- 1Interdisciplinary Centre for Medical Research of Franceville (CIRMF), Franceville, Gabon
- 2Department of Anthropology, Durham University, Durham, United Kingdom
- 3Research Institute for Tropical Ecology (IRET/CENAREST), Libreville, Gabon
- 4MIVEGEC, University of Montpellier, CNRS, IRD, Montpellier, France
- 5Department of Parasitology, Health Science University (USS), Libreville, Gabon
Introduction: Despite all the efforts made to control or even eliminate malaria, the disease continues to claim the highest number of victims of vector-borne pathogens in the world and Sub-Saharan countries bear the heaviest burden. The lack of knowledge of the role of various protagonists involved in the transmission of this parasitic disease, such as mosquito vectors and the plasmodial species they transmit as well as the host species they infect in a locality, constitutes one of the main causes of the persistence of malaria. In Gabon, in several areas, entomological data on malaria transmission remain poorly known. Thus, this study aimed to determine the diversity of Anopheles involved in malaria transmission in different environments of the province of Nyanga in southwest Gabon.
Methods: For this, an entomological study was carried out in the four main localities of the province of Nyanga to provide answers to these shortcomings. Mosquitoes were collected over several nights using the human landing catch method. The identification of Anopheles and malaria parasites circulating in the different sites was achieved by combining morphological and molecular analysis tools.
Results: A total of five hundred and ninety-one (591) mosquitoes belonging to the Culicidae family were collected. From this collection of adult mosquitoes, nine species of Anopheles mosquitoes notably species of the Anopheles nili complex (53.46%) followed by those of Anopheles gambiae complex (22.01%), Anopheles funestus group (18.24%), Anopheles moucheti complex (5.66%) and Anopheles hancocki (0.63%). Approximately 18 percent of these Anopheles species were infected with Plasmodium spp. Anopheles funestus, known to be involved in malaria transmission to humans, and An. moucheti-like, recently discovered in Gabon, and whose status in Plasmodium transmission is not yet elucidated, were found to be infected with great ape Plasmodium.
Discussion: Our results raise the question of the potential switch of simian malaria parasites to humans. If these observations are confirmed in the future, and the infective capacity of the bridge vectors is demonstrated, this new situation could ultimately constitute an obstacle to progress in the fight against malaria.
Introduction
Malaria is the most important parasitic disease based on its impact on human populations. This disease is caused by parasites belonging to the Plasmodium genus and remains most pronounced in many parts of the world, in particular in Sub-Saharan Africa. Indeed, this region of the world accounts for more than 95% of all malaria deaths, and nearly 80% of deaths are among children under 5 years old (WHO, 2020, 2022). Currently, six species are known to infect humans (Plasmodium falciparum, Plasmodium malariae, Plasmodium ovale curtisi, Plasmodium ovale wallikiri, Plasmodium vivax, and Plasmodium knowlesi) (Lalremruata et al., 2017; Rougeron et al., 2022). In fact, malaria is endemic in the majority of countries in Central Africa. The situation concerning the infection varies from country to country (Gemperli et al., 2006). For example, in Cameroon, the prevalence of malaria in humans is 30% (Eleonore et al., 2020) whereas in Central African Republic, it is between 50–60% (Nzoumbou-Boko et al., 2020) and in Congo, the infection level is 52.8% (Mbouloungou et al., 2019; Mayengue et al., 2020).
Malaria remains a major public health problem in Gabon. It is the main cause of medical consultations and hospitalizations (Assele et al., 2015; Manego et al., 2017). Gabon is located in a hyper-endemic area and malaria risk is present throughout the country (Maghendji-Nzondo et al., 2016). Malaria transmission is perennial with high transmission occurring throughout the year. Available malaria data show that the prevalence ranging from 20–34% in urban areas (Maghendji-Nzondo et al., 2016; Larson-Boundenga et al., 2023; Lendongo-Wombo et al., 2023) and between 47–55% in rural areas (Assele et al., 2015; Pegha-Moukandja et al., 2016). More than 90% of malaria cases are due to P. falciparum (Maghendji-Nzondo et al., 2016). Moreover, multidrug-resistant P. falciparum malaria has long been present and documented in several areas in Gabon (Pradines et al., 1998; Ndong et al., 2003; Boukoumba et al., 2021). Recently, a study carried out in departmental capitals of the province of Nyanga revealed a high prevalence of malaria (13.9%) in asymptomatic people, however, data on the vectors involved in the Plasmodium transmission in this province are not available or fragmentary (Imboumy-Limoukou et al., 2020) and dating back several decades (Galliard, 1932).
In the light of these epidemiological data, malaria situations present particular modalities that could be explained by differences related to the biotope, the diversity of vertebrate hosts, the mosquitoes of the genus Anopheles and the parasites they transmit and also the dynamics of their interrelationships (Carnevale et al., 1984; Marsh and Snow, 1997; Mouchet, 2004; Acharya et al., 2017). Of the 131 Anopheles species found in tropical Africa, less than 10% are known to transmit Plasmodium (Sinka et al., 2012; Coetzee, 2020). In Gabon, around 41 Anopheles species have been recorded, however, among all species identified in different areas of the country, only some are known for their role in Plasmodium transmission, with Anopheles funestus and Anopheles gambiae known as the main vectors (Longo-Pendy et al., 2022). In addition, recent studies have revealed that some opportunistic anopheline mosquitoes could play the role of bridge vectors (e.g., Anopheles moucheti, Anopheles vinckei) and promote the exchange of Plasmodium between humans and primates (Paupy et al., 2013; Makanga et al., 2016). Moreover, the fact that the vectors responsible for the transmission of malaria are found in different ecosystems (forest, rural, and urban) (Longo-Pendy et al., 2022) could be a limitation for all control strategies but it could also enhance the risk of parasites exchange between humans and animals in the rural and forest environment.
We think that a potential risk scenario for the future would be the ability of ape’s malaria parasite species to infect humans like was observed with P. knowlesi in Southeast Asia (Setiadi et al., 2016). Thus, a better understanding of the evolution of these different factors involved in malaria transmission is needed through entomological and parasitological monitoring which could provide more information on the ecology of malaria vectors species and their ability to switch hosts and to be infected with and potentially transmit the ape’s Plasmodium species in a specific area. In this article, we present the results of a cross-sectional entomological survey carried out for the first time in the four main localities of the Nyanga Province in southwestern Gabon in Central Africa to characterize the Anopheles species and to identify which of these species are involved in Plasmodium transmission to humans.
Materials and methods
Study sites
Bordered in the North-East by the province of Ngounié, and in the North-West by the province of Ogooué-Maritime, the province of Nyanga is a region that covers an area of 21,285 km2 (Figure 1; Matoumba, 2011). This province is home to the Moukalaba-Doudou protected area, one of the largest national parks in Gabon known for its ape and chimpanzees populations. The sampling sites were selected in both urban and rural environments (Table 1). The factors “population size,” “type of human activity,” “proximity to forest” and “built density” were used to discriminate between urban and rural areas in this study (Table 1). Indeed, a high density of buildings and inhabitants characterizes the urban environment, while the rural environment low density of buildings and very close to the forest environment. Tchibanga (Urban, Latitude: −2.927877; Longitude: 10.997658), the provincial capital, is the first locality with a population of 30,042 (DGS, 2015). Mayumba (Urban, Latitude: −3.442609, Longitude: 10.656261; Population: 5220 inhabitants), a coastal locality, is one of the main tourist destinations in Gabon and Nyanga Province in particular. Moabi (Latitude: −2.444807; Longitude: 10.922204; Population: 3418 inhabitants) and Moulengui-Binza (Latitude: −3.483083; Longitude: 11.743834, Population: 518 inhabitants) (Rural) are villages in this province whose main activities are cassava and banana productions, respectively. These villages have the particularity of being close to National Parks containing a great diversity of fauna, notably great apes [(Gorilla gorilla gorilla and Pan troglodytes troglodytes) (Kami et al., 2019; Freddy and Bakouetila, 2020; Nguema, 2020; Oyaba Yinda et al., 2022)]. Indeed, Moabi is located less than 80 km from Moukalaba-Doudou National Park in Gabon and Moulengui-Binza is less than 60 km from Conkouati-Douli National Park in the Republic of Congo (Figure 1).1
Mosquito collection
To assess the diversity of the anopheline mosquitoes feeding on humans, we performed sessions of collection on humans in both urban and rural environments. These captures were done in April 2018 during two consecutive nights (from 6: 00 PM to 6: 00 AM) in four subsites (Table 1). Captures were made by eight volunteers (volunteers were over 18 years old and all were male) of our team by site. The daily exposure time of the volunteers was 12 h. All mosquitoes were collected by using hemolysis tubes on the exposed legs of the volunteers (all protected with malaria prophylaxis). This study and the methods employed for mosquito collection, including human landing catch (HLC) were approved by Gabon’s National Ethics Committee (authorization number PROT 0031/2014/SG/CNE). After morphological identification of the species using the identification key for Culicidae present in Central Africa and Gabon (Baldacchino and Paupy, 2010), inspired by Gillies and De Meillon (1968) and Gillies and Coetzee (1987), Anopheles specimens were preserved individually in tubes with desiccant.
