- 1Department of Biodiversity and Landscape Ecology, Osnabrück University, Osnabrück, Germany
- 2Institute of Biodiversity and Landscape Ecology (IBL), Münster, Germany
- 3Institute of Applied Entomology, Beverungen, Germany
Introduction: Industrialization has ushered in massive changes in agriculture. Particularly in low mountain ranges, large-scale afforestation with Norway spruce on traditionally managed, semi-natural grasslands has caused a severe decline in biodiversity. Tree removal, hay transfer and resumption of grazing or mowing are typical measures to re-create species-rich grasslands. The aim of this study was to use vascular plants and three insect taxa (leafhoppers, true bugs, and grasshoppers) as bioindicators to evaluate the success of montane grassland restoration on former spruce forests in Central Europe. In addition, we intended to identify the drivers of species richness within the studied grasslands in order to derive suitable recommendations for habitat management.
Methods: We analyzed two different treatments: (i) grazed restoration sites where trees had been cut and species-rich green hay had been applied (N = 9) and (ii) target sites with a long continuity of low-intensity grazing (N = 9).
Results and Discussion: Our study revealed that all studied taxa responded rapidly to the restoration measures. After a development period of 3 to 5 years, we found no differences in species richness and diversity of leafhoppers, true bugs and grasshoppers (all, target and threatened species). In addition, non-metric multidimensional scaling showed a large overlap in species composition between restoration and target grasslands. Among target and threatened species, vascular plants displayed the same pattern as insects and reached similar values when comparing the two treatments. However, total species richness and diversity of vascular plants were still higher on the target sites and species composition overlapped only partially. Grazing intensity was the predictor with the highest explanatory power in multivariable (Generalized) Linear Mixed-effects Models, being negatively related to species richness of leafhoppers and true bugs. We conclude that the measures implemented were effective in re-establishing target communities of different taxa. The transfer of seed-containing hay enabled or accelerated the development of the vegetation. Insects, on the other hand, were able to recolonize the restored grasslands on their own, given that these sites were embedded in a network of species-rich grasslands. With regard to insects (e.g., leafhoppers and true bugs), it should be ensured that grazing is applied at low intensity.
Introduction
For centuries, European landscapes were shaped by traditional land-use practices (Plieninger et al., 2006; Eriksson, 2021; Fartmann, 2023). Small-scale low-intensity farming created a wide variety of interconnected habitats, considerably fostering biodiversity (Plieninger et al., 2006; Halada et al., 2011). With the onset of industrialization, however, drastic changes in land use were ushered in throughout Europe, accelerating from the mid-20th century onwards (Fartmann, 2023). A wide range of organizational and technological innovations, such as land consolidation instruments, progressive mechanization and the increasing use of artificial fertilizers, enabled large-scale intensification of agriculture on productive soils (Stoate et al., 2001; Robinson and Sutherland, 2002; Kleijn et al., 2009). By contrast, marginal land that became increasingly unviable for agricultural use was often abandoned or afforested (Henle et al., 2008; Fartmann, 2023). The transformation from traditional to industrial agricultural landscapes is today considered the major driver of the dramatic decrease in farmland biodiversity across Europe (Plieninger et al., 2006; Pereira et al., 2012; Fartmann, 2023).
Temperate, semi-natural grasslands, arising from traditional management, are very rich in plant and animal species and therefore have an outstanding value for nature conservation (Bonari et al., 2017; Feurdean et al., 2018; Fartmann et al., 2021). However, for decades, they have suffered particularly from persistent pressure exerted by land-use change, which has resulted in continuous habitat loss, deterioration and fragmentation (Isselstein et al., 2005; Fartmann et al., 2021; Fartmann, 2023). The contrasting drivers of this development have been unevenly distributed along elevation gradients: the conversion of semi-natural grassland to cropland and the intensification of management have been more pronounced in lowlands, whereas spontaneous (i.e., succession after abandonment) and artificial afforestation on former grasslands have especially taken place at higher elevations (Mottet et al., 2006; Fartmann, 2023).
In Central European low mountain ranges, montane grasslands in particular have been widely planted with introduced Norway spruce (Picea abies; Hölzel and Tischew, 2019). This has led to rapid changes in habitat conditions. Following site preparation, trees severely alter light and soil conditions as they grow, hampering the development of ground vegetation (Strand et al., 2021). Grassland plant and animal species not only lose habitat but are also hindered in dispersal between remaining habitat patches (Poniatowski et al., 2016). Consequently, the conversion of grasslands to shady high forests, primarily intended to produce firewood and timber, has caused a strong decline in montane species, and semi-natural montane grasslands are seriously threatened today (Finck et al., 2017; Hölzel and Tischew, 2019).
Besides preserving and improving extant fragments of semi-natural montane grasslands, habitat restoration is a key component of nature conservation for increasing biodiversity. In the case of spruce forests, restoration measures include clear-cutting and subsequent tillage. However, the soil seed bank is often depleted after decades of forest use and spontaneous recolonization by dispersing propagules is limited for many plant species (Bakker et al., 2002; Kiehl et al., 2010). Target species are therefore actively introduced by transferring green hay or brush-harvested seeds from nearby species-rich donor grasslands to restoration sites (Kiehl et al., 2010; Durbecq et al., 2022).
However, the success of implemented measures varies between restoration projects as it depends on several factors, such as timing, species composition of donor sites, soil conditions and effectiveness of reintroduced habitat management (Kiehl et al., 2010; Wagner et al., 2020). A careful evaluation of habitat restoration is thus essential. Contrary to common practice, evaluation studies should ideally focus not only on plants but also include animals, as otherwise there is a risk of overestimating restoration success (Baur, 2014; McAlpine et al., 2016). Although plant recovery is an important prerequisite for animal recolonization, sites may lack suitable habitat quality in terms of microclimate or vegetation structure (Jones and Davidson, 2016; Helbing et al., 2021). Moreover, dispersal-limited species often fail to reach restoration sites spontaneously, especially when dispersal corridors are limited (Baur, 2014; Poniatowski et al., 2016). This applies in particular to arthropods that are major drivers of ecosystem functioning in semi-natural grasslands (Soliveres et al., 2016; Poniatowski et al., 2018; Neff et al., 2020). Studies evaluating both the flora and arthropod fauna of restored montane grasslands, though, are still rare (but see Kurtogullari et al., 2020; Neff et al., 2020). In addition, research to date has tended to focus on the restoration of montane grassland habitats in open areas (e.g., Lencová and Prach, 2011; Sullivan et al., 2020; Durbecq et al., 2022), whereas restoration projects that involve clearing spruce forests are still understudied (but see Dassonville et al., 2013).
The objective of this multi-taxon study was to use vascular plants and three insect taxa (leafhoppers [Hemiptera: Auchenorrhyncha], true bugs [Hemiptera: Heteroptera] and grasshoppers [Orthoptera]) as bioindicators to evaluate the success of montane grassland restoration on former spruce forests in a Central European low mountain range. We compared species richness, diversity and composition of grazed restoration sites and nutrient-poor pastures with long-term grazing history (target grassland). The studied insect taxa (i) play an important role both as consumers and as an abundant food source for insectivores in grassland ecosystems, (ii) occupy different ecological niches, (iii) respond quickly to habitat changes and (iv) allow standardized sampling (Kőrösi et al., 2012; Helbing et al., 2020; Fartmann et al., 2022c). With a development period of 3 to 5 years after the implemented measures, we studied the short-term effects of restoration.
In particular, we addressed the following questions:
(i) Do environmental conditions in restored grasslands differ from those in target grasslands?
(ii) What are the effects of restoration measures on species richness and diversity of vascular plants and insects (all, target and threatened species), and how do species compositions differ between both types of studied grasslands?
(iii) Which environmental parameters explain species richness of vascular plants and insects within the studied grasslands, and, on the basis of the results, which recommendations for further habitat management can be given?
Materials and methods
Study area
The study area is located in the Rothaar Mountains, a low mountain range at the northwestern edge of the German uplands (51°11′17 N, 8°32′25 E; Figure 1). It covers an elevation range from 450 to 842 m a.s.l. and is characterized by a cool (mean annual temperature: 5°C) and humid (mean annual precipitation: 1,450 mm) montane climate with snowy winters (mean snow-cover duration: 100 days/year; Fartmann et al., 2022b). Oligotrophic cambisols on acidic bedrock are the prevalent soils (Geologisches Landesamt Nordrhein-Westfalen (NRW), 1998). Interspersed with grasslands, the landscape is dominated by Norway spruce forests (Picea abies), which are non-native to the study region. Although agriculture has been partially intensified here as well, montane grasslands that are grazed or mown at low intensity are still widespread. These comprise mountain hay meadows (order: Arrhenatheretalia, alliance: Polygono-Trisetion) and species-rich Nardus grasslands (order: Nardetalia strictae, alliance: Violion caninae), both of which are protected under the EU Habitats Directive (92/43/EEC, habitat types 6520 and 6230).
Within the study area, a large-scale EU LIFE project (“Mountain hay meadows near Winterberg”) was carried out from 2011 to 2016. It aimed to restore, improve and protect mountain hay meadows and species-rich Nardus grasslands. The project measures included the conversion of spruce forests into species-rich grasslands (Schulte, 2017). After the conduction of the restoration measures, the grasslands have been either grazed or mown at low intensity. In this study, we only considered grazed grasslands that were restored on former spruce forests.
Restoration methods
The restoration measures were conducted 3 to 5 years prior to our study (i.e., between 2012 and 2014) and are described in detail in Schulte (2017). Overall, the restoration comprised four steps: (i) deforestation of spruce forests, (ii) removal of remaining branches, (iii) forestry mulching, and (iv) transfer of autochthonous green hay from adjacent donor sites. Only in exceptional cases was the soil additionally milled and harrowed. Long-existing Nardus grasslands were rarely accessible by heavy machinery due to topographic and edaphic constraints. Thus, oligotrophic hay meadows with a high number of typical Nardus grassland species were mostly chosen as donor sites. For the green hay transfer, these were mown once in early to mid-August of the respective year with a rotary disc mower. The hay was then transported and spread onto the recipient sites using a forage wagon (application rate of 1:1). Only on steeper slopes was spreading by hand necessary.
Study sites and plots
The effectiveness of the restoration measures was evaluated by a comparative approach. We analyzed two treatments:
(i) Montane grasslands where restoration measures described above were carried out and which have since been managed as rough pastures (restored grassland, N = 9, mean size: 0.66 ± 0.11 ha, Figures 2A,B).
(ii) Species-rich pastures with a long continuity of low-intensity grazing. These include Nardus grasslands and closely related oligotrophic grassland communities. The pastures are characterized by a high phytodiversity and represent the target state of the restoration of grazed grasslands in the study area (target grassland, N = 9, mean size: 1.69 ± 0.39 ha, Figures 2C,D).
