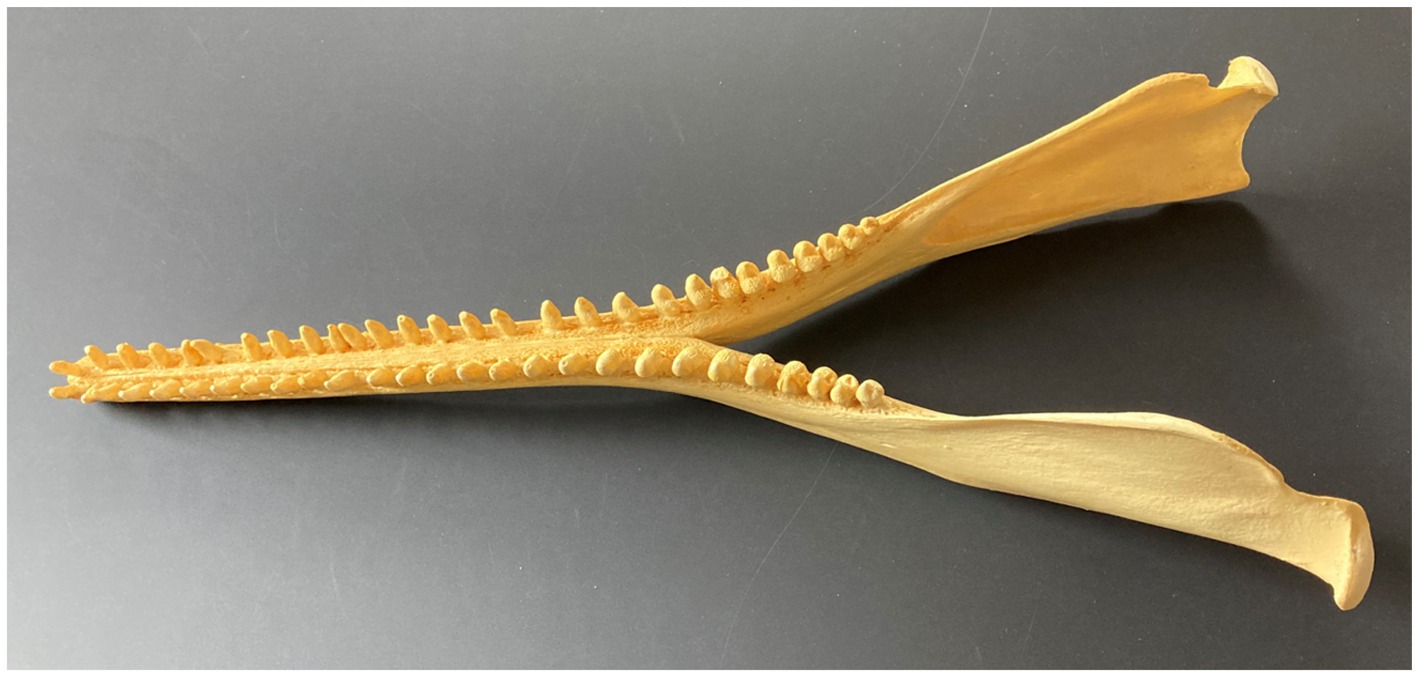
Figure 2. Mandible showing symphysis, coronoid process, and condyle (dorsolateral view) in Amazon river dolphin or boto, Inia geoffrensis.
The mysticete mandible is typically a thick, curved cylinder that lacks not only dentition but also enlarged processes and other prominent bony landmarks found in typical terrestrial mammals (Field et al., 2010; Bisconti and Carnevale, 2022). Baleen whale mandibles are specialized for rotational movements associated with bulk filtration and the subsequent need for expanded gape (Lambertsen, 1983; Lambertsen et al., 1995).
The mandibular symphysis is a key trait that reveals use of the jaws in feeding. Werth (2006a,b) showed that the ratio of jaw length to width, which can be expressed as a mandibular bluntness index (MBI), is a reliable indicator of prey acquisition because it generally correlates to feeding method. Taxa with shorter, blunter heads and jaws tend to be suction feeders (Werth, 2006a), whereas longirostrine taxa with long jaws tend to have more teeth (Werth et al., 2019), which indicates raptorial biting and grasping of prey.
Although short, wide jaws correlate with suction feeding in odontocetes, some taxa that are documented suction feeders nonetheless possess elongated mandibular symphyses (Werth, 2006a). This includes, most notably, sperm whales, Physeter macrocephalus, which exhibit remarkably long symphyses bearing rows of sharp teeth. In fact, there are no teeth borne on the main, paired mandibular bodies (post-symphysis) in the lower jaw of this species. However, the sperm whale is a suction feeder that specializes on large cephalopods and demersal fishes (Werth, 2000). Because there is no traditional oral “cavity” to speak of, it is likely that prey can be sucked directly through the fauces into the oropharynx (Werth, 2004).
Modern mysticetes lack a fused mandibular symphysis, apparently as an adaptation for multiple degrees of freedom of jaw rotation (Lambertsen et al., 1995; Werth et al., 2020) to improve gape and aid in ingesting and expelling larger volumes of water for bulk filtration. Pyenson et al. (2012) described a novel sensory organ between the dentary bones of rorquals to coordinate lunge feeding. Based on histological and gross morphological findings, this appears to be a mechanosensory or proprioceptive receptor, with nerve termini housed within papillae in a gelatinous matrix, to provide feedback regarding relative positions and motions of the dentary bones during feeding. As with sensory hairs along the head and jaws of mysticetes (such as the prominent “stovebolt” hair follicles of humpback whales, Megaptera novaeangliae), this symphyseal organ appears to relay sensory evidence so that whales discern precisely how their jaws are being deployed and the forces they are encountering; sensory feedback can also aid in determining when and how quickly to open and close jaws based on contact with prey and stretching from engulfed water.
The mandibular ramus and CORONOID process (Figure 3) are markedly reduced in all cetaceans relative to typical terrestrial mammals; in some cases, such as bowhead and right whales (Balaenidae), virtually no coronoid can be detected. This relates to a decrease in jaw adductor musculature (masseter, temporalis, and pterygoid muscles), which is notably reduced in cetaceans due to the lack of masticatory food processing, and to a lesser extent to the partial or total loss of teeth (and consequent raptorial grasping) that distinguishes numerous odontocete taxa (Werth, 2000). In some mysticetes, jaw rotation may play important roles in opening and closing the mouth during filter feeding (Lambertsen et al., 1995; El Adli and Demere, 2015; Werth et al., 2020), and slips of temporal musculature inserting on the mandible or on connective tissue “lips” projecting dorsally to the lower jaw, especially in balaenids, may aid in gape alteration (Lambertsen et al., 1995) and hence water ingestion and expulsion.
