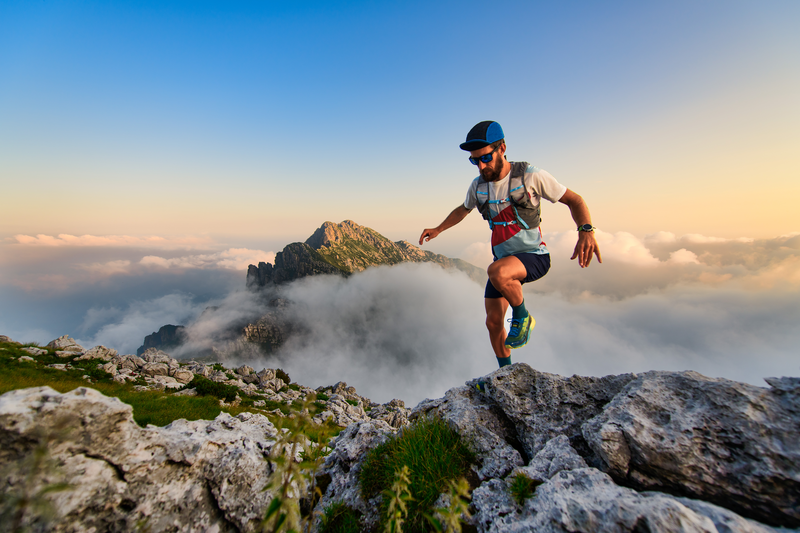
94% of researchers rate our articles as excellent or good
Learn more about the work of our research integrity team to safeguard the quality of each article we publish.
Find out more
ORIGINAL RESEARCH article
Front. Ecol. Evol. , 02 June 2023
Sec. Conservation and Restoration Ecology
Volume 11 - 2023 | https://doi.org/10.3389/fevo.2023.1177827
Nitrogen (N) is frequently a limiting element for primary productivity in karst ecosystems due to its low availability. N mineralization drives N availability and is important for improving N use efficiency in soils. While the use of biochar in agricultural and forest ecosystems has attracted great attention recently, little has been reported on the seasonal dynamics of soil N transformation in karst and its response to biochar application. Therefore, we conducted a pot experiment over 1 year using two types of biochar (rice husk biochar and wood biochar) as soil amendments. The results demonstrated that in the karst mountain region, the soil had the highest content of mineral N (19.41 mg/kg) during summer and the lowest content of mineral nitrogen (3.74 mg/kg) during winter. There was a significant positive correlation (p < 0.01) between soil mineral N content and temperature, and between soil temperature and urease activity. Thus, the higher temperatures during summer enhance soil urease activity, making it easier for soil N mineralization to occur, and increasing soil mineral N content. The soil mineral N content of the CK treatment was 2.61–209.42% higher than the other treatments, indicating that the biochar application reduced the soil mineral N content, which was supported by the negative net N mineralization. This may be due to biochar facilitating the adsorption and immobilization of nitrogen. The soil mineral N content of rice husk biochar was significantly higher than that of woody biochar by 109.55%, indicating that woody biochar would adsorb more N or more readily stimulate N immobilization than rice husk biochar. Furthermore, soil N immobilization (negative net N mineralization) after biochar application varied according to the season. The higher temperatures during summer promoted soil N immobilization more with biochar application. These results are crucial to understanding soil N transformation in karst mountains and guiding effective soil management.
Karst is a distinct type of topography, widely present across the globe, occupying approximately 12% of the land surface excluding the Antarctica, Greenland, and Iceland (Ford and Williams, 2007). Among them, southwest china boasts the largest and most extensive karst region, covering roughly 5.4 million square kilometers (Yuan et al., 1994). Soil characteristics in this area are uniquely defined by high soil exposure and alkali calcareous nature (Su and Li, 2003; Wan et al., 2009; Chen et al., 2010). Nitrogen (N) plays a critical role in primary productivity in karst ecosystems as soil N exiguity limits it (Niinemets and Kull, 2005). Simultaneously, huge N reserves can be stabilized in karst soils by binding to calcium minerals (Clarholm and Skyllberg, 2013). Conversely, highly developed epikarst systems exacerbate the loss of newly solubilized N (Zhang et al., 2011).
The availability of a large fraction of organic N in soil for plants is conditional on its mineralization to mineral N (Ashton et al., 2010). Soil N mineralization plays a significant role in regulating soil N availability and ecosystem productivity (Keuper et al., 2017). However, mineralization releases reactive N, such as NH3, NH4+-N, and NO3–-N, which jeopardizes soil N losses, making it a vital focus of research (Galloway et al., 2008). Recently, the application of biochar in farmland and forest ecosystems has attracted widespread attention (Song et al., 2014; Pereira et al., 2015; Franco et al., 2022), and is considered a feasible solution for reducing soil N loss and improving N use efficiency (Lehmann et al., 2011; Liu et al., 2019). The application of biochar significantly changed soil physicochemical properties that, in turn, affects soil N dynamics (Song et al., 2014). Fatima et al. (2021) observed a reduction in mineral N in soil solely treated with maize cob-biochar. Hale et al. (2013) also reported similar results. Bargmann et al. (2014) found that the biochar application reduced soil mineral N, and the degree of its reduction varied depending on the feedstock. The mechanisms underlying the effect of biochar application on soil mineral N include chemisorption (rich in functional groups), physical adsorption (larger surface area), and biological factors (altered soil microbial activity) (Joseph et al., 2010; Nguyen et al., 2017). Several studies indicate that soil N mineralization responds differently to various biochar feedstock resulting from differences in biochar functional groups (Harvey et al., 2012) and surface area (Bargmann et al., 2014). However, reports on the consequences of biochar feedstock on soil mineral N pool and N mineralization in karst areas remain scarce in the literature.
