- 1Aix Marseille University, Avignon University, CNRS, IRD, IMBE, Marseille, France
- 2Ecological Consultancy ECO-MED, Marseille, France
- 3University Grenoble Alpes, Institut National de recherche pour l'agriculture, l'alimentation et l'environnement (INRAE), Laboratoire des écosystémes et sociétés en montagne (LESSEM), St-Martin-d’Hères, France
- 4Avignon University, Aix Marseille University, CNRS, IRD, IMBE, Avignon, France
Semi-natural mountain grasslands are species-rich and provide a wide range of ecosystem services, such as biomass production for livestock grazing and carbon sequestration. Land abandonment but also habitat destruction are threatening these ecosystems highlighting the need for restoration when degraded. The transfer of plant material containing seed collected at non-degraded reference sites is increasingly used for restoration but the appropriate methods to prepare the transfer and to manage the grassland afterwards are still under debates. This study aims at testing the effects of seed bed preparation by harrowing and livestock grazing on the establishment of transferred targets and community trajectories towards the reference system. In a previous study, first year seedling counts showed a positive effect of seed bed preparation and a marginally significant negative effect of grazing on the seedling recruitment of the transferred species. We asked whether the results on first year seedling densities translate into significant effects on plant cover and whether these effects on plant cover change in the following three years. Both grazing and harrowing effects on species establishment were tested using a full factorial split-plot experimental design on five replicate sites. Data on species richness and vegetation cover for both the whole plant community and the transferred species were analysed during four years after seed transfer. The cover of transferred species increased whereas their species richness remained unchanged. We found a weak positive effect of harrowing on total cover whereas grazing had no effect. Seed bed preparation by harrowing had a clear positive effect on transferred target species cover. The negative effect of grazing on first-year seedling recruitment of transferred target species turned into a positive effect in the fourth year. However, this effect was only apparent in the harrowed plots resulting in a significant grazing-by-harrowing interaction. The similarity of the plant communities to the reference increased from the first to the fourth year and this increase was stronger in grazed and harrowed plots. In conclusion, the results confirmed the positive effect of seed bed preparation on restoration by seed transfer and demonstrated that initial grazing exclusion is not needed in mountain grasslands of our study area.
Introduction
While grasslands occupy an important place in the maintenance and functioning of terrestrial ecosystems, their degradation is still ongoing (Bardgett et al., 2021). Since 2021-2030 was announced the decade of ecosystem restoration by the United Nations, it is becoming crucial to consider grasslands as essential ecosystems to conserve and restore terrestrial biodiversity and ecosystem services, as well as to mitigate climate change (Temperton et al., 2019; Zhao et al., 2020; Programme UNE and Nations, 2022). In their recent review, Bengtsson et al. (2019) addressed readers directly in “Grasslands are more important for ecosystem services than you might think” and detailed numerous grassland ecosystem services. Furthermore, many authors have highlighted the importance of conserving grasslands, integrating them into long-term restoration (and monitoring) projects as well as implementing initiatives to enhance their conservation (Bengtsson et al., 2019; Temperton et al., 2019; Veldman et al., 2019; Buisson et al., 2022).
To restore species-rich grasslands or, at least, to accelerate their recovery, it is widely accepted that the lack of seed dispersal must be overcome (Török et al., 2011; Halassy et al., 2016; Török et al., 2018). The seed bank often contains annual plant species or disturbance-tolerant perennials (Erfanzadeh et al., 2016; Buisson et al., 2018), but it often lacks grassland specialists (Kiss et al., 2016), in particular if the seed bank was diminished by topsoil disturbance (compaction, removal). This may be achieved by active supply of seeds, preferably locally harvested using “near-natural restoration methods” or “nature-based solutions” (Tischew and Kirmer, 2007; Auestad et al., 2016; Valkó et al., 2022). These methods include transferring mown hay (Auestad et al., 2016; Kövendi-Jakó et al., 2019) or seed containing material collected by a brush harvester (Albert et al., 2019; Durbecq et al., 2022) from nearby non-degraded grasslands (Kiehl et al., 2010; Török et al., 2011; Scotton et al., 2012; Scotton, 2019). Furthermore, seed addition experiments showed that enhancing restoration by sowing solely is not always successful. Plant recruitment and establishment are further limiting steps of grassland restoration (Turnbull et al., 2000; Pywell et al., 2002; Bissels et al., 2006; Clark et al., 2007; Buisson et al., 2021). Seed bed preparation by topsoil disturbance before hay or seed transfer is therefore recommended by several authors (Kiehl et al., 2010; Muller et al., 2014; Klaus et al., 2017; Bischoff et al., 2018). Soil disturbance by ploughing, tilling or harrowing at shallow depth (<20 cm) is suggested to enhance the recruitment of the sown species (Hölzel et al., 2003; Schmiede et al., 2012; Long et al., 2014; Czerwiński et al., 2018) by (i) opening space and limiting competition with pre-existing vegetation (Poschlod and Biewer, 2005; Pywell et al., 2006; Klaus et al., 2017; Bischoff et al., 2018), and (ii) improving environmental conditions for germination and seedling recruitment, such as the creation of micro-reliefs increasing seed-soil adhesion (Chambers, 2000; Isselin-Nondedeu et al., 2006; Kiehl et al., 2010). However, soil disturbance may reduce the protecting effect of existing vegetation limiting high solar radiation and desiccation of young seedlings (Gibson, 2009).
The creation of recruitment gaps can also be achieved via livestock trampling (Kladivová and Münzbergová, 2016). European semi-natural grasslands are shaped by extensive grazing or mowing management (Gibson, 2009; Kuneš et al., 2015). Traditional grazing by livestock is needed to prevent natural succession towards woody vegetation and favours the recruitment of typical grassland species (Hejcman et al., 2013). Moreover, as long-term post-restoration management measure, extensive grazing may increase the establishment of semi-natural grassland target species by creating regeneration gaps (Török et al., 2011; Freitag et al., 2021; Tölgyesi et al., 2022). However, at the beginning of ecological restoration including seed addition, the restoration site may need fencing to protect the seedlings of sown species from livestock trampling and feeding during the first years after sowing (Bakker, 2003; Rother et al., 2013; Buisson et al., 2015; Vidaller et al., 2019).
Our objective was to assess the effects of seed bed preparation by harrowing and the effect of initial grazing. The grazing exclusion treatments were fenced only the first two years after sowing while traditional grazing management was applied thereafter. First year seedling counts showed a positive effect of seed bed preparation on seedling recruitment and that grazing had a marginally significant negative effect on seedling recruitment of transferred species (Durbecq et al., 2021). In this study, we asked whether the results on first year seedling densities translate in significant effects on plant cover and whether these effects on plant cover change in the following years. More specifically, we addressed the following questions: (i) does the weak negative effect on seedling recruitment change to a positive effect in the following three years? (ii) does the positive effect of harrowing observed in the beginning persists over the following years and may thus affect the final outcome of restoration? (iii) does the mid-term grazing effect depend on seed bed preparation resulting in a significant interaction of both treatments?
We first hypothesize that an initial negative effect of grazing on transferred species changes into a positive effect after establishment since typical grassland species are favoured by grazing in the long run (Saatkamp et al., 2018; Martin et al., 2022), and that both annual weeds and ruderal plants dominating in early succession later disappear (Labadessa et al., 2020). We further hypothesize that seed preparation has a long-term positive effect on mountain grassland restoration in limiting competition by undesired perennial species and facilitating seedling recruitment of transferred species. (Freitag et al., 2021; Martin et al., 2022).
Methods
Study sites location and description
The study was conducted at five degraded mountain grassland sites in the Southern Alps, in the French Hautes-Alpes department (Figure 1). The degradation was caused by the renovation and extension of an electricity line including the construction of transitory access tracks and platforms, leading to the removal of vegetation and soil compaction-decompaction in spring 2018. The rapid return of species-rich semi-natural grasslands was identified as major objective to compensate for grassland degradation. We selected sites representative of the study zone impacted by construction work. Selection criteria were elevation (between 1100 and 1300 meters above sea level (m asl)), traditional management by grazing not including undergazed sites with shrub encroachment and time since the end of the construction work (< 6 months). We avoided driest and most humid sites, extreme slopes, strong soil modification (gravel addition) and particularly stony sites. Three sites were located in the municipality of L’Argentière-la-Bessée (between 44°78’77’’N, 6°56’32’’E and 44°78’31’’N, 6°56’45’’E; 1,058 to 1,132 m asl). The fourth site was in Embrun (44°58’47’’N; 6°48’31’’E; 1,312 m asl) and the fifth site was in La-Bâtie-Neuve (44°58’54’’N, 6°20’75’’E; 1,264 m asl).