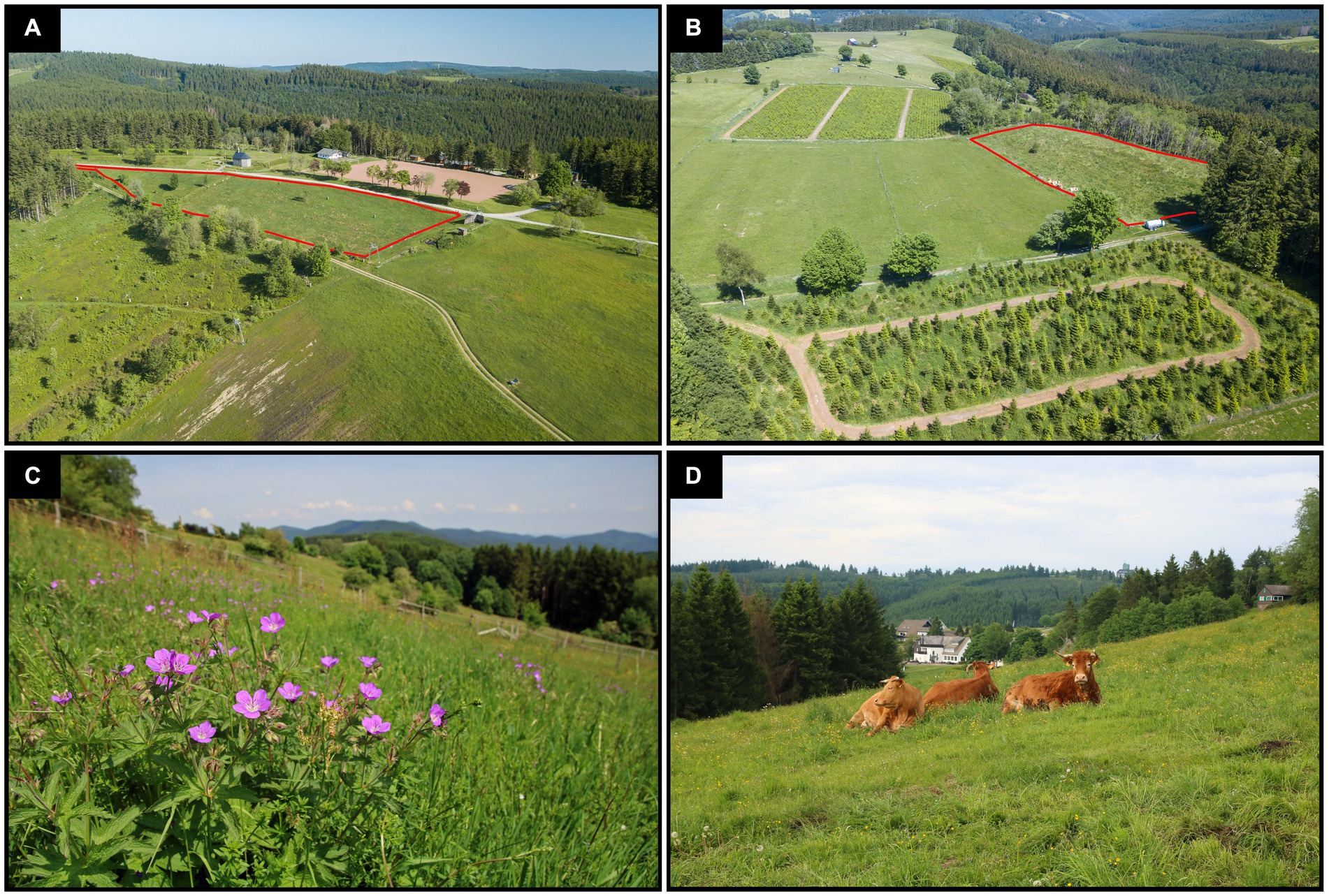
Figure 2. Impressions of the study area: (A,B) aerial views with restored grasslands framed in red (photos: F. Helbing), (C) species-rich pasture with long-term grazing history inhabited by Geranium sylvaticum (photo D. Poniatowski) and (D) long-term pasture grazed by red cattle (photo D. Poniatowski).
The majority of the sites were grazed by cattle (61%), followed by horses (28%) and goats (11%). In the center of each site, we established a rectangular plot with a total size of 500 m2 and a distance of at least 10 m to the site boundary (to avoid edge effects; cf. Kurtogullari et al., 2020). The plots were marked with magnets and tent pegs, which were placed in the ground together. With the help of a magnetic detector, the locations were accurately retrieved during subsequent visits. In order to address potential issues with spatial autocorrelation, the study area was divided into five subareas, each comprising at least one restored grassland site (Figure 1, see also the “Statistical analysis” section: LMM and GLMM with random effects). We attempted to balance the number of sites of the two treatments within each subarea. However, due to the limited availability of suitable pastures, this was not feasible in subarea IV. We therefore increased the number of studied target grasslands in the adjacent subarea V.
Species sampling and classification
We sampled vascular plants and insects in 2017. Insect sampling was always conducted under favorable weather conditions (dry and sunny days with temperatures > 15°C). Depending on the phenology of each species group, the period and frequency of the surveys varied. In order to evaluate the effects of grassland restoration, we classified three groups of vascular plants and insects: all, target and threatened species. Criteria for defining target species were determined individually for each species group and are described below. If available, we obtained the threat status of the species from the latest red data books of the federal state North Rhine-Westphalia (vascular plants, grasshoppers), otherwise red data books of Germany (leafhoppers, true bugs) were used. Hereinafter, species that are listed as “critically endangered,” “endangered,” “vulnerable,” or “near threatened” (red-list categories: 1, 2, 3 and V) are termed “threatened species.” Literature on species identification, scientific nomenclature and the threat status of the studied taxa is listed in Supplementary material.
Vascular plants
We established a subplot covering 16 m2 (4 m × 4 m) in the center of each plot to record vascular plant species richness and cover. The main vegetation survey was carried out in early June. In early August, we revisited the subplots to search for late-flowering plants such as eyebright (Euphrasia spp.). Species cover was estimated according to the Wilmanns scale (Wilmanns, 1998). For statistical analyses, the categories were translated into percentage-cover values: r = 0.1%, + = 0.5%, 1 = 2.5%, 2 m = 2.5%, 2a = 10%, 2b = 20.5%, 3 = 38%, 4 = 63%, and 5 = 88% (Dierschke, 1994).
In addition to the quantitative data gathered within the subplots, we searched the 500 m2 plots in their entirety for additional plant species during both surveys. From the resulting species list, we determined the host plants of sampled leafhoppers (Nickel and Remane, 2002; Nickel, 2003) and true bugs (Wachmann et al., 2004, 2006, 2007, 2008) and used their counts as explanatory variables (see the “Statistical analysis” section). Both the donor and target sites were characterized by plant species typical of Nardus grasslands as well as mountain hay meadows. Thus, we defined all characteristic species of the phytosociological class Nardo-Callunetea, the alliance Polygono-Trisetion and their respective subunits as target species (Dierschke, 1997; Oberdorfer, 2001; Peppler-Lisbach and Petersen, 2001; Supplementary Table 1).
Leafhoppers and true bugs
Leafhoppers and true bugs were surveyed together three times in early June, mid-July and early September. We combined a sweep net (30 cm diameter) and a G-Vac suction apparatus (Stihl SH 56, Waiblingen, Germany; 12.5 cm diameter suction tube; 710 m3h−1 air flow rate) with a fine gauze collection bag (300 μm mesh size) on the inner side of the inlet nozzle to cover all available microhabitats and sample the complete range of herb- and ground-dwelling species (cf. Stewart, 2002; Helbing et al., 2020). We walked in loops over the whole plot and randomly performed 50 sweep net strokes and 50 suction samples. The latter was done by holding the nozzle onto the ground for 10 s (cf. Helbing et al., 2021). The catches were killed with ethyl acetate and identified in the laboratory using a digital microscope (leafhoppers: Keyence VHX-900F, Osaka, Japan) or binoculars (true bugs: Helmut Hund 7–90×, Wetzlar, Germany). Data from both sampling techniques were pooled for statistical analyses. For some genera, identification of females at the species level is not possible (e.g., Aphrodes, Psammotettix, Ribautodelphax). These females were identified at the genus level or, if present, assigned to the corresponding males. In the case of more than one species of a genus, the number of females was assumed to correspond to the number of males (cf. Helbing et al., 2021). We defined typical inhabitants of semi-natural grasslands as target species. For leafhoppers, we listed all sampled grassland species that avoid intensive to very intensive management, based on Nickel and Achtziger (1999). From this list, we then removed species that occur exclusively on unmanaged or wet grasslands, as well as pioneer species (Nickel and Achtziger, 1999; Nickel, 2003; Supplementary Table 2). True bug target species were classified according to Wachmann et al. (2004, 2006, 2007, 2008). We included all species that primarily occur on grasslands with a low management intensity except for typical inhabitants of fringe and wetland habitats as well as pioneer species (Supplementary Table 3).
Grasshoppers
Grasshopper sampling took place once per plot in early August. We used a box quadrat (1.41 × 1.41 m ≙ 2.0 m2), which is a well-approved and accurate tool for surveying species richness and abundance of grasshoppers (Gardiner and Hill, 2006). In each plot, we randomly dropped the box quadrat at 10 different points, covering a total area of 20 m2 (cf. Fartmann et al., 2022a). Species were identified in the field and released afterwards. As with leafhoppers and true bugs, we intended to classify grasshopper species typical of semi-natural grasslands as target species. Poniatowski et al. (2020) presented a species farmland index (SFI) for German grasshopper species that measures the average availability of high nature value farmland (HNV) within a species’ range. HNV is a term used to describe biodiversity-rich farming systems, usually resulting from low-intensity traditional land-use practices (Lomba et al., 2014). Habitat specialists that strongly depend on open, semi-natural habitats receive high SFI values, whereas values for habitat generalists are low. In this study, we classified all species with an SFI value of at least 17.0 as target species (Supplementary Table 4).
Environmental conditions
Local climate
We determined the mean elevation (m a.s.l.) of the study sites from topographic maps using the geographical information system ArcGIS 10.6. Moreover, long-term averages of annual temperature (°C) and precipitation (mm; period 1981–2010) were derived from grid maps with a spatial resolution of 1 km2 (German Meteorological Service, 2022). If sites covered more than one grid cell, we calculated mean values.
During fieldwork, we measured aspect and slope of the plots. In combination with the latitude of the study area (51°), these data were used to calculate the potential amount of direct incident radiation (in MJ cm−2 yr.−1) as an approximate measure of the local climate (McCune and Keon, 2002).
Habitat characteristics
We calculated the size of the study sites (ha) using orthophotos in ArcGIS 10.6. The intensity of grazing was determined on a metric scale. Combining own observations and information provided by farmers, we recorded the composition of grazing livestock and the duration of grazing (with an accuracy of 0.5 months) for each site during the 2017 season. Each type of animal (species and age) was converted into a livestock unit (LSU) using a conversion key for North Rhine-Westphalia (Table 1; LANUV (Landesamt für Natur, Umwelt und Verbraucherschutz Nordrhein-Westfalen), 2022). The grazing intensity of a site was calculated as the product of the density of livestock (summed LSU per hectare) and the months of grazing (MoG).
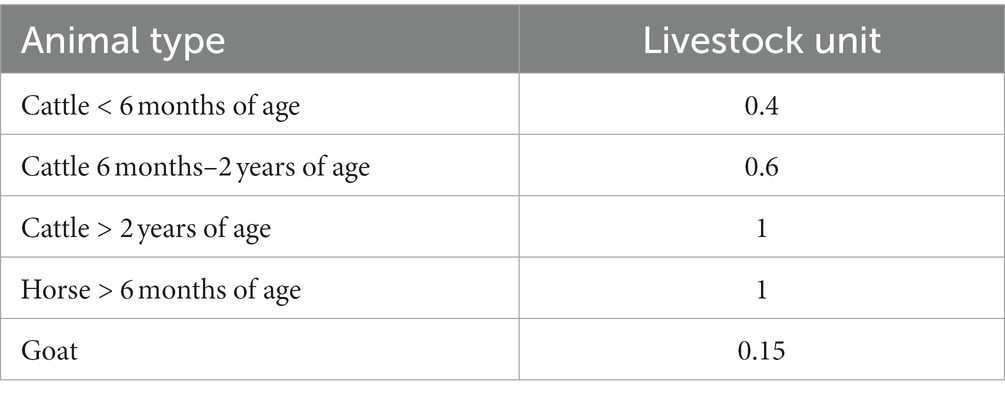
Table 1. Conversion key for the calculation of livestock units per site [LANUV (Landesamt für Natur, Umwelt und Verbraucherschutz Nordrhein-Westfalen), 2022].
In the course of the main vegetation survey in June 2017, we recorded parameters of horizontal and vertical habitat structures within each subplot. We estimated the cover of grasses, herbs, cryptogams, bare soil and litter with an accuracy of 5%. If values were above 95% or below 5%, we used steps of 2.5% (Helbing et al., 2021). The vegetation height of the field layer was measured with an accuracy of 2.5 cm. To characterize soil conditions, we also calculated Ellenberg’s indicator values for vascular plants (Ellenberg et al., 2001; mean values for moisture, nitrogen, and soil reaction).
