- 1Key Laboratory of Ecosystem Network Observation and Modeling, Institute of Geographic Sciences and Natural Resources Research, Chinese Academy of Sciences, Beijing, China
- 2National Ecosystem Science Data Center, Institute of Geographic Sciences and Natural Resources Research, Chinese Academy of Sciences, Beijing, China
- 3University of Chinese Academy of Sciences, Beijing, China
- 4College of Resources and Environment, University of Chinese Academy of Sciences, Beijing, China
- 5School of Earth System Science, Tianjin University, Tianjin, China
The karst region of southwest China showed a significant increase in vegetation cover and vegetation carbon stocks under the implementation of a series of ecological restoration projects. However, the relative contribution of ecological restoration projects to terrestrial carbon sequestration in the context of climate change has yet to be well quantified. Here, we used the Community Land Model (CLM4.5) to investigate the trend of net ecosystem productivity (NEP) and attribution to multiple environmental factors in the karst region of southwest China during 2000–2018. The result showed that ecosystems with a significant increasing trend of NEP covered about 46% of the study region, which were mainly located in the peak forest plain region, colliculus region, peak cluster depression region, and middle-high hill region. The simulation experiments suggested that land use change associated with ecological restoration projects caused a large contribution of 53% to the increasing NEP trend, followed by CO2 fertilization (72%), while climate factors and nitrogen deposition showed minor negative effects. Especially, the NEP trend induced by land use change in the 100 pilot counties with the implementation of rocky desertification control project was significantly higher than that in the other karst area. Moreover, moderate and high levels of restoration efforts invested into recovery led to a larger increasing trend (0.66 gC/m2/yr2 and 0.48 gC/m2/yr2) in NEP than the low efforts level (0.22 gC/m2/yr2). Our results highlight the important role of ecological restoration projects in the enhanced terrestrial carbon sequestration in the karst region of southwest China, and recommend a comprehensive assessment of ecological restoration projects for policymaking.
1. Introduction
The karst region of southwest China holds one of the largest continuous karsts in the world, which is highly sensitive to the increasing climate variability and extreme climate events (Kharin et al., 2013; Seddon et al., 2016). Meanwhile, the increase in population expanded the demand for natural resources and caused a severe rocky desertification (Sweeting, 2012; Delang and Yuan, 2016). Approximately 0.13 million km2 of karst areas were transformed into rocky landscapes from natural vegetation (Yuan, 1997; Wang et al., 2004). To combat land degradation and improve the ecological security situation, Chinese governments have invested more than 130 billion yuan in launching a series of ecological restoration projects since 1999 (Delang and Yuan, 2016). The Grain to Green Project is the most ambitious project for restoring natural vegetation and prevention of sloping farmland in human history (Ouyang et al., 2016; Xu et al., 2017). The government has also set up the rocky desertification control projects in the karst region of Southwest China since 2008, which covered from 100 pilot counties at first to all 451 rocky desertification counties in 2015. With the implementation of these ecological restoration projects, many studies have found that a recent increase in the Normalized Difference Vegetation Index (NDVI) and Leaf Area Index (LAI) (Cai et al., 2014; Xu and Zhang, 2014), aboveground biomass carbon (ABC) (Tong et al., 2020), and vegetation optical depth (VOD) (Brandt et al., 2018) in the karst region of Southwest China. This region’s ecosystem quality increased simultaneously (Zhang M. et al., 2022). Estimation of the effect of ecological restoration projects on ecosystem functions (especially carbon sequestration function) in the karst region of Southwest China is crucial to the ecosystem management and performance assessment of ecological civilization construction.
Previous studies have confirmed the positive effect of ecological restoration projects on vegetation cover and vegetation carbon stocks based on different methods (Zhang et al., 2015; Zhang M. et al., 2016; Tong et al., 2017, 2018). Based on residuals between observed and predicted vegetation cover and biomass trends, Tong et al. (2017) found a direct positive effect of management in Guizhou, Yunnan, and Guangxi. Residual analysis also indicated that ecological services were improved by rocky desertification control in a typical karst region of northwest Guangxi (Zhang M. et al., 2016). The positive impacts of rocky desertification control measures on the vegetation carbon services were found in the typical karst area of northwest Guangxi through the method of canonical correspondence analysis (Zhang et al., 2015). By comparing the results of satellite products and dynamic vegetation models, Tong et al. (2018) pointed out that the areas of high ecological restoration projects have stronger positive trends in vegetation cover and vegetation carbon stocks, while the areas of little ecological restoration projects have weaker positive trends in vegetation cover and vegetation carbon stocks in Guizhou, Yunnan, and Guangxi after 2000. Besides the role of ecological restoration projects, these changes on vegetation cover and vegetation carbon stocks are also associated with other environmental drivers (Wang et al., 2014), such as changing climate, rising atmospheric CO2 concentration, and increasing nitrogen deposition (Choi, 2004; Seabrook et al., 2011). Climatic conditions, human management, and disturbances may influence the effectiveness of ecological restoration projects. High annual rainfall and soil moisture after drought events help increase vegetation cover, especially in the areas where ecological restoration projects are implemented (Brandt et al., 2018). High investments of ecological restoration projects combined with sufficient rainfall generally increase the effectiveness of ecological restoration projects (Tong et al., 2017). However, the relative contribution of ecological restoration projects on ecosystem functions (especially carbon sequestration function) in the context of climate change and human activities in the karst region of Southwest China has not been well quantified.
The primary actions of the ecological restoration projects include expanding the natural vegetation, reducing sloping farmland, and cultivating crops on more fertile and less erodible soils (Tong et al., 2020). The implementation of ecological restoration projects directly influences the ecosystem functions (especially carbon sequestration function) through the land use change (Qin et al., 2022). The objectives of this study are to investigate the trend of NEP in the karst region of southwest China during 2000–2018, and to analyze the relative contribution from multiple environmental factors (i.e., climate change, atmospheric CO2 concentration, nitrogen deposition, and land use change). Here, we used the Community Land Model version 4.5 (CLM4.5) to simulate the temporal variation of net ecosystem productivity (NEP) and region contribution in different karst region, and conducted a series of simulation experiments to quantify the impacts from different drivers on NEP trend.
2. Materials and methods
2.1. Study region
As one of the most typical, complex, and extensive karst landforms worldwide, the karst region of Southwest China includes Chongqing, Hubei, Hunan, Guangdong, Guangxi, Guizhou, Sichuan, and Yunnan provinces (Figure 1). With poor soil formation ability, thin soil depth, and poor surface water retention (Jiang et al., 2014), the environment in this region is vulnerable and very sensitive to climate change and anthropogenic activities (Yuan et al., 1990). Subtropical monsoon climate is the main climate types, with a mean manual temperature of 15.00°C and a mean manual precipitation of 1,100 mm. The vegetation coverage is high with a proportion of forest land and grassland equaling 82.43%. According to similarities of the karst geomorphology and its formation causes, the karst region of Southern China could be divided into eight karst regions, namely Peak forest plain region (I), Trough valley region (II), Colliculus region (III), Gorge region (IV), Peak Cluster Depression region (V), Faulted Basin region (VI), Plateau region (VII), and Middle-high Hill region (VIII) (Supplementary Table S1; Wang et al., 2015). Because of the increasing intensity of human exploitation of natural resources, about 0.13 million km2 of karst areas were turned into rocky landscapes. A series of ecological restoration projects has been implemented to improve the environment (Tong et al., 2018). The 100 pilot counties with the implementation of rocky desertification control project since 2008 are mainly located in the regions of Colliculus (II), Gorge (IV), and Plateau (VII). According to the total area of ecological restoration projects, the 100 pilot counties are divided into three groups: (1) less than 50 km2 (low efforts), (2) 50–100 km2 (moderate efforts), and (3) more than 100 km2 (high efforts) (Tong et al., 2018).
