- Centro Tlaxcala de Biología de la Conducta, Universidad Autónoma de Tlaxcala, Tlaxcala, Tlaxcala, Mexico
Animals vocalize in particular ways in noise, presumably to increase the probability of detection; however, this idea has been seldom put to the test. Vermilion flycatchers produce longer songs in noisy territories. To test the hypothesis that longer songs increase the probability of detection in noise, we ran a field playback experiment on 30 free-living males. Each male was exposed to two treatments with the same songs, but one treatment had traffic noise and the other had no noise. Half of males were exposed to short songs (with four introductory elements – IE) and half to long songs (eight IE). If long songs have a higher probability of detection in noise, we expected that the experimental noise would have little effect when males heard long songs, but a larger effect when they heard short songs (i.e. significant interaction between song length and treatment). We measured call and flight responses, latencies to call and fly, and closest approach to the speaker. We also measured ambient traffic noise in the males’ territories to evaluate a possible association with their responses. Males did not respond differently to long vs short songs; more importantly, the predicted interaction between song length and treatment was not found. Our results, do not support the hypothesis that long songs are detected with a higher probability in noise. Interestingly, males increased their call response as ambient noise increased in their territories. Males also showed a non-significant trend to increase their flight respond toward long songs with experimental noise than to long songs without noise. Our results strongly suggests that noise amplifies territorial response, which may affect the outcome of territorial competition.
Introduction
Anthropogenic noise is a worldwide pollutant that negatively affects many organisms, from aquatic to terrestrial (Kunc and Schmidt, 2019). It has been linked to a lower reproductive success/investment in animals (e.g. Halfwerk et al., 2011a; Injaian et al., 2018; Bowen et al., 2020), which may be in part a result of impaired acoustic communication (reviewed in Brumm and Slabbekoorn, 2005; Patricelli and Blickley, 2006; Ríos-Chelén, 2009). Indeed, it has been shown that noise can change the way receivers respond to conspecific vocal signals (e.g. McMullen et al., 2014), and heterospecific vocal signals (e.g. Morris-Drake et al., 2017). Studies addressing the impact of urban noise on animal communication have dealt mostly with how noise shapes animal acoustic signals (an emitter perspective, reviewed in Patricelli and Blickley, 2006; Gil and Brumm, 2014). For example, some studies have found that animals produce longer acoustic signals (e.g. Lengagne et al., 1999; Brumm et al., 2004; Ríos-Chelén et al., 2013), higher pitched vocalizations (Slabbekoorn and Peet, 2003; Roca et al., 2016), or vocalizations with higher amplitude (Brumm and Zollinger, 2011) in noisy conditions. It is commonly assumed that these noise-related vocal adjustments are an adaptation to noise, by which animals increase the probability of detection in noisy conditions. However, comparatively few studies have looked at the receiver part of the communication process and tested this assumption. Some lab studies are from (Pohl et al. 2009; 2012; 2013). There are a few studies in the field (see below). The study by Templeton et al. (2016) showed that great tits (Parus major) increase the amplitude of their alarm calls (mean amplitude increase of 7.1 dB ± 1.7dB) in noisy conditions, but this amplitude increment is insufficient to restore communication between conspecifics in moderate levels of traffic noise. On the other hand, the study by Halfwerk et al. (2011b), with the same species, showed a perceptual benefit of increasing song pitch in noise during inter-sexual interactions; females emerged more often from their nests when they heard high pitched song types than low pitched ones in noisy conditions.
Noise masking is a common mechanism invoked to explain a reduced response to vocalizations in noise, but other explanations like getting distracted by noise are possible. Zhou et al. (2019) used noise that overlapped with the frequencies of superb fairy-wrens (Malurus cyaneus)’s alarm calls, and noise that did not overlap. They experimentally showed that a reduction in fleeing behavior after hearing alarm calls in noise was due noise masking, and not due other mechanisms like distraction and increased vigilance. Another study showed that male white-crowned sparrows (Zonotrichia leucophrys) living in a relatively noisy environment respond more strongly to songs with noise-related increased pitch than to comparatively lower pitched songs, suggesting higher pitched songs convey a perceptual benefit in relatively noisy conditions (Luther and Derryberry, 2012). A study by Luther and Magnotti (2012), using artificially synthesized songs of northern cardinals (Cardinalis cardinalis), showed that at low ambient noise levels the difference in male responses (songs) between relatively lower and higher pitched songs was relatively large. However, this difference in response decreased as ambient noise increased, suggesting high frequency songs may increase detection at noisy sites. Thus, most studies addressing whether noise-related changes in vocal signals convey a detection benefit in noise have addressed changes in the pitch of acoustic signals. Other noise-related changes in vocalizations, like temporal shifts and to a lesser extent changes in signal amplitude (see above Templeton et al., 2016) have been understudied. More studies are needed to have a better picture of whether noise-related changes (both spectral and temporal) in vocalizations represent a perceptual benefit in noise in wild populations.
In this study we aimed to test whether free-living receivers detect songs that are temporal-adjusted to noise (see below) (evaluated as territorial response) with a higher probability than songs that are not adjusted. Our study species, the vermilion flycatcher (Pyrocephalus rubinus) is a suboscine bird, with marked sexual dimorphism (e.g., males, but not females, have red chests). According to the TheCornellLab webpage (https://www.allaboutbirds.org/guide/Vermilion_Flycatcher/maps-range), vermilion flycatchers in the study site (see below) are year-round, but we have not monitored individuals throughout the year to confirm this. Males, being very territorial, readily defend their territories from conspecific intruders. They produce one song type that varies in the number of introductory elements – IE (Ríos-Chelén et al., 2005), an attribute that shows vocal flexibility (Ríos-Chelén et al., 2005; Ríos-Chelén et al., 2013). A previous study showed that vermilion flycatchers adjust a temporal attribute of their songs with noise; they sing longer songs (songs with more introductory elements) in noisier territories (Ríos-Chelén et al., 2013). This was interpreted as a possible strategy to deal with noise, by which birds produce songs with more elements (i.e. increasing the amount of acoustic stimuli, and redundancy) to increase the probability of detection in noise (Ríos-Chelén et al., 2013; see also Lengagne et al., 1999; Brumm et al., 2004). To test this hypothesis, we conducted a playback experiment simulating an intruder in the focal male’s territory, and exposing males to different treatments differing in song length, and presence/absence of experimental traffic noise. We expected that if males detect long songs with a higher probability than short songs in noisy conditions, the difference in territorial response (i.e. call and flight response, in latencies to call and fly and in closest approach) between the noise and the no noise treatment should be larger when males hear short songs than long songs. That is, experimental noise should affect males’ perception more when they hear short songs than long songs (i.e. interaction effect between song length and treatment). Because some studies have found that ambient noise can influence the territorial responses of birds (e.g. Grabarczyk and Gill, 2019), we also measured ambient noise levels in the territories of the focal males.
