- 1Programa de Pós-Graduação em Ecologia, Instituto de Ciências Biológicas, Universidade Federal do Pará, Belém, Brazil
- 2Programa de Pós-Graduação em Ciências Ambientais, Universidade Federal do Amapá, Macapá, Brazil
- 3Laboratório de Estudos de Impacto Ambiental, Instituto de Ciências e Tecnologia das Águas, Universidade Federal do Oeste do Pará, Santarém, Brazil
Anthropic activities affect the dynamics of aquatic communities and can influence the reproductive behavior of many species. In addition, functional diversity is expected to be influenced by the environment. In this context, we evaluated how the biological functional characteristics of the Odonata adult community respond to impacts caused by human action on streams in the Eastern Amazon, using bionomic characteristics as response variables. Concomitantly, we analyzed which characteristics are responsible for the presence of species in the environment. We sampled adults of Odonata in 98 preserved and altered streams in the Eastern Amazon. We used as functional characteristics: oviposition, thermoregulation and body size, and as morphological characteristics: width of the thorax, width of the wing at the base, length of the abdomen and length of the thorax. We recorded 80 species, distributed in 16 functional groups and three categories: present in all environments, present only in preserved environments, and present only in altered environments. There was variation in the functional characteristics studied between the environments (PerMANOVA; F = 15,655; P < 0.01), with a significant difference in the composition of attributes between the environments studied. Although PCoA did not find a strong relationship between the functional attributes and the level of integrity, the individuals found in altered areas are heliothermic, exophytic oviposition, with a wider wing width at the base and larger size. Individuals with smaller body size and endophytic and epiphytic oviposition, and thermal and endothermic conformators are found in preserved areas. Our study provides evidence that functional attributes are determining factors for the occurrence of species in the environment. The high quality of environment has a significant effect on the composition of functional groups. Exophytic and heliothermic species are favored by altered environments, while in preserved environments, the species that are best adapted are those that present epiphytic and endophytic oviposition and ectothermic thermoregulation (thermal conformers). As for morphology, altered environments favor medium to large individuals, with greater thorax length and abdomen size, preserved environments may favor the smaller and/or specialized species.
1 Introduction
Currently, studies indicate a worrying decline in the diversity of invertebrates, especially insects (Sánchez-Bayo and Wyckhuys, 2019). The main factors causing biodiversity loss are related to human activities such as logging, the expansion and intensification of agriculture, and urbanization (Ceballos et al., 2017). Such human intervention causes a significant change in the riparian vegetation of a waterbody, thus affecting the richness and abundance of species less tolerant to these changes (Ferreira and Petrere, 2007). Factors such as canopy openness (facilitating light entry) and the amount of vegetation around the water body can affect the distribution of aquatic insects (Harabis and Dolný, 2010; Monteiro-Júnior et al., 2013; Suhonen et al., 2013; Rodrigues et al., 2016), the reproductive behavior of many species (Rodrigues et al., 2016), and their functional diversity (Pereira et al., 2019).
The morphological structures of an organism and its dimensions are directly related to the functions performed for its survival (Arnold, 1983). When we evaluate species within the same taxonomic group, it is possible to notice that some individuals are more resistant to environmental changes while others disappear, thus becoming a major challenge for conservation ecology (Powney et al., 2015). Although taxonomic measures are efficient, they alone cannot detect variations in the functional structure of communities (Colzani et al., 2013). There are more recent approaches and diversity metrics that aim to understand the patterns that have reached the distribution of species (Dalzochio et al., 2015; Dalzochio et al., 2018). Among them is the functional diversity approach, whose objective is to group species by function and not by taxonomic group (Poff et al., 2006; Pereira et al., 2019; Resende et al., 2021). This approach is based on biological, morphological and behavioral characteristics that are connected with ecosystem functions, these characteristics are called functional traits (Violle et al., 2007; Dalzochio et al., 2015; Dalzochio et al., 2018). Therefore, the use of airways in functional traits can help identify the characteristics that allow some species of aquatic insects to resist anthropogenic pressures (Firmiano et al., 2021).
Among a variety of aquatic groups, dragonflies (Odonata) stand out due to their great habitat specificity and environmental sensitivity (Banks-Leite et al., 2012; Oliveira-Junior et al., 2022). They are widely used to detect environmental disturbance because their distribution, richness, and composition are highly associated with variations in the conditions that comprise a physical habitat (Williams et al., 2004; Silva-Pinto et al., 2012). Furthermore, they possess a relatively long-life cycle (Miguel et al., 2017), the dragonflies can live up to a year in the tropics (Stoks and Córdoba-Aguilar, 2012), have a broad distribution in aquatic systems (Corbet, 1980), and are affected by environmental change (Monteiro-Júnior et al., 2015; Mendoza-Penagos et al., 2021). Features like these make Odonata especially important for conservation because they provide a greater understanding of the relationship between physical variables, biological variables, and biological communities (Juen and De Marco, 2012).
Morphological variation has implications that need to be considered to increase our understanding of the distribution of species. For example, the width of the wing base is directly related to the ability to glide (Nilsson-Ortman et al., 2012) and inversely related to the maneuverability of the wings, since individuals with a wider wing base cannot perform more elaborate maneuvers (Johansson et al., 2009). The abdomen is important for the thermoregulation, reproduction, and territorialism of these individuals (May, 1976; Michiels and André, 1990). In some species, an increase in wing size is related to the ability to fly (Conrad et al., 2002). The width and length of the hind wing are important for maneuverability, and flight duration (De Marco et al., 2015) and are related to the ability to glide (Corbet, 1962).
The thorax, in contrast, is important for flight because it houses the musculature, and like the abdomen, it also has an important role in thermoregulation because the cooling coefficient is related to small body size (May, 1976). The speed of the group is generally related to body size and thermoregulation (Corbet, 1980). Bigger dragonflies tend to be endothermic and have a high dispersal ability (May, 1991; Corbet and May, 2008). In general, the Anisoptera have more robust bodies and are more capable of dispersal than the Zygoptera, which have slender bodies (Corbet, 1999; Heiser and Schmitt, 2010). Some Odonata species have elaborate territorial and competitive behavior (Corbet, 1999), where the adult male fights against competing males, to restrict access to the territory in which these organisms reproduce (Corbet, 1999).
Another important behavior in the distribution of dragonflies is oviposition. The oviposition environment is essential in the distribution of species because female dragonflies need places with adequate substrate for oviposition (Corbet, 1999; Suhonen et al., 2013). Environmental factors can act as filters for these characteristics (Pereira et al., 2019). Environmental stressors (such as the degradation of freshwater ecosystems) play an important role in shaping the feature composition of the aquatic insect community (Ding et al., 2017). The presence of different microhabitats provides good locations for oviposition and roosting sites (Clark and Samways, 1996). This idea also elucidates the theory of habitat models (Southwood, 1977), which proposes that habitat properties are determined by the composition and diversity of biotic communities (Townsend and Hildrew, 1994; Statzner et al., 2001).
The thermoregulation hypothesis shows that although all thermoregulatory groups are present throughout the entire length of the water body, their proportion in the assemblage composition changes with the width of the stream because of the increase in river order, causing a greater incidence of sunlight (De Marco et al., 2015). This ecophysiological hypothesis is widespread among odonatologists, however, it does not clearly explain the causes of the distribution of species within each of the thermoregulatory groups, especially if one considers the hypothesis that all thermoregulatory groups are found throughout the watercourse, differing only in the proportion of species distribution (Pereira et al., 2019).
The aim of this study was to investigate how the functional characteristics of Odonata respond to impacts caused by human activity in the Eastern Amazon, using bionomic traits as response variables. The hypotheses of the study were: i) species with greater proportions of wing base width, due to reduced maneuverability (Mcauley, 2013), are better related to altered environments, due to the reduction of riparian forest, while for species with more petiolate wings, maneuverability is essential for more preserved riparian forests due to the greater number of obstacles (Hedenström and Møller, 1992). individuals with size and length of the abdomen and larger body size are closely linked to altered environments, dragonflies with such characteristics must perform thermoregulation more efficiently and in places with high solar irradiation, while smaller dragonflies are dependent on the temperature of the environment (De Marco et al., 2005); ii) degraded environments, with low forest cover and altered riparian vegetation favor heliothermic individuals, due to their thermoregulation specificity. While thermal and endothermic conforming individuals will be more frequent in preserved environments (De Marco and Resende, 2002; De Marco et al., 2015). Thus, we expect to have a separation of functional groups according to thermoregulation between the studied areas; iii) oviposition will also be a determining factor in the occurrence of species among the studied areas. Since Odonata species need suitable substrates to lay their eggs (Corbet, 1999; Suhonen et al., 2013), it also acts as a filter that determines the location of occurrence (Pereira et al., 2019). For this reason, we expect a high occurrence of species with endophytic and epiphytic oviposition in preserved environments, once this trait demands the presence of wood and riparian vegetation. In contrast, we expect the occurrence of exophytic species to be higher in altered environments because oviposition of these species is performed directly in the water column.
2 Materials and methods
2.1 Study area
Two drainage basins of Eastern Amazonia were analyzed, one in the municipalities of Santarém, Belterra, and Mojuí dos Campos, and the other in the municipality of Paragominas, located in the State of Pará, Brazil (Figure 1). The municipalities of Santarém (02°26’22”S, 54°41’55”W), Belterra (02°4’54”S, 54°53’18”W), and Mojuí dos Campos (02°40’53”S, 54°38’33”W), are located west of the state. In other words, it is characterized as a rainy tropical climate with a well-defined short dry season, with rainfall of less than 60 mm. The region’s rainfall is, on average 2,000 to 2,300 mm/year, the maximum temperature varies from 25°C and the relative humidity is 86%.
