- School of Business, Zhejiang Wanli University, Ningbo, Zhejiang, China
Carbon neutrality and carbon peak are two important measures to control climate change. They have a huge impact on many companies in the fields of energy, industry, construction, transportation, etc. They can change the development pattern of related industries and increase many new investment and development opportunities. This paper proposes a path analysis of the standardization of energy economic management under the background of carbon neutrality and carbon peak, aiming to study the development forecast of energy economic management under low-carbon conditions. The algorithm proposed in this paper is an energy consumption analysis algorithm based on the IPAT model, which can be combined with the IPAT model to analyze and process energy consumption data. In addition, by analyzing and evaluating the contribution of various factors, people can better understand the impact of energy consumption on the environment and formulate corresponding solutions. The experimental analysis results of energy economic management based on carbon neutrality and carbon peak show that, from 2013 to 2017, under the baseline scenario, carbon emissions increased year by year, from 9.25 billion tons to 10.48 billion tons. Under the carbon neutral scenario, its carbon emissions are 9.22 billion tons, 9.24 billion tons, 9.22 billion tons, 9.19 billion tons and 9.21 billion tons respectively. Obviously, under the carbon neutral scenario, carbon emissions can be better controlled through carbon neutral and carbon peak strategies. Through these experimental prediction results, it can be proved that carbon neutrality and carbon peaking have excellent effects on promoting energy economic management. At the same time, this study also provides valuable reference information for further research on carbon neutrality and carbon peaks.
1. Introduction
Energy economic management refers to the process of meeting the needs of social and economic development through rational planning, development and use of energy resources, including the determination of energy policies and regulations, the formulation of energy planning, the implementation of energy efficiency improvement measures and the development of new energy technologies. The goal of energy economic management is to bring about a balance between energy supply and demand while ensuring the economy, sustainability and security of energy use. In the management of energy economy, various problems may be encountered. Some common problems include supply stability, cost-effectiveness, environmental protection, energy efficiency, etc. The existence of these problems has a great influence on the growth of energy and economy. With the continuous growth of energy demand, the research of energy economic management has become increasingly important. In terms of energy supply, the research contents include the exploitation, processing, transportation and storage of traditional energy (such as coal, oil and natural gas), as well as the development and promotion of new energy (such as wind energy, solar energy and biomass energy). In terms of energy demand, the research contents include the economic efficiency, technical efficiency and energy conversion efficiency of energy use, as well as the prediction, regulation and decision-making of energy demand. Although the research in the field of energy economy and management has made some progress, there are still many problems to be studied and solved in the context of carbon neutrality and carbon peak.
At present, due to the importance of energy management and the proposal of green energy consensus, energy management has become a hot research field, which has been studied by many scholars. Rathor Sumit K believed that the energy crisis and the promotion of global “greening” promoted the development of renewable energy, plug-in electric vehicles and energy storage grids. He pointed out that the variability and volatility of renewable energy and the uncertainty related to plug-in electric vehicles had brought new challenges to the electric energy management system. He reviewed the framework, objectives, architecture, benefits and challenges of the energy management system, and made a comprehensive analysis of the different stakeholders and participants involved, as well as the power quality management implemented in the energy management system. In addition, he also compared and critically analyzed the main optimization technologies used to achieve the objectives of different energy management systems while meeting multiple constraints (Rathor and Saxena, 2020). Zos-Kior Mykola believed that the introduction and optimization of innovative technologies to save energy and resources in the production process of enterprises was one of the main conditions to ensure the stable economic development of any country. His research objective was to develop a method to evaluate the economic benefits of innovative energy and resource conservation projects. He adopted systematic analysis, economic and mathematical modeling, abstract and logical methods to evaluate and predict the potential energy risks, and put forward valuable suggestions for the comprehensive management of energy management and resource conservation innovation (Zos-Kior et al., 2020). Li Zhiyi believed that the surge of distributed energy was reshaping the distribution system. In order to manage the efficiency, reliability, flexibility, security and sustainability of power energy services, he analyzed the scheme developed for the networked microgrid, which used blockchain technology to optimize the financial and physical operation of the distribution system. He also discussed the possibility of customizing blockchain technology to meet the socio-economic requirements of energy management at the distribution level. He proposed other smart contract measures to ensure the best energy transaction between the interconnected microgrid and the local distribution network (Li et al., 2019). Tripathy Bismay Ranjan pointed out that the change of power consumption mode had greatly affected the energy utilization process and socio-economic development. Therefore, it is crucial for predicting energy availability and planning its infrastructure to assess the spatial distribution of power consumption. He modeled the power demand and management of sustainable energy in the future, and also tested the sensitivity of power consumption to GDP and population growth. His research had made great contributions to forecasting power demand (Tripathy et al., 2018). It can be seen that the above research results are relatively comprehensive, and some of the theoretical information and experimental methods are highly referential, which can provide some help for this paper. However, at present, their research does not comprehensively and systematically link energy management research with carbon neutral theory, which is difficult to provide more detailed help.
With the increasing demand for carbon emission reduction, the research of energy management is also changing. In the context of carbon neutrality, the research of energy management has a new research direction and content. At present, some researchers have carried out research in this field. Caineng ZOU’s research pointed out that carbon dioxide was an essential medium of the global carbon cycle, which had the dual attributes of realizing the transformation of organic matter in the ecosystem and causing the greenhouse effect. He believed that carbon neutrality was the consensus of human development, but its implementation still faced many challenges such as politics, resources, technology, market and energy structure. In addition, he believed that new energy had become the leading force of the third energy conversion and would guide the promotion of carbon neutral strategy in the future. His research put forward feasible research directions for carbon neutrality and energy management, and promoted the process of energy independence strategy (Zou et al., 2021). Zhang Shu pointed out in his study that in order to optimize energy management and achieve carbon neutrality, it was necessary to make profound changes in China’s energy system. In his research, he combined Monte Carlo analysis with bottom-up energy, environmental and economic models to generate a number of cases with different carbon peak time, technology evolution path and cumulative carbon budget. By analyzing these cases, he pointed out that the time of emission peak and the cumulative carbon budget had a significant impact on the decarbonization path, technology selection and transition cost. He also believed that the realization of carbon neutrality urgently required technological breakthroughs, changes in production and consumption patterns and policy strengthening (Zhang and Chen, 2022). Through consulting the above literature, their research on carbon neutralization theory can be understood. At the same time, the latest advances in energy management need to be followed to broaden horizons and enrich ideas, which provide clear scientific orientation for this thesis. However, their research on energy management lacks some data and information research support of low carbon and energy. Therefore, more detailed research is needed.
