- 1Department of Biological Sciences, Federal University of Health Sciences, Otukpo, Nigeria
- 2Department of Zoology, Ambrose Alli University, Ekpoma, Nigeria
- 3Department of Animal Biology, Federal University of Technology, Minna, Nigeria
- 4Department of Chemistry, Federal University of Health Sciences, Otukpo, Nigeria
- 5Safety and Security Management Division, Department of Engineering Management Services, Federal Ministry of Works and Housing, Abuja, Nigeria
- 6Department of Highways Construction and Rehabilitation, Federal Ministry of Works and Housing, Abuja, Nigeria
- 7Environment Department, Kenya Industrial Research Institute, Nairobi, Kenya
- 8Department of Environmental Engineering Management, University of Energy and Natural Resources, Sunyani, Ghana
- 9Department of Chemistry, Fourah Bay College, University of Sierra Leone, Freetown, Sierra Leone
- 10Institute for Water Research, Rhodes University, Makhanda, South Africa
Africa harbours about a third of the world’s largest natural resource reserves of mineral such as gold and diamonds. These vast mineral reserves in Africa are essential to the continent's development and modern industrial society. However, these minerals, including gold, are often illegally mined by locals which leads to biodiversity loss and groundwater and surface water contamination. In the present study, we assess the impact of illegal gold mining (i.e., panning) and other anthropogenic activities on the distribution patterns of macroinvertebrate traits in the River Chanchaga, North central Nigeria. Anthropogenic activities including urban development, agricultural activities, household activities and gold mining are impacting the Chanchaga stream sites samples in Nigeria. We selected four sampling stations (i.e., reaches), denoting increasing disturbance order; Station 1 < Station 2 < Station 4 < Station 3. Four macroinvertebrate traits, body size, mode of respiration, mode of locomotion, and body shape, were selected and categorized into 19 attributes. The trait attributes were assigned to taxa using the fuzzy coding method. The relative abundance of traits in the study river showed that very large body size (>40–80 mm) macroinvertebrates dominated Stations 1 and 2 while large body size (>20–40mm) dominated Station 3. The relative abundance of macroinvertebrates possessing an integument for oxygen diffusion dominated Station 4. The RLQ model showed that traits such as medium body size (>10–20 mm), gills as mode of respiration, and streamlined and spherical body shapes were positively associated with Stations 1 and 2. Conversely, small (>5–10 mm) and very large (>40–80 mm) body sizes, spiracle: vegetative respiration using plant stems, climbing mode of locomotion, and sprawling mode of locomotion were positively associated with Stations 3 and 4. The fourth-corner test revealed that macroinvertebrates with very small (<5mm) and medium body sizes (>10–20 mm), spiracles mode of respiration and climbing mode of locomotion were positively associated with at least one of the following physico-chemical variables: 1) electrical conductivity [EC (μS cm−1)], 2) biological oxygen demand [BOD5 (mg l−1)], 3) sulphate (mg l−1), 4) nitrate (mg l−1) and 5) phosphate (mg l−1). These traits were classified as resilient traits to pollution in our study. On the other hand, macroinvertebrates that possess gills and can swim actively were positively associated with dissolved oxygen and were deemed vulnerable to pollution. Based on our findings, we concluded that anthropogenic activities, especially illegal gold mining, alter the distribution patterns of macroinvertebrates traits and, in turn, the ecological balance of the ecosystem. To forestall further damage to the ecological health of the River Chanchaga, the government should regulate the activities of gold miners.
Introduction
Africa harbors about a third of the world’s largest natural resource reserves of gold and diamonds (African Union, 2009; World Bank, 2017; Leuenberger et al, 2021). Mining these minerals, especially gold, significantly affects the continent’s economic development (Obiri-Yeboah et al., 2021). However, illegal gold mining (e.g., mechanized mining and panning) is rampant in most parts of Africa, including Nigeria, leading to severe degradation of the natural environment, including rivers and streams (Merem et al., 2017; Gbedzi et al., 2022). Illegal gold mining activities often lead to tailings (mining wastes) and acid mine drainage that have persistent negative environmental impacts with alarming effects on environmental conditions (Merem et al., 2017; Obiri-Yeboah et al., 2021).
In the last two decades, the economic value of gold has skyrocketed, leading to intense global mining activities (World Gold Council, 2012). Many of these mining activities involve artisanal mining that sometimes requires intense manual labor with zero to minimum involvement of mechanization and minimal geological knowledge (Bester and Groenewald, 2021). Because gold-seeking activities (including mining and panning) are often operated illegally, there is little government oversight of these activities. As a result of the lack of oversight by the government, there is indiscriminate and uncontrolled discharge of effluents containing high levels of acid and heavy metals such as cyanides from mining sites into surface waters such as rivers and streams. Apart from the impact these activities have on water quality and biodiversity loss, the pollution by the illegal gold mining poses health risks to the nearby communities, especially the rural residents who depend directly on raw surface water for their daily livelihood (Duncan, 2020).
In the North Central region of Nigeria, concerns about the effects of anthropogenic activities, like illegal gold mining, on the ecological integrity and aquatic communities (e.g., River Chanchaga) have been raised by previous studies (Edegbene et al., 2015; Keke et al., 2020). The River Chanchaga situated in the Lower Niger-Benue ecoregion of Nigeria is a municipal river housing the Niger State of Nigeria Water Board Authority. The river serves as a source of irrigation, bathing, washing of household cooking utensils, and car washing, as well as industrial activities, which also contribute to the rising anthropogenic activities in the catchment (Edegbene et al., 2015). The River Chanchaga also serves as a source of potable drinking water for the households within the river catchment yet serves as a point source of discharges of domestic wastes including defecation and urination (Edegbene et al., 2019). The River Chanchaga has been exposed to illegal gold mining activities, negatively affecting the water quality and ecological integrity of the river system, which in turn impacts both the local human and invertebrate communities (Edegbene et al., 2019). Despite the importance of the river and the continued water quality degradation from anthropogenic activities, including illegal gold mining, no studies have explicitly investigated the impact of these human activities on ecological integrity and macroinvertebrate communities in the river system. In some reaches of the river, locals engaged in mining activities illegally use small equipment for extracting gold from the river beds and finalize their mining process by using hand pans to separate sediments from gold. The mining activities have redirected the instream flows of some sections of the stations (i.e., reaches) of the river, leading to reduced flow and accumulated sediments in the affected reaches as well leading to a potential increase in heavy metal concentrations. Further, the illegal gold mining activities have also affected the vegetative covers, reduced the usability of the lands for agricultural purposes, and increased soil erosions within the river catchments.
In the past, efforts have been made to assess the ecological risks of point source discharges and other anthropogenic influences, such as mining activities, in a bid to characterize and manage the gradient of pollution in African rivers using aquatic biota (e.g., plankton, macroinvertebrates, and fish species; Zengeya et al., 2013; Arimoro et al., 2014; M’kandawire et al., 2017; Ngole-Jeme and Fantke, 2017; Ghali et al., 2020; Edegbene et al., 2022). Consequently, aquatic invertebrates are one of the most explored aquatic biota to assess the ecological integrity of freshwater ecosystems of riverine systems (Mereta et al., 2013; Fierro et al., 2017). In this regard, analyses and interpreting the differences in the macroinvertebrate community indices, including traits between impacted sites and reference conditions, are used to infer anthropogenic disturbance (Ollis et al., 2006).
The use of macroinvertebrate traits is underpinned by the premise that species respond to anthropogenic stressors including illegal gold mining as a function of their adaptive features (Poff et al., 2006). Thus, the distribution pattern of macroinvertebrate communities in response to anthropogenic stress/pressure results from the differential responses of certain trait combinations along stress gradients (Edegbene et al., 2020a; Edegbene et al, 2021a; Edegbene et al, 2021b; Ntloko et al., 2021). In this regard, the implications of the combined effects of illegal gold mining and other anthropogenic activities on biological communities may involve altering the structural and functional ecology of macroinvertebrate communities. Consequently, sensitive taxa of macroinvertebrates such as Ephemeroptera, Plecoptera, and Trichoptera are expected to disappear, while tolerant ones like Chironomidae and Oligochaeta dominate polluted sites. However, trait assemblage and functional diversity of macroinvertebrates may shift in their distribution pattern in response to increasing potential illegal gold mining activities and other anthropogenic stressors (Pallottini et al., 2017; Desrosiers et al., 2019).
Although the use of traits is increasingly being developed and applied in many parts of the world, including Africa (Edegbene et al., 2020b; Ntloko et al., 2021; Pandey et al., 2022), the combined effects of illegal gold mining and other anthropogenic activities on macroinvertebrate assemblage and their traits have not been explicitly investigated, especially in Africa. This current study focusing on the distribution patterns of macroinvertebrate traits in relation to illegal gold mining and other anthropogenic influences will help to gain insight into how traits may confer taxa resilience to these combined effects.
Based on previous studies conducted in other parts of the world, we have developed several predictions for the impact of anthropogenic activities, like illegal gold mining, on macroinvertebrate traits. In the present study, we predicted that illegal gold mining activities and other anthropogenic influences would pattern the distribution of macroinvertebrate traits along a pollution gradient, with trait combinations such as small body size (>5–10 mm), spiracle respiration, and streamlined body shape (i.e., traits that confer species resilience to anthropogenic disturbance) dominating the highly perturbed stations. Trait combinations such as large body size (>20–40 mm), gills as the mode of respiration, and climbing as the mode of locomotion that are less adapted to depleted oxygen stream sites were assumed to decrease with increasing pollution (with special reference to illegal gold mining activities) at the highly polluted sites. To examine these predictions, we i) determined the relative abundance of macroinvertebrate traits in the stations sampled, ii) explored the patterns of distribution of macroinvertebrate communities and certain traits, iii) determined the responses of individuals with traits to illegal gold mining and other anthropogenic activities by correlating traits with physicochemical variables, and iv) visualized the inter-trait and attribute association across the stations sampled. Inter-trait association is the deployment of more than one trait attribute by taxa to strengthen their survival rate in the face of pollution. For instance, an organism that crawls may not be able to escape pollution immediately but can survive if it possesses a specialized breathing mechanism that will enable it to respire atmospherically. We aim to add this study to the growing pool of knowledge on trait-based biomonitoring globally, especially in the Afrotropical region, where information on trait-based studies is still rare.
Materials and methods
Study area and stations
The River Chanchaga is situated in the southern part of Niger State, between latitudes 8°43′N–9°40′N and longitudes 6°12′E–6°47′E. The river is approximately 215.61 km long, and it receives its source from Mutundaya in Shiroro Local Government Area. It is a municipal river with tributaries such as River Guduko, River Gorax, and River Gbako (Figure 1). Further details about the study area’s hydrology, climate, vegetation, and topography are contained in Edegbene et al. (2019). In the present study area, illegal gold mining activities are the core stressor in the River Chanchaga (Edegbene et al., 2019). Other causes of water quality deterioration in the area include domestic/household, industrial, agricultural, and urban activities.