Materials and methods
Study area and subjects
This study was conducted during the breeding season of vermilion flycatchers (Ríos-Chelén et al., 2005), from April 15th to May 30th 2019, and from around 8:30 a.m. to 12:00 p.m., at the Parque Ecológico Revolución Mexicana (19°01’50.3”N, 98°11’8.3”W), an urban park located in the city of Puebla, Mexico. The Parque Ecológico is a 58 hectare park, including a lake, three botanical gardens, green space, and unpaved (dirt) roads that people use to exercise. The park is surrounded by busy avenues (avenues transited by motor vehicles). We conducted a playback experiment on 30 different vermilion flycatcher males (see below). Before the experiment, in March and early April, we made behavioral observations of the males living in the park. These included male-male vocal interactions where the territory owner called to, in close distance (around 3 meters) with, another male. In this species males defend their territories from conspecific intruders by calling (e.g. Ríos-Chelén and Macías Garcia, 2007; Rivera-Cáceres et al., 2011). We also observed flights from tree to tree where the focal male seemed to be patrolling its territory, and the trees where the focal male spent time feeding (e.g. catching flies and other insects). Instances where the territory owner is involved in physical and violent contact with another male, at the territory boundary, are rare but do occur (AAR-C pers. obs.). We also noted from which trees males sang before dawn. During the breeding season males sing before dawn to presumably defend their territories, or perhaps attract an extra-pair female, and they switch from a few (2–3) different trees as they seem to address different neighbors (AAR-C pers. obs., Ríos-Chelén et al., 2005). These observations allowed us to map males’ territories. We identified males by the territories they occupied and their song perches before dawn. This identification method has proven effective for individual identification in this species because previous studies showed that the same male (identified by color bands) typically use the same song perches, are recaptured near the same song perches in different days and years and some males have been seen to use the same territories for several years (in some cases 4 years, in one case more than 6 years) (Ríos-Chelén et al., 2005; Ríos-Chelén et al., 2013).
Playback experiment and behavioral measures
To test whether long songs have a higher probability of detection than short songs in noisy conditions, we conducted a playback experiment simulating an intruder in the focal male’s territory. We could have experimentally varied song length within males, keeping the experimental noise treatment constant within males. However, previous research suggests that long songs may be perceived as more threatening signals than short songs (Ríos-Chelén and Macías Garcia, 2007). Thus, a result supporting our hypothesis (i.e. stronger response to long songs in noise than to short songs in noise) could be confused by males perceiving long songs as more threatening. Therefore, we followed a different approach that eliminates this potential source of confusion and enables us to directly test our hypothesis. Instead of exposing each male to songs differing in song length, each male exposed twice with the same song length (long or short, see below), but one time with experimental noise (noise treatment) and one time without noise (no noise treatment); that is, the only variable that differed within males was presence/absence of experimental noise, and song length was controlled within males. Half of the males (15) were exposed to long songs (with 8 introductory elements – IE), and half of them to short songs (4 IE). This design allowed us to compare the response of the same male with itself toward the same song (e.g. short) in two different noise conditions (with experimental noise vs. without experimental noise). Half of the males (15) were exposed first to the treatment of songs with noise and then to the treatment of songs without noise, and half of them with the inverse order. We waited at least 20min (range: 20–25min) between each trial to allow males to return to normal behavior after the simulated intrusion (Ríos-Chelén et al., 2018a). Each trial consisted of a pre-playback minute of silence, followed by a playback minute of broadcast songs (with or without noise), followed by a post-playback minute of silence. One minute playback is enough to make vermilion flycatchers respond (Ríos-Chelén and Macías Garcia, 2007, see also the Results section). Because our objective was to compare males’ responses toward different song lengths and toward songs with and without experimental noise, we did not use another type of control like a pure noise stimulus. Instead, we had two other controls. The first control was a pre-playback minute with no sound broadcast (see above), that, when compared to the playback + post-playback minutes, allowed us to evaluate whether males responded to our playback or not. Because our experimental design was a repeated measures design that allowed us to compare the same individual with himself, the second control was within males; that is, the absence of experimental noise as one of the treatments. This allowed us to evaluate whether the same individual changed its behavior when he heard songs without experimental noise vs the same songs with experimental noise. For an explanation on how we analyzed our data see Data analyses. For other studies that have used designs without “extra” controls see e.g. Brumm et al., 2004; Brumm et al., 2009; Purser and Radford, 2011; McMullen et al., 2014). During the pre-playback and post-playback minutes of silence males were exposed to the ambient noise in their territories. Vermilion flycatchers sing in song bouts (Rivera-Cáceres et al., 2011); to mimic the way males sing, the playback minute consisted of song bouts. Each song was copied and pasted 16 times conforming four bouts of four songs each (16 songs/min, which falls within the natural song rate in this species, Ríos-Chelén et al., 2005; Rivera-Cáceres et al., 2011). Each bout had a duration of 10.9s (total duration of bouts 43.816s), and each of three interspaces between bouts lasted 5.3s. These 1min playback parameters were held constant across treatments.
