- 1Kenya Wildlife Service, Nairobi, Kenya
- 2COCON-CEES, University of Groningen, Centre for Life Sciences, Groningen, Netherlands
- 3University of Hohenheim, Institute of Crop Science, Biostatistics Unit, Stuttgart, Germany
- 4University of Groningen, Groningen Institute for Evolutionary Life Sciences, Conservation Ecology Group, Groningen, Netherlands
Large mammals, such as hippopotamuses (Hippopotamus amphibius), can significantly alter the landscape, vegetation composition, and structure in savannas through their grazing habits and ecosystem engineering effects, especially around rivers. However, livestock grazing can strongly change these effects, as seen in the Kenyan Maasai Mara Ecosystem. In this region, the increasing intensity of livestock grazing along local rivers, resulting from the transition of Maasai pastoralists from a semi-nomadic lifestyle to one based on pastoral ranches near the Maasai Mara National Reserve, may alter the impact of hippo grazing on riparian vegetation. We investigated the differences in vegetation structure, plant species richness and composition between landscapes predominantly grazed by hippopotami and livestock. We studied 25 transects, each measuring 5 km and having 13 sampling plots of 10 × 10 m2 located at varying distances from rivers in the reserve (n = 16) and pastoral ranches (n = 9). We measured the height and estimated the percent cover of grasses, forbs, shrubs, and bare ground, as well as plant species composition and richness and grazing intensity. Our results show that the riverine areas were more intensely and homogeneously grazed in the pastoral ranches than in the reserve, and in the dry than in the wet season in both landscapes. In addition, grazing intensity decreased with distance from rivers in the reserve in both seasons, but only in the wet season in the pastoral ranches. The mean plant species richness was similar in both landscapes and decreased linearly with distance from rivers, but varied with grazing intensity due to differences in forb and shrub species. However, plant species composition differed significantly between the reserve and the pastoral ranches. Furthermore, species similarity indices declined with increasing distance from water. These results suggest that the effects of livestock and hippo grazing intensity on vegetation structure and spatial heterogeneity interact, and vary with landscape and season. While both hippopotamus and livestock grazing can modify riparian vegetation, livestock grazing impact on plant species composition, vegetation structure, and spatial heterogeneity appears to be more persistent, declining less rapidly with increasing distance from water. Consequently, hippo and livestock differentially impact landscape heterogeneity.
Introduction
Large herbivores play significant roles in shaping vegetation and landscapes in ecosystems through their grazing habits, which can influence nutrient cycling, vegetation composition, productivity, and structure (McNaughton, 1985, 1988; Frank et al., 1998; Olff et al., 1999; Danell et al., 2006). The impact of grazing on vegetation can vary with the species, density, body size and spatial and temporal distribution of herbivores (Belsky, 1983; McNaughton, 1986; Olff and Ritchie, 1998; Bakker et al., 2006). Furthermore, such variations may reflect interspecific distinctions in the evolutionary history, physiological (e.g., digestive physiology) and behavioural (e.g., degree of gregariousness) characteristics of herbivores and the consequences of these factors for ecosystem functioning and interactions with land use changes, spatial and temporal resource gradients (McCauley et al., 2018; Voysey et al., 2023). Continuous and intense grazing can have a more negative effect on vegetation than periodic, intense grazing that allows enough time for vegetation recovery. As a result, herders usually move animals between pastures to allow plant regrowth (Jordan et al., 2016; Turner and Schlecht, 2019). Traditional pastoralists in East Africa have long recognized this and also practice mobility with their livestock, moving them between pastures and allowing sufficient time for vegetation recovery from heavy utilization. This mobility also helps the livestock efficiently utilize grazing resources that are distributed unevenly in space and time (Homewood and Rodgers, 1991; Oba et al., 2000).
Herbivore grazing and trampling can have multiple impacts on soils and vegetation. For example, the effect of grazing on species richness varies regionally based on soil productivity, with ecosystems with moderate to high productivity tending to have higher plant species diversity than those with lower productivity (Milchunas et al., 1998; Olff and Ritchie, 1998; Bakker et al., 2006). Low to moderate grazing intensity can enhance plant species diversity, while high intensity grazing can reduce it (Milchunas et al., 1988; Hobbs and Huenneke, 1992; Hodgson and Illius, 1996). When herbivores effectively suppress dominant plant species, they can have a significant positive impact on plant species diversity (Olff and Ritchie, 1998; Koerner et al., 2018). As a result, intermediate grazing intensities that create moderate disturbances can support high levels of diversity in grassland communities (Grime, 1973; Milchunas et al., 1988; Hobbs and Huenneke, 1992). Very high grazing intensity can also reduce diversity of other species groups, such as invertebrates (van Klink et al., 2015; Tadey, 2023) with significant consequences for essential ecosystem functions such as pollination (Tadey, 2023).
Grazing can also have other consequences, such as trampling that slows down nutrient cycling (Schrama et al., 2013) and/or create niches for colonizing species and facilitate propagule dispersal (Bullock and Marriott, 2000; Bakker and Olff, 2003). Additionally, grazing by mixed herds of different herbivore species or multiple species associations can enhance vegetation productivity and plant species diversity, although the effect can vary based on the specific herbivore species involved and the availability of resources that limit plant growth, such as water, nutrients, and light (Bell, 1971; Ritchie at al., 1998; Pausas and Austin, 2001; Burkepile et al., 2017). Moreover, livestock grazing can strongly modify plant flowering phenology and fitness such that as grazing intensity increases, blooming starts earlier, reduces in intensity and lasts for a shorter period, leading to earlier fruiting (Tadey, 2020). Overall, the effect of grazing on vegetation can vary with the environment, production system, and spatial and temporal scales.
The grazing of riparian savanna habitats by hippopotamuses or livestock can lead to large ecological impacts, including strong depletion of herbaceous vegetation, increased erosion of river banks, soil compaction and fertilization by dung. Both species groups can have large impacts on riparian nutrient cycling but with different effects between hippo and livestock (Masese et al., 2020). The alteration of vegetation structure due to grazing can negatively affect the habitat’s suitability for some wildlife species, while improving it for herbivores that prefer short grass. In addition, hippopotamuses and livestock may compete for grazing resources, particularly near water sources. However, the relative impacts of these two herbivore groups on vegetation structure, species richness, and composition have received relatively little attention in both protected and pastoral landscapes. In this study, we compare the effects of hippopotamus and livestock grazing on herbaceous plant species richness and composition in the Kenyan Maasai Mara Ecosystem (the Mara) in Narok county. We also examine how the effects of hippopotamus and livestock grazing intensity on plant cover, height, species richness and composition vary with increasing distance from water sources in a protected reserve and adjoining pastoral areas. Grazing intensity is the cumulative effects of grazing animals in rangelands in a given time period. It can be measured in various ways including through percent herbage use, amount of forage standing crop remaining at the end of the grazing cycle, percentages of grazed and ungrazed plants, plant stubble heights, litter, or carry over vegetation from previous years and visual appearance (Holechek et al., 1998).
The Mara region in Africa has a long history of coexistence between pastoralists and wildlife dating back at least 3,000 years (Marshall, 1990; McCabe, 2003). Traditional pastoralism remains a major form of land use and involves herding cattle, sheep and goats, and donkeys to a far lesser extent. The Mara supports a robust and diverse large wild herbivore assemblage, with largely stable but markedly seasonally fluctuating livestock numbers between 1977 and 2002 (Broten and Said, 1995; Serneels and Lambin, 2001; Lamprey and Reid, 2004). The Mara is the main dry season range for the famous Serengeti-Mara migration of over 1.5 million wildebeest (Connochaetes taurinus), zebra (Equus quagga) and eland (Taurogratus oryx). However, marked declines by large wild herbivores in the Mara during 1977–2003 were attributed to their progressive exclusion from the pastoral ranches by land use changes, including expansion of large-scale mechanized commercial and small-scale subsistence agriculture and settlements, human population growth, increasing livestock numbers, illicit wildlife harvests and marked climatic variability (Homewood et al., 2001; Ogutu et al., 2009; Ogutu et al., 2011; Ogutu et al., 2016). These changes progressively degrade, fragment and contract wildlife habitats in the ecosystem, intensifying overlapping use and competition between livestock and wild herbivores. Together with land subdivision, individuation and privatization of land tenure (Kimani and Pickard, 1998), sedentarization of the formerly semi-nomadic Maasai pastoralists and the associated intensification of land use (Western et al., 2009), these processes progressively compress wildlife and livestock distributions and heighten conflicts among the competing and incongruent land uses, including along riparian habitats.
The aim of this study is to examine the differences in vegetation grazing between protected areas dominated by hippopotamuses and pastoral ranches dominated by livestock. It is assumed that these two landscapes will have different vegetation grazing patterns due to the differences in the life histories and grazing strategies of hippopotamuses and livestock. It is expected that hippopotamuses will create fewer patches of bare ground than livestock due to their plucking of grasses and creation of short grass lawns, while livestock (especially cattle) will pull up shallow-rooted grasses due to their bulk-feeding style. It is also anticipated that the intensity of grazing by hippopotamuses and livestock will have differential impacts on vegetation, leading to differences in vegetation structure, plant species abundance and richness, and the dominance of certain plant species. Specifically, it is expected that vegetation height and cover will increase with distance from water sources due to decreasing grazing intensity, and that plant species richness will also increase with distance from water and be higher in areas grazed primarily by hippopotamuses than by livestock. Finally, it is expected that both hippopotamus and livestock grazing will facilitate the establishment and spread of invasive and annual plant species through competitive release, particularly in heavily grazed areas due to more bare soil. Examining these hypotheses is important for understanding the long-term effects of pastoralist sedentarization and the grazing of their livestock on the biodiversity of riparian habitats and associated forage resources in savannas.
Materials and methods
Study area
The Maasai Mara Ecosystem (Mara) is located in southwestern Kenya, between latitudes 34°45´ E and 36°00´ E. It is bordered by the Serengeti National Park in Tanzania to the south and the Siria escarpment to the west, and is part of the larger Greater Serengeti-Mara ecosystem, which spans an area of approximately 40,000 km2 and includes several wildlife conservation areas and multiple land use zones in both Kenya and Tanzania. The Mara itself covers around 7,500 km2, with the Maasai Mara National Reserve (MMNR) comprising 1,530 km2 and the adjacent former pastoral ranches (including Koyiaki, Olkinyei, Siana, Lemek, and Ol Chorro Oiroua) covering a total of around 4,000 km2 (Figure 1). There are also several other former group ranches that together make up the remaining 2,000 km2. Some of the previously communal areas in the Mara region have been divided into private, fenced grazing areas, while others have been transformed into conservancies that continue to support both wildlife and livestock. The Mara region has a bimodal rainfall pattern, with short rains falling from November to February (early wet season) and long rains from March to June (late wet season), though January and February are often dry (Norton-Griffiths et al., 1975). The dry season lasts from July to October and can be divided into the early (July–August) and late (September–October) dry seasons (Figure 2). The Mara is characterized by grassland vegetation, with scattered scrublands and woodlands, particularly along drainage lines and on hilltops (Epp and Agatsiva, 1980). The region is dominated by deep, nutrient-rich clay soils known as black cotton soils (Williams, 1964; Glover, 1966), and receives an annual rainfall of about 877 mm in the southeast (Ololaimutia Gate) to about 1,341 mm in the northwest (Kichwa Tembo) (Ogutu et al., 2011).