Energy economic management refers to the use of economic means to manage and adjust energy consumption in order to achieve energy conservation, environmental protection and promote economic development. It is particularly important in the context of the increasingly severe energy crisis. With the continuous growth of energy demand, the reserves of traditional energy are gradually decreasing, and the greenhouse gases emitted in the process of energy production are also causing global climate change. Energy economic management is one of the effective ways to address energy issues, which is manifested in energy security, energy and resources protection and economic development (Yin et al., 2017). In energy economic management, energy demand and supply can be regulated by means of marketization, and energy consumption costs can be reduced by means of technical improvement, energy conservation and emission reduction measures (Shan et al., 2019; Zhao and Kok Foong, 2022). In this paper, a new research direction is used. The innovation of this paper is to study the management relationship between energy and economy by combining carbon neutral and carbon peak, and to study the feasibility of carbon neutral and low-carbon economic development strategies.
2. Energy management and carbon neutrality
2.1. Main fields of carbon neutralization
Carbon neutrality is a method of reducing greenhouse gas emissions, which aims to offset the emissions of greenhouse gases generated in other activities by reducing the emissions of greenhouse gases generated in some activities (Dong et al., 2021; Ning et al., 2022). In this way, the overall greenhouse gas emissions can be reduced, thus slowing the pace of global warming. Carbon neutralization plays an important role in many fields, and its main application fields are as follows.
2.1.1. Chemical industry
In the chemical industry, carbon neutralization can be used to reduce the carbon emissions of factories and reduce the generation and storage of carbon. One common carbon neutralization technology is carbon capture and storage technology, that is, capture carbon dioxide in the exhaust emissions of factories or power plants and store it underground. This can also be achieved by using absorbent, which can absorb carbon dioxide from the gas and store it in the container. Another common carbon neutralization technology is carbon conversion, that is, carbon dioxide is converted into other substances, such as organic compounds or acids. In addition, carbon neutralization can be used to reduce the carbon emissions of the plant, and can also be used to manufacture carbon neutralization products, such as absorbents or carbon conversion agents. It can also be used to develop low carbon or carbon neutralization processes to reduce the impact on climate.
2.1.2. New energy
In the field of new energy, carbon neutrality is mainly achieved through the use of renewable energy (Shu et al., 2021; Ning et al., 2023). Renewable energy refers to natural renewable energy, such as solar energy, wind energy and biomass energy (Xiao et al., 2022). Renewable energy does not produce carbon dioxide emissions in the process of energy production, so it can help reduce carbon emissions and achieve carbon neutrality. For example, when using solar energy to produce electricity, solar cells convert solar energy into electricity. This process does not produce carbon dioxide emissions, thus reducing the contribution to climate change. Similarly, when using wind energy to produce electricity, wind turbines convert wind energy into electricity, and this process does not produce carbon dioxide emissions. In addition, the use of biomass energy to produce energy is also an effective way of carbon neutralization. Biomass energy refers to the energy that uses organic substances (such as plants, animals and garbage) to produce energy. In the process of biomass energy production, organic matter is burned to generate heat energy and carbon dioxide. However, organic matter has absorbed carbon dioxide in the atmosphere during its growth, so the carbon dioxide emissions from biomass energy production do not increase the carbon dioxide content in the atmosphere. In general, the use of renewable energy is an efficient carbon-neutral strategy that can effectively reduce the contribution to climate change. In addition, there are many other benefits of using renewable energy, such as reducing dependence on fossil fuels, reducing pollutant emissions and economic benefits.
2.1.3. Building materials
The application of carbon neutrality in the field of building materials and buildings refers to taking measures during the construction, maintenance and demolition of buildings to reduce their contribution to climate change. Carbon neutralization in the field of building materials and buildings can be achieved by using low-carbon or carbon-free building materials, implementing energy conservation and emission reduction measures, and building recycling. For example, the use of low-carbon or carbon-free building materials and the use of renewable materials such as recycled plastics and wood can reduce the carbon dioxide emissions generated in the use of buildings. In addition, the implementation of energy conservation and emission reduction measures can also effectively reduce the contribution of buildings to climate change. For example, measures such as improving the thermal insulation performance of buildings, installing energy-saving equipment and saving water can help reduce energy consumption, thus reducing carbon dioxide emissions. Finally, carbon neutralization can also be achieved through building recycling. Building recycling refers to the recycling of materials after the demolition of buildings to reduce the carbon dioxide emissions generated in the production process of materials required for new buildings. Building recycling can not only reduce the contribution of buildings to climate change, but also save resources, thus obtaining economic benefits. In general, the implementation of carbon neutral measures in the field of building materials and buildings can effectively reduce the contribution to climate change, and can also bring many other benefits, such as reducing pollutant emissions, saving resources and improving the sustainability of buildings.
2.1.4. Non-ferrous metals
The application of carbon neutralization in the field of non-ferrous metals refers to taking measures in the production, processing and use of non-ferrous metals to reduce their contribution to climate change. Non-ferrous metals refer to a class of metals including copper, aluminum, nickel, tin, zinc and lead. In addition, non-ferrous metals also include non-ferrous alloys. Non-ferrous metals produce a large amount of carbon dioxide emissions during production and processing, which cause serious environmental pollution. Carbon neutralization measures in the field of non-ferrous metals can be implemented in the following ways. Renewable energy is used to produce non-ferrous metals, such as solar energy and wind energy, which can reduce the dependence on fossil fuels and carbon dioxide emissions. The exhaust gas emissions generated in the process of non-ferrous metal production are reduced. A large amount of waste gas is produced in the process of nonferrous metal production, which contains a large amount of carbon dioxide. Therefore, the use of more stringent waste gas treatment process can minimize the generation of waste gas and thus protect the environment. In general, the implementation of carbon neutralization measures in the field of non-ferrous metals can effectively reduce the contribution to climate change, and can also bring many other benefits, such as saving resources, reducing energy consumption and reducing pollutant emissions.