During the pre-playback minute we recorded ambient noise levels (which was mostly of anthropogenic origin) using a sound level meter (SEW 2310 SL, range 30–130 dB, weight A, fast response, ANSI S1, class II). We pointed the sound level meter toward the sky and recorded one measure of environmental noise every 10 s, for 1 minute (6 measurements). During each minute (pre-, play-, and post-playback), we recorded the following behavioral measures: number of calls and flights from perch to perch. We counted a flight between perches as any flight from a perch where the male did not come back to the same original perch, and this normally included flights between perches of at least 1m, usually more, apart. We also recorded the latencies to call and fly (in seconds, time that took a male to start calling or flying from perch to perch, respectively, since the start of the playback) with a chronometer. Finally, we also recorded the closest approach to the speaker (m) with a tape measure. To playback the sound stimuli we used a Mineroff SME-AFS speaker (frequency response 100Hz–12 kHz) connected with 10 m cable to a Samsung Galaxy S8 cellular phone. The speaker was placed on a tree around 1m high or on the ground (if no tree was available), around 10m from the focal bird and pointing it toward him. The two times the focal male was tested (one with experimental noise and one without experimental noise) the speaker was located in the same place. Placing the speaker at 1m, or on the ground, may lead to some sound degradation, and does not represent a realistic song perch height for vermilion flycatchers. Thus, although our results show that vermilion flycatchers responded to our playback, suggesting they perceived our treatments as realistic (see Results), we think our results should be taken with caution. Two observers were hiding behind a tree or bushes 10m away from the speaker, recording the focal male’s behavior. It was not possible to obtain data blind because the observers could hear whether the played back treatment was the noise or the no noise treatment. This experimental design has proven effective to detect behavioral differences among different acoustic stimuli in vermilion flycatchers (Ríos-Chelén and Macías Garcia, 2007; Ríos-Chelén et al., 2018a).
Preparation of sound stimuli
To prepare the song stimuli we used vermilion flycatcher songs recorded in 2001 and 2015 in another population, 30km away from the studied population (see Ríos-Chelén et al., 2005, and Nakamura-Garcia and Ríos-Chelén, 2022 for details). We used Raven Pro, v.1.5 (www.birds.cornell.edu/raven) to select 30 good quality songs (with a high signal to noise ratio), each from a different male. Half of these songs (15) were long songs (with 8 introductory elements – IE), and half short songs (4 IE). We cleaned the selected songs from background noise by selecting, with the cursor, the lower part of the spectrogram which captured most background noise, but no song, and later removed the selected section with the “filter out active selection” function in Raven (we used Raven default settings like Kaiser window, and stop band attenuation of 100dB, see Ríos-Chelén et al., 2017). Following a similar experiment, each song was normalized (McMullen et al., 2014) to 0dB in Audacity v. 2.1.3 (see also Brumm, 2004; Nemeth and Brumm, 2009 for studies that have normalized to 0dB). Although normalizing to 0dB (instead of for instance to −1dB) has been common practice, this procedure may potentially result in brief overloading and loss of sound quality during playback. This means that, although males responded in a natural way (calling, singing, approaching) to our playback (see Results), suggesting males perceived our treatments as realistic, we cannot be completely sure about this, and our results should be taken cautiously. Normalization results in all songs’ peak amplitude being standardized. The recorded songs had a mean ± SE of 68.7 ± 1.1dB, as measured in Raven with the Avg Power function. These songs, and noise stimuli (see below), were used to prepare the playback minute (see Experiment and behavioral measures for details). The urban noise that we used to prepare the treatments was recorded, using a microphone (Sennheiser K6/ME66) connected to a Marantz digital recorder (PMD221, 16 bit, 44.1 kHz) pointing toward the busy avenue from ca. 1m, from eight different points from two busy avenues in the city of Puebla. This resulted in eight sound files of 15 min each. From these files we selected 30 high-quality 1-min segments (relatively homogenous amplitude with no bird sounds). These segments were copied and saved into individual files. We normalized the 1min noise segments to 0dB in Audacity. As for songs (see above), to know the amplitude of the recorded noise we selected with the cursor in Raven each whole noise file (1min length, from 0 to 22kHz) and automatically obtained the amplitude with the Avg Power function in Raven. Noise files had a mean ± SE of 76.2 ± 0.35dB. Thus, the signal to noise ratio of our treatments (mean amplitude of songs/mean amplitude of noise) was 0.90, which is equivalent to a vermilion flycatcher singing in a higher traffic noise level than its own song. This situation is common in several males defending territories close to busy avenues in the study site.
To prepare the songs with background noise we first randomly selected a song file prepared as mentioned above, and a noise file. We used the function “mix and generate new track” in Audacity to mix the song and noise into a single sound file (McMullen et al., 2014; Zwart et al., 2016). The sound stimuli (songs and noise combined for the noise treatment, songs only for the no noise treatment) were calibrated to 65–70 dB SPL measured at an 8m distance; previous studies show that vermilion flycatchers respond appropriately (e.g. calling, singing, approaching) to this song amplitude (Ríos-Chelén and Macías Garcia, 2007; Rivera-Cáceres et al., 2011). Calibration was done using the same sound level meter that we used to record ambient noise levels (see above).
Data analyses
To evaluate whether males responded to our treatments we ran a paired t-test comparing their call and flight rates between the pre-playback period (baseline state) and the playback + post-playback period. We combined the playback and post-playback minutes because vermilion flycatchers do not stop responding to the playback immediately after the playback has stopped (Ríos-Chelén and Macías Garcia, 2007). Thus, by including the post-playback minute (when males are still responding) with the playback minute we can have a more complete measure of response. Because we used rates (see above) time (1 min of pre-playback, 2 min of playback + post-playback) was controlled.