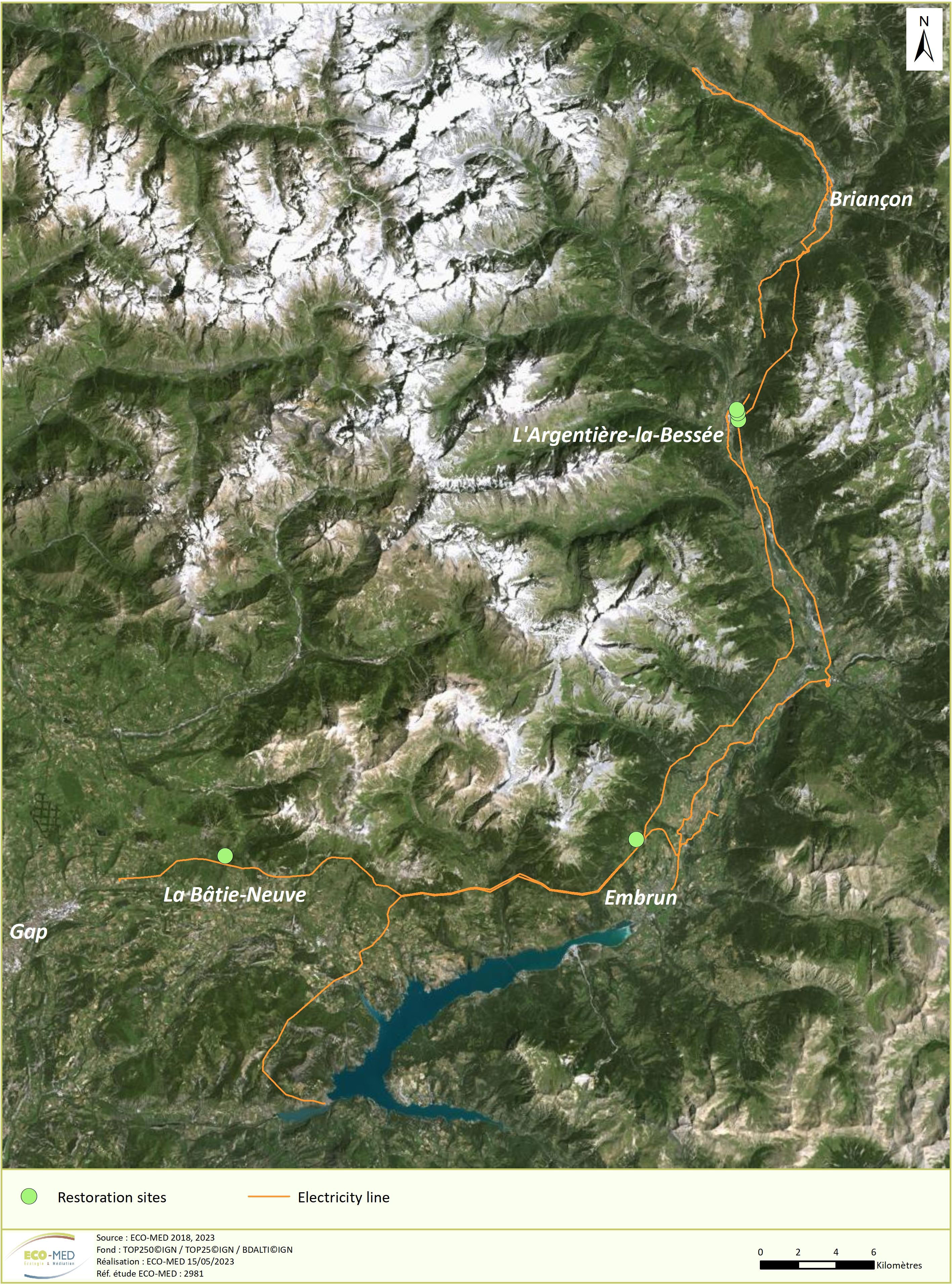
Figure 1 Location of the study area with the three restoration sites (in green) along the construction of a new electricity line (in orange).
The sites were all located in the upper Durance valley at the upper montane altitudinal belt where the climate is temperate to sub-Mediterranean. The Durance valley is characterized by 740 mm of annual precipitation and average July and January temperatures of 20°C and 2°C, respectively, with an annual mean of 10.7°C (Embrun meteorological station, 863 m asl). Due to the higher elevation compared with the meteorological station, temperatures of the study sites are 1.5 to 3°C lower. At the study sites, snow and frost occur from October to April with a usually continuous snow cover in January and February.
The grasslands of the upper Durance valley are traditionally managed and maintained open by cattle or sheep grazing, and to a lesser degree by mowing. The mountain grasslands of the area are part of a transhumance system with winter/spring grazing in the valleys and summer grazing in the alpine belt. Thus, our sites were grazed twice a year in late spring and autumn with low grazing intensity (<0.5 animal units/ha). The prevailing grazing management is transhumance with winter grazing in the bottom of the valley and summer grazing in the alpine part. The montane altitudinal belt of our study site is thus grazed twice a year for a short period in late spring and autumn. At this montane level, grasslands are characterised by Mesobromion plant communities. Accordingly, they belong to the Natura 2000 priority habitat type ‘Semi-natural dry grasslands of Festuco-Brometalia and scrubland facies on calcareous substrates’ (N6210, EU directive habitat 92/43/EEC; Calaciura and Spinelli, 2008). Grasslands of this habitat type are often nutrient-poor and particularly species-rich (WallisDeVries et al., 2002). The main environmental drivers of plant communities are elevation, slope, aspect and bedrock (Durbecq et al., 2020).
Seed material and field experiment
Local seeds were harvested in a traditionally managed and non-degraded semi-natural grassland of the Durance valley, in Freissinières (44°73′61″ N; 6°56′72″ E; 1,100 m asl), where the climatic and soil parameters are similar to the five study sites. Plant material was collected in July 2018 (seed production period for more than 80% of species in this region) with an integrated system combining beater brush harvester and vacuum system, all mounted on a quad to allow access to narrow and steep mountain sites (Appendix 1). We obtained 2.6 kg of dry plant material harvested in 4,000 m² source grassland. Seeds represented 60% of the harvested material and vegetative parts 40%. The average density of collected seeds was 887 seeds/g of plant material (counted in ten samples of 0.5 g of harvested plant material).
We established a full-factorial split-plot experiment at the five replicate study sites with seed bed preparation and seeding as split-plot factors, and grazing as whole-plot factor (see Durbecq et al., 2021). This resulted in eight plots of 4 m × 4 m treatments arranged in rectangles with 1 m distance between treatments (except for one site where they were separated by 50 cm distance due to lack of space; total = 40 plots). After mowing the already existing vegetation with a brush cutter, seed bed preparation and seeding were randomly assigned to eight plots within half-blocks: four plots exposed to livestock grazing comprising i) no seeding, no seed bed preparation (including removing existing vegetation), ii) seeding only, iii) seeding and seed bed preparation, iv) seed bed preparation only. The remaining four other plots, comprising the same treatments were fenced to exclude grazing. The non-sown control plots were only used to distinguish transferred and spontaneously emerging species but not included in the statistical analysis.
In October 2018, we implemented the seed bed preparation treatment by harrowing the soil (8 cm depth) using a rotary cultivator (Appendix 1). For the seeding treatment, we adjusted sown seed density by weight using 3.75 g/m² of seeds which corresponds to 104 g of harvested plant material per plot and approximately 5,700 seeds/m². This density is at the upper range of seed densities recommended for alpine grassland restoration projects in the Southern French Alps (2-4 g/m², Koch et al., 2015). The first half-blocks were fenced before the grazing periods and the other half-blocks were exposed to the local extensive grazing twice a year, in October and June. The exclosures were removed after the second-year vegetation survey, in September 2020. The three sites located in l’Argentière-la-Bessée were grazed by sheep and the two other sites by cattle corresponding to the sheep and cattle grazing ratio of the study region. The sites were neither irrigated nor fertilised.
Measurements and data analysis
Vegetation surveys were conducted at the restoration sites and at the source site of plant material transfer. The cover of all vascular plant species was visually estimated as the vertical projection of all above-ground plant organs.
For each plant species of the source site, percentage cover was estimated in five quadrats of 3 m × 3 m in early June 2018. The total plant cover for each quadrat may exceed 100% due to overlapping vegetation (Londo, 1976). Additionally, the proportion of individuals with mature seeds was estimated for all species occurring at the source site just before the harvest in July 2018. We found altogether 58 species with seeds (Appendix 2).
At restored sites, the 3 m × 3 m quadrats were located in the centre of the 4 m × 4 m quadrats to avoid edge effects, in June 2019, 2020, 2021 and 2022. For each site, species occurring in each sown treatment were compared to control plots, and those occurring in the control were identified as spontaneously emerging species. Species with ripe seeds occurring at the source site of plant material transfer were considered as “harvested species” (58 species, Appendix 2). Those harvested species that did not occur in the four control plots of the same restoration site (with largely higher cover in the seed transfer plots, ratio > 5:1) were identified as “transferred species” (27 species, Table 1). We can, however, not exclude that some species with seeds were finally not harvested because seeds or fruits were too low in the canopy or still strongly attached to the plants. Furthermore, the method may underestimate the density of transferred species since the occurrence of a species in unsown control plots does not necessarily mean that the species was not transferred.
Analysis
We used cover and species richness of the whole plant community (including both transferred species and species emerging from the soil seed bank or seed rain) and of transferred species only for each of the four years after sowing (2019, 2020, 2021 and 2022) to analyse the effect of early grazing and of seed bed preparation on grassland restoration. To focus our analyses on the effects of seed bed preparation and early grazing on both whole community and transferred species, analyses were performed with the two treatments “Grazing” and “Harrowing” and their interactions. Plant cover complied with the assumption of linear models (normality, homoscedasticity). Thus, linear mixed-effects models (LMM) were fitted using lmer function (R package lme4, Bates et al., 2015). Species richness was analysed using generalised linear mixed-effects models (GLMM) with Poisson error distribution and log-link function. No overdispersion occurred in Poisson models. In LMM and GLMM, the significance of treatments and interactions was tested using the Anova function (R package car, Fox & Weisberg 2019). The two treatments “Grazing” and “Harrowing” were fitted as fixed effects, while “site” was fitted as random effect. To consider the split plot experimental design (“Harrowing” and “Seeding” as split plot factors and “Grazing” as whole plot factor), grazing was tested against the site x grazing interaction whereas harrowing and the harrowing × grazing interactions were tested against the model error. As we were interested in the specific effect of treatments on vegetation at different years, data analyses were performed separately for each year. Post-hoc Tukey tests were calculated in the case of significant treatment effects or interactions to analyse which differences between treatment combinations were significant (emmeans package, Lenth, 2020).
To compare the composition of the plant communities in the different treatments in 2019 and in 2022, we applied a Non-Metric Multidimensional Scaling (NMDS) based on Bray-Curtis distance (Borcard et al., 2011), and we used a permutation multivariate analysis of variance (PERMANOVA, Anderson, 2001) with 9,999 permutations to analyse whether the plant composition was significantly different between treatments (R package vegan, Oksanen et al., 2022). Similarity to reference communities was calculated as 1 - the minimum Bray-Curtis distance (Bray and Curtis, 1957) between restoration sites and the reference site. We used the minimum Bray-Curtis distance instead of the average value in order to reduce the effect of the heterogeneity of references (Durbecq et al., 2020). We analysed grazing, harrowing effects and their interaction using the same model design as in the univariate analysis. We performed all analyses using R 4.2.0 (R Core Team, 2022).