Statistical analysis
Comparison of habitat types
All statistical analyses were performed using R 4.1.2 (R Core Team, 2022). We quantified the taxonomic richness and diversity of vascular plants and insects using Hill numbers, which provide a mathematically unified set of diversity indices in units of species (Hill, 1973; Jost, 2006; Chao et al., 2014). The sensitivity of these indices to species relative abundances is controlled by the order q (Chao et al., 2014). We considered Hill numbers at q = 0, which represents species richness, and at q = 1, which is equivalent to the exponential of Shannon entropy (hereinafter termed “diversity”; Jost, 2006; Chao et al., 2014). We tested for significant differences in environmental conditions, species richness and diversity between restored and target grasslands. For the former, we used the Mann–Whitney U-test since the majority of environmental variables showed highly skewed distributions and could thus not be analyzed by mixed-effects models (cf. Helbing et al., 2021). By contrast, species richness and diversity of vascular plants, leafhoppers, true bugs and grasshoppers (all, target and threatened species) were compared via Linear Mixed-effects Models (LMM) or Generalized Linear Mixed-effects Models (GLMM) with habitat type as a nominal predictor and subarea (N = 5; see the “Study sites and plots” section) as a random intercept (R package lme4; Bates et al., 2022). For normally distributed and log-transformed variables with a normal distribution, we applied LMMs, otherwise we calculated GLMMs with a negative-binomial error structure to account for overdispersion (Richards, 2008).
Species compositions of restored and target grasslands were compared via non-metric multidimensional scaling (NMDS; R package vegan, metaMDS function; Oksanen et al., 2022) with the Bray-Curtis distance as a distance measure and a maximum number of 200 random starts in search of a stable solution. The four studied species groups were analyzed separately. We included only those species in the analyses that were sampled on at least three plots. For each species group, we performed a Permutational Multivariate Analysis of Variance with 999 permutations (PERMANOVA; Bray-Curtis distance; R package vegan, adonis2 function; Oksanen et al., 2022) to test for significant differences between restored and target grasslands.
Effects of environmental conditions on species richness of vascular plants and insects
We fitted (G)LMMs with the same random effects and error structure as described above to analyze the effects of environmental parameters on species richness of vascular plants and insects (target and threatened species). For each of the studied species groups, we included a specific and ecologically reasonable set of variables into the models (e.g., the number of leafhopper host plant species was used only to analyze leafhopper species richness, whereas Ellenberg indicator values characterizing soil conditions were included only in the modeling of vascular plant species richness). A detailed assignment of the parameters to the species groups is given in Table 2. Prior to the analyses, we standardized all fixed effects to achieve comparability of effect sizes (cf. Fumy and Fartmann, 2021). Furthermore, we graphically examined all combinations of response and predictor variables before computing the (G)LMMs. If we detected unimodal rather than linear relationships between two variables, we also entered centered and squared values of the predictor into the full model in addition to the untransformed values (cf. Kämpfer and Fartmann, 2022). This was the case for the Ellenberg nitrogen value and threatened vascular plant species. To avoid multicollinearity, we also calculated Spearman’s rank correlation (rS) for all combinations of predictor variables (Dormann et al., 2013). In cases where two or more variables were strongly intercorrelated (|rS| ≥ 0.6, p < 0.05), only one was included in statistical modeling (Supplementary Table 5). We applied model averaging based on an information-theoretic approach for all multivariable analyses (Grueber et al., 2011). In a first step, all possible combinations of environmental parameters were tested, resulting in a set of different candidate models. These candidate models were then ranked based on Akaike’s information criterion (AICC) using the “dredge” function (R package MuMIn; Bartoń, 2022). Finally, the top-ranked models within ΔAICC < 2 were used for model averaging (Grueber et al., 2011). We evaluated the explanatory power of the models by calculating marginal R2 (variance explained by fixed effects) and conditional R2 (variance explained by both fixed and random effects; Nakagawa et al., 2017). The p-values for LMMs were determined with the R package “lmerTest” (Kuznetsova et al., 2022).
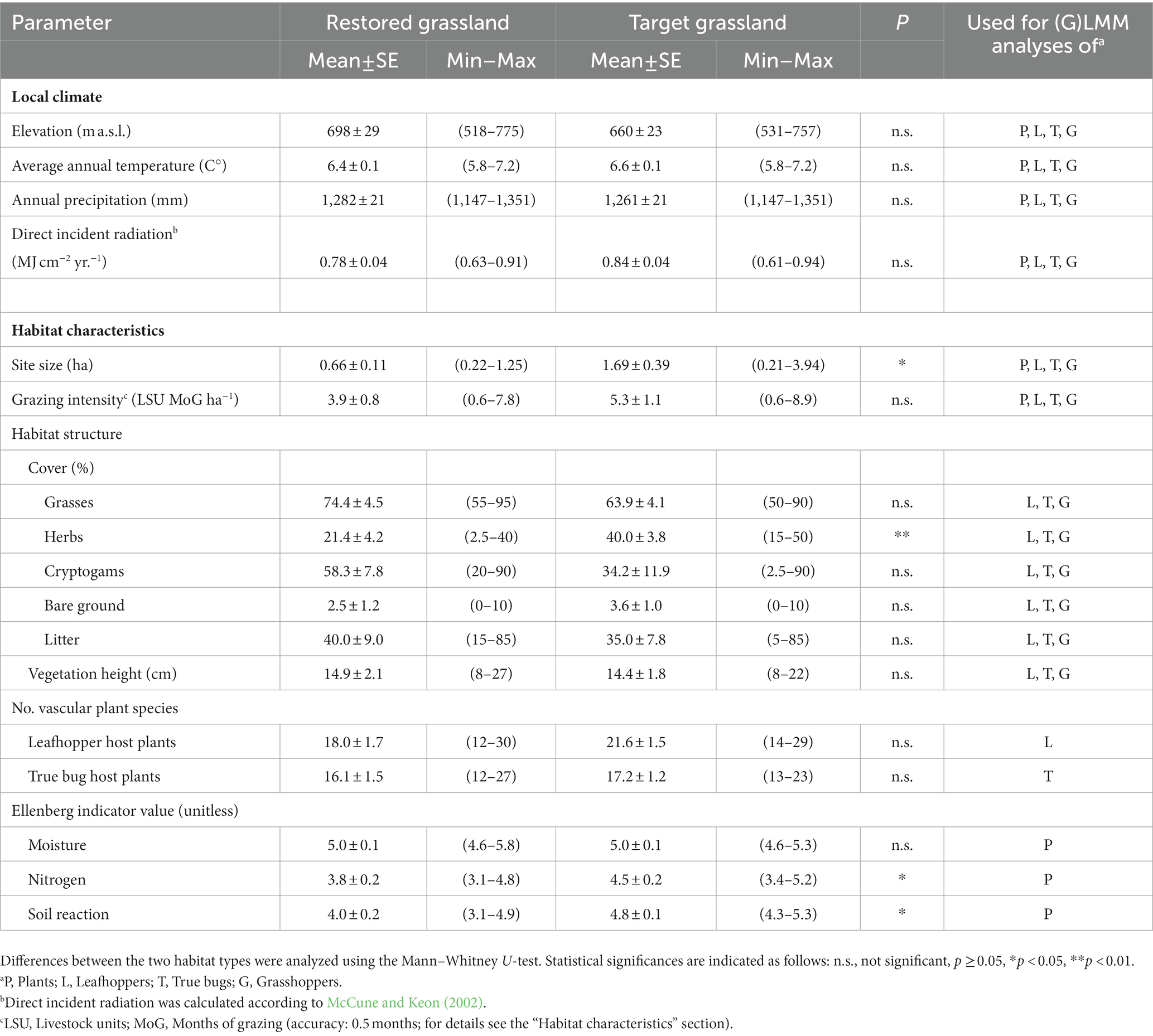
Table 2. Metric environmental parameters [mean ± standard error (SE) as well as minimum (Min) and maximum (Max) values] in restored (N = 9) and target (N = 9) grasslands.
Results
Environmental conditions
Restored and target grasslands differed only slightly in environmental conditions (Table 2). Local climate, grazing intensity, host plants of leafhoppers and true bugs as well as most parameters of habitat structure did not differ. Restored grasslands were merely smaller, had a lower cover of herbs and lower Ellenberg indicator values for nitrogen and soil reaction.
Species richness, diversity, and composition of vascular plants and insects
We sampled a total of 267 species in the four species groups (Table 3; Supplementary Tables 1–4). Vascular plants and leafhoppers were the most species rich taxa, followed by true bugs and grasshoppers. On average, 16% of the species per group were classified as threatened (ranging between 8% in true bugs and 29% in grasshoppers) and 34% as target species (ranging between 15% in vascular plants and 58% in leafhoppers). With a frequency of 100% in both studied habitat types, Agrostis capillaris, Festuca rubra agg., Luzula campestris, and Rumex acetosa were the most widespread vascular plant species in our study. The most abundant species of leafhoppers were Deltocephalus pulicaris (NIndividuals = 4,051; 22%) and Verdanus abdominalis (NIndividuals = 3,451; 18%), those of true bugs were Pachytomella parallela (NIndividuals = 955; 46%) and Nabis flavomarginatus (NIndividuals = 385; 19%), and those of grasshoppers were Chorthippus biguttulus (NIndividuals = 512; 45%) and Pseudochorthippus parallelus (NIndividuals = 414; 36%).
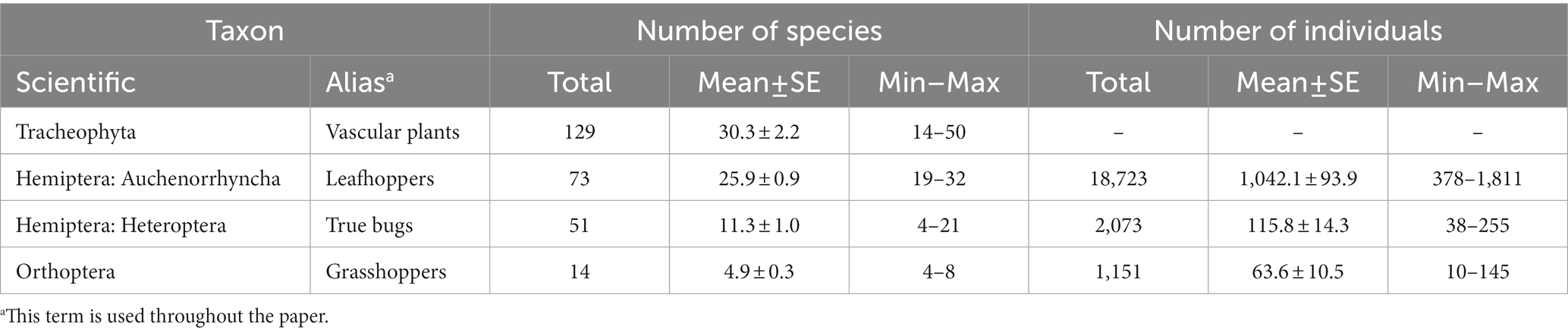
Table 3. Overview of the studied taxa with numbers of species and individuals [total, mean ± standard error (SE) as well as minimum (Min) and maximum (Max) values] per taxon (Nsites = 18).
When comparing restored and target grasslands, no differences in species richness and diversity of leafhoppers, true bugs and grasshoppers (all, target and threatened species) were found (Figures 3C–H). This was also true for target and threatened species of vascular plants (Figures 3A,B). It was only the total species richness and the total species diversity of vascular plants that were higher in target than in restored grasslands (Figures 3A,B). NMDS revealed similar patterns in terms of species composition. There was a large overlap in leafhoppers (F1,16 = 1.39, p = 0.20) and grasshoppers (F1,16 = 1.13, p = 0.34) between restored and target grasslands (Figures 4B,D). True bugs also exhibited similarity between the two grassland types, but only for the centrally located target grasslands, while other target grasslands were more dispersed (F1,16 = 4.20, p < 0.01; Figure 4C). Vascular plants showed some overlap between restored and target grasslands as well, but overall, they were more segregated from one another (F1,16 = 4.28, p < 0.01; Figure 4A).