To obtain measures of territorial response, we subtracted the call and flight rates during the pre-playback minute from that of the playback + post-playback minutes; this allowed us to obtain a difference in activity rate between the pre-playback and the playback + post-playback periods (hereafter, call and flight response, which are continuous variables) that was used in further analyses. This methodology has been previously useful to show subtle differences in territorial responses in vermilion flycatchers (Ríos-Chelén and Macías Garcia, 2007; Ríos-Chelén et al., 2018a). To evaluate whether our response variables (call and flight responses, latencies to call and fly, closest approach) were affected by our treatments (experimental noise, no experimental noise), by the ambient noise in each male’s territory, and by song length, we ran Generalized Estimating Equations (GEE), a type of Generalized Linear Model that allows for repeated measures, and which makes no a priori assumption on data distribution (Pan, 2001). For call and flight responses and closest approach the models had a normal distribution with identity link. For latencies to call and fly we used gamma distribution with log link. In some cases (two individuals) it was difficult to accurately measure latency to call because males immediately called after playback. Their latency to call fell somewhere between 0 and 1 seconds. For these males, we scored latency to call as 0.5 seconds. Preliminary analyses revealed that our response variables were not highly inter-correlated (Spearman rank correlation, r<0.25, r>−0.34, in all); therefore, we analyzed each variable separately. We declared treatment as a within male repeated factor to account for the fact that each male was exposed to the two treatments (songs with and without experimental noise), song length as fixed factor, and ambient noise and time of day as covariates. Because we hypothesized that vermilion flycatchers sing longer songs in noisier places (Ríos-Chelén et al., 2013) to improve song perception of longs songs over short ones in noisy conditions, we expected that the experimental noise would affect the detection of short songs more than the detection of long songs; therefore, we added in the model an interaction term between song length and treatment. Because it is possible that ambient noise could also affect males’ response to our treatments, we also included an interaction term between ambient noise and treatment. All analyses were two-tailed and performed in SPSS.
Results
Males showed a common territorial response toward our playbacks; that is, a higher activity rate (calls, flights looking for the simulated intruder) during the playback + post-playback period relative to the pre-playback minute (paired t test; calls, t= −8.75, df= 29, P= 0.001; flights, t= −6.12, df= 29, P= 0.001), showing that they responded to our playback, and suggesting that they perceived our playback as realistic.
Contrary to our prediction, we found no significant interaction between song length and treatment for any of our response variables (Table 1). For flight response we found a non-significant tendency of an interaction between song length and treatment (P= 0.056, Table 1); however, this non-significant trend was the opposite to what we predicted: males tended to show a greater flight response toward long songs with artificial noise than without noise, and no effect of treatment was seen for short songs (Figure 1). We also found that, regardless of the treatment, males increased their flight response with the time in the morning (Figure 2; Table 1). No other parameter was associated with flight response (Table 1). Song length had no significant effect on males’ territorial responses (Table 1). Males produced a greater call response as ambient noise increased in their territories (Figure 3; Table 1); no other variable was associated with call response (Table 1). Latencies to call and fly, and closest approach, were not associated with any of our explanatory variables (Table 1).
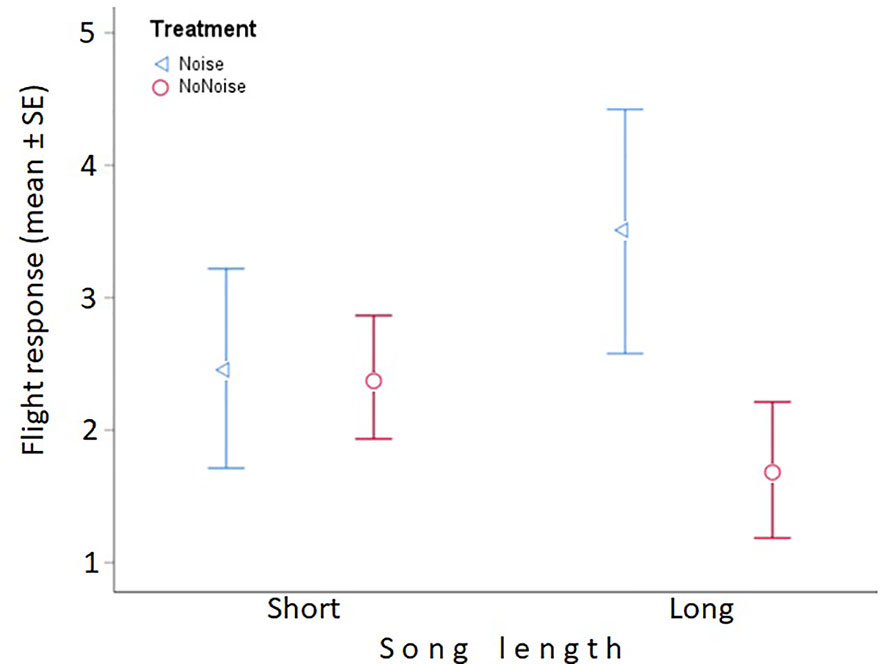
Figure 1 Flight response, song length, and treatment. Males showed a non-significant trend to mount a greater flight response toward long songs with experimental noise (blue triangles) than to long songs with no experimental noise (red circles) (right side of the plot). This non-significant trend was not seen for short songs (left side of the plot). n = 15 males in each group. For details on flight response see text.
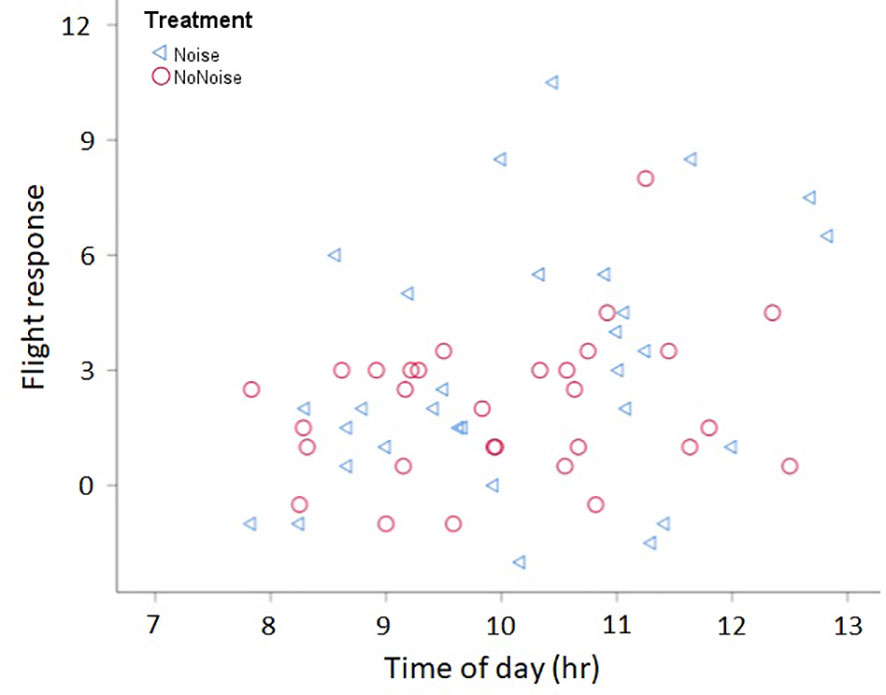
Figure 2 Flight response and morning time. Regardless of the treatment (with noise in blue triangles, without noise in red circles), males showed a stronger flight response as the time passed by in the morning. Time in hours (hr; i.e. 7.5 hr = 7:30AM). For details on flight response see text.