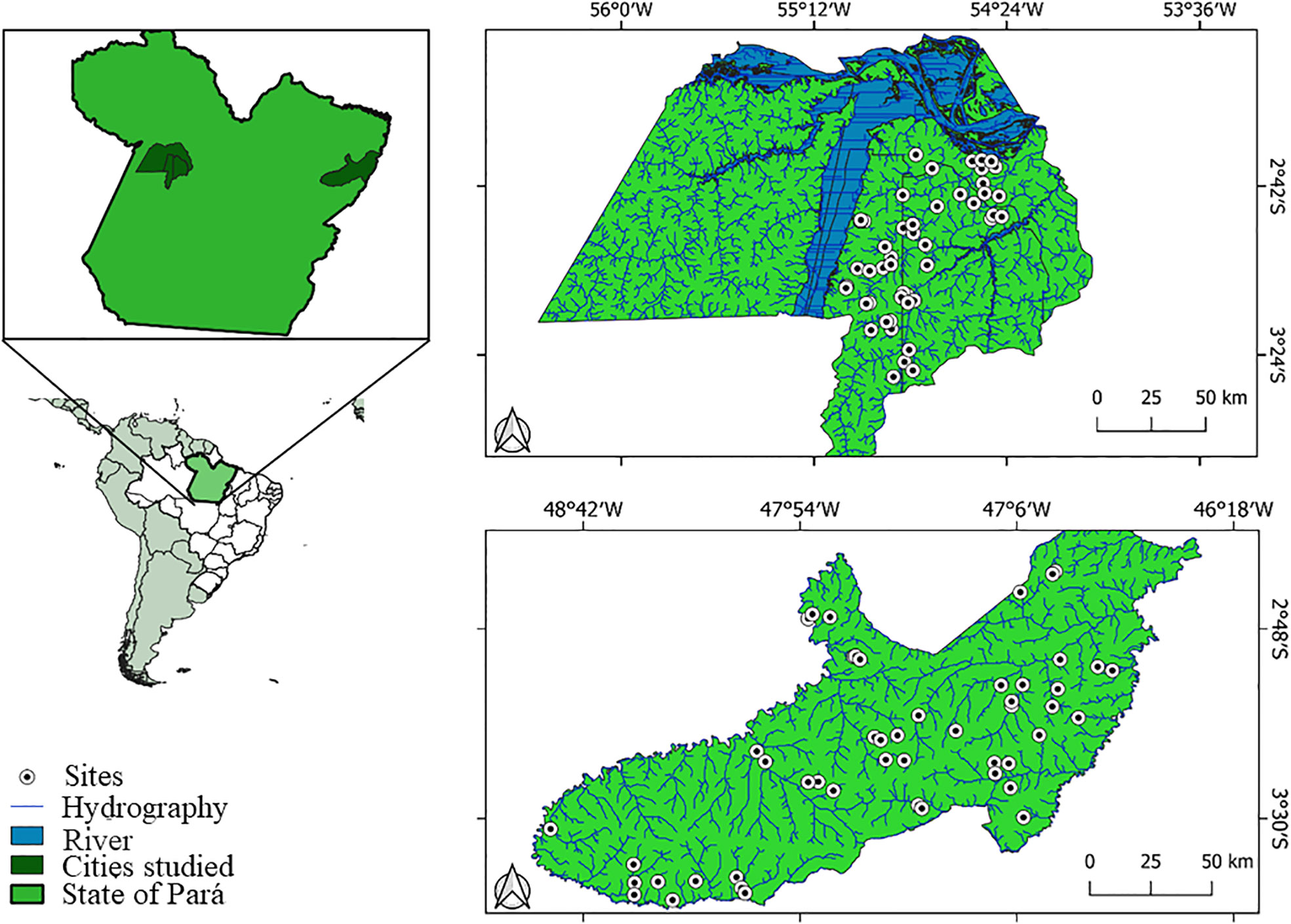
Figure 1 Drainage basins and streams sampled in the regions of Paragominas and Santarém, Belterra and Mojuí dos Campos, in Eastern Amazon, Pará, Brazil.
The rainiest period occurs between December and May, with about 80% of rainfall, and between June and November, the rest of the rainfall is recorded in the region (Nepstad et al., 2002). Paragominas, in turn, is in northeastern Pará (02°26’22”S, 54°41’55”W) with average annual precipitation of 1,766 mm, an average annual temperature of 27°C, and relative humidity of 81% (Watrin and Rocha, 1992). This type of climate corresponds to tropical rainy climates with a short and well-defined dry season.
Santarém, Belterra and Mojuí dos Campos in the Amazon biome present dense rainforest and relief diversification, with regions varying from plateau to floodplains. The predominant vegetation in the region is tropical forest, except Amazon savannahs found in northwestern Santarém (Berenguer et al., 2014). Small reforestation areas are found in the central portion of the municipality, and reference sites can be found in the Tapajós National Forest (Belterra), adjacent to the region (Feitosa et al., 2012). The vegetation of the Paragominas region is classified as a dense tropical forest (Veloso et al., 1991).
Both sampled regions have a land use gradient (Moura et al., 2013), which comprises altered areas that go from secondary forests formed after the complete destruction of the native forest (Putz and Redford, 2010), and areas of cattle grazing and mechanized agriculture and soybean plantation (Gardner et al., 2013; Moura et al., 2013; Oliveira-Junior et al., 2015). However, largely preserved remnants can still be observed consisting of primary forest, with original climax physiognomy that has clearly never been logged (Gardner et al., 2013; Moura et al., 2013).
2.2 Data sampling
2.2.1 Biological sampling
A total of 98 first- to third-order streams were sampled (an average of 2 to 5 m wide, according to Strahler (1957) classification), distributed over a gradient of environmental conditions ranging from fully preserved areas to those heavily modified by cattle ranching and agriculture. The collections were performed in both areas during the dry season, with 48 sampling sites in Santarém, Belterra, and Mojuí dos Campos (July to August 2010) and 50 in Paragominas (July to August 2011). The dry season was selected due to: (i) the ecophysiological requirements of Odonata (May, 1976; May, 1991; Corbet, 1999); (ii) sampling in a single seasonal period can reduce “noise” in statistical analyses (see Heino and Peckarsky, 2014); iii) several studies show that the highest diversity of Odonata can be found during the dry season (Fulan and Henry, 2007); and iv) the low depth of the water column during this season concentrates these insects in smaller areas, which allows us to find and capture them more easily (Oliveira-Junior and Juen, 2019).
At each stream, a 150 m stretch was delimited and subdivided into ten longitudinal sections of 15 m each, separated by bank-to-bank transects (see Oliveira-Junior and Juen, 2019). The 15 m longitudinal sections were subdivided into three segments of five meters each, and only the first two segments of each section were sampled, totaling 20 segments of 5 m in each stream marked with biodegradable strips from A (downstream) to K (upstream) (Figure 2). The fixed area scanning methodology was used successfully by other researchers (Juen and De Marco, 2011; Silva-Pinto et al., 2012; Oliveira-Junior et al., 2013; Calvão et al., 2016; Batista et al., 2021; Cezário et al., 2021). Using an entomological net (40 cm diameter, 65 cm depth, and 90 cm long aluminum handle), the spotted Odonata adults were collected, following the collection protocol by Oliveira-Junior et al. (2015) and conditioned according to Lencioni (2006).
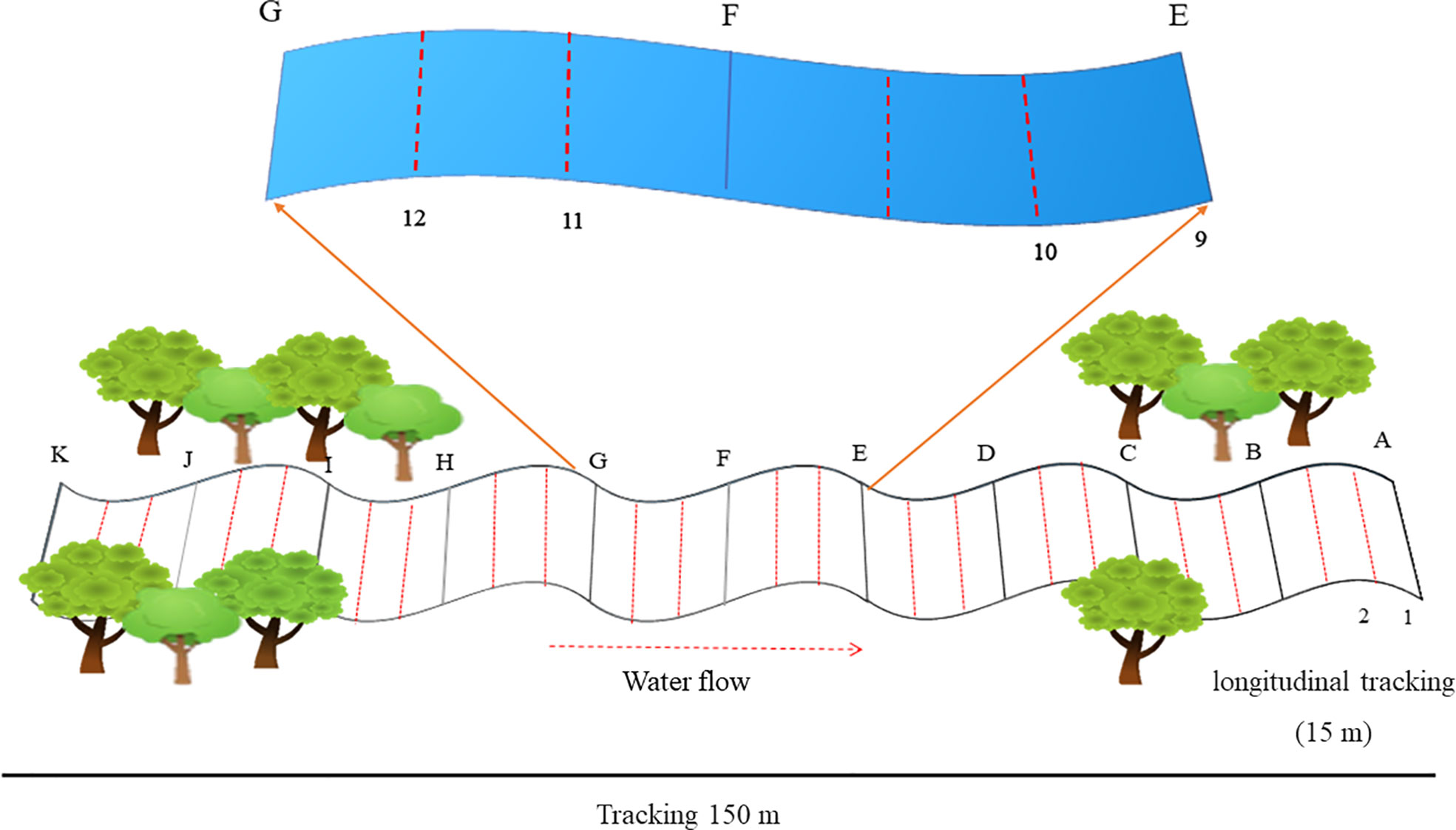
Figure 2 Schematic drawing of the transect established in each stream for Odonata sampling. Each transect was named (with letters from A to K) from downstream to upstream.
Collections were performed between 10 AM and 2 PM, when sunlight reaches the main stream channel, following De Marco and Resende (2002) and Batista et al. (2021), in order to ensure favorable conditions for all Odonata groups, both thermal, heliothermic, and endothermic conformers to be active (May, 1976; May, 1991; Corbet, 1999; De Marco and Resende, 2002; Batista et al., 2021).
To identify the collected specimens, we used specialized taxonomic keys (Garrison, 1990; Lencioni, 2005; Garrison et al., 2006; Lencioni, 2006; Garrison et al., 2010), and compared with the testimony material of the collection from the Zoology Museum of the Universidade Federal do Pará. After identification, the specimens were deposited as testimonial material in the Collection of the Zoology Museum of the Universidade Federal do Pará, Pará state, Brazil.
2.2.2 Morphological traits
With the aid of a digital caliper (precision of 0.01 mm), we measured four morphological characteristics: a) width of the posterior wing at base height (WPWBH); b) abdomen length (AL); c) thorax length (TL); and d) thorax width (TW). Measurements were taken in at least 10 male individuals of each species, taking care to take measurements only in the left side of the specimen, measuring every trait three times, to standardize and minimize errors. The average values of the specimens measured were used as the final measurement for the species in each morphological character.
Body size (BS) was measured as the total length of adults (from the top of the head at the end of the appendices). We measured an average of 10 individuals of each species, using these measurements to obtain the average length of each species. Posteriorly, the species were divided into three size classes: (1) small, individuals measuring 18 to 29 mm; (2) mid-sized, individuals ranging from 30 to 43 mm; and (3) large, individuals over 44 mm (Table 1). To determine the size classes, we followed Dalzochio et al. (2018).