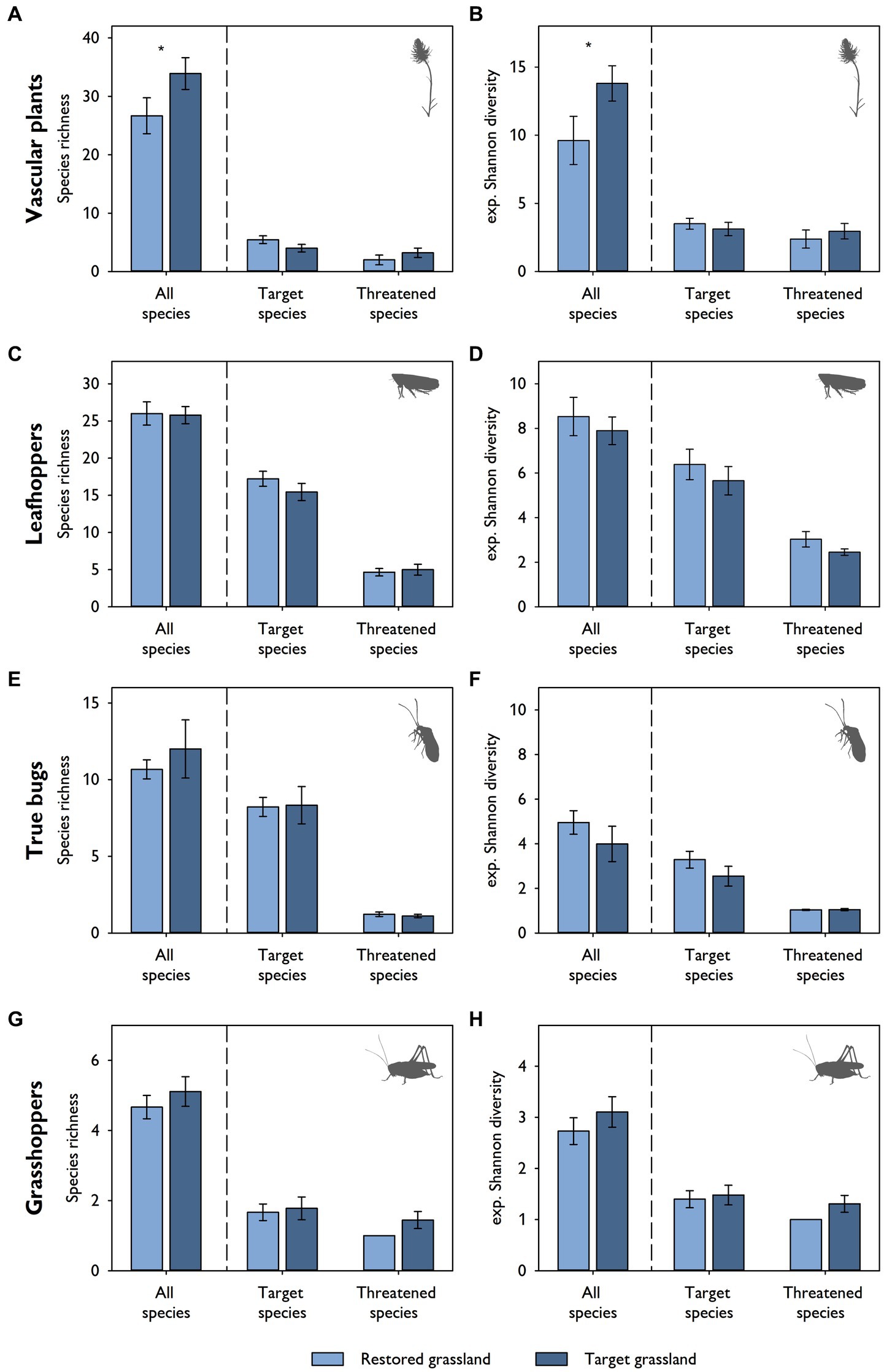
Figure 3. Mean values (± standard error) of species richness (Hill numbers of order q = 0) and diversity (expressed as exponential Shannon diversity; Hill numbers of order q = 1) for all, target and threatened species of (A,B) vascular plants, (C,D) leafhoppers, (E,F) true bugs, and (G,H) grasshoppers in restored (N = 9) and target grasslands (N = 9). Asterisks indicate a significant difference between the two habitat types (tested via mixed-effects models with subarea [N = 5] as a random intercept; *p < 0.05).
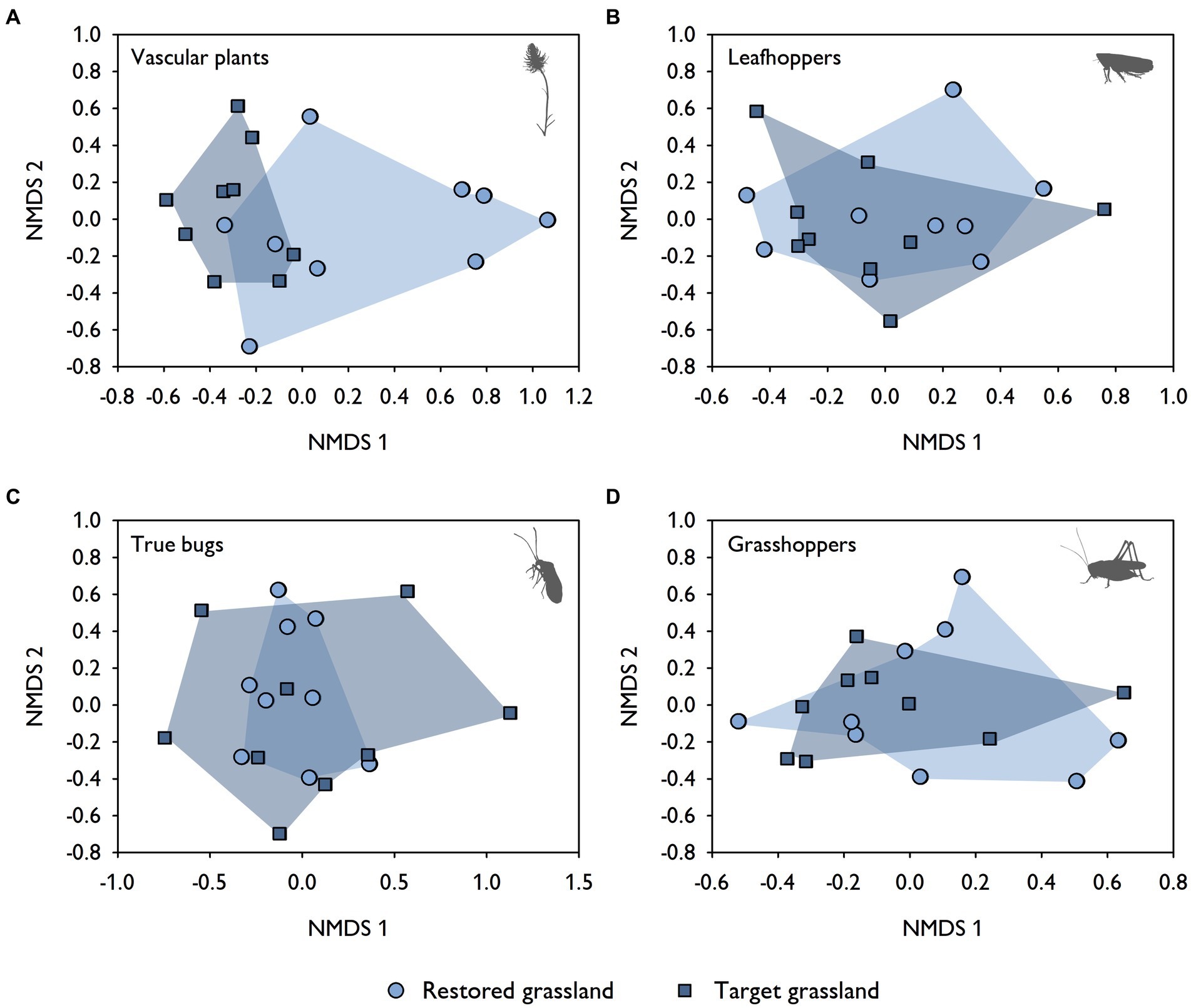
Figure 4. NMDS ordinations (Bray–Curtis distance, four dimensions) for assemblages of (A) vascular plants (based on 65 species, stress = 0.06), (B) leafhoppers (based on 47 species and 18,498 individuals, stress = 0.10), (C) true bugs (based on 22 species and 2,003 individuals, stress = 0.09), and (D) grasshoppers (based on 8 species and 1,133 individuals, stress = 0.05) across restored (N = 9) and target grasslands (N = 9).
Response of vascular plants and insects to environmental conditions
The (G)LMM analyses identified effects of environmental parameters on species richness of vascular plants, leafhoppers and true bugs. The number of vascular plant target species increased with annual precipitation and decreased with Ellenberg nitrogen values (Table 4A; Figures 5A,B). By contrast, the number of threatened vascular plant species was highest at intermediate Ellenberg nitrogen values (Table 4B; Figure 5C). Grazing intensity was the parameter with the highest explanatory power in the multivariable analyses for two of the three insect groups: it negatively affected the species number of leafhoppers (target and threatened species) and true bugs (target species; Tables 4C–E; Figures 5D,E,H). Moreover, direct incident radiation had a positive effect on the number of threatened leafhopper species (Table 4D; Figure 5F) and the number of true bug host plant species was positively related to the number of true bug target species (Table 4E; Figure 5G). For the species richness of grasshoppers (both target and threatened species) and threatened true bugs, there was no relationship with any of the parameters analyzed. The explanatory power of all models was generally high (R2m = 0.30–0.54; R2c = 0.30–0.63).