2.1.5. Energy conservation and environmental protection
The application of carbon neutrality in the field of energy conservation and environmental protection refers to the implementation of energy conservation and environmental protection measures to reduce its contribution to climate change, which can be achieved by protecting biodiversity and paying attention to resource recycling. For example, the protection of biodiversity can help reduce greenhouse gas emissions, because plants and other organisms can absorb carbon dioxide through photosynthesis and store it in soil, wood and other biomass. In addition, the protection of biodiversity also helps to protect the quality of land and water resources, thus reducing the impact on the environment. Finally, the importance of resource recycling is also an essential aspect of energy conservation and environmental protection. Resource recycling refers to the reuse of resources in use to reduce the consumption of raw materials and carbon dioxide emissions. For example, the use of recycled materials, recycling waste and building recycling can help realize resource recycling. In general, the implementation of carbon neutral measures in the field of energy conservation and environmental protection can effectively reduce the contribution to climate change, and can also bring many other benefits, such as saving resources, reducing energy consumption, reducing pollutant emissions and protecting biodiversity.
2.1.6. Mechanical equipment
In the field of mechanical equipment, carbon neutralization technology can be used to reduce the carbon dioxide emissions generated by some mechanical equipment during operation. For example, power generation units or gas turbines can use carbon neutralization technology to capture and store carbon dioxide. This technology can be achieved by using absorbent to absorb carbon dioxide, or by capturing carbon dioxide into carbonaceous gas and storing it underground. In addition, carbon neutralization technology can also be used to improve the energy efficiency of mechanical equipment, thereby reducing its carbon dioxide emissions. In addition, carbon neutralization technology can also be used in other fields, such as industrial production, transportation and construction. The application of carbon neutral technology can help reduce the dependence on fossil fuels and increase the contribution to climate change.
The main areas of carbon neutralization are shown in Figure 1.
2.2. Energy management and its main processes
Energy management refers to the process of meeting the needs of economic development and human life through the rational use, storage and transformation of energy, as well as the prediction, adjustment and control of energy demand (Sadeeq and Zeebaree, 2021; Ning et al., 2022). Energy management is not only the responsibility of the government and enterprises, but also the responsibility of everyone. Everyone should be aware of energy conservation in their lives and participate in energy management efforts. The main processes of energy management are described below.
2.2.1. Data collection
In the process of energy management, data acquisition is an important link (Mahapatra and Nayyar, 2022; Schirmer and Mporas, 2023). Data collection mainly collects data related to energy use and consumption through various sensors, equipment and systems. These data include the production, transformation, storage, transportation, sales and use of energy, as well as people’s energy consumption behavior and demand. Data collection can help people understand the use of energy, analyze and evaluate it according to the actual situation, and provide valuable information and basis for energy management. This information can help formulate reasonable energy planning and strategies to improve energy efficiency and reduce energy costs, thus protecting the environment and promoting economic development. In today’s digital era, data collection has also been greatly developed through network and cloud computing technology. Various energy management systems and platforms can realize remote monitoring, data analysis and management, which provide more accurate, timely and efficient support for energy management.
2.2.2. Energy analysis
Energy analysis refers to the systematic analysis and evaluation of energy use and consumption, and making reasonable suggestions and decisions based on the analysis results. Energy analysis usually analyzes the use of energy, mainly the production, transformation, storage, transportation, sales and use of energy. The energy use in different periods is compared, summarized and evaluated. Energy analysis can help to understand the use and trend of energy and make an overall assessment of it.
2.2.3. Evaluation of energy performance
Energy performance refers to the operating efficiency and economy of the energy system. In energy management, common methods for evaluating energy performance include energy efficiency index, energy cost index, energy use density index and energy renewable utilization index (Ramya et al., 2019). Among them, the energy efficiency indicator is an indicator to measure the operational efficiency of the energy system. Its role is to measure the energy conversion efficiency, energy utilization efficiency and energy utilization efficiency. The energy cost indicator is an indicator to measure the economy of the energy system. The common directions include unit energy cost, energy cost ratio and energy cost–benefit ratio. The energy use density indicator is an indicator to measure the impact of the energy system on the environment. The common contents include energy use per unit area, energy use per unit product and energy use per unit population. The renewable energy utilization rate is an indicator to measure the sustainability of the energy system. The commonly used indicators include the proportion of renewable energy, the utilization rate of renewable energy and the proportion of renewable energy output.
2.2.4. Energy conservation and emission reduction
In energy management, energy conservation and emission reduction refers to taking measures to reduce energy use and greenhouse gas emissions, so as to achieve the purpose of saving energy and protecting the environment. When carrying out energy conservation and emission reduction, common methods include improving energy efficiency, promoting renewable energy, controlling greenhouse gas emissions, adopting energy-saving technologies and equipment, improving energy management level, implementing energy plans and policies, etc. When carrying out energy conservation and emission reduction, different energy systems, different energy use conditions and different economic and social conditions should be taken into account, and practical measures should be taken. At the same time, a sound energy management system should be established to monitor and evaluate energy use, so as to constantly improve energy conservation and emission reduction measures.
The main process of energy management is shown in Figure 2.
3. Carbon neutralization, carbon peak and energy consumption analysis algorithm
3.1. Basic concepts of carbon neutralization and carbon peak
Carbon neutralization is a technology used to reduce greenhouse gas emissions and slow down the process of global warming. This technology is usually achieved by capturing greenhouse gases and storing them underground or converting them into other forms. For example, carbon capture and storage technology can be used to capture carbon dioxide emitted by factories or power plants, and then store it underground to prevent it from entering the atmosphere. Another common carbon neutralization technology is tree planting, because trees can absorb carbon dioxide and convert it into organic matter. Carbon neutrality is an important strategy to mitigate global warming, because it can help reduce greenhouse gas emissions and reduce the rise of the earth’s surface temperature. However, carbon neutrality is not the only way to solve the problem of climate change, nor is it a solution for all. In addressing the issue of climate change, there are many other factors to be considered, including reducing energy use and shifting to renewable energy, as well as policies and measures to promote sustainable development.