DNA extraction and molecular analysis
For each Anopheles collected, DNA was extracted and purified from the head-thorax assembly without the abdomen by using the Cetyltrimethylammonium Bromide (CTAB) extraction protocol (Morlais et al., 2004). Diagnostic PCRs were selected and applied according to the outcome of the morphological identification of different complex and group of Anopheles (Fanello et al., 2002; Cohuet et al., 2003; Kengne et al., 2003, 2007). However, total DNA was also used to amplify a portion of the mitochondrial Cytochrome oxidase II (COII) (Rahola et al., 2014) to confirm the mosquito species, especially for specimens for which the morphological diagnose was doubtful or impossible because of poor preservation of individuals’ morphological characters. For the identification of malaria parasites, we first used the mitochondrial Cytochrome b gene PCR protocol described previously in Boundenga et al. (2017). Then, all positive samples were subjected to PCR following the protocol of Snounou and Singh (2002) in order to discriminate all Plasmodium spp. capable of infecting humans. Small subunit ribosomal RNA (ssrRNA) were used for the phylogenetic analysis of the Plasmodium species. All samples that remained unidentified by this method were reanalyzed using PCR of the Cytochrome b (Cyt-b). The amplification of the portion of Cyt-b gene is based on a nested PCR with 2 sets of primers, one developed by Perkins and Schall (2002) (DW2– DW4) and one from Schwöbel et al. (2003). This combination of primers (despite the fact that some of these primers (Cytb1 and Cytb2) were originally developed to amplify P. falciparum Cytochrome-b gene) can amplify the Cytochrome-b from other haemosporidian parasites infecting a diverse range of hosts (Prugnolle et al., 2010, 2011; Boundenga et al., 2016; Makanga et al., 2016).
Contamination verification
All molecular analyses were repeated and verified in three different separate laboratories at the CIRMF (Entomology and Parasitology department), of which two have never manipulated Plasmodium DNA to avoid the problem of contamination. In each laboratory, a single designated experimenter performed the molecular analyses and the three experimenters obtained identical results. In order to ensure that the results obtained were not the result of any contamination, verification manipulations were carried out. Firstly, the Cyt-b PCR protocol was carried out on thirty-seven (37) non-Anopheles mosquitoes, namely eight (8) Aedes aegypti, fifteen (15) Aedes albopictus, nine (9) Aedes africanus, three (3) Culex spp. and two (2) Toxorhynchites spp. The same protocol was carried out on a plate of 96 water samples. Then, the genetic distance between the different Plasmodium obtained in this study was calculated using the MEGA11 software in order to look at the similarity between the Plasmodium sequences. After, investigation of potential Plasmodium contamination within the laboratory shows that no specific bands of the expected size were amplified from the DNA of the 37 non-Anopheles mosquitoes and the 96 water samples. In addition, the genetic distance analysis between the great ape Plasmodium sequences shows that the sequences are not similar (Supplementary Table S1).
Phylogenetic analyses
For the molecular identification of Anopheles species and their respective malaria, we performed phylogenetic analyses using (i) COII and (ii) Cyt-b sequences for mosquitoes and parasites, respectively. Each analysis was performed using a set of previously known species sequences from different mosquito species and Plasmodium species infecting humans and primates. Multiple alignments of all sequences were performed using ClustalW [(v 1.8.1 in BioEdit v.7.0.9.0.) (Hall, 1999)]. Maximum Likelihood (ML) tree construction was performed based on the Cytochrome-b and mosquito COII sequences of 773 and 603 nucleotides, respectively. The best-fitting ML model according to Akaike’s information criterion was the general time-reversible + γ distribution for nucleotides, as identified by ModelTest (Posada and Crandall, 1998). The most probable DNA trees and corresponding bootstrap support values were obtained by the online software PhyML2 using nearest neighbor exchange and subtree pruning, regrafting, branch exchange, and 100 bootstrap repeats (Guindon et al., 2010). Finally, we differentiated Plasmodium infections of simian origin from those of human origin by analyzing the polymorphism of the sequences obtained. Thus, we identified a polymorphism at positions 3,575 and 3,617 spread over the whole mitochondrial genome and verified on human P. falciparum (AY282930-P. falciparum 3D7 and AY282924-P. falciparum-102) as described in Prugnolle et al. (2011).
Statistical analyses
All statistical analyses were performed using R software version 4.0.2. The comparison of Culicidae proportions between urban and rural areas was carried out using the “chisq. Test” function in R. The Shannon index of diversity (H′) was determined using the “diversity” function of the vegan package (Oksanen et al., 2013).
Results
Taxa of sampled mosquitoes
Five hundred and ninety-one (591) mosquitoes were sampled throughout the survey in the four study sites (Table 1). The mosquitoes mainly belonged to four genera (Culex spp., Anopheles spp., Mansonia spp. and Aedes spp.). The majority of the specimens were assigned to the Culex genus, which represented by 65.7%; followed by Anopheles spp. (31.6%), Mansonia spp. (1.7%) and Aedes spp. (1%) (See Table 1; Figure 2 for more information). Comparative analysis of the populations revealed that Culex species were significantly predominant in urban localities (Tchibanga and Mayumba) compared to rural localities where Anopheles species dominated (χ2 = 289.6, value of p <0.0001).
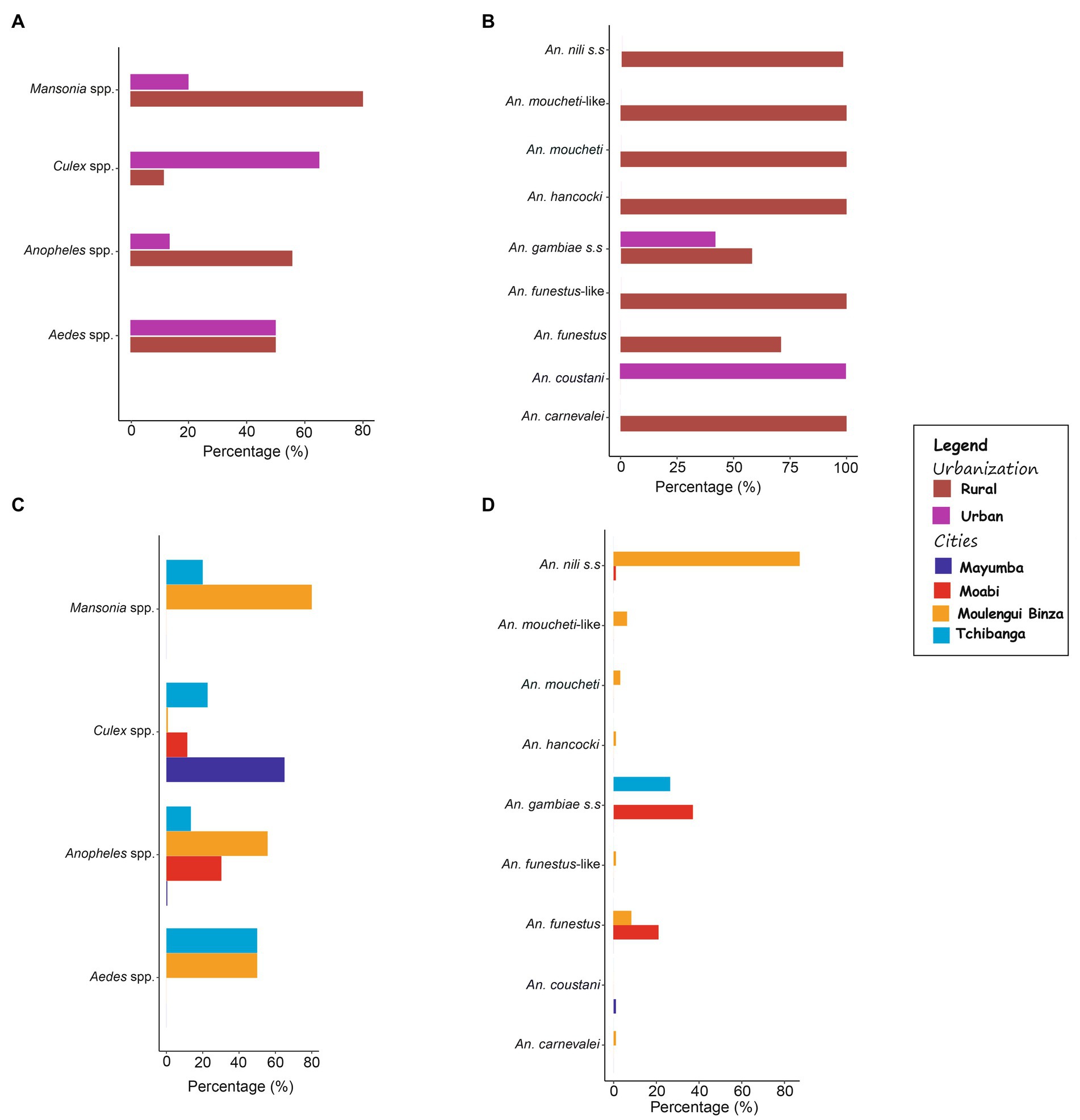
Figure 2. Percentage of different mosquito (A) genera and (B) Anopheles species rural versus urban environment, and population of different mosquito (C) genera and (D) Anopheles species per locality.