Several studies have shown a seasonal succession theory of soil N transformation (Schmidt et al., 2007; Zhang et al., 2008; Kaiser et al., 2011; Hou et al., 2020). This theory proposes that during autumn and winter soil inorganic N is immobilized through microbial uptake and is subsequently released through mineralization during spring and summer. Kaiser et al. (2011) investigated this theory by monitoring soil N dynamics over 2 years in a temperate forest and identified two phases of soil N dynamics, namely the N mineralization phase in summer and the N immobilization phase in winter. Wang et al. (2010) examined the seasonal fluctuations of soil N mineralization in subtropical forests in China and identified soil moisture as a crucial factor driving this process. These findings suggest that soil N transformation is strongly influenced by season, and it remains unclear if the effects of biochar application on soil N mineralization differ during different successional stages.
Here, we conducted a 1 year pot experiment using calcareous soil sourced from typical karst areas in china as the substrates. Two types of biochar, rice husk biochar and woody biochar, were used as additive materials. Our objectives were 2-fold: (1) to examine the seasonal succession pattern of soil N transformation in karst regions and identify key environmental factors that driving the succession, (2) to evaluate the impact of the biochar feedstock and application rate on soil N transformation across different seasons.
The biochar used in the experiments was obtained from Beierfu Biotechnology Co. Ltd., Anhui, China. It was derived from rice husk (R) and forest logging residues (W) through pyrolysis at a temperature of 500°C for 40 min. The soil used in the research was collected from the top 20 cm soil layer of a slope in Puding County, Guizhou province, China (E 105°32’50”, N 26°27’36”). This region exhibits a typical karst topography and has a humid monsoon climate characteristic of northern subtropics, with an annual mean temperature, relative humidity, and rainfall of 15.1°C, 79%, and 1396.9 mm, respectively. The soil has been classed as a sandy loam calcareous soil (Rendolls) (Ditzler, 2017). Table 1 presents the primary properties of both the biochar and the soil.
The biochar used in the pot experiment included woody biochar and rice husk biochar, and three application rates (4, 8, and 12%) were set for each biochar. In addition, the pot without biochar was used as a control treatment (CK), for a total of seven treatments. Each treatment was replicated three times, resulting a total of 21 pots. The soil was screened through a 2 mm sieve and then added to a plastic pot (height of 24 cm and inside diameter of 30 cm), with 10 kg of soil per pot. Prior to being poured into the pots, the biochar was thoroughly mixed with the soil. All pots are randomly placed in the nursery of Xiashu Forest Farm at Nanjing Forestry University in Zhenjiang, China. One annual acacia (Robinia pseudoacacia. L) seedling, with a height of approximately 1 m (±0.1) and a DBH (diameter at breast height) of 1 cm (±0.1), was planted in each pot. All seedlings were propagated asexually from the roots of the same plant. Regular watering, and disease and pest management were supported during the pot trial.
The experiment commenced in March of 2021, and soil samples were collected from the pots on a monthly basis between September 2021 and August 2022. We collected three soil subsamples from each pot through a soil drill and combined them into one sample. Thereafter, the soil samples obtained were divided into two portions, one for determination of soil water content and the other for evaluation of urease activity and mineral N content.
Soil temperature (ST) was determined using a portable thermometer and soil water content (SWC) was assessed by the change in moisture before and after drying at 105°C. NH4+-N and NO3–-N were extracted from the soil with 2 M KCl solution and analyzed using a UV spectrophotometer (Shimadzu UVmini-1240, Agilent Technologies, Kyoto, Japan). Additionally, soil urease activity (SUA) was determined using indophenol blue colorimetry following the method described by Hu et al. (2014).
The net ammonification (NA), net nitrification (NN), and net nitrogen mineralization (NNM) of soil was calculated as follows (Fatima et al., 2021):
Where, NH4+-N[Biochar] and NH4+-N[Control] represent the soil NH4+-N content (mg/kg) with and without biochar application, respectively. NO3–-N[Biochar] and NO3–-N[Control] represent the soil NO3–-N content (mg/kg) with and without biochar application, respectively. Mineral N[Biochar] and Mineral N[Control] represent the soil mineral N content (mg/kg) with and without biochar application, respectively.
Negative NNM was considered as N immobilization or N retention.
Repeated measures ANOVA was used to verify the effects of biochar application on ST, SWC, SUA, NH4+-N, NO3–-N, mineral nitrogen, NA, NN, and NNM. A post-hoc test (LSD method) was performed to compare the mean values of different treatments. Furthermore, Pearson’s correlation coefficients were computed to investigate the relationships between soil properties (i.e., ST, SWC, and SUA) and mineral N content (i.e., NH4+-N and NO3–-N) as well as net N transformation (i.e., NA, NN, and NNM). Lastly, multiple regression analysis was used to determine the relative explanatory power of soil properties on the seasonal dynamics of the inorganic N pool and N transformation. All statistical analyses were done using SPSS v20.0 (IBM, Armonk, NY, USA), and data were visualized using GraphPad Prism v9.0 (GraphPad Software, San Diego, CA, USA).