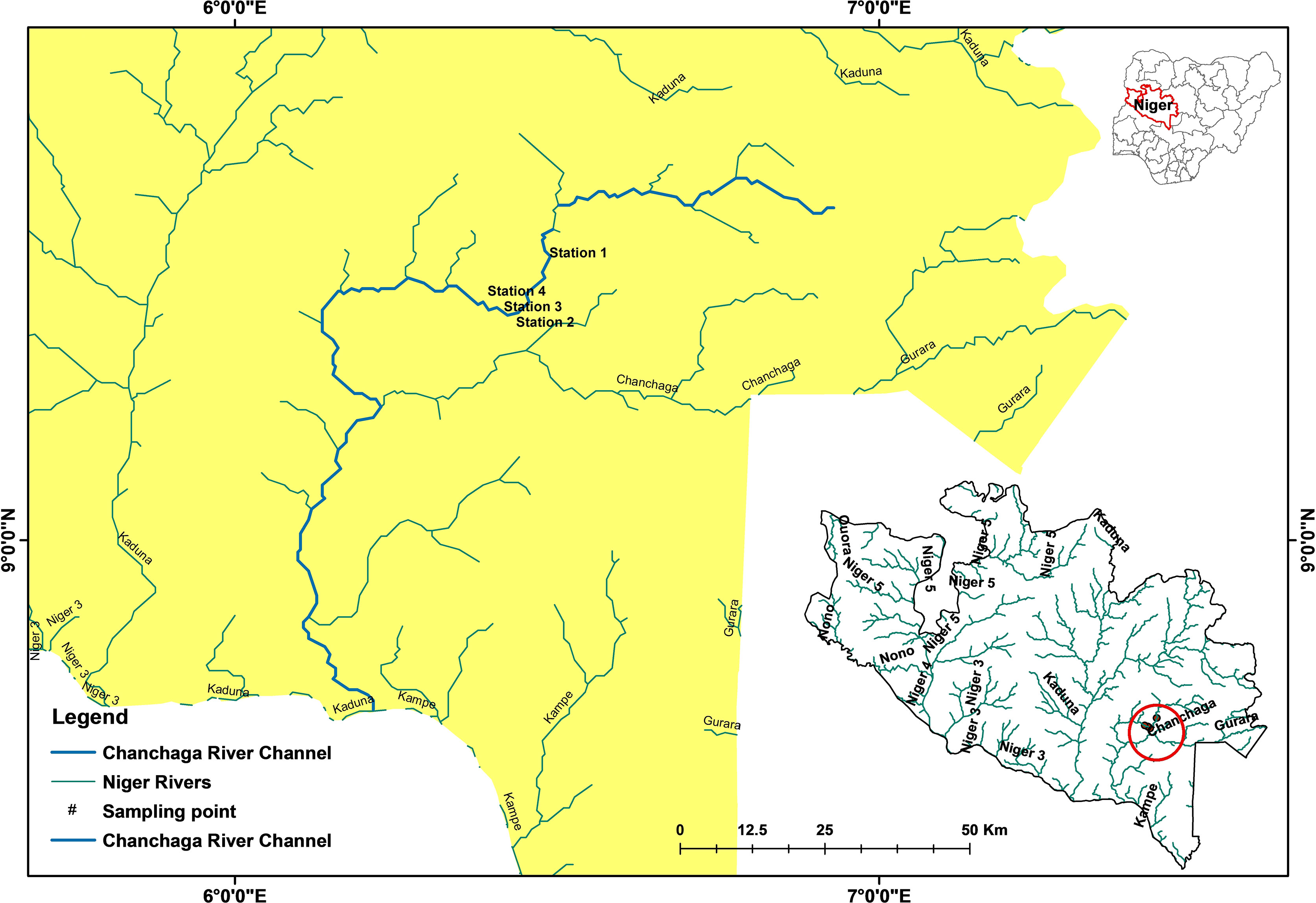
Figure 1 Map of the study area showing the locations of sampling stations on the River Chanchaga. This map has earlier been used in our published paper Edegbene et al. (2023).
We marked out four study stations (i.e., reaches) for the present study (Figure 1) using the degree of anthropogenic influences and accessibility by the community. The first station is relatively unperturbed, with sparsely distributed rural settlements and forest-dominated land use, and is close to the river source (Figure 2). The remaining three stations are subjected to either one or all of the following stressors: illegal gold mining, industrial, agricultural, and urban influences. Stations 3 and 4 are the most perturbed stations, as illegal intensive gold mining activities, agricultural activities, urban settlements, and the localization of industries are expected within the catchments of these stations (Figure 2). Further, the major Niger State Water Board Authority plant is between Stations 3 and 4. The stations represent varying levels of disturbance with Station 1 being the least disturbed and Station 3 being the most disturbed (Station 1: Zhabyala community < Station 2: Tunga Waya community < Station 4: Korokpan community < Station 3: Chanchaga community; Figure 1).
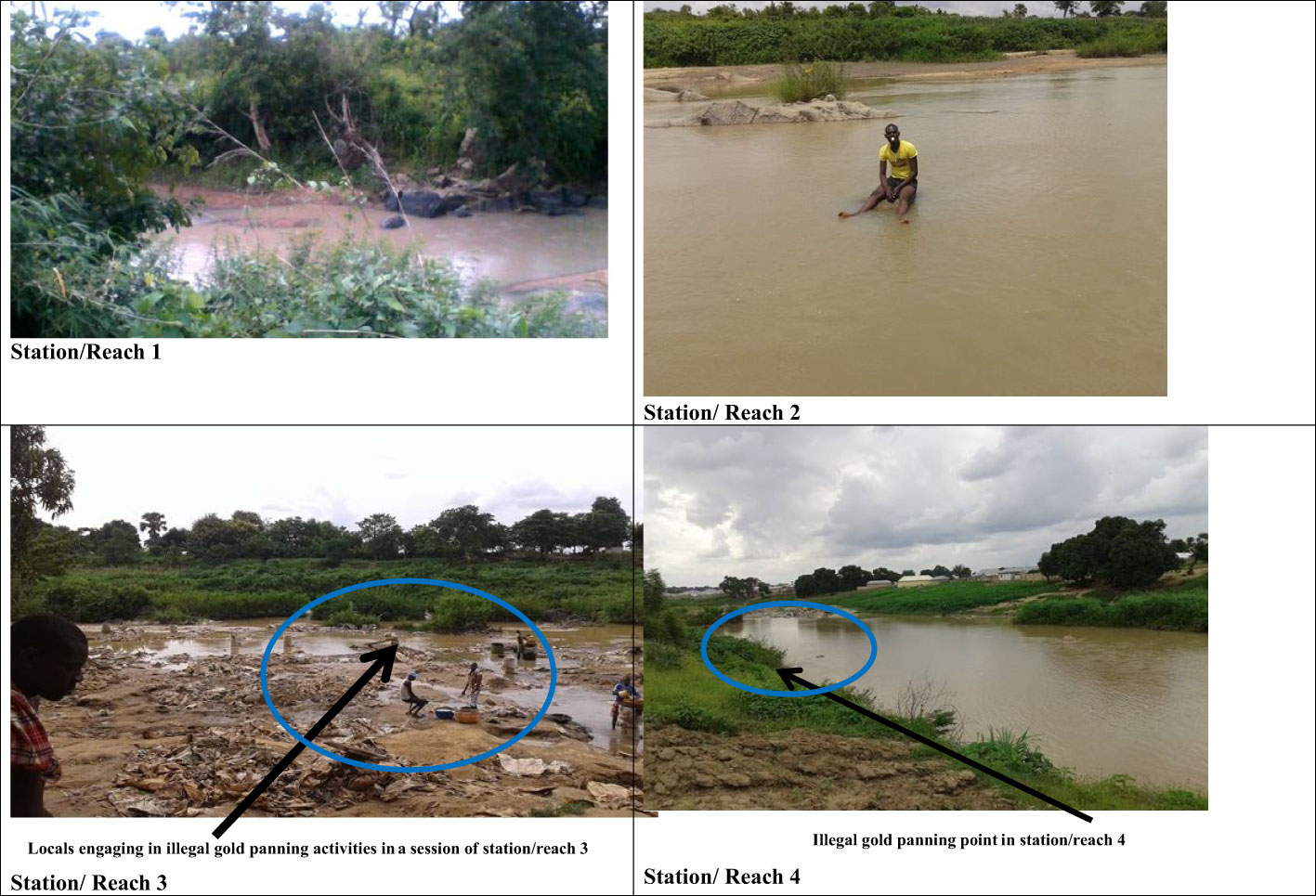
Figure 2 Pictures showing the four sampled stations and the highlights of the level of impact per station in the River Chanchaga, Niger State, Nigeria.
The descriptions of the four stations sampled are as follows. 1) Station 1: the station is relatively unperturbed, with sparely distributed rural settlements and forest-dominated, and close to the river source. The station was marked the control station, as it is relatively unperturbed and hence the reference station in this study. The riparian vegetation is mostly native. Here, the stream flows through a dense canopy cover of bamboo trees (Bambusa sp.). The stream bed is more sand, less mud, boulders, and cobbles. The station is highly sinuous with a large portion of riffle and less of run and pool microhabitats. 2) Station 2: subsistence farming activities are practiced in this station. Farmers here engage in irrigation to grow their crops. The common crops cultivated here include yam, maize, melon, okra, and cowpea. In addition to crop cultivation, cattle grazing, bathing, and washing with detergents are rampant in this station. Illegal gold mining activities were not present at this station. The riparian canopy is sparse with more shrubs and grasses, which results in a year-round open canopy. The station is dominated by a pool microhabitat, while the riffles and runs biotopes are sparse. Due to the level of perturbation, the station is prone to stormwater return flow resulting in erosion. The station streambed consists more of mud and clay, with little sand, silt, and cobbles. 3) Station 3: the station catchment is dominated by residential buildings. Agricultural activities are very high. Local illegal mining activities are carried out in most sections of the station. Further, sand dredging, bathing, defecation, and washing of cars, clothes, and other household items are among the numerous anthropogenic activities occurring at this station. The stream bed consists of sand, boulders, mud, stump of wood, and decaying organic particles. The decayed organic matter is mainly at the littoral zone of the station. The instream cover is relatively poor, rendering the water body open. As we captured in the picture during one of the sampling occasions, locals were carrying out illegal gold mining (Figure 2). 4) Station 4: residential buildings are also present in the station. Subsistence farms also dominate the bank of the station. A very small section of the station is also a site for illegal gold mining by the locals as can be seen in the picture (Figure 2). The stream bed consists of boulders, sand, and mud. The riparian vegetation is mostly mixed with taxa such as Mangifera indica and Nuclea latifolia. The dominant riparian vegetation here is N. latifolia (Rubiaceae).
Physicochemical variables and macroinvertebrate sampling and analyses
The study was conducted for 14 months on four sampling occasions between 2017 and 2018. The first sampling occasion was from March to May 2017, and the second was from September to December 2017. The third sampling was from January to March 2018, and the fourth sampling occasion was from May to August 2018.
A total of 12 physicochemical variables were selected in the course of this study: 1) air temperature (°C), 2) water temperature (°C), 3) pH, 4) electrical conductivity [EC (μS/cm)], 5) flow velocity (m/s), 6) water depth (m), 7) alkalinity (mg/l), 8) dissolved oxygen [DO (mg/l)], 9) biological oxygen demand [BOD5 (mg/l)], 10) sulfate (mg/l), 11) nitrate (mg/l), and 12) phosphate (mg/l). Water and air temperatures were measured using a mercury-in-glass thermometer, and a multiprobe meter (HANNA HI 9828) was used to measure DO, EC, and pH. Flow velocity was measured in three replicates by timing a float as it moved over a distance of 10 m with a stopwatch (Gordon et al., 1994). Water depth was measured with a measuring rod. Water samples were collected in 1-l sample bottles and analyzed for (BOD5), alkalinity, nitrate, sulfate, and phosphate according to APHA (1998) methods. Analysis of all samples commenced within 24 hours of sampling.
The collection of macroinvertebrate samples was performed by kicking all represented biotopes per station, which include sand, gravel, silt, clay, and flow regimes for a period of 3 minutes per biotope using an 800-μm mesh-sized squared-frame net of 14.63 ft2 feet (1.36 m2) along an approximately 25-m-long wadeable stretch of the river. Macroinvertebrates were sorted in the field immediately after sampling. Macroinvertebrates were then collected with forceps and placed in sample bottles containing 70% alcohol for further identification and reference at the laboratory. Macroinvertebrates were identified using keys described by Cranston (2000) and Gerber and Gabriel (2002), after which an abundance count was undertaken.
Selected traits
Four traits associated with physiology, morphology, and behavior were carefully selected in this study: body size, respiration, locomotion, and body shape traits. Respiration traits are related to physiology, while body size and shape are related to morphology. The mode of locomotion is related to behavior. The selected traits were based on their link to anthropogenic pressures such as sedimentation, stormwater return flow, and organic pollution. These pressures are outcomes of industrial, agricultural, urban, and mining activities (Akamagwuna et al., 2019; Edegbene et al., 2021a; Matomela et al., 2021; Ge et al., 2022; Pandey et al., 2022). For instance, body size has been linked with organic pollution, and body shape with stormwater return flow, while body shape and respiration are linked to sedimentation (Ding et al., 2017; Desrosiers et al., 2019; Edegbene et al., 2021a). The selected traits and their linkages with the mentioned stressors had also earlier been used in previous studies to assess the effect of agriculture, urban, and industrial disturbances on the ecological health of aquatic ecosystems (e.g., Chevene and Dolédec, 1994; Akamagwuna et al., 2019; Edegbene et al., 2020a; Akamagwuna et al., 2021; Edegbene et al., 2021b; Ntloko et al., 2021; Edegbene and Akamagwuna, 2022; Ge et al., 2022; Pandey et al., 2022). We categorized the four trait classes into 19 trait attributes, attribute codes, and predicted responses to potential disturbances with a special focus on their responses to illegal gold mining activities (Table 1). We selected five body size trait attributes: 1) very small, 2) small, 3) medium, 4) large, and 5) very large (Table 1). We selected four respiration trait attributes: 1) biological gills, 2) integument, 3) cuticular respiration (cutaneous), and 4) spiracle respiration (spiracle: use of siphon and spiracle: vegetative). In the following paragraph, we highlighted some breathing (respiration) mechanisms exhibited by aquatic insects.
