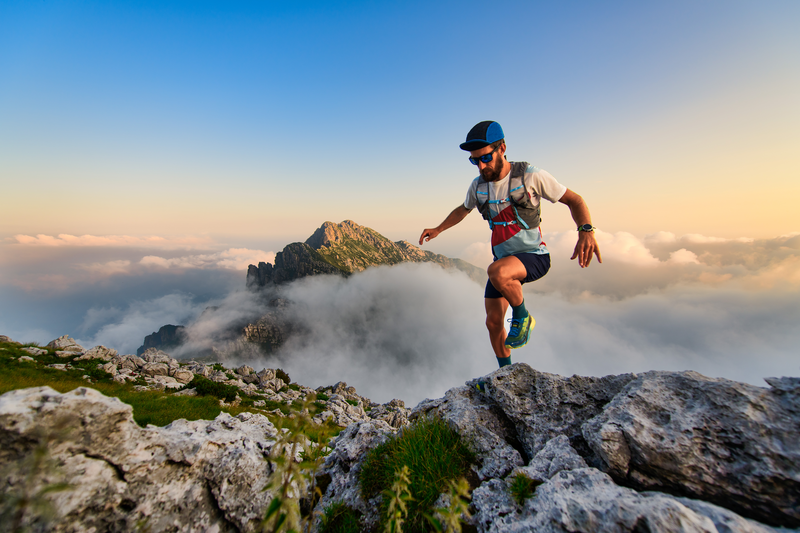
95% of researchers rate our articles as excellent or good
Learn more about the work of our research integrity team to safeguard the quality of each article we publish.
Find out more
ORIGINAL RESEARCH article
Front. Ecol. Evol. , 21 April 2023
Sec. Behavioral and Evolutionary Ecology
Volume 11 - 2023 | https://doi.org/10.3389/fevo.2023.1150736
This article is part of the Research Topic Insect Conservation Behavior View all 11 articles
Wild pollinators are critical to maintaining ecosystem services and facilitating crop production, but habitat degradation and resource loss are leading to worldwide pollinator declines. Nutrient enrichment and changes in rainfall due to global warming are drivers of global environmental change, and likely to impact pollinator foraging behavior and reproductive success through changes to the growth and phenology of flowering plants. Here, we provide a short review of pollinator conservation in the context of nutritional ecology and plant-pollinator interactions. Then, we present novel research into the effects of nutrient and rainfall variation on plant phenology. In this study, we experimentally manipulated the amount of water and supplemental nutrients available to wild sunflower (Helianthus annuus) and goldenrod (Solidago spp.) throughout their growing season. We evaluated how changes in growth and bloom time could impact resource availability for bumble bee (Bombus impatiens) queens preparing to overwinter. We found that fertilizer and rainfall alter plant bloom time by 2–18 days, though flowering response was species-specific. Fertilizer did not significantly affect plant growth or number of flowers produced when plants were grown under drought conditions. When water was not limiting, fertilized sunflowers bloomed in floral pulses. These findings carry important implications for growers and land managers, providing insight into potential drivers of wild pollinator decline and possible conservation strategies.
Wild pollinators are essential to ecosystem function in natural systems and provide critical ecosystem services in agricultural systems (Vanbergen et al., 2013; Goulson et al., 2015). However, insect pollinators are in decline around the world, and their loss could have profound environmental, economic, and social consequences (Vanbergen et al., 2013; Goulson et al., 2015). Though managed honey bees (Apis mellifera Linnaeus) may provide adequate pollination to most crops, a diverse and abundant wild, native insect community can double fruit set even when honey bees account for half of all crop visitation (Garibaldi et al., 2013). Wild pollinators are particularly important to agricultural production as the majority of food crops require pollination to set fruit (Garibaldi et al., 2013). Though native bee communities could provide full pollination services to crops with heavy pollination requirements, the wild bee community on most farms is currently too small to provide sufficient pollination on their own due to lack of semi-natural habitat (Kremen et al., 2002).
Habitat loss and degradation due to climate change and land use intensification are in part responsible for insect pollinator decline (Vanbergen et al., 2013). Habitat alteration can directly impact pollinators or indirectly affect their performance through changes in floral abundance. For example, eutrophication may shift the abundance and timing of flowering in ways that may cause a phenological mismatch between flowering time and pollinator activity (David et al., 2019). Phenological mismatch as a potential driver of pollinator decline is generating increased attention (Ogilvie and Forrest, 2017; Stemkovski et al., 2020). Generalist pollinators rely on an abundant and diverse floral community for food resources from spring through fall in temperate regions (Woodard and Jha, 2017; Leach and Drummond, 2018). Since environmental change may alter plant nutritional chemistry, floral production, and flowering phenology, this may reduce both the quality and quantity of floral resources available to pollinators at critical periods in the foraging season (Ogilvie and Forrest, 2017). Resource loss and nutritional deficiencies leave wild insects more vulnerable to disease and can prohibit reproduction, reducing the pollinator community and pollination services to crops and other flowering plants (Roger et al., 2017). Climate change and agricultural intensification are two major drivers of habitat change that are rapidly altering growing conditions on regional and local scales (Brown et al., 2016; Ogilvie and Forrest, 2017; David et al., 2019; Descamps et al., 2021). Research in the field of nutritional ecology, the study of how an organism interacts with its environment to meet its nutritional needs, could provide a critical link in understanding how landscape-level changes directly impact pollinator health and behavior (Lihoreau et al., 2015; Woodard and Jha, 2017).
Inorganic chemical fertilizer, composed mainly of nitrogen, phosphorus, and potassium, is used widely in agriculture and land management to supplement nutrient-poor soil (Vitousek et al., 1997; Li et al., 2019). Nutrient enrichment from agrochemical runoff and industrial waste, a major driver of habitat change worldwide (David et al., 2019), may affect pollinators in diverse ways. While fertilizer application can result in plants that grow larger, faster, and yield more fruit (Muñoz et al., 2005; Burkle and Irwin, 2009; Li et al., 2019), fertilizer can also alter flower production and bloom time, and nectar and pollen quality (Hoover et al., 2012; David et al., 2019; Russo et al., 2020).
When fertilizer is applied in excess or at the wrong time in the growing season, nutrients can affect non-target plants and habitats through runoff (Shepherd et al., 2018; Russo et al., 2020). The result could be a change in the chemical landscape that reduces floral abundance by promoting nitrogen-limited fast-growth plants and shifting the phenology of those non-target plants (Hunter, 2016; David et al., 2019). Most agricultural fields produce flowers in synchronous pulses that do not offer a continuous supply of flowering plants throughout the growing season and may not offer a full range of essential nutrients (Goulson et al., 2008; Winfree et al., 2008). Wild pollinators must therefore rely on seminatural areas surrounding farms to fill these gaps in plant bloom (Goulson et al., 2008; Winfree et al., 2008).
When fertilizer runs into these adjacent seminatural fields, the timing of bloom may change to potentially widen gaps in resource availability. A change in flowering alters the availability of nectar and pollen to bees and nutrient enrichment can affect the quality of those resources. For example, fertilized Succisa pratensis (Devil’s-Bit) plants, compared against unfertilized plants, produced pollen with a higher total amino acid concentration and altered amino acid profile that was associated with increased larval mortality in bumble bees (Ceulemans et al., 2017).
The effects of chemical fertilizer must be considered in the context of climate change, as environmental conditions alter how plants take up and use soil nutrients (Bassirirad, 2000; Walter, 2018). Though many plants can survive periods of low or high rainfall, extended dry or wet periods can be detrimental to plant growth and to pollinators relying on those plants (Lawson and Rands, 2019; Descamps et al., 2021). Climate change is predicted to bring increasingly long droughts and more frequent extreme precipitation events to many parts of the globe (Trenberth, 2008). Drought-stressed plants tend to bloom earlier, produce fewer flowers, restrict nutrient uptake from soil, and reduce nectar and pollen production (Shavrukov et al., 2017; Walter, 2018; Descamps et al., 2021). While adequate water will increase plant biomass and flower production (Zhang et al., 2020), excessive water that leaves soil saturated for extended periods can inhibit plant growth through reduced soil oxygen, root loss, nitrogen leaching, and limited nutrient uptake by plants (Bedard-Haughn, 2009). As such, fertilizer is likely to affect plants and their pollinators differently when applied under low vs. high rainfall conditions.
Studies in pollinator nutritional ecology examine the distribution and diversity of plants across the landscape and the quality of their floral rewards, which drives foraging behavior, delivery of pollination services, and population stability of both pollinators and plants (Woodard and Jha, 2017). Though insects need food throughout their lives, nutritional deficiencies during certain life cycle stages may have a disproportionate effect on reproductive success and population size. Using wild bees as an example: larvae cannot develop into functional adults without adequate food (Leach and Drummond, 2018); egg-laying females need the protein and fat in pollen to develop and maintain their ovaries (Leach and Drummond, 2018); and diapausing insects have short time frames to build fat body stores ahead of months-long diapause (Hahn and Denlinger, 2011; Woodard and Jha, 2017). Improper nutrition during any of these periods can result in population declines and a breakdown of plant-pollinator networks. In eusocial species like bumble bees (Bombus spp.), which rely on a single queen to survive diapause, initiate nests, and reproduce, the effect of inadequate nutrition on population size and pollination may be magnified.
Bumble bees are among the most important native pollinators for agricultural fields in the US, serving as the primary pollinators of crops like tomatoes, blueberries, and melons, and in some regions providing the majority of crop visitation (Winfree et al., 2008). Several species of bumble bees are in decline in North America and local population distributions are changing, due in large part to habitat loss and disease (Cameron et al., 2011; Carvell et al., 2011). In Europe, bumble bee species declines are closely linked to habitat loss and a narrowing of floral resource diversity and abundance from agricultural intensification (Goulson et al., 2008). As generalist pollinators with months-long flight seasons, bumble bees depend on phenological variation in plant communities to provide diverse floral resources from spring to early fall (Rundlöf et al., 2014; Mallinger et al., 2016; Malfi et al., 2019). The bumble bee colony cycle lasts for approximately 1 year (Alford, 1975; Goulson, 2003). Solitary queens establish nests underground or in thatched grass in the spring and lay eggs that develop into workers. Once workers emerge, queens remain in the nest and no longer forage. During the summer, queens produce workers that forage for food or care for brood before switching from worker production to male and new queen production in the fall. A new queen mates and then diapauses underground through the winter before initiating her own nest in the spring (Alford, 1975; Goulson, 2003).