The carbon peak refers to the process that the global carbon emissions begin to decline after reaching the peak. This is an important concept, because the increase of carbon emissions is the main cause of global warming. When carbon emissions reach the peak and begin to decline, the global climate is likely to be mitigated. Carbon peaking is usually achieved by taking measures to reduce carbon emissions. These measures may include shifting to renewable energy, improving energy efficiency, promoting low-carbon technologies and infrastructure, and encouraging low-carbon behavior through policies and regulations. Carbon peaking is an important strategy to deal with climate change. To truly achieve the carbon peak, it is necessary to take joint action globally. This requires governments, businesses and the public to work together, share responsibility and do their best to reduce carbon emissions in the hope of mitigating the effects of global warming in the future.
The basic concepts of carbon neutralization and carbon peak are illustrated in Figure 3.
3.2. Energy consumption analysis algorithm based on IPAT model
IPAT model is an assessment model of the impact of human activities on the environment, which is used to express the impact of economic growth on the environment, that is, the environmental effect is the result of the comprehensive impact of population, technological factors and the level of affluence. The specific expression of the formula is:
Among them, , P, A and T represent the environmental load, population, per capita GDP and unit GDP, respectively.
The environmental impact is expressed as energy consumption, and the environmental impact formula is the IPAT model of energy consumption. Its specific expression is:
Among them, is the total energy consumption, and G is the total GDP.
In addition, there are relationships of and . The total energy consumption can be converted as follows:
Then, structural decomposition is carried out to get the change of energy consumption in the t period relative to the base period.
Among them, is the energy intensity factor. is the factor of economic growth. is population factor. In addition, is the decomposition allowance. The expression is as follows:
Among them,
Therefore,
To sum up, the total energy consumption can be expressed as follows:
Through these algorithm formulas, the construction of energy consumption analysis algorithm based on IPAT model can be completed. This algorithm is used to analyze and evaluate the impact of human energy consumption on the environment. According to the theory of IPAT model, it decomposes the impact of human energy consumption into three factors: population size, affluence and production technology. Through the analysis and evaluation of these three factors, the impact of energy consumption on the environment is determined. The analysis of energy consumption can provide reference basis for government decision-making and help the government to formulate energy policies and plans. In addition, key areas of energy conservation and emission reduction can also be identified, and corresponding suggestions and measures can be put forward to effectively reduce energy consumption and pollution.
4. Experiment on energy economic management
4.1. Test of energy consumption analysis algorithm based on IPAT model
In this part, the energy consumption analysis algorithm based on IPAT model is tested to explore its operation performance. First, the traditional energy consumption analysis algorithm and the energy consumption analysis algorithm based on IPAT model are synchronized to explore the relationship between the number of analysis threads and the amount of data synchronization processing. The results are illustrated in Figure 4.
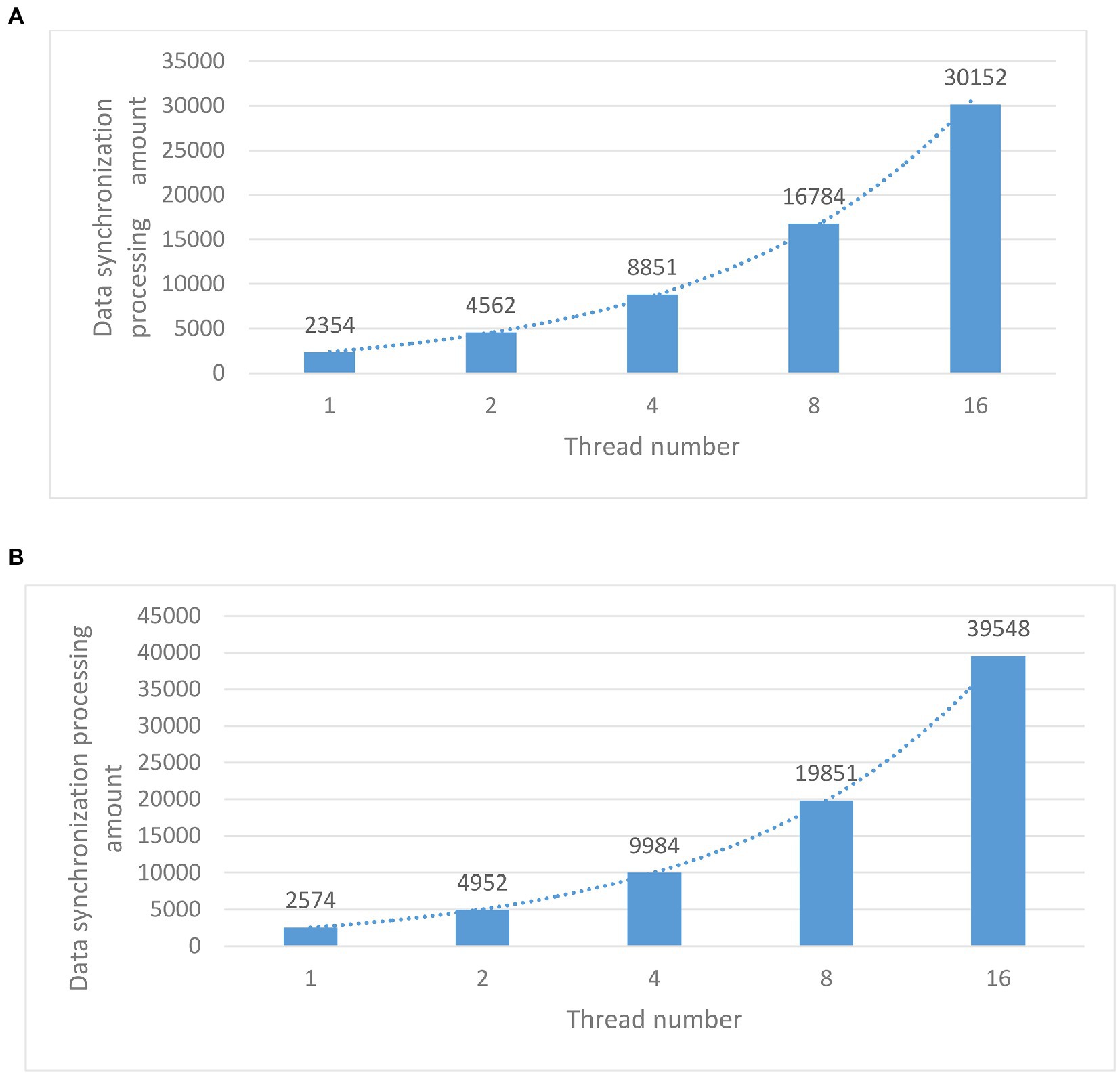
Figure 4. Comparison between the number of analysis threads and the amount of data synchronization processing of the two algorithms. (A) Analysis thread number and data synchronization processing capacity of traditional algorithms. (B) Analysis thread number and data synchronization processing volume based on IPAT model algorithm.