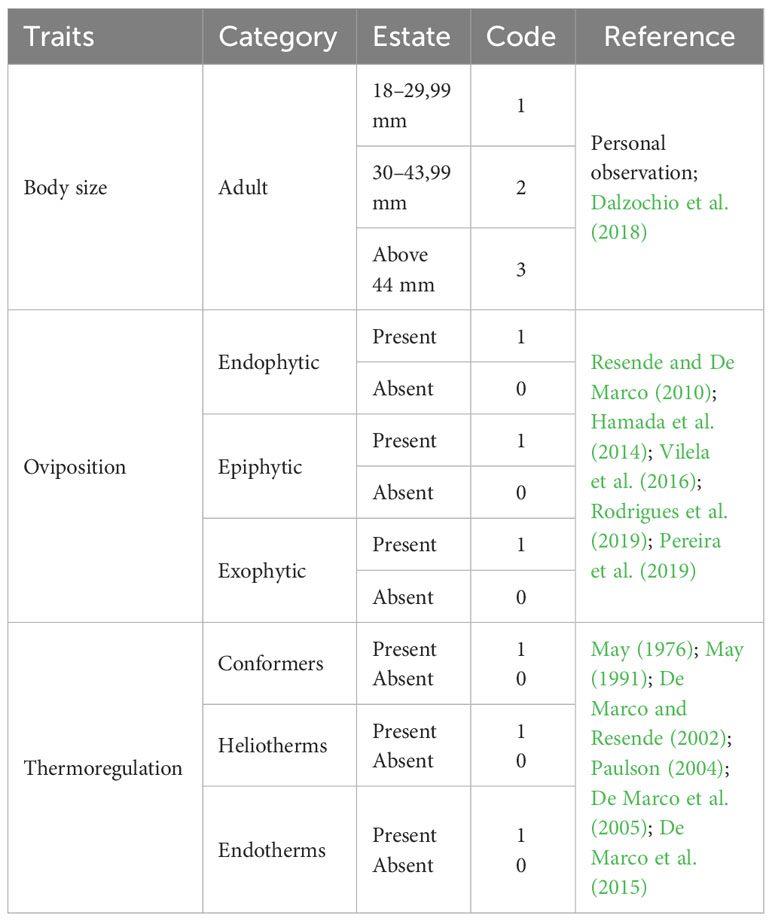
Table 1 Functional traits of Odonata (Insecta) adults, sampled in streams of Eastern Amazon, Pará, Brazil.
2.2.3 Type of oviposition
For Odonata, there are three basic types of oviposition: (i) endophytic – where the eggs are laid inside a plant tissue (living or dead), however, these individuals prefer living tissue; (ii) epiphytic – the eggs are laid at the surface of rocks, trunks, leaves and other substrates, both over and under the water column; (iii) exophytic – eggs are released directly into the water (Fincke, 1986; Corbet, 1999) (Table 1). To determine the type of oviposition of each species, we used the literature as a basis, by means of a direct search of the description of the type of oviposition of each species made in articles or books. In case information about the species was unavailable, we used a category described for the genus level, and in case this was also unavailable, the most frequent oviposition category in the family was used.
2.2.4 Types of thermoregulation
Due to the ecophysiological requirements of adult individuals regarding their ability to thermoregulate, Odonata species can be divided into (i) thermal conformers, of small body size, show increased conductance and the body temperature varies with the environment, mainly due to heat exchange by convection (Corbet, 1999); (ii) heliotherms, have a larger body and, consequently, lower conductance, and their activities are determined mainly by solar irradiation; (iii) endotherms, they produce heat by controlling the circulation of the hemolymph (May, 1976) (Table 1). The strategies and ecophysiological patterns of Odonata also associate thermoregulatory capacity with body size (Corbet, 1999).
The type of thermoregulation for each species was determined based on the literature, through a direct search of the description of the type of oviposition of each species in articles or books. In cases where information about the species was not found, a category already described for the genus level was used; if this was not available, the most frequent category of oviposition in the family was used.
2.2.5 Evaluation of the physical condition of the studied streams
Six variables were used to measure the environmental quality in the 98 studied streams: average canopy cover over the channel, percentage of forest in the surrounding landscape, and four physical and chemical descriptors of the water: water temperature (°C), electrical conductivity (mg/L), hydrogen potential (pH) and dissolved oxygen (mg/L), obtained from a Horiba probe, Model U51. Several previous studies have demonstrated the importance of these physicochemical variables for the structure of aquatic insect communities, including water temperature and electric conductivity (Oliveira-Junior et al., 2013; Oliveira-Junior et al., 2017), dissolved oxygen (Jacob et al., 1984), and pH (Courtney and Clements, 1998), therefore these variables were selected for our study.
To estimate the integrity of the environment in each stream, we used the habitat integrity index (HII) of Nessimian et al. (2008). This index consists of 12 items that describe the environmental conditions of the streams, assessing characteristics such as the pattern of land use adjacent to the riparian vegetation; width of the riparian forest and its state of preservation; state of the riparian forest within a 10 m band; description of the channel condition regarding the type of sediment and presence of retention devices; structure and wear of the marginal ravines of the stream; characterization of the stream bed regarding substrate, aquatic vegetation, detritus, and arrangement of the areas of rapids, pools, and meanders. Each item comprises four to six alternatives that are ordered to represent increasingly more pristine systems, with the index value varying between 0 (least pristine) and 1 (most pristine). This index is directly related to the level of environmental conservation and has been successfully used in many studies assessing the integrity of aquatic systems (Carvalho et al., 2013; Giehl et al., 2014; Juen et al., 2014; Monteiro-Júnior et al., 2015; Bastos et al., 2021), and in a recent meta-analysis, it was shown to be one of the most important metrics for explaining community variation (Brasil et al., 2021).
To estimate mean canopy cover, we used a convex densiometer positioned at the central point of the channel, where we made four measurements: upstream, downstream, and at the left and right margins. Canopy cover is frequently considered one of the main characteristics of the physical habitat that influences Odonata distribution patterns in tropical streams (Oliveira-Junior et al., 2017). For the forest percent cover, we defined forest cover within 100 m buffers, which we used to delineate the landscape within which the percent cover was estimated. The proportion of habitats in preserved environments or natural cover is among the key variables that explain species distribution and community structure in natural environments (Fahrig, 2003).
2.3 Data analyses
2.3.1 Physical and chemical condition of streams
To assess the variation in the studied environments, we summarized the values of six environmental variables (canopy cover, percentage of forest, water temperature, electric conductivity, pH, and dissolved oxygen) through a Principal Component Analysis (PCA), with a correlation matrix to determine the pattern of variation in environmental and structural parameters among samples. To reduce multicollinearity, we assessed the degree of correlation between variables. Since the environmental variables were measured in units of varying orders of magnitude, the values (except pH) were standardized using a Euclidean distance matrix. A random Broken-stick model was used to determine which principal components would be retained for analysis, retaining only those components that describe the main gradients of habitat variation (Jackson, 1993). The HII score (in the PCA ordination) was used to identify the categories of stream integrity (altered and preserved). All analyses were performed in the vegan package (Oksanen et al., 2022), in R software, version 4.0.4 (R Core Team, 2021).
2.3.2 Determination of functional groups
To determine the functional groups (FG), a trait matrix produced from the coding of all species with respect to these features in their respective states was used. After this, we created a dendrogram of Odonata species grouped by functional characteristics, using Ward’s linkage method based on Gower’s distance (Casanoves et al., 2011), using the FD package (Laliberté et al., 2014) in R, version 4.0.4 (R Core Team, 2021). The dendrogram was cut at point “5”, to determine the functional groups (Casanoves et al., 2011; Dalzochio et al., 2018).
2.3.3 Weighted average values of traits at the community level
To assess the variation in the composition of bionomic traits between altered and preserved streams, we first calculated a community-weighted mean (CWM) index, using the FD package (Laliberté et al., 2014), in R (R Core Team, 2021) version 4.0.4. This index represents the relative abundance of each trait inside each studied community, correlating the species-by-trait matrix with the species relative abundance matrix, according to Violle et al. (2007). Thus, after calculating the CWM, a matrix of the studied communities by functional traits was generated. Relative abundance (~ n) was obtained by dividing the number of individuals collected from each species by the total number of individuals in each sample.
2.3.4 Comparison of Odonata communities among the studied locations
With the Functional Groups (FG) and CWM matrices, a Permutational Multivariate Analysis of Variance (PERMANOVA) was performed to test for variation in trait composition among the sites studied, with Gower dissimilarity. To visualize these differences, we proceeded with a Principal Coordinate Analysis (PCoA), also with Gower dissimilarity. The PCoA function calculates the principal coordinate decomposition (also called classical scale) of a distance matrix D (Gower, 1966).
3 Results
3.1 Physical condition of streams and water quality
The studied streams presented considerable variation in physical conditions and physical-chemical properties of water, reflecting environmental change due to anthropogenic activities. The values of the variables were: habitat integrity index – HII (0.83–0.50), average canopy cover on the channel (71.14–84.53%), percentage of primary forest (0.00–100.00%), water temperature (22.50–29.90°C), electrical conductivity (44.64–74.70 µS/cm), dissolved oxygen (5.20–5.33 mg/L), and pH (4.88–4.77).
The HII shows a variation in stream integrity, classifying them into two distinct categories of integrity: 56 altered streams (HII = 0.15 to 0.69) and 42 preserved streams (HII = 0.70 to 0.99). The means and variances of the values of the environmental variables are presented by category of stream integrity in Table 2.

Table 2 Mean and variance (in parentheses) of environmental variables recorded in each type of stream integrity in two regions of the Eastern Amazon, Pará, Brazil.
The association between the two PCA axes accounted for 55.92% of the environmental variation (axis 1 = 30.27%; axis 2 = 25.65%). Only the first axis component was analyzed, since the value of the second component was lower than that estimated from the Broken-stick model. The first component alone accounted for 30.27% of the variance in the results (eigenvalue = 1.82). This analysis showed that there was a clear separation of streams into two distinct integrity categories. The streams with the highest HII values (HII ≥ 0.70; preserved) were grouped with the highest canopy cover, percentage of primary forest, and dissolved oxygen. Streams with lower HII values (HII ≤ 0.69; altered) had a loss or significant change in these same variables (Table 3; Figure 3).