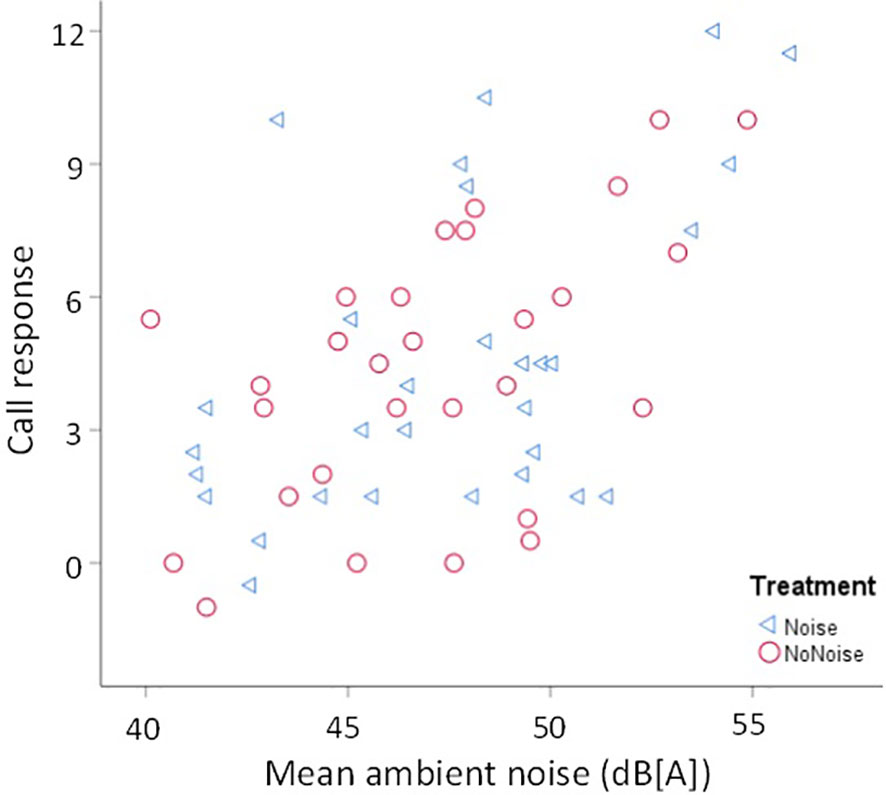
Figure 3 Ambient noise and call response to simulated intrusions in vermilion flycatchers. Males responded with a stronger call response when they heard songs, with experimental noise (blue triangles) or without experimental noise (red circles), as ambient noise increased in their territories. For details on call response see text.
Discussion
Males readily responded to our playback, suggesting that our treatments were perceived as realistic. However, we found no evidence to support the hypothesis that males sing longer songs in noise to improve detectability (Ríos-Chelén et al., 2013, see below).
When it comes to call response, latencies to call and fly, and closest approach, we found no evidence that males responded differentially when they heard long and short songs, with or without experimental noise, and the predicted interaction between song length and treatment was not observed either. We found a non-significant trend for males to show a greater flight response toward long songs with experimental noise than without noise (see below). However, contrary to our prediction, this result is in line with the idea that experimental noise increases the territorial response of males. Therefore, our results do not support the hypothesis that vermilion flycatchers benefit from increased detectability by emitting longer songs in noisy conditions.
It is possible that noise did not alter the perception of short and long songs differentially because of the relatively short distance at which we exposed males to our playbacks (around 10m). However, this distance is biological relevant as it falls well within the observed distance between males in acoustic territorial interactions in this species (Ríos-Chelén pers. obs.). Nevertheless, we cannot discard the possibility that long songs may render a perceptual benefit at larger distances. If, apparently, longer songs do not improve detection, why is it that vermilion flycatchers produce longer songs in noisy conditions (Ríos-Chelén et al., 2013)? Although some of the energy of traffic noise is found above 2kHz, most of its energy is below 2kHz (see Figure 2 in Ríos-Chelén et al., 2018b); this may render the songs of vermilion flycatchers, whose mean minimum frequency is around 2.9 kHz (Ríos-Chelén et al., 2013), as largely unmasked. Therefore, it is possible that traffic noise does not mask the frequencies of vermilion flycatcher songs to an extent that precludes adequate perception, so that both short and long songs are perceived sufficiently well both in quiet and noisy conditions. On the other hand, a possible functional explanation of males singing longer songs in noise, could be related with females. Our study does not discard the possibility that females’ ability to detect different song durations may differ in different noise levels. Although not addressing song durations, but song frequencies, the study by Halfwerk et al. (2011b) showed that great tit (Parus major) males experience reproductive benefits by singing low pitched songs, but these songs are also less efficient for male–female communication in noise. Templeton et al. (2016) showed great tits (Parus major) increase the amplitude of their calls with noise, but that this increase is insufficient to make their calls more easily heard in noise. Contrary to these investigations (Halfwerk et al., 2011b; Templeton et al., 2016), our study showed no evidence of differential detection by vocalizing in a particular way (i.e. longer songs) in noise.
Singing longer songs in noisier territories may also be a by-product of differential settlement in the territories. A study by MacDougall-Shackleton et al. (2009) found that male song sparrows (Melospiza melodia) that sang more complex songs showed a lower stress response (i.e. a smaller increase in circulating glucocorticoids after a stressing event) than males that produced less complex songs, and males with a weaker stress response had a higher probability of survival. Therefore, we can speculate that vermilion flycatchers that sing longer songs may be more capable of coping with stressful noise, and occupy territories with elevated noise levels first. Other studies have found that song characteristics are associated with age (e.g. Gil et al., 2001; Garamszegi et al., 2005; De Kort et al., 2009). Therefore, another possibility is that song length in vermilion flycatchers is related with age/experience, and those males occupying noisier territories are older/more experienced males. The idea that males preferentially occupy noisier territories seems counterintuitive at first; it requires that noisy territories are in some way preferred by males. This could be the case if, for instance, these territories have a lower probability of nest predation (Francis et al., 2009).