The study examined the temporal dynamics of soil temperature, water content, and urease activity during the experiment, as presented in Figure 1. The effects of different treatments on these variables were evaluated using statistical tests. Results indicated that soil temperature, water content, and urease activity were significantly influenced by time (p < 0.001). Specifically, soil temperature, water content, and urease activity decreased steadily from autumn to winter and then increased gradually from winter to summer. The values of soil temperature, water content, and urease activity were 5.47°C, 19.03%, and 92.38 μg/g/d, respectively, in winter, and 28.82°C, 28.55%, and 187.09 μg/g/d in summer. No significant differences in soil temperature were observed between treatments (p > 0.05) (Figure 2A), indicating that biochar application did not affect soil temperature. Conversely, soil water content varied significantly across treatments (p < 0.01). The rice husk biochar treatment showed the highest soil water content (28.64%), while the CK and woody biochar treatments had 13.66 and 26.38%, respectively (Figure 2B). These results suggest that changes in soil water content after biochar application were influenced by the feedstock. However, there were no major differences in soil urease activity observed between the treatments (Figure 2C), suggesting that biochar application may not have a significant impact on soil urease activity.
Figure 1. Soil temperature (A), water content (B), and urease activity (C) at different treatments (Biochar) and at different months (Time). The F- and p-values in the figure are based on repeated measure variance analysis. In the legend, CK is not applying biochar. R4, R8, and R12 were applied rice husk biochar with 4, 8 and 12% concentrations, respectively. W4, W8, and W12 were applied woody biochar with 4, 8, and 12% concentrations, respectively.
Figure 2. Marginal estimated means for soil temperature (A), water content (B), and urease activity (C) were based on repeated measure variance analysis. Different lowercase letters indicated significant differences between treatments (p < 0.05). SUA, soil urease activity.
The soil NH4+-N and NO3–-N contents in different treatments and seasons are displayed in Figure 3. The soil NH4+-N content were highest in the summer season, reaching 3.3 mg/kg, while the content decreased in the other seasons. On the other hand, soil NO3–-N content decreased continuously from autumn to winter but gradually increased from winter to summer. In the winter and summer seasons, soil NO3–-N content values were 2.35 and 16.11 mg/kg, respectively. The soil NH4+-N content of the CK group was significantly higher than that of the other treatment groups (Figure 4A). This implies that the biochar application effectively reduced the soil NH4+-N content, which might be attributed to NH4+-N adsorption by biochar. Although there was no significant difference in NH4+-N content among different feedstock, there was a significant difference among different application amounts. Conversely, the soil NO3–-N content was significantly higher in the CK treatment than in the other treatments (except R8), indicating that the biochar application reduced the soil NO3–-N content, with a varying degree of reduction due to different feedstock. Woody biochar may have a stronger adsorption effect on NO3–-N than rice husk biochar, as indicated by the soil NO3–-N content of rice husk biochar being 159.4% lower than that of woody biochar at 9.34 mg/kg (Figure 4B). The pattern of soil mineral N content in response to the season and treatment was consistent with that of soil NO3–-N.
Figure 3. Soil NH4+-N (A), NO3–-N (B), and mineral N (C) at different treatments (Biochar) and at different months (Time). The F- and p-values in the figure are based on repeated measure variance analysis. In the legend, CK is not applying biochar. R4, R8, and R12 were applied rice husk biochar with 4, 8 and 12% concentrations, respectively. W4, W8, and W12 were applied woody biochar with 4, 8, and 12% concentrations, respectively.
Figure 4. Marginal estimated means for soil NH4+-N (A), NO3–-N (B), and mineral N (C) were based on repeated measure variance analysis. Different lowercase letters indicated significant differences between treatments (p < 0.05).
We observed that soil net ammonification, net nitrification, and NNM were negative after adding biochar to the soil (Figure 5). This indicates that the application of biochar reduced the soil mineral N content, and N immobilization was the main process controlling soil N transformation. Soil net ammonification, net nitrification, and NNM exhibited seasonal variation with an increase from autumn to winter followed by a gradual decrease from winter to summer. During the summer, soil net ammonification (–1.57 mg/kg), net nitrification (–9.19 mg/kg), and NNM (–10.76 mg/kg) were at their lowest levels, indicating that biochar application in summer could improve soil N immobilization. Additionally, woody biochar had a more effective immobilization (or absorption) effect on soil mineral N compared to rice husk biochar, as evidenced by the soil NNM values of –8.23 and –2.38 mg/kg, respectively (Figure 6).
Figure 5. Soil net ammonification (A), net nitrification (B), and net nitrogen mineralization (C) at different treatments (Biochar) and at different months (Time). NA, net ammonification; NN, net nitrification; NNM, net nitrogen mineralization. The F- and p-values in the figure are based on repeated measure variance analysis. In the legend, R4, R8, and R12 were applied rice husk biochar with 4, 8, and 12% concentrations, respectively. W4, W8, and W12 were applied woody biochar with 4, 8, and 12% concentrations, respectively.
Figure 6. Marginal estimated means for soil net ammonification (A), net nitrification (B), and net nitrogen mineralization (C) were based on repeated measure variance analysis. NA, net ammonification; NN, net nitrification; NNM, net nitrogen mineralization. Different lowercase letters indicated significant differences between treatments (p < 0.05).
Table 2 reveals a significant positive correlation (p < 0.01) between soil mineral N content and the levels of both soil temperature and water content, suggesting that moisture and temperature are intricately linked to soil mineral N content. The correlation can be explained by the fact that temperature and moisture directly impact the activity of microorganisms responsible for N transformation. The results of multiple regression analysis displayed that only soil temperature and urease activity had significant linear relationships with the soil mineral N content (Table 3). Thus, temperature plays a crucial role in the seasonal variability of soil mineral N pools. Referring to Table 4, it is evident that soil NNM negatively correlated with soil temperature and urease activity while no significant correlation was observed with soil water content. Table 5 demonstrates that multiple regression analysis revealed a considerable negative linear relationship (p < 0.01) between soil NNM and both temperature and urease activity, with a relatively smaller standardized coefficient for temperature than for urease activity. Findings suggest that temperature is a critical driver of the seasonal dynamics of soil N immobilization after biochar application.