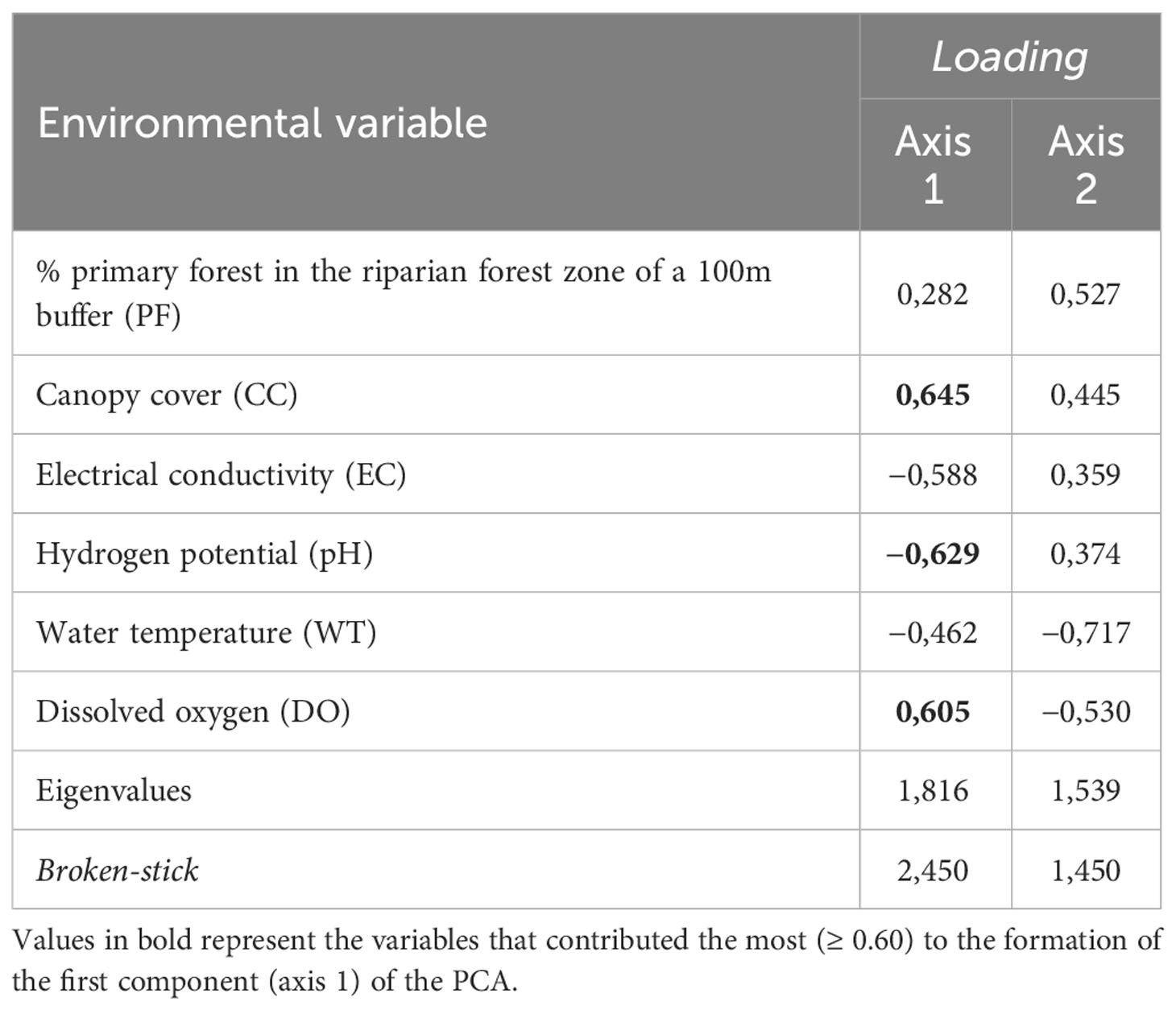
Table 3 The six environmental variables used to describe the environmental conditions of sampled streams in two regions of the Eastern Amazon, Pará, Brazil, and their correlation with the principal components (axes 1 and 2) of the Principal Component Analysis (PCA).
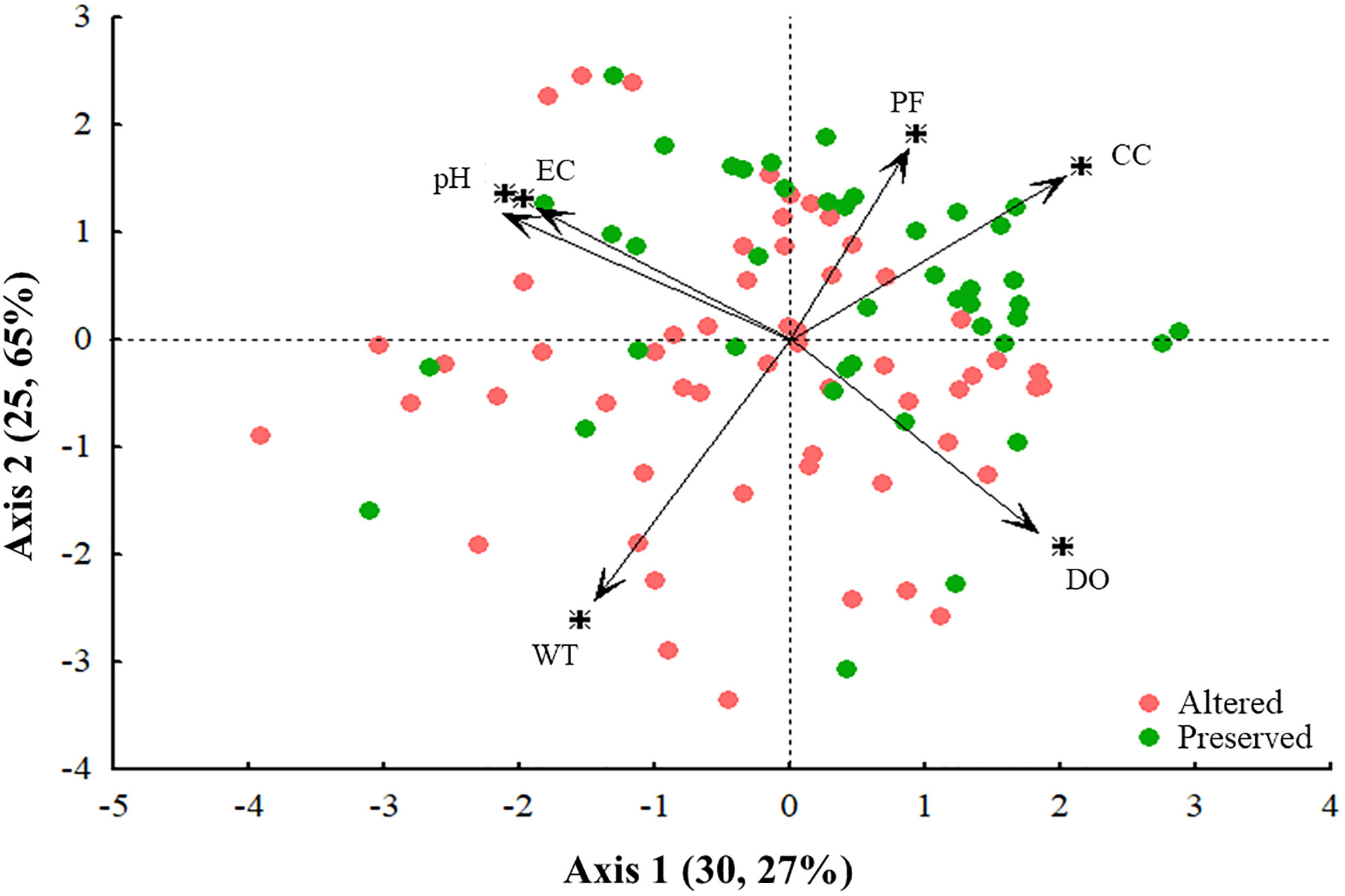
Figure 3 Ordination of the six environmental variables of the sampled streams in two regions of the Eastern Amazon, Pará, Brazil, based on principal component analysis (PCA) (PF, % of primary forest in the riparian network within a 100 m buffer; CC, average canopy cover; EC, electrical conductivity; pH, hydrogen potential; WT, water temperature; DO, dissolved oxygen). The "+" symbol is just a marker for each variable in the figure.
The variables that contributed most to the formation of the first component were related to the physical structure of the riparian forest and water quality, explaining the observed clustering patterns. Canopy cover (CC) and dissolved oxygen (DO) were positively related to the level of stream integrity, while pH was negatively related to integrity levels (Table 3).
3.2 Functional groups
We evaluated 80 species, 48 collected in preserved environments and 70 in altered environments, of these, 44 species belonged to the suborder Zygoptera and 36 belonged to the suborder Anisoptera. The dendrogram based on the designated characteristics resulted in 16 functional groups (Figure 4; Table 4).
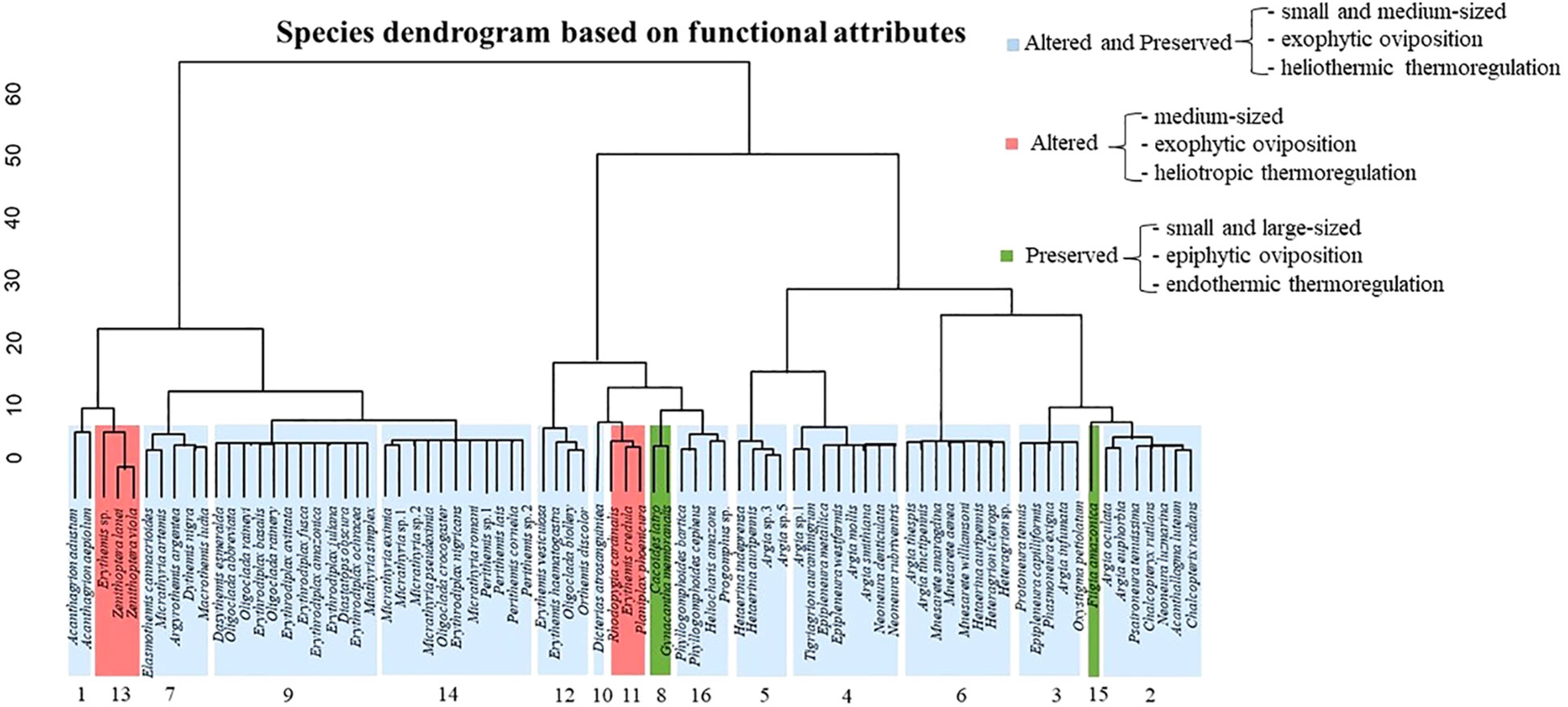
Figure 4 Dendrogram based on functional traits of Odonata species, sampled in streams with different conservation categories (altered and preserved), in the Eastern Amazon, Pará, Brazil.

Table 4 Abundance of functional groups of Odonata (Insecta) adults sampled in altered and preserved streams in the Eastern Amazon, Pará, Brazil.
Of the total functional groups 12 (FG 1, 2, 3, 4, 5, 6, 7, 9, 10, 12, 14, and 16) were present in both altered and preserved streams (Figure 4; Table 4). Most of these groups include species with diverse functional characteristics. Individuals belonging to these groups have the following average morphological traits: width of the posterior wing at base height – WPWBH (4.49 mm), abdomen length – AL (22.66 mm), thorax length – TL (5.72), and thorax width – TW (2.16). For the behavioral traits, the vast majority of the species are small (1) and medium-sized (2), with exophytic oviposition and heliothermic thermoregulation (Appendix 1). The most abundant functional group was GF 9 (n = 20.3), followed by GF 6 (n = 12.3), and the other groups showed low abundance. compared to the presented groups.