Anopheles diversity and distribution
Regarding the Anopheles diversity, after morphological and molecular analysis of 185 specimens belonging to this genus, our results revealed that the specimen identified belonged to nine known species, of which the most frequent were Anopheles nili s.s (45.41%) followed by An. gambiae s.s (32.43%), An. funestus (15.14%), An. moucheti-like (3.24%), An. moucheti (1.62%), An. hancocki (0.54%), An. carnevalei (0.54%), An. coustani (0.54%) and An. funestus-like (0.54%) (Figures 2, 3; Table 2). Rural areas (H′ = 1.27) were more diverse than urban areas (H′ = 0.16) and contained more than 85% of the anopheline fauna. In addition, we observed that this fauna was composed of species of the Anopheles nili complex [(53.46%) (An. nili s.s and An. carnevalei)], followed by those of the Anopheles gambiae complex [(22.01%) (An. gambiae s. s)], the Anopheles funestus group [(18.24%) (An. funestus and An. funestus-like)], the Anopheles moucheti complex [(5.66%) (An. moucheti and An. moucheti-like)], and An. hancocki (0.63%) (Figure 2). However, urban environments were mainly composed of An. gambiae s.s (96%) and one An. coustani (4%) specimen.
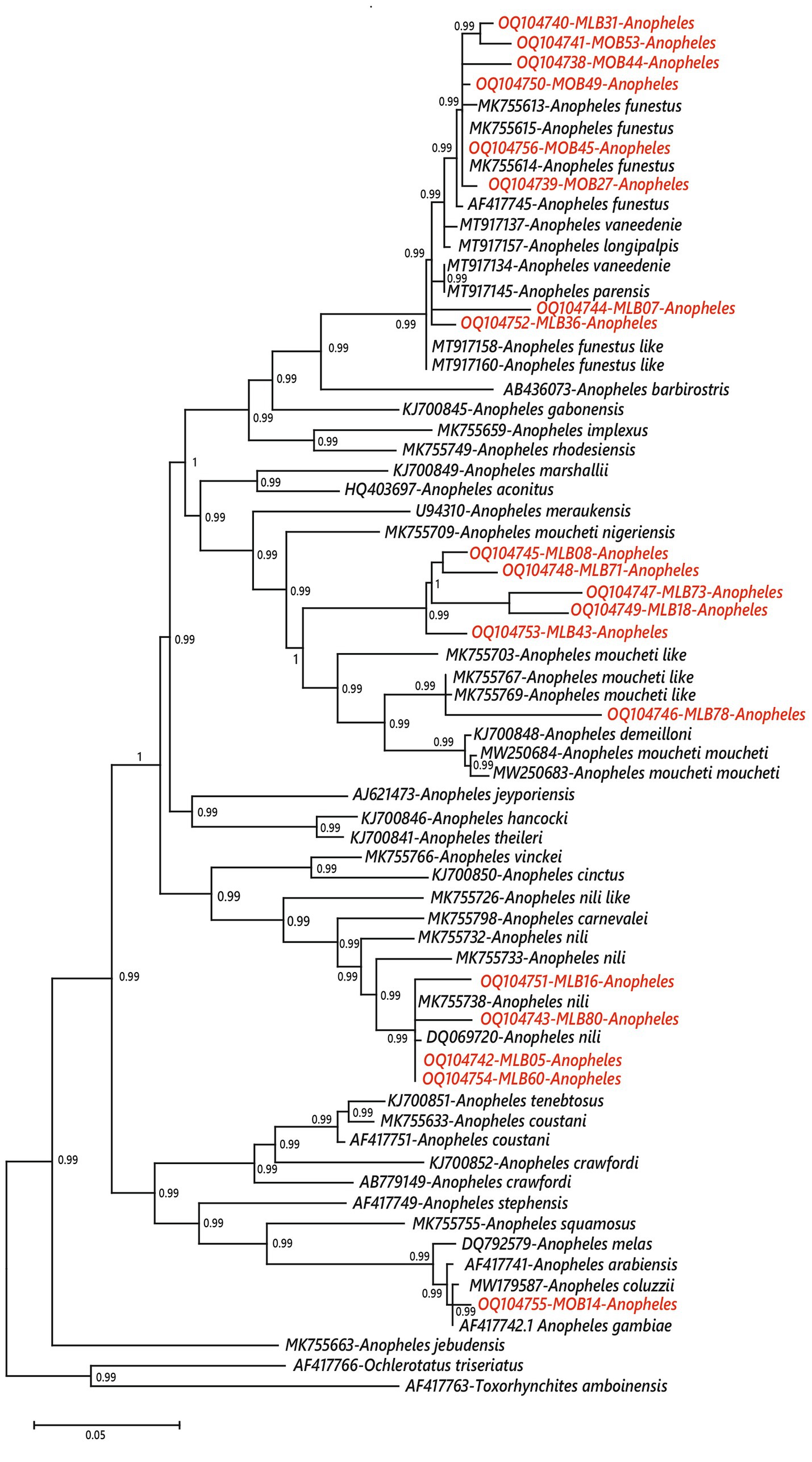
Figure 3. Phylogenetic position of mosquitoes of the Anopheles genus indeterminate by conventional species diagnostic PCR in this study. The tree was constructed from COII sequences using maximum likelihood (ML) methods. Bootstrap values are indicated at each node. The sequences obtained in this study are in red.
Plasmodium infection in malaria vectors
One hundred and eighty-five (185) upper thorax parts of anopheline mosquito samples were tested for the presence of malaria parasites using a Cytochrome b (Cyt-b)-based nested PCR method. Overall, thirty-three (33) samples were positive with malaria parasites, giving a prevalence of 17.84% (Table 2). Our results reveal the variation of average prevalence of Plasmodium-infected Anopheles per site (Table 2). Indeed, we observed significant differences in the rates of Plasmodium-infected Anopheles between the sites: Moabi, Tchibanga, Moulengui-Binza, and Mayumba had 30.36, 16, 11.65 and 0%, respectively (Fisher’s test, value of p = 0.024). However, malaria transmission does not differ between urban and rural environments (Fisher’s test, value of p = 1). Among the nine (n = 9) Anopheles species identified, only six were found to be infected, namely An. gambiae s.s (12/60), An. funestus (9/28), An. nili s.s (7/84), An. moucheti like (3/6), An. moucheti (1/3) and An. funestus like (1/1) (Table 2). Molecular and phylogenetic analyses revealed that the Plasmodium spp. detected belonged to three known species; one which infects human (Plasmodium falciparum) and two which infect apes (Plasmodium reichenowi and Plasmodium praefalciparum). Of all the Plasmodium detected P. falciparum was the most prevalent (15.68%), followed by P. praefalciparum (1.62%) and P. reichenowi (0.54%) (Table 2; Figure 4). Of the twenty-nine P. falciparum positives, the most infected species were An. gambiae s.s (41.4%) followed by An. funestus (24.14%) and An. nili s.s (24.14%). P. falciparum was found in the upper parts of six Anopheles species (An. gambiae s.s, An. funestus, An. funestus-like, An. moucheti, An. moucheti like and An. nili s.s) from urban and rural environments. However, anopheline mosquito infested with apes Plasmodium were observed only in rural areas. Indeed, P. praefalciparum was detected in two species (An. funestus and An. moucheti-like) from Moabi and Moulengui-Binza and P. reichenowi was observed in An. funestus form Moabi (Table 2; Figure 4).