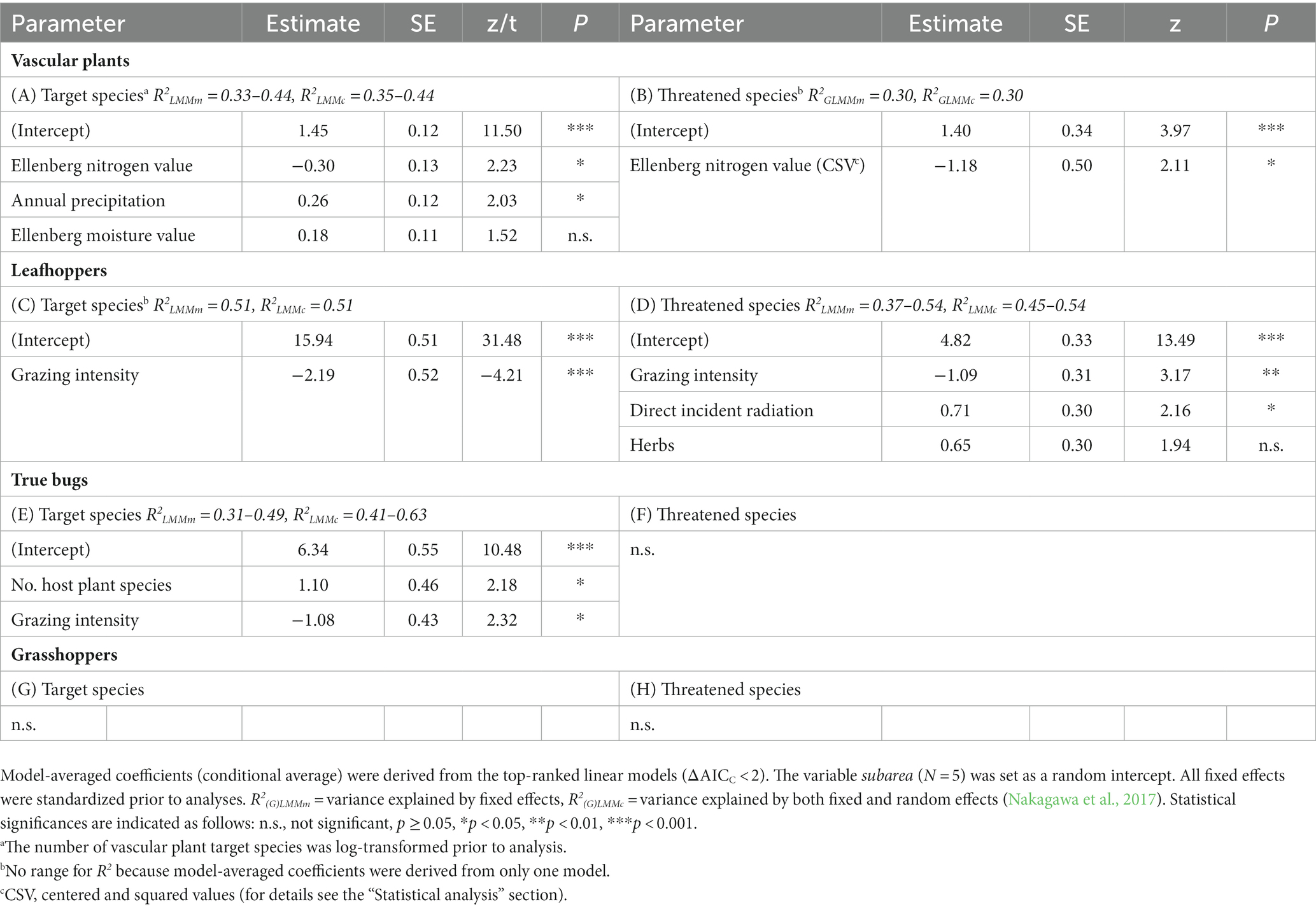
Table 4. Model-averaging results: influence of environmental parameters (predictor variables) on species richness (Hill numbers of order q = 0; target and threatened species) of (A,B) vascular plants, (C,D) leafhoppers, (E,F) true bugs, and (G,H) grasshoppers, analyzed with Linear Mixed-effects Models (LMM) (A,C,D,E) and Generalized Linear Mixed-effects Models (GLMM; negative binomial error structure) (B,F,G,H).
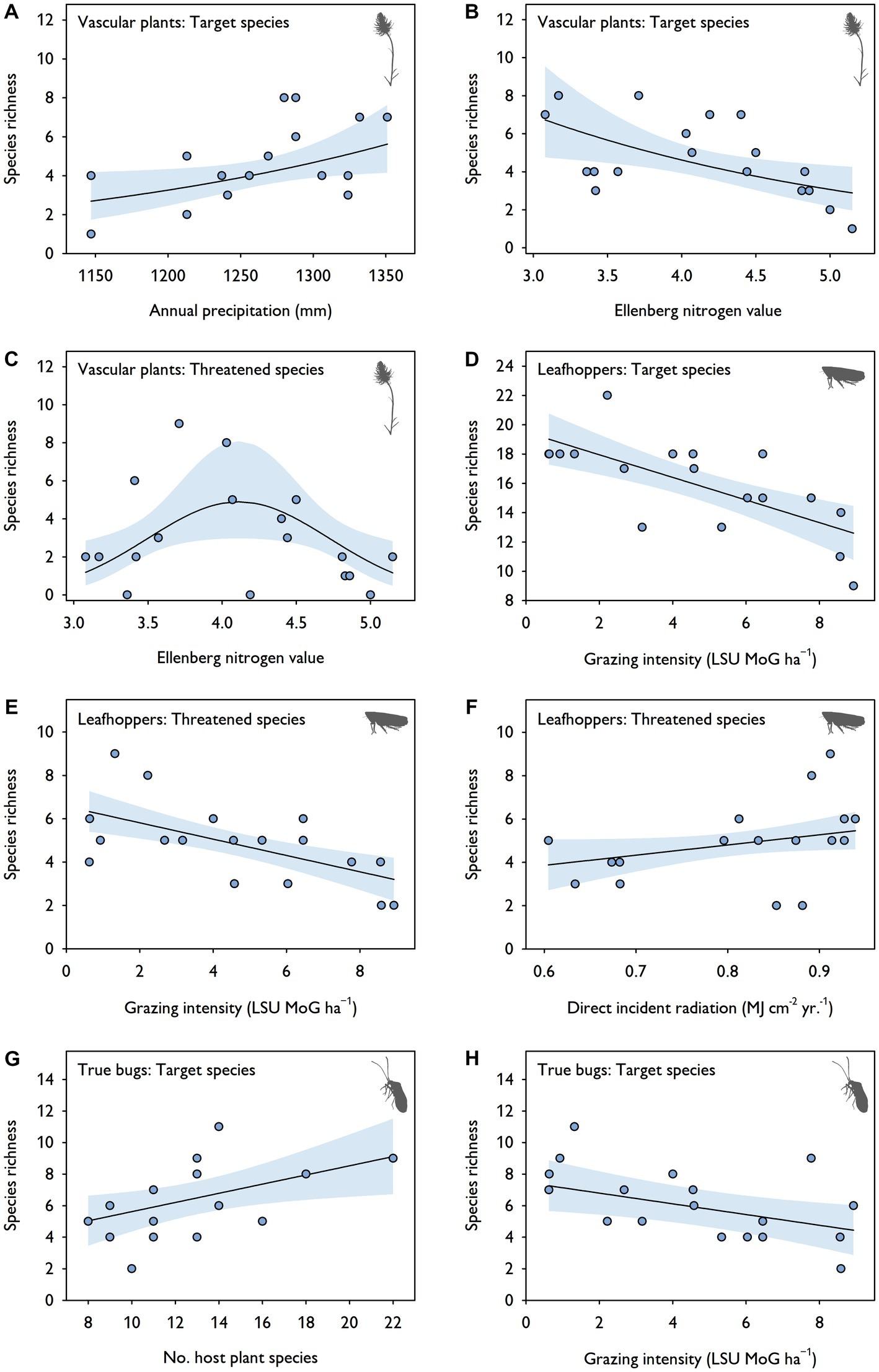
Figure 5. Results of mixed-effects model analyses: relationship between significant environmental parameters of the averaged synthesis models and species richness (Hill numbers of order q = 0; target and threatened species) of (A–C) vascular plants, (D–F) leafhoppers, and (G,H) true bugs. The regression slopes were fitted using multivariable linear models (see Table 4 for detailed model statistics). No relationships are presented for threatened true bugs and for grasshoppers as the analyses did not reveal any significant parameters. Blue bands indicate 95% confidence intervals.
Discussion
Evaluation of restoration measures
Our study revealed that vascular plants and insects (leafhoppers, true bugs, and grasshoppers) responded rapidly to the restoration of species-rich montane grasslands on former spruce forests. After a development period of 3 to 5 years, restored and target grasslands showed a large overlap in the composition of insect species. Furthermore, the studied insect groups neither differed in species richness nor in diversity of all, target and threatened species between the two grassland types. In vascular plants, the same was true for species richness and diversity of target and threatened species. By contrast, total species richness and diversity of vascular plants was still higher in target grasslands and species composition overlapped only partially.
The transfer of seed-containing green hay is an effective method to restore species-rich semi-natural grasslands (Kiehl et al., 2010; Durbecq et al., 2022). Nearby donor sites ensure the preservation of the genetic identity of local plant communities (Albert et al., 2019). In addition, the plant material is usually cut close to the soil surface, enabling the transfer of low-growing plant species as well, and the hay protects seedlings from solar radiation, drought and the competition of ruderal plants (Kiehl et al., 2010; Durbecq et al., 2022). However, a certain proportion of species usually fail to establish on the restoration sites when green hay is applied (Kiehl et al., 2010; Wagner et al., 2020). As we discuss in the following: (i) limitations inherent to the transfer method and (ii) microsite limitations are the key constrains (Kiehl et al., 2010; Wagner et al., 2020).
The study by Wagner et al. (2020) showed that species with low abundance on a donor site often have a reduced establishment rate on a restoration site. This was probably also the case for some species in the study area. For example, Nardus stricta, the eponymous species of Nardus grasslands, was absent from most donor sites and was therefore rarely transferred to the restoration sites (Schulte, 2017). Moreover, the timing of hay cutting is always a compromise that may exclude species with an unusual phenology from the transfer of mature seeds (Bakker et al., 2002; Sullivan et al., 2020). In our study, we recorded low establishment rates for several late flowering species such as Knautia arvensis and Sanguisorba officinalis. This might be explained by the mowing in early to mid-August and hence before seed development had been completed (see also Schulte, 2017; Sullivan et al., 2020).
As with vascular plant and insect species, our study revealed a high degree of similarity between restored and target grasslands in terms of environmental conditions. However, the cover of herbs and Ellenberg indicator values for nitrogen and soil reaction were still lower on the restored grasslands, i.e., on sites that had been covered with spruce forests for decades. Spruce forests are typically characterized by low light availability at the ground and a pronounced layer of needles and other tree components with a limited decomposition rate (Janišová et al., 2007; Fischer and Fischer, 2012). As a consequence, the soil is subject to acidification, a reduced microbial activity and an increased C/N ratio (Fischer and Fischer, 2012; Strand et al., 2021). Additionally, in our case, the restoration sites were only mulched after the trees had been removed, usually without further tillage. Particularly near the mulched tree stumps, this measure produced a layer of wood chips with presumably similar chemical properties as the litter layer (Schulte, 2017). The wood chips may also have limited the direct contact of the seeds with the mineral soil (Schulte, 2017). Nevertheless, according to our results, these sites were suitable for colonization by the majority of target and threatened species adapted to oligotrophic and acidic soils (van Daele et al., 2017; Kurtogullari et al., 2020). Seedling establishment of other grassland species, however, was partially hampered by site characteristics (Roem and Berendse, 2000; Schulte, 2017). This was probably another reason for the differences in species richness, diversity and composition of all vascular plants. There were mainly herbs among the species with low establishment rates, which was also reflected by a generally lower herb cover in restored grasslands. However, the site characteristics in combination with the green hay transfer and subsequent management had the advantage that nitrophilous and ruderal species occurred only rarely on the restored grasslands. In other restoration projects, especially on fertile soils such as on arable land or improved grassland, they may reach high cover and jeopardize the success of the restoration (cf. Kiehl et al., 2010; Lencová and Prach, 2011).
Similar to plants, the recolonization of a restoration site by insects mainly depends on (i) the availability of species-rich sites with abundant source populations in the surroundings and (ii) a suitable habitat quality (Keene et al., 2020; Helbing et al., 2021). The dispersal ability of the studied insect taxa is largely species-specific. On the one hand, numerous species are known to be highly mobile, being able to move long distances either actively or by passive drift (Reynolds et al., 2017; Florio et al., 2020; Poniatowski et al., 2020). On the other hand, there are many dispersal-limited species that rely on a high degree of habitat connectivity with suitable dispersal corridors to migrate (Nickel, 2003; Littlewood et al., 2009; Poniatowski et al., 2016, 2020). Given that the study area still contains a dense network of species-rich grasslands, we assume that even those species with low mobility were often able to reach restoration sites. One remarkable example is the endangered leafhopper target species Delphacinus mesomelas. Although long-winged individuals occasionally occur, the species is usually short-winged. Nevertheless, we sampled it in high abundance on 89% of the restored grasslands, which equals the species’ occupation rate on the target grasslands. We suppose that the transfer of the studied insect taxa with the green hay was negligible for species establishment. The number of transferred individuals was very likely rather small, particularly when mown with a disc mower (Stöckli et al., 2021). Moreover, the restoration sites were not vegetated during the conduction of the restoration measures and therefore did not yet provide a suitable habitat for typical insect species of grasslands.
The interaction of trophic resources, spatial structure of the vegetation and microclimatic conditions is often summarized as habitat quality (Mortelliti et al., 2010; Poniatowski et al., 2018; Helbing et al., 2021). Insects that reach a restoration site will only establish if their requirements in habitat quality are fulfilled (cf. Helbing et al., 2021; Hussain et al., 2022). As described above, environmental conditions 3 to 5 years after restoration hardly differed between restored and target grasslands. Thus, habitat quality was well suited for successful colonization of the restored sites by grassland insects.