Only two groups were present in altered streams (FG 11 and 13). The species in these groups have the following average morphological traits: width of the posterior wing at base height – WPWBH (10.89 mm), abdomen length – AL (16.44 mm), thorax length – TL (5.79 mm), thorax width – TW (2.32 mm). As for behavioral attributes, they have size 2 (medium) species, exophytic oviposition, and heliotropic thermoregulation. Groups GF 8 and 15 were the only ones exclusively present in preserved areas, with species showing the following average morphological traits: width of the posterior wing at base height – WPWBH (8.14 mm), abdomen length – AL (42.43 mm), thorax width – TW (9.09 mm), thorax length TL (5.81) and behavioral traits, epiphytic oviposition, and endothermic thermoregulation, regarding size, we had small (1) and large (3) species.
The composition of biological characteristics was represented by two axes of principal coordinate analysis (PCoA), the first axis explained 73.94% and the second, 24.39% of the functional variation in the community (Figure 5). It can be seen that individuals found in altered areas are heliothermic, with exophytic oviposition and wider width of the posterior wing at base height WPWBH. While individuals with smaller body sizes and endophytic oviposition, and thermal conformers are commonly found in preserved areas. Although some sampling sites were overlapped, PERMANOVA showed that there is a pattern of separation between the studied environments (Pseudo-F = 15.655; P < 0.01) (Figure 5).
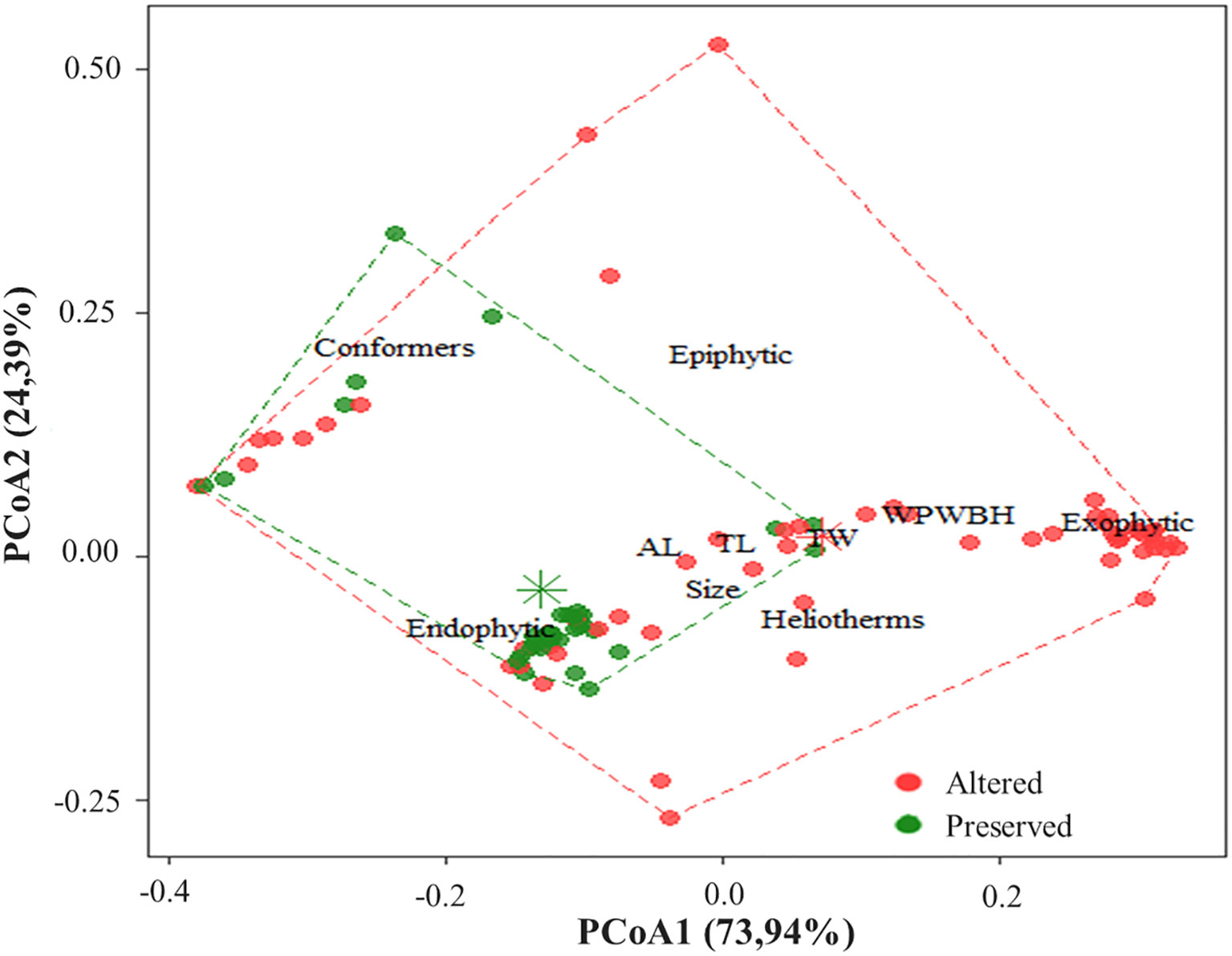
Figure 5 Ordination of the functional composition of Odonata species, sampled in altered and preserved streams, Eastern Amazon, Pará, Brazil.
4 Discussion
The functional characteristics of the Odonata community respond to the impacts caused by human activities in Amazonian streams, which corroborates the hypothesis proposed by this study. Environmental integrity has a negative relationship with some morphological traits such as larger body size and larger thorax width and length, and favor species with exophytic oviposition (Remsburg and Turner, 2009), therefore, there is an increase in the abundance of species that possess these characteristics in altered environments. It is possible to observe that there was a separation of groups between the environments (altered and preserved). Factors such as environmental integrity, canopy cover, as well as the presence or absence of vegetation in the streambeds, affect the distribution of Odonata species (Monteiro-Júnior et al., 2013). The transformation of the terrestrial landscape significantly affects the diversity of dragonflies in terms of taxonomic composition and richness, especially in relation to the loss of vegetation and physical and chemical changes in aquatic environments (Stoks and Córdoba-Aguilar, 2012; Bried and Samways, 2015; De Marco et al., 2015; Monteiro-Júnior et al., 2015; Rodrigues et al., 2016; Rodrigues et al., 2019).
Oviposition is a trait that can influence the distribution of Odonata species and is important in determining the separation of functional groups between environments (Calvão et al., 2022). Although a habitat may appear suitable for many aspects of dragonfly life history, crucial oviposition sites are often of poor quality or absent (Grönroos et al., 2013; Heino and Peckarsky, 2014). Oviposition behavior is an important tool to assess the behavior of Odonata adults in the face of environmental changes caused by different land uses (Rodrigues et al., 2019). Suitable egg-laying characteristics are necessary for successful oviposition and consequently for the population establishment and dynamics of dragonfly larvae (MacCreadie and Adler, 2012; Heino et al., 2014; Tavares et al., 2017).
Studies have shown that characteristics such as size and thermoregulation are key to understanding how anthropogenic environmental changes may affect dragonfly diversity (Juen and De Marco, 2011; Nóbrega and De Marco, 2011; De Marco et al., 2015). Species size is important for thermoregulation (De Marco et al., 2015), while wing base width is related to gliding ability, this same characteristic is inversely related to flight maneuverability (Corbet, 1962; Johansson et al., 2009). Sites with a higher integrity index present greater obstacles, due to the presence of vegetation, so they generate a selectivity in favor of more petiolate wings (Hedenström and Møller, 1992). However, wider wings benefit long flights and are related to open sites with few obstacles (Pereira et al., 2019). Odonata body size influences group competition because larger animals (most Anisoptera), are more attached to open areas (Bastos et al., 2021; Oliveira-Junior et al., 2021). In our study, species that have larger size and heliothermal thermoregulation are better associated with altered environments, as is the case of individuals of Rhodopygia cardinalis, which have exophytic oviposition. On the other hand, Fylgia amazonica had a greater relationship with preserved environments, and these species have a small body size, possibly as an adaptation to shady environments of primary forests (Samways and Taylor, 2004).
Heliotherms were more closely related to altered environments. They are mostly larger in size, and may include some Zygoptera and most Anisoptera, have lower conductance, and their activity is determined mainly by solar irradiance (Corbet and May, 2008). Thermal conformers were better related to preserved environments because this group needs shaded areas (De Marco et al., 2015) and the alteration of habitats ends up excluding species with specific characteristics. Species with large body sizes such as Aeshnidae are considered endothermic, as they regulate their temperature through flight, determining heat production (May, 1991).
The functional groups found in both areas were composed of both Anisoptera and Zygoptera. The most frequent Zygoptera families were Coenagrionidae and Calopterygidae. Dalzochio et al. (2018) obtained similar results, where they state that species from the Calopterygidae family are more generalist and are found in many habitats. The high abundance of species of the genus Argia Rambur may suggest the presence of many individuals of Zygoptera with sizes ranging from small to medium. The Anisopterans found in the groups present in the two categories of integrity were composed almost exclusively of the family Libellulidae, mostly by species of the genera Erythrodiplax Brauer and Micrathyria Kirby. The genus Erythrodiplax Brauer is one of the most diverse genera of Libelullidae (Garrison et al., 2006; Del Palacio and Muzón, 2019). Its species inhabit different types of wetlands, and many of them can be abundant in temporary ponds, swamps, and stream pools (Del Palacio et al., 2020). These characteristics make the species less affected by disturbance events as they support more solar incidence along the stream channel (Ball-Damerow et al., 2014; De Marco et al., 2015; Powney et al., 2015) and consequently allow them to explore more broadly a variety of environments. This explains the occurrence of these groups in both environments.
The groups that were exclusively present in altered streams showed exophytic oviposition and heliothermic thermoregulation, similar to the species of the genus Erythemis that in this study present heliothermic thermoregulation. The relationship of these species with altered areas may be due to the fact that modifications in the stream channels increase the number of backwaters along the water body and the incidence of sunlight due to clearings from human activities (Oliveira-Junior et al., 2015; Rodrigues et al., 2019). In streams with compromised integrity, the removal of riparian vegetation can raise solar incidence and consequently reduce the availability of resources for a wide variety of species. This process may generate homogenization of Odonata species, with the replacement of specialized species by more generalist species (Remsburg and Turner, 2009; Couceiro et al., 2012; Oliveira-Junior et al., 2013).
Altered environments are used by many exophytic species as oviposition sites. This factor contributes to the increase in species richness and abundance in sites with little or no forest cover surrounding streams (Corbet, 1999; Dutra and De Marco, 2015). The exophytic oviposition behavior of Odonata may be related to the alteration of the riparian vegetation, which opens space for greater insolation; these environments also favor species with heliothermic thermoregulation, since heliothermic individuals need sun rays falling directly on their bodies for their metabolic activities (De Marco et al., 2005).
The most abundant family in groups exclusive to altered areas was Libellulidae, the size ranged from medium to large, and morphological characteristics were similar among the species of this family, especially in the abdomen length (AL), which is associated with several ecological behaviors of these individuals such as thermoregulation, oviposition/reproduction, and territorialism (May, 1976). Another factor is the width of the posterior wing at base height (WPWBH), which in the species of this group is wider. The interaction between wing width and integrity index also demonstrates its influence on maneuverability factors (Pereira et al., 2019).