There are several important nutritional windows for bumble bees, with most research focusing on nest initiation in the spring and gyne production in the fall. Early season resources can dictate a colony’s rate of growth, maximum size, and whether the colony will reach the reproductive switch point (Crone and Williams, 2016; Malfi et al., 2019). Late-season resource availability affects new queen production, and the success of these new queens in preparing for diapause (Rundlöf et al., 2014; Woodard et al., 2019; Timberlake et al., 2020). Bumble bees can store food in their colonies for just a few days, requiring food resources to be available near continuously (Goulson, 2003). Larvae require approximately 8 more days of feeding to develop into queens rather than workers (Cnaani et al., 2002). The feeding period for queen larvae is typically 14–20 days followed by approximately 10 days pupation, while worker feeding period lasts approximately 7–11 days followed by a 10-day pupation (Cnaani et al., 2002). Body size is strongly associated with nutrition during larval development, and small queens are unlikely to survive diapause (Owen, 1988; Couvillon and Dornhaus, 2009). For queens that eclose at appropriate body size and weight, nutrition after eclosion is a critical period as mass gain in the week post-eclosion is a strong predictor of overwintering survival (Woodard et al., 2019; Treanore and Amsalem, 2020). New queens have only 6–7 days within their natal nests after emergence to build the necessary fat and energy stores for 6–9 months of overwintering (Woodard et al., 2019).
If a new queen does not consume enough food during larval development or post-eclosion to build energy stores, she may not survive overwintering or will emerge from diapause too weak to initiate a nest (Woodard et al., 2019; Timberlake et al., 2020; Treanore and Amsalem, 2020). Insufficient food during larval development would result in small queens unable to build fat body stores post-eclosion (Owen, 1988; Couvillon and Dornhaus, 2009). Poor nutrition also leaves her more vulnerable to disease or parasitoids, like the gut parasite Crithidia bombi, that can diminish diapause survival and spring nest initiation (Brown et al., 2003; Schlüns et al., 2010). If fertilizer or rainfall extremes alter the timing or quality of blooms in the late summer or early fall, then colonies may not have the food resources needed to produce queens or for queens to survive diapause (Aldridge et al., 2011). In this study, we examine how agricultural practices and climate change are altering the timing of plant growth and flowering in ways that could reduce resource availability to bumble bees during colony reproduction.
Using the common eastern bumble bee (Bombus impatiens) as a model pollinator, we measured growth in wild sunflower (Helianthus annuus) and goldenrod (Solidago spp.) under different fertilizer and precipitation treatments, and tracked bumble bee forager activity and timing of gyne emergence, to identify phenological mismatch between bloom time and colony reproduction. Wild sunflower and goldenrod are both commonly found on farmland in New England and can bloom into early October (Dr. R. Malfi, Dr. L. Russo, personal communication). These species were selected to represent flowering plants on farms or along field margins that provide important late-season food resources to pollinators and could be target or non-target recipients of chemical fertilizer (Kremen and M’Gonigle, 2015; Russo et al., 2020). This study took place over 2 years and captured the effects of fertilizer as it interacted with rain along a continuum from months-long drought in 2020 to extremely high rainfall in 2021.
Several studies have found that fertilizer and changes in rainfall impact plant phenology. Fertilizer can accelerate flowering or extend a plant’s bloom period (Burkle and Irwin, 2009; Russo et al., 2020), while drought can induce early flowering as a mechanism of drought escape (Nord and Lynch, 2009; Shavrukov et al., 2017; Phillips et al., 2018). Plants grown with sufficient water can increase flower production or bloom early, but excessive water can hinder growth (Bedard-Haughn, 2009; Huang et al., 2018). Plant responses to resource variation are species specific, as one study found that nitrogen addition together with water addition accelerated flowering onset in some species of desert annuals, while delaying onset in others (Huang et al., 2018). However, no studies have examined the combined effects of fertilizer and rainfall on plant phenology through the lens of pollinator nutritional ecology.
Given previous research, we predict that fertilizer and drought will create resource gaps in the late growing season that leave bumble bees at risk for nutritional stress as colonies switch to reproduction (Figure 1). Fertilized plants will bloom earlier than unfertilized plants regardless of water availability, with increased bloom duration and flower production in normal to high rainfall. We predict that bloom duration, flower production and plant growth will decline when plants are grown under both low and very high rainfall conditions. However, fertilizer may replace nutrients leached from soil under high rain, mitigating some of the negative effects of excessive water on growth.
Figure 1. Predicted effect of fertilizer and drought on phenology. We predict that fertilizer and rain treatments will result in an increase in blooming floral resources earlier in the season and a reduction in resources available to reproductive colonies in the fall. Lines are colored by precipitation and represent time from summer to fall.
Fertilizer and drought are two stressors affecting pollinators across biological scales, from changes in individual plant physiology and single colony nutrition that trickle up to affect pollinator foraging selection, delivery of pollination services, and population dynamics (Brown et al., 2016; Walter, 2018; David et al., 2019). Studying these drivers of global change biology as they affect pollinator health and reproduction, may provide important insight into disruptions in critical plant-pollination networks.
Experiments to study the effects of nutrient enrichment and precipitation variation on plant growth took place in 2020 and 2021 at the Boston Area Climate Experiment (BACE) in Waltham, MA (42° 23′ 3″ N, 71° 12′ 52″ W). BACE was established in 2007 as a long-term study site to learn how ecosystems would respond to changes in rainfall due to climate change (Hoeppner and Dukes, 2012). The field site is located on a four-acre organic farm with an active community garden. BACE is a previously managed old field system with three experimental blocks each consisting of three precipitation treatments: 100% ambient rainfall, 75% ambient rainfall, and 50% ambient rainfall. The latter two rain treatments represent drought conditions under average rainfall. Precipitation in each treatment was controlled by clear corrugated polycarbonate slats spaced at regular intervals above experimental plots to allow 75 or 50% rainfall to reach the plots below. The ambient treatment plots were covered with deer fencing to reduce photosynthetically active radiation by about 5% to approximate light interception by the polycarbonate slats in the drought treatments (Hoeppner and Dukes, 2012; Scott et al., 2019).
The study site receives approximately 8–11 cm of precipitation per month, based on the last 30 years of rainfall data in eastern Massachusetts (National Oceanic and Atmospheric Administration [NOAA], 2021). In the northeastern US, climate change is predicted to bring more frequent, prolonged droughts punctuated by heavy rainfall events (Runkle et al., 2017). In 2020, a prolonged drought affected BACE from July to October (Lombard et al., 2020), with 6.8 cm rain in June, 5 cm in July, and 5.8 cm in August (National Oceanic and Atmospheric Administration [NOAA], 2021). In 2021, eastern MA experienced the wettest July on record with 25.4 cm of rain. June 2021 received 6.5 cm of rain, and 17.8 cm of rain in August (National Oceanic and Atmospheric Administration [NOAA], 2021). The result is a precipitation continuum that ranges from extremely dry in the 2020 50% ambient (8.8 cm total rain June-August) rainfall treatment to extremely wet in the 2021 100% ambient rainfall treatment (49.7 cm total rain June-August), allowing us to examine the effects of both precipitation extremes predicted with climate change.
The common eastern bumble bee (B. impatiens) is a frequent forager in New England farm fields and a bumble bee species with one of the longest summer foraging periods (Novotny et al., 2021; Pugesek and Crone, 2021). B. impatiens fly until the end of October in Eastern Massachusetts, producing queens and males from August to October (Pugesek and Crone, 2021). As one of the few bees still active in September and October, growers may rely disproportionately on B. impatiens for fall crop and plant pollination. Though this species is not in decline in Massachusetts, a decline in local populations could create a problem for fall crop pollination.
Sunflower and goldenrod are native to North America, common to farm fields in New England, and flower in the late summer or early fall. Wild sunflowers are annuals and at times planted as crops for seeds, oil, or cut flowers (Kaya et al., 2012). Plants typically bloom from July to October in New England. Goldenrod are perennials and often grow along field margins in undisturbed ground, or are planted in pollinator habitat (Werner et al., 1980). Goldenrod occur in clonal stands, in which stems form at a rhizome node and grow outward from a central area (Werner et al., 1980). Goldenrod bloom from August to October. Both species are considered drought tolerant.
Goldenrod and sunflower provide important nutritional resources for late-season foragers and reproductives in bumble bee colonies. Sunflower pollen is typically low in protein but carries important medicinal properties that can reduce instances of the gut pathogen Crithidia bombi—a pathogen that can reduce queen overwintering survival and nest initiation in the spring (Garibaldi et al., 2013; Adler et al., 2020). Goldenrod supplies an important source of pollen and nectar for bumble bees as new queens are produced in the fall at a time when overall floral abundance is declining (Oertel, 1967; Ziska et al., 2016).
For goldenrod (Solidago spp.), severed rhizomes were obtained from an existing clonal stand at BACE in both 2020 and 2021. To limit transplant shock the apical meristem was removed so that each stem was approximately 50 cm in height.