As opposed to what we predicted, males showed a non-significant tendency to show a greater flight response toward long songs with experimental noise than without experimental noise. Along these lines, we also observed that males mounted a stronger call response as ambient noise increased in their territories. Therefore, we provide correlative evidence (for calls) suggesting that noise amplifies the territorial response of vermilion flycatchers, and experimental evidence (for flights, although only a non-significant trend) that goes in line with the idea that noise amplifies the territorial response. From a proximate view, it is not clear why this pattern (i.e. increased territorial response with noise) emerged. One possibility is that noise impairs signal detection and males need to get closer to the emitter to extract the emitted information, increasing the probability of an escalated interaction (Grabarczyk and Gill, 2019). However, and as discussed above, the fact that we found no differences in response between our treatments and interactions in predicted ways, including latency responses (a measure of detectability, Grabarczyk and Gill, 2019), does not support this idea. Another possibility is that males in noisier territories are more stressed (with elevated corticosterone metabolites) (Blickley et al., 2012, but see Injaian et al., 2020), resulting in over-reactive individuals (Rozempolska-Rucińska et al., 2020), than those less exposed to noise. Regardless of the mechanism, ambient noise may influence territorial interactions (this study), potentially increasing the probability of physical injuries and the outcome of territorial competition. Although not very frequently, males have been seen in direct physical, and violent, contact during territorial interactions (AARC, pers. obs). Another study found that experimental noise induces song modifications in European robins (Erithacus rubecula) when responding to simulated intruders (McMullen et al., 2014). Other studies have found that noise may decrease (Kleist et al., 2016; Zwart et al., 2016; Lenis and Guillermo-Ferreira, 2020) or increase (Phillips and Derryberry, 2018; Grabarczyk and Gill, 2019; Wolfenden et al., 2019; Akçay et al., 2020) territorial responses. More studies are necessary to better understand the effect that urban noise may have during territorial interactions in organisms.
We also found that, regardless of the treatment, flight response increased with morning time. This suggests that territorial interactions may be more intense as midday approaches. The reason behind this patten is not known. However, we can speculate that this result may be in part related to time partitioning of different behaviors throughout the day; individuals may be more active feeding in the early morning (e.g. Hutto, 1981), relegating other activities, such as territory patrolling and actively defending the territory (e.g. flying to search for intruders), as midday approaches. This and other ideas could be further studied in the future. In any case, time of day may be an important variable to take into account in future studies addressing the impact of urban contaminants on territorial interactions.
In conclusion, we found no evidence that longer songs have a higher probability of detection in noise, showing that it is important to test assumptions on song adaptation to urban environments. However, we found evidence that noise increases territorial response, suggesting it may influence territorial interactions, possibly influencing the outcome of territorial competition.
Data availability statement
The original contributions presented in the study are included in the article/Supplementary Material. Further inquiries can be directed to the corresponding author.
Ethics statement
This research was conducted with the permission of the administration of the Parque Ecológico Revolución Mexicana.
Author contributions
AR-C conceived the study. NC-M prepared the acoustic stimuli. NC-M and SJ-R ran the experiment and obtained the data. NC-M and AR-C analyzed the data and wrote the manuscript. All authors gave feedback and agree with the final content of the work.
Funding
NC-M and SJ-R were recipients of Master scholarships from the Consejo Nacional de Ciencia y Tecnología (CONACYT, México). This study stems from NECM thesis.
Acknowledgments
We are grateful to Margarita Martínez, Eira Bermúdez-Cuamatzin, Amando Bautista, Luis E Osorio C, Cesar Cerón G, and the Centro Tlaxcala de Biología de la Conducta for logistic support. We are grateful with three reviewers that improved this article with their comments.
Conflict of interest
The authors declare that the research was conducted in the absence of any commercial or financial relationships that could be construed as a potential conflict of interest.
Publisher’s note
All claims expressed in this article are solely those of the authors and do not necessarily represent those of their affiliated organizations, or those of the publisher, the editors and the reviewers. Any product that may be evaluated in this article, or claim that may be made by its manufacturer, is not guaranteed or endorsed by the publisher.
Supplementary material
The Supplementary Material for this article can be found online at: https://www.frontiersin.org/articles/10.3389/fevo.2023.1175732/full#supplementary-material
References
Akçay Ç., Kağan Porsuk Y., Avşar A., Çabuk D., Can Bilgin C. (2020). Song overlapping, noise, and territorial aggression in great tits. Behav. Ecol. 31, 807–814. doi: 10.1093/beheco/araa030
Blickley J. L., Word K. R., Krakauer A. H., Phillips J. L., Sells S. N., Taff C. C., et al. (2012). Experimental chronic noise is related to elevated fecal corticosteroid metabolites in lekking male greater sage-grouse (Centrocercus urophasianus). PLoS One 7, e50462. doi: 10.1371/journal.pone.0050462
Bowen A. E., Gurule-Small G. A., Tinghitella R. M. (2020). Anthropogenic noise reduces male reproductive investment in an acoustically signaling insect. Behav. Ecol. Sociobiol. 74, 103. doi: 10.1007/s00265-020-02868-3
Brumm H. (2004). The impact of environmental noise on song amplitude in a territorial bird. J. Anim. Ecol. 73, 434–440. doi: 10.1111/j.0021-8790.2004.00814.x
Brumm H., Schmidt R., Schrader L. (2009). Noise-dependent vocal plasticity in domestic fowl. Anim. Behav. 78, 741–746. doi: 10.1016/j.anbehav.2009.07.004
Brumm H., Slabbekoorn H. (2005). Acoustic communication in noise. Adv. Stud. Behav. 35, 151–209. doi: 10.1016/S0065-3454(05)35004-2
Brumm H., Voss K., Köllmer I., Todt D. (2004). Acoustic communication in noise: regulation of call characteristics in a New World monkey. J. Exp. Biol. 207, 443–448. doi: 10.1242/jeb.00768
Brumm H., Zollinger S. A. (2011). The evolution of the Lombard effect: 100 years of psychoacoustic research. Behaviour 148, 173–1198. doi: 10.1163/000579511X605759
De Kort S. R., Eldermire E. R. B., Valderrama S., Botero C. A., Vehrencamp S. L. (2009). Trill consistency is an age-related assessment signal in banded wrens. Proc. R. Soc. B. 276, 2315–2321. doi: 10.1098/rspb.2009.0127
Francis C. D., Ortega C. P., Cruz A. (2009). Noise pollution changes avian communities and species interactions. Curr. Biol. 19, 1415–1419. doi: 10.1016/j.cub.2009.06.052
Garamszegi L. Z., Heyle D., Møller A. P., Eens M., de Lope F. (2005). Age-dependent health status and song characteristics in the barn swallow. Behav. Ecol. 16, 580–591. doi: 10.1093/beheco/ari029
Gil D., Brumm H. (2014). “Acoustic communication in the urban environment: patterns, mechanisms, and potential consequences of avian song adjustments,” in Avian urban ecology. Eds. Gil D., Brumm H. (Oxford: Oxford University Press), 69–83.