The absence of riparian forest in altered streams, can increase the entry of sediments in the channel, change lotic channels, form puddles, and/or reduce the streamflow, favoring species that lay eggs directly on the water surface (exophytic oviposition) (Rodrigues et al., 2016). The most representative genera in the groups were Erythemis and Erythrodiplax, these genera are often found in studies of Odonata fauna (Souza and Costa, 2006; Dalzochio et al., 2011). The thermoregulatory behaviors (endothermic and hypothermic) and exophytic oviposition in species of Erythemis and Erythrodiplax may influence their species diversity in environments considered lentic (De Marco et al., 2015; Rodrigues et al., 2018).
The most abundant groups in preserved environments were mostly composed of individuals from the Zygoptera suborder, with endophytic oviposition and largely thermal conformers, all medium-sized. Thermal conformer species have bodies with high conductance, varying according to air temperature (Heinrich, 1974). However, only two groups were unique to pristine areas, which in turn comprised Anisoptera species with epiphytic oviposition, endothermic, and large size. The morphological characteristics were quite similar among the species. One of the groups (GF 8) had only two species: Cacoides latro and Gynacantha membranalis, both from the Gomphidae family. Adults from the Gomphidae family are usually found in areas of denser vegetation, and the nymphs are usually associated with streams or rivers with clear water and currents with sandy sediments (Garrison, 2009), are described as territorialists, being very common in lake environments (Moore and Machado, 1992).
Species from the Gynacantha Rambur genus inhabit mud-bottomed pools and/or temporary ponds within tropical forests (Garrison et al., 2006). They also have a phytotelmata habit, meaning they rely primarily on tree trunks or plants (such as bromeliads) capable of accumulating water for egg laying, this represents a distinctive aquatic habitat and supports a fauna that can be highly specific (Frank, 1983). These habits may explain the association of Gynacantha individuals with preserved environments. Larvae of the genus Cacoides Cowley inhabit vegetated ponds, sandy bank ditches, and lakes in the forest (Garrison et al., 2006), characteristics that are most associated with preserved environments. GF 15 was composed of a single species: Fylgia amazonica, this species is associated with pristine environments (Monteiro-Júnior et al., 2015), although it is considered a common species, individuals of the genus Fylgia Kirby, inhabit lotic and forest environments (Machado, 1954; Garrison et al., 2006).
5 Conclusion
This study provides evidence that functional traits are determining factors for the occurrence of species in the environment. A complex picture was observed where the species evaluated have a set of characteristics that limit them to specific habitats. Environmental quality, measured by the habitat integrity index, has a significant effect on the composition of functional groups. Altered environments favor species with exophytic oviposition and heliothermic thermoregulation, while preserved environments favor individuals with endophytic and epiphytic oviposition and ectothermic thermoregulation (thermal conformers). Species with these characteristics are mainly represented by Zygoptera, although some individuals of this suborder are resistant to changes, the lack of habitat for the oviposition of these individuals ends up causing the exclusion of many species from the environment. As for morphology, altered environments favor medium to large individuals, with greater posterior wing width at base height, thorax length, and abdomen size, while preserved environments may favor smaller and/or specialized species. The effects caused by altered aquatic environments such as landscape changes (e.g., loss of native vegetation) can affect the level of reproductive success of aquatic insects, altering local species composition and richness. The analyses performed show that functional diversity measures can provide additional information on the effects of habitat integrity on biodiversity. From a conservation perspective, the results of this study can be used to incorporate species preservation into the sustainable development of the Eastern Amazon.
Data availability statement
The original contributions presented in the study are included in the article/Supplementary Material. Further inquiries can be directed to the corresponding authors.
Ethics statement
Ethical review and approval was not required for the study on animals in accordance with the local legislation and institutional requirements. Ethical approval for research on invertebrates is under permanent license to collect zoological material (Sisbio number 11841-6).
Author contributions
All authors contributed to the writing of the text: TR, LC, LJ, and JO-J. The collected species data used in the study were made available by the authors: JO-J and LJ. LC provided data and Oviposition. TR, LC, and JO-J performed the statistical analyses. All authors contributed to the article and approved the submitted version.
Funding
This project was financed by the Coodenação de Aperfeiçoamento de Pessoal de Nı́vel Superior (CAPES), for granting a master’s scholarship for two years. This work also received financial support from PROPESP/UFPA through Edital 01/2023 (Programa de Apoio à Publicação Qualificada – PAPQ). JO-J is grateful to CNPq for his productivity grant to (process 307808/2022-0).
Acknowledgments
Laboratory of Ecology and Conservation (LABECO) of the Federal University of Pará (UFPA), for the availability of the database used in this study. The Environmental Impact Studies Laboratory (LEIA) of the Federal University of Western Pará (UFOPA), for academic and logistical support.
Conflict of interest
The authors declare that the research was conducted in the absence of any commercial or financial relationships that could be construed as a potential conflict of interest.
Publisher’s note
All claims expressed in this article are solely those of the authors and do not necessarily represent those of their affiliated organizations, or those of the publisher, the editors and the reviewers. Any product that may be evaluated in this article, or claim that may be made by its manufacturer, is not guaranteed or endorsed by the publisher.
Supplementary material
The Supplementary Material for this article can be found online at: https://www.frontiersin.org/articles/10.3389/fevo.2023.1166057/full#supplementary-material
References
Arnold S. J. (1983). Morphology, performance and fitness. Am. Zool. 2, 347–361. doi: 10.1093/icb/23.2.347
Ball-Damerow J. E., M’Gonigle L. K., Resh V. H. (2014). Changes in occurrence, richness, and biological traits of dragonflies and damselflies (Odonata) in California and Nevada over the past century. Biodivers. Conserv 23, 2107–2126. doi: 10.1007/s10531-014-0707-5
Banks-Leite C., Ewers R. M., Metzger J. P. (2012). Unraveling the drivers of community dissimilarity and species extinction in fragmented landscapes. Ecology 93, 2560–2569. doi: 10.1890/11-2054.1
Bastos R. C., Brasil L. S., Oliveira-Junior J. M. B., Carvalho F. G., Lennox G. D., Barlow J., et al. (2021). Morphological and phylogenetic factors structure the distribution of damselfly and dragonfly species (Odonata) along an environmental gradient in Amazonian streams. Ecol. Indic. 122, 107257. doi: 10.1016/j.ecolind.2020.107257
Batista J. D., Ferreira V. R. S., Cabette H. S. R., De Castro L. A., De Marco P., Juen L. (2021). Sampling efficiency of a protocol to measure Odonata diversity in tropical streams. PloS One 16 (3), e0248216. doi: 10.1371/journal.pone.0248216
Berenguer E., Ferreira J., Gardner T. A., Aragão L. E. O. C., Camargo P. B., Cerri C. E., et al. (2014). A large-scale field assessment of carbon stocks in human-modified tropical forests. Global Change Biol. 20, 3713–3726. doi: 10.1111/gcb.12627
Brasil L. S., Lima E. L., Spigoloni Z. A., Ribeiro-Brasil D. R. G., Juen L. (2021). The habitat integrity index and aquatic insect communities in tropical streams: A meta-analysis. Ecol. Indic. 116, 1470–160X. doi: 10.1016/j.ecolind.2020.106495
Bried J. T., Samways M. J. (2015). A review of odonatology in freshwater applied ecology and conservation science. Freshw. Sci. 34 (3), 1023–1031. doi: 10.1086/682174
Calvão L. B., Nogueira D. S., Montag L. F. A., Lopes M. A., Juen L. (2016). Are Odonata communities impacted by conventional or reduced impact logging? For. Ecol. Manage. 382, 143–150. doi: 10.1016/j.foreco.2016.10.013
Calvão L. B., Siqueira T., Faria A. P. J., Paiva C. K. S., Juen L. (2022). Correlates of Odonata species composition in Amazonian streams depend on dissimilarity coefficient and oviposition strategy. Ecol. Entomol. 47 (6), 998–1010. doi: 10.1111/een.13188
Carvalho F. G., Pinto N. S., Oliveira-Junior J. M. B., Juen L. (2013). Effects of marginal vegetation removal on Odonata communities. Acta Limnol. Bras 25 (1), 10–18. doi: 10.1590/S2179-975X2013005000013
Casanoves F., Pla L., Di Rienzo J. A., Díaz S. (2011). Diversity: a software package for the integrated analysis of functional diversity. Methods Ecol. Evol. 2, 233–237. doi: 10.1111/j.2041-210X.2010.00082.x. F.
Ceballos G., Ehrlich P. R., Dirzo R. (2017). Biological annihilation via the ongoing sixth mass extinction signaled by vertebrate population losses and declines. Proc. Natl. Acad. Sci. 114 (30), 6089–6096. doi: 10.1073/pnas.1704949114
Cezário R. R., Pena-Firme P., Pestana G. C., Vilela D. S., Juen L., Cordero-Rivera A, et al. (2021). In: Santos J. C., Fernandes G. W. (eds) Measuring Arthropod Biodiversity Springer, Cham. doi: 10.1007/978-3-030-53226-0_9
Clark T. E., Samways M. J. (1996). Dragonflies (Odonata) as indicators of biotope quality in the Kruger National Park, South Africa. J. Appl. Ecol. 33, 1001–1012. doi: 10.2307/2404681
Colzani E., Siqueira T., Suriano M. T., Roque F. O. (2013). Responses of aquatic insect functional diversity to landscape changes in Atlantic Forest. Biotropica 45, 343–350. doi: 10.1111/btp.12022
Conrad K. F., Wilson K. H., Wilthifield K., Harvey I. F., Thomas C. J., Sherratt T. N. (2002). Characteristc of dispersing Ischnura elegans and Coenagrion puella (Odonata): age, sex, size, morph and ectoparasitism. Ecography 25, 339–445. doi: 10.1034/j.1600-0587.2002.250406.x
Corbet P. S. (1980). Biology of odonata. Annu. Rev. Entomol. 25, 189–217. doi: 10.1146/annurev.en.25.010180.001201
Corbet P. S. (1999). Dragonflies: behaviour and ecology of odonata (London: Comstock Pub Assoc), 802.
Corbet P. S., May M. L. (2008). Fliers and perchers among Odonata: dichotomy or multidimensional continuum? A provisional reappraisal. Int. J. Odona-tology 11, 155–171. doi: 10.1080/13887890.2008.9748320
Couceiro S., Hamada N., Forberg B. R., Pimentel T. P. (2012). A macroinvertebrate multimetric index to evaluate the biological condition of streams in the Central Amazon region of Brazil. Ecol. Indic. 18, 118–125. doi: 10.1016/j.ecolind.2011.11.001
Courtney L. A., Clements W. H. (1998). Effects of acidic pH on benthic macroinvertebrate communities in stream microcosms. Hydrobiologia 379, 135–145. doi: 10.1023/A:1003442013650
Dalzochio M. S., Baldin R., Stenert C., Malchik L. (2015). How does the management of rice in natural ponds alter aquatic insect Community functional structure? Mar. Freshw. Res. 67, 1644–1654. doi: 10.1071/MF14246
Dalzochio M. S., Costa J. M., Uchôa M. A. (2011). Diversity of Odonata (Insecta) in lotic systems from Serra da Bodoquena, Mato Grosso do Sul State, Brazil. Rev. Bras. Entomologia 5 (1), 88–94. doi: 10.1590/S0085-56262011000100014
Dalzochio M. S., Périco E., Renner S., Sahlén G. (2018). Effect of tree plantations on the functional composition of Odonata species in the highlands of southern Brazil. Hydrobiologia 808, 283–300. doi: 10.1007/s10750-017-3431-9
Del Palacio A., Muzón J. (2019). Redescription of Erythrodiplax chromoptera Borror 1942 with comments on the morphology of the vesica sperMalis (Odonata: Libellulidae). Zool. Anz. 278, 90–94. doi: 10.1016/j.jcz.2018.12.004
Del Palacio A. D., Muzón J., Juen L., Ferreira V. R. S., Batista J. D. (2020). Erythrodiplax nataliae sp. nov., a new species for the state of Mato Grosso, Brazil. |. Ann. Braz. Acad. Sci. 92 (2), 3–7. doi: 10.1590/0001-3765202020181149
De Marco P., Latini A. O., Resende D. C. (2005). Thermoregulatory constraints on behavior: patterns in a Neotropical dragonfly assemblage. Neotrop. Entomol. 34, 155–162. doi: 10.1590/S1519-566X2005000200002
De Marco P., Nóbrega C. C., Souza R. A., Neiss U. G. (2015). Modeling the distribution of a rare Amazonian odonate in relation to future deforestation. Freshw. Sci. 34 (3), 1123–1132. doi: 10.1086/682707
De Marco P. Jr., Resende D. C. (2002). Activity patterns and thermoregulation in a tropical dragonfly assemblage. Odonatologica 31 (2), 129–138.