For wild sunflowers (Helianthus annuus), different seed sources were used for 2020 and 2021. In 2020, goldenrod were transplanted and sunflowers were planted from seed (Silver State seeds, Great Basin Supply) directly into pots in the field on June 12–13th, 2020. Seed germination was low in 2021, requiring us to source plants of three different sunflower varieties. These three varieties included: wild-type H. annuus seeds (American Meadows) planted in basic potting mix (Lambert Professional Growing Media, Germination and Seedlings) in a greenhouse on Tufts University campus; seedlings of the Soraya variety purchased from Russell’s Garden Center in Wayland, MA, USA, and multi-headed wild sunflowers seedlings of unknown heritage sourced from a local grower in Burlington, MA, USA, and grown in basic potting mix. Hereafter, these varieties will be referred to as Wild-Type, Soraya, and S3.
Each sunflower treatment (2 m × 1 m plot) contained 1 Wild-Type, 2–3 S3, and 4 Soraya sunflowers. Sunflower were transplanted on June 24th and goldenrod on June 9th (goldenrod control transplanted June 19th). All plots were watered for the first 2 weeks after transplant as needed, and then watered only by rainwater. In watering control plots, plants were watered when soil moisture was low based on soil moisture measurements and visual inspection. Watering was needed only in June of 2021 as July–September saw record weekly rainfall.
Sunflowers and goldenrod were grown under two fertilization treatments (fertilized and unfertilized) and three precipitation treatments (50, 75, and 100% ambient rainfall) (Supplementary Table 1 and Figure 1). In each of the three precipitation treatments, sunflower and goldenrod were planted in separate 2 m × 2 m (meter) plots, separated by at least 2 m to avoid water pooling between plots, and each plot divided into two 2 m × 1 m sections. Twelve plastic 2-gallon pots were nested below the soil in each 2 m × 1 m section of the plot, with one section randomly assigned to the fertilized treatment (Supplementary Table 1). Plants were arranged in two rows within a treatment, and the orientation of those rows (North-South or East-West) alternated between treatment blocks to control for variation in sunlight. Fertilized plants received 15 mL of controlled-release fertilizer pellets (Osmocote, 14-14-14 NPK) mixed in the top 5 cm of soil on the day of planting. Plants were watered manually in the first 2 weeks after planting, and then watered only by rainfall for the remainder of the growing season.
In 2021, the same planting design was used in the 100, 75, and 50% rainfall plots as in 2020, with 12 plants per goldenrod plot and 8 plants per sunflower plot. An additional manually watered control treatment was added to BACE to ensure at least one treatment with adequate water in the case of drought. Each water control treatment consisted of a 2 m × 1 m plot, divided into two equally sized 1 m × 1 m halves that each contained either 6 pots of goldenrod, or 4 pots of sunflowers.
In 2020, sunflower and goldenrod heights were measured twice per week throughout the month of August, and the number of flowering units within a treatment were counted throughout the bloom period. We recorded an average height of plants for each treatment replicate (2 m × 1 m plot) rather than record height for individual plants. During the month of August, pollen was manually removed from sunflower heads for a separate experiment, and data on flowering phenology were not recorded for sunflowers in 2020.
In 2021, height, flower number, and leaf size were recorded once per week for each sunflower from the time of transplanting through bloom until all flower heads were senesced. The number of leaves and nodes along the stalk were also recorded weekly until the first sunflower blooms appeared in July (Supplementary Table 2). Height and flower number were recorded once per week for each goldenrod plant from the time of transplanting to senescence. The width of 1–3 sunflower heads from each sunflower plant and the length of 1–3 goldenrod inflorescences from each goldenrod plant were recorded once during the growing period (Supplementary Table 2). Volumetric water content was measured weekly from each pot using a soil moisture probe (Campbell Scientific Hydrosense II) (Supplementary Table 3). When plants were in bloom, the number of flowering units in each sunflower or goldenrod treatment replicate was counted three times each week from the time of first bloom until final bloom, to provide more detailed phenology data.
To determine the timing of bumble bee reproductive activity, specifically the duration of queen development and timing of emergence in the field, we surveyed bumble bee visitation to flowers in the neighboring community garden and farm three times per week in 2021 (methods modified from Pollard, 1977). Males are produced before queens, and male emergence is thought to cue the rearing of queen larvae (Goulson, 2003; Belsky et al., 2020). Field observations of males would likely signal the start of queen production within the nest, and the time between male emergence and queen emergence would therefore represent queen larval development plus the 6–7 day preparation to leave the nest (Goulson, 2003; Woodard et al., 2019; Belsky et al., 2020; Treanore and Amsalem, 2020).
We surveyed bumble bee activity three times per week, weather permitting, from July 26th when the first sunflowers opened until October 22nd when the last goldenrod flowers senesced. Surveys took place between 9:00 and 16:00 when temperatures were above 60 degrees F, and never in rainy conditions. In total, we surveyed 4 community garden plots, and 3 locations on the farm. Survey plots were added in the community garden as the growing season progressed and some plants were no longer flowering while others started to bloom. On the farm, we surveyed a strip of pollinator garden in the middle of the farm field, one pollinator garden along the edge of the farm, and a selection of row flowers grown for cut flowers (Supplementary Table 4). For each survey, an observer walked slowly (approximately 20–25 steps/min) once along the perimeter of or within the survey area, depending on size and accessibility, and recorded each bumble bee that was on a flower. Bumble bees were identified to species (Three species largely found in this area from August to October: Bombus impatiens, Bombus griseocollis, and Bombus vagans) and caste (i.e., worker, male, queen), but only B. impatiens data were used for this study (Supplementary Table 4). We chose walking surveys rather than stationary timed surveys because we tested both methods before surveys began and found that walking surveys reduced the likelihood of counting the same individual more than once.
We also surveyed bumble bee visitation in experimental plots within BACE three times per week from the time of the first flower in a treatment replicate until the final flower senesced. Each survey lasted for 5 min, to capture a snapshot of bee visitation. Stationary, timed surveys were used for experimental plots because each plot had fewer flowering units overall than the farm or community garden, and plants were evenly spaced. We were thus unlikely to count the same bee multiple times.
We used generalized linear mixed models (GLMMs) to compare changes in plant growth and flower production as they varied with fertilizer and rain treatments. All statistical analyses were performed in R version 3.6.1. We used the functions glmer() or lmer() from the package “lme4”for all GLMMs (Bates et al., 2015). Separate analyses were performed for goldenrod and sunflower, and each sunflower variety was analyzed both in a single model and separately. Data from 2020 were analyzed separately from 2021 because rain totals varied dramatically (17.6 cm rain from June-August in 2020, 49.7 cm rain from June-August in 2021). Soil moisture content was not measured in 2020 so exact comparisons of rain treatments between years are not possible.
To measure maximum plant height (cm), leaf size (cm), and inflorescence length/width (cm), we used normally distributed GLMMs with fertilizer and rain as fixed effects, and either plot ID, greenhouse, and/or observer as random effects. Whether fertilizer and rain were included as an interactive effect, and which random effect was included, was decided using Akaike Information Criterion (AIC). Models to compare height in 2020 goldenrod included an offset for number of plants because height data were collected as an average value for the entire plot rather than for individual plants. In 2021, sunflower variety was included as a fixed effect when comparing all varieties in a single model. The number of leaves per plant was compared using GLMMS with a Poisson distribution, fertilizer and rain treatment as fixed effects, and greenhouse as a random effect.
To compare the number of flowers produced over the growing period in each treatment, we used either normally distributed or negative binomial GLMs, selecting the distribution that best fit the data using AIC. We summed all flowering unit counts over the full season for each plot and interpreted this value as the number of “flower days,” a value that reflects both how many flowers were produced and how many days these flowers remained open. In this way, a single open flower can be counted multiple times if it remained open and available to pollinators for several days. This resulted in a single flower count for each treatment replicate. For some plant varieties, this count was large enough to fit a Gaussian distribution. Fertilizer and rain variety were included as fixed effects, with observer, greenhouse, or plot ID as random effects. Number of plants in each treatment replicate was included as an offset in all models of flower counts.
We used quantile regression to estimate patterns in the start and end of bloom in goldenrod and sunflowers across treatments, and to estimate timing of bumble bee foraging activity between workers, males and new queens (Cade and Noon, 2003). The onset of bloom or caste observations was estimated at the slope of the 0.2 quantile of flowering unit observations as a function of fertilizer and/or rain, or of the slope of the 0.2 quantile of bumble bee counts (Michielini et al., 2021). The end of bloom or bumble bee observations was estimated at the slope of the 0.8 quantile (Michielini et al., 2021). Predictor variables were compared using marginal hypothesis testing by hand, adding or removing variables from models and using anova.rq() to determine whether the main effects of rain and fertilizer or their interaction had a significant effect on bloom onset. If removing the variable did not result in a significantly worse model, the variable was removed from the analysis. Quantile regression was performed using the rq() function from the package “quantreg” (Koenker et al., 2019).
Phenology analysis of bumble bee activity used only data from farm and community garden surveys because these captured a wide variety of flowering plants and their bee visitors. Experimental plots had low visitation with single plant varieties in small survey areas relative to field surveys and may bias slope coefficients at 0.2 and 0.8 quantiles. The switch to reproduction in bumble bee colonies at this site was identified by the switch from majority workers to majority males in bumble bee counts during surveys. Since male production typically precedes queen production in bumble bee colonies (Goulson, 2003), we used the relative abundance of males to estimate reproductive switchpoint and queen development period.
In 2020, when all plants grew under some level of water deficit, both goldenrod and sunflower height increased with rainfall while fertilizer did not increase plant growth (Figure 2). Rain had a significant effect on height in goldenrod (GLM, χ2 = 7.0, df = 2, p-value = 0.0303) and sunflower (GLM, χ2 = 23.01, df = 2, p-value < 0.0001), but there was no significant difference in height between fertilizer treatments in either species. Goldenrod height increased with rainfall in both fertilized and unfertilized treatments. Fertilized sunflowers grew larger in the 100% (t = 3.319) and 75% (t = −4.601) rain treatments than in the 50% rain treatments (pairwise comparison, Tukey’s adjustment, p-value < 0.0226).