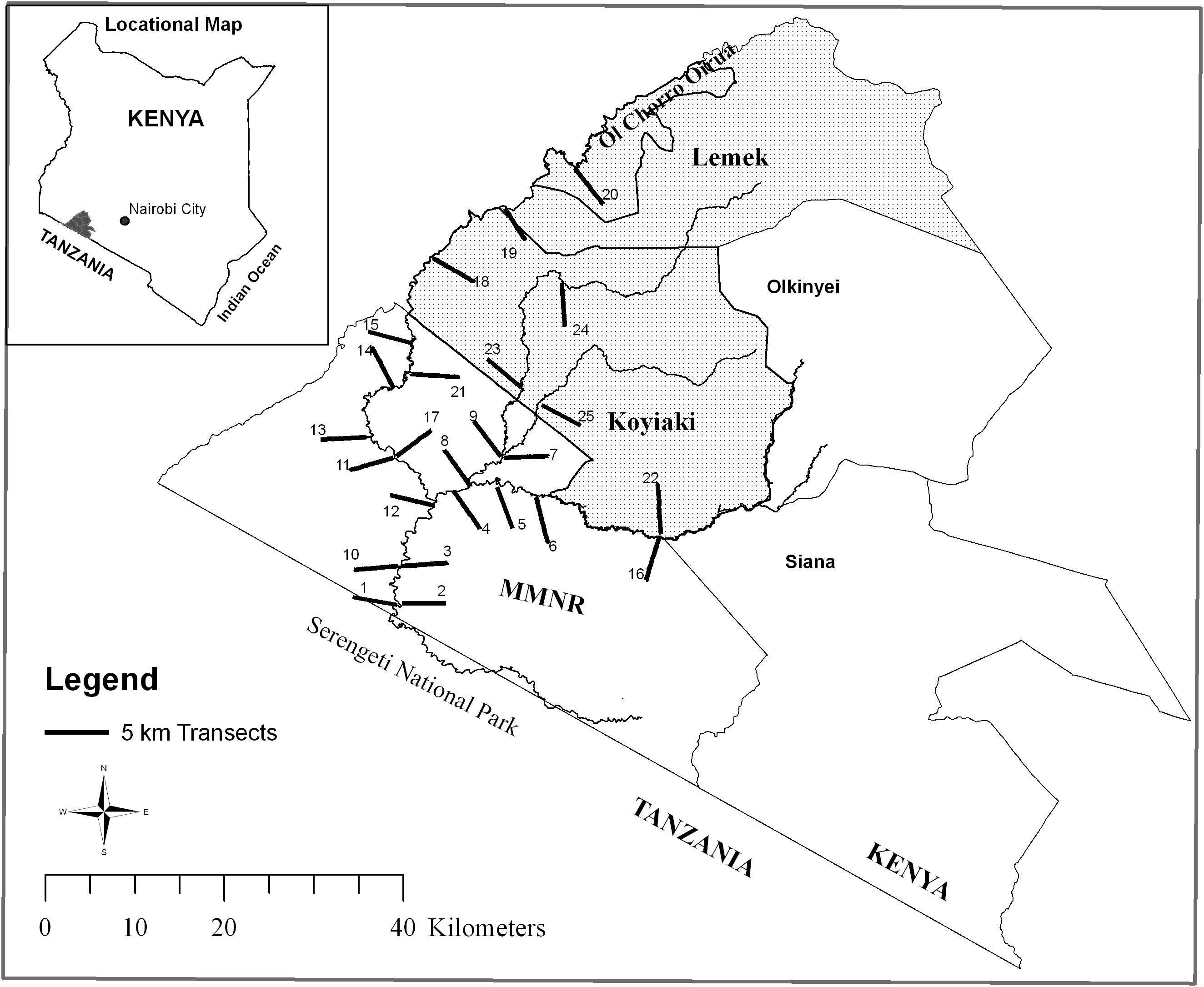
Figure 1 Map of the Maasai Mara National Reserve and the adjoining pastoral ranches showing the transects (numbered) radiating from rivers and sampled during 2007–2008. The study area encompassed the protected Maasai Mara National Reserve and the Koyiaki, Lemek and Ol Chorro Oiroua pastoral ranches.
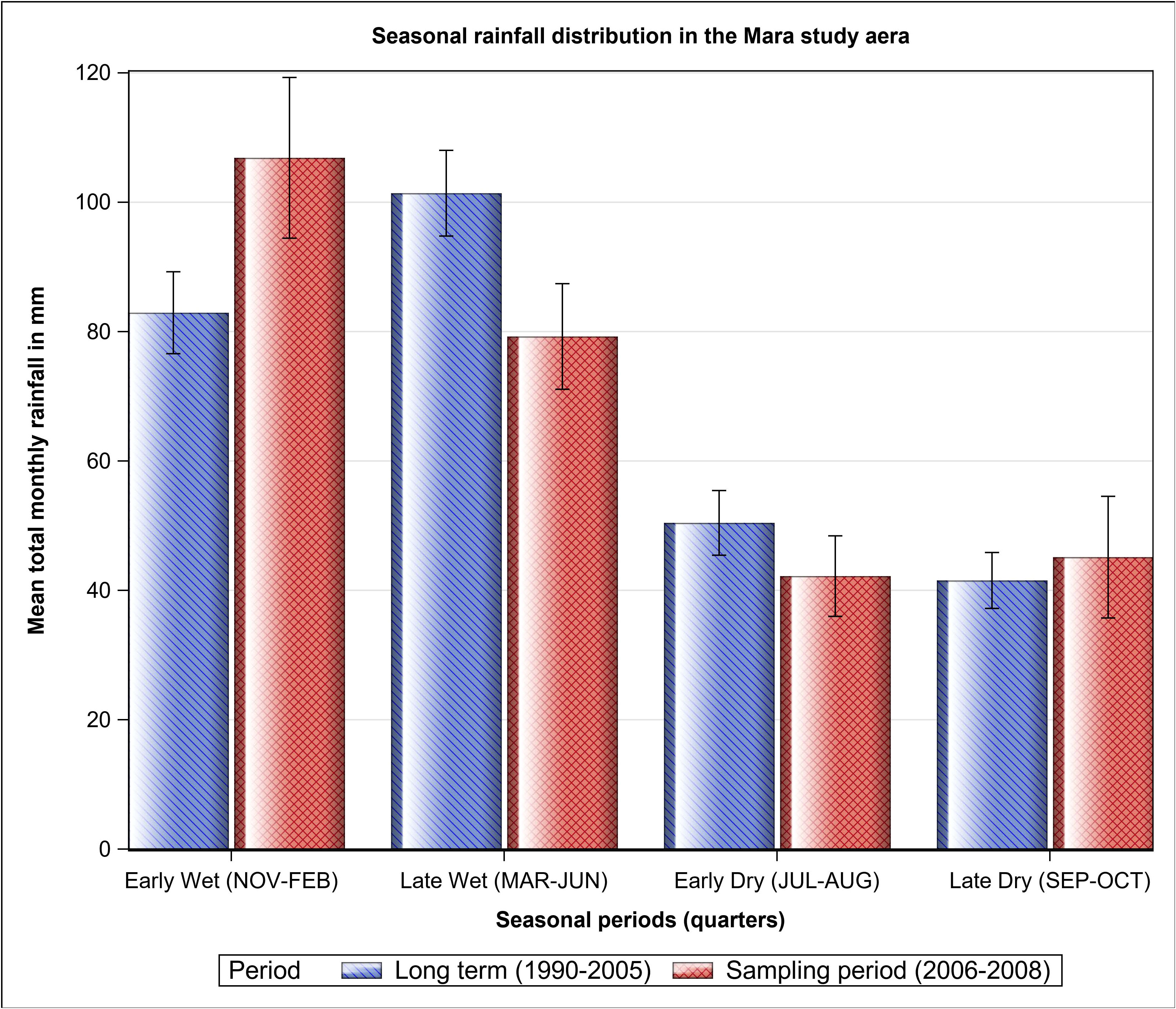
Figure 2 Seasonal distribution of the average total monthly rainfall in the Maasai Mara Ecosystem of Kenya during the sampling period (2006–2008) and over the long-term (1990–2005).
Sampling design
In this study, two landscapes were selected: the Maasai Mara National Reserve (MMNR), a protected conservation area, and the adjacent former community pastoral ranches of Koyiaki, Lemek, and Ol Chorro Oiroua (these areas currently contain Mara North, Naboisho, Olare-Motorogi, Lemek and Ol Chorro Oirorua Conservancies and Pardamat Conservation Area). While livestock grazing is not allowed in the MMNR, it is permitted in the adjacent pastoral, where livestock and wildlife graze together. The study sites within the MMNR was located far from areas known for illegal livestock incursions and no livestock or evidence of grazing were observed during the study period. To examine the differences in vegetation grazing between the two landscapes, 25 random transects were established, each 5 km long and radiating from the Mara, Talek, and Olare Orok Rivers. Sixteen of the transects were located in areas grazed by hippopotamuses and other wild herbivores, while the remaining nine transects were placed in areas grazed by both livestock and wild herbivores (Figure 1). There were no physical barriers separating the MMNR and ranch areas, allowing for the free movement of wildlife between the two, depending on wildlife species and season (Bhola et al., 2012a). Livestock were the dominant grazers in the ranch areas, while hippopotamuses were the dominant grazers in the MMNR as livestock is not permitted there. The rest of the wild herbivore communities and the distributions of their density and grazing pressure were comparable between the reserve and the ranch areas (Bhola et al., 2012b; Kanga et al., 2013; Ogutu et al., 2014). Along the 5 km riparian strip, the main resident grazers were hippopotamuses in the MMNR and livestock in the pastoral ranches. The topography of the study area was gently sloping plains and pediplains.
For each of the 25 transects, 13 sampling plots were established at 0, 100, 250, 500, 750, 1,000, 1,250, 1,500, 2,000, 2,500, 3,000, 4,000, and 5,000 meters from the rivers. These plots were each 10 × 10 m2 in size and were used to measure the percent cover of three vegetation growth forms (grasses, forbs, and shrubs) and bare ground. The grasses were further divided into three height classes: less than 10 cm tall, 10–30 cm tall, and greater than 30 cm tall. The cover measurements were used as a simple and efficient way to assess the rangeland condition. In addition, plant species richness and abundance were estimated by identifying and counting the individuals of each plant species in each plot. Specimens that could not be identified in the field were taken to the East African Herbarium for further identification. Plant nomenclature follows Agnew and Agnew (1994) and Beentje (1994). The grazing intensity within a 30 m radius of the center of each plot was also scored and recorded on a scale of 1 (no clear evidence of grazing) to 4 (heavily grazed), based on a visual assessment of the vegetation state, species composition, and structure, as well as the presence of dung, spoor, and herbivore movement patterns.
Finally, to assess the impact of hippopotamuses on herbaceous vegetation and denudation, we counted the number of hippopotamus trails within a 50 m radius of the center of each plot. Sampling took place during the early dry season (July–August), late dry season (September–October) of 2007 and 2008, and the late wet season (March–April) of 2008. It was not possible to access the study area to obtain samples for the early wet season (January–February) of 2008 due to widespread post-election violence in Kenya. Transects were treated as the unit of replication, resulting in a total of 650 samples (325 plots × 2 seasons). However, 16 of these samples (8 from the reserve and 8 from the pastoral ranches) were discarded due to the plots being either burned in the dry season or inaccessible due to heavy rainfall in the wet season. This left a total of 406 sampling points from the 16 transects in the reserve and 228 samples from the 9 transects in the pastoral ranches over the wet and dry seasons. In addition to the vegetation measurements, we also recorded the location of each plot relative to its catena position. All the data used in this study are provided in Supplementary Data Sheets 1–4.
Statistical analysis
Before analysis, the percentage cover of the five vegetation components comprising forbs, shrubs, grasses less than 10 cm, 10–30 cm and greater than 30 cm tall and bare ground were arcsine square-root transformed. We then used the Mann-Whitney U-test to examine the relationship between grazing intensity scores and distance from water and landscape, with two levels, namely Mara Reserve and pastoral ranches. The distance from water was treated as a categorical predictor and the grazing intensity score (1 to 4) as a continuous response variable. We performed multiple pairwise comparisons of the estimated mean grazing intensity scores among all the distance classes and used a Bonferroni adjustment for multiplicity. A doubly multivariate repeated measures analysis of variance (ANOVA) was used to test for differences in the combined mean proportions (arcsine square root transformed) of bare ground, forbs, shrubs, grasses less than 10 cm, 10–30 cm and greater than 30 cm tall between seasons, landscapes, distance from water and all their interactions. A multivariate analysis of variance (MANOVA) was used to test for differences between the four levels of grazing intensity treated as a categorical predictor variable. A doubly multivariate repeated measures ANOVA was also used to test for differences in the combined mean cover of the four different plant life forms, forbs, grasses, shrubs and trees between landscapes, seasons, distance from water and all their interactions.
The mean frequency of each plant species at each sampling distance from water per landscape was calculated across all transects, and the contribution of each plant life form, grasses, forbs, shrubs and trees, to the total species richness (number of different species) was estimated for landscapes, seasons, and along distance from water. Univariate Analysis of Covariance (ANCOVA) was used to relate the number of different species (species richness) of each life form, namely forbs, grasses, shrubs and trees, separately to season, landscape, distance from water and all their interactions, treated as categorical predictors, and grazing intensity treated as a continuous covariate. To estimate the proportion of plant species shared between landscapes, we used the Jaccard coefficient, a measure of the similarity in species composition between two communities based on the number of species present in both communities and the number present in only one community. The coefficient ranges from 0 for two communities with no species in common to 1 for two communities with identical sets of species. Indirect gradient analysis was used to examine plant species compositions between the two landscapes based on species frequency of occurrence along distance from water. First, a Detrended Correspondence Analysis (DCA) was used to determine the length of the gradient, which was 1.3 (showing that a linear method such as the PCA is justified for the data, ter Braak, 1985), after which a Principal Components Analysis (PCA) was used for further analysis. Samples from plots on river banks were removed from the analysis to reveal more continuous effects of gradients. We correlated grazing intensity, number of hippopotamus trails, and distance from water as passive environmental variables with species composition along the axes. All models were fitted using Statistica (version 8), Statistical Analysis System (SAS/STAT 15.2, SAS Institute Inc, 2023) and PC-ORD (McCune and Mefford, 2006).
