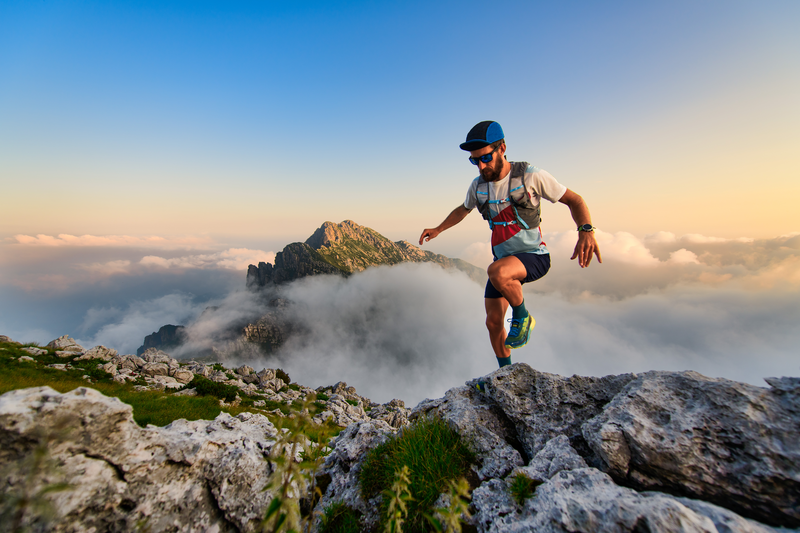
94% of researchers rate our articles as excellent or good
Learn more about the work of our research integrity team to safeguard the quality of each article we publish.
Find out more
EDITORIAL article
Front. Ecol. Evol. , 21 February 2023
Sec. Behavioral and Evolutionary Ecology
Volume 11 - 2023 | https://doi.org/10.3389/fevo.2023.1157198
This article is part of the Research Topic Signals in Motion View all 10 articles
Editorial on the Research Topic
Signals in motion
Animals use signals and cues to derive information about their environment, including the presence of food, enemies and mates, the location of nesting and provisioning sites, and the assessment of reproductive and social partners. The nature and reception of signals and cues is therefore a key focus of evolutionary and behavioral ecology, and our understanding of animal signals has expanded with the development of conceptual and technical advances. Nevertheless, our insights about the function and efficacy of animal color patterns remains largely shaped by a focus on stationary animals, typically in a static background, which rarely reflects the natural world (Cuthill et al., 2019; Tan and Elgar, 2021): most animals are mobile in their search for food and mates, and their surrounding environment is usually dynamic. There is considerable variation in animal signals within different signal modalities, including the color patterns of visual signals; the odor cocktails of chemical signals; and the amplitude and frequency of acoustic signals. These signals may act multimodally, which makes disentangling the additional contributions of animal motion and a dynamic environment even more challenging.
Our Research Topic Signals in motion asks how the efficacy of these signals to convey information to the intended or unintended receiver is affected by both animal movement and a potentially dynamic background. For example, there is emerging interest in how individual motion can reveal information about the signaler to the receiver but can also be a means of concealing visual cues to unintended receivers. In contrast, the impact of movement when signaling in acoustic or olfactory modalities has received little, if any, attention. The primary intention of this Research Topic is to draw attention to the importance of motion in animal signaling. Contributing authors report on diverse organisms with different behaviors, signaling modalities and background environments, and detail how motion is linked to signaling and how signalers and background movement may affect signal reception. There are few direct tests of the effects of signals in motion, but the studies in this Research Topic provide the initial steps toward understanding how motion affects signals.
Morphology and patterns can act together to affect the signal that is perceived by predators (Linke et al.). For example, the shape of butterfly wings affects their aerodynamic performance (Ortega Ancel et al., 2017), but whether these shapes can act as cues that indicate unprofitability to potential predators has been largely overlooked. Linke et al. examined how the shape and markings of butterfly wings can affect signaling unprofitability to predators. Their experiments revealed that blue tits Cyanistes caeruleus can associate white bands and hindwing tails with the difficulty of capture of dummy butterflies. This raises the intriguing possibility that butterfly tails act both to signal evasiveness as well as to divert attacks to non-essential parts of the wing.