Table 2. Coefficient (r) and p-value of pearson’s correlation between soil inorganic N content (NH4+-N, NO3–-N and mineral N) and soil temperature, water content and soil urease activity (SUA).
Table 3. Multiple linear regression analysis of soil NH4+-N, NO3–-N, and mineral N using soil temperature (ST), soil water content (SWC), and soil urease activity (SUA) as independent variables.
Table 4. Coefficient (r) and p-value of Pearson’s correlation of soil net ammonification (NA), net nitrification (NN), and net nitrogen mineralization (NNM) with soil temperature, water content, and urease activity.
Table 5. Multiple linear regression analysis of oil net ammonification (NA), net nitrification (NN), and net nitrogen mineralization (NNM) using soil temperature (ST), soil water content (SWC), and soil urease activity (SUA) as independent variables.
The season has a significant impact on soil N transformation in karst by altering the inorganic N pool. Specifically, the soil mineral N content decreases significantly from autumn to winter, with the lowest concentration occurring during winter. During this period, N immobilization is the main process that regulates soil N transformation, which supports earlier findings (Schmidt et al., 2007; Kaiser et al., 2011) that demonstrate plant growth and N uptake cease gradually in autumn and winter, while soil N transformation displays an N immobilization pattern. Kaiser et al. (2010) suggest that in autumn and winter, plants return some nutrients to the soil from litter decomposition. At this time, microbial immobilization of mineral N is critical for soil N transformation, since it minimizes soil N losses due to leaching or volatilization, and most of the immobilized N will be available for plant uptake through mineralization in the subsequent spring (Lipson et al., 1999). Conversely, soil mineral N content increases significantly from spring to summer, reaching the highest amount during summer when N mineralization dominates soil N transformation. During this period, which is the growing season for plants, strong net N mineralization and low microbial N uptake characterize soil N transformation, facilitating increased soil N activity and addressing the high N demand required for rapid plant growth (Zhu and Carreiro, 2004; Zhang et al., 2008).
Soil N transformation exhibits seasonal dynamics that are typically attributed to temperature and moisture availability regulation (Piccolo et al., 1994; Smith et al., 1998). Pérez et al. (2004) reported that low temperatures and humidity levels reduced soil NNM as well as net nitrification. Similarly, Yan et al. (2009) revealed that the seasonal dynamics of soil NNM and net nitrification in subtropical forests were consistent with the seasonal changes in soil temperature and moisture. Their study demonstrated that soil temperature, water content, and urease activity were all significantly and positively correlated with soil mineral N content, while temperature had the most substantial impact. This agrees with prior findings (Dalias et al., 2002; Yan et al., 2008) that temperature plays a pivotal role in regulating seasonal dynamics of soil N transformation, as soil temperature directly impacts soil microbial activity (Nicolardot et al., 2001; Schimel et al., 2004). Additionally, soil urease activity is a crucial factor that influences soil mineral N content (Cui et al., 2022). Cong and Eriksen (2018) proposed that urease is directly implicated in the conversion of organic N to mineral N, making it a pivotal participant in soil N mineralization. During summer, higher temperatures boosted urease activity, which led to increased soil mineralization and higher soil mineral N content.
The biochar application was found to decrease soil mineral N content by 2.6–209.41%, compared to CK (Figure 4C), consistent with the findings of Fatima et al. (2021) who also observed a negative response of soil mineral N content to biochar addition alone. This suggests that soil N availability is reduced by biochar application, leading to two main effects: (1) decreased soil mineral N leaching and (2) reduced soil mineral N uptake by plants. Nguyen et al. (2017) integrated analyzed 56 publications and found an overall 10% reduction in soil mineral N content in biochar-treated soils compared to those treated with CK due to the adsorption and immobilization of soil mineral N by biochar. Biochar has a large surface area and various functional groups that facilitate the adsorption of mineral nitrogen (Saleh et al., 2012; Clough et al., 2013; Lehmann and Joseph, 2015; Zhang et al., 2020; He et al., 2022) and promote the immobilization of soil nitrogen (Ducey et al., 2013). Microorganisms primarily drive soil N immobilization, leading to inefficient mineral N uptake by plants (Hart et al., 1994). Factors that influence the soil N immobilization include biochar feedstock and biochar C/N.
The influence of biochar application on the sorption or immobilization of soil mineral nitrogen was more significantly determined by feedstock than application rate. Woody biochar exhibited a higher sorption or immobilization capacity for inorganic N than rice husk biochar. Grass biochar, according to Harvey et al. (2012), demonstrated a higher sorption capacity than woody biochar due to higher concentrations of functional groups. However, Nguyen et al. (2017) observed that woody biochar was less effective in reducing soil mineral N than other types of biochar, which contradicts our results and may be attributed to differences in soil type and environmental conditions (Clough et al., 2013). Biochar’s sorption capacity is shown to be positively correlated with its internal surface area (Zhang et al., 2012), so woody biochar, with a larger surface area, has a greater physical sorption capacity than rice husk biochar. Another plausible explanation could be that woody biochar readily activates N immobilization rather than N mineralization compared to rice husk biochar (Lehmann et al., 2006). Soil N was immobilized after woody biochar application at all application rates, whereas in soils where 4 and 12% rice husk biochar were applied, suggesting that rice husk biochar application may encourage soil N mineralization.