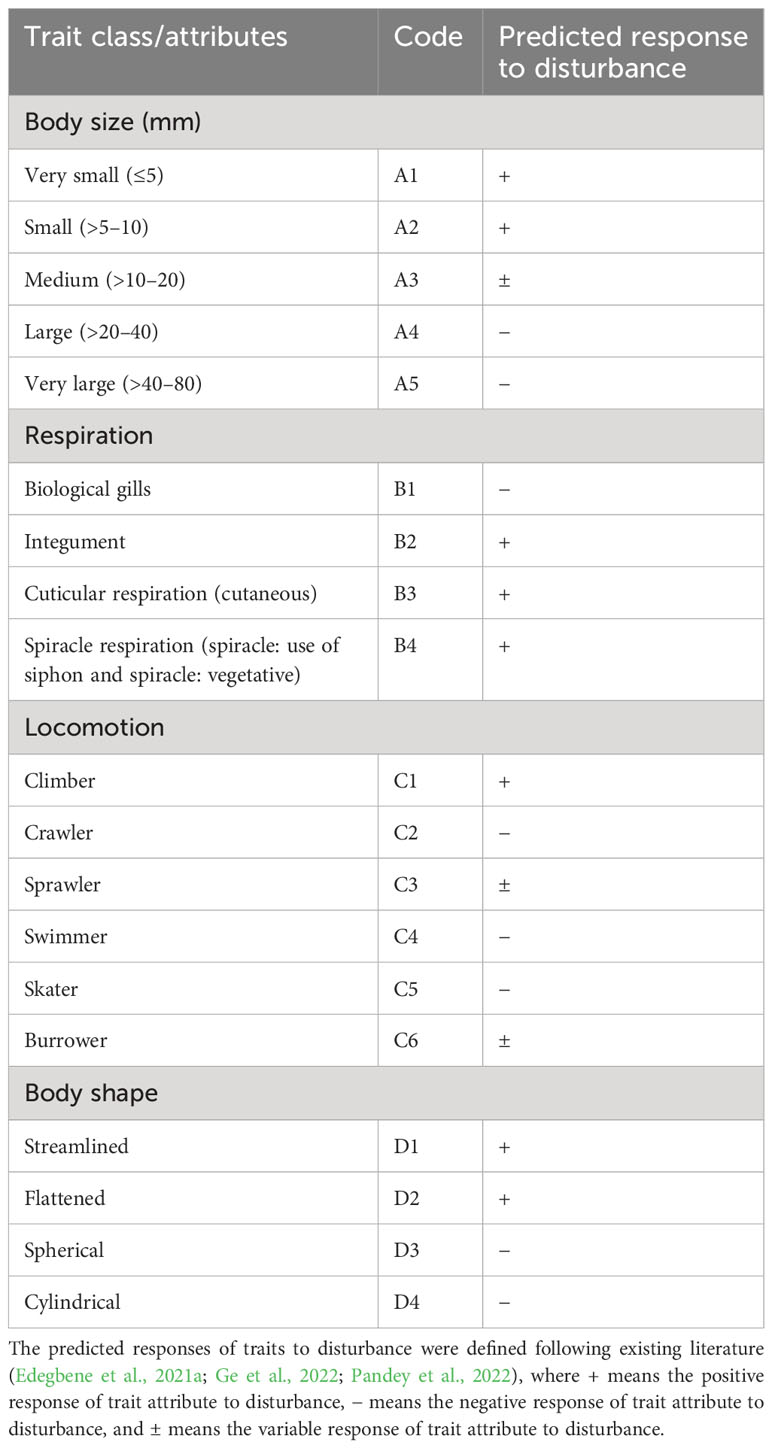
Table 1 Macroinvertebrate selected trait classes and trait attributes (with code) used in the current study.
Biological gill respiration entails mainly the use of two processes in breathing: plastron and bubble (Balmert et al., 2011). Plastron is a series of hairlike bumps on the surface of the gill of an aquatic insect body surface, which traps oxygen against the body of insects (Watson et al., 2017). Some taxa in order Gerridae are examples of insects that make use of plastron for trapping oxygen from the aquatic environment. In addition to the possession of plastron, some insect taxa also form oxygen bubbles, which they carry under the water in a bid to maintain their oxygen balance in the face of depleting concentrations of dissolved oxygen in a water system (Hershey et al., 2010). By carrying an oxygen bubble, some Belostomatidae can dive and remain underwater in a range of water qualities. Cuticular respiration (integument/cutaneous) is a passive form of respiration used by some smaller-bodied insect larvae (Buck, 1962). Examples are some taxa in the order Diptera (e.g., Chironomidae, Culicidae, and some other midges). In general, Chironomidae larvae respire through cuticular absorption, but some have red blood cells that enable them to remain in very low dissolved oxygen waters by increasing blood oxygen concentration with red blood cells. Oxygen enters the trachea of insects either through the use of an oxygen bubble (e.g., Belostomatidae) or through a spiracle that functions like a snorkel. The spiracle absorbs oxygen either directly from the atmosphere as the insect hangs at the water surface (e.g., Culicidae) or by puncturing an underwater plant stem and using a spiracle to absorb oxygen through the plant aerenchyma (e.g., Elmidae).
Fuzzy coding of macroinvertebrate trait attributes
We used the fuzzy coding system to describe the link between a taxon and each trait attribute. The system uses affinity scores that account for functional differences between members of the same family and different life stages within a taxon (Chevene and Dolédec, 1994; Edegbene et al., 2021a). Hence, in the present study, fuzzy coding was performed at the macroinvertebrate family level (see Table S1). The fuzzy affinity scores of 0–5 were used in the current study, and fuzzy scores of 0–5 have been said to account for potential variability that may exist among genus/species with a given family, as the fuzzy codes (scores) were applied at the family level of the macroinvertebrates sampled (Chevene and Dolédec, 1994; Edegbene et al., 2021a). Therefore, scores 0, 1, 3, and 5 denote no, low, moderate, and high affinities, respectively (Chevene and Dolédec, 1994).
Data analyses
Visualizing the distribution patterns of trait-based community responses to anthropogenic influences along the four sampled stations
To analyze the distribution patterns of the responses of trait communities to stressors at the four sampled stations over the study period, we pooled macroinvertebrate taxa at each station together, resulting in taxon–station information for the entire study period. We created a table that contains trait–taxon with trait attributes coded for each taxon (Table S1). Then, we multiplied the trait–taxon matrix by the abundance of each macroinvertebrate family per season for the entire study period containing trait–station information as described in Tomanova et al. (2008) and recently used by Edegbene et al. (2021b). The abundance of each trait attribute within each station was then transformed into relative abundance (Tomanova et al., 2008).
Our preliminary analyses visualized the distribution patterns of trait-based community responses to anthropogenic influences among the four sampled stations using bar charts. The bar charts were plotted using the trait attributes relative abundance transformation.
Responses of macroinvertebrate trait attributes to anthropogenic influences: identifying and classifying vulnerable and resilient traits
We further confirmed the distribution patterns and responses of selected trait attributes to anthropogenic influences by employing multivariate approaches and procedures known as RLQ and fourth-corner analyses developed by Dolédec et al. (1996). RLQ is an ordination procedure with multiple tests embedded in one single analysis, and it performs ordination analyses on three matrices, namely, R (physicochemical variables), L (macroinvertebrate taxon abundance), and Q (trait attributes of macroinvertebrates). Further, we tested the significance level of the first two RLQ axes using the Monte Carlo test at 999 permutations. In determining the relationship between each trait attribute and the analyzed physicochemical variables in the current study, we used the fourth-corner test to visualize whether a given trait attribute correlates positively or negatively to single or multiple physicochemical variables (Brown et al., 2014). We performed RLQ and fourth-corner tests using version 2.5.4 of the ade4 package within the R programming language (Dray and Dufour, 2007; Oksanen et al., 2019; R Core Team, 2020).
In classifying macroinvertebrate traits based on their vulnerability and resilience to anthropogenic influences, we followed the recent thinking of Edegbene et al. (2021a) and Edegbene et al. (2021b). For instance, we classified traits that associated positively with Stations 3 and 4 on the RLQ ordination and correlated negatively with increased DO or correlated positively with any three of EC, BOD5, and nutrients on the fourth-corner test as resilient traits to anthropogenic influences (e.g., illegal gold mining). However, we classified traits that associated positively with Station 1 on the RLQ ordination and correlated negatively with increased DO or positively correlated with any three of EC, BOD5, and nutrients or correlated positively with increased DO on the fourth-corner test as vulnerable traits to anthropogenic influences (e.g., illegal gold mining).
Visualizing macroinvertebrate inter-trait and attribute association across the sampled stations
Finally, cluster analysis based on the Bray–Curtis similarity was performed to elucidate the pattern of inter-trait and attribute association among the taxa of macroinvertebrates. Box plots were used to visualize the variation of the relative abundances of macroinvertebrates in each identified trait cluster across the four sampled stations. Cluster analysis was selected to elucidate inter-trait and attribute association because it is a multidimensional representation (Poff et al., 2006; Edegbene et al., 2021a , Edegbene et al., 2021b). The Kruskal–Wallis multiple comparison tests were also used to ascertain whether the relative abundances of macroinvertebrates in each trait cluster showed whether trait combinations differed significantly among the sampling stations. Cluster analysis was undertaken using the paleontological statistical software (PAST; Hammer et al., 2001), while the box plot was plotted using Statistica version 12 (Tibco Software, 2020).
Predictive performance of the variation in the taxa and trait responses to physicochemical variables among the four stations sampled
In order to determine the relationships among taxa, traits, and physicochemical variables, we performed a linear regression by employing two model approaches: taxa * environmental (physicochemical variables) and traits * environmental (physicochemical variables). Variation was computed as a measure of predictive performance based on the ratio of deviance (R2) at 95% confidence level (Hui et al., 2013; Brown et al., 2014). This model was constructed in PAST (Hammer et al., 2001).
Results
Visualizing the distribution patterns of trait-based community responses to anthropogenic influences along the four sampled stations
The present study recorded 36 macroinvertebrate taxa (families) within eight orders/classes. Diptera was the most represented family with eight taxa, followed by Hemiptera (seven taxa). Odonata was represented by five taxa, while Coleoptera, Ephemeroptera, and Trichoptera were represented by four taxa each. Three and one taxa represented Oligochaeta and Mollusca, respectively (Table S1). We assigned 19 trait attributes to the 36 macroinvertebrate taxa (families) across the four stations sampled (Table S1).
The patterns of distribution of traits and attributes in the sampled stations are presented in Figure 3. The relative abundance of macroinvertebrates that have very large body sizes (>40–80 mm) was higher in Stations 1 and 2, and that of large body sizes (>20–40 mm) dominated Station 3 (Figure 3A). Station 4 was dominated by macroinvertebrates with small body sizes (>5–10 mm), as they had the highest macroinvertebrate relative abundance (Figure 3A). The relative abundance of macroinvertebrates that have very large body sizes was the lowest in Station 4 (Figure 3A). The majority of the body size traits showed a marked distribution pattern among the four sampled stations. For instance, very large body size decreases markedly from Stations 1 to 4 (Figure 3A).