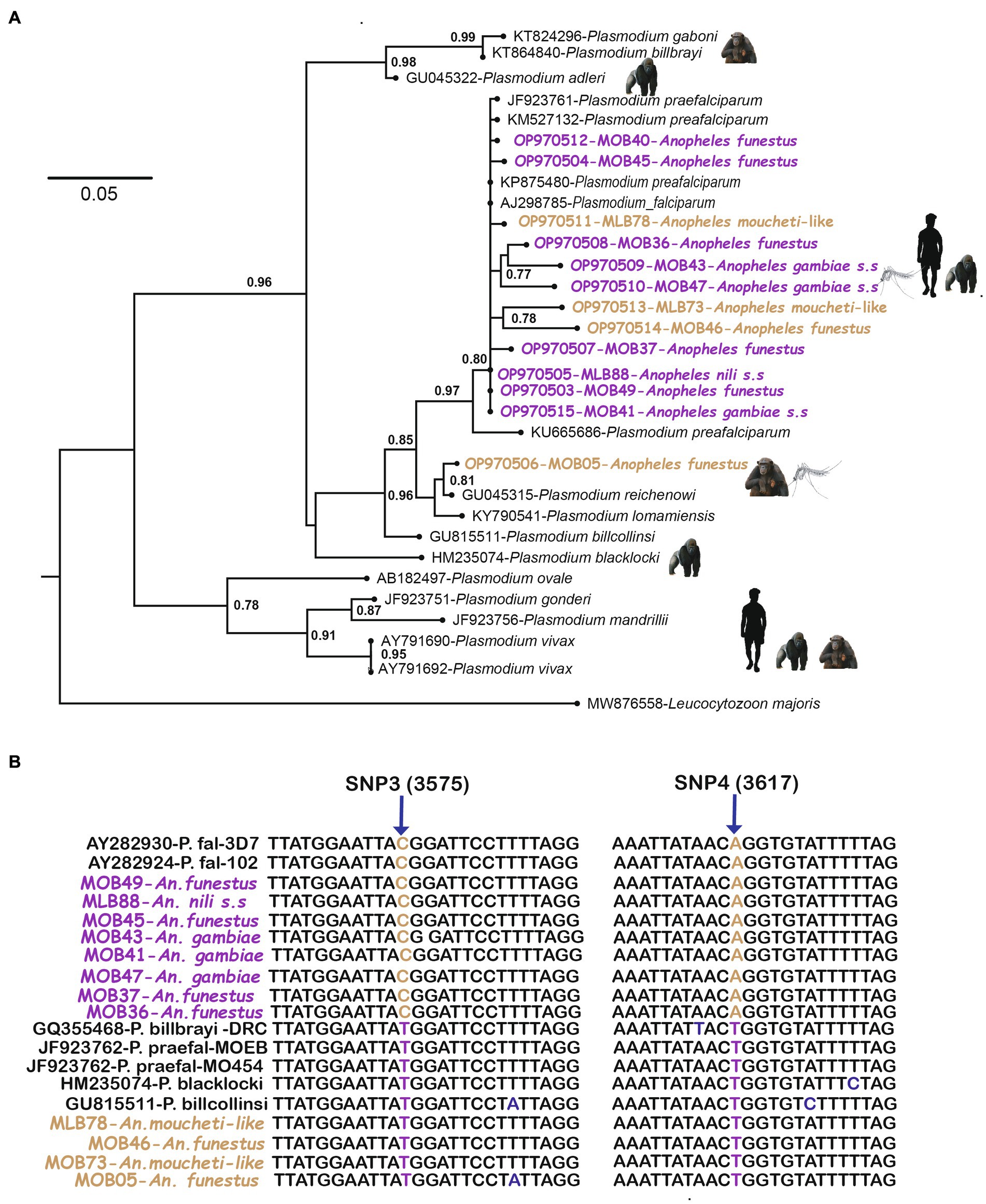
Figure 4. (A) Phylogenetic relationships between Plasmodium isolates obtained after sequencing of the Cytochrome b gene. The tree was constructed using Maximum likelihood (ML) methods. Bootstrap values are shown at each node except those below 0.5. Leucocytozoon majoris was used to root the tree. (B) Shown the result of comparison at the level of two diagnostic mitochondrial SNPs of our twelve suspect samples against those present in Plasmodium falciparum and non-human isolates.
Discussion
In this study, we sought to identify Anopheles species involved in human malaria transmission in urban and rural areas. To do so, we conducted a study of anopheline communities in the Nyanga Province in southern Gabon (Central Africa). Indeed, the lack of knowledge of the different vector species potentially involved in the transmission of malaria remains an obstacle to the implementation of an effective malaria control system (WHO, 2020, 2022) especially for countries located in hyper-endemic areas such as Gabon, where the most vulnerable groups are pregnant women and children under 5 years old (Jäckle et al., 2013; Mawili-Mboumba et al., 2013; Imboumy-Limoukou et al., 2020). Thus, this cross-sectional entomological study was carried out following a parasitological study which revealed a significant average malaria prevalence of 13.9% in Nyanga Province (Imboumy-Limoukou et al., 2020).
Urbanization influences on Culicidae composition in Nyanga
In the present study, the results showed that four groups of mosquitoes feed on human subjects in the four sites surveyed. However, the spatial distribution of the different identified mosquitoes was not homogeneous across the different environments. Indeed, mosquitoes of the genus Culex were significantly more abundant in urban areas, whereas in rural areas, mosquitoes of the genus Anopheles were predominant and very diverse. This contrasting abundance and diversity of Anopheles species between urban and rural areas could be explained by environmental disturbances resulting from anthropogenic land use, which in most cases could eliminate a greater diversity of larval breeding sites in urban areas than in rural areas (Johnson and Munshi-South, 2017; Epelboin et al., 2021; Nieto-Sanchez et al., 2022; Verrelli et al., 2022). Thus, the higher species diversity identified in rural areas could be explained by the stability of rural environments that favors the abundance of anopheline species (Imbahale et al., 2011; Mzilahowa et al., 2016). Indeed, studies on the impact of urbanization shows that both faunal and floral species richness along an urban–rural gradient decreases from the periphery to the middle of the city (Johnson and Munshi-South, 2017). These observations could be explained by environmental conditions such as deforestation, industrial and domestic pollution of surface waters, which destroy the ecological niches of certain species and thus create new environmental conditions unfavorable to the normal life cycle of indigenous species (Clergeau et al., 1998; Chace and Walsh, 2006), particularly mosquito vectors of Plasmodium (Longo-Pendy et al., 2021). Seven of the nine Anopheles species recorded were exclusive to rural areas, namely An. nili s.s, An. funestus, An. funestus like, An. moucheti, An. moucheti like, An. carnevalei and An. hancocki. Therefore, only mosquito species that are able to adapt to environmental changes (such as those generated by pollution of any kind) in urban areas such as the larvae of the Culex genus (Doby and Mouchet, 1957; Subra, 1981) and very recently the sister species of the Anopheles gambiae complex, An. gambiae s.s and An. coluzzii (Antonio-Nkondjio et al., 2011; Kamdem et al., 2012; Tene Fossog et al., 2012; Longo-Pendy et al., 2021; Nieto-Sanchez et al., 2022), can proliferate in mostly polluted urban breeding sites (Brown et al., 2008). This is coherent with our results, which showed that the Culicidae fauna in urban localities in Nyanga Province was mainly composed of adult mosquitoes of the Culex genus and of the species An. gambiae s.s. Thus, the high abundance of the genus Culex that we observed in the urban areas of Mayumba and Tchibanga confirms that rapid unplanned urbanization could contribute to the proliferation of Culex species by creating suitable environmental conditions for their development (Brown et al., 2008; Mohammed et al., 2021). This could constitute a risk for human health in urban centers in Gabon, as Culex species are known to be vectors of several zoonotic pathogens such as Rift Valley fever virus (Ferraguti et al., 2021). Moreover, the very low occurrence of Anopheles mosquitoes observed in Mayumba, with only one specimen detected, An. coustani, which is known to be a minor vector of malaria in sub-Saharan Africa and in Gabon in particular (Longo-Pendy et al., 2022), allows us to justify the observations made by Imboumy-Limoukou et al. (2020) (Imboumy-Limoukou et al., 2020) who showed that there were no cases of malaria in this coastal town of Gabon (Imboumy-Limoukou et al., 2020). The impact of environmental changes on the spatial dynamics of mosquitoes is not only specific to Anopheles species but has also been observed in mosquitoes of the Aedes genus (Albrieu Llinás et al., 2018; Zettle et al., 2022). This modification of spatial distribution could modulate their trophic behavior and influence the occurrence of the plasmodial species they host as recently described for arboviruses (Li et al., 2013; Kolimenakis et al., 2021) and helminths in the giant toad Rhinella horribilis (Jacinto-Maldonado et al., 2022).