Results
Whole community
The plant cover of the whole community (considering all species) in the four sown treatments increased over years with an average total cover of 28% (all treatments combined) in 2019, 54% in 2020, 61% in 2021, and 67% in 2022 (Figure 2). The grazing treatment had no effect on total cover. In the first year after sowing (2019, Figure 2A), the harrowing × grazing interaction was significant, with a greater cover in the non-grazed and non-harrowed treatment (Table 2A). One year later (2020, Figure 2B), this interaction was marginally significant but for different reasons than in 2019: the total plant cover was lower in the grazed and non-harrowed plots leading also to a significant harrowing main effect with plant cover being lower in non-harrowed plots (Table 2A, Figure 2B). In 2021 (Table 2A, Figure 2C), the third year after sowing, there was no effect of the treatments, whereas in 2022 the total plant cover was again lower in the non-harrowed plots (marginally significant, Table 2A, Figure 2D).
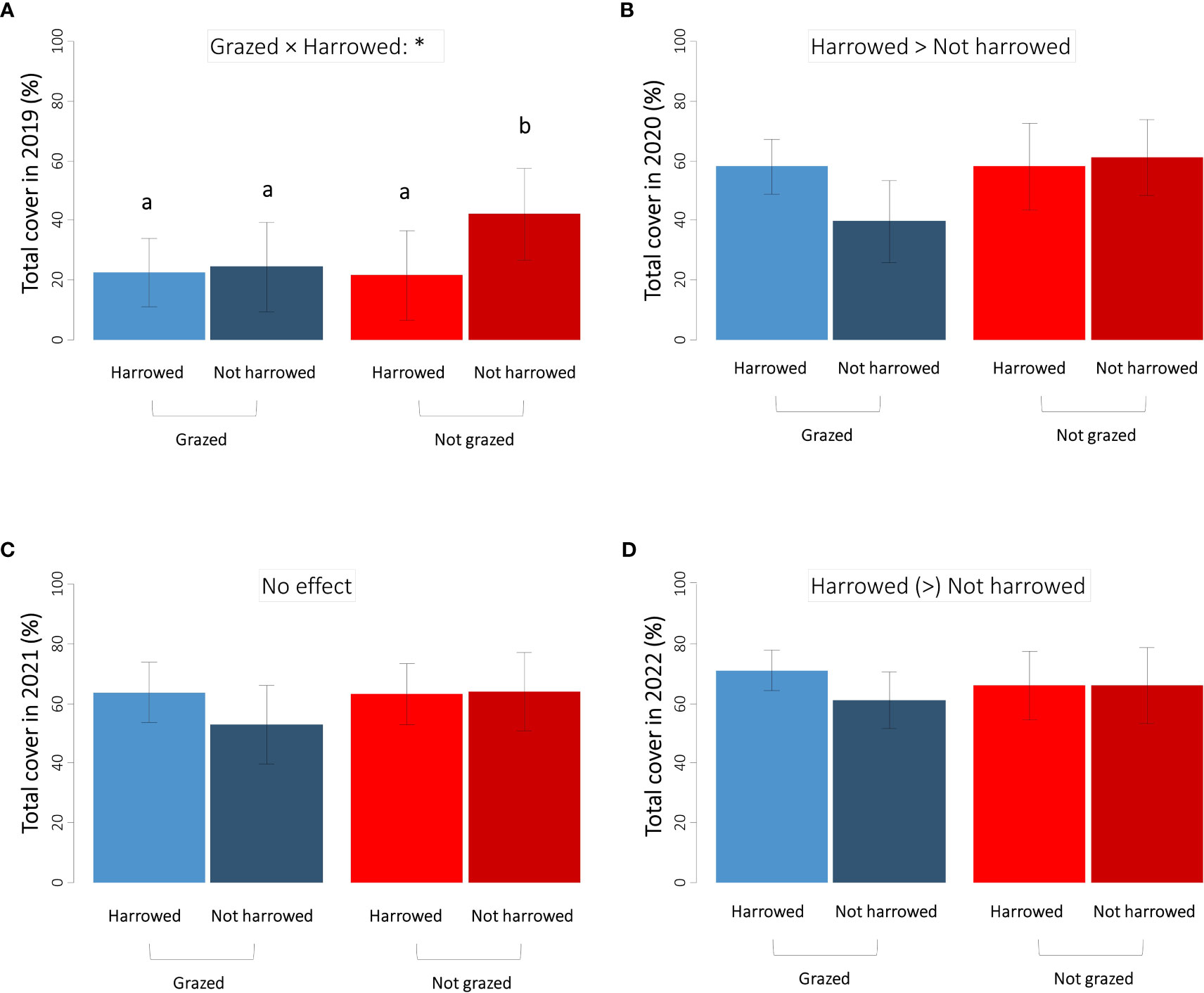
Figure 2 Total plant cover of the four sown treatments in (A) 2019, (B) 2020, (C) 2021 and (D) 2022. The direction of significant effects is specified using “>”: p < 0.05 and “(>)”: p < 0.1 (marginally significant). Significant harrowing × grazing interactions are indicated using “*” (p < 0.05). Error bars represent ±SE and different letters indicate significant differences between treatments (p < 0.05).
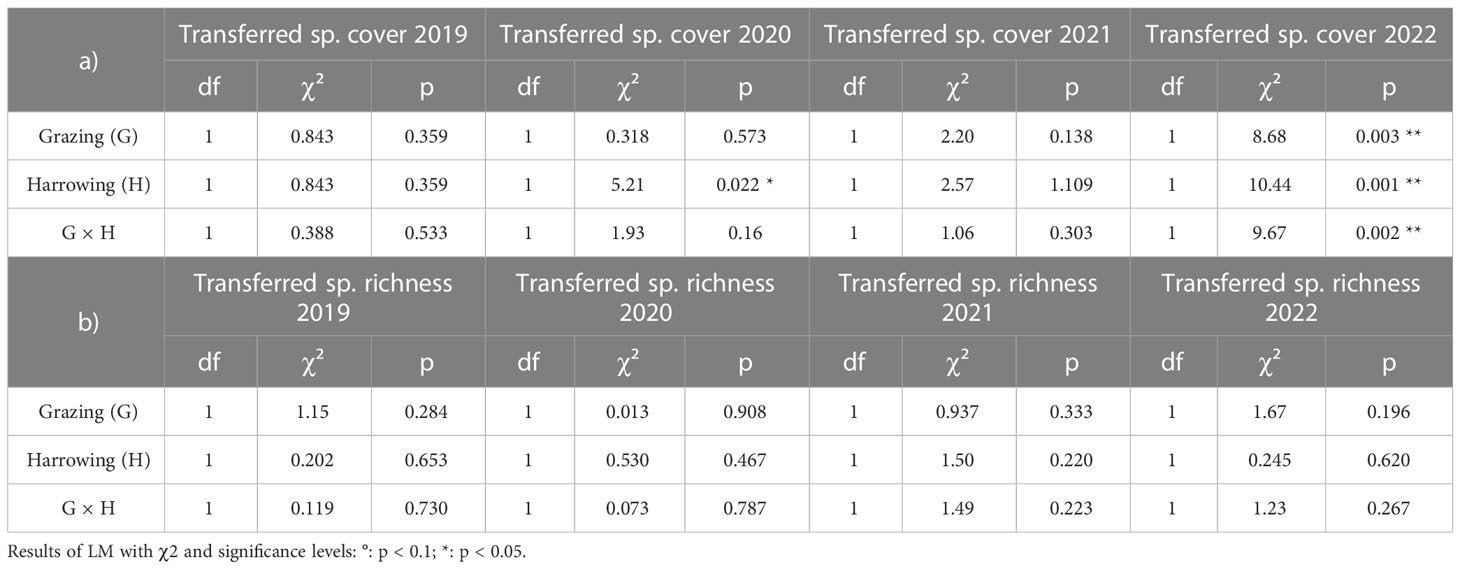
Table 2 Effect of grazing, harrowing and their interactions on a) cover and b) richness of the whole plant community in 2019, 2020, 2021 and 2022.
The average species richness of the plant communities was 27 species in 2019 compared to an average of 32 species for the following three years (Figure 3). No difference in total species richness was observed between the treatments and the interaction was never significant (Table 2B).