Figure 2. Maximum goldenrod and sunflower height (cm) in 2020 and 2021 from July to August. Rain level along the x-axis displays precipitation treatments (A–C represent 50–100% rainfall in 2020; D–F represent 50–100% rainfall in 2021). Boxplots represent the maximum height reached in each of 3 treatment replicates, measured by average plant height within the plot (n = 12 in goldenrod; n = 7 in sunflower). Averages for sunflower include three sunflower varieties in each plot. Fertilized treatments in green, unfertilized in orange. Rainfall totals for each treatment from June to August of each year from low to high: 8.8 cm (A), 13.2 cm (B), 17.6 cm (C), 24.9 cm (D), 37.3 cm (E), 49.7 cm (F). Each *represents statistical difference (p < 0.05) in average maximum height (cm) between fertilized vs. unfertilized plants.
In 2021, one of the wettest summers on record in Massachusetts, goldenrod and sunflower height generally increased with fertilizer, but plateaued or decreased as rain increased from 50 to 100% ambient rainfall (Figure 2). However, specific growth responses to fertilizer and rainfall varied between species and among sunflower varieties (Figure 3). Fertilizer had a significant effect on goldenrod height (GLM, χ2 = 13.06, df = 1, p = 0.0003) and fertilized goldenrod grew larger than unfertilized in 100% rain (pairwise comparison, Tukey’s adjustment, t = 2.75, p = 0.0174) (Figure 2). There was a significant effect of fertilizer (GLM, χ2 = 51.21, df = 1, p-value < 0.0001), plant variety (GLM, χ2 = 157.02, df = 2, p-value < 0.0001), and the interaction between fertilizer and plant variety (GLM, χ2 = 18.07, df = 2, p-value = 0.0024) on sunflower growth (Figure 3). The S3 sunflower variety showed no significant difference in growth between rain and fertilizer treatments. In the Soraya variety, there was a significant effect of fertilizer but not rainfall on growth (GLM, χ2 10.99, df = 1, p-value = 0.0009), and fertilized plants in 100% (t = 2.76) and 75% (t = 2.42) rain grew larger than unfertilized (pairwise comparison, Tukey’s adjustment, p-value < 0.0328). And in the Wild-Type variety, fertilizer (GLM, χ2 = 8.12, df = 1, p-value = 0.0044) and rain (GLM, χ2 = 7.82, df = 2, p-value = 0.0200) main effects were significant predictors of plant growth but with no interaction.
Figure 3. Average height (cm) of three sunflower varieties in July–October of 2021. Height is averaged over three replicate treatments of each rainfall and fertilizer treatment. Each replicate plot contained 4 Soraya plants, 2–3 S3 plants, and 1–2 Wild-Type plants. Each *represents statistical difference (p < 0.05) in average maximum height (cm) between fertilized vs. unfertilized plants. In all species, there was no significant difference in maximum growth between rain treatments. Error bars represent standard error.
For full summary of plant growth data, refer to Supplementary Table 5.
In 2020 we counted a total of 2,545 flowers on fertilized goldenrod and 2,709 flowers on unfertilized goldenrod. In 2021, we counted 2,453 flowers on fertilized goldenrod and 1,134 flowers on unfertilized goldenrod. Goldenrod produced more flowers with more water in both fertilized and unfertilized treatments, but only when water was limiting in 2020. When water was not limiting (2021), fertilized goldenrod produced more flowers than unfertilized and flower production increased with rainfall (Figure 4). In 2020, we found that rain has a significant effect on goldenrod flower production (negative binomial GLM, χ2 = 8.04, df = 2, p-value = 0.018) while fertilizer did not influence number of flowers. A total of 100% rain treatments produced significantly more flowers (348 ± 65 95% CI) than 50% rain treatment (245 ± 41) (pairwise comparison, Tukey’s adjustment, t = 2.71, p-value = 0.0182). In 2021, fertilizer (negative binomial GLM, χ2 = 41.89, df = 1, p-value < 0.0001) and the interaction between fertilizer and rain (negative binomial GLM, χ2 7.28, df = 2, p-value = 0.0263) had significant effects on goldenrod flower production. Rain alone did not have a significant effect on flower production (negative binomial GLM, χ2 0.422, df = 2, p-value = 0.8096). Fertilized goldenrod produced more flowers in 100% (241 ± 50 fertilized, 130 ± 25 unfertilized) (t = 4.30) and 75% (267 ± 46 fertilized, 126 ± 24 unfertilized) (t = 5.31) rain treatments (pairwise comparison, Tukey’s adjustment, p-value < 0.0001).
Figure 4. Average number of flowering units in goldenrod treatments over time in 2020 (dry year) and 2021 (wet year). Number of flowers averaged over three replicate treatments of each rainfall and fertilizer treatment. Each replicate contained approximately 12 plants. Fertilized treatments in green and unfertilized in orange. Each *represents statistical difference (p < 0.05) in total flowers produced between fertilized vs. unfertilized plants. Letters represent difference in flower production between rainfall treatments within a given year (row). Error bars represent standard error.
In 2021, we counted a total of 2,616 flowers in fertilized sunflowers and 2,765 flowers in unfertilized sunflowers. Sunflowers grown in 50% rain had significantly more “flower days” than those under 75 and 100% rainfall. However, specific responses to fertilizer and rainfall varied between sunflower varieties (Figure 5). Flower production of sunflowers in 2020 was not analyzed due to manipulation of flowering heads for a separate experiment that affected number of flowers per plant. In a model that includes all plant varieties, rainfall and plant variety had significant effects on the number of flowers produced (negative binomial GLM, χ2 = 7.93, 203.55; df = 2, 2; p-value < 0.0190). Each of the three varieties produced more flowers over the growing period in the 50% rain treatment than in the 75 and 100% rain treatments.
Figure 5. Average number of flowering units in three sunflower varieties over time in 2021 (wet year). Number of flowers averaged over three replicate treatments of each rainfall and fertilizer treatment. Each replicate plot contained 4 Soraya plants, 2–3 S3 plants, and 1–2 Wild-Type plants. Fertilized treatments in green and unfertilized in orange. Each *represents statistical difference (p < 0.05) in total flower days (not flowering rate) between fertilized vs. unfertilized plants. Upper case letters (AB) represent differences in total flower days with rain treatments among fertilized plants in a given sunflower variety (row). Lower case letters (ab) represent differences with rain treatment among unfertilized plants in a given variety. Error bars represent standard error.
In analyzing each 2021 variety separately, fertilizer (GLM, χ2 = 9.60, df = 1, p-value = 0.0020) and rain (GLM, χ2 = 16.72, df = 2, p-value = 0.0002) had significant effects on number of flowers produced in S3 sunflowers. S3 sunflowers in 50% rain produced significantly more flowers than those growing in 75% (t = 2.55) and 100% (t = −4.04) rain and unfertilized S3 sunflowers produced significantly more flowers across all rain treatments (pairwise comparison, Tukey’s adjustment, p-value < 0.0289).
In Soraya variety, fertilizer (negative binomial GLM, χ2 = 5.02, df = 1, p-value = 0.0250) and the interaction between fertilizer and rain (negative binomial GLM, χ2 = 7.40, df = 2, p-value = 0.0248) had significant effects on number of flowers produced. Unfertilized Soraya produced more flowers than fertilized in 75% rain (pairwise comparison, Tukey’s adjustment, t = −3.14, p = 0.0017), and fertilized plants in 75% rain produced fewer flowers than fertilized in 100% (t = 3.20) and 50% (t = 2.60) rain (pairwise comparison, Tukey’s adjustment, p-value < 0.0259). There was no significant difference in number of flowers produced between rain treatments in unfertilized plants, but overall unfertilized Soraya produced more flowers than fertilized.
In Wild-Type sunflowers, fertilizer but not rainfall had a significant effect on open flowers (negative binomial GLM, χ2 = 7.33, df = 1, p-value = 0.0068). Unfertilized Wild-Type plants produced fewer flowers than fertilized (pairwise comparison, Tukey’s adjustment, t = 2.20, p-value = 0.0281).
For full summary of plant growth and flower data, refer to Supplementary Table 5.
In both 2020 and 2021, fertilized goldenrod bloomed and senesced earlier than unfertilized goldenrod across all rain treatments (Figure 6). In both years, fertilizer alone had a significant effect on bloom onset and end (quantile regression, marginal hypothesis test, p-value < 0.0306) with no significant interaction between rain and fertilizer. In 2020, goldenrod bloom onset and end occurred 4 days earlier in fertilized plants than unfertilized. Bloom duration was 2 days longer in unfertilized plants. In 2021, bloom began 4 days earlier in fertilized plants, and ended 2 days earlier. Thus, bloom duration was 2 days shorter in unfertilized plants. Fertilized goldenrod bloomed earlier under all rain treatments, and bloomed for a longer period than unfertilized goldenrod in 2021 when water was not limited.
Figure 6. Activity period for B. impatiens and flowering period of four plant varieties influenced by fertilizer and rain treatments. Black lines represent abundance of B. impatiens workers, males and queens. As lab studies predict, queen development within the nest likely occurred during the male activity period. Green and orange lines represent bloom start and end points at 0.2 and 0.8 quantiles in 2021, color by fertilizer treatment and line style by rain treatment. X-axis represents number day of year, and y-axis represents plant species or variety. We observed 2,950 workers, 1,902 males, and 55 queens. Each goldenrod treatment represents flower counts from approximately 36 plants. Each sunflower treatment represents counts from 12 Soraya, 6–9 S3, and 3–5 Wild-Type.