In rural areas, Anopheles harbor apes malaria parasites
Vectors analysis allowed us to observe a prevalence of infection of 17.84% in the Anopheles species surveyed. The prevalence we obtained in this study is higher than those reported in previous studies in Gabon. It has been reported that Anopheles infestation rates range from 0.15 to 0.8% in Libreville (Mourou et al., 2010, 2012), 0.64% in Port-Gentil (Mourou et al., 2010) and 2.2% in Lambaréné (Boussougou-Sambe et al., 2022). This difference in the level of Anopheles infection could be justified, as mentioned above, by the influence of environmental factors specific to each study site. However, the prevalence of malaria observed in Anopheles in our study seems consistent with the 14% level of malaria infection in humans observed in Nyanga Province in 2018 (Imboumy-Limoukou et al., 2020). This observation reflects a high risk of exposure to malaria infection by populations with each Anopheles bite in this region of Gabon. Thus, given the heterogeneity of malaria prevalence from one locality to another, the establishment of an epidemiological surveillance system in this region would allow a better characterization of the transmission facies, and consequently the adoption of more effective malaria control strategies.
Concerning malaria parasites species, our study revealed that some of these Anopheles species were carriers of sporozoites of three plasmodial species belonging to the subgenus Laverania in particular P. falciparum, P. reichenowi, and P. praefalciparum. Among these parasite species, the most represented was P. falciparum (87.9%) followed by P. praefalciparum (9.1%) and P. reichenowi (3%). The vector species of P. falciparum were mainly An. gambiae s.s, An. nili s.s and An. funestus, species known in sub-Saharan Africa and particularly in Gabon, for their strong involvement in malaria transmission (Sinka et al., 2012; Longo-Pendy et al., 2022). Surprisingly, An. funestus was found to be infected for the first time by P. reichenowi and P. praefalciparum, both species of simian plasmodia. This result shows that this Anopheles species, described in the literature as very anthropophilic, can adopt an opportunistic behavior and adapt its feeding habits depending on the environment it lives. This species would be able to take its blood meal on primates other than humans in rural areas where human populations live near forests. In addition, our study has also enabled us to highlight for the first time the presence of malaria parasite in An. moucheti-like, a species described very recently as being very close to the species of the Anopheles moucheti complex (Ayala et al., 2019). In this study, two specimens of this species were found to be infected with the gorillas Plasmodium (P. praefalciparum). All these new findings combined, allow us to add to the list of potential bridge-vectors of malaria parasite between apes and human (Makanga et al., 2016), An. funestus and An. moucheti-like. Thus, the presence of a wide diversity of Anopheles species with opportunistic behavior could constitute a risk for human health and raises the question of the potential existence of zoonotic infections in this part of Gabon as already described in the Asian region with P. knowlesi (Setiadi et al., 2016), in Latin America with P. simium (Brasil et al., 2017; Mourier et al., 2021; Lempang et al., 2022), and in Central Africa with P. vivax-like (Prugnolle et al., 2013). Thus, it could be that the identical morphology of Plasmodium belonging to the subgenus Laverania, as seen between P. falciparum and P. reichenowi (Coatney, 1971; Martin et al., 2005), could lead to a proportion of human malaria cases being wrongly attributed to P. falciparum in this region of Gabon where diagnosis of malaria is done only via microscopy and malaria rapid detection test (RDT) methods. Only a molecular characterization would allow to distinguish these Plasmodium species. However, it would be necessary to carry out a longitudinal entomological and parasitological follow-up with molecular tools that would allow us to confirm the involvement of An. funestus and An. moucheti-like in malaria transmissions from great apes. In addition, it would be necessary to highlight the role of bridge vector in the simians malaria transmission to humans and finally to determine the occurrence of Plasmodium species of non-human primates in humans and the associated clinical signs.
Conclusion
Our study highlighted that among the large diversity of Anopheles species found in the province of Nyanga in south-eastern Gabon, one Anopheles species known to be highly involved in human malaria transmission, An. funestus, and a very recently described, An. moucheti-like, were infected with P. reichenowi and P. praefalciparum which are great ape Plasmodium species genetically very close to P. falciparum. One of the scenarios that could be imagined in this region of the country, would be the existence of a zoonotic transmission of malaria that is undetectable in humans with the conventional tools of plasmodial morpho-taxonomy, notably the blood smear, and which could be untreatable with artemisinin derivatives. Thus, a better characterization of this zoonotic transmission would require the implementation of molecular diagnostic tools that would allow to reveal it and to strategize ways to manage it.
Data availability statement
The datasets presented in this study can be found in online repositories. The names of the repository/repositories and accession number(s) can be found at: https://www.ncbi.nlm.nih.gov/genbank/, OP970503-OP970515 and OQ104738-OQ104756.
Ethics statement
The studies involving human participants were reviewed and approved by National Ethical Research Committee in Gabon (No. PROT 0031/2014/SG/CNE and N °001/PR/SG/CNE/2018). The patients/participants provided their written informed consent to participate in this study.
Author contributions
N-ML-P, J-BL-D, and PK: conceptualization. BM: fieldwork and morpho-taxonomy of mosquitoes. N-ML-P, OA-E, CM-B, LaB, and SL-D: molecular analysis and sequencing. N-ML-P and LaB: phylogenetic and bio-statistical analyses. N-ML-P and LaB: writing–original draft. BM, CM-B, LeB, OA-E, LN-N, J-BL-D, PK, LaB, and N-ML-P: writing–review and editing. PK and J-BL-D: supervision. J-BL-D: project management and funding acquisition. All authors contributed to the article and approved the submitted version.
Funding
This work was supported by the MCDI (Medical Care Development International, European and developing Countries clinical Trial Partnership) and the logistics and transport aspects by the CIRMF (CIRMF is funded by Total Energy Gabon). CIRMF is a member of CANTAM funding by EDCTP CSA2020NoE-3100-CANTAM 3.
Acknowledgments
The authors would like to thank the volunteers in Tchibanga, Mayumba, Moabi, and Moulengui-Binza for their collaboration and diligence during the field surveys. They also like to thank Patrick Yangari and Alain Prince Okouga for coordinating the field surveys and Franck Prugnolle, Judicaël Obame-Nkoghe, Franck Mounioko, Elsa Rush Eyang Assengone, and Patrick Voua Otomo for their assistance and discussion.
Conflict of interest
The authors declare that the research was conducted in the absence of any commercial or financial relationships that could be construed as a potential conflict of interest.
Publisher’s note
All claims expressed in this article are solely those of the authors and do not necessarily represent those of their affiliated organizations, or those of the publisher, the editors and the reviewers. Any product that may be evaluated in this article, or claim that may be made by its manufacturer, is not guaranteed or endorsed by the publisher.
Supplementary material
The Supplementary material for this article can be found online at: https://www.frontiersin.org/articles/10.3389/fevo.2023.1176687/full#supplementary-material
Footnotes
References
Acharya, P., Garg, M., Kumar, P., Munjal, A., and Raja, K. (2017). Host–parasite interactions in human malaria: clinical implications of basic research. Front. Microbiol. 8:889. doi: 10.3389/fmicb.2017.00889
Albrieu Llinás, G., Espinosa, M. O., Quaglia, A. I. E., Abril, M., and Scavuzzo, C. M. (2018). Urban environmental clustering to assess the spatial dynamics of Aedes aegypti breeding sites. Geospat Health 13. doi: 10.4081/gh.2018.654
Antonio-Nkondjio, C., Fossog, B. T., Ndo, C., Djantio, B. M., Togouet, S. Z., Awono-Ambene, P., et al. (2011). Anopheles gambiae distribution and insecticide resistance in the cities of Douala and Yaounde (Cameroon): influence of urban agriculture and pollution. Malar. J. 10:154. doi: 10.1186/1475-2875-10-154
Assele, V., Ndoh, G. E., Nkoghe, D., and Fandeur, T. (2015). No evidence of decline in malaria burden from 2006 to 2013 in a rural province of Gabon: implications for public health policy. BMC Public Health 15, 1–8. doi: 10.1186/s12889-015-1456-4
Ayala, D., Akone-Ella, O., Rahola, N., Kengne, P., Ngangue, M. F., Mezeme, F., et al. (2019). Natural Wolbachia infections are common in the major malaria vectors in Central Africa. Evol. Appl. 12, 1583–1594. doi: 10.1111/eva.12804
Baldacchino, F., and Paupy, C. (2010). Clé de détermination des Culicidae présents en Afrique Centrale et au Gabon. Document de travail, IRD/CIRMF 108.