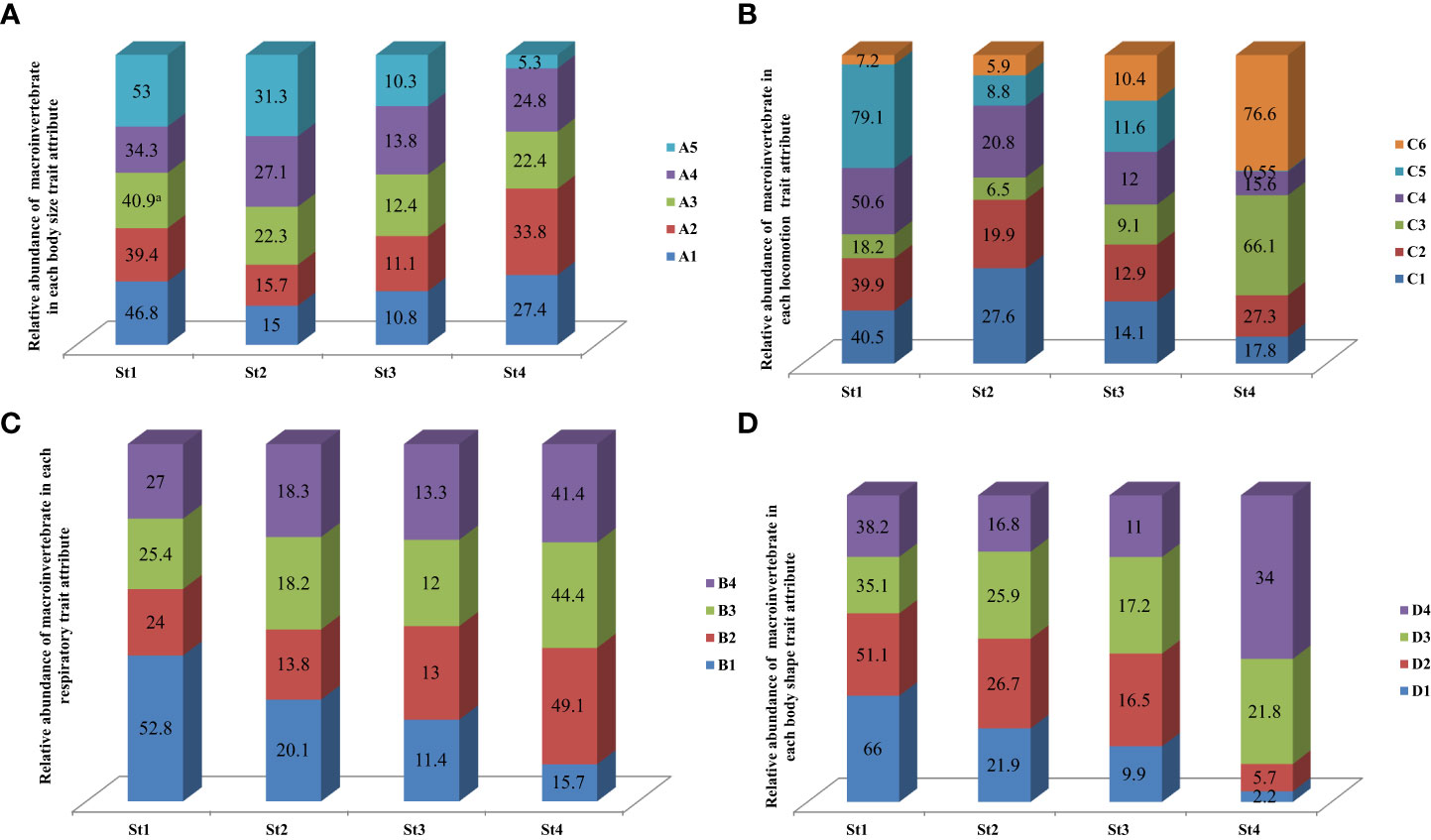
Figure 3 Relative abundance of macroinvertebrates in each of the analyzed body size (A), respiration (B), locomotion (C), and body shape (D) trait attributes at the four sampling stations in the River Chanchaga during the study. St1, Station 1; St2, Station 2; St3, Station 3; St4, Station 4.
The relative abundance of macroinvertebrates using cuticular respiration and those using spiracles to respire was the highest in Station 4. The relative abundance of macroinvertebrates with the gill trait was the highest in Station 1 compared to the remaining three stations. Gills decrease markedly from Stations 1 to 4, and spiracle respiratory systems increase from Stations 1 to 4 (Figure 3B). Of the six locomotion trait attributes analyzed, the relative abundance of macroinvertebrates that are climbers was higher in Stations 2 and 3. At the same time, skaters and burrowers dominated Stations 1 and 4, respectively, with the highest relative abundance (Figure 3C). The relative abundance of macroinvertebrates that are skaters decreases sharply from Stations 1 to 4, and that of burrowers decreases significantly from Stations 1 to 4 (Figure 3C). Macroinvertebrate taxa with climbing as the mode of locomotion and crawling showed no particular distribution pattern, as they decreased slightly from Stations 1 to 3 and then increased slightly in Station 4 (Figure 3C). The relative abundance of macroinvertebrates with streamlined body shapes was the highest in Station 1, and that of cylindrical body shapes was the highest in Station 4 (Figure 3D). Streamlined and flattened body shapes decreased from Stations 1 to 4, while spherical and cylindrical body shapes decreased markedly from Stations 1 to 3 and then increased in Station 4 (Figure 3D).
The result of our subsequent analysis to ascertain and confirm the patterns of distribution of macroinvertebrate traits using RLQ revealed that Axes 1 and 2 accounted for 72.02% and 13.58%, respectively, of the total variance in the RLQ ordination. Hence, the cumulative variance of the first two axes of the RLQ was 85.6%, indicating that the RLQ ordination was good. The eigenvalues of Axes 1 and 2 were 2.4 and 1.03, respectively. Axis 1 of the RLQ ordination enabled the distinction of the less perturbed Stations 1 and 2 from the relatively perturbed Stations 3 and 4 (Figure 4). In the first Axis 1 of the RLQ, traits such as medium body size, gills, swimming as a mode of locomotion, and streamlined and spherical body shapes were positively associated with Stations 1 and 2 (Figure 4). These trait attributes were represented by Caenidae, Gerridae, Naididae, Coenagrionidae, Belostomatidae, Gomphidae, Elmidae, Aeshnidae, Dytiscidae, and Heptagenidae (Figure 4). Equally, small and very large body sizes, spiracle respiration, climbing as the mode of locomotion, sprawling as the mode of locomotion, and cylindrical body shapes were associated positively with the perturbed Stations 3 and 4 (Figure 4). These traits were represented most by tolerant macroinvertebrate taxa such as Chironomidae, Dugesiidae, Planorbidae, Syrphidae, and Ceratopogonidae (Figure 4).
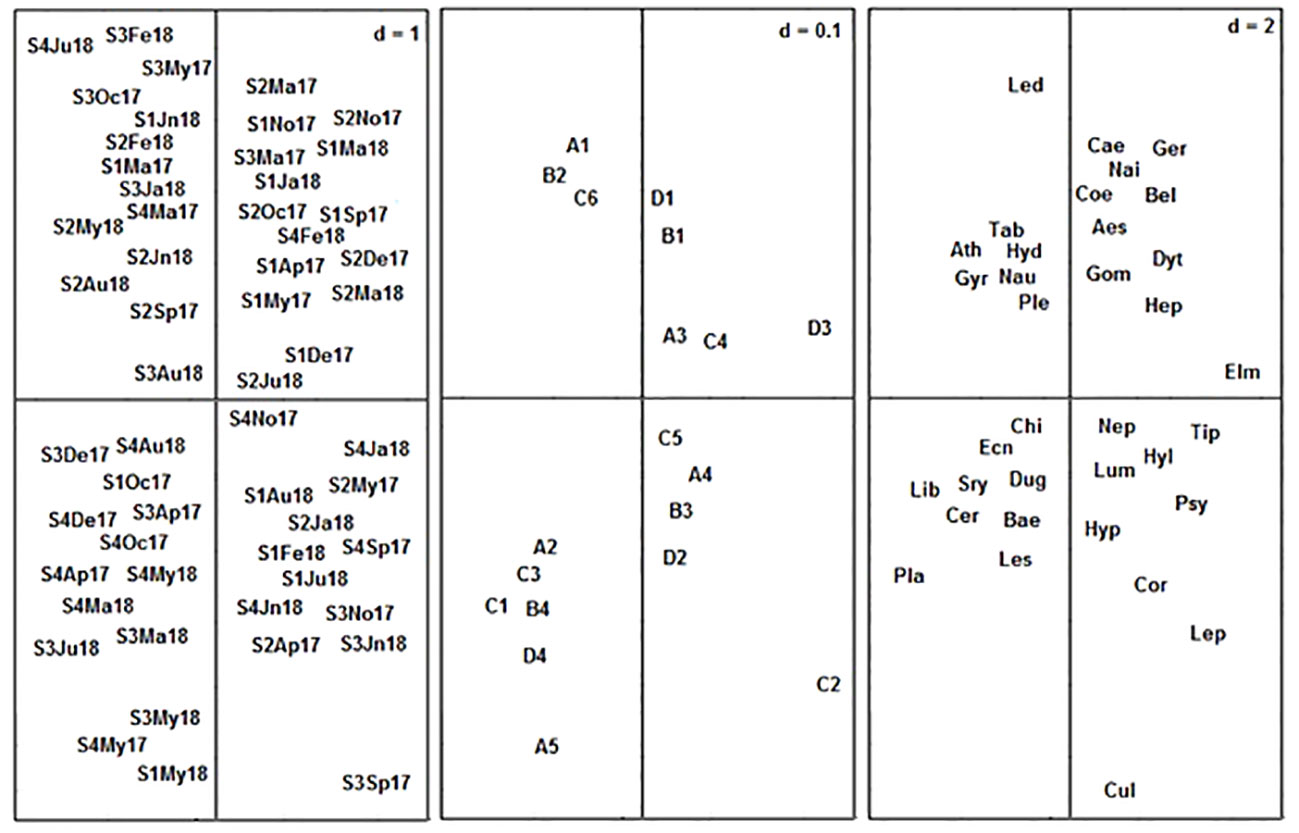
Figure 4 RLQ ordination visualizing the distribution of macroinvertebrate traits and taxa in relation to stations sampled. Abbreviations: Months: Ja (January), Fe (February), Ma (March), Ap (April), My (May), Jn (June), Ju (July), Au (August), Sp (September), Oc (October), No (November), and De (December). Stations: S1 (Station 1), S2 (Station 2), S3 (Station 3), and S4 (Station 4). Sampling years: 17 (2017) and 18 (2018). Traits: Body size: A1 (very small), A2 (small), A3 (medium), A4 (large), and A5 (very large). Respiration: B1 (gill), B2 (integument for oxygen diffusion), B3 (spiracle: hanging at the water surface using a siphon to obtain oxygen), and B4 (spiracle: vegetative respiration using plant stems to obtain oxygen). Locomotion: C1 (climber), C2 (crawler), C3 (sprawler), C4 (swimmer), C5 (skater), and C6 (burrower). Body shape: D1 (streamlined), D2 (flattened), D3 (spherical), and D4 (cylindrical). Taxa: Coe (Coenagrionidae), Les (Lesticidae), Aes (Aeshnidae), Gom (Gomphidae), Lib (Libellulidae), Dyt (Dytiscidae), Gyr (Gyrinidae), Hyd (Hydrophilidae), Elm (Elmidae), Bel (Belostomatidae), Nau (Naucoridae), Nep (Nepidae), Ple (Pleidae), Ger (Gerridae), Cor (Corixidae), Not (Notonectidae), Psy (Psychodidae), Chi (Chironomidae), Tip (Tipulidae), Cul (Culicidae), Sry (Syrphidae), Tab (Tabanidae), Ath (Athericidae), Cer (Ceratopogonidae), Hep (Heptageniidae), Cae (Caenidae), Bae (Baetidae), Lep (Leptophlebiidae), Hyp (Hydropsychidae), Hyl (Hydroptilidae).
Responses of macroinvertebrate trait attributes to anthropogenic influences: identifying and classifying vulnerable and resilient traits
Of the 19 macroinvertebrate trait attributes evaluated, the result of the fourth-corner test revealed that five trait attributes were positively correlated with at least one of the pollution-indicating physicochemical variables (EC, BOD5, sulfate, nitrate, and phosphate). The trait attributes were as follows: 1) small body size, 2) medium body size, 3) spiracle respiration, and 4) climbing as the mode of locomotion (Figure 5). Further, the gill and swimming as a mode of locomotion were positively associated with increasing DO concentration (Figure 5).