Drivers of species richness
We found only minor differences between restored and target grasslands with regard to species richness of vascular plants and insects as well as environmental conditions. By contrast, the data showed greater variation in species richness (target and threatened species) within all studied grassland sites, and statistical modeling revealed several drivers responsible for this.
The species number of vascular plants was related to the Ellenberg nitrogen value, i.e., to soil fertility. In the case of target species, this relationship was negative. The majority of target species are characteristic of Nardus grasslands and are therefore adapted to very oligotrophic conditions (Ellenberg and Leuschner, 2010; van Daele et al., 2017). Only some character species of mountain hay meadows, such as Crepis mollis, grow preferentially at more mesotrophic soils. However, these species were hardly present on the study plots. The number of target vascular plant species therefore increased with decreasing soil fertility. For threatened vascular plant species, intermediate Ellenberg nitrogen values resulted in the highest species richness. This unimodal response corresponds with the intermediate productivity hypothesis: it states that only a few species can tolerate the environmental stress at very low productivity and that at the same time a few highly competitive species dominate sites with high productivity (Fraser et al., 2015).
Vascular plant species were also associated with precipitation. The higher the precipitation, the more target species occurred. We assume that an indirect relationship explains this pattern. Precipitation increased with elevation (rs = 0.63**). At higher elevations—i.e. in areas with higher precipitation—the overall intensity of land use is usually lower than at lower elevations (e.g., Strebel and Bühler, 2015; Buzhdygan et al., 2020). Accordingly, we interpret the environmental variable “precipitation” as a proxy for the overall land-use intensity.
Leafhoppers (target and threatened species) and true bugs (target species) were sensitive to grazing intensity. Although the studied gradient was rather small and did not include intensive grazing, it exhibited a negative relationship with species richness. This is typical of both taxa. Numerous studies have shown that species richness and abundance of small insects such as leafhoppers and true bugs often reach the highest values on grasslands with very low land-use intensity or even on abandoned sites (e.g., Dennis et al., 1998; Kruess and Tscharntke, 2002; Biedermann et al., 2005). The reason for this is that species-rich grassland communities characterized by the coexistence of different life strategy types rely on a pronounced field layer with a complex plant architecture (Biedermann et al., 2005; Zurbrügg and Frank, 2006; Helbing et al., 2017, 2021). Each grazing period reduces the height and structural complexity of the vegetation, leading to negative responses in numerous leafhopper and true bug species (Biedermann et al., 2005; Zurbrügg and Frank, 2006). However, very low-intensity grazing or abandonment is favorable only in the short to medium term since succession in the long run results in a lower richness of (host) plant species and an adverse (cool) microclimate (Biedermann et al., 2005). Furthermore, some habitat specialists of both taxa (Nickel, 2003; Wachmann et al., 2004, 2006, 2007, 2008) and other species groups, such as grasshoppers (Fumy et al., 2021), depend on spatial heterogeneity within grasslands, including areas of short turf and bare ground. This is best achieved through a low to intermediate intensity of grazing (van Klink et al., 2015; Fumy et al., 2021).
Threatened leafhopper species were also positively associated with direct incident radiation. Insects are thermophilic organisms, and the local climate of a habitat is an important driver of insect species richness (e.g., Kurtogullari et al., 2020). Helbing et al. (2017) studied leafhopper assemblages on acidic grasslands adjacent to the study area at slightly lower elevations (300–680 m a.s.l.). Numerous of the species detected by the authors were absent in our study. We suggest that climatic limitations may explain these differences. Some species reached their upper range limit in the study area. They probably prefer sites with a warm local climate.
The occurrence of insect herbivores is generally fostered by high plant species richness (Haddad et al., 2001; Joern and Laws, 2013). The majority of true bugs feed on plants, and many species are monophagous or oligophagous (Wachmann et al., 2004, 2006, 2007, 2008). Accordingly, our results revealed a positive relationship between the number of host plant species and the number of target species in true bugs. The same observation was made for leafhoppers in other studies (e.g., Rösch et al., 2013; Helbing et al., 2021). However, although this group of insects is entirely phytophagous and many species exhibit a high degree of host plant specialism (Nickel, 2003; Biedermann et al., 2005), we found no relationship with leafhopper host plants. This is probably a result of the different host plant preferences of leafhoppers and true bugs. For example, a total of 29 sampled leafhopper species (40%) feed on the four vascular plant species that occurred on all study plots (see the “Results” section). In contrast, only 12 true bug species (24%) are known to feed on these widespread plants. We therefore assume that the occurrence of host plants was not a limiting factor for leafhopper species in the study area.
We found no effect of environmental parameters on threatened true bugs or threatened and target grasshoppers. This was likely due to the low number of species in these groups, resulting in limited variance between the sites.
Conclusion
Based on our findings, we conclude that the recovery of species-rich communities of the studied taxa has progressed well in the study area, particularly with respect to insects. The restoration of montane grasslands is thus an important tool for increasing habitat networks and enhancing biodiversity in upland landscapes. This is particularly important in times of global warming since species adapted to cool-humid mountain climates rely on the ability to migrate between habitat patches (Freeman et al., 2018; Stuhldreher and Fartmann, 2018). However, we analyzed only the short-term effects of restoration. The development of the grassland sites should ideally be monitored in the long term. In a positive scenario, species richness and diversity will remain stable or even increase over time. Missing vascular plant species may be reintroduced through selective sowing by conservationists (Wagner et al., 2020). Moreover, species colonization is likely to progress, facilitated by the dense network of grassland patches in the study area (Waldén et al., 2017). On the other hand, target plant species that occur with few individuals on the restoration sites run the risk of not being able to persist in the long term (Kiehl et al., 2010). Moreover, atmospheric nitrogen deposition represents a continuous threat to the studied types of grassland (Bobbink et al., 2010). Insufficient management often results in the dominance of a few grass species such as Agrostis capillaris or Deschampsia flexuosa, while other species are suppressed (Stevens et al., 2004; Ellenberg and Leuschner, 2010). This also affects insects, as exemplified by true bug host plants. To counteract grass encroachment and in order to promote different insect taxa, including those sensitive to grazing intensity (e.g., leafhoppers and true bugs), lenient grazing with low stocking density is recommended. As an option, annually rotating small areas could be excluded from grazing, as this has been shown to have a positive effect on insect diversity (Kruess and Tscharntke, 2002).
Data availability statement
The raw data supporting the conclusions of this article will be made available by the authors, without undue reservation.
Author contributions
FH, TF, and DP contributed to the conception and design of the study. FH conducted the field work, identification of leafhopper species in the laboratory and statistical analysis, and wrote the first draft of the manuscript. CM performed the identification of true bug species in the laboratory. All authors contributed to the article and approved the submitted version.
Funding
This study was funded by the German Federal Agency for Nature Conservation (Bundesamt für Naturschutz; grant number 3516892017).
Acknowledgments
We sincerely thank Axel M. Schulte (Biologische Station Hochsauerlandkreis e.V.) for his dedicated assistance throughout the research work. Moreover, we are grateful to Raïna Wendland for support during fieldwork, to Herbert Nickel for assistance in the identification of difficult taxa, and to two reviewers for valuable comments on an earlier version of the manuscript. We further acknowledge support by Deutsche Forschungsgemeinschaft (DFG) and Open Access Publishing Fund of Osnabrück University. The restoration measures were co-financed by the EU LIFE project “Mountain hay meadows near Winterberg.”
Conflict of interest
The authors declare that the research was conducted in the absence of any commercial or financial relationships that could be construed as a potential conflict of interest.
Publisher’s note
All claims expressed in this article are solely those of the authors and do not necessarily represent those of their affiliated organizations, or those of the publisher, the editors and the reviewers. Any product that may be evaluated in this article, or claim that may be made by its manufacturer, is not guaranteed or endorsed by the publisher.
Supplementary material
The Supplementary material for this article can be found online at: https://www.frontiersin.org/articles/10.3389/fevo.2023.1148266/full#supplementary-material
References
Albert, Á.-J., Mudrák, O., Jongepierová, I., Fajmon, K., Frei, I., Ševčíková, M., et al. (2019). Grassland restoration on ex-arable land by transfer of brush-harvested propagules and green hay. Agric. Ecosyst. Environ. 272, 74–82. doi: 10.1016/j.agee.2018.11.008
Bakker, J. P., Elzinga, J. A., and de Vries, Y. (2002). Effects of long-term cutting in a grassland system: perspectives for restoration of plant communities on nutrient-poor soils. Appl. Veg. Sci. 5, 107–120. doi: 10.1111/j.1654-109X.2002.tb00540.x
Bartoń, K. (2022). Multi-model inference (package “MuMIn,” version 1.47.1). Available at: https://cran.r-project.org/web/packages/MuMIn/MuMIn.pdf (Accessed 21 October 2022).
Bates, D., Maechler, M., Bolker, B., Walker, S., Christensen, R. H. B., Singmann, H., et al. (2022). Linear mixed-effects models using “Eigen” and S4 (package “lme4,” version 1.1–31). Available at: https://cran.r-project.org/web/packages/lme4/lme4.pdf (Accessed 1 November 2022).
Baur, B. (2014). Dispersal-limited species—a challenge for ecological restoration. Basic Appl. Ecol. 15, 559–564. doi: 10.1016/j.baae.2014.06.004
Biedermann, R., Achtziger, R., Nickel, H., and Stewart, A. J. A. (2005). Conservation of grassland leafhoppers: a brief review. J. Insect Conserv. 9, 229–243. doi: 10.1007/s10841-005-0531-z
Bobbink, R., Hicks, K., Galloway, J., Spranger, T., Alkemade, R., Ashmore, M., et al. (2010). Global assessment of nitrogen deposition effects on terrestrial plant diversity: a synthesis. Ecol. Appl. 20, 30–59. doi: 10.1890/08-1140.1
Bonari, G., Fajmon, K., Malenovský, I., Zelený, D., Holuša, J., Jongepierová, I., et al. (2017). Management of semi-natural grasslands benefiting both plant and insect diversity: the importance of heterogeneity and tradition. Agric. Ecosys. Environm. 246, 243–252. doi: 10.1016/j.agee.2017.06.010
Buzhdygan, O. Y., Tietjen, B., Rudenko, S. S., Nikorych, V. A., and Petermann, J. S. (2020). Direct and indirect effects of land-use intensity on plant communities across elevation in semi-natural grasslands. PLoS One 15:e0231122. doi: 10.1371/journal.pone.0231122
Chao, A., Chiu, C.-H., and Jost, L. (2014). Unifying species diversity, phylogenetic diversity, functional diversity, and related similarity and differentiation measures through Hill numbers. Annu. Rev. Ecol. Evol. Syst. 45, 297–324. doi: 10.1146/annurev-ecolsys-120213-091540
Dassonville, N., Herremans, J.-P., and Tanghe, M. (2013). Valeur des coupes à blanc de pessières pour la conservation de la biodiversité en Wallonie; restauration de milieux semi-naturels à partir du stock grainier du sol. Acta Bot. Gallica 153, 201–218. doi: 10.1080/12538078.2006.10515537
Dennis, P., Young, M. R., and Gordon, I. J. (1998). Distribution and abundance of small insects and arachnids in relation to structural heterogeneity of grazed, indigenous grasslands. Ecol. Entomol. 23, 253–264. doi: 10.1046/j.1365-2311.1998.00135.x
Dierschke, H. (1997). Molinio-Arrhenatheretea (E 1) – Kulturgrasland und verwandte Vegetationstypen, Teil 1: Arrhenatheretalia – Wiesen und Weiden frischer Standorte. Syn. Pflanzenges. Deutschl. 3, 1–74.