Ding N., Yang W., Zhou Y., GonzaLez-Bergonzoni I., Zhang J., Chen K., et al. (2017). Different responses of functional traits and diversity of stream macroinvertebrates to environmental and spatial factors in the Xishuangbanna watershed of the upper Mekong River Basin, China. Sci. Total Environ. 574, 288–299. doi: 10.1016/j.scitotenv.2016.09.053
Dutra S., De Marco P. Jr. (2015). Bionomic differences in odonates and their influence on the efficiency of indicator species of environmental quality. Ecol. Indic. 49, 132–142. doi: 10.1016/j.ecolind.2014.09.016
Fahrig L. (2003). Effects of habitat fragmentation on biodiversity. Annu. Rev. Ecol. Evol. Syst. 34, 487–515. doi: 10.1146/annurev.ecolsys.34.011802.132419
Feitosa M. A. C., Julião G. M., Costa M. D. P., Belém B., Pessoa F. A. C. (2012). Diversity of sand flies in domiciliary environment of Santarém, state of Pará, Brazil: species composition and abundance patterns in rural and urban areas. Acta Amazonica 42 (4), 507–514. doi: 10.1590/S0044-59672012000400008
Ferreira F. C., Petrere J. M. (2007). Anthropic effects on the fish community of Ribeirão Claro, Rio Claro, SP, Brazil. Braz. J. Biol. 67, 23–32. doi: 10.1590/S1519-69842007000100004
Fincke O. (1986). Lifetime reproductive success and the opportunity for selection in a nonterritorial damselfly (Odonata: Coenagrionidae). Evolution 40, 791–803. doi: 10.1111/j.1558-5646.1986.tb00539.x
Firmiano K. R., Castro D. M. P., Linares M. S., Callisto M. (2021). Functional responses of aquatic invertebrates to anthropogenic stressors in riparian zones of Neotropical savanna streams. Sci. Total Environ. 753, 141865. doi: 10.1016/j.scitotenv.2020.141865bb
Frank J. H. (1983). “Bromeliad phytotelmata and their biota, especially mosquitoes,” in Phytotelmata: terrestrial plants as host for aquatic insect communities. Eds. Frank J. H., Lounibos L. P. (Marlton, New Jersey, EUA: Plexus), 101–128.
Fulan J. A., Henry R. (2007). Distribuição temporal de imaturos de Odonata (Insecta) associada a Eichhornia azurea (Kunth) na Lagoa do Camargo, Rio Paranapanema, São Paulo. Rev. Bras. Entomologia 51 (2), 224–227. doi: 10.1590/S0085-56262007000200013
Gardner T. A., Ferreira J., Barlow J., Lees A. C., Parry L., Vieira I. C. G., et al. (2013). A social and ecological assessment of tropical land-uses at multiple scales: The Sustainable Amazon Network. Philos. Trans. R. Soc. Ser. B 368 (1619), 20120166. doi: 10.1098/rstb.2012.0166
Garrison R. W. (1990). A synopsis of the genus Hetaerina with descriptions of four new species (Odonata: Calopterigidae). Trans. Am. Entomological Soc. 116 (1), 175–259.
Garrison R. W. (2009). A synopsis of the genus Telebasis (Odonata: Coenagrionidae). Int. J. Odonatol. 12 (1), 1–121. doi: 10.1080/13887890.2009.9748331
Garrison R. W., Von Ellenrieder N., Louton J. A. (2006). Dragonfly genera of the new world: an illustrated and annotated key to the anisoptera (Baltimore: The Johns Hopkins University Press), 368.
Garrison R. W., Von Ellenrieder N., Louton J. A. (2010). Damselfly genera of the new world: an illustrated and annotated key to the zygoptera (Baltimore: The Johns Hopkins University Press), 490.
Giehl N. F. S., Dias-Silva K., Juen L., Batista J. D., Cabette H. S. R. (2014). Taxonomic and numerical resolutions of Nepomorpha (Insecta: Heteroptera). Cerrado streams. PloS One 9 (8), e103623. doi: 10.1371/journal.pone.0103623
Gower J. C. (1966). Some distance properties of latent root and vector methods used in multivariate analysis. Biometrika 53 (3-4), 325–338. doi: 10.1093/biomet/53.3-4.325
Grönroos M., Heino J., Siqueira T., Landeiro V. L., Kotanen J., Bini L. M. (2013). Metacommunity structuring in stream networks: roles of dispersal mode, distance type, and regional environmental context. Ecol. Evol. 3 (13), 4473–4487. doi: 10.1002/ece3.834
Hamada N., Nessimian J. L., Querino R. B. (2014). Insetos aquáticosaquáticos na Amazônia brasileira: taxonomia, biologia e ecologia (Manaus, Brazil: Editora do INPA).
Harabis F., Dolný A. (2010). Ecological factors determining the density-distribution of Central European dragonflies (Odonata). Eur. J. Entomol. 107 (4), 571–577. doi: 10.14411/eje.2010.066
Hedenström A., Møller A. P. (1992). Morphological adaptations to song flight in passerine birds: a comparative study. Proc. R. Soc. London Ser. B Biol. Sci. 247, 183–187. doi: 10.1098/rspb.1992.0026
Heino J., Melo A. S., Siqueira T., Soininen J., Valenko S., Bini M. (2014). Metacommunity organization, spatial extent and dispersal in aquatic systems: patterns, processes and prospects. Freshw. Biol. 60 (5), 845–869. doi: 10.1111/fwb.12533
Heino J., Peckarsky B. L. (2014). Integrating behavioral, population and large-scale approaches for understanding stream insect communities. Insect Sci. 2, 7–13. doi: 10.1016/j.cois.2014.06.002
Heinrich B. (1974). Thermoregulation in endothermic insects. Science 185, 747–756. doi: 10.1126/science.185.4153.747
Heiser M., Schmitt T. (2010). Do different dispersal capacities influence the biogeography of the western Palearctic dragonflies (Odonata)? Biol. J. Linn. Soc. 99 (1), 177–195. doi: 10.1111/j.1095-8312.2009.01349.x
Jackson D. A. (1993). Stopping rules in principal components analysis: a comparison of heuristical and statistical approaches. Ecology 74, 2204–2214. doi: 10.2307/1939574
Jacob U., Walther H., and Klenke R. (1984). Aquatic insect larvae as indicators of limiting minimal contents of dissolved oxygen-part II. Aquat. Insects 6 (3), 185–190. doi: 10.1080/01650428409361183
Johansson F., Söderquist M., Bokma F. (2009). Insect wing shape evolution: independent effects of migratory and mate guarding flight on dragonfly wings. Biol. Jounal Linn. Soc. 97, 362–372. doi: 10.1111/j.1095-8312.2009.01211.x
Juen L., De Marco P. Jr. (2011). Odonate biodiversity in terra- firme forest streamlets in Central Amazonia: on the relative effects of neutral and niche drivers at small geographical extents. Insect Conserv. Diversity 4 (4), 265–274. doi: 10.1111/j.1752-4598.2010.00130.x
Juen L., De Marco P. Jr. (2012). Dragonfly endemism in the Brazilian Amazon: competing hypotheses for biogeographical patterns. Biodiversity Conserv. 21, 3507–3521. doi: 10.1007/s10531-012-0377-0
Juen L., Oliveira-Junior J. M. B., Shimano Y., Mendes T. P., Cabette H. S. R. (2014). Composição e riqueza de Odonata (Insecta) em riachos com diferentes níveis de conservação em um ecótone Cerrado-Floresta Amazônica. Acta Amazonica 44, 175–184. doi: 10.1590/S0044-59672014000200008
Laliberté E., Legendre P., Shipley B. (2014). FD: measuring functional diversity from multiple traits, and other tools for functional ecology. R Package version 1, 0–12.
Lencioni F. A. A. (2005). The Damselflies of Brazil: An Illustrated Identification Guide 1 – The Noncoenagrionidae families. All Print Editora, São Paulo, Brazil, 324 p.
Lencioni F. A. A. (2006). Damselflies of Brazil, an illustrated identification guide: coenagrionidae families (São Paulo, Brazil: All Print Editora), 330.
MacCreadie J. W., Adler P. H. (2012). The roles of abiotic factors, dispersal, and species interactions in structuring stream assemblages of black flies (Diptera: Simuliidae). Aquat. Biosyst. 8 (14), 1–14. doi: 10.1186/2046-9063-8-14
Machado A. B. M. (1954). Elga santosi sp. n. e redescrição de Elga leptostyla Ris 1911 (Odonata, Libellulidae). Rev. Bras. Biol. 14 (3), 303–312.
May M. L. (1976). Thermoregulation and adaptation to temperature in dragonflies (Odonata: Anisoptera). Ecol. Monogr. 46, 1–32. doi: 10.2307/1942392
Mendoza-Penagos C., Calvão L. B., Juen L. (2021). A new biomonitoring method using taxonomic families as substitutes for the suborders of the Odonata (Insecta) in Amazonian streams. Ecol. Indic. 124, 1470–160x. doi: 10.1016/j.ecolind.2021.107388
Mcauley S. J. (2013). Relationship between morphology dispersal, and habitat distribution in three species of Libellula (Odonata: Anisoptera). Aquat. Insect 34 (3–4), 195–204. doi: 10.1080/01650424.2013.800557
Michiels N. K., André A. (1990). Costs and benefits associated with oviposition site selection in the dragonfly Sympetrum danae (Odonata: Libellulidae). Anim. Behav. 40 (4), 668–678. Dhondt. doi: 10.1016/S0003-3472(05)80696-7
Miguel T. B., Oliveira-Junior J. M. B., Ligeiro R., Juen L. (2017). Odonata (Insecta) as a tool for the biomonitoring of environmental quality. Ecol. Indic 81, 555–566. doi: 10.1016/j.ecolind.2017.06.010
Monteiro-Júnior C. S., Juen L., Hamada N. (2015). Analysis of urban impacts on aquatic habitats in the central Amazon basin: adult odonates as bioindicators of environmental quality. Ecol. Indic. 48, 303–311. doi: 10.1016/j.ecolind.2014.08.021
Monteiro-Júnior C. S., Couceiro S. R. M., Hamada N., Juen L. (2013). Effect of vegetation removal for road building on richness and composition of Odonata communities in Amazonia, Brazil. Int. J. Odonat. 16 (2), 135–144. doi: 10.1080/13887890.2013.764798
Moore A. J., Machado A. B. M. (1992). A note on Cacoides latro (Erichson), a territorial lacustrine gomphid (Anisoptera: Gomphidae). Odonatologica 21 (4), 499–503.