The impact of animal movement on species that signal with volatile odors is rarely considered. Johnson et al. conducted inter-specific comparative analyses to examine how the complexity of moth antennae have evolved with the mobility of female signallers, who use sex pheromones to reveal their location to males. These analyses revealed that the loss of flight in female moths has evolved more frequently in species where males have elaborate antennae, and that elaborate antennae in males evolved more frequently in species where females are monoandrous (where remating frequency is <30%). Together, these data highlight how elaborate antennae are associated with selection favoring efficient signal detection, which in turn allows females to invest less in movement and dispersal.
Technological advances, especially high-speed cameras have allowed research to investigate complex animal signals involved in flight (Linke et al.; Martin et al.; Pohl et al.). Martin et al. and Pohl et al. show that animal responses, in grasshoppers and phasmids respectively, can be a collection of behaviors in motion, employing a range of antipredator strategies. The Carolina grasshopper Dissosteira carolina has a mostly brown colouration, with contrasting black and cream hindwings that are displayed when the grasshopper takes flight. By recording high-speed videos of the grasshopper flights in the field, Martin et al. discovered that the hindwing signals change during flight, depending on the size of the hindwing in view and the markings displayed. Martin et al. suggest that the Carolina grasshopper use their contrasting hindwings to deter predators through three mechanisms: first, by startling predators through deimatic defense; secondly, by confusing the predators and disrupting their search images through protean defense; and finally, returning to crypsis. Pohl et al. examined the behavioral responses of phasmids at different ontogenetic stages to a wind stimulus (a proxy for a dynamic environment). They found that adult behaviors were mostly species-specific, while nymph behaviors varied with appearance and environmental condition. Pohl et al. suggest that the behavioral responses interact with the morphology of the insects—depending on their species and ontogeny—to help reduce detection or recognition cues by potential predators.
Animals may employ signals from several modalities. For example, male wolf spiders Schizocosa are thought to use static and dynamic visual signals together with vibratory signals. Nevertheless, mating in S. retrorsa takes place in the absence of visual and vibratory stimuli. Kundu et al. show, experimentally, that these spiders use another signal modality—air particle movement or near field sound, that is generated by rapid leg waving. Interestingly, these signals are specific to courtship: female foraging success did not depend on near field sound (Kundu et al.).
Many signals can vary over both space and time, requiring an experimental procedure that is more nuanced than the traditional approach of investigating static models against a static background. Miller et al. provide details on how the dynamic visual signals of birds can be investigated using three dimensional multi-spectral models, which allow measures of color (including in the UV), pattern, 3D shape and motion to be considered quantitatively. This novel approach is likely to be a crucial first step at analyzing visual signals in motion.
Signals in motion may serve to benefit the animal when it resumes a stationary position. Flash signals are displayed by cryptic animals when they move: for example, the cryptic blue-winged grasshopper Oedipoda caerulescens, flashes highly conspicuous blue wings when in flight. While this behavior may startle the predator, the conventional view is that the putative predator incorrectly assumes the prey is always conspicuous, which compromises its subsequent searching efforts. This view has some empirical support but the underlying process leading to this benefit has not been investigated. Sherratt and Loeffler-Henry use Bayesian search theory to show that the degree of the conspicuousness of a prey item is negatively correlated with the time the predator should give up searching for it in an area where it appears to have settled. The model makes several predictions that can be readily tested experimentally.
Most experimental studies of signaling utilize a static background, yet this is rarely the experience for animals (Cuthill et al., 2019; Tan and Elgar, 2021). Environmental noise is often dynamic and multi-faceted, and the individual effects on signals can be difficult to disentangle (Pohl et al.; Ramos and Peters). Ramos and Peters examined the displays of six closely related agamid lizards of the Ctenophorus decresii complex. They compared the signal structures and contrasts in the context of the lizards' natural habitats and showed that the signaling behavior of the different lizard clades reflected local adaptations to their specific habitats. Ramos and Peters suggest common morphological and behavioral traits are likely from the ancestral state, and their study highlights the importance of taking account of the natural habitat or environment when interpreting signals and signaling behavior.