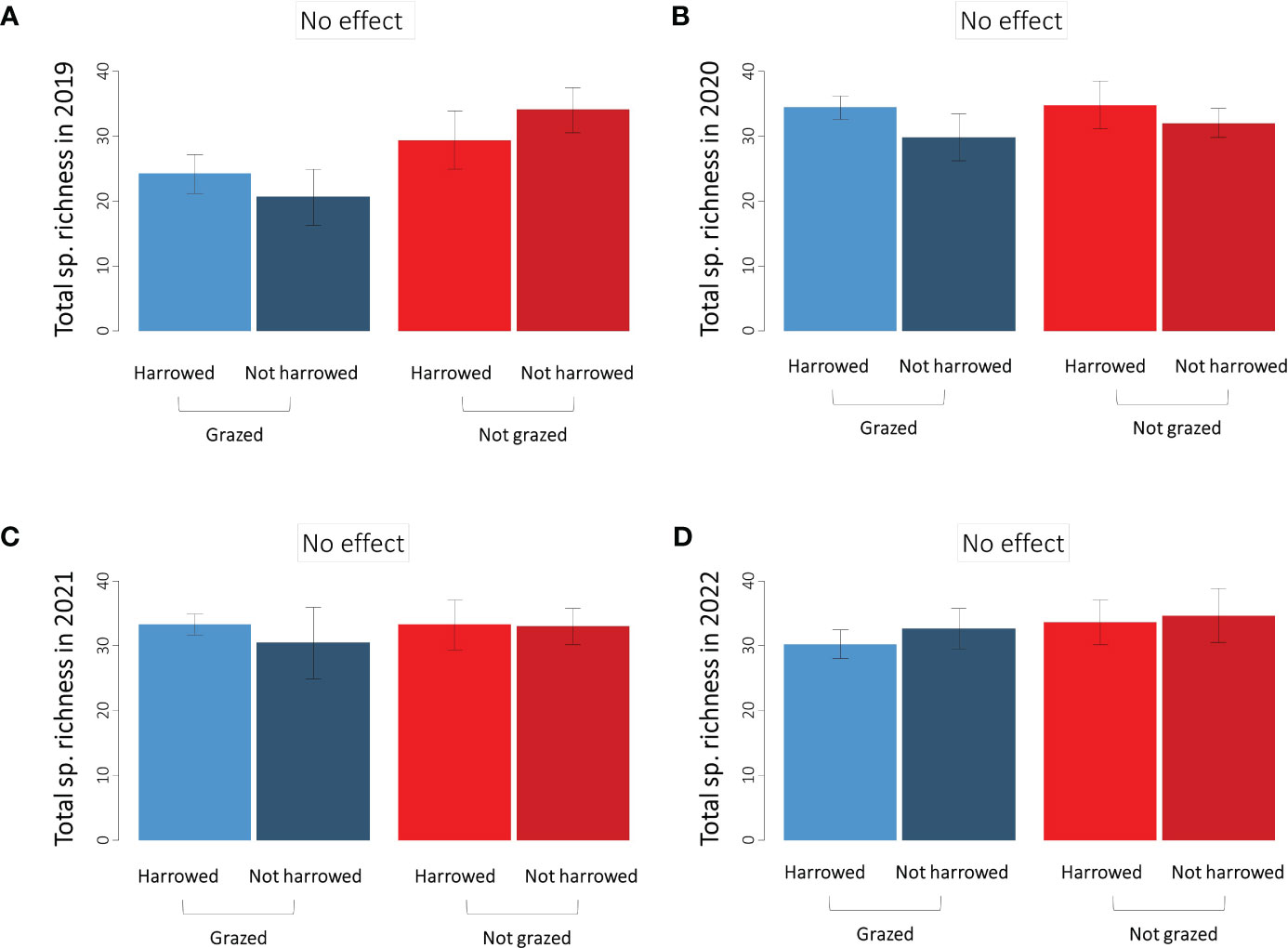
Figure 3 Species richness of the whole plant community in the four sown treatments in (A) 2019, (B) 2020, (C) 2021 and (D) 2022. Error bars represent ±SE.
The NMDS ordination revealed a clear separation of plant community composition between restoration treatments in 2019 and in 2022 (Figure 4). 2022 polygons were smaller than 2019 polygons and approached the source site in terms of species composition, indicating higher species similarity with the source site after three years. However, the source site was still clearly separated from the restoration sites in 2022. The PERMANOVA showed differences in plant species composition of the plant communities between treatments, both in 2019 and 2022. In 2019, a marginally significant effect of the grazing treatment was found (F1,19 = 1.50(*)), with typical species of the grazed plots occurring more on the bottom of NMDS axis 2, such as Alyssum alyssoides (L.) L., Reseda phyteuma L., Eryngium campestre L., Centaurea paniculata L., Stachys recta L., Saponaria ocymoides L. and Ononis pusilla L. Neither the harrowing effect nor harrowing × grazing interactions were significant. In 2022, the effect of grazing and the harrowing × grazing interaction on species composition were marginally significant (respectively F1,19 = 1.61(*) and F1,19 = 1.71(*)), distinguishing the two non-grazed plots from the two grazed plots. The similarity to references was not significantly affected by grazing or by harrowing treatments in 2019 (χ²G = 0.30 and χ²H = 1.95). However, in 2022, grazing, harrowing and their interactions had a significant effect on similarity to the reference site (χ²G = 16.3***, χ²H = 6.40* and χ²G×H = 7.41**). The grazed and harrowed plots had the highest (0.45+-0.04 SE) while the non-grazed plots had the lowest similarity to the reference site (0.31+-0.02 SE and 0.36+-0.03 SE for harrowed and not harrowed respectively, Appendix 3).
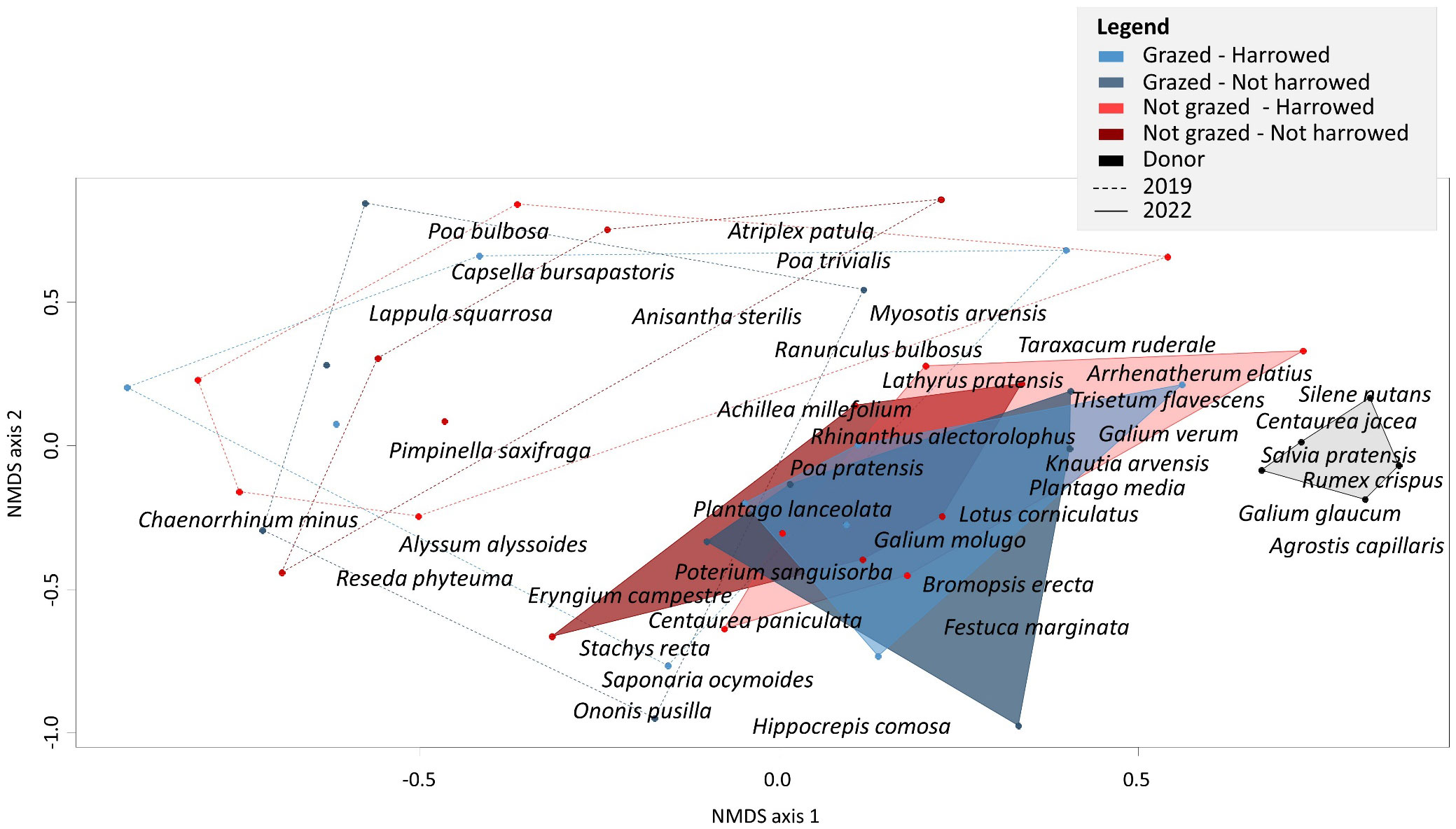
Figure 4 NMDS of plant species composition of the whole community in the four sown treatments in 2019 (dotted lines) and 2022 (full lines) compared to the plant species composition at the donor site. The two intermediate years 2020 and 2021 are not shown for clarity. Polygons indicate the position of the outmost plots in each treatment (stress = 0. 24). Transferred species are shown in bold.
Transferred species
Altogether 27 species of the source grassland were found in the sown restoration treatments (Table 1). After correction for species also occurring in control treatments, the number of considered transferred species was between four and eight per plot depending on year and treatment. The cover sum of these transferred species (pooled for treatments) clearly increased over the years with, on average, 1% in 2019, 9% in 2020, 18% in 2021, and 22% in 2022 (Figure 5). In the first and third years after sowing (2019 and 2021), the treatments had no effect on the transferred species cover (Table 3A, Figures 5A, C). However, both in 2020 and 2022, transferred species cover was significantly higher in the harrowed plots, particularly when the latter where grazed (Table 3A, Figures 5B, D). The year 2022 was characterised by significant effects of both treatments and their interaction (Table 3A). Transferred species cover was the highest in the grazed and harrowed plots, averaging at 34% (Figure 5D) and representing almost half of the whole community cover (76%, Figure 2D). In grazed and non-harrowed plots, however, the cover was only 14%, and it was 24% in the non-grazed and non-harrowed plots (Figure 5D). The significant harrowing × grazing interaction showed that the grazing effect depended on the seed bed preparation by harrowing and vice versa.