Boukoumba, F. M., Lekana-Douki, J. B., Matsiegui, P. B., Moukodoum, D. N., Adegnika, A. A., and Oyegue-Liabagui, S. L. (2021). High prevalence of genotypes associated with sulfadoxine/pyrimethamine resistance in the rural area of Fougamou, Gabon. J. Global Antimicrob. Resist. 25, 181–186. doi: 10.1016/j.jgar.2021.03.003
Boundenga, L., Makanga, B., Ollomo, B., Gilabert, A., Rougeron, V., Mve-Ondo, B., et al. (2016). Haemosporidian parasites of antelopes and other vertebrates from Gabon, Central Africa. PLoS One 11:e0148958. doi: 10.1371/journal.pone.0148958
Boundenga, L., Perkins, S. L., Ollomo, B., Rougeron, V., Leroy, E. M., Renaud, F., et al. (2017). Haemosporidian parasites of reptiles and birds from Gabon, Central Africa. J. Parasitol. 103, 330–337. doi: 10.1645/16-118
Boussougou-Sambe, S. T., Woldearegai, T. G., Doumba-Ndalembouly, A. G., Ngossanga, B., Mba, R. B., Edoa, J. R., et al. (2022). Assessment of malaria transmission intensity and insecticide resistance mechanisms in three rural areas of the Moyen Ogooué Province of Gabon. Parasit. Vectors 15, 1–13. doi: 10.1186/s13071-022-05320-9
Brasil, P., Zalis, M. G., De Pina-Costa, A., Siqueira, A. M., Júnior, C. B., Silva, S., et al. (2017). Outbreak of human malaria caused by Plasmodium simium in the Atlantic Forest in Rio de Janeiro: a molecular epidemiological investigation. Lancet Glob. Health 5, e1038–e1046. doi: 10.1016/S2214-109X(17)30333-9
Brown, H. E., Childs, J. E., Diuk-Wasser, M. A., and Fish, D. (2008). Ecological factors associated with West Nile virus transmission, northeastern United States. Emerg. Infect. Dis. 14, 1539–1545. doi: 10.3201/eid1410.071396
Carnevale, P., Robert, V., Molez, J.-F., and Baudon, D. (1984). Epidémiologie générale: faciès épidémiologiques des paludismes en Afrique subsaharienne. Etudes Médicales, 15177, 123–133.
Chace, J. F., and Walsh, J. J. (2006). Urban effects on native avifauna: a review. Landsc. Urban Plan. 74, 46–69. doi: 10.1016/j.landurbplan.2004.08.007
Clergeau, P., Savard, J.-P. L., Mennechez, G., and Falardeau, G. (1998). Bird abundance and diversity along an urban-rural gradient: a comparative study between two cities on different continents. Condor 100, 413–425. doi: 10.2307/1369707
Coatney, G. R. (1971). The primate malarias. Rockville, MD: US National Institute of Allergy and Infectious Diseases.
Coetzee, M. (2020). Key to the females of Afrotropical Anopheles mosquitoes (Diptera: Culicidae). Malar. J. 19, 1–20. doi: 10.1186/s12936-020-3144-9
Cohuet, A., Simard, F., Toto, J.-C., Kengne, P., Coetzee, M., and Fontenille, D. (2003). Species identification within the Anopheles funestus group of malaria vectors in Cameroon and evidence for a new species. Am. J. Trop. Med. Hygiene 69, 200–205. doi: 10.4269/ajtmh.2003.69.200
DGS (2015). Résultats globaux du Recensement Général de la Population et des Logements de 2013 du Gabon (RGPL-2013). Libreville, Publication de la Direction Générale de la Statique (DGS).
Doby, J., and Mouchet, J. (1957). Écologie larvaire de quelques espèces de Culicidés dans la région de Yaoundé (Sud-Cameroun). Bulletin de la Société de Pathologie Exotique 50, 945–957.
Eleonore, N. L. E., Cumber, S. N., Charlotte, E. E., Lucas, E. E., Edgar, M. M. L., Nkfusai, C. N., et al. (2020). Malaria in patients with sickle cell anaemia: burden, risk factors and outcome at the Laquintinie hospital, Cameroon. BMC Infect. Dis. 20, 1–8. doi: 10.1186/s12879-019-4757-x
Epelboin, A., Gazin, P., Goujon, C., and Pichard, E. (2021). Pollutions environnementales et santé sous les tropiques: Journée scientifique dématérialisée de la SFMTSI. Jeudi 20 mai 2021. Médecine Tropicale et Santé Internationale-Bulletin.
Fanello, C., Santolamazza, F., and Della Torre, A. (2002). Simultaneous identification of species and molecular forms of the Anopheles gambiae complex by PCR-RFLP. Med. Vet. Entomol. 16, 461–464. doi: 10.1046/j.1365-2915.2002.00393.x
Ferraguti, M., Heesterbeek, H., Martínez-De La Puente, J., Jiménez-Clavero, M., Vázquez, A., Ruiz, S., et al. (2021). The role of different Culex mosquito species in the transmission of West Nile virus and avian malaria parasites in Mediterranean areas. Transbound. Emerg. Dis. 68, 920–930. doi: 10.1111/tbed.13760
Freddy, G., and Bakouetila, M. (2020). Conflictualité homme-éléphant et écotourisme au parc national Conkouati-Douli (PNCD), République du Congo. Études caribéennes.
Galliard, H. (1932). Culicides du Gabon. III.—Anophelinés. Ann. Parasitol. Hum. Comp. 10, 85–95. doi: 10.1051/parasite/1932101085
Gemperli, A., Sogoba, N., Fondjo, E., Mabaso, M., Bagayoko, M., Briët, O. J., et al. (2006). Mapping malaria transmission in west and Central Africa. Tropical Med. Int. Health 11, 1032–1046. doi: 10.1111/j.1365-3156.2006.01640.x
Gillies, M., and Coetzee, M. (1987). A supplement to the Anophelinae of Africa South of the Sahara. Publ. S Afr. Inst. Med. Res. 55, 1–143.
Gillies, M. T., and De Meillon, B. (1968). The Anophelinae of Africa south of the Sahara (Ethiopian zoogeographical region).
Guindon, S., Dufayard, J. F., Lefort, V., Anisimova, M., Hordijk, W., and Gascuel, O. (2010). New algorithms and methods to estimate maximum-likelihood phylogenies: assessing the performance of PhyML 3.0. Syst. Biol. 59, 307–321. doi: 10.1093/sysbio/syq010
Hall, T. A. (1999). “BioEdit: a user-friendly biological sequence alignment editor and analysis program for windows 95/98/NT” in Nucleic acids symposium series (London: Information Retrieval Ltd., c1979-c2000), 95–98.
Imbahale, S. S., Paaijmans, K. P., Mukabana, W. R., Van Lammeren, R., Githeko, A. K., and Takken, W. (2011). A longitudinal study on Anopheles mosquito larval abundance in distinct geographical and environmental settings in western Kenya. Malar. J. 10:81. doi: 10.1186/1475-2875-10-81
Imboumy-Limoukou, R. K., Maghendji-Nzondo, S., Ondo-Enguier, P. N., Niemczura De Carvalho, J., Tsafack-Tegomo, N. P., Buekens, J., et al. (2020). Malaria in children and women of childbearing age: infection prevalence, knowledge and use of malaria prevention tools in the province of Nyanga, Gabon. Malar. J. 19, 1–8. doi: 10.1186/s12936-020-03411-5
Jacinto-Maldonado, M., García Peña, G. E., Lesbarrères, D., Meza-Figueroa, D., Robles-Morúa, A., Salgado-Maldonado, G., et al. (2022). Urbanization impacts parasite diversity in the cane toad Rhinella horribilis (Anura: Bufonidae). Global Ecol. Conserv. 38:e02275. doi: 10.1016/j.gecco.2022.e02275
Jäckle, M. J., Blumentrath, C. G., Zoleko, R. M., Akerey-Diop, D., Mackanga, J.-R., Adegnika, A. A., et al. (2013). Malaria in pregnancy in rural Gabon: a cross-sectional survey on the impact of seasonality in high-risk groups. Malar. J. 12, 1–6. doi: 10.1186/1475-2875-12-412
Johnson, M. T., and Munshi-South, J. (2017). Evolution of life in urban environments. Science 358:eaam8327. doi: 10.1126/science.aam8327
Kamdem, C., Fossog, B. T., Simard, F., Etouna, J., Ndo, C., Kengne, P., et al. (2012). Anthropogenic habitat disturbance and ecological divergence between incipient species of the malaria mosquito Anopheles gambiae. PLoS One 7:e39453. doi: 10.1371/journal.pone.0039453
Kami, T. J. V., Moutsambote, J.-M., Fdez-Navarro, S., Darby, L., and Atencia, R. (2019). Forest feeding phenology of Pan troglodytes (chimpanzee) east of Conkouati-Douli National Park, republic of Congo. Int. J. Innov. Appl. Stud. 27, 133–145.