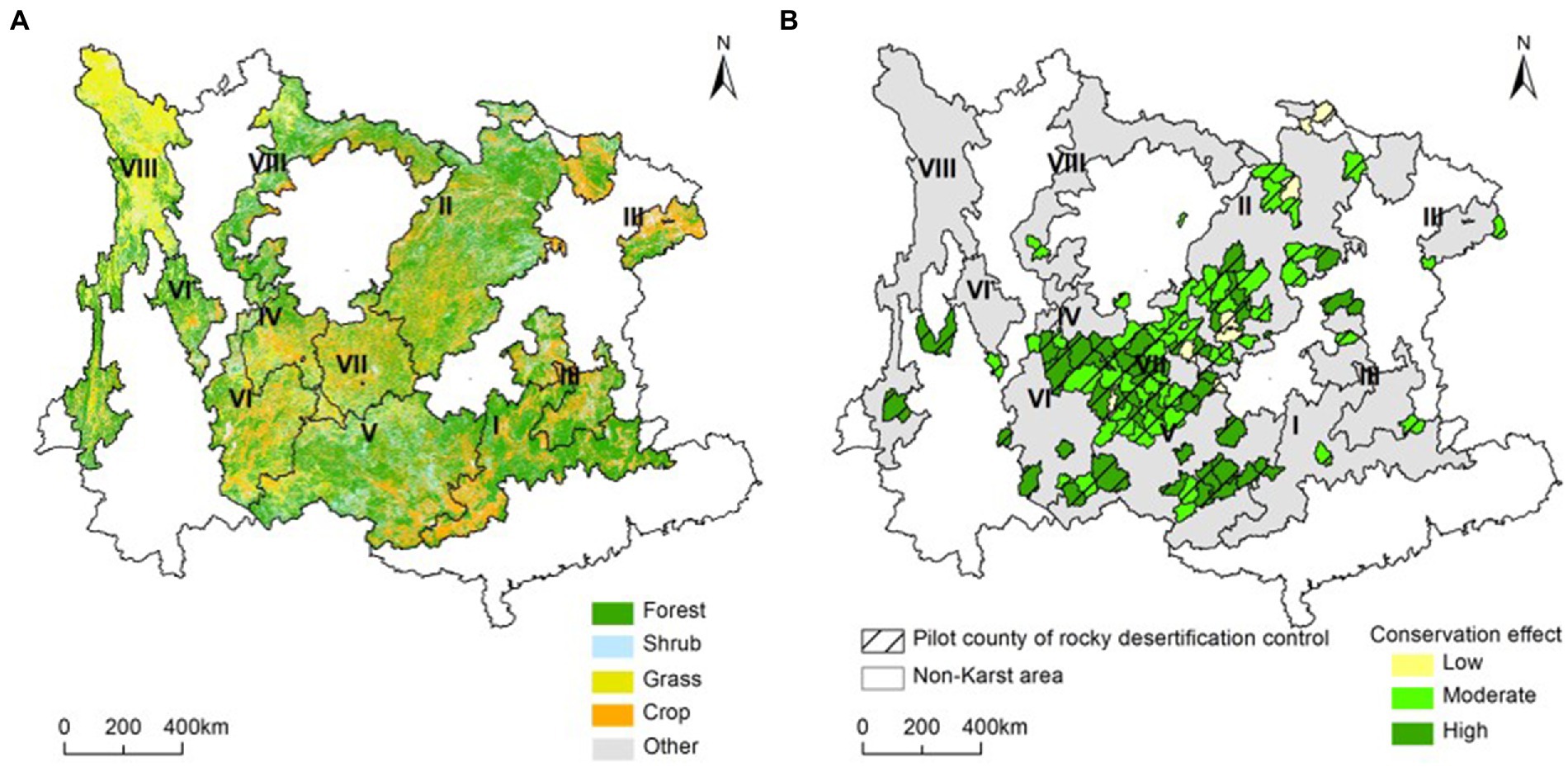
Figure 1. The vegetation type in karst region in Southwest China (A) and three groups of the 100 pilot counties of rocky desertification control since 2008 (B): (1) less than 50 km2 (low efforts), (2) 50–100 km2 (moderate efforts), and (3) more than 100 km2 (high efforts). I: Peak forest plain, II: Trough valley, III: Colliculus, IV: Gorge, V: Peak Cluster Depression, VI: Faulted Basin, VII: Plateau, VIII: Middle-high Hill.
2.2. Data
The input data of CLM4.5 mainly include seven climate variables (i.e., air temperature, precipitation, relative humidity, downwelling longwave radiation, downwelling short-wave radiation, surface pressure, and wind speed), two atmospheric environment variables (i.e., atmospheric CO2 concentration and nitrogen deposition), and other environment variables, such as plant function type, and dynamic land use and land cover change data. Climate data (1979–2018) were extracted from the high spatial and temporal resolution surface meteorological element driven dataset in China with a temporal resolution of 3 h and a spatial resolution of 0.1o (He et al., 2020). The atmospheric CO2 concentration (1850–2015) with a spatial resolution of 1o comes from Mauna Loa Observatory (Lamarque et al., 2010). The atmospheric nitrogen deposition data (1980–2015) with a spatial resolution of 0.05o were calculated based on the atmospheric inorganic nitrogen wet deposition data proposed by Jia et al. (2019) and the dry and wet nitrogen deposition ratio developed by Yu et al. (2019). The plant functional type and dynamic land use and land cover changes data (1990–2015) with a spatial resolution of 0.05o were processed based on the land cover data of China (ChinaCover) produced by Wu et al. (2014) and climate variables (Wang et al., 2017) using the method reported in Bonan et al. (2018). The parameter values of ratio of leaf carbon to nitrogen for each plant functional type were revised against the inventory data (Tang Z. et al., 2018). All input data of CLM4.5 was resampled to a unified spatial resolution (0.5o). Climate data has a temporal resolution of 6 h. Other input data have a temporal resolution of 1 year. Then we could use these data to force the CLM4.5.
Modelled outputs were evaluated by estimated carbon flux production from Yao et al. (2018) and Jung et al. (2011), and field inventory data of vegetation and soil carbon density (Fang et al., 2018; Tang X. et al., 2018) in the study region. Yao et al. (2018) used a machine learning algorithm to develop a GPP dataset based on observation data from 40 stations in China and surrounding countries during 1982–2015. Jung et al. (2011) also used a machine learning algorithm to develop a terrestrial land-atmosphere energy fluxes dataset based on observation data from FLUXNET during 1982–2011. Machine learning algorithms have been applied to the upscaling of eddy covariance measurements from local to continental (Xiao et al., 2018). Therefore, these products produced by machine learning algorithms could be approximately regarded as the observed data.
We used the method in Vicente-Serrano (2006) to calculate Standardized Precipitation Index (SPI) data using monthly precipitation data (Lv et al., 2022). The SPI computed on shorter scales (e.g., 3 months) describes the short-term moisture conditions that affect the ecosystem. We used the SPI 3 to identify drought events. The drought events were extracted with SPI 3 data <−1.0, which indicates when water shortages were developing or imminent (Vicente-Serrano et al., 2014). To quantify the influence of annual water stress on the karst ecosystems, the SPI 3 products at monthly resolution were aggregated to an annual mean.
2.3. CLM4.5
2.3.1. Model description
The CLM4.5 model is a state-of-the-art land surface model for simulating bio-geophysical and bio-geochemical processes in the atmosphere-vegetation-soil continuum (Oleson et al., 2013), which has a good performance in estimating carbon and water fluxes for terrestrial ecosystems (Zhang L. et al., 2016; Li et al., 2018) and has been well applicated in China’s terrestrial ecosystem (Jia et al., 2018; Lu et al., 2021; Xue et al., 2021).
2.3.2. Experiment design
We performed five simulations to assess the relative contributions of changing climate, rising atmospheric CO2 concentration, increasing nitrogen deposition, and historical land use change on the carbon sequestration (Table 1). In simulation S1, we kept the climate driver constant for the entire period 1990–2018 and varied the other environmental factors. In simulation S2, only the atmospheric CO2 concentration was hold constant. In simulation S3, we used the varied climatic factors, atmospheric CO2 concentration, and land use change and held the nitrogen deposition at 1990. In simulation S4, we only kept the land use constant, while varying the other environmental factors. Finally, in simulation S5, we allowed all factors to change throughout the full simulation. The effects of each individual factor are calculated by subtracting S1, S2, S3, and S4 from simulation S5. The simulated yearly variables for gross primary productivity (GPP), ecosystem respiration (ER), and NEP from 2000 to 2018 were used for the analysis in this study.
2.3.3. Model simulation
We determined the initial conditions by first running the CLM4.5 to equilibrium using a two-stage spin-up method. Initial spin-up followed the accelerated decomposition approach for 600 simulation years with the long-term average climate and all other environmental factors. Then a normal decomposition spin-up was operated for 1,000 years using a repeating 40-year (1979–2018) cycle of climatic factors and constant other environmental factors until a dynamic equilibrium was reached. After initialization, we performed a transient run from 1901 to 2018 of the time series data of all driving factors. In this study, we choose 1990 as the base year. The annual temperature (13.64°C) and annual precipitation (1,364 mm) in 1990 are close to the multi-year average value during 1990–2018 (13.96°C and 1,370 mm). Moreover, the “base year” of emission reduction stipulated in the Kyoto Protocol is 1990. The emission reduction effect of greenhouse gases needs to be referenced and measured relative to 1990.
3. Results
3.1. Model evaluation
We evaluated modelled annual GPP by CLM4.5 with the GPP estimation extracted from the results of Yao et al. (2018) and Jung et al. (2011). Modelled annual GPP has a similar spatial pattern with the estimation based on eddy-flux observations in the karst region of Southwest China (Figures 2A–C). The Pearson correlation coefficients between GPP modelled by CLM4.5 and the estimated data of Yao et al. (2018) and Jung et al. (2011) across the eight regions were 0.94 and 0.87, respectively. For those areas with high GPP in the Peak forest plain region (I), Trough valley region (II), Colliculus region (III), Peak Cluster Depression region (V), and Plateau region (VII), the model bias for GPP varied from −8.65 to 3.35% (Figure 2D). The mean total annual GPP in the study area modelled by CLM4.5 is 1.56 Pg C/yr., which was slightly higher than that in the previous studies by 11–16%.