Gil D., Cobb J. L. S., Slater P. J. B. (2001). Song characteristics are age dependent in the willow warbler, Phylloscopus trochilus. Anim. Behav. 62, 689–694. doi: 10.1006/anbe.2001.1812
Grabarczyk E. E., Gill S. A. (2019). Anthropogenic noise affects male house wren response to but not detection of territorial intruders. PLoS One 14, e0220576. doi: 10.1371/journal.pone.0220576
Halfwerk W., Botb S., Buikxa J., van der Velde M., Komdeur J., ten Cate C., et al. (2011b). Low-frequency songs lose their potency in noisy urban conditions. P. Natl. Acad. Sci. U.S.A. 108, 14549–14554. doi: 10.1073/pnas.1109091108
Halfwerk W., Holleman L. J. M., Lessells C. M., Slabbekoorn H. (2011a). Negative impact of traffic noise on avian reproductive success. J. Appl. Ecol. 48, 210–219. doi: 10.1111/j.1365-2664.2010.01914.x
Hutto R. L. (1981). Temporal patterns of foraging activity in some wood warblers in relation to the availability of insect prey. Behav. Ecol. Sociobiol. 9, 195–198. doi: 10.1007/BF00302937
Injaian A., Francis C., Ouyang J., Dominoni D., Donald J., Fuxjager M., et al. (2020). Baseline and stress-induced corticosterone levels across birds and reptiles do not reflect urbanization levels. Conserv. Physiol. 8, coz110. doi: 10.1093/conphys/coz110
Injaian A. S., Poon L. Y., Patricelli G. L. (2018). Effects of experimental anthropogenic noise on avian settlement patterns and reproductive success. Behav. Ecol. 29, 1181–1189. doi: 10.1093/beheco/ary097
Kleist N. J., Guralnick R. P., Cruz A., Francis C. D. (2016). Anthropogenic noise weakens territorial response to intruder’s songs. Ecosphere 7, e01259. doi: 10.1002/ecs2.1259
Kunc H. P., Schmidt R. (2019). The effects of anthropogenic noise on animals: a meta-analysis. Biol. Lett. 15, 20190649. doi: 10.1098/rsbl.2019.0649
Lengagne T., Aubin T., Lauga J., Jouventin P. (1999). How do king penguins (Aptenodytes patagonicus) apply the mathematical theory of information to communicate in windy conditions? Proc. R. Soc. Lond. B. 266, 623–1628. doi: 10.1098/rspb.1999.0824
Lenis P. R., Guillermo-Ferreira R. (2020). Effect of noise on behavioural response to simulated territorial intrusion in the great kiskadee (Pitangus sulphuratus). Urban. Ecosyst. 23, 93–96. doi: 10.1007/s11252-019-00906-1
Luther D. A., Derryberry E. P. (2012). Birdsongs keep pace with city life: changes in song over time in an urban songbird affects communication. Anim. Behav. 83, 1059–1066. doi: 10.1016/j.anbehav.2012.01.034
Luther D., Magnotti J. (2012). Can animals detect differences in vocalizations adjusted for anthropogenic noise? Anim. Behav. 92, 111–116. doi: 10.1016/j.anbehav.2014.03.033
MacDougall-Shackleton S. A., Dindia L., Newman A. E. M., Potvin D. A., Stewart K. A., MacDougall-Shackleton E. A. (2009). Stress, song and survival in sparrows. Biol. Lett. 5, 746–748. doi: 10.1098/rsbl.2009.0382
McMullen H., Schmidt R., Kunc H. P. (2014). Anthropogenic noise affects vocal interactions. Behav. Processes. 103, 125–128. doi: 10.1016/j.beproc.2013.12.001
Morris-Drake A., Bracken A. M., Kern J. M., Radford A. N. (2017). Anthropogenic noise alters dwarf mongoose responses to heterospecific alarm calls. Environ Pollut. 223, 476–483. doi: 10.1016/j.envpol.2017.01.049
Nakamura-Garcia M. T., Ríos-Chelén A. A. (2022). More than noise: light, moon phase, and singing behavior in a passerine. Urban. Ecosyst. 25, 291–303. doi: 10.1007/s11252-021-01142-2
Nemeth E., Brumm H. (2009). Blackbirds sing higher-pitched songs in cities: adaptation to habitat acoustics or side-effect of urbanization? Anim. Behav. 78, 637-641. doi: 10.1016/j.anbehav.2009.06.016
Pan W. (2001). Akaike’s information criterion in generalized estimating equations. Biometrics 57, 120–125. doi: 10.1111/j.0006-341X.2001.00120.x
Patricelli G. L., Blickley J. L. (2006). Avian communication in urban noise: causes and consequences of vocal adjustment. Auk 123, 639–649. doi: 10.1093/auk/123.3.639
Phillips J. N., Derryberry E. P. (2018). Urban sparrows respond to a sexually selected trait with increased aggression in noise. Sci. Rep. 8, 7505. doi: 10.1038/s41598-018-25834-6
Pohl N. U., Leadbeater E., Slabbekoorn H., Klump G. M., Langemann U. (2012). Great tits in urban noise benefit from high frequencies in song detection and discrimination. Anim. Behav. 83, 711–721. doi: 10.1016/j.anbehav.2011.12.019
Pohl N. U., Slabbekoorn H., Klump G. M., Langemann U. (2009). Effects of signal features and environmental noise on signal detection in the great tit, Parus major. Anim. Behav. 78, 1293–1300. doi: 10.1016/j.anbehav.2009.09.005