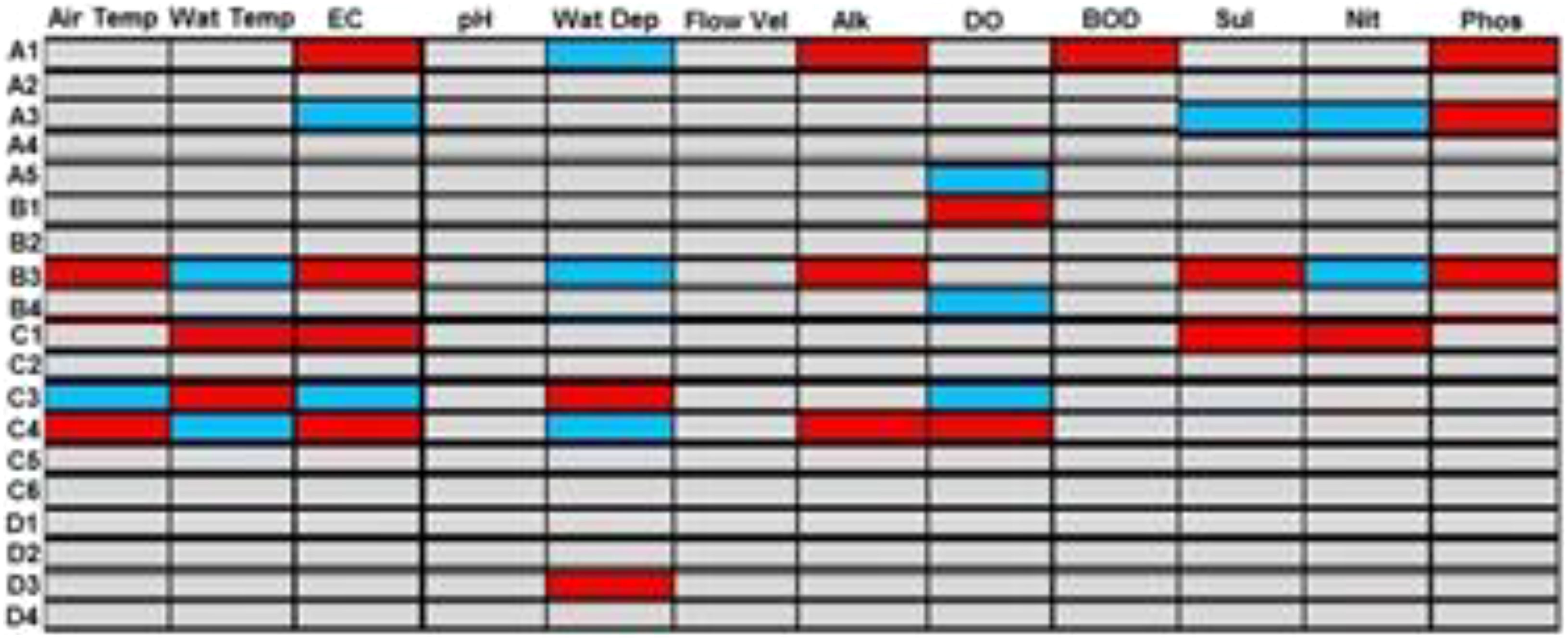
Figure 5 Fourth-corner analysis explaining the relationship between macroinvertebrate traits and physicochemical variables. Traits: Body size: A1 (very small), A2 (small), A3 (medium), A4 (large), and A5 (very large). Respiration: B1 (gill), B2 (integument for oxygen diffusion), B3 (spiracle: hanging at the water surface using a siphon to obtain oxygen), and B4 (spiracle: vegetative respiration using plant stems to obtain oxygen). Locomotion: C1 (climber), B2 (crawler), B3 (sprawler), C4 (swimmer), C5 (skater), and C6 (burrower). Body shape: D1 (streamlined), B2 (flattened), D3 (spherical), and D4 (cylindrical). Physicochemical variables: Air Temp (air temperature) Wat Temp (water temperature), EC (electrical conductivity), Wat Dep (water depth), Flow Vel (flow velocity), DO (dissolved oxygen), BOD (biochemical oxygen demand), Sul (sulfate), Nit (nitrate), and Phos (phosphate). Note that blue boxes indicate a significant negative correlation (p ≤ 0.05), and red boxes indicate a significant positive correlation (p ≤ 0.05).
Therefore, in determining the responses of macroinvertebrate trait attributes to anthropogenic influences to identify and classify vulnerable and resilient traits, we pointed out traits that were either positively or negatively correlated with some of the 12 selected physicochemical variables. Thus, traits that either correlated positively with increasing DO concentration or negatively with any three of the pollution-indicating physicochemical variables (EC, BOD5, sulfate, nitrate, and phosphate) were deemed vulnerable traits to anthropogenic influences. The traits are medium body size, gill respiration, and swimming as a mode of locomotion (Figure 5). However, very small and very large body sizes, integument respiration, spiracle respiration, and climbing as the mode of locomotion were identified and classified as resilient traits to anthropogenic influences, as they either correlated positively to at least three of the pollution indicating physicochemical variables or correlated negatively to increasing DO concentration (Figure 5).
Visualizing macroinvertebrate inter-trait and attribute association across the sampled stations
We performed a cluster analysis to visualize the inter-trait and attribute association across the four sampled stations. The cluster analysis showed a similarity value greater than 0.84 (0.84%), with four distinct clusters of inter-trait co-occurrence (Table 2; Figure 6). The four trait classes evaluated were represented in the first cluster. The represented trait attributes are very large body size, flattened and streamlined body shapes, gill respiration trait, and swimming mode of locomotion (Table 2; Figure 6). The relative abundance of macroinvertebrates exhibiting these traits in cluster 1 was higher in Station 1 than in the remaining stations (Figure 7). Cluster 2 traits consist of only the respiration trait class, and the trait attributes were cuticular respiration and spiracle respiration traits (Table 2; Figure 6). The relative abundance of macroinvertebrates in the second cluster decreased slightly from Stations 1 to 3 but increased markedly in Station 4 (Figure 8). Station 4 had the highest relative abundance of macroinvertebrate composition based on the mode of respiration association in cluster 2 and showed no significant differences among the four sampled stations (Figure 8). Cluster 3 had the highest trait class association with eight attributes (Table 2; Figure 6). This cluster is a mixture of all trait classes except the mode of respiration. Most of the trait attributes were related to body size (very small, small, medium, and large body sizes). Locomotion and body shape traits classes had two representatives each. Climbing as the mode of locomotion and crawling as the mode of locomotion were the representatives of locomotion traits, while spherical and cylindrical body shapes were the representatives of body shape traits. Cluster 3 had the highest relative abundance of the traits association in Station 1 and changed intermittently from Stations 2 to 4 (Figure 9). Cluster 4 had only two trait attributes representing only locomotion trait classes, namely, sprawling and burrowing as modes of locomotion (Table 2; Figure 6). The relative abundance of the locomotion trait in cluster 4 showed no marked difference (Figure 10). Among all the biological trait classes analyzed, only one did not form any of the four identified clusters: skating as a mode of locomotion.
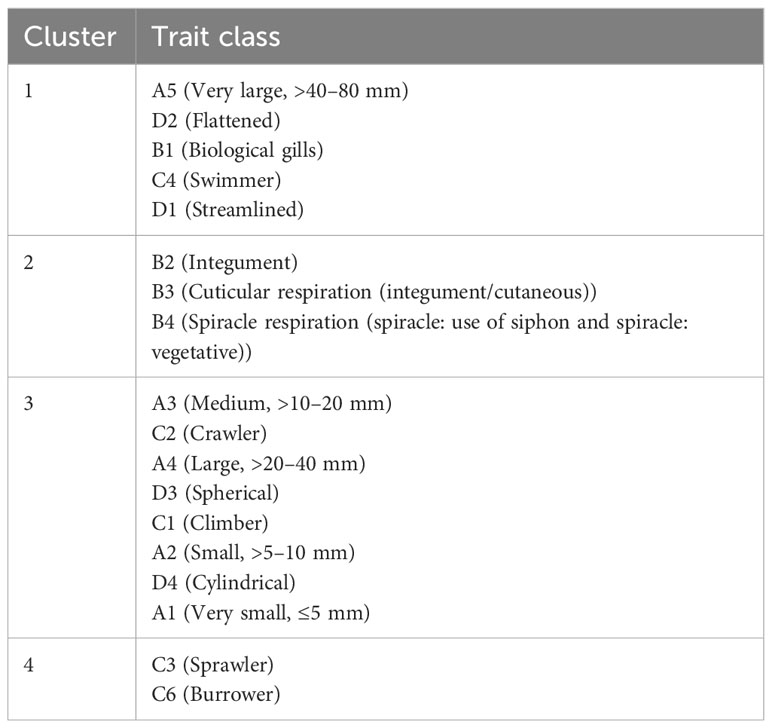
Table 2 The four distinct clusters of macroinvertebrate inter-trait and attribute clustering at >0.84 (84%) Bray–Curtis similarity in the River Chanchaga based on the dendrogram in Figure 5.
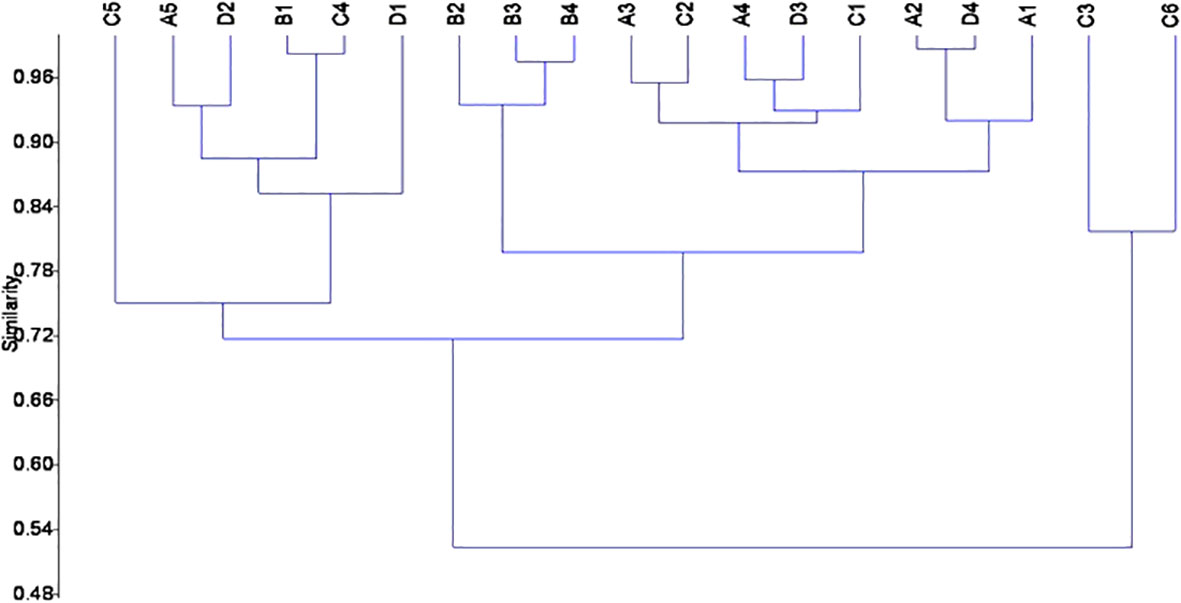
Figure 6 Dendrogram of cluster analysis based on Bray–Curtis similarity index showing inter-trait and attribute groupings in the River Chanchaga during the study period.
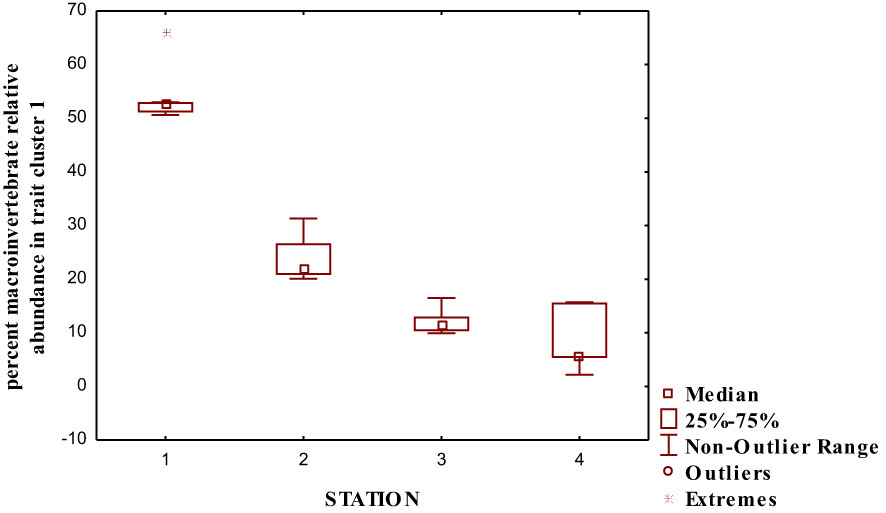
Figure 7 Relative abundance of macroinvertebrate inter-traits and attributes in cluster 1 based on the dendrogram in Figure 6.
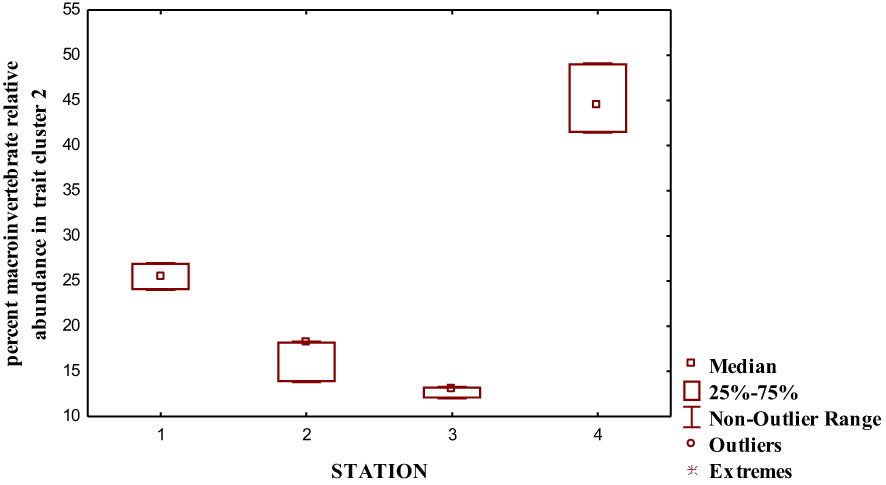
Figure 8 Relative abundance of macroinvertebrate inter-traits and attributes in cluster 2 based on the dendrogram in Figure 6.