However, the coronoid process (and overall mandible) of early cetaceans more closely resembled that of terrestrial ancestors. In most archaeocetes, the jaw’s form, like its coronoid and dentition, indicate “traditional” mammalian jaw movements, including biting and chewing. The extent to which the mandible and its constituent elements changed over time within Archaeoceti, and in odontocetes and mysticetes–becoming longer, more rod-like, less robust, and less characterized by bony protuberances and processes–all signify ecomorphological shifts in cetacean foraging methods and feeding mechanisms, namely away from typical mammalian biting and mastication to other feeding methods better suited to aquatic life.
Intraoral transport
The crucial role of the tongue and hyoid in prey acquisition have been discussed above. Unsurprisingly, these structures also play central roles in the transport of ingested food within the mouth (Hiiemae, 2000), including transport to and from tooth rows (in cases where prey items are grasped and/or dentally processed), and transport to and from the main portion of the oral cavity to its posterior border at the oropharyngeal isthmus, or pillars of the fauces, for swallowing.
Unlike many mammals, cetaceans exhibit a “notched” or open “Pac-man” gape, with few discernible soft tissues to create cheeks or lips. In this way, cetacean mouths often resemble those of crocodilians more than of typical mammals. As such, the mouth does not often involve a true oral “cavity” or enclosed space bounded by fleshy cheeks. Nor is there usually a truly circular anterior oral aperture, or mouth proper, that provides the entry to the buccal region. However, there are in certain taxa some soft tissues that, even in greatly reduced form, create some sort of oral aperture or even cheek-like boundaries lateral to the tooth rows. These are best seen in delphinoid odontocete taxa that depend heavily on suction feeding. Beluga whales, Delphinapterus leucas, offer a prime example; they can readily “purse” their mouth to create a more circular opening, the better to suck in water and prey, and to control the direction of incurrent water flow (Werth, 2006a).
In such odontocetes, various facial or superficial muscles, especially the buccinator and orbicularis oris, figure prominently among such soft tissues, along with conspicuous adipose and other connective tissues (Werth, 2000). There are roughened attachment points along the upper jaws of the skull that reveal the presence and indicate the prominence of such muscles. Even in some odontocete taxa where such facial muscles exist solely in greatly reduced (even vestigial) form, the muscles, and their bony attachment points, provide some indication of the extent to which these cetaceans can alter the shape of the mouth to acquire and especially to transport food items intraorally (Werth, 2006b).
Like odontocetes, mysticetes also possess a clearly notched gape and lack obvious or conventional lips, but there are small bands of tissue, especially around the mandibles and projecting dorsally above them (Werth et al., 2020), that might function to constrain or “hold” baleen racks in place during filtration, to prevent keratinous baleen plates from bending or moving laterally as immense volumes of water are rapidly expelled through the baleen with consequent high forces being generated (Werth, 2013). Again, the presence and condition of bony surfaces adjacent to these jaw structures can be indicative of soft tissue features in extant cetaceans, just as changes in such features throughout the fossil record can reveal shifts in feeding. In this regard, it is noteworthy that Peredo et al. (2022) concluded that palatal foramina, traditionally interpreted as evidence of baleen presence in Recent and fossil mysticetes, probably instead relate more to gingival or associated tissues unrelated to baleen (or to filter feeding), as in artiodactyls and other typical terrestrial mammals.
Mastication
The zygomatic arches of cetaceans (Figure 4) underwent major change, and specifically near-total loss (to the point where they are nearly vestigial in many taxa), due to the loss of mastication from archaeocete ancestors to modern crown cetaceans. Thus the arches and their constituent bones, whether classified as zygomatic, malar, or jugal, are, in most dolphins and porpoises, as thin as toothpicks. However, early cetaceans possessed prominent, robust zygomatic arches and bones befitting their role, along with the mandibular coronoid process, in serving as attachment points for masticatory musculature. Although the zygomatic arch is retained in greatly reduced form in modern odontocetes, there are prominent postorbital processes that provide a similar role (with much additional surface area) for the attachment of jaw-closing musculature.
At the same time, the temporal fossa that symbolizes the mammalian braincase (Figure 4) also underwent prominent transformation, and similar near loss, throughout cetacean evolution, once again signifying the loss of masticatory function and hence considerable reduction in jaw adductor muscles to rapidly and forcefully close and move the jaws (in rotational and translational movements).
The mandibular condyle of most cetaceans (Figure 5) underwent a similar transition with the shift from traditional mammalian masticatory function to a basic role of jaws in acquiring/ingesting and swallowing prey, and in many cases grasping and transporting prey following prehension, yet without any comminution or trituration. Thus the condyles are rounded, reflecting not hinge-like attachment and scissor-like motion for biting, nor robust movement (as in herbivorous artiodactyls) for mastication, but instead, simple jaw closure (Bouetel, 2005).
Again, the earliest whales exhibited some apparent adaptations for aquatic existence, particularly with regard to their ear bones, but in terms of feeding morphology archaeocetes possessed anatomy much more in keeping with terrestrial mammals–not just at first but even as their postcranial skeletons showed indications of aquatic locomotion and other signs of life in water. The temporal fossa, zygomatic arch, and mandibular condyloid and coronoid processes appear to have been somewhat slower to change than postcranial bones.
Deglutition
Extant cetaceans have an unusual soft palate: not a free soft palate extending caudally from the bony palate, and delineating the nasopharynx from the oropharynx, as in typical mammals, but instead a palatopharyngeal sphincter. This ring of muscles holds the larynx in a permanent intranarial position (Reidenberg and Laitman, 1987), such that cetaceans cannot breathe through the mouth nor aspirate water into airways through the mouth. They breathe solely through the blowhole (single in odontocetes, double in mysticetes). The larynx is snugly bound by the palatopharyngeal sphincter such that it abuts to internal nares, with the larynx protruding through the center of the oropharynx or asymmetrically located slightly closer to one side of the lateral pharyngeal wall. This laryngeal position, together with the overall size of the esophagus, limits the amount that can be swallowed in a single bolus of food. The extent to which the larynx can be dislodged during vocalization (Reidenberg and Laitman, 2007) or swallowing (Gil et al., 2022) is unclear.
Due to the contribution of various pharyngeal muscles that together constitute this sphincter and the soft palate overall (mainly the palatopharyngeus but also the pterygopharyngeus, stylopharyngeus, thyropharyngeus, and occipitothyroid), the palate is important in swallowing. In typical mammals the bony pterygoid hamulae form a pulley around which tendons can pull and thus tense or elevate the soft palate (the tensor and levator veli palatini muscles, respectively; Putz and Kroyer, 1999). Cetaceans, however, have unusual pterygoids, largely because these bones are lightly constructed to house air-filled sinuses presumed to play a role in vocalization, especially in odontocetes (Racicot and Berta, 2013).