Sunflower bloom phenology data were collected only in 2021 due to a different experiment in 2020 that altered flowering. Therefore, sunflower bloom phenology was collected during the year of record rainfall but not in the drought year. In 2021, the timing bloom onset and end in sunflowers were highly variable among plant variety (Figure 6). In Wild-Type plants, there was no significant difference in date of bloom between different fertilizer and rain treatments, but both the interaction between rain and fertilizer (quantile regression, marginal hypothesis test, p-value = 0.0497) and the main effect of rain (p-value < 0.0001) had significant effects on the end of bloom. In fertilized Wild-Type sunflowers, plants in 75% rain ended bloom approximately 17 days before those in 50 and 100% rain. Among unfertilized Wild-Type sunflowers, plants grown under 50% rain reached the end of bloom approximately 18 days before those grown in 100% rain. Bloom duration in Wild-Type sunflowers ranged from 14 days in fertilized 75% rain to 37 days in unfertilized 50% rain. All Wild-Type plants, regardless of treatment, began to bloom at approximately the same time. However, end of bloom varied by up to 18 days between rain and fertilizer treatments.
In Soraya plants, the interaction of rain and fertilizer had a significant effect on the start and end of bloom (quantile regression, marginal hypothesis test, p-value < 0.0407), but the main effects of fertilizer and rain were not significant predictors of bloom time. Among fertilized Soraya, the dates of bloom and senescence were latest in 100% rain and earliest in 75% rain, by a difference of approximately 7 days. In unfertilized Soraya, the dates of bloom and senescence were earliest in 100% rain and latest in 50% rain, by a difference of approximately 5 days. Bloom duration in Soraya was approximately 2 days longer in fertilized plants. The interaction between fertilizer and rainfall resulted in a non-additive effect on bloom onset and end in Soraya sunflowers, without any pattern that tracks with rain or fertilizer treatment alone.
In the S3 variety, fertilizer had a significant effect on bloom onset (quantile regression, marginal hypothesis test, p-value = 0.0022), while both rain (p-value = 0.0014) and fertilizer (p-value = 0.0006) had significant effects on bloom end, though there was no significant interaction between the two predictors. Fertilized S3 plants bloomed 8 days later than unfertilized S3 plants. Unfertilized S3 senesced 8 days earlier than fertilized, and bloom end occurred approximately 8 days later in 100% rain than in 75 and 50% rain in both fertilized and unfertilized plants. Bloom duration in S3 plants was longest in 100% rain, with bloom lasting approximately 8 days longer in 100% rain in both fertilized and unfertilized treatments than 75 and 50% of ambient rain. On average, bloom onset and bloom end was later in fertilized S3 sunflowers than in unfertilized S3 sunflowers.
In 2021 we spent approximately 68 h surveying bumble bees and observed a total of 2,950 B. impatiens workers, 1902 males B. impatiens, and 55 B. impatiens queens. The observed activity periods for males and queens in the field suggest that there is a period of approximately 16 days, measured by the number of days between the 0.2 quantile slope of male activity and that of queen activity, in which most colonies have reached the point of queen larval development and feeding (Figure 6). If measured by the 0.1 quantile slope of male and queen emergence, queen development occurs over approximately 23 days, which provides an estimate of queen development in this field site that likely encompasses colonies that switched to reproduction early or late. We used the slopes from 0.2 to 0.8 quantiles to estimate the reproductive period with which the majority of colonies coincide.
Observations prior to July 30th were removed from the dataset, along with observations by a single observer on August 20th and 21st due to mistakes in species and/or caste identification. The first 20% of male observations occurred on day 258, just 2 days before 80% of worker observations were recorded, and at which point we estimate most colonies reached the reproductive switch point. The first 20% of queen observations occurred on day 274 and queens were observed through the end of the survey period. These queens were likely developing from larvae to eclosion and then feeding prior to foraging within the nest (Cnaani et al., 2002; Woodard et al., 2019) during the 16-day period in which most males were observed from September 15th (day 258) to October 3rd (day 276) (Supplementary Table 6).
We experimentally manipulated the amount of water and supplemental nutrients available to plants throughout their growing season to understand the effects of these treatments on flowering phenology. We then evaluated how these changes could impact resource availability for B. impatiens queens preparing to overwinter. We found that fertilizer and rain interact to affect the growth and phenology of sunflower and goldenrod, altering bloom time between 2 and 18 days, but that this effect varies between species and varieties. Our original hypothesis was that fertilized plants would grow more and bloom earlier than unfertilized plants because fertilizer would accelerate growth (Burkle and Irwin, 2009; Russo et al., 2020). We expected that under average conditions, plants grown in 50% rainfall would grow smaller and bloom earlier than those grown in 100% rainfall, since water limitation can reduce growth and result in drought escape (Nord and Lynch, 2009; Shavrukov et al., 2017).
However, neither 2020 nor 2021 were average rainfall years: the summer and fall of 2020 brought a prolonged drought, and summer 2021 was one of the wettest summers on record in the Boston area. We found that fertilizer can increase both sunflower and goldenrod growth when water is sufficient, but fertilizer does not affect maximum height or flowers produced when water is limiting. In sunflower, fertilized plants produce most flowers within a narrow window, resulting in a floral resource pulse. In goldenrod, fertilizer results in earlier bloom and senescence regardless of rainfall. Finally, field observations of bumble bees suggest the majority of colonies are simultaneously producing and feeding new queens over an approximately 16-day window. This indicates a short, critical nutritional window in which the most colonies would benefit from available floral resources.
Finding no effect of fertilizer on plant growth under drought conditions somewhat contradicts some studies that find fertilizer to increase plant growth under moderate drought, mitigating the negative effects of drought on plant growth (Garg et al., 2004; Barbosa et al., 2014; Kelso et al., 2020). We may have seen this difference because the total rainfall in 2020 surpassed moderate drought intensity: rainfall totals in July and August of 2020 were 50 and 68% of average rainfall, respectively, leaving the 50% ambient rainfall treatments at just 25 and 34% average rainfall (National Oceanic and Atmospheric Administration [NOAA], 2021). Plants tend to reduce uptake of nutrients during drought (Bista et al., 2018) and as soil dries it becomes primed to leach nutrients during the first significant rewetting (Shepherd et al., 2018). It is possible that in 2020, additional nutrients provided by fertilizer did not affect growth because plants were restricting nutrient uptake to conserve water, and that nutrients were lost from soil during the first significant rainfall. In 2021, growth may have declined in 100% rainfall among unfertilized plants because heavy rainfall leached nutrients from the soil (Bedard-Haughn, 2009) which were supplemented in fertilized treatments, resulting in no growth decline in fertilized plants as water increased. This finding carries important implications for growers and land managers, suggesting that fertilizer should not be applied as insurance against damage from dry conditions (Shepherd et al., 2018).
Flowering phenology was also altered in the experiments. Fertilizer resulted in floral pulses—narrow windows of resource availability (Hemberger and Gratton, 2018)—in sunflower in 2021 but not in goldenrod. Specifically, sunflower blooms were produced all at once rather than produced at an even rate over the bloom period. Comparing the sum of open flowers over time revealed that S3 and Soraya varieties produced significantly more flowers in unfertilized treatments regardless of rainfall. We concluded that fertilizer may have induced accelerated bloom in which plants produce more flowers over a short period, so that most flowers are open and available to pollinators over a shorter window of time than in unfertilized plants. Goldenrod, on the other hand, produced flowers at a similar rate between fertilizer treatments rather than in floral pulses. The number of goldenrod flowers and flower days did not differ significantly between fertilizer treatments in 2020, but was significantly higher in fertilized treatments in 2021. Flowering and flower days increased with rainfall in both study years.
Plants may respond to stress or external stimuli in two ways: flower to produce seed for the next generation, or delay flowering through slowed metabolism (Cho et al., 2017). Sunflowers are considered drought tolerant largely due to drought escape, which results in earlier and more rapid flowering (Hussain et al., 2018). It is possible that these sunflower varieties respond to other stimuli with rapid flowering as well, which could increase the number of flowers produced all at once and decrease the number of flowers produced later in the season. Goldenrod responds to drought and herbivory stress by reallocating resources to asexual reproduction via rhizome maintenance rather than increased or rapid flowering (Shibel and Heard, 2016; Rosenblatt, 2021). This stress response would explain why we observed a similar flowering rate across treatments but higher overall flowers and flower days with more rainfall and, in 2021, fertilizer addition.
Floral pulses that produce abundant food resources can support bumble bee colony growth, but may not increase colony reproduction (Riedinger et al., 2015; Hemberger et al., 2020). Hemberger et al. (2020) found that bumble bee microcolony reproduction was maximized when food rations were high and constantly available, but may be resilient to pulsed food sources if those pulses produce abundant food. Microcolony growth suffered under low abundance regardless of temporal availability. However, this study does not examine queen production, which is more costly than male production (Rundlöf et al., 2014), and colonies can store food for only a few days in the nest (Goulson, 2003) which could result in reduced resiliency to food pulses during queen reproduction. In field studies, bumble bee colony reproduction did not benefit from mass-flowering crops when colony flight periods extended beyond the floral pulse (Riedinger et al., 2015), but queen and male abundance increased when this floral pulse coincided with colony reproduction (Rundlöf et al., 2014).
Bloom time in each plant species and variety grown in this study varied with fertilizer and rain treatments by as much as 18 days. This species-specific response is a common finding among resource manipulation studies that make broad predictions difficult (Tilman and Wedin, 1991; Burkle and Irwin, 2009; Burkle and Runyon, 2016; Cho et al., 2017). Burkle and Irwin (2009) tested the effects of nutrient addition on floral characteristics in two subalpine plant species and found that life-history traits likely played a role in determining the growth response of each plant to soil nutrients. Even within a single species, individual response to resource availability can differ (Burkle et al., 2013; Alvarez-Maldini et al., 2020).
Though there was no single pattern in plant response across species, both fertilizer and rainfall significantly alter the timing and duration of bloom, creating potential phenological mismatch between late-season flowering plants and reproductive bumble bee colonies. Food availability and nectar quality in the fall is one of the strongest predictors of bumble bee populations in the spring (Timberlake et al., 2020). Because the effects of fertilizer and rainfall vary dramatically with plant species, it is essential that habitat surrounding farmland include a diverse community of fall-blooming plants. In this way, low levels of nutrient runoff will increase heterogeneity in bloom time rather than uniformly shift the bloom time of a few species in any direction.