Results
Overall, the cover of shrubs, forbs and bare ground all declined with distance from rivers and are replaced with grasses. These grasses were taller in the reserve than in the pastoral ranches with livestock, likely due to different grazing patterns such that hippo grazing is concentrated around rivers and livestock grazing is spread out over a greater distance from the rivers.
Grazing intensity, distribution of hippopotamus trails, vegetation cover and species diversity
Grazing intensity was higher in the pastoral ranches (mean rank = 397.33, n = 228) than in the MMNR (mean rank = 272.67, n = 406; Mann-Whitney U = 28083, P < 0.001) and in the dry than in the wet season. Grazing intensity did not change significantly along distance from water in the pastoral ranches, particularly in the dry season (Kendall tau b = −0.069, P > 0.05, Figure 3), but declined significantly with distance from water in the MMNR in both seasons (Kendall Tau b = −0.401, P < 0.001, Figure 3). The mean number of hippopotamus trails was significantly higher in the MMNR (0.31 ± 0.02, n = 406; F1, 618 = 17.6, P < 0.001) than in the pastoral ranches (0.14 ± 0.01, n = 228) and declined significantly with distance from rivers (F3, 618 = 36.2, P < 0.001), with the decline being steeper for the MMNR (P = 0.013). The mean plant species richness increased with increasing grazing intensity in the reserve in both seasons (Figure 4). But in the pastoral ranches the mean species richness first increased up to a peak at intermediate grazing levels and then decreased with further increase in grazing intensity (Figure 4).
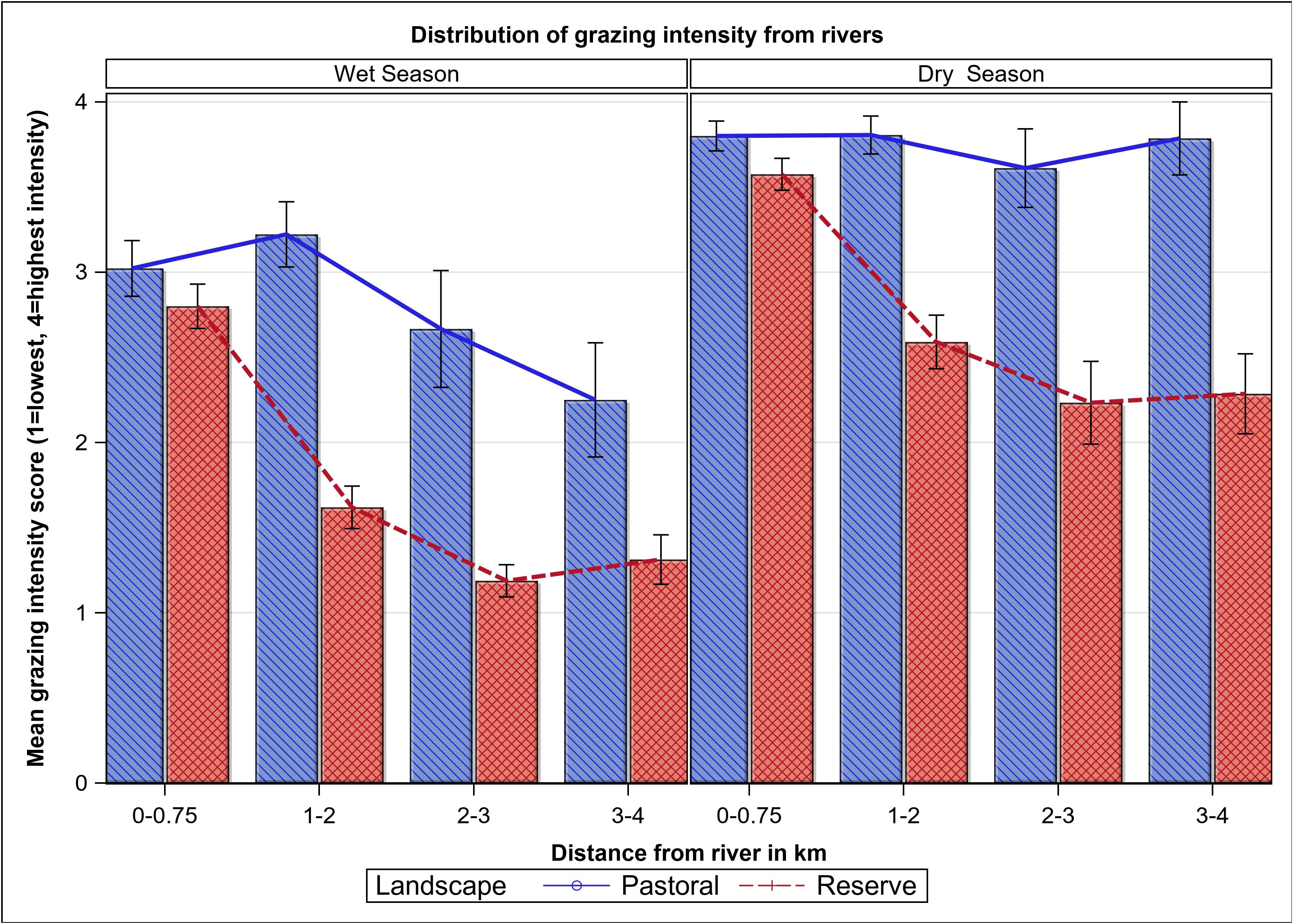
Figure 3 Mean grazing intensity as a function of distance from rivers in the dry and wet seasons in the pastoral land and the Maasai Mara Reserve in the Maasai Mara Ecosystem of Kenya during 2007–2008.
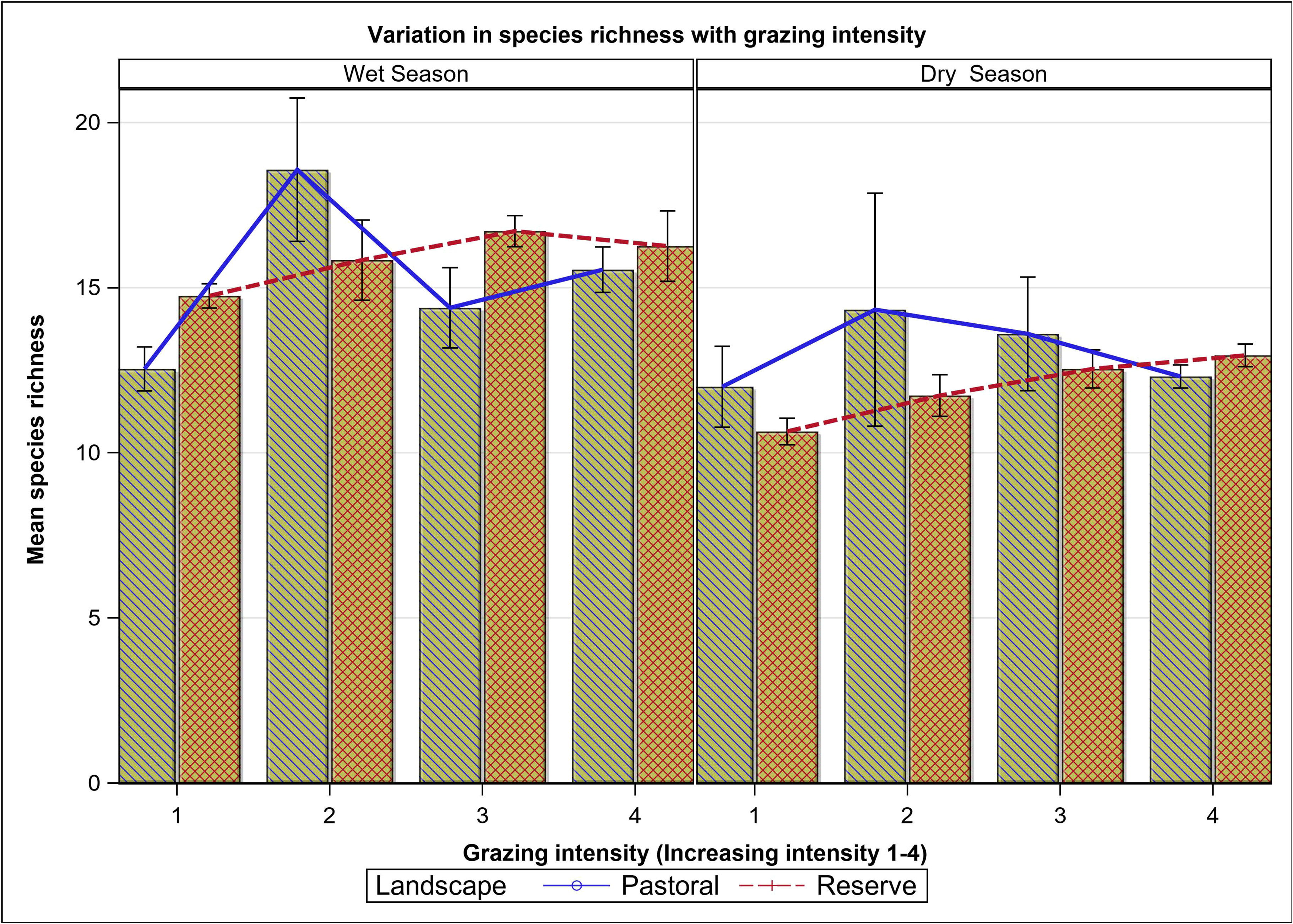
Figure 4 The variation in mean plant species richness as a function of grazing intensity in the Maasai Mara Reserve and pastoral ranches in the wet and dry seasons during 2007–2008.
The doubly multivariate repeated measures ANOVA showed that the combined mean percentage cover of the bare ground and the five vegetation components (grasses less than 10 cm, 10–30 cm and greater than 30 cm tall, forbs, shrubs) differed significantly across seasons (F6, 303 = 18.47, P < 0.0001), landscapes (F6, 303 = 13.86, P < 0.0001) and along distance from water (F18, 857.5 = 5.12, P < 0.0001, Figures 5 and 6). The variation in the combined mean parentage cover along distance from water differed between the two landscapes (F18, 857.5 = 2.26, P = 0.002). The pattern of variation in the combined mean percentage cover between landscapes or along distance from water were similar for both seasons but the pattern of variation among landscape × distance differed between seasons (F18, 857.5 = 1.70, P = 0.0348). The mean percentage cover of bare ground was similar between the dry and the wet seasons but varied between landscapes (P= 0.0136), with increasing distance from water (P < 0.0001) and the variation along distance from water differed between landscapes (P < 0.0001, Figures 5A and 6), such that it was lower in the pastoral ranches than in the MMNR within 750 m from rivers but became higher in the pastoral ranches than in the MMNR at greater distances from rivers. The mean percentage cover of grasses shorter than 10 cm was higher in the dry season (0.81 ± 0.02, n = 312) than in the wet season (0.59 ± 0.02, n = 322, P < 0.001) and in the pastoral ranches (0.92 ± 0.03, n = 228) than in the MMNR (0.57 ± 0.02, n = 406, P < 0.0001). It declined significantly with increasing distance from rivers (P < 0.0001), but the decline was only significant for the MMNR (Figures 5B and 6). The mean percentage cover of grasses 10–30 cm tall was similar in both seasons and landscapes but increased significantly with distance from water similarly in both landscapes (P < 0.001, Figures 5C and 6) except for 1–2 km from water at which the mean cover was far lower in the pastoral land than in the reserve (P = 0.0394). The mean percentage cover of grasses taller than 30 cm was higher in the wet season (0.59 ± 0.03, n = 322) than in the dry season (0.32 ± 0.02, n = 312, P < 0.0001), as well as in the MMNR (0.58 ± 0.03, n = 406) than in the pastoral ranches (0.24 ± 0.03, n = 228, P < 0.0001). It also increased with increasing distance from rivers (P < 0.0001), but the increase was only significant for the MMNR (Figures 5D and 6).