Fossils of the earliest cetaceans appear to exhibit pterygoid bones (Figure 4) like those of most terrestrial mammals. When and how the hamulus of the pterygoid was lost or altered is unknown, but likely relates to adaptations for aquatic life: namely, true separation of airways and digestive passages, and consequent alteration of swallowing, due to the intranarial position of the larynx (Reidenberg and Laitman, 1987) and its permanent protrusion through the pharynx. Apart from alteration of the pterygoid bones (e.g., loss of the hamulus) relating to soft palatal modification, diminution and weakening/thinning of pterygoid bones also reflects loss of pterygoid jaw adductor musculature during cetacean evolution, consistent with decreased emphasis on mastication or other prey processing involving complex mandibular motions.
Discussion
As noted earlier, many cetacean fossils, particularly of the earliest Eocene and Oligocene taxa, are highly fragmentary. They often include distinctive details, such as of the temporal region and auditory bullae, that allow for diagnostic identification as cetacean, even if they do not include full (or indeed any) bones of the hyoid apparatus, palate, or zygoma. Fossils may not reveal details, particularly on bony surfaces, relating to the sorts of muscle attachments we outline here, which would be especially informative in drawing conclusions related to feeding morphology and ecology. Nonetheless, we hope our catalog of features (Table 1) will prove useful in interpreting current and as-yet-undescribed osteological specimens.
The presumed “missing link” ancestor to all Cetacea was Indohyus, a small, amphibious digitigrade artiodactyl of the extinct Raoellidae (Thewissen, 1998; Thewissen et al., 2007, 2009). Indohyus had heavy bones, perhaps as an adaptation to spending greater time in water; its jaws and zygoma were robust. It was presumably omnivorous based on dental morphology, and isotopic analysis (Marx et al., 2016) suggest it fed occasionally or rarely in water and was still at least semi-terrestrial. Ears of the earliest archaeocete cetaceans, such as Pakicetus and Protocetus, exhibit clear adaptation to aquatic life, and their teeth display signs of wear from heavy chewing (Thewissen et al., 2011). However, the earliest archaeocetes display a trend toward longer jaws and presumably more of a laterally open or notched gape. This continued in later (more recent) archaeocetes, including Ambulocetus, Maiacetus, and Rodhocetus. Larger taxa, including Basilosaurus, Cynthiacetus, and Dorudon, had strong skulls, indicating powerful feeding on large prey (unsurprising given the large body size of these early whales). By the time early odontocetes appeared, skulls and jaws were less robust and dentition became more homodont, with the appearance of the simple, conical pegs that predominate among Odontoceti today.
Although toothed and baleen crown cetaceans are highly disparate, both stem from the same early progenitors such as Indohyus whose cranial and oral anatomy was vastly unlike that of extant cetaceans, and which was apparently largely similar to that of other raoellid artiodactyls. This holds for the heterodont dentition of both upper (maxillary) and lower (mandibular) jaws, with large conical and serrated triangular teeth serving as piercing, grasping, and shearing surfaces, many of which exhibit apical, buccal, and lateral wear facets (Marx et al., 2016), separated by pronounced diastemas. Although in some ways the cetacean fossil record is fragmentary, in other ways it reveals a wealth of taxa from the presumed earliest cetaceans to myriad extinct odontocete and mysticete taxa, including such striking groups as the shark-toothed squalodontids, sword-snouted eurhinodelphids, walrus-like odobenocetopsids, mystacodontids, llanocetids, and macroraptorial sperm whales (Lambert et al., 2010, 2017; Racicot et al., 2014), as well as diverse toothed stem mysticetes such as mammalodontids and aetiocetids. Probably the most instructive fossil cetacean taxa can be found in many archeocete families, from the earliest pakicetids, ambulocetids, remingtonocetids, and protocetids, to larger, later basilosaurids including dorudontines, all of which were raptorial heterodont predators (Carpenter and White, 1986; Fahlke, 2012; Cooper et al., 2014). Still, some cetacean fossils, archaeocete and otherwise, exhibits somewhat brevirostrine forms that depart from the typical longirostrine template. Other key osteological changes, such as modification of orbits and telescoping of the braincase and rostrum concomitant with posterior migration of the nares for more efficient aquatic breathing, are evident within Archaeoceti. The larger diameter of the mandibular foramen, beginning with Ambulocetidae (Thewissen and Bajpai, 2001), may mark the origin of the mandibular fat body that aids sound transmission in extant odontocete jaws.
In terms of feeding morphology and ecology, basilosaurids (Figure 6) are among the best-studied ancient cetaceans, perhaps due to their abundance and early discovery of well-preserved fossil remains. Basilosaurids have traditionally been presumed to prey on fish and squid using their large teeth and grasping jaws (Kellogg, 1928, 1936; Barnes and Mitchell, 1978; Martinez-Caceres et al., 2017), but Van Valen (1968) suggested that the prominently notched, serrated teeth of basilosaurids are strikingly reminiscent of the distinctively lobed dental cusps of crabeater seals (Lobodon carcinophagus), and hence that these archaeocetes might, like Lobodon, have fed via filtration on krill or other zooplankton. Carpenter and White (1986), relying on mammalian anatomy described by Turnbull (1970), determined that Zygorhiza’s skull and dentition, bearing sharp carnassial teeth (Uhen, 2000), were best suited to grasp., pierce, and slice fish or other large, elusive prey. The zygomatic arch is much less slender than in extant cetaceans, and the temporal fossae/fenestrae are very large, with a prominent lambdoidal crest, indicating robust temporalis muscles. There is also much more surface area (relative to recent taxa) for the attachment of pterygoid muscles. The dentaries are often strongly fused with a long symphysis that occupies half the mandibular length (Carpenter and White, 1986; however, the ligamentous symphysis of basilosaurids is unfused), and the digastric muscle also appears to have been prominent. The mandibular condyloid and coronoid processes are large and thickly developed. There is a large mandibular foramen on the lower jaw’s medial surface but no fossa on the lateral surface of the mandibular coronoid process. The basilosaurid hyoid is long and well-developed but slender: not small, but not as robust as in many living cetaceans.