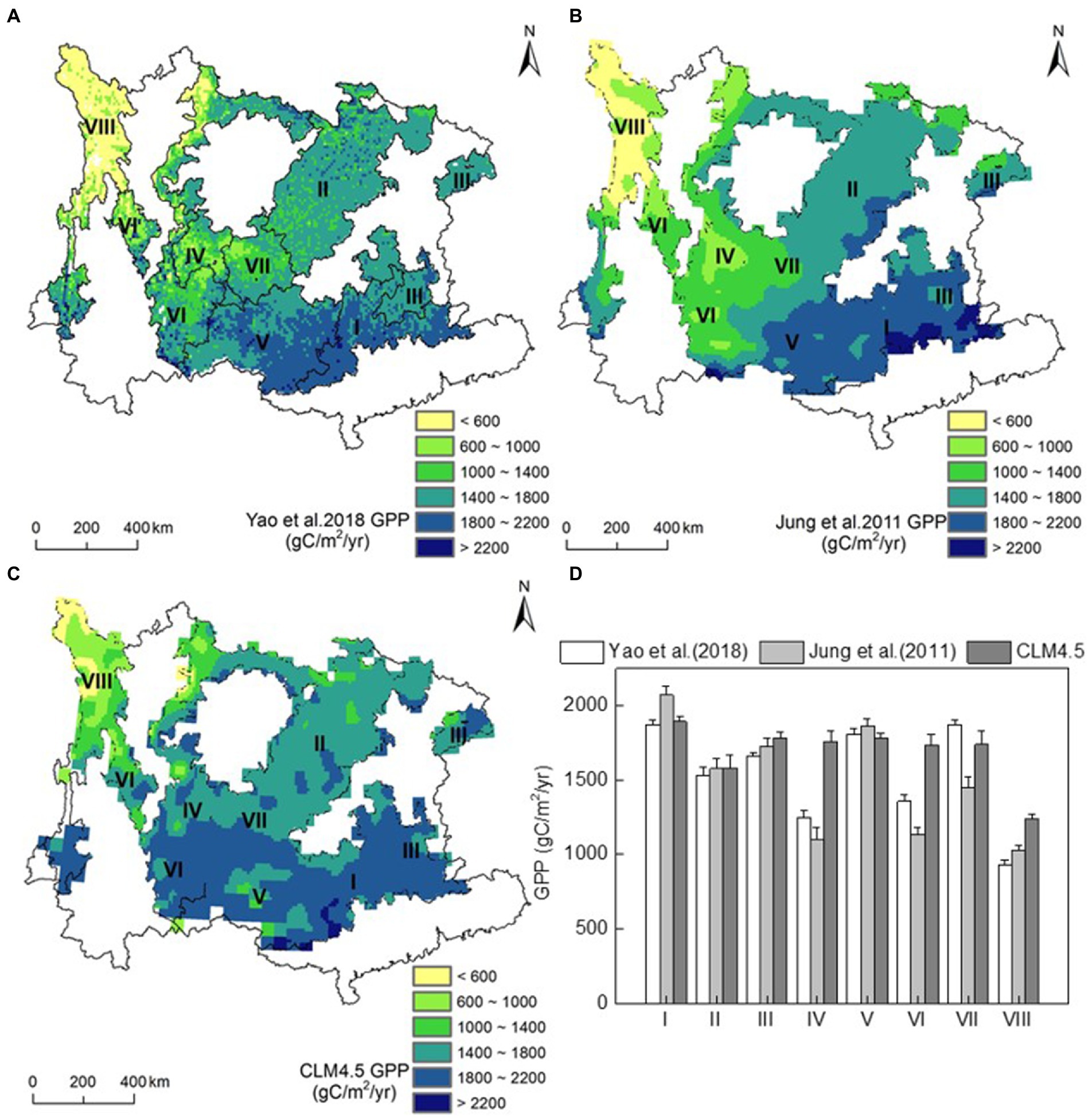
Figure 2. Comparison of mean gross primary productivity (GPP) between Yao et al. (2018), Jung et al. (2011), and the CLM4.5 model. The spatial pattern of mean GPP in different karst regions in Yao et al. (2018), Jung et al. (2011), and the CLM4.5 model (A–C). The mean GPP in different karst regions in Yao et al. (2018), Jung et al. (2011), and the CLM4.5 model (D). I: Peak forest plain, II: Trough valley, III: Colliculus, IV: Gorge, V: Peak Cluster Depression, VI: Faulted Basin, VII: Plateau, VIII: Middle-high Hill.
Figure 3 shows the comparison of observed and modelled mean vegetation carbon and soil carbon density for different forest types in the karst region of Southwest China. The CLM4.5 model could capture the variation in vegetation carbon density among different forest types but overestimate by 14.0% for ENF, 19.7% for DBF, 25.3% for MF, and 26.8% for EBF (Figure 3A). The mean values of the modelled soil carbon density were well consistent with the inventory data, with relative errors ranging from −2.6 to 3.9% for DBF, EBF, and MF, except 17.7% for ENF (Figure 3B).
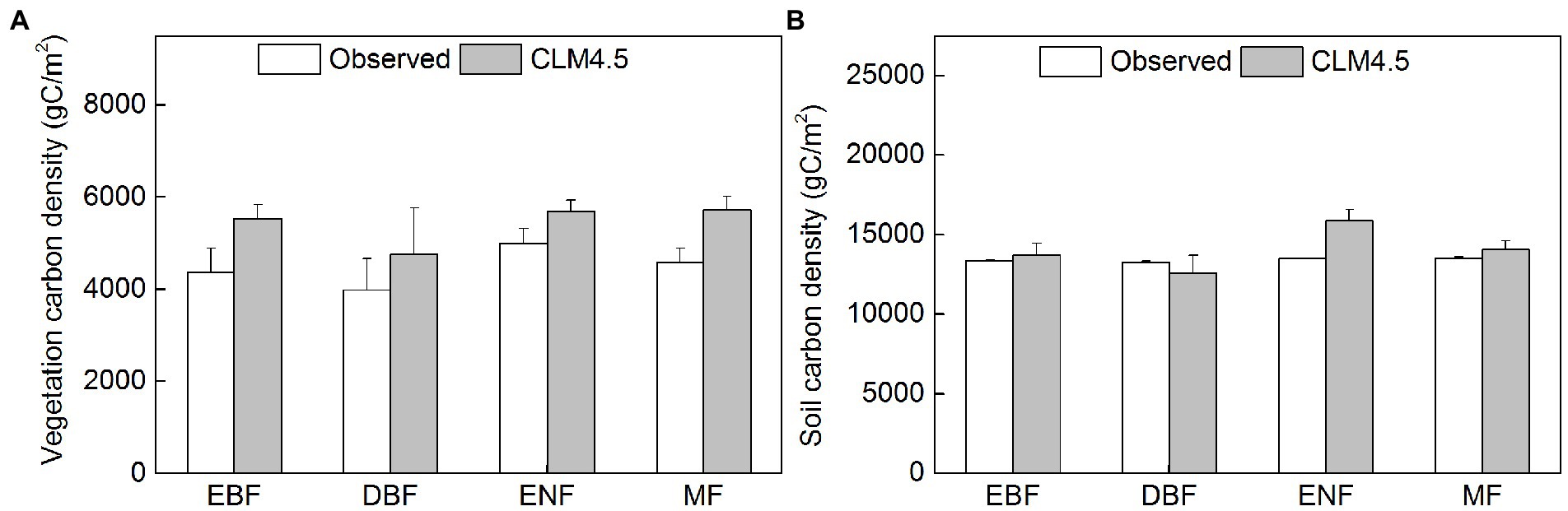
Figure 3. Comparison of vegetation carbon density (A) and soil carbon density (B) in different plant types in karst region of Southwest China between the observation and CLM4.5. EBF: evergreen broadleaved forest, DBF: deciduous broadleaved forest, ENF: evergreen needle leaved forest, MF: mixed forest.
3.2. Long-term trend of NEP and regional contribution
There was an increasing trend in NEP, although it was not statistically significant (0.16 Tg C/yr2, p = 0.87). However, the trend of NEP showed a large spatial heterogeneity in the karst region of Southwest China. About 76% of the study region shows an increasing trend, among which 33% is significant at p = 0.05 level, which were mainly located in the Peak forest plain region (I), Colliculus region (III), Peak Cluster Depression region (V), and Middle-high Hill region (VIII) (Figures 4A,B). The increasing trend in NEP in these areas reached to 0.93 Tg C/yr2 (Figure 4C), because a larger increasing rate in GPP (3.46 Tg C/yr2) than that in ER (2.53 Tg C/yr2). The accumulated NEP increased from 24.95 to 380.16 Tg C/yr in these areas (Figure 4C), which highlighted the important role in these areas in carbon sequestration.