By analyzing the data in Figure 4, it can be known that when the number of online trips is 1, the data synchronization processing capacity of the traditional energy consumption analysis algorithm and the energy consumption analysis algorithm based on IPAT model are 2,354 and 2,574 respectively, and the data between them is not different. When the number of threads is 16, their data synchronization processing capacity is 30,152 and 39,548, respectively. It can be seen that there is a huge gap between the data synchronization processing capacity of the two algorithms. It shows that the energy consumption analysis algorithm based on IPAT model has higher data synchronization processing capacity in the case of multi-thread operation.
Then, the memory occupation of the two algorithms is investigated, and the change of memory occupation with the running time is studied. The results are shown in Figure 5.
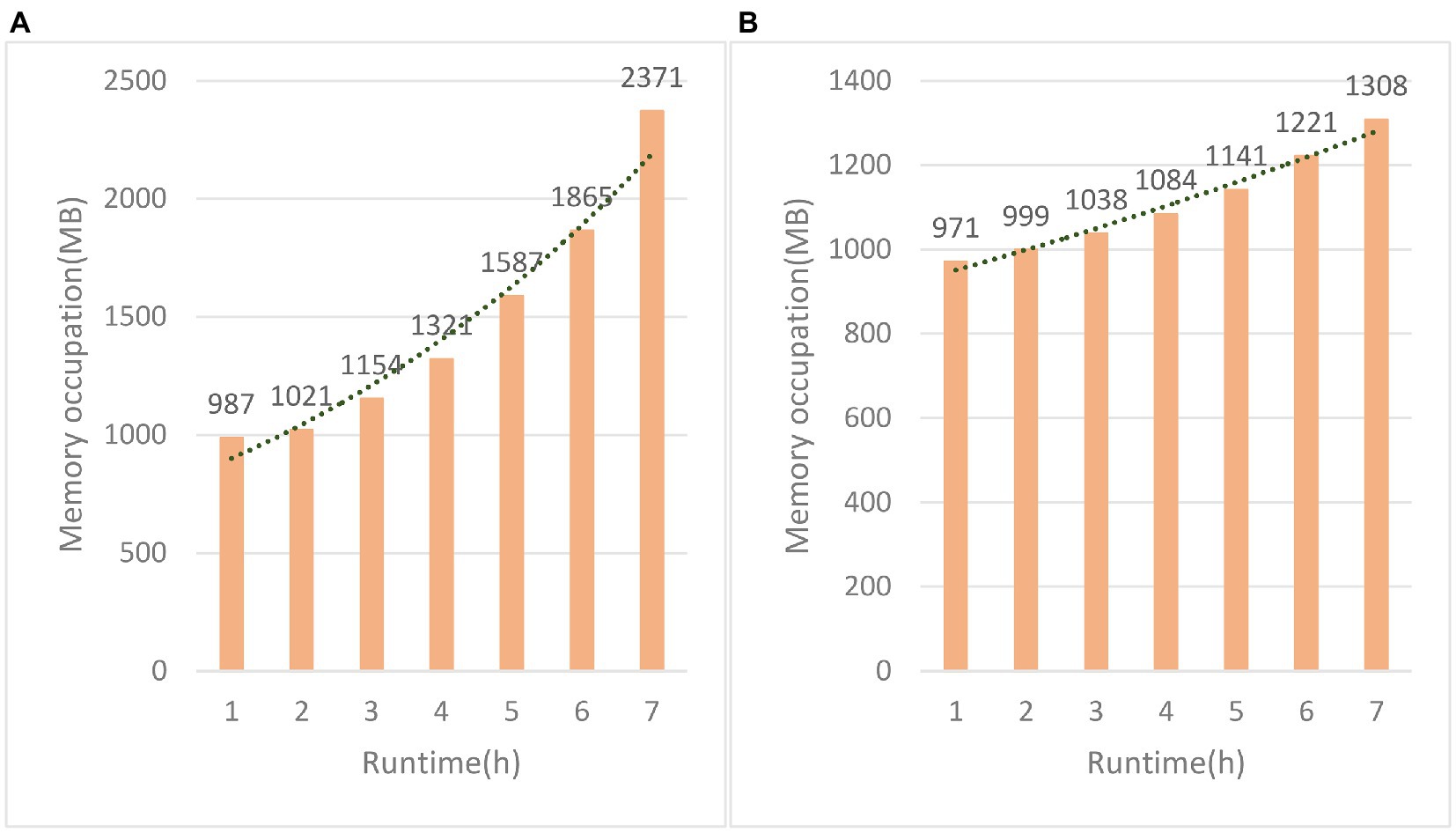
Figure 5. Comparison of memory usage of the two algorithms. (A) Memory occupation diagram of traditional algorithms (B) Memory occupation diagram of algorithms based on IPAT model.
Through the analysis of the experimental data in Figure 5, it can be learned that when the running time is 1 h, the memory occupation of the traditional energy consumption analysis algorithm and the energy consumption analysis algorithm based on IPAT model are 987 MB and 971 MB respectively, and the gap between them is relatively small. When the running time is 7 h, their memory consumption is 2,371 MB and 1,308 MB, respectively. Obviously, the energy consumption analysis algorithm based on IPAT model occupies less memory.
4.2. Experiment of energy economic management under the background of carbon neutralization and carbon peak
First, China’s carbon emissions and economic development in recent years are investigated. The total carbon emissions, per capita carbon emissions and GDP are statistically investigated. The results are described in Table 1.
As can be seen from Table 1, from 2008 to 2012, the total carbon emissions have increased from 7.38 billion tons to 9.00 billion tons. The per capita carbon emissions have increased from 1.42 tons of coal to 1.71 tons of coal. In addition, the economy has also developed rapidly, with GDP increasing from 31924.4 billion yuan to 53857.9 billion yuan. This shows that with the development of economy, energy consumption has increased significantly, and carbon emissions have also increased rapidly.