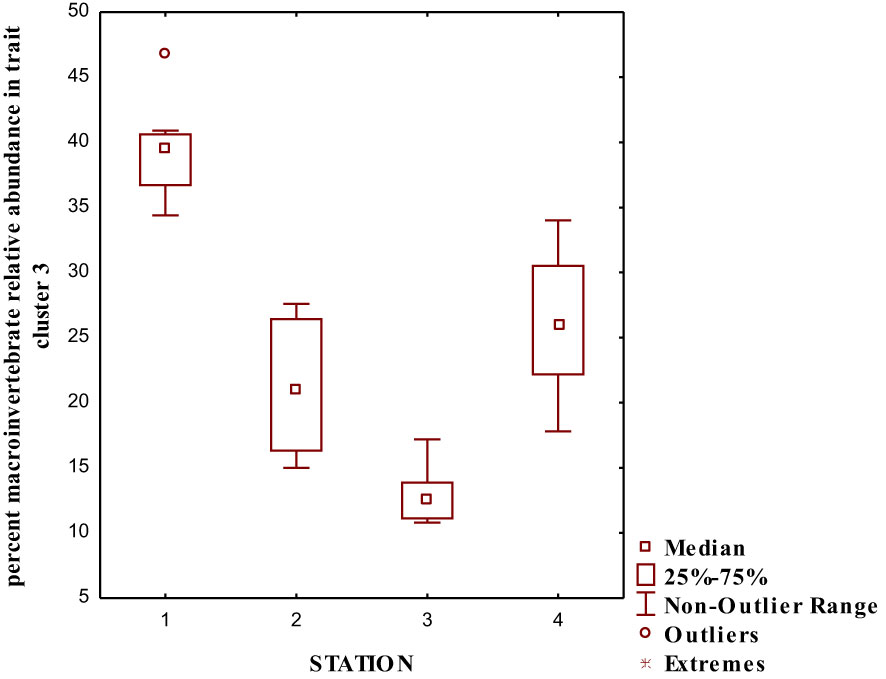
Figure 9 Relative abundance of macroinvertebrate inter-trait and attributes in cluster 3 based on the dendrogram in Figure 6.
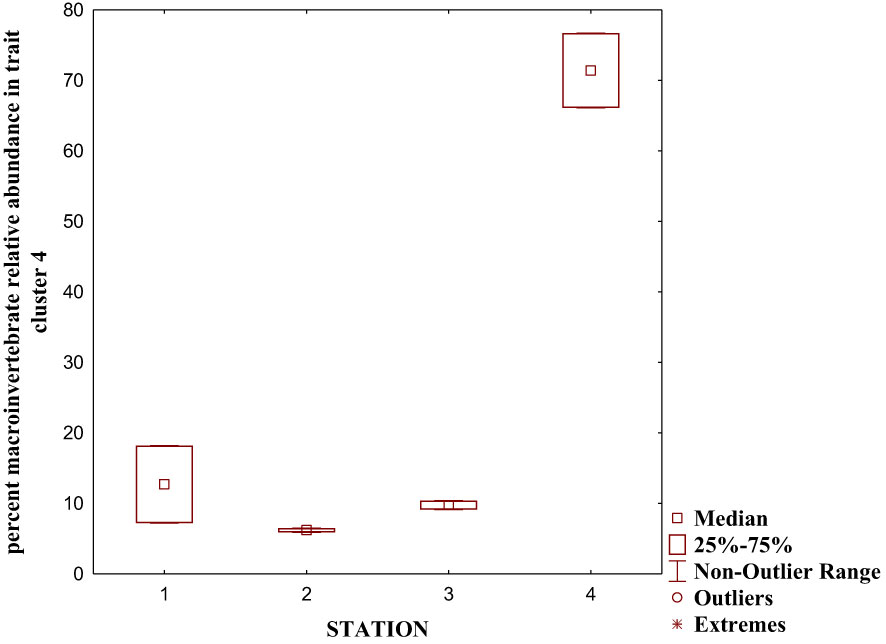
Figure 10 Relative abundance of macroinvertebrate inter-traits and attributes in cluster 4 based on the dendrogram in Figure 6.
Predictive performance of the variation in the taxa and trait responses to physicochemical variables among the four stations sampled
Among the four stations sampled in the course of the study, the trait model was a significantly better fit in all the stations except for Station 4 with a proportionately better fit for taxa than traits (Figure 11). There were no significant differences between the taxa and trait models among the four stations (p > 0.05) except for Station 4, which was different in the taxa model (p = 0.044, p < 0.05; Figure 11).
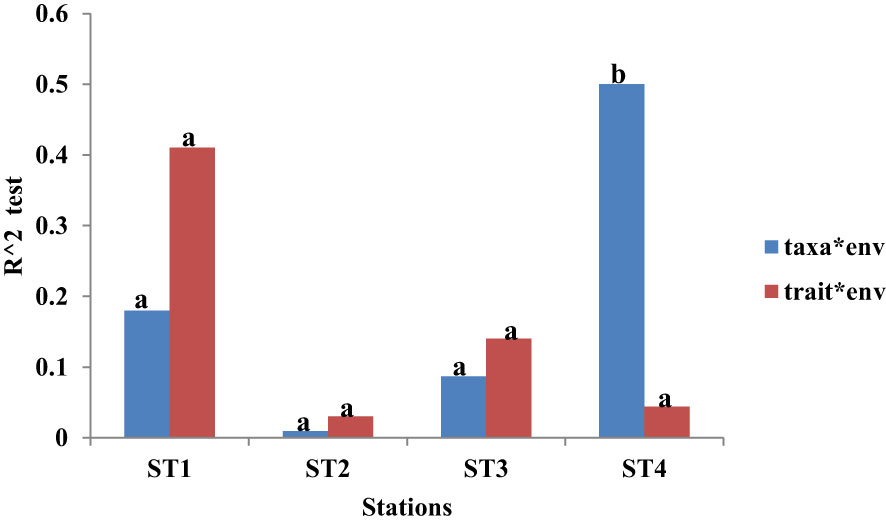
Figure 11 Predictive performance of the variation in the taxa and trait responses to physicochemical variables among the four stations sampled. The predictive performance was measured based on the ratio of deviance (R2 test) at 0.05 level of significance.
Discussion
The present study explored the potential responses of macroinvertebrate traits to illegal gold mining activities. We observed that illegal gold mining and other human activities impacted vegetation cover and instream flows at the affected stations and, in turn, influenced the distribution patterns of macroinvertebrate traits in the riverine system. In earlier studies, the combined effects of anthropogenic influences of industrial, agricultural, and urban activities have been noted to constitute grave implications on the structural (taxa) and functional (traits) assemblages of freshwater systems (Garba et al., 2022; Ge et al., 2022; Pandey et al., 2022). This study’s results showed that anthropogenic influences most especially illegal gold mining activities are major drivers that pattern the structural and functional assemblages of the Chanchaga river system. Our predictions were largely supported by the illegal gold mining and other anthropogenic activities in the surrounding catchment influencing the distribution pattern of macroinvertebrate traits along a pollution gradient. For example, macroinvertebrate traits displayed differential associations with the study area’s highly disturbed and reference stations, with pollution-tolerant and pollution-vulnerable traits being identified. Similar studies have shown that anthropogenic activities threaten macroinvertebrates’ functional assemblages and phylogeny in riverine systems (Ge et al., 2022). Furthermore, the result from the distribution pattern of macroinvertebrates taxa, as revealed from the RLQ model, agrees with previous studies that reference stations favor pollution-sensitive taxa (e.g., Caenidae, Gerridae, Dytiscidae, and Elmidae), whereas the impacted stations harbor more pollution-tolerant macroinvertebrate taxa such as Chironomidae, Dugesiidae, Planorbidae, and Syrphidae (Arimoro et al., 2015; Edegbene et al., 2015; Li et al., 2019; Edegbene, 2020; Garba et al., 2022; Ge et al., 2022).
Macroinvertebrate traits such as medium body size, gill respiration, and swimming as a mode of locomotion were associated with Station 1 on the RLQ and were either positively correlated with DO or negatively correlated with any EC, BOD5, nitrate, phosphate, and sulfate. Studies have shown medium-body-sized macroinvertebrates to dominate less perturbed sites (e.g., Edegbene et al., 2021b; Pandey et al., 2022). For instance, Pandey et al. (2022) recently reported the dominance of macroinvertebrate taxa with medium and large body sizes in an undisturbed site, which they ascribed to the fact that relatively undisturbed or pristine environments support more long-lived and large carnivorous taxa. Other studies have also reported a similar pattern of distribution of large-bodied macroinvertebrate taxa (e.g., Pil´o et al., 2016; Kenny et al., 2018; Hu et al., 2019). The dominance of medium-body-sized macroinvertebrates in the reference station also agreed with the River Habitat Template Concept (RHTC; Townsend and Hildrew, 1994) that large-bodied organisms associated more with the less-disturbed environment.
Previous studies have reported macroinvertebrates that obtained DO using gills to be highly sensitive to clogging in the face of organic pollution (Bilotta and Brazier, 2008; Akamagwuna et al., 2019). The vulnerability of filamentous gills to clogging by fine sediments may explain the negative associations of gill breathers to the highly disturbed stations and their dominance in the reference stations observed in this study. Nevertheless, although clogging can hinder the ability of gill-breathing macroinvertebrates to dwell in relatively unperturbed sites, some macroinvertebrate taxa in the Order Hemiptera (e.g., Gerridae and Belostomatidae) exhibited positive associations with Station 1 (the reference/control station) in this study. This trend may be attributed to the fact that they have the ability to carry air bubbles and plastrons to dive using their spiracle respiration mechanism.
Locomotion is an important trait that could enable an organism to seek refuge in the face of increasing disturbance (Ladle and Ladle, 1992). In our study, the relative abundance of locomotion traits, such as swimmers and skaters, decreased in the impacted stations. Therefore, taxa with active swimming abilities probably moved to the less-impacted stations during increased pollution, reflecting their adaptive potential and deteriorating water quality in the Chanchaga river system. Similarly, other studies have revealed that modes of locomotion (e.g., crawling, swimming, skating, sprawling, climbing as the mode of locomotion, and burrowing) significantly correlate with stressors (Ward et al., 2013; Pandey et al., 2022). In this study, swimming and climbing as the mode of locomotion traits were considered vulnerable to pollution. However, the classification of the swimming mobility trait as vulnerable to pollution is contrary to recent reports that classified swimming as a mode of locomotion to be tolerant to organic pollution (Pandey et al., 2022). The disparate responses of mobility traits may be attributed to or affirm our assertion of the concept of trait syndrome, as, ordinarily, organisms that are swimmers can swim away from perturbed sites (Edegbene et al., 2021b). However, our classification of swimming as a mode of locomotion as a vulnerable trait agrees with Wilkes et al. (2017), who classified swimmers as sensitive to pollution.
Our results showed that very-small- and very-large-body-sized traits are resilient to pollution. The association of very small body size to impacted stations and their resultant positive correlation with pollution indicating physicochemical variables (e.g., EC, BOD5, and nutrients) is in line with the prediction of Townsend and Hildrew (1994), known as the RHTC. They asserted that small-body-sized organisms undergo rapid population growth and short generation time, producing many offspring per reproductive event, which confers resilience and resistance to pollution. Other studies have reported similar findings (e.g., Serra et al., 2017; Castro et al., 2018). However, very-large-body-sized taxa were also resilient to pollution. This finding agrees with Odume (2020), who identified large-body-sized organisms as potentially tolerant traits of urban pollution. However, other studies have reported contrary findings (e.g., Pil´o et al., 2016; Kenny et al., 2018; Edegbene et al., 2021b; Pandey et al., 2022). As reported by various studies, these inconsistencies in trait response to pollution can also be linked to trait syndrome, as we stated earlier. In this study, most of the large-body-sized macroinvertebrate taxa (e.g., Planorbidae and Dugesiidae) were associated with Station 4 (one of the heaviest impacted stations) on the RLQ and negatively correlated with DO on the fourth-corner test.
We found out that macroinvertebrates possessing spiracles increased markedly in relative abundance in Station 4. This confirmed the assertion by Statzner and Bêche (2010), who opined that the possession of spiracles and other specialized breathing techniques (breathing tube and straps/other apparatus, e.g., elytra) is an adaptive mechanism for obtaining atmospheric oxygen in a dissolved oxygen-deficient environment, as organisms possessing such traits become less reliant on dissolved oxygen concentration. Further, organisms that exhibit spiracle respiration increased in Stations 3 and 4. This may be attributed to warmer temperatures due to the exposed nature of the stations all year round. Diaz et al. (2008) reported the dominance of some Coleoptera and Diptera, which respire aerially and dwell in a polluted environment. In this current study, the dominance of Diptera (e.g., Culicidae) in Stations 3 and 4, as revealed by the RLQ model, may also be attributed to the increased breathing by spiracle: vegetative respiration mechanism. Hence, we classified spiracle: vegetative respiration method traits as pollution-resilient traits, contrary to our predicted response of the trait attributed to pollution.