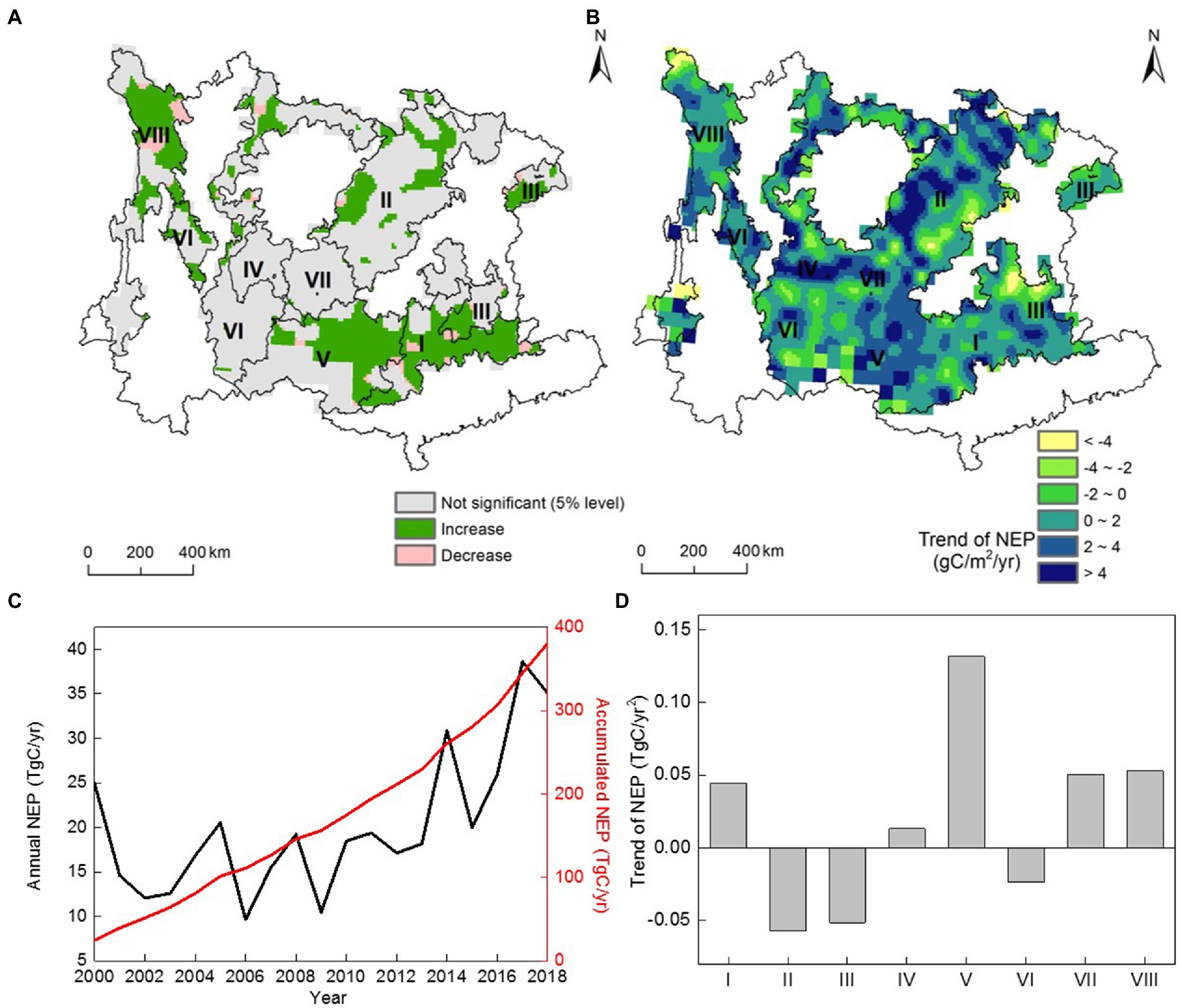
Figure 4. Temporal change and regional contribution of net ecosystem productivity (NEP) in karst region of Southwest China over the period 2000–2018 estimated by the CLM4.5 model. (A) Significance of the NEP trend in karst region of Southwest China during 2000–2018. (B) Temporal changes of the NEP in karst region of Southwest China during 2000–2018. (C) Annual NEP and accumulated NEP in the region of significant variation in NEP during 2000–2018. (D) Regional contribution of the NEP trend. I: Peak forest plain, II: Trough valley, III: Colliculus, IV: Gorge, V: Peak Cluster Depression, VI: Faulted Basin, VII: Plateau, VIII: Middle-high Hill.
The trend of NEP in different karst regions over the period 2000–2018 was shown in Figure 4D. Ecosystems in the Peak forest plain region (I), Peak Cluster Depression region (V), and Middle-high Hill region (VIII) dominated the increase in NEP, with the contribution of 28, 82, and 33%, respectively. The increasing rate of NEP in the Peak Cluster Depression region (V) was the highest (0.14 Tg C/yr2, p = 0.06), followed by Peak forest plain region (I) and Middle-high Hill region (VIII) with similar trend of 0.057–0.059 Tg C/yr2. Besides these three regions, the Plateau region (VII) also had a large contribution to the NEP trend (32%). The increases in NEP in these regions were all resulted from the higher enhancement in GPP than that in ER.
3.3. Contribution of environmental factors to the trend in NEP
Simulation experiments suggested that atmospheric CO2 concentration (73%) and land use change (53%) dominated the increasing NEP trend, while climate change and nitrogen deposition led to the decrease in NEP (−26% and − 0.2%, respectively). CO2 fertilization significantly stimulate NEP due to larger response of GPP than ER (3.05 vs. 2.96 Tg C/ppm), especially in the Peak forest plain region (I), Trough valley region (II), and Middle-high Hill region (VIII) (Figure 5B).
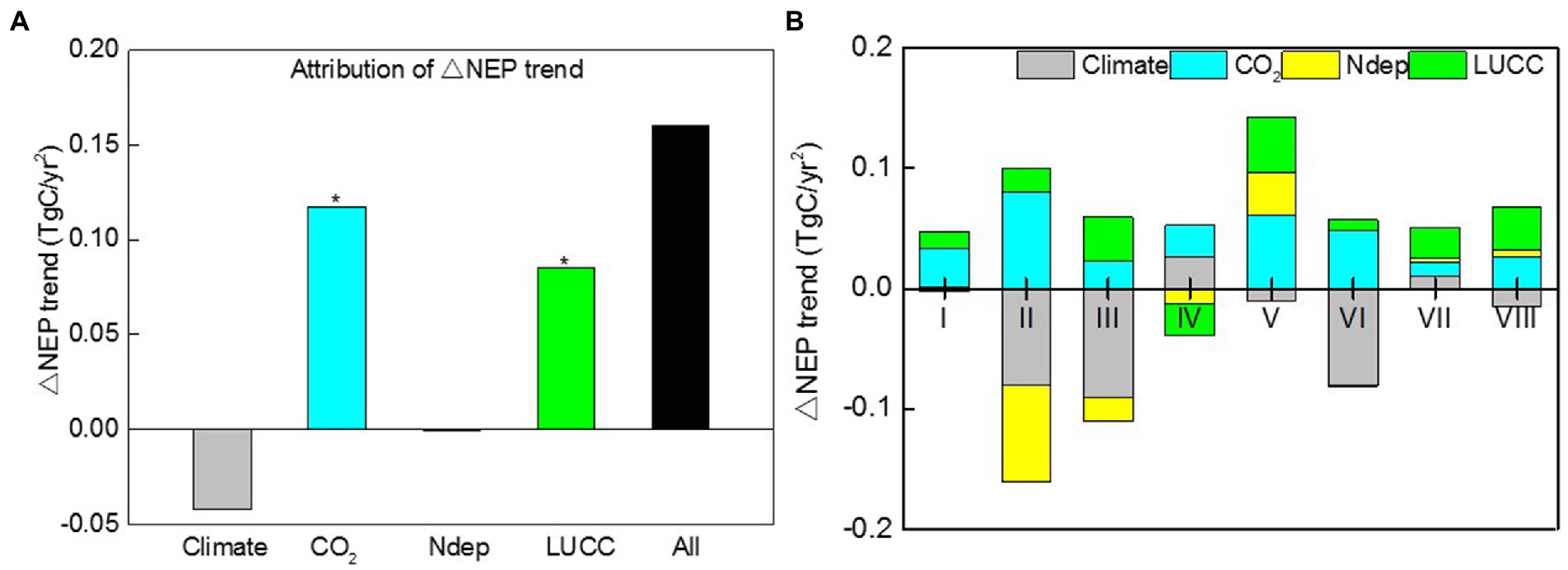
Figure 5. Effects of environmental factors (i.e., climatic factors (Climate), atmospheric carbon dioxide concentration (CO2), nitrogen deposition (Ndep), land use change (LUCC), and all environmental factors (All)) on the net ecosystem productivity (NEP) trend (A) in karst region of Southwest China and different karst regions (B). I: Peak forest plain, II: Trough valley, III: Colliculus, IV: Gorge, V: Peak Cluster Depression, VI: Faulted Basin, VII: Plateau, VIII: Middle-high Hill. * means p < 0.05.