Before 2013, China’s carbon emissions were in a period of rapid growth. After 2013, it is necessary to change the strategy of energy economic management and re-design the standardized path of energy economic management. Therefore, it is necessary to implement energy conservation and emission reduction measures through the concept of carbon neutralization and carbon peak, so as to make the carbon emissions and economic development enter a stable period. The energy consumption analysis algorithm based on IPAT model is used to analyze and forecast China’s carbon emissions and GDP. The carbon emissions and GDP values under the baseline scenario and the carbon neutral scenario can be analyzed.
First, the carbon emissions are predicted, and the carbon emissions under the baseline scenario and the carbon neutral scenario are analyzed, respectively. The results are shown in Figure 6.
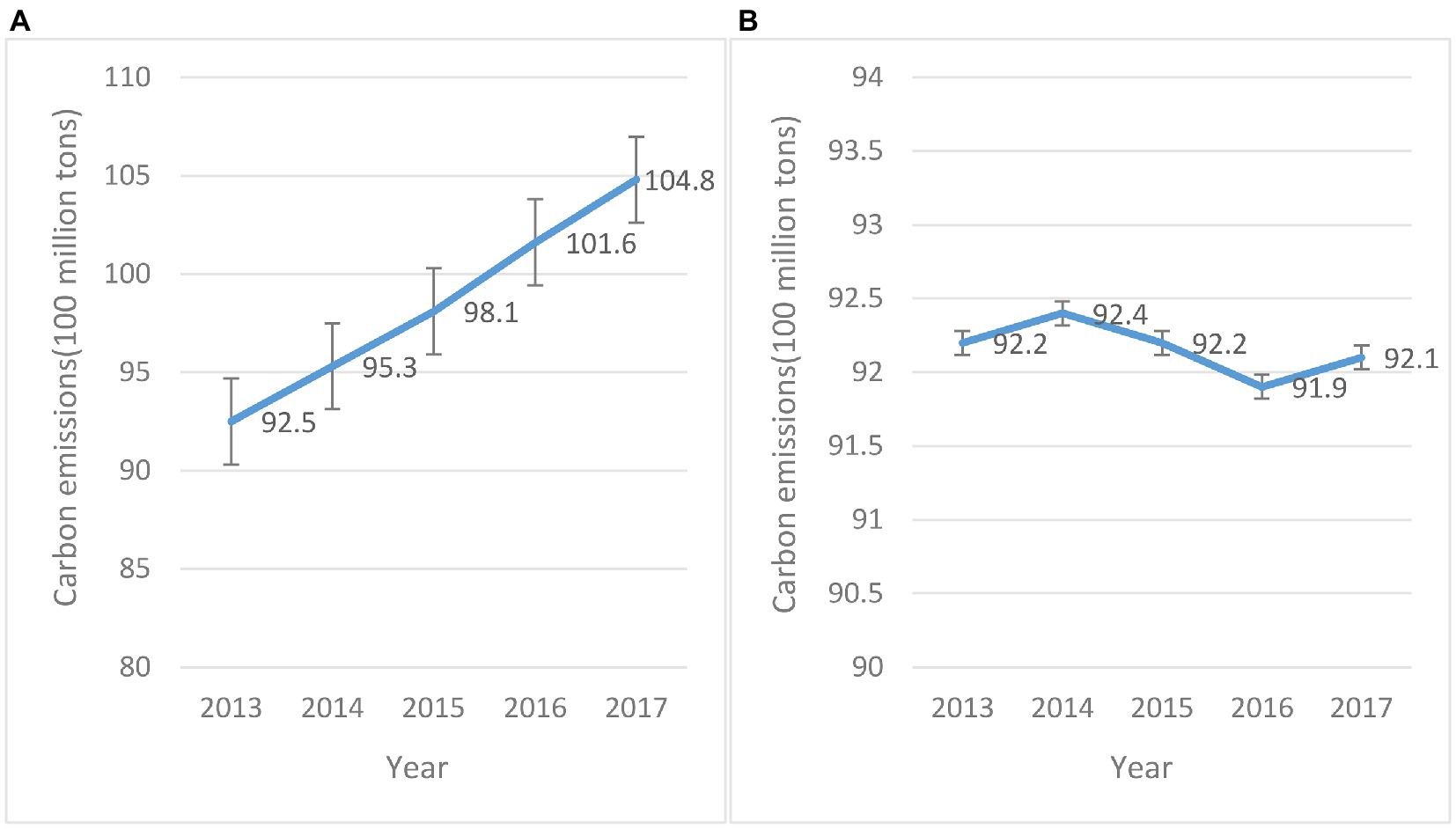
Figure 6. Carbon emission pre-measurement diagram. (A) Carbon emission prediction under the baseline scenario. (B) Carbon emission prediction under the carbon neutral scenario.
Through the analysis of Figure 6, it can be learned that in 2013–2017, the carbon emissions under the baseline scenario have increased year by year, from 9.25 billion tons to 10.48 billion tons. Under the carbon neutral scenario, its carbon emissions in 2013–2017 are 9.22 billion tons, 9.24 billion tons, 9.22 billion tons, 9.19 billion tons and 9.21 billion tons, respectively. It can be learned that under the carbon neutral scenario, its carbon emissions tend to be stable.
Then, by using the same method, the GDP under the forecast baseline scenario and the carbon neutral scenario are analyzed separately. The results are shown in Figure 7.