The biochar application can either stimulate soil N mineralization (Singh et al., 2012; Naisse et al., 2015) or N immobilization (Zavalloni et al., 2011; Tsai and Chang, 2020; Fatima et al., 2021). Chan and Xu (2012) proposed that the C/N ratio of biochar determines whether soil N mineralization or N immobilization will occur after its application. Kuzyakov et al. (2000) posited that a threshold C/N ratio exists between 20 and 32, and a ratio exceeding this threshold results in N immobilization, while a ratio below the limit causes N mineralization. In this study, rice husk biochar had a C/N ratio of 31.28, while woody biochar had a ratio of 37.97. Consequently, the use of rice husk biochar stimulated both soil N immobilization and N mineralization, whereas the regulation of woody biochar only stimulated soil N immobilization, which agrees with previous studies (Lehmann et al., 2006) reporting that woody biochar primarily encourages soil N immobilization. Furthermore, rice husk biochar may stimulate soil N mineralization more than woody biochar due to lower C/N and more unstable organic compounds, which determine the rate of soil organic matter mineralization (Singh et al., 2012). Of note, the response of soil NNM (net N mineralization) varied following the use of woody biochar and rice husk biochar (Figure 5C), indicating that the effect of biochar on soil N transformation is complex, influenced by a combination of factors, such as N chemisorption, N physisorption, N immobilization, and N mineralization.
The immobilization of soil nitrogen by biochar is affected by seasonal variations. Specifically, N immobilization was weaker in spring and winter, and stronger in autumn and summer. This pattern is similar to the seasonal dynamics of urease activity, suggesting that the interplay between biochar properties and environmental factors influences the effect of biochar on soil N transformation (Nguyen et al., 2017). In summer, when compared to winter, higher temperatures, greater microbial activity, and increased soil N mineralization and availability (Kaiser et al., 2011) may be more conducive to the adsorption and immobilization of soil mineral N by biochar. The effects of biochar on soil N transformation include biological mechanisms (Zimmerman et al., 2011; Bruun et al., 2012; Ulyett et al., 2014), physical mechanisms (Bargmann et al., 2014), and chemical mechanisms (Lehmann and Joseph, 2015), which may be limited by environmental conditions (such as temperature, humidity), particularly in diverse regions. Therefore, we suggest that future studies should report basic environmental information to improve comparability across studies.
The results demonstrate that the soil mineral nitrogen (N) content in karst mountains reaches its peak during summer due to the strong soil NNM, while the lowest content is observed in winter because of the microbial uptake. Moreover, soil temperature is significantly and positively related to soil mineral N levels and acts as a crucial driver of seasonal fluctuations in soil mineral N pools. Specifically, higher temperatures in summer promote soil urease activity, benefiting soil mineralization and elevating the soil mineral N content. After the biochar application, the soil mineral N content decreases due to it was adsorbed and immobilized by biochar, which is in line with the negative NNM. The feedstock exerts a greater influence on soil mineral N than the application rate. Woody biochar has a stronger adsorption capacity for soil mineral N compared to rice husk biochar, and it more readily stimulates soil N immobilization rather than mineralization. Furthermore, the effect of biochar on soil NNM varies depending on the season. In the summer, higher temperatures enhance soil N immobilization with biochar applications. To conclude, soil mineral N pool alteration by biochar is intricate and is the product of a combination of influencing mechanisms, including chemisorption, physisorption, N mineralization, and N immobilization.
The original contributions presented in this study are included in this article/supplementary material, further inquiries can be directed to the corresponding author.
HW: conceptualization, methodology, data curation, visualization, writing—original draft, and writing—review and editing. JL: data curation and writing—review and editing. YW: supervision. JX: conceptualization, funding acquisition, and writing—review and editing. All authors contributed to the article and approved the submitted version.
This work was supported by the Major State Basic Research Development Program of China (grant number: 2016YFC0502605) and the Postgraduate Research and Practice Innovation Program of Jiangsu Province (grant number: KYCX21_0866).
The authors declare that the research was conducted in the absence of any commercial or financial relationships that could be construed as a potential conflict of interest.
All claims expressed in this article are solely those of the authors and do not necessarily represent those of their affiliated organizations, or those of the publisher, the editors and the reviewers. Any product that may be evaluated in this article, or claim that may be made by its manufacturer, is not guaranteed or endorsed by the publisher.
Ashton, I. W., Miller, A. E., Bowman, W. D., and Suding, K. N. (2010). Niche complementarity due to plasticity in resource use: plant partitioning of chemical N forms. Ecology 91, 3252–3260. doi: 10.1890/09-1849.1
Bargmann, I., Martens, R., Rillig, M. C., Kruse, A., and Kücke, M. (2014). Hydrochar amendment promotes microbial immobilization of mineral nitrogen. J. Plant Nutr. Soil Sci. 177, 59–67. doi: 10.1002/jpln.201300154
Bruun, E. W., Ambus, P., Egsgaard, H., and Hauggaard-Nielsen, H. (2012). Effects of slow and fast pyrolysis biochar on soil C and N turnover dynamics. Soil Biol. Biochem. 46, 73–79. doi: 10.1016/j.soilbio.2011.11.019
Chan, K. Y., and Xu, Z. (2012). “Biochar: nutrient properties and their enhancement,” in Biochar for Environmental Management, eds J. Lehmann and S. D. Joseph (London: Routledge), 99–116.