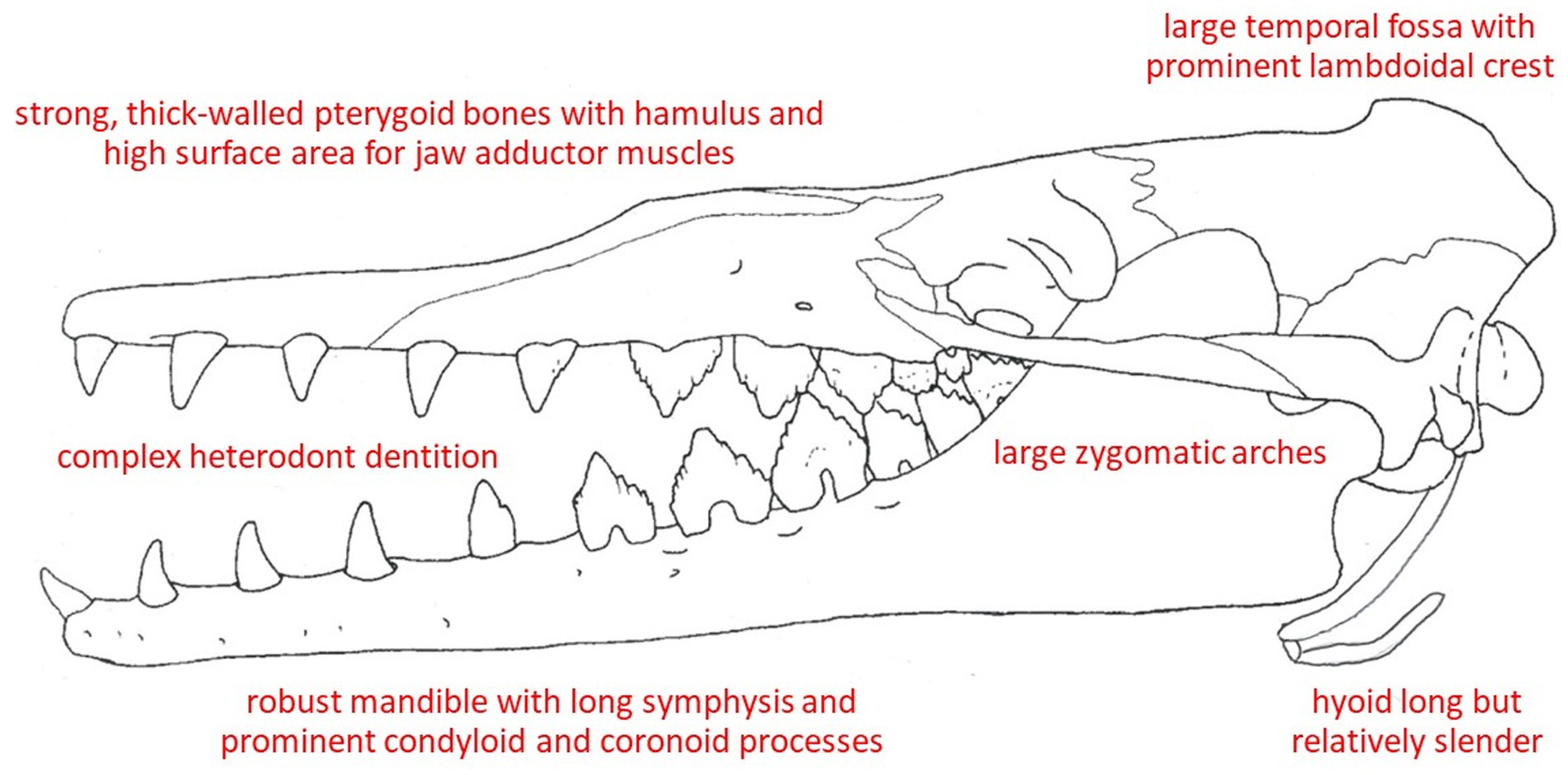
Figure 6. Osteological correlates of feeding in a dorudontine basilosaurid, Zygorhiza kochii, that is presumed similar to the ancestral condition of modern cetaceans.
Altogether, the osteological features of the skull and jaws of Dorudon, Zygorhiza, Cynthiacetus, and other well-described basilosaurids (Figure 6), along with tooth size, shape, and wear facets (Clementz and Uhen, 2012; Fahlke et al., 2013), present an embodiment not only of mammals well suited to capturing aquatic prey but also to processing them orally (Uhen, 2004; Martinez-Caceres et al., 2017). Apart from certain modern delphinids (notably Orcinus, Feresa, and Pseudorca) and possibly Inia, there is likely little intraoral prey processing in extant cetaceans. One can conclude that cetaceans have become adept at capturing and ingesting prey, not only with their well-adapted morphology but especially via versatile and resourceful foraging behaviors and echolocatory abilities, while they have at the same time apparently largely dispensed with the masticatory and other intraoral prey processing that characterize Mammalia. Alteration of the pharynx for aquatic breathing has also led to changes in the soft palate that somewhat affect swallowing (and preclude the possibility of food or water entry into airways except via blowholes).
The earliest mysticetes, including Mystacodon, Llanocetus, and Janjucetus, retained teeth as well as mixed indicators that they fed via suction or raptorial (biting) ingestion or by some combination of these mechanisms. Given how resourceful and opportunistic modern cetaceans are–being heavily motivated to use diverse foraging methods to prey upon all sorts of potential food items–it would not be surprising that early mysticetes, before becoming obligate filter feeders, were highly adaptive and versatile in attempts to catch many types of prey (de Muizon et al., 2019). Along with dental changes (namely, gradual and steadily progressive shifts in decreased tooth size and number) in later taxa of early mysticetes, including Mammalodon to Aetiocetus to Cetotherium, there were also changes in the mandible and skull presumed to reflect the switch to bulk filtration (Kimura, 2002; Demere and Berta, 2008; Fitzgerald, 2010; Gol’din et al., 2014). Specifically, the jaw joint and mandible appear to be better suited for rotational changes to increase gape rather than to catch or clamp down on individual prey items. As teeth continued to dwindle in size and number in genera such as Llanocetus and Maiabalaena, proto-baleen tissues to capture and retain small bulk prey (fish, cephalopods, or zooplankton) likely appeared and probably proved highly adaptive, such that these tissues, once established, presumably both quickly replaced any remaining teeth just as these keratinous plates quickly grew in size and number. As mentioned above, Peredo et al. (2022) concluded that the palatal foramina traditionally accepted as a signal indicator of the presence of baleen may instead relate simply to gingiva or other palatal tissues unrelated to baleen. There is a tremendous diversity of mysticete fossils (O’Leary and Uhen, 1999; Uhen, 2007, 2010; Marx et al., 2016; Fordyce, 2018), and obviously many pronounced ecological and morphological shifts from the earliest stem baleen whales to modern crown taxa. Ideally, the recent surge in newly described fossils will continue, helping to flesh out the story of whale evolution, and its key osteological events and transitions, in far greater detail. What is the ultimate outcome of these comparisons (Figures 7, 8; Table 1)? At first glance it might appear that either specialization has led to numerous unique adaptations, or that too many of these anatomical details just lead to generalizations. What can one do with such information? By summarizing the extremes found in the forms described above (Table 1), one could, at the very least, estimate a range of possible specializations by the way in which one’s organism of interest fits within the ranges of the categories outlined above. For example, a tightly fused symphysis may be indicative of stronger bite forces or more precise occlusion, yet a wide oral aperture might be less indicative of a suction feeding form of prey capture. One could readily apply these anatomical directions to estimate how anatomical specializations might be optimized for all stages of feeding. Ideally, rather than allowing an expected progression from one feeding mode to another be a factor in interpretation, one could instead let the anatomical features observed in the fossil record determine the interpretation of the progression through these feeding specializations (Figures 7, 8). Just as one must not forget that all anatomical structures have a phylogenetic history constrained by natural selection on ancestral forms, one must also remember that organisms live and behave with no aim besides survival in mind, and each anatomical specialization is only going to be used by that organism for its own purposes.