Field observations of bumble bee activity suggest a period of just 16 days between male and queen emergence in which most colonies have reached the reproductive switch point. Though each colony varies in the exact timing of reproduction, these finding suggest that available resources during this short period would support reproduction for the largest number of colonies. Colony reproductive success hinges on a short window with two sensitive nutritional periods: queen larval development, and post-eclosion mass-gain (Owen, 1988; Couvillon and Dornhaus, 2009; Woodard et al., 2019). Food reserves within the nest last only 24–48 h (Goulson, 2003; Rotheray et al., 2017), so food resources outside the nest must be continuously available during reproduction. If food is insufficient during the development period, the colony fails to reproduce or queens do not prepare effectively for winter, leading to lower winter survival and fewer nests in the spring (Goulson, 2003; Fliszkiewicz and Wilkaniec, 2007; Woodard et al., 2019). The changes we observed in plant bloom—most notably the 8-day delay in fertilized S3 sunflower bloom and 4-day acceleration in fertilized goldenrod bloom—may be biologically significant to reproductive bumble bee colonies and warrant further investigation.
Changes in the timing of food availability locally can be overcome if workers fly farther to forage for their queens, or if queens delay diapause to forage after leaving the nest. Queens cannot fly for the first 3–5 days post-eclosion but can delay mating and overwintering by several days to forage for themselves farther from the nest if they have not gained needed energy stores (Watrous et al., 2021). Overwintering survival is optimized when queens enter diapause between 6 and 17 days post-eclosion (Treanore and Amsalem, 2020). However, the sublethal effects of delayed weight gain and the energy expenditure for queens to fly further from the nest to find food are unknown (Watrous et al., 2021). Further, a shift in colony foraging range to overcome local misalignment in bloom time and reproduction could result in changes to local pollination networks. Bumble bees show strong floral constancy (Ogilvie et al., 2017), and this shift in range could persist through the end of the season, resulting in new pollination patterns and potentially fewer visitors to some late-blooming plants.
Nutrient pollution from fertilizer is a major problem and its interactive effect with rain on plant growth may be exacerbated as rainfall frequency and intensity become more variable each year (Vitousek et al., 1997; Lawson and Rands, 2019). Chemical fertilizer has increased farm yield, but at the cost of eutrophication in soils and waterways, and at high economic expense to growers. Methods to make fertilizer use more efficient and reduce its use altogether are therefore important to the sustainability of food production (Watrous et al., 2021). More research is needed into how plant communities, rather than single plant species, respond to fertilizer and rainfall variation. This study does not capture the potential effects of belowground interactions between plants and competition for resources that would also drive changes in bloom in plant communities. Research is also needed to study changes not only in resource availability, but in resource quality measured by pollen and nectar nutritional value.
There is no pinpointing a single cause of pollinator decline, largely because it is the combined effects of stressors that have the largest impact on pollinator foraging, health and population size (Goulson et al., 2015). It is therefore essential that more studies examine how global drivers of change interact to affect potentially sensitive life cycle stages of pollinators through changes to plant growth and physiology. Overall, our study suggests that nutrient enrichment and changes in rainfall affect plant bloom time, and in some plant species this shift falls uniformly along nutrient treatments. Variation in the floral landscape determines pollinator foraging behavior, delivery of pollination services, and gyne production (Cardoza et al., 2012; Mallinger et al., 2016; Ceulemans et al., 2017; Adler et al., 2020; Timberlake et al., 2020). Anthropogenic nutrient deposition and increasingly varied rainfall alter this landscape, threatening the plant-pollinator interactions that support biodiversity and plant productivity (Brown et al., 2016; David et al., 2019). Wild pollinators are critical to successful and reliable crop pollination, especially under an increasingly unpredictable climate (Winfree et al., 2007). Our findings suggest that nutrient addition on managed landscapes without consideration for environmental factors like climate change could further reduce suitable habitat for already threatened pollinators. The results of our study have important implications for agricultural management in a time of increasingly variable climate that can prioritize both crop yield and native pollinator conservation.
The raw data supporting the conclusions of this article will be made available by the authors, without undue reservation.
JT lead author on this manuscript and led the research. CD, MP, and NJ assisted in field work, data collection and entry, and editing. CO helped with project development and manuscript editing. PS advised the project, helped with project development, and manuscript editing. All authors contributed to the article and approved the submitted version.
This work was supported by the National Science Foundation Research Experience for Undergraduates Program (NSF DBI 1852438).
We gratefully acknowledge the assistance of Dr. Elizabeth Crone for help and guidance with statistical analysis, and Heidy Acevedo, Avalon Owens, Isaac Weinberg, and Nicholas Dorian for additional field and data collection support. We thank DI and HH reviewers for the comments on this manuscript. Dr. Lynn Adler of UMass Amherst, Dr. Laura Russo of the University of Tennessee, and Dr. Rosemary Malfi of UMass Amherst consulted on plant selection and study design. The Waltham Fields Community Farm, the University of Massachusetts Boston Area Climate Experiment, and the City of Waltham provided access to study sites.
The authors declare that the research was conducted in the absence of any commercial or financial relationships that could be construed as a potential conflict of interest.
All claims expressed in this article are solely those of the authors and do not necessarily represent those of their affiliated organizations, or those of the publisher, the editors and the reviewers. Any product that may be evaluated in this article, or claim that may be made by its manufacturer, is not guaranteed or endorsed by the publisher.
The Supplementary Material for this article can be found online at: https://www.frontiersin.org/articles/10.3389/fevo.2023.1150736/full#supplementary-material
Adler, L. S., Fowler, A. E., Malfi, R. L., Anderson, P. R., Coppinger, L. M., Deneen, P. M., et al. (2020). Assessing chemical mechanisms underlying the effects of sunflower pollen on a gut pathogen in bumble bees. J. Chem. Ecol. 46, 649–658. doi: 10.1007/s10886-020-01168-4
Aldridge, G., Inouye, D. W., Forrest, J. R. K., Barr, W. A., and Miller-Rushing, A. J. (2011). Emergence of a mid-season period of low floral resources in a montane meadow ecosystem associated with climate change. J. Ecol. 99, 905–913. doi: 10.1111/j.1365-2745.2011.01826.x
Alvarez-Maldini, C., Acevedo, M., Dumroese, R. K., González, M., and Cartes, E. (2020). Intraspecific variation in drought response of three populations of Cryptocarya alba and Persea lingue, two native species from Mediterranean central Chile. Front. Plant Sci. 11:1042. doi: 10.3389/fpls.2020.01042
Barbosa, E. R. M., Tomlinson, K. W., Carvalheiro, L. G., Kirkman, K., de Bie, S., Prins, H. H. T., et al. (2014). Short-term effect of nutrient availability and rainfall distribution on biomass production and leaf nutrient content of savanna tree species. PLoS One 9:e92619. doi: 10.1371/journal.pone.0092619
Bassirirad, H. (2000). Kinetics of nutrient uptake by roots: Responses to global change. New Phytol. 147, 155–169. doi: 10.1046/j.1469-8137.2000.00682.x
Bates, D., Mächler, M., Bolker, B., and Walker, S. (2015). Fitting linear mixed-effects models using lme4. J. Stat. Softw. 67, 1–48. doi: 10.18637/jss.v067.i01
Bedard-Haughn, A. (2009). Managing excess water in Canadian prairie soils: A review. Can. J. Soil Sci. 89, 157–168. doi: 10.4141/CJSS07071
Belsky, J. E., Camp, A. A., and Lehmann, D. M. (2020). The importance of males to bumble bee (Bombus Species) nest development and colony viability. Insects 11:506. doi: 10.3390/insects11080506
Bista, D. R., Heckathorn, S. A., Jayawardena, D. M., Mishra, S., and Boldt, J. K. (2018). Effects of drought on nutrient uptake and the levels of nutrient-uptake proteins in roots of drought-sensitive and -tolerant grasses. Plants 7:28. doi: 10.3390/plants7020028
Brown, M. J. F., Dicks, L. V., Paxton, R. J., Baldock, K. C. R., Barron, A. B., Chauzat, M.-P., et al. (2016). A horizon scan of future threats and opportunities for pollinators and pollination. PeerJ 4:e2249. doi: 10.7717/peerj.2249
Brown, M. J. F., Schmid-Hempel, R., and Schmid-Hempel, P. (2003). Strong context-dependent virulence in a host–parasite system: Reconciling genetic evidence with theory. J. Anim. Ecol. 72, 994–1002. doi: 10.1046/j.1365-2656.2003.00770.x
Burkle, L. A., and Irwin, R. E. (2009). The effects of nutrient addition on floral characters and pollination in two subalpine plants, Ipomopsis aggregata and Linum lewisii. Plant Ecol. 203, 83–98. doi: 10.1007/s11258-008-9512-0
Burkle, L. A., and Runyon, J. B. (2016). Drought and leaf herbivory influence floral volatiles and pollinator attraction. Glob. Chang. Biol. 22, 1644–1654.