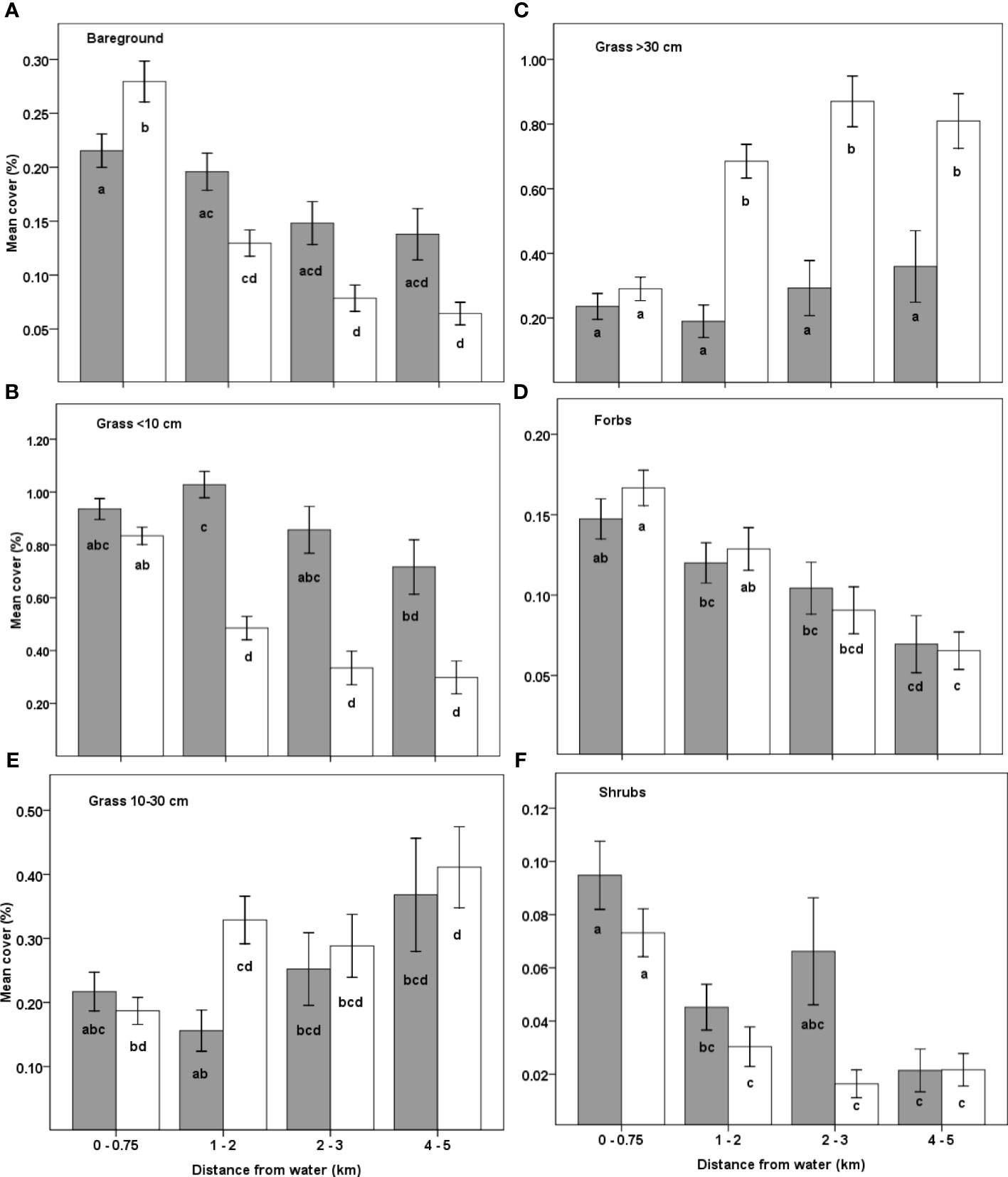
Figure 5 Mean percent cover of (A) bare soil, (B) grass < 10 cm, (C) grass 10–30 cm, (D) grass > 30 cm, (E) forbs and (F) shrubs in the pastoral ranches (grey bars) and the Maasai Mara National Reserve (white bars) as functions of distance from water during 2007–2008. Means not sharing any letter are significantly different at the 5% level of significance.
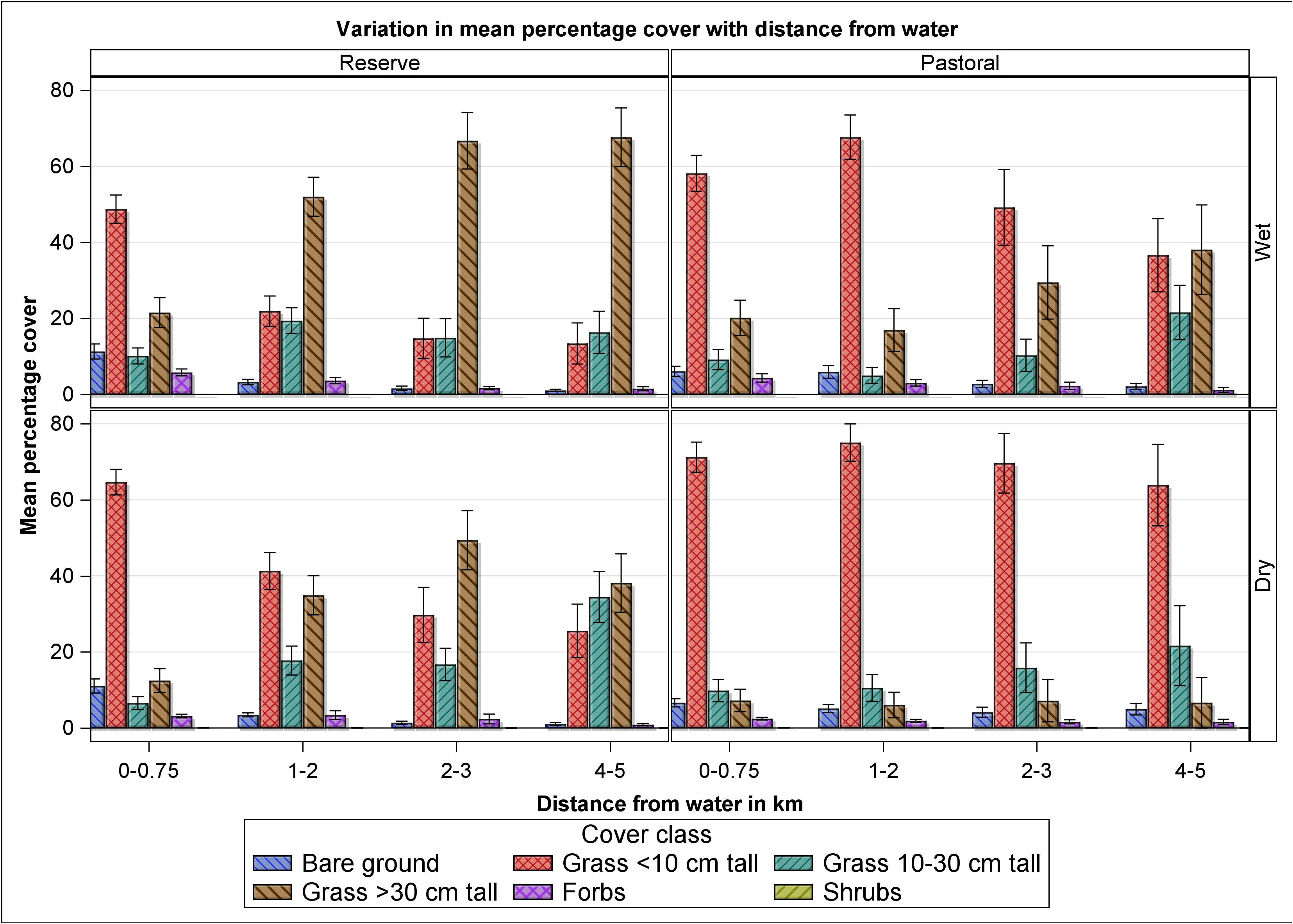
Figure 6 Variation on mean percent cover of bare ground, grasses less than 10 cm, 10–30 cm and greater than 30 cm tall, forbs and shrubs with distance from water in the Mara Reserve and adjoining pastoral lands in the wet and dry seasons of 2007–2008.
The mean percentage cover of forbs was higher in the wet season (0.14 ± 0.01, n = 322) than in the dry season (0.11 ± 0.01, n = 312, P = 0.058) but similar in both landscapes and declined with increasing distance from water similarly in both landscapes (P < 0.0001, Figures 5E and 6). The mean percentage cover of shrubs was comparable between the dry and wet seasons but was higher in the pastoral ranches (0.07 ± 0.01, n = 228) than in the MMNR (0.04 ± 0.01, n = 406, P = 0.0106). Furthermore, it declined significantly with distance from water, similarly in both landscapes (P < 0.0001, Figures 5F and 6).
A multivariate ANOVA test showed that the combined mean percent cover of bare ground and the five vegetation cover categories varied significantly among the four levels of grazing intensity (Wilks’ Lambda = 0.195, F18, 1768 = 76.8, P < 0.0001), such that the cover of bare ground (P < 0.0001), grasses shorter than 10 cm (P < 0.0001), forbs (P < 0.0001) and shrubs (P <=0.0004) increased significantly with increasing grazing intensity, whereas the cover of grasses 10–30 cm tall (P < 0.0001) and taller than 30 cm (P < 0.0001) declined with increasing grazing intensity.
Although high grazing intensity can lead to bush (shrub and tree) encroachment, shrubs are the only woody component we observed (Figures 5 and 7). We also observed the same pattern of change in shrub cover with distance from water in the reserve and the pastoral ranches, despite differences in grazing intensity. At no distance from the river was shrub cover significantly different between the reserve and the ranches, including at 2–3 km (Figures 5 and 7). Consequently, grazing may not be the only factor responsible for the pattern of change in shrub cover we observed. Therefore, other factors such as position on the catena, soil moisture, seedling recruitment bottlenecks and fire could provide plausible alternative explanations. As a result, the data do not appear to provide unequivocal support that livestock grazing alone leads to bush encroachment.
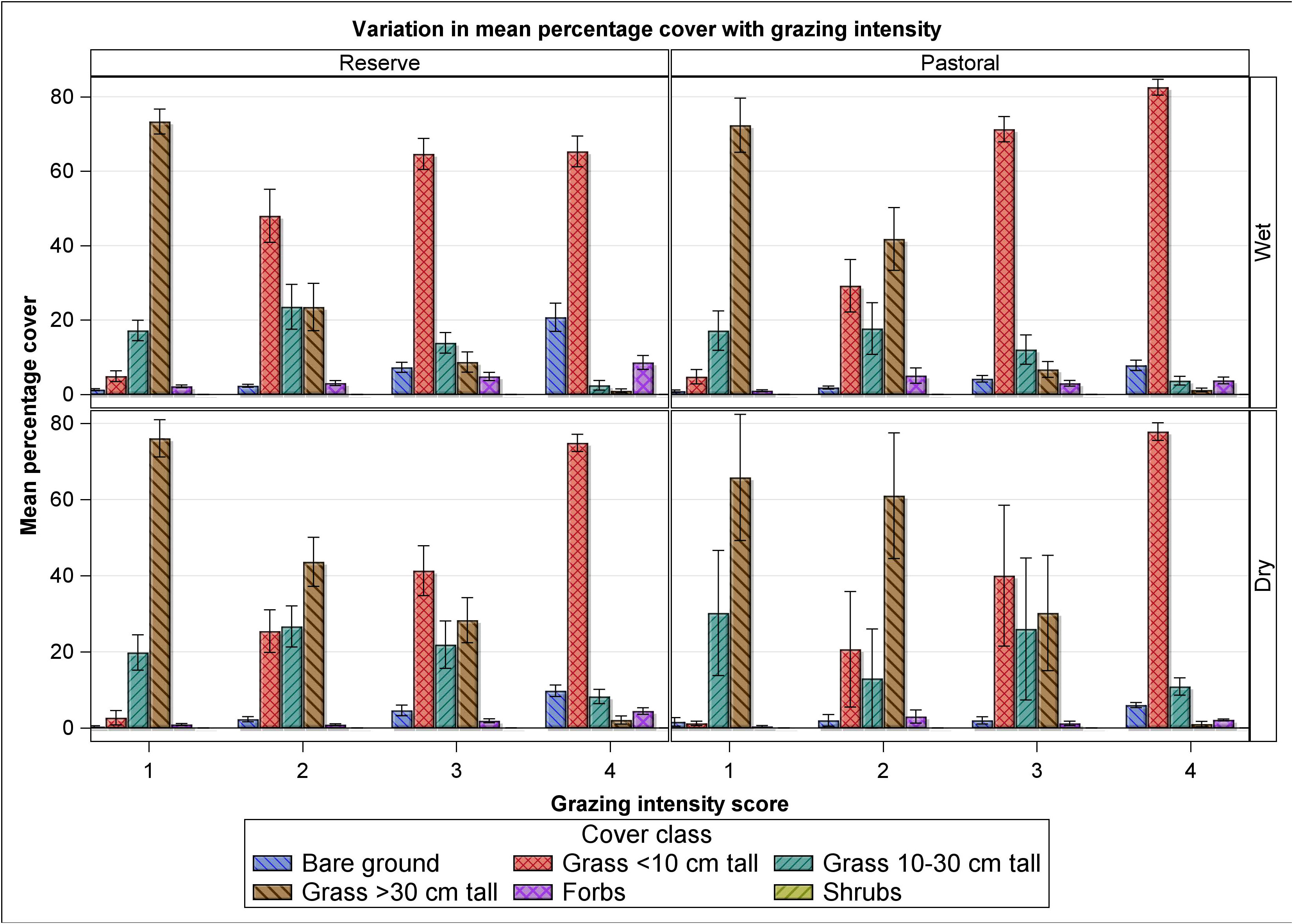
Figure 7 Variation in mean percent cover of bare ground, grasses less than 10 cm, 10–30 cm and greater than 30 cm tall, forbs and shrubs with distance from water in the Mara Reserve and adjoining pastoral lands in the wet and dry seasons of 2007–2008.