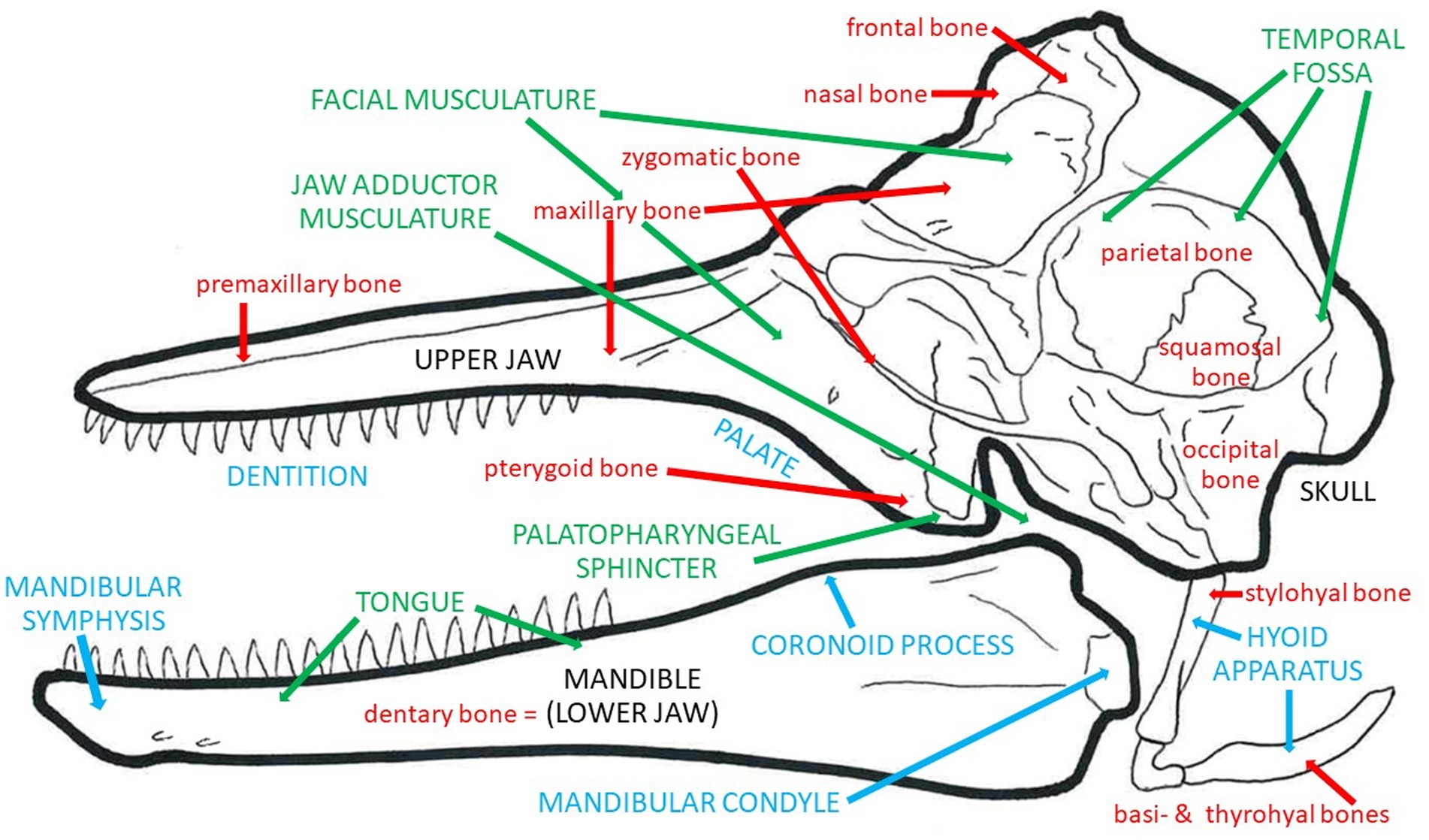
Figure 7. Summary of osteological correlates of feeding in a Recent odontocete, showing individual bones in red, muscle groups and other soft tissues in green, and bony features, landmarks, and complexes in blue.
Hopefully, observations of these osteological correlates will lead to further evaluations of soft tissues and influence our understanding of feeding specializations such that we do not rely so heavily on teeth. Paleoanthropology is rife with examples of conflict between research groups in which different methods and anatomical features are used by opposing groups to argue over paleobiological interpretations that might be instead used to help describe a more complex picture of their organisms of interest. Likewise, marine mammal paleontology should aim to use all possible information to inform us of the complex ways in which marine mammals evolved anatomical specializations for feeding. The fossil record is already missing so much of the original data once there, such as soft tissues, biochemistry, and behavior, that it would be a shame to ignore what is available because it does not fit within a simplified, clean story that we wish to tell.
Author contributions
AW and BB wrote and edited this manuscript. All authors contributed to the article and approved the submitted version.
Acknowledgments
We thank the reviewers and editor for their helpful suggestions on this manuscript, and our numerous colleagues for their continued findings in vertebrate paleontology and morphology, many of which inspired this review article.
Conflict of interest
The authors declare that the research was conducted in the absence of any commercial or financial relationships that could be construed as a potential conflict of interest.
Publisher’s note
All claims expressed in this article are solely those of the authors and do not necessarily represent those of their affiliated organizations, or those of the publisher, the editors and the reviewers. Any product that may be evaluated in this article, or claim that may be made by its manufacturer, is not guaranteed or endorsed by the publisher.
References
Alexander, R. M. (1985). “Body support, scaling, and allometry” in Functional Vertebrate Morphology. eds. M. Hildebrand, D. M. Bramble, K. F. Liem, and D. B. Wake (Cambridge: Harvard University Press), 26–37.
Barnes, L., and Mitchell, E. (1978). “Cetacea” in Evolution of African Mammals. eds. V. Maglio and H. Cooke (Cambridge MA: Harvard University Press), 582–602.
Barroso, C., Cranford, T. W., and Berta, A. (2012). Shape analysis of odontocete mandibles: functional and evolutionary implications. J. Morphol. 273, 1021–1030. doi: 10.1002/jmor.20040
Bergqvist, L. P. (2003). The role of teeth in mammal history. Brazil J. Oral. Sci. 2, 249–257. doi: 10.20396/bjos.v2i6.8641693
Bisconti, M., and Carnevale, G. (2022). Skeletal transformations and the origins of baleen whales (Mammalia, Cetacea, Mysticeti): a study on evolutionary patterns. Diversity 14:221. doi: 10.3390/d14030221
Bloodworth, B., and Marshall, C. D. (2007). A functional comparison of the hyolingual complex in pygmy and dwarf sperm whales (Kogia breviceps & K. sima), and bottlenose dolphins (Tursiops truncatus). J. Anat. 211, 78–91. doi: 10.1111/j.1469-7580.2007.00755.x
Bouetel, V. (2005). Phylogenetic implications of skull structure and feeding behaviors in balaenopterids (Cetacea: Mysticeti). Mammalogy 86, 139–146. doi: 10.1644/1545-1542(2005)086<0139:PIOSSA>2.0.CO;2
Brand, R. A., Pederson, D. R., and Friederich, J. A. (1986). The sensitivity of muscle force prediction to changes in physiological cross-section area. J. Biomechanics 19, 589–596. doi: 10.1016/0021-9290(86)90164-8
Buono, M. R., and Vlachos, E. (2022). Breaking the mold: telescoping drives the evolution of more integrated and heterogeneous skulls in cetaceans. PeerJ 10:e13392. doi: 10.7717/peerj.13392
Carpenter, K., and White, W. (1986). Feeding in the archaeocete Zygorhiza kochii (Cetacea: Archaeoceti). Miss. Geol. 7, 1–14.