Kengne, P., Antonio-Nkondjio, C., Awono-Ambene, H., Simard, F., Awolola, T., and Fontenille, D. (2007). Molecular differentiation of three closely related members of the mosquito species complex, Anopheles moucheti, by mitochondrial and ribosomal DNA polymorphism. Med. Vet. Entomol. 21, 177–182. doi: 10.1111/j.1365-2915.2007.00681.x
Kengne, P., Awono-Ambene, P., Nkondjio, C. A., Simard, F., and Fontenille, D. (2003). Molecular identification of the Anopheles nili group of African malaria vectors. Med. Vet. Entomol. 17, 67–74. doi: 10.1046/j.1365-2915.2003.00411.x
Kolimenakis, A., Heinz, S., Wilson, M. L., Winkler, V., Yakob, L., Michaelakis, A., et al. (2021). The role of urbanisation in the spread of Aedes mosquitoes and the diseases they transmit—a systematic review. PLoS Negl. Trop. Dis. 15:e0009631. doi: 10.1371/journal.pntd.0009631
Lalremruata, A., Jeyaraj, S., Engleitner, T., Joanny, F., Lang, A., Bélard, S., et al. (2017). Species and genotype diversity of Plasmodium in malaria patients from Gabon analysed by next generation sequencing. Malar. J. 16, 1–11. doi: 10.1186/s12936-017-2044-0
Larson-Boundenga, M. B., Dibakou, S.-E., Mombo, L. E., Jauvert, C., Moukagni-Mussadji, D. M., Wora, F. K.-K., et al. (2023). Increase in malaria prevalence from 2017 to 2019 in Franceville, Southeast Gabon, Central Africa. J. Public Health Africa 14:1865. doi: 10.4081/jphia.2023.1865
Lempang, M. E. P., Dewayanti, F. K., Syahrani, L., Permana, D. H., Malaka, R., Asih, P. B. S., et al. (2022). Primate malaria: an emerging challenge of zoonotic malaria in Indonesia. One. Health 14:100389. doi: 10.1016/j.onehlt.2022.100389
Lendongo-Wombo, J. B., Ibinga, E., Oyegue-Liabagui, S. L., Imboumy Limoukou, R. K., Okouga, A. P., Mounioko, F., et al. (2023). Severe malaria in children and adolescents in Southeast Gabon. BMC Infect. Dis. 23:207. doi: 10.1186/s12879-023-08133-y
Li, S., Tao, H., and Xu, Y. (2013). Abiotic determinants to the spatial dynamics of dengue fever in Guangzhou. Asia Pacific J. Public Health 25, 239–247. doi: 10.1177/1010539511418819
Longo-Pendy, N. M., Boundenga, L., Kutomy, P. O. O., Mbou-Boutambe, C., Makanga, B., Moukodoum, N., et al. (2022). Systematic review on diversity and distribution of Anopheles species in Gabon: a fresh look at the potential malaria vectors and perspectives. Pathogens 11:668. doi: 10.3390/pathogens11060668
Longo-Pendy, N. M., Tene-Fossog, B., Tawedi, R. E., Akone-Ella, O., Toty, C., Rahola, N., et al. (2021). Ecological plasticity to ions concentration determines genetic response and dominance of Anopheles coluzzii larvae in urban coastal habitats of Central Africa. Sci. Rep. 11, 1–13. doi: 10.1038/s41598-021-94258-6
Maghendji-Nzondo, S., Nzoughe, H., Lemamy, G. J., Kouna, L. C., Pegha-Moukandja, I., Lekoulou, F., et al. (2016). Prevalence of malaria, prevention measures, and main clinical features in febrile children admitted to the Franceville regional hospital, Gabon. Parasite 23:16032. doi: 10.1051/parasite/2016032
Makanga, B., Yangari, P., Rahola, N., Rougeron, V., Elguero, E., Boundenga, L., et al. (2016). Ape malaria transmission and potential for ape-to-human transfers in Africa. Proc. Natl. Acad. Sci. 113, 5329–5334. doi: 10.1073/pnas.1603008113
Manego, R. Z., Mombo-Ngoma, G., Witte, M., Held, J., Gmeiner, M., Gebru, T., et al. (2017). Demography, maternal health and the epidemiology of malaria and other major infectious diseases in the rural department Tsamba-Magotsi, Ngounie Province, in central African Gabon. BMC Public Health 17, 1–7. doi: 10.1186/s12889-017-4045-x
Marsh, K., and Snow, R. W. (1997). Host—parasite interaction and morbidity in malaria endemic areas. Philosophical transactions of the Royal Society of London. Series B Biol. Sci. 352, 1385–1394.
Martin, M. J., Rayner, J. C., Gagneux, P., Barnwell, J. W., and Varki, A. (2005). Evolution of human-chimpanzee differences in malaria susceptibility: relationship to human genetic loss of N-glycolylneuraminic acid. Proc. Natl. Acad. Sci. 102, 12819–12824. doi: 10.1073/pnas.0503819102
Mawili-Mboumba, D. P., Akotet, M. K. B., Kendjo, E., Nzamba, J., Medang, M. O., Mbina, J.-R. M., et al. (2013). Increase in malaria prevalence and age of at risk population in different areas of Gabon. Malar. J. 12:3. doi: 10.1186/1475-2875-12-3
Mayengue, P. I., Batsimba, D. K., Niama, R. F., Ottia, R. I., Malonga-Massanga, A., Fila-Fila, G. P. U., et al. (2020). Variation of prevalence of malaria, parasite density and the multiplicity of Plasmodium falciparum infection throughout the year at three different health centers in Brazzaville, republic of Congo. BMC Infect. Dis. 20, 1–10. doi: 10.1186/s12879-020-4913-3
Mbouloungou, A., Koumba, A. A., Mombo, J. B., Iyangui, N. N., Mavoungou, J. F., and Djeki, J. (2019). Géographie du paludisme dans la région de Libreville-Owendo-Akanda, Gabon. Eur. Sci. J. 15, 362–382. doi: 10.19044/esj.2019.v15n27p362
Mohammed, B. R., Yayo, A. M., Ajanusi, O. J., and Lawal, I. A. (2021). Relative abundance and molecular identification of Culex pipiens complex (Diptera: Culicidae), in Kura local government area, North-western Nigeria. Parasite Epidemiol Control 14:e00213. doi: 10.1016/j.parepi.2021.e00213
Morlais, I., Ponçon, N., Simard, F., Cohuet, A., and Fontenille, D. (2004). Intraspecific nucleotide variation in Anopheles gambiae: new insights into the biology of malaria vectors. Am. J. Trop. Med. Hygiene 71, 795–802. doi: 10.4269/ajtmh.2004.71.795
Mourier, T., De Alvarenga, D. A. M., Kaushik, A., De Pina-Costa, A., Douvropoulou, O., Guan, Q., et al. (2021). The genome of the zoonotic malaria parasite Plasmodium simium reveals adaptations to host switching. BMC Biol. 19, 1–17. doi: 10.1186/s12915-021-01139-5
Mourou, J.-R., Coffinet, T., Jarjaval, F., Cotteaux, C., Pradines, E., Godefroy, L., et al. (2012). Malaria transmission in Libreville: results of a one year survey. Malar. J. 11:40. doi: 10.1186/1475-2875-11-40
Mourou, J.-R., Coffinet, T., Jarjaval, F., Pradines, B., Amalvict, R., Rogier, C., et al. (2010). Malaria transmission and insecticide resistance of Anopheles gambiae in Libreville and Port-Gentil, Gabon. Malar. J. 9, 1475–2875. doi: 10.1186/1475-2875-9-321
Mzilahowa, T., Luka-Banda, M., Uzalili, V., Mathanga, D. P., Campbell, C. H. Jr., Mukaka, M., et al. (2016). Risk factors for Anopheles mosquitoes in rural and urban areas of Blantyre District, southern Malawi. Malawi Med. J. 28, 154–158. doi: 10.4314/mmj.v28i4.2
Ndong, J. M. M., Atteke, C., Aubouy, A., Bakary, M., Lébibi, J., and Deloron, P. (2003). In vitro activity of chloroquine, quinine, mefloquine and halofantrine against Gabonese isolates of Plasmodium falciparum. Tropical Med. Int. Health 8, 25–29. doi: 10.1046/j.1365-3156.2003.00967.x
Nguema, P. M. (2020). Quantification de la résistance aux antibiotiques dans la faune sauvage des forets du Gabon: Conséquences pour la compréhension des mécanismes d'émergence de nouvelles résistances aux antibiotiques chez l'homme.