The increasing NEP trend induced by land use change (0.09 Tg C/yr2, p < 0.05, Figure 5A) was mainly related to the large-scale afforestation (3,964 km2), and cropland abandonment (13,517 km2) under the implementation of a series of ecological restoration projects since 2000 (Supplementary Figure S1D). Especially in the Colliculus region (III) and Plateau region (VII), the area of afforestation is eight times that of other karst regions (Supplementary Table S3). In addition, the contribution of land use change to the NEP trend is higher than the contribution of CO2 in the Colliculus region (III), Plateau region (VII), and Middle-high Hill region (VIII) (Figure 5B).
There was a larger increase in NEP (+0.44 gC/m2/yr2, p < 0.05, Figure 6B) induced by land use change in the 100 pilot counties of rocky desertification control than that in the other karst area due to higher trend in GPP (+0.21 gC/m2/yr2, p < 0.05) and less in ER (−0.23 gC/m2/yr2, p < 0.05). Moreover, the positive effect of land use change on NEP trend varied with ecological restoration projects efforts. The difference in impacts of land use change were analysed at the county level by dividing the 100 pilot counties of rocky desertification control into three groups according to the total ecological restoration projects areas (Figure 1B). The difference in the area of conversion of farmland into forest in the low ecological restoration projects area (625 km2) and the moderate and high ecological restoration projects areas (3,900 and 3,075 km2, respectively) led to the difference of NEP trend driven by land use change in three groups. The NEP trend induced by land use change in the counties with moderate and high ecological restoration projects efforts (0.66 and 0.58 gC/m2/yr2, p < 0.05, respectively) significantly greater than those with low efforts (0.22 gC/m2/yr2, p < 0.05). In addition, the trend in NEP increased significantly with increasing forest coverage (Slope = 0.46, p < 0.05, Figure 6C) in the 100 pilot counties of rocky desertification control. These results indicated that the implementation of ecological restoration projects in the karst region of Southwest China play a remarkable role in enhancing carbon sequestration, especially in the moderate and high ecological restoration projects efforts areas. The positive NEP trend induced by land use change in counties of high ecological restoration projects efforts area was slightly lower than those with moderate efforts area, which might be associated with the drought events in 2009 and 2011. The karst region of Southwest China experience drought events in 2009 and 2011, of which 13 and 5%, respectively, in the high ecological restoration projects area (Supplementary Figure S2).
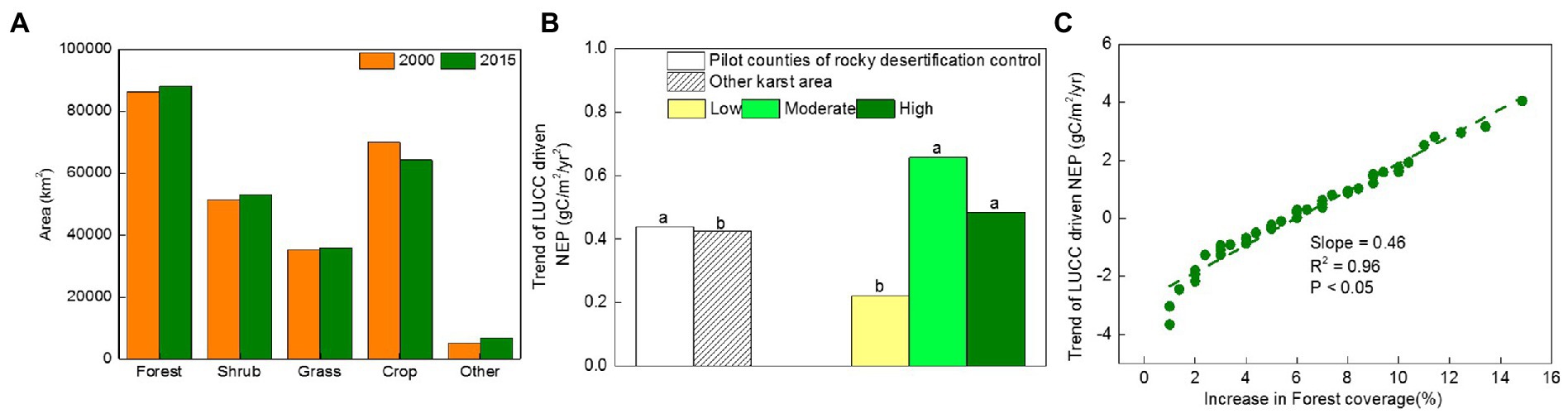
Figure 6. Temporal change of land use change (LUCC) in 2000 and 2015 in the 100 pilot counties of rocky desertification control (A), land use change (LUCC) driven net ecosystem productivity (NEP) in the 100 pilot counties of rocky desertification control and other karst area, and in the three groups of the 100 pilot counties of rocky desertification control during 2008–2018 (B), and the relationship between the increase in forest coverage and trend of LUCC driven NEP (C). According to their total area of ecological restoration projects, the 100 pilot counties of rocky desertification control were divided in three groups: (1) less than 50 km2 (low efforts), (2) 50–100 km2 (moderate efforts), and (3) more than 100 km2 (high efforts). a and b on the bar chart mean that there is a significant difference in the trend of NEP between different groups.
The negative effect of climate on the NEP trend in this region (−0.04 Tg C/yr2, p = 0.83, Figure 5A) was mainly caused by the significant warming with the rate of 0.023°C/yr (p < 0.05; Supplementary Figure S1C). The increasing temperature led to a faster increase in ER (2.65 Tg C/yr2, p = 0.09) than GPP (2.10 Tg C/yr2, p < 0.05). This negative effect of climate was mainly in the Trough valley region (II), Colliculus region (III), and Faulted Basin region (VI) (Figure 5B). The Trough valley region (II) and Colliculus region (III) had the large increase of temperature and the high response of NEP to temperature (Supplementary Table S4). In addition, the minor contribution of nitrogen deposition to the trend in NEP was mainly due to the significant decrease of nitrogen deposition (Supplementary Table S2) and the large response of NEP to nitrogen deposition in the Trough valley region (II) (Supplementary Table S4).
4. Discussion
Southwest China has been one of the largest coherent regions with positive biomass and vegetation cover trends since 2000 (Brandt et al., 2018). Consequently, vegetation carbon sequestration has increased since 2000 as revealed by both remote sensing data (Zhang et al., 2017; Tong et al., 2020) and ecosystem process models (Zhang M. et al., 2016; Tong et al., 2018). Our study demonstrated the increase in carbon sequestration in the karst region of Southwest China during 2000–2018, especially in the Peak Forest Plain region (I), the Peak Cluster Depression region (V), and the Middle-high Hill region (VIII). This increasing trend of carbon sequestration in the karst region of Southwest China is also supported by the terrestrial land-atmosphere energy fluxes dataset from Jung et al. (2011) with a significant increasing rate of 11.74 Tg C/yr2 (p < 0.05) for GPP and 5.75 Tg C/yr2 (p < 0.05) for NEP in 2000–2011, and Yao et al. (2018) with the increasing rate of GPP (1.24 Tg C/yr2, p = 0.50) during 2000–2015.
The increase in carbon sequestration in the karst region of southwest China has been proven to be associated with high ecological restoration projects after 2000 (Zhang M. et al., 2016; Brandt et al., 2018; Tong et al., 2018; Zhang X. et al., 2022). Our results suggested that land use change was the second large contributor to the sustained carbon sequestration besides elevated atmospheric CO2 concentration. To alleviate ecological degradation and ameliorate regional poverty, the Chinese government has invested in a series of mitigation initiatives, which are aimed at converting farmlands and degraded lands into conservation or production forests and grasslands (Delang and Yuan, 2016). The land use change resulted in a large increase in carbon sequestration by increasing the aboveground and underground carbon density (Hong et al., 2020) and the turnover of carbon storage (Chen and Tian, 2007). With the increase of forest coverage driven by ecological restoration projects, the average ratio of the ecosystem service index increased (Zhang M. et al., 2022). With the increase of ecological restoration projects efforts, the growth rate of vegetation cover and carbon density became faster (Lv et al., 2018; Tong et al., 2018). We found the differences of increasing trend in carbon sequestration between low and other (i.e., moderate and high) ecological restoration projects efforts (Figure 6B). The positive impact of the ecological restoration projects may be influenced by climatic conditions, human management, and disturbances. The temperate climate with sufficient rainfall and high investments generally lead to the high effectiveness of ecological restoration projects in Peak Forest Plain region (I) and Peak Cluster Depression region (V) (Tong et al., 2017).