Considering the natural context is equally important for theoretical modeling. Tan et al. corrected a false assumption underpinning a model explaining swaying behavior in phasmid leaf insects. The model assumed that phasmids perched on top of the substrate and argued that the swaying behavior served a balancing function. However, Tan et al. point out that as most phasmids hang from the substrate, gravity removes any need for “balance.” Tan et al. thus caution that drawing functional explanations about animal motion, including motion signaling behavior, requires some understanding of the species' natural history.
Several themes emerge within the Signals in motion Research Topic: the coevolution of morphology and signals; the complexity of behaviors in motion; and the impact of environmental complexity. A common insight across these papers is a reiteration of John Endler's seminal paper highlighting the importance of considering the natural context (Endler, 1990). It is clear, from the contributions to the Signals in motion Research Topic, that this includes the dynamic nature of both the signal and the background, neither of which are likely to be entirely stationary. While the papers in the Signals in motion Research Topic identify technical and logistic challenges in developing ways of experimentally and theoretically investigating dynamic signals and backgrounds, they also highlight a rich seam of research possibilities. We hope this stimulates increasing interest in dynamic signals in dynamic backgrounds.
ET and ME conceived, wrote and edited the manuscript, and approved the submitted version. All authors contributed to the article and approved the submitted version.
This work was supported by the Ministry of Education, Singapore and Yale-NUS College Start-up Grant to ET.
We thank the authors of the nine papers in this Research Topic for their contributions.
The authors declare that the research was conducted in the absence of any commercial or financial relationships that could be construed as a potential conflict of interest.
All claims expressed in this article are solely those of the authors and do not necessarily represent those of their affiliated organizations, or those of the publisher, the editors and the reviewers. Any product that may be evaluated in this article, or claim that may be made by its manufacturer, is not guaranteed or endorsed by the publisher.
Cuthill, I. C., Matchette, S. R., and Scott-Samuel, N. E. (2019). Camouflage in a dynamic world. Curr. Opin. Behav. Sci. 30, 109–115. doi: 10.1016/j.cobeha.2019.07.007
Endler, J. A. (1990). On the measurement and classification of colour in studies of animal colour patterns. Biol. J. Linnean Soc. 41, 315–352. doi: 10.1111/j.1095-8312.1990.tb00839.x
Ortega Ancel, A., Eastwood, R., Vogt, D., Ithier, C., Smith, M., Wood, R., et al. (2017). Aerodynamic evaluation of wing shape and wing orientation in four butterfly species using numerical simulations and a low-speed wind tunnel, and its implications for the design of flying micro-robots. Interface Focus 7:20160087. doi: 10.1098/rsfs.2016.0087
Keywords: motion signals, flash behavior, crypsis, signaling environment, environmental noise, attack avoidance, mate location
Citation: Tan EJ and Elgar MA (2023) Editorial: Signals in motion. Front. Ecol. Evol. 11:1157198. doi: 10.3389/fevo.2023.1157198
Received: 02 February 2023; Accepted: 06 February 2023;
Published: 21 February 2023.
Edited and reviewed by: Jordi Figuerola, Doñana Biological Station (CSIC), Spain
Copyright © 2023 Tan and Elgar. This is an open-access article distributed under the terms of the Creative Commons Attribution License (CC BY). The use, distribution or reproduction in other forums is permitted, provided the original author(s) and the copyright owner(s) are credited and that the original publication in this journal is cited, in accordance with accepted academic practice. No use, distribution or reproduction is permitted which does not comply with these terms.
*Correspondence: Eunice J. Tan, eW5jdGplQG51cy5lZHUuc2c=
Disclaimer: All claims expressed in this article are solely those of the authors and do not necessarily represent those of their affiliated organizations, or those of the publisher, the editors and the reviewers. Any product that may be evaluated in this article or claim that may be made by its manufacturer is not guaranteed or endorsed by the publisher.
Research integrity at Frontiers
Learn more about the work of our research integrity team to safeguard the quality of each article we publish.