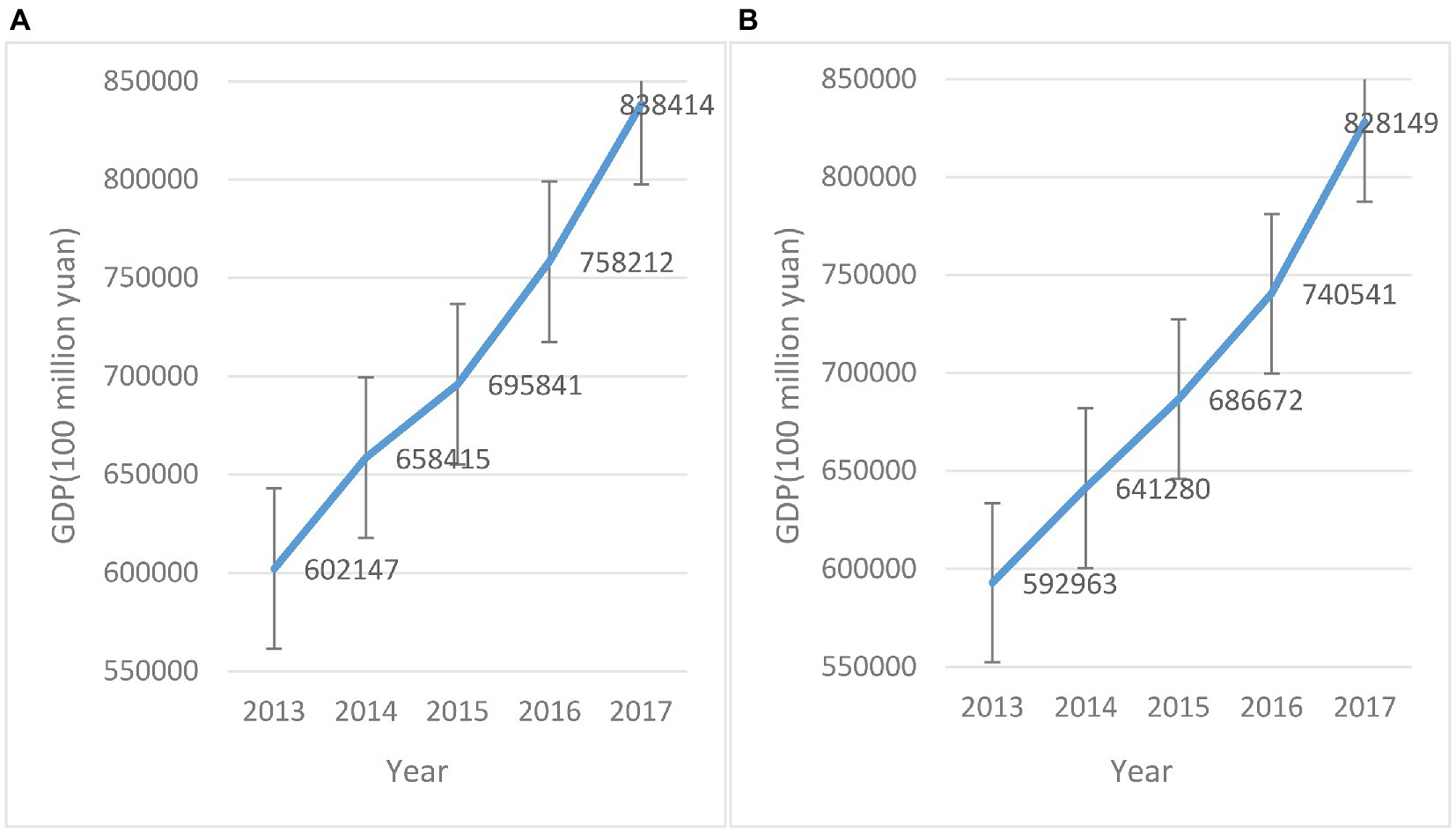
Figure 7. GDP forecast. (A) GDP forecast under the baseline scenario. (B) GDP forecast under the carbon neutral scenario.
According to the data in Figure 7, it can be analyzed that the GDP under the baseline scenario has increased from 60214.7 billion yuan to 83841.4 billion yuan in 2013–2017. Under the carbon neutral scenario, its GDP has increased from 59296.3 billion yuan to 82814.9 billion yuan. It can be seen that although the GDP under the carbon neutral scenario is smaller than that under the baseline scenario, it is not much smaller.
To sum up, after the implementation of the concept of carbon neutrality and carbon peaking, and the implementation of energy conservation and emission reduction measures, the carbon emissions have decreased significantly, while the GDP has decreased by a small amount. This shows that the environment and optimize energy management can be protected by sacrificing a little GDP. It can also prove that the standardization path of energy economic management based on the background of carbon neutrality and carbon peak is feasible.
5. Conclusion
Carbon neutrality refers to reducing greenhouse gas emissions by removing carbon dioxide from the atmosphere, thus curbing climate change. Carbon peak refers to that in the next few decades, the carbon dioxide emitted by human beings reaches the peak, and then begins to decline gradually. Under the background of carbon neutrality and carbon peak, energy economic management is particularly important. Energy economic management refers to the management measures to promote sustainable economic development through rational allocation and use of energy resources, improve energy efficiency and reduce energy consumption and environmental pollution. In the context of carbon neutrality and carbon peak, the main task of energy economic management is to promote the promotion and use of low-carbon energy and reduce carbon emissions. In order to achieve this goal, a variety of measures can be taken, such as improving energy efficiency, developing low-carbon energy, implementing energy price policies, promoting energy conservation and emission reduction technologies, establishing energy markets, and establishing energy information platforms. This paper analyzed the energy economic management path in the context of carbon neutralization and carbon peak. The energy consumption analysis algorithm based on IPAT model proposed in this paper had an excellent role for research. This study believed that the energy consumption analysis algorithm based on IPAT model could effectively improve the synchronous processing of energy data under multi-thread operation. In addition, it could also reduce the memory footprint at runtime. Through the research and experiment of energy economic management in the context of carbon neutralization and carbon peaking, it can be understood that under the concept of carbon neutralization and carbon peaking, carbon emissions were greatly reduced, while the economic reduction was very small. Through this experiment, the development route of low carbon economy could be explained, which provided an excellent hint for energy management under low carbon economy. As one of the effective ways to solve energy problems, energy economic management has a very broad development prospect. With the continuous growth of energy demand, energy economic management has received more and more attention. Energy economic management should strengthen support for energy conservation and emission reduction. By promoting energy-saving technologies and improving energy efficiency, energy consumption costs and pollution have been reduced. In addition, energy economic management should strengthen the protection of energy resources, and reduce the consumption of energy resources by improving energy utilization efficiency and promoting renewable energy, so as to protect the sustainable use of energy resources.
Data availability statement
The original contributions presented in the study are included in the article/supplementary material, further inquiries can be directed to the corresponding author.