Such a positive effect of land use change on the increase of carbon sequestration in China on the national scale had been demonstrated by Tian et al. (2011) using process-based models and Houghton and Nassikas (2017) using the bookkeeping model but was underestimated by Jia et al. (2020) based on results of the Multi-scale Synthesis and Terrestrial Model Intercomparison Project (MsTMIP). The low contributions of land use change to GPP might be related to the underestimates of the forest area in China (33,292 km2) in the land use change dataset produced by Hurtt et al. (2006) and Yu et al. (2022). On the other hand, the model assumptions relating to whether the productivity of secondary vegetation is smaller or larger than that of the vegetation it replaces are also important (Zhu et al., 2016). We found the land use change has a higher contribution to carbon sequestration (0.44 Tg C/yr2) in the karst region of southwest China, compared with the effect of land use change on carbon sequestration in China (0.27 Tg C/yr2). These results suggested that the ecological restoration projects in the karst region of southwest China play a crucial role in the increase of carbon sequestration in China. When considering the carbon emissions caused by land use change, the net biome productivity (NBP) still increased in the karst region of southwest China (1.23 Tg C/yr2, p = 0.28, Supplementary Figure S3).
The effects of atmospheric CO2 concentrations on carbon sequestration were consistent with previous studies in China (Tian et al., 2011; Jia et al., 2020) and the globe (Keenan et al., 2021) due to photosynthesis increases as CO2 concentrations rise (Huntingford and Oliver, 2021). In contrast, climate had a negative effect on the carbon sequestration in this karst region of southwest China as shown in both this study and Tong et al. (2017), which might be due to the increase in temperature and decrease in soil water during drought events in recent years (e.g., 2009 and 2011) (Song et al., 2019). Compared to the result of Tian et al. (2011), the nitrogen deposition’s contribution to carbon sequestration was lower in this study. The main reason is that the reference level of nitrogen deposition and other factors was assigned to the value in 1990 not that in 1900 as Tian et al. (2011). Right now, the nitrogen deposition level increased by 7.06 TgN/yr. than that of 1990 and 11.09 TgN/yr. than that of 1900 (data came from Tian et al., 2011) in China, but the nitrogen utilization efficiency (10.6 gC/gN) was similar to that (10.1 gC/gN) of Tian et al. (2011).
Under the recommendations for formulating China’s 14th five-year development plan and the long-range goals for 2035, the karst regions of southwest China will control more than 10,760 km2 of rocky desertification in Hubei, Guizhou, and Yunnan provinces. In Hunan province, more than 7.50 km2 of afforestation and grassland construction were carried out (Gao et al., 2021). Moreover, constructing the national rocky desert park is essential, which could realize the combination of rocky desertification control and poverty control. The implementation of these actions in 451 counties of karst desertification control will continue to increase carbon sequestration in forest and grassland, protect against soil erosion, and create a more biodiversity-rich landscape (Hogarth et al., 2013; Tong et al., 2020). Nevertheless, large-scale ecological restoration projects can have an adverse impact on the ecosystem by decreasing soil moisture and water availability (Feng et al., 2016; Jia et al., 2017; Zhao et al., 2021). Therefore, the ecological restoration projects require adjusted according to different situations. Such as, better water-conservancy areas are suitable for tropical and subtropical economic fruit species, while water-deficient areas should be planted drought tolerant savanna woody species (Zhang et al., 2012). And the benefits of ecological restoration projects should be comprehensively evaluated when considering the sustainability of ecosystems.
The impacts of multiple environmental factors on carbon sequestration have been in the spotlight of science (Qian et al., 2019; Liu et al., 2023). Based on process-based model, the simulation experiments could quantify the influence of changing climate, rising atmospheric CO2 concentration, increasing nitrogen deposition, and historical land use change on the carbon sequestration (Tian et al., 2011; He et al., 2019). The large contribution (53%) of land use change to the increasing NEP trend in our study is similar to the result of Yu et al. (2022), who pointed out that forest expansion increased China’s land carbon sink since 1980 using the simulation experiments in DLEM with a contribution of 44%. In addition, such an important role of land use change in stimulating the positive trend in carbon sequestration in China is also supported by previous studies using other approaches, such as residual analysis (Tong et al., 2017), canonical correspondence analysis (Zhang et al., 2015), comparison of the differences between satellite products and dynamic vegetation models (Tong et al., 2018), and comparison of the differences between the baseline period and post-period (Lu et al., 2018). However, we did not include the legacy of previous land use change, which might cause uncertainty about the estimated effect of land use change. Tian et al. (2011) pointed out that the land use change could cause a change of 2.7 to 4.1% in total carbon stocks in the first half of the 20th century. These land use legacy effects in the karst regions of southwest China require further exploration in the future. Other factors, such as forest management, grazing, irrigation and disturbances, were not included in the CLM4.5 in our study. Such a comprehensive assessment of ecological restoration projects requires the strengthening of long-term observation and research, and more efforts to better simulate the effects of land cover changes and land management practices on karst ecosystems (Chen et al., 2020) in the next generation process-based models.
5. Conclusion
In this study, we investigated the trend of carbon sequestration and the impacts of environmental factors in the karst region of Southwest China during 2000–2018 using the CLM 4.5 model. Our result showed that areas with a significant trend of NEP covered about 45.6% of the study region. This increasing NEP trend was mainly contributed by the peak forest plain region, peak cluster depression region, and the middle-high hill region. We found that land use change plays an important role in stimulating the positive trend in NEP besides atmospheric CO2 concentration in this region, while climate and nitrogen deposition had relatively small negative contributions. Moreover, with the large-scale afforestation and cropland abandonment, a notable increase in NEP was found in the 100 pilot counties of rocky desertification control project, when compared with the other karst areas. Furthermore, the areas with moderate and high ecological restoration efforts had a higher increasing rate of NEP than the low effort areas. Our study demonstrated the benefits of ecological restoration projects on carbon sequestration in the karst region of Southwest China, which could keep contributing to the achievement of China’s carbon neutrality.
Data availability statement
The datasets presented in the study are shown in the article, further inquiries can be directed to the corresponding author. Climate data were extracted from the high spatial and temporal resolution surface meteorological element driven dataset in China (He et al., 2020). The atmospheric CO2 concentration comes from Mauna Loa Observatory (Lamarque et al., 2010). The estimated carbon flux production comes from Yao et al. (2018) and Jung et al. (2011). The outputs of CLM4.5 are available upon request from Li Zhang (bGkuemhhbmdAaWdzbnJyLmFjLmNu).
Author contributions
YL: methodology, validation, and writing (original draft). LZ: experiment design, conceptualization, and editing. PL: investigation. HH: editing. XR: editing. MZ: editing. All authors contributed to the article and approved the submitted version.
Funding
This work was supported by the National Natural Science Foundation of China (31971512, 31988102, and 42141005).
Conflict of interest
The authors declare that the research was conducted in the absence of any commercial or financial relationships that could be construed as a potential conflict of interest.
Publisher’s note
All claims expressed in this article are solely those of the authors and do not necessarily represent those of their affiliated organizations, or those of the publisher, the editors and the reviewers. Any product that may be evaluated in this article, or claim that may be made by its manufacturer, is not guaranteed or endorsed by the publisher.
Supplementary material
The Supplementary material for this article can be found online at: https://www.frontiersin.org/articles/10.3389/fevo.2023.1179608/full#supplementary-material
References
Bonan, G. B., Levis, S., Kergoat, L., and Oleson, K. W. (2018). Landscapes as patches of plant functional types: an integrating concept for climate and ecosystem models. Global Biogeochem Cycles 16, 5-1–5-23. doi: 10.1029/2000GB001360
Brandt, M., Yue, Y., Wigneron, J. P., Tong, X., Tian, F., Jepsen, M. R., et al. (2018). Satellite-observed major greening and biomass increase in South China karst during recent decade. Earth’s Future 6, 1017–1028. doi: 10.1029/2018EF000890
Cai, H., Yang, X., Wang, K., and Xiao, L. (2014). Is forest restoration in the Southwest China karst promoted mainly by climate change or human-induced factors? Remote Sens. 6, 9895–9910. doi: 10.3390/rs6109895
Chen, A., Tang, R., Mao, J., Yue, C., Li, X., Gao, M., et al. (2020). Spatiotemporal dynamics of ecosystem fires and biomass burning-induced carbon emissions in China over the past two decades. Geogr Sustain 1, 47–58. https://doi:10.1016/j.geosus.2020.03.002. doi: 10.1016/j.geosus.2020.03.002
Chen, G., and Tian, H. (2007). Land use/cover change effects on carbon cycling in terrestrial ecosystems. Chin. J. Plant Ecol. 31, 189–204. doi: 10.17521/cjpe.2007.0024
Choi, Y. D. (2004). Theories for ecological restoration in changing environment: toward ‘futuristic’ restoration. Ecol. Res. 19, 75–81. doi: 10.1111/j.1440-1703.2003.00594_19_1.x
Delang, C. O., and Yuan, Z. (2016). China’s Grain for Green Program. New York, United States: Springer International.