Moura N. G. M., Lees A. C. L., Andretti C., Davis B., Solar R., Aleixo A., et al. (2013). Avian biodiversity in multiple-use landscapes of the Brazilian Amazon. Biol. Conserv. 167, 339–348. doi: 10.1016/j.biocon.2013.08.023
Nepstad D. C., Moutinho P. R. S., Dias-Filho M. B., Davidson E., Cardinot G., Markewitz D., et al. (2002). The effects of rainfall exclusion on canopy processes and biogeochemistry of an Amazon Forest. J. Geophysical Res. 107, 80–85. doi: 10.1029/2001JD000360
Nessimian J. L., Venticinque E., Zuanon J., De Marco P., Gordo M., Fidelis L., et al. (2008). Land use, habitat integrity, and aquatic insect assemblages in Central Amazonian streams. Hydrobiologia 614, 117–131. doi: 10.1007/s10750-008-9441-x
Nilsson-Ortman V., Stoks R., De Block M., Johansson F. (2012). Generalists and specialists along a latitudinal transect: patterns of thermal adaptation in six species of damselflies. Ecology 93, 1340–1352. doi: 10.1890/11-1910.1
Nóbrega C. C., De Marco P. (2011). Unprotecting the rare spe- cies: a niche-based gap analysis for odonates in a core Cerrado area. Diversity Distributions 17 (3), 491–505. doi: 10.1111/j.1472-4642.2011.00749.x
Oksanen J., Simpson G., Blanchet F., Kindt R., Legendre P., Minchin P., et al. (2022). Vegan community ecology package. R package version 2.6-4. Available at: https://CRAN.R-project.org/package=vegan.
Oliveira-Junior J. M. B., Cabette H. S. R., Pinto N. S., Juen L. (2013). As variações na comunidade de Odonata (Insecta) em córregos podem ser preditas pelo Paradoxo do Plâncton? Explicando a riqueza de espécies pela variabilidade ambiental. EntomoBrasilis 6, 1–8. doi: 10.12741/ebrasilis.v6i1.250
Oliveira-Junior J. M. B., De Marco P., Dias-Silva K., Leitão R. P., Leal C. G., Pompeu P. S., et al. (2017). Effects of human disturbance and riparian conditions on Odonata (Insecta) assemblages in eastern Amazon basin streams. Limnologica 66, 31–39. doi: 10.1016/j.limno.2017.04.007
Oliveira-Junior J. M. B., Juen L. (2019). Structuring of dragonfly communities (Insecta: odonata) in eastern Amazon: effects of environmental and spatial factors in preserved and altered streams. Insects 10 (10), 322. doi: 10.3390/insects10100322
Oliveira-Junior J. M. B., Rocha T. S., Vinagre S. F., MIranda-Filho J. C., Mendoza-Penagos C. C., Dias-Silva K., et al. (2022). A bibliometric analysis of the global research in odonata: trends and gaps. Diversity 14, 1074. doi: 10.3390/d14121074
Oliveira-Junior J. M. B., Shimano Y., Gardner T. A., Hughes R. M., De Marco P. Jr, Juen. L. (2015). Neotropical dragonflies (Insecta: Odonata) as indicators of ecological condition of small streams in the eastern Amazon. Austral Ecol. 40 (6), 733–744. doi: 10.1111/aec.12242
Oliveira-Junior J. M. B., Teodósio M. A., Juen L. (2021). Patterns of co-occurrence and body size in dragonflies and damselflies (Insecta: Odonata) in preserved and altered Amazonian streams. Austral Entomol. 60 (2), 436–450. doi: 10.1111/aen.12535
Paulson D. R. (2004). Why do some zygopterans (Odonata) perch with open wings? Int. J. Odonatatology 7 (3), 505–515. doi: 10.1080/13887890.2004.9748235
Pereira D. F. G., Oliveira-Junior J. M. B., Juen L. (2019). Environmental changes promote larger species of Odonata (Insecta) in Amazonian streams. Ecol. Indic. 98, 179–192. doi: 10.1016/j.ecolind.2018.09.020
Poff N. L., Olden J. D., Vieira N. K. M., Finn D. S., Simmons M. P., Kondratieff B. C. (2006). Functional trait niches of North American lotic insects: traits-based ecological applications in light of phylogenetic relationships. J. North Am. Benthological Soc. 25, 730–755. doi: 10.1899/0887-3593(2006)025[0730:FTNONA]2.0.CO,2
Powney G. D., Cham S. S. A., Smallshire D., Isaac N. J. B. (2015). Trait correlates of distribution trends in the Odonata of Britain and Ireland. PeerJ 3, e1410. doi: 10.7717/peerj.1410
Putz F. E., Redford H. K. (2010). The importance of defining ‘Forest’: Tropical forest degradation, deforestation, long-term phase shifts, and further transitions. Biotropica 42 (1), 10–20. doi: 10.1111/j.1744-7429.2009.00567.x
R Core Team (2021). R: A language and environment for statistical computing (Vienna, Austria: R Foundation for Statistical Computing). Available at: https://www.R-project.org/.
Remsburg A. J., Turner M. C. (2009). Aquatic and terrestrial drivers of dragonfly (Odonata) assemblages within and among north-temperate lakes. J. North Am. Benthological Soc. 28 (1), 44–56. doi: 10.1899/08-004.1
Resende B. O., Ferreira V., Brasil L., Calvão L. B., Mendes T. P., Carvalho F. G., et al. (2021). Impact of environmental changes on the behavioral diversity of the Odonata (Insecta) in the Amazon. Sci. Rep. 11 (1), 2045–2322. doi: 10.1038/s41598-021-88999-7
Resende D. C., De Marco P. Jr. (2010). First description of reproductive behavior of the Amazonian damselfly Chalcopteryx rutilans (Rambur) (Odonata, Polythoridae). Rev. Bras. Entomologia 54 (3), 436–440. doi: 10.1590/S0085-56262010000300013
Rodrigues M. E., Moura E. B., Koroiva R., Borges A. C. P., Roque F. O. (2018). Survey of dragonflies (Odonata) in palm swamps of cerrado hotspot. Entomol. News 128 (1), 24–38. doi: 10.3157/021.128.0104
Rodrigues M. E., Roque F. O., Guillermo-Ferreira R., Saito V. S., Samways M. J. (2019). Egg-laying traits reflect shifts in dragonfly assemblages in response to different amount of tropical forest cover. Insect Conserv. Diversity 12 (3), 231–240. doi: 10.1111/icad.12319
Rodrigues M. E., Roque F. O., Quintero J. M. O., Castro-Pena J. C. (2016). Nonlinear responses in damselfly community along a gradient of habitat loss in a savanna landscape. Biol. Conserv. 194, 113–120. doi: 10.1016/j.biocon.2015.12.001
Samways M. J., Taylor S. (2004). Impacts of invasive alien plants on Red-Listed South African dragonflies (Odonata). South Afr. J. Sci. 100, 78–80.
Sánchez-Bayo F., Wyckhuys K. A. G. (2019). Worldwide decline of the entomofauna: A review of its drivers. Biol. Conserv. 232, 8–27. doi: 10.1016/j.biocon.2019.01.020
Silva-Pinto N., Juen L., Cabette H. S. R., De Marco P. Jr. (2012). Fluctuating asymmetry and wing size of Argia tinctipennis Selys (Zygoptera: Coenagrionidae) in relation to Riparian Forest Preservation Status. Neotrop. Entomol. 41, 1–9. doi: 10.1007/s13744-012-0029-9
Southwood T. R. (1977). Habitat, the templet for ecological strategies? J. Anim. Ecol. 46, 337–365. doi: 10.2307/3817
Souza L. O. I., Costa J. M. (2006). “Inventário da Odonatofauna no Complexo Aporé-Sucuriú,” in Biodiversidade do Complexo Aporé-Sucuriú. Subsídios à conservação e manejo do bioma Cerrado. Eds. Pagotto T. C. S., Souza P. R. (Campo Grande: Editora da UFMS), 81–88, 308. org.
Statzner B., Bis B., Dolédec S., Usseglio-Polatera P. (2001). Perspectives for biomonitoring at large spatial scales: a unified measure for the functional composition of invertebrate communities in European running waters. Basic Appl. Ecol. 2, 73–85. doi: 10.1078/1439-1791-00039
Stoks R., Córdoba-Aguilar A. (2012). Evolutionary ecology of Odonata: A complex life cycle perspective. Annu. Rev. Entomol. 57, 249–265. doi: 10.1146/annurev-ento-120710-100557
Strahler A. N. (1957). Quantitative analysis of watershed geomorphology. Trans. Am. Geophysical Union 38 (6), 913–920. doi: 10.1029/tr038i006p00913
Suhonen J., Suutari E., Kaunisto K. M., Krams K. (2013). Patch area of macrophyte Stratioites aloides as a critical resource for declining dragonfly Aeshna viridis. J. Insect Conserv. 2, 393–398. doi: 10.1007/s10841-012-9521-0
Tavares R. I. S., Mandelli A. M., Mazão G. R., Guillermo-Ferreira R. (2017). The relationship between habitat complexity and emergence time in damselflies. Limnologica 65 (1), 1–3. doi: 10.1016/j.limno.2017.04.009
Townsend C. R., Hildrew A. G. (1994). Species traits in relation to a habitat templet for river systems. Freshw. Biol. 31, 265–275. doi: 10.1111/j.1365-2427.1994.tb01740.x
Veloso H. P., Rangel Filho A. L. R., Lima J. C. A. (1991). Classificação da vegetação brasileira adaptada a um sistema universal (Rio de Janeiro, Brazil: IBGE), 123.
Vilela D. S., Ferreira R. G., Del-Claro K. (2016). The Odonata community of a Brazilian vereda: seasonal patterns, species diversity and rarity in a palm swamp environment. Biosci. J. 32 (1), 486–495. doi: 10.14393/BJ-v32n2a2016-30491
Violle C., Navas M. L., Vile D., Kazakou E., Fortunel C., Hummel I., et al. (2007). Let the concept of trait be functional. Oikos 116, 882–892. doi: 10.1111/j.0030-1299.2007.15559.x
Watrin O. S., Rocha A. M. A. (1992). Levantamento da Vegetação Natural e do Uso da Terra no Município de Paragominas (PA) Utilizando Imagens TM/Landsat Vol. p (Belém, Brazil: EMBRAPA/CPATU), Boletim de Pesquisa), 40. EMBRAPA/CPATU.
Keywords: Amazon, dragonflies, oviposition, thermoregulation, environmental change
Citation: Rocha TS, Calvão LB, Juen L and Oliveira-Junior JMB (2023) Effect of environmental integrity on the functional composition of the Odonata (Insecta) community in streams in the Eastern Amazon. Front. Ecol. Evol. 11:1166057. doi: 10.3389/fevo.2023.1166057
Received: 14 February 2023; Accepted: 15 August 2023;
Published: 06 September 2023.
Edited by:
Rachael E. Bonoan, Providence College, United StatesReviewed by:
Eduardo Périco, Universidade do Vale do Taquari – Univates, BrazilCharl Deacon, Stellenbosch University, South Africa
Copyright © 2023 Rocha, Calvão, Juen and Oliveira-Junior. This is an open-access article distributed under the terms of the Creative Commons Attribution License (CC BY). The use, distribution or reproduction in other forums is permitted, provided the original author(s) and the copyright owner(s) are credited and that the original publication in this journal is cited, in accordance with accepted academic practice. No use, distribution or reproduction is permitted which does not comply with these terms.
*Correspondence: Tainã Silva Rocha, dGFpbmFzaWx2YS50ckBnbWFpbC5jb20=; Lenize Batista Calvão, bGVuaXplY2FsdmFvQGdtYWlsLmNvbQ==