Burkle, L. A., Souza, L., Genung, M. A., and Crutsinger, G. M. (2013). Plant genotype, nutrients, and G × E interactions structure floral visitor communities. Ecosphere 4:art113. doi: 10.1890/ES13-00039.1
Cade, B. S., and Noon, B. R. (2003). A gentle introduction to quantile regression for ecologists. Front. Ecol. Environ. 1, 412–420. doi: 10.1890/1540-92952003001[0412:AGITQR]2.0.CO;2
Cameron, S. A., Lozier, J. D., Strange, J. P., Koch, J. B., Cordes, N., Solter, L. F., et al. (2011). Patterns of widespread decline in North American bumble bees. Proc. Natl. Acad. Sci. U.S.A. 108, 662–667. doi: 10.1073/pnas.1014743108
Cardoza, Y. J., Harris, G. K., and Grozinger, C. M. (2012). Effects of soil quality enhancement on pollinator-plant interactions. Psyche 2012:581458. doi: 10.1155/2012/581458
Carvell, C., Osborne, J. L., Bourke, A. F. G., Freeman, S. N., Pywell, R. F., and Heard, M. S. (2011). Bumble bee species’ responses to a targeted conservation measure depend on landscape context and habitat quality. Ecol. Applic. 21, 1760–1771. doi: 10.1890/10-0677.1
Ceulemans, T., Hulsmans, E., Ende, W. V., and Honnay, O. (2017). Nutrient enrichment is associated with altered nectar and pollen chemical composition in Succisa pratensis Moench and increased larval mortality of its pollinator Bombus terrestris L. PLoS One 12:e0175160. doi: 10.1371/journal.pone.0175160
Cho, L.-H., Yoon, J., and An, G. (2017). The control of flowering time by environmental factors. Plant J. 90, 708–719. doi: 10.1111/tpj.13461
Cnaani, J., Schmid-Hempel, R., and Schmidt, J. O. (2002). Colony development, larval development and worker reproduction in Bombus impatiens Cresson. Insectes Sociaux 49, 164–170. doi: 10.1007/s00040-002-8297-8
Couvillon, M. J., and Dornhaus, A. (2009). Location, location, location: Larvae position inside the nest is correlated with adult body size in worker bumble-bees (Bombus impatiens). Proc. R. Soc. B Biol. Sci. 276, 2411–2418.
Crone, E. E., and Williams, N. M. (2016). Bumble bee colony dynamics: Quantifying the importance of land use and floral resources for colony growth and queen production. Ecol. Lett. 19, 460–468. doi: 10.1111/ele.12581
David, T. I., Storkey, J., and Stevens, C. J. (2019). Understanding how changing soil nitrogen affects plant–pollinator interactions. Arthropod Plant Interact. 13, 671–684. doi: 10.1007/s11829-019-09714-y
Descamps, C., Quinet, M., and Jacquemart, A.-L. (2021). The effects of drought on plant–pollinator interactions: What to expect? Environ. Exp. Bot. 182:104297. doi: 10.1016/j.envexpbot.2020.104297
Fliszkiewicz, M., and Wilkaniec, Z. (2007). Fatty acids and amino acids in the fat body of bumblebee Bombus terrestris (L.) in diapausing and non-diapausing queens. J. Apicult. Sci. 51, 55–63.
Garg, B., Burman, U., and Kathju, S. (2004). The influence of phosphorus nutrition on the physiological response of moth bean genotypes to drought. J. Plant Nutr. Soil Sci. 167, 503–508. doi: 10.1002/JPLN.200320368
Garibaldi, L. A., Steffan-Dewenter, I., Winfree, R., Aizen, M. A., Bommarco, R., Cunningham, S. A., et al. (2013). Wild pollinators enhance fruit set of crops regardless of honey bee abundance. Science 339, 1608–1611. doi: 10.1126/science.1230200
Goulson, D., Lye, G. C., and Darvill, B. (2008). Decline and conservation of bumble bees. Annu. Rev. Entomol. 53, 191–208.
Goulson, D., Nicholls, E., Botías, C., and Rotheray, E. L. (2015). Bee declines driven by combined stress from parasites, pesticides, and lack of flowers. Science 347:1255957. doi: 10.1126/science.1255957
Hahn, D. A., and Denlinger, D. L. (2011). Energetics of insect diapause. Annu. Rev. Entomol. 56, 103–121. doi: 10.1146/annurev-ento-112408-085436
Hemberger, J., and Gratton, C. (2018). Floral resource pulse decreases bumble bee foraging trip duration in central Wisconsin agroecosystem. Ecol. Entomol. 43, 447–457. doi: 10.1111/een.12516
Hemberger, J., Frappa, A., Witynski, G., and Gratton, C. (2020). Saved by the pulse? Separating the effects of total and temporal food abundance on the growth and reproduction of bumble bee microcolonies. Basic Appl. Ecol. 45, 1–11. doi: 10.1016/j.baae.2020.04.004
Hoeppner, S. S., and Dukes, J. S. (2012). Interactive responses of old-field plant growth and composition to warming and precipitation. Glob. Chang Biol. 18, 1754–1768. doi: 10.1111/j.1365-2486.2011.02626.x
Hoover, S. E. R., Ladley, J. J., Shchepetkina, A. A., Tisch, M., Gieseg, S. P., and Tylianakis, J. M. (2012). Warming, CO2, and nitrogen deposition interactively affect a plant-pollinator mutualism. Ecol. Lett. 15, 227–234. doi: 10.1111/j.1461-0248.2011.01729.x
Huang, G., Li, C., and Li, Y. (2018). Phenological responses to nitrogen and water addition are linked to plant growth patterns in a desert herbaceous community. Ecol. Evol. 8, 5139–5152. doi: 10.1002/ece3.4001
Hunter, M. (2016). The phytochemical landscape: Linking trophic interactions and nutrient dynamics. Princeton, NJ: Princeton University Press.
Hussain, M., Farooq, S., Hasan, W., Ul-Allah, S., Tanveer, M., Farooq, M., et al. (2018). Drought stress in sunflower: Physiological effects and its management through breeding and agronomic alternatives. Agric. Water Manage. 201, 152–166. doi: 10.1016/j.agwat.2018.01.028
Kaya, Y., Jocic, S., and Miladinovic, D. (2012). “Sunflower,” in Technological innovations in major world oil crops, volume 1: Breeding, ed. S. K. Gupta (Berlin: Springer), 85–129. doi: 10.1007/978-1-4614-0356-2_4
Kelso, M. A., Wigginton, R. D., and Grosholz, E. D. (2020). Nutrients mitigate the impacts of extreme drought on plant invasions. Ecology 101:e02980. doi: 10.1002/ecy.2980
Koenker, R., Portnoy, S., Ng, P. T., Zeileis, A., Grosjean, P., and Ripley, B. D. (2019). Package ‘quantreg. Available online at: https://www.vps.fmvz.usp.br/CRAN/web/packages/quantreg/quantreg.pdf (accessed December 2022).
Kremen, C., and M’Gonigle, L. K. (2015). EDITOR’S CHOICE: Small-scale restoration in intensive agricultural landscapes supports more specialized and less mobile pollinator species. J. Appl. Ecol. 52, 602–610. doi: 10.1111/1365-2664.12418
Kremen, C., Williams, N. M., and Thorp, R. W. (2002). Crop pollination from native bees at risk from agricultural intensification. Proc. Natl. Acad. Sci. U.S.A. 99, 16812–16816. doi: 10.1073/pnas.262413599
Lawson, D. A., and Rands, S. A. (2019). The effects of rainfall on plant–pollinator interactions. Arthropod Plant Interact. 13, 561–569. doi: 10.1007/s11829-019-09686-z
Leach, M. E., and Drummond, F. (2018). A review of native wild bee nutritional health. Int. J. Ecol. 2018:9607246. doi: 10.1155/2018/9607246
Li, Z., Zhang, R., Xia, S., Wang, L., Liu, C., Zhang, R., et al. (2019). Interactions between N, P and K fertilizers affect the environment and the yield and quality of satsumas. Glob. Ecol. Conserv. 19:e00663. doi: 10.1016/j.gecco.2019.e00663
Lihoreau, M., Buhl, J., Charleston, M. A., Sword, G. A., Raubenheimer, D., and Simpson, S. J. (2015). Nutritional ecology beyond the individual: A conceptual framework for integrating nutrition and social interactions. Ecol. Lett. 18, 273–286. doi: 10.1111/ele.12406
Lombard, P. J., Barclay, J. R., and McCarthy, D.-A. E. (2020). 2020 drought in New England. Reston, VA: U.S. Geological Survey, doi: 10.3133/ofr20201148
Malfi, R. L., Crone, E., and Williams, N. (2019). Demographic benefits of early season resources for bumble bee (B. vosnesenskii) colonies. Oecologia 191, 377–388. doi: 10.1007/s00442-019-04472-3
Mallinger, R. E., Gibbs, J., and Gratton, C. (2016). Diverse landscapes have a higher abundance and species richness of spring wild bees by providing complementary floral resources over bees’ foraging periods. Landsc. Ecol. 31, 1523–1535. doi: 10.1007/s10980-015-0332-z
Michielini, J. P., Dopman, E. B., and Crone, E. E. (2021). Changes in flight period predict trends in abundance of Massachusetts butterflies. Ecol. Lett. 24, 249–257. doi: 10.1111/ele.13637
Muñoz, A., Celedon-Neghme, C., Cavieres, L. A., and Arroyo, M. T. K. (2005). Bottom-up effects of nutrient availability on flower production, pollinator visitation, and seed output in a high-Andean shrub. Oecologia 143, 126–135. doi: 10.1007/s00442-004-1780-3
National Oceanic and Atmospheric Administration [NOAA] (2021). NOAA’s national weather service. Climate. NOWData. Available online at: https://www.weather.gov/wrh/Climate?wfo=box (accessed September 27, 2021).