Fang, J., Yu, G., Liu, L., Hu, S., and Chapin, F. S. (2018). Climate change, human impacts, and carbon sequestration in China. Proc. Natl. Acad. Sci. U. S. A. 115, 4015–4020. doi: 10.1073/pnas.1700304115
Feng, X., Fu, B., Piao, S., Wang, S., Ciais, P., Zeng, Z., et al. (2016). Revegetation in China’s loess plateau is approaching sustainable water resource limits. Nat. Clim. Chang. 6, 1019–1022. doi: 10.1038/nclimate3092
Gao, J., Li, G., Zhang, Y., and Zou, C. (2021). Analysis on Goals, Tasks and Realization Path of China's 14th Five Year Plan for Ecological and Environmental Protection. Environmental Protection. Environmental Protection. 49, 45–51. doi: 10.14026/j.cnki.0253-9705.2021.02.008
He, H., Wang, S., Zhang, L., Wang, J., Ren, X., Zhou, L., et al. (2019). Altered trends in carbon uptake in China's terrestrial ecosystems under the enhanced summer monsoon and warming hiatus. Natl. Sci. Rev. 6, 505–514. doi: 10.1093/nsr/nwz021
He, J., Yang, K., Tang, W., Lu, H., Qin, J., Chen, Y., et al. (2020). The first high-resolution meteorological forcing dataset for land process studies over China. Sci Data. 7:25. doi: 10.1038/s41597-020-0369-y
Hogarth, N. J., Belcher, B., Campbell, B., and Stacey, N. (2013). The role of forest-related income in household economies and rural livelihoods in the border-region of southern China. World Dev. 43, 111–123. doi: 10.1016/j.worlddev.2012.10.010
Hong, S., Yin, G., Piao, S., Dybzinski, R., Cong, N., Li, X., et al. (2020). Divergent responses of soil organic carbon to afforestation. Nat. Sustain. 3, 694–700. doi: 10.1038/s41893-020-0557-y
Houghton, R. A., and Nassikas, A. A. (2017). Global and regional fluxes of carbon from land use and land cover change 1850–2015. Global Biogeochem. Cycles 31, 456–472. doi: 10.1002/2016GB005546
Huntingford, C., and Oliver, R. J. (2021). Constraints on estimating the CO2 fertilization effect emerge. Nature 600, 224–225. doi: 10.1038/d41586-021-03560-w
Hurtt, G. C., Frolking, S., Fearon, M. G., Moore, B., Shevliakova, E., Malyshev, S., et al. (2006). The underpinnings of land-use history: three centuries of global gridded land-use transitions, wood-harvest activity, and resulting secondary lands. Glob. Chang. Biol. 12, 1208–1229. doi: 10.1111/j.1365-2486.2006.01150.x
Jia, B., Luo, X., Cai, X., Jain, A., Huntzinger, D. N., Xie, Z., et al. (2020). Impacts of land use change and elevated CO2 on the interannual variations and seasonal cycles of gross primary productivity in China. Earth Syst. Dynam. 11, 235–249. doi: 10.5194/esd-11-235-2020
Jia, X., Shao, M., Zhu, Y., and Luo, Y. (2017). Soil moisture decline due to afforestation across the loess plateau, China. J. Hydrol. 546, 113–122. doi: 10.1016/j.jhydrol.2017.01.011
Jia, B., Wang, Y., and Xie, Z. (2018). Responses of the terrestrial carbon cycle to drought over China: modeling sensitivities of the interactive nitrogen and dynamic vegetation. Ecol. Model. 368, 52–68. doi: 10.1016/j.ecolmodel.2017.11.009
Jia, Y., Wang, Q., Zhu, J., Chen, Z., He, N., and Yu, G. (2019). A spatial and temporal dataset of atmospheric inorganic nitrogen wet deposition in China (1996–2015). China Sci. Data. 4, 1–10.
Jiang, Z., Luo, W., Deng, Y., Cao, J., Qin, X., Li, Y., et al. (2014). The leakage of water and soil in the karst peak cluster depression and its prevention and treatment. Acta Geosci. Sin. 5, 535–542. doi: 10.3975/cagsb.2014.05.02 (In Chinese).
Jung, M., Reichstein, M., Margolis, H. A., Cescatti, A., Richardson, A. D., Arain, M. A., et al. (2011). Global patterns of land-atmosphere fluxes of carbon dioxide, latent heat, and sensible heat derived from eddy covariance, satellite, and meteorological observations. J. Geophys. Res. Biogeosci. 116:G3. doi: 10.1029/2010JG001566
Keenan, T. F., Luo, X., De Kauwe, M. G., Medlyn, B., Prentice, I. C., Stocker, B., et al. (2021). A constraint on historic growth in global photosynthesis due to increasing CO2. Nature 600, 253–258. doi: 10.1038/s41586-021-04096-9
Kharin, V. V., Zwiers, F. W., Zhang, X., and Wehner, M. (2013). Changes in temperature and precipitation extremes in the CMIP5 ensemble. Clim. Chang. 119, 345–357. doi: 10.1007/s10584-013-0705-8
Lamarque, J. F., Bond, T. C., Eyring, V., Granier, C., Heil, A., Klimont, Z., et al. (2010). Historical (1850–2000) gridded anthropogenic and biomass burning emissions of reactive gases and aerosols: methodology and application. Atmos. Chem. Phys. 10, 7017–7039. doi: 10.5194/acp-10-7017-2010
Li, P., Zhang, L., Yu, G., Liu, C., Ren, X., He, H., et al. (2018). Interactive effects of seasonal drought and nitrogen deposition on carbon fluxes in a subtropical evergreen coniferous forest in the east Asian monsoon region. Agric. Forest Meteorol. 263, 90–99. doi: 10.1016/j.agrformet.2018.08.009
Liu, M., Bai, X., Tan, Q., Luo, G., Zhao, C., Wu, L., et al. (2023). Climate change enhanced the positive contribution of human activities to net ecosystem productivity from 1983 to 2018. Front. Ecol. Evol. 10:1101135. doi: 10.3389/fevo.2022.1101135
Lu, F., Hu, H., Sun, W., Zhu, J., Liu, G., Zhou, W., et al. (2018). Effects of national ecological restoration projects on carbon sequestration in China from 2001 to 2010. Proc. Natl. Acad. Sci. U. S. A. 115, 4039–4044. doi: 10.1073/pnas.1700294115
Lu, T., Zhang, J., Xue, W., Qiao, Y., Zhou, L., and Che, Y. (2021). Impacts of aerosol direct radiative forcing on terrestrial ecosystem respiration in China from 2001 to 2014. Atmos. Res. 260:105713. doi: 10.1016/j.atmosres.2021.105713
Lv, Y., He, H., Ren, X., Zhang, L., Qin, K., Wu, X., et al. (2022). High resistance of deciduous forests and high recovery rate of evergreen forests under moderate droughts in China. Ecol. Indic. 144:109469. doi: 10.1016/j.ecolind.2022.109469
Lv, Y., Zhang, L., Yan, H., Ren, X., Wang, J., Niu, Z., et al. (2018). Spatial and temporal patterns of changing vegetation and the influence of environmental factors in the karst region of Southwest China. Acta Ecol. Sin. 38, 8774–8786.
Oleson, K., Lawrence, D., Bonan, G., Drewniak, B., Huang, M., Koven, C. D., et al. (2013). Technical Description of Version 4.5 of the Community Land Model (CLM), NCAR Technical Note: NCAR/TN-503+ STR. National Center for Atmospheric Research (NCAR), Boulder, CO, USA.
Ouyang, Z., Zheng, H., Xiao, Y., Polasky, S., Liu, J., Xu, W., et al. (2016). Improvements in ecosystem services from investments in natural capital. Science 352, 1455–1459. doi: 10.1126/science.aaf2295
Qian, C., Shao, L., Hou, X., Zhang, B., Chen, W., and Xia, X. (2019). Detection and attribution of vegetation greening trend across distinct local landscapes under China's grain to green program: a case study in Shaanxi Province. Catena 183:104182. doi: 10.1016/j.catena.2019.104182
Qin, C., Li, S., Yu, G., Bass, A. M., Yue, F., and Xu, S. (2022). Vertical variations of soil carbon under different land uses in a karst critical zone observatory (CZO), SW China. Geoderma 412:115741. doi: 10.1016/j.geoderma.2022.115741
Seabrook, L., Mcalpine, C. A., and Bowen, M. E. (2011). Restore, repair or reinvent: options for sustainable landscapes in a changing climate. Landscape Urban Plan. 100, 407–410. doi: 10.1016/j.landurbplan.2011.02.015
Seddon, A. W., Macias-Fauria, M., Long, P. R., Benz, D., and Willis, K. J. (2016). Sensitivity of global terrestrial ecosystems to climate variability. Nature 531, 229–232. doi: 10.1038/nature16986
Song, L., Li, Y., Ren, Y., Wu, X., Guo, B., Tang, X., et al. (2019). Divergent vegetation responses to extreme spring and summer droughts in southwestern China. Agric. Forest Meteorol. 279:107703. doi: 10.1016/j.agrformet.2019.107703
Sweeting, M. M. (2012). Karst in China: Its Geomorphology and Environment, vol. 15 Springer Berlin, Heidelberg: Springer Science & Business Media.