Nieto-Sanchez, C., Dens, S., Solomon, K., Haile, A., Yuan, Y., Hawer, T., et al. (2022). Beyond eves and cracks: an interdisciplinary study of socio-spatial variation in urban malaria transmission in Ethiopia. PLoS Global Public Health 2:e0000173. doi: 10.1371/journal.pgph.0000173
Nzoumbou-Boko, R., Yambiyo, B. M., Ngoagouni, C., Vickos, U., Manirakiza, A., and Nakouné, E. (2020). Falciparum malaria in febrile patients at sentinel sites for influenza surveillance in the Central African Republic from 2015 to 2018. Interdiscipl. Pers. Infect. Dis. 2020, 1–7. doi: 10.1155/2020/3938541
Oksanen, J., Blanchet, F. G., Kindt, R., Legendre, P., Minchin, P. R., Ohara, R., et al. (2013). Package ‘vegan’. Community ecology package, version 2, 1–295.
Oyaba Yinda, L. E. D., Onanga, R., Mbehang Nguema, P. P., Akomo-Okoue, E. F., Nsi Akoue, G., Longo Pendy, N. M., et al. (2022). Phylogenetic groups, Pathotypes and antimicrobial resistance of Escherichia coli isolated from Western lowland Gorilla Faeces (Gorilla gorilla gorilla) of Moukalaba-Doudou National Park (MDNP). Pathogens 11:1082. doi: 10.3390/pathogens11101082
Paupy, C., Makanga, B., Ollomo, B., Rahola, N., Durand, P., Magnus, J., et al. (2013). Anopheles moucheti and Anopheles vinckei are candidate vectors of ape Plasmodium parasites, including Plasmodium praefalciparum in Gabon. PLoS One 8:e57294. doi: 10.1371/journal.pone.0057294
Pegha-Moukandja, I., Essone J C, B. B., Sagara, I., Kassa Kassa, R. F., Ondzaga, J., Lékana Douki, J. B., et al. (2016). Marked rise in the prevalence of asymptomatic Plasmodium falciparum infection in rural Gabon. PLoS One 11:e0153899. doi: 10.1371/journal.pone.0153899
Perkins, S. L., and Schall, J. (2002). A molecular phylogeny of malarial parasites recovered from cytochrome b gene sequences. J. Parasitol. 88, 972–978. doi: 10.1645/0022-3395(2002)088[0972:AMPOMP]2.0.CO;2
Posada, D., and Crandall, K. A. (1998). MODELTEST: testing the model of DNA substitution. Bioinformatics 14, 817–818. doi: 10.1093/bioinformatics/14.9.817
Pradines, B., Mamfoumbi, M. M., Parzy, D., Medang, M. O., Lebeau, C., Mbina, J. M., et al. (1998). In vitro susceptibility of Gabonese wild isolates of Plasmodium falciparum to artemether, and comparison with chloroquine, quinine, halofantrine and amodiaquine. Parasitology 117, 541–545. doi: 10.1017/S0031182098003400
Prugnolle, F., Durand, P., Neel, C., Ollomo, B., Ayala, F. J., Arnathau, C., et al. (2010). African great apes are natural hosts of multiple related malaria species, including Plasmodium falciparum. Proc. Natl. Acad. Sci. 107, 1458–1463. doi: 10.1073/pnas.0914440107
Prugnolle, F., Ollomo, B., Durand, P., Yalcindag, E., Arnathau, C., Elguero, E., et al. (2011). African monkeys are infected by Plasmodium falciparum nonhuman primate-specific strains. Proc. Natl. Acad. Sci. 108, 11948–11953. doi: 10.1073/pnas.1109368108
Prugnolle, F., Rougeron, V., Becquart, P., Berry, A., Makanga, B., Rahola, N., et al. (2013). Diversity, host switching and evolution of Plasmodium vivax infecting African great apes. Proc. Natl. Acad. Sci. 110, 8123–8128. doi: 10.1073/pnas.1306004110
Rahola, N., Makanga, B., Yangari, P., Jiolle, D., Fontenille, D., Renaud, F., et al. (2014). Description of Anopheles gabonensis, a new species potentially involved in rodent malaria transmission in Gabon, Central Africa. Infect. Genet. Evol. 28, 628–634. doi: 10.1016/j.meegid.2014.05.012
Rougeron, V., Boundenga, L., Arnathau, C., Durand, P., Renaud, F., and Prugnolle, F. (2022). A population genetic perspective on the origin, spread and adaptation of the human malaria agents Plasmodium falciparum and Plasmodium vivax. FEMS Microbiol. Rev. 46:fuab047. doi: 10.1093/femsre/fuab047
Schwöbel, B., Alifrangis, M., Salanti, A., and Jelinek, T. (2003). Different mutation patterns of atovaquone resistance to Plasmodium falciparum in vitro and in vivo: rapid detection of codon 268 polymorphisms in the cytochrome b as potential in vivo resistance marker. Malar. J. 2, 5–7. doi: 10.1186/1475-2875-2-5
Setiadi, W., Sudoyo, H., Trimarsanto, H., Sihite, B. A., Saragih, R. J., Juliawaty, R., et al. (2016). A zoonotic human infection with simian malaria, Plasmodium knowlesi, in Central Kalimantan, Indonesia. Malar. J. 15, 1–6. doi: 10.1186/s12936-016-1272-z
Sinka, M. E., Bangs, M. J., Manguin, S., Rubio-Palis, Y., Chareonviriyaphap, T., Coetzee, M., et al. (2012). A global map of dominant malaria vectors. Parasit. Vectors 5, 1–11. doi: 10.1186/1756-3305-5-69
Snounou, G., and Singh, B. (2002). “Nested PCR analysis of Plasmodium parasites” in Malaria methods and protocols. ed. Doolan, D. L. (New York: Springer), 189–203.
Subra, R. (1981). Biology and control of Culex pipiens quinquefasciatus* say, 1823 (Diptera, Culicidae) with special reference to Africa. Int. J. Trop. Insect Sci. 1, 319–338. doi: 10.1017/S1742758400000618
Tene Fossog, B., Kopya, E., Ndo, C., Menze-Djantio, B., Costantini, C., Njiokou, F., et al. (2012). Water quality and Anopheles gambiae larval tolerance to pyrethroids in the cities of Douala and Yaounde (Cameroon). J. Trop. Med. 2012, 1–10. doi: 10.1155/2012/429817
Verrelli, B. C., Alberti, M., Des Roches, S., Harris, N. C., Hendry, A. P., Johnson, M. T., et al. (2022). A global horizon scan for urban evolutionary ecology. Trends Ecol. Evol. 37, 1006–1019. doi: 10.1016/j.tree.2022.07.012
Keywords: malaria transmission, Anopheles diversity, plasmodium diversity, bridge vectors, Gabon
Citation: Longo-Pendy N-M, Boundenga L, Makanga BK, Mbou-Boutambe C, Bouafou L, Akone-Ella O, Nkoghe-Nkoghe LC, Lekana-Douki S, Lekana-Douki J-B and Kengne P (2023) A cross-sectional study of malaria transmission in suggests the existence of a potential bridge vector susceptible of ensuring the transfer of simian malaria parasites to humans. Front. Ecol. Evol. 11:1176687. doi: 10.3389/fevo.2023.1176687
Edited by:
Mauro Marrelli, University of São Paulo, BrazilReviewed by:
Rafael Gutiérrez López, Centro de Investigacao em Biodiversidade e Recursos Geneticos (CIBIO-InBIO), PortugalEmily Pascoe, Wageningen University and Research, Netherlands
Copyright © 2023 Longo-Pendy, Boundenga, Makanga, Mbou-Boutambe, Bouafou, Akone-Ella, Nkoghe-Nkoghe, Lekana-Douki, Lekana-Douki and Kengne. This is an open-access article distributed under the terms of the Creative Commons Attribution License (CC BY). The use, distribution or reproduction in other forums is permitted, provided the original author(s) and the copyright owner(s) are credited and that the original publication in this journal is cited, in accordance with accepted academic practice. No use, distribution or reproduction is permitted which does not comply with these terms.
*Correspondence: Neil-Michel Longo-Pendy, bG9uZ28ybWljaGVsQGdtYWlsLmNvbQ==; Larson Boundenga, Ym91bmRlbmdhQGdtYWlsLmNvbQ==
†These authors have contributed equally to this work