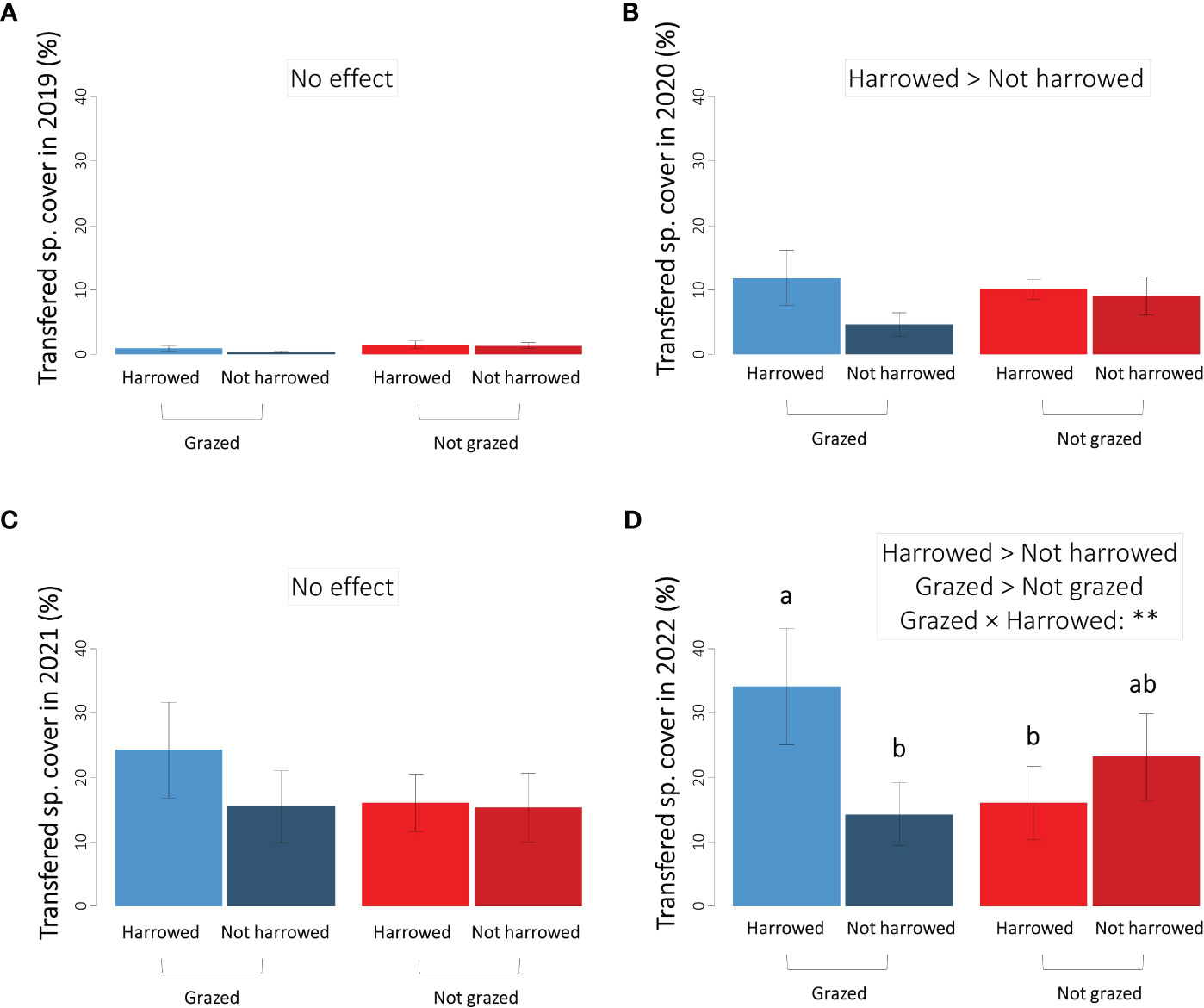
Figure 5 Sum of cover of transferred species in the four sown treatments in (A) 2019, (B) 2020, (C) 2021 and (D) 2022. The direction of significant effects is specified using “>”: p < 0.05. Significant harrowing × (p < 0.05).
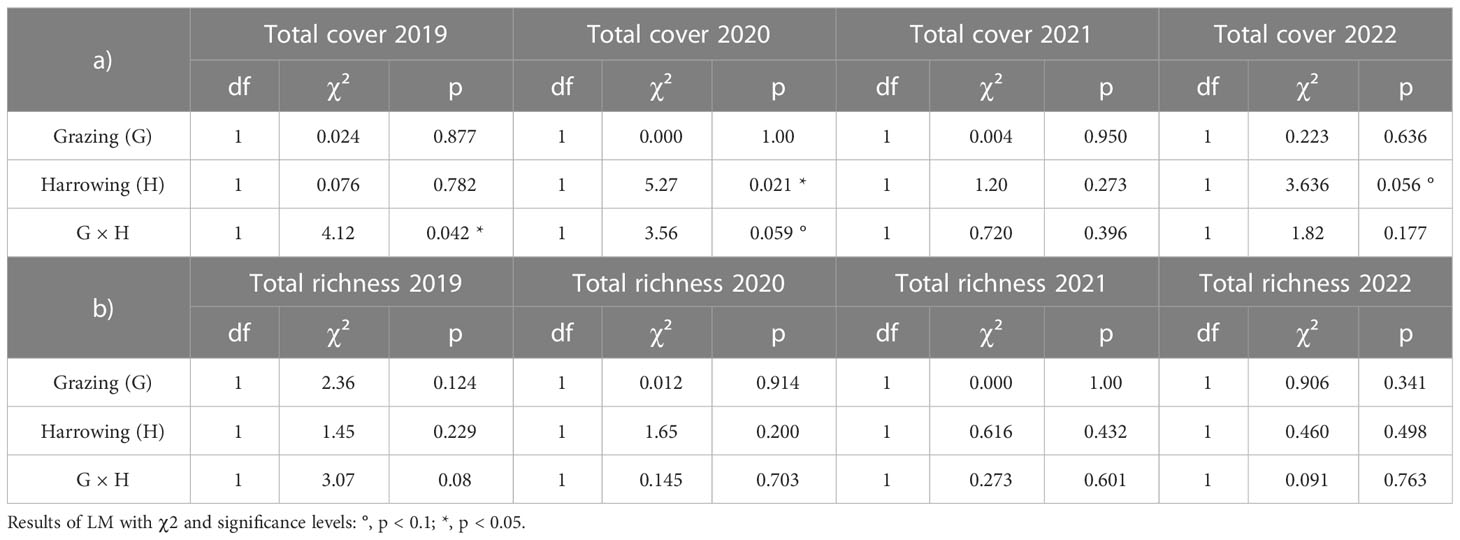
Table 3 Effect of grazing, harrowing and their interaction on transferred species a) cover, and b) richness, in 2019, 2020, 2021 and 2022.
The transferred species richness was similar between treatments in the first three years after sowing (Table 3B, Figures 6A–C). We found a marginally significant effect of the grazing treatment in 2022 with slightly more transferred species in the grazed than in non-grazed plots (Table 3B, Figure 6D).
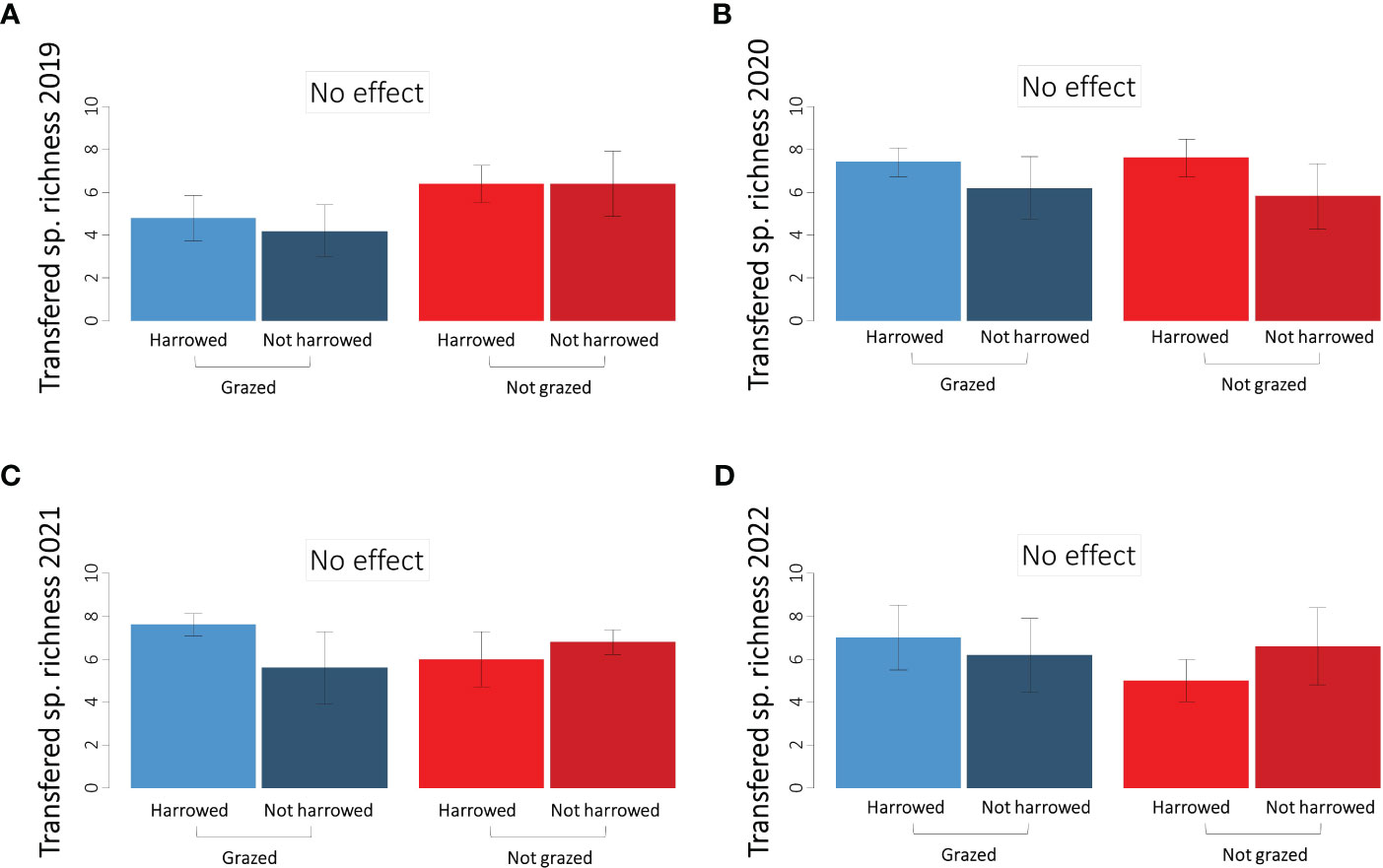
Figure 6 Richness of transferred species in the four sown treatments in (A) 2019, (B) 2020, (C) 2021 and (D) 2022. Error bars represent ±SE.