Tang, Z., Xu, W., Zhou, G., Bai, Y., Li, J., Tang, X., et al. (2018). Patterns of plant carbon, nitrogen, and phosphorus concentration in relation to productivity in China’s terrestrial ecosystems. Proc. Natl. Acad. Sci. U. S. A. 115, 4033–4038. doi: 10.1073/pnas.1700295114
Tang, X., Zhao, X., Bai, Y., Tang, Z., Wang, W., Zhao, Y., et al. (2018). Carbon pools in China’s terrestrial ecosystems: new estimates based on an intensive field survey. Proc. Natl. Acad. Sci. U. S. A. 115, 4021–4026. doi: 10.1073/pnas.1700291115
Tian, H., Melillo, J., Lu, C., Kicklighter, D., Liu, M., Ren, W., et al. (2011). China’s terrestrial carbon balance: contributions from multiple global change factors. Global Biogeochem. Cycles 25:1. doi: 10.1029/2010gb003838
Tong, X., Brandt, M., Yue, Y., Ciais, P., Rudbeck, J. M., Penuelas, J., et al. (2020). Forest management in southern China generates short term extensive carbon sequestration. Nat. Commun. 11:129. doi: 10.1038/s41467-019-13798-8
Tong, X., Brandt, M., Yue, Y., Horion, S., Wang, K., Keersmaecker, W. D., et al. (2018). Increased vegetation growth and carbon stock in China karst via ecological engineering. Nat. Sustain. 1, 44–50. doi: 10.1038/s41893-017-0004-x
Tong, X., Wang, K., Yue, Y., Brandt, M., Liu, B., Zhang, C., et al. (2017). Quantifying the effectiveness of ecological restoration projects on long-term vegetation dynamics in the karst regions of Southwest China. Int J Appl Earth Obs. 54, 105–113. doi: 10.1016/j.jag.2016.09.013
Vicente-Serrano, S. (2006). Differences in spatial patterns of drought on different time scales: an analysis of the Iberian Peninsula. Water Resour. Manag. 20, 37–60. doi: 10.1007/s11269-006-2974-8
Vicente-Serrano, S. M., Julio Camarero, J., and Azorin-Molina, C. (2014). Diverse responses of forest growth to drought time-scales in the northern hemisphere. Glob. Ecol. Biogeogr. 23, 1019–1030. doi: 10.1111/geb.12183
Wang, S., Liu, Q., and Zhang, D. (2004). Karst rocky desertification in southwestern China: geomorphology, landuse, impact and rehabilitation. Land Degrad. Dev. 15, 115–121. doi: 10.1002/ldr.592
Wang, X., Piao, S., Ciais, P., Friedlingstein, P., Myneni, R. B., Cox, P., et al. (2014). A two-fold increase of carbon cycle sensitivity to tropical temperature variations. Nature 506, 212–215. doi: 10.1038/nature12915
Wang, J., Wang, J., Ye, H., Liu, Y., and He, H. (2017). An interpolated temperature and precipitation dataset at 1-km grid resolution in China (2000-2012). China Sci. Data. 2, 73–80. doi: 10.11922/csdata.170.2016.0112
Wang, S., Zhang, X., and Bai, X. (2015). An outline of karst geomorphology zoning in the karst areas of southern China. Mt. Res. Dev. 33, 641–648.
Wu, B., Yuan, Q., Yan, C., Wang, Z., Yu, X., Li, A., et al. (2014). Land cover changes of China from 2000 to 2010. Quat Sci 34, 723–731.
Xiao, J., Zhuang, Q., Baldocchi, D. D., Law, B. E., Richardson, A. D., Chen, J., et al. (2018). Estimation of net ecosystem carbon exchange for the conterminous United States by combining MODIS and Ameri flux data. Agric. For. Meteorol. 148, 1827–1847. doi: 10.1016/j.agrformet.2008.06.015
Xu, W., Xiao, Y., Zhang, J., Yang, W., Zhang, L., Hull, V., et al. (2017). Strengthening protected areas for biodiversity and ecosystem services in China. Proc. Natl. Acad. Sci. U. S. A. 114, 1601–1606. doi: 10.1073/pnas.1620503114
Xu, E., and Zhang, H. (2014). Characterization and interaction of driving factors in karst rocky desertification: a case study from Changshun, China. Solid Earth. 5, 1329–1340. doi: 10.5194/se-5-1329-2014
Xue, W., Zhang, J., Ji, D., Che, Y., Lu, T., Deng, X., et al. (2021). Aerosol-induced direct radiative forcing effects on terrestrial ecosystem carbon fluxes over China. Environ. Res. 200:111464. doi: 10.1016/j.envres.2021.111464
Yao, Y., Wang, X., Li, Y., Wang, T., Shen, M., Du, M., et al. (2018). Spatiotemporal pattern of gross primary productivity and its covariation with climate in China over the last thirty years. Glob. Chang. Biol. 24, 184–196. doi: 10.1111/gcb.13830
Yu, Z., Ciais, P., Piao, S., Houghton, R. A., Lu, C., Tian, H., et al. (2022). Forest expansion dominates China’s land carbon sink since 1980. Nat. Commun. 13:5374. doi: 10.1038/s41467-022-32961-2
Yu, G., Jia, Y., He, N., Zhu, J., Chen, Z., Wang, Q., et al. (2019). Stabilization of atmospheric nitrogen deposition in China over the past decade. Nat. Geosci. 12, 424–429. doi: 10.1038/s41561-019-0352-4
Yuan, D. (1997). Rock desertification in the subtropical karst of South China. Z. Geomorphol. 108, 81–90.
Yuan, D., Drogue, C., Dai, A., Lao, W., Cai, W., Bidaux, P., et al. (1990). Hydrology of the karst aquifer at the experimental site of Guilin in southern China. J. Hydrol. 115, 285–296. doi: 10.1016/0022-1694(90)90210-O
Zhang, X., Brandt, M., Yue, Y., Tong, X., Wang, K. L., and Fensholt, R. (2022). The carbon sink potential of southern China after two decades of afforestation. Earth’s Future 10:e2022EF002674. doi: 10.1029/2022EF002674
Zhang, L., Mao, J., Shi, X., Ricciuto, D., He, H., Thornton, P., et al. (2016). Evaluation of the community land model simulated carbon and water fluxes against observations over China FLUX sites. Agric. For. Meteorol. 226-227, 174–185. doi: 10.1016/j.agrformet.2016.05.018
Zhang, J. L., Poorter, L., and Cao, K. F. (2012). Productive leaf functional traits of Chinese savanna species. Plant Ecol. 213, 1449–1460. doi: 10.1007/s11258-012-0103-8
Zhang, C., Qi, X., Wang, K., Zhang, M., and Yue, Y. (2017). The application of geospatial techniques in monitoring karst vegetation recovery in Southwest China. Prog. Phys. Geogr. 41, 450–477. doi: 10.1177/0309133317714246
Zhang, M., Wang, K., Liu, H., Wang, J., Zhang, C., Yue, Y., et al. (2016). Spatio-temporal variation and impact factors for vegetation carbon sequestration and oxygen production based on rocky desertification control in the karst region of Southwest China. Remote Sens. 8:102. doi: 10.3390/rs8020102
Zhang, M., Wang, K., Liu, H., Zhang, C., Wang, J., Yue, Y., et al. (2015). How ecological restoration alters ecosystem services: an analysis of vegetation carbon sequestration in the karst area of Northwest Guangxi, China. Environ Earth Sci. 74, 5307–5317. doi: 10.1007/s12665-015-4542-0
Zhang, M., Zhang, L., He, H., Ren, X., Lv, Y., and Chang, Q. (2022). Improvement of ecosystem quality in National key Ecological Function Zones in China during 2000–2015. J. Environ. Manag. 324:116406. doi: 10.1016/j.jenvman.2022.116406
Zhao, M., Zhang, J., Velicogna, I., Liang, C., and Li, Z. (2021). Ecological restoration impact on total terrestrial water storage. Nat. Sustain. 4, 56–62. doi: 10.1038/s41893-020-00600-7
Keywords: net ecosystem productivity, temporal variation, environmental factors, the CLM4.5 model, the ecological restoration projects
Citation: Lv Y, Zhang L, Li P, He H, Ren X and Zhang M (2023) Ecological restoration projects enhanced terrestrial carbon sequestration in the karst region of Southwest China. Front. Ecol. Evol. 11:1179608. doi: 10.3389/fevo.2023.1179608
Edited by:
Wei Zhao, Institute of Geographic Sciences and Natural Resources Research, Chinese Academy of Sciences (CAS), ChinaReviewed by:
Yuemin Yue, Institute of Subtropical Agriculture, Chinese Academy of Sciences (CAS), ChinaChong Jiang, Guangzhou Institute of Geography, China
Copyright © 2023 Lv, Zhang, Li, He, Ren and Zhang. This is an open-access article distributed under the terms of the Creative Commons Attribution License (CC BY). The use, distribution or reproduction in other forums is permitted, provided the original author(s) and the copyright owner(s) are credited and that the original publication in this journal is cited, in accordance with accepted academic practice. No use, distribution or reproduction is permitted which does not comply with these terms.
*Correspondence: Li Zhang, bGkuemhhbmdAaWdzbnJyLmFjLmNu