Species richness and floristic composition
We recorded 145 plant species belonging to 98 genera and 40 families and comprising 31% forbs, 28% grasses, 23% trees and 18% shrubs. Plant species richness varied between landscapes, seasons and along distance from water (F13, 620 = 9.35, P < 0.001, Table 1). The mean number of plant species per 100 m2 plot was higher in the wet season (15.44 ± 0.25, n = 322) than in the dry season (11.89 ± 0.26, n = 312). There was no significant difference in plant species richness between the MMNR (13.86 ± 0.21, n = 406) and the pastoral ranches (13.74 ± 0.32, n = 228; Table 1; Figure 8). However, the number of plant species declined with distance from water in both landscapes (P = 0.012, Figure 8) and increased significantly linearly with increasing grazing intensity (P < 0.001).
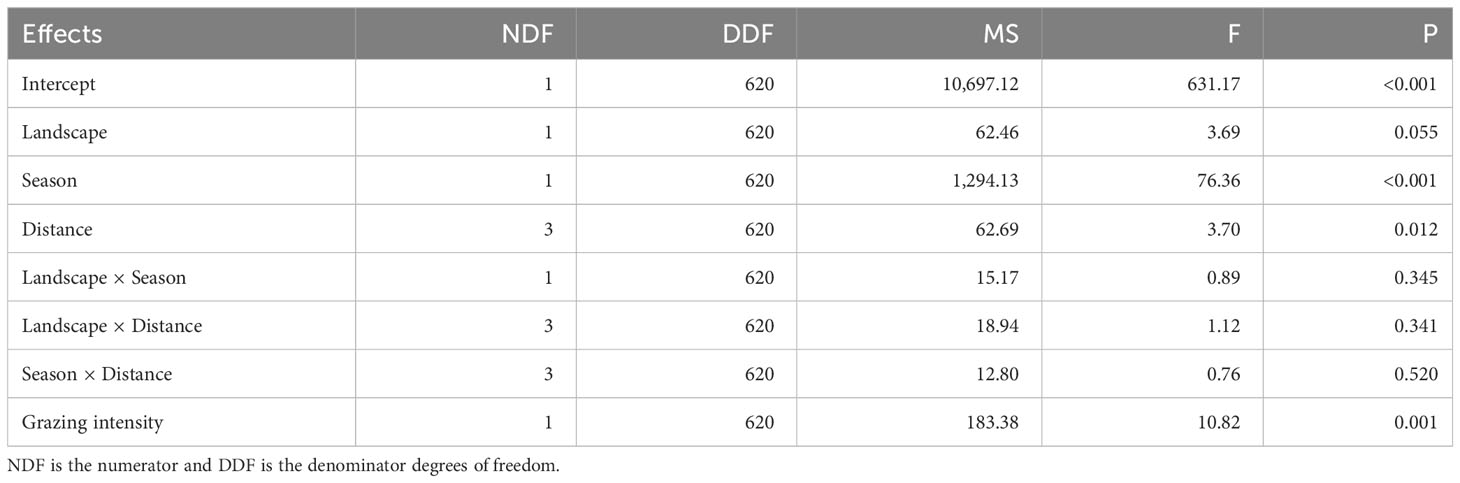
Table 1 Results of doubly multivariate repeated measures analysis of variance of the effects of season, landscape, distance from water and their interactions on plant species richness in the Mara Region of Kenya.
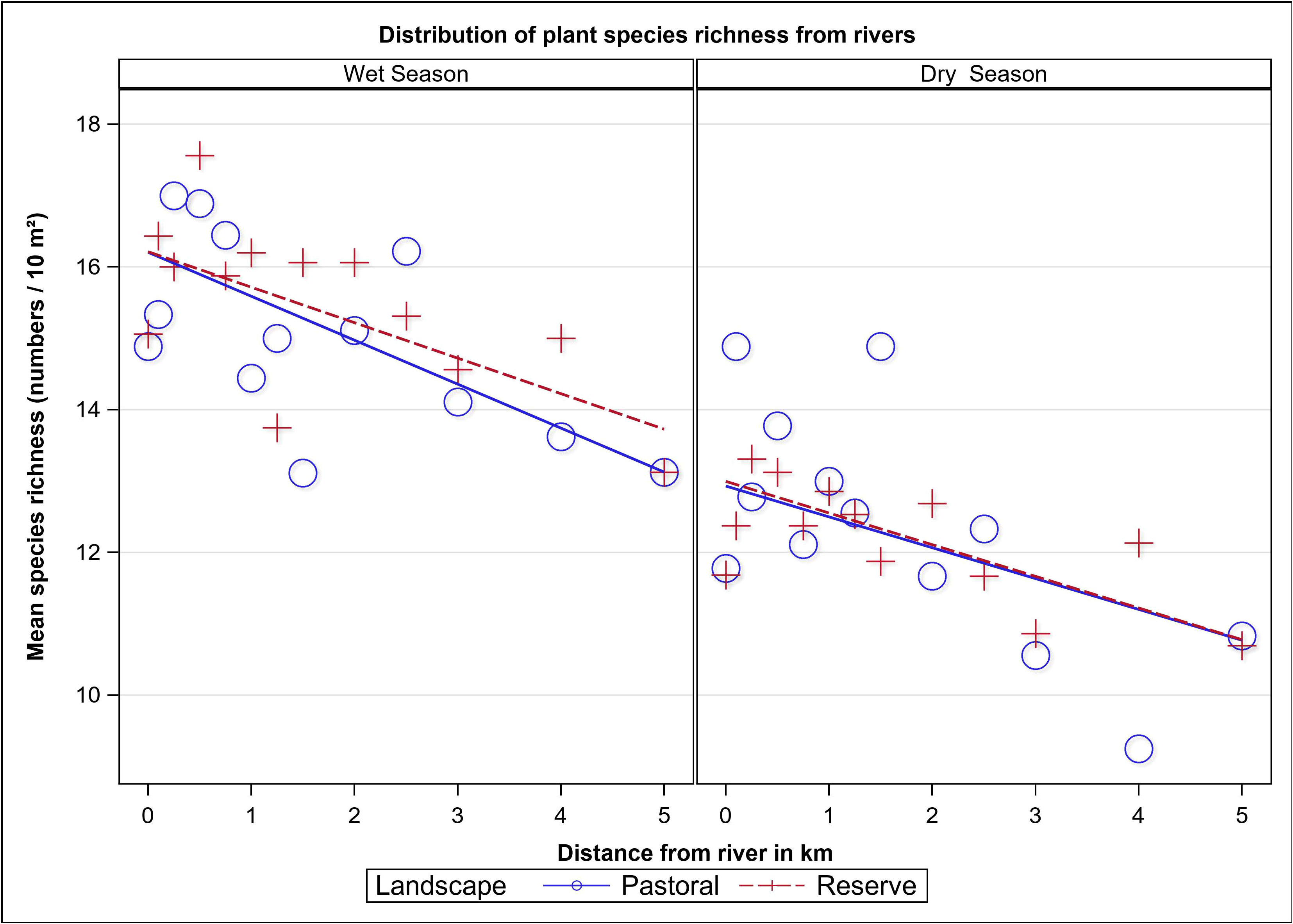
Figure 8 Mean number of plant species per 10 m2 as a function of distance from rivers in the dry and wet seasons in the Maasai Mara Ecosystem of Kenya during 2007–2008.
Growth form characterization
The doubly multivariate repeated measures ANOVA showed that the combined mean number of forb, grass, shrub and tree species differed between landscapes (F4, 305 = 5.31, P =0.0004), seasons (F4, 305 = 36.84, P < 0.0001) and along distance from water (F12, 807.3 = 3.53, P < 0.0001). The pattern of difference in mean species richness between landscapes was modified by season (F4, 305 = 3.03, P = 0.0108). Grazing intensity significantly modified the number of forb species (P < 0.001) but only marginally influenced the grass species (P = 0.085, Table 2). More forb species were recorded in the wet (4.54 ± 0.13, n = 322) than the dry (3.61 ± 0.09, n = 312, P < 0.001) season, in the MMNR (4.26 ± 0.10, n = 406) than in the pastoral ranches (3.78 ± 0.13, n = 228, P < 0.001) and the number of forb species declined with distance from water (P = 0.010), similarly in both landscapes (Table 2). The number of grass species was higher in the wet season (9.37 ± 0.15, n = 322) than in the dry season (7.08 ± 0.10, n=312, P < 0.001), marginally higher in the MMNR (8.40 ± 0.14, n = 406) than in the pastoral ranches (7.98 ± 0.16, n = 228, P = 0.046, Table 2) and did not change with distance from water in either landscape. The number of shrub species was similar in both seasons, higher in the pastoral ranches (1.68 ± 0.12, n = 228) than in the MMNR (1.00 ± 0.05, n = 406, P = 0.001) and declined significantly with distance from water in both landscapes (P < 0.001, Table 2). The decline was more marked in the pastoral ranches (P = 0.008). The mean number of tree species was similar between landscapes but declined with increasing distance from water (P = 0.018). Moreover, the rate of decline was steeper for the pastoral ranches (P = 0.025, Table 2).
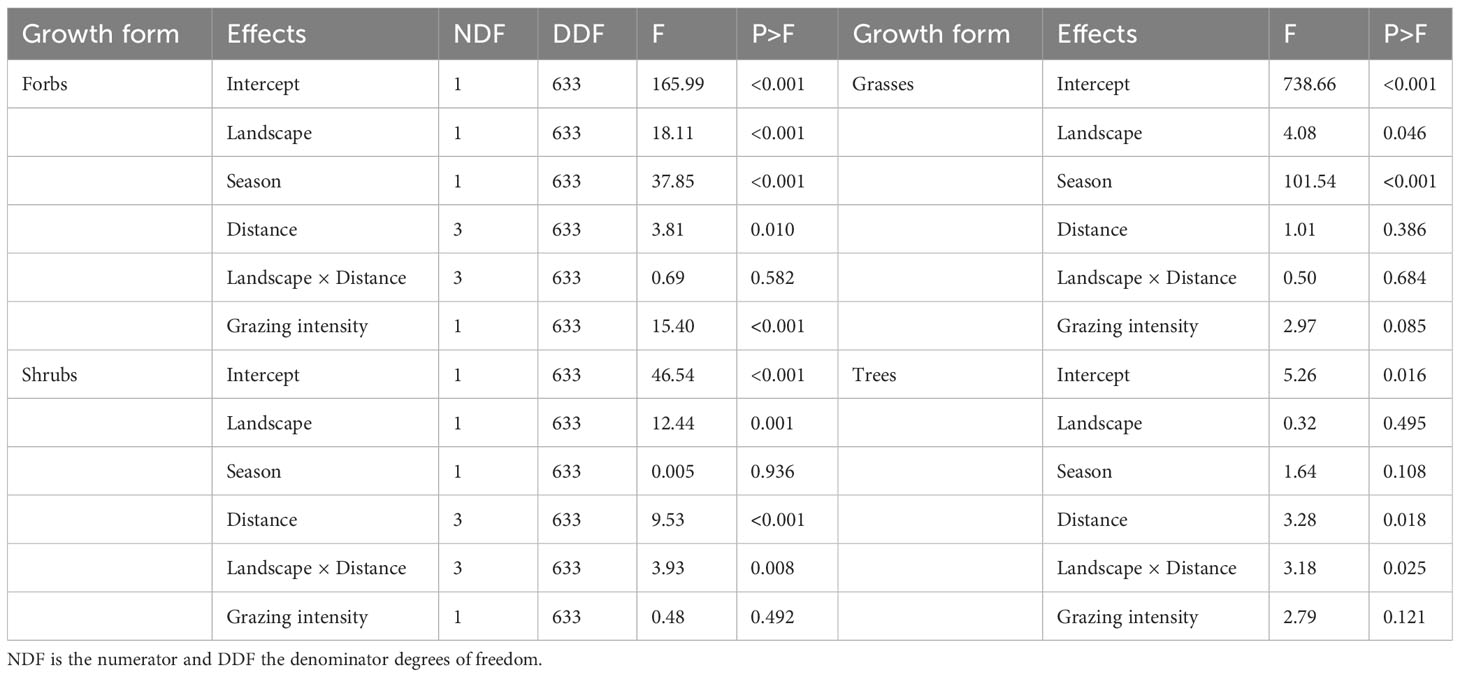
Table 2 Results of statistical tests of the effects of landscape, distance from water, season, grazing intensity and their interactions on the mean number of forb, grass, shrub and tree species in the Mara region of Kenya.