Author contributions
The author confirms being the sole contributor of this work and has approved it for publication.
Funding
This study is funding by Yongjiang Talent Project of Ningbo (2022B-034-G).
Conflict of interest
The author declares that the research was conducted in the absence of any commercial or financial relationships that could be construed as a potential conflict of interest.
Publisher’s note
All claims expressed in this article are solely those of the authors and do not necessarily represent those of their affiliated organizations, or those of the publisher, the editors and the reviewers. Any product that may be evaluated in this article, or claim that may be made by its manufacturer, is not guaranteed or endorsed by the publisher.
References
Dong, L., Miao, G., and Wen, W. (2021). China’s carbon neutrality policy: objectives, impacts and paths. East Asian Policy 13, 5–18. doi: 10.1142/S1793930521000015
Li, Z., Bahramirad, S., Paaso, A., Yan, M., and Shahidehpour, M. (2019). Blockchain for decentralized transactive energy management system in networked microgrids. Electr. J. 32, 58–72. doi: 10.1016/j.tej.2019.03.008
Sadeeq, M. A. M., and Zeebaree, S. (2021). Energy management for internet of things via distributed systems. J. Appl. Sci. Technol. Trends 2, 59–71. doi: 10.38094/jastt20285
Mahapatra, B., and Nayyar, A. (2022). Home energy management system (HEMS): concept, architecture, infrastructure, challenges and energy management schemes. Energy Syst. 13, 643–669. doi: 10.1007/s12667-019-00364-w
Ning, X., Tian, W., He, F., Bai, X., Sun, L., and Li, W. (2023). Hyper-sausage coverage function neuron model and learning algorithm for image classification. Pattern Recogn. 136:109216. doi: 10.1016/j.patcog.2022.109216
Ning, X., Tian, W., Yu, Z., Li, W., Bai, X., and Wang, Y. (2022). HCFNN: high-order coverage function neural network for image classification. Pattern Recogn. 131:108873. doi: 10.1016/j.patcog.2022.108873
Ning, X., Xu, S. H., Nan, F. Z., Zeng, Q. L., Wang, C., Cai, W. W., et al. (2022). Face editing based on facial recognition features IEEE transactions on cognitive and developmental systems. 1–1. doi: 10.1109/TCDS.2022.3182650
Ramya, K., Teekaraman, Y., and Ramesh Kumar, K. A. (2019). Fuzzy-based energy management system with decision tree algorithm for power security system. Int. J. Comput. Intell. Syst. 12, 1173–1178. doi: 10.2991/ijcis.d.191016.001
Rathor, S. K., and Saxena, D. (2020). Energy management system for smart grid: an overview and key issues. Int. J. Energy Res. 44, 4067–4109. doi: 10.1002/er.4883
Schirmer, P. A., and Mporas, I. (2023). On the non-intrusive extraction of residents’ privacy- and security-sensitive information from energy smart meters. Neural Comput. Applic. 35, 119–132. doi: 10.1007/s00521-020-05608-w
Shan, P., Lai, X., and Liu, X. (2019). Correlational analytical characterization of energy dissipation-liberation and acoustic emission during coal and rock fracture inducing by underground coal excavation. Energies 12:2382. doi: 10.3390/en12122382
Shu, Y., Zhang, L., Zhang, Y., Wang, Y., Lu, G., Yuan, B., et al. (2021). Carbon peak and carbon neutrality path for China’s power industry. Strategic Study Chin. Acad. Eng. 23, 1–14. doi: 10.15302/J-SSCAE-2021.06.001
Tripathy, B. R., Sajjad, H., Elvidge, C. D., Ting, Y., Pandey, P. C., Rani, M., et al. (2018). Modeling of electric demand for sustainable energy and management in India using spatio-temporal DMSP-OLS night-time data. Environ. Manag. 61, 615–623. doi: 10.1007/s00267-017-0978-1
Xiao, G., Wang, T., Chen, X., and Zhou, L. (2022). Evaluation of ship pollutant emissions in the ports of Los Angeles and Long Beach. J. Marine Sci. Eng. 10:1206. doi: 10.3390/jmse10091206
Yin, L., Li, X., Gao, L., Lu, C., and Zhang, Z. (2017). A novel mathematical model and multi-objective method for the low-carbon flexible job shop scheduling problem. Sustainable Comput. Inf. Syst. 13, 15–30. doi: 10.1016/j.suscom.2016.11.002
Zhang, S., and Chen, W. (2022). Assessing the energy transition in China towards carbon neutrality with a probabilistic framework. Nat. Commun. 13, 87–15. doi: 10.1038/s41467-021-27671-0
Zhao, Y., and Kok Foong, L. (2022). Predicting electrical power output of combined cycle power plants using a novel artificial neural network optimized by electrostatic discharge algorithm. Measurement 198:111405. doi: 10.1016/j.measurement.2022.111405
Zos-Kior, M., Hnatenko, I., Isai, O., Shtuler, I., Samborskyi, O., and Rubezhanska, V. (2020). Management of efficiency of the energy and resource saving innovative projects at the processing enterprises. Manage. Theory Stud. Rural Bus. Infrastruct. Dev. 42, 504–515. doi: 10.15544/mts.2020.52
Keywords: energy economic management, carbon neutral, peak carbon dioxide emissions, energy consumption analysis algorithm, IPAT model
Citation: Liu J (2023) Path analysis of energy economic management standardization in the context of carbon neutralization and carbon peak. Front. Ecol. Evol. 11:1155401. doi: 10.3389/fevo.2023.1155401
Edited by:
Xin Ning, Institute of Semiconductors (CAS), ChinaReviewed by:
Yitong Niu, Sofia University, United StatesQazi Salman Khalid, University of Engineering and Technology, Peshawar, Pakistan
Hu Qinhui, Guilin University of Aerospace Technology, China
Copyright © 2023 Liu. This is an open-access article distributed under the terms of the Creative Commons Attribution License (CC BY). The use, distribution or reproduction in other forums is permitted, provided the original author(s) and the copyright owner(s) are credited and that the original publication in this journal is cited, in accordance with accepted academic practice. No use, distribution or reproduction is permitted which does not comply with these terms.
*Correspondence: Jilu Liu, bGl1amxAend1LmVkdS5jbg==