Discussion
Four years after the transfer of seed-containing plant material to degraded mountain grassland sites in the southern French Alps, transferred species reached cover values of 15 to 35% depending on the restoration treatments (Figure 5D). Seed bed preparation favoured the establishment of these species (35% in the grazed treatment). This positive effect of such soil disturbance had already been found in the first-year seedling counts (Durbecq et al., 2021) but not in first and second-year plant covers. Similarly, initial grazing increased the establishment of the transferred species by the fourth year. The small negative effect of initial grazing on first-year seedling recruitment (Durbecq et al., 2021) was not significant in the longer term and turned into a small positive effect at the end of the study period after the removal of fences. The effects of grazing on total cover and total species richness were not or marginally significant in the end showing that effects of treatments on transferred species were not strong enough to change these whole community parameters. Plant community composition, however, showed a clear development towards the reference community used as a source for seed material. This development was favoured by initial grazing whereas the effect of seed bed preparation was not significant.
The positive effects of harrowing that were observed on transferred species establishment in our restored sites indicated that the potential negative effects of seed bed preparation, such as the elimination of the protective effect of pre-existing vegetation on seedlings (e.g. against UV and drought, Gibson, 2009) were compensated by positive effects. Such positive effects include the destruction of the dense and deep root systems of competitive perennial species not targeted for restoration, reducing interspecific competition (Pywell et al., 2006; Klaus et al., 2017; Bischoff et al., 2018). Recruitment success may also be improved by a better seed adhesion to the soil after seed bed preparation (Chambers, 2000; Isselin-Nondedeu et al., 2006). Moreover, harrowing allows soil aeration increasing nitrogen mineralization (Davies et al., 2001; Steinmann, 2002). Thus, preparing the soil before seed transfer releases nutrients that increase biomass production as a short-term effect of soil tillage that disappears later on (Kristensen et al., 2003; Seabloom et al., 2003). Kiss et al. (2021) also found a beneficial effect of soil disturbance on the cover of transferred species during dry grasslands restoration after five years of revegetation monitoring. Similarly, Schmiede et al. (2012) showed improved establishment of plant species by harrowing. In a previous study on first-year seedling recruitment in our restoration sites, the number of seedlings of spontaneously emerging species was lower in the harrowed plots and the number of seedlings of transferred species and their richness were higher (Durbecq et al., 2021). Although not apparent in first-year cover, the current study confirms the positive effect of harrowing on seedling recruitment on transferred species cover in the long run. Four years after sowing the cover values of transferred species were also higher in harrowed than in non-harrowed plots. Several studies already suggested to prepare the soil before sowing but there are few studies analysing mid- to long-term effects on restoration success. In a recent meta-analysis, Slodowicz et al. (2023) found 187 publications that studied active grassland restoration, but only 13 lasted more than three years. Seed bed preparation has often positive effects on the recruitment of sown species (Edwards et al., 2007; Schmiede et al., 2012; Bischoff et al., 2018) but contrary to our mid-term results, long-term restoration studies found that such initial soil disturbance effects are less visible in the long run (Harvolk-Schöning et al., 2020; Sommer et al., 2023).
In our study, seed bed preparation had no significant effect on species richness. Similarly, Edwards et al. (2007) observed no effect of soil tillage on the restoration of calcareous grasslands monitored over four years. After grassland degradation, a rise of species richness is typically observed in the first years of restoration due to the germination of short-lived species abundant in the seed bank (Freitag et al., 2021, Valkó et al., 2022). However, Freitag et al. (2021) found a decrease in ruderal species richness over years in favour of transferred species. This effect was not observed in our study, since the transferred species richness did not change during the four years monitoring. We thus conclude that most transferred species were already recruited in the first year and just increased in cover.
Total plant cover was not affected by grazing, but our results showed a strong interaction between grazing and seed bed preparation in the beginning. The initially higher total cover in the non-grazed, non-harrowed treatment suggested that both grazing and seed bed preparation contributed to a reduction in competition the first year. This significant interaction disappeared afterwards. In several studies, grazing exclusion failed to increase grassland plant cover in early successional stages but long-term grazing maintained relatively higher diversity or stability (Milotić et al., 2010; Song et al., 2020). In our study, the plant species composition of the whole community developed towards the reference community demonstrating the success of seed transfer four years after sowing. Interestingly, initial grazing had a marginally significant positive effect on this development towards the reference community, whereas the effect of grazing on seedling recruitment of transferred species was slightly negative in a previous study on seedling recruitment (Durbecq et al., 2021).
Although the magnitude of the effect on seedling recruitment was small, our results indicated that mountain grassland communities are finally favoured by grazing even in the beginning and that an initial damage of young seedlings does not translate into a worse establishment of transferred species. Grazing is an important driver in grassland ecosystems. It influences the composition of grassland communities by creating spatial heterogeneity and constantly producing new micro-patterns by plant removal and trampling favourable for the establishment of grassland species (Kladivová and Münzbergová, 2016, Sommer et al., 2023, Martin et al., 2022). Similarly, grazing promotes environmental heterogeneity and biodiversity by redistributing and selectively concentrating nutrients (Austrheim and Eriksson, 2001). Grazing is therefore crucial to maintain the high species richness of grasslands (Dupré and Diekmann, 2001; Pykälä, 2003; Saatkamp et al., 2018). However, it remains unclear whether its intensity, frequency and/or timing drive this effect (Adler et al., 2001).
In our study, the grazing effect was still apparent after four years although grazing was only excluded for four years. Transferred species establishment was higher in the initially grazed treatments, thus explaining the development of the plant community towards the reference. Initial negative effects of grazing due to seedling damage later turned into a positive effect because grazing damages other ruderal species more than typical mountain grassland species of the reference community, thus leading to a selective effect of grazing on species establishment over the years. However, the positive grazing effect was particularly strong in the harrowed plots resulting in a significant grazing-by-harrowing interaction effect on transferred species cover in the fourth year. Freitag et al. (2021) noted that it is still not well known whether grazing interacts with soil disturbance to shape grassland species establishment, but grazing exclusion in the first years may favour transferred species that need protection against solar radiation provided by ruderal species particularly occurring in non-grazed and/or non-harrowed plots. Conversely, the non-harrowed plots may be more attractive for grazing animals than the harrowed plots due to both a higher plant biomass and palatability. In agreement with Freitag et al. (2021), our results suggest that a better understanding of interactions between grazing and seed bed preparation in driving the long-term establishment of transferred species is needed to improve management recommendations in grassland restoration involving seed-addition.
Conclusion
Sowing seeds harvested in a species-rich reference community allowed the restoration of the plant species composition of mountain grasslands towards this targeted reference state. In agreement with Labadessa et al. (2020), we found that even early extensive grazing had a small positive effect on transferred species suggesting that initial grazing exclusion is not necessary in this grassland type of our study region. Seed bed preparation had a positive effect not only on seedling recruitment but also in later stages of plant succession and can thus be strongly recommended for restoration in montane grasslands although negative effects on soil organisms and functioning need to be considered. The strong grazing-by-harrowing interaction showed that a combination of both is the best strategy to restore this grassland type. However, research is still needed to better understand how soil disturbance interacts with grazing management in the long run.
Data availability statement
The original contributions presented in the study are included in the article/Supplementary Material, further inquiries can be directed to the corresponding author/s.
Author contributions
The project was conceived by AB, RJ and EB. Experimental design was set up by AB, AD, RJ and EB. All the authors contribute to floristic surveys. AD, CF, NM and RJ performed data analysis with assistance of the other authors. AD wrote the first draft of the manuscript. All authors contributed and approved the final version.
Funding
Financial supports were provided by ANRT (National Agency of Research and Technology) via a grant CIFRE N°2017/0478, the environmental consultancy ECO-MED, RTE (Electricity Transmission Network) and the project “France Relance” funded by the French government and the European Union.
Acknowledgments
We thank Alexis Versino, Alice Dupré-la-Tour, Charlotte Rohmer, Emile Melloul, Léo Rocher, Lola Gogniat, Margaux d’Ambly and Moritz Hammerl for field assistance. Many thanks to Daniel Pavon for his botanist expertise and Alexandre Cluchier from ECO-MED for supporting the project. We also thank the reviewers for helpful suggestions on the manuscript. The authors declare that they have no conflict of interests.
Conflict of interest
Author AD was employed by the company Ecological consultancy ECO-MED.
The remaining authors declare that the research was conducted in the absence of any commercial or financial relationships that could be construed as a potential conflict of interest.
Publisher’s note
All claims expressed in this article are solely those of the authors and do not necessarily represent those of their affiliated organizations, or those of the publisher, the editors and the reviewers. Any product that may be evaluated in this article, or claim that may be made by its manufacturer, is not guaranteed or endorsed by the publisher.
Supplementary material
The Supplementary Material for this article can be found online at: https://www.frontiersin.org/articles/10.3389/fevo.2023.1152549/full#supplementary-material
References
Adler P., Raff D., Lauenroth W. (2001). The effect of grazing on the spatial heterogeneity of vegetation. Oecologia 128 (4), 465–479. doi: 10.1007/s004420100737
Albert Á.-J., Mudrák O., Jongepierová I., Fajmon K., Frei I., Ševčíková M., et al. (2019). Grassland restoration on ex-arable land by transfer of brush-harvested propagules and green hay. Agric. Ecosyst. Environ. 272, 74–82. doi: 10.1016/j.agee.2018.11.008
Anderson M. J. (2001). A new method for non-parametric multivariate analysis of variance. Austral Ecol. 26, 32–46.