Pohl N. U., Slabbekoorn H., Neubauer H., Heil P., Klump G. M., Langemann U. (2013). Why longer song elements are easier to detect: threshold level-duration functions in the great tit and comparison with human data. J. Comp. Physiol. A 199, 239–252. doi: 10.1007/s00359-012-0789-z
Purser J., Radford A. N. (2011). Acoustic noise induces attention shifts and reduces foraging performance in three-spined sticklebacks (Gasterosteus aculeatus). PLoS One 6, e17478. doi: 10.1371/journal.pone.0017478
Ríos-Chelén A. A. (2009). Bird song: the interplay between urban noise and sexual selection. Oecol. Brasil. 13, 153–116. doi: 10.4257/oeco.2009.1301.12
Ríos-Chelén A. A., Crisanto-Téllez L. G., Quiros-Guerrero E., Rivera-Caceres K. D. (2018a). Territorial responses to song components in a suboscine, the vermilion flycatcher. Behav. Process. 157, 478–483. doi: 10.1016/j.beproc.2018.06.012
Ríos-Chelén A. A., Cuatianquiz-Lima C., Bautista A., Martínez-Gómez M. (2018b). No reliable evidence for immediate noise-induced song flexibility in a suboscine. Urban. Ecosyst. 21, 15–25. doi: 10.1007/s11252-017-0690-1
Ríos-Chelén A. A., Macías Garcia C. (2007). Responses of a sub-oscine bird during playback: effects of different song variants and breeding period. Behav. Process. 74, 319–325. doi: 10.1016/j.beproc.2006.11.007
Ríos-Chelén A. A., Macías-García C., Riebel K. (2005). Variation in the song of a sub-oscine, the vermilion flycatcher. Behaviour 142, 1121–1138. doi: 10.1163/156853905774405326
Ríos-Chelén A. A., McDonald A. N., Berger A., Perry A. C., Krakauer A. H., Patricelli G. L. (2017). Do birds vocalize at higher pitch in noise, or is it a matter of measurement? Behav. Ecol. Sociobiol. 71, 29. doi: 10.1007/s00265-016-2243-7
Ríos-Chelén A. A., Quirós-Guerrero E., Gil D., Macías-García C. (2013). Dealing with urban noise: vermilion flycatchers sing longer songs in noisier territories. Behav. Ecol. Sociobiol. 67, 145–152. doi: 10.1007/s00265-012-1434-0
Rivera-Cáceres K., Macías Garcia C., Quirós-Guerrero E., Ríos-Chelén A. A. (2011). An interactive playback experiment shows song bout size discrimination in the suboscine vermilion flycatcher (Pyrocephalus rubinus). Ethology 117, 1120–1127. doi: 10.1111/j.1439-0310.2011.01968.x
Roca I. T., Desrochers L., Giacomazzo M., Bertolo A., Bolduc P., Deschesnes R., et al. (2016). Shifting song frequencies in response to anthropogenic noise: a meta-analysis on birds and anurans. Behav. Ecol. 27, 1269–1274. doi: 10.1093/beheco/arw060
Rozempolska-Rucińska I., Czech A., Kasperek K., Zięba G., Ziemiańska A. (2020). Behaviour and stress in three breeds of laying hens kept in the same environment. S. Afr. J. Anim. Sci. 50, 272–280. doi: 10.4314/sajas.v50i2.10
Slabbekoorn H., Peet M. (2003). Birds sing at higher pitch in urban noise. Nature 426, 267–267. doi: 10.1038/424267a
Templeton C. N., Zollinger S. A., Brumm H. (2016). Traffic noise drowns out great tit alarm calls. Curr. Biol. 26, R1167–R1176. doi: 10.1016/j.cub.2016.09.058
Wolfenden A. D., Slabbekoorn H., Kluk. K., de Kort S. R. (2019). Aircraft sound exposure leads to song frequency decline and elevated aggression in wild chiffchaffs. J. Anim. Ecol. 88, 1720–1731. doi: 10.1111/1365-2656.13059
Zhou Y., Radford A. N., Magrath R. D. (2019). Why does noise reduce response to alarm calls? Experimental assessment of masking, distraction and greater vigilance in wild birds. Funct. Ecol. 33, 1280–1289. doi: 10.1111/1365-2435.13333
Keywords: traffic noise, territorial response, bird song, suboscine, acoustic communication, Pyrocephalus rubinus, sexual selection
Citation: Chavez-Mendoza NE, José-Ramírez S and Ríos-Chelén AA (2023) Evidence that traffic noise increases territorial response in vermilion flycatchers. Front. Ecol. Evol. 11:1175732. doi: 10.3389/fevo.2023.1175732
Received: 28 February 2023; Accepted: 17 July 2023;
Published: 01 August 2023.
Edited by:
Javier delBarco-Trillo, University College Cork, IrelandReviewed by:
Christopher B. Sturdy, University of Alberta, CanadaBeate Anna Apfelbeck, University of Salzburg, Austria
Luis Sandoval, University of Costa Rica, Costa Rica
Copyright © 2023 Chavez-Mendoza, José-Ramírez and Ríos-Chelén. This is an open-access article distributed under the terms of the Creative Commons Attribution License (CC BY). The use, distribution or reproduction in other forums is permitted, provided the original author(s) and the copyright owner(s) are credited and that the original publication in this journal is cited, in accordance with accepted academic practice. No use, distribution or reproduction is permitted which does not comply with these terms.
*Correspondence: Alejandro Ariel Ríos-Chelén, YWxlamFuZHJvLnJpb3MuY0B1YXR4Lm14
†ORCID: Alejandro Ariel Ríos-Chelén, orcid.org/0000-0002-9936-6146