Churchill, M., Geisler, J. H., Beatty, B. L., and Goswami, A. (2018). Evolution of cranial telescoping in echolocating whales (Cetacea: Odontoceti). Evolution 72, 1092–1108. doi: 10.1111/evo.13480
Churchill, M., Martinez-Caceres, M., de Muizon, C., Mnieckowski, J., and Geisler, J. H. (2016). The origin of high-frequency hearing in whales. Curr. Biol. 26, 2144–2149. doi: 10.1016/j.cub.2016.06.004
Clementz, M. T., and Uhen, M. D. (2012). Ontogenetic variation in dental stable isotope values of two species of basilosaurids (Zygorhiza kochii and Dorudon atrox). J. Vertebr. Paleontol. 26, 355–370.
Cooper, L. N., Hieronymous, T. L., Vineyard, C. J., Bajpai, S., and Thewissen, J. G. M. (2014). “New applications for constrained ordination: reconstructing feeding behaviors in fossil Remingtonocetinae (Cetacea: Mammalia)” in Experimental Approaches to Understanding Fossil Organisms. eds. D. I. Hembre, B. F. Platt, and J. J. Smith (Dordrecht: Springer), 89–107.
de Muizon, C. (1993). Walrus-like feeding adaptation in a new cetacean from the Pliocene of Peru. Nature 365, 745–748. doi: 10.1038/365745a0
de Muizon, C., and Domning, D. P. (2002). The anatomy of Odobenocetops (Delphinoidea, Mammalia), the walrus-like dolphin from the Pliocene of Peru and its palaeobiological implications. Zool. J. Linnean Soc. 134, 423–452. doi: 10.1046/j.1096-3642.2002.00015.x
de Muizon, C., Lambert, O., Martínez-Cáceres, M., and Bianucci, G. (2019). Mystacodon selenensis, the earliest known toothed mysticete (Cetacea, Mammalia) from the late Eocene of Peru: anatomy, phylogeny, and feeding adaptations. Geodiversitas 41, 401–499. doi: 10.5252/geodiversitas2019v41a11
Demere, T. A., and Berta, A. (2008). Skull anatomy of the Oligocene toothed mysticete Aetiocetus weltoni (Mammlia: Cetacea): implications for mysticete evolution and functional anatomy. Zool. J. Linnaean Soc. 154, 308–352. doi: 10.1111/j.1096-3642.2008.00414.x
El Adli, J. J., and Demere, T. A. (2015). On the anatomy of the temporomandibular joint and the muscles that act upon it: observations on the gray whale, Eschrichtius robustus. Anat. Rec. 298, 680–690. doi: 10.1002/ar.23109
Esperante, R., Brand, L., Nick, K. E., Poma, O., and Urbina, M. (2008). Exceptional occurrence of fossil baleen in shallow marine sediments of the Neogene Pisco formation, southern Peru. Palaeogeogr. Palaeoclimatol. Palaeoecol. 257, 344–360. doi: 10.1016/j.palaeo.2007.11.001
Fahlke, J. M. (2012). Archaeocete predation: bite marks revisited–evidence for middle-to-late Basilosaurus isis predation on Dorudon atrox (both Cetacea, Basilosauridae). Paleontologica Electronica 15:32a. doi: 10.26879/341
Fahlke, J. M., Bastl, K. A., Semprebon, G. M., and Gingerich, P. D. (2013). Paleoecology of archaeocete whales throughout the Eocene: dietary adaptations revealed by microwear analysis. Palaeogeogr. Palaeoclimatol. Palaeoecol. 386, 690–701. doi: 10.1016/j.palaeo.2013.06.032
Field, D. A., Campbell-Malone, R., Goldbogen, J. A., and Shadwick, R. E. (2010). Quantitative computed tomography of humpback whale (Megaptera novaeangliae) mandibles: mechanical implications for rorqual lunge-feeding. Anat. Rec. 293, 1240–1247. doi: 10.1002/ar.21165
Fitzgerald, E. M. G. (2010). The morphology and systematics of Mammalodon colliveri (Cetacea: Mysticeti), a toothed mysticete from the Oligocene of Australia. Zool. J. Linnaean Soc. 158, 367–476. doi: 10.1111/j.1096-3642.2009.00572.x
Fordyce, R. E. (2018). “Cetacean evolution” in Encyclopedia of Marine Mammals 32. eds. B. Würsig, J. G. M. Thewissen, and K. Kovacs (New York: Elsevier), 180–185.
Gil, K. N., Vogl, A. W., and Shadwick, R. E. (2022). Anatomical mechanism for protecting the airway in the largest animals on earth. Curr. Biol. 32, 898–903.e1. doi: 10.1016/j.cub.2021.12.040
Gol’din, P., Startsev, D., and Krakhmalnaya, T. (2014). The anatomy of the late Miocene baleen whale Cetotherium riabinini from Ukraine. Paleontologica Polonica 59, 795–814. doi: 10.4202/app.2012.0107
Goldbogen, J. A., Cade, D., Calambokidis, J. A., Friedlaender, A. S., Potvin, J., Segre, P. S., et al. (2017). How baleen whales feed: the biomechanics of engulfment and filtration. Annu. Rev. Mar. Sci. 9, 367–386. doi: 10.1146/annurev-marine-122414-033905
Gordon, K. R. (1984). Models of tongue movement in the walrus (Odobenus rosmarus). J. Morph. 182, 179–196. doi: 10.1002/jmor.1051820206
Helgason, B., Perilli, E., Schileo, E., Taddei, F., Brynjólfsson, S., and Viceconti, M. (2008). Mathematical relationships between bone density and mechanical properties: a literature review. Clin. Biomech. 23, 135–146. doi: 10.1016/j.clinbiomech.2007.08.024
Herring, S. W. (1993). Functional morphology of mammalian feeding. Am. Zool. 33, 289–299. doi: 10.1093/icb/33.3.289
Heyning, J. E., and Mead, J. G. (1996). Suction feeding in beaked whales: morphological and observational evidence. Contrib. Sci. Nat. Hist. Mus. LA County 464, 1–12. doi: 10.5962/p.226802
Hiiemae, K. M. (2000). “Mammalian feeding” in Feeding: Form, Function and Evolution in Tetrapod Vertebrates. ed. K. Schwenk (San Diego: Academic), 411–448.