Species similarity index and grazing impact signatures
The reserve and pastoral ranches had an overall plant species similarity index of 0.57. This index declined consistently with distance from water such that it was 0.50, 0.49, 0.41 and 0.29 at 0–0.75, 1–2, 2–3 and 4–5 km from water, respectively. The first two ordination axes (PCA) explained 31% of the variation in plant species composition between the two landscapes. Grazing intensity was the most important factor influencing species composition along the first axis independent of the number of hippo trails, while distance from water and number of hippo trails influenced species composition along the second axis (Figure 9). The PCA ordination space clearly separated the reserve and the pastoral ranches based on plant species composition, with four species clusters apparent: (a) species associated with a high number of hippo trails and close to water, were exemplified by Aristida adoensis, Loudetia simplex, Sida ovate and Tragus berteronianus; (b) species associated with greater distances from water in areas of low numbers of hippo trails clustered together and included Eragrostis racemosa, Acacia drepanolobium, and Athroisma psylloides; (c) species responding to heavy grazing irrespective of the number of hippo trails and distance from water were represented by Cynodon dactylon, Microchloa kunthii, Sida massaica and Solanum incanum; and (d) species common in low or moderately grazed areas irrespective of the distance from water were exemplified by Chloris gayana, Cymbopogon spp. and Setaria incrassate (Figure 9).
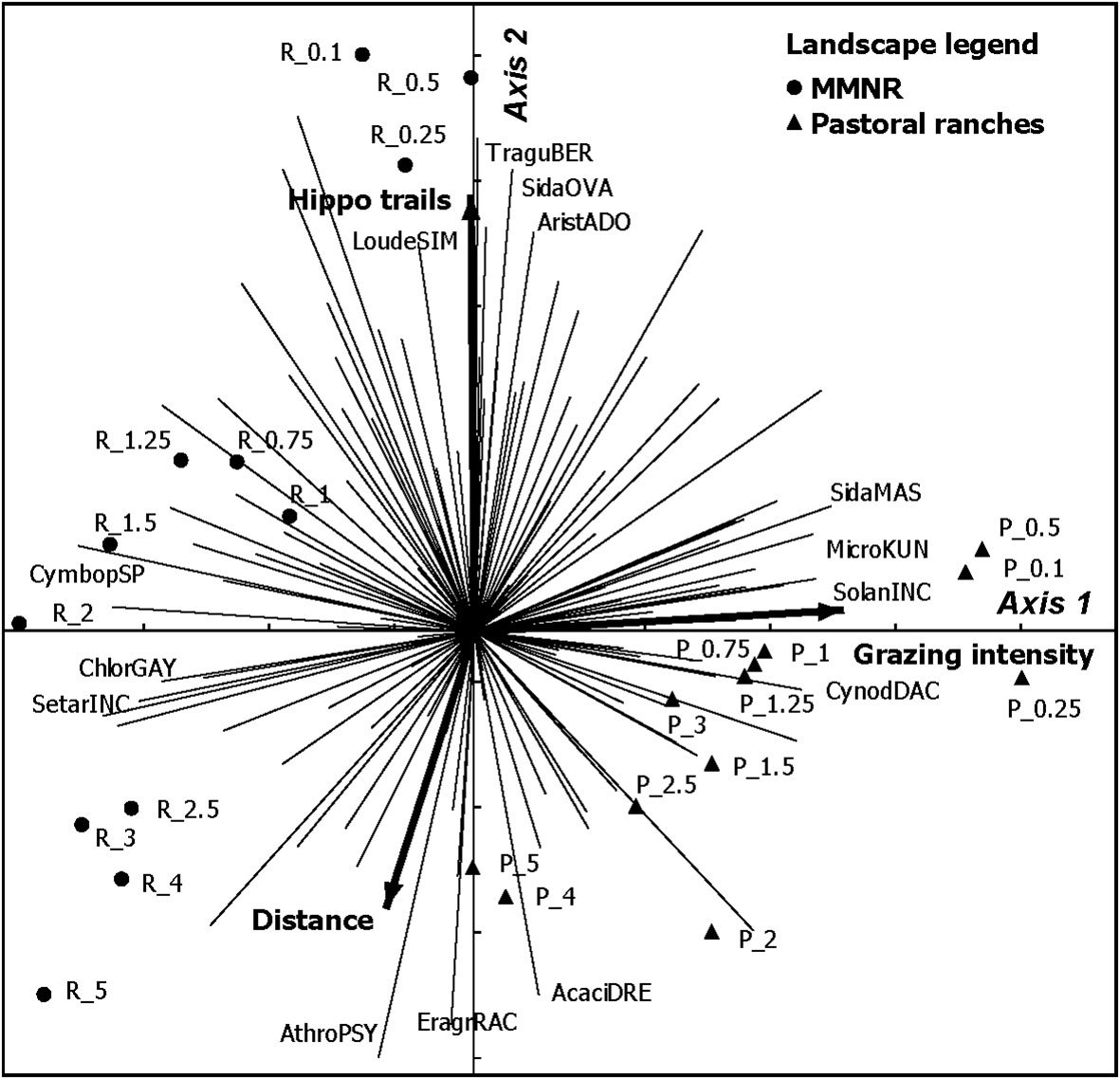
Figure 9 Ordination diagram (PCA) based on plant species frequency, showing the influence of distance from water, grazing intensity and number of hippo trails on plant species composition in the Mara region of Kenya. Abbreviations refer to sampling locations (R denotes reserve and P denotes pastoral ranches and the associated numeric suffixes denote the distance of the location from water in kilometers). For species (the first 5 letters denote the generic name whereas the next 3 letters denote the specific name).
Discussion
We examined differences in hippopotamus and livestock grazing patterns along transects radiating from rivers in the Masai Mara National Reserve and its adjacent pastoral ranches in southwestern Kenya during wet and dry seasons. We used grazing intensity scores to measure the variation in grazing intensity with distance from rivers and investigated the influence of hippopotamus and livestock grazing on plant height, species abundance, richness, and composition. The spatial patterns of grazing and their impacts on vegetation varied, resulting in discernible spatial heterogeneity in vegetation structure and species composition (McNaughton, 1983, 1984; Olff and Ritchie, 1998), particularly in the reserve. The high grazing intensity near water in both landscapes is characteristic of gradients created and maintained by herbivores in areas of concentrated resource utilization (Thornton, 1971; Thrash, 2000; Oba et al., 2001), such as near water during the dry season when water is scarce. However, grazing intensity declined steeply away from water in the reserve, but not in the pastoral ranches, indicating a more homogeneous distribution of grazing impact in the pastoral ranches than in the reserve, especially during the dry season. As a result, areas closer to water were typically more heavily grazed than distant areas in the reserve, similar to other observations of utilization gradients emanating from points of concentrated use known as piospheres (Andrew, 1988; James et al., 1999; Ogutu et al., 2010; Ogutu et al., 2014). The heavily grazed strip right next to water had the highest number of hippopotamus trails in the reserve, reflecting concentrated utilization by hippopotamus as they left and returned to water daily. In contrast, the more widespread grazing impact in the pastoral ranches was almost certainly due to year-round intense livestock grazing.
Higher livestock concentrations reduced vegetation cover and plant height over longer distances from water in the pastoral ranches than in the reserve. The differences in the patterns of spatial heterogeneity in vegetation structure away from rivers between the two landscapes likely reflects differences in hippopotamus and livestock grazing styles and their resultant impacts on vegetation. The difference in grazing intensity between the reserve and the pastoral ranches suggests that hippos predominantly graze closer to rivers. The difference also suggests that wild herbivore grazing impact is more diffusely distributed over the landscape and fades faster away from rivers, resulting in the lower grazing intensity farther from water for the reserve. Although differences in the herbivore communities likely contributed to these differences, other factors besides grazing impacts not measured in this study may also have contributed to the observed vegetation patterns.
Repeated grazing and trampling by hippopotami leaving and returning to water daily in the reserve created sacrificial zones devoid of most vegetation cover and extending for nearly 750 m from river banks, similar to findings of earlier studies (Thornton, 1971; Lock, 1972; Eltringham, 1999; Thrash and Derry, 1999). These high-impact zones were characteristically denuded and barren and had the highest grazing intensity scores and percent cover of short grasses and forbs. In contrast, the higher percent cover of bare ground beyond 750 m from water in the pastoral ranches than in the reserve can largely be attributed to heavy and sustained grazing and trampling by large livestock herds. Although high grazing intensity-maintained grass cover at relatively low levels, grasses still constituted the major fraction of the herbaceous layer. Grass height was markedly shorter closer to water in both landscapes and increased significantly with increasing distance from water in the reserve but not in the pastoral ranches, where short grasses were dominant up to 5 km from water. Forbs were well represented for the first 2–3 km from water in both landscapes but their contribution to ground cover decreased sharply thereafter, implying that forbs favoured short grass lawns created and maintained by heavy grazing close to water. Shrub cover was higher closer to water in both landscapes and farther from water in the ranches than in the reserve, implying that heavy grazing likely enhanced the thickening and spread of shrubs, through suppression of fires and reduced competition with grasses (Belsky, 1992; Moleele and Perkins, 1998; Thrash and Derry, 1999; Oba et al., 2000; Sharp and Whittaker, 2003; Lunt et al., 2007; Veldhuis et al., 2019). Also, the observed seasonal variation in vegetation cover suggests that forage availability is limiting in the dry season as indicated by intensification in grazing impacts closer to water.
Intense large herbivore grazing can reduce the effect of fire on vegetation by reducing fuel loads and allowing increased woody plant recruitment. Thus, even though the Maasai traditionally use fire as a management tool to improve grazing quality, intense and sustained livestock grazing has gradually eliminated fire from large parts of the pastoral ranches around the Serengeti-Mara Ecosystem, including in Maasai Mara (Butz, 2009; Veldhuis et al., 2019). Fuel loads and fire regimes can also be affected by the marked rainfall gradient because grass biomass production increases with rainfall in the ecosystem (Sinclair, 1975; McNaughton, 1985). Moreover, even though fuel loads can be higher in the depressions with taller grasses than in the elevations in catenas, higher soil moisture in the catenary depressions can reduce fire intensity and frequency. Lastly, woody plant seedlings may be more exposed to herbivory, depending on the locally common herbivore community, where grazing has strongly reduced grass height. Nevertheless, heavily grazed pastoral lands around the Serengeti-Mara Ecosystem generally have more woody cover and increasingly less frequent fires, than the protected areas (Veldhuis et al., 2019).
Despite their differences in grazing intensity and vegetation structure, both landscapes had similar plant species richness. Species richness was the highest proximal to the rivers and decreased linearly and at similar rates with distance from rivers regardless of season, landscape or grazing intensity. This pattern contradicts predictions of the intermediate disturbance hypothesis for mesic savanna rangelands (Grime, 1973), and suggests, surprisingly, that intense grazing pressure close to rivers enabled coexistence of many plant species thereby enhancing herbaceous species richness (Whicker and Detling, 1988; Olff and Ritchie, 1998; Oba et al., 2001).
However, plant species richness increased with increasing grazing intensity in the reserve in both seasons due to release from domination by a few species, as has also been observed elsewhere (Del-Val and Crawley, 2005; Lunt et al., 2007; Koerner et al., 2018). This was not the case in the pastoral ranches, where the mean species richness peaked at intermediate levels of grazing intensity and declined with further increases in grazing intensity. This distinction further highlights the contrasting grazing strategies of hippopotamus and livestock, the two dominant constituents of the grazing large mammal community in the riparian-edge habitats of the Mara. In the reserve, the grazing impact of hippopotamus created shifting mosaics of tall, medium, and short vegetation cover that promoted the coexistence of herbaceous species, especially at heavily grazed locations near water. In contrast, in the pastoral ranches, heavy livestock grazing created wide patches of bare ground and homogenized the structural vegetation pattern, which enhanced the establishment of annual and invasive species and elevated species richness at intermediate distances from water, similar to patterns observed elsewhere (Zerihun and Saleem, 2000). Therefore, hippopotamus and livestock grazing in the riparian zone in the Mara had contrasting impacts, but both allowed a diverse set of herbaceous species to coexist.