Dormann, C. F., Elith, J., Bacher, S., Buchmann, C., Carl, G., Carré, G., et al. (2013). Collinearity: a review of methods to deal with it and a simulation study evaluating their performance. Ecography 36, 27–46. doi: 10.1111/j.1600-0587.2012.07348.x
Durbecq, A., Rocher, L., Jaunatre, R., Dupré la Tour, A., Buisson, E., and Bischoff, A. (2022). Mountain grassland restoration using hay and brush material transfer combined with temporary wheat cover. Ecol. Eng. 174:106447. doi: 10.1016/j.ecoleng.2021.106447
Ellenberg, H., and Leuschner, C. (2010). Vegetation Mitteleuropas mit den Alpen. Stuttgart: Eugen Ulmer.
Ellenberg, H., Weber, H. E., Düll, R., Wirth, V., Werner, W., and Paulißen, D. (2001). Zeigerwerte von Pflanzen in Mitteleuropa. Scripta Geobotanica 18, 1–262.
Eriksson, O. (2021). The importance of traditional agricultural landscapes for preventing species extinctions. Biodivers. Conserv. 30, 1341–1357. doi: 10.1007/s10531-021-02145-3
Fartmann, T. (2023). “Grasslands” in Routledge handbook of insect conservation. eds. J. Pryke, M. J. Samways, T. New, P. Cardoso, and R. Gaigher (London: Routledge)
Fartmann, T., Brüggeshemke, J., Poniatowski, D., and Löffler, F. (2022a). Summer drought affects abundance of grassland grasshoppers differently along an elevation gradient. Ecol. Entomol. 47, 778–790. doi: 10.1111/een.13168
Fartmann, T., Drung, M., and Freienstein, M. (2022b). Rejuvenation and restoration measures foster specialised and threatened carabid beetle species in montane heathland ecosystems. Insect Conserv. Divers. 15, 348–358. doi: 10.1111/icad.12560
Fartmann, T., Jedicke, E., Stuhldreher, G., and Streitberger, M. (2021). Insektensterben in Mitteleuropa – Ursachen und Gegenmaßnahmen. Stuttgart: Eugen Ulmer.
Fartmann, T., Poniatowski, D., and Holtmann, L. (2022c). Effects of land-use and climate change on grasshopper assemblages differ between protected and unprotected grasslands. Basic Appl. Ecol. 63, 83–92. doi: 10.1016/j.baae.2022.06.005
Feurdean, A., Ruprecht, E., Molnár, Z., Hutchinson, S. M., and Hickler, T. (2018). Biodiversity-rich European grasslands: ancient, forgotten ecosystems. Biol. Conserv. 228, 224–232. doi: 10.1016/j.biocon.2018.09.022
Finck, P., Heinze, S., Raths, U., Riecken, U., and Ssymank, A. (2017). Rote Liste der gefährdeten Biotoptypen Deutschlands. Naturschutz und Biologische Vielfalt 156, 1–642.
Fischer, A., and Fischer, H. (2012). “Restoration of temperate forests: an European approach,” in Restoration ecology: The New frontier, 2nd Edn., eds J. Andelvan and J. Aronson (Chichester: John Wiley & Sons), 145–160.
Florio, J., Verú, L. M., Dao, A., Yaro, A. S., Diallo, M., Sanogo, Z. L., et al. (2020). Diversity, dynamics, direction, and magnitude of high-altitude migrating insects in the Sahel. Sci. Rep. 10:20523. doi: 10.1038/s41598-020-77196-7
Fraser, L. H., Pither, J., Jentsch, A., Sternberg, M., Zobel, M., Askarizadeh, D., et al. (2015). Worldwide evidence of a unimodal relationship between productivity and plant species richness. Science 349, 302–305. doi: 10.1126/science.aab3916
Freeman, B. G., Lee-Yaw, J. A., Sunday, J. M., and Hargreaves, A. L. (2018). Expanding, shifting and shrinking: the impact of global warming on species’ elevational distributions. Glob. Ecol. Biogeogr. 27, 1268–1276. doi: 10.1111/geb.12774
Fumy, F., and Fartmann, T. (2021). Climate and land-use change drive habitat loss in a mountain bird species. Int J Avian Sci 163, 1189–1206. doi: 10.1111/ibi.12954
Fumy, F., Kämpfer, S., and Fartmann, T. (2021). Land-use intensity determines grassland Orthoptera assemblage composition across a moisture gradient. Agric. Ecosyst. Environ. 315:107424. doi: 10.1016/j.agee.2021.107424
Gardiner, T., and Hill, J. (2006). A comparison of three sampling techniques used to estimate population density and assemblage diversity of Orthoptera. J. Orthop. Res. 15, 45–51. doi: 10.1665/1082-6467(2006)15[45:ACOTST]2.0.CO;2
Geologisches Landesamt Nordrhein-Westfalen (NRW). (1998). Geologische Karte von Nordrhein-Westfalen 1: 100 000, Blatt C 4714 Arnsberg. Krefeld, Germany: Geologisches Landesamt Nordrhein-Westfalen.
German Meteorological Service. (2022). Vieljährige Mittelwerte. Available at: https://www.dwd.de/DE/leistungen/klimadatendeutschland/vielj_mittelwerte.html (Accessed 17 January 2022).
Grueber, C. E., Nakagawa, S., Laws, R. J., and Jamieson, I. G. (2011). Multimodel inference in ecology and evolution: challenges and solutions. J. Evol. Biol. 24, 699–711. doi: 10.1111/j.1420-9101.2010.02210.x
Haddad, N. M., Tilman, D., Haarstad, J., Ritchie, M., and Knops, J. M. H. (2001). Contrasting effects of plant richness and composition on insect communities: a field experiment. Am. Nat. 158, 17–35. doi: 10.1086/320866
Halada, L., Evans, D., Romão, C., and Petersen, J.-E. (2011). Which habitats of European importance depend on agricultural practices? Biodivers. Conserv. 20, 2365–2378. doi: 10.1007/s10531-011-9989-z
Helbing, F., Fartmann, T., Löffler, F., and Poniatowski, D. (2017). Effects of local climate, landscape structure and habitat quality on leafhopper assemblages of acidic grasslands. Agric. Ecosyst. Environ. 246, 94–101. doi: 10.1016/j.agee.2017.05.024
Helbing, F., Fartmann, T., and Poniatowski, D. (2020). Suction samplers are a valuable tool to sample arthropod assemblages for conservation translocation. Entomol. Exp. Appl. 168, 688–694. doi: 10.1111/eea.12952
Helbing, F., Fartmann, T., and Poniatowski, D. (2021). Restoration measures foster biodiversity of important primary consumers within calcareous grasslands. Biol. Conserv. 256:109058. doi: 10.1016/j.biocon.2021.109058
Henle, K., Alard, D., Clitherow, J., Cobb, P., Firbank, L., Kull, T., et al. (2008). Identifying and managing the conflicts between agriculture and biodiversity conservation in Europe – a review. Agric. Ecosyst. Environ. 124, 60–71. doi: 10.1016/j.agee.2007.09.005
Hill, M. O. (1973). Diversity and evenness: a unifying notation and its consequences. Ecology 54, 427–432. doi: 10.2307/1934352
Hölzel, N., and Tischew, S. (2019). “Zwergstrauchheiden und bodensaure Magerrasen” in Renaturierungsökologie. eds. J. Kollmann, A. Kirmer, S. Tischew, N. Hölzel, and K. Kiehl (Berlin: Springer Spektrum), 289–310.
Hussain, R. I., Brandl, M., Maas, B., Krautzer, B., Frank, T., and Moser, D. (2022). Establishing new grasslands on crop fields: short-term development of plant and arthropod communities. Restor. Ecol. 30:e13641. doi: 10.1111/rec.13641
Isselstein, J., Jeangros, B., and Vilem, P. (2005). Agronomic aspects of biodiversity targeted management of temperate grasslands in Europe – a review. Agron. Res. 3, 139–151.
Janišová, M., Hrivnák, R., Gömöry, D., Ujházy, K., Valachovič, M., Gömöryová, E., et al. (2007). Changes in understorey vegetation after Norway spruce colonization of an abandoned grassland. Ann. Bot. Fennici 44, 256–266.
Joern, A., and Laws, A. N. (2013). Ecological mechanisms underlying arthropod species diversity in grasslands. Annu. Rev. Entomol. 58, 19–36. doi: 10.1146/annurev-ento-120811-153540
Jones, M. E., and Davidson, N. (2016). Applying an animal-centric approach to improve ecological restoration. Restor. Ecol. 24, 836–842. doi: 10.1111/rec.12447
Kämpfer, S., and Fartmann, T. (2022). Natural coastal dunes on Wadden Sea islands as a refuge for an endangered wader species. J. Coast. Conserv. 26:53. doi: 10.1007/s11852-022-00897-w
Keene, K., Malmstrom, C. M., Alexander, H. M., Wayadande, A., and Denning, K. R. (2020). Low conservatism of leafhopper communities in remnant and reconstructed prairie sites in a working agroecological landscape. J. Insect Conserv. 24, 35–48. doi: 10.1007/s10841-019-00198-y
Kiehl, K., Kirmer, A., Donath, T. W., Rasran, L., and Hölzel, N. (2010). Species introduction in restoration projects – evaluation of different techniques for the establishment of semi-natural grasslands in central and northwestern Europe. Basic Appl. Ecol. 11, 285–299. doi: 10.1016/j.baae.2009.12.004
Kleijn, D., Kohler, F., Baldi, A., Batary, P., Concepcion, E., Clough, Y., et al. (2009). On the relationship between farmland biodiversity and land-use intensity in Europe. Proc. Biol. Sci. 276, 903–909. doi: 10.1098/rspb.2008.1509
Kőrösi, A., Batáry, P., Orosz, A., Rédei, D., and Báldi, A. (2012). Effects of grazing, vegetation structure and landscape complexity on grassland leafhoppers (Hemiptera: Auchenorrhyncha) and true bugs (Hemiptera: Heteroptera) in Hungary. Insect Conserv. Divers. 5, 57–66. doi: 10.1111/j.1752-4598.2011.00153.x
Kruess, A., and Tscharntke, T. (2002). Contrasting responses of plant and insect diversity to variation in grazing intensity. Biol. Conserv. 106, 293–302. doi: 10.1016/S0006-3207(01)00255-5
Kurtogullari, Y., Rieder, N. S., Arlettaz, R., and Humbert, J.-Y. (2020). Conservation and restoration of Nardus grasslands in the Swiss northern Alps. Appl. Veg. Sci. 23, 26–38. doi: 10.1111/avsc.12462
Kuznetsova, A., Brockhoff, P. B., Christensen, R. H. B., and Jensen, S. P. (2022). Tests in linear mixed effects models (package “lmerTest,” version 3.1–3). Available at: https://cran.r-project.org/web/packages/lmerTest/lmerTest.pdf (Accessed 22 October 2022).
LANUV (Landesamt für Natur, Umwelt und Verbraucherschutz Nordrhein-Westfalen) (2022). Umrechnungsschlüssel zur Ermittlung des zulässigen Viehbesatzes. Available at: http://vns.naturschutzinformationen.nrw.de/vns/de/fachinfo/rahmenrichtlinie/umrechnungsschluessel (Accessed 16 May 2022).