Chen, H. S., Zhang, W., Wang, K. L., and Fu, W. (2010). Soil moisture dynamics under different land uses on karst hillslope in northwest Guangxi, China. Environ. Earth Sci. 61, 1105–1111. doi: 10.1007/s12665-009-0428-3
Clarholm, M., and Skyllberg, U. (2013). Translocation of metals by trees and fungi regulates pH, soil organic matter turnover and nitrogen availability in acidic forest soils. Soil Biol. Biochem. 63, 142–153. doi: 10.1016/j.soilbio.2013.03.019
Clough, T., Condron, L., Kammann, C., and Müller, C. (2013). A review of biochar and soil nitrogen dynamics. Agronomy 3, 275–293. doi: 10.3390/agronomy3020275
Cong, W.-F., and Eriksen, J. (2018). Forbs differentially affect soil microbial community composition and functions in unfertilized ryegrass-red clover leys. Soil Biol. Biochem. 121, 87–94. doi: 10.1016/j.soilbio.2018.03.008
Cui, Q., Xia, J. B., Peng, L., Zhao, X. M., and Qu, F. Z. (2022). Positive effects on alfalfa productivity and soil nutrient status in coastal wetlands driven by biochar and microorganisms mixtures. Front. Ecol. Evol. 9:798520. doi: 10.3389/fevo.2021.798520
Dalias, P., Anderson, J. M., Bottner, P., and Coûteaux, M. M. (2002). Temperature responses of net nitrogen mineralization and nitrification in conifer forest soils incubated under standard laboratory conditions. Soil Biol. Biochem. 34, 691–701. doi: 10.1016/S0038-0717(01)00234-6
Ditzler, C. A. (2017). “Soil properties and classification (soil taxonomy),” in The soils of the USA, eds. L. T. West, M. J. Singer, and A. E. Hartemink. (Cham: Springer International Publishing), 29–41. doi: 10.1007/978-3-319-41870-4_3
Ducey, T. F., Ippolito, J. A., Cantrell, K. B., Novak, J. M., and Lentz, R. D. (2013). Addition of activated switchgrass biochar to an aridic subsoil increases microbial nitrogen cycling gene abundances. Appl. Soil Ecol. 65, 65–72. doi: 10.1016/j.apsoil.2013.01.006
Fatima, S., Riaz, M., Al-Wabel, M. I., Arif, M. S., Yasmeen, T., Hussain, Q., et al. (2021). Higher biochar rate strongly reduced decomposition of soil organic matter to enhance C and N sequestration in nutrient-poor alkaline calcareous soil. J. Soils Sediments 21, 148–162. doi: 10.1007/s11368-020-02753-6
Ford, D., and Williams, P. D. (2007). Karst Hydrogeology and Geomorphology. London: John Wiley & Sons. doi: 10.1002/9781118684986
Franco, C. R., Page-Dumroese, D. S., and Archuleta, J. (2022). Forest management and biochar for continued ecosystem services. J. Soil Water Conservat. 77, 60A–64A. doi: 10.2489/jswc.2022.0603A
Galloway, J. N., Townsend, A. R., Erisman, J. W., Bekunda, M., Cai, Z., Freney, J. R., et al. (2008). Transformation of the nitrogen cycle: recent trends, questions, and potential solutions. Science 320, 889–892. doi: 10.1126/science.1136674
Hale, S. E., Alling, V., Martinsen, V., Mulder, J., Breedveld, G. D., and Cornelissen, G. (2013). The sorption and desorption of phosphate-P, ammonium-N and nitrate-N in cacao shell and corn cob biochars. Chemosphere 91, 1612–1619. doi: 10.1016/j.chemosphere.2012.12.057
Hart, S. C., Stark, J. M., Davidson, E. A., and Firestone, M. K. (1994). Nitrogen Mineralization, Immobilization, and Nitrification. London: Wiley Online Library.
Harvey, O. R., Herbert, B. E., Kuo, L. J., and Louchouarn, P. (2012). Generalized two-dimensional perturbation correlation infrared spectroscopy reveals mechanisms for the development of surface charge and recalcitrance in plant-derived biochars. Environ. Sci. Technol. 46, 10641–10650. doi: 10.1021/es302971d
He, Z. J., Cao, H. X., Liang, J. P., Hu, Q. Y., Zhang, Y., Nan, X. P., et al. (2022). Effects of biochar particle size on sorption and desorption behavior of NH4+-N. Industrial Crops Products 189:115837. doi: 10.1016/j.indcrop.2022.115837
Hou, R., Li, T., Fu, Q., Liu, D., Li, M., Zhou, Z., et al. (2020). The effect on soil nitrogen mineralization resulting from biochar and straw regulation in seasonally frozen agricultural ecosystem. J. Cleaner Product. 255:120302. doi: 10.1016/j.jclepro.2020.120302
Hu, B., Liang, D., Liu, J., Lei, L., and Yu, D. (2014). Transformation of heavy metal fractions on soil urease and nitrate reductase activities in copper and selenium co-contaminated soil. Ecotoxicol. Environ. Safety 110, 41–48. doi: 10.1016/j.ecoenv.2014.08.007
Joseph, S. D., Camps-Arbestain, M., Lin, Y., Munroe, P., Chia, C. H., Hook, J., et al. (2010). An investigation into the reactions of biochar in soil. Soil Res. 48, 501–515. doi: 10.1071/SR10009
Kaiser, C., Fuchslueger, L., Koranda, M., Gorfer, M., Stange, C. F., Kitzler, B., et al. (2011). Plants control the seasonal dynamics of microbial N cycling in a beech forest soil by belowground C allocation. Ecology 92, 1036–1051. doi: 10.1890/10-1011.1
Kaiser, C., Koranda, M., Kitzler, B., Fuchslueger, L., Schnecker, J., Schweiger, P., et al. (2010). Belowground carbon allocation by trees drives seasonal patterns of extracellular enzyme activities by altering microbial community composition in a beech forest soil. New Phytol. 187, 843–858. doi: 10.1111/j.1469-8137.2010.03321.x