The numerical dominance of forbs within heavily grazed patches is consistent with findings of several other studies (Whicker and Detling, 1988; Del-Val and Crawley, 2005), while the increase in the number of plant species recorded in the wet season probably reflects the germination of annual species (Zerihun and Saleem, 2000). The number of forb species decreased with increasing distance from water, indicating that grasses outcompeted forbs in areas of low grazing intensity (Oba et al., 2001; Del-Val and Crawley, 2005). Interestingly, the number of grass species did not change along the distance-to-water gradient in both landscapes, suggesting that it was insensitive to average vegetation height and only slightly responsive to grazing intensity. Woody species (shrubs and trees) were consistently more abundant closer to water and in the pastoral ranches, implying that intense grazing near water by hippopotamus in the reserve and livestock in the ranches kept grasses short all year, thereby suppressing fires and stimulating the encroachment of woody species (Moleele and Perkins, 1998; Sharp and Whittaker, 2003; Lunt et al., 2007). These results demonstrate that low grazing intensities are associated with reduced species richness by altering forb and shrub species. The greater shrub abundance near rivers could also partly be a consequence of higher grazing there. These results partly contrast with findings for xeric environments demonstrating that high livestock stocking rates, densities or intense livestock grazing simplifies and removes vegetation patches and reduces plant cover, abundance, vegetation density and species richness (Tadey, 2006; Pelliza et al., 2021; Tadey, 2023). Our results also partly reinforce those of other studies showing that hippo grazing significantly reduces grassland canopy height but increases the leafiness of common grasses, reduces woody plant abundance and size, and increases the concentrations of several soil elements (McCauley et al., 2018).
But low fire frequency caused by high grazing intensity at greater distances from water in the ranches, imply that shrub density should not decline with distance from rivers if the mechanism operates through grazing pressure reducing fires, thus resulting in more shrubs. This suggests the additional involvement of something else as an important driver of vegetation type close to rivers, such as edaphic factors, including soils and moisture that also covary with distance from rivers.
Although plant species richness was similar between the reserve and the pastoral ranches, the differences in species composition were large, as seen by the clear separation of the two landscapes in the PCA ordination space. Jaccard’s similarity index also showed that both landscapes shared 57% of the species recorded, and the similarity index declined considerably with increasing distance from water in response to differences in grazing gradients between the two landscapes. Variations in species composition in the reserve are explained by distance from water, number of hippo trails, and grazing intensity. In contrast, variations in species composition in the pastoral ranches are explained less by distance from water and mostly by grazing intensity. Notably, areas far from water (4–5 km) in the pastoral ranches have similar species compositions to areas close to water (0.1–0.5 km) in the reserve, while species compositions within 1–5 km in the reserve and 0.1–3 km in the pastoral ranches are distinctive to each landscape (Figure 9). The differences in species composition between the reserve and pastoral ranches are clearly discernible, probably due to the additional effects of heavy, year-round livestock grazing in the pastoral ranches. Instead, large herds of migratory herbivores only use the reserve for half of the year, allowing sufficient time for vegetation recovery. Heavily grazed areas especially in the pastoral ranches retained species such as Cynodon dactylon, a grazing lawn species, Microchloa kunthii which establishes well in degraded areas, an increaser species such as Sida massaica and invasive species such as Solanum incanum (Figure 9). Areas highly utilized by hippos and mostly in the reserve had typical species such as Aristida adoensis which is common in highly grazed areas; an increaser species such as Sida ovate, weeds such as Tragus berteronianus, and a low palatable grass Loudetia simplex. Species associated with distance from water and in areas less utilized by hippos but common in the pastoral ranches included Eragrostis racemosa, Athroisma psylloides and Acacia drepanolobium. However, areas in the reserves that were moderately grazed irrespective of distance from water had atypical species cluster that included Setaria incrassate which only survives in moderately grazed areas; Cymbopogon spp. and Chloris gayana, a high-quality pasture grass. It follows, therefore, that contrasting modes and intensities of grazing by hippopotamus and livestock along riparian-edge habitats in the Mara have significantly and differentially modified plant species composition. Furthermore, changes in vegetation structure, plant species richness and composition induced by hippopotamus and livestock created habitat and forage diversity that may influence the suitability of riparian habitats for other mammalian herbivore species.
Conclusions
These results show that the sedentarization of pastoralists and concentration of their livestock grazing in a smaller area year-round, even without significantly increasing their stocking rate (Homewood and Rodgers, 1991), has important consequences for vegetation, making the landscape more uniform and causing a large shift in plant community composition compared to the protected area, albeit at the same small-scale plant diversity. In contrast, in the protected area, intense hippo grazing close to rivers strongly contributes to landscape heterogeneity, causing strong gradients in vegetation structure, species richness, and composition. The increasing sedentarization of the Maasai pastoralists and their livestock in the pastoral ranches of the Mara will likely progressively increase both the intensity and extent of the livestock grazing impact. This will likely shift the vegetation composition towards annuals and invasive weedy species especially near settled areas, further suppress fires, promote the establishment and spread of woody species encroachment, and lower the quality of the pastoral rangelands for wildlife. The long-term consequences of these impacts are still unknown and difficult to predict, but continued densification of woody species may reduce the suitability of the pastoral landscapes for livestock and grazing wild herbivores.
Data availability statement
The original contributions presented in the study are included in the article/Supplementary Material. Further inquiries can be directed to the corresponding author.
Ethics statement
Ethical review and approval was not required for the study on animals in accordance with the local legislation and institutional requirements.
Author contributions
EK, JO and HO conceived, supervised and coordinated the project. EK led data collection and in-country coordination. EK, JO, H-PP and HO developed the analytical approach and EK and JO conducted the analyses. EK and JO led the writing of the manuscript, with support from H-PP, and HO. All authors contributed to the article and approved the submitted version.
Funding
EK was supported by the Netherlands Fellowship Program (NFP) and the University of Groningen through the Government of Kenya and by the Frankfurt Zoological Society (FZS). JO was supported by a grant from the German Research Foundation (DFG, Grant # 257734638).
Acknowledgments
We thank Charles Matankory and Sospeter Kiambi for assistance with field work and for arranging field logistics. We also thank the Kenya Wildlife Service (KWS) rangers for providing security during field work, wardens of the Maasai Mara National Reserve and the management of the Koyiaki, Lemek and Ol Chorro Oiroua pastoral ranches for allowing us unlimited access to the study area.
Conflict of interest
The authors declare that the research was conducted in the absence of any commercial or financial relationships that could be construed as a potential conflict of interest.
Publisher’s note
All claims expressed in this article are solely those of the authors and do not necessarily represent those of their affiliated organizations, or those of the publisher, the editors and the reviewers. Any product that may be evaluated in this article, or claim that may be made by its manufacturer, is not guaranteed or endorsed by the publisher.
Author disclaimer
Any opinions, findings, and conclusions or recommendations expressed in this material are those of the authors.
Supplementary material
The Supplementary Material for this article can be found online at: https://www.frontiersin.org/articles/10.3389/fevo.2023.1161079/full#supplementary-material
Supplementary Data Sheet 1 | (Rainfall). Total monthly rainfall for five rain gauges located within the Maasai Mara study landscape during 1990–2008.
Supplementary Data Sheet 2 | (December-working-data-vege2010_2-2011-March). Complete vegetation, hippo and livestock grazing data for the Maasai Mara study landscape.
Supplementary Data Sheet 3 | (Lifeform-Speciesnumbers-April2010). Number of forb, shrub and tree species at various distances from water in the pastoral land and protected reserve in the Masai Mara study landscape.
Supplementary Data Sheet 4 | (Ordination-Plant-Species-97-coded2-May2010). Plant species data used in the ordination analysis.
References
Agnew A. D. Q., Agnew S. (1994). Upland Kenya Wild Flowers: A flora of the ferns and herbaceous flowering plants of upland Kenya. (Nairobi: East African Natural History Society).
Andrew M. H. (1988). Grazing impacts in relation to livestock watering points. Trends Ecol. Evol. 3, 336–339. doi: 10.1016/0169-5347(88)90090-0
Bakker E. S., Olff H. (2003). Impacts of different sized herbivores on recruitment opportunities for subordinate herbs in grassland. J. Vegetation Sci. 14, 465–474. doi: 10.1111/j.1654-1103.2003.tb02173.x
Bakker E. S., Ritchie M. E., Olff H., Milchunas D. G., Knop J. M. H. (2006). Herbivore impact on grassland plant diversity depends on habitat productivity and herbivore size. Ecol. Lett. 9, 780–788. doi: 10.1111/j.1461-0248.2006.00925.x
Bell R. H. V. (1971). A grazing system in the Serengeti. Sci. Am. 225, 86–93. doi: 10.1038/scientificamerican0771-86
Belsky A. J. (1983). Small-scale pattern in grassland communities in the Serengeti National Park, Tanzania. Vegetatio 55, 141–151. doi: 10.1007/BF00045015
Belsky A. J. (1992). Effects of grazing, competition, disturbance and fire on species composition and diversity in grassland communities. J. Vegetation Sci. 3, 187–200. doi: 10.2307/3235679
Bhola N., Ogutu J. O., Piepho H. P., Said M. Y., Reid R. S., Hobbs N. T., et al. (2012a). Comparative changes in density and demography of large herbivores in the Masai Mara Reserve and its surrounding human-dominated pastoral ranches in Kenya. Biodiversity Conserv. 21 (6), 1509–1530. doi: 10.1007/s10531-012-0261-y
Bhola N., Ogutu J. O., Said M. Y., Piepho H. P., Olff H. (2012b). The distribution of large herbivore hotspots in relation to environmental and anthropogenic correlates in the Mara region of Kenya. J. Anim. Ecol. 81 (6), 1268–1287. doi: 10.1111/j.1365-2656.2012.02000.x
Broten M. D., Said M. (1995). “Population trends of ungulates in and around Kenya’s Maasai Mara Reserve,” in Serengeti II: Dynamics, Management and Conservation of an Ecosystem. Eds. Sinclair A. R. E., Arcese P. (Chicago: University of Chicago Press), 169–193.
Bullock J. M., Marriott C. A. (2000). “Plant responses to grazing and opportunities for manipulation,” in Grazing Management, the principles and practice of grazing, for profit and environmental gain, within temperate grassland systems. Eds. Rook A. J., Penning P. D. (UK: British Grassland Society), 27–32.
Burkepile D. E., Fynn R. W. S., Thompson D. I., Lemoine N. P., Koerner S. E., Eby S., et al. (2017). Herbivore size matters for productivity–richness relationships in African savannas. J. Ecol. 105, 674–686. doi: 10.1111/1365-2745.12714
Butz R. J. (2009). Traditional fire management: historical fire regimes and land use change in pastoral East Africa. Int. J. Wildland Fire 18 (4), 442–450. doi: 10.1071/WF07067
Danell K., Duncan P., Bergström R., Pastor J. (2006). Large herbivore ecology, ecosystem dynamics and conservation. (Cambridge: Cambridge University Press).
Del-Val E., Crawley M. J. (2005). What limits herb biomass in grasslands: competition or herbivory? Oecologia 142, 202–211. doi: 10.1007/s00442-004-1719-8
Epp H., Agatsiva J. (1980). Habitat types of the Mara-Narok Area, western Kenya. (Nairobi, Kenya: Kenya Rangeland and Ecological Monitoring Programme (KREMU), Ministry of Environment and Natural Resources).
Frank D. A., McNaughton S. J., Tracy B. F. (1998). The ecology of the Earth’s grazing ecosystems. Bioscience 48 (7), 513–521. doi: 10.2307/1313313
Glover P. E. (1966). An ecological survey of the Narok District of Kenya Maasailan-1965. Part 1: geology, soils, climate and hydrology. (Nairobi: Kenya National Parks).
Grime J. P. (1973). Competitive exclusion in herbaceous vegetation. Nature 242, 343–347. doi: 10.1038/242344a0
Hobbs R. J., Huenneke L. F. (1992). Disturbance, diversity and invasion: implications for conservation. Conserv. Biol. 6, 324–337. doi: 10.1046/j.1523-1739.1992.06030324.x
Hodgson J., Illius A. W. (1996). The ecology and management of grazing systems (Wallingford, UK: CAB International).
Holechek J. L., Gomes H. D. S., Molinar F., Galt D. (1998). Grazing intensity: critique and approach. Rangelands Arch. 20 (5), 15–18. doi: 10.1126/science.aav0564
Homewood K. M., Rodgers W. A. (1991). Maasailand ecology: Pastoralist development and wildlife conservation in Ngorongoro, Tanzania. (Cambridge, UK: Cambridge University Press).
Homewood K., Lambin E. F., Coast E., Kariuki A., Kikula I., Kivelia J., et al. (2001). Long-term changes in Serengeti-Mara wildebeest and land cover: pastoralism, population, or policies? Proc. Natl Acad. Sci. U.S.A. 98 (22), 12544–12549.