Lencová, K., and Prach, K. (2011). Restoration of hay meadows on ex-arable land: commercial seed mixtures vs. spontaneous succession. Grass Forage Sci. 66, 265–271. doi: 10.1111/j.1365-2494.2011.00786.x
Littlewood, N. A., Pakeman, R. J., and Woodin, S. J. (2009). Isolation of habitat patches limits colonisation by moorland Hemiptera. J. Insect Conserv. 13, 29–36. doi: 10.1007/s10841-007-9114-5
Lomba, A., Guerra, C., Alonso, J., Honrado, J. P., Jongman, R., and McCracken, D. (2014). Mapping and monitoring high nature value farmlands: challenges in European landscapes. J. Environ. Manage. 143, 140–150. doi: 10.1016/j.jenvman.2014.04.029
McAlpine, C., Catterall, C. P., Mac Nally, R., Lindenmayer, D., Reid, J. L., Holl, K. D., et al. (2016). Integrating plant- and animal-based perspectives for more effective restoration of biodiversity. Front. Ecol. Environ. 14, 37–45. doi: 10.1002/16-0108.1
McCune, B., and Keon, D. (2002). Equations for potential annual direct incident radiation and heat load. J. Veg. Sci. 13, 603–606. doi: 10.1111/j.1654-1103.2002.tb02087.x
Mortelliti, A., Amori, G., and Boitani, L. (2010). The role of habitat quality in fragmented landscapes: a conceptual overview and prospectus for future research. Oecologia 163, 535–547. doi: 10.1007/s00442-010-1623-3
Mottet, A., Ladet, S., Coqué, N., and Gibon, A. (2006). Agricultural land-use change and its drivers in mountain landscapes: a case study in the Pyrenees. Agric. Ecosyst. Environ. 114, 296–310. doi: 10.1016/j.agee.2005.11.017
Nakagawa, S., Johnson, P. C. D., and Schielzeth, H. (2017). The coefficient of determination R2 and intra-class correlation coefficient from generalized linear mixed-effects models revisited and expanded. J.R. Soc. Interface 14, 1–11. doi: 10.1098/rsif.2017.0213
Neff, F., Resch, M. C., Marty, A., Rolley, J. D., Schütz, M., Risch, A. C., et al. (2020). Long-term restoration success of insect herbivore communities in seminatural grasslands: a functional approach. Ecol. Appl. 30:e02133. doi: 10.1002/eap.2133
Nickel, H. (2003). The leafhoppers and Planthoppers of Germany (Hemiptera, Auchenorrhyncha): Patterns and strategies in a highly diverse Group of Phytophagous Insects. Sofia: Pensoft Publishers.
Nickel, H., and Achtziger, R. (1999). Wiesen bewohnende Zikaden (Auchenorrhyncha) im Gradienten von Nutzungsintensität und Feuchte. Beitr. Zikadenk. 3, 65–80.
Nickel, H., and Remane, R. (2002). Artenliste der Zikaden Deutschlands, mit Angaben von Nährpflanzen, Nahrungsbreite, Lebenszyklus, Areal und Gefährdung (Hemiptera, Fulgoromorpha et Cicadomorpha). Beitr. Zikadenk. 5, 27–64.
Oberdorfer, E. (2001). Pflanzensoziologische Exkursionsflora für Deutschland und angrenzende Gebiete, 8th Edn. Stuttgart: Eugen Ulmer.
Oksanen, J., Simpson, G. L., Blanchet, F. G., Kindt, R., Legendre, P., Minchin, P. R., et al. (2022). Community ecology package (package “vegan,” version 2.6–4). Available at: https://cran.r-project.org/web/packages/vegan/vegan.pdf (Accessed 21 October 2022).
Peppler-Lisbach, C., and Petersen, J. (2001). Calluno-Ulicetea (G3), Teil 1: Nardetalia strictae – Borstgrasrasen. Syn. Pflanzenges. Deutschl. 8, 1–117.
Pereira, H. M., Navarro, L. M., and Martins, I. S. (2012). Global biodiversity change: the bad, the good, and the unknown. Annu. Rev. Env. Resour. 37, 25–50. doi: 10.1146/annurev-environ-042911-093511
Plieninger, T., Höchtl, F., and Spek, T. (2006). Traditional land-use and nature conservation in European rural landscapes. Environ. Sci. Policy 9, 317–321. doi: 10.1016/j.envsci.2006.03.001
Poniatowski, D., Beckmann, C., Löffler, F., Münsch, T., Helbing, F., Samways, M. J., et al. (2020). Relative impacts of land-use and climate change on grasshopper range shifts have changed over time. Glob. Ecol. Biogeogr. 29, 2190–2202. doi: 10.1111/geb.13188
Poniatowski, D., Löffler, F., Stuhldreher, G., Borchard, F., Krämer, B., and Fartmann, T. (2016). Functional connectivity as an indicator for patch occupancy in grassland specialists. Ecol. Indic. 67, 735–742. doi: 10.1016/j.ecolind.2016.03.047
Poniatowski, D., Stuhldreher, G., Löffler, F., and Fartmann, T. (2018). Patch occupancy of grassland specialists: habitat quality matters more than habitat connectivity. Biol. Conserv. 225, 237–244. doi: 10.1016/j.biocon.2018.07.018
R Core Team (2022). R: A language and environment for statistical computing. R foundation for statistical computing, Vienna, Austria. Available at: https://www.r-project.org/index.html (Accessed 27 April 2022).
Reynolds, D. R., Chapman, J. W., and Stewart, A. J. A. (2017). Windborne migration of Auchenorrhyncha (Hemiptera) over Britain. Eur. J. Entomol. 114, 554–564. doi: 10.14411/eje.2017.070
Richards, S. A. (2008). Dealing with overdispersed count data in applied ecology. J. Appl. Ecol. 45, 218–227. doi: 10.1111/j.1365-2664.2007.01377.x
Robinson, R., and Sutherland, W. J. (2002). Post-war changes in arable farming and biodiversity in Great Britain. J. Appl. Ecol. 39, 157–176. doi: 10.1046/j.1365-2664.2002.00695.x
Roem, W. J., and Berendse, F. (2000). Soil acidity and nutrient supply ratio as possible factors determining changes in plant species diversity in grassland and heathland communities. Biol. Conserv. 92, 151–161. doi: 10.1016/S0006-3207(99)00049-X
Rösch, V., Tscharntke, T., Scherber, C., and Batáry, P. (2013). Landscape composition, connectivity and fragment size drive effects of grassland fragmentation on insect communities. J. Appl. Ecol. 50, 387–394. doi: 10.1111/1365-2664.12056
Schulte, A. M. (2017). E.05.1 Vegetationskundliches Monitoring zur Restitution/Optimierung von Bergmähwiesen und Borstgrasrasen auf Waldumwandlungs-und Grünlandflächen insbesondere durch Mahdgut-Übertragung. Available at: http://www.bergwiesen-winterberg.de/images/Mon2017_Bericht_mitAnlagen.pdf (Accessed 25 October 2022).
Soliveres, S., van der Plas, F., Manning, P., Prati, D., Gossner, M. M., and Renner, S. C. (2016). Biodiversity at multiple trophic levels is needed for ecosystem multifunctionality. Nature 536, 456–459. doi: 10.1038/nature19092
Stevens, C. J., Dise, N. B., Mountford, J. O., and Gowing, D. J. (2004). Impact of nitrogen deposition on the species richness of grasslands. Science 303, 1876–1879. doi: 10.1126/science.1094678
Stewart, A. J. A. (2002). Techniques for sampling Auchenorrhyncha in grasslands. Denisia 4, 491–512.
Stoate, C., Boatman, N. D., Borralho, R. J., Carvalho, C. R., de Snoo, G. R., and Eden, P. (2001). Ecological impacts of arable intensification in Europe. J. Environ. Manage. 63, 337–365. doi: 10.1006/jema.2001.0473
Stöckli, A., Slodowicz, D., Arlettaz, R., and Humbert, J.-Y. (2021). Transfer of invertebrates with hay during restoration operations of extensively managed grasslands in Switzerland. J. Insect Conserv. 25, 189–194. doi: 10.1007/s10841-020-00282-8
Strand, L. T., Fjellstad, W., Jackson-Blake, L., and De Wit, H. A. (2021). Afforestation of a pasture in Norway did not result in higher soil carbon, 50 years after planting. Landscape Urban Plan. 207:104007. doi: 10.1016/j.landurbplan.2020.104007
Strebel, N., and Bühler, C. (2015). Recent shifts in plant species suggest opposing land-use changes in alpine pastures. Alp. Bot. 125, 1–9. doi: 10.1007/s00035-015-0145-3
Stuhldreher, G., and Fartmann, T. (2018). Threatened grassland butterflies as indicators of microclimatic niches along an elevational gradient – implications for conservation in times of climate change. Ecol. Indic. 94, 83–98. doi: 10.1016/j.ecolind.2018.06.043
Sullivan, E., Hall, N., and Ashton, P. (2020). Restoration of upland hay meadows over an 11-year chronosequence: an evaluation of the success of green hay transfer. Restor. Ecol. 28, 127–137. doi: 10.1111/rec.13063
van Daele, F., Wasof, S., Demey, A., Schelfhout, S., De Schrijver, A., Baeten, L., et al. (2017). Quantifying establishment limitations during the ecological restoration of species-rich Nardus grassland. Appl. Veg. Sci. 20, 594–607. doi: 10.1111/avsc.12330
van Klink, R., van der Plas, F., van Noordwijk, C. G. E., Wallis DeVries, M. F., and Olff, H. (2015). Effects of large herbivores on grassland arthropod diversity. Biol. Rev. 90, 347–366. doi: 10.1111/brv.12113
Wachmann, E., Melber, A., and Deckert, J. (2004). “Wanzen Band 2 – Cimicomorpha: Microphysidae (Flechtenwanzen), Miridae (Weichwanzen)” in Die Tierwelt Deutschlands, vol. 75 (Keltern: Goecke & Evers), 1–288.
Wachmann, E., Melber, A., and Deckert, J. (2006). “Wanzen band 1 – Dipsocoromorpha, Nepomorpha, Gerromorpha, Leptopodomorpha, Cimicomorpha (Teil 1)” in Die Tierwelt Deutschlands, vol. 77 (Keltern: Goecke & Evers), 1–263.
Wachmann, E., Melber, A., and Deckert, J. (2007). “Wanzen Band 3 – Pentatomomorpha I: Aradidae, Lygaeidae, Piesmatidae, Berytidae, Pyrrhocoridae, Alydidae, Coreidae, Rhopalidae, Stenocephalidae” in Die Tierwelt Deutschlands, Vol. 78 (Keltern: Goecke & Evers), 1–272.
Wachmann, E., Melber, A., and Deckert, J. (2008). “Wanzen band 4 – Pentatomomorpha II, Pentatomoidea: Cydnidae, Thyreocoridae, Plataspidae, Acanthosomatidae, Scutelleridae, Pentatomidae” in Die Tierwelt Deutschlands, Vol. 81 (Keltern: Goecke & Evers), 1–230.
Wagner, M., Hulmes, S., Hulmes, L., Redhead, J. W., Nowakowski, M., and Pywell, R. F. (2020). Green hay transfer for grassland restoration: species capture and establishment. Restor. Ecol. 29:e13259. doi: 10.1111/rec.13259
Waldén, E., Öckinger, E., Winsa, M., and Lindborg, R. (2017). Effects of landscape composition, species pool and time on grassland specialists in restored semi-natural grasslands. Biol. Conserv. 214, 176–183. doi: 10.1016/j.biocon.2017.07.037
Keywords: Auchenorrhyncha, green hay transfer, habitat quality, Heteroptera, host plant, nature conservation, Orthoptera, Picea abies
Citation: Helbing F, Fartmann T, Morkel C and Poniatowski D (2023) Rapid response of vascular plants and insects to restoration of montane grasslands. Front. Ecol. Evol. 11:1148266. doi: 10.3389/fevo.2023.1148266
Edited by:
Armin Bischoff, University of Avignon, FranceReviewed by:
Paulo A. V. Borges, University of the Azores, PortugalOlivier Blight, University of Avignon, France
Copyright © 2023 Helbing, Fartmann, Morkel and Poniatowski. This is an open-access article distributed under the terms of the Creative Commons Attribution License (CC BY). The use, distribution or reproduction in other forums is permitted, provided the original author(s) and the copyright owner(s) are credited and that the original publication in this journal is cited, in accordance with accepted academic practice. No use, distribution or reproduction is permitted which does not comply with these terms.
*Correspondence: Felix Helbing, felix.helbing@uos.de
†ORCID: Felix Helbing, https://orcid.org/0000-0002-5161-709X
Thomas Fartmann, https://orcid.org/0000-0002-2050-9221
Carsten Morkel, https://orcid.org/0000-0002-0623-9827
Dominik Poniatowski, https://orcid.org/0000-0002-9955-688X