Auestad I., Rydgren K., Austad I. (2016). Near-natural methods promote restoration of species-rich grassland vegetation—Revisiting a road verge trial after 9 years. Restor. Ecol. 24 (3), 381–389. doi: 10.1111/rec.12319
Austrheim G., Eriksson O. (2001). Plant species diversity and grazing in the Scandinavian mountains—Patterns and processes at different spatial scales. Ecography 24 (6), 683–695. doi: 10.1111/j.1600-0587.2001.tb00530.x
Bakker E. S. (2003) Herbivores as mediators of their environment: The impact of large and small species on vegetation dynamics [Phd, S.n.]. Available at: https://library.wur.nl/WebQuery/wurpubs/327304.
Bardgett R. D., Bullock J. M., Lavorel S., Manning P., Schaffner U., Ostle N., et al. (2021). Combatting global grassland degradation. Nat. Rev. Earth Environ. 2 (10). doi: 10.1038/s43017-021-00207-2. Article 10.
Bates D., Mächler M., Bolker B., Walker S. (2015). Fitting linear mixed-effects models Usinglme4. J. Stat. Softw. 67 (1), 1–48. doi: 10.18637/jss.v067.i01
Bengtsson J., Bullock J. M., Egoh B., Everson C., Everson T., O’Connor T., et al. (2019). Grasslands—More important for ecosystem services than you might think. Ecosphere 10 (2), e02582. doi: 10.1002/ecs2.2582
Bischoff A., Hoboy S., Winter N., Warthemann G. (2018). Hay and seed transfer to re-establish rare grassland species and communities: How important are date and soil preparation? Biol. Conserv. 221, 182–189. doi: 10.1016/j.biocon.2018.02.033
Bissels S., Donath T. W., Hölzel N., Otte A. (2006). Effects of different mowing regimes on seedling recruitment in alluvial grasslands. Basic Appl. Ecol. 7 (5), 433–442. doi: 10.1016/j.baae.2005.10.002
Borcard D., Gillet F., Legendre P. (2011). Numerical Ecology with R (New York: Springer-Verlag) 2, 688. doi: 10.1007/978-1-4419-7976-6
Bray J. R., Curtis J. T. (1957). An ordination of the upland forest communities of Southern Wisconsin. Ecol. Monogr. 27 (4), 325–349. doi: 10.2307/1942268
Buisson E., Archibald S., Fidelis A., Suding K. N. (2022). Ancient grasslands guide ambitious goals in grassland restoration. Science 377 (6606), 594–598. doi: 10.1126/science.abo4605
Buisson E., Corcket E., Dutoit T. (2015). Limiting processes for perennial plant reintroduction to restore dry grasslands. Restor. Ecol. 23 (6), 947–954. doi: 10.1111/rec.12255
Buisson E., De Almeida T., Durbecq A., Arruda A. J., Vidaller C., Alignan J.-F., et al. (2021). Key issues in Northwestern Mediterranean dry grassland restoration. Restor. Ecol. 29, e13258. doi: 10.1111/rec.13258
Buisson E., Jaunatre R., Römermann C., Bulot A., Dutoit T. (2018). Species transfer via topsoil translocation: lessons from two large Mediterranean restoration projects. Restor. Ecol. 26, S179–S188. doi: 10.1111/rec.12682
Calaciura B., Spinelli O. (2008). Management of Natura 2000 habitats. 6210 Semi-natural dry grasslands and scrubland facies on calcareous substrates (Festuco-Brometalia) (*important orchid sites). Technical Report 2008 12/24, European Commission. Available at: http://ec.europa.eu/environment/nature/natura2000/management/habitats/pdf/6210_Seminatural_dry_grasslands.pdf.
Chambers J. C. (2000). Seed movements and seedling fates in disturbed sagebrush steppe ecosystems: Implications for restoration. Ecol. Appl. 10 (5), 1400–1413. doi: 10.1890/1051-0761(2000)010[1400:SMASFI]2.0.CO;2
Clark C. J., Poulsen J. R., Levey D. J., Osenberg C. W. (2007). Are plant populations seed limited? A critique and meta-analysis of seed addition experiments. Am. Nat. 170 (1), 128–142. doi: 10.1086/518565
Czerwiński M., Woodcock B. A., Golińska B., Kotowski W. (2018). The effect of tillage management and its interaction with site conditions and plant functional traits on plant species establishment during meadow restoration. Ecol. Eng. 117, 28–37. doi: 10.1016/j.ecoleng.2018.03.017
Davies M., Smith K., Vinten A. (2001). The mineralisation and fate of nitrogen following ploughing of grass and grass-clover swards. Biol. Fertil. Soils 33, 423–434. doi: 10.1007/s003740100348
Dupré C., Diekmann M. (2001). Differences in species richness and life-history traits between grazed and abandoned grasslands in southern Sweden. Ecography 24 (3), 275–286. doi: 10.1111/j.1600-0587.2001.tb00200.x
Durbecq A., d’Ambly M., Buisson E., Jaunatre R., Cluchier A., Bischoff A. (2021). Seedling recruitment in mountain grassland restoration: Effects of soil preparation and grazing. Appl. Veg. Sci. 24 (1), e12564. doi: 10.1111/avsc.12564
Durbecq A., Jaunatre R., Buisson E., Cluchier A., Bischoff A. (2020). Identifying reference communities in ecological restoration: The use of environmental conditions driving vegetation composition. Restor. Ecol. 28 (6), 1445–1453. doi: 10.1111/rec.13232
Durbecq A., Rocher L., Jaunatre R., Dupré la Tour A., Buisson E., Bischoff A. (2022). Mountain grassland restoration using hay and brush material transfer combined with temporary wheat cover. Ecol. Eng. 174, 106447. doi: 10.1016/j.ecoleng.2021.106447
Edwards A. R., Mortimer S. R., Lawson C. S., Westbury D. B., Harris S. J., Woodcock B. A., et al. (2007). Hay strewing, brush harvesting of seed and soil disturbance as tools for the enhancement of botanical diversity in grasslands. Biol. Conserv. 134 (3), 372–382. doi: 10.1016/j.biocon.2006.08.025
Erfanzadeh R., KaMali P., Ghelichnia H., Pétillon J. (2016). Effect of grazing removal on aboveground vegetation and soil seed bank composition in sub-alpine grasslands of northern Iran. Plant Ecol. Diversity 9 (3), 309–320. doi: 10.1080/17550874.2016.1221479
Freitag M., Klaus V. H., Bolliger R., Hamer U., Kleinebecker T., Prati D., et al. (2021). Restoration of plant diversity in permanent grassland by seeding: Assessing the limiting factors along land-use gradients. J. Appl. Ecol. 58 (8), 1681–1692. doi: 10.1111/1365-2664.13883
Halassy M., Singh A. N., Szabó R., Szili-Kovács T., Szitár K., Török K. (2016). The application of a filter-based assembly model to develop best practices for Pannonian sand grassland restoration. J. Appl. Ecol. 53 (3), 765–773. doi: 10.1111/1365-2664.12618
Harvolk-Schöning S., Michalska-Hejduk D., Harnisch M., Otte A., Donath T. W. (2020). Floodplain meadow restoration revisited: Long-term success of large scale application of diaspore transfer with plant material in restoration practice. Biol. Conserv. 241, 108322. doi: 10.1016/j.biocon.2019.108322
Hejcman M., Hejcmanová P., Pavlů V., Beneš J. (2013). Origin and history of grasslands in Central Europe – a review. Grass Forage Sci. 68 (3), 345–363. doi: 10.1111/gfs.12066
Hölzel N., Otte A., Bakker J. P. (2003). Restoration of a species-rich flood meadow by topsoil removal and diaspore transfer with plant material. Appl. Veg. Sci. 6 (2), 131–140. doi: 10.1658/1402-2001(2003)006[0131:ROASFM]2.0.CO;2
Isselin-Nondedeu F., Rey F., Bédécarrats A. (2006). Contributions of vegetation cover and cattle hoof prints towards seed runoff control on ski pistes. Ecol. Eng. 27 (3), 193–201. doi: 10.1016/j.ecoleng.2006.02.006
Kiehl K., Kirmer A., Donath T. W., Rasran L., Hölzel N. (2010). Species introduction in restoration projects – Evaluation of different techniques for the establishment of semi-natural grasslands in Central and Northwestern Europe. Basic Appl. Ecol. 11 (4), 285–299. doi: 10.1016/j.baae.2009.12.004
Kiss R., Deák B., Tóthmérész B., Miglécz T., Tóth K., Török P., et al. (2021). Establishment gaps in species-poor grasslands: Artificial biodiversity hotspots to support the colonization of target species. Restor. Ecol. 29 (S1), e13135. doi: 10.1111/rec.13135
Kiss R., Valkó O., Tóthmérész B., Török P. (2016). “Seed bank research in Central-European grasslands - An overview,” in Seed Banks: Types, Roles and Research (Nova Science Publisher), 1–34.
Kladivová A., Münzbergová Z. (2016). Interacting effects of grazing and habitat conditions on seedling recruitment and establishment. J. Veg. Sci. 27 (4), 834–843. doi: 10.1111/jvs.12395
Klaus V. H., Schäfer D., Kleinebecker T., Fischer M., Prati D., Hölzel N. (2017). Enriching plant diversity in grasslands by large-scale experimental sward disturbance and seed addition along gradients of land-use intensity. J. Plant Ecol. 10 (4), 581–591. doi: 10.1093/jpe/rtw062
Koch E. M., Spiegelberger T., Barrel A., Bassignana M., Curtaz A., Spiegelberger T., et al. (2015). Les semences locales dans la restauration écologique en montagne—Production et utilisation de mélanges pour la préservation (Institut Agricole Régional), 98. Available at: https://hal.inrae.fr/hal-02607421.
Kövendi-Jakó A., Halassy M., Csecserits A., Hülber K., Szitár K., Wrbka T., et al. (2019). Three years of vegetation development worth 30 years of secondary succession in urban-industrial grassland restoration. Appl. Veg. Sci. 22 (1), 138–149. doi: 10.1111/avsc.12410
Kristensen H. L., Debosz K., McCarty G. W. (2003). Short-term effects of tillage on mineralization of nitrogen and carbon in soil. Soil Biology and Biochemistry 35 (7), 979–986.