James C. D., Landsberg. J., Morton S. R. (1999). Provision of watering points in the Australian arid zone: a review of effects on biota. J. Arid Environments 41, 87–121. doi: 10.1006/jare.1998.0467
Jordan G., Goenster S., Munkhnasan T., Shabier A., Buerkert A., Schlecht E. (2016). Spatio-temporal patterns of herbage availability and livestock movements: A cross-border analysis in the Chinese-Mongolian Altay. Pastoralism 6, 1–17. doi: 10.1186/s13570-016-0060-2
Kanga E. M., Ogutu J. O., Piepho H. P., Olff H. (2013). Hippopotamus and livestock grazing: influences on riparian vegetation and facilitation of other herbivores in the Mara Region of Kenya. Landscape Ecol. Eng. 9, 47–58. doi: 10.1007/s11355-011-0175-y
Kimani K., Pickard J. (1998). Recent trends and implications of group ranch subdivision and fragmentation in Kajiado District, Kenya. Geographic J. 164, 202–213. doi: 10.2307/3060370
Koerner S. E., Smith M. D., Burkepile D. E., Hanan N. P., Avolio M. L., Collins S. L., et al. (2018). Change in dominance determines herbivore effects on plant biodiversity. Nat. Ecol. Evol. 2, 1925–1932. doi: 10.1038/s41559-018-0696-y
Lamprey R. H., Reid R. S. (2004). Expansion of human settlement in Kenya’s Maasai Mara: what future for pastoralism and wildlife? J. Biogeography 31, 997–1032. doi: 10.1111/j.1365-2699.2004.01062.x
Lock J. M. (1972). The effects of hippopotamus grazing on grasslands. J. Ecol. 60, 445–467. doi: 10.2307/2258356
Lunt I. D., Eldridge D. J., Morgan J. W., Witt G. B. (2007). Turner review, 13. A framework to predict the effects of livestock grazing and grazing exclusion on conservation values in natural ecosystems in Australia. Aust. J. Bot. 55, 401–415. doi: 10.1071/BT06178
Marshall F. (1990). “Cattle herds and caprine flocks,” in Early pastoralists of South-western Kenya. Ed. Sutton J. E. G. (Nairobi: Memoirs of the British Institute in Eastern Africa), 205–260.
Masese F. O., Kiplagat M. J., González-Quijano C. R., Subalusky A. L., Dutton C. L., Post D. M., et al. (2020). Hippopotamus are distinct from domestic livestock in their resource subsidies to and effects on aquatic ecosystems. Proc. R Soc. B 287, 20193000. doi: 10.1098/rspb.2019.3000
McCabe J. T. (2003). Sustainability and livelihood diversification among the Maasai of Northern Tanzania. Hum. Organ. 62, 100–111. doi: 10.17730/humo.62.2.4rwrt1n3xptg29b8
McCauley D. J., Graham S. I., Dawson T. E., Power M. E., Ogada M., Nyingi W. D., et al. (2018). Diverse effects of the common hippopotamus on plant communities and soil chemistry. Oecologia 188, 821–835. doi: 10.1007/s00442-018-4243-y
McCune B., Mefford M. J. (2006). Multivariate analysis of ecological data. PC-ORD 5.0: Gleneden Beach, Oregon, USA.
McNaughton S. J. (1983). Compensatory plant growth as a response to herbivory. Oikos 40, 329–336. doi: 10.2307/3544305
McNaughton S. J. (1984). Grazing Lawns: Animals in herds, plant form and co-evolution. Am. Nat. 124, 863–886. doi: 10.1086/284321
McNaughton S. J. (1985). Ecology of a grazing system: the Serengeti. Ecol. Monogr. 55, 259–294. doi: 10.2307/1942578
McNaughton S. J. (1988). Mineral nutrition and seasonal movements of African migratory ungulates. Nature 345, 613–615. doi: 10.1038/345613a0
Milchunas D. G., Lauenroth W. K., Burke I. C. (1998). Livestock Grazing: animal and plant biodiversity of shortgrass steppe and the relationship to ecosystem function. Oikos 83, 65–74. doi: 10.2307/3546547
Milchunas D. G., Sala O. E., Lauenroth W. K. (1988). A generalized model of the effects of grazing by large herbivores on grassland community structure. Am. Nat. 132, 87–106. doi: 10.1086/284839
Moleele N. M., Perkins J. S. (1998). Encroaching woody plant species and boreholes: is cattle density the main driving factor in the Olifants Drift communal grazing lands, south-eastern Botswana? J. Arid Environments 40, 245–253. doi: 10.1006/jare.1998.0451
Norton-Griffiths M., Herlocker D. J., Pennycuick L. (1975). The patterns of rainfall in the Serengeti ecosystem. East Afr. Wildlife J. 13, 347–374. doi: 10.1111/j.1365-2028.1975.tb00144.x
Oba G., Stenseth NC., Lusigi WJ. (2000). New perspectives on sustainable grazing management in arid zones of sub-Saharan Africa. BioScience 50(1), 35–31.
Oba G., Vetaas O. R., Stenseth N. C. (2001). Relationships between biomass and plant species richness in arid-zone grazing lands. J. Appl. Ecol. 38, 836–845. doi: 10.1046/j.1365-2664.2001.00638.x
Ogutu J. O., Owen-Smith N., Piepho H. P., Said M. Y. (2011). Continuing wildlife population declines and range contraction in the Mara region of Kenya during 1977–2009. J. Zoology 285 (2), 99–109. doi: 10.1111/j.1469-7998.2011.00818.x
Ogutu J. O., Piepho H. P., Dublin H. T., Bhola N., Reid R. S. (2009). Dynamics of Mara-Serengeti ungulates in relation to land use changes. J. Zoology 278, 1–14. doi: 10.1111/j.1469-7998.2008.00536.x
Ogutu J. O., Piepho H. P., Reid R. S., Rainy M. E., Kruska R. L., Worden J. S., et al. (2010). Large herbivore responses to water and settlements in savannas. Ecol. Monogr. 80 (2), 241–266. doi: 10.1890/09-0439.1
Ogutu J. O., Piepho H. P., Said M. Y., Ojwang G. O., Njino L. W., Kifugo S. C., et al. (2016). Extreme wildlife declines and concurrent increase in livestock numbers in Kenya: What are the causes? PloS One 11 (9), e0163249. doi: 10.1371/journal.pone.0163249
Ogutu J. O., Reid R. S., Piepho H. P., Hobbs N. T., Rainy M. E., Kruska R. L., et al. (2014). Large herbivore responses to surface water and land use in an East African savanna: implications for conservation and human-wildlife conflicts. Biodiversity Conserv. 23, 573–596. doi: 10.1007/s10531-013-0617-y
Olff H., Brown V. K., Drent R. (1999). Herbivores: Between Plants and Predators. (Oxford: Blackwell Science).
Olff H., Ritchie M. E. (1998). Effects of herbivores on grassland plant diversity. Trends Ecol. Evol. 13, 261–265. doi: 10.1016/S0169-5347(98)01364-0
Pausas J. G., Austin M. P. (2001). Patterns of plant species richness in relation to different environments: An appraisal. J. Vegetation Sci. 12, 153–166. doi: 10.2307/3236601
Pelliza Y. I., Fernandez A., Saiz H., Tadey M. (2021). Together we stand, divided we fall: effects of livestock grazing on vegetation patches in a desert community. J. vegetation Sci. 32 (2), e13015. doi: 10.1111/jvs.13015
Ritchie M. E., Tilman D., Knops J. M. H. (1998). Herbivore effects on plant and nitrogen dynamics in Oak savanna. Ecology 79, 165–177. doi: 10.1890/0012-9658(1998)079[0165:HEOPAN]2.0.CO;2
Schrama M., Veen G. F., Bakker E. S., Ruifrok J. L., Bakker J. P., Olff H. (2013). An integrated perspective to explain nitrogen mineralization in grazed ecosystems. Perspect. Plant Ecol. Evol. systematics 15, 32–44. doi: 10.1016/j.ppees.2012.12.001
Serneels S., Lambin E. F. (2001). Impact of land-use changes on the wildebeest migration in the northern part of the Serengeti-Mara ecosystem. J. Biogeography 28, 391–407. doi: 10.1046/j.1365-2699.2001.00557.x
Sinclair ARE (1975). The resource limitation of trophic levels in tropical grassland ecosystems. J. Anim. Ecol. 44, 497–520.
Sharp B. R., Whittaker R. J. (2003). The irreversible cattle-driven transformation of a seasonally flooded Australian savanna. J. Biogeography 30, 783–802. doi: 10.1046/j.1365-2699.2003.00840.x
Tadey M. (2006). Grazing without grasses: Effects of introduced livestock on plant community composition in an arid environment in northern Patagonia. Appl. Vegetation Sci. 9, 109–116. doi: 10.1111/j.1654-109X.2006.tb00660.x
Tadey M. (2020). Reshaping phenology: Grazing has stronger effects than climate on flowering and fruiting phenology in desert plants. Perspect. Plant Ecology Evol. Systematics 42, 125501. doi: 10.1016/j.ppees.2019.125501
Tadey M. (2023). Cascading effects of livestock grazing on insect functional groups associated to flowers in arid lands. Agric. For. Entomology, 25, (3) 1–16. doi: 10.1111/afe.12557
ter Braak C. J. F. (1985). Correspondence analysis of incidence and abundance data: properties in terms of a unimodal response model. Biometrics 41, 859–873. doi: 10.2307/2530959
Thornton D. D. (1971). The effect of complete removal of hippopotamus on grasslands in the Queen Elizabeth National Park, Uganda. East Afr. Wildlife J. 9, 47–53. doi: 10.1111/j.1365-2028.1971.tb00218.x
Thrash I. (2000). Determinants of the extent of indigenous large herbivores impact on herbaceous vegetation at watering points in the north-eastern lowveld, South Africa. J. Arid Environments 44, 61–72. doi: 10.1006/jare.1999.0452
Thrash I., Derry J. F. (1999). The nature and modeling of piospheres: A review. Koedoe 42, 73–94. doi: 10.4102/koedoe.v42i2.234
Turner M. D., Schlecht E. (2019). Livestock mobility in sub-Saharan Africa: A critical review. Pastoralism 9 (1), 1–15. doi: 10.1186/s13570-019-0150-z
van Klink R., van der Plas F., van Noordwijk C. G. E. T., Wallisdevries M. F. F., Olff H. (2015). Effects of large herbivores on grassland arthropod diversity. Biol. Rev. 90, 347–366. doi: 10.1111/brv.12113
Veldhuis M. P., Ritchie M. E., Ogutu J. O., Morrison T. A., Beale C. M., Estes A. B., et al. (2019). Cross-boundary human impacts compromise the Serengeti-Mara ecosystem. Science 363 (6434), 1424–1428. doi: 10.1126/science.aav0564
Voysey M. D., de Bruyn P. N., Davies A. B. (2023). Are hippos Africa's most influential megaherbivore? A review of ecosystem engineering by the semi-aquatic common hippopotamus. Biol. Rev. 98, (5) doi: 10.1111/brv.12960
Western D., Groom R., Worden J. (2009). The impact of subdivision and sedentarization of pastoral lands on wildlife in an African savanna ecosystem. Biol. Conserv. 142, 2538–2546. doi: 10.1016/j.biocon.2009.05.025
Whicker A. D., Detling J. K. (1988). Ecological consequences of prairie dog disturbances. Bioscience 38, 778–785. doi: 10.2307/1310787
Williams L. A. J. (1964). Geology of the Mara River-Siana Area. Report no. 66. Geological survey of Kenya (Nairobi: Ministry of Natural Resources).
Keywords: grazing intensity, hippo grazing, pastoral livestock grazing, distance from water, protected and human-dominated pastoral landscapes, spatial heterogeneity, rainfall seasonality, Serengeti-Mara ecosystem
Citation: Kanga EM, Ogutu JO, Piepho H-P and Olff H (2023) Hippopotamus and livestock grazing near water points: consequences for vegetation cover, plant species richness and composition in African savannas. Front. Ecol. Evol. 11:1161079. doi: 10.3389/fevo.2023.1161079
Received: 07 February 2023; Accepted: 18 September 2023;
Published: 04 October 2023.
Edited by:
Dan Rubenstein, Princeton University, United StatesReviewed by:
Kari Veblen, Utah State University, United StatesMariana Tadey, National University of Comahue, Argentina
Copyright © 2023 Kanga, Ogutu, Piepho and Olff. This is an open-access article distributed under the terms of the Creative Commons Attribution License (CC BY). The use, distribution or reproduction in other forums is permitted, provided the original author(s) and the copyright owner(s) are credited and that the original publication in this journal is cited, in accordance with accepted academic practice. No use, distribution or reproduction is permitted which does not comply with these terms.
*Correspondence: Joseph O. Ogutu, jogutu2007@gmail.com
†These authors share first authorship