Conclusion
Our study showed that illegal gold mining and other anthropogenic activities in the surrounding catchment negatively affected trait combinations expressed by macroinvertebrates in the River Chanchaga. Trait combinations such as medium body size, gills and spiracle respiration, and streamlined and spherical body shapes were positively associated with Stations 3 and 4, the impacted stations. These traits were considered pollution-tolerant, as they also showed positive associations with increasing electrical conductivity, biological oxygen demand, sulfate, nitrate, and phosphate. Overall, traits such as small and large body sizes, climbing as the mode of locomotion, sprawling as the mode of locomotion, and cylindrical body shape were adjudged to reflect the potential impact of illegal gold mining in the affected Stations 3 and 4 due to their positive association with these stations, as revealed by the RLQ model. However, based on the fourth-corner test we conducted, these traits either correlated positively to increased dissolved oxygen concentration or correlated negatively with increasing nutrient variables; hence, they were deemed vulnerable traits. The results of this study provided further insights and data on the growing literature on trait-based biomonitoring of anthropogenic pollution arising mainly from gold mining activities; this information can contribute to the development of local trait-based tools in the region. However, we suggest caution be applied in interpreting trait responses to stressors, as limited seasonal data were used. Also, attention should be given to trait co-occurrence and correlation vis-à-vis trait syndrome when analyzing trait response to stressors such as illegal gold mining activities. We, therefore, recommend that further studies should be conducted that will collect more environmental variables including heavy metals, which are among the determining variables of gold mining pollution across large spatial and time scales, to extrapolate our findings further.
Data availability statement
The raw data supporting the conclusions of this article will be made available by the authors, without undue reservation.
Ethics statement
The manuscript presents research on animals that do not require ethical approval for their study.
Author contributions
Study conceptualization and design: AE. Material preparation and data collection: AE. Data analysis: AE, TEO and CN. Preparation of the first draft of the manuscript: AE, TEO, CN, and FCA. Fund acquisition for the research: AE. Editing and reviewing of the manuscript: AE, LE, FOA, EO, TEO, EA, NU, CN, AS, and FCA. Finalization of the manuscript: AE and TEO. All authors contributed to the article and approved the submitted version.
Funding
The study was supported by the doctoral grant awarded to the first author by the Tertiary Education Trust Fund (TETFUND) of Nigeria at Sule Lamido University, Kafin Hausa, Nigeria with TETFUND letter referenced: TETF/DASTD/UNIV/JIGAWA STATE/VOL.1 and Sule Lamido University letter referenced: SLU/VC/TIC/002/VOL.1.
Acknowledgments
The study was supported by the doctoral grant awarded to the first author by the Tertiary Education Trust Fund (TETFUND) of Nigeria. Special thanks to Bawinile Mahlaba of the Institute for Water Research, Rhodes University, Makhanda, South Africa, for helping with the study area map. Special thanks to our undergraduate research students—Meshack Sanda, Osmond Obinnna Odoh, Helen Onyenche Adekunle, and Hassana Usman—for their support during the field and laboratory exercise. Mr. Prince Ossai is also acknowledged for his assistance in the laboratory. We acknowledge the staff of Albany Museum, Makhanda, South Africa, for the confirmation of some of the taxa and trait-based allocation. Our unreserved appreciation goes to the two reviewers whose critical and constructive comments/input helped in refining this paper.
Conflict of interest
The authors declare that the research was conducted in the absence of any commercial or financial relationships that could be construed as a potential conflict of interest.
Publisher’s note
All claims expressed in this article are solely those of the authors and do not necessarily represent those of their affiliated organizations, or those of the publisher, the editors and the reviewers. Any product that may be evaluated in this article, or claim that may be made by its manufacturer, is not guaranteed or endorsed by the publisher.
Supplementary material
The Supplementary Material for this article can be found online at: https://www.frontiersin.org/articles/10.3389/fevo.2023.1149227/full#supplementary-material
References
African Union (2009). Africa mining vision: “Transparent, equitable and optimal exploitation of mineral resources to underpin broad-based sustainable growth and socio-economic development” (Ethiopia: African Union Summit held in February 2009 at Addis Ababa), 55. Available at: https://au.int/sites/default/files/documents/30995-doc-africa_mining_vision_english_1.pdf.
Akamagwuna F. C., Mensah P. K., Nnadozie C. F., Odume O. N. (2019). Traits-based responses of Ephemeroptera, Plecoptera and Trichoptera to sediment stress in the Tsitsa River and its tributaries, Eastern Cape, South Africa. River Res. Appl. 35 (7), 999–1012. doi: 10.1002/rra.3458
Akamagwuna F. C., Ntloko P., Edegbene A. O., Odume O. N. (2021). Are Ephemeroptera, Plecoptera and Trichoptera traits reliable indicators of semi-urban pollution in the Tsitsa River, Eastern Cape Province of South Africa? Environ. Monit. Assess. 193 (5), 309. doi: 10.1007/s10661-021-09093-z
APHA. (1998). Standard methods for the examination of water and wastewater, WEF and AWWA. 20th Edition. (Washington, DC: American Public Health Association).
Arimoro F. O., Ikomi R. B., Nwadukwe F. O., Eruotor O. D., Edegbene A. O. (2014). Fluctuating salinity levels and increasing pollution gradient on fish community structure and trophic levels in a small creek in the Niger Delta, Nigeria. Int. Aquat. Res. 6 (4), 187–202. doi: 10.1007/s40071-014-0078-2
Arimoro F. O., Odume O. N., Uhunoma S. I., Edegbene A. O. (2015). Anthropogenic impact on water chemistry and benthic macroinvertebrate associated changes in a southern Nigeria stream. Environ. Monit. Assess. 1871 doi: 10.1007/s10661-014-4251-2
Balmert A., Bohn H. F., Ditsche-Kuru P., Barthlott W. (2011). Dry under water: Comparative morphology and functional aspects of air-retaining insect surfaces. J. morphology 272, 442–451. doi: 10.1002/jmor.10921
Bester V., Groenewald L. (2021). Corporate social responsibility and artisanal mining: Towards a fresh South African perspective. Resour. Policy 72, 102124. doi: 10.1016/j.resourpol.2021.102124
Bilotta G. S., Brazier R. E. (2008). Understanding the influence of suspended solids on water quality and aquatic biota. Water Res. 422849 doi: 10.1016/j.watres.2008.03.018
Brown A. M., Warton D. I., Andrew N. R., Binns M., Cassis G., Gibb H. (2014). The fourth-corner solution – using predictive models to understand how species traits interact with the environment. Methods Ecol. Evol. 5, 344–352. doi: 10.1111/2041-210X.12163
Buck J. (1962). Some physical aspects of insect respiration. Annu. Rev. Entomology 7 (1), 27–56. doi: 10.1146/annurev.en.07.010162.000331
Castro D. M. P., Dolédec S., Callisto M. (2018). Land cover disturbance homogenizes aquatic insect functional structure in neotropical savanna streams. Ecol. Indic. 84, 573–582. doi: 10.1016/j.ecolind.2017.09.030
Chevene F., Dolédec S. (1994). A fuzzy coding approach for analysis of long-term ecological data. Freshw. Biol. 31, 295–309. doi: 10.1111/j.1365-2427.1994.tb01742.x
Cranston P. S. (2000). Electronic guide to the Chironomidae of Australia. http://www/entomologyucdavis.edu/chiropage. Accessed 29 August 2018.
Desrosiers M., Usseglio-Polatera P., Archaimbault V., Larras F., Methot G., Pinel-Alloul B. (2019). Assessing anthropogenic pressure in the St. Lawrence River using traits of benthic macroinvertebrates. Sci. Total Environ. 649, 233–246. doi: 10.1016/j.scitotenv.2018.08.267
Diaz A. M., Alonso M. L. S., Gutierrez M. R. V. (2008). Biological traits of stream macroinvertebrates from a semi-arid catchment: patterns along complex environmental gradients. Freshw. Biol. 531, 21. doi: 10.1111/j.1365-2427.2007.01854.x
Ding N., Yang W., Zhou Y., Gonález-Bergonzoni I., Zhang J., Chen K., et al. (2017). Different responses of functional traits and diversity of stream macroinvertebrates to environmental and spatial factors in the Xishuangbanna watershed of the upper Mekong River Basin, China. Sci. Total Environ. 574, 288–299. doi: 10.1016/j.scitotenv.2016.09.053
Dolédec S., Chessel D., ter Braak C. J. F., Champely S. (1996). Matching species traits to environmental variables: a new three-table ordination method. Environ. Ecol. Stat 3 (2), 143–166. doi: 10.1007/BF02427859
Dray S., Dufour A. B. (2007). The ade4 package: implementing the duality diagram for ecologists. J. Stat. Software 22, 1–20. doi: 10.18637/jss.v022.i04
Duncan A. E. (2020). The dangerous couple: illegal mining and water pollution—A case study in fena river in the ashanti region of Ghana. J. Chem. 2378560, 9. doi: 10.1155/2020/2378560
Edegbene A. O. (2020). Developing macroinvertebrate trait-and taxonomically-based approaches for biomonitoring wadeable riverine systems in the Niger Delta, Nigeria (Grahamstown, South Africa: Ph.D. thesis Rhodes University). doi: 10.21504/10962/140660
Edegbene A. O., Abdullahi Y., Akamagwuna F. C., Omovoh B. O., Osimen E. C., Ogidiaka E. (2022). Are zooplankton useful indicators of ecological quality in Afrotropical Ephemeral River impacted by human activities? Environ. Monit. Assess. 194, 399. doi: 10.1007/s10661-022-10061-4
Edegbene A. O., Adam M. B., Gambo J., Osimen E. C., Ikomi R. B., Ogidiaka E., et al. (2021b). Searching for indicator macroinvertebrate traits in an Afrotropical riverine system: implication for ecosystem biomonitoring and sustainability. Environ. Monit. Assess. 193 (11), 711. doi: 10.1007/s10661-021-09450-y
Edegbene A. O., Akamagwuna F. C. (2022). Insights from the Niger Delta Region, Nigeria on the impacts of urban pollution on the functional organisation of Afrotropical macroinvertebrates. Sci. Rep. 12, 22551. doi: 10.1038/s41598-022-26659-0
Edegbene A. O., Elakhame L. A., Arimoro F. O., Osimen E. C., Akamagwuna F. C., Edegbene Ovie T. T., et al. (2023). Preliminary studies on macroinvertebrate biomonitoring of freshwater systems in the Afrotropics: a case study of the Chanchaga River in the Lower Niger-Benue Ecoregion of Nigeria. Biologia. doi: 10.1007/s11756-023-01445-z
Edegbene A. O., Arimoro F. O., Odoh O., Ogidiaka E. (2015). Effect of anthropogenicity on the composition and diversity of aquatic insects of a municipal river in North Central Nigeria. Biosci. Res. Today’s World 1 (1), 55–66.
Edegbene A. O., Arimoro F. O., Odume O. N. (2020a). Exploring the distribution patterns of macroinvertebrate signature traits and ecological preferences and their responses to urban and agricultural pollution in selected rivers in the Niger Delta ecoregion, Nigeria. Aquat. Ecol. 54, 553–573. doi: 10.1007/s10452-020-09759-9
Edegbene A. O., Arimoro F. O., Odume O. N. (2020b). How does urban pollution infuence macroinvertebrate traits in forested riverine systems? Water 12 (11), 3111. doi: 10.3390/w12113111
Edegbene A. O., Elakhame L. A., Arimoro F. O., Osimen E. C., Odume O. N. (2019). Development of macroinvertebrate multimetric index for ecological evaluation of a river in North Central Nigeria. Environ. Monit. Assess. 191 (5), 274. doi: 10.1007/s10661-019-7438-8
Edegbene A. O., Odume O. N., Arimoro F. O., Keke U. N. (2021a). Identifying and classifying macroinvertebrate indicator signature traits and ecological preferences along urban pollution gradient in the Niger Delta. Environ. pollut. 281, 117076. doi: 10.1016/j.envpol.2021.117076
Fierro P., Bertran C., Hauenstein E., Pena-Cortes F., Vergara C., Cerna C., et al. (2017). Effects of local land-use on riparian vegetation, water quality and the functional organisation of macroinvertebrate assemblages. Sci. Total Environ. 609, 724.e734. doi: 10.1016/j.scitotenv.2017.07.197
Garba F., Ogidiaka E., Akamagwuna F. C., Nwaka K. H., Edegebene A. O. (2022). Deteriorating water quality state on the structural assemblage of aquatic insects in a North-Western Nigerian River. Water Sci. 3622 (1), 31. doi: 10.1080/23570008.2022.2034396
Gbedzi D. D., Ofosu E. A., Mortey E. M., Obiri-Yeboah A., Nyantakyi E. K., Siabi E. K., et al. (2022). Impact of mining on land use land cover change and water quality in the asutifi north district of Ghana, west africa. Environ. Challenges. 6, 100441. doi: 10.1016/j.envc.2022.100441
Ge Y., Liu Z., García-Gir´on J., Chen X., Yan Y., Li Z., et al. (2022). Human-induced loss of functional and phylogenetic diversity is mediated by concomitant deterministic processes in subtropical aquatic insect communities. Ecol. Indic. 136, 108600. doi: 10.1016/j.ecolind.2022.108600
Gerber A., Gabriel M. J. M. (2002). Aquatic invertebrates of South African rivers – field guide. (Pretoria: Resource Quality Services, Department of Water Affairs).