Hiiemae, K. M., and Crompton, A. W. (1985). “Mastication, food transport, and swallowing” in Functional Vertebrate Morphology. eds. M. Hildebrand, D. M. Bramble, K. F. Liem, and D. B. Wake (Cambridge: Harvard University Press), 262–290.
Hillson, S. (2005). “Teeth” in Cambridge Manuals in Archaeology (Cambridge: Cambridge University Press), 373.
Kellogg, R. (1928). The history of whales: their adaptation to life in water. Q. Rev. Biol. 3, 29–76. doi: 10.1086/394293
Kimura, T. (2002). Feeding strategy of an early Miocene cetothere from the Toyama and Akeyo formations, Central Japan. Paleontol. Res. 6, 179–189. doi: 10.2517/prpsj.6.179
Lambert, O., Bianucci, G., and Beatty, B. (2014). Bony outgrowths on the jaws of an extinct sperm whale support macroraptorial feeding in several stem physeteroids. Naturwissenschaften 101, 517–521. doi: 10.1007/s00114-014-1182-2
Lambert, O., Bianucci, G., and de Muizon, C. (2017). Macroraptorial sperm whales (Cetacea, Odontoceti) from the Miocene of Peru. Zool. J. Linnean Soc. 179, 404–474. doi: 10.1111/zoj.12456
Lambert, O., Bianucci, G., Post, K., de Muizon, C., Salas-Gismondi, R., Urbina, M., et al. (2010). The giant bite of a new raptorial sperm whale from the Miocene epoch of Peru. Nature 466, 105–108. doi: 10.1038/nature09067
Lambertsen, R. L., Ulrich, N., and Straley, J. (1995). Frontomandibular stay of Balaenopteridae: a mechanism for momentum recapture during feeding. J. Mammal. 76, 877–899. doi: 10.2307/1382758
Lanyon, L. E., and Rubin, C. T. (1985). “Functional adaptation in skeletal structures” in Functional Vertebrate Morphology. eds. M. Hildebrand, D. M. Bramble, K. F. Liem, and D. B. Wake (Cambridge: Harvard Univ Press), 1–25.
Lauder, G. (1985). “Aquatic feeding in lower vertebrates” in Functional Vertebrate Morphology. eds. M. Hildebrand, D. M. Bramble, K. F. Liem, and D. B. Wake (Cambridge: Harvard Univ Press), 210–229.
Lawrence, B., and Schevill, W. E. (1965). Gular musculature in delphinids. Bull. Mus. Comp. Zool. 133, 1–65.
Loch, C., Kieser, J. A., and Fordyce, R. E. (2015). Enamel ultrastructure in fossil cetaceans (Cetacea: Archaeoceti and Odontoceti). PLoS One 10:e0116557. doi: 10.1371/journal.pone.0116557
Lucas, P. W. (2004) Dental Functional Morphology: How Teeth Work. Cambridge University Press, New York.
Marshall, C. D., and Goldbogen, J. A. (2015). “Marine mammal feeding mechanisms” in Marine Mammal Physiology: Requisites for Ocean Living. eds. M. A. Castellini and J. A. Mellish (Boca Raton, FL: CRC), 95–117.
Marshall, C. D., and Pyenson, N. D. (2019). “Feeding in aquatic mammals: an evolutionary and functional approach” in Feeding in Vertebrates: Anatomy, Biomechanics, Evolution. eds. V. Bels and I. Q. Whishaw (New York: Springer), 743–785.
Marshall, C. D., Rosen, D. A., and Trites, A. W. (2015). Feeding kinematics and performance of basal otariid pinnipeds, Steller Sea lions and northern fur seals: implications for the evolution of mammalian feeding. J. Exp. Biol. 218, 3229–3240. doi: 10.1242/jeb.126573
Martinez-Caceres, M., Lambert, O., and de Muizon, C. (2017). The anatomy and phylogenetic affinities of Cynthiacetus peruvianus, a large dorudon-like basilosaurid (Cetacea, Mammalia) from the late Eocene of Peru. Geodiversitas 39, 7–163. doi: 10.5252/g2017n1a1
Maughan, R. J., Watson, J. S., and Weir, J. (1983). Strength and cross-sectional area of human skeletal muscle. J Physiol (London) 338, 37–49. doi: 10.1113/jphysiol.1983.sp014658
Norris, K. S., and Møhl, B. (1983). Can odontocetes debilitate prey with sound? Am. Nat. 122, 85–104. doi: 10.1086/284120
Nybakken, J. W., and Bertness, M. D. (2005). Marine Biology: An Ecological Approach 6e. Pearson Benjamin Cummings: New York.
O’Leary, M. A., and Uhen, M. D. (1999). The time of origin of whales and the role of behavioral changes in the terrestrial-aquatic transition. Paleobiology 25, 534–556. doi: 10.1017/S0094837300020376
Oelschlager, H. A. (1986). Tympanohyal bone in toothed whales and the formation of the tympano-periotic complex (Mammalia: Cetacea). J. Morphol. 188, 157–165. doi: 10.1002/jmor.1051880203
Peredo, C. M., Pyenson, N. D., and Uhen, M. D. (2022). Lateral palatal foramina do not indicate baleen in fossil whales. Sci. Rep. 12:11448. doi: 10.1038/s41598-022-15684-8
Putz, R., and Kroyer, A. (1999). Functional morphology of the pterygoid hamulus. Ann. Anat. 181, 85–88. doi: 10.1016/S0940-9602(99)80099-5
Pyenson, N. D., Goldbogen, J. A., Vogl, A. W., Szathmary, G., Drake, R. L., and Shadwick, R. E. (2012). Discovery of a sensory organ that coordinates lunge feeding in rorqual whales. Nature 485, 498–501. doi: 10.1038/nature11135
Racicot, R. A., and Berta, A. (2013). Comparative morphology of porpoise (Cetacea: Phocoenidae) pterygoid sinuses: phylogenetic and functional implications. J Morph. 274, 49–62. doi: 10.1002/jmor.20075
Racicot, R. A., Demere, T. A., Beatty, B. L., and Boessenecker, R. W. (2014). Unique feeding morphology in a new prognathous extinct porpoise from the Pliocene of California. Curr. Biol. 24, 774–779. doi: 10.1016/j.cub.2014.02.031
Ray, G. C., and Schevill, W. E. (1974). Feeding of a captive gray whale, Eschrichtius robustus. Mar. Fish. Rev. 36, 31–38.