Kuneš P., Svobodová-Svitavská H., Kolář J., Hajnalová M., Abraham V., Macek M., et al. (2015). The origin of grasslands in the temperate forest zone of east-central Europe: Long-term legacy of climate and human impact. Quat. Sci. Rev. 116, 15–27. doi: 10.1016/j.quascirev.2015.03.014
Labadessa R., Deák B., Valkó O. (2020). No need for grazing exclusion - Sheep grazing supports grassland recovery even from the early successional stages. Tuexenia 40, 429–443. doi: 10.14471/2020.40.014
Lenth R. (2020) R. Lenth, emmeans: estimated marginal means, aka least-squares means. R package version 1.4.5.. Available at: https://CRAN.R-project.org/package=emmeans.
Long Q., Foster B. L., Kindscher K. (2014). Seed and microsite limitations mediate stochastic recruitment in a low-diversity prairie restoration. Plant Ecol. 215 (11), 1287–1298. doi: 10.1007/s11258-014-0387-y
Martin G., Courtial A., Génin A., Ramone H., Dutoit T. (2022). Why grazing and soil matter for dry grassland diversity: New insights from multigroup structural equation modelling of micro-patterns. Front. Ecol. Evol. 10. doi: 10.3389/fevo.2022.879060
Milotić T., Erfanzadeh R., Pétillon J., Maelfait J.-P., Hoffmann M. (2010). Short-term impact of grazing by sheep on vegetation dynamics in a newly created salt-marsh site. Grass Forage Sci. 65, 121–132. doi: 10.1111/j.1365-2494.2009.00725.x
Muller I., Mesléard F., Buisson E. (2014). Effect of topsoil removal and plant material transfer on vegetation development in created Mediterranean meso-xeric grasslands. Appl. Veg. Sci. 17 (2), 246–261. doi: 10.1111/avsc.12059
Oksanen J., Simpson G., Blanchet F., Kindt R., Legendre P., O’Hara R. B., et al. (2022) vegan: Community Ecology Package_. R package version 2.6-2. Available at: https://CRAN.R-project.org/package=vegan.
Poschlod P., Biewer H. (2005). Diaspore and gap availability are limiting species richness in wet meadows. Folia Geobot. 40 (1), 13–34. doi: 10.1007/BF02803041
Programme, U. N. E., Nations, F. and A. O. of the U. (2022) The UN Decade on Ecosystem Restoration, (2021-2030): Flagship Initiatives. Available at: https://wedocs.unep.org/xmlui/handle/20.500.11822/37848.
Pykälä J. (2003). Effects of restoration with cattle grazing on plant species composition and richness of semi-natural grasslands. Biodivers. Conserv. 12 (11), 2211–2226. doi: 10.1023/A:1024558617080
Pywell R. F., Bullock J. M., Hopkins A., Walker K. J., Sparks T. H., Burke M. J. W., et al. (2002). Restoration of species-rich grassland on arable land: Assessing the limiting processes using a multi-site experiment. J. Appl. Ecol. 39 (2), 294–309. doi: 10.1046/j.1365-2664.2002.00718.x
Pywell R. F., Bullock J. M., Tallowin J. B., Walker K. J., Warman E. A., Masters G. (2006). Enhancing diversity of species-poor grasslands: An experimental assessment of multiple constraints: Enhancing diversity of species-poor grasslands. J. Appl. Ecol. 44 (1), 81–94. doi: 10.1111/j.1365-2664.2006.01260.x
R Core Team (2022). R: A language and environment for stria (Vienna, AU: R Foundation for Statistical Computing). Available at: https://www.R-project.org/.statisticalcomputing.
Rother D. C., Jordano P., Rodrigues R. R., Pizo M. A. (2013). Demographic bottlenecks in tropical plant regeneration: A comparative analysis of causal influences. Perspect. Plant Ecol. Evol. Syst. 15 (2), 86–96. doi: 10.1016/j.ppees.2012.12.004
Saatkamp A., Henry F., Dutoit T. (2018). Vegetation and soil seed bank in a 23-year grazing exclusion chronosequence in a Mediterranean dry grassland. Plant Biosyst. 152 (5), 1020–1030. doi: 10.1080/11263504.2017.1407375
Seabloom E. W., Borer E. T., Boucher V. L., Burton R. S., Cottingham K. L., Goldwasser L., et al. (2003). Competition, seed limitation, disturbance, and reestablishment of California native annual forbs. Ecological applications 13 (3), 575–592.
Schmiede R., Otte A., Donath T. W. (2012). Enhancing plant biodiversity in species-poor grassland through plant material transfer – the impact of sward disturbance. Appl. Veg. Sci. 15 (2), 290–298. doi: 10.1111/j.1654-109X.2011.01168.x
Scotton M. (2019). Mountain grassland restoration: Effects of sowing rate, climate and soil on plant density and cover. Sci. Total Environ. 651, 3090–3098. doi: 10.1016/j.scitotenv.2018.10.192
Scotton M., Kirmer A., Krautzer B. (2012). Practical handbook for seed harvest and ecological restoration of species-rich grasslands (Cooperativa Libraria Editrice Università di Padova). Available at: https://agris.fao.org/agris-search/search.do?recordID=US201300010888.
Slodowicz D., Durbecq A., Ladouceur E., Eschen E., Humbert J.-Y., Arlettaz A. (2023). The relative effectiveness of different grassland restoration methods: a meta-analysis. Ecol. Solut. Evid. 4, e12221. doi: 10.1002/2688-8319.12221
Sommer L., Klinger Y. P., Donath T. W., Kleinebecker T., Harvolk-Schöning S. (2023). Long-term success of floodplain meadow restoration on species-poor grassland. Front. Ecol. Evol. 10. doi: 10.3389/fevo.2022.1061484
Song S., Zhu J., Zheng T., Tang Z., Zhang F., Ji C., et al. (2020). Long-term grazing exclusion reduces species diversity but increases community heterogeneity in an alpine grassland. Front. Ecol. Evol. 8. doi: 10.3389/fevo.2020.00066
Steinmann H. H. (2002). Impact of harrowing on the nitrogen dynamics of plants and soil. Soil and Tillage Research 65(1), 53–59. doi: 10.1016/S0167-1987(01)00278-1
Temperton V. M., Buchmann N., Buisson E., Durigan G., Kazmierczak Ł., Perring M. P., et al. (2019). Step back from the forest and step up to the Bonn Challenge: How a broad ecological perspective can promote successful landscape restoration. Restor. Ecol. 27 (4), 705–719. doi: 10.1111/rec.12989
Tischew S., Kirmer A. (2007). Implementation of basic studies in the ecological restoration of surface-mined land. Restor. Ecol. 15 (2), 321–325. doi: 10.1111/j.1526-100X.2007.00217.x
Tölgyesi C., Vadász C., Kun R., Csathó A. I., Bátori Z., Hábenczyus A., et al. (2022). Post-restoration grassland management overrides the effects of restoration methods in propagule-rich landscapes. Ecol. Appl. 32 (1), e02463. doi: 10.1002/eap.2463
Török P., Helm A., Kiehl K., Buisson E., Valkó O. (2018). Beyond the species pool: Modification of species dispersal, establishment, and assembly by habitat restoration. Restor. Ecol. 26 (S2), S65–S72. doi: 10.1111/rec.12825
Török P., Vida E., Deák B., Lengyel S., Tóthmérész B. (2011). Grassland restoration on former croplands in Europe: An assessment of applicability of techniques and costs. Biodivers. Conserv. 20 (11), 2311–2332. doi: 10.1007/s10531-011-9992-4
Turnbull L. A., Crawley M. J., Rees M. (2000). Are plant populations seed-limited? A review of seed sowing experiments. Oikos 88 (2), 225–238. doi: 10.1034/j.1600-0706.2000.880201.x
Valkó O., Rádai Z., Deák B. (2022). Hay transfer is a nature-based and sustainable solution for restoring grassland biodiversity. J. Environ. Manage. 311, 114816. doi: 10.1016/j.jenvman.2022.114816
Veldman J. W., Aleman J. C., Alvarado S. T., Anderson T. M., Archibald S., Bond W.-J., et al. (2019). Comment on “The global tree restoration potential”. Science 366 (6463), eaay7976. doi: 10.1126/science.aay7976
Vidaller C., Dutoit T., Ramone H., Bischoff A. (2019). Factors limiting early establishment of the Mediterranean grassland species Brachypodium retusum at disturbed sites. Basic Appl. Ecol. 37, 10–19. doi: 10.1016/j.baae.2019.04.003
WallisDeVries M. F., Poschlod P., Willems J. H. (2002). Challenges for the conservation of calcareous grasslands in northwestern Europe: Integrating the requirements of flora and fauna. Biol. Conserv. 104 (3), 265–273. doi: 10.1016/S0006-3207(01)00191-4
Keywords: community ecology, exclosures, livestock grazing, near-natural restoration methods, semi-natural grassland, soil disturbance
Citation: Durbecq A, Jaunatre R, Buisson E, Favale C, Maudieu N and Bischoff A (2023) Persisting effects of seed bed preparation and early grazing on plant communities in grassland restoration. Front. Ecol. Evol. 11:1152549. doi: 10.3389/fevo.2023.1152549
Received: 27 January 2023; Accepted: 17 July 2023;
Published: 09 August 2023.
Edited by:
Julien Pétillon, University of Rennes 1, FranceReviewed by:
Reza Erfanzadeh, Tarbiat Modares University, IranCsaba Tölgyesi, University of Szeged, Hungary
Copyright © 2023 Durbecq, Jaunatre, Buisson, Favale, Maudieu and Bischoff. This is an open-access article distributed under the terms of the Creative Commons Attribution License (CC BY). The use, distribution or reproduction in other forums is permitted, provided the original author(s) and the copyright owner(s) are credited and that the original publication in this journal is cited, in accordance with accepted academic practice. No use, distribution or reproduction is permitted which does not comply with these terms.
*Correspondence: Aure Durbecq, YXVyZS5kdXJiZWNxQGdtYWlsLmNvbQ==