Ghali H., Osimen E. C., Ogidiaka E., Akamagwuna F. C., Keke U.N. and Edegbene A. O. (2020). Preliminary assessment of the deteriorating state of an irrigation dam in north western Nigeria using phytoplankton structural assemblage and environmental factors. Water Sci. 34 (1), 181–189. doi: 10.1080/11104929.2020.1816152
Gordon N. D., McMahon T.A., Finlayson B.L. (1994). Stream Hydrology, an Introduction for Ecologists. (New York, NY: John Wiley & Sons).
Hammer Ø., Harper D. A. T., Ryan P. D. (2001). PAST: paleontological statistics software package for education and data analysis. Palaeontologia Electronica 4 (1), 9Pp. Available at: http://palaeo-electronica.org/2001_1/past/issue1_01.htm.
Hershey A. E., Lamberti G. A., Chaloner D. T., Northington R. M. (2010). “Aquatic insect ecology,” in Ecology and classification of north american freshwater invertebrates, 3rd edEds. Thorp J. H., Covich A. P., 659–694doi: 10.1016/B978-0-12-374855-3.00017-0
Hu C., Dong J., Gao L., Yang X., Wang Z., Zhang X. (2019). Macrobenthos functional trait responses to heavy metal pollution gradients in a temperate lagoon. Environ. Pollut. 253, 1107–1116. doi: 10.1016/j.envpol.2019.06.117
Hui F. K. C., Warton D. I., Foster S. D., Dunstan P. K (2013). To mix or not to mix: comparing the predictive performance of mixture models vs. separate species distribution models. Ecology 94, 1913–1919. doi: 10.1890/12-1322.1
Keke U. N., Arimoro F. O., Ayanwale A. V., Odume O. N., Edegbene A. O. (2020). Exploratory accounts of biodiversity patterns along seasonality and environmental factors of stream communities in an Afro-tropical biodiversity hotspot, Nigeria. Egyptian J. Aquat. Biol. Fisheries 24 (4), 521–534. doi: 10.21608/EJABF.2020.102102
Kenny A. J., Jenkins C., Wood D., Bolam S. G., Mitchell P., Scougal C., et al. (2018). Assessing cumulative human activities, pressures, and impacts on North Sea benthic habitats using a biological traits approach. ICES J. Mar. Sci. 75, 1080–1092. doi: 10.1093/icesjms/fsx205
Ladle M., Ladle R. J. (1992). Life history patterns of river invertebrates. Hydrobiologia 24831, 37 doi: 10.1007/BF00008883
Leuenberger A., Winkler M. S., Cambaco O., Cossa H., Kihwele F., Lyatuu I., et al. (2021). Health impacts of industrial mining on surrounding communities: Local perspectives from three sub-Saharan African countries. PloS One 16 (6), e0252433. doi: 10.1371/journal.pone.0252433
Li Z., Wang J., Liu Z., Meng X., Heino J., Jiang X., et al. (2019). Different responses of taxonomic and functional structures of stream macroinvertebrate communities to local stressors and regional factors in a subtropical biodiversity hotspot. Sci. Total Environ. 655, 1288–1300. doi: 10.1016/j.scitotenv.2018.11.222
Matomela N. H., Chakona A., Kadye W. T. (2021). Comparative assessment of macroinvertebrate communities within three Afromontane headwater streams influenced by different land use patterns. Ecol. Indic. 129, 107972. doi: 10.1016/j.ecolind.2021.107972
Merem E. C., Twumasi Y., Wesley J., Isokpehi P., Shenge M., Fageir S., et al (2017). Assessing the ecological effects of mining in West Africa: the case of Nigeria. Int. J. Min. Eng. Mineral Process. 6 (1), 1–19. doi: 10.5923/j.mining.20170601.01
Mereta S. T., Boets P., De Meester L., Goethals P. L. M. C. (2013). Development of multimetric index based on benthic macroinvertebrates for the assessment of natural wetlands in southwest Ethiopia. Ecol. Indic. 29, 510e521. doi: 10.1016/j.ecolind.2013.01.026
M’kandawire E., Choongo K., Yabe J., Mwase M., Saasa N., Nakayama S. M. M. M., et al. (2017). Sediment metal contamination in the kafue river of Zambia and ecological risk assessment. Bull. Environ. Contam. Toxicol. 99, 108–116. doi: 10.1007/s00128-017-2089-3
Ngole-Jeme V. M., Fantke P. (2017). Ecological and human health risks associated with andoned gold mine tailings contaminated soil. PloS One 12, e0172517. doi: 10.1371/journal.pone.0172517
Ntloko P., Palmer C. G., Akamagwuna F. C., Odume O. N. (2021). Exploring macroinvertebrates ecological preferences and trait-based indicators of suspended fne sediment efects in the Tsitsa River and its tributaries, Eastern Cape. South Africa. Water 13 (6), 798. doi: 10.3390/w13060798
Obiri-Yeboah A., Nyantakyi E. K., Mohammed A. R., Yeboah S. I. I. K., Domfeh M. K., Abokyi E. (2021). Assessing potential health effect of lead and mercury and the impact of illegal mining activities in the bonsa river, tarkwa nsuaem, Ghana. Sci. Afr. 13, e00876. doi: 10.1016/j.sciaf.2021.e00876
Odume O. N. (2020). Searching for urban pollution signature and sensitive macroinvertebrate traits and ecological preferences in a river in the Eastern Cape of South Africa. Ecol. Indic. 108, 105759. doi: 10.1016/j.ecolind.2019.105759
Oksanen J., Blanchet D., Minchin P. R., O’Hara R. B., Simpson G. L., Solymos P., et al. (2019) Vegan: community ecology package. Available at: https://cran.r-project.org,https://github.com/veganders/vegan.
Ollis D. J., Dallas H. F., Esler K. J., Boucher C. (2006). Bioassessment of the ecological integrity of river ecosystems using aquatic macroinvertebrates: an overview with a focus on South Africa. Afr. J. Aquat. Sci. 2, 205–227. doi: 10.2989/16085910609503892
Pallottini M., Cappelletti D., Fabrizi A., Gaino E., Goretti E., Selvaggi R., et al. (2017). Macroinvertebrate functional trait responses to chemical pollution in agricultural–industrial landscapes. River Res. Appl. 33 (4), 505–513.
Pandey V., Jha D. K., Kumar P. S., Santhanakumar J., Venkatnarayanan S., Jebakumar J. P. P., et al. (2022). Effect of multiple stressors on the functional traits of sub-tidal macrobenthic fauna: A case study of the southeast coast of India. Mar. pollut. Bull. 175, 113355. doi: 10.1016/j.marpolbul.2022.113355
Pil´o D., Ben-Hamadou R., Pereira F., Carriço A., Pereira P., Corzo A., et al. (2016). How functional traits of estuarine macrobenthic assemblages respond to metal contamination? Ecol. Indic. 71, 645–659. doi: 10.1016/j.ecolind.2016.07.019
Poff N. L., Olden J. D., Vieira N. K. M., Finn D. S., Simmons M. P., Konddratieff B. C. (2006). Functional trait niches of North American lotic insects: traits-based ecological applications in light of phylogenetic relationships. J. North America Benthological Soc. 25, 730e755. doi: 10.1899/0887-3593(2006)025[0730:FTNONA]2.0.CO;2
R Core Team (2020). “R: A language and environment for statistical computing,” in R foundation for statistical computing(Vienna, Austria). Available at: http://www.r-project.org/R.
Serra R. Q. S., Graca M. A. S., Dolédec S., Feio M. J. (2017). Chironomidae traits and life history strategies as indicators of anthropogenic disturbance. Environ. Monit. Assess. 189 (7), 326. doi: 10.1007/s10661-017-6027-y
Statzner B., Bêche L. (2010). Can biological invertebrate traits resolve effects of multiple stressors on running water ecosystems? Freshw. Biol. 5580 (1), 199. doi: 10.1111/j.1365-2427.2009.02369.x
Tibco Software (2020). TIBCO statistica ™ Quick reference (Palo Alto, California: TIBCO Software Inc), 11.
Tomanova S., Moya N., Oberdorff T. (2008). Using macroinvertebrate biological traits for assessing biotic integrity of neotropical streams. River Res. Appl. 241230, 1239 doi: 10.1002/rra.1148
Townsend C. R., Hildrew A. G. (1994). Species traits in relation to a habitat templet for river systems. Freshw. Biol. 170243, 255 doi: 10.1111/j.1365-2427.1994.tb01740.x
Ward D. J., Simpson S. L., Jolley D. F. (2013). Slow avoidance response to contaminated sediments elicits sublethal toxicity to benthic invertebrates. Environ. Sci. Technol. 47, 5947–5953. doi: 10.1021/es400152a
Watson G. S., Watson J. A., Cribb B. W. (2017). Diversity of cuticular micro and nano structures on insects: properties, functions, and potential applications. Annu. Rev. Entomology 62, 185–205. doi: 10.1146/annurev-ento-031616-035020
Wilkes M. A., Mckenzie M., Murphy J. F., Chadd R. P. (2017). Assessing the mechanistic basis for fine sediment biomonitoring: inconsistencies among the literature, traits and indices. River Res. Appl. 33 (10), 1618–1629. doi: 10.1002/rra.3139
World Bank (2017). The growing role of minerals and metals for a low carbon future (Washington, D.C., USA: World Bank).
World Gold Council (2012). “World gold council conflict-free gold standard,” A presentation by Terry Heymann on the 2nd of May 2012 at the “Meeting of the OECD-hosted forum on implementation of due diligence in the gold supply chain” held at the OECD Conference Centre, 2 rue André Pascal, 75016 Paris, France, 24. Available at: https://www.oecd.org/daf/inv/investmentfordevelopment/50471988.pdf.
Zengeya T. A., Robertson M. P., Booth A. J., Chimimba C. T. (2013). A qualitative ecological risk assessment of the invasive nile tilapia, Oreochromis niloticus in a sub-tropical african river system (Limpopo river, South Africa). Aquat. Conserv. Mar. Freshw. Ecosyst. 23, 51–64. doi: 10.1002/aqc.2258
Keywords: illegal gold mining, trait-based approach, RLQ and fourth-corner model, River Chanchaga, Nigeria
Citation: Edegbene AO, Elakhame LA, Arimoro FO, Osimen EC, Edegbene Ovie TT, Akumabor EC, Ubanatu NC, Njuguna CW, Sankoh AA and Akamagwuna FC (2023) How do the traits of macroinvertebrates in the River Chanchaga respond to illegal gold mining activities in North Central Nigeria. Front. Ecol. Evol. 11:1149227. doi: 10.3389/fevo.2023.1149227
Received: 21 January 2023; Accepted: 22 September 2023;
Published: 16 October 2023.
Edited by:
Lynne Beaty, The Behrend College, United StatesReviewed by:
Deepthi Wickramasinghe, University of Colombo, Sri LankaHeather Fair, University of Minnesota Twin Cities, United States
Copyright © 2023 Edegbene, Elakhame, Arimoro, Osimen, Edegbene Ovie, Akumabor, Ubanatu, Njuguna, Sankoh and Akamagwuna. This is an open-access article distributed under the terms of the Creative Commons Attribution License (CC BY). The use, distribution or reproduction in other forums is permitted, provided the original author(s) and the copyright owner(s) are credited and that the original publication in this journal is cited, in accordance with accepted academic practice. No use, distribution or reproduction is permitted which does not comply with these terms.
*Correspondence: Augustine O. Edegbene, b3ZpZWVkZXNAZ21haWwuY29t
†ORCID: Augustine O. Edegbene, orcid.org/0000-0003-4557-5361