Keuper, F., Dorrepaal, E., Van Bodegom, P. M., Van Logtestijn, R., Venhuizen, G., Van Hal, J., et al. (2017). Experimentally increased nutrient availability at the permafrost thaw front selectively enhances biomass production of deep-rooting subarctic peatland species. Global Change Biol. 23, 4257–4266. doi: 10.1111/gcb.13804
Kuzyakov, Y., Friedel, J., and Stahr, K. (2000). Review of mechanisms and quantification of priming effects. Soil Biol. Biochem. 32, 1485–1498. doi: 10.1016/S0038-0717(00)00084-5
Lehmann, J., Gaunt, J., and Rondon, M. (2006). Bio-char sequestration in terrestrial ecosystems–a review. Mitigation Adaptation Strategies Global Change 11, 403–427. doi: 10.1007/s11027-005-9006-5
Lehmann, J., and Joseph, S. (2015). “Biochar for environmental management: an introduction,” in Biochar for Environmental Management, eds J. Lehmann and S. Joseph (London: Science and Technology), 1–13. doi: 10.4324/9780203762264
Lehmann, J., Rillig, M. C., Thies, J., Masiello, C. A., Hockaday, W. C., and Crowley, D. (2011). Biochar effects on soil biota – a review. Soil Biol. Biochem. 43, 1812–1836. doi: 10.1016/j.soilbio.2011.04.022
Lipson, D. A., Schmidt, S. K., and Monson, R. K. (1999). Links between microbial population dynamics and nitrogen availability in an alpine ecosystem. Ecology 80, 1623–1631. doi: 10.1890/0012-9658(1999)080[1623:LBMPDA]2.0.CO;2
Liu, Q., Liu, B. J., Zhang, Y. H., Hu, T. L., Lin, Z. B., Liu, G., et al. (2019). Biochar application as a tool to decrease soil nitrogen losses (NH3 volatilization, N2O emissions, and N leaching) from croplands: options and mitigation strength in a global perspective. Global Change Biol. 25, 2077–2093. doi: 10.1111/gcb.14613
Naisse, C., Girardin, C., Davasse, B., Chabbi, A., and Rumpel, C. (2015). Effect of biochar addition on C mineralisation and soil organic matter priming in two subsoil horizons. J. Soils Sediments 15, 825–832. doi: 10.1007/s11368-014-1002-5
Nguyen, T. T. N., Xu, C.-Y., Tahmasbian, I., Che, R., Xu, Z., Zhou, X., et al. (2017). Effects of biochar on soil available inorganic nitrogen: a review and meta-analysis. Geoderma 288, 79–96. doi: 10.1016/j.geoderma.2016.11.004
Nicolardot, B., Recous, S., and Mary, B. (2001). Simulation of C and N mineralisation during crop residue decomposition: a simple dynamic model based on the C: N ratio of the residues. Plant Soil 228, 83–103. doi: 10.1023/A:1004813801728
Niinemets, Ü, and Kull, K. (2005). Co-limitation of plant primary productivity by nitrogen and phosphorus in a species-rich wooded meadow on calcareous soils. Acta Oecol. 28, 345–356. doi: 10.1016/j.actao.2005.06.003
Pereira, E. I. P., Suddick, E. C., Mansour, I., Mukome, F. N., Parikh, S. J., Scow, K., et al. (2015). Biochar alters nitrogen transformations but has minimal effects on nitrous oxide emissions in an organically managed lettuce mesocosm. Biol. Fertility Soils 51, 573–582. doi: 10.1007/s00374-015-1004-5
Pérez, C. A., Carmona, M. R., Aravena, J. C., and Armesto, J. J. (2004). Successional changes in soil nitrogen availability, non-symbiotic nitrogen fixation and carbon/nitrogen ratios in southern Chilean forest ecosystems. Oecologia 140, 617–625. doi: 10.1007/s00442-004-1627-y
Piccolo, M. C., Neill, C., and Cerri, C. C. (1994). Net nitrogen mineralization and net nitrification along a tropical forest-to-pasture chronosequence. Plant Soil 162, 61–70. doi: 10.1007/BF01416090
Saleh, M. E., Mahmoud, A. H., and Rashad, M. J. (2012). Peanut biochar as a stable adsorbent for removing NH4+-N from wastewater: a preliminary study. Adv. Environ. Biol. 6, 2170–2177.
Schimel, J. P., Bilbrough, C., and Welker, J. M. (2004). Increased snow depth affects microbial activity and nitrogen mineralization in two Arctic tundra communities. Soil Biol. Biochem. 36, 217–227. doi: 10.1016/j.soilbio.2003.09.008
Schmidt, S. K., Costello, E. K., Nemergut, D. R., Cleveland, C. C., Reed, S. C., Weintraub, M. N., et al. (2007). Biogeochemical consequences of rapid microbial turnover and seasonal succession in soil. Ecology 88, 1379–1385. doi: 10.1890/06-0164
Singh, B. P., Cowie, A. L., and Smernik, R. J. (2012). Biochar carbon stability in a clayey soil as a function of feedstock and pyrolysis temperature. Environ. Sci. Technol. 46, 11770–11778. doi: 10.1021/es302545b
Smith, C. K., Gholz, H. L., and De Assis Oliveira, F. (1998). Soil nitrogen dynamics and plant-induced soil changes under plantations and primary forest in lowland Amazonia. Brazil. Plant Soil 200, 193–204. doi: 10.1023/A:1004348116786
Song, Y. J., Zhang, X. L., Ma, B., Chang, S. X., and Gong, J. (2014). Biochar addition affected the dynamics of ammonia oxidizers and nitrification in microcosms of a coastal alkaline soil. Biol. Fert. Soils 50, 321–332. doi: 10.1007/s00374-013-0857-8
Su, Z. M., and Li, X. K. (2003). The types of natural vegetation in karst region of Guangxi and its classified system. Guangxi Zhiwu 23, 289–293.