Nord, E. A., and Lynch, J. P. (2009). Plant phenology: A critical controller of soil resource acquisition. J. Exp. Bot. 60, 1927–1937. doi: 10.1093/jxb/erp018
Novotny, J. L., Reeher, P., Varvaro, M., Lybbert, A., Smith, J., Mitchell, R. J., et al. (2021). Bumble bee species distributions and habitat associations in the Midwestern USA, a region of declining diversity. Biodivers. Conserv. 30, 865–887. doi: 10.1007/s10531-021-02121-x
Ogilvie, J. E., and Forrest, J. R. (2017). Interactions between bee foraging and floral resource phenology shape bee populations and communities. Curr. Opin. Insect Sci. 21, 75–82. doi: 10.1016/j.cois.2017.05.015
Ogilvie, J. E., Griffin, S. R., Gezon, Z. J., Inouye, B. D., Underwood, N., Inouye, D. W., et al. (2017). Interannual bumble bee abundance is driven by indirect climate effects on floral resource phenology. Ecol. Lett. 20, 1507–1515. doi: 10.1111/ele.12854
Owen, R. E. (1988). Body size variation and optimal body size of bumble bee queens (Hymenoptera: Apidae). Can. Entomol. 120, 19–27. doi: 10.4039/Ent12019-1
Phillips, B. B., Shaw, R. F., Holland, M. J., Fry, E. L., Bardgett, R. D., Bullock, J. M., et al. (2018). Drought reduces floral resources for pollinators. Glob. Chang. Biol. 24, 3226–3235. doi: 10.1111/gcb.14130
Pollard, E. (1977). A method for assessing changes in the abundance of butterflies. Biol. Conserv. 12, 115–134. doi: 10.1016/0006-3207(77)90065-9
Pugesek, G., and Crone, E. E. (2021). Contrasting effects of land cover on nesting habitat use and reproductive output for bumble bees. Ecosphere 12:e03642. doi: 10.1002/ecs2.3642
Riedinger, V., Mitesser, O., Hovestadt, T., Steffan-Dewenter, I., and Holzschuh, A. (2015). Annual dynamics of wild bee densities: Attractiveness and productivity effects of oilseed rape. Ecology 96, 1351–1360. doi: 10.1890/14-1124.1
Roger, N., Michez, D., Wattiez, R., Sheridan, C., and Vanderplanck, M. (2017). Diet effects on bumblebee health. J. Insect Physiol. 96, 128–133. doi: 10.1016/j.jinsphys.2016.11.002
Rosenblatt, A. E. (2021). Drought rewires an old field food web through shifts in plant nutrient content and herbivore feeding behaviors. Clim. Change Ecol. 2:100019. doi: 10.1016/j.ecochg.2021.100019
Rotheray, E. L., Osborne, J. L., and Goulson, D. (2017). Quantifying the food requirements and effects of food stress on bumble bee colony development. J. Apicult. Res. 56, 288–299. doi: 10.1080/00218839.2017.1307712
Rundlöf, M., Persson, A. S., Smith, H. G., and Bommarco, R. (2014). Late-season mass-flowering red clover increases bumble bee queen and male densities. Biol. Conserv. 172, 138–145. doi: 10.1016/j.biocon.2014.02.027
Runkle, J., Kunkel, K., Frankson, R., Easterling, D., DeGaetano, A. T., Stewart, B., et al. (2017). Massachusetts state climate summary. Washington, DC: NOAA.
Russo, L., Buckley, Y. M., Hamilton, H., Kavanagh, M., and Stout, J. C. (2020). Low concentrations of fertilizer and herbicide alter plant growth and interactions with flower-visiting insects. Agric. Ecosyst. Environ. 304:107141. doi: 10.1016/j.agee.2020.107141
Schlüns, H., Sadd, B. M., Schmid-Hempel, P., and Crozier, R. H. (2010). Infection with the trypanosome Crithidia bombi and expression of immune-related genes in the bumblebee Bombus terrestris. Dev. Comp. Immunol. 34, 705–709. doi: 10.1016/j.dci.2010.02.002
Scott, E. R., Li, X., Kfoury, N., Morimoto, J., Han, W.-Y., Ahmed, S., et al. (2019). Interactive effects of drought severity and simulated herbivory on tea (Camellia sinensis) volatile and non-volatile metabolites. Environ. Exp. Bot. 157, 283–292. doi: 10.1016/j.envexpbot.2018.10.025
Shavrukov, Y., Kurishbayev, A., Jatayev, S., Shvidchenko, V., Zotova, L., Koekemoer, F., et al. (2017). Early flowering as a drought escape mechanism in plants: How can it aid wheat production? Front. Plant Sci. 8:1950. doi: 10.3389/fpls.2017.01950
Shepherd, M., Lucci, G., Vogeler, I., and Balvert, S. (2018). The effect of drought and nitrogen fertiliser addition on nitrate leaching risk from a pasture soil; an assessment from a field experiment and modelling. J. Sci. Food Agric. 98, 3795–3805.
Shibel, Z., and Heard, S. B. (2016). Synergistic and additive effects of drought stress and simulated herbivory on two goldenrods, Solidago altissima and S. gigantea. Botany 94, 635–642. doi: 10.1139/cjb-2016-0060
Stemkovski, M., Pearse, W. D., Griffin, S. R., Pardee, G. L., Gibbs, J., Griswold, T., et al. (2020). Bee phenology is predicted by climatic variation and functional traits. Ecol. Lett. 23, 1589–1598. doi: 10.1111/ele.13583
Tilman, D., and Wedin, D. (1991). Plant traits and resource reduction for five grasses growing on a nitrogen gradient. Ecology 72, 685–700. doi: 10.2307/2937208
Timberlake, T. P., Vaughan, I. P., Baude, M., and Memmott, J. (2020). Bumblebee colony density on farmland is influenced by late-summer nectar supply and garden cover. J. Appl. Ecol. 1, 1006–1016. doi: 10.1111/1365-2664.13826
Treanore, E., and Amsalem, E. (2020). The effect of intrinsic physiological traits on diapause survival and their underlying mechanisms in an annual bee species Bombus impatiens. Conserv. Physiol. 8:coaa103. doi: 10.1093/conphys/coaa103
Trenberth, K. E. (2008). “The impact of climate change and variability on heavy precipitation, floods, and droughts,” in Encyclopedia of hydrological sciences, eds M. G. Anderson and J. J. McDonnell (Hoboken, NJ: John Wiley & Sons, Ltd), hsa211. doi: 10.1002/0470848944.hsa211
Vanbergen, A. J., Baude, M., Biesmeijer, J. C., Britton, N. F., Brown, M. J. F., Bryden, J., et al. (2013). Threats to an ecosystem service: Pressures on pollinators. Front. Ecol. Environ. 11, 251–259. doi: 10.1890/120126
Vitousek, P. M., Aber, J. D., Howarth, R. W., Likens, G. E., Matson, P. A., Schindler, D. W., et al. (1997). Human alteration of the global nitrogen cycle: Sources and consequences. Ecol. Applic. 7, 737–750. doi: 10.1890/1051-07611997007[0737:HAOTGN]2.0.CO;2
Walter, J. (2018). Effects of changes in soil moisture and precipitation patterns on plant-mediated biotic interactions in terrestrial ecosystems. Plant Ecol. 219, 1449–1462. doi: 10.1007/s11258-018-0893-4
Watrous, K. M., Costa, C. P., Diaz, Y. R., and Woodard, S. H. (2021). Flexibility in the Critical Period of Nutrient Sequestration in Bumble Bee Queens. Integr. Organ. Biol. 3:obab009. doi: 10.1093/iob/obab009
Werner, P. A., Gross, R. S., and Bradbury, I. K. (1980). THE BIOLOGY OF CANADIAN WEEDS.: 45. Solidago canadensis L. Can. J. Plant Sci. 60, 1393–1409. doi: 10.4141/cjps80-194
Winfree, R., Williams, N. M., Dushoff, J., and Kremen, C. (2007). Native bees provide insurance against ongoing honey bee losses. Ecol. Lett. 10, 1105–1113. doi: 10.1111/j.1461-0248.2007.01110.x
Winfree, R., Williams, N. M., Gaines, H., Ascher, J. S., and Kremen, C. (2008). Wild bee pollinators provide the majority of crop visitation across land-use gradients in New Jersey and Pennsylvania, USA. J. Appl. Ecol. 45, 793–802. doi: 10.1111/j.1365-2664.2007.01418.x
Woodard, S. H., and Jha, S. (2017). Wild bee nutritional ecology: Predicting pollinator population dynamics, movement, and services from floral resources. Curr. Opin. Insect Sci. 21, 83–90. doi: 10.1016/j.cois.2017.05.011
Woodard, S. H., Duennes, M. A., Watrous, K. M., and Jha, S. (2019). Diet and nutritional status during early adult life have immediate and persistent effects on queen bumble bees. Conserv. Physiol. 7:coz048. doi: 10.1093/conphys/coz048
Zhang, J., Zuo, X., Zhao, X., Ma, J., and Medina-Roldán, E. (2020). Effects of rainfall manipulation and nitrogen addition on plant biomass allocation in a semiarid sandy grassland. Sci. Rep. 10:9026. doi: 10.1038/s41598-020-65922-0
Keywords: pollination, drought, rainfall, nutrient enrichment, agriculture, bumble bees, phenology, global change biology
Citation: Thuma JA, Duff C, Pitera M, Januario N, Orians CM and Starks PT (2023) Nutrient enrichment and rainfall affect plant phenology and floral resource availability for pollinators. Front. Ecol. Evol. 11:1150736. doi: 10.3389/fevo.2023.1150736
Received: 24 January 2023; Accepted: 03 April 2023;
Published: 21 April 2023.
Edited by:
Silvio Erler, Julius Kühn-Institut, GermanyReviewed by:
Harmen P. Hendriksma, Wageningen University & Research, NetherlandsCopyright © 2023 Thuma, Duff, Pitera, Januario, Orians and Starks. This is an open-access article distributed under the terms of the Creative Commons Attribution License (CC BY). The use, distribution or reproduction in other forums is permitted, provided the original author(s) and the copyright owner(s) are credited and that the original publication in this journal is cited, in accordance with accepted academic practice. No use, distribution or reproduction is permitted which does not comply with these terms.
*Correspondence: Jessie A. Thuma, amVzc2llLnRodW1hQHR1ZnRzLmVkdQ==
Disclaimer: All claims expressed in this article are solely those of the authors and do not necessarily represent those of their affiliated organizations, or those of the publisher, the editors and the reviewers. Any product that may be evaluated in this article or claim that may be made by its manufacturer is not guaranteed or endorsed by the publisher.
Research integrity at Frontiers
Learn more about the work of our research integrity team to safeguard the quality of each article we publish.