Reidenberg, J. S., and Laitman, J. T. (1987). Position of the larynx in Odontoceti (toothed whales). Anat. Rec. 218, 98–106. doi: 10.1002/ar.1092180115
Reidenberg, J. S., and Laitman, J. T. (1994). Anatomy of the hyoid apparatus in Odontoceti (toothed whales): specializations of their skeleton and musculature compared with those of terrestrial mammals. Anat. Rec. 240, 598–624. doi: 10.1002/ar.1092400417
Reidenberg, J. S., and Laitman, J. T. (2007). Blowing bubbles: an aquatic adaptation that risks protection of the respiratory tract in humpback whales (Megaptera novaeangliae). Anat. Rec. 290, 569–580. doi: 10.1002/ar.20537
Rubenstein, D. I., and Koehl, M. A. R. (1977). The mechanisms of filter feeding: some theoretical considerations. Amer. Natur. 111, 981–994. doi: 10.1086/283227
Rudwick, M. J. S. (1998). Georges Cuvier, Fossil Bones, and Geological Catastrophes: New Translations & Interpretations of the Primary Texts. Chicago, Ill: University of Chicago Press
Sanderson, S. L., Roberts, E., Lineburg, J., and Brooks, H. (2016). Fish mouths as engineering structures for vortical cross-step filtration. Nat. Commun. 7:e11092. doi: 10.1038/ncomms11092
Thewissen, J. G. M. (1998) The Emergence of Whales: Evolutionary Patterns in the Origins of Cetacea Plenum. Springer Science & Business Media, New York.
Thewissen, J. G. M., and Bajpai, S. (2001). Whale origins as a poster child for macroevolution: fossils collected in the last decade document the ways in which Cetacea (whales, dolphins, and porpoises) became aquatic, a transition that is one of the best documented examples of macroevolution in mammals. Bio Sci. 51, 1037–1049.
Thewissen, J. G. M., Cooper, L. N., Clementz, M. T., Bajpai, S., and Tiwari, B. N. (2007). Whales originated from aquatic artiodactyls in the Eocene epoch of India. Nature 450, 1190–1194. doi: 10.1038/nature06343
Thewissen, J. G. M., Cooper, L. N., George, J. C., and Bajpai, S. (2009). From land to water: the origin of whales, dolphins, and porpoises. Evol. Educ. Outreach. 2, 272–288. doi: 10.1007/s12052-009-0135-2
Thewissen, J. G. M., Sensor, J. D., Clementz, M. T., and Bajpia, S. (2011). Evolution of dental wear and diet during the origin of whales. Paleobiology 37, 655–669. doi: 10.1666/10038.1
Uhen, M. D. (2000). Replacement of deciduous first premolars and dental eruption in archaeocete whales. J. Mammal. 81, 123–133. doi: 10.1644/1545-1542(2000)081<0123:RODFPA>2.0.CO;2
Uhen, M. D. (2004). Form, function, and anatomy of Dorudon atrox (Mammalia Cetacea): an archaeocete from the middle to late Eocene of Egypt. Univ. Michigan Papers Paleontol. 34, 1–222.
Uhen, M. D. (2007). Evolution of marine mammals: back to the sea after 300 million years. Anat. Rec. 290, 514–522. doi: 10.1002/ar.20545
Uhen, M. D. (2010). The origin(s) of whales. Annu. Rev. Earth Planet. Sci. 38, 189–219. doi: 10.1146/annurev-earth-040809-152453
Ungar, P. S. (2010) Mammal Teeth: Origin, Evolution, and Diversity. Johns Hopkins University Press, Baltimore.
Van Valen, L. (1968). Monophyly or diphyly in the origin of whales. Evolution 22, 37–41. doi: 10.2307/2406647
von Koenigswald, W. (1997) Evolutionary trends in the differentiation of mammalian enamel ultrastructure. In: W. Koenigswaldvon and P. M. Sander (eds) Tooth Enamel Microstructure. Balkema, Rotterdam, 203–225
Wainwright, P. C., McGee, M. D., Longo, S. J., and Hernandez, L. P. (2015). Origins, innovations, and diversification of suction feeding in vertebrates. Integr. Comp. Biol. 55, 134–145. doi: 10.1093/icb/icv026
Werth, A. J. (2000). “Marine mammals” in Feeding: Form, Function and Evolution in Tetrapod Vertebrates. ed. K. Schwenk (New York: Academic), 475–514.
Werth, A. J. (2004). Functional morphology of the sperm whale (Physeter macrocephalus) tongue, with reference to suction feeding. Aq. Mamm. 30, 405–418. doi: 10.1578/AM.30.3.2004.405
Werth, A. J. (2006a). Mandibular and dental variation and the evolution of suction feeding in Odontoceti. J. Mamm. 87, 579–588. doi: 10.1644/05-MAMM-A-279R1.1
Werth, A. J. (2006b). Odontocete suction feeding: experimental analysis of water flow and head shape. J Morph 267, 1415–1428. doi: 10.1002/jmor.10486
Werth, A. J. (2007). Adaptations of the cetacean hyolingual apparatus for aquatic feeding and thermoregulation. Anat. Rec. 290, 546–568. doi: 10.1002/ar.20538
Werth, A. J. (2013). Flow-dependent porosity of baleen from the bowhead whale (Balaena mysticetus). J. Exp. Biol. 216, 1152–1159. doi: 10.1242/jeb.078931
Werth, A. J., Ito, H., and Ueda, K. (2020). Multiaxial movements of the minke whale temporomandibular joint. J. Morphol. 281, 402–412. doi: 10.1002/jmor.21107
Werth, A. J., Loch, C., and Fordyce, R. E. (2019). Enamel microstructure in Cetacea: a case study in evolutionary loss of complexity. J. Mamm. Evol. 27, 789–805. doi: 10.1007/s10914-019-09484-7
Werth, A. J., and Potvin, J. (2016). Baleen hydrodynamics and morphology of cross-flow filtration in balaenid whale suspension feeding. PLoS One 11:e0150106. doi: 10.1371/journal.pone.0150106
Keywords: Cetacea, feeding, morphology, fossil, bone
Citation: Werth AJ and Beatty BL (2023) Osteological correlates of evolutionary transitions in cetacean feeding and related oropharyngeal functions. Front. Ecol. Evol. 11:1179804. doi: 10.3389/fevo.2023.1179804
Edited by:
Sylvain Charbonnier, Muséum National d’Histoire Naturelle, FranceReviewed by:
Alexandra Quilhac, Sorbonne Universités, FranceChristian De Muizon, Centre National de la Recherche Scientifique (CNRS), France
Copyright © 2023 Werth and Beatty. This is an open-access article distributed under the terms of the Creative Commons Attribution License (CC BY). The use, distribution or reproduction in other forums is permitted, provided the original author(s) and the copyright owner(s) are credited and that the original publication in this journal is cited, in accordance with accepted academic practice. No use, distribution or reproduction is permitted which does not comply with these terms.
*Correspondence: Alexander J. Werth, YXdlcnRoQGhzYy5lZHU=
†ORCID: Alexander J. Werth, https://orcid.org/0000-0002-7777-478X
Brian L. Beatty, https://orcid.org/0000-0002-5464-0041