Tsai, C.-C., and Chang, Y.-F. (2020). Nitrogen availability in biochar-amended soils with excessive compost application. Agronomy-Basel 10:444. doi: 10.3390/agronomy10030444
Ulyett, J., Sakrabani, R., Kibblewhite, M., and Hann, M. (2014). Impact of biochar addition on water retention, nitrification and carbon dioxide evolution from two sandy loam soils. Eur. J. Soil Sci. 65, 96–104. doi: 10.1111/ejss.12081
Wan, Y., Ju, X., Ingwersen, J., Schwarz, U., Stange, C. F., Zhang, F., et al. (2009). Gross nitrogen transformations and related nitrous oxide emissions in an intensively used calcareous soil. Soil Sci. Soc. Am. J. 73, 102–112. doi: 10.2136/sssaj2007.0419
Wang, F. M., Zhu, W. X., Xia, H. P., Fu, S. L., and Li, Z. A. (2010). Nitrogen mineralization and leaching in the early stages of a subtropical reforestation in Southern China. Restoration Ecol. 18, 313–322. doi: 10.1111/j.1526-100X.2009.00642.x
Yan, E. R., Wang, X. H., Guo, M., Zhong, Q., Zhou, W., and Li, Y. F. (2009). Temporal patterns of net soil N mineralization and nitrification through secondary succession in the subtropical forests of eastern China. Plant Soil 320, 181–194. doi: 10.1007/s11104-008-9883-y
Yan, E. R., Wang, X. H., Huang, J. J., Li, G. Y., and Zhou, W. (2008). Decline of soil nitrogen mineralization and nitrification during forest conversion of evergreen broad-leaved forest to plantations in the subtropical area of Eastern China. Biogeochemistry 89, 239–251. doi: 10.1007/s10533-008-9216-5
Yuan, D. X., Zhu, D. H., and Weng, J. T. (1994). Karstology of China. Beijing: GeologicalPublishing House.
Zavalloni, C., Alberti, G., Biasiol, S., Delle Vedove, G., Fornasier, F., Liu, J., et al. (2011). Microbial mineralization of biochar and wheat straw mixture in soil: a short-term study. Appl. Soil Ecol. 50, 45–51. doi: 10.1016/j.apsoil.2011.07.012
Zhang, A. F., Liu, Y. M., Pan, G. X., Hussain, Q., Li, L. Q., Zheng, J. W., et al. (2012). Effect of biochar amendment on maize yield and greenhouse gas emissions from a soil organic carbon poor calcareous loamy soil from Central China Plain. Plant Soil 351, 263–275. doi: 10.1007/s11104-011-0957-x
Zhang, M., Song, G., Gelardi, D. L., Huang, L., Khan, E., Masek, O., et al. (2020). Evaluating biochar and its modifications for the removal of ammonium, nitrate, and phosphate in water. Water Res. 186:116303. doi: 10.1016/j.watres.2020.116303
Zhang, X. B., Bai, X. Y., and He, X. B. (2011). Soil creeping in the weathering crust of carbonate rocks and underground soil losses in the karst mountain areas of southwest China. Carbonates Evaporites 26, 149–153. doi: 10.1007/s13146-011-0043-8
Zhang, X. L., Wang, Q. B., Li, L. H., and Han, X. G. (2008). Seasonal variations in nitrogen mineralization under three land use types in a grassland landscape. Acta Oecol. 34, 322–330. doi: 10.1016/j.actao.2008.06.004
Zhu, W. X., and Carreiro, M. M. (2004). Temporal and spatial variations in nitrogen transformations in deciduous forest ecosystems along an urban–rural gradient. Soil Biol. Biochem. 36, 267–278. doi: 10.1016/j.soilbio.2003.09.013
Keywords: karst mountains, biochar, calcareous soil, soil N transformation, seasonal dynamics
Citation: Wang H, Liu J, Wu Y and Xue J (2023) The effect on the seasonal dynamics of soil N transformation resulting from biochar application in karst mountains. Front. Ecol. Evol. 11:1177827. doi: 10.3389/fevo.2023.1177827
Received: 03 March 2023; Accepted: 17 May 2023;
Published: 02 June 2023.
Edited by:
Arnaldo Marín, University of Murcia, SpainReviewed by:
Bhupinder Pal Singh, University of New England, AustraliaCopyright © 2023 Wang, Liu, Wu and Xue. This is an open-access article distributed under the terms of the Creative Commons Attribution License (CC BY). The use, distribution or reproduction in other forums is permitted, provided the original author(s) and the copyright owner(s) are credited and that the original publication in this journal is cited, in accordance with accepted academic practice. No use, distribution or reproduction is permitted which does not comply with these terms.
*Correspondence: Jianhui Xue, amh4dWVAbmpmdS5lZHUuY24=
Disclaimer: All claims expressed in this article are solely those of the authors and do not necessarily represent those of their affiliated organizations, or those of the publisher, the editors and the reviewers. Any product that may be evaluated in this article or claim that may be made by its manufacturer is not guaranteed or endorsed by the publisher.
Research integrity at Frontiers
Learn more about the work of our research integrity team to safeguard the quality of each article we publish.